- 1The Second Clinical Hospital of Beijing University of Chinese Medicine, Beijing, China
- 2Dongfang Hospital of Beijing University of Chinese Medicine, Beijing, China
Lung cancer has high metastasis and drug resistance. The prognosis of lung cancer patients is poor and the patients’ survival chances are easily neglected. Ferroptosis is a programmed cell death proposed in 2012, which differs from apoptosis, necrosis and autophagy. Ferroptosis is a novel type of regulated cell death which is driven by iron-dependent lipid peroxidation and subsequent plasma membrane ruptures. It has broad prospects in the field of tumor disease treatment. At present, multiple studies have shown that biological compounds can induce ferroptosis in lung cancer cells, which exhibits significant anti-cancer effects, and they have the advantages in high safety, minimal side effects, and less possibility to drug resistance. In this review, we summarize the biological compounds used for the treatment of lung cancer by focusing on ferroptosis and its mechanism. In addition, we systematically review the current research status of combining nanotechnology with biological compounds for tumor treatment, shed new light for targeting ferroptosis pathways and applying biological compounds-based therapies.
1 Introduction
Lung cancer is a prevalent malignant neoplasm affecting the respiratory system. In 2022, lung cancer is estimated to account for 13% of new cancer diagnoses in the United States and 22% of all cancer-caused deaths. Although the mortality rate of lung cancer continues to decline with the decrease in the number of smokers and the early rational diagnosis and treatment, lung cancer is still the highest fatality rate of cancer. Due to the fact that smokers are more common among males, the number of deaths of lung cancer in males is higher than in females (Siegel et al., 2023). Therefore, improving the clinical efficacy and prognosis of lung cancer treatment, seeking more effective intervention targets and mechanisms, and exploring more advantageous treatment plans are urgent issues that need to be addressed.
Ferroptosis was first proposed by Dixon et al. in Cell Magazine in 2012. It is a regulated form of cell death that differs from other programmed deaths like morphology, biochemistry and regulatory mechanism (Dixon et al., 2012). Ferroptosis is based on divalent iron, characterized by the accumulation of iron-dependent polyunsaturated fatty acid peroxides, leading to membrane damage and cell lysis (Riegman et al., 2020; Du et al., 2023). The occurrence of ferroptosis is the result of various metabolic imbalances, and the disruption of metabolic homeostasis is related to tumors, inflammation, nerve damage, ischemic organ damage, etc. (Liang et al., 2022; Li et al., 2023; Ye et al., 2023). Ferroptosis regulators as promising biomarkers and therapeutic targets associated with tumor immune infiltration are shown to have accurate diagnostic and prognostic predictive performance with superior specificity and sensitivity (Tang et al., 2021a). Therefore, in-depth researches on ferroptosis have broad prospects in the field of tumor disease treatment.
Anti-cancer drugs mostly work by inducing tumor cell apoptosis, but long-term and high-dose use may lead to resistance to drugs that induce apoptosis, resulting in poor therapeutic effects. Previous studies have shown that ferroptosis is closely related to the occurrence of tumors. Due to the high metabolic levels, cancer cells require more iron during production process compared with normal cells (Hassannia et al., 2019). Also, the imbalance in iron metabolism is able to increase the risk of cancer and promote tumor growth, making them more sensitive to ferroptosis. As a result, ferroptosis is considered as an effective mean of treating with tumors (Tsoi et al., 2018), providing new directions for the development of anti-cancer drugs. It has been continuous reports that biological compounds play a potential anti-tumor role in regulating ferroptosis, which has the advantages in high safety, small side effects, and less susceptibility to drug resistance (Yan et al., 2021). This review will further explore the therapeutic targets and pathways of ferroptosis, analyze the mechanism of ferroptosis, and summarize the existing biological compounds targeting ferroptosis in order to provide theoretical basis for further research on ferroptosis.
2 The mechanism of ferroptosis
Ferroptosis is controlled and executed by a comprehensive and precise signal network, including genetic, transcriptional, post-transcriptional, and post-translational level (Tang et al., 2021b). Ferroptosis is a programmed cell death distinct from apoptosis, necrosis and autophagy, which is the result of various metabolic imbalances. In the case of metabolic disorders, iron, lipids, and reactive oxygen species (ROS) cannot maintain normal cell function. Iron affects lipid peroxidation during ferroptosis by producing ROS and activating iron-containing enzymes (such as arachidonic acid lipoxygenase (ALOXs)). The mass production of lipid ROS promotes cell membrane damage and destruction, leading to cell death (Zhou et al., 2020; Zheng et al., 2023). Ferroptosis has two characteristics: firstly, morphological features, include cell membrane rupture, mitochondrial shrinkage, mitochondrial ridge reduction or even disappearance, increased mitochondrial membrane density, outer membrane rupture, and normal nuclear morphology, but lacking chromatin agglutination; secondly, biological characteristics, include plenty of ROS production, aggregation of ferrous ions, activation of mitogen-activated protein kinase system (MAPK), glutathione (GSH) reduction, and cysteine-glutamate antiporter system (System Xc-), etc (Jiang et al., 2021a); (Figure 1).
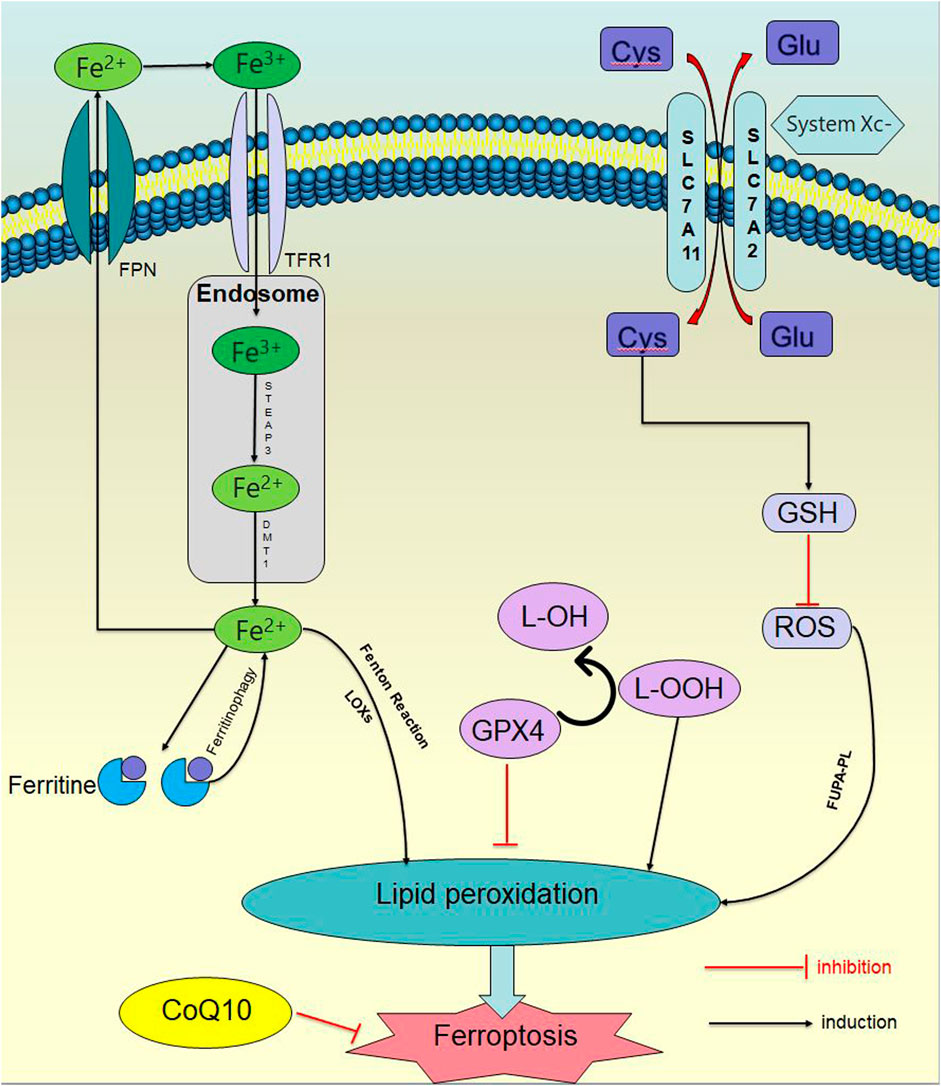
Figure 1. Ferroptosis signaling pathway. In the case of metabolic disorders, iron, lipids, and ROS) cannot maintain normal cellular function. Iron affects lipid peroxidation during ferroptosis by producing ROS and activating iron containing enzymes. The excessive production of lipid ROS promotes damage and destruction of cell membranes, leading to cell death.
2.1 Iron metabolism disorder
Under physiological conditions, extracellular iron exists in the form of trivalent iron (Fe3+), binds to transferrin receptor 1 (TFR1) and enters the cell. After entering the nucleus, it is immediately reduced to divalent iron ions (Fe2+) under the catalysis of iron reductase prostate six transmembrane epithelial antigen 3 (STEAP3). Afterwards, Fe2+ is transported to the cytoplasm through the endosomal membrane under the action of divalent metal transporter 1 (DMT1), and form an unstable iron pool. The excess iron is transported to the extracellular space through the iron transporter protein, or stored in ferritin and reacted with intracellular H2O2 to generate highly active hydroxyl radicals (•OH) under slightly acidic conditions, which is known as the Fenton reaction (Fe2+ + H2O2→Fe3+ + OH− +·OH). •OH then reacts with intracellular liposomes to generate lipid peroxidation (LPO) (Sutton and Winterbourn, 1989; Liu et al., 2018a). At the same time, it has been reported that ferritin-bound iron can be mobilized through selective autophagy to degrade ferritin (which is known as ferritinophagy). In other words, when intracellular iron is deficient, the ferritinophagy receptor NCOA4 (nuclear receptor coactivator 4) is stabilized, interacting with ferritin and delivering ferritin to the autophagosomes. NCOA4 is engulfed by lysosomal degradation and releases iron, then the sensitivity to ferroptosis is increased (Shan et al., 2023; Wu et al., 2023).
