- 1Beijing University of Chinese Medicine, Beijing, China
- 2Dongfang Hospital, Beijing University of Chinese Medicine, Beijing, China
Background: Ischemic stroke is a serious and sudden cerebrovascular condition that significantly affects individual’s health and imposes a substantial economic burden on medical management. Despite its widespread use in China, there is still a lack of reliable evidence regarding the efficacy of Shenmai injection (SMI) in acute ischemic stroke (AIS). We aimed to comprehensively assess the effectiveness and safety of SMI in treating AIS through a systematic review and meta-analysis.
Methods: Randomized controlled studies (RCTs) investigating the efficacy of SMI in treating AIS were searched for in eight databases from the inception of each database till January 2024. We utilized the ROB 2.0 to assess the risk of bias. A meta-analysis was conducted using Review Manager 5.4, while sensitivity analyses and publication bias assessments were conducted using Stata 16.1.
Results: A total of 17 studies involving 1,603 AIS patients were included in our meta-analysis. Our results showed that SMI plus conventional treatments (CTs) was more effective than CTs alone in improving the total effective rate (RR 1.22, 95% CI: 1.14 to 1.30, p < 0.00001), the Barthel index (BI) (MD 12.18, 95% CI: 10.30 to 14.06, p < 0.00001), and reducing the National Institute of Health Stroke Scale Score (NIHSS) score (MD -3.05, 95% CI: 3.85 to −2.24, p < 0.00001) and Modified Rankin Scale (mRS) (MD -0.68, 95% CI: 0.86 to-0.49, p < 0.00001). In addition, SMI combination therapy was better than CTs alone in decreasing the levels of IL-6, IL-18, and hs-CRP. SMI therapy also enhanced the cerebral hemorheology of patients by reducing levels of fibrinogen and plasma viscosity. However, there was no significant difference in the incidence of adverse events, including elevated transaminase, rash, nausea, bleeding, urticaria, headache, vomiting, chest tightness, and facial flushes. Moreover, no serious adverse effects or life-threatening events were reported.
Conclusion: Our study shows that combining SMI with CTs effectively enhances the neurological function of patients with acute cerebral infarction. However, our findings should be interpreted considering the significant heterogeneity and suboptimal quality of the analyzed trials.
Systematic review registration: https://www.crd.york.ac.uk/prospero/display_record.php?ID=CRD42024504675, Identifier PROSPERO, CRD42024504675
1 Introduction
Acute ischemic stroke (AIS) refers to sudden focal ischemia of the brain tissue lasting more than 24 h and causing neurological dysfunction (Mendelson and Prabjakaran, 2021). Ischemic stroke, accounting for 87% of all stroke occurrences, is a major cause of mortality and disability globally, and the clinical prognosis of AIS is poor (Liu et al., 2020; Tsao et al., 2022). Currently, stroke is the leading cause of death in China, with an annual increase of 2.5 million cases and 1.6 million deaths (Jia et al., 2022).
Stroke can result in various complications, including neurological abnormalities, infections, movement impairment, thrombosis, and emotional issues (Lin et al., 2022). Among these complications, recurrent stroke is regarded as the most serious; patients with repeated stroke frequently become locked in a vicious cycle, resulting in fast functional decline (Saengsuwan et al., 2017). Furthermore, a study conducted in rural China found that the age-standardized risk of recurrent stroke within 5 years was 22.5% (24.0% for men and 20.2% for women) (Han et al., 2020). AIS is a significant health issue that imposes a substantial economic burden on families and society.
The main objective of AIS treatment is to reopen the arteries and restore tissue blood flow (Sapir et al., 2021). Recent advances in AIS management, including intravenous thrombolysis (IVT) and endovascular thrombectomy (EVT), have significantly improved post-stroke survival rates (Powers et al., 2019). However, the use of IVTs and endovascular thrombectomy is limited due to the occurrence of hemorrhagic transformation and reperfusion injury after recanalization (Zhang et al., 2021; Bo et al., 2022). Furthermore, it is essential to consider the constraints imposed by the narrow therapeutic window (Dong et al., 2021). Hence, identifying effective therapies for AIS is critical.
Shenmai injection (SMI) is a well-known botanical drug injection extracted from Panax ginseng C.A. Mey. [Araliaceae; Radix ginseng rubra] and Ophiopogon japonicus (Thunb.) Ker Gawl. [Asparagaceae; Ophiopogonis Radix], which was included in the National Essential Drugs List (2018 Edition) in China. Regarding the preparation thereof, the pharmaceutical factory acquired 100 g each of Radix ginseng rubra and Ophiopogonis Radix. These were heated and refluxed with 90% ethanol for extraction, then subjected to reduced pressure to yield 0.3–0.4 g of raw medicine per 1 mL. Following filtration with activated carbon and dilution with water, 5 g of polysorbate 80 was added. Injection water was added to reach a volume of 1,000 mL, and the pH was adjusted to 5.0–6.5 using 10% NaOH. Lastly, the mixture underwent filtration, sealing, and sterilization to produce SMI. In accordance with the drug standards set by the China Food and Drug Administration (China Food and Drug Administration, 2010), the concentration of Ginsenoside Re, an active metabolite traditionally employed to ensure the quality of SMI, should not be lower than 0.8 g/L. The details of SMI, including the composition, source, actions, quality control, etc., are shown in Supplementary Table S2, and the principal chemical constituents of SMI under fingerprint methods are summarized in Supplementary Table S3. Research has shown that SMI can successfully mitigate the damage caused by ischemia-reperfusion, enhance cerebral blood flow, and decrease the extent of cerebral infarction (Zuo et al., 2015). In addition, pharmacological studies have proven that SMI exerts neuro-protective effects by preserving blood-brain barrier functional integrity, reducing oxidative stress, and inhibiting neuronal apoptosis (Dang et al., 2017; Xiao et al., 2019; Xu et al., 2019). Moreover, SMI has demonstrated the capability to lower the levels of NO, NOS, and MDA in brain tissue while increasing SOD activity to enhance the removal of oxygen radicals (Zhao et al., 2012). Clinical trials have revealed that SMI improves neurological function, cerebral hemodynamics, and reduces inflammatory factor levels in patients with AIS (Sun, 2020).
A previous meta-analysis studied SMI in the treatment of AIS (Liu et al., 2017), but with certain restrictions. Firstly, the inclusion of other TCM interventions in the literature considered in this study could have affected the precision of the findings. Secondly, the study exhibited significant heterogeneity and did not conduct subgroup and sensitivity analyses to assess stability. Additionally, the assessment of the effectiveness and safety of SMI was not comprehensive due to the restricted range of outcome measures. Due to these limitations, the results are less reliable and less applicable to clinical settings.
We, therefore, aimed to conduct a subgroup analysis to determine the influence of SMI on the efficacy and safety of AIS, and use standardized criteria to improve the accuracy of the pharmacological efficacy evaluation. Moreover, serum inflammatory markers and coagulation indices were included as important indicators to assess effectiveness. The GRADE system was utilized to evaluate evidence quality, offering clinicians a reliable and objective reference for SMI.
2 Materials and methods
This systematic review and meta-analysis follows the Preferred Reporting Items for Systematic Reviews and Meta-Analyses Statement. The study protocol was registered with PROSPERO (registration number: CRD42024504675).
