- 1State Key Laboratory of Southwestern Chinese Medicine Resources, Chengdu, China
- 2Pharmacy College of Chengdu University of Traditional Chinese Medicine, Chengdu, China
Formononetin (FMNT) is a secondary metabolite of flavonoids abundant in legumes and graminaceous plants such as Astragalus mongholicus Bunge [Fabaceae; Astragali radix] and Avena sativa L. [Poaceae]. Astragalus is traditionally used in Asia countries such as China, Korea and Mongolia to treat inflammatory diseases, immune disorders and cancers. In recent years, inflammation and oxidative stress have been found to be associated with many diseases. A large number of pharmacological studies have shown that FMNT, an important bioactive metabolite of Astragalus, has a profoundly anti-inflammatory and antioxidant potential. This review focuses on providing comprehensive and up-to-date findings on the efficacy of the molecular targets and mechanisms involve of FMNT and its derivatives against inflammation and oxidative stress in both in vitro and in vivo. Relevant literature on FMNT against inflammation and oxidative stress between 2013 and 2023 were analyzed. FMNT has antioxidant and anti-inflammatory potential and shows mild or no toxicity in various diseases. Moreover, in the medical field, FMNT has shown potential in the prevention and treatment of cancers, neurological diseases, fibrotic diseases, allergic diseases, metabolic diseases, cardiovascular diseases, gastrointestinal diseases and autoimmune diseases. Thus, it is expected to be utilized in more products in the medical, food and cosmetic industries in the future.
1 Introduction
Inflammation and oxidative stress have been implicated as key mechanisms in a range of disease processes. Several studies have presented the scientific basis about the interconnecting paths governing oxidative stress-induced inflammation and vice versa. Therefore, it has become imperative to selectively develop therapies and drugs for many diseases based on the relevant mechanisms (Khansari et al., 2009; Reuter et al., 2010; Salzano et al., 2014).
Traditional botanical medicine is extensively used in the cure of inflammation and oxidative stress-related diseases due to its multi-metabolite, multi-target, multi-pathway and mild toxicity attributes. This is why the traditional application of botanical drugs and their active ingredients has been a top priority when researchers tap into modern medicine. Formononetin (FMNT) is an isoflavone, a type of flavonoid, and is one of the main bioactive metabolites of many botanical drugs such as Trifolium pratense L. [Fabaceae] and Astragalus mongholicus Bunge [Fabaceae; Astragali radix]. The chemical structure of FMNT is shown in Figure 1.
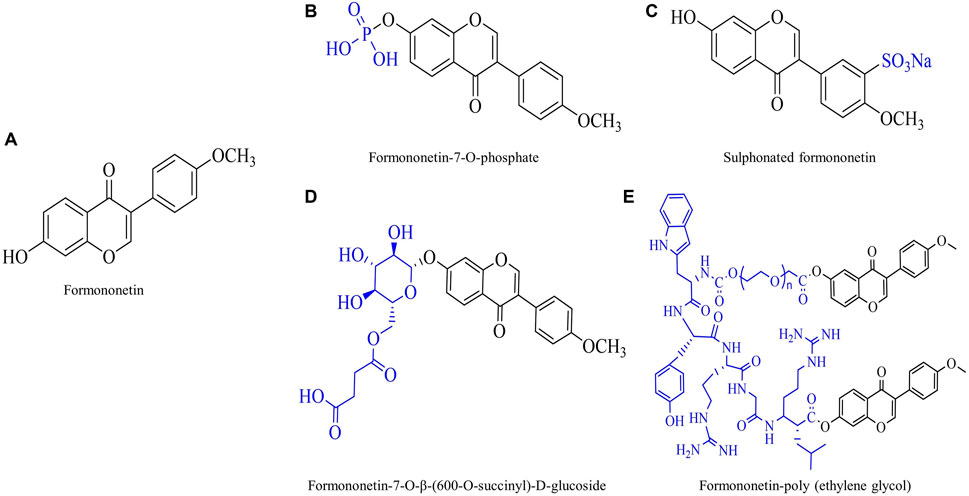
Figure 1. Chemical structure of formononetin (FMNT) and its derivatives. (A) Chemical structure of FMNT. (B) Chemical structure of FMNT-7-O-phosphate. (C) Chemical structure of sulphonated FMNT. (D) Chemical structure of FMNT-7-O-β-(600-O-succinyl)-D-glucoside. (E) Chemical structure of FMNT-poly (ethylene glycol).
Various studies have previously shown that FMNT has a wealth of pharmacological effects, including antioxidant effects, anti-inflammatory activity, neuroprotective effects, anti-cancer effects, healing action, osteogenic action, anti-microbial and anti-viral activity, and treatment of diabetes and hypertension. Inspired by traditional uses, many researchers have explored the efficacy and molecular mechanisms of FMNT for inflammation and oxidative stress-induced diseases through the use of in vivo and in vitro models.
Although the pharmacological effects of FMNT have been summarized to some extent by previous authors, there is no review focusing on the anti-inflammatory and antioxidant pharmacological effects of FMNT, as well as its intrinsic in-depth molecular mechanisms. Thus, in present review we will provide an up-to-date and comprehensive overview of the anti-oxidative stress and anti-inflammatory activities of FMNT and assess its molecular mechanisms. It is desired to provide a detailed scientific basis and fundemental for future research directions and application development.
2 Anti-inflammatory molecular targets and mechanisms of FMNT
Inflammation, an early host immune response mediated by cytokines released from immune cells, is a critically underlying pathological process that usually accompanies the development of a wide range of diseases. Generally, the inflammatory response is a protection of cells exposed to various stimuli, whereas excessive inflammation can induce severe organismal damage and even tumor. When cells are stimulated by inflammation, mitogen-activated protein kinase (MAPK) first transmits inflammatory signals from the cell surface to the cell, promoting the activation of downstream kinases extracellular signal-regulated kinase 1/2 (ERK1/2) and c-Jun N-terminal kinase (JNK) as well as the expression of forkhead box O3 (FOXO3a) protein, which translocates to the nucleus and participates in the transcription of related inflammatory factors. In parallel, activated ERK1/2 can also promote inhibitor of kappa B kinase (IκBα) phosphorylation, accelerate IκBα ubiquitination degradation, and release the p50/p65 complex. The p50/p65 complex is transported to the nucleus to regulate transcription. Moreover, activated JNK can also promote signal transducer and activator of transcription 3 (STAT3) phosphorylation after entering the nucleus. Phosphorylated STAT3 acts in concert with the p50/p65 complex and FOXO3a to promote the expression of interleukin (IL), tumor necrosis factor-α (TNF-α), nitric oxide (NO), Prostaglandin E2 (PEG2) and other inflammatory factors. In addition to the MAPK dependency, the nuclear factor kappa B (NF-κB) and janus kinase/signal transducer and activator of transcription (JAK/STAT) pathways are also directly activated by TNF-α, granulocyte colony-stimulating factor (G-CSF), or IL-6, regulating the activity of downstream transcription factors and related enzymes. These highly expressed inflammatory factors further activate related inflammatory pathways, creating a vicious cycle. Noteworthy, FMNT has been shown to quench these processes and reduce the production of inflammatory factors, thereby alleviating inflammation.
2.1 Inflammatory mediators secretion regulation
2.1.1 Cytokine release modulators
Cytokines are vital cellular signaling molecules and mediators of the immune system that monitor and regulate immune and inflammatory responses through complex networks. Unusual cytokine levels can contribute to cytokine storms and disease. The level of cytokines is therefore of great value in the diagnosis and therapy of diseases (Liu et al., 2021). A wealth of studies have shown remarkable anti-inflammatory activity of FMNT on luxuriant inflammatory models induced by manifold inflammagens in vivo and in vitro (Table 1). The primary macrophage-secreted pro-inflammatory cytokine, such as TNF-α and multiple ILs, chemokines, adhesion molecules and NOsynthesized by iNOS via the L-arginine pathway, are widely accepted to be compactly implicated in the development and amplification of inflammation.
It has been reported that FMNT had a neuroprotective effect on brain injury by alleviating neuroinflammation. For example, the researchers found that levels of inflammatory cytokines were not only reduced by FMNT in the injured brain tissues. In addition, the team enriched the intervention effect of FMNT on inflammation in serum and brain tissue of the cortical neurons in follow-up studies. This also reflects the critical role of FMNT in brain disease inflammation. (Li et al., 2014; Li et al., 2018). In addition, FMNT was also able to alleviate side effects of drugs with multiple organ toxicity, and organ damage due to serious diseases. FMNT improved liver dysfunction in rat models of Ritonavir induced hepatotoxicity via inhibiting irregular IL-1β, IL-6, and TNF-α secretion (Alauddin et al., 2018). Besides, researchers demonstrated pro-inflammatory cytokine inhibitory activity of FMNT against acute lung injury induced by LPS. The authors observed a downregulation of increased IL-6 and TNF-α levels in dose-dependent manners after FMNT administration (Ma et al., 2013). Besides that, pre-treatment with FMNT distinctly attenuated the hyperoxia-induced increase of pro-inflammatory cytokine secretion (Chen et al., 2021). Similarly, other researchers found that FMNT administration mitigated the overexpression of TNF-α and IL-1β in cisplatin-induced acute kidney injury models on male Wistar rats (Hao et al., 2021).