2.2 Abnormal antioxidant mechanism
Multiple antioxidants are present in the cell. GSH is the main intracellular antioxidant, and it is also a cofactor for the normal function of selenase glutathione peroxidase 4 (GPX4). The System Xc-on the cell membrane is an important part of keeping the homeostasis of the cellular antioxidant system, consisting of two parts: a light chain (xCT, transcribed by SLC7A11) and a heavy chain (4F2, transcribed by SLC3A2) (Xu et al., 2023a), which can exchange intracellular glutamate with extracellular cysteine (Parker et al., 2021). After cystine enters the cell, it is reduced to cysteine by nicotinamide adenine dinucleotide phosphate (NADPH). Due to the limited concentration of cysteine in cells, cysteine is considered as a rate-limiting precursor for GSH synthesis, which further synthesizes GSH with glycine and glutamate acid to reduce intracellular ROS.
When System Xc - is inhibited, decrease in intracellular GSH synthesis leads to a significant accumulation of ROS, causing an imbalance in the cellular antioxidant system. When ROS accumulates in large quantities, it reacts directly with polyunsaturated fatty acid phospholipids (PUFA-PL) on the cell membrane to form lipid hydroperoxides, which in turn forms LPO and initiates ferroptosis. In addition, lipid hydroperoxides can also be directly catalyzed by the combined action of lipoxygenases (LOXs) and Fe2+.
Besides, GPX4 is mainly involved in the redox reaction of ferroptosis (Ingold et al., 2018). GPX4 is selenium protein, which is the only enzyme that directly reduces lipid hydroperoxides in biofilms, catalyzing the reduction of lipid hydroperoxides, converting toxic lipid hydroperoxides (L-OOH) to non-toxic lipid alcohols (L-OH) in the lipid bilayer (Ursini et al., 1982), reducing LPO and maintaining cellular redox homeostasia. Once the production of the (e)ine/GSH/GPX4 axis is inhibited, lethal amounts of LPO will accumulate, resulting in disruptions such as cell membrane rupture, cell swelling, mitochondrial rupture and leading to ferroptosis (Li and Li, 2020; Shan et al., 2023). The expression of GPX4 is regulated by GSH and selenium, whose catalytic requirement is fulfilled by replacing cysteine and two electrons with selenium supplied by GSH, and GPX4 is inactivated while GSH is consuming.
The pathway of ferroptosis is influenced by various enzymes as well. Lipoxygenases (LOXs), non-heme iron-dependent dioxygenases, can selectively act on polyunsaturated fatty acids (PUFAs) and lipid-containing PUFAs in oxidative biofilms, thereby increasing the likelihood of ferroptosis. LOXs mainly induce ferroptosis through cysteine deprivation, rather than depletion of GPX4. Cytochrome p450 oxidoreductase (POR) affects iron metabolism by removing hydrogen from polyunsaturated fatty acids, or by reducing Fe3+ to Fe2+ for electron transfer, to accelerate the circulation between Fe3+ and Fe2+ to promote ferroptosis (Zou et al., 2021). Lipoxygenase isoenzymes and p450 oxidoreductase can catalyze PUFA-PL to produce PLOOH and induce ferroptosis (Ni and Li, 2024).
Another study has found that nuclear factor erythroid 2-related factor (Nrf2) is an important antioxidant transcription factor, which plays a key role in oxidative defense by regulating the expression of various antioxidant factors. At the same time, Nrf2 is also a crucial regulatory factor for maintaining oxidative homeostasis in oxidative damage induced by the ROS production factor, NADPH oxidase 4 (NOX4). Nrf2 is a negative regulator of ferroptosis as well, which can regulate the expression of several key genes involved in iron concentration by activating the GSH transport system, improving ferritin autophagy, regulating mitochondrial activity and lipid peroxidation (Hou et al., 2024).
2.3 Peroxidation of membrane phospholipids
Unrestricted peroxidation of phospholipids is another vital mechanism that promotes ferroptosis. Lipid metabolomics has shown that polyunsaturated fatty acids such as arachidonic acid (AA) or adrenergic acid (AdA) is the most easily oxidized lipids during ferroptosis and are regulated by three synthases. Acyl-CoA synthase long-chain family member 4 (ACSL4) catalyzes the conversion of AA or AdA to AA-CoA and AdA-CoA. Subsequently, lysine phosphatidylcholine acyltransferase 3 (LPCAT3) esterifies it to phosphatidylethanolamine (PE) to form AA-PE and AdA-PE, and finally oxidized to PE-AA-OOH and PE-AdA-OOH through polyunsaturated fatty acid lipoxygenase 15 (ALOX15) (Jiang et al., 2024).
The sensitivity of cells to ferroptosis is mainly determined by the unsaturation exhibited by the phospholipid bilayer. Biological cell membranes and organelle membranes are particularly vulnerable to ROS because they are rich in PUFA (Viswanathan et al., 2017) that is easily damaged by oxidation, then the lipid bilayer is destroyed and membrane function is affected, which are becoming drivers for ferroptosis. Phospholipids peroxidation begins with the lipid bilayer containing PUFA-PL, removing two hydrogen atoms (located between two carbon-carbon double bonds) from the diallyl group of PUFA-PL’s unsaturated acyl group, and forming a carbon-centered phospholipid radical that reacts with oxygen to produce a phospholipid hydrogen peroxide radical. Then two hydrogen atoms are removed from polyunsaturated fatty acid (PUFA) and form phospholipid hydroperoxide (PLOOH). If PLOOH is not reduced to corresponding alcohols by GPX4, these substances will continue to oxidize PUFA-PL to produce more PLOOH (Conrad and Pratt, 2019), triggering the accumulation of lethal lipid ROS (Ma et al., 2022), causing organelle and/or cell membrane rupture (Jiang et al., 2021b), and inducing ferroptosis. On the contrary, monounsaturated fatty acid (MUFA), such as saturated fat palmitic acid (SFA), is incorporated into phospholipids in an ACSL3 dependent manner through stearoyl CoA desaturase-1 (SCD1). This binding interferes with the formation of PUFA phospholipids and protects cells from cell death caused by ferroptosis (Xiang et al., 2024).
Moreover, a study in 2019 (Doll et al., 2019) showed that the FSP1-CoQ10-NAD(P)H pathway existed as an independent parallel system, which inhibited ferroptosis by producing the antioxidant CoQ10 on the plasma membrane to capture phospholipid peroxidation free radicals, and collaborated with GPX4 and glutathione (GLU) to inhibit phospholipid peroxidation and ferroptosis. Later, DHODH, located in the inner mitochondrial membrane, reduced CoQ10 to panthenol and played a role in ferroptosis inhibition. Thus, several anti-ferroptotic pathways, including SLC7A11/GPX4, FSP1/CoQ, GCH1/BH4, and mitochondria DHODH/CoQ, were established as LPO defending systems to prevent ferroptosis (Gao et al., 2022).
The acyl chain saturation of membrane phospholipids is strongly correlated with the ferroptotic sensitivity of cells, but the influence of specific phospholipid categories on ferroptosis remains obscure. Some scholars (Zhu et al., 2023)have found that phosphatidylcholine (PC) reduction strongly induced ferroptosis, but they excluded the possibility that the sensitivity to ferroptosis is caused by elevated PUFA levels. Meanwhile, PC was required in the construction of new membranes, which made cells are more sensitive to PC deficiency than other tissues. Therefore, it was feasible to induce ferroptosis by simultaneous treatment with canonical ferroptotic inducers and PC synthesis inhibitors.
3 Clinical researches progress of targeting ferroptosis in the treatment of tumors
Ferroptosis has dual properties in the progression of cancer: on the one hand, ferroptosis can help tumor cells evade immune surveillance and reduce the effectiveness of early tumor treatment; on the other hand, it can resist tumor drug resistance, increase sensitivity to radiation, and assist in enhancing immunotherapy (Li et al., 2024). Targeting ferroptosis has been clinically used to treat tumors, whose mechanisms mainly include inhibiting System Xc-, inhibiting/degrading/inactivating GPX4, consuming coenzyme Q10, and inducing lipid peroxidation through peroxides, iron, or PUFA overload (Bian et al., 2024). Diffuse large B-cell lymphoma (DLBCL) is a heterogeneous lymphoid malignancy. It has found that (Zhang et al., 2019; Schmitt et al., 2021; Hong et al., 2022) dimethyl fumarate (DMF) consumes GSH and inhibits NF-κB and JAK/STAT survival signals; APR-246 promotes the binding of repaired p53 mutants to target genes and their transcription factors; Imidazolone Erastine (IKE) is a potent and metabolically stable systemic Xc-inhibitor and ferroptosis inducer by consuming GSH. All of these possess the ability to cause ferroptosis and treat DLBCL. Radiation therapy (RT) is the main treatment method for tumors, but it inevitably damage to normal cells. Ning et al. (2024) found that some plant extracts, exosomes, and plasmids exhibited the repair of radiation-induced hematopoietic and skin damage by inhibiting ferroptosis; Melatonin promoted the binding of Nrf2 and pyruvate kinase isoenzyme M2 (PKM2), which reduced iron concentration and inhibited ferroptosis to alleviate radiation-induced hippocampal neuronal death. Yue et al. (2024)summarized that various anti-tumor drugs such as cisplatin, paclitaxel, capecitabine, apatinib and artemisinin could induce ferroptosis in gastric cancer (GC) cells. And even dexmedetomidine (DEX), levobupivacaine and other local anesthetics had potential value in the treatment of GC due to their ability in regulating ferroptosis and inhibiting GC ell proliferation.
