- Department of Human Genetics, Emory University, Atlanta, GA, United States
Patients with mutations that alter the function of the sodium channel SCN8A present with a range of clinical features, including mild to severe seizures, developmental delay, intellectual disability, autism, feeding dysfunction, motor impairment, and hypotonia. In an effort to identify compounds that could be potentially beneficial in SCN8A-associated epilepsy, Atkin et al. conducted an in vitro screen which resulted in the identification of 90 compounds that effectively reduced sodium influx into the cells expressing the human SCN8A R1872Q mutation. The top compounds that emerged from this screen included amitriptyline, carvedilol, and nilvadipine. In the current study, we evaluated the ability of these three compounds to increase resistance to 6 Hz or pentylenetetrazole (PTZ)-induced seizures in wild-type CF1 mice and in a mouse line expressing the human SCN8A R1620L mutation. We also evaluated the effects of fenfluramine administration, which was recently associated with a 60%–90% decrease in seizure frequency in three patients with SCN8A-associated epilepsy. While amitriptyline, carvedilol, and fenfluramine provided robust protection against induced seizures in CF1 mice, only carvedilol was able to significantly increase resistance to 6 Hz- and PTZ-induced seizures in RL/+ mutants. These results provide support for further evaluation of carvedilol as a potential treatment for patients with SCN8A mutations.
Introduction
The first SCN8A-associated epilepsy mutation was identified in 2012 (Veeramah et al., 2012), and since then, over 250 SCN8A mutations have been reported (Johannesen et al., 2022). SCN8A encodes the voltage-gated sodium channel (VGSC) Nav1.6 which is localized to the axon initial segment and the nodes of Ranvier (Caldwell et al., 2000; Boiko et al., 2001; Boiko et al., 2003) where it plays an important role in regulating neuronal excitability (Caldwell et al., 2000; Boiko et al., 2001; Boiko et al., 2003). Patients with SCN8A mutations present with a wide range of clinical features, including mild to severe seizures, developmental delay, intellectual disability, autism, feeding dysfunction, motor impairment, and hypotonia (Johannesen et al., 2022; Andrews et al., 2023). Thus, it is possible that multiple treatment strategies may be necessary to effectively address the range of clinical phenotypes. Gain-of-function SCN8A mutations have been shown to increase persistent and resurgent sodium currents in vitro and in vivo (Lopez-Santiago et al., 2017; Liu et al., 2019; Wengert et al., 2019), and drugs that reduce these sodium currents can mitigate neuronal hyperexcitability (Wengert et al., 2019). While randomized clinical trials for SCN8A-associated disease have not yet been completed, high doses of sodium channel blockers are efficacious in some patients. In a study by Gardella and others, the sodium channel blockers oxcarbazepine, carbamazepine, and phenytoin, or benzodiazepines provided the best seizure control in a cohort of 22 patients with SCN8A mutations (Gardella et al., 2018).
In an effort to find additional compounds that could be beneficial in SCN8A-associated epilepsy, Atkin et al., conducted an in vitro screen of 1,320 pharmaceutical compounds to identify those that reduce sodium influx into HEK293 cells transfected with constructs expressing either wild-type SCN8A or the SCN8A R1872Q mutation. From this screen, 90 compounds displayed inhibition at a level greater than twice the standard deviation (≥63% inhibition). Amitriptyline, carvedilol, and nilvadipine emerged as lead compounds, and additional electrophysiological analyses showed that these three drugs inhibited sodium currents at levels that were comparable to carbamazepine, an established anti-seizure drug (Atkin et al., 2018). In addition, the serotonergic drug fenfluramine is gaining interest as a possible treatment for SCN8A-associated epilepsy. Previously used in the treatment of depression and obesity, fenfluramine recently received Food and Drug Administration (FDA) approval for the treatment of Dravet syndrome, Lennox-Gastaut syndrome, and Tuberous Sclerosis Complex. In a recent case report, fenfluramine was found to decrease seizure frequency by 60%–90% in three patients with SCN8A-associated epilepsy (Aledo-Serrano et al., 2022).
In the current study, we evaluated the ability of amitriptyline, carvedilol, nilvadipine, and fenfluramine to increase resistance to induced seizures in CF1 wild-type (WT) mice and in mice expressing the human SCN8A R1620L mutation which was identified in a patient with behavioral seizures, ADHD, autism, and social behavior challenges (Rossi et al., 2017). We previously reported that heterozygous mutants (RL/+) exhibit increased susceptibility to induced seizures, infrequent spontaneous seizures, and several behavioral deficits (Wong et al., 2021a). We selected the RL/+ mutants for this in vivo drug screen as these mutants do not exhibit the high rate of premature death observed in other SCN8A epilepsy mouse models (Wagnon et al., 2015; Bunton-Stasyshyn et al., 2019). Furthermore, the R1620L mutation is associated with both gain-of-function and loss-of-function properties (Liu et al., 2019; Wong et al., 2021a); thus, we speculated that the RL/+ mutants would provide the opportunity to identify drugs that could be broadly therapeutic. In the current study, we found that amitriptyline, carvedilol, and fenfluramine increased resistance against induced seizures in WT mice. However, only carvedilol was able to provide significant protection against 6 Hz- and pentylenetetrazole-induced seizures in the RL/+ mutants.
Materials and methods
Animals
Two-month-old wild-type CF1 males (Stock No. 023, Charles River) were used to generate the dose-response curves and for the 6 Hz and pentylenetetrazole (PTZ) seizure induction experiments. Heterozygous Scn8a R1620L (RL/+) mutants and WT littermates (2–4 months) at the N10 generation were used for 6 Hz and PTZ seizure induction. RL/+ mutants and WT littermates were genotyped as previously described (Wong et al., 2021a) and maintained on a C57BL/6J background (Stock No. 000664, Jackson Laboratories). Mice were housed on a 12H light-dark cycle and food and water were provided ad libitum. All experiments were conducted in accordance with the guidelines of the Emory University Institutional Animal Care and Use Committee and the Animal Research: Reporting of In Vivo Experiments (ARRIVE) guidelines.
