- 1Department of Physiology, College of Medicine, University of Bisha, Bisha, Saudi Arabia
- 2Department of Engineering Process, National Higher Polytechnic School of Douala, University of Douala, Douala, Cameroon
- 3Department of Pharmacology, College of Medicine, University of Bisha, Bisha, Saudi Arabia
- 4Department of Child Health College of Medicine, University of Bisha, Bisha, Saudi Arabia
- 5Neoteriks Health Research and Innovation, Avon, IN, United States
- 6Department of Basic Medical Sciences, Faculty of Medicine, Majmaah University, Al Majma’ah, Saudi Arabia
- 7Department of Pathology College of Medicine University of Bisha, Bisha, Saudi Arabia
- 8Department of Internal Medicine, College of Medicine, University of Bisha, Bisha, Saudi Arabia
- 9Department of Surgery, College of Medicine, University of Bisha, Bisha, Saudi Arabia
- 10Department of Ophthalmology, College of Medicine, King Khalid University, Abha, Saudi Arabia
- 11Department of Anatomy, College of Medicine, University of Bisha, Bisha, Saudi Arabia
- 12Department of Community and Family Medicine, College of Medicine University of Bisha, Bisha, Saudi Arabia
- 13Department of Biochemistry, Faculty of Science, University of Dschang, Dschang, Cameroon
Introduction: There is a growing interest in studying natural products for the identification of novel lead compounds for drug development for treating inflammatory diseases. Although some studies have focused anti-inflammatory activity of benzophenones and xanthones, exploring additional targets such as enzymes and cytokines, involved in their inflammatory response could provide more comprehensive understanding of the compounds’ anti-inflammatory effects. In this study, four xanthones ananixanthone (1), smeathxanthone A (2), smeathxanthone B (3), and 1,3,5,8-tetrahydroxy-2-(3-methybut-2-enyl)-4-(3,7-dimethyloct-2,6-dienyl) xanthone (4); and three benzophenones guttiferone O (5), guttiferone M (6), and aristophenone A (7) from Garcinia smeathmannii (Planch. & Triana) Oliv. were investigated for their effect on nitric oxide production, cyclooxygenase, lipoxygenase inhibition, and Th1/Th2 cytokines production in activated RAW 264.7 macrophages.
Methods: The Griess reagent method and the ferrous oxidation-xylenol orange assay were used to evaluate the inhibition of NO production and the 15-lipoxygenase activity respectively. Cyclooxygenase activity was assessed using the fluorometric COX activity assay kit and measurement of Th1/Th2 cytokines was performed using a flow cytometer.
Results: All the tested compounds exhibited a dose-dependent inhibition of NO production with varying degrees of inhibitory effects on 15-LOX activity. Compound (6), displays the best inhibitory effect on COX-1/COX-2 activity. A general trend of the tested compounds on cytokines profiles revealed that compound (5) showed a pronounced enhancement of anti-inflammatory cytokines (IL-4 and IL-10).
Conclusion: This observation supports future exploration of ananixanthone (1), guttiferone O (5), and guttiferone (6) as potential candidates for the development of anti-inflammatory drugs.
1 Introduction
Dysregulated inflammation is a complex biological response orchestrated by the immune system which can lead to a myriad of chronic diseases, including autoimmune disorders, cardiovascular complications, and various cancers (Calhelha et al., 2023). It involves the activation of immune and inflammatory cells such as macrophages (Kolliniati et al., 2022). Macrophages play a crucial role in the initiation and development of multiple inflammatory diseases and could be activated to release inflammatory cytokines and mediators such as nitric oxide (NO), interleukins, interferon-γ (IFN-γ), and tumour necrosis factor-α (TNF-α) (Marrocco and Ortiz, 2022). Additionally, inflammatory enzymes cyclooxygenase (COX) and lipoxygenase (LOX) play pivotal roles in the initiation and progression of inflammation (Cui et al., 2022). Cyclooxygenase, existing in two isoforms (COX-1 and COX-2), catalyzes the conversion of arachidonic acid into prostaglandins, while LOX is responsible for the synthesis of leukotrienes (Smith et al., 2011). Available literature data indicated that pro-inflammatory enzymes and cytokines play an important role in the pathogenesis of inflammation via different pathways (Chen et al., 2018). Hence, the inhibition of these enzymes and other inflammatory mediators is considered an important target for the management of inflammation-related diseases.
The current therapeutic strategy for managing chronic inflammation is largely dominated by synthetic drugs especially non-steroidal anti-inflammatory drugs (NSAIDs). These drugs are often accompanied by limited efficacy and unintended side effects including gastrointestinal complications and immunosuppression (Ziesenitz et al., 2022; Farah et al., 2023). Therefore, there is a need to explore safer and more effective alternatives to overcome painful and other inflammatory conditions. Hence, there has been an increasing interest in the exploration of naturally occurring compounds as potential anti-inflammatory agents (Bakar et al., 2018). Furthermore, a particular focus has emerged on the study of naturally occurring benzophenones and xanthones which are compounds abundant in various plants, and renowned for their diverse biological activities (Xue et al., 2020). Due to their interesting scaffolds and great pharmacological importance, some benzophenone and xanthone compounds have been investigated as potential anti-inflammatory drug candidates (Huang et al., 2020). The ability of several benzophenones and xanthones derivates compounds to alleviate inflammation has been demonstrated so far, with several studies pointing out inhibition of COX enzymes and inflammatory mediators as the main anti-inflammatory mechanisms. 1,3,5,6-tetrahydroxyxanthone (3) and guttiferone E (7) were reported as potential inhibitors of NO production and 15-LOX activity (Dzoyem et al., 2015). In the study of Folquitto et al. (2022), some benzophenones analogs including 2′-hydroxy-4′-benzoylphenyl-β-D-glucopyranoside, 4-hydroxy-4′-methoxybenzophenone and 4′-(4″-methoxybenzoyl) phenyl-β-D-glucopyranoside showed interestig values of Glide Score in COX-2 docking evaluation and selectively inhibited COX-2 and COX-1 in an vitro enzymatic assay (Folquitto et al., 2022). Malik et al. (2022) showed the ability of some benzophenone derivatives to inhibit pro-inflammatory mediators including IFN-γ, TNF-α, IL-1β, IL-6, GM-CSF, IL-2and nitric oxide (NO) (Dzoyem et al., 2015; Folquitto et al., 2022; Malik et al., 2022; Saikia et al., 2023). Another study reported the potential of hydroxyxanthone derivatives to inibit COX-2 enzyme (Dzoyem et al., 2015; Folquitto et al., 2022; Malik et al., 2022; Saikia et al., 2023). Despite these previous work, the full anti-inflammatory potential of the great diversity of benzophenones and xanthones has not yet been fully investigated, exploring additional targets such as enzymes and cytokines, involved in their inflammatory response could provide more comprehensive understanding of the compounds’ anti-inflammatory effects. Therefore, new anti-inflammatory agents having multitarget mechanisms with improved safety profiles that prevent the release of prostaglandins, leukotrienes, and Th1/Th2 cytokines are needed. Therefore, this work was undertaken to investigate seven naturally occurring benzophenones and xanthones derivatives isolated from Garcinia smeathmannii (Planch. & Triana) Oliv. as potent anti-inflammatory agents with a different probable mechanism of action. Although several studies have demonstrated the ability of some benzophenones and xanthones to modulate various targets involved in the inflammatory response, detailed evaluation of ananixanthone, smeathxanthones A and B, 1,3,5,8-tetrahydroxy-2-(3-methybut-2-enyl)-4-(3,7-dimethyloct-2,6-dienyl) xanthone, guttiferone O and M, and aristophenone in modulating key mediators of inflammation such as LOX, COX and cytokines has not been carried out. In order to explore the role of the above mentioned benzophenones and xanthones in managing inflammation associated with various diseases, this study was designed to investigate in vitro effect on nitric oxide production, COX/15-LOX inhibition and Th1/Th2 cytokines production in RAW264.7 activated macrophages.