4 Biological compounds intervene in ferroptosis in lung cancer
At present, most studies on inducing ferroptosis mainly focus on western medicine pathways, including targeted dysregulation of iron metabolism, targeted reduction-oxidation pathways, targeted lipid metabolism pathways and other strategies. However, to date, there has been no specific summary of the progress about biological compounds curing lung cancer. Natural plants are a rich source of bioactive ingredients and extracts, with various biological activities, including anti-cancer properties, antioxidation, immunoregulation, and antibacterial effects. Natural compounds have always been of great pharmacological significance, especially in chemoprevention, since they have high availability and affordability. Biological compounds can be performed as substitutes or adjuncts for other anti-tumor therapies for cancer treatment, which increase the sensitivity of tumor cells to radiotherapy and chemotherapy, and even reversing the MDR response to chemotherapy. They can also regulate tumor related immune responses and improve the prognosis of anti-tumor therapy. The latest researches on the promotion of ferroptosis in lung cancer by biological compounds show that they mainly block the progression in the G2/M phase and cause a decrease in the number of G0/G1 and S phase cells (Chen et al., 2020a; Luo and Xu, 2022; Wu et al., 2022; Zhou et al., 2023a). Several key factors, such as glutathione peroxidase 4 (GPX4), reactive oxygen species (ROS), glutathione (GSH), solvecarrierfamily7, and (ionicamino acidtransporter, y + system) member11 (SLC7A11), play important roles in this process. The research progress on the induction of ferroptosis by biological compounds in the treatment of lung cancer is listed in Table 1.
Biological compounds can be classified into different categories based on their chemical composition, containing polyphenols, terpenoids, alkaloids, flavonoids, etc., all of which have an undeniable ability to induce ferroptosis in tumors. In 2015, Professor Tu Youyou was awarded the Nobel Prize in Medicine for extracting artemisinin from the plant Artemisia annua as an anti-malaria drug, which was proved to be a promising drug for treating various diseases, embodying cancer. Further researches have shown that artemisinin and its derivatives can stimulate the ferroptosis cell pathway, containing down-regulating GPX4 expression, increasing ROS and intracellular iron production. There are some natural compounds defined as ferroptosis inducers, such as Ferroptoside (Llabani et al., 2019), Erianin (Luo and Xu, 2022) and Wogonin (Liu et al., 2023a). Ferrotocide, a diterpenoid natural product, is a thioredoxin inhibitor which inducs ferroptosis of breast cancer by inactivating thioredoxin (a cell antioxidant enzyme). Erianin is a natural product derived from Dendrobium chrysotoxum Lindl, and Ca2+/CaM signaling is a key medium of ferroptosis erianin causing. Wogonin, which is extracted from the root of Scutellaria baicalensis Georgi, significantly inhibited the proliferation of pancreatic cancer cells and induced ferroptosis through Nrf2/GPX4 axis.
In addition, although some natural compounds with biological activity like curcumin are shown to activate ferroptosis, they can also be used as ferroptosis inhibitors for tissue damage treatment. Curcumin is the main phenolic pigment extracted from turmeric (the powdery rhizome of turmeric), which inhibits the expression of GPX4 and FSP1 in lung cancer cells (Zhou et al., 2023b), disrupting GPX4 activity in glioblastoma cells (Chen et al., 2020b) and stimulating ferroptosis. On the contrary, curcumin has strong anti-inflammatory and antioxidant properties, being able to eliminate free radicals such as superoxide anions and hydroxyl groups to neutralize ROS. It has been reported that curcumin has ability to inhibit lipid peroxidation in renal epithelial cells, thereby preventing the cytotoxic effects of hydrogen peroxide (Ahangarpour et al., 2019). Thus, more research is needed to demonstrate the biochemical and/or cellular conditions of the action modes of these biological compounds.
Ferroptosis has been recognized as the pathogenic mechanism of many diseases, such as neurodegenerative diseases, ischemia-reperfusion (I/R) injury, etc. And some natural compounds can cure diseases depending on ferroptosis. For example, Hinokitiol (β- Thujaplicin, HIK) is a natural monoterpene small molecule compound with antibacterial, anti-inflammatory, anti-tumor and various biological activities. Hinokitiol is capable to alleviate excessive glutamate to induce ROS, lipid peroxidation, and Fe2+accumulation in neuronal cells and relieve brain tissue damage (Tang et al., 2023). Quercetin (QRC) is absorbed through the intake of diverse fruits, vegetables, and legumes, which upregulates GPX4 and FTH1 and downregulate ACSL4 to inhibit the production of lipid peroxides and ferroptosis. It exerts neuroprotective effects, and demonstrates the therapeutic potential for ischemic stroke (Peng et al., 2023). Baicalin supresses oxidative stress by reducing ROS and protects GPX4 from membrane lipid peroxidation, thereby it curbs ferroptosis and plays a neuroprotective role in cerebrovascular diseases, neurodegenerative diseases, and other central nervous system diseases (Yang et al., 2023).
5 Biological compounds improve lung cancer drug resistance through ferroptosis
The therapy for lung cancer mainly involves surgery combined with radiotherapy and chemotherapy. Cisplatin, gefitinib, osimertinib, etc. are well-known chemotherapy drugs used to treat tumors. However, acquiring resistance to chemotherapy is a major clinical issue, and the mechanism of this resistance is still unknown. So overcoming resistance is the main challenge. Ferroptosis is a special form compared with other forms of cell death, caused by the accumulation of iron-related lipid ROS. Cancer cells are more iron-addicted than normal cells because iron is one of the essential elements in the body. Therefore, cancer cells require more iron in the production process, so that the imbalance of iron metabolism leads to increase the risk of cancer and facilitates tumor growth. By accelerating ferroptosis progress, the development of lung cancer can be suppressed, while the drug resistance and radiation resistance of lung cancer is also resisted to a certain extent (Mou et al., 2019). Studies have shown that achieving ferroptosis increases ROS levels in cells and the cytotoxicity of cisplatin (Golbashirzadeh et al., 2023). Nevertheless, osimertinib-resistant cells are prone to ferroptosis due to raised accumulation of ferrous ions (Konishi et al., 2023). Therefore, a deeper understanding of the process of ferroptosis may guide the discovery of new therapies to overcome tumor resistance.
Biological compounds can be used together with chemotherapy drugs to enhance the sensitivity of the body to chemotherapy drugs and slow down the resistance process by ferroptosis of tumor cells. Feng et al. (2023a) found that the combination of isoorientin (IO) and DDP noticeably decreased the survival ability of drug-resistant cells, regulated ferroptosis and reversed\ drug resistance in lung cancer cells by controlling the SIRT6/Nrf2/GPX4 signaling pathway. Lai et al. (2023) observed that the combination therapy of dihydroartemisinin (DHA) and gefitinib had a better inhibitory effect on lung adenocarcinoma than gefitinib alone. Therefore, ROS is the key factor for DHA to resist the proliferation of drug-resistant lung adenocarcinoma cells and trigger ferroptosis. Li et al. (2022c) used D-borneol and cisplatin to treat non-small cell lung cancer (NSCLC) cells resistant to cisplatin and discovered that it could shrink tumor volume and weight, enhance anti-tumor effect, promote ROS accumulation, stimulate ferritin uptake mediated by nuclear receptor co activator 4(NCOA4), and upregulate PRNP and downregulate PCBP2 to adjust intracellular iron transport to achieve ferroptosis. Yu et al. (2023a) identified a natural NQO1 substrate, 2-methoxy-6-acetyl-7-methyljuglone (MAM), which has potent anti-cancer effects. MAM activates and binds to quinone oxidoreductase 1 (NQO1) which triggers ROS generation, unstable iron pool (LIP) expansion, and lipid peroxidation, activating NQO1-mediated ferroptosis to combat drug resistance.
Plenty of research has identified the crucial part of GPX4 in the susceptibility to drug-mediated ferroptosis in lung cancer, and targeting GPX4 can re-sensitize drug-resistant lung cancer to chemotherapy drugs (Zhang et al., 2022b; Cheng et al., 2023). Wu et al. (2022) have explored that the β-elemene integrated with erlotinib enhanced the cytotoxicity of erlotinib against EGFR mutated primary EGFR-TKI resistant NSCLC cells, and diminished the content of ferroptosis negative regulatory proteins (glutaminase, FTH1, Nrf2, Slc7a11 and GPX4), added ROS and lipid peroxidation levels, and accomplished anti-tumor effects through ferroptosis. In the bargain, Ni et al. (2023) found out that Manoalide (MA) enhanced the sensitivity of osimertinib-resistant lung cancer cells to osimertinib by inhibiting the NRF2-SLC7A11 axis and mitochondrial Ca2+ overload inducing -FTH1 pathway to activate ferroptosis.
6 Nano-drug delivery strategy for inducing ferroptosis in tumor
In recent years, emerging biomedical developments and technological innovations have opened up more possibilities for the effective treatment of cancer. The research on nano-drug delivery system has become an essential choice in cancer therapy. This system overcomes some drawbacks of direct-drug delivery and has significant advantages like improving water solubility, prolonging circulation time, enhancing tumor accumulation, increasing cell uptake and reducing toxicity. It has enormous potential in increasing therapeutic effects.
Nano-technology enables to deliver the active ingredients in sufficient concentrations to the desired site of action, and maintain the biological distribution and accumulation of therapeutic drugs at the desired target site. It avoids premature exposure to the biological environment during delivery, enhances its permeability, and heightens its ability to be absorbed by cells. A number of studies have shown that nanoparticles have the potential to scale up the solubility and stability of phytochemical extracts (Lawson and Gardner, 2005; Putnik et al., 2019). As a result, the use of nanotechnology with biological compounds has advantages in the utilization, which are considered as potential approaches (Lu et al., 2022). Table 2 presents the research on the use of nanotechnology combined with biological compounds to treat tumors through ferroptosis. At present, the biological effects of bioactive inorganic nanomaterials and metal ions have attracted widespread attention (Pei et al., 2023). Nano-carriers whose surfaces are designed to contain active ligands (cancer cell markers or antibodies) - binding structures, based on metals (such as systems carrying transferrin drugs), polymers (nanocapsules and nanospheres), or lipids (such as sulfur-containing glycoside nanoliposomes) carries ferroptosis inducers, which can be modified to target specific cancer cells and achieve anti-tumor effects (Neganova et al., 2022).
Nanoparticles has the ability to load chemotherapy drugs or tumor selective molecules, making them highly suitable for exploring nanoparticle-based malignant tumor treatments and combined therapies. Firstly, there is insufficient H2O2 in the acidic tumor microenvironment (TME) (Yu et al., 2023b), and a number of nanoparticles are designed to accelerate the generation of H2O2 near the tumor cell membrane (Zhang et al., 2023a), triggering Fenton/Fenton-like reaction to produce hydroxyl radicals (• OH) and ROS, amplifying the oxidative stress response (Liu et al., 2023b; Zhang et al., 2023b), and accomplishing ferroptosis within tumor cells. Secondly, overexpression of GSH in TME clears ROS and weakens the performance of ferroptosis therapy (FT) in tumors. The use of nanoparticles consumes the highly expressed GSH in tumors, which leads to the inactivation of GPX4 and accumulation of iron (Sun et al., 2023), thereby triggering ferroptosis and achieving high-performance FT.
