- 1Endocrine Physiology Research Center, Research Institute for Endocrine Sciences, Shahid Beheshti University of Medical Sciences, Tehran, Iran
- 2Cellular and Molecular Endocrine Research Center, Research Institute for Endocrine Sciences, Shahid Beheshti University of Medical Sciences, Tehran, Iran
- 3Department of Molecular, Cellular, and Biomedical Sciences, Sophie Davis School of Biomedical Education, City University of New York School of Medicine, New York, NY, United States
Introduction: Menopause is associated with reduced nitric oxide (NO) bioavailability and lower tolerance against myocardial ischemia-reperfusion (IR) injury. This study investigated whether long-term nitrate administration provides resistance against myocardial IR injury in ovariectomized (OVX) rats.
Method: After ovariectomy, female rats were assigned to the OVX and the OVX + nitrate groups (n = 14/group); the latter group consumed nitrate (100 mg/L) for 9 months. At month 9, each group was divided into two subgroups (n = 7/subgroup), of which one subgroup was exposed to myocardial IR (IR+ hearts) and the other was not exposed (IR− hearts). The hearts of rats were isolated, and NO metabolite (NOx), oxidative stress indices, and mRNA expressions of endothelial (eNOS), inducible (iNOS), and neuronal (nNOS) NO synthases, as well as markers of apoptosis, were measured in the IR− and IR+ hearts. In the IR+ hearts, cardiac function indices (CFI) and the infarct size were also measured.
Results: Nitrate increased catalase activity (97%) and eNOS expression (2.94-fold) in the IR− hearts. In the IR+ hearts, nitrate reduced left ventricular (LV) end-diastolic pressure (11.6%) and infarct size (26.2%) and increased recovery of LV developed pressure (44.0%) and peak rate of positive (28.9%) and negative (15.4%) changes in LV pressure. In addition, in the IR+ hearts, nitrate increased eNOS and B-cell lymphoma-2 (Bcl-2) as well as decreased iNOS, Bcl-2 associated X protein (Bax), caspase-3, caspase-8, caspase-9, and tumor necrosis factor-α (TNF-α) expression. Nitrate increased total antioxidant capacity (TAC) and catalase (CAT) activity and decreased malondialdehyde (MDA) levels at month nine in serum and IR+ hearts.
Conclusion: The favorable effects of nitrate against IR injury were associated with higher eNOS and Bcl-2 expression, CAT activity, TAC, and lower iNOS, Bax, caspase-3, caspase-8, caspase-9 and TNF-α expression, and MDA in the heart tissue. Nitrate preconditioning alleviated IR-induced myocardial injury in OVX rats; this effect was associated with eNOS upregulation before IR and the blunting of OVX-induced eNOS downregulation, iNOS upregulation, apoptosis, and oxidative stress in heart tissue after IR.
1 Introduction
Ischemic heart disease (IHD) is a leading cause of death globally (NCD Countdown collaborators, 2020) and is responsible for 9.14 million deaths in 2019, registering a 60% increase from 1990 (Safiri et al., 2022). The risk of IHD is 30% for premenopausal women (Lloyd-Jones et al., 1999), which increases after menopause by 200%–400% (Costello et al., 2017). Surgical menopause is a leading cause of early menopause in women (Faubion et al., 2015), and compared to natural menopause, it increases the risk of IHD by 50% (Muka et al., 2016). Hormone replacement therapy (HRT) is the first-line treatment in women with surgical menopause; however, the frequency of HRT decreased from ∼90% before 2002 to 10%–50% in 2020 (Marjoribanks et al., 2012; Sarrel et al., 2016; Garg et al., 2020) due to its possible side effects.
Reduced tolerance against myocardial ischemia-reperfusion (IR) injury and increased infarct size by 45%–75% has been reported in ovariectomized (OVX) rats following 2 (Kaya and Agan, 2022), 6 (Garvin et al., 2017), 12 (Kumar et al., 2021), and 44 (Yousefzadeh and Jeddi, 2023) weeks after ovariectomy. We previously reported that these effects of OVX in heart tissue are at least in part associated with reduced nitric oxide (NO) levels, reduced endothelial NO synthase (eNOS) expression, and increased inducible NOS (iNOS) expression (Yousefzadeh and Jeddi, 2023). In addition, in heart tissue of OVX rats, increased activation of Fas-receptor-dependent extrinsic pathway of apoptosis, including Fas ligand, caspase-3, caspase-8 and tumor necrosis factor-α (TNF-α), as well as mitochondrion-dependent intrinsic pathway of apoptosis, including Bcl-2 associated X protein (Bax), B-cell lymphoma 2 (Bcl-2), cytochrome c, caspase-3, and caspase-9 has been reported (Lee et al., 2008). Dietary nitrate and nitrite can boost NO in NOS-disrupted conditions (Lundberg et al., 2015) and reduce IHD risk by 21% (Bryan, 2017). In support, in male mice, a low-nitrite diet for 1 week exacerbates myocardial IR injury (Bryan et al., 2007), and in humans, consuming one fruit/vegetable serving per day as a source of inorganic nitrate reduces the risk of coronary heart disease by 4% (Joshipura et al., 2001). In addition, nitrate may also have estradiol-like effects in heart tissue; following conversion from nitrate, nitrite directly binds to the ligand-binding domain of estrogen receptor alpha (ERα) (Veselik et al., 2008), while NO S-nitrosylates ERα (Garbán et al., 2005). These actions mediate and restore the favorable effects of estradiol in heart tissue (Caulin-Glaser et al., 1997).
The protective effects of nitrate against myocardial IR have been studied in the short-term (only 1 week before ischemia) and in male rodents (Bryan et al., 2007; Salloum et al., 2015). Only two studies from our laboratory addressed the favorable long-term effects of nitrate against myocardial IR for 2 (Jeddi et al., 2016) and 9 (Yassaghi et al., 2023b) months in male diabetic (Jeddi et al., 2016) and normal female (Yassaghi et al., 2023b) rats. Time-dependent effects of nitrate in OVX rats have been reported previously; for example, glucose-lowering and anti-osteoporotic effects of nitrate have been observed only after 6 months of intervention (Yousefzadeh et al., 2022b; Yousefzadeh et al., 2022c). In addition, compared to males, following ischemia, females have reduced myocardial IR injury (Besík et al., 2007) and infarct size (Bae and Zhang, 2005), as well as higher eNOS and neural NOS (nNOS) expression (Sun et al., 2006) and lower apoptosis (Chen et al., 2013; Medzikovic et al., 2023) in heart tissue. Therefore, the current study was designed to investigate whether the long-term (9 months) nitrate administration provides resistance against myocardial IR injury in OVX rats.
2 Methods
2.1 Ethical approval
Following the principles of the 3Rs (Replacement, Reduction, and Refinement), to minimize the number of rats used in the study, isolated hearts and serum were obtained from an earlier study in which we reported that long-term nitrate administration improved carbohydrate metabolism in OVX rats (Yousefzadeh et al., 2022c). The present study focused on the effect of nitrate administration on myocardial IR injury in OVX rats. All experiments in this study were conducted according to the published guidelines for the care and use of laboratory animals in Iran (Ahmadi-Noorbakhsh et al., 2021) and were reported following ARRIVE guidelines (Percie du Sert et al., 2020). The Research Institute for Endocrine Sciences ethics committee, affiliated with Shahid Beheshti University of Medical Sciences, approved all experimental procedures of the present study (Ethic Code: IR. SBMU.ENDOCRINE.REC.1402.084).
2.2 Study protocol
This experimental interventional study was conducted in OVX rats (6-month-old, 200–215 g) maintained under standardized conditions with unrestricted access to a standard diet and drinking water. The study protocol is presented in Figure 1. A total of 28 rats were randomly assigned to two groups (n = 14/group): the OVX group, which received tap water, and the OVX + nitrate (OVX + N) group, which consumed sodium nitrate (100 mg/L) in tap water for 9 months. The period of study (9 months) was chosen for two reasons. First, previous studies have shown that long-term periods (>6 months) are needed to observe the adverse effects of ovariectomy on heart function (Shinmura et al., 2008; Tang et al., 2016; Yousefzadeh et al., 2022a). Second, long-term administration is necessary to observe the potential protective effects of nitrates in OVX rats, including glucose-lowering and anti-osteoporotic effects, as indicated by previous studies (Yousefzadeh et al., 2022b; Yousefzadeh et al., 2022c). At the end of the intervention, both groups were further subdivided into two subgroups (n = 7/subgroup), with the hearts of one subgroup subjected to IR (IR+ hearts) and the other subgroup left untreated (IR− hearts).
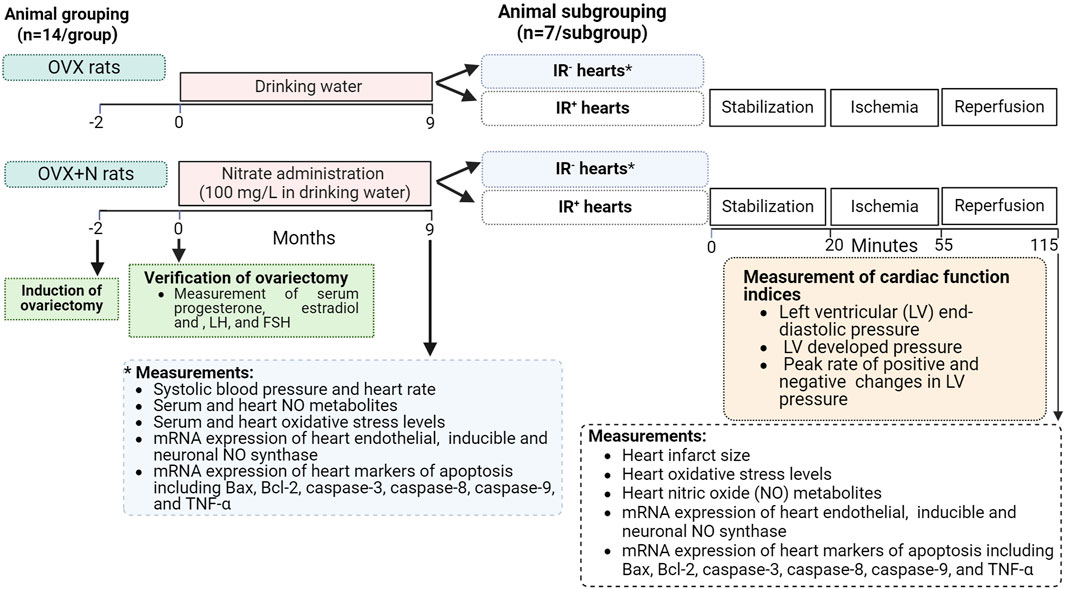
Figure 1. Study protocol. Bax, Bcl-2 associated X protein; Bcl-2, B-cell lymphoma-2; FSH, follicle-stimulating hormone; IR+, exposed to ischemia-reperfusion; IR−, not-exposed to ischemia-reperfusion; LH, luteinizing hormone; OVX, ovariectomized; OVX + N, OVX + nitrate; TNF-α, tumor necrosis factor-α.