6 Hz seizure induction
6 Hz seizures were induced as previously described (Wong et al., 2016; Lamar et al., 2017; Shapiro et al., 2019; Wong et al., 2019; Inglis et al., 2020; Wong et al., 2021a; Wong et al., 2021b; Shapiro et al., 2022; Shiu et al., 2022). A topical anesthetic (proparacaine hydrochloride) was administered to the cornea of the mice prior to corneal stimulation (6 Hz, 0.2 ms pulse width, 3 s) using a constant current device (ECT unit, 57800; Ugo Basile, Comerio, Italy). Following corneal stimulation, behavioral seizure responses were scored using a modified Racine scale (RS): RS0, no behavioral seizure response, RS1, immobile ≥3 s, RS2, forelimb clonus, head bobbing, paw waving; and RS3, generalized tonic-clonic seizure (GTCS) with loss of posture. CF1 mice were tested at current intensities of 22 or 44 mA, and RL/+ mutants and WT littermates were tested at 16 mA.
Pentylenetetrazole seizure induction
Pentylenetetrazole (PTZ) seizure induction was performed as previously described (Wong et al., 2016; Shapiro et al., 2019; Wong et al., 2019; Wong et al., 2021a; Wong et al., 2021b; Shapiro et al., 2022). PTZ was dissolved in 0.9% saline and administered subcutaneously to CF1 mice (85 mg/kg) and RL/+ mutants and WT littermates (100 mg/kg). Mice were observed for 30 min, and the latencies to the first myoclonic jerk (MJ) and GTCS were recorded.
Pharmaceutical compounds
Amitriptyline hydrochloride (Fisher Scientific) and fenfluramine hydrochloride (Millipore Sigma) were dissolved in 0.9% saline. Carvedilol (VWR) and nilvadipine (VWR) were dissolved in 30% DMSO and 0.9% saline. All pharmaceutical compounds were administered intraperitoneally.
Statistical analyses
For dose-response curves, a Kruskal-Wallis test followed by Dunn’s multiple comparisons was used to compare the effect of different doses of each drug on Racine scores following 6 Hz seizure induction in CF1 mice. A Mann-Whitney test was used to compare vehicle and treatment following 6 Hz seizure induction in CF1 mice. A Friedman’s test was used to compare the effect of treatment on 6 Hz seizures in the RL/+ mutants and WT littermates. A log-rank Mantel-Cox test was used to compare the effect of vehicle and treatment on PTZ-induced seizures. Data are presented as mean ± SEM with p ≤ 0.05.
Results
Amitriptyline and carvedilol protect against induced seizures in CF1 mice
We selected amitriptyline (AMI), carvedilol (CVD), and nilvadipine for testing in the Scn8aR1620L/+ (RL/+) mouse line based on a previous in vitro screen that suggested these drugs might be efficacious in SCN8A-derived epilepsy due to their ability to inhibit sodium influx (Atkin et al., 2018). Fenfluramine (FF) was selected because it is efficacious in patients with Dravet syndrome and Lennox-Gastaut syndrome (Ceulemans et al., 2012; Ceulemans et al., 2016; Knupp et al., 2022; Knupp et al., 2023), and was recently reported to reduce seizure frequency in three patients with SCN8A-derived epilepsy (Aledo-Serrano et al., 2022).
Table 1 provides a summary of all of the results. For each drug, we first generated a ¼ log dose-response curve based on the ability to increase resistance to 6 Hz seizures in CF1 mice. The range of drug doses tested was based on previous studies in mouse models of other neurological disorders (Parra et al., 2002; El-Kharashi and Abd El Samad, 2011; Goel et al., 2011; Goel, 2013; Vanelderen et al., 2013; Morin et al., 2018; Morin et al., 2020). We found that significant protection against 6 Hz seizures was achieved at a dose of 30 mg/kg AMI; five of 8 CF1 mice treated with this dose did not exhibit a seizure (RS0; Figure 1A). In a separate cohort of CF1 mice, we found that 30 mg/kg AMI prevented 6 Hz-induced seizures in all treated mice, confirming effective protection (Figure 1B). We next tested whether 30 mg/kg AMI could also protect against 6 Hz seizures induced at twice the convulsive current (2xCC, 44 mA), which is a current intensity previously shown in CF1 mice to be predictive of drugs that might be efficacious in refractory epilepsies (Barton et al., 2001). However, while 33% (3/9) of AMI-treated mice did not exhibit a behavioral seizure at 2xCC (RS0 score), this was not statistically different from the response of vehicle-treated CF1 mice (Figure 1C). Finally, we found that AMI (30 mg/kg) administration also resulted in a robust increase in the latency to the first GTCS following PTZ administration in the CF1 mice (Figure 1D). Notably, six of 10 (60%) of AMI-treated CF1 mice did not have a GTCS during the 30-min observation, while all vehicle-treated CF1 mice exhibited a GTCS.
