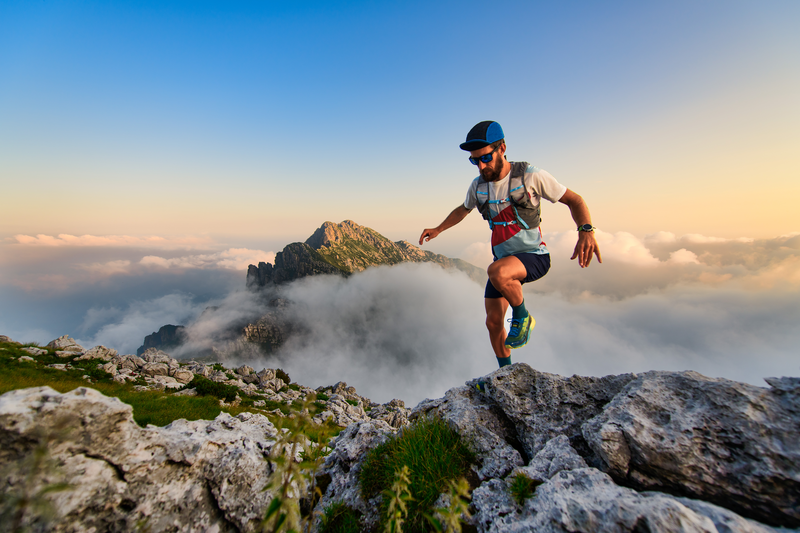
94% of researchers rate our articles as excellent or good
Learn more about the work of our research integrity team to safeguard the quality of each article we publish.
Find out more
ORIGINAL RESEARCH article
Front. Pharmacol. , 07 August 2024
Sec. Neuropharmacology
Volume 15 - 2024 | https://doi.org/10.3389/fphar.2024.1392832
This article is part of the Research Topic Neuropharmacological Intervention for Severe Mental Illness and Suicide Prevention View all 10 articles
Introduction: Suicide represents a significant public health problem whose neurobiology is not yet fully understood. In many cases, suicidal behavior and psychiatric spectrum disorders are linked, in particular, to major depression. An emerging pathophysiological hypothesis underlines the role of neurotrophic factors, proteins involved in neurogenesis, in synaptic plasticity in response to stressors. Our research aims to evaluate the degree of expression of brain neurotrophic factor (BDNF) in brain areas involved in depressive disorder in suicidal subjects. Furthermore, we want to evaluate the expression of glial cell line-derived neurotrophic factor (GDNF) in suicidal subjects.
Methods: We selected twenty confirmed cases of suicide among subjects with a clinical history of depressive pathology and possible psychopharmacological treatment, compared to ten controls of individuals who died of non-suicidal causes. For all selected cases and controls, immunohistochemical investigations were performed using a panel of antibodies against the BDNF and GDNF antigens on samples from the various brain areas.
Results and discussion: The results show that BDNF was under-expressed in the cerebral parenchyma of subjects who died by suicide compared to controls, while there was an overexpression of GDNF in suicide victims, these data could be useful for a clinical application as potential markers for suicidal risk, to assess the severity of depression and development of specific pharmacological therapies for depression.
According to the WHO, every year, about 700,000 people end their lives through suicide, making it the fourth leading cause of death in young people (datadot, 2024). According to estimates, for every suicide carried out, there are between 10 and 40 suicide attempts (Krug et al., 2002; Chang et al., 2011). Suicide turns out to be a real social problem, as it cannot leave public opinion indifferent. According to ISTAT (Italian National Institute of Statistics) data from 2016, 3780 committed suicides in Italy, 78.8% of whom were male. The death rate from suicide is 11.8 per 100,000 population for men and 3.0 per 100,000 population for women (Vichi et al., 2019). In Western countries, the suicide rate among men is three to four times higher than among women. This difference is likely to be the case because men use reliably more lethal means of taking their own lives. This difference is even more marked in men aged 65 years and older, who are likely to be ten times more than women (Sue et al., 2016). In the Eastern Mediterranean countries, on the other hand, female and male suicide rates are almost identical. In many countries, suicide rates appear to be higher in middle age or old age. The absolute number of suicides, however, is highest in the age group between 15 and 29 years old (Pitman et al., 2012; Yip et al., 2012). Suicide is also related to age, with young people under 25 and older adults interested the most in the risk of suicide, although it proportionally increases with age (OECD, 2012). In 2018, the ISTAT estimated that 3,820 people committed suicide, with the highest incidence in males between 35 and 64 years old. The main methods used to commit suicide are hanging, pesticide poisoning, and the use of firearms. In a survey (Ajdacic-Gross et al., 2008) carried out in 56 countries, hanging was by far the most common method used by 53% of male suicides and 39% of female suicides (O Connor et al., 2011; Suicide in the Province, 2022). Suicide presents numerous risk factors (Beghi et al., 2021; Risk and Protective Factors, 2023), such as individuals (previous suicide attempts, chronic psychiatric and physical pathologies, drug addiction), relationships (history of family suicide, isolation, bullying), community (violence, discrimination), and social-linked (spectacularization by the media, access to lethal means). One of the most important risk factors is the presence of psychiatric pathology, primarily major depression, followed by anxiety disorder, bipolar disorder, and drug addiction (Beghi et al., 2021). Also, genetics can influence the risk of committing a self-suppressive act (Brent and Melhem, 2008). Among psychiatric pathologies, it is clear that patients suffering from major depression present a significant risk of suicide or attempted suicide (Conejero et al., 2018; Fusar-Poli et al., 2021). Identifying risk factors can recognize a part of the population at high risk of committing extreme acts (Granier and Boulenger, 2002).
The neurobiology underlying suicide is exceptionally complex, not being the result of a single pathophysiological entity but of a multiplicity of behavioral, socio-environmental, and psychological causal factors that act together simultaneously. According to the literature, suicide follows the “diathesis-stress” model: a behavioral disorder derives from a genetic predisposition that makes one more vulnerable to stress, usually caused by life events (Mann, 2003; Van Heeringen and Mann, 2014; Zeigler-Hill and Shackelford, 2019). In particular, traumatic life events and stress acts as triggers for suicidal behavior (Dwivedi, 2012). Furthermore, the interaction of numerous biological systems is involved (Girardi and Pompili, 2015), including the neurotrophic system. Indeed, the pathogenesis of depression and suicidal behavior involves changes in neuronal plasticity (Garcia, 2002), reducing the brain’s ability to adapt.
Neurotrophins are growth factors that play a crucial role in regulating structural and synaptic plasticity, maintaining neuronal functions, and modulating neurotransmission (Thoenen, 1995). Neurotrophins are essential for the survival, growth, and regeneration of different neuronal populations belonging to the central and peripheral nervous systems in the fetal and adult brain (Allen et al., 2013). The first neurotrophin discovered in the 1950s was Nerve Growth Factor (NGF) (Levi-Montalcini, 1987). Neurotrophin is transported from the production site to the nerve terminal via axonal retrograde flow during development. Neurons that present this retrograde flow survive; otherwise, they degenerate (“neurotrophic hypothesis”) (Hamburger et al., 1981; Ginty and Segal, 2002). There are five neurotrophic factors expressed in mammals: Brain-Derived Neurotrophic Factor (BDNF), Neurotrophins 3 and 4 (NT-3 and NT-4), Glial Derived Neurotrophic Factor (GDNF) and Ciliar Neurotrophic Factor (CNTF). Neurotrophins are initially synthesized as pro-neurotrophins, and then through proteolytic processes, they are converted into mature neurotrophins that interact with two classes of receptors (p75NRT and Trk) to act (Ibáñez and Simi, 2012; Neurotrophic, 2017). Neurotrophins also have numerous actions outside the nervous system, such as immune (Thorpe and Perez Polo, 1987; Otten et al., 1989; Donovan et al., 2000) and hematopoietic function (Thorpe et al., 1987). They also control some neuroendocrine functions (for example, the development of the female rat’s hypothalamus) (Ojeda et al., 1991) and are involved in the transmission of pain (Skaper, 2017). Furthermore, these molecules can increase cholinergic function and protect against neurodegeneration (Hock et al., 2000; Hoxha et al., 2018), hypothesizing a role in Alzheimer’s disease (Hefti, 1986). Similarly, the GDNF can be involved in Parkinson’s disease, as it acts on dopaminergic neurons (Yasuda and Mochizuki, 2010). Therefore, a pathological alteration of neurotrophic factors could determine defects in neural maintenance and regeneration, possible structural anomalies in the brain, and a reduction in neural plasticity, thus compromising the individual’s ability to adapt in critical situations.