Body weight, systolic blood pressure (SBP), and heart rate (HR) were measured at the end of the study (month 9). Blood samples were taken from the tail tips of all rats under isoflurane inhalation anesthesia at month 9, centrifuged (3,000 g for 10 min), and sera were used to measure progesterone, estradiol, follicle-stimulating (FSH), and luteinizing hormone (LH) as well as oxidative stress indices. In addition, the hearts of all rats were isolated under sodium pentobarbital anesthesia (60 mg/kg, intraperitoneal injection). Nitric oxide metabolite (NOx) concentration, oxidative stress indices, and mRNA expressions of eNOS, iNOS, and nNOS, as well as Bax, Bcl-2, caspase-3, caspase-8, and caspase-9, as well as TNF-α were measured in IR− and IR+ hearts. In IR+ hearts, cardiac function indices (CFI) and infarct size were also measured in addition to these parameters.
2.3 Measurement of parameters for verification of ovariectomy
Following an overnight fast, rats (200–215 g body weight) were anesthetized with sodium pentobarbital (60 mg/kg, intraperitoneally), and the ovaries were removed via a dorsolateral skin incision method, which has been described in detail in our previous publication (Yousefzadeh et al., 2020). To confirm the ovariectomy, serum progesterone, estradiol, FSH, and LH levels were measured 2 months after ovariectomy at month 0. The sensitivities of the assay kits were 0.1 ng/mL for progesterone, 10 pg/mL for estradiol, 0.7 mIU/mL for FSH, and 0.15 mIU/mL for LH. The kits for measuring progesterone and estradiol were obtained from Diagnostics Biochem (Ontario, Canada), and those for measuring FSH and LH were obtained from Cusabio Biotech (Wuhan, China). The intra-assay coefficients of variation (CVs) were less than 7% for all assays.
2.4 Measurement of SBP and HR
At month 9, rats were individually placed in a restrainer, and the noninvasive tail-cuff method (AD Instruments, MLT125R, Australia) was used to assess in vivo SBP and HR. For each rat, SBP and HR values were averaged from three consecutive measurements.
2.5 Isolated heart and assessment of CFI
The isolation of the heart and assessment of CFI were described in detail in our previous publication (Yassaghi et al., 2023b). In brief, at month 9, OVX rats were anesthetized with sodium pentobarbital (60 mg/kg, intraperitoneal injection), and hearts were surgically removed. The isolated hearts were immediately immersed in ice-cold, carbogenated Krebs-Henseleit solution (composition in mM: 118.6 NaCl; 4.7 KCl; 2.5 CaCl2; 1.6 MgSO4; 1.2 KH2PO4; 25 NaHCO3; 11.1 glucose - all from Merck, Darmstadt, Germany) to minimize ischemic damage and after that cannulated in Langendorff apparatus. Aortic cannulation enabled retrograde Langendorff perfusion in isolated hearts. After being inserted into the LV, a deflated LV balloon was inflated to 5–10 mm Hg and connected to a pressure transducer (MLT844-Sweden) via a water-filled PE-50 tube. The transducer was coupled with a PowerLab system (AD Instruments, ML866, Australia) to record CFI parameters. CFI included left ventricular end-diastolic pressure (LVEDP), LV systolic pressure (LVSP), LV developed pressure (LVDP, obtained by subtracting the LVEDP from the LVSP), and peak rate of positive (+dp/dt) and negative (-dp/dt) changes in LV pressure. CFI was recorded during stabilization (20 min), global ischemia (35 min), and reperfusion (60 min) periods. After a 20-min stabilization, hearts underwent 35 min of no-flow global ischemia, induced by interrupting Krebs-Henseleit perfusion to the cannulated isolated heart and confirmed by the cessation of coronary effluent. Following the IR period, the hearts were removed from the Langendorff apparatus and stored at −80°C for future examinations.
2.6 Measurement of heart NO metabolites
At the end of the study, IR− hearts and IR+ hearts were homogenized in phosphate-buffered saline (500 µL) and then centrifuged (10 min at 10,000 g); NOx and nitrite in all heart homogenates were then measured by the modified Griess method (Miranda et al., 2001) that reported previously in detail (Yousefzadeh et al., 2023). In brief, for deproteinization, zinc sulfate (10 μL, 15 mg/mL) and NaOH (10 μL, 3.72 M) were added to each sample (100 mg of tissue in 500 µL phosphate-buffered saline), centrifuged at 10,000 g for 10 min, and supernatants were used for NOx measurements. To measure NOx concentrations, nitrate was converted to nitrite by adding vanadium trichloride (8 mg/mL in 1 M HCl), after which N-(1-naphthyl) ethylenediamine (0.1% in ddH2O) and sulfanilamide (2% in 5% HCl) were added, and samples were incubated for 30 min at 37°C. The optical density was read at 540 nm. Nitrite was measured similarly, except that 1 M HCl was added to the samples to replace vanadium trichloride. The nitrate concentration in heart samples was determined by subtracting nitrite from NOx concentrations. The Bradford method was used to measure protein concentration in the samples (Bradford, 1976). NOx and nitrite levels are expressed as per mg protein, and intra-assay CVs of NOx and nitrite in heart tissue were less than 4%.
2.7 Measurement of infarct size
After the IR period, the triphenyl tetrazolium chloride (TTC) method was used to assess infarct size in isolated IR+ hearts. In brief, the iced hearts were meticulously sliced into thin segments, incubated in 1% TTC (in phosphate buffer solution) at 37°C for 10 min, and embedded in 10% formalin for 24 h (Ghanbari et al., 2015). This procedure vividly differentiated viable (red-stained) areas from necrotic (gray-stained) regions. The sliced heart sections were photographed, analyzed using ImageJ software, and represented as a percentage of the total area.
2.8 Measurement of mRNA expression
The sequences of the primers are provided in Table 1. The detailed protocols for RNA extraction, cDNA synthesis, and amplification have been previously described (Yousefzadeh et al., 2023). For RNA extraction, the RNX-Plus solution kit (Cinagen Co., Tehran, Iran) was used. For cDNA synthesis, the SMOBiO Technology cDNA synthesis kit (Taiwan) was used. To amplify cDNA, the Ampliqon SYBR Green Master Mix (Ampliqon Company, Denmark) was utilized using a real-time PCR machine (Rotor-Gene 6000; Corbett Life Science, Australia).
2.9 Measurement of heart oxidative stress indices
Details on the measurement of serum and tissue oxidative stress indices, including total antioxidant capacity (TAC), catalase (CAT) activity, and malondialdehyde (MDA) levels, have been previously reported (Yousefzadeh et al., 2022b). In brief, TAC was measured by the ferric-reducing antioxidant power (FRAP) assay, which involves incubating the sample with a ferric-tripyridyltriazine complex and measuring the reduction of the complex to the ferrous form. CAT activity was measured by the Hadwan method, which involves incubating the sample with hydrogen peroxide and measuring the reduction of dichromate acetic acid. MDA levels were measured by the Satoh method, which involves extracting the MDA from the sample, converting it to a pink-colored complex, and measuring the absorbance of the complex. Intra-assay CVs for TAC, CAT, and MDA were less than 5%.
2.10 Statistical analyses
Data analysis was performed using GraphPad Prism software. Mean ± SEM was used for all parameters except mRNA expressions, which were presented as relative fold changes. The Student’s t-test compared parameters, including, SBP, HR, infarct size, and oxidative stress indices. The paired t-test compared body weight, serum progesterone and estradiol, FSH, and LH concentrations. One-way ANOVA followed by the Bonferroni post hoc test was used to compare nitrite, nitrate, and NOx levels. To compare CFI in IR+ hearts at different time points, two-way mixed (between-within) ANOVA was applied, followed by the Bonferroni post-hoc test. Relative gene expression was calculated using REST software. p-values <0.05 were considered statistically significant.
3 Results
3.1 Verification of ovariectomy
Compared to before ovariectomy (month −2), 2 months after ovariectomy (month 0), OVX rats had a lower concentration of progesterone by 74% (p < 0.001) and estradiol by 64% (p = 0.005) and a higher concentration of FSH by 6-fold (p = 0.011) and LH by 28-fold (p = 0.014) in serum (Table 2). In addition, OVX rats had higher body weight at month 0 by 21% (250.1 ± 5.4 vs 206.0 ± 2.3, p < 0.001). All of these changes confirm the inducing of the OVX rat model at month 0 before nitrate intervention.

Table 2. Effect of ovariectomy on serum estradiol, progesterone, luteinizing hormone, and follicle-stimulating hormone in rats.