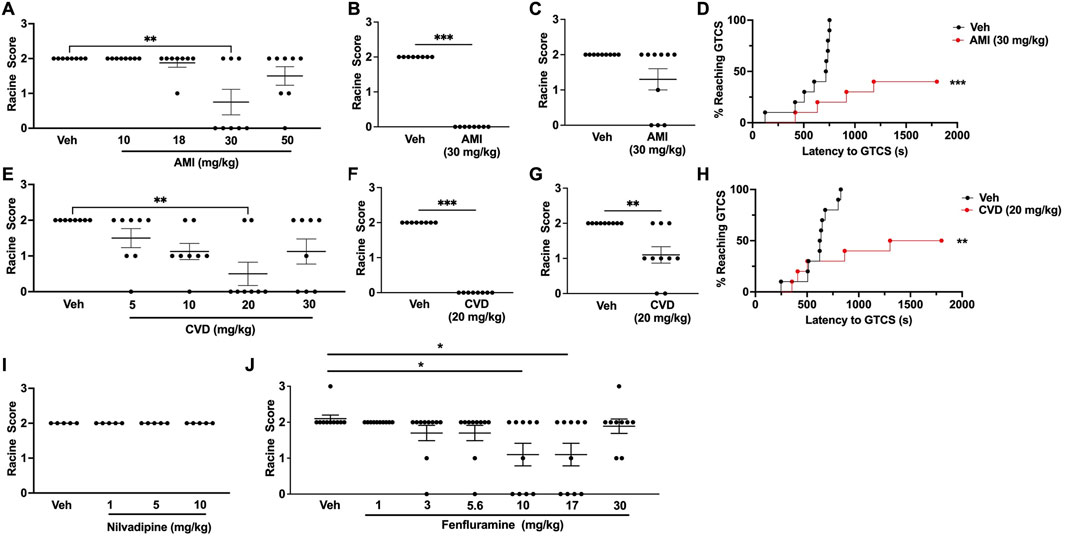
Figure 1. Generation of dose-response curves in CF1 wild-type mice. (A) Treatment with 30 mg/kg amitriptyline (AMI) was able to significantly increase resistance against 6 Hz-induced seizures in CF1 wild-type (WT) mice. N = 8/group. (B) In a separate cohort of mice, we confirmed that 30 mg/kg AMI was able to protect against 6 Hz seizures (22 mA). N = 8/group. (C) When tested at twice the convulsive current (2xCC, 44 mA), 30 mg/kg AMI did not protect against 6 Hz seizures. N = 9/group. (D) 30 mg/kg AMI was able to increase the latency to the first generalized tonic-clonic seizure (GTCS) following administration of pentylenetetrazole (PTZ). Six of 10 AMI-treated mice were completely protected against PTZ-induced seizures. N = 10/group. (E) Carvedilol (CVD, 20 mg/kg) was able to significantly increase resistance against 6 Hz seizures. N = 8/group. (F) We confirmed 20 mg/kg CVD protects against 6 Hz-induced seizures in a separate cohort of mice (22 mA). N = 8/group. (G) At 2xCC, 20 mg/kg CVD also significantly increased resistance against 6 Hz seizures. N = 9–10/group. (H) CVD (20 mg/kg) was also able to increase the latency to the first GTCS following PTZ administration. Of the CVD-treated mice, 50% did not exhibit a GTCS during the 30-min observation period. N = 10/group. (I) Nilvadipine did not increase resistance against 6 Hz-induced seizures at the doses tested. N = 5/group. (J) We found that 10 and 17 mg/kg fenfluramine was able to protect against 6 Hz seizures. N = 9–10/group. *p ≤ 0.05, **p < 0.01, ***p < 0.001.
We observed a dose-dependent response with carvedilol, in which increasing CVD doses provided greater protection against 6 Hz-induced seizures, with maximum protection achieved with 20 mg/kg CVD (Figure 1E). At this dose of CVD, six of 8 CF1 mice were protected against 6 Hz seizures (RS0, Figure 1E). The ability of this dose to effectively block 6 Hz-induced seizures was confirmed in a separate cohort of CF1 mice (Figure 1F). Unlike AMI, CVD (20 mg/kg) also conferred significant protection when the CF1 mice were tested at 2xCC (Figure 1G). CVD was also able to significantly increase the latency to the first GTCS following PTZ administration, and 50% of the CVD-treated mice (5 of 10 mice) did not exhibit a GTCS during the 30-min observation period (Figure 1H).
In contrast to AMI and CVD, nilvadipine did not significantly protect against 6 Hz seizures at any of the doses tested (Figure 1I). We were unable to prepare higher doses of nilvadipine due to its low solubility; thus, this compound was not examined further. Finally, we also observed a dose-dependent increase in protection against 6 Hz seizures with fenfluramine, with doses of 10 and 17 mg/kg leading to increased resistance against 6 Hz seizures when tested in CF1 mice (Figure 1J).
Carvedilol also provides robust protection against induced seizures in Scn8a RL/+ mutant mice
Since AMI, CVD, and FF conferred seizure protection in CF1 mice, we next investigated whether these compounds could similarly protect against induced seizures in the Scn8a RL/+ mutants. Surprisingly, we found that 30 mg/kg AMI caused excessive sedation and 5–10oC reduction in body temperature in the RL/+ mutants and their WT littermates, alterations that were not observed in the CF1 mice. We also observed myoclonic jerks in two RL/+ mutants that were administered 30 mg/kg AMI. Thus, we tested a lower AMI dose (10 mg/kg) in the RL/+ mutants and WT littermates in order to avoid these side effects. However, the lower AMI dose did not protect against 6 Hz seizures in the RL/+ mutants and appeared to slightly increase susceptibility to 6 Hz seizures in the WT littermates (Figure 2A). In contrast, AMI (10 mg/kg) did prevent PTZ-induced GTCSs during the 30-min observation period in 7 of 9 (78%) WT littermates. However, there was no statistically significant difference in the latency to the first GTCS or the number of mice exhibiting a GTCS between the AMI- and vehicle-treated RL/+ mutants following PTZ administration (Figure 2B).
