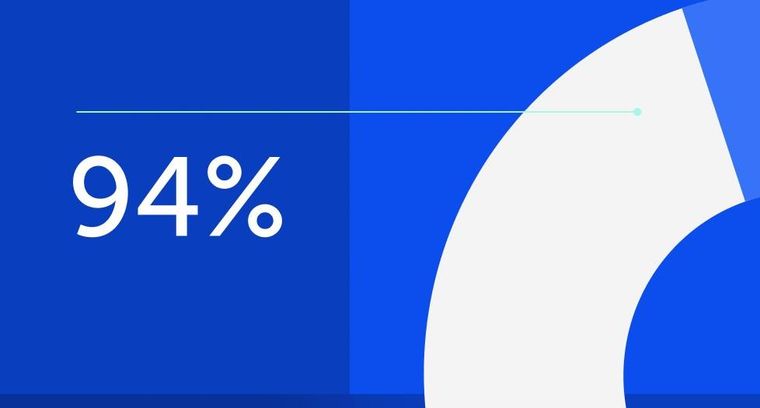
94% of researchers rate our articles as excellent or good
Learn more about the work of our research integrity team to safeguard the quality of each article we publish.
Find out more
OPINION article
Front. Pharmacol., 05 April 2024
Sec. Ethnopharmacology
Volume 15 - 2024 | https://doi.org/10.3389/fphar.2024.1366551
This article is part of the Research TopicHerbal Medical Products for Metabolic Diseases - New Integrated Pharmacological ApproachesView all 12 articles
Metabolic diseases remain the most worrying global health problem as their prevalence is increasing globally every year (Choi et al., 2008). Moreover, most metabolic diseases and their complications including coronary artery disease, diabetes mellitus, hypertension, and obesity are chronic and associated with a very high financial burden. Consequently, various efforts have been made to develop safe drugs with mild side effects for long-term consumption.
Epigenetics are changes in gene expression without changes in DNA sequences and plays a role in cellular processes in various diseases (Ling and Ronn, 2019; Guo et al., 2021). Epigenetic changes can be caused by at least three mechanisms including DNA methylation, post-translational histone modifications, and regulation of gene expression by non-coding RNA (ncRNA) which can be microRNA (miRNA), long non-coding RNA (lncRNA), etc (Xiao et al., 2019). Increased DNA methylation at gene promoters causes decreased gene expression, increased ncRNA activity reduces gene expression through degradation of messenger RNA (mRNA), while the effect of histone modifications on gene expression is more variable depending on the type and location of substrate attachment to the histone tail (Xiao et al., 2019). Epigenetics plays an important role in the development of many metabolic diseases (Tikoo et al., 2008; Stančáková and Laakso, 2016; Ling and Ronn, 2019; Rizzacasa et al., 2019; Samblas et al., 2019; Wu et al., 2023) and since they are reversible, restoring epigenetic status to normal provides opportunities for developing pharmacotherapies for metabolic diseases (Hamm and Costa, 2015).
Currently, botanical drug is an interesting topic of discussion for the treatment of metabolic diseases because of its low toxicity and promising therapeutic effects (Ariyanto et al., 2023a; Ariyanto et al., 2023b; Wu et al., 2023). Botanical drugs are composed of many compounds, some of which provide therapeutic effects (Ariyanto et al., 2023a; Ariyanto et al., 2023b; Wu et al., 2023) and are usually consumed in the form of decoction, pills, powder, etc. extracted from the leaves, fruit, roots, or stems of plants (Wu et al., 2023).
Several studies have determined the effect of botanical drugs on metabolic diseases but its effectiveness in modifying the epigenetic status of molecular pathways involved in the pathogenesis and pathophysiology of metabolic diseases has not yet been revealed. This article aims to comprehensively analyze the efficacy of botanical drugs in treating metabolic diseases through epigenetic changes to provide insight into research and development strategies for botanical drugs as a pharmacotherapy for metabolic diseases.
Previous studies have shown that botanical drugs can produce epigenetic changes through several mechanisms including modulating DNA methylation, posttranslational histone modifications, as well as ncRNA-mediated gene regulation by modulating the expression or activity of DNA methyltransferase (DNMT) and histone deacetylase (HDAC) (Wu et al., 2023). DNMT catalyzes DNA methylation while HDAC catalyzes the release of acetyl groups from histones to inhibit gene expression (Verdin et al., 2003; Mirza et al., 2013).
