- 1Laboratory of Microbiology, Institute of Biosciences, Federal University of Mato Grosso do Sul (UFMS), Campo Grande, Brazil
- 2Laboratory of Bioactive Natural Products Research (PRONABio), Institute of Chemistry, Federal University of Mato Grosso do Sul (UFMS), Campo Grande, Brazil
- 3Laboratory of Trypanosomatid Biochemistry, Oswaldo Cruz Foundation (FIOCRUZ), Rio de Janeiro, Brazil
Introduction: Leishmaniasis, a neglected tropical parasitic disease, is regarded as a major public health problem worldwide. The first-line drugs for leishmaniasis suffer from limitations related to toxicity and the development of resistance in certain parasitic strains. Therefore, the discovery of alternative treatments for leishmaniasis is imperative, and natural products represent a valuable source of potential therapeutic agents.
Methods: The present study aimed at finding new potential antileishmanial agents from the aerial parts of the medicinal plant Momordica charantia. This study was based on bioassay-guided fractionation of the M. charantia extract against promastigotes and amastigotes of Leishmania (Leishmania) amazonensis. The cytotoxicity of the extract, fractions, and isolated compounds were evaluated against peritoneal murine macrophages by employing the MTT assay for assessing cell metabolic activity.
Results: Antileishmanial assay-guided fractionation of the M. charantia extract led to the bioactive cucurbitacin-enriched fraction and the isolation of four bioactive cucurbitacin-type triterpenoids, which exhibited significant antileishmanial activity, with IC50 values between 2.11 and 3.25 μg.mL−1 against promastigote and amastigote forms, low toxicity and selectivity indexes ranging from 8.5 to 17.2.
Conclusion: Our findings demonstrate that the fractions and cucurbitacin-type triterpenoids obtained from the aerial parts of M. charantia are promising natural leishmanicidal candidates.
1 Introduction
Leishmaniasis, a neglected tropical parasitic disease, is considered a major public health problem worldwide. This ailment is caused by unicellular protozoan organisms of the Leishmania genus, which belong to the Trypanosomatidae family (Mishra et al., 2009). Leishmaniasis accounts for an estimated 700,000 to a million new cases worldwide each year. In 2020, over 90% of the new cases of visceral leishmaniasis and 85% of cutaneous leishmaniasis cases reported to the World Health Organization (WHO) originated from 10 countries (Medenica et al., 2023). In Brazil, Leishmania (Viannia) braziliensis and L. (Leishmania) amazonensis species are widely distributed. Among these, L. (Leishmania) amazonensis is known to cause a broad spectrum of clinical diseases, ranging from localized cutaneous leishmaniasis to visceral leishmaniasis and mucocutaneous forms (Anversa et al., 2018).
Pentavalent antimonials are the first-line drugs for the treatment of all types of leishmaniasis (Polonio and Efferth, 2008). However, despite the development of an array of chemotherapeutic agents, none of the drugs are entirely free from side effects; a number of these suffer limitations related to unaffordability, difficulty of administration, and toxicity. In addition, antileishmanial activity seems to be dependent on the evaluated Leishmania species (Barrett and Croft, 2012). The development of resistance in some parasitic strains is an additional concern (Padma, 2013). These limitations, combined with the absence of an effective vaccine, demonstrate the urgency for the development of new alternatives for the treatment of leishmaniasis (Sen and Chatterjee, 2011).
In recognition of the impact of leishmaniasis worldwide, WHO has proposed that the diffusion of the disease should be controlled and evaluation of the efficacy of new medicines should be promoted as one of the strategies of the control program (World Health Organization WHO, 2010). Plant extracts and plant-derived compounds have been used to treat leishmaniasis and represent potential sources of new medicinal agents and antileishmanial drugs (Newman and Cragg, 2020).
As part of our ongoing investigation on the use of Cerrado plants as a source of bioactive molecules, ethanol extracts from 14 species belonging to 12 different families have been analyzed for their activity against promastigote forms of L. amazonensis (Marques et al., 2013). It was determined that the crude ethanol extract obtained from the aerial parts of Momordica charantia L. (Cucurbitaceae) showed a promising activity profile, with an IC50 of 6.25 μg.mL−1.