2.1 Search strategy
We searched the PubMed, Embase, Web of Science, Cochrane Library, China National Knowledge Infrastructure, Chinese Sci-tech Periodical Database (VIP), Chinese Biological Medicine Database (Sinomed), and the Chinese Wan Fang Database (Wan fang) from the inception of the database to February 2024. The search strategies for each database are depicted in Supplementary Material S1.
2.2 Selection criterion
2.2.1 Types of studies
The study comprised randomized controlled trials (RCTs) with participants meeting the diagnostic criteria for AIS.
2.2.2 Types of interventions
Conventional treatments (CTs) were used in the control group (antiplatelet, anticoagulation, improvement of cerebral circulation, nerve nutrition, radical scavenging, defibrase, and statins) (Chinese Society of Neurology and Chinese Stroke Society, 2018). Patients in the experimental group were administered SMI in conjunction with conventional treatments. Studies with the other TCM treatments were excluded.
2.2.3 Outcome measures
The primary outcome was total effective rate, which is determined by calculating the decrease in the National Institute of Health Stroke Scale Score (NIHSS); we defined the total effective rate as the ratio of patients whose NIHSS score reduced by >18%. Secondary outcomes included the NIHSS, Barthel Index (BI), modified Rankin Scale (mRS), and other related scales, interleukin-6 (IL-6), interleukin-18 (IL-18), C-reactive protein (hs-CRP), fibrinogen levels, plasma viscosity, hematocrit, and adverse events.
2.3 Exclusion criteria
The following studies were excluded from our analysis: 1) case reports, experiments, reviews, and conference papers; 2) studies that included other forms of traditional Chinese medicine, such as herbal remedies, acupuncture, massage, and scraping; 3) studies with three arms; 4) studies with data that was incomplete or unable to be extracted; and 5) studies with patients treated with IVTs or EVTs.
2.4 Study selection and data extraction
EndNote X9.1 was used to organize the studies for analysis. After removing duplicates, two reviewers (ZS and STY) independently evaluated the titles and abstracts to exclude non-compliant papers. Then, to find other studies to include, the whole texts of the remaining studies were downloaded and thoroughly reviewed. For each included study, two writers separately retrieved information on the authors, publication date, gender, age, sample size, intervention, the injection dosage of SMI, length of treatment, and outcome metrics. A third author (ZM) arbitrated any disputes between the two reviewers.
2.5 Risk of bias
Two reviewers (ZS and STY) independently assessed and cross-checked the risk of bias for eligible RCTs using the Cochrane risk of bias tool 2.0 (RoB 2.0). Six domains were evaluated: 1) randomization process, 2) deviations from the intended interventions, 3) missing outcome data, 4) measurement of the outcome, 5) selection of the reported outcome, and 6) overall bias. The trials were all categorized as having a low, uncertain, or high risk of bias. Disputes were resolved through consultation with a third researcher (ZM) or through mutual negotiation.
2.6 Data analysis
A meta-analysis was performed using the RevMan 5.4 or Stata 16.1 software. Dichotomous results were analyzed using risk ratio (RR) and 95% confidence intervals (CIs), whereas continuous results were examined using mean difference (MD) and 95% CIs. A p-value of <0.05 was deemed to show statistical significance for the overall impact.
To explore potential sources of heterogeneity, we conducted subgroup analyses, considering the dosage of SMI (20 mL, 40 mL, 50 mL, 60 mL, 60–100 mL), intervention duration (10 D, 2 W, 3 W, 4 W). Heterogeneity was evaluated using the I2 value, and values exceeding 50% were considered indicative of significant statistical heterogeneity (Sun et al., 2010). We selected a random effect model when there was significant heterogeneity. Otherwise, a fixed effects model was adopted. Heterogeneity was explained by subgroup or sensitivity analyses if the random-effects model was applicable. The sensitivity analyses were conducted by systematically excluding individual studies one at a time to assess the impact of their heterogeneity on the combined results of the meta-analyses. We evaluated publication bias by creating funnel plots and conducting both the Egger’s and Harbord tests. When the p-value of Egger’s tests was <0.05, the trim-and-fill calculation was used to evaluate the effect of publication bias.
3 Results
3.1 Study selection
Overall, we initially gathered 488 studies showing relevance from the eight databases. After eliminating 260 duplicates, the titles and abstracts of the remaining 228 studies were examined. Among them, 99 studies were removed due to not meeting the inclusion criteria. A total of 112 articles were further excluded because they were not true RCTs (n = 8), were other interventions (n = 81), had outcome indicators that did not meet the inclusion criteria (n = 17), had data that could not be extracted (n = 4), had unclear courses of treatment (n = 1), and were studies with three arms (n = 1). Finally, 17 studies (Tang, 2011; Lu and Yang, 2013; Wang et al., 2013; Wang, 2014; Liu et al., 2016; Chen and Liu, 2018; Han, 2018; Hu, 2018; Liang et al., 2018; Wu et al., 2018; Zhang et al., 2018; Chen, 2019; Li and Chen, 2019; Liu et al., 2020; Sun, 2020; Li and Dai, 2022; Wu, 2022) were included for the final meta-analysis (Figure 1).
3.2 Study characteristics
A total of 1,603 individuals were enrolled, of which 804 were in the group that received SMI combined with CTs and 799 were in the group that received CTs alone. All the included studies were RCTs conducted in China, with a publication time range from 2011 to 2022. The participants had an average age ranging from 56.9 to 78.9 years old, with onset within 14 days. The dosage of SMI treatment exhibited variation, with a minimum of 20 mL (Li and Dai, 2022) and a maximum of 100 mL (Han, 2018). The duration of treatment ranged from a minimum of 10 days (Liu et al., 2016) to a maximum of 4 weeks (Han, 2018; Liu et al., 2020; Li and Dai, 2022). The administration of SMI to experimental groups was conducted by intravenous route in all studies, with a frequency of once-daily treatment. A summary of the fundamental characteristics and specific information of the studies included in the analysis is presented in Table 1.
3.3 Risk of bias assessment
In general, most of the analyzed trials demonstrated low-to-moderate levels of quality. Among the analyzed studies, 11 (Lu and Yang, 2013; Wang, 2014; Chen and Liu, 2018; Han, 2018; Hu, 2018; Liang et al., 2018; Wu et al., 2018; Li and Chen, 2019; Liu et al., 2020; Sun, 2020; Li and Dai, 2022) were randomly assigned using the random number table method, one (Chen, 2019) used the lottery method, and another (Zhang et al., 2018) employed the randomized block design method. The methodology for random grouping was not explicated in detail in the remaining experiments. Furthermore, the trials included no information about participant blinding, outcome assessment, or allocation concealment. We, therefore, rated the bias risk as uncertain. All 17 articles included in the study contained comprehensive data and did not have any selectively reported findings. Additional biases were categorized as unclear due to inadequate information. Figures 2A, B display a summary and graph of the ROB assessment.