Several studies have reported the effect of botanical drugs in changing DNA methylation levels to improve metabolic disease conditions. Zhou showed that geniposide present in Hedyotis diffusa, Radix scrophulariae, Eucommia ulmoides, and Paederia scandens has antiatherosclerotic effects through regulating DNA methylation (Zhou, 2019). Ma et al showed that resveratrol has important benefits in preventing cardiovascular disease because it can inhibit homocysteine-induced PTEN hypermethylation to inhibit smooth muscle cell proliferation, one of the stages of atherogenesis (Ma et al., 2018). Another study indicated the role of curcumin in increasing the methylation of the RNA18S5 gene through activation of DNMT2 which produces atheroprotective effects (Lewinska et al., 2015). An in vivo study reported the role of naoluoxintong (NLXT) in a rat model of ischemic stroke with middle cerebral artery occlusion (MCAO) (Hong et al., 2021). NLXT regulated NogoA, NgR1, NgR2, RhoA, and Rock2 gene expression through downregulation of DNA methylation (Hong et al., 2021).
Resveratrol, a metabolite contained in melinjo seeds, improved outcomes in type 2 diabetes mellitus patients through epigenetic modification (Bo et al., 2018). Administering 40 mg and 500 mg for 6 months to type 2 diabetes mellitus patients increased Sirtuin-1 (Sirt1) expression which was associated with a decrease in H3K56 acetylation and body fat (Bo et al., 2018). Naringenin and hesperetin, Quzhou Fructus aurantia-derived metabolites, inhibited AMPK-mediated p300 induction to decrease histone acetylation, thereby decreasing Txnip expression in pancreatic β cells in diabetic mice and the INS-1 pancreatic β cell line (Wang et al., 2021).
Several in vivo studies reported the potential of esculetin, a derivative of coumarin, in improving diabetes mellitus and its complications through epigenetic modification (Kadakol et al., 2015; Kadakol et al., 2017). Esculetin administered at doses of 50 and 100 mg/kg/day for 2 weeks reduced dimethylation at lysine 4 of histone 3 (H3K4me2) and H3K36me2, as well as acetylation at lysine 27 of histone 3 (H3K27ac) in the hearts of type 2 diabetes mice (Kadakol et al., 2015). The administration of 50 mg/kg/day esculetin for 6 weeks also improved cardiomyopathy caused by type 2 diabetes mellitus by reducing levels of H3K9ac, H2AK119ub, and H2BK120ub (Kadakol et al., 2017). Therefore, esculetin has the potential to be developed for the treatment of diabetes mellitus and its complications through epigenetic modifications, especially in histones.
In vivo studies in mouse models and in vitro studies reported that icariin pretreatment (4 μM) prevented ischemia/reperfusion (I/R)-induced injury by increasing the activity of sirtuin-1, a histone deacetylase, which then reduced FOXO1 (Wu et al., 2018). This mechanism improved the quality of heart contractions, limited the size of cardiac infarction, and leakage of creatine kinase-MB from damaged myocardium, as well as reduced oxidative stress in heart cell mitochondria (Wu et al., 2018). Moreover, administration of sirtuin-1 inhibitors and Sirt1 siRNA reduced the visible cardioprotective effects (Wu et al., 2018).
Suxiao Jiuxin pill (SJP) is an botanical drug that contains tetramethylpyrazine and borneol and has often been used as a therapy for coronary artery disease in China (Ruan et al., 2018). In the context of cell-cell communication in the heart, exosomes play a pivotal role in cardiac mesenchymal stem cell and cardiomyocyte communication, some of which can occur through the modulation of epigenetic changes (Ruan et al., 2018). Ruan et al showed that SJP treatment can cause changes in C-MSC-derived exosomes to increase H3K27me3 and decrease Utx expression, as well as increase Pcna expression, a marker of cardiomyocyte proliferation in HL-1 cells, a mouse cardiomyocyte line (Ruan et al., 2018).