In addition to anti-inflammatory cytokine release in vivo, FMNT also has the potential to diminish the overexpression of pro-inflammatory cytokines in inflammatory models in vitro. Recently, researchers evaluated the effect of FMNT in treating osteoarthritis. According to the authors, 1.25 μg/mL FMNT treatment visibly lowered IL-1β secretion in IL-1β stimulated chondrocytes (Xiong et al., 2021). Notably, the FMNT used in the experiments in both in vivo and in vitro in this article is the same concentration, which is inconsistent with the doses of the ordinary article and deserves more rigorous consideration. The potential of FMNT in remedying inflammation has been evaluated using the RAW264.7 macrophage model triggered by LPS. According to the results, FMNT showed reduction of the NOx level in the inflammatory macrophages, exhibiting favorable antioxidant activity (Bottamedi et al., 2021). Other authors stated that 32 μg/mL FMNT significantly reversed the strong inflammatory response caused by streptococcus suis serotype 2 infection in macrophage-like J774 cells (Wang et al., 2020).
2.1.2 Chemokine release modulators
The chemokines (or chemotactic cytokines) are a large family of small, secreted proteins that signal via G protein-coupled heptahelical chemokine receptors on the cell surface (Hughes and Nibbs, 2018). They act primarily by stimulating cell migration and are the “primary regulators” of the movement and positioning of many inflammation-associated cells such as macrophages, lymphocytes, neutrophils and dendritic cells in the body. Chemokines therefore play a remarkable role in the development of inflammatory responses in vivo in terms of leukocyte recruitment and are involved in all destructive or protective inflammatory responses (Zlotnik and Yoshie, 2012). The studies reporting the inhibitory actions of FMNT on chemokines are discussed (Table 1).
Some researchers have reported the chemokines inhibitory effect of FMNT. In this study, the authors noted that FMNT treatment reduced eotaxin and granulocyte-macrophage colony-stimulating factor (GM-CSF) secretion in JME/CF15 cells after IL-13 stimulation in a dose-dependent manner (Huang et al., 2021). In another study, the chemokine inhibitory features of FMNT also have been displayed in rat chondrocytes induced by IL-1β. According to the authors, FMNT lowered the level of several chemokines, including fractalkine, macrophage inflammatory protein-3α, and monocyte chemoattractant protein-1, and showed brilliant osteoarthritis relief (Cho et al., 2019).
2.2 Mechanism intervention
2.2.1 COX2/PGE2 pathway inhibition
PGE2 produced by mPGES-1 plays a crucial role in inflammation (Chang and Meuillet, 2011; Finetti et al., 2012). Isoform two of cyclooxygenase (COX2) converts arachidonic acid (AA) to PGH2, an unstable metabolite, which is then isomerized by mPGES-1 to inflammatory PGE2. Thus, COX2 inhibition has a specific value in the onset and duration of inflammatory diseases. Several studies reporting COX2 inhibitory properties of FMNT are summarized in this section (Table 2).
Studies have proven that chondrocyte dysfunction, such as type II collagen degradation and inflammation, is associated with joint disease like rheumatoid arthritis and osteoarthritis (Menu and Vince, 2011). Researchers reported anti-inflammatory role of FMNT via primary Rat Chondrocytes. According to the authors, 25 and 50 μM FMNT markedly downregulated the expression of PGE2 as well as COX2 in primary rat chondrocytes treated with 10 ng/mL IL-1β, in which the specific production of PGE2 was inhibited by 98% ± 3% and 77% ± 2.2%, respectively compared to control group. Results above suggested the counteracting role of FMNT on IL-1β-induced catabolic pro-inflammatory effects in primary rat chondrocytes and may counteract IL-1β-induced articular cartilage degeneration (Cho et al., 2019).
Scholars investigated the protective effect of FMNT on ox-LDL-induced endothelial dysfunction via network pharmacology and experimental validation. According to the authors, FMNT had an effect on anesthetizing ox-LDL-induced inflammation and apoptosis in HUVECs through downregulation of COX2, eNOS, and cleaved caspase-3 expression (Zhang et al., 2021). FMNT treatment also suppressed the level of PGE2 in LPS-treated RAW264.7 macrophage cells in a dose-dependent manner (Kim et al., 2019). What’s more valuable is that the authors of this study synthesized a novel bioinnovative product (formononetin 7-O-phosphate), which has lower cytotoxicity and stronger anti-inflammatory activity compared to FMNT. Moreover, other researchers reported the influence of FMNT on neuroinflammation in LPS-treated BV2 microglia. According to the authors, FMNT dramatically decreased the level of PGE2, iNOS and COX2, providing strong support for the development of new compounds with selective neuroprotective effects in the brain (El-Bakoush and Olajide, 2018). In addition, the COX2 inhibitory properties of FMNT against methotrexate-induced acute kidney injury in Wistar rats were evaluated. The authors verified that rats treated with FMNT had enhanced anti-inflammatory features, compared with positive controls due to its capacity to significantly downregulate COX2 production (Aladaileh et al., 2019). All in all, these results provide evidence that FMNT is capable of blocking the conversion of arachidonic acid to PGE2 by inhibiting COX2, thereby ameliorating the inflammatory response. Additionally, the inhibition of COX2 may be the result of restrain of one or more inflammatory intracellular and intercellular signaling cascades.
2.2.2 NF-κB pathway inhibition
NF-κB is a key transcriptional regulator that controls the expression of many inflammatory and immune genes. It is inactivated in the cytoplasm by binding to I-κB. Once activated by stimuli such as viruses, oxidants and inflammatory cytokines, NF-κB dissociates from I-κB and binds to a specific promoter in the nucleus, thereby regulating gene expression and activate the transcription of TNF-α, IL-6, IL-1β and COX2. Excessive activation of NF-κB is associated with cancers, immune cell dysplasia, delayed cell growth as well as many chronic inflammatory diseases, including rheumatoid arthritis, asthma, AIDS, atherosclerosis and neurodegenerative diseases. Thus, in this portion, we review several studies that have reported the inhibitory properties of FMNT on NF-κB (Table 3).
Allergic diseases, which include food allergies, asthma, anaphylaxis, sinusitis and atopic dermatitis, are triggered by allergens and feature adaptive allergic inflammation. In a study, the NF-κB inhibitory role of FMNT was assessed in a mast cell-mediated allergic inflammation model. Results indicated that the properties of FMNT were correlated with a restriction of NF-κB activation and upstream IκKα phosphorylation in a dose-dependent manner (Xu and An, 2017). However, this study was lack of the exploration of the dosage of FMNT, which was addressed in other articles. The NF-κB inhibitory effect of FMNT in an ovalbumin-induced asthmatic female BALB/c mice model was also been evaluated. According to the authors, FMNT treatments appeared to observably inhibit the activation of NF-κB, certifying distinct therapeutic effects of FMNT in attenuating airway inflammation (Yi et al., 2020). A20 is a negative regulator of NF-κB, which plays an integral role in the regulation of immune and inflammatory responses (Priem et al., 2020). In another study, researchers supplied evidence of the anti-inflammatory ability of FMNT against FITC-treated atopic dermatitis mice model as well as siA20 and siGPER-transfected HaCaT cells model. As reported, FMNT (10 mg/kg, i.p.) was capable of attenuating atopic dermatitis by enhancing A20 expression via GPER activation (Yuan et al., 2021). All of the above studies indicated that FMNT had a clear therapeutic effect on allergic inflammation.
A study investigated the anxiolytic role of FMNT against chronic inflammatory pain responses in C57BL/6 male mice. The FMNT administration has shown to inhibit NF-κB signaling and microglial activation in the mice with chronic inflammatory pain, thereby relieving the anxiety (Wang et al., 2019). Meanwhile, FMNT also has a non-negligible role in the regulation of autoimmune diseases. For example, studies showed that FMNT decreased the level of p-NF-κB p65 and increased the level of IκBα, the inhibitor of NF-κB, in the liver from concanavalin-A-induced autoimmune hepatitis mice, showing a powerful immunomodulatory property (Liu et al., 2021). FMNT also possess activity of restraining monocrotaline-treated pulmonary arterial hypertension, whose pharmacological mechanism was related to the downregulation of phosphorylated ERK and NF-κB (Wu et al., 2020). In addition to the inflammation model mentioned above, NF-κB pathway plays a critical role in mastitis. Scholars determined the protective effect of FMNT in LPS-induced mastitis in female lactating C57BL/6 mice and mouse mammary epithelial cells EpH4-Ev, and they observed an obvious inhibition of NF-κB signaling pathway activation in both in vitro and in vivo experiments (Xiang et al., 2022).