Momordica charantia is used in traditional medicine as an antidiabetic, antihelmintic, and antimalarial, and to treat leprosy, skin diseases and scabies, and it has been described as a producer of biologically active phytochemicals such as triterpenoids, alkaloids, and steroids (Grover and Yadav, 2004). Several studies regarding the biological activities of M. charantia have detailed its use as an adjuvant for the treatment of diabetes to reduce glucose levels (Rathi et al., 2002), as well as oxidative stress (Sathishsekar and Subramanian, 2005), further, it has been used as an antiviral therapy for HIV infection (Cunnick et al., 1990), as a cytostatic agent in certain types of cancers (Ganguly et al., 2000), and as an antiprotozoal agent against Trypanosoma and Plasmodium spp. (Munoz et al., 2000; Kohler et al., 2002). Previous investigations on the leishmanicidal potential of M. charantia were performed with the ethanolic extract of the leaves, which exhibited IC50 values of 58.4 and 500 μg.mL−1 against promastigote forms of L. amazonensis (García et al., 2012) and L. brasiliensis (Santos et al., 2013), respectively. The ethanolic extract of the green fruits of M. charantia proved to be active against Leishmania donovani promastigotes, and further investigation led to the identification of momordicatin [4-(O-carboethoxyphenyl) butanol] as the active compound (Gupta et al., 2010).
In the present study, by using a bioassay-guided approach, we assessed the activity and toxicity of the extract, phases, and isolated compounds obtained from M. charantia, against extracellular promastigote and intracellular amastigote stages of L. (Leishmania) amazonensis.
2 Materials and methods
2.1 Plant extract preparation
Momordica charantia L. (Cucurbitaceae) samples were collected in Corumbá (19°34′37″S, 57°00′42″W), Mato Grosso do Sul, Brazil, in December 2007. The plant material was identified by Dr. Arnildo Pott and M. Sc. Ubirazilda Maria Rezende (CGMS Herbarium, Universidade Federal de Mato Grosso do Sul, Brazil), where a voucher specimen (No. 19894) is deposited. The research with this plant material was registered in the National System of Genetic Resource Management and Associated Traditional Knowledge (SisGen) at number A685E68. The aerial parts (leaves and stems) of M. charantia (150 g) were dried, triturated and extracted with 95% EtOH (×5, 750 mL) for 5 days at room temperature. The resulting extracts were combined and the solvent was removed under reduced pressure. The residue obtained (53.1 g) was stored at −18°C.
2.2 Antileishmanial bioassay-guided isolation of cucurbitacins
The ethanol extract of M. charantia (EEMc), which exhibited antileishmanial activity (IC50 = 6.25 μg.mL−1), was re-suspended in MeOH-H2O (8:2) and successively partitioned with hexanes and EtOAc (Supplementary Figure S1). The resulting phases were tested for antileishmanial activity, wherein the EtOAc phase was found to possess a superior activity profile (IC50 = 6.17 μg.mL−1). The EtOAc phase was chromatographed on a silica gel 70–230 mesh column, using step gradient elution with hexanes-EtOAc to yield 14 fractions (F1−F14). Fraction F7 showed the highest antileishmanial activity (IC50 = 3.02 μg.mL−1), and an aliquot of this fraction (0.50 g) was further chromatographed on a Sephadex LH-20 column eluted with CHCl3, to give 11 subfractions (A1−A11), of which A1 (44.8 mg), A3 (92.3 mg), and A5 (17.6 mg) exhibited antileishmanial activity. Fraction A1 was re-chromatographed on a silica gel 200–400 mesh column [hexanes-EtOAc (6:4)] to afford 11 subfractions (A1.1-A1.11), of which A1.3 yielded 25-methoxy-3,7-dihydroxycucurbita-5,23(E)-dien-19-al (1, 10.1 mg). 3,7,25-trihydroxycucurbita-5,23(E)-dien-19-al (2, 90.0 mg), was isolated from subfraction A3, after reversed-phase semi-preparative HPLC (CH3CN-H2O, 50→100%). Fraction A5 was re-chromatographed on a silica gel 200–400 mesh column [hexanes-EtOAc-MeOH (6:4:0.5)] to afford nine subfractions (A5.1-A5.9). 19(R),25-dimethoxy-5-19-epoxycucurbita-6,23(E)-dien-3-ol (3, 2.1 mg), was obtained from subfraction A5.2, while 19(R)-methoxy-5-19-epoxycucurbita-6,23(E)-dien-3,25-diol (4, 1.7 mg) was isolated from subfraction A5.3, after preparative TLC on silica gel PF254, solvent system hexanes-EtOAc-MeOH (6:2:0.5). Compounds 1–4 were identified by 1D and 2D NMR spectroscopy recorded at 300 MHz for 1H and 75 MHz for 13C NMR, on a Bruker DPX-300 spectrometer, and by comparison with literature data.