Nowadays, extensive experiments and studies have been conducted on the combination of nanoparticles and biological compounds for treatment, but there is relatively little research on ferroptosis. DHA is a first-line antimalarial drug that acts as an inhibitor of system Xc- and GPX4. System Xc-is a heterodimeric cell surface amino acid reverse transporter protein, including transmembrane transporter SLC7A11 which is crucial in initiating ferroptosis. Based on current research, the targets of ferroptosis in lung cancer mainly focus on the regulation of antioxidant pathways, among which GPX4 and SLC7A11 are the core targets of GSH-dependent antioxidant pathways (Xing et al., 2023). On the contrary, due to the inherent drawbacks of DHA including poor stability, low water solubility, and short plasma half-life, its therapeutic efficacy is compromised. To overcome these shortcomings, nanoscale drug delivery systems (NDDSs), such as polymer nanoparticles (NPs), have been introduced to maximize the efficacy of DHA in single or multiple drug therapies (Wong et al., 2022). Fu et al. (2023) constructed an inhalable biomineralized liposome LDM loaded with DHA and pH-responsive calcium phosphate (CaP) as an iron transport nano-inducer for Fenton-like reaction to finish intracellular ROS production and ferroptosis. Zhang et al. (2023c) combined polyglutamic acid-stable Fe3O4 magnetic nanoparticles (Fe3O4-PGA-DHA) loaded with DHA with polyaspartic acid-stable Fe3O4 magnetic nanoparticles (Fe3O4-PASP-DOX) loaded with DOX to achieve significantly more intracellular accumulation of ROS and lipid peroxides for ferroptosis to enhance chemotherapy of triple negative breast cancer. Ta et al. (2023) constructed photosynthetic microcapsules (PMCs) by encapsulating cyanobacteria and up-conversing nanoparticles in alginate microcapsules which driven by external near-infrared photons. The combination of PMC and X-rays activated ferroptosis in MM cells and heterologous plants, delivering evidence for the development of lipid peroxidation, GPX4 inhibition, Fe2+release, and GSH reduction.
What’s more, there have been several research reports on other biological compounds associated with nanotechnology inducing ferroptosis in tumor cells. Feng et al. (2023b) reported that Fe(III)-Shikonin (FeShik) supramolecular nanomedicine could respond to the tumor microenvironment and be decomposed into Fe2+and shikonin, leading to immunogenic cell death through ferroptosis in tumor cells. Wang et al. (2023) constructed a BSA-FA functionalized iron-containing metal organic framework (TPL@TFBF). Through the coordination between tannic acid (TA) and Fe3+, a metal organic framework is formed, then loaded with Triptolide (TPL) and coated with FA modified BSA. The nanoparticles were targeted to modify tumor cells through FA, releasing TPL, Fe3+ and TA. After that, Fe3+ was reduced to Fe2+by TA, triggering Fenton reaction and resulting in ROS production. Additionally, TPL increased intracellular ROS production and induced ferroptosis in tumor cells by preventing the expression of nuclear factor erythroid-2(Nrf2) related factors.
7 Conclusion and prospects
Since ferroptosis was discovered, it has become increasingly associated with tumors and expected to deliver new avenues for anti-cancer treatments. First, some tumor suppressors, such as p53 and BRCA1-associated protein 1 (BAP1), are involved in regulating ferroptosis in cancer cells (Jiang et al., 2015; Zhang et al., 2018). Second, cancer cells require more iron to grow and have higher ROS levels compared to normal cells, which makes cancer cells more susceptible to ferroptosis (Hassannia et al., 2019; Lei et al., 2022). Third, ferroptosis has shown potential to reverse drug resistance in tumor cells (Zhang et al., 2022d). The mesenchymal cell state of cancer cells is generally thought to be related with the spread of metastasis and the acquired drug resistance (Pattabiraman and Weinberg, 2014), and this high mesenchymal cell state has been found to be dependent on the GPX4-mediated lipid peroxidation pathway, which prevents ferroptosis. Therefore, inhibition of this pathway can induce ferroptosis to reverse tumor-acquired drug resistance (Viswanathan et al., 2017). From the above, targeting ferroptosis may provide a new strategy for cancer treatment.
In this review, we summarize the current research status of some biological compounds targeting ferroptosis in the treatment of lung cancer, some of which even reduce tumor cell resistance by inducing ferroptosis. Based on the above facts, research on ferroptosis may make a significant contribution to the development of anti-cancer drugs. Meanwhile, nanomedicine-based therapies have promising results in treating tumor diseases, confirming the therapeutic potential of this cutting-edge technology. We have summarized various nanomedicine delivery strategies that have crucial therapeutic value for tumor diseases in recent years. These nanocarriers, with their unique physical and chemical properties, reach the target site and accumulate, then avoid loss and obstruction along the way, to realize the effect of treating tumors through ferroptosis.
Although ferroptosis has shown great potential in cancer treatment, there are still many unresolved issues. For example, ferroptosis has shown a strong susceptibility to various cancers since its discovery, but its dynamic regulatory mechanisms have been still lacking in research. Is there any other substance that affects the sensitivity of ferroptosis, in addition to the accumulation of iron and lipid peroxides? Besides, as the realization of ferroptosis is usually achieved through lipid peroxidation accumulation and cell membrane damage, it remains to be determined whether lipid peroxides will damage normal cells and whether there will be other potential side effects caused by ferroptosis. Next, the occurrence of ferroptosis is due to the production of ROS by excessive free Fe2+ under the action of Fenton reaction, which produce lipid peroxidation caused by oxidized lipids. Cuproptosis is a newly discovered programmed cell death caused by Cu2+ as well (Shen et al., 2023b). Finally, accumulated research has found that some biological compounds have multiple effects in controlling malignant tumors, such as inducing autophagy to prevent apoptosis of malignant tumor cells. Is this related to factors such as the dosage of traditional Chinese medicine monomers worth further investigation (Zhai et al., 2019; Wu et al., 2022). Therefore, whether other ions can also activate ferroptosis is still a direction well-worth exploring.
Author contributions
QL: Writing–original draft. YW: Writing–review and editing. YiL: Writing–review and editing. JW: Writing–review and editing. CL: Writing–review and editing. YgL: Writing–review and editing.
Funding
The author(s) declare that financial support was received for the research, authorship, and/or publication of this article. This research is supported by National Natural Youth Science Foundation of China grant number 82104957 and New Teacher Project of Beijing University of Chinese Medicine grant number 2023-JYB-XJSJJ050.
Conflict of interest
The authors declare that the research was conducted in the absence of any commercial or financial relationships that could be construed as a potential conflict of interest.
Publisher’s note
All claims expressed in this article are solely those of the authors and do not necessarily represent those of their affiliated organizations, or those of the publisher, the editors and the reviewers. Any product that may be evaluated in this article, or claim that may be made by its manufacturer, is not guaranteed or endorsed by the publisher.