BDNF (“Brain-Derived Neurotrophic Factor”) is the most widespread neurotrophin in the brain of mammals, both in adults and in the developing phase (Anisman et al., 2012). It is involved in neurogenesis, the development of neurons, neuronal homeostasis, the structural integrity and maintenance of neuronal plasticity in the adult brain (Altar et al., 1997; Bartrup et al., 1997; Edelmann et al., 2014; Panja and Bramham, 2014), synaptic connection, in the mechanisms that regulate learning and memory (Kang and Schuman, 1995), drug addiction, and in stress adaptation mechanisms. Numerous exogenous and endogenous stimuli (such as stress, physical activity, diet, and brain injury) regulate BDNF expression (Aid et al., 2007; Pruunsild et al., 2007). Several neuropathologies cause a reduction in BDNF protein levels and serum in patients’ brains (Autry and Monteggia, 2012; Borba et al., 2016). Unfortunately, it is unclear whether serum BDNF levels reflect brain levels, as studies contradict each other (Sartorius et al., 2009; Klein et al., 2011). However, serum BDNF levels should be higher than those in plasma and cerebrospinal fluid (Radka et al., 1996; Serra-Millàs, 2016). According to some clinical studies that examined the blood levels of BDNF in vivo, the BDNF can cross the blood-brain barrier in both directions via a high-capacity saturable transport system, which determines an early influx and rapid in the brain (Pan et al., 1998). BDNF concentrations can be measured in serum, plasma, or whole blood and appear highly correlated to those in cerebrospinal fluid. Therefore, BDNF levels in the blood could be related to the concentration of the same neurotrophin at the level of the cerebral cortex (Klein et al., 2011). The blood quantification of BDNF in vivo could be a marker of neuronal plasticity (Shimizu et al., 2003). Furthermore, BDNF also plays an essential role in neuroinflammation and aging, identifying a role in degenerative diseases such as Parkinson’s and Alzheimer’s (Lima et al., 2019). In humans, BDNF is considered a promising biomarker for various psychiatric pathologies (Chen et al., 2017). In some animal models, mice heterozygous for the BDNF gene showed increased aggression and anxiety, significant weight gain, and memory impairment, suggesting that its depletion could be associated with the development of some psychiatric symptoms (Lindholm and Castracn, 2014). This hypothesis also seems supported by the fact that there is an increase in BDNF levels after treatment of psychiatric pathologies (Nuernberg et al., 2016; Castrén and Monteggia, 2021). Patients with major depression show an appreciable reduction in serum levels of BDNF (Shimizu et al., 2003), probably due to a reduction of the protein in the brain (Karege et al., 2005a). There is also a single nucleotide polymorphism of the BDNF gene (Val66Met) that is associated with the severity of depressive symptoms and memory deficits (Youssef et al., 2018; Zhao et al., 2018), as well as a higher risk of suicide attempts (Zai et al., 2012). Typically, treatment with antidepressant drugs increases BDNF levels in serum and plasma (Molendijk et al., 2011). Some post-mortem studies have shown that treatment-resistant patients had significantly low levels of BDNF, especially in areas such as the hippocampus, which otherwise is generally rich (Colla et al., 2007; Brunoni et al., 2014). Other studies have demonstrated reductions in levels of BDNF mRNA and its protein from post-mortem brain samples of depressed patients, in particular in the hippocampus and amygdala (Guilloux et al., 2012; Ray et al., 2014). The reduction of serum BDNF levels has also been ascertained in other psychiatric pathologies, such as schizophrenia (Ray et al., 2014), autism (Katoh Semba et al., 2007), and bipolar disorder (Fernandes et al., 2015). Furthermore, in animal models, BDNF levels in the prefrontal cortex and hippocampus were increased after pharmacological treatment with mood stabilizers (Yasuda et al., 2009; Jornada et al., 2010). In the literature, there are numerous studies carried out on the brains of suicidal victims, which observe a reduction in the levels of BDNF protein and its receptor in the hippocampus (Karege et al., 2005a; University, 2021) and the prefrontal cortex (Zheng et al., 2016; Misztak et al., 2020), as well as in its mRNA (Hamburger et al., 1981). Thus, BDNF levels could represent an interesting biological marker of suicidal behavior.
Glial cell line-derived neurotrophic factor (GDNF) is a neurotrophin expressed primarily during neuronal development and differentiation. In the adult, its expression decreases and remains limited to some regions, such as the cortex, the hippocampus, the substantia nigra, and the striatum nucleus (Duarte Azevedo et al., 2020). GDNF promotes the differentiation of dopaminergic (Christophersen et al., 2007) and serotonergic (Ducray et al., 2006) neurons. Furthermore, one of its most important roles is the protection of neurons (Hochstrasser et al., 2011; Uzdensky et al., 2013; Jaumotte and Zigmond, 2014) from oxidative stress and inflammation (Pascual et al., 2008). GDNF levels appear to be reduced in patients who have Alzheimer’s disease, leading to the hypothesis of its use as a biomarker for the pathology [ (Sharif et al., 2021; Kimhy et al., 2015)]. Although there are not many studies in the literature relating to the involvement of GDNF in the suicidal phenomenon, there is evidence linking this neurotrophin to the onset of mood disorders. In fact, according to some studies, there are lower serum GDNF levels in patients suffering from depression, with a subsequent increase after pharmacological treatment (Lin and Tseng, 2015; Wang et al., 2023). In a post-mortem study, an increase in GDNF was observed in the parietal cortex (site of emotion regulation (Anderson et al., 2004)) of patients with depressive disorder (Michel et al., 2008), while in other studies, a reduction in the expression of its mRNA was measured (Otsuki et al., 2008). In the literature, GDNF levels increase following ischemic or inflammatory damage (Wei et al., 2000; Michel et al., 2007) as neuronal resilience and plasticity compromise, hypothesizing that the increase in GDNF is an adaptive and compensatory response to neuronal damage (Chao and Lee, 1999; Tokumine et al., 2003).
Currently, many studies on the possible link between neurotrophins and suicide are carried out in vivo or plasma (Kim et al., 2007; Salas Magana et al., 2017; Sonal and Raghavan, 2018). Further interesting data could come from post-mortem studies carried out directly on the brains of suicidal subjects. An essential element is that many of these studies focus on the link between neurotrophins and neuro-psychiatric pathology. However, not all subjects who commit suicide suffer from a diagnosed psychiatric pathology. A recent literature review (De Simone et al., 2022) shows a paucity of experimental work on BDNF and GDNF. It shows that the altered regulation of BDNF, such as the Val66Met polymorphism, can favor the onset of psychiatric disorders linked to stress (Felmingham et al., 2013; Zhao et al., 2018; Miao et al., 2020), leading to an increase in suicidal risk according to some works (Zai et al., 2012; Paska et al., 2013), or no correlation with suicide, according to another study (Ratta-apha et al., 2013). Some of the studies examining the level of BDNF in post-mortem brain samples showed reduced values compared to controls, regardless of psychiatric diagnosis, in the prefrontal cortex and hippocampus (Dwivedi et al., 2003; Karege et al., 2005b; Ducray et al., 2006; Hochstrasser et al., 2011), as well as in the amygdala (Ray et al., 2014) and the anterior cingulate cortex (Tripp et al., 2012) of subjects suffering from major depressive disorder. Schneider et al. (2015) also evaluated subjects suffering from depression, detecting increased methylation of the BDNF promoter in the frontal cortex, in agreement with other studies (Keller et al., 2010; Kang et al., 2013). Another interesting finding, supported by further evidence (Pan et al., 1998; Klein et al., 2011), is that the brain level of BDNF was higher in suicidal subjects suffering from major depression on pharmacological therapy compared to non-suicidal controls (Gadad et al., 2021). As regards GDNF, in the study by Michel et al. (Michel et al., 2007), it was not possible to demonstrate a statistically significant increase in the levels of this neurotrophin in different brain areas of depressed patients taking antidepressant drug therapy.
The results of this systematic review partially support the hypothesis that a lower level of neurotrophins is connected to an increased risk of suicide. The identification of a suicide biomarker remains a challenge for the scientific and forensic community. The objective of the present study is to analyze the correlation between suicide and the degree of expression of BDNF and GDNF on autopsy samples in specific brain areas. These neurotrophic factors could have an important role both in the prevention of suicidal events in the population at high risk for anti-conservative behavior, allowing early action to limit this risk preventively, and in the search for a new potential pharmacological target. In the literature, there are no valuable markers for the identification of suicidal risk, so BDNF and GDNF could be promising for identifying suicidal risk in people with well-defined risk factors.