3.2 Effect of nitrate administration on heart function and SBP
Nitrate administration for 9 months to OVX rats did not affect baseline heart function [LVEDP (8.1 ± 0.7 vs 7.1 ± 0.4 mm Hg), LVSP (83.8 ± 3.9 vs 81.8 ± 3.3 mm Hg), LVDP (75.7 ± 4.3 vs 74.8 ± 3.4 mm Hg), +dp/dt (2048 ± 108 vs 2076 ± 152 mm Hg/s), and–dp/dt (1555 ± 98 vs 1708 ± 84 mm Hg/s)]. However, as shown in Figure 2, when subjected to ischemia, hearts from OVX + N rats showed decreased LVEDP (p < 0.001) and increased recovery of LVSP, LVDP, +dp/dt, and–dp/dt (all p < 0.001) during IR period. These results indicate that nitrate exerts protective effects against impaired heart function in OVX rats after IR but had no positive effect before IR.
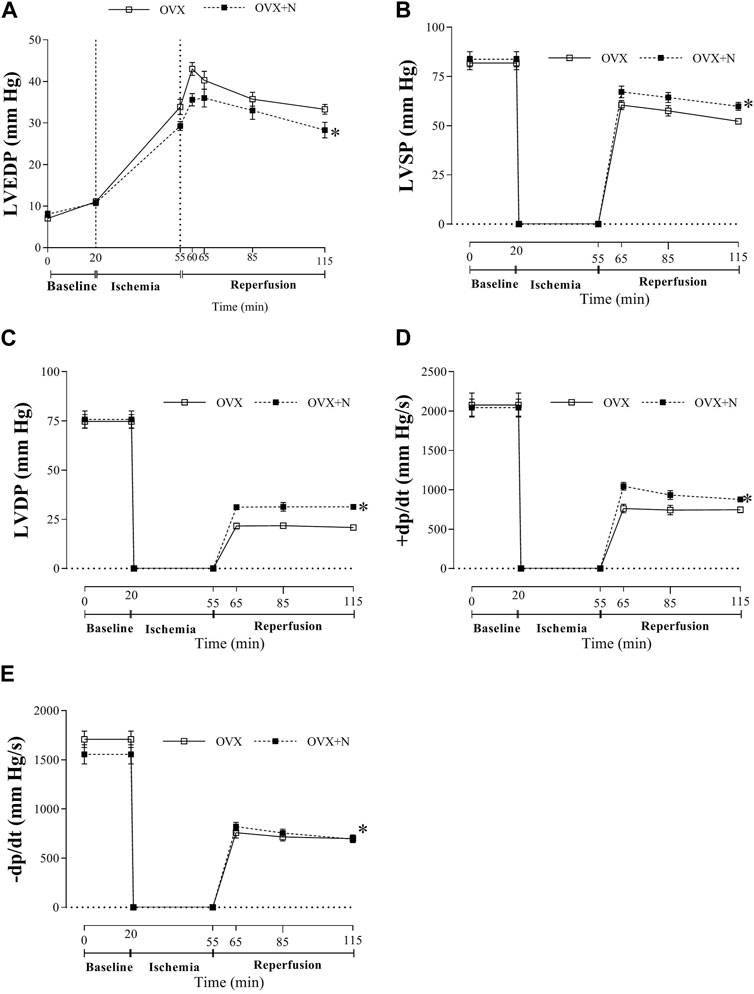
Figure 2. Tolerance to myocardial ischemia-reperfusion injury following nitrate administration in ovariectomized (OVX) rats as measured by left ventricular end-diastolic pressure (LVEDP, (A), LV systolic pressure (LVSP, (B), LV developed pressure (LVDP, (C), peak rate of positive (+dp/dt, (D) and negative (-dp/dt, (E) changes in LV pressure. (n = 7/subgroup). OVX, ovariectomized; OVX + N, OVX + nitrate. * Significant difference compared to OVX rats.
In addition, nitrate administration decreased in vivo SBP (111 ± 6 vs 126 ± 3, p = 0.040) but did not affect in vivo HR (318 ± 19 vs 322 ± 14) in OVX rats.
3.3 Effect of nitrate administration on NO metabolites
Nitrate administration in OVX rats increased nitrite (142%, p < 0.001), nitrate (41%, p = 0.043), and NOx concentrations (91%, p < 0.001) in IR− hearts. Exposed to IR increased nitrite (389%, p = 0.002), nitrate (175%, p < 0.001), and NOx (274%, p = 0.002) levels in OVX rats. Nitrate administration in OVX rats decreased nitrite (40%, p < 0.001), nitrate (43%, p = 0.027), and NOx (35%, p = 0.004) levels in IR+ hearts (Figure 3).
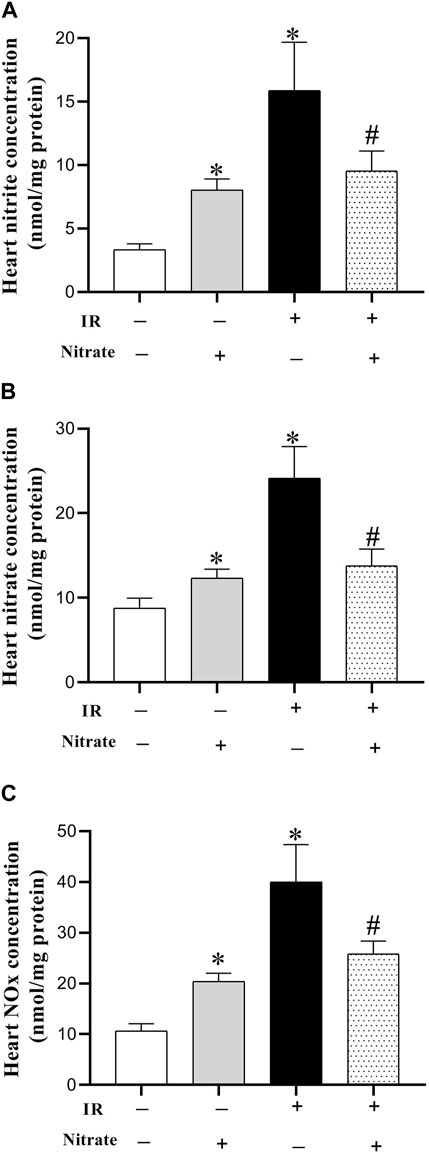
Figure 3. Concentration of nitrite (A), nitrate (B), and nitric oxide (NO) metabolites (NOx) (C), at month nine in not-exposed (IR−) and exposed to ischemia-reperfusion (IR+) heart in ovariectomized rats. Results are mean ± SEM (n = 7/group). *, and # significant difference compared to IR− and IR+ hearts from OVX rats, respectively.
3.4 Effect of nitrate administration on mRNA expression of NOS enzymes and marker of apoptosis
As shown in Figure 4, nitrate administration to OVX rats increased eNOS (2.94-fold, p < 0.001), did not affect iNOS, and decreased nNOS (42%, p = 0.027) expressions in IR− hearts. Exposed to IR in OVX rats, decreased eNOS (81%, p = 0.038), increased iNOS (5.82-fold, p < 0.001), and did not affect nNOS expressions. While nitrate administration to OVX rats increased eNOS (p = 0.004), decreased iNOS (p < 0.001), and did not affect nNOS mRNA expression in IR+ hearts; however, the eNOS expression remained lower by 18% (p = 0.077), and iNOS expression remained higher by 186% (p < 0.001) compared to IR− hearts.
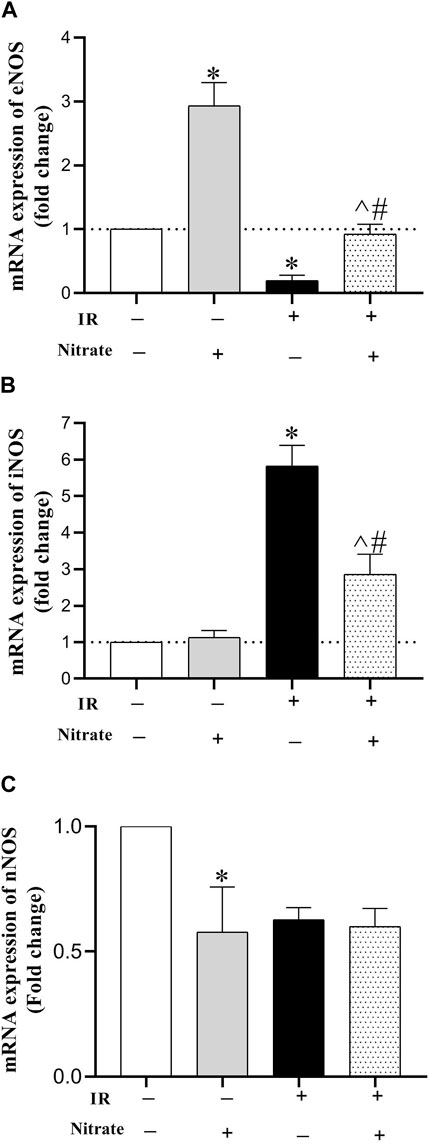
Figure 4. mRNA expression of the endothelial NO synthesis (eNOS) (A), inducible NOS (iNOS) (B) and neural NOS (C) at month nine in not-exposed (IR−) and exposed to ischemia-reperfusion (IR+) heart in ovariectomized rats. (n = 7/group).*, #, and ^ significant difference compared to IR− and IR+ hearts from OVX rats and IR− hearts from OVX + N rats, respectively.
As shown in Figure 5, nitrate administration to OVX rats did not affect the expression of Bax, Bcl-2, caspase-3, caspase −8, caspase-9, and TNF-α in IR− hearts. Exposed to IR in OVX rats increased Bax (319%, p < 0.001), caspase-3 (251%, p < 0.001), caspase-8 (375%, p < 0.001), caspase-9 (380%, p < 0.001), and TNF-α (759%, p < 0.001) expression. Nitrate administration decreased Bax (p < 0.001), caspase-3 (p < 0.001), caspase-8 (p = 0.003), caspase-9 (p = 0.003), and TNF-α (p = 0.011) expression, while increased Bcl-2 expression (p = 0.002) in IR+ hearts. However, the expression of Bax, caspase-3, caspase-8, and TNF-α remained higher by 67%, (p = 0.041), 47%, (p = 0.032), 144%, (p < 0.001) and 349% (p < 0.001) compared to IR− hearts. These results indicate the anti-apoptotic effect of nitrate administration in IR+ hearts from OVX rats.