References
Ahangarpour, A., Sayahi, M., and Sayahi, M. (2019). The antidiabetic and antioxidant properties of some phenolic phytochemicals: a review study. Diabetes Metab. Syndr. 13 (1), 854–857. doi:10.1016/j.dsx.2018.11.051
Bian, W., Li, H., Chen, Y., Yu, Y., Lei, G., Yang, X., et al. (2024). Ferroptosis mechanisms and its novel potential therapeutic targets for DLBCL. Biomed. Pharmacother. 173, 116386. Epub ahead of print. doi:10.1016/j.biopha.2024.116386
Chen, P., Lv, X., and Zheng, Z. (2023). Gigantol exerts anti-lung cancer activity by inducing ferroptosis via SLC7A11-GPX4 axis. Biochem. Biophys. Res. Commun. 690, 149274. doi:10.1016/j.bbrc.2023.149274
Chen, P., Wu, Q., Feng, J., Yan, L., Sun, Y., Liu, S., et al. (2020a). Erianin, a novel dibenzyl compound in Dendrobium extract, inhibits lung cancer cell growth and migration via calcium/calmodulin-dependent ferroptosis. Signal Transduct. Target Ther. 5 (1), 51. doi:10.1038/s41392-020-0149-3
Chen, Q., Ma, X., Xie, L., Chen, W., Xu, Z., Song, E., et al. (2021). Iron-based nanoparticles for MR imaging-guided ferroptosis in combination with photodynamic therapy to enhance cancer treatment. Nanoscale 13 (9), 4855–4870. doi:10.1039/d0nr08757b
Chen, T. C., Chuang, J. Y., Ko, C. Y., Kao, T. J., Yang, P. Y., Yu, C. H., et al. (2020b). AR ubiquitination induced by the curcumin analog suppresses growth of temozolomide-resistant glioblastoma through disrupting GPX4-Mediated redox homeostasis. Redox Biol. 30, 101413. doi:10.1016/j.redox.2019.101413
Cheng, F., Dou, J., Yang, Y., Sun, S., Chen, R., Zhang, Z., et al. (2023). Drug-induced lactate confers ferroptosis resistance via p38-SGK1-NEDD4L-dependent upregulation of GPX4 in NSCLC cells. Cell Death Discov. 9 (1), 165. doi:10.1038/s41420-023-01463-5
Cheng, J., Zhu, Y., Xing, X., Xiao, J., Chen, H., Zhang, H., et al. (2021). Manganese-deposited iron oxide promotes tumor-responsive ferroptosis that synergizes the apoptosis of cisplatin. Theranostics 11 (11), 5418–5429. doi:10.7150/thno.53346
Conrad, M., and Pratt, D. A. (2019). The chemical basis of ferroptosis. Nat. Chem. Biol. 15 (12), 1137–1147. doi:10.1038/s41589-019-0408-1
Dixon, S. J., Lemberg, K. M., Lamprecht, M. R., Skouta, R., Zaitsev, E. M., Gleason, C. E., et al. (2012). Ferroptosis: an iron-dependent form of nonapoptotic cell death. Cell 149 (5), 1060–1072. doi:10.1016/j.cell.2012.03.042
Doll, S., Freitas, F. P., Shah, R., Aldrovandi, M., da Silva, M. C., Ingold, I., et al. (2019). FSP1 is a glutathione-independent ferroptosis suppressor. Nature 575 (7784), 693–698. doi:10.1038/s41586-019-1707-0
Du, S., Zeng, F., and Deng, G. (2023). Tumor neutrophils ferroptosis: a targetable immunosuppressive mechanism for cancer immunotherapy. Signal Transduct. Target Ther. 8 (1), 77. doi:10.1038/s41392-023-01357-z
Feng, S., Li, Y., Huang, H., Huang, H., Duan, Y., Yuan, Z., et al. (2023a). Isoorientin reverses lung cancer drug resistance by promoting ferroptosis via the SIRT6/Nrf2/GPX4 signaling pathway. Eur. J. Pharmacol. 954, 175853. doi:10.1016/j.ejphar.2023.175853
Feng, W., Shi, W., Cui, Y., Xu, J., Liu, S., Gao, H., et al. (2023b). Fe(III)-Shikonin supramolecular nanomedicines as immunogenic cell death stimulants and multifunctional immunoadjuvants for tumor vaccination. Theranostics 13 (15), 5266–5289. doi:10.7150/thno.81650
Fu, F., Wang, W., Wu, L., Wang, W., Huang, Z., Huang, Y., et al. (2023). Inhalable biomineralized liposomes for cyclic Ca2+-burst-centered endoplasmic reticulum stress enhanced lung cancer ferroptosis therapy. ACS Nano 17 (6), 5486–5502. doi:10.1021/acsnano.2c10830
Fu, J., Li, T., Yang, Y., Jiang, L., Wang, W., Fu, L., et al. (2021). Activatable nanomedicine for overcoming hypoxia-induced resistance to chemotherapy and inhibiting tumor growth by inducing collaborative apoptosis and ferroptosis in solid tumors. Biomaterials 268, 120537. doi:10.1016/j.biomaterials.2020.120537
Gao, M., Fan, K., Chen, Y., Zhang, G., Chen, J., and Zhang, Y. (2022). Understanding the mechanistic regulation of ferroptosis in cancer: the gene matters. J. Genet. Genomics 49 (10), 913–926. doi:10.1016/j.jgg.2022.06.002
Golbashirzadeh, M., Heidari, H. R., Talebi, M., and Yari Khosroushahi, A. (2023). Ferroptosis as a potential cell death mechanism against cisplatin-resistant lung cancer cell line. Adv. Pharm. Bull. 13 (1), 176–187. doi:10.34172/apb.2023.019
Hassannia, B., Vandenabeele, P., and Vanden Berghe, T. (2019). Targeting ferroptosis to iron out cancer. Cancer Cell 35 (6), 830–849. doi:10.1016/j.ccell.2019.04.002
Hong, Y., Ren, T., Wang, X., Liu, X., Fei, Y., Meng, S., et al. (2022). APR-246 triggers ferritinophagy and ferroptosis of diffuse large B-cell lymphoma cells with distinct TP53 mutations. Leukemia 36 (9), 2269–2280. doi:10.1038/s41375-022-01634-w
Hou, H., Qin, X., Li, G., Cui, Z., Zhang, J., Dong, B., et al. (2024). Nrf2-mediated redox balance alleviates LPS-induced vascular endothelial cell inflammation by inhibiting endothelial cell ferroptosis. Sci. Rep. 14 (1), 3335. doi:10.1038/s41598-024-53976-3
Huang, F., Pang, J., Xu, L., Niu, W., Zhang, Y., Li, S., et al. (2022). Hedyotis diffusa injection induces ferroptosis via the Bax/Bcl2/VDAC2/3 axis in lung adenocarcinoma. Phytomedicine 104, 154319. doi:10.1016/j.phymed.2022.154319
Huang, L., Chang, Y., Cao, P., Nakai, A., Tan, K., Chen, F., et al. (2021). Ferroptosis inducer erastin sensitizes NSCLC cells to celastrol through activation of the ROS-mitochondrial fission-mitophagy axis. Mol. Oncol. 15 (8), 2084–2105. doi:10.1002/1878-0261.12936
Huang, L., Zhu, J., Xiong, W., Feng, J., Yang, J., Lu, X., et al. (2023). Tumor-generated reactive oxygen species storm for high-performance ferroptosis therapy. ACS Nano 17 (12), 11492–11506. doi:10.1021/acsnano.3c01369
Ingold, I., Berndt, C., Schmitt, S., Doll, S., Poschmann, G., Buday, K., et al. (2018). Selenium utilization by GPX4 is required to prevent hydroperoxide-induced ferroptosis. Cell 172 (3), 409–422. doi:10.1016/j.cell.2017.11.048
Jiang, L., Kon, N., Li, T., Wang, S. J., Su, T., Hibshoosh, H., et al. (2015). Ferroptosis as a p53-mediated activity during tumour suppression. Nature 520 (7545), 57–62. doi:10.1038/nature14344
Jiang, X., Stockwell, B. R., and Conrad, M. (2021a). Ferroptosis: mechanisms, biology and role in disease. Nat. Rev. Mol. Cell Biol. 22(4), 266–282. doi:10.1038/s41580-020-00324-8
Jiang, X., Stockwell, B. R., and Conrad, M. (2021b). Ferroptosis: mechanisms, biology and role in disease. Nat. Rev. Mol. Cell Biol. 22 (4), 266–282. doi:10.1038/s41580-020-00324-8
Jiang, Y., Yu, Y., Pan, Z., Glandorff, C., and Sun, M. (2024). Ferroptosis: a new hunter of hepatocellular carcinoma. Cell Death Discov. 10 (1), 136. doi:10.1038/s41420-024-01863-1
Jiaqi, L., Siqing, H., Qin, W., di, Z., Bei, Z., and Jialin, Y. (2023). Andrographolide promoted ferroptosis to repress the development of non-small cell lung cancer through activation of the mitochondrial dysfunction. Phytomedicine 109, 154601. doi:10.1016/j.phymed.2022.154601
Konishi, H., Haga, Y., Lin, Y., Tsujino, H., Higashisaka, K., and Tsutsumi, Y. (2023). Osimertinib-tolerant lung cancer cells are susceptible to ferroptosis. Biochem. Biophys. Res. Commun. 641, 116–122. doi:10.1016/j.bbrc.2022.12.029
Lai, X. Y., Shi, Y. M., and Zhou, M. M. (2023). Dihydroartemisinin enhances gefitinib cytotoxicity against lung adenocarcinoma cells by inducing ROS-dependent apoptosis and ferroptosis. Kaohsiung J. Med. Sci. 39 (7), 699–709. doi:10.1002/kjm2.12684
Lawson, L. D., and Gardner, C. D. (2005). Composition, stability, and bioavailability of garlic products used in a clinical trial. J. Agric. Food Chem. 53 (16), 6254–6261. doi:10.1021/jf050536+
Lei, G., Zhuang, L., and Gan, B. (2022). Targeting ferroptosis as a vulnerability in cancer. Nat. Rev. Cancer 22 (7), 381–396. doi:10.1038/s41568-022-00459-0
Lei, H., Li, Q., Pei, Z., Liu, L., Yang, N., and Cheng, L. (2023). Nonferrous ferroptosis inducer manganese molybdate nanoparticles to enhance tumor immunotherapy. Small 19 (45), e2303438. doi:10.1002/smll.202303438
Li, B., Chen, X., Qiu, W., Zhao, R., Duan, J., Zhang, S., et al. (2022d). Synchronous disintegration of ferroptosis defense Axis via engineered exosome-conjugated magnetic nanoparticles for glioblastoma therapy. Adv. Sci. (Weinh) 9 (17), e2105451. doi:10.1002/advs.202105451
Li, D., and Li, Y. (2020). The interaction between ferroptosis and lipid metabolism in cancer. Signal Transduct. Target Ther. 5 (1), 108. doi:10.1038/s41392-020-00216-5