2.3 HPLC analysis
HPLC analysis was performed on a Shimadzu system (Shimadzu Corporation, Kyoto, Japan) equipped with LC-6AD pumps, a SIL-20A autosampler, SPDV-6AV UV/VIS detector, CBM-20A communications module and LC Solution 1.24 SP2 software to record and process the data. Acetonitrile (CH3CN) was HPLC grade (Merck, Darmstadt, Germany), and H2O was purified using a Milli-Q system (Millipore, Bedford, MA, United States). The analyses were performed using a Phenomenex Luna® C18 column (5 μm, 100 Å, LC column 4.6 × 250 mm). Cucurbitacins 1–4 were eluted with an isocratic mobile phase system consisting of CH3CN-H2O (70:30) and detected at 210 nm. The extracts were completely dissolved in CH3CN, filtered (0.22 µm pore size, Merck), and analyzed at room temperature (25°C), at a flow rate of 1 mL.min−1 and an injection volume of 10 µL. Peaks were identified by comparison of their retention times (tR) (1 = 7.12 min; 2 = 9.42 min; 3 + 4 = 19.29 min) and co-injection with the isolated standards (Supplementary Figure S10). The identities and purities of the compounds were confirmed by NMR spectroscopy.
2.4 L. (Leishmania) amazonensis culture
The L. (L.) amazonensis strain MHOM/BR/77/LTB0016 was kept at 26°C in Schneider’s insect medium (Sigma-Aldrich, St. Louis, United States), at pH 6.9, and supplemented with 10% heat-inactivated fetal calf serum, 100 mg.mL−1 of streptomycin, and 100 IU/mL of penicillin. Parasites were maintained in culture until the 10th passage and new parasites were routinely isolated from infected lesions of BALB/c mice.
2.5 Determination of leishmanicidal activity in vitro
2.5.1 Antipromastigote activity
The effects of the crude extract, fractions, and isolated compounds on the viability of the extracellular forms of L. amazonensis were determined using the thiazolyl blue tetrazolium bromide (MTT) assay (Sigma-Aldrich, St. Louis, United States). A stock solution of testing materials and pentamidine was prepared in DMSO (Sigma Aldrich). The maximum solvent concentration used in the assay was 0.5% in a final volume per well of 200 µL. Untreated infected cells and pentamidine isethionate (3.125–200 μM.mL−1) were used as a negative and positive control, respectively. The logarithmic phase of growth was maintained, and the final concentration of parasites was adjusted to 1 × 106 promastigotes.mL−1. The cells were transferred to 96-well plates, incubated for 72 h, and kept at 27°C with the testing material (extract and fractions: 1.56–50 μg.mL−1; pure compounds: 3.125–200 μM.mL−1). Following incubation, 22 µL of MTT solution (5 μg.mL−1) was added to each well, and samples were incubated for 2 h, followed by the addition of 80 µL of DMSO. The optical density was measured at 570 nm on a µQuant system (Bio-Tek Instruments, Winooski, United States). The results were expressed as the mean ± standard deviation (SD) of the IC50 of three independent experiments done in triplicate. Compounds with IC50 ≤ 50 μg.mL−1 were considered active.
2.5.2 Anti-amastigote activity
For the evaluation of activity against intracellular amastigotes, murine peritoneal macrophage cells were obtained by peritoneal washing with 5 mL of iced Roswell Park Memorial Institute–1640 (RPMI-1640) medium (Sigma). The peritoneal fluid was adjusted to a concentration of 2 × 106 macrophages. mL−1, plated (0.4 mL/well) in Lab-Tek 8-chambers slides (Nunc, Roskilde, Denmark) and incubated for 1 h at 37°C and 5% CO2. The cultures were washed with phosphate-buffered saline (PBS) at 37°C to remove the non-adherent cells. The adhered cells were incubated with L. amazonensis promastigotes in the ratio of 3:1 (0.4 mL/well) at 37°C and 5% CO2. After 4 h, the chambers were washed again to remove free parasites. For the assay against amastigote forms, monolayers of the cells containing the intracellular parasites were incubated with the test compounds and the controls at a range of concentrations (1.5625–50 μg.mL−1) for 72 h at 37°C and 5% CO2. After the incubation period, the cells were washed with phosphate buffer saline (0.02 M, pH 7.2), fixed with methanol and stained with Giemsa, to count live amastigotes in macrophage by bright-field microscopy. The anti-amastigote activities of the compounds were determined microscopically by counting the number of amastigotes and examining at least 100 infected macrophages per experiment. The results were expressed as an infection index (% infected macrophage×number of amastigote/total number of macrophages) and IC50 values were calculated by plotting the infection index in amastigotes against drug concentrations tested by logarithmic nonlinear regression analysis, using GraphPad Prism software version 5.0. Due to limited quantities of 3 and 4, their anti-amastigote activity and cytotoxicity against mammalian cells could not be evaluated, and accordingly, their selectivity indexes. The results were expressed as the mean ± standard deviation (SD) of the IC50 of three independent experiments done in triplicate.
