- 1Department of Ophthalmology, Collegium Medicum, Cardinal Stefan Wyszynski University, Warsaw, Poland
- 2Hospital in Ostrow Mazowiecka, Ostrów Mazowiecka, Poland
- 3Department of Nuclear Medicine, Pomeranian Medical University in Szczecin, Szczecin, Poland
- 4Orthopedic and Rehabilitation Department, Medical University of Warsaw, Warsaw, Poland
- 5Medical Faculty, Lazarski University, Warsaw, Poland
- 6Institute of Genetics and Animal Biotechnology of the Polish Academy of Sciences, Magdalenka, Poland
- 7Laboratory of Natural Products and Medicinal Chemistry (LNPMC), Center for Global Health Research, Saveetha Medical College and Hospital, Saveetha Institute of Medical and Technical Sciences (SIMATS), Chennai, India
- 8Ludwig Boltzmann Institute Digital Health and Patient Safety, Medical University of Vienna, Vienna, Austria
Diabetes mellitus (DM) is a prevalent metabolic disease. The clinical impact of sustained hyperglycemia on ligament healing has not been well characterized. Diabetes is a known cause of macro-, microvascular, and diabetic ulcer healing difficulties among tissues. Therefore, we aimed to investigate the healing potential occurring in injured and healthy ligaments among diabetic and healthy individuals using a rat model. We hypothesize that DM may contribute to altering the knee medial collateral ligament (MCL), thus its morphology, biochemical fitness, and functionality. The study cohort consisted of 40 rats. The animals were randomized into four equal groups. Groups I and II (20 rats) received saline subcutaneously and served as controls. Groups III and IV (20 rats) were injected with a single dose of streptozotocin (STZ). All animals underwent surgery to cut the left tibial collateral ligament in the hind limb and suture it. The access site was sutured to create inflammation and study the regenerative capacities of animals with normal carbohydrate metabolism and pharmacologically induced diabetes. Each animal then underwent sham surgery to access and suture the right tibial collateral ligament in the hind limb without ligament intervention. After the animals had undergone surgeries, groups II and IV were given melatonin supplementation for 4 weeks. Rats with DM presented with more fibrosis and calcification of the MCL and decreased healing potential. Treatment with melatonin in diabetic rats mitigated alterations and improved the antioxidant status of ligaments from the diabetic group.
1 Introduction
Ligaments play a unique and important role in the musculoskeletal system. However, the knowledge about the molecular mechanisms in the connective tissue is not as advanced as knowledge about other tissues (Longo et al., 2009; Leong et al., 2020). This is partly because, until recently, the transcription factors crucial for the formation and maintenance of ligaments remained unknown, and there is still much to discover (Nelson et al., 2006). Understanding the pathogenesis of non-insulin-dependent diabetes mellitus and the development of more effective therapies may be facilitated by the establishment of animal models with pathophysiology closely resembling human disease (Fleming et al., 2005). Tendons and ligaments arise from different developmental processes. The regulatory pathways and morphologies of the two tissues predispose them to play heterogeneous roles (Fleming, 2003). Therefore, the available findings in tendinopathies are not well adopted in the concept of ligament regeneration and require further study.
Systemic diseases with long-lasting inflammation impact the organism in various ways (Fleming, 2003; Stolarczyk et al., 2018). Diabetes mellitus (DM) is a prevalent metabolic disorder that impairs barrier functioning and healing responses throughout the human body. In particular, DM inhibits the expression of mitogenic growth factors and simultaneously stimulates that of pro-inflammatory cytokines through epigenetic mechanisms. Moreover, hyperglycemia and oxidative stress induced by DM prevent the expansion of mesenteric cells that are involved in both soft and hard tissue wounds (Hill et al., 2005; Akbarzadeh et al., 2007; Mullaji et al., 2008). Those mechanisms can be investigated in animal models, and further comprehensive studies are needed to address the issues. Therefore, research on ligament alteration is of great significance.
Not many researchers correlate the damage to ligaments with possible systemic diseases and instead focus on trauma as the cause of damage (Akbarzadeh et al., 2007; Mullaji et al., 2008; Parasuraman et al., 2010; Bicer et al., 2011). However, DM is one of the most common and debilitating medical conditions (Fleming, 2003; Stolarczyk et al., 2018).
Patients with DM may experience increased surgical risks, including a higher risk of infection, compromised tissue quality for repair, and a propensity for problems with wound healing (Sotoudeh and Namavar, 2022).
A major interest of this study was to compare both the regenerative abilities of ligament and skin injury in diabetic rats and the regenerative abilities of ligament and skin injury among nondiabetic rats, as well as the putative benefits of melatonin supplementation. We hypothesize that DM may contribute to the impaired healing potential of the knee medial collateral ligament regardless of the extent of the damage. The sacrifice of rats in the present study enhanced the current state of the art by collecting tissues for microscopic evaluation. Furthermore, the study aimed to identify alterations in the microarchitecture of MCL and remodeling of the molecules for insights into the treatment of patients presenting with DM and musculoskeletal complications. Ligament recovery is demanding because of its multifactorial role in the musculoskeletal system. The healing of the joint soft tissues in comorbidity with diabetes is challenging in clinical practice. Accumulated evidence and data from the present study support future preclinical and clinical experiments. Melatonin’s beneficial effects on insulin signaling and lipid metabolism in obesity, as well as decreasing inflammation and antioxidant activity in diabetes and related comorbidities, have been proved. This is important due to the abundance of patients with obesity in clinical practice. DM and obesity are common comorbidities in the orthopedic department, and the result again stresses the need for holistic therapy for soft tissue damage in patients and the role of sleep and melatonin at high levels. The results provide insights into the pathogenesis of ligament alteration in diabetes and uncover melatonin’s potential for downregulating inflammation, oxidative stress, and inhibitors of systemic metabolism.
2 Materials and methods
Male Sprague–Dawley rats with a weight ranging from 280 to 300 g, 12 weeks of age, were purchased from the Central Laboratory of Experimental Animal Models, Medical University of Warsaw, Warsaw, Poland. The rats were kept in plastic cages with metal lids, with two rats per cage. The animals received a commercial diet and had free access to water. The rats were placed in a conventional laboratory housing with a 12 h light/12 h dark cycle at a temperature of 21°C ± 2°C and 55% ± 10% humidity.
All the animals received housing conditions according to the criteria outlined in the Guide for the Care and the Use of Laboratory Animals prepared by EU Directive 2010/63/EU for animal experiments. The ethical regulations were followed in accordance with national and institutional guidelines for the protection of animal welfare during experiments. The study was designed according to the ARRIVE guidelines.
The animals used in the study were randomly separated into four groups.
Group I (n = 10) general control group: the group was not induced with DM and fed on a normal diet.
Group II (n = 10) melatonin-supplemented control group: the group was fed a normal diet and supplemented with 3 mg/kg/day melatonin per os (p.o.) for 4 weeks (Bicer et al., 2011).
Groups III and IV were injected with streptozotocin (STZ) at a dose of 60 mg/kg of body weight, dissolved in a freshly prepared buffer (0.1 mol/L citrate, pH 4.5). The rats were fasted for 8 h before the STZ injection. The solution was injected into rats intraperitoneally (i.p.), with the aim to induce diabetes (Akbarzadeh et al., 2007). Fasting blood glucose level was assessed on the day of the intraperitoneal injection of STZ and 72 h after the injection, and a venous blood glucose test was performed by glucometer strips. The animals were immobilized in a plastic tube and placed on the heating platform to dilate the vessels in their tails. A drop of blood was obtained by puncturing the vein with a needle (Parasuraman et al., 2010).
Rats with consistent blood glucose levels ≥200 mg/dL 72 h after the STZ injection were regarded as successful diabetic models. To reduce the risk of mortality caused by STZ, the animals used in the study were relatively young. No animal died, and no animal experienced an ineffective DM induction.
Group III (n = 10) diabetic group: the group in which DM was induced by an i.p. injection of 60 mg/kg STZ and was not supplemented with melatonin.
Group IV (n = 10) melatonin-supplemented diabetic group: the group in which DM was induced by an i.p. injection of 60 mg/kg STZ and was supplemented with 3 mg/kg/day melatonin p. o. for 4 weeks (Bicer et al., 2011).
2.1 Tissue preparation
A surgical dissection of the MCL was performed on the left lower extremity of an animal, and a sham procedure was performed on the right lower extremity of the same animal to simulate the surgical conditions in surrounding tissues and to compare the process of regeneration. The procedure was performed under general anesthesia (ketamine 75 mg/kg of body weight + xylazine 7 mg/kg of body weight) (Sotoudeh and Namavar, 2022). After disinfection, a longitudinal cut from 3 mm below the knee joint to 3 mm above the joint was made, then the skin and fascia were cut to reveal the MCL (Sairyo et al., 2007) (Figure 1). The ligament was dissected and cut in the middle. Fascia, ligaments, and skin were closed with a surgical suture (Vicryl 6.0, Ethicon) (Figure 2). A sham operation was performed on the right limb. All steps were the same as the operation of dissecting the left MCL, except that the ligament remained intact after preparation. Post-operative care and pain relief (paracetamol in drinking water 300 mg/kg of body weight) were provided for 2 days from the day of surgery and possibly repeated in the case of pain distress caused by the operation. Animals received 2.5% Baytril antibiotic prophylaxis (enrofloxacinum) s.c. mg/kg of body weight dose for a period of 3 days post-operatively (Trouchon and Lefebvre, 2016; Sotoudeh and Namavar, 2022).
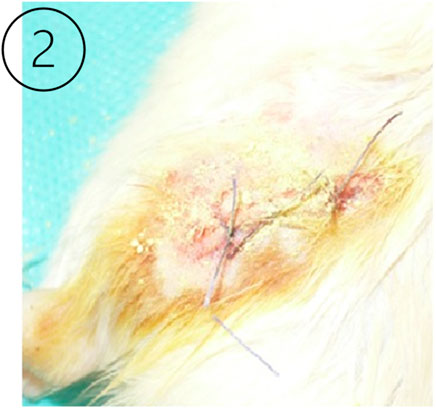
Figure 1. The right image (Leong et al., 2020) shows the animal model knee joint surgical site during the ligament preparation procedure.
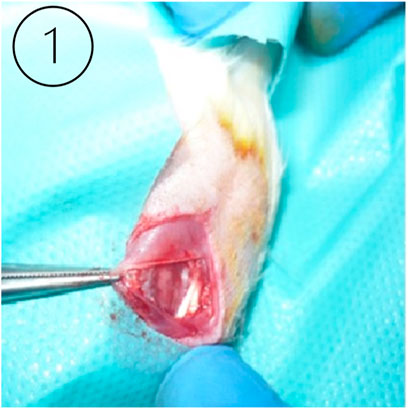
Figure 2. The left image (Longo et al., 2009) shows a sutured surgical wound in the experimental animal model after the intervention in the knee joint ligament.