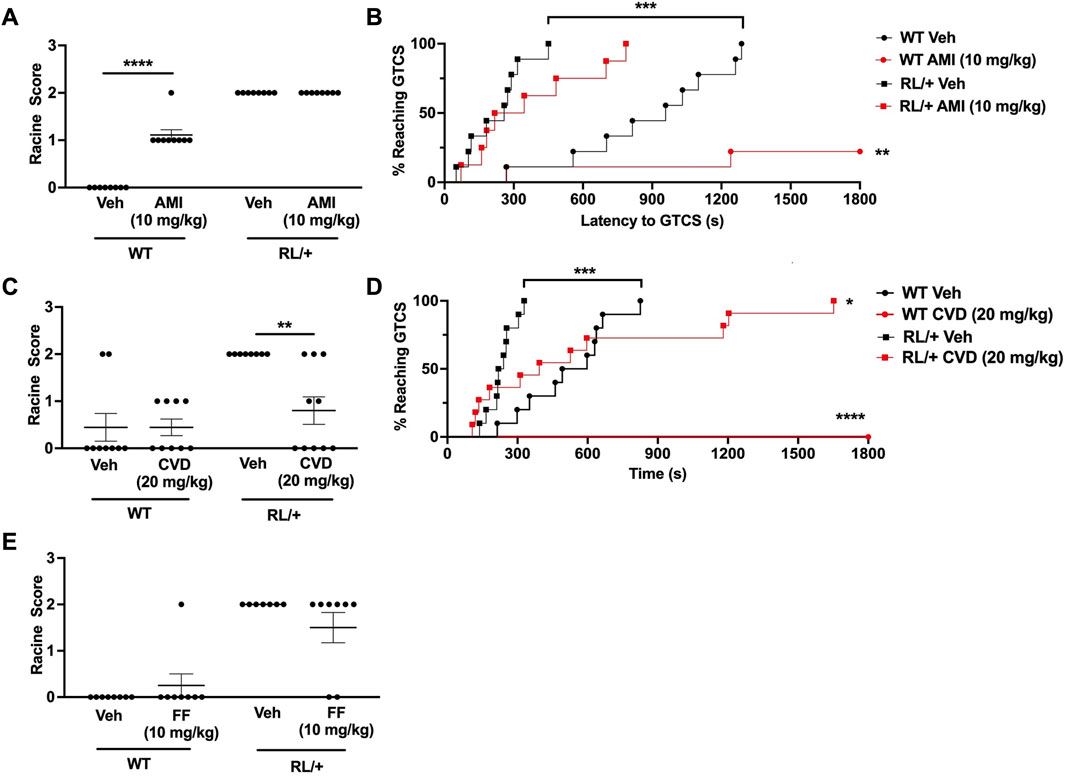
Figure 2. Carvedilol increases seizure resistance in RL/+ mutants. (A) Amitriptyline (AMI, 10 mg/kg) had no effect on seizure susceptibility in the RL/+ mutants, but slightly increased seizure susceptibility in the wild-type (WT) littermates. (B) AMI increased the latency to the first GTCS in the RL/+ mutants and WT littermates. Of the AMI-treated WT mice, seven of nine mice did not exhibit a GTCS during the 30-min observation period. (C) Carvedilol (20 mg/kg) was able to increase resistance against 6 Hz-induced seizures in the RL/+ mutants. (D) Carvedilol significantly increased the latency to the first GTCS following PTZ administration in the RL/+ mutants. All of the WT littermates treated with CVD were completely protected against PTZ-induced seizures. (E) Fenfluramine (17 mg/kg) had no effect on susceptibility to 6 Hz-induced seizures in the RL/+ mutants. *p ≤ 0.05, **p < 0.01, ***p < 0.001, ****p < 0.0001.
Similar to its effect in CF1 mice, we found that CVD (20 mg/kg) was able to protect against induced seizures in the RL/+ mutants (Figures 2C, D). Unlike vehicle-treated RL/+ mutants which all exhibited RS2 seizures in the 6 Hz paradigm, 50% (5 of 10) of CVD-treated RL/+ mutants were completely protected (RS0), and 2 CVD-treated RL/+ mutants displayed a less severe response (RS1). CVD was also able to significantly increase the latency to the first PTZ-induced GTCS in the RL/+ mutants (Figure 2D). Furthermore, PTZ-induced GTCSs were not observed in any of the WT littermates that were treated with CVD during the 30-min observation period.
Finally, we examined the ability of fenfluramine to protect against 6 Hz seizures in the RL/+ mutants. In contrast to the CF1 mice, we found that fenfluramine (17 mg/kg) was only able to prevent a 6 Hz-induced seizure in 2 RL/+ mutants (RS0), while the remaining treated mutants seized (RS2; Figure 2E). At a higher fenfluramine dose (30 mg/kg), we observed proconvulsant effects in CF1 mice and significant side effects including hyperactivity and head twitching in the RL/+ mutants and WT littermates (not shown). Thus, we did not proceed with further testing of fenfluramine in the RL/+ mutants.
Discussion
Using an in vitro drug screen, Atkin and others found that amitriptyline, carvedilol, and nilvadipine were able to reduce sodium influx and inhibit sodium currents (Atkin et al., 2018). Given that SCN8A mutations are often gain-of-function and result in increased sodium currents, it was speculated that these drugs might be efficacious in SCN8A-derived epilepsy. In addition, fenfluramine has garnered interest for its ability to significantly reduce seizure frequency in several severe pediatric forms of epilepsy. A recent study also reported a significant reduction in seizure frequency in three patients with SCN8A epilepsy mutations who were treated with fenfluramine (Aledo-Serrano et al., 2022).
To further explore the clinical potential of these compounds, we tested their ability to increase resistance to induced seizures in CF1 mice and a mouse model of Scn8a-derived epilepsy. We found that AMI and CVD provided robust protection against 6 Hz- and PTZ-induced seizures in CF1 WT mice; however, at the doses tested, nilvadipine did not alter seizure susceptibility (Figure 1). The dose range of nilvadipine that we tested was based on previous mouse studies (Morin et al., 2018; Morin et al., 2020); thus, it is possible that higher doses might be required to increase seizure resistance. However, due to the limited solubility of this compound, we were unable to test higher doses.