3.4 Findings from meta-analyses
3.4.1 Primary efficacy outcome
3.4.1.1 Total effective rate
Eight studies with a total of 732 patients reported the total effective rate, including 366 patients in the SMI combining CTs group and 366 in the CTs alone group (Lu and Yang, 2013; Wang, 2014; Chen and Liu, 2018; Liang et al., 2018; Zhang et al., 2018; Chen, 2019; Liu et al., 2020; Wu, 2022). The meta-analysis findings indicated that the combination of SMI with CTs significantly enhanced the total effective rate compared to CTs alone (RR 1.22, 95% CI: 1.14 to 1.30, p < 0.00001, Figure 3). A subgroup analysis was conducted based on the SMI dosage. The total effective rate was significantly higher in groups combining CTs with 60 mL of SMI (RR 1.31, 95% CI: 1.05 to 1.63, p = 0.02, Figure 3), 50 mL of SMI (RR 1.20, 95% CI: 1.11 to 1.30, p < 0.00001, Figure 3), and 40 mL of SMI (RR 1.21, 95% CI: 1.00 to 1.46, p = 0.04, Figure 3) compared to the control group. Furthermore, the sensitivity analysis confirmed the stability of the findings obtained from the meta-analysis (Supplementary Figure S1).
3.4.2 Secondary outcomes
3.4.2.1 NIHSS score
The NIHSS score serves as a crucial indicator to assess neurological deficits in patients with AIS. Twelve studies (Lu and Yang, 2013; Wang, 2014; Liu et al., 2016; Hu, 2018; Liang et al., 2018; Wu et al., 2018; Chen, 2019; Li and Chen, 2019; Liu et al., 2020; Sun, 2020; Li and Dai, 2022; Wu, 2022) involving 1,276 patients assessed the changes in NIHSS score. The meta-analysis results showed that combining SMI with CTs led to a significant reduction in the NIHSS score compared to using CTs alone (MD -3.05, 95% CI: 3.85 to −2.24, p < 0.00001, Figure 4). A subgroup analysis based on the dosage of SMI revealed significantly lower NIHSS score in groups combining CTs with 50 mL (MD -3.26, 95% CI: 4.41 to −2.11, p < 0.00001, I2 = 96%, Figure 4), 40 mL (MD -2.67, 95% CI: 3.17 to −2.17, p < 0.00001, I2 = 0%, Figure 4), and 20 mL (MD -2.03, 95% CI: 2.86 to −1.20, p < 0.00001, Figure 4) of SMI compared to CTs alone.
Due to high heterogeneity, the random-effects model was employed (p < 0.0001, I2 = 93%). A subgroup analysis indicated that treatment dosage might significantly influence heterogeneity, although it may not account for all sources thereof. Egger’s test (p = 0.053) suggested no significant publication bias in our analysis (Supplementary Figure S2). Furthermore, a sensitivity analysis was performed to validate the robustness of the meta-analytic results (Supplementary Figure S1).
3.4.2.2 BI
Five studies (Wang, 2014; Chen and Liu, 2018; Zhang et al., 2018; Chen, 2019; Wu, 2022) involving 470 patients assessed the changes in BI. The meta-analysis results showed that combining SMI with CTs considerably improved the BI compared to using CTs alone (MD 12.18, 95% CI: 10.30 to 14.06, p < 0.00001, I2 = 38%, Figure 5). A SMI dosage-based subgroup analysis was carried out. The BI was significantly higher in groups combining CTs with 60 mL of SMI (MD 10.53, 95% CI: 8.35 to 12.71, p < 0.00001, Figure 5), 50 mL of SMI (MD 13.51, 95% CI: 11.75 to 15.27, p < 0.00001, I2 = 0%, Figure 5), and 40 mL of SMI (MD 10.43, 95% CI: 3.63 to 17.23, p = 0.003, Figure 5) compared to the control group. The results of Egger’s test (p = 0.754) indicated that there was no substantial publication bias in our study (Supplementary Figure S2). A sensitivity study was conducted to verify the reliability of the meta-analytic findings (Supplementary Figure S1).
3.4.2.3 Modified rankin scale (mRS)
The significant heterogeneity that was found (p < 0.0001, I2 = 83%) led to the selection of the random-effects model. Three studies (Zhang et al., 2018; Chen, 2019; Wu, 2022) involving 320 patients assessed the changes in mRS. The meta-analysis revealed that combining SMI with CTs significantly reduced the mRS compared to CTs alone (MD -0.68, 95% CI: 0.86 to −0.49, p < 0.00001, Figure 6). Egger’s test (p = 0.567) indicated no significant publication bias in our study (Supplementary Figure S2). Nonetheless, the reliability of the meta-analysis results is compromised by the limited sample size and high heterogeneity.
3.4.2.4 Levels of inflammatory factors
Serum IL-6 levels were reported in seven studies (Lu and Yang, 2013; Wang et al., 2013; Liu et al., 2016; Han, 2018; Liu et al., 2020; Sun, 2020; Li and Dai, 2022), and serum IL-18 levels were reported in four (Zhang et al., 2018; Liu et al., 2020; Li and Dai, 2022; Wu, 2022), whereas serum hs-CRP levels were reported in five studies (Tang, 2011; Wang, 2014; Liu et al., 2020; Sun, 2020; Li and Dai, 2022). The random-effects model was also adopted because of the significant variability observed across the studies (p < 0.0001, I2 = 94%; p < 0.0001, I2 = 88%; p < 0.0001, I2 = 97%). Due to inconsistencies in the units used across studies, we utilized the standardized mean difference (SMD) as the effect size indicator. The meta-analysis revealed that combining SMI with CTs significantly reduced the serum IL-6 levels (SMD -2.20, 95% CI: 3.04 to −1.37, p < 0.00001, Figure 7A), the serum IL-18 levels (SMD -2.30, 95% CI: 3.07 to −1.52, p < 0.00001, Figure 7B), and the serum hs-CRP levels (SMD -3.59, 95% CI: 5.42 to −1.76, p = 0.0001, Figure 7C) compared to CTs alone. High heterogeneity might stem from variations in the baseline inflammatory index levels among the included cases. As depicted in the forest plot of IL-18, the studies by Li and Dai, and Liu et al. exhibit greater homogeneity, while those by Wu and Zhang et al. also show homogeneity. Egger’s test results indicated no significant publication bias in serum IL-18 and hs-CRP levels (Supplementary Figure S2). However, a potential publication bias was observed in serum IL-6 levels following the Egger’s test (p = 0.032) (Supplementary Figure S2). Therefore, we conducted a trim-and-fill calculation, which revealed no need for trimming. Furthermore, A sensitivity analysis was performed to validate the reliability of the meta-analytic findings (Supplementary Figure S1).