2.2.3 MAPK pathway inhibition
The MAPK signaling pathway, a highly conserved serine/threonine protein kinase, is one of the most fundamental regulatory mechanisms in eukaryotic cells and part of a vital signaling system (Krishna and Narang, 2008). There are three well-characterized subfamilies of MAPKs in mammals: the ERKs, the JNKs and the p38 kinases (Cargnello and Roux, 2011). Of the three types of MAPKs, ERKs and JNKs are closely associated with some physiological processes, such as immunity, inflammation and tissue growth.
Several studies have shown the inhibition of FMNT to the activation of MAPK signaling pathways (Table 4). For instance, researchers revealed that FMNT administration was capable of down-regulating the expression of JNK1, ERK1 and p38α, which are compactly associated with MAPK pathway in LPS-induced inflammatory zebrafish models, thus showing a dramatic anti-inflammatory activity (Luo et al., 2019). Besides, in another experiment, the potential of FMNT for ameliorating cholestasis has been assessed. This study found that FMNT attenuated alpha-naphthyl isothiocyanate-induced inflammatory response through inactivation of JNK inflammatory pathway in a PPARα-dependent manner (Yang et al., 2019).
2.2.4 JAK-STAT pathway inhibition
JAK/STAT signaling pathway, one of the key central communication nodes for many cellular functions and processes, is a means of intracellular signaling in response to growth hormones and cytokines (Darnell, 1997). Its aberrant phosphorylation triggers the overexpression of key mediators associated with various autoimmune and inflammatory diseases (Liongue and Ward, 2013; Schwartz et al., 2017). Several of these studies presenting the JAK-STAT inhibitory effects of FMNT are reviewed in this section (Table 4).
In a study, researchers tested anti-inflammatory properties of FMNT on cerebral ischemia-reperfusion injury model in SD rats. Using Western blotting they found that FMNT inhibited the protein expression of p-JAK2 and p-STAT3 at a dose of 30 mg/kg body weight, indicating prominent JAK2/STAT3 signaling pathway inhabitation (Yu et al., 2022). In another study, FMNT was shown to lessen constitutive STAT3 and STAT5 activation in U266 and RPMI 8226 cells belonging to myeloma cell line after FMNT therapy. More interestingly, the raised production of reactive oxygen species (ROS) due to a GSH/GSSG imbalance was involved in above effect (Kim et al., 2018).
2.2.5 NLRP3 inflammasome inhibition
Inflammasome is a group of intracellular polymeric protein complexes that activate inflammatory caspase-1. Its activation is a major inflammatory pathway and a key participant in innate immunity discovered in recent years (Franchi et al., 2009). Several inflammasomes have been identified, including NLRP2, NLRP7 and NLRP12, of which NLRP3 is the best characterized (Khare et al., 2012; Vladimer et al., 2012; Minkiewicz et al., 2013). NLRP3 is essential for host immune defense and is closely related to a variety of autoimmune and autoinflammatory diseases when it is disrupted (Menu and Vince, 2011). Several of these studies presenting the NLRP3 inflammasome inhibitory effects of FMNT are reviewed in this section (Table 4).
In a recent study, scholars explored therapies for lung injury and fibrosis and found that FMNT nanomedicines could block macrophage pyrodeath involved in NLRP3, thereby improving lung function and longevity in mice (Ouyang et al., 2023). FMNT was also found to suppress streptozotocin-induced NLRP3 protein overexpression in diabetic male C57BL/6 J mice (Wang et al., 2018). Other scholars assessed the NLRP3 inflammasome signaling pathway inhibitory activity of FMNT in vitro. They discovered that FMNT (25 μM, 50 μM) treatment to acute injury model of human colon carcinoma cell line (HCT-116) induced by TNF-α for 12 h led to the downregulation of NLRP3 inflammasome expression levels. Furthermore, they also performed simultaneous in vivo experiments and found that NLRP3 expression was diminished in the colon tissue of dextran sulfate sodium-induced acute colitis mice after FMNT treatment. This finding is consistent with the in vitro experiments (Wu et al., 2018).
The above evidence suggests that FMNT is a very promising anti-inflammatory agent that can inhibit the release of cytokines and chemokines by down-regulating the over-activated COX2/PGE2 pathway, NF-κB pathway, MAPK pathway, JAK-STAT pathway and reducing the production of NLRP3 inflammasome. It is well known that FMNT can be used in the treatment of multiple diseases such as liver injury, kidney injury, central nervous system and airway inflammation. Based on in vitro and in vivo studies of the mechanisms involved, we have summarized schematically the interaction pathways for the anti-inflammatory activity of FMNT as shown in Figure 2.
3 Anti-oxidative stress molecular targets and mechanisms of FMNT
Oxidative stress is an imbalance between oxidants and antioxidants that tends to favor oxidants, which leads to dysfunction of redox signaling and control and/or molecular damage (Sies et al., 2017). Oxidative stress can be triggered in a variety of diseases, including liver diseases, cardiovascular diseases and psychiatric disorders (Ng et al., 2008; Magenta et al., 2013; Jadeja et al., 2017). Noxious external stimuli first causes a large accumulation of ROS in the cell. On the one hand, excess ROS promotes the phosphorylation of its downstream AKT by activating phosphatidylinositol-3-kinase (PI3K). Normally, Kelch-like ECH-associated protein1 (Keap1) binds to nuclear factor erythroid-2 related factor 2 (Nrf2) in the cytoplasm in an inactive state. Phosphorylated AKT acts on the Keap1-Nrf2 conjugate, destabilizing its binding and releasing Nrf2. Free Nrf2 is translocated to the nucleus and binds to antioxidant response element (ARE), activating the transcription of downstream genes and increasing the expression of heme oxygenase-1 (HO-1) and antioxidant enzymes. On the other hand, excess ROS can also activate silent information regulator 1 (SIRT1). Activated SIRT1 directly promotes the deacetylation and subsequent activation of Nrf2, ultimately leading to the upregulation of Nrf2 target genes and increased antioxidant capacity. At the same time, excessive ROS can also increase the gene expression of peroxisome proliferator-activated receptor gamma (PPARγ), further enhancing antioxidant capacity. Interestingly, FMNT has been demonstrated to have the potential to promote these processes, leading to the production of more antioxidant enzymes and alleviating the damage to the body by oxidative stress.
3.1 ROS inactivation
ROS in the body refers to the general term of oxygen-containing and active substances composed of oxygen. Normal intracellular ROS acts as messengers in gene expression, signal transduction, and homeostasis. When ROS is excessive, oxidative stress will be triggered, which destroys many of the biological macromolecules in the cell and leads to apoptosis. High level of ROS leads to the formation of free radicals, which are highly oxidizing and can damage the body’s tissues and cells, leading to chronic disease and the effects of aging (Liochev, 2013).
FMNT may reduce oxidative stress in liver disease, showing hepatoprotective potential. Mitochondrial dysfunction produces excess ROS, while FMNT promoted PHB2/PINK1/Parkin-mediated mitophagy pathway, reduced the production of ROS and then alleviated ischemia/reperfusion-induced liver injury (Ma et al., 2022). The H2O2 molecule is a precursor of free radicals that can cause cell death (Halliwell, 1989). A study explored the inhibiting effect of FMNT on oxidative stress in H2O2-treated Chang Liver cells. The authors discovered that H2O2 decreased antioxidant activity and increased ROS levels, which was reversed after FMNT treatment (Li et al., 2015). The study lacks of reliability because it did not mention the dosage of FMNT. Ritonavir is a protease inhibitor of human immunodeficiency virus that has multiple adverse effects, including hepatotoxicity. Fortunately, FMNT was found to be succeed in mitigating ritonavir-induced hepatotoxicity through lowering the formation of ROS, thereby reducing its side effects and increasing its safety (Alauddin et al., 2018). In addition, researchers observed the gastric protective effect of FMNT (10 mg/kg) on a gastric ulcer rat model induced by ethanol and non-steroidal anti-inflammatory drugs. In this study, they were surprised to find that FMNT significantly reduced the total gastric injury area and gastric fluid secretion, and increased the amount of mucus secretion in rats with gastric ulcer by scavenging free radicals produced by oxidative stress (Mendonça et al., 2020). Besides, FMNT (40 mg/kg) also markedly alleviated airway oxidative stress in mice with allergic asthma by reducing ROS (Yi et al., 2020).