Table 1 provides a summarized division of the animals into the four groups, each with 10 models. Figure 3 represents the schematic overview of the study design.
2.2 Controlled treatment with melatonin supplementation
Melatonin (Mel) (Sigma M-5250) supplementation was given to the animals in groups II and IV at a dose of 3 mg/kg/day for 4 weeks after the surgical procedures (Bicer et al., 2011).
2.3 Tissue acquisition and preparation
The operating surgeon obtained tissue from the central portion of the MCL (entire layer) to minimize tissue damage and optimize the harvest of the ligament only. After all fat tissue was removed, the tissue specimens were washed with saline to remove blood and bodily fluid contaminants. All specimens were transported under sterile conditions to the laboratory within 20 min of excision. The harvesting and processing procedures were identical in all rats. Two experienced scientists and the first author performed the surgical interventions.
The specimens were fixed in 4% formaldehyde in phosphate-buffered saline and prepared for paraffin embedding. Twenty consecutive sections, each 4-µm thick, were cut on a microtome and treated with the appropriate stains. A light microscope (Nikon, Japan) was used to view and photograph the specimens.
2.4 Fibrosis and elastin fiber loss grading
Four cross sections of each specimen were randomly selected for histologic analysis and graded independently by four of the authors (A.S., A.W., M.P., and O.A.), all of whom were blinded to the group. The severity of fibrosis (based on Masson trichrome staining) was graded on four-point scale, according to the guidelines set by Sairyo et al. (2007), Ding et al. (1988): 0—normal tissue showing no fibrotic region; grade 1—fibrosis of less than 25% of the entire area; 2—fibrosis of 25%–50% of the entire area; grade 3—fibrosis of 50%–75% of the entire area; 4—more than 75% fibrosis. The same method was used to evaluate elastin fiber loss (based on elastica van Gieson and Orcein staining). Average grades of fibrosis and elastin fiber loss were calculated for every sample. The results are presented in Figure 3.
2.5 Tissue homogenates
Rats from all groups were terminally anesthetized after 6 weeks by an intraperitoneal injection of 1.5–2 mL/kg (199.95–267 mg/kg) of body weight of sodium pentobarbital + 40.05–53.4 mg/kg of body weight of pentobarbital. Blood samples were drawn by cardiac puncture into tubes containing EDTA. Ligament samples were also collected from the different experimental groups for histopathological and biochemical examination.
In the first part, ligament harvesting was done immediately, and the collected tissue was homogenized in a volume/tissue ratio of 100 mM phosphate buffer (pH 7.4) containing 22 mg% EDTA. Organs from different experimental groups were fixed in 10% buffered formalin for 24 h. After fixation, tissues were washed in tap water, then dehydrated in an ascending series of 70%, 90%, 95%, and 100% ethanol, followed by clearing in xylene and embedding in paraffin wax at 55°C. Five sections (4 µm thickness) were cut from each tissue, followed by staining with hematoxylin and eosin. In brief, slides were put in xylene to dissolve the paraffin wax, then in absolute alcohol for 2 min, 95% alcohol for 1 min, and 70% alcohol for 1 min. Slides were stained with hematoxylin for 5 min, then washed in tap water. Excess stain was removed in acid alcohol for a few seconds. Sections were stained with eosin for 4 min to 5 min, washed in water, and then washed in absolute alcohol (95%) and xylene.
The tissue homogenate was then stored at −20°C for determination of lipid peroxides, total thiols, activity of catalase, superoxide dismutase (SOD), and glutathione S-transferase (GST). The ligament homogenates were retrieved to analyze the concentrations of oxidative biomarkers: tissue total antioxidant status (TAS), total oxidant status (TOS), ROS activities, and oxidative stress index (OSI). Lipid peroxidation was determined using malondialdehyde (MDA) and glutathione (GSH). Blood samples were centrifuged to separate plasma and tested for levels of fasting blood glucose, total lipid, triglyceride levels, cholesterol levels, lipid peroxides, nitric oxide (NO), total thiols, and total albumin and activities of catalase, uric acid, SOD and GST.
2.6 Biochemical analyses
2.6.1 Lipid peroxidase and nitric oxide
Lipid peroxide concentration was detected in obtained plasma and tissue homogenates. The product of the reaction between malondialdehyde and barbituric acid was measured. NO was determined in plasma as nitrite concentration after the reduction of nitrate to nitrite. The reaction took place at 22°C for 20 min, and the absorbance at 546 nm was measured using an NaNO3 solution as the standard (Ding et al., 1988).
2.6.2 SOD, catalase, GST, MDA, and ceruloplasmin activities
SOD activity was measured in three tubes: plasma, hemolysates, and tissue homogenates, and was calculated using its inhibitory activity of the autooxidation of epinephrine in an alkaline medium (Misra and Fridovich, 1972). GST activity in hemolysates and tissue homogenates was measured through chemical determination using 1-chloro2,4-dinitrobenzene as the substrate (Habig et al., 1974). Catalase activity in hemolysate and tissue homogenates was determined based on its ability to decompose H2O2 and measured at 240 nm (Luck and Bergmeyer, 1963). Serum MDA was determined by a rat MDA ELISA Kit (MBS268427, MyBioSource, United States). SOD activity was determined by a rat SOD ELISA Kit (MBS266897, MyBioSource, United States). A Bio-Rad protein assay was used to measure total protein concentration in tissue homogenates. Ceruloplasmin activity was determined using a para-phenylenediamine dihydrochloride method (Schosinsky et al., 1974).
2.6.3 Total plasma thiols, uric acid, and albumin
Total thiols were detected using the chemical method described by Ellman (1959). Albumin detection was performed colorimetrically with a commercial kit (Sclavo Diagnostics, Italy). Uric acid was determined by enzymatic colorimetric method using a commercial kit (Biocon, Burbachy, Germany).
2.6.4 Plasma cholesterol, triglycerides, and total lipids
Cholesterol concentration was determined using an enzymatic colorimetric kit (Biocon). Triglyceride concentration was measured using an enzymatic hydrolysis of triglycerides with subsequent determination of liberated glycerol by colorimetry (Boehringer). Total lipids were chemically determined by the phosphovanillin method (Knight et al., 1972).
2.6.5 Total protein and blood glucose
Total proteins in plasma and tissue homogenates of ligaments and muscles were determined by a biuret tartrate method using a commercial kit (Sclavo Diagnostics). Blood glucose level was determined by a commercial kit (Biocon).
2.6.6 Oxidative status
Ligament harvesting was done, and bone samples were excised and homogenized in cold 0.9% NaCl using glass equipment to obtain a 10% (w/v) homogenate. The homogenates were centrifuged at 10,000×g for 15 min at 4°C so as to obtain the necessary clear supernatants required for the experiments. TAS, TOS, and ROS activities and OSI were assessed using the method described by Erel (2004) and Erel (2005).
2.6.7 Lipid peroxidation parameters
MDA analyses were performed in the bone tissue as mentioned earlier, and the levels were given as nmol/g/protein (Draper and Hadley, 1990). The glutathione GSH levels in the bone tissue were determined, as mentioned earlier. The GSH level was determined as mg/dL/g protein (Atroshi et al., 1981).
2.7 Statistical analysis
The collected data were analyzed using the STATISTICA 13.3 TIBCO Software, Inc., statistical package. Data are expressed as mean ± SD for all parameters in plasma, erythrocyte lysate, and tissue homogenates. The evaluation of the distribution of the analyzed variables was verified with the Shapiro–Wilk test. When two dependent variables were compared, and the distribution was consistent with a normal distribution, Student’s t-test was used. When comparing the two dependent variables and non-compliance of the distribution with the normal distribution, the Wilcoxon pair order test was applied.
In the case of multiple independent samples (such as a comparison of animal models from different groups) with a distribution that did not match the normal distribution, the Kruskal–Wallis ANOVA test was used. Post hoc group comparisons were carried out using the Newman–Keuls test. To determine the occurrence of statistically significant differences between specific variables, multiple comparisons of average ranks for all trials (such as a comparison of parameters from a single-animal model) were used. Trait correlations were assessed using the Spearman test:
• Rxy > 0 positive correlation—when the value of X increases, so does Y,
• Rxy = 0 no correlation—when X increases, Y sometimes increases and sometimes decreases,
• Rxy < 0 negative correlation—when X increases, Y decreases.
The correlation strength was determined according to the following guidelines:
• Rxy = 0 variables are not correlated
• 0 < Rxy < slight correlation
• 0.1 < Rxy < 0.3 weak correlation
• 0.3 < Rxy < 0.5 average correlation
• 0.5 < Rxy < 0.7 high correlation
• 0.7 < Rxy < 0.9 very high correlation
• 0.9 < Rxy < 1 almost complete correlation
The statistical significance level of p < 0.05 was adopted.
3 Results
3.1 Histological screening of stained samples: Sairyo score assessment of the fibrosis of knee ligament samples
The values presented in the tables are compared in the following manner: group I is a control group for groups II and III, and group II is a control group for group IV.
The left MCLs that underwent the surgical procedure presented with worsened overall status compared to the right MCLs that experienced a sham procedure (Table 2). Specifically noticeable was significant fibrosis in the left MCL compared to the right MCL in group III of animals with a DM score of 3.43 ± 0.88; p < 0.05 and in group I animals with a DM score of 1.44 ± 0.7; p < 0.05. The third section (a Sairyo score of 1.44) of dissected ligament tissue from the normoglycemic group appeared fibrotic, and 75% of the MCLs dissected from diabetic rats were fibrotic. Increased loss of elastin fiber was observed in the dissected MCLs from all groups, p < 0.05. Group II, the melatonin-supplemented control group, had less elastin fiber loss than group I. Right MCLs from group II achieved 0 in Sairyo Score and right MCLs from the group I achieved 0.5 ± 0.2, p < 0.05. Left MCLs from group II achieved 0 in Sairyo Score and left MCLs from the group I achieved 1.05 ± 0.55, p < 0.05. The right MCL samples from all groups showed statistically significant more calcification than the tissue retrieved from the left MCL from the same groups. The nondiabetic group presented with calcification in the dissected left MCL (1.05, ± 1.7) and no sign of calcification among the right MCL with statistical significance (p < 0.05).
Specifically, the specimens belonging to group III, animals with DM, present with hypercellularity and fibrosis compared to the integrated, linear fibroblasts in the control group. What is interesting is that group IV, animals with DM receiving supplemental melatonin, presented with an improved overall quality of the dissected ligaments (Figure 4).