2 Materials and methods
2.1 Natural products
2.1.1 Plant material
The stem bark of Garcinia. smeathmannii (Planch. & Triana) Oliv. was collected from Cheffou-Baham (5° 20′9.33″N, 10° 23′34.84″E), western province, Cameroon in April 2010 and was identified by Victor Nana of the Cameroon National Herbarium (CNH), Yaoundé, where a voucher specimen (35169/HNC) has been deposited.
2.1.2 General experimental procedures
Melting points were determined on a Büchi-540 melting point apparatus. IR spectra were determined on Nicolet 380 Fourier Transform IR spectrometer. UV spectra were determined on a Spectronic Unicam spectrophotometer. The 1H, 13C, and DEPT NMR spectra, as well as two-dimensional experiments (COSY, NOESY, HSQC, HMBC using pulsed field gradients), were recorded on a Bruker DRX 500 FT-NMR spectrometer, operating at 500 MHz (1H) and 125 MHz (13C) and Avance 600 FT-NMR spectrometer, operating at 600 MHz (1H) and 150 MHz (13C) in CDCl3, C3D6O or DMSO-d6 with TMS as internal standard.
EI Mass spectra and accurate mass spectra were recorded with a LC linear ion trap instrument (Esquire 3000) using electrospray ionization in the negative or positive mode and Sector field mass spectrometer (Autospec X). Vacuum Liquid Chromatography (VLC) was carried out using Merck Silica Gel 60 GF254 (230–400 mesh), column chromatography using Silica Gel 60 (230–400 mesh, 70–230 mesh), TLC analysis was performed on Silica Gel plates (Merck kieselgel 60 GF254, 0.25 mm, 20 × 20 cm) with different mixtures of petrol ether, cyclohexane, dichloromethane, ethyl acetate, and acetone and methanol as eluents; spots were visualized under UV lamps (254 nm) and (365 nm) or by MeOH–H2SO4 reagent. Solvent evaporation was done using Rota vapor (laborota 4000; Heidolph).
2.1.3 Isolation and identification of compounds
The air-dried, powdered stem bark of G. smeathmannii (2.5 kg) was extracted at room temperature for 3 days using distilled methanol (12 L). The crude methanol extract (207 g) obtained was partitioned with petroleum ether (88 g; 2.5 L), dichloromethane (20.4 g; 1.5 L), and ethyl acetate (32 g; 2 L).
The petroleum ether fraction (80 g) was subjected to flash column chromatography using silica gel (230–400 mesh; 800 g) eluted with pure petroleum ether, petroleum ether-EtOAc (9: 1), petroleum ether-EtOAc (7.5: 2.5), petroleum ether-EtOAc (1: 1), EtOAc and EtOAc-MeOH (7.5: 2.5) to give five main fractions labeled A (27 g), B (22 g), C (14 g), D (17 g), and E (6.4 g), respectively.
Fraction A (25 g) was then subjected to column chromatography (5 × 100 cm) on silica gel (600 g, 230–400 mesh) and eluted by a petroleum ether-EtOAc mixture of increasing polarity (20: 1–3: 1). A total of 90 fractions of ca. 300 mL each were collected, concentrated and combined based on TLC to give five subfractions indexed A1 to A4. The subfraction A3 (1.9 g) was further subjected to column chromatography (4 × 30 cm) on silica gel (25 g, 70–230 mesh) and eluted with petroleum ether-EtOAc (18: 2) to yield compound 4 (8 mg, ≥98% purity). The subfraction A4 (19.2 g), after column chromatography (5 × 50 cm) on silica gel (350 g, 70–230 mesh), yielded a mixture of polyprenylated benzophenones. Fractions were then mixed again and submitted to LH-20 Sephadex column chromatography (1.5 × 150 cm), eluted with a mixture of DCM-MeOH (1:1) to afford compound 5 (13.5 mg, ≥95% purity) and 6 (24 mg, ≥98% purity).
Fraction B (20 g) was also subjected to column chromatography (5 × 100 cm) on silica gel (600 g, 230–400 mesh) and eluted with a petroleum ether-EtOAc mixture of increasing polarity (20: 1–3: 1). Fifty fractions of ca. 300 mL each were collected and regrouped into three subfractions, B1 to B3, based on their TLC profile. Subfraction B1 (1.8 g) was subjected to column chromatography (4 × 30 cm) on silica gel (20.0 g, 70–230 mesh) and eluted with a mixture of petroleum ether-EtOAc (17: 3) to give compound 3 (5.0 mg, ≥99% purity). Subfraction B2 (8.0 g) was subjected to column chromatography (4 × 30 cm) on silica gel (20 g, 70–230 mesh) and eluted with a mixture of petroleum ether-EtOAc (13: 7) to yield compounds 1 (25.0 mg, ≥97% purity). Subfraction B3 (2.6 g) was also subjected to column chromatography (4 × 30 cm) on silica gel (30 g, 70–230 mesh) and eluted with a mixture of petroleum ether- EtOAc (12: 8) to afford compounds 7 (15.0 mg, ≥98% purity) and 2 (22.5 mg, ≥98% purity).
Ananixanthone (1): pale colorless amorphous, mp 107-171 (MeOH); UV (MeOH) λmax (logε) 253.3 (4.19), 269.6 (4.19), 332.8 (3.74); UV (MeOH + NaOH) λmax (logε) 269.6 (4.23), 285 (4.13) sh, 348 (3.62); UV (MeOH + NaOAc) λmax (logε) 269.6 (4.27), 285 (4.15) sh, 338.3 (3.62); UV (MeOH + AlCl3) λmax (logε) unchanged. IR (KBr) νmax: 3377, 2919, 2852, 1648 cm−1 (Bayma et al., 1998).
Smeathxanthone A (2): Yellow crystals, mp. 216°C–218°C; UV (EtOH) λmax (logε): 408 (0.32), 338 (0.21), 337 (0.78), 297 (2.63), 223 (1.27), 205 (5.00); IR (KBr) νmax: 3315, 2891, 2350, 2200, 1962, 1869, 1579, 1440, 1290, 1193, 1084, 936, 822, 784 cm−1 (Komguem et al., 2005).
Smeathxanthone B (3): Yellow powder; mp. 187°C–189°C;
1,3,5,8-tetrahydroxy-2-(3-methybut-2-enyl)-4-(3,7-dimethyloct-2,6-dienyl) xanthone (4): yellow powder, mp 172°C–173°C; UV (MeOH) λmax (logε): 283 (4.6), 325 (4.2),350 (4.3) 400 (3.8) nm; IR (solid) νmax: 2919, 2851, 1627, 1617, 1580, 1485, 1463, 1314, 1217, 1176, 1099, 1007, 967, 830, 809, 719, 704, 618, 589 cm−1 (Fouotsa et al., 2015).
Guttiferone O (5): Yellow powder; mp: 103°C;
Guttiferone M (6): yellow oil;
Aristophenone A (7): yellow cubes (CHCl3); mp. 82°C;
2.2 Chemicals
Lipopolysaccharide (LPS) from Escherichia coli 0111:B4 was obtained from Sigma-Aldrich, Darmstadt, Germany. Penicillin/streptomycin/fungizone (PSF), Dulbecco’s modified Eagle’s medium (DMEM), and fetal calf serum (FCS) were purchased from Highveld Biological Products (South Africa). Whitehead Scientific (South Africa) provided trypsin and phosphate-buffered saline (PBS). 3-(4, 5-dimethylthiazol-2-yl)-2, 5-diphenyl-tetrazolium bromide (MTT) and quercetin were provided by Sigma-Aldrich St. Louis, MO, United States. Linoleic acid was purchased from Merck (Darmstadt), xylenol orange from Searle (England), and sodium carbonate from Holpro Analytic (South Africa). Tris (hydroxymethyl) aminomethane was purchased from Sigma, (Switzerland) while 15-lipoxygenase from Glycine max, ferrous sulfate, indomethacin, and sodium nitrite were obtained from Sigma (Germany).
2.3 Nitric oxide inhibitory activity in LPS-activated RAW 264.7 macrophages
2.3.1 Cell culture and maintenance
Murine macrophage RAW 264.7 cell line obtained from the American Type Culture Collection (Rockville, MD, United States) was maintained in DMEM supplemented with 10% fetal calf serum (FCS) and 1% penicillin/streptomycin/fungizone (PSF) under standard cell culture conditions at 37°C and 5% CO2 in a humidified environment.