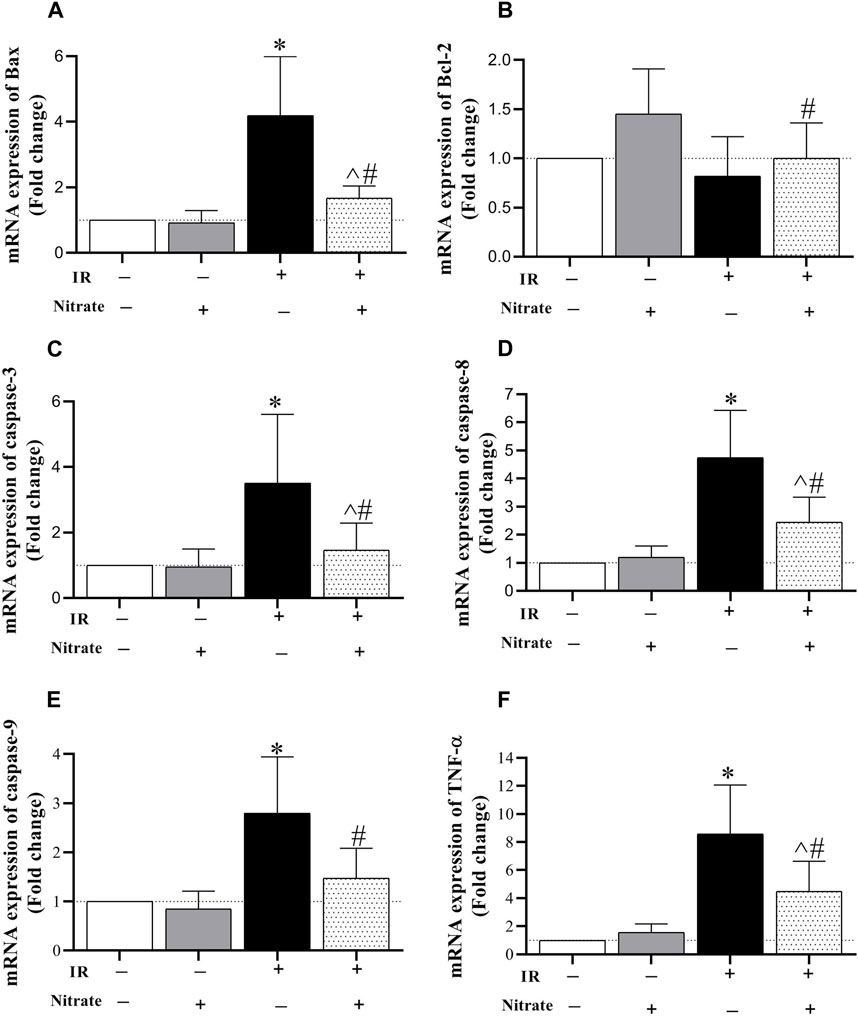
Figure 5. mRNA expression of the Bcl-2 associated X protein (Bax, (A), B-cell lymphoma 2 (Bcl-2, (B), caspase-3 (C), caspase-8 (D), caspase-9 (E), and tumor necrosis factor-α (TNF-α, (F) expression at month nine in not-exposed (IR−) and exposed to ischemia-reperfusion (IR+) heart in ovariectomized rats. (n = 7/group).*, #, and ^ significant difference compared to IR− and IR+ hearts from OVX rats and IR− hearts from OVX + N rats, respectively.
3.5 Effect of nitrate administration on infarct size levels
Compared to IR− hearts from OVX rats, nitrate administration decreased infarct size by 26.2% (p < 0.001) in IR+ hearts from OVX + N rats (Figure 6).
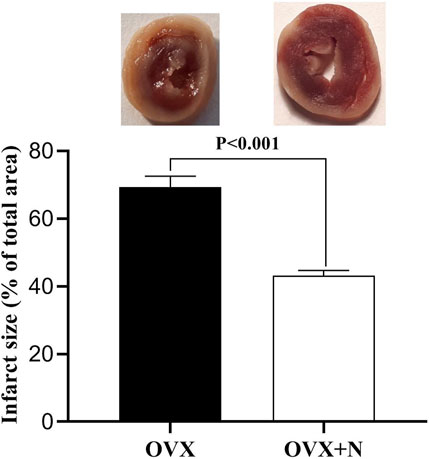
Figure 6. Levels of infarct size in the ischemia-exposed hearts from OVX and OVX + N rats. n = 7/subgroup. OVX, ovariectomized; OVX + N, OVX + nitrate.
3.6 Effect of nitrate on oxidative stress indices in serum and heart tissue
Nitrate administration for 9 months increased serum TAC levels (p = 0.006) and CAT activity (p = 0.016) and decreased MDA levels (p = 0.022) at month 9. As shown in Table 3, nitrate administration for 9 months only increased CAT (p = 0.020) activity in IR− hearts but increased TAC levels (p = 0.002), CAT activity (p < 0.001), and decreased MDA levels (p = 0.023) in IR+ hearts; that indicating inhibitory effects of nitrate against OVX-induced oxidative stress in circulation and heart tissue.
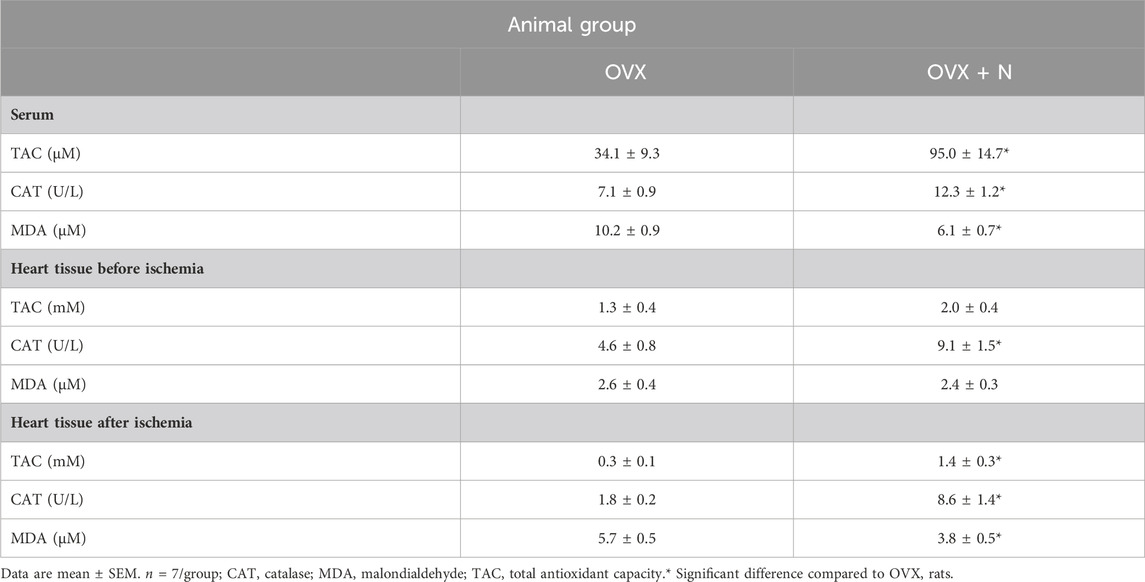
Table 3. Changes in serum and heart oxidants and antioxidants levels following 9 months of nitrate administration in ovariectomized (OVX) rats.
4 Discussion
The findings of the present study show that long-term (9 months) low-dose (100 mg/L) nitrate preconditioning alleviated IR-induced myocardial injury in OVX rats that were confirmed by the higher recovery of CFI (decreased LVEDP and increased LVDP and ±dp/dt), and lower infarct size. The favorable effects of nitrate in OVX rats were associated with the eNOS upregulation before ischemia and the blunting of OVX-induced eNOS downregulation, iNOS upregulation, apoptosis, and oxidative stress in heart tissue after IR.
In the current study, in addition to the increase in body weight, serum concentrations of progesterone and estradiol decreased, while those of LH and FSH increased at month 0 (2 months after ovariectomy and before nitrate intervention), confirming the successful establishment of the OVX model (Yousefzadeh et al., 2020). The body weight-lowering effect of nitrate in OVX rats is attributed to its ability to mitigate OVX-induced adiposity (Yousefzadeh et al., 2022c), as evidenced by reduced adipocyte size and increased levels of brown adipose tissue (Yousefzadeh et al., 2022c; Yousefzadeh and Jeddi, 2022).
In this study, IR+ hearts from OVX rats showed higher recovery of CFI and lower infarct size following nitrate administration. Reduced CFI and increased infarct size in rats following 2 (Kaya and Agan, 2022), 4 (Ibañez et al., 2022), 5 (Ibañez et al., 2022), 6 (Garvin et al., 2017), 12 (Kumar et al., 2021), and 44 (Yousefzadeh and Jeddi, 2023) weeks of ovariectomy have previously been documented. Our findings align with previous studies (summarized in Table 4) showing reduced infarct size and improved post-ischemic cardiac function in healthy mice following short-term nitrate administration (7 days) at doses of 1000 mg/L (5 mg/day) (Bryan et al., 2007) and 43 mg/L (0.22 mg/day; equivalent to 10 g/L beetroot juice containing ∼0.7 mM nitrate) (Salloum et al., 2015). In addition, we previously reported the protective effects of long-term nitrate administration (100 mg/L, 3.5 mg/day) against myocardial IR injury in male diabetic rats (60 days) (Jeddi et al., 2016) and normal female rats (270 days) (Yassaghi et al., 2023b). Furthermore, nitrite administration (50 mg/L, 0.25 mg/day for 7 days) decreased infarct size in healthy and eNOS-deficient mice (Bryan et al., 2007; Bryan et al., 2008). Our data extend this effect to OVX rats and is in line with the results of a recent meta-analysis reported that nitrite/nitrate administration reduces myocardial infarct size by ∼15% in normal animals (Yassaghi et al., 2023a) and that nitrate is more effective if it is used as a preconditioning (Yassaghi et al., 2023a), as it was used in our study.