When administered to RL/+ mutants and WT littermates, we found that CVD but not AMI, was able to increase resistance against 6 Hz- and PTZ-induced seizures (Figures 2C,D). This observation was surprising given the evidence that both AMI and CVD can inhibit sodium channels (Barber et al., 1991; Bankston and Kass, 2010). Furthermore, AMI has high affinity for binding to the open and inactivated sodium channel (Wang et al., 2004), which is a feature of several drugs that have antiseizure effects (Xie et al., 1995). The electrophysiological assays conducted by Atkin et al., also suggested that AMI has a greater inhibitory effect on Nav1.6 channels compared to CVD, nilvadipine, and carbamazepine (Atkin et al., 2018). It is possible that other factors, such as the bioavailability or half-life of AMI, or the biophysical effect of the R1620L mutation, could have influenced the effect of AMI in the RL/+ mutants.
While a recent study reported that fenfluramine was able to significantly reduce seizure frequency in three patients with SCN8A-derived epilepsy (Aledo-Serrano et al., 2022), we did not observe any significant effect on susceptibility to 6 Hz-induced seizures in RL/+ mutants treated with fenfluramine (Figure 2E). We did not test higher doses of fenfluramine in the RL/+ mutants as a higher dose of fenfluramine (30 mg/kg) in CF1 WT mice was associated with increased seizure susceptibility and significant side effects were observed in the RL/+ mutants and WT littermates.
In the current study, we found that only CVD was able to increase resistance to 6 Hz- and PTZ-induced seizures in the RL/+ mutants. While there is no standard of care treatment for patients with SCN8A-derived epilepsy, from several published studies, sodium channel blockers like oxcarbazepine (OXC) can provide some seizure protection and are well-tolerated (Gardella et al., 2018; Talwar and Hammer, 2021; Johannesen et al., 2022). We previously observed a dose-dependent increase in OXC-mediated seizure protection in RL/+ mutants, with 50% and 100% of the mutants being protected against 6 Hz seizures with 15–20 mg/kg and 50 mg/kg OXC, respectively (Wong et al., 2021a). In the current study, approximately 50% of the RL/+ mutants were protected against 6 Hz seizures with 20 mg/kg CVD treatment.
Altogether, we found that the serotonergic drugs, AMI and fenfluramine, did not increase seizure resistance in the RL/+ mutants; however, CVD, which is a β-adrenergic receptor blocker, was able to increase seizure resistance. Previous studies have suggested a role for β-adrenergic receptors in modulating seizure susceptibility (Lints and Nyquist-Battie, 1985; Goel, 2013). Activation of β-adrenergic receptors contribute to the generation and propagation of audiogenic seizures (Lints and Nyquist-Battie, 1985), while antagonism of the β-adrenergic receptor increases seizure resistance (Goel, 2013). CVD has been shown to increase seizure resistance and potentiate the antiseizure effects of sodium valproate in WT mice (Goel, 2013). In addition, CVD treatment decreased the duration and severity of post-ischemic seizures in a rat model (El-Kharashi and Abd El Samad, 2011). However, Wengert et al., observed no protection against audiogenic seizure-induced mortality following administration of the β-adrenergic receptor blocker sotalol in adult mice expressing the SCN8A N1768D mutation (Wengert et al., 2021). Thus, further research will be required to better understand the therapeutic potential of modulating the noradrenergic system in SCN8A-derived epilepsy.
Common biophysical effects of SCN8A epilepsy mutations include greater levels of persistent and/or resurgent sodium currents (Lopez-Santiago et al., 2017; Wengert et al., 2019; Pan and Cummins, 2020; Tidball et al., 2020). These underlying biophysical abnormalities provide the opportunity for targeted treatment development which might result in greater efficacy and less side effects when compared to other drugs. In the current study, we tested AMI, CVD, and nilvadipine in the Scn8a RL/+ mutants as these drugs demonstrated the ability to inhibit sodium current influx in vitro. We also examined fenfluramine based on recent clinical evidence suggesting that it might be efficacious in SCN8A-derived epilepsy. Other drugs that target persistent and/or resurgent sodium currents have recently been described. For example, Prax330 was shown to reduce persistent and resurgent sodium currents in subiculum neurons isolated from Scn8a N1768D/+ mutant mice (Wengert et al., 2019). Cenobamate (YKP3089), which received FDA approval for the treatment of focal seizures in adults in 2019, works, in part, by enhancing the inactivated state of VGSCs and inhibiting persistent sodium currents (Nakamura et al., 2019). Based on its mechanism of action, we speculate that cenobamate might also be beneficial in SCN8A-associated epilepsy. Finally, Johnson et al. recently reported the development of a Nav1.6 selective sodium channel inhibitor (NBI-921352) that blocks persistent and resurgent sodium currents (Johnson et al., 2022). NBI-921352 was able to inhibit firing of glutamatergic neurons and increase seizure resistance in Scn8a N1768D/+ mutant mice (Johnson et al., 2022). Whether these observations in preclinical rodent models translate to humans has not yet been established. While it is beneficial to develop novel drugs for SCN8A-derived epilepsy, there is also value in testing FDA-approved compounds, such as CVD, as repurposing drugs could provide faster clinical application.
In summary, we evaluated the ability of four drugs to increase resistance to induced seizures in a mouse model of SCN8A epilepsy. Considering the differences in drug metabolism between mice and humans (Nair and Jacob, 2016), the doses of the drugs we tested fell within the range of doses administered in humans (Table 1). AMI, CVD, and nilvadipine are currently used in the treatment of conditions such as heart disease and depression; thus, the doses we tested in the mice may not correspond to a seizure-protective dose in humans. Fenfluramine is currently used as an add-on therapy in several forms of pediatric epilepsy, and while the doses we tested are within the current range used in patients with epilepsy (0.2–0.7 mg/kg/day), we only examined the effect of fenfluramine as a monotherapy treatment in the mice. It is possible we may observe greater seizure protection when using fenfluramine as an add-on treatment. Overall, we found that carvedilol, a β-adrenergic receptor blocker, robustly increased resistance to 6 Hz- and PTZ-induced seizures in the RL/+ mutants. While this mouse line offered the advantage of a SCN8A mutation with both gain-of-function and loss-of-function properties, RL/+ mutants exhibit infrequent spontaneous seizures, and thus, we were unable to evaluate whether carvedilol could also reduce spontaneous seizure frequency. In future studies, it would be important to also evaluate the effect of these drugs in female RL/+ mutants and WT littermates. The results of this study and previous evidence for the role of noradrenergic modulation in SCN8A-associated epilepsy, provide support for the continued investigation of the potential of CVD and other β-adrenergic receptor blockers as treatments for SCN8A-associated epilepsy.