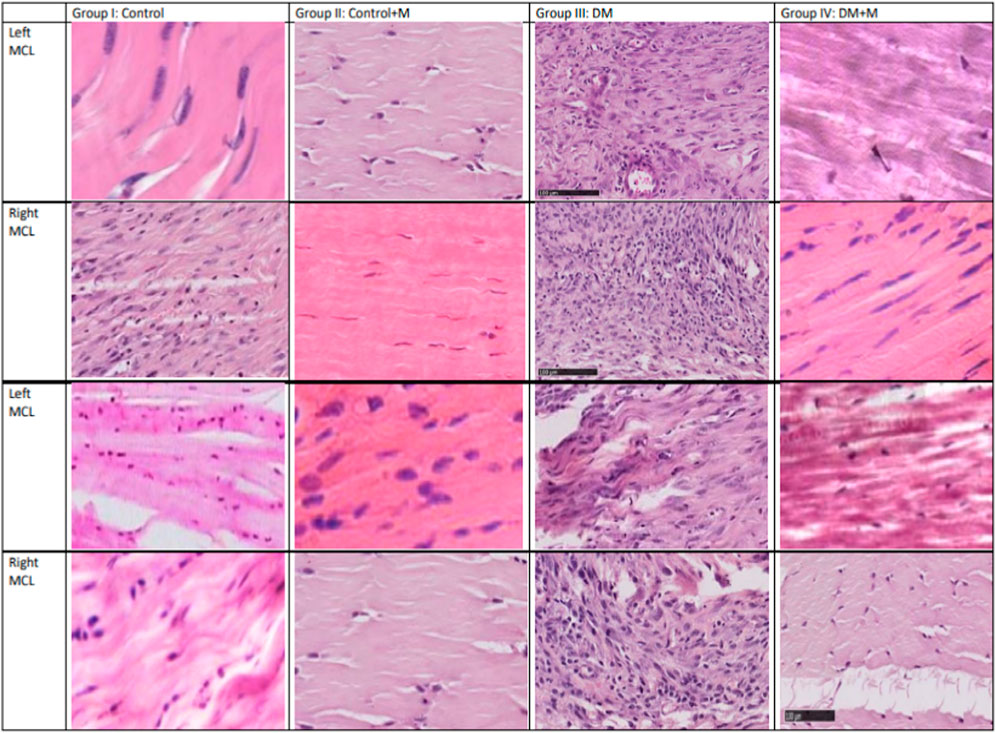
Figure 4. Histology-stained sections (longitudinal dissection) from the four groups: normoglycemia and diabetes mellitus, with and without melatonin supplementation. Each column contains light microscopy images obtained from a single representative model and from the same section. A scale bar is shown on the left bottom corner (100 µm). Each row is indicated for one model from which the tissue was retrieved. Sample imaging was used to assess the status of the tissue. The right MCL comes from the extremity on which the sham procedure was performed, and the left MCL comes from the extremity on which a ligament incision was performed. Quantitative results are presented in Tables 3, 4.
3.2 Microscopic histopathological assessment: optic quantitative screening for alterations in the extracted ligament samples from different experimental groups
All (100%) of the connective tissue of the right and left MCL samples from group I, normoglycemic animals, appeared with typical ligament components with statistical significance (p < 0.05) (Table 3). An assessment of the remaining groups indicated that in group II of animals without DM receiving Mel supplementation, the right MCL appeared with a characteristic pattern in each sample with statistical significance (p < 0.05). In the left MCL, most samples (8; p < 0.05) appeared to be typical, but two samples presented with single lymphocytic infiltration, loosened structure, mast cell infiltration, and increased fibroblasts compared to group I (p < 0.05).
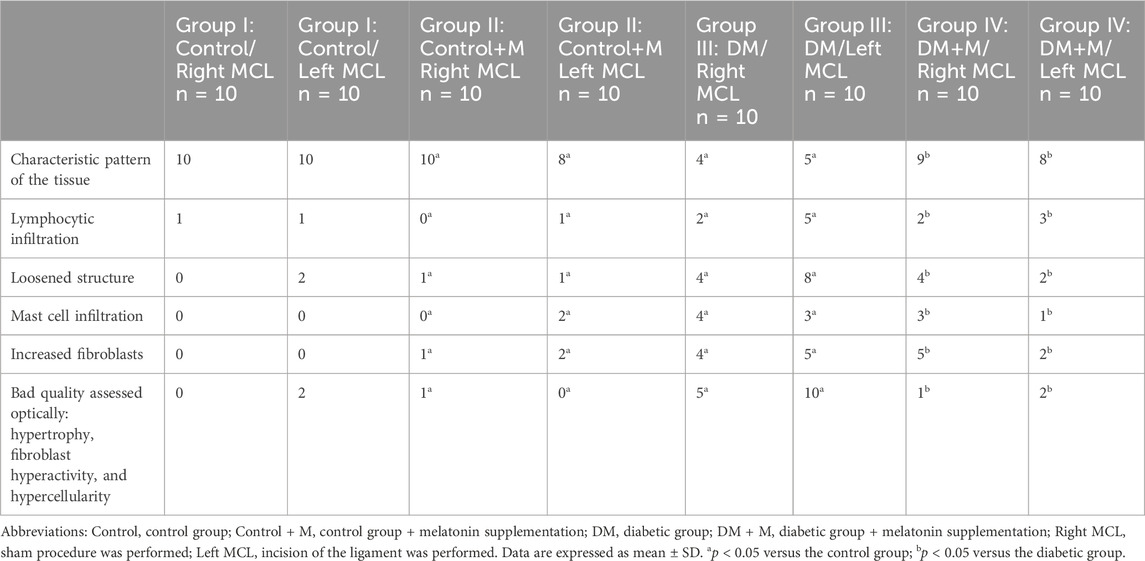
Table 3. Optical quantitative analysis of the ligaments from groups I to IV using the light microscope.
Histopathological screening of the left MCL from group III with DM revealed a loosened structure of the connective tissue (8; p < 0.05) together with lymphocytic infiltration (5; p < 0.05), increased quantities of fibroblasts (5; p < 0.05) and mast cell infiltration (3; p < 0.05) and features of increased fibroblast activity influencing the overall optically assessed quality of the tissue. Overall hypertrophy of the tissue, hypercellularity, and invalid fibroblast function were described in half of the samples (5; p < 0.05) compared to group I.
A minority of the right MCL samples from group III presented the characteristic pattern of the tissue (4; p < 0.05), with increased fibroblasts (4; p < 0.05), mast cell infiltration (4; p < 0.05), loosened structure (4; p < 0.05), and lymphocytic infiltration (2; p < 0.05). Overall hypertrophy of the tissue, hypercellularity, and invalid fibroblast function were described in half of the samples (5; p < 0.05) compared to group I.
Nine samples from group IV, animals with DM and Mel supplementation, presented with a characteristic pattern of the right MCL, which is statistically significant compared to group II of animals without DM and with Mel supplementation (p <0.05). Lymphocytic infiltration appeared in two samples (p < 0.05), and increased fibroblasts (5; p < 0.05), mast cell infiltration (3; p < 0.05), and loosened structure (4; p < 0.05) appeared in other samples. Only one sample was assessed as having bad quality (p < 0.05) compared to the corresponding group II.
Eight of the left MCL samples from group IV presented with the characteristic pattern of the right MCL, which is statistically significant compared to group II (p < 0.05). Lymphocytic infiltration appeared in three samples (p < 0.05), and increased fibroblasts (2; p < 0.05), mast cell infiltration (1; p < 0.05), and loosened structure (2; p < 0.05) appeared in others. The quality of two samples was assessed as bad compared to group II (p < 0.05).
All the left MCL samples from group III, animals with DM, seemed to be disintegrated and frayed. Their elasticity was consistent (90%; p = 0.001). Some samples were found to be mildly hypertrophic (2 ± 0.92; p = 0.001), and some were intensively hypertrophic (6 ± 0.22; p = 0.001). The right MCL samples from group III seemed hypercellular (8; p > 0.05) and showed lymphocytic infiltration (2; p = 0.00865). However, the structure differed from the physiological appearance of the ligament tissue. Numerous collagen fibers seemed loosened with mast cell infiltrations in some cases (4; p = 0.8567). The overall screening revealed significantly better qualitative measures in group IV, the melatonin-supplemented diabetic group, compared to the values from group III, with diabetes (p = 0.01).
The process of dissection facilitates morphological and histological changes in the appearance of the MCL. The glistening white appearance under the microscope was not no longer recognizable. DM was found to be a cofactor for exacerbating the change. Severely affected fragments were grayish and amorphous, disproportionally distinguished into fusiform or nodular thickening portions. Compared to group III, the melatonin supplementation (group IV) brought statistically significant improvement in all the morphological and histological measures, p < 0.05. Melatonin supplementation in group II of animals without DM and with Mel supplementation diminished elastin fiber loss compared to the control group from 0.5 ± 0.2, p < 0.05 and 0, p < 0.05 for the right MCL samples from group I and the right MCL samples from group II, respectively. The same observation was made about the left MCL samples from group I, which had a score of 1.05 ± 0.55, p < 0.05, and the left MCL samples from group II, which had a score of 0. Melatonin supplementation in group II of animals without DM and with Mel supplementation did not cause any significant changes in other parameters.
3.3 Laboratory tests: blood plasma assessment of molecule concentration
Blood samples were drawn from the experimental animals in all groups (I–IV). In diabetic rats from group III, blood glucose, total lipids, cholesterol, and triglyceride presented significantly increased values (p < 0.05) compared to control animals (Table 4). However, group IV, the diabetic group that received melatonin, presented with reduced concentrations of blood glucose, triglyceride, total lipids, and cholesterol (p < 0.05).
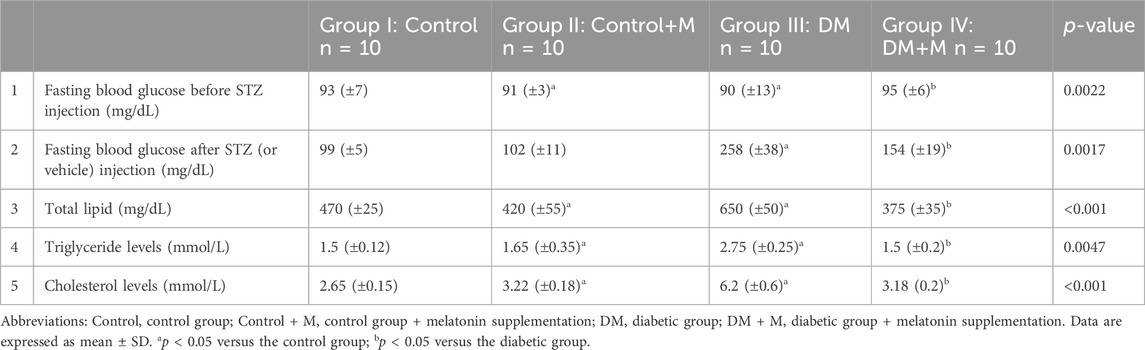
Table 4. Fasting blood glucose levels before (Leong et al., 2020) and after (Longo et al., 2009) STZ injection, total lipids (Nelson et al., 2006), triglyceride levels (Fleming et al., 2005), and cholesterol levels (Fleming, 2003) represented through statistical analysis of the different experimental groups.