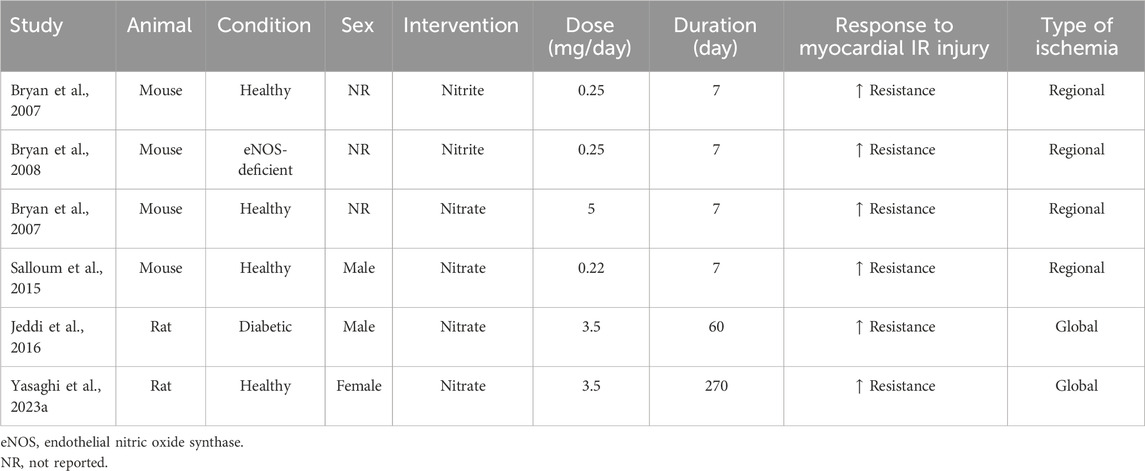
Table 4. Effect of nitrite/nitrate administration on myocardial ischemia-reperfusion injury in rodents.
In our study, the effect of nitrate against IR-induced myocardial injury in OVX animals was related to increased eNOS-derived NO both before ischemia and after IR, as well as decreased iNOS-derived NO after IR. Lower eNOS-derived NO (lower nitrate, nitrite, and NOx concentration and eNOS expression) before ischemia (Chou et al., 2010; Yousefzadeh and Jeddi, 2023) and higher iNOS-derived NO (higher nitrate, nitrite, and NOx concentration and higher iNOS expression) during IR (Sakanashi et al., 2013; Yousefzadeh and Jeddi, 2023) in the heart tissue have been reported in OVX rats. In line with our study, nitrate administration (7 days, 1000 mg/L (Bryan et al., 2007); 60 days, 100 mg/L (Jeddi et al., 2016); and 270 days, 100 mg/L (Yassaghi et al., 2023b)) increased eNOS-derived NO before ischemia and blunted decrease in eNOS and increased in iNOS expressions after IR in the heart tissue of diabetic male rats (Jeddi et al., 2016), normal female rats (Yassaghi et al., 2023b), and normal mice (Bryan et al., 2007). In normal heart tissue, eNOS produces about 80% of the heart’s NO, but during ischemia, iNOS is the predominant source of NO (Ghasemi and Jeddi, 2022). eNOS downregulation (Sharp et al., 2002) and iNOS overexpression (Di Napoli et al., 2001) exacerbate myocardial IR injury in animals; in contrast, eNOS overexpression (Jones et al., 2004) and iNOS suppression decrease myocardial IR injury (Sam et al., 2001) in animals.
Another potential mechanism underlying the favorable effects of nitrate against myocardial IR injury, observed in our study, is the reduction of circulating and myocardial oxidative stress before and after exposure to IR. Lower glutathione (GSH) levels and CAT activities and higher MDA and reactive oxygen species (ROS) levels have been reported in the heart tissue of OVX rats before and after exposure to IR (Tang et al., 2016; Kumar et al., 2021). Nitrate exerts antioxidant effects, as have been documented in the serum of OVX rats (Yang et al., 2017), the cardiovascular system of hypertensive rats (Amaral et al., 2019), rats with congestive heart failure (Donnarumma et al., 2016), and aged mice (Sindler et al., 2011). Nitrate inhibits the expression and activity of pro-oxidant enzymes and enhances the expression of antioxidant enzymes (Gao et al., 2015; Ghasemi et al., 2023), thereby exerting its antioxidant effects.
In the current study, nitrate administration reduced myocardial apoptosis in IR+ hearts from OVX rats, evidenced by the decreased mRNA expression of Bax, caspase-3, caspase-8, and caspase-9 as well as TNF-α. Activation of Fas receptor-dependent (TNF-α, Fas ligand, Fas death receptors, Fas-associated death domain, and caspase-8) and mitochondrion-dependent (Bax, Bax/Bcl-2 ratio, cytochrome c, and caspase-9) apoptotic pathways have been reported in the hearts of OVX rats (Huang et al., 2016; Wu et al., 2022). Consistent with our findings, beetroot juice (150 and 300 mg/kg for 28 days) (Raish et al., 2019) and nitrite/nitrate administration (60-min nitrite infusion 0.20 μmol/min/kg (Gonzalez et al., 2008) or 50 μL containing 1.2–1,920 nmol sodium nitrite or sodium nitrate (Duranski et al., 2005)) decreased apoptosis in IR+ hearts of control rats. Bryan et al. proposed that nitrite, derived from dietary nitrate, plays two critical roles in heart tissue during IR; it acts as a non-enzymatic NO source during ischemia and also reacts with thiol groups to form S-nitrosothiols, which prevent protein/lipid oxidation (Bryan et al., 2007) and apoptosis (Mannick et al., 1999). NO-derived nitrate mitigates apoptosis by inhibiting caspase activity, preventing mitochondrial membrane potential loss, and reducing cytochrome c release (Sellers et al., 2012). Nitrite S-nitrosates complex I of the mitochondrial electron transport chain, decreasing electron flow and ROS formation during reperfusion (Shiva et al., 2007), which inhibits the opening of the mitochondrial permeability transition pore, reducing cytochrome c release and limiting apoptosis (Gonzalez et al., 2008).
Nitrate is reduced to nitrite and then to NO; while nitrite itself can directly bind to ERα (Veselik et al., 2008), NO primarily S-nitrosylates the receptor (Garbán et al., 2005), potentially enhancing its activity. Thus, it can be speculated that nitrate has estradiol-like effects in cardiac tissue, including decreasing oxidative stress (Sedeek et al., 2013; Vrtačnik et al., 2014), iNOS activity (Chang et al., 2002), endogenous eNOS inhibitor (Monsalve et al., 2007), and increasing eNOS expression and activity (Wassmann et al., 2002; Stirone et al., 2003). The effectiveness of estrogen therapy for women with surgical menopause has been questioned because of its side effects (Marjoribanks et al., 2012; Sarrel et al., 2016; Garg et al., 2020), and inorganic nitrate, which can be easily obtained through vegetable consumption, may potentially be an alternative.
This study has some strengths. First, the dose of nitrate used in rats (100 mg/L equals 11 mg/kg/day) is translated to 1.8 mg/kg in humans, which is in the range of the median nitrate intake in humans (1.28–2.14 mg/kg/day) (Reagan-Shaw et al., 2008; Babateen et al., 2018). This dose is found in green leafy vegetables and has beneficial effects against cardiometabolic diseases (Lundberg et al., 2018; Kapil et al., 2020). Second, the OVX rat model used in the present study mimics the menopause-induced changes observed in women after surgical menopause (Yousefzadeh et al., 2020). Finally, given that a living day in rats is roughly equivalent to 26 days in humans (Quinn, 2005), a 9-month nitrate intervention could be considered a long-term intervention in humans. This study has some limitations; first, we did not use pharmacological interventions to directly assess the causal roles of NOS enzymes and ERα in the observed cardioprotective effects of nitrate. However, considering the protective effect of eNOS-derived NO (Sharp et al., 2002) and the detrimental effect of iNOS-derived NO (Parlakpinar et al., 2005; Li et al., 2006) following myocardial IR injury, it can be assumed that the protective effects of nitrate against myocardial IR injuries in OVX rats could be blunted after acute inhibition of eNOS or enhances after acute inhibition of iNOS. Second, the study did not measure heart histology to investigate the potential protective effects of nitrate against OVX-induced structural damage in heart tissue. Third, this study used a global ischemia model, commonly used in the Langendorff-perfused heart model. While the regional ischemia model may be clinically more relevant (Kim et al., 2012), the global ischemia model offers simplicity and reproducibility of results (Bibli et al., 2012). However, in the global ischemia model, the direct measurement of infarcted and non-infarcted areas is impractical or inaccurate. Fourth, the study did not measure coronary flow, an indicator of isolated heart function, such as the heart’s ability to maintain cardiac output. Finally, the rats were followed for 9 months after nitrate treatment, and the response to myocardial IR injury was measured at month 9. Longer or shorter follow-up periods may yield different results.
5 Conclusion
This study demonstrates that preconditioning with low-dose (100 mg/L) of nitrate for a long term (9 months) mitigates IR-induced myocardial injury in OVX animals. The protective effects of nitrate were evidenced by the enhanced recovery of cardiac function (decreased LVEDP and increased LVDP, ±dp/dt) and reduced infarct size. These favorable outcomes are associated with nitrate’s ability to upregulate eNOS levels before IR and to prevent OVX-induced eNOS downregulation, iNOS upregulation, apoptosis, and oxidative stress in heart tissue after IR.