Anacardic acid was reported to have an inhibitory effect on histone acetylation in a cardiac hypertrophy mice model. Administration of 3.75 mg/kg anacardic acid improved cardiac hypertrophy through modulating histone acetylation (Li et al., 2019) by inhibiting the expression of p300 and p300/CBP-associated factor (PCAF), thereby reducing H3K9ac levels and normalizing the transcriptional activity of Mef2 (Li et al., 2019). Anacardic acid also inhibits the activity of histone acetylases in mouse cardiac hypertrophy, causing changes in the expression of several important genes in the heart and reducing cardiac hypertrophy (Peng et al., 2017).
In vitro studies reported the effectiveness of kaempferol and piperine in inhibiting adipocyte differentiation and increasing lipolytic gene expression, respectively, through epigenetic modification (Park et al., 2019; Park et al., 2022). Administration of 100 μM kaempferol inhibited the expression of several Pparg target adipogenic genes including Adipoq, Fabp4, and Lpl by reducing H3K27me3 deposition in the gene promoter region during 3T3-L1 adipocyte differentiation (Park et al., 2022). Administration of 50 μM piperine for 8 days to the 3T3-L1 cell line decreased H3K27me3 enrichment in Pparg, decreased H3K9ac, and increased Ezh2, increasing the expression of Ezh2-associated lipolytic genes (Park et al., 2019).
Qian Yang Yu Yin (QYYY) granules improve renal injury conditions through epigenetic regulation in HEK293T cells whose proliferation was induced by Ang II as a renal damage model (Zhang et al., 2020). QYYY inhibits the proliferation of HEK293T cells, acetyl-cortactin, and DNA methylation, as well as increasing Sirt1 and H3K4me3 (Zhang et al., 2020).
Several in vivo studies demonstrated the role of botanical drugs in improving the pathological conditions of metabolic diseases through miRNA regulation. Supplementation with plant-derived polyphenols reduces weight gain, liver steatosis, insulin resistance, and histopathological damage in diet-induced fatty liver disease in hyperlipidemic mice through regulation of the miRNA paralogs miR-103/107 and miR-122 which then modulated glucose and lipid metabolism (Joven et al., 2012). Supplementation with 0.05% lycopene for 8 weeks inhibited liver steatosis in high-fat-fed mice through miRNA-21 induction, which then caused Fabp7 degradation and decreased fatty acid-binding protein 7 (FABP7) expression (Ahn et al., 2012). Another in vivo study unraveled that the administration of 40 mg/kg Xuesaitong increased myocardial blood vessel formation in a myocardial infarction mouse model through inhibiting miR-3158-3p targeting Nur77 (Liao et al., 2023).
Yang et al showed the effect of administering 25 μmol/L dihydromyricetin in increasing endothelial nitric oxide (NO) synthesis and inhibiting atherosclerosis through inhibiting miR-21 in the apolipoprotein E-deficient mice model (Yang et al., 2020). Inhibition of miR-21 further increased the expression of the gene encoding dimethylarginine dimethylaminohydrolase-1, which in turn decreased asymmetric dimethylarginine and increased endothelial NO synthase to increase NO production (Yang et al., 2020). In vivo research on a rat myocardial infarction model showed that Wenxin granules improved myocardial infarction by regulating miR-1 and protein kinase C (PKC)-mediated signal transduction which protected gap junctions and increased the ventricular fibrillation threshold (Wu et al., 2017).
A study using human coronary artery endothelial cell-derived exosomes found that chrysin treatment reduced endothelial cell-derived miR-92a-containing exosomes which then caused an increase in Klf2 expression for an atheroprotective effect (Lin et al., 2021). Yunpi Heluo decoction improved insulin resistance in Zucker diabetic fatty rats by reducing miR-29a-3p expression causing increased Irs1 expression, a target of miR-29a-3p (Mao et al., 2019), thereby increasing the protein expression of IRS1, protein kinase B, and pyruvate dehydrogenase lipoamide kinase isozyme 1 (PDK1) (Mao et al., 2019). Another study found that quercetin, perillyl alcohol, and berberine improved pathological conditions in pulmonary arterial hypertension by regulating miR-204 and miR-27a, as well as factors that play a role in inflammation, fibrosis, and apoptosis (Rajabi et al., 2021). Moreover, Cao et al suggested the role of Astragalus polysaccharide in accelerating the differentiation of C3H10T 1/2 cells into brown adipocytes through the regulation of miR-1258-5p and Cux1 (Cao et al., 2021).