2.3.2 Cytotoxicity assay
The cytotoxic effect of compounds on RAW 264.7 cells was assessed using the 3-(4, 5-dimethylthiazol-2-yl)-2, 5-diphenyl tetrazolium bromide (MTT) assay as established by Mosmann (Mosmann, 1983) with slight modifications. Briefly, cells were seeded at a density of 1 × 105 cells/mL (100 µL) in 96-well microtitre plates and incubated at 37°C and 5% CO2 in a humidified environment. After 24 h incubation, 100 µL of compounds (at concentration ranged from to 250 μM–1.95 µM) were added to the wells containing cells and further incubated for 48 h in a CO2 incubator. A suitable control (untreated cells) was included and doxorubicin was used as a positive reference. Thereafter, the medium in each well was aspirated from the cells, which were then washed with PBS, and finally fresh medium (200 µL) was added to each well. Then, 30 µL of MTT (5 mg/mL in PBS) was added to each well and the plates were incubated at 37°C for 4 h. The medium was aspirated from the wells and DMSO was added to solubilize the formed formazan crystals. The absorbance was read at 570 nm (SpectraMax 190, Molecular devices). The percentage of cell inhibition was calculated with reference to the control (untreated cells taken as 100% viability). Then, the IC50 values of plant compounds showing more than 50% cell growth inhibition were calculated by plotting the percentage inhibition against the concentration.
2.3.3 Inhibition of nitric oxide (NO) production in LPS-activated RAW 264.7 macrophages
The RAW 264.7 macrophage cells were seeded in 96 well-microtitre plates (2 × 105 cells/mL). They were activated by incubation in a medium containing 1 μg/mL LPS alone (control) or lipopolysaccharide, and compounds at concentration of 3.12 µM, 6.25 µM, 12.5 µM and 25 µM dissolved in DMSO. Quercetin served as a positive control NO inhibitor for the reduction of NO production. After 24h, the amount of nitric oxide released from macrophages was determined using the Griess reagent as previously described (Dzoyem and Eloff, 2015).
2.4 Soybean 15-LOX inhibition assay
The assay was performed according to a previously described procedure (Pinto et al., 2007) with slight modifications. The assay is based on measuring the formation of the complex Fe3+/xylenol orange in a spectrophotometer at 560 nm. 15-LOX from G. max was incubated with compounds or standard inhibitors at 25°C for 5 min. The concentration range of the samples were from 250 μM to 1.95 µM. Then linoleic acid (final concentration, 140 µM) in Tris-HCl buffer (50 mM, pH 7.4) was added and the mixture was incubated at 25°C for 20 min in the dark. The assay was terminated by the addition of 100 µL of FOX reagent consisting of sulfuric acid (30 mM), xylenol orange (100 µM), iron (II) sulfate (100 µM) in methanol/water (9:1). For the control, only LOX solution and buffer were pipetted into the wells. Blanks (background) contained the enzyme LOX during incubation, but the substrate (linoleic acid) was added after the FOX reagent. Quercetin was used as a reference compound for the inhibition of 15-LOX activity (Sadik et al., 2003). The LOX inhibitory activity was evaluated by calculating the percentage of the inhibition of hydroperoxide production from the changes in absorbance values at 560 nm after 30 min at 25°C. % inhibition = [(Acontrol – Ablank) – (Asample – Ablank)/(Acontrol – Ablank)] x100. Where Acontrol is the absorbance of control well, Ablank is the absorbance of blank well and Asample is the absorbance of sample well.
2.5 Evaluation of COX-1 and COX-2 activity
The fluorometric cyclooxygenase activity assay kit (Biovision) was used to evaluate the activity of COX-1 and COX-2 enzymes. Raw 264.7 cells were seeded at 2 × 105 cells/mL in a 48-well microplate, and allowed to adhere for 24 h, then treated with LPS 0.1 μg/mL and compounds (1), (5) and (6) at 20 µM against Raw 264.7 cells. After 24 h the cyclooxygenase enzyme (COX-1 and COX-2) activity assay was performed. Cells were detached with TNE buffer (Tris 40 mM, NaCl 150 mM, EDTA 1 mM) and washed with PBS (1x), then re-suspended in 1 mL PBS (1x), transferred into 1.5 mL tube, and centrifuged at 500 x g for 3 min. The pellet was then re-suspended in 0.5 mL of lysis buffer with a protease inhibitor cocktail, vortexed, and incubated at 4°C for 5 min. The cell lysate was centrifuged at 12000 x g for 3 min and the supernatant was collected for COX activity assay. The COX activity was assessed using the fluorometric cyclooxygenase activity assay kit (Biovision) following the manufacturer’s instructions. The assay includes COX-1 and COX-2 specific inhibitors to differentiate the activity of COX-1 and COX-2 as well as other peroxidases. Indomethacin (10 µM) was used as a standard drug and appropriate controls were also included.
2.6 Measurement of Th1/Th2 cytokines
Raw 264.7 cells were seeded at 2 × 105 cells/mL in a 48-well microplate and allowed to adhere for 24 h, then treated with LPS 0.1 μg/mL and with compounds (1), (5) and (6) at 20 µM. Untreated cells and indomethacin (10 µM) were used as controls. After 24 h of incubation, the supernatant was collected and the amount of pro-inflammatory Th1 cytokines (IFN-γ, TNF-α, and IL-2) and anti-inflammatory Th2 cytokines (IL-4, IL-6, and IL-10) released measured. The experiment was performed according to the manufacturer’s instructions using BD™ Cytometric Bead Array (CBA) Human Th1/Th2 Cytokine Kit (BD-Biosciences), and data were acquired on a BD LSR Fortessa™ cell analyzer flow cytometer.
2.7 Statistical analysis
The data are presented as the mean ± standard deviation (SD) of three independent experiments or triplicate (n = 3). Differences between the means of each group were assessed by a one-way analysis of variance followed by Sidak’s multiple comparisons test using GraphPad Prism 9.
3 Results
3.1 Isolation and structural elucidation of compounds
Extensive column chromatography on methanol (MeOH) extract of the stem bark of Garcinia Smeathmannii (Planch. & Triana) Oliv. using silica gel and Sephadex LH-20 led to the isolation of seven known compounds (1–7). Their structures were established through spectroscopic (1D, 2D NMR) data, spectrometric analysis (MS), and by comparison with literature data. Isolated compounds were identified by comparison of their spectroscopic data with literature values. They are Ananixanthone (1), Smeathxanthone A (2), Smeathxanthone B (3), 1,3,5,8-tetrahydroxy-2-(3-methybut-2-enyl)-4-(3,7-dimethyloct-2,6-dienyl) xanthone (4), Guttiferone O (5), Guttiferone M (6), Aristophenone A (7) (Figure 1).