One possible implication of our findings that needs to be tested in human studies is that regular intake of nitrite/nitrate-rich foods may offer protection against myocardial IR injury in women after surgical menopause. Estimates indicate that consuming nitrite-containing foods, such as leafy green vegetables and fruits, can decrease the risk of IHD (Bryan, 2017). This notion is supported by studies showing a reduced risk of IHD mortality in vegetarians, who often consume higher amounts of nitrite/nitrate-rich vegetables (average follow-up of 10.68 years), compared to non-vegetarians (Jabri et al., 2021). Furthermore, increasing daily fruit and vegetable intake has been linked to a decreased risk of coronary heart disease by 4% (Joshipura et al., 2001). Regular consumption of nitrite/nitrate-rich foods can compensate for disturbances in endogenous NO synthesis (Lundberg et al., 2006), making this a potential protective strategy for individuals at risk of CVD (Bryan, 2006). This risk is particularly elevated in women after surgical menopause, an NO-deficient state linked to a 50% higher risk of IHD compared to natural menopause (Muka et al., 2016). In addition, some studies proposed reclassifying nitrite as a dietary mineral due to its natural presence in some foods (Bryan, 2017). They also suggest exploring potential food fortification with nitrate and nitrite (Bryan and Ivy, 2015) as strategies worth further investigation. Therefore, modest dietary changes to include nitrite/nitrate-rich foods could be particularly beneficial for women who have undergone surgical menopause and are at a higher risk of myocardial IR.
Data availability statement
The original contributions presented in the study are included in the article/Supplementary material, further inquiries can be directed to the corresponding authors.
Ethics statement
The animal study was approved by The Research Institute for Endocrine Sciences ethics committee, affiliated with Shahid Beheshti University of Medical Sciences, approved all experimental procedures of the present study (Ethic Code: IR.SBMU.ENDOCRINE.REC.1402.084). The study was conducted in accordance with the local legislation and institutional requirements.
Author contributions
SJ: Conceptualization, Formal Analysis, Investigation, Methodology, Writing–original draft. NY: Conceptualization, Formal Analysis, Investigation, Methodology, Writing–original draft. MZ: Investigation, Methodology, Writing–original draft. KK: Conceptualization, Validation, Writing–review and editing. AG: Conceptualization, Formal Analysis, Funding acquisition, Project administration, Supervision, Validation, Writing–original draft, Writing–review and editing.
Funding
The author(s) declare that financial support was received for the research, authorship, and/or publication of this article. This research was funded by Shahid Beheshti University of Medical Sciences, grant number 43007937-4.
Conflict of interest
The authors declare that the research was conducted in the absence of any commercial or financial relationships that could be construed as a potential conflict of interest.
The author(s) declared that they were an editorial board member of Frontiers, at the time of submission. This had no impact on the peer review process and the final decision.
Publisher’s note
All claims expressed in this article are solely those of the authors and do not necessarily represent those of their affiliated organizations, or those of the publisher, the editors and the reviewers. Any product that may be evaluated in this article, or claim that may be made by its manufacturer, is not guaranteed or endorsed by the publisher.
References
Ahmadi-Noorbakhsh, S., Mirabzadeh Ardakani, E., Sadighi, J., Aldavood, S. J., Farajli Abbasi, M., Farzad-Mohajeri, S., et al. (2021). Guideline for the care and use of laboratory animals in Iran. Lab. Anim. 50 (11), 303–305. doi:10.1038/s41684-021-00871-3
Amaral, J. H., Rizzi, E. S., Alves-Lopes, R., Pinheiro, L. C., Tostes, R. C., and Tanus-Santos, J. E. (2019). Antioxidant and antihypertensive responses to oral nitrite involves activation of the Nrf2 pathway. Free Radic. Biol. Med. 141, 261–268. doi:10.1016/j.freeradbiomed.2019.06.028
Babateen, A. M., Fornelli, G., Donini, L. M., Mathers, J. C., and Siervo, M. (2018). Assessment of dietary nitrate intake in humans: a systematic review. Am. J. Clin. Nutr. 108 (4), 878–888. doi:10.1093/ajcn/nqy108
Bae, S., and Zhang, L. (2005). Gender differences in cardioprotection against ischemia/reperfusion injury in adult rat hearts: focus on Akt and protein kinase C signaling. J. Pharmacol. Exp. Ther. 315 (3), 1125–1135. doi:10.1124/jpet.105.090803
Besík, J., Szárszoi, O., Kunes, J., Netuka, I., Malý, J., Kolár, F., et al. (2007). Tolerance to acute ischemia in adult male and female spontaneously hypertensive rats. Physiol. Res. 56 (3), 267–274. doi:10.33549/physiolres.930998
Bibli, S. I., Toli, E. V., Vilaeti, A. D., Varnavas, V. C., Baltogiannis, G. G., Papalois, A., et al. (2012). Endothelin-B receptors and left ventricular dysfunction after regional versus global ischaemia-reperfusion in rat hearts. Cardiol. Res. Pract. 2012, 986813. doi:10.1155/2012/986813
Bradford, M. M. (1976). A rapid and sensitive method for the quantitation of microgram quantities of protein utilizing the principle of protein-dye binding. Anal. Biochem. 72, 248–254. doi:10.1006/abio.1976.9999
Bryan, N. S. (2006). Nitrite in nitric oxide biology: cause or consequence? A systems-based review. Free Radic. Biol. Med. 41 (5), 691–701. doi:10.1016/j.freeradbiomed.2006.05.019
Bryan, N. S. (2017). “3 - an overview of nitrite and nitrate: new paradigm of nitric oxide,” in Sustained energy for enhanced human functions and activity. Editor D. Bagchi (Cambridge, Massachusetts, United States: Academic Press), 53–65.
Bryan, N. S., Calvert, J. W., Elrod, J. W., Gundewar, S., Ji, S. Y., and Lefer, D. J. (2007). Dietary nitrite supplementation protects against myocardial ischemia-reperfusion injury. Proc. Natl. Acad. Sci. U. S. A. 104 (48), 19144–19149. doi:10.1073/pnas.0706579104
Bryan, N. S., Calvert, J. W., Gundewar, S., and Lefer, D. J. (2008). Dietary nitrite restores NO homeostasis and is cardioprotective in endothelial nitric oxide synthase-deficient mice. Free Radic. Biol. Med. 45 (4), 468–474. doi:10.1016/j.freeradbiomed.2008.04.040
Bryan, N. S., and Ivy, J. L. (2015). Inorganic nitrite and nitrate: evidence to support consideration as dietary nutrients. Nutr. Res. 35 (8), 643–654. doi:10.1016/j.nutres.2015.06.001
Caulin-Glaser, T., García-Cardeña, G., Sarrel, P., Sessa, W. C., and Bender, J. R. (1997). 17 beta-estradiol regulation of human endothelial cell basal nitric oxide release, independent of cytosolic Ca2+ mobilization. Circ. Res. 81 (5), 885–892. doi:10.1161/01.res.81.5.885
Chang, S. P., Yang, W. S., Lee, S. K., Min, W. K., Park, J. S., and Kim, S. B. (2002). Effects of hormonal replacement therapy on oxidative stress and total antioxidant capacity in postmenopausal hemodialysis patients. Ren. Fail 24 (1), 49–57. doi:10.1081/jdi-120002660
Chen, C., Hu, L. X., Dong, T., Wang, G. Q., Wang, L. H., Zhou, X. P., et al. (2013). Apoptosis and autophagy contribute to gender difference in cardiac ischemia-reperfusion induced injury in rats. Life Sci. 93 (7), 265–270. doi:10.1016/j.lfs.2013.06.019
Chou, S.-H., Lee, Y.-C., Huang, C.-F., Wang, Y.-R., Yu, H.-P., and Lau, Y.-T. (2010). Gender-specific effects of caloric restriction on the balance of vascular nitric oxide and superoxide radical. Cardiovasc. Res. 87 (4), 751–759. doi:10.1093/cvr/cvq095
Costello, B. T., Sprung, K., and Coulter, S. A. (2017). The rise and fall of estrogen therapy: is testosterone for "manopause" next? Tex. Heart Inst. J. 44 (5), 338–340. doi:10.14503/THIJ-17-6360
Di Napoli, P., Antonio Taccardi, A., Grilli, A., Spina, R., Felaco, M., Barsotti, A., et al. (2001). Simvastatin reduces reperfusion injury by modulating nitric oxide synthase expression: an ex vivo study in isolated working rat hearts. Cardiovasc Res. 51 (2), 283–293. S0008636301003066. doi:10.1016/s0008-6363(01)00306-6
Donnarumma, E., Bhushan, S., Bradley, J. M., Otsuka, H., Donnelly, E. L., Lefer, D. J., et al. (2016). Nitrite therapy ameliorates myocardial dysfunction via H2S and nuclear factor-erythroid 2-related factor 2 (Nrf2)-Dependent signaling in chronic heart failure. J. Am. Heart Assoc. 5 (8), e003551. doi:10.1161/jaha.116.003551
Duranski, M. R., Greer, J. J., Dejam, A., Jaganmohan, S., Hogg, N., Langston, W., et al. (2005). Cytoprotective effects of nitrite during in vivo ischemia-reperfusion of the heart and liver. J. Clin. Invest. 115 (5), 1232–1240. doi:10.1172/JCI22493