The burgeoning field of botanical drugs has garnered significant attention in recent years due to its perceived potential and effectiveness in the treatment of metabolic diseases. Several investigations and empirical data discussed earlier provide detailed insights into the potential and effectiveness of botanical drugs in managing metabolic diseases. Several studies have indicated that even at low doses, botanical drugs can yield significant effects, suggesting considerable efficacy.
Despite the promising outcomes observed in the previous studies, the precise molecular mechanisms underlying the therapeutic effects of botanical drugs remain incompletely understood. A key area of inquiry pertains to the epigenetic alterations and gene regulation induced by secondary metabolites. Delving deeper into these mechanisms necessitates elucidating, for instance, the specific binding proteins or transcription factors involved in mediating posttranslational histone modifications, modulating gene expression, and, subsequently, producing biological effects. This intricate interplay between secondary metabolites and molecular pathways warrants further exploration through more advanced molecular and biochemical analyses.
Moreover, alongside unraveling the molecular intricacies, it is crucial to conduct rigorous investigations into the safety profile of botanical drugs. While botanical drugs offer potential therapeutic benefits, ensuring their safety is paramount. Comprehensive toxicity studies and pharmacological evaluations are essential to ascertain any potential adverse effects associated with prolonged usage or interactions with other medications and compounds. Such thorough assessments are fundamental for mitigating risks and promoting the responsible use of botanical drugs in clinical settings.
Furthermore, the exploration of potential side effects and drug interactions extends beyond individual metabolites to encompass their synergistic effects and interactions with conventional pharmaceuticals. Understanding how botanical drugs interact with other molecules, including prescription drugs, is essential for preventing adverse reactions and optimizing therapeutic outcomes. Integrating pharmacokinetic and pharmacodynamic studies can provide valuable insights into the bioavailability, metabolism, and potential drug interactions of herbal formulations.
While empirical evidence highlights the therapeutic potential of botanical drugs in managing metabolic diseases, further research is imperative to elucidate the underlying molecular mechanisms and ensure their safety and efficacy. By leveraging advanced scientific methodologies and conducting comprehensive evaluations, we can unlock the optimal therapeutic potential of botanical drugs while safeguarding patient health and wellbeing.
Botanical drugs in relatively small doses produce beneficial effects in various pathological conditions involved in metabolic diseases by changing the level of DNA methylation or post-translational histone modifications, or modulating ncRNAs. However, further studies elaborating more specific molecular mechanisms, safety, adverse effects and potential interactions with other molecules are required to accelerate the development of novel drugs.
EA: Conceptualization, Funding acquisition, Investigation, Resources, Validation, Writing–original draft, Writing–review and editing.
The author(s) declare financial support was received for the research, authorship, and/or publication of this article. This study was funded by Universitas Padjadjaran grant for EA.
I would like to thank Universitas Padjadjaran for supporting this study.
The author declares that the research was conducted in the absence of any commercial or financial relationships that could be construed as a potential conflict of interest.
All claims expressed in this article are solely those of the authors and do not necessarily represent those of their affiliated organizations, or those of the publisher, the editors and the reviewers. Any product that may be evaluated in this article, or claim that may be made by its manufacturer, is not guaranteed or endorsed by the publisher.