Group II, which received melatonin, did not show any significant changes in any of the measured parameters compared to the healthy control group I. Fasting blood glucose levels from group III, the untreated DM group, increased significantly compared to the healthy control. Group IV, the DM+M group, showed higher fasting blood glucose levels than group I but significantly lower than those in group III, the DM group, at 258 ± 38 and 154 ± 19; p < 0.05, respectively.
All total lipid, triglyceride levels, and cholesterol levels increased significantly in non-treated diabetic group III, at 650 ± 50, 2.75 ± 0.25, and 6.2 ± 0.6, respectively, compared to healthy controls, at 470 ± 25, 1.5 ± 0.12, and 2.65 ± 0.15 for total lipids, triglycerides, and cholesterol, respectively, as shown in Table 5. A significant decline in total lipids, triglyceride levels, and cholesterol levels was observed in the melatonin-treated diabetic group IV (375 ± 35, 1.5 ± 0.2, 3.18 ± 0.2, respectively) compared to the non-treated diabetic group.
3.4 Laboratory tests: oxidative stress markers in blood plasma
Lipid peroxides and uric acid showed increased concentration in group III (p < 0.05), and reduced albumin concentration was found in group III (p < 0.05) (Table 5). Nitric oxide was also increased (p > 0.05) in group III, while total thiol and ceruloplasmin were reduced (p > 0.05) when compared to the group I. Group II significantly increased total thiol and ceruloplasmin activity. In addition, group II significantly decreased (p < 0.001, p < 0.05) lipid peroxides and uric acid, respectively. Rats group II only showed a decrease (p > 0.05) in nitric oxide. Group IV showed decreased values of LPO, NO and uric acid compared to group III. However the melatonin supplementation in group IV increased the results of total thiols, SOD, ceruroplasmin and albumin concentration in comarison to group III.
3.5 Laboratory tests: oxidative status of ligamentous homogenates
Ligament harvesting included samples for homogenization. The probes of ligament homogenates showed a significant increase in lipid peroxides in diabetic rats compared to the controls (p < 0.05) (Table 6). GST activities increased significantly (p < 0.001) in group IV, diabetic rats treated with melatonin. Total thiols presented with similar values (p < 0.05) in group II, rats treated with melatonin, and group I, the control group. Samples from group IV rats treated with melatonin showed significantly increased (p < 0.05, p < 0.001) activities of superoxide dismutase and catalase, respectively, compared to the samples from group III rats with DM and showed a similar range of values compared to the group I control.
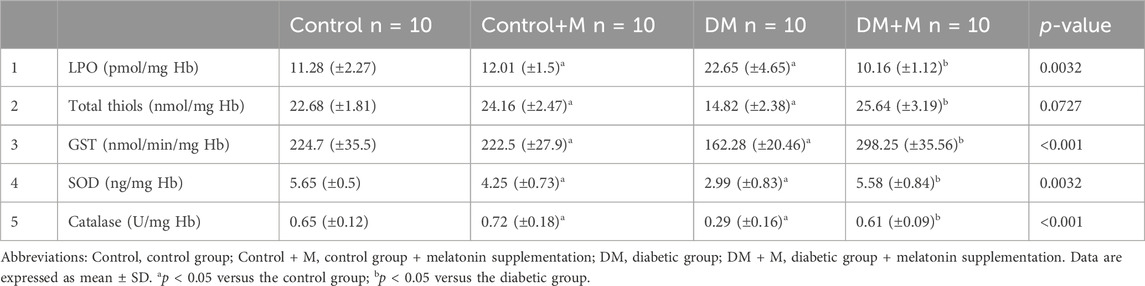
Table 6. Levels of lipid peroxides (LPO), total thiols, and the activities of superoxide dismutase (SOD), catalase, and glutathione S-transferase (GST) in the ligament homogenates of the different experimental groups.
Oxidative and antioxidative status were measured among all the groups (I–IV) (Figure 5). In the diabetic groups, it was found that TAS levels decreased while TOS, ROS levels, and OSI rates increased. These changes were found to be meaningful when compared to the control group (p < 0.0001, p < 0.0001, p < 0.0001, and p < 0.0001, respectively). Treatment with melatonin in diabetic rats mitigated these changes (p < 0.0001, p < 0.0001, p < 0.0001, p < 0.0001, respectively).
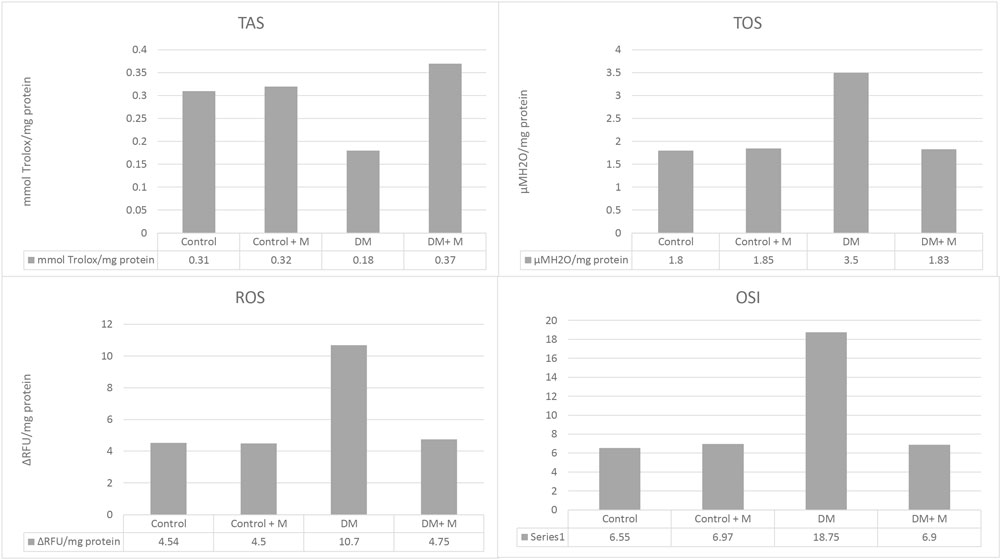
Figure 5. Ligament homogenate TAS, TOS, ROS levels, and OSI rates of all groups. Abbreviations: Control, control group; Control + M, control group + melatonin supplementation; DM, diabetic group; DM + M, diabetic group + melatonin supplementation; OSI, oxidative stress index; ROS, reactive oxygen species; TAS, total antioxidant status; TOS, total oxidant status. Abundance of groups: Control: n = 10; Control + M: n = 10; DM: n = 10; DM + M: n = 10. Data are expressed as mean ± SD. ap < 0.05 versus the control group; bp < 0.05 versus the diabetic group.
MDA and GSH concentrations were measured (Figure 6). The highest MDA value in the ligament homogenates was obtained in group III, the DM group. MDA levels in the bone tissue in group IV, with DM and melatonin supplementation, were lower than in group III, the DM group, but higher than in all other groups. The GSH values appeared higher in the melatonin-supplemented groups than in groups without supplementation. The highest GSH levels were measured in group IV, the diabetic group supplemented with melatonin.
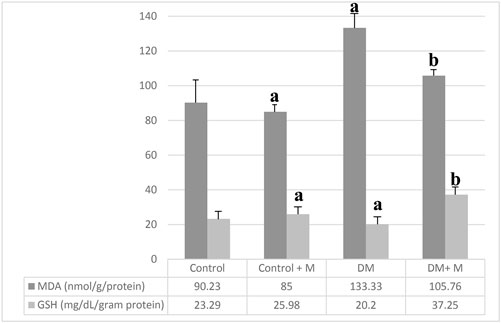
Figure 6. Levels of MDA and GSH in the various ligament homogenates. Abbreviations: Control, control group; Control + M, control group + melatonin supplementation; DM, diabetic group; DM + M, diabetic group + melatonin supplementation; MDA, malondialdehyde; GSH, glutathione. Data are expressed as mean ± SD. ap < 0.05 versus the control group; bp < 0.05 versus the diabetic group.
6 Discussion
Many factors are responsible for the successful healing and structural recovery of injured ligaments. Uncorrectable risk factors for poor healing potential after trauma or surgery are comorbidities, muscle degeneration and atrophy, autoimmunological diseases, larger tear size, poor soft tissue quality, repetitive trauma, smoking, and diabetes (Loots et al., 1999).
The results of our study evidence the high grade of morphological changes occurring in the ligaments in a hyperglycemic state. We confirm a positive relationship between hyperglycemia and immune response, inflammation, and extracellular matrix synthesis. In addition, high glucose levels have been shown to inhibit the proliferation and regeneration of ligament fibroblast cells, which might partly account for delayed regeneration and healing in animals with diabetes (Furnary et al., 1999; Damasceno et al., 2014a).
Ligaments play a significant role in stabilizing the body and carrying mechanical forces (Yamaguchi et al., 2006; Shemesh et al., 2018; Adamska et al., 2022).
The enhanced concentration of collagen cross-linking stimulated in inflammatory conditions of DM worsens the quality of tissue and disables its significant roles in the functioning of the body (Al-Mulla et al., 2011; Kato et al., 2015; Wilkinson et al., 2019a; Hippensteel et al., 2019).
Our study posits that DM significantly enables the physiological response to either stress or trauma. We provide evidence of the mechanisms responsible for the alteration of antioxidant defense. Furthermore, identifying specific molecular markers for ligament progenitor cells in adult tissues might help elucidate their contribution to ligament homeostasis and regeneration and would allow treatment of some of the complications of diabetes. Asahara et al. (2017) hypothesized that Egr-1 expression may be compromised in DM, leading to impaired collateral vessel growth and impairing healing and homeostasis. This evidence shows that DM provokes pathological tissue responses through multiple mechanisms.