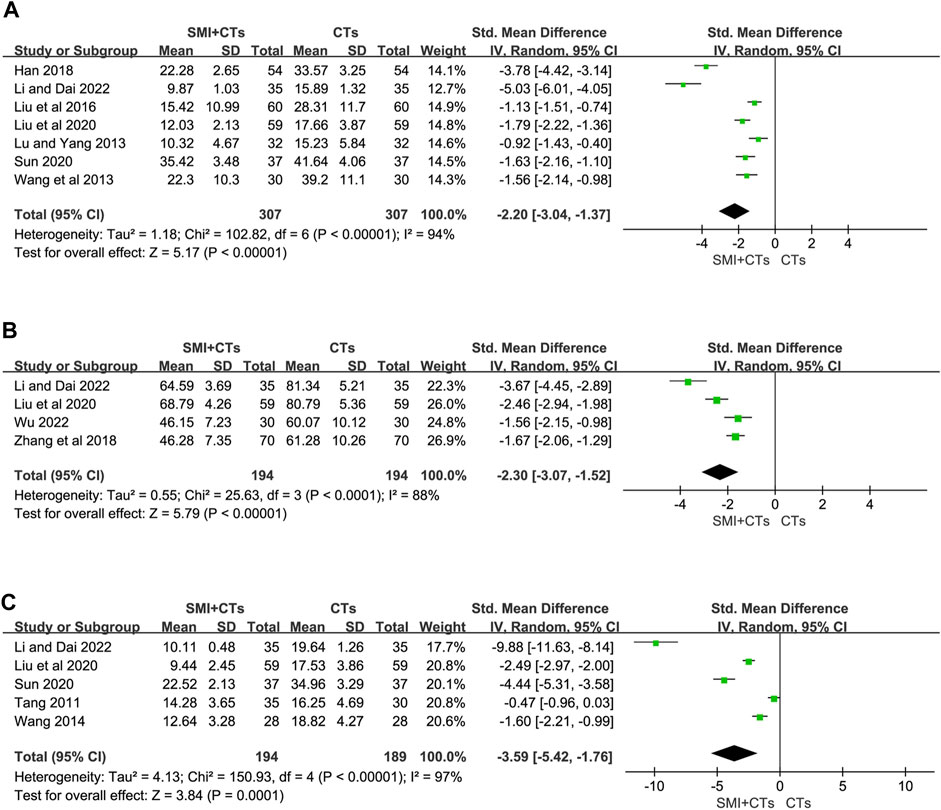
Figure 7. Forest plot for levels of Inflammatory Factors. (A) Serum IL-6. (B) Serum IL-18. (C) Serum hs-CRP.
3.4.2.5 Levels of hemorheology
Fibrinogen levels were reported in five studies (Tang, 2011; Chen and Liu, 2018; Zhang et al., 2018; Li and Dai, 2022; Wu, 2022), and two (Wang et al., 2013; Li and Dai, 2022) reported hematocrit and plasma viscosity. The random-effects model was also employed in our analysis, with the MD serving as the effect size indicator. We conducted a subgroup analysis on fibrinogen levels depending on the length of the intervention. The fibrinogen levels were significantly lower in groups combining CTs with SMI for 4 weeks (MD -0.83, 95% CI: 1.00 to −0.67, p < 0.00001, Figure 8A), as well as for 2 weeks (MD -0.63, 95% CI: 0.87 to −0.39, p < 0.00001, Figure 8A) compared to the control group. The findings indicated that the combination of SMI and CTs led to a reduction in plasma viscosity (MD -0.43, 95% CI: 0.61 to −0.25, p < 0.00001, Figure 8C). However, the hematocrit decrease did not show a significant difference between patients receiving CTs combined with SMI and those receiving CTs alone (MD -9.28, 95% CI: 19.28 to 0.73, p = 0.07, Figure 8C). The results of Egger’s test indicated that there was no substantial publication bias in our study (Supplementary Figure S2). A sensitivity study was performed to confirm the stability and reliability of the meta-analytic results; however, the sources of heterogeneity could not be determined (Supplementary Figure S1). Variations in dose and duration in the trials may have led to this heterogeneity. Further studies are required to enhance the results accuracy and reduce variability.
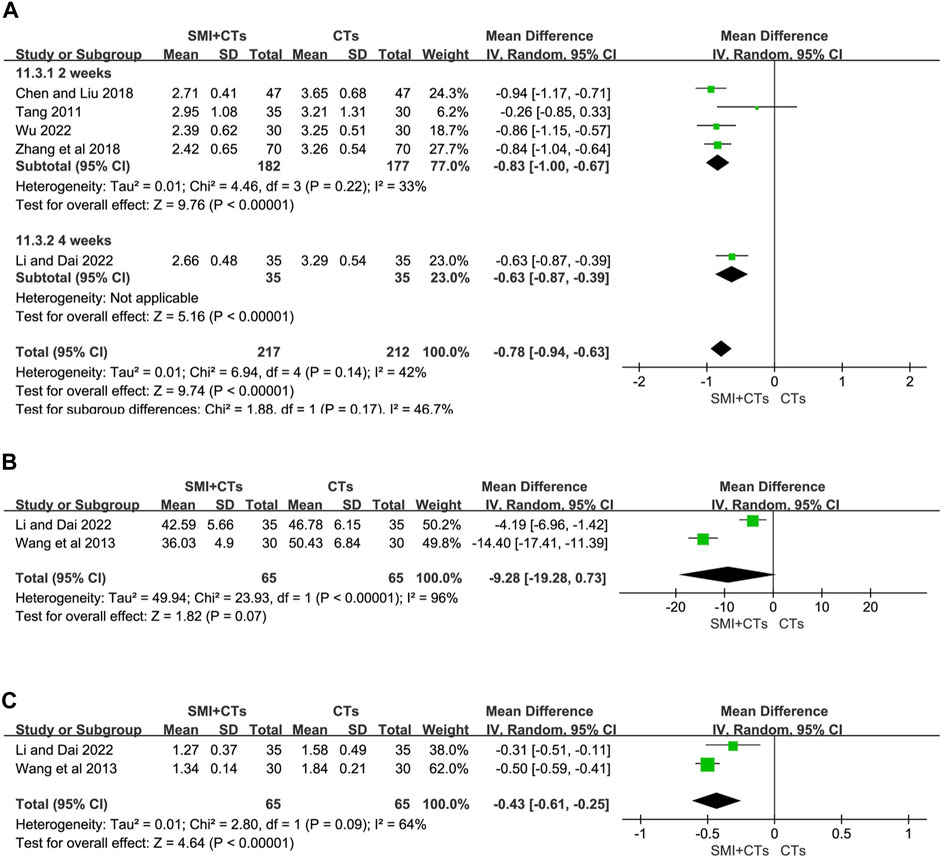
Figure 8. Forest plot for levels of Hemorheology. (A) Fibrinogen. (B) Hematocrit. (C) Plasma viscosity.
3.4.3 Adverse events
Adverse events were documented in six out of 17 studies (Hu, 2018; Zhang et al., 2018; Li and Chen, 2019; Liu et al., 2020; Sun, 2020; Wu, 2022). One study (Sun, 2020) found that adverse events were recorded in 1 out of 37 patients in the SMI combining CTs group and 1 out of 37 cases in the CTs alone group. The frequency of adverse events did not change significantly between the two groups (RR = 1.00, 95% CI: 0.06 to 15.40, p = 1.00). The precise symptoms were not stated, however.
Five studies documented nine side effects, including elevated transaminase, rash, nausea, bleeding, urticaria, chest tightness, facial blushing, headache, and vomiting. Furthermore, a subgroup analysis based on the symptoms was conducted. As depicted in Table 2, no significant difference was seen in the occurrence of elevated transaminase (RR 0.67, 95% CI: 0.11 to 3.87, p = 0.65), rash (RR 0.33, 95% CI: 0.08 to 1.37, p = 0.13), nausea (RR 0.57, 95% CI: 0.22 to 1.42, p = 0.23), bleeding (RR 0.43, 95% CI: 0.06 to 2.85, p = 0.38), urticaria (RR 1.00, 95% CI: 0.18 to 5.69, p = 1.00), chest tightness (RR 1.00, 95% CI: 0.23 to 4.35, p = 1.00), facial blushing (RR 2.33, 95% CI: 0.35 to 15.58, p = 0.38), headache (RR 0.67, 95% CI: 0.12 to 3.85, p = 0.65), and vomiting (RR 0.50, 95% CI: 0.05 to 5.37, p = 0.57). Additionally, a sensitivity analysis confirmed the stability of the findings obtained from the meta-analysis (Supplementary Figure S1).