2.6 In vitro cytotoxic concentration (CC50) against mammalian cells
The cytotoxicity of the compounds was determined using murine macrophages (2 × 106 in 200 µL) of BALB/c mice incubated with various compound concentrations for 72 at 37°C and 5% CO2. The effect on macrophage viability was assessed using the MTT assay. The concentration of extracts/compounds that caused 50% macrophage cytotoxicity (CC50) was determined by linear regression analysis using GraphPad Prism software, version 5.0. The selectivity index (SI) of the extracts/compounds was determined using the following equation: SI = CC50 against mammalian cells/IC50 against L. amazonensis amastigotes.
2.7 Statistical analyses
The in vitro antileishmanial activity and cytotoxicity were expressed as the IC50 and CC50, respectively, by a non-linear regression curve calculated using the statistical GraphPad Prism software version 5.0, with a confidence interval of 95%.
3 Results
3.1 Isolation and characterization of cucurbitacins
A bioassay-guided fractionation of the bioactive fraction F7 led to the isolation and characterization of four cucurbitacins: 25-methoxy-3,7-dihydroxycucurbita-5,23(E)-dien-19-al (1), 3,7,25-trihydroxycucurbita-5,23(E)-dien-19-al (2), 19(R),25-dimethoxy-5-19-epoxycucurbita-6,23(E)-dien-3-ol (3), and 19(R)-methoxy-5-19-epoxycucurbita-6,23(E)-dien-3,25-diol (4) (Figure 1). Their structures were established based on NMR experiments (Supplementary Table S1). Tetracyclic triterpenes with cucurbitane-type rearranged skeletons, such as compounds 1–4, have been previously isolated from the leaves and fruits of M. charantia collected in Japan and Nigeria. The NMR data obtained for these compounds in our study were consistent with those reported in the literature (Fatope et al., 1990; Kimura et al., 2005). Additionally, cucurbitacins 1–4 have been identified in the leaves of Momordica foetida collected in South Africa (Mulholland et al., 1997).
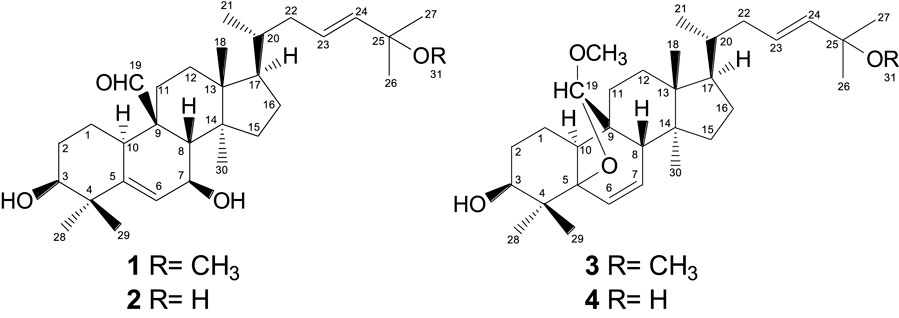
Figure 1. Structures of the isolated compounds obtained from the ethanol extract of aerial parts of Momordica charantia.
3.2 HPLC profiles
The HPLC profiles of the ethanol extract and fraction F7 were similar, demonstrating that both contained a mixture of cucurbitacins 1–4 as the major components (Figure 2).
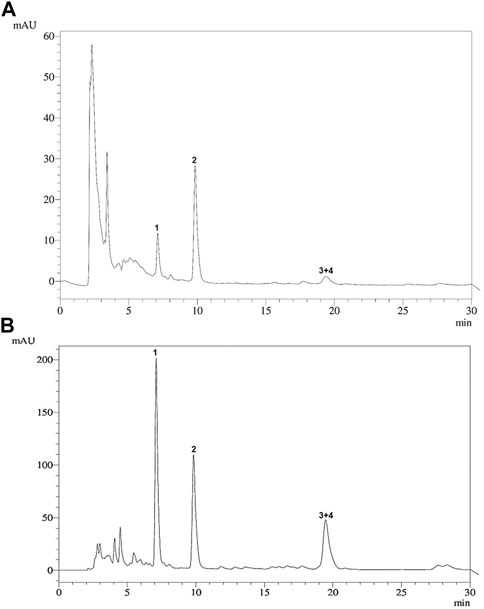
Figure 2. HPLC profile of (A) the ethanol extract of the aerial parts and (B) cucurbitacin-enriched fraction F7 of Momordica charantia. 1: 25-methoxy-3, 7-dihydroxycucurbita-5,23(E)-dien-19-al (7.12 min); 2: 3,7,25-trihydroxycucurbita-5,23(E)-dien-19-al (9.42 min); 3 + 4: 19(R),25-dimethoxy-5-19-epoxycucurbita-6,23(E)-dien-3-ol and 19(R)-methoxy-5-19-epoxycucurbita-6,23(E)-dien-3,25-diol (19.29 min).