Findings show a decreased proliferation rate, higher turnover, and breakdown of fibroblasts derived from soft tissues induced by DM (Lerman et al., 2003). The results from the histopathological examination of samples from rats with DM in groups III and IV presented a disintegrated structure, diminished fibroblast functioning, and hypercellularity, enabling physiological response to trauma. These suggest that excessive proliferation and an altered structure of the fibroblasts may contribute to poor production of the main component of ligaments, namely, collagen, and thus account for their impaired healing (Lerman et al., 2003; Ko et al., 2021).
Studies found that DM delays oral wound healing, which agrees with our finding of peripheral ligaments (Ko et al., 2021). Diabetic wounds were exacerbated by increased inflammation, as noted by greater numbers of neutrophils, enhanced re-epithelialization, and increased levels of TNF compared to wounds in nondiabetic patients (Vicente et al., 2021). In skin wounds, DM contributes to prolonged inflammation (Wang and Graves, 2020).
Ligament wounds have reduced angiogenic response compared to skin wounds in general because of excessive inflammation (Wilkinson et al., 2019b). Jeon et al. (2021) promote the primary benefit of inflammatory pathway inhibition in early phases of wound healing by enhanced production of connective tissue matrix, increased myofibroblast formation, and greater angiogenesis in diabetic wounds. This is important because reducing prolonged inflammation improves the healing of wounds in type 2 DM animals. Thulasingam et al. (2019) confirm that normoglycemia prevents complications and comorbidities in healthy mice and can reverse degeneration in DM mice. Our study confirmed that animals from normoglycemic groups I and II presented with better overall antioxidative status, and, in contrast, groups III and IV, namely, animals with DM, had high oxidative status values.
Endoplasmic reticulum (ER) stress mainly involves transcription factors. Although moderate ER stress can effectively protect the body, excessive ER stress causes degeneration, as happens during DM involvement (Ruan et al., 2021). Song et al. (2021) evidenced ER effects in apoptosis of the periodontal ligament (PDL) and vascular calcification in a rat model. Furthermore, our study confirms that DM also provokes those complications in the MCL.
There is evidence that streptozotocin-induced diabetes releases free radicals (Damasceno et al., 2014b). As glutathione is closely linked to glucose metabolism via NADPH, it is logical that free radical metabolism is altered in diabetes (Johansen et al., 2005).
In the present experiment, streptozotocin treatment caused a significant increase in lipid peroxidation in plasma. Increased glycation of collagen and plasma proteins in diabetes may stimulate the oxidation of lipids, which in turn may stimulate autooxidative reactions of sugars, enhancing damage to both lipids and proteins in the circulation and continuing the cycle of oxidative stress (Volpe et al., 2018; Ito et al., 2019).
It is evident from the present study that melatonin supplementation may help in the prevention and protection against the production of free radicals in diabetes. The antioxidant effects of melatonin caused a marked elevation in the antioxidant system activity and reduced oxidative stress.
Nitric oxide is produced in the endocrine pancreas and contributes to the synthesis and secretion of insulin. The potential role of NO in insulin secretion is disputable—both stimulatory and inhibitory effects have been reported (Gheibi and Ghasemi, 2020). In the present study, plasma nitrite as an end product of nitric oxide activity was elevated in the untreated diabetic rats. Similar results have been previously obtained in other studies (Anggård, 1994; Welsh et al., 1994; Nagareddy et al., 2006; Pacher et al., 2007).
Uric acid is considered a non-enzymatic antioxidant, but increased production of uric acid means increased free radical production due to activation of the xanthine oxidase enzyme system (Sekizuka, 2022). In our experiment, uric acid levels were increased in diabetic rats. In the present study, streptozotocin treatment caused a significant depletion of both enzymatic and non-enzymatic antioxidants in plasma or erythrocyte lysates or the homogenates of both ligaments and muscles. Accordingly, SOD treatment can protect in vivo or in vitro against the high toxic potential of the superoxide radicals in alloxan-induced diabetic rats (Gandy et al., 1982; Najafi et al., 2021). A significant decrease in plasma ceruloplasmin and albumin was also found. However, uric acid increased. In our experiment, melatonin treatment generally normalized oxidative stress in streptozotocin-induced diabetic rats. Melatonin treatment reduces oxidative stress (lipid peroxidation, nitric oxide, and uric acid) while elevating enzymatic and non-enzymatic antioxidant systems in blood, ligaments, and muscle homogenates. Anwar et al. reported that melatonin had a potent reducing effect on the production of lipid peroxides in rats exposed to cytotoxic drugs (Anwar and Meki, 2003). Nitric oxide, involved in the neuropathy that is one of the complications of diabetes as a result of oxidative stress, is reduced after melatonin treatment (Sáenz et al., 2002; Huang et al., 2015; Bicer et al., 2018; Hosseini et al., 2022). Storr et al. (2002) reported that melatonin inhibited nitric oxide synthase enzyme and reduced nitric oxide production. The reduction in uric acid levels after melatonin treatment may be due to the reduction of lipid peroxidation, triglycerides, and cholesterol, while the elevation of these substances may increase uric acid synthesis (Ferlazzo et al., 2020).
According to the following articles, the highest MDA levels in ligament tissues were found in diabetic groups that were not supplemented with melatonin. This result is consistent with literature, which states that oxidant stress that increased in diabetes had a negative impact on bone metabolism. What is more, it was reported that endurance exercise was able to enhance bone quality by restoring bone blood flow (Atalay et al., 1997; Hara et al., 1997; Turgut et al., 2003; Bae et al., 2018; Zhao et al., 2022; Marcucci et al., 2023).
However, those results differ from the results of a study conducted by Bicer et al. (2018). This study interestingly found continuously high bone MDA levels in the diabetic group despite subjecting them to swimming exercises. Those elevated MDA values that were established in the bone tissue demonstrate that the lipid peroxidation that is enhanced in diabetic rats cannot be offset by exercise.
The abovementioned results suggest that MDA values in the bone tissue in group IV, diabetic rats supplemented with melatonin, should be lower than the values in the diabetic group without supplementation but higher than those in all other groups. The results of the current study indicate that melatonin supplementation suppresses MDA values in diabetic groups. It was reported that melatonin had a protective effect on tissues throughout the body (Bazyar et al., 2021) and prevented lipid peroxidation in the bone tissue (Bazyar et al., 2022; Dludla et al., 2023). The current results confirm that it presents the same protective effects in bones. Group IV, the melatonin-supplemented diabetic group, had the highest bone tissue GSH values. This result demonstrates that melatonin supplementation significantly increases GSH levels in diabetic rats. Melatonin supplementation was also reported to significantly increase antioxidant activity in rats with induced diabetes.
The most pronounced effect of melatonin administration was the prevention of an increase in NO levels in blood plasma during streptozotocin-induced diabetes, which implies that melatonin may operate as an NO scavenger and carrier.
The biochemical analyses done in the present study prove the existence of oxidative stress in diabetes. These parameters are ROS, TOS, TAS, and OSI, and they indicate the level of accumulated oxidants and possible tissue damage caused by DM. The typical pathophysiology of oxidative stress provokes an ROS and TOS increase and a TAS level decrease. It is explained by TAS activity in the effective reduction of oxidative stress complications, and, furthermore, it shows an increased antioxidant level. The results are in accordance with the literature findings (Rehman and Akash, 2017; Hu et al., 2022).
In addition, melatonin diminishes the levels of oxidative stress in organisms directly by inducing the antioxidant defense systems (Görgün et al., 2002; Ghanbari et al., 2016; Raygan et al., 2019; Ertik et al., 2023). Melatonin can decrease oxidative stress by stimulating the gene expression of antioxidant enzymes such as SOD and GSH-Px (Abdulwahab et al., 2021; Grabia et al., 2023; Mirapoğlu et al., 2023; Rahbarghazi et al., 2023). Ahmad et al. (2018) found that 1 mg/kg of melatonin given to the diabetic group IV increased the GSH level, CAT, and SOD activities but decreased the LPO level in the pancreas. Albazal et al. (2021) found that the GSH levels and CAT and SOD activities decreased in diabetic animals, while LPO and ROS levels increased. Treatment with melatonin at 10 mg/kg/day for 7 weeks reversed the negative impact of oxidative stress (Ahmad et al., 2018). Another study reported that administration of 10 mg/kg melatonin to diabetic rats for 14 days improved oxidative stress parameters significantly (Albazal et al., 2021; Kahya and Nazıroğlu, 2016). In the diabetic group, GSH, TAS levels, and GPx activity diminished, while LPO, ROS, and TOS levels increased. It was observed that oxidative stress parameters were reversed upon administering melatonin to diabetic rats by reducing oxidative stress (Kahya and Nazıroğlu, 2016). The present study examined oxidative stress parameters such as TAS, TOS, ROS, and OSI. It was found that the oxidative stress in the bone tissue caused by diabetes decreased upon the administration of melatonin for 4 weeks. When both the effect of melatonin on antioxidant enzymes and the free radical scavenging effect are considered, the outcomes are compatible with previous reports.
Melatonin has a pleiotropic effect on the organism. Because many organs have melatonin receptors, it is a potent antioxidant, anti-inflammatory, free radical scavenger, and helpful in fighting inflammatory diseases (Guan et al., 2022). Melatonin receptors might have therapeutic potential as they belong to the G protein-coupled receptor superfamily and, therefore, are abundant throughout the organism. Understanding the role of melatonin and its receptors on glucose homeostasis is urgent because of the wide use of melatonin for many indications, either as a prescribed medication or as a supplement without medical prescription, in many countries (Gomes et al., 2021). The antagonistic relationship between melatonin and insulin defines its influence in regulating insulin secretion and action. Melatonin treatment improved glucose homeostasis, energy balance, and overall health in diabetes mellitus. Literature reports melatonin’s cytoprotective role as an antioxidant and free radical scavenger in combating oxidative stress, preserving beta-cell function, and influencing the development of diabetic complications (Wajid et al., 2020).
In the present study, an increased level of circulating melatonin was associated with a decrease in blood glucose level, reducing the pro-oxidant susceptibility and pro-inflammatory responses. The role played by melatonin on the endocrine system, including insulin metabolism and proving the presence of melatonin receptors in many different organs, allows us to assume that the processes taking place during regeneration, for example, at night when melatonin secretion is the highest, are not a coincidence, and that melatonin is a hormone that affects reactions regulating homeostasis (Guan et al., 2022). However, with age, the concentration of melatonin decreases, and its role becomes less important. Therefore, many researchers use exogenous melatonin to demonstrate a protective effect on circadian rhythm, immune system, response to inflammation, wound healing, and tissue regeneration (Karamitri and Jockers, 2019).