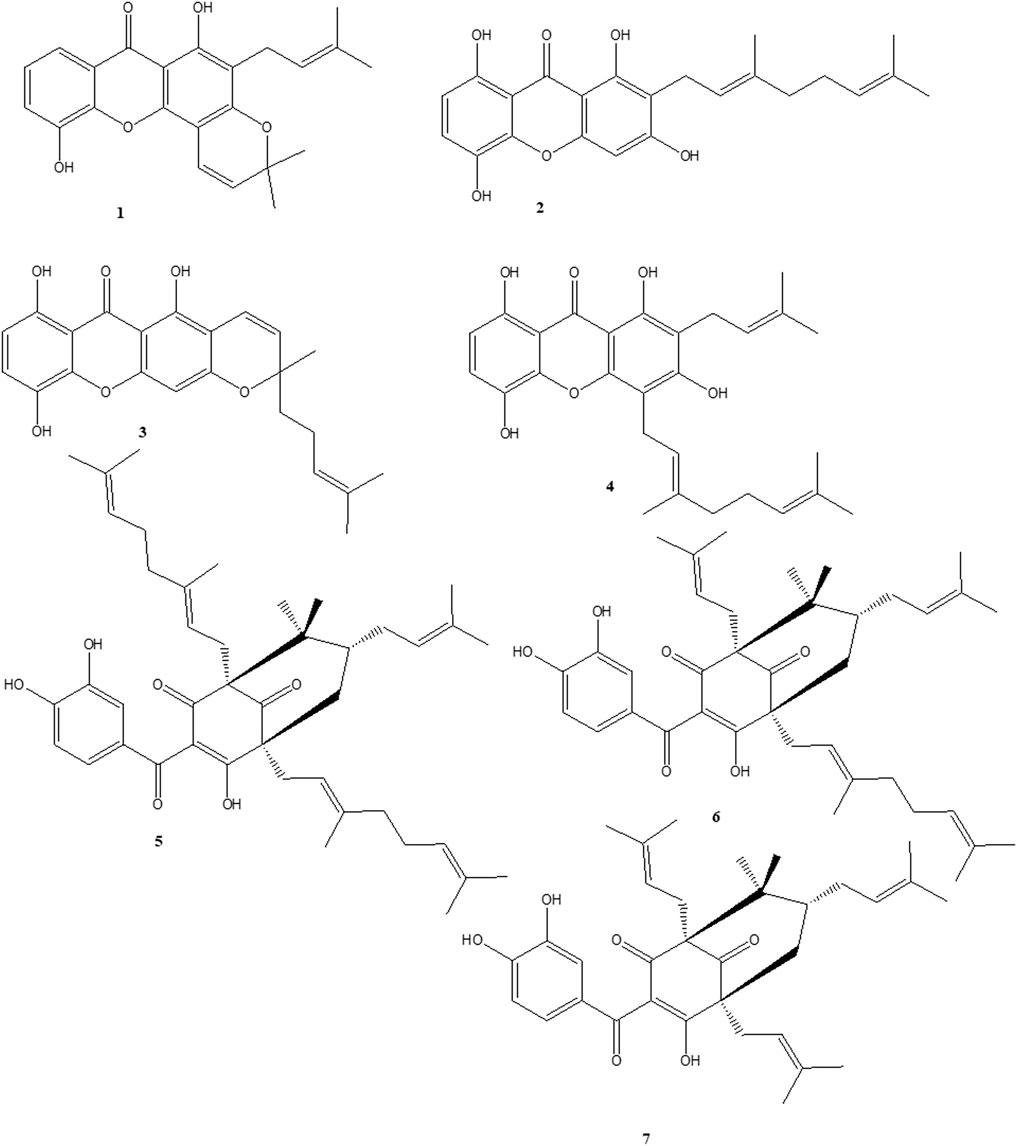
Figure 1. Chemical structures of naturally occurring benzophenones and xanthones. ananixanthone (1), smeathxanthone A (2), smeathxanthone B (3), 1,3,5,8-tetrahydroxy-2-(3-methybut-2-enyl)-4-(3,7-dimethyloct-2,6-dienyl) xanthone (4), guttiferone O (5), guttiferone M (6), and aristophenone A (7).
3.2 Cytotoxicity of compounds against RAW 264.7 macrophages
In order to assess the safety of the investigated compounds towards the RAW 264.7 cells and to select the sub-inhibitory concentration for further experiments, a cytotoxicity assay was conducted and the results are presented in Figure 2. It appears from this Figure 2 that, compounds (1), (3), and (7) exhibit relatively higher IC50 values. These values are above 100 μM, suggesting that they might have lesser cytotoxic effects on RAW264.7 cells compared to the other compounds. Compounds (5) and (6) have the lowest IC50 values (64.54 µM and 61.25 µM respectively), indicating greater potency in inhibiting cell growth or inducing cell death in RAW264.7 cells. Doxorubicin, a known chemotherapeutic drug with cytotoxic properties, serves as a reference. It exhibits an IC50 value of 4.63 µM, which is lower than all the compounds tested. Based on these data, sub-inhibitory concentration of 25 µM was chosen for NO assay.
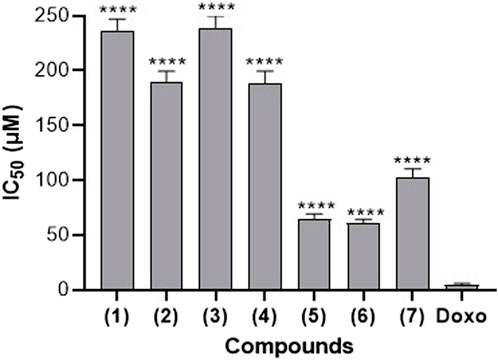
Figure 2. Cytotoxic effect of seven naturally occurring benzophenones and xanthones from Garcinia smeathmannii on RAW264.7 cells. Data are the mean from three independent experiments. Sidak’s multiple comparisons test using one-way ANOVA was performed: ∗∗∗∗p < 0.0001 for compounds versus reference drug doxorubicin (Doxo).
3.3 Nitric oxide (NO) production inhibition and cell viability
The ability of compounds obtained from G. smeathmannii, to inhibit NO production in the RAW 264.7 cell line after stimulation with lipopolysaccharide (LPS) was evaluated and results are shown in Figure 3. All the tested compounds exhibited a dose-dependent inhibition of NO production. At the highest concentration (25 µM), guttiferone O (5), and guttiferone M (6) exhibit the most potent inhibitory activities reducing the amount of NO produced from 6.08 µM in untreated and stimulated control to 0.3 µM and 0.54 µM respectively.
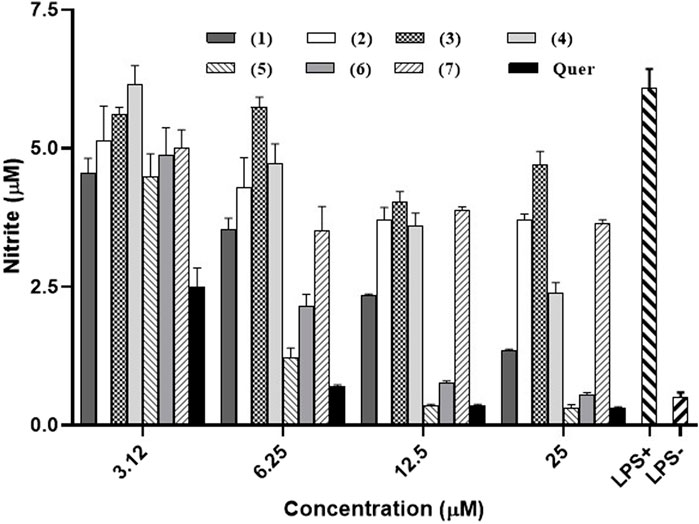
Figure 3. Inhibitory activities of seven naturally occurring benzophenones and xanthones from Garcinia smeathmannii on NO production in LPS-stimulated in RAW 264.7 macrophages. Quer: quercetin.
3.4 Inhibition of 15-lipoxygenase activity
Figure 4 presents the inhibitory effects of the seven naturally occurring benzophenones and xanthones on 15-Lipoxygenase (15-LOX) activity, expressed in terms of IC50 values. These results suggested that the evaluated compounds possess varying degrees of inhibitory effects on 15-LOX activity. The IC50 values obtained ranged between 45.01 μM and 160.73 μM. Ananixanthone (1), Guttiferone O (5) and Guttiferone M (6) demonstrated IC50 values of 63.91µM, 45.01 µM and 52.97 µM, respectively, which appears to be the most effective among the seven tested compounds, as compared to quercetin, a well-studied flavonoid, used as a positive control which showed an IC50 value of 64.35 µM.
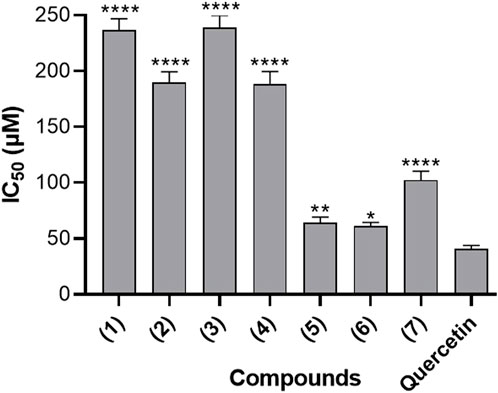
Figure 4. 15-Lipoxygenase inhibitory activity of seven naturally occurring benzophenones and xanthones from Garcinia smeathmannii. Data are the mean from three independent experiments. Sidak’s multiple comparisons test using one-way ANOVA was performed: ∗∗p < 0.01, ∗∗∗p < 0.001, and ∗∗∗∗p < 0.0001 for compounds versus reference compound quercetin (Quer).