3.3 Antileishmanial activities
The antileishmanial activities of the extracts, fractions and cucurbitacins obtained from the aerial parts of M. charantia against promastigotes and amastigotes of L. (L.) amazonensis are reported in Table 1. The effects of the M. charantia ethanol extract and fractions on the promastigote forms of L. (L.) amazonensis were monitored for 72 h, and it was established that the ethanol extract and fraction F7 showed activity against the promastigote form of L. amazonensis, with IC50 values of 6.25 and 3.02 μg.mL−1, respectively. The isolated cucurbitacins 1–4 showed an activity profile against promastigotes with IC50 values ranging from 4.68 to 5.21 μg.mL−1 (Table 1).
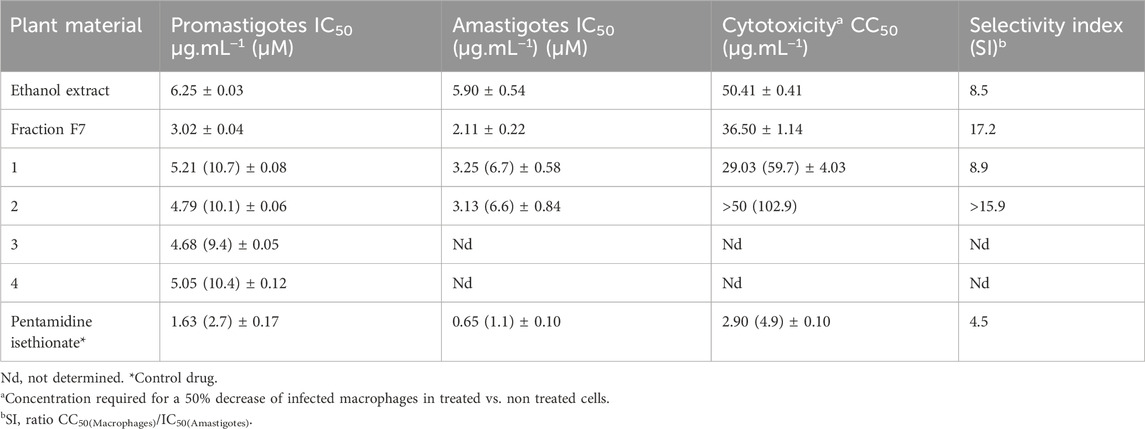
Table 1. Antileishmanial activity of ethanol extract, fraction, and isolated compounds from the aerial parts of Momordica charantia against Leishmania (L.) amazonensis.
4 Discussion
Promising results were obtained against amastigote forms of L. amazonensis, whereby fraction F7 exhibited the highest activity with an IC50 value of 2.11 μg.mL−1, followed by 1 and 2, with IC50 values of 3.25 and 3.13 μg.mL−1, respectively. The ethanol extract was likewise active against amastigotes, exhibiting an IC50 of 5.9 μg.mL−1.
To date, a large number of cucurbitacins and cucurbitacin-derived compounds have been isolated from the Cucurbitaceae species. The structures of cucurbitacins 1–4 isolated from M. charantia have the same degree of oxygenation, a similar side chain at C-17 bearing hydroxyl or methoxy substituents, and oxygenated C-19 bearing aldehyde or acetal functional groups, as well as an oxygenated C-3 position. It has been suggested that these structural characteristics are important for antipromastigote activity of triterpenes (Filho et al., 2009).
The skeleton of the cucurbitacin-type tetracyclic triterpenes exhibits lipophilic behavior, particularly in free form, as is the case for 1–4. The lipophilic characteristics of these compounds may promote the efficiency of parasite membrane transportation, increasing antiprotozoan activity (Basselin and Robert-Gero, 1998; Cargnin et al., 2013).