Li, J., He, D., Li, S., Xiao, J., and Zhu, Z. (2023). Ferroptosis: the emerging player in remodeling triple-negative breast cancer. Front. Immunol. 14, 1284057. doi:10.3389/fimmu.2023.1284057
Li, J., Yuan, J., Li, Y., Wang, J., Xie, Q., Ma, R., et al. (2022c). d-Borneol enhances cisplatin sensitivity via autophagy dependent EMT signaling and NCOA4-mediated ferritinophagy. Phytomedicine 106, 154411. doi:10.1016/j.phymed.2022.154411
Li, L., Gao, Q., Wang, J., Gu, L., Li, Z., Zhang, S., et al. (2022a). Induction of ferroptosis by ophiopogonin-B through regulating the gene signature AURKA in NSCLC. Front. Oncol. 12, 833814. doi:10.3389/fonc.2022.833814
Li, L. G., Peng, X. C., Yu, T. T., Xu, H. Z., Han, N., Yang, X. X., et al. (2022b). Dihydroartemisinin remodels macrophage into an M1 phenotype via ferroptosis-mediated DNA damage. Front. Pharmacol. 13, 949835. doi:10.3389/fphar.2022.949835
Li, Y., Li, X., and Li, J. (2024). Ferroptosis in lung cancer: dual role, multi-level regulation, and new therapeutic strategies. Front. Oncol. 14, 1360638. doi:10.3389/fonc.2024.1360638
Liang, D., Minikes, A. M., and Jiang, X. (2022). Ferroptosis at the intersection of lipid metabolism and cellular signaling. Mol. Cell 82 (12), 2215–2227. doi:10.1016/j.molcel.2022.03.022
Liu, B., Ji, Q., Cheng, Y., Liu, M., Zhang, B., Mei, Q., et al. (2022c). Biomimetic GBM-targeted drug delivery system boosting ferroptosis for immunotherapy of orthotopic drug-resistant GBM. J. Nanobiotechnology 20 (1), 161. doi:10.1186/s12951-022-01360-6
Liu, D., Lin, M., Shu, L., Sun, J., and Chen, X. (2023b). A versatile supramolecular assembly platform for tumor microenvironment motivated drug release and ferroptosis synergistic therapy. Nano Lett. 23 (12), 5713–5721. doi:10.1021/acs.nanolett.3c01440
Liu, G., Liu, M., Li, X., Ye, X., Cao, K., Liu, Y., et al. (2023c). Peroxide-simulating and GSH-depleting nanozyme for enhanced chemodynamic/photodynamic therapy via induction of multisource ROS. ACS Appl. Mater Interfaces 15 (41), 47955–47968. doi:10.1021/acsami.3c09873
Liu, T., Liu, W., Zhang, M., Yu, W., Gao, F., Li, C., et al. (2018a). Ferrous-supply-regeneration nanoengineering for cancer-cell-specific ferroptosis in combination with imaging-guided photodynamic therapy. ACS Nano 12 (12), 12181–12192. doi:10.1021/acsnano.8b05860
Liu, T., Liu, W., Zhang, M., Yu, W., Gao, F., Li, C., et al. (2018b). Ferrous-Supply-Regeneration nanoengineering for cancer-cell-specific ferroptosis in combination with imaging-guided photodynamic therapy. ACS Nano 12 (12), 12181–12192. doi:10.1021/acsnano.8b05860
Liu, X., Hai, Y., Dong, J., Xu, L., Hou, W., Su, J., et al. (2022b). Realgar-induced KRAS mutation lung cancer cell death via KRAS/Raf/MAPK mediates ferroptosis. Int. J. Oncol. 61 (6), 157. doi:10.3892/ijo.2022.5447
Liu, X., Peng, X., Cen, S., Yang, C., Ma, Z., and Shi, X. (2023a). Wogonin induces ferroptosis in pancreatic cancer cells by inhibiting the Nrf2/GPX4 axis. Front. Pharmacol. 14, 1129662. doi:10.3389/fphar.2023.1129662
Liu, X., Zhang, Y., Gao, H., Hou, Y., Lu, J. J., Feng, Y., et al. (2020). Induction of an MLKL mediated non-canonical necroptosis through reactive oxygen species by tanshinol A in lung cancer cells. Biochem. Pharmacol. 171, 113684. doi:10.1016/j.bcp.2019.113684
Liu, X. Y., Wei, D. G., and Li, R. S. (2022a). Capsaicin induces ferroptosis of NSCLC by regulating SLC7A11/GPX4 signaling in vitro. Sci. Rep. 12 (1), 11996. doi:10.1038/s41598-022-16372-3
Llabani, E., Hicklin, R. W., Lee, H. Y., Motika, S. E., Crawford, L. A., Weerapana, E., et al. (2019). Diverse compounds from pleuromutilin lead to a thioredoxin inhibitor and inducer of ferroptosis. Nat. Chem. 11 (6), 521–532. doi:10.1038/s41557-019-0261-6
Lou, J. S., Zhao, L. P., Huang, Z. H., Chen, X. Y., Xu, J. T., Tai, W. C., et al. (2021). Ginkgetin derived from Ginkgo biloba leaves enhances the therapeutic effect of cisplatin via ferroptosis-mediated disruption of the Nrf2/HO-1 axis in EGFR wild-type non-small-cell lung cancer. Phytomedicine 80, 153370. doi:10.1016/j.phymed.2020.153370
Lu, X., Wang, C., Zhao, M., Wu, J., Niu, Z., Zhang, X., et al. (2022). Improving the bioavailability and bioactivity of garlic bioactive compounds via nanotechnology. Crit. Rev. Food Sci. Nutr. 62 (30), 8467–8496. doi:10.1080/10408398.2021.1929058
Luo, L., and Xu, G. (2022). Fascaplysin induces apoptosis and ferroptosis, and enhances anti-PD-1 immunotherapy in non-small cell lung cancer (NSCLC) by promoting PD-L1 expression. Int. J. Mol. Sci. 23 (22), 13774. doi:10.3390/ijms232213774
Ma, X. H., Liu, J. H., Liu, C. Y., Sun, W. Y., Duan, W. J., Wang, G., et al. (2022). ALOX15-launched PUFA-phospholipids peroxidation increases the susceptibility of ferroptosis in ischemia-induced myocardial damage. Signal Transduct. Target Ther. 7 (1), 288. doi:10.1038/s41392-022-01090-z
Mou, Y., Wang, J., Wu, J., He, D., Zhang, C., Duan, C., et al. (2019). Ferroptosis, a new form of cell death: opportunities and challenges in cancer. J. Hematol. Oncol. 12 (1), 34. doi:10.1186/s13045-019-0720-y
Neganova, M. E., Aleksandrova, Y. R., Sukocheva, O. A., and Klochkov, S. G. (2022). Benefits and limitations of nanomedicine treatment of brain cancers and age-dependent neurodegenerative disorders. Semin. Cancer Biol. 86 (2), 805–833. doi:10.1016/j.semcancer.2022.06.011
Ni, C., and Li, D. (2024). Ferroptosis and oxidative stress in endometriosis: a systematic review of the literature. Med. Baltim. 103 (11), e37421. doi:10.1097/MD.0000000000037421
Ni, Y., Liu, J., Zeng, L., Yang, Y., Liu, L., Yao, M., et al. (2023). Natural product manoalide promotes EGFR-TKI sensitivity of lung cancer cells by KRAS-ERK pathway and mitochondrial Ca2+ overload-induced ferroptosis. Front. Pharmacol. 13, 1109822. doi:10.3389/fphar.2022.1109822
Nie, Q., Chen, W., Zhang, T., Ye, S., Ren, Z., Zhang, P., et al. (2023). Iron oxide nanoparticles induce ferroptosis via the autophagic pathway by synergistic bundling with paclitaxel. Mol. Med. Rep. 28 (4), 198. doi:10.3892/mmr.2023.13085
Ning, J., Chen, L., Zeng, Y., Xiao, G., Tian, W., Wu, Q., et al. (2024). The scheme, and regulative mechanism of pyroptosis, ferroptosis, and necroptosis in radiation injury. Int. J. Biol. Sci. 20 (5), 1871–1883. doi:10.7150/ijbs.91112
Oh, E. S., Ryu, H. W., Song, Y. N., Kang, M. J., Huh, Y. H., Park, J. Y., et al. (2023). Diplacone isolated from paulownia tomentosa mature fruit induces ferroptosis-mediated cell death through mitochondrial Ca2+ influx and mitochondrial permeability transition. Int. J. Mol. Sci. 24 (8), 7057. doi:10.3390/ijms24087057
Parker, J. L., Deme, J. C., Kolokouris, D., Kuteyi, G., Biggin, P. C., Lea, S. M., et al. (2021). Molecular basis for redox control by the human cystine/glutamate antiporter system xc<sup/>. Nat. Commun. 12 (1), 7147. doi:10.1038/s41467-021-27414-1
Pattabiraman, D. R., and Weinberg, R. A. (2014). Tackling the cancer stem cells - what challenges do they pose? Nat. Rev. Drug Discov. 13 (7), 497–512. doi:10.1038/nrd4253
Pei, Z., Lei, H., Wu, J., Tang, W., Wei, K., Wang, L., et al. (2023). Bioactive vanadium disulfide nanostructure with "dual" antitumor effects of vanadate and gas for immune-checkpoint blockade-enhanced cancer immunotherapy. ACS Nano 17 (17), 17105–17121. doi:10.1021/acsnano.3c04767
Peng, C., Ai, Q., Zhao, F., Li, H., Sun, Y., Tang, K., et al. (2023). Quercetin attenuates cerebral ischemic injury by inhibiting ferroptosis via Nrf2/HO-1 signaling pathway. Eur. J. Pharmacol. 963, 176264. doi:10.1016/j.ejphar.2023.176264
Putnik, P., Gabrić, D., Roohinejad, S., Barba, F. J., Granato, D., Mallikarjunan, K., et al. (2019). An overview of organosulfur compounds from Allium spp.: from processing and preservation to evaluation of their bioavailability, antimicrobial, and anti-inflammatory properties. Food Chem. 276, 680–691. doi:10.1016/j.foodchem.2018.10.068
Qian, X., Zhu, L., Xu, M., Liu, H., Yu, X., Shao, Q., et al. (2023). Shikonin suppresses small cell lung cancer growth via inducing ATF3-mediated ferroptosis to promote ROS accumulation. Chem. Biol. Interact. 382, 110588. doi:10.1016/j.cbi.2023.110588
Riegman, M., Sagie, L., Galed, C., Levin, T., Steinberg, N., Dixon, S. J., et al. (2020). Ferroptosis occurs through an osmotic mechanism and propagates independently of cell rupture. Nat. Cell Biol. 22 (9), 1042–1048. doi:10.1038/s41556-020-0565-1