3.5 Publication bias
Due to the low heterogeneity and limited number of studies available for analysis, the Harbord test was adopted. The results indicated no obvious publication bias for total effective rate (p = 0.403; Figure 9B) and the incidence of adverse events (p = 0.802; Figure 9D). Additionally, the funnel plot of the total effective rate and the incidence of adverse events did not show visual asymmetry (Figures 9A, C).
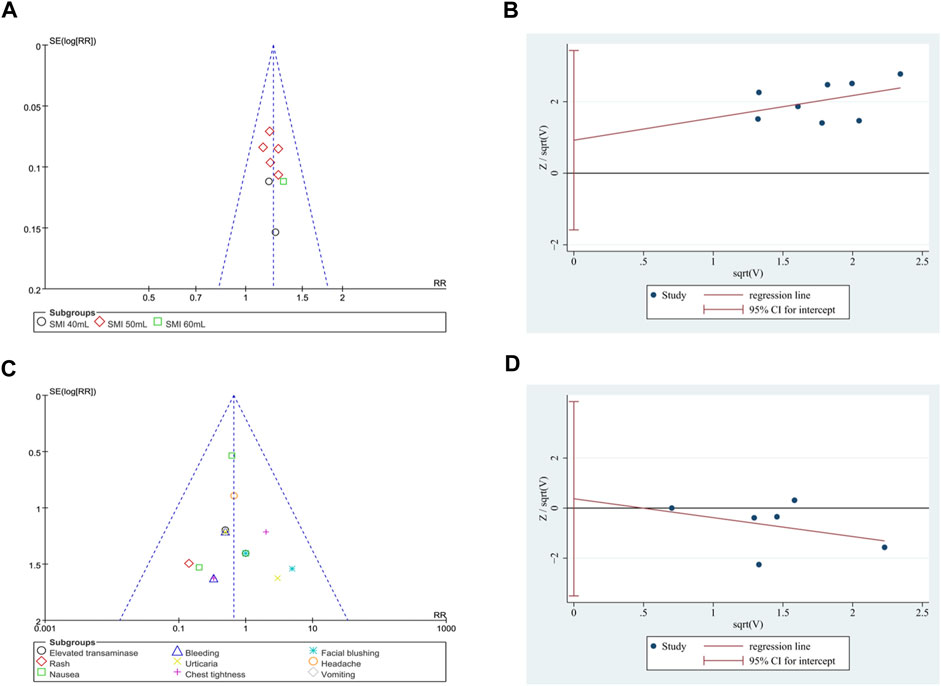
Figure 9. Publication bias. (A) Funnel plot of total effective rate. (B) The Harbord’s test on total effective rate. (C) Funnel plot of the incidence of adverse events. (D) The Harbord’s test on the incidence of adverse events.
3.6 Quality of evidence
The GRADE system was utilized to methodically assess the quality of 10 outcome indicators (Table 3). The findings indicated moderate-quality evidence for total effective rate, BI, and fibrinogen levels. Additionally, low-quality evidence was identified for NIHSS score and serum IL-6 levels, followed by very low evidence for mRS, serum IL-18 levels, serum hs-CRP levels, hematocrit, and plasma viscosity. The significant risks of deviations and reported deviations are attributed to the following five factors: 1) a potential for publication bias was present; 2) the heterogeneity among the studies is significant (p < 0.1, I2 > 50%); 3) the 95% confidence interval includes 1; 4) sample sizes of <400 were included; and 5) the number of included RCTs is fewer than 5. More extensive samples and well-conducted RCTs are needed to improve the evidence on the effectiveness of SMI in treating AIS owing to limitations in the present study.
4 Discussion
4.1 Summary of findings
This systematic review discusses the efficacy and safety of SMI in treating AIS. A total of 17 RCTs were considered, with 1,603 participants. The treatment with SMI appears effective and safe, as it can lower levels of mRS, NIHSS scores, and inflammatory markers, while improving overall effective rate, BI, and cerebral hemodynamics without increasing the occurrence of adverse reactions.
Our subgroup analysis revealed that, compared to CTs, the combination of 20, 40, 50, and 60 mL SMI with CTs significantly enhanced overall effective rate and BI, while reducing NIHSS score. This indicates that within an appropriate dosage range, the combination of SMI effectively alleviates clinical symptoms, facilitates neurological function recovery, and enhances daily living abilities in patients. Moreover, our study highlights the necessity for future investigations to explore the optimal dosage regimens for SMI in AIS treatment. Through systematic evaluation of the effectiveness and safety of various dosage regimens, we can deepen our understanding of the therapeutic potential of SMI and optimize its clinical application. Regarding the intervention duration, SMI combined with CTs treatment for 2 and 4 weeks significantly decreased fibrinogen levels. Additionally, these findings suggest that the effect of SMI is sustained and rapid. The meta-analysis found that SMI did not increase the incidence of patients experiencing increased transaminases, rash, nausea, chest tightness, bleeding, urticaria, facial flushing, headache, or vomiting in patients. However, this implies the necessity of closely monitoring the coagulation function, transaminase levels, and allergic reactions in patients receiving SMI.
Sensitivity analysis suggests that the combined results are robust. However, the origin of heterogeneity cannot be determined. The restricted number of included studies, small sample size, and large variability in early laboratory markers across patients may all contribute to significant heterogeneity. Nonetheless, due to the absence of available data, further discussion was not pursued in the article.
4.2 Interpretation of findings
Inflammation is involved in the occurrence, progression, and development of AIS, which can induce thrombosis. This plays a crucial role in the pathophysiology of ischemic stroke and is a primary focus for the creation of novel stroke treatments (Jiang et al., 2021; Zuo et al., 2021). Increased levels of the pro-inflammatory factor IL-6 induced by ischemia often cause endothelial cells to express adhesion factors and chemokines, which in turn activate monocytes to release relevant inflammatory cytokines, leading to the formation of atherosclerotic thrombosis (Lei et al., 2014). Prospective studies have shown that high levels of IL-6 in community-dwelling individuals are directly linked to an increased risk of developing ischemic stroke in the long run, regardless of traditional vascular risk factors (Papadopoulos et al., 2022). Moreover, higher CRP levels have been demonstrated to independently predict survival after ischemic stroke and functional outcomes following thrombolytic stroke (Nishi et al., 2020). According to the results of this meta-analysis, combination therapy with SMI and CTs may lower blood levels of IL-6, IL-18, and hs-CRP compared to CTs alone. Thrombosis typically develops from coagulation disorders induced by vascular endothelial cell injury, platelet aggregation, and alterations in hemorheology (Ge et al., 2018). Fibrinogen, a vascular inflammatory mediator, has recently been identified as a driver of the neurodegenerative process in cerebrovascular stroke (Wang et al., 2020). The meta-analysis showed that combining SMI with CTs effectively reduces fibrinogen levels and plasma viscosity, leading to an improvement in hemorheological parameters. We refer to the ConPhyMP statement to ensure the rigor of our conclusions (Heinrich et al., 2022). The main botanical drugs of SMI are P. ginseng C.A. Mey. [Araliaceae; Radix ginseng rubra] and O. japonicus (Thunb.) Ker Gawl. [Asparagaceae; Ophiopogonis Radix], which are utilized to invigorate Qi and nourish Yin. Treatment with Ginsenoside Rg1 inhibits the interaction between CKLF1 and CCR5 after ischemic stroke, reducing pyroptosis generated by the CKLF1/CCR5 axis (Long et al., 2024). Ginsenosides also inhibit thrombogenesis by interfering with multiple signaling pathways involved in platelet aggregation, thereby inhibiting the production of thromboxane A2 (TXA2) and phosphorylation of MAPKs (Zhou et al., 2019; Luo et al., 2020) found that different dosage groups of O. japonicus polysaccharides can inhibit NF-κB activation in the B signaling pathway, thereby reducing the oxidative stress response, alleviating macrophage damage, and decreasing the secretion of inflammatory factors. In summary, SMI can exert neuroprotective effects on patients with AIS by reducing the levels of inflammatory factors and improving cerebral hemodynamics.