Interestingly, fraction F7 presented the highest antileishmanial activity, exceeding that of the isolated compounds 1–4. The four cucurbitacins were found to be present as the major metabolites of fraction F7, as demonstrated by HPLC analysis (Figure 2). A similar aspect was observed for the cucurbitane-type triterpenoid-enriched fraction obtained from Trichosanthes dioica, which showed an inhibitory effect on the in vitro growth of L. donovani promastigotes (Bhattacharya et al., 2013). Although cucurbitacins have previously undergone evaluation for various biological activities (Hill and Connolly, 2017), studies supporting the antileishmanial activity of this class of secondary metabolites remain scarce.
When evaluated for their selective toxicity on mammalian macrophage cells, no significant cytotoxic effects on the viability of macrophages were observed for the ethanol extract, fraction F7, and 1 and 2. Their CC50/IC50 ratios ranged from 8.5 to 17.2, indicating good selectivity indexes, which were higher than that obtained for the control pentamidine isethionate, one of the primary drugs employed against leishmaniasis (Table 1). According to Katsuno et al. (2015), for a compound to be considered with low cytotoxicity, the selectivity index (SI) must be ≥ 10.
5 Conclusion
By using a bioassay-guided fractionation approach, the extract, fractions, and four cucurbitacin-type triterpenoids, obtained from the aerial parts of the medicinal plant M. charantia, were revealed to exhibit significant leishmanicidal activity against L. amazonensis. The fraction enriched with cucurbitacin-type triterpenoids presented a superior activity profile to those of the isolated compounds. In addition, the tested cucurbitacins 1 and 2, and the active fraction proved to be noncytotoxic to mammalian cells, thereby displaying good selectivity indexes. Further studies are being conducted to investigate the medicinal applicability of the cucurbitacins and the cucurbitacin-enriched fraction obtained from M. charantia as potential antileishmanial candidates and/or as an adjuvant for the treatment and control of leishmaniasis.
Data availability statement
The original contributions presented in the study are included in the article/Supplementary Material, further inquiries can be directed to the corresponding author.
Ethics statement
The animal study was approved by the Ethics Committtee of Fiocruz—CEUA/Fiocruz. The study was conducted in accordance with the local legislation and institutional requirements.
Author contributions
MM: Conceptualization, Formal Analysis, Investigation, Methodology, Resources, Validation, Writing–original draft, Writing–review and editing. NY: Conceptualization, Supervision, Visualization, Writing–original draft, Writing–review and editing. ET: Data curation, Methodology, Validation, Writing–original draft. FG: Funding acquisition, Project administration, Writing–original draft, Writing–review and editing. WG: Funding acquisition, Project administration, Validation, Writing–original draft.
Funding
The author(s) declare that financial support was received for the research, authorship, and/or publication of this article. This work was supported by Conselho Nacional de Desenvolvimento Científico e Tecnológico (CNPq) and Fundação de Apoio ao Desenvolvimento do Ensino, Ciência e Tecnologia de Mato Grosso do Sul (FUNDECT-MS, 020793) grants. This study was financed in part by the Coordenação de Aperfeiçoamento de Pessoal de Nível Superior—Brasil (CAPES)—Finance Code 001, and CPq-PROPP UFMS.
Acknowledgments
WG, MM, NY, ET, and FG are grateful to CNPq and FUNDECT-MS for the awarded research grant. Thanks are also due to Prof. Arnildo Pott, Vali J. Pott, and Ubirazilda M. Resende (INBIO-UFMS) for their assistance in the collection and identification of the plant material. And we thank Mariela Ferreira de Vasconcelos for her support in the investigations.
Conflict of interest
The authors declare that the research was conducted in the absence of any commercial or financial relationships that could be construed as a potential conflict of interest.
Publisher’s note
All claims expressed in this article are solely those of the authors and do not necessarily represent those of their affiliated organizations, or those of the publisher, the editors and the reviewers. Any product that may be evaluated in this article, or claim that may be made by its manufacturer, is not guaranteed or endorsed by the publisher.