3.2 Enzymatic antioxidants activation
Enzymatic antioxidants, comprised of CAT, GSH-Px, SOD and Trx systems, provide a more efficient protection against brisk oxidative attacks due to their competence to break down ROS. By degrading the hydrogen peroxide into molecular oxygen and water, CAT has the capacity to neutralise the hydrogen peroxide. The main biomolecular function of GSH-Px is to remove lipid hydroperoxides, protecting cells from oxidative damage (Dokic et al., 2012). As for SOD, the most powerful antioxidant in the body, can be categorised into mitochondrial Mn-SOD, cytosolic CuZn-SOD and extracellular SOD (He et al., 2018). As a result, these antioxidases play immeasurable roles in pathological states that include I/R injury, viscera injury, acute myocardial infarction, traumatic brain injury and inflammation (Table 5).
Study found that FMNT could stimulate the activation of the Nrf2 pathway, producing the majority of cytoprotective proteins. These processes regulate the transcription of antioxidant genes (such as SOD and GSH-Px) through a series of biochemical cascades, thereby alleviating neuroinflammation and playing a neuroprotective role (Li et al., 2014). Another study revealed that FMNT was able to elevate levels of SOD, CAT and GSH-Px in db/db mice, alleviating diabetic nephropathy (Lv et al., 2020). Studies of ischemia reperfusion showed apparent roles of FMNT in improving the activities of CAT, SOD and GSH-Px, and relieved damage of the cell membrane and increase in permeability evoked by superfluous oxygen-free radicals (Zhao et al., 2021; Ma et al., 2022). Except for ischemia-reperfusion, oxidative stress caused by overproduction of ROS and depletion of antioxidants in the defense system has also been implicated in the pathogenesis of acute myocardial infarction (AMI). Not surprisingly, FMNT reduced H2O2-induced apoptosis, via increasing the level or activity of antioxidant enzymes and inhibiting the activation of NF-κB, a key transcription factor for apoptosis (Jia et al., 2014). In addition, sodium formononetin-3′-sulphonate (Sul-F), a derivative of FMNT, has been reported to boost the expression of antioxidant enzymes that were consumed in heart tissue in the AMI model (Zhang et al., 2011). However, in this article, the author did not make comparison with FMNT and could not directly observe the advantages of Sul-F. Taken together, these findings suggested that FMNT may serve as a promising drug candidate to exert antioxidant effects in a variety of acute and chronic diseases.
3.3 Nrf2/HO-1 pathway activation
Nrf2 is an emerging cellular antioxidant regulator, controlling the basal and inducible expression of a number of antioxidant response element-dependent genes, regulating the physiological and pathophysiological consequences of ROS exposure (Ma, 2013). HO-1 is a heme degradation enzyme, and heme metabolites can act as antioxidative mediators of HO-1 cell protection, reducing ROS damage. As an indispensable regulatory pathway for intracellular antioxidative stress defense, Nrf2/HO-1 signaling pathway is actively engaged in apoptosis, anti-inflammation, antioxidation and other processes (Table 6), making it of great interest (Morita, 2005; Luo et al., 2019).
Oxidative stress is considered a major contributor to traumatic brain injury. A study assessed the HO-1 stimulatory properties of FMNT in rats with traumatic brain injury and found that FMNT pretreatment for 5 days can significantly upregulate HO-1 expression in model rats, showing neuroprotective property (Li et al., 2017). In addition, another study reported the Nrf2 stimulatory activities of FMNT in human non-tumor hepatic cells LO2 and an acetaminophen-induced hepatotoxicity mouse model. The authors observed that FMNT had the potential to enhance Nrf2 expression in both in vivo and in vitro liver disease models (Jin et al., 2017). In parallel, FMNT treatment was able to enhance Nrf2 level and then upregulate HO-1 expression in hyperoxic acute lung injury mice. To verify this result, Nrf2 inhibitors was administered to pulmonary microvascular endothelial cells and found that when Nrf2 was silenced, the upregulation of HO-1 by FMNT was abrogated (Chen et al., 2021). Several studies have revealed that FMNT treatment had the ability to upregulate Nrf2/HO-1 signaling and prevented oxidative stress in rats with kidney damage caused by different factors, exhibiting renal protection (Aladaileh et al., 2019; Hao et al., 2021; Althunibat et al., 2022).
3.4 PI3K/AKT pathway regulation
Elevated levels of ROS induced oxidative stress can lead to cell death. Akt is a serine/threonine protein kinase phosphorylated and activated by extracellular factors in a PI3K-dependent way (Yang et al., 2017). As a key inhibitor of apoptosis, the PI3K/Akt signaling pathway has been shown to play an indispensable role in participating in cell metabolism and promoting cell survival (Table 7), thereby alleviating oxidative stress (Garcia-Echeverria and Sellers, 2008).
Flap necrosis is one of the main post-operative complications. In a recent study, FMNT (25 and 50 mg/kg) was inhibition of oxidative damage and enhancement of the survival of random skin flaps by activating PI3K/Akt-driven Nrf2 antioxidant defense system in a dose-dependent manner (Li et al., 2022). What’s more, researchers reported that FMNT could ameliorate chronic kidney disease-induced muscle atrophy and TNF-α-induced C2C12 myotubes via reversing myostatin-generated dephosphorylation of the PI3K/Akt pathway as well as the defective proliferation and differentiation behavior of satellite cells (Liu et al., 2021). FMNT is also known to induce cancer cells to apoptosis. FMNT was found to be able to inactivate PI3K/AKT pathway and restrained ovarian cancer cell proliferation through arrest in the sub-G0/G1 cell phase. Moreover, FMNT induced cell apoptosis involving DNA fragmentation through MMP degradation and ROS accumulation, with subsequent P38 activation in OV90 and ES2 cells (Park et al., 2018).
3.5 SIRT1 activation
As a class III nicotinamide adenine dinucleotide (NAD)-dependent histone deacetylase, SIRT1 has been shown to regulate critical metabolic activities such as apoptosis, ageing and oxidative stress by deacetylating a diverse range of zymolytes (Oberdoerffer et al., 2008; Yu and Auwerx, 2010). An elevation of ROS activates SIRT1, which in turn leads to a decrease of the ROS (Zhang et al., 2017). Therefore, SIRT1 plays a considerable role in anti-oxidative stress, which has been validated by quite a few studies (Table 7).
Type 2 diabetes brings multiple complications in the clinic, the most common of which are vascular complications, including diabetic nephropathy, diabetic neuropathy, and more, most of which are affiliated with inactivated SIRT1. In a study, Male db/db mice were gavaged with 25 and 50 mg/kg/day FMNT for 8 weeks and sacrificed. While the result emerged that FMNT upregulated the level of quenched Sirt1 to activation of the Nrf2/ARE pathway (Zhuang et al., 2020). In other studies, Oza assessed the SIRT1 stimulatory properties of FMNT to kidney damage and neuropathy in type 2 diabetes rats, and spotted that the low expression of SIRT1 was observably reversed after administration with FMNT for 16 weeks (Oza and Kulkarni, 2019; 2020). Above articles illustrated an efficacious antioxidation of FMNT in the control of type 2 diabetes. Other researchers used an in-depth method of small interfering RNA-mediated gene silencing to explore the inhibition effect of FMNT in LPS-induced high-mobility group box 1 release through PPARδ-dependent activation of SIRT1 (Hwang et al., 2018). These findings have profound connotation for our comprehension of the molecular mechanism underlying the transcriptional regulation of SIRT1.
3.6 PPARγ activation
PPARγ is a member of the nuclear hormone receptor transcription factor superfamily and is an active participant in the regulation of genes involved in metabolism, inflammation, immunity, cell proliferation and differentiation (Willson et al., 2000; Schupp et al., 2009; Rogue et al., 2010). Oxidative stress induces a decline in PPARγ levels and activity by downregulating PPARγ transcription, potentially involved in the activation of inhibitory redox-regulated transcription factors (Blanquicett et al., 2010). Thus, elevation of PPARγ represents a promising strategy.
Previous studies have demonstrated that FMNT exerted a promoting effect on PPARγ (Table 7). In the case of acute lung injury mice induced by LPS, the downregulated PPAR-γ gene expression was obviously improved upon FMNT treatment (Ma et al., 2013). In addition, treatment with FMNT also resulted in a provoke of PPAR-γ signaling in ox-LDL-stimulated endothelial injury in HUVECs, thus supplying a theoretical basis for FMNT as a potential anti-atherosclerotic drug (Zhang et al., 2021). However, the results of this experiment were relatively simple, as only one concentration of FMNT (40 µM) was tested and no positive drugs were compared.