3.5 Inhibition of cyclooxygenase enzyme activity
The results of the inhibitory effect of three selected naturally occurring benzophenones and xanthone on COX-1 & COX-2 enzyme activity are provided in Figure 5. A perusal of this figure reveals that all three compounds exhibited some levels of inhibition on COX-1/COX-2 activity. Compared to the untreated controls, all the compounds significantly inhibit the activity of COX-1 (p < 0.0001) reducing the activity of the enzyme from 85.19 µU/mg to 52.55 µU/mg, 32.28 µU/mg, and 5.13 µU/mg for compound (1), (5) and (6) respectively. Compound (6), guttiferone M displays the best inhibitory effect on COX-1 with a value (15.13 µU/mg). Likewise, all the compounds significantly inhibit the activity of COX-2 (p < 0.0001 for compounds (1) and (6), p < 0.01 for compound (5)). The activity of COX-2 was reduced from 99.85µU/mg in untreated control to 59.33 µU/mg, 88.10 µU/mg, and 43.84 µU/mg for compounds (1), (5) and (6) respectively.
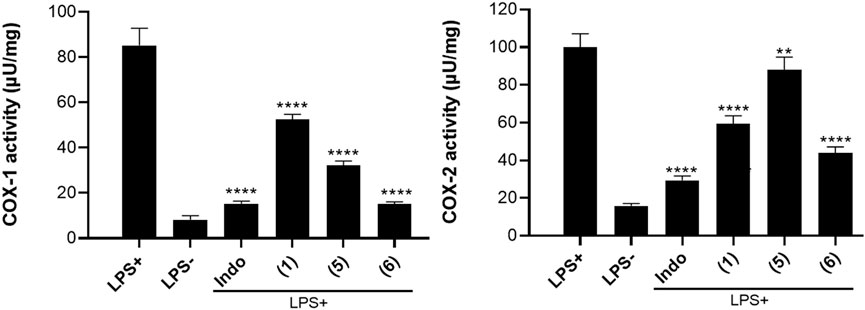
Figure 5. Results of COX-1 and COX-2 activity in LPS-stimulated MH-S cell lysate treated with compounds (1), (5) and (6) at 20 µM. The positive control indomethacin (Indo) was tested at 10 μM. One unit (U) of COX activity is the amount of enzyme that generates 1.0 μmol of resorufin per minute at pH 8.0°C and 25°C. Data are the mean of three independent experiments. Sidak’s multiple comparisons test using one-way ANOVA was performed: ∗∗p < 0.01, ∗∗∗p < 0.001, and ∗∗∗∗p < 0.0001 for compounds versus stimulated and untreated control.
3.6 Modulation of Th1/Th2 cytokines
In this work, the effects of compounds (1), (5), and (6) on the production of human Th1/Th2 cytokines were evaluated. The cytokines measured include pro-inflammatory Th1 cytokines (IFN-γ, TNF-α, IL-2, IL-6) and anti-inflammatory Th1 cytokines (IL-4, IL-10). Results presented in Figure 6 indicated that the compounds tested exhibit diverse effects on the production of the pro- and anti-inflammatory cytokines.
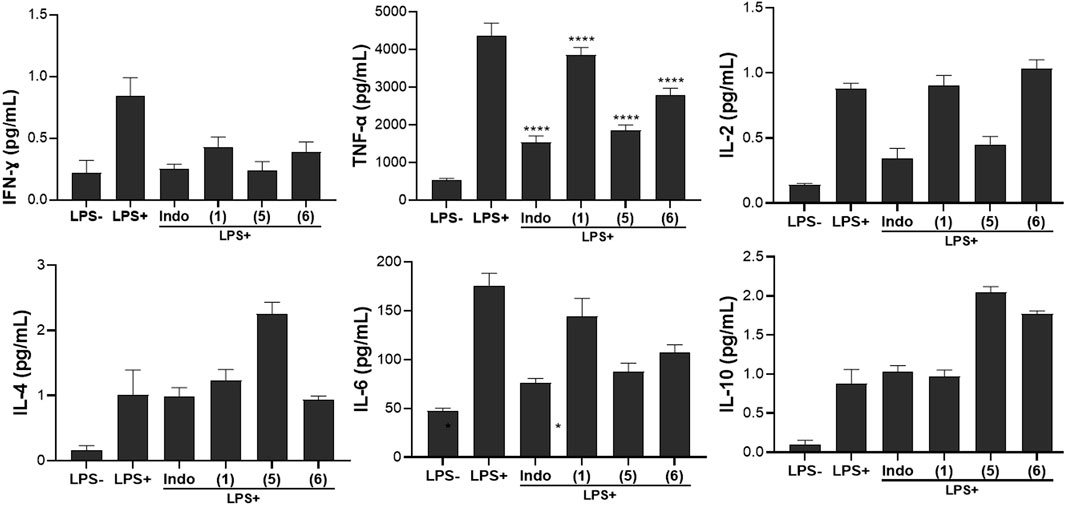
Figure 6. Effect of compounds (1), (5), and (6) on inflammatory cytokines production in LPS-stimulated Raw 264.7 macrophage cells. Cells were treated for 24 h with compounds (1), (5) and (6) at 20µM, then cytokines secretion levels were measured. Indomethacin (Indo) was tested at 10 µM. Values are the mean of experiments done in triplicate (n = 3) ± standard deviation. Statistical analysis was performed with Sidak’s multiple comparisons test using one-way ANOVA. ∗∗∗∗p < 0.0001 between the sample and stimulated and untreated control.
The stimulated and untreated control groups had different levels of pro-inflammatory cytokines viz, 0.84 pg/mL for IFN-γ, 4356.86 pg/mL for TNF-α, and 0.88 pg/mL for IL-2; while the levels of anti-inflammatory cytokines were 1.01 pg/mL, 175.96 pg/mL, and 0.88 pg/mL for IL-4, IL-6, and IL-10, respectively. Upon treatment, compound (1) indicated a moderate decrease in IFN-γ and IL-2 production (0.43 pg/mL and 0.90 pg/mL respectively) but had a minimal effect on TNF-α (3854.61 pg/mL) compared to the stimulated and untreated control. This demonstrated a potential anti-inflammatory effect, though not as pronounced as seen with indomethacin.
4 Discussion
Nitric oxide (NO) plays a pivotal role in the physiological process of inflammation and has been involved in endotoxin-induced tissue injury due to its overproduction (Goncharov, 2020). Therefore, the inhibition of NO production has been considered a target for anti-inflammatory therapeutics (Wong and Lerner, 2015). Many plants of the Garcinia genus containing benzophenones and xanthones as bioactive constituents have been traditionally used as anti-inflammatory agents (Espirito Santo et al., 2020). Among the xanthone derivatives tested, ananixanthone (1) shows a remarkable inhibitory effect at the highest concentration (25 µM), suggesting its potential anti-inflammatory activity. Previous studies have highlighted the ability of these compounds to modulate inflammatory pathways, including the inhibition of NO production (Dzoyem et al., 2015). A benzophenone derivative clusiacyclol A was shown to have a significant NO inhibitory activity in RAW 264.7 macrophage cells with over 85% inhibition without obvious cytotoxicity at a final concentration of 100 μM (Sukandar et al., 2023). Similarly, xanthones derivatives garcinoxanthones B and C significantly inhibited NO production with IC50 values of 11.3 μM and 18.0 μM respectively (Liu et al., 2016). In this study, the observed inhibitory effects suggest that compounds (1), (5), and (6), may interfere with the inflammatory cascade by suppressing NO production.
