- 1School of Clinical Medical, North China University of Science and Technology, Tangshan, Hebei, China
- 2Critical Care Department, Hebei General Hospital, Shijiazhuang, Hebei, China
- 3School of Graduate, Hebei Medical University, Shijiazhuang, Hebei, China
- 4Department of Orthopaedics, The People’s Hospital of Shizhu, Chongqing, China
Objective: We conducted a systematic review to assess the advantages and disadvantages of levosimendan in patients with sepsis compared with placebo, milrinone, and dobutamine and to explore the clinical efficacy of different concentrations of levosimendan.
Methods: PubMed, Web of Science, Cochrane Library, Embase, CNKI, Wanfang data, VIP, and CBM databases were searched using such keywords as simendan, levosimendan, and sepsis. The search time was from the establishment of the database to July 2023. Two researchers were responsible for literature screening and data collection respectively. After the risk of bias in the included studies was evaluated, network meta-analysis was performed using R software gemtc and rjags package.
Results: Thirty-two randomized controlled trials (RCTs) were included in the network meta-analysis. Meta-analysis results showed that while levosimendan significantly improved CI levels at either 0.1 µg/kg/min (mean difference [MD] [95%CrI] = 0.41 [−0.43, 1.4]) or 0.2 µg/kg/min (MD [95%CrI] =0.54 [0.12, 0.99]). Levosimendan, at either 0.075 µg/kg/min (MD [95% CrI] =0.033 [−0.75, 0.82]) or 0.2 µg/kg/min (MD [95% CrI] = −0.014 [−0.26, 0.23]), had no significant advantage in improving Lac levels. Levosimendan, at either 0.1 µg/kg/min (RR [95% CrI] = 0.99 [0.73, 1.3]) or 0.2 µg/kg/min (RR [95% CrI] = 1.0 [0.88, 1.2]), did not have a significant advantage in reducing mortality.
Conclusion: The existing evidence suggests that levosimendan can significantly improve CI and lactate levels in patients with sepsis, and levosimendan at 0.1 µg/kg/min might be the optimal dose. Unfortunately, all interventions in this study failed to reduce the 28-day mortality.
Systematic Review Registration: https://www.crd.york.ac.uk/prospero/display_record.php?ID=CRD42023441220.
1 Introduction
Sepsis is one of the leading causes of admission to the intensive care unit (ICU), accounting for approximately 20%–30% of ICU admissions (Vincent et al., 2014). About 15% of sepsis patients progress to septic shock (Fleischmann et al., 2016; Liu, 2017). Up to 18%–40% of septic shock patients were complicated with heart failure and myocardial depression (Liu, 2017; Ravikumar et al., 2021; Koya and Paul, 2023). If they are not treated in time, the mortality rate can reach 70%–90% (Dugar et al., 2020). The occurrence of these complications may be related to the imbalance of myocardial energy metabolism, the production of a large number of negative regulatory factors in myocardium, and apoptosis of myocardial cells (Zuo et al., 2023). Therefore, in the treatment, attention should be paid not only to the control of sepsis infection, but also to the protection of cardiac function (Vincent and Bakker, 2021; Chen and Fu, 2023).
Levosimendan is a Ca2+ sensitizer that enhances myocardial contractility by increasing the sensitivity of cardiac troponin to Ca2+, but does not increase myocardial oxygen consumption and cause calcium overload, thus avoiding arrhythmias caused by traditional positive inotropic drugs (Sun et al., 2023). Because of its good cardiotonic effect, it has been widely used in the treatment of acute heart failure and cardiogenic shock (Fan et al., 2019). The results of current studies are inconsistent as to whether levosimendan is effective in the treatment and overall prognosis of sepsis patients. Studies by Morelli et al. showed that levosimendan can increase cardiac output, reduce lactate levels, improve intestinal microcirculation, and modulate inflammatory responses (Matejovic et al., 2005; Morelli et al., 2005; Morelli et al., 2006; Tasouli et al., 2007; Pan et al., 2019; Yang et al., 2019). However, other studies indicated that levosimendan does not improve organ dysfunction or reduce mortality in patients with sepsis (Antcliffe et al., 2019). Hence, further investigation is needed to determine the therapeutic value of levosimendan in sepsis (Saevik et al., 2021).