4.3 Advantages and limitations
A study conducted in 2017 showed that SMI is effective and safe for the treatment of AIS. However, compared with previous studies, ours possesses the following advantages. Firstly, integrating efficacy evaluation criteria based on the decline rate of NIHSS score in our study enables a more comprehensive assessment of the effectiveness of SMI. This not only reduces inconsistency but also enhances the credibility of the results. Moreover, by excluding interventions from other traditional Chinese medicine therapies, we minimized the impact on the accuracy of results, thus providing stronger confirmation of the efficacy of SMI. Furthermore, we employed a subgroup analysis to investigate the effects of medication dosage and duration on efficacy, and further explored the sources of heterogeneity. Finally, we utilized ROB 2.0 and the GRADE profiler to evaluate the risk of bias and the quality of evidence, enhancing the credibility of the findings.
Nevertheless, our study has some limitations. First, most of the included studies showed a risk of bias, reducing the trustworthiness of the findings. Second, although we conducted a sensitivity analysis to assess the stability of the results, caution is warranted in interpreting these findings due to factors such as small sample size, high heterogeneity, and potential publication bias. Third, the study population included in this analysis exclusively consisted of individuals from China, neglecting the potential influence of race and population diversity. This limitation may hinder the broader promotion and application of SMI. Fourth, IVT and EVTs are crucial treatment options for acute cerebral infarction. However, due to their potential impact on outcome consistency, we chose not to include studies in this area. Fifth, the scientific rigor of the efficacy evaluation criteria we have selected may still be subject to debate, particularly considering the variations in stroke severity among participants. Finally, the studies included in our analysis lacked allocation concealment or blinding, and some failed to adequately describe methods for generating random sequences. Consequently, the overall methodological quality was deemed to be low.
4.4 Implications for future studies
We demonstrate the effectiveness of SMI for AIS; however, this has certain limitations that can inspire future research designs. Firstly, considering the extended recovery period of AIS, future clinical studies should incorporate a longer follow-up duration to confirm the potential benefits of SMI in enhancing AIS prognosis. Secondly, there is currently limited research on the combination of SMI with IVT and EVT, necessitating further investigation in this area in the future. Thirdly, when possible, blinding should be implemented using random and covert allocation techniques, and suitable methods to determine sample size should be used. Fourthly, the test report should adhere to the Uniform Standards of Reporting Trials (CONSORT) statement, facilitating the acquisition of more comprehensive information. Finally, the selection of outcome indicators should adhere to uniform standards to comprehensively evaluate the effectiveness and safety of SMI.
5 Conclusion
Overall, this meta-analysis suggests that the combination of SMI with CTs appears to be more effective in treating AIS. Furthermore, none of the adverse effects were severe enough to be considered life-threatening. However, larger, higher-quality trials are required to further corroborate and validate the clinical efficacy and safety of SMI in the treatment of AIS, given the substantial heterogeneity and low quality of evidence.
Data availability statement
The datasets presented in this study can be found in online repositories. The names of the repository/repositories and accession number(s) can be found in the article/Supplementary Material.
Author contributions
SZ: Conceptualization, Data curation, Formal Analysis, Investigation, Project administration, Validation, Visualization, Writing–original draft. TS: Data curation, Formal Analysis, Investigation, Validation, Writing–original draft. MZ: Data curation, Formal Analysis, Validation, Writing–original draft. MY: Data curation, Formal Analysis, Validation, Writing–original draft. KW: Data curation, Formal Analysis, Investigation, Validation, Writing–review and editing. LL: Data curation, Formal Analysis, Investigation, Validation, Writing–review and editing. JL: Conceptualization, Funding acquisition, Project administration, Supervision, Writing–review and editing.
Funding
The author(s) declare that financial support was received for the research, authorship, and/or publication of this article. This study was supported by the Fundamental Research Funds for Beijing University of Chinese Medicine (2020-tsxk-001) and Beijing-Tianjin-Hebei Chinese Medicine Collaborative Development Program (GZY-GCS-2017-0).
Conflict of interest
The authors declare that the research was conducted in the absence of any commercial or financial relationships that could be construed as a potential conflict of interest.
Publisher’s note
All claims expressed in this article are solely those of the authors and do not necessarily represent those of their affiliated organizations, or those of the publisher, the editors and the reviewers. Any product that may be evaluated in this article, or claim that may be made by its manufacturer, is not guaranteed or endorsed by the publisher.
Supplementary material
The Supplementary Material for this article can be found online at: https://www.frontiersin.org/articles/10.3389/fphar.2024.1394936/full#supplementary-material
Abbreviations
AIS, Acute ischemic stroke; BI, Barthel index; CI, Confidence intervals; CTs, Conventional treatments; IVT, Intravenous thrombolysis; MD, Mean difference; NIHSS, National Institute of Health Stroke Scale Score; RCTs, Randomized controlled trials; SMD, Standardized mean difference; SMI, Shenmai injection.
References
Bo, C., Wang, T., Hou, C., Han, J., Chen, L., Zhang, H., et al. (2022). Evolution of ischemic stroke drug clinical trials in mainland China from 2005 to 2021. CNS Neurosci. Ther. 28 (8), 1229–1239. doi:10.1111/cns.13867
Chen, F. (2019). Clinical efficacy of Shenmai Injection combined with butylphthalide in the treatment of elderly patients with acute cerebral infarction. Med. Equip. 32 (16), 70–71. doi:10.3969/j.issn.1002-2376.2019.16.047
Chen, Z., and Liu, W. (2018). The effect of Shenmai Injection as an adjuvant therapy on red blood cell immune function, coagulation function, and cytokines in elderly patients with acute cerebral infarction. Chin. J. Gerontol. 38 (05), 1043–1045. doi:10.3969/j.issn.1005-9202.2018.05.008
China Food and Drug Administration (2010) National drug standards WS3-B-3428-98-2010Z. Beijing, China: China Food and Drug Administration.