Lipoxygenase (LOX) and cyclooxygenase (COX) are the enzymes responsible for the conversion of arachidonic acid to eicosanoids which are essential to initiate the immunological response during inflammation (Mukhopadhyay et al., 2023). Targeting the dual inhibition of COX/LOX is a promising strategy to develop novel anti-inflammatory agents (Cheng et al., 2022; Rudrapal et al., 2023). In our continued search for novel, more efficacious, and safer anti-inflammatory agents having dual mechanisms that could prevent the release of both prostaglandins and leukotrienes, benzophenones and xanthones compounds herein investigated. The compounds were screened for their ability to inhibit the activity of 15-LOX, then the most potent were selected and tested for their effect on the inhibition of COX-1 and COX-2 activities. Although not exhaustive, the literature on benzophenones and xanthones compounds supports the idea that some of them exhibit anti-inflammatory properties through the inhibition of LOX enzymes (Yamashita et al., 1990; Santos et al., 2017; Lončarić et al., 2021). The observed IC50 values provide insights into the concentration at which these compounds exert their inhibitory effects, allowing for a better understanding of their potential therapeutic relevance. From these results, ananixanthone (1) guttiferone O (5) and guttiferone M (6) which appear as the most effective in inhibiting the activity of 15-LOX were selected emphasized the need for further investigation for their effect on the activity of COX enzymes. This finding is noteworthy as COX-2 is often upregulated in response to inflammatory stimuli, and its inhibition is a key target for anti-inflammatory drugs (Gandhi et al., 2017). Once more, compound (6) exhibited the most potent inhibitory effect on COX-2 suggesting its multitarget potential. Although no previous report focusing on the effect of compounds (1), (5) and (6) on COX enzyme activity is documented, the observed inhibitory activity on both COX-1 and COX-2 is consistent with the broader literature on benzophenones and xanthones derivatives. The benzophenones and xanthones group of compounds has been recognized for their anti-inflammatory potential, and the modulation of COX as the plausible mechanism underlying their effects (Miladiyah et al., 2017; Qiao et al., 2021; Dzoyem et al., 2022). The observation that compound (6) had comparable result with the indomethacin positive controls was encouraging, as it suggests the potential for developing novel anti-inflammatory agents. Moreover, some well-known NSAIDs such as ibuprofen and aspirin (Acetylsalicylic Acid) are non-selective inhibitors of cyclooxygenase (COX) by reducing the production of prostaglandins, which are mediators of inflammation and pain.
Cytokines are endogenous mediators that play an important role in the pathophysiology of the systemic inflammatory response (Ishii and Yoshida, 2010). The production of both pro- and anti-inflammatory cytokines is strictly controlled by complex feedback mechanisms (Kishen and Muralidharan, 2020). Pro-inflammatory cytokines are responsible for initiating an effective inflammatory process against pathogens, whereas their overproduction has been associated with harmful effects on the body (Cole et al., 2018). In contrast, anti-inflammatory cytokines are involved in down-regulating the exacerbated inflammatory response and maintaining homeostasis (Ishii and Yoshida, 2010; Muzamil et al., 2021). Cytokines are mainly produced by macrophages and lymphocytes. In this study we used RAW 264.77 cells as model for cytokines production after stimulation with lipopolysaccharide (LPS) from E. coli 0111:B4. The result obtained with compound (1) corroborated with the increase in the levels of the anti-inflammatory cytokine IL-4 (1.23 pg/mL). However, its effect was minimal towards IL-10 (0.971 pg/mL) and there was a reduction in IL-6 (144.25 pg/mL), indicating instead its potential modulation of pro-inflammatory responses. To the best of our knowledge, this is the first study reporting the effect of ananixanthone on cytokine production. However, several xanthone derivatives have been shown to regulate various inflammatory activities and signaling pathways in immune cells, especially macrophages (Ng and Chua, 2019; Feng et al., 2020). Another xanthone derivative, α-mangostin was shown to inhibit the production of interleukin (IL)-6 (Ibrahim et al., 2016). The results of the effect of the two benzophenone compounds tested showed that compound (5) significantly reduces the production of IFN-γ, TNF-α, and IL-2 cytokines, similar to the effect of Indomethacin. This suggests a strong anti-inflammatory impact, potentially inhibiting the inflammatory response induced by LPS. Compound (6) exhibited a moderate decrease in IFN-γ and TNF-α production but had a limited effect on IL-2 compared to the stimulated and untreated control, indicating a partial anti-inflammatory effect, with a more substantial impact on IFN-γ and TNF-α. A substantial increase in the production of both IL-4 (2.25 pg/mL) and IL-10 (2.05 pg/mL) upon treatment with compound (5) was observed, indicating a robust enhancement of anti-inflammatory cytokines. Compound (6) had a moderate effect on IL-4 (0.94 pg/mL) and IL-10 (1.77 pg/mL). A general trend of the tested compounds on cytokines profiles revealed that compound (5) showed a pronounced enhancement of anti-inflammatory cytokines (IL-4 and IL-10), suggesting a potent anti-inflammatory effect while compound (6) exhibits a moderate effect on anti-inflammatory cytokines. Previous studies reporting the anti-inflammatory effect of benzophenones via modulation of cytokines are very scarce. However, a study by Rachoń et al. showed that benzophenone-2 was able to shift the Th1/Th2 balance toward a Th2 response (lower IFN-γ production and higher IL-10) (Rachoń et al., 2006). Another study showed that a 4-aminobenzophenone derivative displayed potent inhibition against TNF-α and IL-1β in LPS-stimulated human peripheral blood mononuclear cells (Khanum et al., 2004). Another benzophenone derivative otogirinin A was reported to suppress TNF-α generation via blocking the phosphorylation of MAPK/JNK and degradation of IκBα (Huang et al., 2020). Overall, the results in Figure 6 highlight the modulatory effects of the three selected compounds on inflammatory cytokine production. The observed changes in cytokine levels indicate potential anti-inflammatory and modulatory properties, emphasizing the need for further studies to elucidate the underlying mechanisms and validate the therapeutic potential of these compounds in the context of inflammatory disorders.
5 Conclusion
In this study, we have shown that compounds (1), (5) and (6) exerted anti-inflammatory by inhibition of NO, inhibition of the activity LOX, COX-1, and COX-2, as well as by regulating the production of cytokines. These data contributed to the growing body of evidence supporting the anti-inflammatory potential of benzophenones and xanthones derivatives and the use of natural compounds in the development of novel anti-inflammatory therapeutics. Our findings further support the exploration of ananixanthone (1), guttiferone O (5), and guttiferone (6) as potential candidates for the development of anti-inflammatory drugs. Although this study lacks information on the mechanism underlying the inhibitory effects of psoralen on the synthesis of cyclooxygenase enzymes and the studied of other inflammatory cytokines. Results are promising and further studies, including in vivo experiments, exploration of the signaling pathways such as NF-κB and MAPKs, synergistic effects of psoralen with other isolated compounds, are needed to confirm the efficacy and safety of psoralen as an anti-inflammatory agent.
Data availability statement
The original contributions presented in the study are included in the article/Supplementary Material, further inquiries can be directed to the corresponding authors.
Author contributions
MJ: Conceptualization, Writing–original draft. HF: Conceptualization, Writing–original draft. OM: Writing–review and editing, Methodology, Writing–original draft. JA: Writing–original draft, Resources. SA: Writing–review and editing, Writing–original draft. MA: Software, Writing–original draft. AY: Formal Analysis, Writing–original draft. HE: Data curation, Writing–original draft. EB: Validation, Writing–original draft. NM: Methodology, Writing–original draft. YA: Validation, Writing–original draft. AA: Writing–original draft, Validation. AR: Investigation, Writing–original draft. MA: Formal Analysis, Writing–original draft. JD: Writing–review and editing, Supervision, Writing–original draft. AI: Writing–review and editing, Supervision, Writing–original draft, Project administration.
Funding
The author(s) declare that no financial support was received for the research, authorship, and/or publication of this article.
Acknowledgments
Authors are thankful to the Deanship of Graduate Studies and Scientific Research at the University of Bisha for supporting this work through the Fast-Track Research Support Program.
Conflict of interest
The authors declare that the research was conducted in the absence of any commercial or financial relationships that could be construed as a potential conflict of interest.