We conducted a retrospective study based on a series of judicial autopsies performed at the Forensic Medicine of the University of Foggia and the University of Ferrara.
The sample consists of twenty subjects who died following suicide between November 2020 and March 2023 (Table 1). The autopsies were performed 36–48 after the death and we excluded from the study corpses in an advanced stage of decomposition, corpses testing positive for common substances of abuse, and subjects suffering from neurodegenerative diseases, Alzheimer’s, and Parkinson’s disease. For subjects who used drugs, only benzodiazepines in three cases, we dosed the active ingredients, ensuring that they were at therapeutic doses (between 2 and 5 mg/day). We selected ten case controls, chosen among subjects who died of natural or only chest traumatic causes, without a history of psychiatric pathology or drug addiction, and who were negative for toxicological analyses.
In our sample, six individuals did not have a psychiatric diagnosis. However, they presented particularly critical and stressful recent events (e.g., bereavement, loss of job, imprisonment). The remaining fourteen cases, however, had a known psychiatric pathology; of these, six were affected by mood disorders (five from major depressive disorder, one from bipolar disorder), eight from other types of diagnosed psychiatric pathology (pathological gambling, substance use disorder, problematic use of alcohol, autism spectrum disorder, self-harm, adjustment disorder). The characteristics of these disorders are summarized in Table 2 based on the Diagnostic and Statistical Manual of Mental Disorders, 5th Edition (DSM-5). In all cases, we performed toxicological analyses on biological fluids to test the primary substances of abuse and the most common pharmacological active ingredients.
Table 2. The table shows the most relevant features of psychiatric disorders diagnosed in the selected cases, based on the Diagnostic and Statistical Manual of Mental Disorders, 5th Edition (DSM-5).
To exclude a possible influence of psychopharmacological active ingredients on the expression of BDNF and GDNF, we excluded subjects treated with antidepressant and antipsychotic drugs or positive for narcotic substances. Three of the twenty subjects studied regularly took only a mild therapy with sedative drugs (benzodiazepines), whose active ingredients, based on the results of toxicological analyses, still fell within the therapeutic prescribed dosage range.
For each case, the entire brain was removed during the autopsy examination, with appropriate preservation in formol solution for 3 weeks to perform a correct fixation of the tissues. The brain was dissected using sagittal cuts, identifying and taking four areas of interest from the right hemisphere: the prefrontal cortex, cingulate gyrus, basal nuclei, and hippocampus.
All the samples obtained were embedded in paraffin and treated by immunohistochemical staining for anti-BDNF and anti-GDNF antibodies to proceed with the search for neurotrophins. The two antibodies used are BDNF (monoclonal mouse antibody Catalog Number: 66292-1-ig, Proteintech Group, Chicago, United States), and GDNF (monoclonal rabbit antibody, Catalog Number: orb572592, Biorbyt Ltd., Cambridge, United Kingdom). For both antibodies, it was used the Protein Atlas website for the selection of positive and negative controls, and then it was performed various tests for the individuation of the correct pretreatment and dilution, according to the indications of the Production Company, published papers, and the suggestions of www.atlasantibodies.com.
For each case, sections of approximately 4 μm were made on the microtome. The sections, mounted on a slide, were hydrated in decreasing alcohol solutions. After inhibiting endogenous peroxidase, the sections were subjected to antigen retrieval in Citric acid 0.1 M and subsequently incubated with the primary antibody (dilution 1:200 for BDNF and 1:500 for GDNF). The formation of the immune complex was highlighted by applying a Streptavidin-Biotin system (HRP-DAB system research and development kit CTS005, R&D Systems, Inc., Minneapolis, MN, United States). The reaction was visualized by peroxidation of 3,3′-diaminobenzidine (DAB). Once the reaction was verified, the nuclei were counter-stained with hematoxylin, and subsequently slides were subjected to dehydration. The slide was then mounted and observed using a Nikon Eclipse E90i optical microscope, using the NIS – Elements F program. BDNF and GDNF immunopositivity in the right frontal cortex, hippocampus (dentate gyrus (DG) -Lacunar-molecular, Radiate, Granular layers and Hilus:.), girus of the cingulum, and basal nuclei. For the hippocampus, the granular layer and hilus are the selected areas for the statistical analysis because they are more significant. Four visual fields of approximately 350 μm × 350 μm were randomly selected in interest of each area for each sample of cases and controls. Images for each slide were acquired with a digital camera (Nikon) connected to the microscope at 10x for an exhaustive semiquantitative, preliminary evaluation of the reaction and after at 40 × magnification in the more significant area for quantification. The parameters for image acquisition were established at the beginning of the observation and kept constant for all images. Quantifying cells positive for DAB staining was performed using ImageJ software (imagej.nih.gov/ij/) and expressed as the number of positively stained cells per analyzed area. Quantifications were expressed as the number of positive-stained cells/analyzed area. For hippocampus we quantized the radiate layer and hilus because are the areas. Blind researchers performed histological analyses concerning the information about the cases. The blinding of the data was maintained until the analysis was terminated.
Data were analyzed using Windows GraphPad Prism 10 software for Windows (La Jolla, CA, United States). The data were analyzed through the two-way ANOVA analytical system (two-way ANOVA) for two independent factors. A P-value < 0.05 was considered statistically significant. Results are expressed as means ± standard deviation.
The two-way ANOVA highlighted a statistically significant difference in both cases (p < 0.0001). In particular, a reduced expression of BDNF was noted in suicide cases compared to controls, while the expression of GDNF was increased in cases compared to controls (Figures 1, 2).
Figure 1. BDNF immunohistochemical expression in the prefrontal cortex. Prefrontal cortex immunohistochemistry results: normal immunohistochemistry reaction of BDNF in the control case, see arrows (A) 10x magnification (B) 40x magnification; reduced expression of BDNF in a sample of suicide cases, see arrows (C) 10x magnification (D) 40x magnification. In the lower part of the figures, the graphical representation of the statistical analysis, the percentage decrease of BDNF in the suicide cases group is 24%.
Figure 2. GDNF immunohistochemical expression in the prefrontal cortex. Prefrontal cortex immunohistochemistry results: basal immunohistochemistry reaction of GDNF in thecontrol case (the control case used is the same as Figure 1, you can see in consecutive slice section the difference of reaction between the two markers), see arrows (A) 10x magnification (B) 40x magnification; over-expression of GDNF in a sample of suicide case, see arrows (C) 10x magnification (D) 40x magnification. The graphical representation of the statistical analysis is in the lower part of the figures.
The application of the two-way ANOVA test returned a statistically significant difference between the expression of BDNF (p < 0.001) and GDNF (<0.0001). In particular, it was possible to highlight a reduced expression of BDNF in suicide cases compared to controls, while the expression of GDNF was found to be increased in cases compared to controls (Figures 3, 4).
Figure 3. BDNF immunohistochemical expression in the hippocampus. Hippocampus (dentate gyrus) immunohistochemistry results: normal immunohistochemistry reaction of BDNF in the control case (granular layer of dentate gyrus), see arrows (A) 10x magnification (B) 40x magnification; reduced expression of BDNF in a sample of suicide case (granular layer of dentate gyrus), see arrows (C) 10x magnification (D) 40x magnification. In the lower part of the figures, the graphical representation of the statistical analysis, the percentage decrease of BDNF in the suicide cases group is 27%.
Figure 4. GDNF immunohistochemical expression in the hippocampus. Hippocampus (dentate gyrus) immunohistochemistry results: basal immunohistochemistry reaction of GDNF in the control case (hilus of dentate gyrus), see arrows (A) 10x magnification (B) 40x magnification; over-expression of GDNF in a sample of suicide case (granular layer of dentate gyrus), see arrows (C) 10x magnification (D) 40x magnification. In the lower part of the figures is the graphical representation of the statistical analysis.
The two-way ANOVA analysis highlighted a statistically significant difference in both cases (p < 0.0001). In particular, a reduced expression of BDNF was noted in suicide cases compared to controls, although less marked than in the other areas examined. Also, in this case, the expression of GDNF is increased in cases compared to controls (Figures 5, 6).
Figure 5. BDNF immunohistochemical expression in cingulum. Cingulum immunohistochemistry results: normal immunohistochemistry reaction of BDNF in the control case, see arrows (A) 10x magnification (B) 40x magnification; reduced expression of BDNF in a sample of suicide cases, see arrows (C) 10x magnification (D) 40x magnification. In the lower part of the figures, the graphical representation of the statistical analysis shows that the percentage decrease of BDNF in the suicide cases group is 21%.