Ahn, J., Lee, H., Jung, C. H., and Ha, T. (2012). Lycopene inhibits hepatic steatosis via microRNA-21-induced downregulation of fatty acid-binding protein 7 in mice fed a high-fat diet. Mol. Nutr. Food Res. 56 (11), 1665–1674. doi:10.1002/mnfr.201200182
Ariyanto, E. F., Danil, A. S., Rohmawaty, E., Sujatmiko, B., and Berbudi, A. (2023a). Effect of resveratrol in melinjo seed (gnetum gnemon L) extract on type 2 diabetes mellitus patients and its possible mechanism: a review. Curr. Diabetes Rev. 19 (2), e280222201512. doi:10.2174/1573399818666220228160908
Ariyanto, E. F., Wirajati, F., Rahman, P. H., Berbudi, A., and Rohmawaty, E. (2023b). Mechanism of action of Indonesian medicinal plants in inhibiting 3T3-L1 adipocyte differentiation: a review. J. Appl. Pharm. Sci. 13 (05), 050–057. doi:10.7324/JAPS.2023.6711
Bo, S., Togliatto, G., Gambino, R., Ponzo, V., Lombardo, G., Rosato, R., et al. (2018). Impact of sirtuin-1 expression on H3K56 acetylation and oxidative stress: a double-blind randomized controlled trial with resveratrol supplementation. Acta Diabetol. 55 (4), 331–340. doi:10.1007/s00592-017-1097-4
Cao, Y., Deng, B., Zhang, S., Gao, H., Song, P., Zhang, J., et al. (2021). Astragalus polysaccharide regulates brown adipogenic differentiation through miR-1258-5p-modulated cut-like homeobox 1 expression. Acta Biochim. Biophys. Sin. (Shanghai) 53 (12), 1713–1722. doi:10.1093/abbs/gmab151
Choi, B. C., McQueen, D. V., Puska, P., Douglas, K. A., Ackland, M., Campostrini, S., et al. (2008). Enhancing global capacity in the surveillance, prevention, and control of chronic diseases: seven themes to consider and build upon. J. Epidemiol. Commun. Health 62, 391–397. doi:10.1136/jech.2007.060368
Guo, W., Ma, H., Wang, C. Z., Wan, J. Y., Yao, H., and Yuan, C. S. (2021). Epigenetic studies of Chinese herbal medicine: pleiotropic role of DNA methylation. Front. Pharmacol. 12, 790321. doi:10.3389/fphar.2021.790321
Hamm, C. A., and Costa, F. F. (2015). Epigenomes as therapeutic targets. Pharmacol. Ther. 151, 72–86. doi:10.1016/j.pharmthera.2015.03.003
Hong, L., Chen, W., He, L., Tan, H., Peng, D., Zhao, G., et al. (2021). Effect of Naoluoxintong on the NogoA/RhoA/ROCK pathway by down-regulating DNA methylation in MCAO rats. J. Ethnopharmacol. 281, 114559. doi:10.1016/j.jep.2021.114559
Joven, J., Espinel, E., Rull, A., Aragonès, G., Rodríguez-Gallego, E., Camps, J., et al. (2012). Plant-derived polyphenols regulate expression of miRNA paralogs miR-103/107 and miR-122 and prevent diet-induced fatty liver disease in hyperlipidemic mice. Biochim. Biophys. Acta 1820 (7), 894–899. doi:10.1016/j.bbagen.2012.03.020
Kadakol, A., Malek, V., Goru, S. K., Pandey, A., and Gaikwad, A. B. (2015). Esculetin reverses histone H2A/H2B ubiquitination, H3 dimethylation, acetylation and phosphorylation in preventing type 2 diabetic cardiomyopathy. J. Funct. Foods 17, 127–136. doi:10.1016/j.jff.2015.05.017
Kadakol, A., Malek, V., Goru, S. K., Pandey, A., and Gaikwad, A. B. (2017). Telmisartan and esculetin combination ameliorates type 2 diabetic cardiomyopathy by reversal of H3, H2A, and H2B histone modifications. Indian J. Pharmacol. 49, 348–356. doi:10.4103/ijp.IJP_710_16
Lewinska, A., Wnuk, M., Grabowska, W., Zabek, T., Semik, E., Sikora, E., et al. (2015). Curcumin induces oxidation-dependent cell cycle arrest mediated by SIRT7 inhibition of rDNA transcription in human aortic smooth muscle cells. Toxicol. Lett. 233 (3), 227–238. doi:10.1016/j.toxlet.2015.01.019
Li, S., Peng, B., Luo, X., Sun, H., and Peng, C. (2019). Anacardic acid attenuates pressure-overload cardiac hypertrophy through inhibiting histone acetylases. J. Cell Mol. Med. 23 (4), 2744–2752. doi:10.1111/jcmm.14181