Publisher’s note
All claims expressed in this article are solely those of the authors and do not necessarily represent those of their affiliated organizations, or those of the publisher, the editors and the reviewers. Any product that may be evaluated in this article, or claim that may be made by its manufacturer, is not guaranteed or endorsed by the publisher.
References
Bakar, F. I. A., Bakar, M. F. A., Abdullah, N., Endrini, S., and Rahmat, A. (2018). A review of Malaysian medicinal plants with potential anti-inflammatory activity. Adv. Pharmacol. Sci. 2018, 8603602. doi:10.1155/2018/8603602
Bayma, J. C., Arruda, M. S., and Neto, M. S. (1998). A prenylated xanthone from the bark of symphonia globulifera. Phytochemistry 49, 1159–1160. doi:10.1016/S0031-9422(98)00085-5
Calhelha, R. C., Haddad, H., Ribeiro, L., Heleno, S. A., Carocho, M., and Barros, L. (2023). Inflammation: what’s there and what’s new? Appl. Sci. 13, 2312. doi:10.3390/app13042312
Chen, L., Deng, H., Cui, H., Fang, J., Zuo, Z., Deng, J., et al. (2018). Inflammatory responses and inflammation-associated diseases in organs. Oncotarget 9, 7204–7218. doi:10.18632/oncotarget.23208
Cheng, X., Kong, , Zhi, D., and Li, Y. (2022). Screening of dual targeted inhibitors of 5-lipoxygenase and cyclooxygenase-2 from Oroxylum indicum by off-line two-dimensional liquid chromatography coupled with mass spectrometry. Ind. Crops Prod. 186, 115243. doi:10.1016/j.indcrop.2022.115243
Cole, C. L., Kleckner, I. R., Jatoi, A., Schwarz, E. M., and Dunne, R. F. (2018). The role of systemic inflammation in cancer-associated muscle wasting and rationale for exercise as a therapeutic intervention. JCSM Clin. Rep. 3, 1–19. doi:10.17987/jcsm-cr.v3i2.65
Cuesta-Rubio, O., Padron, A., Velez Castro, H., Pizza, C., and Rastrelli, L. (2001). Aristophenones A and B. A new tautomeric pair of polyisoprenylated benzophenones from Garcinia aristata. J. Nat. Prod. 64, 973–975. doi:10.1021/np0100035
Cui, J., Shan, K., Yang, Q., Chen, W., Feng, N., and Chen, Y. Q. (2022). Eicosanoid production by macrophages during inflammation depends on the M1/M2 phenotype. Prostagl. Other Lipid Mediat 160, 106635. doi:10.1016/j.prostaglandins.2022.106635
Dzoyem, J. P., and Eloff, J. N. (2015). Anti-inflammatory, anticholinesterase and antioxidant activity of leaf extracts of twelve plants used traditionally to alleviate pain and inflammation in South Africa. J. Ethnopharmacol. 160, 194–201. doi:10.1016/j.jep.2014.11.034
Dzoyem, J. P., Lannang, A. M., Fouotsa, H., Mbazoa, C. D., Nkengfack, A. E., Sewald, N., et al. (2015). Anti-inflammatory activity of benzophenone and xanthone derivatives isolated from Garcinia (Clusiaceae) species. Phytochem. Lett. 14, 153–158. doi:10.1016/j.phytol.2015.10.003
Dzoyem, J. P., Pinnapireddy, S. R., Fouotsa, H., Brüßler, J., Runkel, F., and Bakowsky, U. (2022). Liposome-Encapsulated Bioactive Guttiferone e Exhibits Anti-Inflammatory Effect in Lipopolysaccharide-Stimulated MH-S Macrophages and Cytotoxicity against Human Cancer Cells. Mediat. Inflamm. 2022, 8886087. doi:10.1155/2022/8886087
Espirito Santo, B. L. S. do, Santana, L. F., Kato Junior, W. H., de Araújo, F. de O., Bogo, D., Freitas, K. de C., et al. (2020). Medicinal potential of Garcinia species and their compounds. Molecules 25, 4513. doi:10.3390/molecules25194513
Farah, R. I., Khatib, A. E., Abu Ziyad, H. J., Jiad, D. K., Al Qusous, L. R., Ababneh, A. J., et al. (2023). Pattern of use and awareness of side-effects of non-steroidal anti-inflammatory drugs in the Jordanian population. Ann. Med. 55, 2242248. doi:10.1080/07853890.2023.2242248
Feng, Z., Lu, X., Gan, L., Zhang, Q., and Lin, L. (2020). Xanthones, a promising anti-inflammatory scaffold: structure, activity, and drug likeness analysis. Molecules 25, 598. doi:10.3390/molecules25030598
Folquitto, L. R. S., De Souza, T. B., Januario, J. P., Nascimento, I. M., Brandão, B. T. V., Moreira, M. E. C., et al. (2022). Benzophenone Derivatives Showed Dual Anti-Inflammatory and Antiproliferative Activities by Inhibiting COX Enzymes and Promote Cyclin e Downregulation. J. Braz Chem. Soc. 33. doi:10.21577/0103-5053.20210154
Fouotsa, H., Lannang, A. M., Dzoyem, J. P., Tatsimo, S. J. N., Neumann, B., Mbazoa, C. D., et al. (2015). Antibacterial and antioxidant xanthones and benzophenone from garcinia smeathmannii. Planta Med. 81, 594–599. doi:10.1055/s-0035-1545841
Gandhi, J., Khera, L., Gaur, N., Paul, C., and Kaul, R. (2017). Role of modulator of inflammation cyclooxygenase-2 in gammaherpesvirus mediated tumorigenesis. Front. Microbiol. 8, 538. doi:10.3389/fmicb.2017.00538
Goncharov, N. P. (2020). Nitric oxide (NO): physiology and metabolism (lecture). Androl. i Genital’naa Hir. 21, 75–79. doi:10.17650/2070-9781-2020-21-3-75-79
Huang, C. Y., Chang, T. C., Wu, Y. J., Chen, Y., and Chen, J. J. (2020). Benzophenone and benzoylphloroglucinol derivatives from hypericum sampsonii with anti-inflammatory mechanism of otogirinin a. Molecules 25, 4463. doi:10.3390/molecules25194463
Ibrahim, M. Y., Hashim, N. M., Mariod, A. A., Mohan, S., Abdulla, M. A., Abdelwahab, S. I., et al. (2016). α-Mangostin from Garcinia mangostana Linn: an updated review of its pharmacological properties. Arab. J. Chem. 9, 317–329. doi:10.1016/j.arabjc.2014.02.011
Ishii, H., and Yoshida, M. (2010). Inflammatory cytokines. Nippon. rinsho Jpn. J. Clin. Med. 68, 819–822. doi:10.1046/j.1471-4159.2000.740457.x
Khanum, S. A., Shashikanth, S., and Deepak, A. V. (2004). Synthesis and anti-inflammatory activity of benzophenone analogues. Bioorg Chem. 32, 211–222. doi:10.1016/j.bioorg.2004.04.003
Kishen, A., and Muralidharan, N. P. (2020). Role of cytokines in inflammation. Int. J. Pharm. Res. 12. doi:10.31838/ijpr/2020.SP1.019
Kolliniati, O., Ieronymaki, E., Vergadi, E., and Tsatsanis, C. (2022). Metabolic regulation of macrophage activation. J. Innate Immun. 14, 51–68. doi:10.1159/000516780
Komguem, J., Meli, A. L., Manfouo, R. N., Lontsi, D., Ngounou, F. N., Kuete, V., et al. (2005). Xanthones from Garcinia smeathmannii (Oliver) and their antimicrobial activity. Phytochemistry 66, 1713–1717. doi:10.1016/j.phytochem.2005.03.010
Lannang, A. M., Louh, G. N., Biloa, B. M., Komguem, J., Mbazoa, C. D., Sondengam, B. L., et al. (2010). Cytotoxicity of natural compounds isolated from the seeds of garcinia afzelii. Planta Med. 76, 708–712. doi:10.1055/s-0029-1240627
Liu, Q., Li, D., Wang, A., Dong, Z., Yin, S., Zhang, Q., et al. (2016). Nitric oxide inhibitory xanthones from the pericarps of Garcinia mangostana. Phytochemistry 131, 115–123. doi:10.1016/j.phytochem.2016.08.007