Figure 6. GDNF immunohistochemical expression in cingulum. Cingulum immunohistochemistry results: basal immunohistochemistry reaction of GDNF in the control case, see arrows (A) 10x magnification (B) 40x magnification; over-expression of GDNF in a sample of suicide case, see arrows (C) 10x magnification (D) 40x magnification. The graphical representation of the statistical analysis is in the lower part of the figures.
The two-way ANOVA showed a statistically significant difference in the expression of BDNF (p < 0.001), while no statistically significant differences were highlighted in GDNF (p < 0.1). In particular, a reduced expression of BDNF was noted in suicide cases compared to controls, while a minimal increase in GDNF expression was observed in cases compared to controls (Figures 7, 8).
Figure 7. BDNF immunohistochemical expression in basal nuclei. Basal nuclei immunohistochemistry results: normal immunohistochemistry reaction of BDNF in the control case, see arrows (A) 10x magnification (B) 40x magnification; reduced expression of BDNF in a sample of suicide case, see arrows (C) 10x magnification (D) 40x magnification. In the lower part of the figures, the graphical representation of the statistical analysis shows that the percentage decrease of BDNF in the suicide cases group is 21%.
Figure 8. GDNF immunohistochemical expression in basal nuclei. Basal nuclei immunohistochemistry results: basal immunohistochemistry reaction of GDNF in the control case, see arrows (A) 10x magnification (B) 40x magnification; similar basal expression of GDNF in a sample of suicide case, see arrows (C) 10x magnification (D) 40x magnification. In the lower part of the figures is the graphical representation of the statistical analysis.
There are few studies in the literature relating to the measure of BDNF and GDNF on post-mortem samples of suicidal subjects, especially those without psychiatric pathologies. Our work aims to contribute to science to identify molecules useful as markers of suicide risk. These neurotrophins could also be potential pharmacological targets for researching and developing new targeted therapies.
The sample examined in our study was composed of twenty subjects who died by suicide and ten controls who died of non-suicidal causes, matched for sex and age, who underwent autopsy examination. 90% of subjects were male (18 of 20), 10% were female (2 of 20), 13 of them (65%) were under 50 years old, and 7 (35%) were over 50 years old. Furthermore, 6 out of 20 individuals (30%) did not have any diagnosis of psychiatric disorder. However, they had been involved in recent stressful events, for example, bereavement, job loss, and imprisonment. The remaining 14 cases (70%), however, presented a psychiatric pathology; of these, six were affected by mood disorders (five from major depressive disorder, one from bipolar disorder), eight from other types of diagnosed psychiatric pathology (pathological gambling, substance use disorder, problematic use of alcohol, autism spectrum disorder, self-harm, adjustment disorder). Five of the 20 cases analyzed (25%) were subjects subjected to a prison regime, another risk factor.
To exclude the possible influence of psychopharmacological active ingredients on the expression of BDNF and GDNF, the authors excluded patients on therapy with antidepressant and antipsychotic drugs and those who tested positive for narcotic and psychoactive substances in the toxicological analyses. Therefore, subjects who committed suicide due to overdose were also excluded. Only three of the 20 subjects studied were taking mild therapy based on non-neuroleptic or antidepressant drugs (mainly benzodiazepines) whose active ingredients, based on the results of toxicological analyses, were still within the therapeutic dosage range.
11 out of 20 subjects (55%) died from hanging [the most used method, according to literature data (Baldari et al., 2021)], 4 out of 20 (20%) from precipitation, 2 out of 20 (10%) from stab wounds, 2 in 20 (10%) by drowning and 1 in 20 (5%) by gunshot wounds.
The results of our work, which used an immunohistochemical method widely used in the field of forensic pathology (Baldari et al., 2021), highlighted a statistically significant reduction in BDNF levels in the various brain regions examined in all twenty suicidal subjects compared to the ten controls. In particular, the areas most affected are the prefrontal cortex and cingulate gyrus (p < 0.0001), to a slightly lesser extent, hippocampus and basal nuclei (p < 0.001). These data agree with the literature (Middlemas et al., 1991; Karege et al., 2002; Dwivedi et al., 2003; Karege et al., 2005b; Tripp et al., 2012), which reports significant decreases in BDNF levels in the prefrontal cortex, hippocampus, and cingulate gyrus. Furthermore, it is interesting to consider that the global examination of the different brain areas highlighted a statistically significant reduction in BDNF in suicidal subjects compared to controls (p < 0.0001).
The encephalic expression of GDNF was increased in all 20 study subjects. In particular, the authors observed a statistically significant (p < 0.0001) increase in GDNF in suicidal subjects compared to controls at the prefrontal cortex, hippocampus, and cingulate gyrus. In the basal nuclei of suicidal subjects, however, there was a slight increase in GDNF levels compared to controls, which was not statistically significant. Interestingly, the global examination of the different brain areas highlighted an increase in GDNF in suicidal subjects, compared to controls, which was statistically significant (p < 0.0001).
Our research is unique, as we analyzed the expression of GDNF on the brain tissue of suicidal subjects without therapy. The few studies in the literature on GDNF have evaluated its concentration in the peripheral blood of patients with depressive or, more generally, mood disorders.
The results obtained agree with the data of another work (Rosa et al., 2006), which documented an increase in GDNF in the peripheral blood of patients who have bipolar disorder in the depressive phase. Thus, increased BDNF synthesis could be a characteristic of acute episodes of mood disorders, although the literature is unclear (Takebayashi et al., 2006; Otsuki et al., 2008).
An important point of the study is that we have only selected autoptic cases of subjects who have not undergone drug therapy. In literature, most of the studies concern subjects on antidepressant drug therapy, finding a reduction in the expression of GDNF in plasma or blood (Maheu et al., 2015). On the contrary, in our study, the GDNF molecule in the brain is increased. It suggests that central GDNF signaling may represent a novel target for antidepressant treatment (Maheu et al., 2015).
In our study, we evaluate neurotrophic expression at the brain tissue, while other studies evaluate it at the plasma peripheral level (Sun et al., 2019). It would be interesting to conduct a multidisciplinary clinic-based assessment to observe whether the reduction in GDNF correlates with an improvement in symptoms.
The authors analyzed three further subgroups of the 20 cases: six subjects suffering from psychiatric pathology, eight subjects suffering from mood disorders, and six normal subjects. This analysis revealed a statistically significant reduction (p < 0.0001) in the expression of BDNF in all psychiatric subjects compared to the control group in all encephalic areas. In contrast, no statistically significant variations appeared in comparison between depressed individuals and those with other mental disorders. The same result was obtained with the GNDF, with a statistically significant increase (p < 0.0001) in all brain areas of psychiatric subjects compared to the control group. In contrast, there were no statistically significant variations in the comparison between depressed individuals and those with other psychic disorders.
The results we obtained on the expression of BDNF and GDNF, in agreement with data from works published in the literature, support the hypothesis that lower levels of BDNF and higher levels of GDNF correlate with an increased risk of suicide. The use of these markers could have several clinical implications. BDNF and GDNF testing could be valuable markers for screening patients most at risk of suicide based on demographic and clinical data to estimate suicidal risk. These markers measured in vivo and correlated with clinical symptomatology could help assess the severity of depressive symptoms, such as the presence of polymorphism of the BDNF gene (Val66Met). Further studies could have important clinical implications using BDNF and GDNF as targets for specific pharmacological therapies for depression.
This study could be a pilot study to continue the research on a larger sample of subjects who died as a result of suicide. It would, therefore, be necessary to increase the sample size, standardize the data collection methods, and group the samples according to their characteristics. In our study, there is a wide heterogeneity regarding the method of suicide and psychiatric pathology. In addition, the exclusion of psychoactive substance users may have led to a selection bias, although it was necessary to identify the modification in BDNF and GDNF correctly.
The raw data supporting the conclusions of this article will be made available by the authors, without undue reservation.
The studies involving humans were approved by Ethics Committee N 57/CE/ 2024 of the “Riuniti” Hospital of Foggia, Italy. The studies were conducted in accordance with the local legislation and institutional requirements. The study was performed in accordance with the guidelines of the Declaration of Helsinki. This study was performed by using human post-mortem brain samples collected during autopsies ordered by the prosecutor and used at the end of the investigations; It is a retrospective study on samples collected during the autopsy, and the acquisition of informed consent is not possible, because the heirs cannot be traced.