We aimed to evaluate the effect of melatonin administration in STZ-induced diabetic rats and healthy rats. Melatonin administration in STZ-induced diabetic rats reduced hyperglycemia, hyperlipidemia, and oxidative stress. Melatonin acted as an anti-inflammatory agent that reduced pro-inflammatory mechanisms and oxidative stress biomarkers, such as MDA, GSH, and NO. Melatonin succeeded in enhancing ligament cell regeneration under severe inflammation caused by surgical conditions and injury.
The authors of a study evaluating the role of melatonin in foot ulcer regeneration underlined its potential in reducing the risk of hyperglycemia, neuropathy, vascular damage, and immune system impairment in diabetic patients. All those characteristics are proven to play a role in ligament healing. Correspondingly, melatonin use brings promising results in diabetic patients with joint and soft tissue complications (Sajjadpour et al., 2023).
The results of the present study indicated that melatonin supplementation prevents increased free radical production and inhibits antioxidant activity resulting from diabetes in the bone tissue. Melatonin has many positive effects and antioxidant properties. In the present study, the effect of melatonin on diabetes was examined using different biochemical parameters. It was observed that melatonin had a healing effect and restored normal biochemical parameters. The data indicate that the use of melatonin with or without insulin may be effective in preventing or at least retarding the development of some diabetic complications.
6.1 Summary
Surgical intervention within the ligament contributes to the impaired healing potential and loss of the morphological structure in the group of animals with induced diabetes.
Inflammatory changes caused by autooxidative activities are high in diabetic groups. Melatonin supplementation in a group of animals with induced diabetes contributed to an increase in the concentration of antioxidants, providing a protective effect on the ligaments in conditions of hyperglycemia.
These findings evidence the degenerative influence of DM on the ligament tissue, inhibiting physiological protective mechanisms for oxidative stress. Treatment with melatonin in diabetic rats mitigated alterations and improved the antioxidant status of ligaments in the diabetic group.
Data availability statement
The raw data supporting the conclusions of this article will be made available by the authors, without undue reservation.
Ethics statement
The animal study was approved by the Institutional Ethics Committee of the Warsaw School of Applied Sciences. The study was conducted in accordance with the local legislation and institutional requirements.
Author contributions
OA: conceptualization, project administration, software, supervision, writing–original draft, and writing–review and editing. AW: conceptualization, software, and writing–review and editing. AK: investigation, visualization, and writing–review and editing. MP: methodology, software, validation, and writing–review and editing. BM: funding acquisition, project administration, supervision, and writing–review and editing. MK: investigation, resources, and writing–review and editing. AS: conceptualization, methodology, project administration, supervision, and writing–review and editing. MM: formal analysis, methodology, validation, and writing–review and editing. AA: formal analysis, funding acquisition, investigation, methodology, project administration, resources, visualization, and writing–original draft. MŁ: data curation, formal analysis, methodology, validation, visualization, and writing–review and editing. AJ: data curation, investigation, methodology, resources, and writing–review and editing.
Funding
The authors declare that financial support was received for the research, authorship, and/or publication of this article. The authors are thankful for the support from the Project: POPUL/SP/0120/2023/01.
Conflict of interest
The authors declare that the research was conducted in the absence of any commercial or financial relationships that could be construed as a potential conflict of interest.
Publisher’s note
All claims expressed in this article are solely those of the authors and do not necessarily represent those of their affiliated organizations, or those of the publisher, the editors, and the reviewers. Any product that may be evaluated in this article, or claim that may be made by its manufacturer, is not guaranteed or endorsed by the publisher.
References
Abdulwahab, D. A., El-Missiry, M. A., Shabana, S., Othman, A. I., and Amer, M. E. (2021). Melatonin protects the heart and pancreas by improving glucose homeostasis, oxidative stress, inflammation and apoptosis in T2DM-induced rats. Heliyon 7 (3), e06474. doi:10.1016/j.heliyon.2021.e06474
Adamska, O., Stolarczyk, A., Gondek, A., Maciąg, B., Świderek, J., Czuchaj, P., et al. (2022). Ligament alteration in diabetes mellitus. J. Clin. Med. 11 (19), 5719. doi:10.3390/jcm11195719
Ahmad, H. Y., Rai, S., Basheer, M., Ghosh, H., and Singh, S. (2018). Protective role of melatonin in streptozotocin induced pancreatic damages in diabetic wistar rat. Pak J. Biol. Sci. 21 (9), 423–431. doi:10.3923/pjbs.2018.423.431
Akbarzadeh, A., Norouzian, D., Mehrabi, M. R., Jamshidi, Sh, Farhangi, A., Verdi, A. A., et al. (2007). Induction of diabetes by Streptozotocin in rats. Indian J. Clin. Biochem. 22 (2), 60–64. doi:10.1007/BF02913315
Albazal, A., Delshad, A. A., and Roghani, M. (2021). Melatonin reverses cognitive deficits in streptozotocin-induced type 1 diabetes in the rat through attenuation of oxidative stress and inflammation. J. Chem. Neuroanat. 112, 101902. doi:10.1016/j.jchemneu.2020.101902
Al-Mulla, F., Leibovich, S. J., Francis, I. M., et al. (2011). Impaired TGF-β signaling and a defect in resolution of inflammation contribute to delayed wound healing in a female rat model of type 2 diabetes. Mol. Biosyst. (7), 11. doi:10.1039/c0mb00317d
Anggård, E. (1994). Nitric oxide: mediator, murderer, and medicine. Lancet. 343 (8907), 1199–1206. doi:10.1016/s0140-6736(94)92405-8
Anwar, M. M., and Meki, A. R. (2003). Oxidative stress in streptozotocin-induced diabetic rats: effects of garlic oil and melatonin. Comp. Biochem. Physiol. A Mol. Integr. Physiol. 135 (4), 539–547. doi:10.1016/s1095-6433(03)00114-4
Asahara, H., Inui, M., and Lotz, M. K. (2017). Tendons and ligaments: connecting developmental biology to musculoskeletal disease pathogenesis. J. Bone Min. Res. 32 (9), 1773–1782. doi:10.1002/jbmr.3199
Atalay, M., Laaksonen, D. E., Niskanen, L., Uusitupa, M., Hänninen, O., and Sen, C. K. (1997). Altered antioxidant enzyme defences in insulin-dependent diabetic men with increased resting and exercise-induced oxidative stress. Acta Physiol. Scand. 161 (2), 195–201. doi:10.1046/j.1365-201X.1997.00200.x
Atroshi, F., Sankari, S., Osterberg, S., and Sandholm, M. (1981). Variation of erythrocyte glutathione peroxidase activity in Finn sheep. Res. Vet. Sci. 31 (3), 267–271. PMID: 7342221. doi:10.1016/s0034-5288(18)32454-8
Bae, W. J., Park, J. S., Kang, S. K., Kwon, I. K., and Kim, E. C. (2018). Effects of melatonin and its underlying mechanism on ethanol-stimulated senescence and osteoclastic differentiation in human periodontal ligament cells and cementoblasts. Int. J. Mol. Sci. 19 (6), 1742. doi:10.3390/ijms19061742
Bazyar, H., Zare Javid, A., Bavi Behbahani, H., Moradi, F., Moradi Poode, B., and Amiri, P. (2021). Consumption of melatonin supplement improves cardiovascular disease risk factors and anthropometric indices in type 2 diabetes mellitus patients: a double-blind, randomized, placebo-controlled trial. Trials 22 (1), 231. doi:10.1186/s13063-021-05174-z
Bazyar, H., Zare Javid, A., Zakerkish, M., Yousefimanesh, H. A., and Haghighi-Zadeh, M. H. (2022). Effects of melatonin supplementation in patients with type 2 diabetes mellitus and chronic periodontitis under nonsurgical periodontal therapy: a double-blind randomized controlled trial. J. Res. Med. Sci. 27, 52. doi:10.4103/jrms.JRMS_927_19
Bicer, M., Akil, M., Avunduk, M. C., Kilic, M., Mogulkoc, R., and Baltaci, A. K. (2011). Interactive effects of melatonin, exercise and diabetes on liver glycogen levels. Endokrynol. Pol. 62 (3), 252–256.
Bicer, M., Baltaci, S. B., Patlar, S., Mogulkoc, R., and Baltaci, A. K. (2018). Melatonin has a protective effect against lipid peroxidation in the bone tissue of diabetic rats subjected to acute swimming exercise. Horm. Mol. Biol. Clin. Investig. 34, 34. doi:10.1515/hmbci-2017-0079
Damasceno, D. C., Netto, A. O., Iessi, I. L., Gallego, F. Q., Corvino, S. B., Dallaqua, B., et al. (2014b). Streptozotocin-induced diabetes models: pathophysiological mechanisms and fetal outcomes. Biomed. Res. Int. 2014, 819065. doi:10.1155/2014/819065
Damasceno, D. C., Netto, A. O., Iessi, I. L., Gallego, F. Q., Corvino, S. B., Dallaqua, B., et al. (2014a). Streptozotocin-induced diabetes models: pathophysiological mechanisms and fetal outcomes. Biomed. Res. Int. 2014, 819065. doi:10.1155/2014/819065
Ding, A. H., Nathan, C. F., and Stuehr, D. J. (1988). Release of reactive nitrogen intermediates and reactive oxygen intermediates from mouse peritoneal macrophages. Comparison of activating cytokines and evidence for independent production. J. Immunol. 141 (7), 2407–2412. PMID: 3139757. doi:10.4049/jimmunol.141.7.2407
Dludla, P. V., Ziqubu, K., Mabhida, S. E., Mazibuko-Mbeje, S. E., Hanser, S., Nkambule, B. B., et al. (2023). Dietary supplements potentially target plasma glutathione levels to improve cardiometabolic health in patients with diabetes mellitus: a systematic review of randomized clinical trials. Nutrients 15 (4), 944. doi:10.3390/nu15040944
Draper, H. H., and Hadley, M. (1990). Malondialdehyde determination as index of lipid peroxidation. Methods Enzymol. 186, 421–431. doi:10.1016/0076-6879(90)86135-i
Ellman, G. L. (1959). Tissue sulfhydryl groups. Arch. Biochem. Biophys. 82 (1), 70–77. doi:10.1016/0003-9861(59)90090-6
Erel, O. (2004). A novel automated direct measurement method for total antioxidant capacity using a new generation, more stable ABTS radical cation. Clin. Biochem. 37 (4), 277–285. doi:10.1016/j.clinbiochem.2003.11.015
Erel, O. (2005). A new automated colorimetric method for measuring total oxidant status. Clin. Biochem. 38 (12), 1103–1111. doi:10.1016/j.clinbiochem.2005.08.008
Ertik, O., Sener, G., and Yanardag, R. (2023). The effect of melatonin on glycoprotein levels and oxidative liver injury in experimental diabetes. J. Biochem. Mol. Toxicol. 37 (3), e23268. doi:10.1002/jbt.23268
Ferlazzo, N., Andolina, G., Cannata, A., Costanzo, M. G., Rizzo, V., Currò, M., et al. (2020). Is melatonin the cornucopia of the 21st century? Antioxidants 9 (11), 1088. doi:10.3390/antiox9111088
Fleming, B. C. (2003). Biomechanics of the anterior cruciate ligament. J. Orthop. Sports Phys. Ther. 33 (8), A13–A15.