Lončarić, M., Strelec, I., Moslavac, T., Šubarić, D., Pavić, V., and Molnar, M. (2021). Lipoxygenase inhibition by plant extracts. Biomolecules 11, 152. doi:10.3390/biom11020152
Malik, H. N., Jabeen, A., Ashraf, S., Haq, Z. U., Salar, U., Arshia, , et al. (2022). Benzophenone and coumarin derivatives as 3-CLPro inhibitors: targeting cytokine storm through in silico and in vitro approaches. J. Mol. Struct. 1265, 133478. doi:10.1016/j.molstruc.2022.133478
Marrocco, A., and Ortiz, L. A. (2022). Role of metabolic reprogramming in pro-inflammatory cytokine secretion from LPS or silica-activated macrophages. Front. Immunol. 13, 936167. doi:10.3389/fimmu.2022.936167
Masullo, M., Bassarello, C., Suzuki, H., Pizza, C., and Piacente, S. (2008). Polyisoprenylated benzophenones and an unusual polyisoprenylated tetracyclic xanthone from the fruits of Garcinia cambogia. J. Agric. Food Chem. 56, 5205–5210. doi:10.1021/jf800416j
Miladiyah, I., Jumina, J., Haryana, S. M., and Mustofa, M. (2017). In silico MOLECULAR DOCKING OF XANTHONE DERIVATIVES AS CYCLOOXYGENASE-2 INHIBITOR AGENTS. Int. J. Pharm. Pharm. Sci. 9, 98. doi:10.22159/ijpps.2017v9i3.15382
Mosmann, T. (1983). Rapid colorimetric assay for cellular growth and survival: application to proliferation and cytotoxicity assays. J. Immunol. Methods 65, 55–63. doi:10.1016/0022-1759(83)90303-4
Mukhopadhyay, N., Shukla, A., Makhal, P. N., and Kaki, V. R. (2023). Natural product-driven dual COX-LOX inhibitors: overview of recent studies on the development of novel anti-inflammatory agents. Heliyon 9, e14569. doi:10.1016/j.heliyon.2023.e14569
Muzamil, A., Tahir, H. M., Ali, S., Liaqat, I., Ali, A., and Summer, M. (2021). Inflammatory process and role of cytokines in inflammation: an overview. Punjab Univ. J. Zool. 36. doi:10.17582/JOURNAL.PUJZ/2021.36.2.237.252
Ng, I. J., and Chua, C. L. (2019). The potential of xanthones as a therapeutic option in macrophage-associated inflammatory diseases. Pharmacogn. Rev. 13, 28. doi:10.4103/phrev.phrev_25_18
Pinto, M. D. C., Tejeda, A., Duque, A. L., and Macías, P. (2007). Determination of lipoxygenase activity in plant extracts using a modified ferrous oxidation-xylenol orange assay. J. Agric. Food Chem. 55, 5956–5959. doi:10.1021/jf070537x
Qiao, Y., Qin, Y., Liu, L., Chen, X., Li, Y., and Li, Q. (2021). An investigation into the interaction between double hydroxide-based antioxidant benzophenone derivatives and cyclooxygenase 2. Molecules 26, 6622. doi:10.3390/molecules26216622
Rachoń, D., Rimoldi, G., and Wuttke, W. (2006). In vitro effects of benzophenone-2 and octyl-methoxycinnamate on the production of interferon-γ and interleukin-10 by murine splenocytes. Immunopharmacol. Immunotoxicol. 28, 501–510. doi:10.1080/08923970600927751
Rudrapal, M., Eltayeb, W. A., Rakshit, G., El-Arabey, A. A., Khan, J., Aldosari, S. M., et al. (2023). Dual synergistic inhibition of COX and LOX by potential chemicals from Indian daily spices investigated through detailed computational studies. Sci. Rep. 13, 8656. doi:10.1038/s41598-023-35161-0
Sadik, C. D., Sies, H., and Schewe, T. (2003). Inhibition of 15-lipoxygenases by flavonoids: structure-activity relations and mode of action. Biochem. Pharmacol. 65, 773–781. doi:10.1016/S0006-2952(02)01621-0
Saikia, R., Pathak, K., Das, A., Tayeng, D., Ahmad, M. Z., Das, J., et al. (2023). Design, QSAR methodology, synthesis and assessment of some structurally different xanthone derivatives as selective cox-2 inhibitors for their anti-inflammatory properties. Med. Chem. (Los Angeles) 20, 78–91. doi:10.2174/1573406419666230818092253
Santos, C. M. M., Ribeiro, D., Silva, A. M. S., and Fernandes, E. (2017). 2,3-Diarylxanthones as potential inhibitors of arachidonic acid metabolic pathways. Inflammation 40, 956–964. doi:10.1007/s10753-017-0540-6
Smith, W. L., Urade, Y., and Jakobsson, P. J. (2011). Enzymes of the cyclooxygenase pathways of prostanoid biosynthesis. Chem. Rev. 111, 5821–5865. doi:10.1021/cr2002992
Sukandar, E. R., Kaennakam, S., Wongsuwan, S., Chatwichien, J., Krobthong, S., Yingchutrakul, Y., et al. (2023). Schomburginones A‒J, geranylated benzophenones from the leaves of Garcinia schomburgkiana and their cytotoxic and anti-inflammatory activities. Phytochemistry 211, 113701. doi:10.1016/j.phytochem.2023.113701
Wong, V. C., and Lerner, E. (2015). Nitric oxide inhibition strategies. Futur Sci. OA 1. doi:10.4155/fso.15.35
Xue, Q., Chen, Y., Yin, H., Teng, H., Qin, R., Liu, H., et al. (2020). Prenylated xanthones and benzophenones from the fruits of Garcinia bracteata and their potential antiproliferative and anti-inflammatory activities. Bioorg Chem. 104, 104339. doi:10.1016/j.bioorg.2020.104339
Yamashita, A., Schaub, R. G., Bach, M. K., White, G. J., Toy, A., Ghazal, N. B., et al. (1990). 1,4-Dihydronaphthoquinones, hydroindoloquinones, benzofurans, and benzothiophenes as inhibitors of 5-lipoxygenase. Synthesis and structure-activity studies. J. Med. Chem. 33, 775–781. doi:10.1021/jm00164a050
Keywords: benzophenone, xanthone, nitric oxide, lipoxygenase, cyclooxygenase, cytokines
Citation: Jeelani M, Fouotsa H, Mohammed OA, Alfaifi J, Adebayo S, Ahmed MM, Yahia AIO, Eissa H, Bahashwan E, Mohammed NA, Alotaibi YA, Asiri AY, Rezigallah A, Alharthi MH, Dzoyem JP and Isa AI (2024) Naturally occurring benzophenones and xanthones from Garcinia smeathmannii (Planch. & Triana) Oliv. displayed anti-inflammatory effects by modulating the activities of inflammatory mediators in LPS-stimulated RAW 264.7 macrophages. Front. Pharmacol. 15:1370073. doi: 10.3389/fphar.2024.1370073
Received: 13 January 2024; Accepted: 13 May 2024;
Published: 03 June 2024.
Edited by:
Carmenza Spadafora, Instituto de Investigaciones Científicas y Servicios de Alta Tecnología, PanamaReviewed by:
Laiba Arshad, Forman Christian College, PakistanAhmed S. Doghish, Badr University in Cairo, Egypt
Copyright © 2024 Jeelani, Fouotsa, Mohammed, Alfaifi, Adebayo, Ahmed, Yahia, Eissa, Bahashwan, Mohammed, Alotaibi, Asiri, Rezigallah, Alharthi, Dzoyem and Isa. This is an open-access article distributed under the terms of the Creative Commons Attribution License (CC BY). The use, distribution or reproduction in other forums is permitted, provided the original author(s) and the copyright owner(s) are credited and that the original publication in this journal is cited, in accordance with accepted academic practice. No use, distribution or reproduction is permitted which does not comply with these terms.
*Correspondence: Adamu Imam Isa, aiisa@ub.edu.sa; Jean Paul Dzoyem, jpdzoyem@yahoo.fr