Faubion, S. S., Kuhle, C. L., Shuster, L. T., and Rocca, W. A. (2015). Long-term health consequences of premature or early menopause and considerations for management. Climacteric 18 (4), 483–491. doi:10.3109/13697137.2015.1020484
Gao, X., Yang, T., Liu, M., Peleli, M., Zollbrecht, C., Weitzberg, E., et al. (2015). NADPH oxidase in the renal microvasculature is a primary target for blood pressure-lowering effects by inorganic nitrate and nitrite. Hypertension 65 (1), 161–170. doi:10.1161/HYPERTENSIONAHA.114.04222
Garbán, H. J., Márquez-Garbán, D. C., Pietras, R. J., and Ignarro, L. J. (2005). Rapid nitric oxide-mediated S-nitrosylation of estrogen receptor: regulation of estrogen-dependent gene transcription. Proc. Natl. Acad. Sci. U. S. A. 102 (7), 2632–2636. doi:10.1073/pnas.0409854102
Garg, N., Behbehani, S., Kosiorek, H., and Wasson, M. (2020). Hormone replacement therapy prescription after premature surgical menopause. J. Minim. Invasive Gynecol. 27 (7), 1618–1623. doi:10.1016/j.jmig.2020.03.002
Garvin, A. M., Aurigemma, N. C., Hackenberger, J. L., and Korzick, D. H. (2017). Age and ischemia differentially impact mitochondrial ultrastructure and function in a novel model of age-associated estrogen deficiency in the female rat heart. Pflugers Arch. 469 (12), 1591–1602. doi:10.1007/s00424-017-2034-7
Ghanbari, M., Jeddi, S., Bagheripuor, F., and Ghasemi, A. (2015). The effect of maternal hypothyroidism on cardiac function and tolerance to ischemia-reperfusion injury in offspring male and female rats. J. Endocrinol. Invest. 38 (8), 915–922. doi:10.1007/s40618-015-0267-x
Ghasemi, A., Gheibi, S., Kashfi, K., and Jeddi, S. (2023). Anti-oxidant effect of nitrite in the pancreatic islets of type 2 diabetic male rats. Iran. J. Basic Med. Sci. 26 (4), 420–428. doi:10.22038/ijbms.2023.68245.14900
Ghasemi, A., and Jeddi, S. (2022). Quantitative aspects of nitric oxide production in the heart. Mol. Biol. Rep. 49 (11), 11113–11122. doi:10.1007/s11033-022-07889-x
Gonzalez, F. M., Shiva, S., Vincent, P. S., Ringwood, L. A., Hsu, L. Y., Hon, Y. Y., et al. (2008). Nitrite anion provides potent cytoprotective and antiapoptotic effects as adjunctive therapy to reperfusion for acute myocardial infarction. Circulation 117 (23), 2986–2994. doi:10.1161/CIRCULATIONAHA.107.748814
Huang, C. Y., Lin, Y. Y., Hsu, C. C., Cheng, S. M., Shyu, W. C., Ting, H., et al. (2016). Antiapoptotic effect of exercise training on ovariectomized rat hearts. J. Appl. Physiol. 121 (2), 457–465. doi:10.1152/japplphysiol.01042.2015
Ibañez, A. M., González Arbeláez, L. F., Ciocci Pardo, A., Mosca, S., Lofeudo, J. M., Velez Rueda, J. O., et al. (2022). Chronic GPER activation prevents ischemia/reperfusion injury in ovariectomized rats. Biochimica Biophysica Acta (BBA) - General Subj. 1866 (2), 130060. doi:10.1016/j.bbagen.2021.130060
Jabri, A., Kumar, A., Verghese, E., Alameh, A., Kumar, A., Khan, M. S., et al. (2021). Meta-analysis of effect of vegetarian diet on ischemic heart disease and all-cause mortality. Am. J. Prev. Cardiol. 7, 100182. doi:10.1016/j.ajpc.2021.100182
Jeddi, S., Khalifi, S., Ghanbari, M., Bageripour, F., and Ghasemi, A. (2016). Effects of nitrate intake on myocardial ischemia-reperfusion injury in diabetic rats. Arq. Bras. Cardiol. 107, 339–347. doi:10.5935/abc.20160137
Jones, S. P., Greer, J. J., Kakkar, A. K., Ware, P. D., Turnage, R. H., Hicks, M., et al. (2004). Endothelial nitric oxide synthase overexpression attenuates myocardial reperfusion injury. Am. J. Physiol. Heart Circ. Physiol. 286 (1), H276–H282. doi:10.1152/ajpheart.00129.2003
Joshipura, K. J., Hu, F. B., Manson, J. E., Stampfer, M. J., Rimm, E. B., Speizer, F. E., et al. (2001). The effect of fruit and vegetable intake on risk for coronary heart disease. Ann. Intern Med. 134 (12), 1106–1114. doi:10.7326/0003-4819-134-12-200106190-00010
Kapil, V., Khambata, R., Jones, D., Rathod, K., Primus, C., Massimo, G., et al. (2020). The noncanonical pathway for in vivo nitric oxide generation: the nitrate-nitrite-nitric oxide pathway. Pharmacol. Rev. 72 (3), 692–766. doi:10.1124/pr.120.019240
Kaya, S. T., Agan, K., Fulden-Agan, A., Agyar-Yoldas, P., Ozarslan, T. O., Kekecoglu, M., et al. (2022). Protective effect of propolis on myocardial ischemia/reperfusion injury in males and ovariectomized females but not in intact females. J. Food Biochem. 46, e14109. doi:10.1111/jfbc.14109
Kim, J. H., Kim, J., Park, Y. H., Chun, K. J., Kim, J. S., Jang, Y. H., et al. (2012). Cardiodynamics and infarct size in regional and global ischemic isolated heart model: comparison of 1 hour and 2 hours reperfusion. Korean Circ. J. 42 (9), 600–605. doi:10.4070/kcj.2012.42.9.600
Kumar, A., Boovarahan, S. R., Prem, P. N., Ramanathan, M., Chellappan, D. R., and Kurian, G. A. (2021). Evaluating the effects of carbon monoxide releasing molecule-2 against myocardial ischemia-reperfusion injury in ovariectomized female rats. Naunyn Schmiedeb. Arch. Pharmacol. 394 (10), 2103–2115. doi:10.1007/s00210-021-02129-8
Lee, S. D., Kuo, W. W., Ho, Y. J., Lin, A. C., Tsai, C. H., Wang, H. F., et al. (2008). Cardiac Fas-dependent and mitochondria-dependent apoptosis in ovariectomized rats. Maturitas 61 (3), 268–277. doi:10.1016/j.maturitas.2008.07.004
Li, D., Qu, Y., Tao, L., Liu, H., Hu, A., Gao, F., et al. (2006). Inhibition of iNOS protects the aging heart against beta-adrenergic receptor stimulation-induced cardiac dysfunction and myocardial ischemic injury. J. Surg. Res. 131 (1), 64–72. doi:10.1016/j.jss.2005.06.038
Lloyd-Jones, D. M., Larson, M. G., Beiser, A., and Levy, D. (1999). Lifetime risk of developing coronary heart disease. Lancet 353 (9147), 89–92. doi:10.1016/s0140-6736(98)10279-9
Lundberg, J. O., Carlström, M., and Weitzberg, E. (2018). Metabolic effects of dietary nitrate in health and disease. Cell Metab. 28 (1), 9–22. doi:10.1016/j.cmet.2018.06.007
Lundberg, J. O., Feelisch, M., Björne, H., Jansson, E. A., and Weitzberg, E. (2006). Cardioprotective effects of vegetables: is nitrate the answer? Nitric Oxide 15 (4), 359–362. doi:10.1016/j.niox.2006.01.013
Lundberg, J. O., Gladwin, M. T., and Weitzberg, E. (2015). Strategies to increase nitric oxide signalling in cardiovascular disease. Nat. Rev. Drug Discov. 14 (9), 623–641. doi:10.1038/nrd4623
Mannick, J. B., Hausladen, A., Liu, L., Hess, D. T., Zeng, M., Miao, Q. X., et al. (1999). Fas-induced caspase denitrosylation. Science 284 (5414), 651–654. doi:10.1126/science.284.5414.651
Marjoribanks, J., Farquhar, C., Roberts, H., and Lethaby, A. (2012). Long term hormone therapy for perimenopausal and postmenopausal women. Cochrane Database Syst. Rev. (7), Cd004143. doi:10.1002/14651858.CD004143.pub4
Medzikovic, L., Azem, T., Sun, W., Rejali, P., Esdin, L., Rahman, S., et al. (2023). Sex differences in therapies against myocardial ischemia-reperfusion injury: from basic science to clinical perspectives. Cells 12 (16), 2077. doi:10.3390/cells12162077
Miranda, K. M., Espey, M. G., and Wink, D. A. (2001). A rapid, simple spectrophotometric method for simultaneous detection of nitrate and nitrite. Nitric Oxide 5 (1), 62–71. doi:10.1006/niox.2000.0319
Monsalve, E., Oviedo, P. J., García-Pérez, M. A., Tarín, J. J., Cano, A., and Hermenegildo, C. (2007). Estradiol counteracts oxidized LDL-induced asymmetric dimethylarginine production by cultured human endothelial cells. Cardiovasc Res. 73 (1), 66–72. doi:10.1016/j.cardiores.2006.09.020
Muka, T., Oliver-Williams, C., Kunutsor, S., Laven, J. S., Fauser, B. C., Chowdhury, R., et al. (2016). Association of age at onset of menopause and time since onset of menopause with cardiovascular outcomes, intermediate vascular traits, and all-cause mortality: a systematic review and meta-analysis. JAMA Cardiol. 1 (7), 767–776. doi:10.1001/jamacardio.2016.2415
NCD Countdown collaborators (2020). NCD Countdown 2030: pathways to achieving sustainable development goal target 3.4. Lancet 396 (10255), 918–934. doi:10.1016/s0140-6736(20)31761-x
Parlakpinar, H., Ozer, M. K., and Acet, A. (2005). Effect of aminoguanidine on ischemia-reperfusion induced myocardial injury in rats. Mol. Cell Biochem. 277 (1-2), 137–142. doi:10.1007/s11010-005-5779-9
Percie du Sert, N., Hurst, V., Ahluwalia, A., Alam, S., Avey, M. T., Baker, M., et al. (2020). The ARRIVE guidelines 2.0: updated guidelines for reporting animal research. PLoS Biol. 18 (7), e3000410. doi:10.1371/journal.pbio.3000410
Quinn, R. (2005). Comparing rat's to human's age: how old is my rat in people years? Nutrition 21 (6), 775–777. doi:10.1016/j.nut.2005.04.002