Data availability statement
The raw data supporting the conclusion of this article will be made available by the authors, without undue reservation.
Ethics statement
The animal study was approved by the Emory University Institutional and Animal Care and Use Committee (IACUC). The study was conducted in accordance with the local legislation and institutional requirements.
Author contributions
JW: Conceptualization, Data curation, Formal Analysis, Writing–original draft, Writing–review and editing, Funding acquisition, Investigation, Methodology. AE: Conceptualization, Formal Analysis, Funding acquisition, Investigation, Methodology, Writing–review and editing.
Funding
The author(s) declare that financial support was received for the research, authorship, and/or publication of this article. This project was supported by the National Institutes of Health (JW, R21NS114795; AE, R01NS126392).
Acknowledgments
The authors would like to acknowledge Quinn Eastman for editorial assistance and Dr. Michael Epstein for guidance with statistical analyses.
Conflict of interest
The authors declare that the research was conducted in the absence of any commercial or financial relationships that could be construed as a potential conflict of interest.
Publisher’s note
All claims expressed in this article are solely those of the authors and do not necessarily represent those of their affiliated organizations, or those of the publisher, the editors and the reviewers. Any product that may be evaluated in this article, or claim that may be made by its manufacturer, is not guaranteed or endorsed by the publisher.
References
Aledo-Serrano, A., Cabal-Paz, B., Gardella, E., Gomez-Porro, P., Martinez-Mugica, O., Beltran-Corbellini, A., et al. (2022). Effect of fenfluramine on seizures and comorbidities in SCN8A-developmental and epileptic encephalopathy: a case series. Epilepsia Open 7, 525–531. doi:10.1002/epi4.12623
Andrews, J. G., Galindo, M. K., Hack, J. B., Watkins, J. C., Conecker, G. A., Hammer, M. F., et al. (2023). The international SCN8A patient registry: a scientific resource to advance the understanding and treatment of a rare pediatric neurodevelopmental syndrome. J. Regist. Manag. 50, 4–10.
Atkin, T. A., Maher, C. M., Gerlach, A. C., Gay, B. C., Antonio, B. M., Santos, S. C., et al. (2018). A comprehensive approach to identifying repurposed drugs to treat SCN8A epilepsy. Epilepsia 59, 802–813. doi:10.1111/epi.14037
Bankston, J. R., and Kass, R. S. (2010). Molecular determinants of local anesthetic action of beta-blocking drugs: implications for therapeutic management of long QT syndrome variant 3. J. Mol. Cell Cardiol. 48, 246–253. doi:10.1016/j.yjmcc.2009.05.012
Barber, M. J., Starmer, C. F., and Grant, A. O. (1991). Blockade of cardiac sodium channels by amitriptyline and diphenylhydantoin. Evidence for two use-dependent binding sites. Circ. Res. 69, 677–696. doi:10.1161/01.res.69.3.677
Barton, M. E., Klein, B. D., Wolf, H. H., and White, H. S. (2001). Pharmacological characterization of the 6 Hz psychomotor seizure model of partial epilepsy. Epilepsy Res. 47, 217–227. doi:10.1016/s0920-1211(01)00302-3
Boiko, T., Rasband, M. N., Levinson, S. R., Caldwell, J. H., Mandel, G., Trimmer, J. S., et al. (2001). Compact myelin dictates the differential targeting of two sodium channel isoforms in the same axon. Neuron 30, 91–104. doi:10.1016/s0896-6273(01)00265-3
Boiko, T., Van Wart, A., Caldwell, J. H., Levinson, S. R., Trimmer, J. S., and Matthews, G. (2003). Functional specialization of the axon initial segment by isoform-specific sodium channel targeting. J. Neurosci. 23, 2306–2313. doi:10.1523/JNEUROSCI.23-06-02306.2003
Bunton-Stasyshyn, R. K. A., Wagnon, J. L., Wengert, E. R., Barker, B. S., Faulkner, A., Wagley, P. K., et al. (2019). Prominent role of forebrain excitatory neurons in SCN8A encephalopathy. Brain 142, 362–375. doi:10.1093/brain/awy324
Caldwell, J. H., Schaller, K. L., Lasher, R. S., Peles, E., and Levinson, S. R. (2000). Sodium channel Na(v)1.6 is localized at nodes of ranvier, dendrites, and synapses. Proc. Natl. Acad. Sci. U. S. A. 97, 5616–5620. doi:10.1073/pnas.090034797
Ceulemans, B., Boel, M., Leyssens, K., Van Rossem, C., Neels, P., Jorens, P. G., et al. (2012). Successful use of fenfluramine as an add-on treatment for Dravet syndrome. Epilepsia 53, 1131–1139. doi:10.1111/j.1528-1167.2012.03495.x
Ceulemans, B., Schoonjans, A. S., Marchau, F., Paelinck, B. P., and Lagae, L. (2016). Five-year extended follow-up status of 10 patients with Dravet syndrome treated with fenfluramine. Epilepsia 57, e129–e134. doi:10.1111/epi.13407
El-Kharashi, O. A., and Abd El Samad, A. A. (2011). The potential pharmacological and histological benefits of carvedilol on the hippocampal post- stroke seizures in rats. Life Sci. Journal-Acta Zhengzhou Univ. Overseas Ed. 8, 951–960.