Chinese Society of Neurology and Chinese Stroke Society (2018). Chinese guidelines for diagnosis and treatment of acute ischemic stroke 2018. Chin. J. Neurol. 51 (9), 666–682. doi:10.3760/cma.j.issn.1006-7876.2018.09.004
Dang, J., Sun, H., Dong, W., Li, Q., Feng, Z., and Lei, X. (2017). Protective effect of Shenmai Injection on the behavior chang ge in adult rats after neonatal maternal separation. Chin. J. Clin. Pharmacol. 33 (04), 335–337+342. doi:10.13699/j.cnki.1001-6821.2017.04.013
Dong, R., Huang, R., Shi, X., Xu, Z., and Mang, J. (2021). Exploration of the mechanism of luteolin against ischemic stroke based on network pharmacology, molecular docking and experimental verification. Bioengineered 12 (2), 12274–12293. doi:10.1080/21655979.2021.2006966
Ge, Y. H., Chen, Y. Y., Zhou, G. S., Liu, X., Tang, Y. P., Liu, R., et al. (2018). A novel antithrombotic protease from marine worm sipunculus nudus. Int. J. Mol. Sci. 19 (10), 3023. doi:10.3390/ijms19103023
Han, J., Mao, W., Ni, J., Wu, Y., Liu, J., Bai, L., et al. (2020). Rate and determinants of recurrence at 1 Year and 5 Years after stroke in a low-income population in rural China. Front. Neurol. 11, 2. doi:10.3389/fneur.2020.00002
Han, M. (2018). Clinical effect of Shenmai Injection in acute cerebral infarction and its effect on hemodynamics and inflammatory factors. Clini. Res. Pract. 3 (14), 112–113. doi:10.19347/j.cnki.2096-1413.201814053
Heinrich, M., Jalil, B., Abdel-Tawab, M., Echeverria, J., Kulić, Ž., McGaw, L. J., et al. (2022). Best Practice in the chemical characterisation of extracts used in pharmacological and toxicological research-The ConPhyMP-Guidelines. Front. Pharmacol. 13, 953205. doi:10.3389/fphar.2022.953205
Hu, M. (2018). Effect of shenmai injection combined with ozagrel on NIHSS score and serum levels of hcy and LP(a) in patients with acute ischemia stroke. J. Med. Theory. Pract. 31 (07), 943–945. doi:10.19381/j.issn.1001-7585.2018.07.003
Jia, X., Xie, L., Liu, Y., Liu, T., Yang, P., Hu, J., et al. (2022). Astragalus polysaccharide (APS) exerts protective effect against acute ischemic stroke (AIS) through enhancing M2 micoglia polarization by regulating adenosine triphosphate (ATP)/purinergic receptor (P2X7R) axis. Bioengineered 13 (2), 4468–4480. doi:10.1080/21655979.2021.1980176
Jiang, X., Xu, J., Hao, X., Xue, J., Li, K., Jin, A., et al. (2021). Elevated lipoprotein(a) and lipoprotein-associated phospholipase A 2 are associated with unfavorable functional outcomes in patients with ischemic stroke. J. Neuroinflammation 18 (1), 307. doi:10.1186/s12974-021-02359-w
Lei, H., Xu, J., Cheng, L. J., Guo, Q., Deng, A. M., and Li, Y. S. (2014). An increase in the cerebral infarction area during fatigue is mediated by il-6 through an induction of fibrinogen synthesis. Clin. (Sao Paulo) 69 (6), 426–432. doi:10.6061/clinics/2014(06)10
Li, X., and Dai, Z. (2022). Clinical study of Shenmai Injection in the treatment of patients with acute ischaemic stroke. Mod. Med. Health. Res. Elect. J. 6 (10), 24–27.
Li, Y., and Chen, L. (2019). Clinical effect of edaravone injection combined with Shenmai Injection in treating elderly patients with ischemic stroke and the impact on serum levels of vaspin and resistin, and cerebral hemodynamics. Pract. J. Card. Cereb. Pneum. Vasc. Dis. 27 (10), 112–116. doi:10.3969/j.issn.1008-5971.2019.10.021
Liang, X., Wang, W., Shi, Q., Chen, X., and Hu, G. (2018). Clinical observation on shenmai injection combined with rosuvastatin calcium for acute ischemic stroke. New. Chin. Med. 50 (11), 62–66. doi:10.13457/j.cnki.jncm.2018.11.017
Lin, S. Y., Law, K. M., Yeh, Y. C., Wu, K. C., Lai, J. H., Lin, C. H., et al. (2022). Applying machine learning to carotid sonographic features for recurrent stroke in patients with acute stroke. Front. Cardiovasc. Med. 9, 804410. doi:10.3389/fcvm.2022.804410
Liu, H., Liu, M., and Ma, L. (2016). Effect of Shenmai Injection on blood interleukin 6 in patients with cerebral infarction. Chin. J. Pract. Nerv. Dis. 19 (012), 124. doi:10.3969/j.issn.1673-5110.2016.12.081
Liu, M., Zhang, Q., Chen, X., and Li, K. (2017). Efficacy and safety of shenmai injection in treatment of acute cerebral infarction: a meta analysis. Liaoning J. Tradit. Chin. Med. 44 (02), 228–232. doi:10.13192/j.issn.1000-1719.2017.02.002
Liu, W., Miao, Y., Zhang, L., Xu, X., and Luan, Q. (2020). MiR-211 protects cerebral ischemia/reperfusion injury by inhibiting cell apoptosis. Bioengineered 11 (1), 189–200. doi:10.1080/21655979.2020.1729322
Liu, Y., Zhao, H., Zhang, H., Li, J., Shang, S., and Yu, X. (2020). Efficacy of shenmai injection in the treatment of neurological deterioration in early stage of acute ischemic stroke and its effects on serum cytokines. Eval. Anal. Drug-Use. Hosp. Chin. 20 (03), 286–289. doi:10.14009/j.issn.1672-2124.2020.03.007
Long, J., Sun, Y., Liu, S., Chen, C., Yan, Q., Lin, Y., et al. (2024). Ginsenoside Rg1 treats ischemic stroke by regulating CKLF1/CCR5 axis-induced neuronal cell pyroptosis. Phytomedicine 123, 155238. doi:10.1016/j.phymed.2023.155238
Lu, R., and Yang, P. (2013). Effect of Shenmai Injection on serum TNF-α and IL-6 in patients with acute ischaemic stroke. Cardiovasc. Dis. J. Integr. Tradit. Chin. West. Med. 11 (011), 1399–1400. doi:10.3969/j.issn.1672-1349.2013.11.070
Luo, B. Y., Jiang, J. L., Fang, Y. F., Yang, F., Yin, M. D., Zhang, B. C., et al. (2020). The effects of ginsenosides on platelet aggregation and vascular intima in the treatment of cardiovascular diseases: from molecular mechanisms to clinical applications. Pharmacol. Res. 159, 105031. doi:10.1016/j.phrs.2020.105031
Mendelson, S. J., and Prabhakaran, S. (2021). Diagnosis and management of transient ischemic attack and acute ischemic stroke: a review. JAMA 325 (11), 1088–1098. doi:10.1001/jama.2020.26867
Nishi, H., Hosomi, N., Ohta, K., Aoki, S., Nakamori, M., Nezu, T., et al. (2020). Serum immunoglobulin G antibody titer to Fusobacterium nucleatum is associated with unfavorable outcome after stroke. Clin. Exp. Immunol. 200 (3), 302–309. doi:10.1111/cei.13430