SD: Writing–review and editing, Writing–original draft, Resources, Validation, Conceptualization, Project administration. LA: Software, Writing–review and editing, Visualization, Writing–original draft, Resources. MAB: Writing–original draft, Formal Analysis. SC: Writing–original draft, Investigation, Supervision. MC: Writing–review and editing, Writing–original draft. LC: Methodology, Project administration, Writing–review and editing. MN: Conceptualization, Writing–original draft, Investigation, Project administration, Validation, Writing–review and editing, Supervision.
The author(s) declare that no financial support was received for the research, authorship, and/or publication of this article.
Thanks to Dott.ssa Mika and Dott.ssa Formalina for the friendly and constant support.
The authors declare that the research was conducted in the absence of any commercial or financial relationships that could be construed as a potential conflict of interest.
The author(s) declared that they were an editorial board member of Frontiers, at the time of submission. This had no impact on the peer review process and the final decision.
All claims expressed in this article are solely those of the authors and do not necessarily represent those of their affiliated organizations, or those of the publisher, the editors and the reviewers. Any product that may be evaluated in this article, or claim that may be made by its manufacturer, is not guaranteed or endorsed by the publisher.
Aid, T., Kazantseva, A., Piirsoo, M., Palm, K., and Timmusk, T. (2007). Mouse and rat BDNF gene structure and expression revisited. J Neurosci. Res. 85, 525–535. doi:10.1002/jnr.21139
Ajdacic-Gross, V., Weiss, M. G., Ring, M., Hepp, U., Bopp, M., Gutzwiller, F., et al. (2008). Methods of suicide: international suicide patterns derived from the WHO mortality database. Bull. World Health Organ 86, 726–732. doi:10.2471/blt.07.043489
Allen, S. J., Watson, J. J., Shoemark, D. K., Barua, N. U., and Patel, N. K. (2013). GDNF, NGF and BDNF as therapeutic options for neurodegeneration. Pharmacol. Ther. 138, 155–175. doi:10.1016/j.pharmthera.2013.01.004
Altar, C. A., Cai, N., Bliven, T., Juhasz, M., Conner, J. M., Acheson, A. L., et al. (1997). Anterograde transport of brain-derived neurotrophic factor and its role in the brain. Nature 389, 856–860. doi:10.1038/39885
Anderson, A., Oquendo, M. A., Parsey, R. V., Milak, M. S., Campbell, C., and Mann, J. J. (2004). Regional brain responses to serotonin in major depressive disorder. J. Affect. Disord. 82, 411–417. doi:10.1016/j.jad.2004.04.003
Anisman, H., Merali, Z., and Poulte, M. O. (2012). “Gamma-aminobutyric acid involvement in depressive illness interactions with corticotropin-releasing hormone and serotonin,” in The neurobiological Basis of suicide. Frontiers in neuroscience. Editor Y. Dwivedi (Boca Raton (FL): CRC Press/Taylor and Francis).
Autry, A. E., and Monteggia, L. M. (2012). Brain-derived neurotrophic factor and neuropsychiatric disorders. Pharmacol. Rev. 64, 238–258. doi:10.1124/pr.111.005108
Baldari, B., Vittorio, S., Sessa, F., Cipolloni, L., Bertozzi, G., Neri, M., et al. (2021). Forensic application of monoclonal anti-human glycophorin A antibody in samples from decomposed bodies to establish vitality of the injuries. A preliminary experimental study. Healthcare 9, 514. doi:10.3390/healthcare9050514
Bartrup, J. T., Moorman, J. M., and Newberry, N. R. (1997). BDNF enhances neuronal growth and synaptic activity in hippocampal cell cultures. NeuroReport 8, 3791–3794. doi:10.1097/00001756-199712010-00027
Beghi, M., Butera, E., Cerri, C. G., Cornaggia, C. M., Febbo, F., Mollica, A., et al. (2021). Suicidal behaviour in older age: a systematic review of risk factors associated to suicide attempts and completed suicides. Neurosci. Biobehav. Rev. 127, 193–211. doi:10.1016/j.neubiorev.2021.04.011
Borba, E. M., Duarte, J. A., Bristot, G., Scotton, E., Camozzato, A. L., and Chaves, M. L. F. (2016). Brain-derived neurotrophic factor serum levels and hippocampal volume in mild cognitive impairment and dementia due to alzheimer disease. Dement. Geriatr. Cogn. Disord. Extra 6, 559–567. doi:10.1159/000450601
Brent, D. A., and Melhem, N. (2008). Familial transmission of suicidal behavior. Psychiatric Clin. N. Am. 31, 157–177. doi:10.1016/j.psc.2008.02.001
Brunoni, A. R., Machado-Vieira, R., Zarate, C. A., Vieira, E. L. M., Vanderhasselt, M.-A., Nitsche, M. A., et al. (2014). BDNF plasma levels after antidepressant treatment with sertraline and transcranial direct current stimulation: results from a factorial, randomized, sham-controlled trial. Eur. Neuropsychopharmacol. 24, 1144–1151. doi:10.1016/j.euroneuro.2014.03.006
Castrén, E., and Monteggia, L. M. (2021). Brain-derived neurotrophic factor signaling in depression and antidepressant action. Biol. Psychiatry 90, 128–136. doi:10.1016/j.biopsych.2021.05.008
Chang, B., Gitlin, D., and Patel, R. (2011). The depressed patient and suicidal patient in the emergency department: evidence-based management and treatment strategies. Emerg. Med. Pract. 13 (1–23), 1–24.
Chao, C. C., and Lee, E. H. Y. (1999). Neuroprotective mechanism of glial cell line-derived neurotrophic factor on dopamine neurons: role of antioxidation. Neuropharmacology 38, 913–916. doi:10.1016/S0028-3908(99)00030-1
Chen, S., Jiang, H., Liu, Y., Hou, Z., Yue, Y., Zhang, Y., et al. (2017). Combined serum levels of multiple proteins in tPA-BDNF pathway may aid the diagnosis of five mental disorders. Sci. Rep. 7, 6871. doi:10.1038/s41598-017-06832-6
Christophersen, N. S., Grønborg, M., Petersen, T. N., Fjord-Larsen, L., Jørgensen, J. R., Juliusson, B., et al. (2007). Midbrain expression of Delta-like 1 homologue is regulated by GDNF and is associated with dopaminergic differentiation. Exp. Neurol. 204, 791–801. doi:10.1016/j.expneurol.2007.01.014
Colla, M., Kronenberg, G., Deuschle, M., Meichel, K., Hagen, T., Bohrer, M., et al. (2007). Hippocampal volume reduction and HPA-system activity in major depression. J. Psychiatric Res. 41, 553–560. doi:10.1016/j.jpsychires.2006.06.011
Conejero, I., Olié, E., Calati, R., Ducasse, D., and Courtet, P. (2018). Psychological pain, depression, and suicide: recent evidences and future directions. Curr. Psychiatry Rep. 20, 33. doi:10.1007/s11920-018-0893-z
datadot (2024). Suicide mortality rate (per 100 000 population). Available at: https://data.who.int/indicators/i/16BBF41 (Accessed February 26, 2024).
De Simone, S., Bosco, M. A., La Russa, R., Vittorio, S., Di Fazio, N., Neri, M., et al. (2022). Suicide and neurotrophin factors: a systematic review of the correlation between BDNF and GDNF and self-killing. Healthcare 11, 78. doi:10.3390/healthcare11010078
Donovan, M. J., Lin, M. I., Wiegn, P., Ringstedt, T., Kraemer, R., Hahn, R., et al. (2000). Brain derived neurotrophic factor is an endothelial cell survival factor required for intramyocardial vessel stabilization. Development 127, 4531–4540. doi:10.1242/dev.127.21.4531
Duarte Azevedo, M., Sander, S., and Tenenbaum, L. (2020). GDNF, A neuron-derived factor upregulated in glial cells during disease. JCM 9, 456. doi:10.3390/jcm9020456
Ducray, A., Krebs, S. H., Schaller, B., Seiler, R. W., Meyer, M., and Widmer, H. R. (2006). GDNF family ligands display distinct action profiles on cultured GABAergic and serotonergic neurons of rat ventral mesencephalon. Brain Res. 1069, 104–112. doi:10.1016/j.brainres.2005.11.056
Dwivedi, Y. (2012). The neurobiological basis of suicide (Boca Raton, FL: Taylor and Francis/CRC Press), 454.