Fleming, B. C., Hulstyn, M. J., Oksendahl, H. L., and Fadale, P. D. (2005). Ligament injury, reconstruction and osteoarthritis. Curr. Opin. Orthop. 16 (5), 354–362. doi:10.1097/01.bco.0000176423.07865.d2
Furnary, A. P., Zerr, K. J., Grunkemeier, G. L., and Starr, A. (1999). Continuous intravenous insulin infusion reduces the incidence of deep sternalwound infection in diabetic patients after cardiac surgical procedures. Ann. Thorac. Surg. 67, 352–360. doi:10.1016/s0003-4975(99)00014-4
Gandy, S. E., Buse, M. G., and Crouch, R. K. (1982). Protective role of superoxide dismutase against diabetogenic drugs. J. Clin. Investig. 70 (3), 650–658. doi:10.1172/jci110659
Ghanbari, E., Nejati, V., and Khazaei, M. (2016). Improvement in serum biochemical alterations and oxidative stress of liver and pancreas following use of royal jelly in streptozotocin-induced diabetic rats. Cell. J. 18 (3), 362–370. doi:10.22074/cellj.2016.4564
Gheibi, S., and Ghasemi, A. (2020). Insulin secretion: the nitric oxide controversy. EXCLI J. 19, 1227–1245. PMID: 33088259; PMCID: PMC7573190. doi:10.17179/excli2020-2711
Gomes, P. R. L., Vilas-Boas, E. A., Leite, E. D. A., Munhoz, A. C., Lucena, C. F., Amaral, F. G. d., et al. (2021). Melatonin regulates maternal pancreatic remodeling and B-cell function during pregnancy and lactation. J. Pineal Res. 71, e12717. doi:10.1111/jpi.12717
Görgün, F. M., Oztürk, Z., Gümüştaş, M. K., and Kökogu, E. (2002). Melatonin administration affects plasma total sialic acid and lipid peroxidation levels in streptozotocin induced diabetic rats. J. Toxicol. Environ. Health A 65 (10), 695–700. doi:10.1080/00984100290071045
Grabia, M., Socha, K., Soroczyńska, J., Bossowski, A., and Markiewicz-Żukowska, R. (2023). Determinants related to oxidative stress parameters in pediatric patients with type 1 diabetes mellitus. Nutrients 15 (9), 2084. doi:10.3390/nu15092084
Guan, Q., Wang, Z., Cao, J., Dong, Y., and Chen, Y. (2022). Mechanisms of melatonin in obesity: a review. Int. J. Mol. Sci. 23 (1), 218. doi:10.3390/ijms23010218
Habig, W. H., Pabst, M. J., and Jakoby, W. B. (1974). Glutathione S-transferases. J. Biol. Chem. 249 (22), 7130–7139. PMID: 4436300. doi:10.1016/s0021-9258(19)42083-8
Hara, M., Iigo, M., Ohtani-Kaneko, R., Nakamura, N., Suzuki, T., Reiter, R. J., et al. (1997). Administration of melatonin and related indoles prevents exercise-induced cellular oxidative changes in rats. Biol. Signals 6 (2), 90–100. doi:10.1159/000109113
Hill, C. L., Seo, G. S., Gale, D., Totterman, S., Gale, M. E., and Felson, D. T. (2005). Cruciate ligament integrity in osteoarthritis of the knee. Arthritis Rheum. 52 (3), 794–799. doi:10.1002/art.20943
Hippensteel, K. J., Johnson, J., McCormick, J., and Klein, S. (2019). A comparison of wound complications with surgical treatment of achilles tendon conditions using 2 surgical approaches. Foot Ankle Orthop. 4 (1), 2473011418814004. doi:10.1177/2473011418814004
Hosseini, A., Samadi, M., Baeeri, M., Rahimifard, M., and Haghi-Aminjan, H. (2022). The neuroprotective effects of melatonin against diabetic neuropathy: a systematic review of non-clinical studies. Front. Pharmacol. 13, 984499. doi:10.3389/fphar.2022.984499
Hu, A., Zou, H., Chen, B., and Zhong, J. (2022). Posttranslational modifications in diabetes: mechanisms and functions. Rev. Endocr. Metab. Disord. 23 (5), 1011–1033. Epub 2022 Jun 13. doi:10.1007/s11154-022-09740-x
Huang, C. C., Lai, C. J., Tsai, M. H., Wu, Y. C., Chen, K. T., Jou, M. J., et al. (2015). Effects of melatonin on the nitric oxide system and protein nitration in the hypobaric hypoxic rat hippocampus. BMC Neurosci. 16, 61. doi:10.1186/s12868-015-0199-6
Ito, F., Sono, Y., and Ito, T. (2019). Measurement and clinical significance of lipid peroxidation as a biomarker of oxidative stress: oxidative stress in diabetes, atherosclerosis, and chronic inflammation. Antioxidants 8 (3), 72. doi:10.3390/antiox8030072
Jeon, H. H., Yu, Q., Witek, L., Lu, Y., Zhang, T., Stepanchenko, O., et al. (2021). Clinical application of a FOXO1 inhibitor improves connective tissue healing in a diabetic minipig model. Am. J. Transl. Res. 13 (2), 781–791. Published 2021 Feb 15.
Johansen, J. S., Harris, A. K., Rychly, D. J., and Ergul, A. (2005). Oxidative stress and the use of antioxidants in diabetes: linking basic science to clinical practice. Cardiovasc Diabetol. 4, 5. doi:10.1186/1475-2840-4-5
Kahya, M. C., and Nazıroğlu, M. (2016). Melatonin reduces lens oxidative stress level in STZ-induced diabetic rats through supporting glutathione peroxidase and reduced glutathione values. J. Cell. Neurosci. Oxidative Stress 8 (2), 588–594. doi:10.37212/jcnos.334113
Karamitri, A., and Jockers, R. (2019). Melatonin in type 2 diabetes mellitus and obesity. Nat. Rev. Endocrinol. 15, 105–125. doi:10.1038/s41574-018-0130-1
Kato, S., Saito, M., Funasaki, H., and Marumo, K. (2015). Distinctive collagen maturation process in fibroblasts derived from rabbit anterior cruciate ligament, medial collateral ligament, and patellar tendon in vitro. Knee Surg. Sports Traumatol. Arthrosc. 23 (5), 1384–1392. doi:10.1007/s00167-013-2773-8
Knight, J. A., Anderson, S., and Rawle, J. M. (1972). Chemical basis of the sulfo-phospho-vanillin reaction for estimating total serum lipids. Clin. Chem. 18 (3), 199–202. PMID: 5020813. doi:10.1093/clinchem/18.3.199
Ko, K. I., Sculean, A., and Graves, D. T. (2021). Diabetic wound healing in soft and hard oral tissues. Transl. Res. 236, 72–86. doi:10.1016/j.trsl.2021.05.001
Leong, N. L., Kator, J. L., Clemens, T. L., James, A., Enamoto-Iwamoto, M., and Jiang, J. (2020). Tendon and ligament healing and current approaches to tendon and ligament regeneration. J. Orthop. Res. 38 (1), 7–12. doi:10.1002/jor.24475
Lerman, O. Z., Galiano, R. D., Armour, M., Levine, J. P., and Gurtner, G. C. (2003). Cellular dysfunction in the diabetic fibroblast: impairment in migration, vascular endothelial growth factor production, and response to hypoxia. Am. J. Pathol. 162 (1), 303–312. doi:10.1016/S0002-9440(10)63821-7
Longo, U. G., Ronga, M., and Maffulli, N. (2009). Acute ruptures of the achilles tendon. Sports Med. Arthrosc. Rev. 17 (2), 127–138. doi:10.1097/JSA.0b013e3181a3d767
Loots, M. A., Lamme, E. N., Mekkes, J. R., Bos, J. D., and Middelkoop, E. (1999). Cultured fibroblasts from chronic diabetic wounds on the lower extremity (non-insulin-dependent diabetes mellitus) show disturbed proliferation. Arch. Dermatol Res. 291 (2-3), 93–99. doi:10.1007/s004030050389
Luck, H. (1963). “Catalase,” in Methods of enzymatic analysis. Editor H. U. Bergmeyer (New York: Academic Press), 885–888.