Supplementary material
The Supplementary Material for this article can be found online at: https://www.frontiersin.org/articles/10.3389/fphar.2024.1390715/full#supplementary-material
References
Anversa, L., Tiburcio, M. G. S., Richini-Pereira, V. B., and Ramirez, L. E. (2018). Human leishmaniasis in Brazil: a general review. Rev. Assoc. Méd. Bras. 64 (3), 281–289. doi:10.1590/1806-9282.64.03.281
Barrett, M. P., and Croft, S. L. (2012). Management of trypanosomiasis and leishmaniasis. Br. Med. Bull. 104 (1), 175–196. doi:10.1093/bmb/lds031
Basselin, M., and Robert-Gero, M. (1998). Alterations in membrane fluidity, lipid metabolism, mitochondrial activity, and lipophosphoglycan expression in pentamidine-resistant Leishmania. Parasitol. Res. 84, 78–83. doi:10.1007/s004360050361
Bhattacharya, S., Biswas, M., and Haldar, P. K. (2013). The triterpenoid fraction from Trichosanthes dioica root exhibits in vitro antileishmanial effect against Leishmania donovani promastigotes. Pharmacogn. Res. 5 (2), 109–112. doi:10.4103/0974-8490.110540
Cargnin, S. T., Vieira, P. deB., Cibulski, S., Cassel, E., Vargas, R. M. F., Montanha, J., et al. (2013). Anti-Trichomonas vaginalis activity of Hypericum polyanthemum extract obtained by supercritical fluid extraction and isolated compounds. Parasitol. Int. 62 (2), 112–117. doi:10.1016/j.parint.2012.10.006
Cunnick, J. E., Sakamoto, K., Chapes, S. K., Fortner, G. W., and Takemoto, D. J. (1990). Induction of tumor cytotoxic immune cells using a protein from the bitter-melon (Momordica charantia). Cell. Immunol. 126, 278–289. doi:10.1016/0008-8749(90)90321-H
Fatope, M. O., Takeda, Y., Yamashita, H., Okabe, H., and Yamauchi, T. (1990). New cucurbitane triterpenoids from Momordica charantia. J. Nat. Prod. 53 (6), 1491–1497. doi:10.1021/np50072a014
Filho, A. A. S., Resende, D. O., Fukui, M. J., Santos, F. F., Pauletti, P. M., Cunha, W. R., et al. (2009). In vitro antileishmanial, antiplasmodial and cytotoxic activities of phenolics and triterpenoids from Baccharis dracunculifolia D. C. (Asteraceae). Fitoterapia 80 (8), 478–482. doi:10.1016/j.fitote.2009.06.007
Ganguly, C., De, S., and Das, S. (2000). Prevention of carcinogen-induced mouse skin papilloma by whole fruit aqueous extract of Momordica charantia. Eu. J. Cancer Prev. 9 (4), 283–288. doi:10.1097/00008469-200008000-00009
García, M., Monzote, L., Scull, R., and Herrera, P. (2012). Activity of Cuban plants extracts against Leishmania amazonensis. ISRN Pharmacol. 2012, 104540. Article ID 104540. doi:10.5402/2012/104540
Grover, J. K., and Yadav, S. P. (2004). Pharmacological actions and potential uses of Momordica charantia: a review. J. Ethnopharmacol. 93 (1), 123–132. doi:10.1016/j.jep.2004.03.035
Gupta, S., Raychaudhuri, B., Banerjee, S., Das, B., Mukhopadhaya, S., and Datta, S. C. (2010). Momordicatin purified from fruits of Momordica charantia is effective to act as a potent antileishmania agent. Parasitol. Int. 59 (2), 192–197. doi:10.1016/j.parint.2010.01.004
Hill, R. A., and Connolly, J. D. (2017). Triterpenoids. Nat. Prod. Rep. 34, 90–122. doi:10.1039/c6np00094k
Katsuno, K., Burrows, J. N., Duncan, K., Hooft van Huijsduijnen, R., Kaneko, T., Kita, K., et al. (2015). Hit and lead criteria in drug discovery for infectious diseases of the developing world. Nat. Rev. Drug Discov. 14 (11), 751–758. doi:10.1038/nrd4683
Kimura, Y., Akihisa, T., Yuasa, N., Ukiya, M., Suzuki, T., Toriyama, M., et al. (2005). Cucurbitane-type triterpenoids from the fruit of Momordica charantia. J. Nat. Prod. 68 (5), 807–809. doi:10.1021/np040218p
Kohler, I., Jenett-Siems, K., Siems, K., Hernandez, M. A., Ibarra, R. A., Berendsohn, W. G., et al. (2002). In vitro antiplasmodial investigation of medicinal plants from El Salvador. Z Naturforsch C J. Biosci. 57 (3-4), 277–281. doi:10.1515/znc-2002-3-413
Marques, M. C. S., Hamerski, L., Garcez, F. R., Tieppo, C., Vasconcelos, M., Torres-Santos, E. C., et al. (2013). In vitro biological, screening and evaluation of free radical scavenging activities of medicinal plants from the Brazilian Cerrado. J. Med. Plants Res. 7 (15), 957–962. doi:10.5897/JMPR12.882