Papadopoulos, A., Palaiopanos, K., Björkbacka, H., Peters, A., de Lemos, J. A., Seshadri, S., et al. (2022). Circulating interleukin-6 levels and incident ischemic stroke: a systematic review and meta-analysis of prospective studies. Neurology 98 (10), e1002–e1012. doi:10.1212/WNL.0000000000013274
Powers, W. J., Rabinstein, A. A., Ackerson, T., Adeoye, O. M., Bambakidis, N. C., Becker, K., et al. (2019). Guidelines for the early management of patients with acute ischemic stroke: 2019 update to the 2018 guidelines for the early management of acute ischemic stroke: a guideline for healthcare professionals from the American heart association/American stroke association. Stroke 50 (12), e344–e418. doi:10.1161/STR.0000000000000211
Saengsuwan, J., Suangpho, P., and Tiamkao, S. (2017). Knowledge of stroke risk factors and warning signs in patients with recurrent stroke or recurrent transient ischaemic attack in Thailand. Neurol. Res. Int. 2017, 8215726. doi:10.1155/2017/8215726
Sapir, G., Shaul, D., Lev-Cohain, N., Sosna, J., Gomori, M. J., and Katz-Brull, R. (2021). LDH and PDH activities in the ischemic brain and the effect of reperfusion-an ex vivo MR study in rat brain slices using hyperpolarized [1-13C] pyruvate. Metabolites 11 (4), 210. doi:10.3390/metabo11040210
Sun, X. (2020). Observation on the effect of Shenmai Injection combined with butylphthalide Injection in the treatment of patients with acute watershed cerebral infarction. Chin. J. Mod. Drug. Appl. 14 (11), 114–115. doi:10.14164/j.cnki.cn11-5581/r.2020.11.052
Sun, X., Briel, M., Walter, S. D., and Guyatt, G. H. (2010). Is a subgroup effect believable? Updating criteria to evaluate the credibility of subgroup analyses. BMJ 340, c117. doi:10.1136/bmj.c117
Tang, Y. (2011). Observation on the therapeutic effect of Shenmai Injection in the treatment of 35 cases of acute watershed cerebral infarction. Zhejiang J. Tradit. Chin. Med. 46 (4), 2. doi:10.3969/j.issn.0411-8421.2011.04.002
Tsao, C. W., Aday, A. W., Almarzooq, Z. I., Alonso, A., Beaton, A. Z., Bittencourt, M. S., et al. (2022). Heart disease and stroke statistics-2022 update: a report from the American heart association. Circulation 145 (8), e153–e639. doi:10.1161/CIR.0000000000001052
Wang, J. (2014). Influence of ginseng injection on blood serum C-reactive protein of patients with acute ischemic brain stroke. Henan. Tradit. Chin. Med. 34 (04), 638–639. doi:10.16367/j.issn.1003-5028.2014.04.119
Wang, Q., Fang, F., Feng, W., and Li, X. (2013). Effects of Shenmai Injection on cerebral hemodynamics, hemorheology and serum TNF-α, IL-6 of acute cerebral infarction patients. Chin. J. Tradit. Med. Sci. Technol. 20 (03), 224–225+215. doi:10.3969/j.issn.1005-7072.2013.03.008
Wang, T., Yuan, F., Chen, Z., Zhu, S., Chang, Z., Yang, W., et al. (2020). Vascular, inflammatory and metabolic risk factors in relation to dementia in Parkinson's disease patients with type 2 diabetes mellitus. Aging 12 (15), 15682–15704. doi:10.18632/aging.103776
Wu, H., Liang, Y., Chen, J., Chen, W., Huang, T., Huang, T., et al. (2018). Effect of Shenmai Injection on scores of Qi deficiency syndrome, Yin deficiency syndrome and motor function of hemiplegic limbs in patients with cerebral watershed infarction. Chin. J. Integr. Tradit. West. Med. 38 (08), 950–953. doi:10.7661/j.cjim.20180627.185
Wu, W., Pu, Y., and Shi, J. (2022). Nanomedicine-enabled chemotherapy-based synergetic cancer treatments. VIP. Med. 20 (11), 4. doi:10.1186/s12951-021-01181-z
Xiao, W., Yang, Z., and Li, Y. (2019). In vitro study on the antioxidant activities of Shenmai Injection. Tianjin J. Tradit. Chin. Med. 36 (07), 697–700. doi:10.11656/j.issn.1672-1519.2019.07.18
Xu, H., Liu, Y., Wang, D., and Zhang, Z. (2019). Shenmai Injection maintains blood-brain barrier integrity following focal cerebral ischemia via modulating the expression and trafficking of occludin in lipid rafts. J. Ethnopharmacol. 237, 55–63. doi:10.1016/j.jep.2019.03.034
Zhang, F., Sun, Y., Zhang, L., Dong, Q., Chen, Z., and Liu, B. (2018). Clinical study on Shenmai Injection combined with butylphthalide in treatment of acute cerebral infarction. Drugs and Clin. 33 (07), 1635–1639. doi:10.7501/j.issn.1674-5515.2018.07.017
Zhang, K. J., Jin, H., Xu, R., Zhang, P., Guo, Z. N., and Yang, Y. (2021). N-terminal pro-brain natriuretic peptide is associated with hemorrhagic transformation and poor outcomes in patients with stroke treated with intravenous thrombolysis. Front. Mol. Neurosci. 14, 758915. doi:10.3389/fnmol.2021.758915
Zhao, M., Fu, L., and Chen, S. (2012). Protection effects of shenmai injection on focal cerebral lschemia in rats. Milit. Med. Jt. Logis. 26 (03), 212–215+228. doi:10.13730/j.1009-2595.2012.03.028
Zhou, M., Yuan, H., and Wu, S. (2019). Protective effects and mechanism of Ophiopogon japonicus polysaccha-ride on lipopolysaccharide-induced macrophage damage. Pharm. Clin. Res. 27 (6), 416–420. doi:10.13664/j.cnki.pcr.2019.06.004
Zuo, L., Xie, J., Liu, Y., Leng, S., Zhang, Z., and Yan, F. (2021). Down-regulation of circular RNA CDC14A peripherally ameliorates brain injury in acute phase of ischemic stroke. J. Neuroinflammation 18 (1), 283. doi:10.1186/s12974-021-02333-6
Keywords: acute ischemic stroke, Shenmai injection, efficacy, safety, randomized controlled trials, systematic review, meta-analysist
Citation: Zhao S, Sun T, Zhang M, Yan M, Wang K, Li L and Liu J (2024) Efficacy and safety of Shenmai injection for acute ischemic stroke: a systematic review and meta-analysis. Front. Pharmacol. 15:1394936. doi: 10.3389/fphar.2024.1394936
Received: 02 March 2024; Accepted: 14 May 2024;
Published: 04 June 2024.
Edited by:
Rolf Teschke, Hospital Hanau, GermanyReviewed by:
Yongsheng Chen, Jinan University, ChinaHoward Prentice, Florida Atlantic University, United States
Copyright © 2024 Zhao, Sun, Zhang, Yan, Wang, Li and Liu. This is an open-access article distributed under the terms of the Creative Commons Attribution License (CC BY). The use, distribution or reproduction in other forums is permitted, provided the original author(s) and the copyright owner(s) are credited and that the original publication in this journal is cited, in accordance with accepted academic practice. No use, distribution or reproduction is permitted which does not comply with these terms.
*Correspondence: Jinmin Liu, jmvip@vip.163.com