Sang, M., Luo, R., Bai, Y., Dou, J., Zhang, Z., Liu, F., et al. (2019). Mitochondrial membrane anchored photosensitive nano-device for lipid hydroperoxides burst and inducing ferroptosis to surmount therapy-resistant cancer. Theranostics 9 (21), 6209–6223. doi:10.7150/thno.36283
Schmitt, A., Xu, W., Bucher, P., Grimm, M., Konantz, M., Horn, H., et al. (2021). Dimethyl fumarate induces ferroptosis and impairs NF-κB/STAT3 signaling in DLBCL. Blood 138 (10), 871–884. doi:10.1182/blood.2020009404
Shan, X., Li, J., Liu, J., Feng, B., Zhang, T., Liu, Q., et al. (2023). Targeting ferroptosis by poly(acrylic) acid coated Mn3O4 nanoparticles alleviates acute liver injury. Nat. Commun. 14 (1), 7598. doi:10.1038/s41467-023-43308-w
Shao, M., Jiang, Q., Shen, C., Liu, Z., and Qiu, L. (2022). Sinapine induced ferroptosis in non-small cell lung cancer cells by upregulating transferrin/transferrin receptor and downregulating SLC7A11. Gene 827, 146460. doi:10.1016/j.gene.2022.146460
Shen, H., Wei, Y., Yang, Q., Cai, Y., Zhu, K., and Chen, X. (2023a). Scoparone induces both apoptosis and ferroptosis via multiple mechanisms in non-small-cell lung cancer cells. Toxicol Vitro 91, 105627. doi:10.1016/j.tiv.2023.105627
Shen, Y., Li, D., Liang, Q., Yang, M., Pan, Y., and Li, H. (2023b). Cross-talk between cuproptosis and ferroptosis regulators defines the tumor microenvironment for the prediction of prognosis and therapies in lung adenocarcinoma. Front. Immunol. 13, 1029092. doi:10.3389/fimmu.2022.1029092
Shen, Z., Liu, T., Li, Y., Lau, J., Yang, Z., Fan, W., et al. (2018). Fenton-reaction-acceleratable magnetic nanoparticles for ferroptosis therapy of orthotopic brain tumors. ACS Nano 12 (11), 11355–11365. doi:10.1021/acsnano.8b06201
Siegel, R. L., Miller, K. D., Wagle, N. S., and Jemal, A. (2023). Cancer statistics, 2023. CA Cancer J. Clin. 73 (1), 17–48. doi:10.3322/caac.21763
Su, M., Chen, Y., Jia, L., and Zhang, Z. (2022). Camptothecin-loaded and manganese dioxide-coated polydopamine nanomedicine used for magnetic resonance imaging diagnosis and chemo-photothermal therapy for lung cancer. Int. J. Nanomedicine 17, 6687–6705. doi:10.2147/IJN.S359300
Sun, S., Shen, J., Jiang, J., Wang, F., and Min, J. (2023). Targeting ferroptosis opens new avenues for the development of novel therapeutics. Signal Transduct. Target Ther. 8 (1), 372. doi:10.1038/s41392-023-01606-1
Sutton, H. C., and Winterbourn, C. C. (1989). On the participation of higher oxidation states of iron and copper in Fenton reactions. Free Radic. Biol. Med. 6, 53–60. doi:10.1016/0891-5849(89)90160-3
Ta, N., Jiang, X., Zhang, Y., and Wang, H. (2023). Ferroptosis as a promising therapeutic strategy for melanoma. Front. Pharmacol. 14, 1252567. doi:10.3389/fphar.2023.1252567
Tang, B., Xu, W., Wang, Y., Zhu, J., Wang, H., Tu, J., et al. (2021a). Identification of critical ferroptosis regulators in lung adenocarcinoma that RRM2 facilitates tumor immune infiltration by inhibiting ferroptotic death. Clin. Immunol. 232, 108872. doi:10.1016/j.clim.2021.108872
Tang, D., Chen, X., Kang, R., and Kroemer, G. (2021b). Ferroptosis: molecular mechanisms and health implications. Cell Res. 31 (2), 107–125. doi:10.1038/s41422-020-00441-1
Tang, H., He, K., Zhao, K., Zheng, C., Wu, W., Jin, W., et al. (2023). Protective effects of Hinokitiol on neuronal ferroptosis by activating the KEAP1/NRF-2/HO-1 pathway in traumatic brain injury. J. Neurotrauma 14, 734–750. doi:10.1089/neu.2023.0150
Tian, H. X., Mei, J., Cao, L., Song, J., Rong, D., Fang, M., et al. (2023). Disruption of iron homeostasis to induce ferroptosis with albumin-encapsulated Pt(IV) nanodrug for the treatment of non-small cell lung cancer. Small 22, e2206688. doi:10.1002/smll.202206688
Tian, Y. Y., Yang, A. L., Chen, X. N., Ren, H. M., Liu, Y. X., Qiu, H. L., et al. (2020). Effect of Huaier aqueous extract on growth and metastasis of human non-small cell lung cancer NCI-H1299 cells and its underlying mechanisms. Zhongguo Zhong Yao Za Zhi 45 (15), 3700–3706. Chinese. doi:10.19540/j.cnki.cjcmm.20200310.401
Tsai, Y., Xia, C., and Sun, Z. (2020). The inhibitory effect of 6-gingerol on ubiquitin-specific peptidase 14 enhances autophagy-dependent ferroptosis and anti-tumor in vivo and in vitro. Front. Pharmacol. 11, 598555. doi:10.3389/fphar.2020.598555
Tsoi, J., Robert, L., Paraiso, K., Galvan, C., Sheu, K. M., Lay, J., et al. (2018). Multi-stage differentiation defines melanoma subtypes with differential vulnerability to drug-induced iron-dependent oxidative stress. Cancer Cell 33 (5), 890–904. doi:10.1016/j.ccell.2018.03.017
Ursini, F., Maiorino, M., Valente, M., Ferri, L., and Gregolin, C. (1982). Purification from pig liver of a protein which protects liposomes and biomembranes from peroxidative degradation and exhibits glutathione peroxidase activity on phosphatidylcholine hydroperoxides. Biochim. Biophys. Acta 710, 197–211. doi:10.1016/0005-2760(82)90150-3
Viswanathan, V. S., Ryan, M. J., Dhruv, H. D., Gill, S., Eichhoff, O. M., Seashore-Ludlow, B., et al. (2017). Dependency of a therapy-resistant state of cancer cells on a lipid peroxidase pathway. Nature 547 (7664), 453–457. doi:10.1038/nature23007
Wang, C., Wen, L., Wang, K., Wu, R., Li, M., Zhang, Y., et al. (2023c). Visualization of ferroptosis in brain diseases and ferroptosis-inducing nanomedicine for glioma. Am. J. Nucl. Med. Mol. Imaging 13 (5), 179–194.
Wang, L., Fu, H., Song, L., Wu, Z., Yu, J., Guo, Q., et al. (2023b). Overcoming AZD9291 resistance and metastasis of NSCLC via ferroptosis and multitarget interference by nanocatalytic sensitizer plus AHP-DRI-12. Small 19 (4), e2204133. doi:10.1002/smll.202204133
Wang, S., Guo, Q., Xu, R., Lin, P., Deng, G., and Xia, X. (2023a). Combination of ferroptosis and pyroptosis dual induction by triptolide nano-MOFs for immunotherapy of Melanoma. J. Nanobiotechnology 21 (1), 383. doi:10.1186/s12951-023-02146-0
Wang, S., Li, F., Qiao, R., Hu, X., Liao, H., Chen, L., et al. (2018). Arginine-rich manganese silicate nanobubbles as a ferroptosis-inducing agent for tumor-targeted theranostics. ACS Nano 12 (12), 12380–12392. doi:10.1021/acsnano.8b06399
Wong, K. H., Yang, D., Chen, S., He, C., and Chen, M. (2022). Development of nanoscale drug delivery systems of dihydroartemisinin for cancer therapy: a review. Asian J. Pharm. Sci. 17 (4), 475–490. doi:10.1016/j.ajps.2022.04.005
Wu, C. Y., Yang, Y. H., Lin, Y. S., Chang, G. H., Tsai, M. S., Hsu, C. M., et al. (2021). Dihydroisotanshinone I induced ferroptosis and apoptosis of lung cancer cells. Biomed. Pharmacother. 139, 111585. doi:10.1016/j.biopha.2021.111585
Wu, H., Liu, Q., Shan, X., Gao, W., and Chen, Q. (2023). ATM orchestrates ferritinophagy and ferroptosis by phosphorylating NCOA4. Autophagy 19 (7), 2062–2077. doi:10.1080/15548627.2023.2170960
Wu, Y., Wang, D., Lou, Y., Liu, X., Huang, P., Jin, M., et al. (2022). Regulatory mechanism of α-hederin upon cisplatin sensibility in NSCLC at safe dose by destroying GSS/GSH/GPX2 axis-mediated glutathione oxidation-reduction system. Biomed. Pharmacother. 150, 112927. doi:10.1016/j.biopha.2022.112927
Xiang, D., Zhou, L., Yang, R., Yuan, F., Xu, Y., Yang, Y., et al. (2024). Advances in ferroptosis-inducing agents by targeted delivery system in cancer therapy. Int. J. Nanomedicine 19, 2091–2112. doi:10.2147/IJN.S448715
Xing, N., Du, Q., Guo, S., Xiang, G., Zhang, Y., Meng, X., et al. (2023). Ferroptosis in lung cancer: a novel pathway regulating cell death and a promising target for drug therapy. Cell Death Discov. 9 (1), 110. doi:10.1038/s41420-023-01407-z
Xu, C., Chen, Y., Yu, Q., Song, J., Jin, Y., and Gao, X. (2023a). Compounds targeting ferroptosis in breast cancer: progress and their therapeutic potential. Front. Pharmacol. 14, 1243286. doi:10.3389/fphar.2023.1243286
Xu, C., Jiang, Z. B., Shao, L., Zhao, Z. M., Fan, X. X., Sui, X., et al. (2023b). β-Elemene enhances erlotinib sensitivity through induction of ferroptosis by upregulating lncRNA H19 in EGFR-mutant non-small cell lung cancer. Pharmacol. Res. 191, 106739. doi:10.1016/j.phrs.2023.106739
Xu, R., Wu, J., Luo, Y., Wang, Y., Tian, J., Teng, W., et al. (2022). Sanguinarine represses the growth and metastasis of non-small cell lung cancer by facilitating ferroptosis. Curr. Pharm. Des. 28 (9), 760–768. doi:10.2174/1381612828666220217124542
Xu, T., Ma, Y., Yuan, Q., Hu, H., Hu, X., Qian, Z., et al. (2020). Enhanced ferroptosis by oxygen-boosted phototherapy based on a 2-in-1 nanoplatform of ferrous hemoglobin for tumor synergistic therapy. ACS Nano 14 (3), 3414–3425. doi:10.1021/acsnano.9b09426