Dwivedi, Y., Rizavi, H. S., Conley, R. R., Roberts, R. C., Tamminga, C. A., and Pandey, G. N. (2003). Altered gene expression of brain-derived neurotrophic factor and receptor tyrosine kinase B in postmortem brain of suicide subjects. Arch. Gen. Psychiatry 60, 804–815. doi:10.1001/archpsyc.60.8.804
Edelmann, E., Leßmann, V., and Brigadski, T. (2014). Pre- and postsynaptic twists in BDNF secretion and action in synaptic plasticity. Neuropharmacology 76, 610–627. doi:10.1016/j.neuropharm.2013.05.043
Felmingham, K. L., Dobson-Stone, C., Schofield, P. R., Quirk, G. J., and Bryant, R. A. (2013). The brain-derived neurotrophic factor Val66Met polymorphism predicts response to exposure therapy in posttraumatic stress disorder. Biol. Psychiatry 73, 1059–1063. doi:10.1016/j.biopsych.2012.10.033
Fernandes, B. S., Molendijk, M. L., Köhler, C. A., Soares, J. C., Leite, CMGS, Machado-Vieira, R., et al. (2015). Peripheral brain-derived neurotrophic factor (BDNF) as a biomarker in bipolar disorder: a meta-analysis of 52 studies. BMC Med. 13, 289. doi:10.1186/s12916-015-0529-7
Fusar-Poli, L., Aguglia, A., Amerio, A., Orsolini, L., Salvi, V., Serafini, G., et al. (2021). Peripheral BDNF levels in psychiatric patients with and without a history of suicide attempt: a systematic review and meta-analysis. Prog. Neuro-Psychopharmacology Biol. Psychiatry 111, 110342. doi:10.1016/j.pnpbp.2021.110342
Gadad, B. S., Vargas-Medrano, J., Ramos, E. I., Najera, K., Fagan, M., Forero, A., et al. (2021). Altered levels of interleukins and neurotrophic growth factors in mood disorders and suicidality: an analysis from periphery to central nervous system. Transl. Psychiatry 11, 341. doi:10.1038/s41398-021-01452-1
Garcia, R. (2002). Stress, metaplasticity, and antidepressants. Curr. Mol. Med. 2, 629–638. doi:10.2174/1566524023362023
Ginty, D., and Segal, R. A. (2002). Retrograde neurotrophin signaling: Trk-ing along the axon. Curr. Opin. Neurobiol. 12, 268–274. doi:10.1016/S0959-4388(02)00326-4
Guilloux, J.-P., Douillard-Guilloux, G., Kota, R., Wang, X., Gardier, A. M., Martinowich, K., et al. (2012). Molecular evidence for BDNF- and GABA-related dysfunctions in the amygdala of female subjects with major depression. Mol. Psychiatry 17, 1130–1142. doi:10.1038/mp.2011.113
Hamburger, V., Brunso-Bechtold, J., and Yip, J. (1981). Neuronal death in the spinal ganglia of the chick embryo and its reduction by nerve growth factor. J. Neurosci. 1, 60–71. doi:10.1523/JNEUROSCI.01-01-00060.1981
Hefti, F. (1986). Nerve growth factor promotes survival of septal cholinergic neurons after fimbrial transections. J. Neurosci. 6, 2155–2162. doi:10.1523/JNEUROSCI.06-08-02155.1986
Hochstrasser, T., Ullrich, C., Sperner-Unterweger, B., and Humpel, C. (2011). Inflammatory stimuli reduce survival of serotonergic neurons and induce neuronal expression of indoleamine 2,3-dioxygenase in rat dorsal raphe nucleus organotypic brain slices. Neuroscience 184, 128–138. doi:10.1016/j.neuroscience.2011.03.070
Hock, C., Heese, K., Hulette, C., Rosenberg, C., and Otten, U. (2000). Region-specific neurotrophin imbalances in alzheimer disease: decreased levels of brain-derived neurotrophic factor and increased levels of nerve growth factor in Hippocampus and cortical areas. Arch. Neurol. 57, 846–851. doi:10.1001/archneur.57.6.846
Hoxha, E., Lippiello, P., Zurlo, F., Balbo, I., Santamaria, R., Tempia, F., et al. (2018). The emerging role of altered cerebellar synaptic processing in alzheimer’s disease. Front. Aging Neurosci. 10, 396. doi:10.3389/fnagi.2018.00396
Ibáñez, C. F., and Simi, A. (2012). p75 neurotrophin receptor signaling in nervous system injury and degeneration: paradox and opportunity. Trends Neurosci. 35, 431–440. doi:10.1016/j.tins.2012.03.007
Jaumotte, J. D., and Zigmond, M. J. (2014). Comparison of GDF5 and GDNF as neuroprotective factors for postnatal dopamine neurons in ventral mesencephalic cultures. J Neurosci. Res. 92, 1425–1433. doi:10.1002/jnr.23425
Jornada, L. K., Moretti, M., Valvassori, S. S., Ferreira, C. L., Padilha, P. T., Arent, C. O., et al. (2010). Effects of mood stabilizers on hippocampus and amygdala BDNF levels in an animal model of mania induced by ouabain. J. Psychiatric Res. 44, 506–510. doi:10.1016/j.jpsychires.2009.11.002
Kang, H., and Schuman, E. M. (1995). Long-lasting neurotrophin-induced enhancement of synaptic transmission in the adult Hippocampus. Science 267, 1658–1662. doi:10.1126/science.7886457
Kang, H.-J., Kim, J.-M., Lee, J.-Y., Kim, S.-Y., Bae, K.-Y., Kim, S.-W., et al. (2013). BDNF promoter methylation and suicidal behavior in depressive patients. J. Affect. Disord. 151, 679–685. doi:10.1016/j.jad.2013.08.001
Karege, F., Bondolfi, G., Gervasoni, N., Schwald, M., Aubry, J.-M., and Bertschy, G. (2005a). Low Brain-Derived Neurotrophic Factor (BDNF) levels in serum of depressed patients probably results from lowered platelet BDNF release unrelated to platelet reactivity. Biol. Psychiatry 57, 1068–1072. doi:10.1016/j.biopsych.2005.01.008
Karege, F., Perret, G., Bondolfi, G., Schwald, M., Bertschy, G., and Aubry, J.-M. (2002). Decreased serum brain-derived neurotrophic factor levels in major depressed patients. Psychiatry Res. 109, 143–148. doi:10.1016/S0165-1781(02)00005-7
Karege, F., Vaudan, G., Schwald, M., Perroud, N., and La Harpe, R. (2005b). Neurotrophin levels in postmortem brains of suicide victims and the effects of antemortem diagnosis and psychotropic drugs. Mol. Brain Res. 136, 29–37. doi:10.1016/j.molbrainres.2004.12.020
Katoh Semba, R., Wakako, R., Komori, T., Shigemi, H., Miyazaki, N., Ito, H., et al. (2007). Age-related changes in BDNF protein levels in human serum: differences between autism cases and normal controls. Intl J Devlp Neurosci. 25, 367–372. doi:10.1016/j.ijdevneu.2007.07.002
Keller, S., Sarchiapone, M., Zarrilli, F., Videtič, A., Ferraro, A., Carli, V., et al. (2010). Increased BDNF promoter methylation in the wernicke area of suicide subjects. Arch. Gen. Psychiatry 67, 258–267. doi:10.1001/archgenpsychiatry.2010.9
Kim, Y.-K., Lee, H.-P., Won, S.-D., Park, E.-Y., Lee, H.-Y., Lee, B.-H., et al. (2007). Low plasma BDNF is associated with suicidal behavior in major depression. Prog. Neuro-Psychopharmacology Biol. Psychiatry 31, 78–85. doi:10.1016/j.pnpbp.2006.06.024
Kimhy, D., Vakhrusheva, J., Bartels, M. N., Armstrong, H. F., Ballon, J. S., Khan, S., et al. (2015). The impact of aerobic exercise on brain-derived neurotrophic factor and neurocognition in individuals with schizophrenia: a single-blind, randomized clinical trial. SCHBUL 41, 859–868. doi:10.1093/schbul/sbv022
Klein, A. B., Williamson, R., Santini, M. A., Clemmensen, C., Ettrup, A., Rios, M., et al. (2011). Blood BDNF concentrations reflect brain-tissue BDNF levels across species. Int. J. Neuropsychopharm 14, 347–353. doi:10.1017/S1461145710000738
Krug, E. G., Mercy, J. A., Dahlberg, L. L., and Zwi, A. B. (2002). The world report on violence and health. Lancet 360, 1083–1088. doi:10.1016/S0140-6736(02)11133-0
Levi-Montalcini, R. (1987). The nerve growth factor 35 Years later. Science 237, 1154–1162. doi:10.1126/science.3306916
Lima, G. B., Doorduin, J., Klein, H. C., Dierckx, RAJO, Bromberg, E., and De Vries, E. F. J. (2019). Brain-derived neurotrophic factor in brain disorders: focus on neuroinflammation. Mol. Neurobiol. 56, 3295–3312. doi:10.1007/s12035-018-1283-6
Lin, P.-Y., and Tseng, P.-T. (2015). Decreased glial cell line-derived neurotrophic factor levels in patients with depression: a meta-analytic study. J. Psychiatric Res. 63, 20–27. doi:10.1016/j.jpsychires.2015.02.004