The above evidence implies that FMNT is a highly potent antioxidant that can stimulate the expression of antioxidant enzymes by promoting the activation of Nrf2/HO-1 pathway, PI3K/AKT pathway, SIRT1 and PPARγ, which in turn eliminates the excessive accumulation of ROS, the “killer” for oxidative stress, and alleviate ROS-induced tissue and cell damage. It plays an exceptional antioxidant role in various pathological models such as cancer, neurological diseases, fibrotic diseases, allergic diseases, metabolic diseases, cardiovascular diseases, gastrointestinal diseases and autoimmune diseases. We have summarized the interaction pathway of FMNT’s antioxidant activity based on previous studies of relevant mechanisms in vitro and in vivo, as shown in Figure 3.
4 Absorption, bioavailability, metabolism and toxicity of FMNT
The pharmacokinetic study of FMNT in rats showed that FMNT had poor oral activity, with a bioavailability of 21.8% after a single oral dose. FMNT is rapidly absorbed in the intestinal tract of animals, distributed throughout the body for a short period of time, then rapidly eliminated and metabolized to sulfate and glucuronide for excretion (Jeong et al., 2005; Luo et al., 2018). The relatively low bioavailability of FMNT may be due to its poor water solubility as well as its short half-life (2.1 h).
To date, the vast majority of reports have indicated that FMNT is non-toxic. However, when used with ultra-high doses, FMNT was found to cause low toxicity in some models. Researchers conducted a 90-day subchronic toxicity study using Sul-Fat doses of 0, 33.3, 100 and 300 mg/kg. The results of this study showed that intravenous administration of Sul-F at a dose of 300 mg/kg produced a white-crystal (non-metabolic Sul-F) and transient vomiting in Beagle dogs. Under these conditions, the no observed adverse effect level (NOAEL) of Sul-F was 100 mg/kg in dogs (Li et al., 2016). Recently an acute and sub-acute toxicity research of FMNT has been conducted in Swiss albino mice. During the acute toxicity trial, it was found that the LD50 for FMNT was assessed to be 103.6 mg/kg, with a NOAEL of 50 mg/kg, whereas mortality was existing in the 300 mg/kg dose group and pathological changes like a mild diffuse granular degeneration in the liver were noted. Noteworthily, during subacute study, there were no changes in the mice’s organ tissue structures or metabolism after 28 days of intraperitoneal injections of FMNT at doses of 12.5, 25 and 50 mg/kg (Pingale and Gupta, 2023).
5 Conclusion and future prospects
Traditional knowledge-based botanicals and their active metabolites are usually treated as one of the alternatives of therapy. In recent years, bioactive metabolites derived from natural plants have appealed great interest to researchers due to their wide range of anti-inflammatory and antioxidant properties. FMNT is a widely available isoflavone with a wide range of biological activities. It is currently in use and has several patent outputs in human and animal nutritional supplementation, crop fungicide, and weight control. Oxidative stress and inflammation are inextricably linked, so limiting oxidative stress is a strategy for controlling inflammation. This present study comprehensively reviews and summarizes the antioxidant and anti-inflammatory activities of FMNT and their relevant mechanisms of action in vitro and in vivo. It was found that FMNT has an excellent alleviating effect in inflammation accompanied with cancer, neurological diseases, fibrotic diseases, allergic diseases, metabolic diseases, cardiovascular diseases, gastrointestinal diseases and autoimmune diseases. However, the results of these effects are inconsistent and the doses used vary widely across reports, thus the results need to be critically assessed and analyzed as they depend on the level and type of health of the subject. Studies have shown that most human studies of isoflavones and flavonoids have been unsuccessful, mainly due to their poor oral bioavailability. Noteworthy, the researchers synthesized several derivatives of FMNT through chemical and biological modifications: FMNT-7-o-phosphate, sulphonated FMNT, FMNT-7-o-β -(600-O-succinyl) -d-Glucoside, and FMNT-poly (ethylene glycol). Their chemical structures are shown in Figure 1. These novel FMNT derivatives improve FMNT’s relatively low bioavailability, poor water solubility, and systemic toxicity, thereby enhancing its anti-inflammatory and antioxidant activity. More interestingly, coupling with targeting peptide, FMNT derivatives enable targeted and precise drug delivery. Taken together, we clearly understand that FMNT derivatives are more potent than FMNT, and in some of the literature we have found the benefits of long-term FMNT supplementation. Despite the strong anti-inflammatory and antioxidant potential of FMNT in disease models, more research is required into the side effects of long-term and heavy dose of FMNT in healthy tissues and cells before it can be considered for use in areas other than medicine.
At present, the mechanism research of FMNT still has the following shortcomings. First, there is a great variation in dosage, so it is necessary to explore the minimum active concentration of FMNT and standardize the dosage. Second, these articles are very original, but future research needs improvement in the normativity of species names. This is what most articles about FMNT lack of. Third, the signal pathways are relatively common, and the effects of FMNT on new or rare pathways are rarely explored. Finally, gene knockout and inhibitor research methods are also rarely used in published studies. To fully understand the mechanistic pathways behind the anti-inflammatory and antioxidant properties of FMNT, more research gaps need to be filled. Future perspectives should focus on investigating the optimal benefits of FMNT, especially recommendations for long-term intake and forms of intake. Its mild or no toxicity allows it to be formed into more products for commercial applications. For example, in the field of pharmaceuticals, it may be a lead molecule for the treatment of diseases related to oxidative stress and inflammation. Alternatively, as a material or supplement for the manufacture of anti-tumour, lipid-lowering, anti-allergy and immunosuppressive drugs. It can also be used to promote wound healing, reduce scar hyperplasia and infection and other post-operative sequelae by promoting skin flap survival. In the field of food, it may be used as dietary supplements for purposes of improvement of function, balance of metabolism and reduction of disease risk. As to the field of cosmetics, it is a wise choice to develop FMNT into cosmeceuticals related to the alleviation of inflammation and removal of acne in the skin. Consider the oxidation resistance of FMNT, it may be a wonderful innovation as a source for cosmeceuticals against skin aging. Research of FMNT in the future should focus on how to maximize the bioactive effects of FMNT with appropriate dosing regimens and supplementation forms for humans.
Author contributions
MD: Investigation, Validation, Writing–original draft, Writing–review and editing. YB: Conceptualization, Writing–review and editing. HL: Formal Analysis, Validation, Writing–review and editing. XZ: Supervision, Writing–review and editing. BL: Writing–review and editing. RY: Funding acquisition, Methodology, Supervision, Validation, Writing–review and editing. NZ: Funding acquisition, Methodology, Supervision, Writing–review and editing.
Funding
The author(s) declare financial support was received for the research, authorship, and/or publication of this article. This research was funded by the Open Science Fund of Chengdu University of Traditional Chinese Medicine (2020XSGG002), Xinglin Scholar Program of Chengdu University of Traditional Chinese Medicine (QJJJ2022012).
Conflict of interest
The authors declare that the research was conducted in the absence of any commercial or financial relationships that could be construed as a potential conflict of interest.
Publisher’s note
All claims expressed in this article are solely those of the authors and do not necessarily represent those of their affiliated organizations, or those of the publisher, the editors and the reviewers. Any product that may be evaluated in this article, or claim that may be made by its manufacturer, is not guaranteed or endorsed by the publisher.