Medenica, S., Miladinović-Tasić, N., Stojanović, N. M., Lakićević, N., and Rakočević, B. (2023). Climate variables related to the incidence of human leishmaniosis in Montenegro in southeastern europe during seven decades (1945-2014). Int. J. Environ. Res. Public Health 20 (3), 1656. doi:10.3390/ijerph20031656
Mishra, B. B., Kale, R. R., Singh, R. K., and Tiwari, V. K. (2009). Alkaloids: future prospective to combat leishmaniasis. Fitoterapia 80 (2), 81–90. doi:10.1016/j.fitote.2008.10.009
Mulholland, D. A., Sewram, V., Osborne, R., Pegel, K. H., and Connolly, J. D. (1997). Cucurbitane triterpenoids from the leaves of Momordica foetida. Phytochemistry 45 (2), 391–395. doi:10.1016/s0031-9422(96)00814-x
Munoz, V., Sauvain, M., Bourdy, G., Callapa, J., Rojas, I., Vargas, L., et al. (2000). The search for natural bioactive compounds through a multidisciplinary approach in Bolivia. Part II. Antimalarial activity of some plants used by Mosetene Indians. J. Ethnopharmacol. 69 (2), 139–155. doi:10.1016/S0378-8741(99)00096-3
Newman, D. J., and Cragg, G. M. (2020). Natural products as sources of new drugs over the nearly four decades from 01/1981 to 09/2019. J. Nat. Prod. 83 (3), 770–803. doi:10.1021/acs.jnatprod.9b01285
Padma, T. V. (2013). Leishmaniasis drug fails a fifth of patients: high failure rate of front-line therapy puts eradication campaign at risk. Nat. News. doi:10.1038/nature.2013.12553
Polonio, T., and Efferth, T. (2008). Leishmaniasis: drug resistance and natural products (Review). Int. J. Mol. Med. 22 (3), 277–286. doi:10.3892/ijmm_00000020
Rathi, S. S., Grover, J. K., and Vats, V. (2002). The effect of Momordica charantia and Mucuna pruriens in experimental diabetes and their effect on key metabolic enzymes involved in carbohydrate metabolism. Phytother. Res. 16 (3), 236–243. doi:10.1002/ptr.842
Santos, K. K. A., Rolón, M., Veja, C., Arias, A. R., Costa, J. G. M., and Coutinho, H. D. M. (2013). Atividade leishmanicida in vitro de Eugenia uniflora e Momordica charantia. Rev. Ciências Farm. Básica Apl. 34 (1), 47–50.
Sathishsekar, D., and Subramanian, S. (2005). Antioxidant properties of Momordica charantia (bitter gourd) seeds on Streptozotocin induced diabetic rats. Asia Pac. J. Clin. Nutr. 14 (2), 153–158.
Sen, R., and Chatterjee, M. (2011). Plant-derived therapeutics for the treatment of Leishmaniasis. Phytomedicine 18 (12), 1056–1069. doi:10.1016/j.phymed.2011.03.004
World Health Organization (WHO) (2010). Report of a meeting of the WHO Expert Committee on the control of leishmaniasis. Available at: http://whqlibdoc.who.int/trs/WHO_TRS_949_eng.pdf (Accessed April 18, 2016).
Keywords: Momordica charantia, Cucurbitaceae, leishmanicidal activity, Leishmania amazonensis, neglected tropical disease, cucurbitacin
Citation: Marques MCS, Yoshida NC, Torres-Santos EC, Garcez FR and Garcez WS (2024) Bioassay-guided isolation of leishmanicidal cucurbitacins from Momordica charantia. Front. Pharmacol. 15:1390715. doi: 10.3389/fphar.2024.1390715
Received: 23 February 2024; Accepted: 15 May 2024;
Published: 11 July 2024.
Edited by:
Germain Sotoing Taiwe, University of Buea, CameroonReviewed by:
Sergio R. Peraza-Sanchez, Scientific Research Center of Yucatán (CICY), MexicoEsther Del Olmo, University of Salamanca, Spain
Copyright © 2024 Marques, Yoshida, Torres-Santos, Garcez and Garcez. This is an open-access article distributed under the terms of the Creative Commons Attribution License (CC BY). The use, distribution or reproduction in other forums is permitted, provided the original author(s) and the copyright owner(s) are credited and that the original publication in this journal is cited, in accordance with accepted academic practice. No use, distribution or reproduction is permitted which does not comply with these terms.
*Correspondence: Maria Carolina Silva Marques, bWFyaWEubWFycXVlc0B1Zm1zLmJy
†These authors have contributed equally to this work and share first authorship
‡These authors share senior authorship