Given the large heterogeneity of control groups in existing studies on levosimendan and the different regimens of levosimendan used in different studies, we want to explore the advantages and disadvantages of levosimendan compared with placebo, milrinone, and dobutamine in patients with sepsis and the clinical efficacy of different concentrations of levosimendan. A network meta-analysis can analyze more than two interventions simultaneously, based on indirect comparisons or a combination of indirect and direct comparisons. It allows for quantitative comparisons of different interventions for the same disease and ranks them according to the effect on a certain outcome measure. This study used systematic review and network meta-analysis to synthesize the available evidence. The objective of this study is to directly and indirectly compare the efficacy of dobutamine, milrinone, and levosimendan in patients with sepsis and explore the efficacy of levosimendan at different doses.
2 Methods
2.1 Protocol and registration
This study followed the PRISMA guideline (Hutton et al., 2015) and was pre-registered on the PROSPERO platform (registration number #CRD42023441220) (Tan et al., 2023).
2.2 Search strategy
The main terms used to construct the search strategy were simendan, levosimendan, and sepsis. PubMed, Web of Science, Cochrane Library, Embase, CNKI, Wanfang Data, VIP, and CBM were searched from the date of database inception to July 2023. In addition, the references of the included studies were traced to supplement relevant studies.
2.3 Literature screening
Literature screening, data extraction, and cross-checking were conducted by two independent researchers. In case of any disagreements, a third researcher was consulted for resolution. Reasons for excluding literature were clearly recorded, and an exclusion criteria list was created. Efforts were made to contact the original authors via email or phone for any missing data. During the screening and evaluation of literature, the titles and abstracts were initially reviewed to remove duplicates and articles that did not meet the requirements. Subsequently, the full texts were further examined to determine the included studies.
2.4 Eligibility criteria
2.4.1 Inclusion criteria
1) Type of study: randomized controlled trials (RCT); 2) Participants: patients met the definition criteria of sepsis or septic shock in international guidelines at that time, age>18 years old; gender, nationality, race, source of infection, pathogen and course of disease were not limited; 3) Intervention measures and comparison: The control group was treated with dobutamine, milrinone or placebo, while the experimental group was treated with levosimendan intravenous injection or intravenous pump, and other conventional treatments were the same as those in the control group; 4) Outcome measures: Cardiac index (CI) was used as the primary outcome measure, while lactic acid (Lac) level and 28-day mortality were used as secondary outcome measures.
2.4.2 Exclusion criteria
1) Studies that included underage patients; 2) duplicate publications; 3) studies without outcome measure data; 4) studies with incomplete data or unavailable data; 5) non-Chinese/English studies; and 6) animal experiments.
2.5 Bias risk assessment for included studies
The risk of bias was assessed using the RCT bias risk assessment tool built into Cochrane Review Manager (5.4.1). Two investigators independently evaluated the bias risk of the included studies, and cross-checked the evaluation results. Disagreements were discussed or adjudicated by a third investigator. The evaluation domains included: (1) whether the random allocation method was correct; (2) whether the allocation hiding method was used; (3) whether the blinding method was used; (4) whether the outcome data were complete; (5) whether there was selective reporting of study results; (6) other sources of bias. Each evaluation domain was judged as high, uncertain, or low risk of bias.
2.6 Data extraction
The following data were extracted: (1) general information, including publication country, publication year, publication journal, first author and title; (2) basic characteristics of study subjects, including sample size, gender composition, age distribution and disease severity; (3) study characteristics, including study objective, study type, administration method, dose, duration and results of levosimendan; (4) key elements of bias risk evaluation; (5) outcome indicators, including 28-day mortality, CI, and Lac.