Supplementary material
The Supplementary Material for this article can be found online at: https://www.frontiersin.org/articles/10.3389/fphar.2024.1368765/full#supplementary-material
References
Aladaileh, S. H., Hussein, O. E., Abukhalil, M. H., Saghir, S. A. M., Bin-Jumah, M., Alfwuaires, M. A., et al. (2019). Formononetin upregulates Nrf2/HO-1 signaling and prevents oxidative stress, inflammation, and kidney injury in methotrexate-induced rats. Antioxidants (Basel) 8, 430. doi:10.3390/antiox8100430
Alauddin, , Chaturvedi, S., Malik, M. Y., Azmi, L., Shukla, I., Naseem, Z., et al. (2018). Formononetin and biochanin A protects against ritonavir induced hepatotoxicity via modulation of NfκB/pAkt signaling molecules. Life Sci. 213, 174–182. doi:10.1016/j.lfs.2018.10.023
Althunibat, O. Y., Abukhalil, M. H., Aladaileh, S. H., Qaralleh, H., Al-Amarat, W., Alfwuaires, M. A., et al. (2022). Formononetin ameliorates renal dysfunction, oxidative stress, inflammation, and apoptosis and upregulates Nrf2/HO-1 signaling in a rat model of gentamicin-induced nephrotoxicity. Front. Pharmacol. 13, 916732. doi:10.3389/fphar.2022.916732
Blanquicett, C., Kang, B.-Y., Ritzenthaler, J. D., Jones, D. P., and Hart, C. M. (2010). Oxidative stress modulates PPAR gamma in vascular endothelial cells. Free Radic. Biol. Med. 48, 1618–1625. doi:10.1016/j.freeradbiomed.2010.03.007
Bottamedi, M., Pereira Dos Santos Nascimento, M. V., Fratoni, E., Kinoshita Moon, Y. J., Faqueti, L., Tizziani, T., et al. (2021). Antioxidant and anti-inflammatory action (in vivo and in vitro) from the trunk barks of Cabreúva (Myrocarpus frondosus Allemao, Fabaceae). J. Ethnopharmacol. 267, 113545. doi:10.1016/j.jep.2020.113545
Cargnello, M., and Roux, P. P. (2011). Activation and function of the MAPKs and their substrates, the MAPK-activated protein kinases. Microbiol. Mol. Biol. Rev. 75, 50–83. doi:10.1128/MMBR.00031-10
Chang, H.-H., and Meuillet, E. J. (2011). Identification and development of mPGES-1 inhibitors: where we are at? Future Med. Chem. 3, 1909–1934. doi:10.4155/fmc.11.136
Chen, Y., Wei, D., Zhao, J., Xu, X., and Chen, J. (2021). Reduction of hyperoxic acute lung injury in mice by Formononetin. PLoS One 16, e0245050. doi:10.1371/journal.pone.0245050
Cho, I.-A., Kim, T.-H., Lim, H., Park, J.-H., Kang, K.-R., Lee, S.-Y., et al. (2019). Formononetin antagonizes the interleukin-1β-induced catabolic effects through suppressing inflammation in primary rat chondrocytes. Inflammation 42, 1426–1440. doi:10.1007/s10753-019-01005-1
Darnell, J. E. (1997). STATs and gene regulation. Science 277, 1630–1635. doi:10.1126/science.277.5332.1630
Dokic, I., Hartmann, C., Herold-Mende, C., and Régnier-Vigouroux, A. (2012). Glutathione peroxidase 1 activity dictates the sensitivity of glioblastoma cells to oxidative stress. Glia 60, 1785–1800. doi:10.1002/glia.22397
El-Bakoush, A., and Olajide, O. A. (2018). Formononetin inhibits neuroinflammation and increases estrogen receptor beta (ERβ) protein expression in BV2 microglia. Int. Immunopharmacol. 61, 325–337. doi:10.1016/j.intimp.2018.06.016
Finetti, F., Terzuoli, E., Bocci, E., Coletta, I., Polenzani, L., Mangano, G., et al. (2012). Pharmacological inhibition of microsomal prostaglandin E synthase-1 suppresses epidermal growth factor receptor-mediated tumor growth and angiogenesis. PLoS One 7, e40576. doi:10.1371/journal.pone.0040576
Franchi, L., Eigenbrod, T., Muñoz-Planillo, R., and Nuñez, G. (2009). The inflammasome: a caspase-1-activation platform that regulates immune responses and disease pathogenesis. Nat. Immunol. 10, 241–247. doi:10.1038/ni.1703
Garcia-Echeverria, C., and Sellers, W. R. (2008). Drug discovery approaches targeting the PI3K/Akt pathway in cancer. Oncogene 27, 5511–5526. doi:10.1038/onc.2008.246
Halliwell, B. (1989). Protection against tissue damage in vivo by desferrioxamine: what is its mechanism of action? Free Radic. Biol. Med. 7, 645–651. doi:10.1016/0891-5849(89)90145-7
Hao, Y., Miao, J., Liu, W., Peng, L., Chen, Y., and Zhong, Q. (2021). Formononetin protects against cisplatin-induced acute kidney injury through activation of the PPARα/Nrf2/HO-1/NQO1 pathway. Int. J. Mol. Med. 47, 511–522. doi:10.3892/ijmm.2020.4805
He, L., Zhang, J., Zhao, J., Ma, N., Kim, S. W., Qiao, S., et al. (2018). Autophagy: the last defense against cellular nutritional stress. Adv. Nutr. 9, 493–504. doi:10.1093/advances/nmy011
Huang, J., Chen, X., and Xie, A. (2021). Formononetin ameliorates IL-13-induced inflammation and mucus formation in human nasal epithelial cells by activating the SIRT1/Nrf2 signaling pathway. Mol. Med. Rep. 24, 832. doi:10.3892/mmr.2021.12472
Hughes, C. E., and Nibbs, R. J. B. (2018). A guide to chemokines and their receptors. FEBS J. 285, 2944–2971. doi:10.1111/febs.14466
Hwang, J. S., Kang, E. S., Han, S. G., Lim, D.-S., Paek, K. S., Lee, C.-H., et al. (2018). Formononetin inhibits lipopolysaccharide-induced release of high mobility group box 1 by upregulating SIRT1 in a PPARδ-dependent manner. PeerJ 6, e4208. doi:10.7717/peerj.4208
Jadeja, R. N., Devkar, R. V., and Nammi, S. (2017). Oxidative stress in liver diseases: pathogenesis, prevention, and therapeutics. Oxid. Med. Cell Longev. 2017, 8341286. doi:10.1155/2017/8341286
Jeong, E. J., Jia, X., and Hu, M. (2005). Disposition of formononetin via enteric recycling: metabolism and excretion in mouse intestinal perfusion and Caco-2 cell models. Mol. Pharm. 2 (4), 319–328. PMID: 16053335. doi:10.1021/mp0498852
Jia, W. C., Liu, G., Zhang, C. D., and Zhang, S. P. (2014). Formononetin attenuates hydrogen peroxide (H2O2)-induced apoptosis and NF-κB activation in RGC-5 cells. Eur. Rev. Med. Pharmacol. Sci. 18, 2191–2197.
Jin, F., Wan, C., Li, W., Yao, L., Zhao, H., Zou, Y., et al. (2017). Formononetin protects against acetaminophen-induced hepatotoxicity through enhanced NRF2 activity. PLoS One 12, e0170900. doi:10.1371/journal.pone.0170900
Khansari, N., Shakiba, Y., and Mahmoudi, M. (2009). Chronic inflammation and oxidative stress as a major cause of age-related diseases and cancer. Recent Pat. Inflamm. Allergy Drug Discov. 3, 73–80. doi:10.2174/187221309787158371
Khare, S., Dorfleutner, A., Bryan, N. B., Yun, C., Radian, A. D., de Almeida, L., et al. (2012). An NLRP7-containing inflammasome mediates recognition of microbial lipopeptides in human macrophages. Immunity 36, 464–476. doi:10.1016/j.immuni.2012.02.001
Kim, C., Lee, S.-G., Yang, W. M., Arfuso, F., Um, J.-Y., Kumar, A. P., et al. (2018). Formononetin-induced oxidative stress abrogates the activation of STAT3/5 signaling axis and suppresses the tumor growth in multiple myeloma preclinical model. Cancer Lett. 431, 123–141. doi:10.1016/j.canlet.2018.05.038
Kim, M.-S., Park, J.-S., Chung, Y. C., Jang, S., Hyun, C.-G., and Kim, S.-Y. (2019). Anti-inflammatory effects of formononetin 7-O-phosphate, a novel biorenovation product, on LPS-stimulated RAW 264.7 macrophage cells. Molecules 24, 3910. doi:10.3390/molecules24213910
Krishna, M., and Narang, H. (2008). The complexity of mitogen-activated protein kinases (MAPKs) made simple. Cell Mol. Life Sci. 65, 3525–3544. doi:10.1007/s00018-008-8170-7
Li, C., Li, G., Gao, Y., Sun, C., and Wang, X. (2016). A 90-day subchronic toxicity study with sodium formononetin-3'-sulphonate (Sul-F) delivered to dogs via intravenous administration. Regul. Toxicol. Pharmacol. 77, 87–92. doi:10.1016/j.yrtph.2016.02.016
Li, H., Jiang, R., Lou, L., Jia, C., Zou, L., and Chen, M. (2022). Formononetin improves the survival of random skin flaps through PI3K/Akt-Mediated Nrf2 antioxidant defense system. Front. Pharmacol. 13, 901498. doi:10.3389/fphar.2022.901498
Li, J., Han, L., Ma, Y.-f., and Huang, Y.-f. (2015). Inhibiting effects of three components of Astragalus membranaceus on oxidative stress in Chang Liver cells. Zhongguo Zhong Yao Za Zhi 40, 318–323.