Liao, J., Shao, M., Wang, Y., Yang, P., Fu, D., Liu, M., et al. (2023). Xuesaitong promotes myocardial angiogenesis in myocardial infarction mice by inhibiting MiR-3158-3p targeting Nur77. Aging (Albany NY) 15 (10), 4084–4095. doi:10.18632/aging.204671
Lin, C. M., Wang, B. W., Pan, C. M., Fang, W. J., Chua, S. K., Cheng, W. P., et al. (2021). Chrysin boosts KLF2 expression through suppression of endothelial cell-derived exosomal microRNA-92a in the model of atheroprotection. Eur. J. Nutr. 60 (8), 4345–4355. doi:10.1007/s00394-021-02593-1
Ling, C., and Ronn, T. (2019). Epigenetics in human obesity and type 2 diabetes. Cell Metab. 29, 1028–1044. doi:10.1016/j.cmet.2019.03.009
Ma, S. C., Zhang, H. P., Jiao, Y., Wang, Y. H., Zhang, H., Yang, X. L., et al. (2018). Homocysteine-induced proliferation of vascular smooth muscle cells occurs via PTEN hypermethylation and is mitigated by resveratrol. Mol. Med. Rep. 17 (4), 5312–5319. doi:10.3892/mmr.2018.8471
Mao, Z. J., Weng, S. Y., Lin, M., and Chai, K. F. (2019). Yunpi Heluo decoction attenuates insulin resistance by regulating liver miR-29a-3p in Zucker diabetic fatty rats. J. Ethnopharmacol. 243, 111966. doi:10.1016/j.jep.2019.111966
Mirza, S., Sharma, G., Parshad, R., Gupta, S. D., Pandya, P., and Ralhan, R. (2013). Expression of DNA methyltransferases in breast cancer patients and to analyze the effect of natural compounds on DNA methyltransferases and associated proteins. J. Breast Cancer 16, 23–31. doi:10.4048/jbc.2013.16.1.23
Park, U., Hwang, J., Youn, H., Kim, E., and Um, S. (2019). Piperine inhibits adipocyte differentiation via dynamic regulation of histone modifications. Phytother. Res. 33, 2429–2439. doi:10.1002/ptr.6434
Park, U. H., Hwang, J. T., Youn, H., Kim, E. J., and Um, S. J. (2022). Kaempferol antagonizes adipogenesis by repressing histone H3K4 methylation at PPARγ target genes. Biochem. Biophys. Res. Commun. 617, 48–54. doi:10.1016/j.bbrc.2022.05.098
Peng, C., Luo, X., Li, S., and Sun, H. (2017). Phenylephrine-induced cardiac hypertrophy is attenuated by a histone acetylase inhibitor anacardic acid in mice. Mol. Biosyst. 13 (4), 714–724. doi:10.1039/c6mb00692b
Rajabi, S., Najafipour, H., Jafarinejad-Farsangi, S., Joukar, S., Beik, A., Askaripour, M., et al. (2021). Quercetin, perillyl alcohol, and berberine ameliorate right ventricular disorders in experimental pulmonary arterial hypertension: effects on miR-204, miR-27a, fibrotic, apoptotic, and inflammatory factors. J. Cardiovasc Pharmacol. 77 (6), 777–786. doi:10.1097/FJC.0000000000001015
Rizzacasa, B., Amati, F., Romeo, F., Novelli, G., and Mehta, J. L. (2019). Epigenetic modification in coronary atherosclerosis: JACC review topic of the week. J. Am. Coll. Cardiol. 74 (10), 1352–1365. doi:10.1016/j.jacc.2019.07.043
Ruan, X. F., Li, Y. J., Ju, C. W., Shen, Y., Lei, W., Chen, C., et al. (2018). Exosomes from Suxiao Jiuxin pill-treated cardiac mesenchymal stem cells decrease H3K27 demethylase UTX expression in mouse cardiomyocytes in vitro. Acta Pharmacol. Sin. 39 (4), 579–586. doi:10.1038/aps.2018.18
Samblas, M., Milagro, F. I., and Martínez, A. (2019). DNA methylation markers in obesity, metabolic syndrome, and weight loss. Epigenetics 14 (5), 421–444. doi:10.1080/15592294.2019.1595297
Stančáková, A., and Laakso, M. (2016). Genetics of type 2 diabetes. Endocr. Dev. 31, 203–220. doi:10.1159/000439418