Lindholm, J. S. O., and CastrAcn, E. (2014). Mice with altered BDNF signaling as models for mood disorders and antidepressant effects. Front. Behav. Neurosci. 8, 8. doi:10.3389/fnbeh.2014.00143
Maheu, M., Lopez, J. P., Crapper, L., Davoli, M. A., Turecki, G., and Mechawar, N. (2015). MicroRNA regulation of central glial cell line-derived neurotrophic factor (GDNF) signalling in depression. Transl. Psychiatry 5, e511. doi:10.1038/tp.2015.11
Mann, J. J. (2003). Neurobiology of suicidal behaviour. Nat. Rev. Neurosci. 4, 819–828. doi:10.1038/nrn1220
Miao, Z., Wang, Y., and Sun, Z. (2020). The relationships between stress, mental disorders, and epigenetic regulation of BDNF. IJMS 21, 1375. doi:10.3390/ijms21041375
Michel, T. M., Frangou, S., Camara, S., Thiemeyer, D., Jecel, J., Tatschner, T., et al. (2008). Altered glial cell line-derived neurotrophic factor (GDNF) concentrations in the brain of patients with depressive disorder: a comparative post-mortem study. Eur. Psychiatr. 23, 413–420. doi:10.1016/j.eurpsy.2008.06.001
Michel, T. M., Frangou, S., Thiemeyer, D., Camara, S., Jecel, J., Nara, K., et al. (2007). Evidence for oxidative stress in the frontal cortex in patients with recurrent depressive disorder—a postmortem study. Psychiatry Res. 151, 145–150. doi:10.1016/j.psychres.2006.04.013
Middlemas, D. S., Lindberg, R. A., and Hunter, T. (1991). trkB, a neural receptor protein-tyrosine kinase: evidence for a full-length and two truncated receptors. Mol. Cell. Biol. 11, 143–153. doi:10.1128/mcb.11.1.143
Misztak, P., Pańczyszyn-Trzewik, P., Nowak, G., and Sowa-Kućma, M. (2020). Epigenetic marks and their relationship with BDNF in the brain of suicide victims. PLoS ONE 15, e0239335. doi:10.1371/journal.pone.0239335
Molendijk, M. L., Bus, B. A. A., Spinhoven, P., Penninx, BWJH, Kenis, G., Prickaerts, J., et al. (2011). Serum levels of brain-derived neurotrophic factor in major depressive disorder: state–trait issues, clinical features and pharmacological treatment. Mol. Psychiatry 16, 1088–1095. doi:10.1038/mp.2010.98
Neurotrophic (2017). Neurotrophic factors: methods and protocols. New York, NY: Springer Science+Business Media.
Nuernberg, G. L., Aguiar, B., Bristot, G., Fleck, M. P., and Rocha, N. S. (2016). Brain-derived neurotrophic factor increase during treatment in severe mental illness inpatients. Transl. Psychiatry 6, e985. doi:10.1038/tp.2016.227
O Connor, R. C., Platt, S., and Gordon, J. (2011). International handbook of suicide prevention: research, policy and practice. Chichester, West Sussex, U.K: Wiley-Blackwell, 677.
Ojeda, S. R., Hill, D. F., and Katz, K. H. (1991). The genes encoding nerve growth factor and its receptor are expressed in the developing female rat hypothalamus. Mol. Brain Res. 9, 47–55. doi:10.1016/0169-328X(91)90129-L
Otsuki, K., Uchida, S., Watanuki, T., Wakabayashi, Y., Fujimoto, M., Matsubara, T., et al. (2008). Altered expression of neurotrophic factors in patients with major depression. J. Psychiatric Res. 42, 1145–1153. doi:10.1016/j.jpsychires.2008.01.010
Otten, U., Ehrhard, P., and Peck, R. (1989). Nerve growth factor induces growth and differentiation of human B lymphocytes. Proc. Natl. Acad. Sci. U. S. A. 86, 10059–10063. doi:10.1073/pnas.86.24.10059
Pan, W., Banks, W. A., Fasold, M. B., Bluth, J., and Kastin, A. J. (1998). Transport of brain-derived neurotrophic factor across the blood–brain barrier. Neuropharmacology 37, 1553–1561. doi:10.1016/S0028-3908(98)00141-5
Panja, D., and Bramham, C. R. (2014). BDNF mechanisms in late LTP formation: a synthesis and breakdown. Neuropharmacology 76, 664–676. doi:10.1016/j.neuropharm.2013.06.024
Pascual, A., Hidalgo-Figueroa, M., Piruat, J. I., Pintado, C. O., Gómez-Díaz, R., and López-Barneo, J. (2008). Absolute requirement of GDNF for adult catecholaminergic neuron survival. Nat. Neurosci. 11, 755–761. doi:10.1038/nn.2136
Paska, A. V., Zupanc, T., and Pregelj, P. (2013). The role of brain-derived neurotrophic factor in the pathophysiology of suicidal behavior. Psychiatr. Danub 25 (Suppl. 2), S341–S344.
Pitman, A., Krysinska, K., Osborn, D., and King, M. (2012). Suicide in young men. Lancet 379, 2383–2392. doi:10.1016/S0140-6736(12)60731-4
Pruunsild, P., Kazantseva, A., Aid, T., Palm, K., and Timmusk, T. (2007). Dissecting the human BDNF locus: bidirectional transcription, complex splicing, and multiple promoters. Genomics 90, 397–406. doi:10.1016/j.ygeno.2007.05.004
Radka, S. F., Hoist, P. A., Fritsche, M., and Altar, C. A. (1996). Presence of brain-derived neurotrophic factor in brain and human and rat but not mouse serum detected by a sensitive and specific immunoassay. Brain Res. 709, 122–130. doi:10.1016/0006-8993(95)01321-0
Ratta-apha, W., Hishimoto, A., Yoshida, M., Ueno, Y., Asano, M., Shirakawa, O., et al. (2013). Association study of BDNF with completed suicide in the Japanese population. Psychiatry Res. 209, 734–736. doi:10.1016/j.psychres.2013.05.030
Ray, M. T., Shannon Weickert, C., and Webster, M. J. (2014). Decreased BDNF and TrkB mRNA expression in multiple cortical areas of patients with schizophrenia and mood disorders. Transl. Psychiatry 4, e389. doi:10.1038/tp.2014.26
Risk and Protective Factors (2023). Risk and protective factors | suicide prevention. China: CDC. Available at: https://www.cdc.gov/suicide/factors/index.html (Accessed November 7, 2022).
Rosa, A. R., Frey, B. N., Andreazza, A. C., Ceresér, K. M., Cunha, A. B. M., Quevedo, J., et al. (2006). Increased serum glial cell line-derived neurotrophic factor immunocontent during manic and depressive episodes in individuals with bipolar disorder. Neurosci. Lett. 407, 146–150. doi:10.1016/j.neulet.2006.08.026
Salas Magaña, M., Tovilla-Zárate, C. A., González-Castro, T. B., Juárez-Rojop, I. E., López-Narváez, M. L., Rodríguez-Pérez, J. M., et al. (2017). Decrease in brain-derived neurotrophic factor at plasma level but not in serum concentrations in suicide behavior: a systematic review and meta-analysis. Brain Behav. 7, e00706. doi:10.1002/brb3.706
Sartorius, A., Hellweg, R., Litzke, J., Vogt, M., Dormann, C., Vollmayr, B., et al. (2009). Correlations and discrepancies between serum and brain tissue levels of neurotrophins after electroconvulsive treatment in rats. Pharmacopsychiatry 42, 270–276. doi:10.1055/s-0029-1224162
Schneider, E., El Hajj, N., Müller, F., Navarro, B., and Haaf, T. (2015). Epigenetic dysregulation in the prefrontal cortex of suicide completers. Cytogenet Genome Res. 146, 19–27. doi:10.1159/000435778
Serra-Millàs, M. (2016). Are the changes in the peripheral brain-derived neurotrophic factor levels due to platelet activation? WJP 6, 84–101. doi:10.5498/wjp.v6.i1.84
Sharif, M., Noroozian, M., and Hashemian, F. (2021). Do serum GDNF levels correlate with severity of Alzheimer’s disease? Neurol. Sci. 42, 2865–2872. doi:10.1007/s10072-020-04909-1
Shimizu, E., Hashimoto, K., Okamura, N., Koike, K., Komatsu, N., Kumakiri, C., et al. (2003). Alterations of serum levels of brain-derived neurotrophic factor (BDNF) in depressed patients with or without antidepressants. Biol. Psychiatry 54, 70–75. doi:10.1016/S0006-3223(03)00181-1
Skaper, S. D. (2017). Nerve growth factor: a neuroimmune crosstalk mediator for all seasons. Immunology 151, 1–15. doi:10.1111/imm.12717
Sonal, A., and Raghavan, V. (2018). Brain derived neurotrophic factor (BDNF) and suicidal behavior: a review of studies from Asian countries. Asian J. Psychiatry 33, 128–132. doi:10.1016/j.ajp.2018.03.004
Sue, D., Sue, D. W., Sue, D. M., and Sue, S. (2016). Understanding abnormal behavior. Eleventh edition. Australia; Mexico: Cengage Learning, 567.