Gardella, E., Marini, C., Trivisano, M., Fitzgerald, M. P., Alber, M., Howell, K. B., et al. (2018). The phenotype of SCN8A developmental and epileptic encephalopathy. Neurology 91, e1112–e1124. doi:10.1212/WNL.0000000000006199
Goel, R., Goel, A., and Kumar, Y. (2011). Influence of carvedilol on anticonvulsant effect of gabapentin. Acta Neurol. Belg 111, 296–305.
Goel, R. G. A., and Goel, A. (2013). Interactions between carvedilol and sodium valproate along with neurobehavioural co-morbidities in various epilepsy models. Drug Invent. Today 5, 87–91. doi:10.1016/j.dit.2013.05.003
Inglis, G. A. S., Wong, J. C., Butler, K. M., Thelin, J. T., Mistretta, O. C., Wu, X., et al. (2020). Mutations in the Scn8a DIIS4 voltage sensor reveal new distinctions among hypomorphic and null Nav 1.6 sodium channels. Genes Brain Behav. 19, e12612. doi:10.1111/gbb.12612
Johannesen, K. M., Liu, Y., Koko, M., Gjerulfsen, C. E., Sonnenberg, L., Schubert, J., et al. (2022). Genotype-phenotype correlations in SCN8A-related disorders reveal prognostic and therapeutic implications. Brain 145, 2991–3009. doi:10.1093/brain/awab321
Johnson, J. P., Focken, T., Khakh, K., Tari, P. K., Dube, C., Goodchild, S. J., et al. (2022). NBI-921352, a first-in-class, Na(V)1.6 selective, sodium channel inhibitor that prevents seizures in Scn8a gain-of-function mice, and wild-type mice and rats. Elife 11, e72468. doi:10.7554/eLife.72468
Knupp, K. G., Scheffer, I. E., Ceulemans, B., Sullivan, J., Nickels, K. C., Lagae, L., et al. (2023). Fenfluramine provides clinically meaningful reduction in frequency of drop seizures in patients with Lennox-Gastaut syndrome: interim analysis of an open-label extension study. Epilepsia 64, 139–151. doi:10.1111/epi.17431
Knupp, K. G., Scheffer, I. E., Ceulemans, B., Sullivan, J. E., Nickels, K. C., Lagae, L., et al. (2022). Efficacy and safety of fenfluramine for the treatment of seizures associated with lennox-gastaut syndrome: a randomized clinical trial. JAMA Neurol. 79, 554–564. doi:10.1001/jamaneurol.2022.0829
Lamar, T., Vanoye, C. G., Calhoun, J., Wong, J. C., Dutton, S. B. B., Jorge, B. S., et al. (2017). SCN3A deficiency associated with increased seizure susceptibility. Neurobiol. Dis. 102, 38–48. doi:10.1016/j.nbd.2017.02.006
Lints, C. E., and Nyquist-Battie, C. (1985). A possible role for beta-adrenergic receptors in the expression of audiogenic seizures. Pharmacol. Biochem. Behav. 22, 711–716. doi:10.1016/0091-3057(85)90518-0
Liu, Y., Schubert, J., Sonnenberg, L., Helbig, K. L., Hoei-Hansen, C. E., Koko, M., et al. (2019). Neuronal mechanisms of mutations in SCN8A causing epilepsy or intellectual disability. Brain 142, 376–390. doi:10.1093/brain/awy326
Lopez-Santiago, L. F., Yuan, Y., Wagnon, J. L., Hull, J. M., Frasier, C. R., O'malley, H. A., et al. (2017). Neuronal hyperexcitability in a mouse model of SCN8A epileptic encephalopathy. Proc. Natl. Acad. Sci. U. S. A. 114, 2383–2388. doi:10.1073/pnas.1616821114
Morin, A., Mouzon, B., Ferguson, S., Paris, D., Browning, M., Stewart, W., et al. (2020). Nilvadipine suppresses inflammation via inhibition of P-SYK and restores spatial memory deficits in a mouse model of repetitive mild TBI. Acta Neuropathol. Commun. 8, 166. doi:10.1186/s40478-020-01045-x
Morin, A., Mouzon, B., Ferguson, S., Paris, D., Saltiel, N., Lungmus, C., et al. (2018). Treatment with nilvadipine mitigates inflammatory pathology and improves spatial memory in aged hTau mice after repetitive mild TBI. Front. Aging Neurosci. 10, 292. doi:10.3389/fnagi.2018.00292
Nair, A. B., and Jacob, S. (2016). A simple practice guide for dose conversion between animals and human. J. Basic Clin. Pharm. 7, 27–31. doi:10.4103/0976-0105.177703
Nakamura, M., Cho, J. H., Shin, H., and Jang, I. S. (2019). Effects of cenobamate (YKP3089), a newly developed anti-epileptic drug, on voltage-gated sodium channels in rat hippocampal CA3 neurons. Eur. J. Pharmacol. 855, 175–182. doi:10.1016/j.ejphar.2019.05.007
Pan, Y., and Cummins, T. R. (2020). Distinct functional alterations in SCN8A epilepsy mutant channels. J. Physiol. 598, 381–401. doi:10.1113/JP278952
Parra, A., Everss, E., Monleón, S., Vinader-Caerols, C., and Arenas, M. C. (2002). Effects of acute amitriptyline administration on memory, anxiety and activity in male and female mice. Neurosci. Res. Commun. 31, 135–144. doi:10.1002/nrc.10046
Rossi, M., El-Khechen, D., Black, M. H., Farwell Hagman, K. D., Tang, S., and Powis, Z. (2017). Outcomes of diagnostic exome sequencing in patients with diagnosed or suspected autism spectrum disorders. Pediatr. Neurol. 70, 34–43. doi:10.1016/j.pediatrneurol.2017.01.033
Shapiro, L., Escayg, A., and Wong, J. C. (2022). Cannabidiol increases seizure resistance and improves behavior in an Scn8a mouse model. Front. Pharmacol. 13, 815950. doi:10.3389/fphar.2022.815950