2.7 Statistical analysis
The statistical analysis in this study was performed using the gemtc package (version 1.0-1) in R software (version 4.0.4). Mean difference (MD) was used for continuous data, odds ratio (OR) was used for binary data, and 95% confidence intervals (95%CrI) were obtained for each effect size (ES). The I2 test was used to assess the heterogeneity among studies. A 95% CrI that does not include 0 (for continuous data) or 1 (for binary data) indicates a statistically significant difference. First, the “mtc.network” function was used to construct a network, followed by generating models using the “mtc.model”. Finally, Bayesian analysis was performed using the “mtc.run” function. In the “mtc.model” function, the likelihood/link was set to “binom/log” to compute the log risk ratio (logRR) with a 95% CrI based on collected binary data. The JAGS software (through the “rjags” package) was used for model estimation. Markov Chain Monte Carlo (MCMC) method was used to compute a fixed-effect model based on 5,000 adaptive simulations and 20,000 iterations. A ranking table was generated to display the relationships between all treatment concentrations, and the “exp” function was used to calculate the relative risk (RR) from the log risk ratio (LogRR). Additionally, a forest plot of relative effects was provided to visualize the relative effects of different treatment processes compared to the standard treatment process. The surface under the cumulative ranking curve (SUCRA) was calculated to compare the differences in treatment efficacy among different interventions. The convergence of iterations was quantified by calculating potential scale reduction factor (PSRF) values. The consistency assumption was tested using the node-splitting method, with inconsistency considered significant at p < 0.05. Furthermore, the “mtc.anohe” function was employed to test the homogeneity hypothesis. I2 > 50% indicated significant heterogeneity. A sensitivity analysis was conducted by comparing the pooled results of the random-effects model with the fixed-effect model.
3 Results
3.1 Search results
A total of 1,237 studies were retrieved, including 140 from PubMed, 119 from Web of Science, 65 from Cochrane Library, 536 from Embase, 93 from CNKI, 107 from Wanfang data, 88 from VIP, and 89 from CBM. The search strategies and results are listed in Supplementary Material S2. After filtering for duplicates and excluding studies that did not match PICOS based on titles and abstracts, a total of 38 full-text articles were retrieved. After carefully reading the entire texts, 32 studies (Morelli et al., 2005; Morelli et al., 2006; Morelli et al., 2010; Fang and Dong, 2014; Torraco et al., 2014; Huang et al., 2015; Gordon et al., 2016; Lai et al., 2016; Meng et al., 2016; Wu and Chang, 2016; Yan et al., 2016; Hajjej et al., 2017; Huang et al., 2017; Janssens, 2017; Wang D. et al., 2018; Wang W. et al., 2018; Lan et al., 2018; Su et al., 2018; Xu et al., 2018; Fan et al., 2019; Pan, 2019; Yang and Li, 2019; Bian et al., 2020; Liu et al., 2020; Lu et al., 2020; Shi and Lin, 2020; Liu et al., 2021; Yang and Yi, 2021; Hua et al., 2022; Chen and Fu, 2023; Sun et al., 2023; Zhou, 2023) (involving 2,813 patients) were included in the Bayesian analysis. The PRISMA flowchart is shown in Figure 1. Among them, there were 20 Chinese studies and 12 English studies.
3.2 Study characteristics
A total of 32 studies (involving 2,813 sepsis patients) were included, with 23 studies comparing two or more different inotropic drugs and 9 studies comparing single levosimendan or its combination with other drugs versus placebo. Four trials were multicenter and mainly conducted in developed regions such as Europe and North America. There were no significant differences in gender distribution and age distribution among the included studies, with most patients being middle-aged or elderly. In the assessment of initial disease severity, SAPS II scores were provided in four studies, APACHE II scores in 20 studies, and SOFA scores in 15 studies, indicating a generally consistent severity of the patients’ diseases. In the evaluation of initial cardiac function, 18 studies provided BNP levels and 19 studies provided CI levels, with no significant differences observed in the initial cardiac function of the patients (Table 1). Through the comparative analysis of study design, outcome measures, patient characteristics, and inclusion/exclusion criteria, we found that conducting a network meta-analysis was appropriate for the comprehensive evaluation of the evidence. Homogeneity and consistency assumptions were statistically confirmed (Supplementary Material S1).