Raish, M., Ahmad, A., Ansari, M. A., Alkharfy, K. M., Ahad, A., Khan, A., et al. (2019). Beetroot juice alleviates isoproterenol-induced myocardial damage by reducing oxidative stress, inflammation, and apoptosis in rats. 3 Biotech. 9 (4), 147. doi:10.1007/s13205-019-1677-9
Reagan-Shaw, S., Nihal, M., and Ahmad, N. (2008). Dose translation from animal to human studies revisited. Faseb J. 22 (3), 659–661. doi:10.1096/fj.07-9574LSF
Safiri, S., Karamzad, N., Singh, K., Carson-Chahhoud, K., Adams, C., Nejadghaderi, S. A., et al. (2022). Burden of ischemic heart disease and its attributable risk factors in 204 countries and territories, 1990-2019. Eur. J. Prev. Cardiol. 29 (2), 420–431. doi:10.1093/eurjpc/zwab213
Sakanashi, M., Matsuzaki, T., Noguchi, K., Nakasone, J., Sakanashi, M., Uchida, T., et al. (2013). Long-term treatment with san’o-shashin-to, a kampo medicine, markedly ameliorates cardiac ischemia-reperfusion injury in ovariectomized rats via the redox-dependent mechanism. Circulation J. 77 (7), 1827–1837. doi:10.1253/circj.CJ-12-1434
Salloum, F. N., Sturz, G. R., Yin, C., Rehman, S., Hoke, N. N., Kukreja, R. C., et al. (2015). Beetroot juice reduces infarct size and improves cardiac function following ischemia–reperfusion injury: possible involvement of endogenous H2S. Exp. Biol. Med. 240 (5), 669–681. doi:10.1177/1535370214558024
Sam, F., Sawyer, D. B., Xie, Z., Chang, D. L., Ngoy, S., Brenner, D. A., et al. (2001). Mice lacking inducible nitric oxide synthase have improved left ventricular contractile function and reduced apoptotic cell death late after myocardial infarction. Circ. Res. 89 (4), 351–356. doi:10.1161/hh1601.094993
Sarrel, P. M., Sullivan, S. D., and Nelson, L. M. (2016). Hormone replacement therapy in young women with surgical primary ovarian insufficiency. Fertil. Steril. 106 (7), 1580–1587. doi:10.1016/j.fertnstert.2016.09.018
Sedeek, M., Nasrallah, R., Touyz, R. M., and Hébert, R. L. (2013). NADPH oxidases, reactive oxygen species, and the kidney: friend and foe. J. Am. Soc. Nephrol. 24 (10), 1512–1518. doi:10.1681/ASN.2012111112
Sellers, S. L., Trane, A. E., and Bernatchez, P. N. (2012). Caveolin as a potential drug target for cardiovascular protection. Front. Physiol. 3, 280. doi:10.3389/fphys.2012.00280
Sharp, B. R., Jones, S. P., Rimmer, D. M., and Lefer, D. J. (2002). Differential response to myocardial reperfusion injury in eNOS-deficient mice. Am. J. Physiol. Heart Circ. Physiol. 282 (6), H2422–H2426. doi:10.1152/ajpheart.00855.2001
Shinmura, K., Nagai, M., Tamaki, K., and Bolli, R. (2008). Loss of ischaemic preconditioning in ovariectomized rat hearts: possible involvement of impaired protein kinase C epsilon phosphorylation. Cardiovasc Res. 79 (3), 387–394. doi:10.1093/cvr/cvn086
Shiva, S., Sack, M. N., Greer, J. J., Duranski, M., Ringwood, L. A., Burwell, L., et al. (2007). Nitrite augments tolerance to ischemia/reperfusion injury via the modulation of mitochondrial electron transfer. J. Exp. Med. 204 (9), 2089–2102. doi:10.1084/jem.20070198
Sindler, A. L., Fleenor, B. S., Calvert, J. W., Marshall, K. D., Zigler, M. L., Lefer, D. J., et al. (2011). Nitrite supplementation reverses vascular endothelial dysfunction and large elastic artery stiffness with aging. Aging Cell 10 (3), 429–437. doi:10.1111/j.1474-9726.2011.00679.x
Stirone, C., Chu, Y., Sunday, L., Duckles, S. P., and Krause, D. N. (2003). 17 Beta-estradiol increases endothelial nitric oxide synthase mRNA copy number in cerebral blood vessels: quantification by real-time polymerase chain reaction. Eur. J. Pharmacol. 478 (1), 35–38. doi:10.1016/j.ejphar.2003.08.037
Sun, J., Picht, E., Ginsburg, K. S., Bers, D. M., Steenbergen, C., and Murphy, E. (2006). Hypercontractile female hearts exhibit increased S-nitrosylation of the L-type Ca2+ channel alpha1 subunit and reduced ischemia/reperfusion injury. Circ. Res. 98 (3), 403–411. doi:10.1161/01.RES.0000202707.79018.0a
Tang, Y., Li, S., Zhang, P., Zhu, J., Meng, G., Xie, L., et al. (2016). Soy isoflavone protects myocardial ischemia/reperfusion injury through increasing endothelial nitric oxide synthase and decreasing oxidative stress in ovariectomized rats. Oxidative Med. Cell. Longev. 2016, 5057405. doi:10.1155/2016/5057405
Veselik, D. J., Divekar, S., Dakshanamurthy, S., Storchan, G. B., Turner, J. M., Graham, K. L., et al. (2008). Activation of estrogen receptor-alpha by the anion nitrite. Cancer Res. 68 (10), 3950–3958. doi:10.1158/0008-5472.can-07-2783
Vrtačnik, P., Ostanek, B., Mencej-Bedrač, S., and Marc, J. (2014). The many faces of estrogen signaling. Biochem. medica 24 (3), 329–342. doi:10.11613/BM.2014.035
Wassmann, S., Laufs, U., Stamenkovic, D., Linz, W., Stasch, J. P., Ahlbory, K., et al. (2002). Raloxifene improves endothelial dysfunction in hypertension by reduced oxidative stress and enhanced nitric oxide production. Circulation 105 (17), 2083–2091. doi:10.1161/01.cir.0000014618.91633.67
Wu, X. B., Lai, C. H., Ho, Y. J., Kuo, C. H., Lai, P. F., Tasi, C. Y., et al. (2022). Anti-apoptotic effects of diosgenin on ovariectomized hearts. Steroids 179, 108980. doi:10.1016/j.steroids.2022.108980
Yang, T., Zhang, X. M., Tarnawski, L., Peleli, M., Zhuge, Z., Terrando, N., et al. (2017). Dietary nitrate attenuates renal ischemia-reperfusion injuries by modulation of immune responses and reduction of oxidative stress. Redox Biol. 13, 320–330. doi:10.1016/j.redox.2017.06.002
Yassaghi, Y., Jeddi, S., Kashfi, K., and Ghasemi, A. (2023a). Myocardial infarct size is reduced by nitrite and nitrate administration: a systematic review and meta-analysis of animal studies. EXCLI J., doi:10.1186/s12872-023-03425-2
Yassaghi, Y., Jeddi, S., Yousefzadeh, N., Kashfi, K., and Ghasemi, A. (2023b). Long-term inorganic nitrate administration protects against myocardial ischemia-reperfusion injury in female rats. BMC Cardiovasc Disord. 23 (1), 411. doi:10.1186/s12872-023-03425-2
Yousefzadeh, N., and Jeddi, S. (2023). Long-term ovariectomy reduces tolerance of rats to myocardial ischemia-reperfusion injury. Int. J. Endocrinol. Metab. 21 (3), e135101. doi:10.5812/ijem-135101
Yousefzadeh, N., Jeddi, S., Afzali, H., Kashfi, K., and Ghasemi, A. (2022). Chronic nitrate administration increases the expression the genes involved in the browning of white adipose tissue in female rats. Cell Biochem. Funct. 40 (7), 750–759. doi:10.1002/cbf.3741
Yousefzadeh, N., Jeddi, S., and Ghasemi, A. (2022a). The effect of ovariectomy on hemodynamic functions and the level of nitric oxide metabolites in the heart of rats. Med. J. Tabriz Univ. Med. Sci. 44 (3), 178–188. doi:10.34172/mj.2022.026
Yousefzadeh, N., Jeddi, S., Kashfi, K., and Ghasemi, A. (2022b). Long-term inorganic nitrate administration protects against ovariectomy-induced osteoporosis in rats. EXCLI J. 21, 1151–1166. doi:10.17179/excli2022-5082
Yousefzadeh, N., Jeddi, S., Shokri, M., Afzali, H., Norouzirad, R., Kashfi, K., et al. (2022c). Long term sodium nitrate administration positively impacts metabolic and obesity indices in ovariectomized rats. Arch. Med. Res. 53 (2), 147–156. doi:10.1016/j.arcmed.2021.09.007
Yousefzadeh, N., Jeddi, S., Zarkesh, M., Kashfi, K., and Ghasemi, A. (2023). Altered sialin mRNA gene expression in type 2 diabetic male Wistar rats: implications for nitric oxide deficiency. Sci. Rep. 13 (1), 4013. doi:10.1038/s41598-023-31240-4
Keywords: ovariectomy, menopause, nitric oxide, nitrate, cardiac function, female rats
Citation: Jeddi S, Yousefzadeh N, Zarkesh M, Kashfi K and Ghasemi A (2024) Effect of long-term inorganic nitrate administration on myocardial ischemia-reperfusion injury in ovariectomized rats. Front. Pharmacol. 15:1369379. doi: 10.3389/fphar.2024.1369379
Received: 12 January 2024; Accepted: 15 March 2024;
Published: 27 March 2024.
Edited by:
Mahmoud El-Mas, Alexandria University, EgyptReviewed by:
Adebayo Oluwafemi Adekunle, Xuzhou Medical University, ChinaAgnaldo Bruno Chies, Medical School of Marilia, Brazil
Copyright © 2024 Jeddi, Yousefzadeh, Zarkesh, Kashfi and Ghasemi. This is an open-access article distributed under the terms of the Creative Commons Attribution License (CC BY). The use, distribution or reproduction in other forums is permitted, provided the original author(s) and the copyright owner(s) are credited and that the original publication in this journal is cited, in accordance with accepted academic practice. No use, distribution or reproduction is permitted which does not comply with these terms.
*Correspondence: Asghar Ghasemi, R2hhc2VtaUBzYm11LmFjLmly; Khosrow Kashfi, S2FzaGZpQG1lZC5jdW55LmVkdQ==