Li, Z., Dong, X., Zhang, J., Zeng, G., Zhao, H., Liu, Y., et al. (2014). Formononetin protects TBI rats against neurological lesions and the underlying mechanism. J. Neurol. Sci. 338, 112–117. doi:10.1016/j.jns.2013.12.027
Li, Z., Wang, Y., Zeng, G., Zheng, X., Wang, W., Ling, Y., et al. (2017). Increased miR-155 and heme oxygenase-1 expression is involved in the protective effects of formononetin in traumatic brain injury in rats. Am. J. Transl. Res. 9, 5653–5661.
Li, Z., Zeng, G., Zheng, X., Wang, W., Ling, Y., Tang, H., et al. (2018). Neuroprotective effect of formononetin against TBI in rats via suppressing inflammatory reaction in cortical neurons. Biomed. Pharmacother. 106, 349–354. doi:10.1016/j.biopha.2018.06.041
Liochev, S. I. (2013). Reactive oxygen species and the free radical theory of aging. Free Radic. Biol. Med. 60, 1–4. doi:10.1016/j.freeradbiomed.2013.02.011
Liongue, C., and Ward, A. C. (2013). Evolution of the JAK-STAT pathway. JAKSTAT 2, e22756. doi:10.4161/jkst.22756
Liu, C., Chu, D., Kalantar-Zadeh, K., George, J., Young, H. A., and Liu, G. (2021a). Cytokines: from clinical significance to quantification. Adv. Sci. (Weinh) 8, e2004433. doi:10.1002/advs.202004433
Liu, G., Zhao, W., Bai, J., Cui, J., Liang, H., and Lu, B. (2021b). Formononetin protects against concanavalin-A-induced autoimmune hepatitis in mice through its anti-apoptotic and anti-inflammatory properties. Biochem. Cell Biol. 99, 231–240. doi:10.1139/bcb-2020-0197
Liu, L., Hu, R., You, H., Li, J., Liu, Y., Li, Q., et al. (2021c). Formononetin ameliorates muscle atrophy by regulating myostatin-mediated PI3K/Akt/FoxO3a pathway and satellite cell function in chronic kidney disease. J. Cell Mol. Med. 25, 1493–1506. doi:10.1111/jcmm.16238
Luo, L., Zhou, J., Zhao, H., Fan, M., and Gao, W. (2019a). The anti-inflammatory effects of formononetin and ononin on lipopolysaccharide-induced zebrafish models based on lipidomics and targeted transcriptomics. Metabolomics 15, 153. doi:10.1007/s11306-019-1614-2
Luo, L. Y., Fan, M. X., Zhao, H. Y., Li, M. X., Wu, X., and Gao, W. Y. (2018). Pharmacokinetics and bioavailability of the isoflavones formononetin and ononin and their in vitro absorption in ussing chamber and caco-2 cell models. J. Agric. Food Chem. 66 (11), 2917–2924. doi:10.1021/acs.jafc.8b00035
Luo, M., Tian, R., and Lu, N. (2019b). Nitric oxide protected against NADPH oxidase-derived superoxide generation in vascular endothelium: critical role for heme oxygenase-1. Int. J. Biol. Macromol. 126, 549–554. doi:10.1016/j.ijbiomac.2018.12.252
Lv, J., Zhuang, K., Jiang, X., Huang, H., and Quan, S. (2020). Renoprotective effect of formononetin by suppressing Smad3 expression in Db/Db mice. Diabetes Metab. Syndr. Obes. 13, 3313–3324. doi:10.2147/DMSO.S272147
Ma, Q. (2013). Role of nrf2 in oxidative stress and toxicity. Annu. Rev. Pharmacol. Toxicol. 53, 401–426. doi:10.1146/annurev-pharmtox-011112-140320
Ma, Z., Ji, W., Fu, Q., and Ma, S. (2013). Formononetin inhibited the inflammation of LPS-induced acute lung injury in mice associated with induction of PPAR gamma expression. Inflammation 36, 1560–1566. doi:10.1007/s10753-013-9700-5
Ma, Z., Zhang, D., Sun, J., Zhang, Q., Qiao, Y., Zhu, Y., et al. (2022). Formononetin inhibits hepatic I/R-Induced injury through regulating PHB2/PINK1/parkin pathway. Oxid. Med. Cell Longev. 2022, 6481192. doi:10.1155/2022/6481192
Magenta, A., Greco, S., Gaetano, C., and Martelli, F. (2013). Oxidative stress and microRNAs in vascular diseases. Int. J. Mol. Sci. 14, 17319–17346. doi:10.3390/ijms140917319
Mendonça, M. A. A. d., Ribeiro, A. R. S., Lima, A. K. d., Bezerra, G. B., Pinheiro, M. S., Albuquerque-Júnior, R. L. C. d., et al. (2020). Red propolis and its dyslipidemic regulator formononetin: evaluation of antioxidant activity and gastroprotective effects in rat model of gastric ulcer. Nutrients 12, 2951. doi:10.3390/nu12102951
Menu, P., and Vince, J. E. (2011). The NLRP3 inflammasome in health and disease: the good, the bad and the ugly. Clin. Exp. Immunol. 166, 1–15. doi:10.1111/j.1365-2249.2011.04440.x
Minkiewicz, J., de Rivero Vaccari, J. P., and Keane, R. W. (2013). Human astrocytes express a novel NLRP2 inflammasome. Glia 61, 1113–1121. doi:10.1002/glia.22499
Morita, T. (2005). Heme oxygenase and atherosclerosis. Arterioscler. Thromb. Vasc. Biol. 25, 1786–1795. doi:10.1161/01.ATV.0000178169.95781.49
Ng, F., Berk, M., Dean, O., and Bush, A. I. (2008). Oxidative stress in psychiatric disorders: evidence base and therapeutic implications. Int. J. Neuropsychopharmacol. 11, 851–876. doi:10.1017/S1461145707008401
Oberdoerffer, P., Michan, S., McVay, M., Mostoslavsky, R., Vann, J., Park, S.-K., et al. (2008). SIRT1 redistribution on chromatin promotes genomic stability but alters gene expression during aging. Cell 135, 907–918. doi:10.1016/j.cell.2008.10.025
Ouyang, B., Deng, L., Yang, F., Shi, H., Wang, N., Tang, W., et al. (2023). Albumin-based formononetin nanomedicines for lung injury and fibrosis therapy via blocking macrophage pyroptosis. Mater Today Bio 20, 100643. doi:10.1016/j.mtbio.2023.100643
Oza, M. J., and Kulkarni, Y. A. (2019). Formononetin attenuates kidney damage in type 2 diabetic rats. Life Sci. 219, 109–121. doi:10.1016/j.lfs.2019.01.013
Oza, M. J., and Kulkarni, Y. A. (2020). Formononetin ameliorates diabetic neuropathy by increasing expression of SIRT1 and NGF. Chem. Biodivers. 17, e2000162. doi:10.1002/cbdv.202000162
Park, S., Bazer, F. W., Lim, W., and Song, G. (2018). The O-methylated isoflavone, formononetin, inhibits human ovarian cancer cell proliferation by sub G0/G1 cell phase arrest through PI3K/AKT and ERK1/2 inactivation. J. Cell Biochem. 119, 7377–7387. doi:10.1002/jcb.27041
Pingale, T. D., and Gupta, G. L. (2023). Acute and sub-acute toxicity study reveals no dentrimental effect of formononetin in mice upon repeated i.p. dosing. Toxicol. Mech. Methods 33, 688–697. doi:10.1080/15376516.2023.2234026
Priem, D., van Loo, G., and Bertrand, M. J. M. (2020). A20 and cell death-driven inflammation. Trends Immunol. 41, 421–435. doi:10.1016/j.it.2020.03.001
Reuter, S., Gupta, S. C., Chaturvedi, M. M., and Aggarwal, B. B. (2010). Oxidative stress, inflammation, and cancer: how are they linked? Free Radic. Biol. Med. 49, 1603–1616. doi:10.1016/j.freeradbiomed.2010.09.006
Rogue, A., Spire, C., Brun, M., Claude, N., and Guillouzo, A. (2010). Gene expression changes induced by PPAR gamma agonists in animal and human liver. PPAR Res. 2010, 325183. doi:10.1155/2010/325183
Salzano, S., Checconi, P., Hanschmann, E.-M., Lillig, C. H., Bowler, L. D., Chan, P., et al. (2014). Linkage of inflammation and oxidative stress via release of glutathionylated peroxiredoxin-2, which acts as a danger signal. Proc. Natl. Acad. Sci. U. S. A. 111, 12157–12162. doi:10.1073/pnas.1401712111