3.3 Quality evaluation
All 32 studies mentioned the use of random allocation methods, with 18 studies using random number tables, 13 studies using simple randomization, and one study using sequential allocation. Two studies employed double-blinding, and two studies employed single-blinding, while the remaining 28 studies did not mention the use of blinding. The outcome data of all included studies were relatively complete, with no bias due to incomplete outcome reporting, and the reported methods and results were consistent. Other sources of bias were unclear. The risk of bias assessment results for the included studies are illustrated in Figure 2.
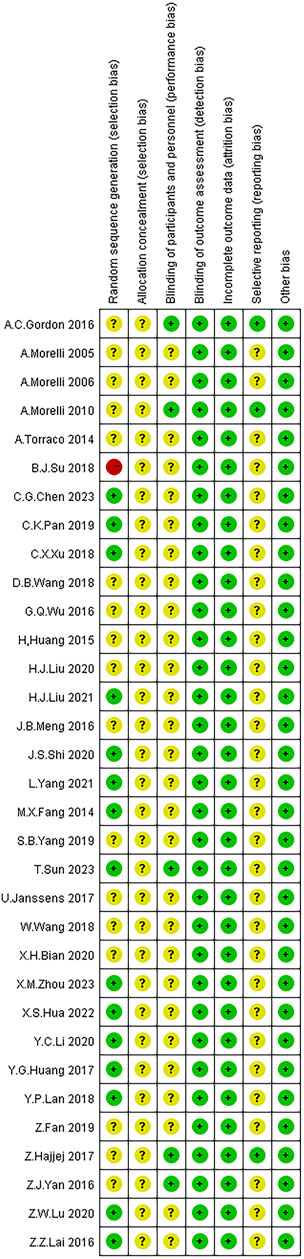
FIGURE 2. Risk of bias assessment of the included studies. Note: Red color: high risk; Yellow color: unclear risk; Green color: low risk.
3.4 Efficacy for outcome measures
Heterogeneity analysis using the “mtc.anohe” function showed that heterogeneity did not significantly affect the results overall. The consistency hypothesis using the node-splitting method showed that all p-values were ≥0.05, indicating no significant inconsistency. Convergence of the iterations was quantified by calculating the PSRF, and a PSRF value of 1.00 indicated satisfactory convergence. In conclusion, conducting network meta-analysis for these outcome measures was reasonable.
3.4.1 Cardiac index
Regarding the meta-analysis of CI level, a total of 17 studies with 1,414 patients were included. Four different interventions were involved, with levosimendan having two different doses of 0.1 µg/kg/min and 0.2 µg/kg/min. Three studies only used a placebo intervention, 14 studies used dobutamine intervention with no specific dose, four studies used levosimendan intervention at a dose of 0.1 µg/kg/min, and 13 studies used levosimendan intervention at a dose of 0.2 µg/kg/min (Supplementary Figure SI-1 in Supplementary Material S1).
In comparison to the placebo group, dobutamine (MD [95% CrI] = 0.014 [−0.43, 0.47]) did not show a significant advantage in improving CI. However, levosimendan, at 0.1 µg/kg/min (MD [95% CrI] = 0.41 [-0.43, 1.4]) and 0.2 µg/kg/min (MD [95% CrI] = 0.54 [0.12, 0.99]), significantly improved the CI levels of patients. (Supplementary Figure SI-3.1 in Supplementary Material S1). Additionally, it was observed that levosimendan at 0.1 µg/kg/min was superior to 0.2 µg/kg/min (MD [95% CrI] = 0.34 [−0.06, 0.72) (Supplementary Table SI-3.2 in Supplementary Material S1). The probability ranking based on SUCRA values showed a consistent trend with the forest plot and league table: dobutamine (17.61%), levosimendan at 0.1 µg/kg/min (98.57%), levosimendan at 0.2 µg/kg/min (67.80%), and placebo (16.02%). According to the SUCRA values, levosimendan 0.1 µg/kg/min was superior to levosimendan 0.2 µg/kg/min, with a significant difference. Therefore, levosimendan at 0.1 µg/kg/min may be preferred in clinical practice. The reason for this difference may be related to the small number of included studies and the limited evidence base.