Shapiro, L., Wong, J. C., and Escayg, A. (2019). Reduced cannabinoid 2 receptor activity increases susceptibility to induced seizures in mice. Epilepsia 60, 2359–2369. doi:10.1111/epi.16388
Shiu, F. H., Wong, J. C., Yamamoto, T., Lala, T., Purcell, R. H., Owino, S., et al. (2022). Mice lacking full length Adgrb1 (Bai1) exhibit social deficits, increased seizure susceptibility, and altered brain development. Exp. Neurol. 351, 113994. doi:10.1016/j.expneurol.2022.113994
Talwar, D., and Hammer, M. F. (2021). SCN8A epilepsy, developmental encephalopathy, and related disorders. Pediatr. Neurol. 122, 76–83. doi:10.1016/j.pediatrneurol.2021.06.011
Tidball, A. M., Lopez-Santiago, L. F., Yuan, Y., Glenn, T. W., Margolis, J. L., Clayton Walker, J., et al. (2020). Variant-specific changes in persistent or resurgent sodium current in SCN8A-related epilepsy patient-derived neurons. Brain 143, 3025–3040. doi:10.1093/brain/awaa247
Vanelderen, P., Rouwette, T., Kozicz, T., Heylen, R., Van Zundert, J., Roubos, E. W., et al. (2013). Effects of chronic administration of amitriptyline, gabapentin and minocycline on spinal brain-derived neurotrophic factor expression and neuropathic pain behavior in a rat chronic constriction injury model. Reg. Anesth. Pain Med. 38, 124–130. doi:10.1097/AAP.0b013e31827d611b
Veeramah, K. R., O'brien, J. E., Meisler, M. H., Cheng, X., Dib-Hajj, S. D., Waxman, S. G., et al. (2012). De novo pathogenic SCN8A mutation identified by whole-genome sequencing of a family quartet affected by infantile epileptic encephalopathy and SUDEP. Am. J. Hum. Genet. 90, 502–510. doi:10.1016/j.ajhg.2012.01.006
Wagnon, J. L., Korn, M. J., Parent, R., Tarpey, T. A., Jones, J. M., Hammer, M. F., et al. (2015). Convulsive seizures and SUDEP in a mouse model of SCN8A epileptic encephalopathy. Hum. Mol. Genet. 24, 506–515. doi:10.1093/hmg/ddu470
Wang, G. K., Russell, C., and Wang, S. Y. (2004). State-dependent block of voltage-gated Na+ channels by amitriptyline via the local anesthetic receptor and its implication for neuropathic pain. Pain 110, 166–174. doi:10.1016/j.pain.2004.03.018
Wengert, E. R., Saga, A. U., Panchal, P. S., Barker, B. S., and Patel, M. K. (2019). Prax330 reduces persistent and resurgent sodium channel currents and neuronal hyperexcitability of subiculum neurons in a mouse model of SCN8A epileptic encephalopathy. Neuropharmacology 158, 107699. doi:10.1016/j.neuropharm.2019.107699
Wengert, E. R., Wenker, I. C., Wagner, E. L., Wagley, P. K., Gaykema, R. P., Shin, J. B., et al. (2021). Adrenergic mechanisms of audiogenic seizure-induced death in a mouse model of SCN8A encephalopathy. Front. Neurosci. 15, 581048. doi:10.3389/fnins.2021.581048
Wong, J. C., Dutton, S. B., Collins, S. D., Schachter, S., and Escayg, A. (2016). Huperzine A provides robust and sustained protection against induced seizures in Scn1a mutant mice. Front. Pharmacol. 7, 357. doi:10.3389/fphar.2016.00357
Wong, J. C., Grieco, S. F., Dutt, K., Chen, L., Thelin, J. T., Inglis, G. A. S., et al. (2021a). Autistic-like behavior, spontaneous seizures, and increased neuronal excitability in a Scn8a mouse model. Neuropsychopharmacology 46, 2011–2020. doi:10.1038/s41386-021-00985-9
Wong, J. C., Shapiro, L., Thelin, J. T., Heaton, E. C., Zaman, R. U., D'souza, M. J., et al. (2021b). Nanoparticle encapsulated oxytocin increases resistance to induced seizures and restores social behavior in Scn1a-derived epilepsy. Neurobiol. Dis. 147, 105147. doi:10.1016/j.nbd.2020.105147
Wong, J. C., Thelin, J. T., and Escayg, A. (2019). Donepezil increases resistance to induced seizures in a mouse model of Dravet syndrome. Ann. Clin. Transl. Neurol. 6, 1566–1571. doi:10.1002/acn3.50848
Keywords: SCN8A, epilepsy, carvedilol, amitriptyline, seizure susceptibility
Citation: Wong JC and Escayg A (2024) Carvedilol increases seizure resistance in a mouse model of SCN8A-derived epilepsy. Front. Pharmacol. 15:1397225. doi: 10.3389/fphar.2024.1397225
Received: 07 March 2024; Accepted: 14 May 2024;
Published: 04 June 2024.
Edited by:
Bin Gu, The Ohio State University, United StatesReviewed by:
Ian Wenker, University of Virginia, United StatesAmanda E. Hernan, Nemours Children’s Health, United States
Copyright © 2024 Wong and Escayg. This is an open-access article distributed under the terms of the Creative Commons Attribution License (CC BY). The use, distribution or reproduction in other forums is permitted, provided the original author(s) and the copyright owner(s) are credited and that the original publication in this journal is cited, in accordance with accepted academic practice. No use, distribution or reproduction is permitted which does not comply with these terms.
*Correspondence: Jennifer C. Wong, amVubmlmZXIuYy53b25nQGVtb3J5LmVkdQ==