Schupp, M., Cristancho, A. G., Lefterova, M. I., Hanniman, E. A., Briggs, E. R., Steger, D. J., et al. (2009). Re-expression of GATA2 cooperates with peroxisome proliferator-activated receptor-gamma depletion to revert the adipocyte phenotype. J. Biol. Chem. 284, 9458–9464. doi:10.1074/jbc.M809498200
Schwartz, D. M., Kanno, Y., Villarino, A., Ward, M., Gadina, M., and O'Shea, J. J. (2017). JAK inhibition as a therapeutic strategy for immune and inflammatory diseases. Nat. Rev. Drug Discov. 16, 843–862. doi:10.1038/nrd.2017.201
Sies, H., Berndt, C., and Jones, D. P. (2017). Oxidative stress. Annu. Rev. Biochem. 86, 715–748. doi:10.1146/annurev-biochem-061516-045037
Vladimer, G. I., Weng, D., Paquette, S. W. M., Vanaja, S. K., Rathinam, V. A. K., Aune, M. H., et al. (2012). The NLRP12 inflammasome recognizes Yersinia pestis. Immunity 37, 96–107. doi:10.1016/j.immuni.2012.07.006
Wang, G., Liu, H., Liu, Y., Li, H., Li, Z., Shao, G., et al. (2020). Formononetin alleviates Streptococcus suis infection by targeting suilysin. Microb. Pathog. 147, 104388. doi:10.1016/j.micpath.2020.104388
Wang, J., Wang, L., Zhou, J., Qin, A., and Chen, Z. (2018). The protective effect of formononetin on cognitive impairment in streptozotocin (STZ)-induced diabetic mice. Biomed. Pharmacother. 106, 1250–1257. doi:10.1016/j.biopha.2018.07.063
Wang, X.-S., Guan, S.-Y., Liu, A., Yue, J., Hu, L.-N., Zhang, K., et al. (2019). Anxiolytic effects of Formononetin in an inflammatory pain mouse model. Mol. Brain 12, 36. doi:10.1186/s13041-019-0453-4
Willson, T. M., Brown, P. J., Sternbach, D. D., and Henke, B. R. (2000). The PPARs: from orphan receptors to drug discovery. J. Med. Chem. 43, 527–550. doi:10.1021/jm990554g
Wu, D., Wu, K., Zhu, Q., Xiao, W., Shan, Q., Yan, Z., et al. (2018). Formononetin administration ameliorates dextran sulfate sodium-induced acute colitis by inhibiting NLRP3 inflammasome signaling pathway. Mediat. Inflamm. 2018, 3048532. doi:10.1155/2018/3048532
Wu, Y., Cai, C., Yang, L., Xiang, Y., Zhao, H., and Zeng, C. (2020). Inhibitory effects of formononetin on the monocrotaline-induced pulmonary arterial hypertension in rats. Mol. Med. Rep. 21, 1192–1200. doi:10.3892/mmr.2020.10911
Xiang, K., Shen, P., Gao, Z., Liu, Z., Hu, X., Liu, B., et al. (2022). Formononetin protects LPS-induced mastitis through suppressing inflammation and enhancing blood-milk barrier integrity via AhR-induced src inactivation. Front. Immunol. 13, 814319. doi:10.3389/fimmu.2022.814319
Xiong, W., Lan, Q., Liang, X., Zhao, J., Huang, H., Zhan, Y., et al. (2021). Cartilage-targeting poly(ethylene glycol) (PEG)-formononetin (FMN) nanodrug for the treatment of osteoarthritis. J. Nanobiotechnology 19, 197. doi:10.1186/s12951-021-00945-x
Xu, N., and An, J. (2017). Formononetin ameliorates mast cell-mediated allergic inflammation via inhibition of histamine release and production of pro-inflammatory cytokines. Exp. Ther. Med. 14, 6201–6206. doi:10.3892/etm.2017.5293
Yang, K., Zhang, H., Luo, Y., Zhang, J., Wang, M., Liao, P., et al. (2017). Gypenoside XVII prevents atherosclerosis by attenuating endothelial apoptosis and oxidative stress: insight into the erα-mediated PI3K/akt pathway. Int. J. Mol. Sci. 18, 77. doi:10.3390/ijms18020077
Yang, S., Wei, L., Xia, R., Liu, L., Chen, Y., Zhang, W., et al. (2019). Formononetin ameliorates cholestasis by regulating hepatic SIRT1 and PPARα. Biochem. Biophys. Res. Commun. 512, 770–778. doi:10.1016/j.bbrc.2019.03.131
Yi, L., Cui, J., Wang, W., Tang, W., Teng, F., Zhu, X., et al. (2020). Formononetin attenuates airway inflammation and oxidative stress in murine allergic asthma. Front. Pharmacol. 11, 533841. doi:10.3389/fphar.2020.533841
Yu, J., and Auwerx, J. (2010). Protein deacetylation by SIRT1: an emerging key post-translational modification in metabolic regulation. Pharmacol. Res. 62, 35–41. doi:10.1016/j.phrs.2009.12.006
Yu, L., Zhang, Y., Chen, Q., He, Y., Zhou, H., Wan, H., et al. (2022). Formononetin protects against inflammation associated with cerebral ischemia-reperfusion injury in rats by targeting the JAK2/STAT3 signaling pathway. Biomed. Pharmacother. 149, 112836. doi:10.1016/j.biopha.2022.112836
Yuan, W., Chen, Y., Zhou, Y., Bao, K., Yu, X., Xu, Y., et al. (2021). Formononetin attenuates atopic dermatitis by upregulating A20 expression via activation of G protein-coupled estrogen receptor. J. Ethnopharmacol. 266, 113397. doi:10.1016/j.jep.2020.113397
Zhang, B., Hao, Z., Zhou, W., Zhang, S., Sun, M., Li, H., et al. (2021). Formononetin protects against ox-LDL-induced endothelial dysfunction by activating PPAR-γ signaling based on network pharmacology and experimental validation. Bioengineered 12, 4887–4898. doi:10.1080/21655979.2021.1959493
Zhang, S., Tang, X., Tian, J., Li, C., Zhang, G., Jiang, W., et al. (2011). Cardioprotective effect of sulphonated formononetin on acute myocardial infarction in rats. Basic Clin. Pharmacol. Toxicol. 108, 390–395. doi:10.1111/j.1742-7843.2011.00676.x
Zhang, W., Huang, Q., Zeng, Z., Wu, J., Zhang, Y., and Chen, Z. (2017). Sirt1 inhibits oxidative stress in vascular endothelial cells. Oxid. Med. Cell Longev. 2017, 7543973. doi:10.1155/2017/7543973
Zhao, L., Han, J., Liu, J., Fan, K., Yuan, T., Han, J., et al. (2021). A novel formononetin derivative promotes anti-ischemic effects on acute ischemic injury in mice. Front. Microbiol. 12, 786464. doi:10.3389/fmicb.2021.786464
Zhuang, K., Jiang, X., Liu, R., Ye, C., Wang, Y., Wang, Y., et al. (2020). Formononetin activates the Nrf2/ARE signaling pathway via Sirt1 to improve diabetic renal fibrosis. Front. Pharmacol. 11, 616378. doi:10.3389/fphar.2020.616378
Zlotnik, A., and Yoshie, O. (2012). The chemokine superfamily revisited. Immunity 36, 705–716. doi:10.1016/j.immuni.2012.05.008
Glossary
Keywords: formononetin, oxidative stress, inflammation, mechanism, derivate
Citation: Ding M, Bao Y, Liang H, Zhang X, Li B, Yang R and Zeng N (2024) Potential mechanisms of formononetin against inflammation and oxidative stress: a review. Front. Pharmacol. 15:1368765. doi: 10.3389/fphar.2024.1368765
Received: 15 January 2024; Accepted: 26 April 2024;
Published: 10 May 2024.
Edited by:
Rong-Rong He, Jinan University, ChinaReviewed by:
Huang Jinling, Anhui University of Chinese Medicine, ChinaXinyu Wang, Philadelphia College of Osteopathic Medicine—Georgia Campus, United States
Copyright © 2024 Ding, Bao, Liang, Zhang, Li, Yang and Zeng. This is an open-access article distributed under the terms of the Creative Commons Attribution License (CC BY). The use, distribution or reproduction in other forums is permitted, provided the original author(s) and the copyright owner(s) are credited and that the original publication in this journal is cited, in accordance with accepted academic practice. No use, distribution or reproduction is permitted which does not comply with these terms.
*Correspondence: Nan Zeng, MTk5MzIwMTVAY2R1dGNtLmVkdS5jbg==; Ruocong Yang, eWFuZ3J1b2NvbmdAY2R1dGNtLmVkdS5jbg==