Yan, H. F., Zou, T., Tuo, Q. Z., Xu, S., Li, H., Belaidi, A. A., et al. (2021). Ferroptosis: mechanisms and links with diseases. Signal Transduct. Target Ther. 6 (1), 49. doi:10.1038/s41392-020-00428-9
Yang, K., Zeng, L., Zeng, J., Deng, Y., Wang, S., Xu, H., et al. (2023). Research progress in the molecular mechanism of ferroptosis in Parkinson's disease and regulation by natural plant products. Ageing Res. Rev. 91, 102063. doi:10.1016/j.arr.2023.102063
Yang, S., Wong, K. H., Hua, P., He, C., Yu, H., Shao, D., et al. (2022). ROS-responsive fluorinated polyethyleneimine vector to co-deliver shMTHFD2 and shGPX4 plasmids induces ferroptosis and apoptosis for cancer therapy. Acta Biomater. 140, 492–505. doi:10.1016/j.actbio.2021.11.042
Ye, J., Zhang, F., Li, B., Liu, Q., and Zeng, G. (2023). Knockdown of ATF3 suppresses the progression of ischemic stroke through inhibiting ferroptosis. Front. Mol. Neurosci. 15, 1079338. doi:10.3389/fnmol.2022.1079338
Yu, J., Zhong, B., Zhao, L., Hou, Y., Ai, N., Lu, J. J., et al. (2023a). Fighting drug-resistant lung cancer by induction of NAD(P)H:quinone oxidoreductase 1 (NQO1)-mediated ferroptosis. Drug Resist Updat 70, 100977. doi:10.1016/j.drup.2023.100977
Yu, W., Jia, F., Fu, J., Chen, Y., Huang, Y., Jin, Q., et al. (2023b). Enhanced transcutaneous chemodynamic therapy for melanoma treatment through cascaded fenton-like reactions and nitric oxide delivery. ACS Nano 17 (16), 15713–15723. doi:10.1021/acsnano.3c02964
Yue, R., Zhou, M., Li, X., Xu, L., Lu, C., Dong, Z., et al. (2023). GSH/APE1 cascade-activated nanoplatform for imaging therapy resistance dynamics and enzyme-mediated adaptive ferroptosis. ACS Nano 17 (14), 13792–13810. doi:10.1021/acsnano.3c03443
Yue, Z., Yuan, Y., Zhou, Q., Sheng, J., and Xin, L. (2024). Ferroptosis and its current progress in gastric cancer. Front. Cell Dev. Biol. 12, 1289335. doi:10.3389/fcell.2024.1289335
Zeng, Y. Y., Luo, Y. B., Ju, X. D., Zhang, B., Cui, Y. J., Pan, Y. B., et al. (2022). Solasonine causes redox imbalance and mitochondrial oxidative stress of ferroptosis in lung adenocarcinoma. Front. Oncol. 12, 874900. doi:10.3389/fonc.2022.874900
Zhai, B., Zhang, N., Han, X., Li, Q., Zhang, M., Chen, X., et al. (2019). Molecular targets of β-elemene, a herbal extract used in traditional Chinese medicine, and its potential role in cancer therapy: a review. Biomed. Pharmacother. 114, 108812. doi:10.1016/j.biopha.2019.108812
Zhai, F. G., Liang, Q. C., Wu, Y. Y., Liu, J. Q., and Liu, J. W. (2022). Red ginseng polysaccharide exhibits anticancer activity through GPX4 downregulation-induced ferroptosis. Pharm. Biol. 60 (1), 909–914. doi:10.1080/13880209.2022.2066139
Zhang, C., Liu, X., Jin, S., Chen, Y., and Guo, R. (2022d). Ferroptosis in cancer therapy: a novel approach to reversing drug resistance. Mol. Cancer 21 (1), 47. doi:10.1186/s12943-022-01530-y
Zhang, C., Wang, C., Yang, Z., Bai, Y., Shukuya, T., Poh, M. E., et al. (2022b). Identification of GPX4 as a therapeutic target for lung adenocarcinoma after EGFR-TKI resistance. Transl. Lung Cancer Res. 11 (5), 786–801. doi:10.21037/tlcr-22-318
Zhang, J., Zhou, K., Lin, J., Yao, X., Ju, D., Zeng, X., et al. (2023c). Ferroptosis-enhanced chemotherapy for triple-negative breast cancer with magnetic composite nanoparticles. Biomaterials 303, 122395. doi:10.1016/j.biomaterials.2023.122395
Zhang, Q., Kuang, G., Wang, H., Zhao, Y., Wei, J., and Shang, L. (2023d). Multi-bioinspired MOF delivery systems from microfluidics for tumor multimodal therapy. Adv. Sci. (Weinh) 10 (33), e2303818. doi:10.1002/advs.202303818
Zhang, Q., Yi, H., Yao, H., Lu, L., He, G., Wu, M., et al. (2021b). Artemisinin derivatives inhibit non-small cell lung cancer cells through induction of ROS-dependent apoptosis/ferroptosis. J. Cancer 12 (13), 4075–4085. doi:10.7150/jca.57054
Zhang, R., Pan, T., Xiang, Y., Zhang, M., Xie, H., Liang, Z., et al. (2021a). Curcumenol triggered ferroptosis in lung cancer cells via lncRNA H19/miR-19b-3p/FTH1 axis. Bioact. Mater 13, 23–36. doi:10.1016/j.bioactmat.2021.11.013
Zhang, W., Jiang, B., Liu, Y., Xu, L., and Wan, M. (2022a). Bufotalin induces ferroptosis in non-small cell lung cancer cells by facilitating the ubiquitination and degradation of GPX4. Free Radic. Biol. Med. 180, 75–84. doi:10.1016/j.freeradbiomed.2022.01.009
Zhang, X., Ding, Y., Zhang, Z., Ma, Y., Sun, X., Wang, L., et al. (2023a). In situ construction of ferrocene-containing membrane-bound nanofibers for the redox control of cancer cell death and cancer therapy. Nano Lett. 23 (16), 7665–7674. doi:10.1021/acs.nanolett.3c02362
Zhang, Y., Du, X., He, Z., Gao, S., Ye, L., Ji, J., et al. (2023e). A vanadium-based nanoplatform synergizing ferroptotic-like therapy with glucose metabolism intervention for enhanced cancer cell death and antitumor immunity. ACS Nano 17 (12), 11537–11556. doi:10.1021/acsnano.3c01527
Zhang, Y., Li, L., Li, Y., Fei, Y., Xue, C., Yao, X., et al. (2022c). An ROS-activatable nanoassembly remodulates tumor cell metabolism for enhanced ferroptosis therapy. Adv. Healthc. Mater 11 (2), e2101702. doi:10.1002/adhm.202101702
Zhang, Y., Shi, J., Liu, X., Feng, L., Gong, Z., Koppula, P., et al. (2018). BAP1 links metabolic regulation of ferroptosis to tumour suppression. Nat. Cell Biol. 20 (10), 1181–1192. doi:10.1038/s41556-018-0178-0
Zhang, Y., Tan, H., Daniels, J. D., Zandkarimi, F., Liu, H., Brown, L. M., et al. (2019). Imidazole ketone erastin induces ferroptosis and slows tumor growth in a mouse lymphoma model. Cell Chem. Biol. 26 (5), 623–633. doi:10.1016/j.chembiol.2019.01.008
Zhang, Y., Zhang, Q., Wang, F., Li, M., Shi, X., and Li, J. (2023b). Activatable semiconducting polymer nanoinducers amplify oxidative damage via sono-ferroptosis for synergistic therapy of bone metastasis. Nano Lett. 23 (16), 7699–7708. doi:10.1021/acs.nanolett.3c02414
Zheng, Y., Wang, Y., Lu, Z., Wan, J., Jiang, L., Song, D., et al. (2023). PGAM1 inhibition promotes HCC ferroptosis and synergizes with anti-PD-1 immunotherapy. Adv. Sci. (Weinh) 14, e2301928. doi:10.1002/advs.202301928
Zhou, B., Liu, J., Kang, R., Klionsky, D. J., Kroemer, G., and Tang, D. (2020). Ferroptosis is a type of autophagy-dependent cell death. Semin. Cancer Biol. 66, 89–100. doi:10.1016/j.semcancer.2019.03.002
Zhou, C., Yu, T., Zhu, R., Lu, J., Ouyang, X., Zhang, Z., et al. (2023a). Timosaponin AIII promotes non-small-cell lung cancer ferroptosis through targeting and facilitating HSP90 mediated GPX4 ubiquitination and degradation. Int. J. Biol. Sci. 19 (5), 1471–1489. doi:10.7150/ijbs.77979
Zhou, J., Zhang, L., Yan, J., Hou, A., Sui, W., and Sun, M. (2023b). Curcumin induces ferroptosis in A549 CD133+ cells through the GSH-GPX4 and FSP1-CoQ10-NAPH pathways. Discov. Med. 35 (176), 251–263. doi:10.24976/Discov.Med.202335176.26
Zhu, J., Meng, W., Lam, S. M., Shui, G., and Huang, X. (2023). Phosphatidylcholine deficiency increases ferroptosis susceptibility in the Caenorhabditis elegans germline. J. Genet. Genomics 50 (5), 318–329. doi:10.1016/j.jgg.2023.03.005
Zhu, M., Wu, P., Li, Y., Zhang, L., Zong, Y., and Wan, M. (2022). Synergistic therapy for orthotopic gliomas via biomimetic nanosonosensitizer-mediated sonodynamic therapy and ferroptosis. Biomater. Sci. 10 (14), 3911–3923. doi:10.1039/d2bm00562j
Keywords: ferroptosis, lung cancer, biological compound, resistance, nanotechnology
Citation: Liang Q, Wang Y, Li Y, Wang J, Liu C and Li Y (2024) Ferroptosis: emerging roles in lung cancer and potential implications in biological compounds. Front. Pharmacol. 15:1374182. doi: 10.3389/fphar.2024.1374182
Received: 21 January 2024; Accepted: 22 April 2024;
Published: 09 May 2024.
Edited by:
Jianqiang Xu, Dalian University of Technology, ChinaCopyright © 2024 Liang, Wang, Li, Wang, Liu and Li. This is an open-access article distributed under the terms of the Creative Commons Attribution License (CC BY). The use, distribution or reproduction in other forums is permitted, provided the original author(s) and the copyright owner(s) are credited and that the original publication in this journal is cited, in accordance with accepted academic practice. No use, distribution or reproduction is permitted which does not comply with these terms.
*Correspondence: Chuanbo Liu, bGNiNDgyMEAxNjMuY29t; Yicong Li, bGl5aWNvbmczMzBAMTYzLmNvbQ==