Tikoo, K., Meena, R. L., Kabra, D. G., and Gaikwad, A. B. (2008). Change in post-translational modifications of histone H3, heat-shock protein-27 and MAP kinase p38 expression by curcumin in streptozotocin-induced type I diabetic nephropathy. Br. J. Pharmacol. 153, 1225–1231. doi:10.1038/sj.bjp.0707666
Verdin, E., Dequiedt, F., and Kasler, H. G. (2003). Class II histone deacetylases: versatile regulators. Trends Genet. 19, 286–293. doi:10.1016/S0168-9525(03)00073-8
Wang, S. W., Sheng, H., Bai, Y. F., Weng, Y. Y., Fan, X. Y., Zheng, F., et al. (2021). Inhibition of histone acetyltransferase by naringenin and hesperetin suppresses Txnip expression and protects pancreatic β cells in diabetic mice. Phytomedicine 88, 153454. doi:10.1016/j.phymed.2020.153454
Wu, A., Zhao, M., Lou, L., Zhai, J., Zhang, D., Zhu, H., et al. (2017). Effect of Wenxin granules on gap junction and MiR-1 in rats with myocardial infarction. Biomed. Res. Int. 2017, 3495021. doi:10.1155/2017/3495021
Wu, B., Feng, J. Y., Yu, L. M., Wang, Y. C., Chen, Y. Q., Wei, Y., et al. (2018). Icariin protects cardiomyocytes against ischaemia/reperfusion injury by attenuating sirtuin 1-dependent mitochondrial oxidative damage. Br. J. Pharmacol. 175 (21), 4137–4153. doi:10.1111/bph.14457
Wu, Y. Y., Xu, Y. M., and Lau, A. T. Y. (2023). Epigenetic effects of herbal medicine. Clin. Epigenetics 15 (1), 85. doi:10.1186/s13148-023-01481-1
Xiao, Y., Su, M., Ou, W., Wang, H., Tian, B., Ma, J., et al. (2019). Involvement of noncoding RNAs in epigenetic modifications of esophageal cancer. Biomed. Pharmacother. 117, 109192. doi:10.1016/j.biopha.2019.109192
Yang, D., Yang, Z., Chen, L., Kuang, D., Zou, Y., Li, J., et al. (2020). Dihydromyricetin increases endothelial nitric oxide production and inhibits atherosclerosis through microRNA-21 in apolipoprotein E-deficient mice. J. Cell Mol. Med. 24 (10), 5911–5925. doi:10.1111/jcmm.15278
Zhang, S. F., Mao, X. J., Jiang, W. M., and Fang, Z. Y. (2020). Qian Yang Yu Yin Granule protects against hypertension-induced renal injury by epigenetic mechanism linked to Nicotinamide N-Methyltransferase (NNMT) expression. J. Ethnopharmacol. 255, 112738. doi:10.1016/j.jep.2020.112738
Keywords: botanical drugs, epigenetics, DNA methylation, histone modifications, non-coding RNA, metabolic disorder
Citation: Ariyanto EF (2024) The efficacy of botanical drugs in orchestrating epigenetic modifications for ameliorating metabolic disorders. Front. Pharmacol. 15:1366551. doi: 10.3389/fphar.2024.1366551
Received: 06 January 2024; Accepted: 22 March 2024;
Published: 05 April 2024.
Edited by:
Abd El-Latif Hesham, Beni-Suef University, EgyptReviewed by:
Yasufumi Katanasaka, University of Shizuoka, JapanCopyright © 2024 Ariyanto. This is an open-access article distributed under the terms of the Creative Commons Attribution License (CC BY). The use, distribution or reproduction in other forums is permitted, provided the original author(s) and the copyright owner(s) are credited and that the original publication in this journal is cited, in accordance with accepted academic practice. No use, distribution or reproduction is permitted which does not comply with these terms.
*Correspondence: Eko Fuji Ariyanto, ZnVqaUB1bnBhZC5hYy5pZA==
Disclaimer: All claims expressed in this article are solely those of the authors and do not necessarily represent those of their affiliated organizations, or those of the publisher, the editors and the reviewers. Any product that may be evaluated in this article or claim that may be made by its manufacturer is not guaranteed or endorsed by the publisher.
Research integrity at Frontiers
Learn more about the work of our research integrity team to safeguard the quality of each article we publish.