Marcucci, G., Domazetovic, V., Nediani, C., Ruzzolini, J., Favre, C., and Brandi, M. L. (2023). Oxidative stress and natural antioxidants in osteoporosis: novel preventive and therapeutic approaches. Antioxidants (Basel) 12 (2), 373. doi:10.3390/antiox12020373
Mirapoğlu, S. L., Kaymakcı, A., Akın, S., Gültekin, F., and Güler, E. M. (2023). Oxidative stress and inflammation markers in undescended testes patients. Med. Rec. 5 (1), 29–32. doi:10.37990/medr.1140268
Misra, H. P., and Fridovich, I. (1972). The role of superoxide anion in the autoxidation of epinephrine and a simple assay for superoxide dismutase. J. Biol. Chem. 247 (10), 3170–3175. PMID: 4623845. doi:10.1016/s0021-9258(19)45228-9
Mullaji, A. B., Marawar, S. V., Simha, M., and Jindal, G. (2008). Cruciate ligaments in arthritic knees: a histologic study with radiologic correlation. J. Arthroplasty 23 (4), 567–572. doi:10.1016/j.arth.2007.05.024
Nagareddy, P. R., Xia, Z., MacLeod, K. M., and McNeill, J. H. (2006). N-acetylcysteine prevents nitrosative stress-associated depression of blood pressure and heart rate in streptozotocin diabetic rats. J. Cardiovasc Pharmacol. 47 (4), 513–520. doi:10.1097/01.fjc.0000211744.93701.25
Najafi, A., Pourfarzam, M., and Zadhoush, F. (2021). Oxidant/antioxidant status in Type-2 diabetes mellitus patients with metabolic syndrome. J. Res. Med. Sci. 26, 6. doi:10.4103/jrms.JRMS_249_20
Nelson, F., Billinghurst, R. C., Pidoux, I., Reiner, A., Langworthy, M., McDermott, M., et al. (2006). Early post-traumatic osteoarthritis-like changes in human articular cartilage following rupture of the anterior cruciate ligament. Osteoarthr. Res. Soc. 14 (2), 114–119. doi:10.1016/j.joca.2005.08.005
Pacher, P., Beckman, J. S., and Liaudet, L. (2007). Nitric oxide and peroxynitrite in health and disease. Physiol. Rev. 87 (1), 315–424. doi:10.1152/physrev.00029.2006
Parasuraman, S., Raveendran, R., and Kesavan, R. (2010). Blood sample collection in small laboratory animals. J. Pharmacol. Pharmacother. 1 (2), 87–93. doi:10.4103/0976-500X.72350
Rahbarghazi, A., Alamdari, K. A., Rahbarghazi, R., and Salehi-Pourmehr, H. (2023). Co-administration of exercise training and melatonin on the function of diabetic heart tissue: a systematic review and meta-analysis of rodent models. Diabetol. Metab. Syndr. 15 (1), 67. doi:10.1186/s13098-023-01045-6
Raygan, F., Ostadmohammadi, V., Bahmani, F., Reiter, R. J., and Asemi, Z. (2019). Melatonin administration lowers biomarkers of oxidative stress and cardio-metabolic risk in type 2 diabetic patients with coronary heart disease: a randomized, double-blind, placebo-controlled trial. Clin. Nutr. 38 (1), 191–196. doi:10.1016/j.clnu.2017.12.004
Rehman, K., and Akash, M. S. H. (2017). Mechanism of generation of oxidative stress and pathophysiology of type 2 diabetes mellitus: how are they interlinked? J. Cell. Biochem. 118 (11), 3577–3585. Epub 2017 May 31. doi:10.1002/jcb.26097
Ruan, L., Li, F., Li, S., Zhang, M., Wang, F., Lv, X., et al. (2021). Effect of different exercise intensities on hepatocyte apoptosis in HFD-induced NAFLD in rats: the possible role of endoplasmic reticulum stress through the regulation of the IRE1/JNK and eIF2α/CHOP signal pathways. Oxid. Med. Cell. Longev. 2021, 6378568. Published 2021 Mar 15. doi:10.1155/2021/6378568
Sáenz, D. A., Turjanski, A. G., Sacca, G. B., Marti, M., Doctorovich, F., Sarmiento, M. I., et al. (2002). Physiological concentrations of melatonin inhibit the nitridergic pathway in the Syrian hamster retina. J. Pineal Res. 33 (1), 31–36. doi:10.1034/j.1600-079x.2002.01880.x
Sairyo, K., Biyani, A., Goel, V. K., Leaman, D. W., Booth, R., Thomas, J., et al. (2007). Lumbar ligamentum flavum hypertrophy is due to accumulation of inflammation-related scar tissue. Spine (Phila Pa 1976) 32 (11), E340–E347. doi:10.1097/01.brs.0000263407.25009.6e
Sajjadpour, Z., Hoseini Tavassol, Z., Aghaei Meybodi, H. R., Eskandarynasab, M., Pejman Sani, M., Hasani-Ranjbar, S., et al. (2023). Evaluating the effectiveness of melatonin in reducing the risk of foot ulcers in diabetic patients. J. Diabetes Metab. Disord. 22 (2), 1073–1082. Published 2023 Sep 9. doi:10.1007/s40200-023-01289-z
Schosinsky, K. H., Lehmann, H. P., and Beeler, M. F. (1974). Measurement of ceruloplasmin from its oxidase activity in serum by use of o-dianisidine dihydrochloride. Clin. Chem. 20 (12), 1556–1563. doi:10.1093/clinchem/20.12.1556
Sekizuka, H. (2022). Uric acid, xanthine oxidase, and vascular damage: potential of xanthine oxidoreductase inhibitors to prevent cardiovascular diseases. Hypertens. Res. 45 (5), 772–774. doi:10.1038/s41440-022-00891-7
Shemesh, S., Sidon, E., Kaisler, E., Sheinis, D., Velkes, S., Ohana, N., et al. (2018). Diabetes mellitus is associated with increased elastin fiber loss in ligamentum flavum of patients with lumbar spinal canal stenosis: results of a pilot histological study. Eur. Spine J. 27 (7), 1614–1622. doi:10.1007/s00586-017-5315-0
Song, X., Li, J., Jiao, M., Chen, Y., and Pan, K. (2021). Effect of endoplasmic reticulum stress-induced apoptosis in the role of periodontitis on vascular calcification in a rat model. J. Mol. Histol. 52 (5), 1097–1104. doi:10.1007/s10735-021-10015-z
Sotoudeh, N., and Namavar, M. R. (2022). Optimisation of ketamine-xylazine anaesthetic dose and its association with changes in the dendritic spine of CA1 hippocampus in the young and old male and female Wistar rats. Vet. Med. Sci. 8 (6), 2545–2552. doi:10.1002/vms3.936
Stolarczyk, A., Sarzyńska, S., Gondek, A., and Cudnoch-Jędrzejewska, A. (2018). Influence of diabetes on tissue healing in orthopaedic injuries. Clin. Exp. Pharmacol. Physiol. 45 (7), 619–627. doi:10.1111/1440-1681.12939
Storr, M., Koppitz, P., Sibaev, A., Saur, D., Kurjak, M., Franck, H., et al. (2002). Melatonin reduces non-adrenergic, non-cholinergic relaxant neurotransmission by inhibition of nitric oxide synthase activity in the gastrointestinal tract of rodents in vitro. J. Pineal Res. 33 (2), 101–108. doi:10.1034/j.1600-079x.2002.02909.x
Thulasingam, S., Krishnasamy, S., Raj, C. D., Lasch, M., Vedantham, S., and Deindl, E. (2019). Insulin treatment forces arteriogenesis in diabetes mellitus by upregulation of the early growth response-1 (Egr-1) pathway in mice. Int. J. Mol. Sci. 20 (13), 3320. doi:10.3390/ijms20133320
Trouchon, T., and Lefebvre, S. (2016). A review of enrofloxacin for veterinary use. Open J. Veterinary Med. 6, 40–58. doi:10.4236/ojvm.2016.62006
Turgut, M., Uslu, S., Uysal, A., Yurtseven, M. E., and Ustün, H. (2003). Changes in vascularity of cartilage endplate of degenerated intervertebral discs in response to melatonin administration in rats. Neurosurg. Rev. 26 (2), 133–138. doi:10.1007/s10143-003-0259-8
Vicente, A., Bravo-González, L. A., Navarro, J. A., Buendía, A. J., and Camacho-Alonso, F. (2021). Effects of diabetes on oxidative stress, periodontal ligament fiber orientation, and matrix metalloproteinase 8 and 9 expressions during orthodontic tooth movement. Clin. Oral Investig. 25, 1383–1394. doi:10.1007/s00784-020-03446-7
Volpe, C. M. O., Villar-Delfino, P. H., Dos Anjos, P. M. F., and Nogueira-Machado, J. A. (2018). Cellular death, reactive oxygen species (ROS) and diabetic complications. Cell. Death Dis. 9 (2), 119. doi:10.1038/s41419-017-0135-z
Wajid, F., Poolacherla, R., Mim, F. K., Bangash, A., and Rutkofsky, I. H. (2020). Therapeutic potential of melatonin as a chronobiotic and cytoprotective agent in diabetes mellitus. J. Diabetes Metab. Disord. 19 (2), 1797–1825. Published 2020 Jul 21. doi:10.1007/s40200-020-00585-2
Wang, Y., and Graves, D. T. (2020). Keratinocyte function in normal and diabetic wounds and modulation by FOXO1. J. Diabetes Res. 2020, 3714704–3714709. doi:10.1155/2020/3714704
Welsh, N., Eizirik, D. L., and Sandler, S. (1994). Nitric oxide and pancreatic beta-cell destruction in insulin dependent diabetes mellitus: don't take NO for an answer. Autoimmunity 18 (4), 285–290. doi:10.3109/08916939409009530
Wilkinson, H. N., Clowes, C., Banyard, K. L., Matteuci, P., Mace, K. A., and Hardman, M. J. (2019a). Elevated local senescence in diabetic wound healing is linked to pathological repair via CXCR2. J. Investig. Dermatol. 139 (5), 1171–1181. doi:10.1016/j.jid.2019.01.005
Wilkinson, H. N., Clowes, C., Banyard, K. L., Matteuci, P., Mace, K. A., and Hardman, M. J. (2019b). Elevated local senescence in diabetic wound healing is linked to pathological repair via CXCR2. J. Investig. Dermatol 139 (5), 1171–1181. doi:10.1016/j.jid.2019.01.005
Yamaguchi, K., Ditsios, K., Middleton, W. D., Hildebolt, C. F., Galatz, L. M., and Teefey, S. A. (2006). The demographic and morphological features of rotator cuff disease. A comparison of asymptomatic and symptomatic shoulders. J. bone Jt. Surg. Am. volume 88 (8), 1699–1704. doi:10.2106/JBJS.E.00835
Keywords: ligaments alteration in diabetes mellitus, diabetes mellitus, ligaments alteration, orthopedic complications, diabetes ligaments
Citation: Adamska O, Wnuk A, Kamińska A, Poniatowska M, Maciąg B, Kamiński M, Stolarczyk A, Matin M, Atanasov AG, Łapiński M and Jóźwik A (2024) Melatonin supplementation counteracts fiber loss in knee ligaments of diabetes-induced rats. Front. Pharmacol. 15:1399719. doi: 10.3389/fphar.2024.1399719
Received: 12 March 2024; Accepted: 24 June 2024;
Published: 29 July 2024.
Edited by:
Raghuram Kandimalla, James Graham Brown Cancer Center, United StatesReviewed by:
Raman Khurana, O2M Technologies, LLC, United StatesSrinivasa Rao Sirasanagandla, Sultan Qaboos University, Oman
Copyright © 2024 Adamska, Wnuk, Kamińska, Poniatowska, Maciąg, Kamiński, Stolarczyk, Matin, Atanasov, Łapiński and Jóźwik. This is an open-access article distributed under the terms of the Creative Commons Attribution License (CC BY). The use, distribution or reproduction in other forums is permitted, provided the original author(s) and the copyright owner(s) are credited and that the original publication in this journal is cited, in accordance with accepted academic practice. No use, distribution or reproduction is permitted which does not comply with these terms.
*Correspondence: Olga Adamska, b2xnYWFkYW05OEBnbWFpbC5jb20=
†ORCID: Olga Adamska, orcid.org/0000-0003-1875-2510; Agnieszka Kamińska, orcid.org/0000-0001-6977-6411; Artur Stolarczyk, orcid.org/0000-0002-5633-8153; Atanas G. Atanasov, orcid.org/0000-0003-2545-0967; Artur Jóźwik, orcid.org/0000-0001-5546-9891