3.4.2 Lactic acid
Twenty-one studies reported Lac levels, including 1,605 patients. There were six interventions, including three different doses of levosimendan: 0.075 µg/kg/min, 0.1 µg/kg/min, and 0.2 µg/kg/min. Three studies used placebo as the intervention, 16 studies used dobutamine with no specific dose, two studies used milrinone with no specific dose, two studies used levosimendan at a dose of 0.075 µg/kg/min, five studies used levosimendan at a dose of 0.1 µg/kg/min, and 14 studies used levosimendan at a dose of 0.2 µg/kg/min (Supplementary Figure SII-1 in Supplementary Material S1).
Compared to the placebo group, dobutamine (MD [95% CrI] = 1.3 [0.94, 1.6]) significantly increased Lac levels. Additionally, milrinone (MD [95% CrI] = 0.43 [−0.84, 1.7]) showed a trend of increasing Lac levels, but this trend was not statistically significant due to the wide width of the 95% CrI, which included the null value. Levosimendan, at both 0.075 µg/kg/min (MD [95% CrI] = 0.033 [−0.75, 0.82]) and 0.2 µg/kg/min (MD [95% CrI] = −0.014 [−0.26, 0.23]), did not show a significant advantage in improving Lac. However, levosimendan at 0.1 µg/kg/min (MD [95% CrI] = −0.47 [−0.90, −0.043]) significantly reduced Lac levels in patients (Supplementary Figure SII-3.1 in Supplementary Material S1). In addition, according to the league table, levosimendan at 0.1 µg/kg/min showed a significant superiority over dobutamine (MD [95% CrI] = 1.72 [1.4, 2.04]) (Supplementary Table SII-3.2 in Supplementary Material S1). The probability rankings based on SUCRA values reflected a consistent trend with the forest plot and league table: dobutamine (97.98%), milrinone (65.04%), levosimendan at 0.075 µg/kg/min (44.92%), levosimendan at 0.1 µg/kg/min (4.34%), levosimendan at 0.2 µg/kg/min (42.73%), and placebo (45.00%).
3.4.3 28-day mortality
Twenty-two studies reported 28-day mortality rates, including 2,130 patients. There are 6 intervention measures involved, among which levosimendan has three different doses: 0.075 µg/kg/min, 0.1 µg/kg/min, and 0.2 µg/kg/min. Five studies used a placebo as the intervention, fifteen studies used dobutamine, two studies used milrinone, two studies used levosimendan at a dose of 0.075 µg/kg/min, six studies used levosimendan at a dose of 0.1 µg/kg/min, and fourteen studies used levosimendan at a dose of 0.2 µg/kg/min (Supplementary Figure SIII-1 in Supplementary Material S1).
Compared to the placebo group, dobutamine (RR [95% CrI] = 1.3 [1.0, 1.7]) was associated with an increased 28-day mortality rate, while milrinone (RR [95% CrI] = 1.5 [0.65, 3.6]) showed a trend towards increasing the mortality, although the wide 95% CrI included the null value, making this trend non-significant. Levosimendan, at 0.1 µg/kg/min (RR [95% CrI] = 0.99 [0.73, 1.3]) and 0.2 µg/kg/min (RR [95% CrI] = 1.0 [0.88, 1.2]), did not show significant advantages in improving mortality rates. Levosimendan at 0.075 µg/kg/min (RR [95% CrI] = 0.72 [0.30, 1.6]) showed a trend towards reducing the 28-day mortality rate. However, this trend was not statistically significant due to the wide 95% CrI, which included the null value (Supplementary Figure SIII-3.1 in Supplementary Material S1). Additionally, the league table showed that levosimendan at 0.075 µg/kg/min was superior to milrinone (RR [95% CrI] = 2.07 [0.77, 6.18)] (Supplementary Table SIII-3.2 in Supplementary Material S1). The SUCRA rankings align with the trends observed in the forest plot and league table: dobutamine (15.43%), milrinone (19.31%), levosimendan at a dose of 0.075 µg/kg/min (84.05%), levosimendan at a dose of 0.1 µg/kg/min (63.69%), levosimendan at a dose of 0.2 µg/kg/min (53.94%), and placebo (63.57%). Based on the SUCRA values, there is a substantial difference between levosimendan at 0.075 µg/kg/min and dobutamine, as well as milrinone. Therefore, the clinical use of levosimendan at 0.075 µg/kg/min may lead to greater benefits for patients. However, the observed differences did not reach statistical significance as levosimendan at 0.075 µg/kg/min was only reported in two studies, and there was heterogeneity within these studies.