Suicide in the Province (2022) “Suicide in the Province of Foggia: retrospective study and analysis of the difference between men and women,”, 18. USA: JOMH, 1. doi:10.31083/j.jomh1808166
Sun, J., Kong, L., Wu, F., Wei, Y., Zhu, Y., Yin, Z., et al. (2019). Decreased plasma glial cell line-derived neurotrophic factor level in major depressive disorder is associated with age and clinical severity. J. Affect. Disord. 245, 602–607. doi:10.1016/j.jad.2018.11.068
Takebayashi, M., Hisaoka, K., Nishida, A., Tsuchioka, M., Miyoshi, I., Kozuru, T., et al. (2006). Decreased levels of whole blood glial cell line-derived neurotrophic factor (GDNF) in remitted patients with mood disorders. Int. J. Neuropsychopharm 9, 607–612. doi:10.1017/S1461145705006085
Thoenen, H. (1995). Neurotrophins and neuronal plasticity. Science 270, 593–598. doi:10.1126/science.270.5236.593
Thorpe, L. W., and Perez Polo, J. R. (1987). The influence of nerve growth factor on the in vitro proliferative response of rat spleen lymphocytes. J Neurosci. Res. 18, 134–139. doi:10.1002/jnr.490180120
Thorpe, L. W., Werrbach-Perez, K., and Perez-Polo, J. R. (1987). Effects of nerve growth factor on the expression of interleukin-2 receptors on cultured human lymphocytes. Ann. N. Y. Acad. Sci. 496, 310–311. doi:10.1111/j.1749-6632.1987.tb35781.x
Tokumine, J., Kakinohana, O., Cizkova, D., Smith, D. W., and Marsala, M. (2003). Changes in spinal GDNF, BDNF, and NT-3 expression after transient spinal cord ischemia in the rat. J Neurosci. Res. 74, 552–561. doi:10.1002/jnr.10760
Tripp, A., Oh, H., Guilloux, J.-P., Martinowich, K., Lewis, D. A., and Sibille, E. (2012). Brain-derived neurotrophic factor signaling and subgenual anterior cingulate cortex dysfunction in major depressive disorder. AJP 169, 1194–1202. doi:10.1176/appi.ajp.2012.12020248
University, I. (2021). Department of psychiatry, malatya, Turkey, gonenir erbay L, karlidag R, inonu university, department of psychiatry, malatya, Turkey, oruc M, inonu university, department of forensic medicine, malatya, Turkey, cigremis Y, inonu university, department of medical biology and genetics, malatya, Turkey, celbis O, inonu university, department of forensic medicine, malatya, Turkey. Association of BDNF/trkb and NGF/trka levels in postmortem brain with major depression and suicide. Psychiat Danub 33, 491–498. doi:10.24869/psyd.2021.491
Uzdensky, A., Komandirov, M., Fedorenko, G., and Lobanov, A. (2013). Protection effect of GDNF and neurturin on photosensitized crayfish neurons and glial cells. J. Mol. Neurosci. 49, 480–490. doi:10.1007/s12031-012-9858-6
Van Heeringen, K., and Mann, J. J. (2014). The neurobiology of suicide. Lancet Psychiatry 1, 63–72. doi:10.1016/S2215-0366(14)70220-2
Vichi, M., Ghirini, S., Pompili, M., Erbuto, D., and Siliquini, R. (2019). In rapporto Osservasalute 2018: stato di salute e qualità dell’assistenza nelle regioni italiane. Milano: Prex S.p.A.
Wang, H., Yang, Y., Pei, G., Wang, Z., and Chen, N. (2023). Neurotrophic basis to the pathogenesis of depression and phytotherapy. Front. Pharmacol. 14, 1182666. doi:10.3389/fphar.2023.1182666
Wei, G., Wu, G., and Cao, X. (2000). Dynamic expression of glial cell line-derived neurotrophic factor after cerebral ischemia. NeuroReport 11, 1177–1183. doi:10.1097/00001756-200004270-00007
Yasuda, S., Liang, M.-H., Marinova, Z., Yahyavi, A., and Chuang, D.-M. (2009). The mood stabilizers lithium and valproate selectively activate the promoter IV of brain-derived neurotrophic factor in neurons. Mol. Psychiatry 14, 51–59. doi:10.1038/sj.mp.4002099
Yasuda, T., and Mochizuki, H. (2010). Use of growth factors for the treatment of Parkinson’s disease. Expert Rev. Neurother. 10, 915–924. doi:10.1586/ern.10.55
Yip, P. S., Caine, E., Yousuf, S., Chang, S.-S., Wu, K. C.-C., and Chen, Y.-Y. (2012). Means restriction for suicide prevention. Lancet 379, 2393–2399. doi:10.1016/S0140-6736(12)60521-2
Youssef, M. M., Underwood, M. D., Huang, Y.-Y., Hsiung, S., Liu, Y., Simpson, N. R., et al. (2018). Association of BDNF Val66Met polymorphism and brain BDNF levels with major depression and suicide. Int. J. Neuropsychopharmacol. 21, 528–538. doi:10.1093/ijnp/pyy008
Zai, C. C., Manchia, M., De Luca, V., Tiwari, A. K., Chowdhury, N. I., Zai, G. C., et al. (2012). The brain-derived neurotrophic factor gene in suicidal behaviour: a meta-analysis. Int. J. Neuropsychopharm 15, 1037–1042. doi:10.1017/S1461145711001313
Zeigler-Hill, V., and Shackelford, T. K. (2019). Encyclopedia of personality and individual differences (Cham, Switzerland: Springer), 1.
Zhao, M., Chen, L., Yang, J., Han, D., Fang, D., Qiu, X., et al. (2018). BDNF Val66Met polymorphism, life stress and depression: a meta-analysis of gene-environment interaction. J. Affect. Disord. 227, 226–235. doi:10.1016/j.jad.2017.10.024
Keywords: suicide, neurotrophic factor, immunohistochemistry, BDNF, GDNF
Citation: De Simone S, Alfieri L, Bosco MA, Cantatore S, Carpinteri M, Cipolloni L and Neri M (2024) The forensic aspects of suicide and neurotrophin factors: a research study. Front. Pharmacol. 15:1392832. doi: 10.3389/fphar.2024.1392832
Received: 28 February 2024; Accepted: 26 July 2024;
Published: 07 August 2024.
Edited by:
Ewa Litwa, Polish Academy of Sciences, PolandReviewed by:
Daniela Vanesa Navarro, Miguel Hernández University of Elche, SpainCopyright © 2024 De Simone, Alfieri, Bosco, Cantatore, Carpinteri, Cipolloni and Neri. This is an open-access article distributed under the terms of the Creative Commons Attribution License (CC BY). The use, distribution or reproduction in other forums is permitted, provided the original author(s) and the copyright owner(s) are credited and that the original publication in this journal is cited, in accordance with accepted academic practice. No use, distribution or reproduction is permitted which does not comply with these terms.
*Correspondence: Margherita Neri, bWFyZ2hlcml0YS5uZXJpQHVuaWZlLml0
†These authors have contributed equally to this work and share first authorship
‡These authors have contributed equally to this work and share last authorship
Disclaimer: All claims expressed in this article are solely those of the authors and do not necessarily represent those of their affiliated organizations, or those of the publisher, the editors and the reviewers. Any product that may be evaluated in this article or claim that may be made by its manufacturer is not guaranteed or endorsed by the publisher.
Research integrity at Frontiers
Learn more about the work of our research integrity team to safeguard the quality of each article we publish.