4 Discussion
The results of this network meta-analysis showed that levosimendan significantly improved CI levels and Lac levels in patients with sepsis. All interventions in this study failed to reduce 28-day mortality. Levosimendan 0.1 µg/kg/min may be the optimal dose to improve CI levels and Lac levels in patients with sepsis.
Dobutamine, milrinone, and levosimendan all have positive effects on heart muscle contraction, but they work through different mechanisms. Dobutamine selectively activates the heart β1 receptor, increasing the concentration of calcium ions inside the heart muscle cells, thereby enhancing myocardial contractility and significantly reducing the levels of plasma cTnI and heart-type fatty acid binding protein (H-FABP) (Stratton et al., 2017). Milrinone can improve cardiac function (Wang et al., 2021; Zhuang et al., 2023) by inhibiting the intracellular nucleotide phosphodiesterase pathway, increasing the concentration of intracellular cyclic adenosine monophosphate (cAMP) (Dünser et al., 2009), enhancing the release of intracellular calcium, relaxing vascular smooth muscle (Werner et al., 1995; Zhao et al., 2021) and reducing the expression of inflammatory factors (Kern et al., 2001). However, both dobutamine and milrinone have limitations as they increase myocardial oxygen consumption, posing a risk of adverse events (Schmittinger et al., 2012; Chang et al., 2020), and are thus not recommended for long-term clinical use (Fang et al., 2021). Levosimendan, on the other hand, improves myocardial contractility by stabilizing the “cTnC-Ca2+” complex without increasing intracellular calcium concentration and cell oxygen consumption (Ponikowski et al., 2016). Levosimendan also directly activates eNOS on endothelial cells, leading to increased production of nitric oxide (NO) (Pataricza et al., 2003), as well as indirectly activate KATP channels and Ca2+and voltage-sensitive K+ channels on vascular smooth muscle cells through modulation of certain signaling molecules (Grossini et al., 2009). This leads to the dilation of coronary and systemic blood vessels, thus improving myocardial ischemia (Pollesello et al., 2016). Additionally, levosimendan has the ability to suppress inflammatory reactions and myocardial apoptosis, as well as to combat oxidative damage. It reduces the release of oxidative markers (such as TBARS), increases plasma GSH levels (Grossini et al., 2020), and enhances cell autophagy (Schellekens et al., 2015), which plays a role in early-stage cell repair (Schauer et al., 2021) and long-term heart protection (Paraskevaidis et al., 2005). Furthermore, it has advantages in improving cellular metabolism in septic patients and provides certain protective effects on lung, kidney (Lannemyr et al., 2018), and diaphragm function (Doorduin et al., 2012).
We found that levosimendan significantly increased CI in patients with sepsis, and different doses also affected the extent of the increase in CI. Currently, the optimal dose of levosimendan is still controversial. Our results suggest that levosimendan 0.1 µg/kg/min may benefit patients more. Levosimendan has a short half-life of only 1–1.5 h, with a plasma protein binding rate of 95%, and is metabolized by the liver and intestine into OR-1855 and OR-1896. OR-1855 is inactive, while OR-1896 has a structure and function similar to levosimendan, with a half-life of nearly 77 h (Banfor et al., 2008). The typical loading dose for levosimendan is 6–24 μg/kg, with a maintenance dose starting at 0.1 μg/kg/min, which can be adjusted based on the situation within the range of 0.05–0.2 μg/kg/min (Leppikangas et al., 2011). To avoid sudden drop in blood pressure, worsening of coronary ischemia, and arrhythmias caused by the loading dose, it may be advisable to avoid using the loading dose or to use other positive inotropic and vasopressor drugs concurrently (Mehta et al., 2017; Herpain et al., 2019). Hence, we believe that recommending a dosage of 0.1 μg/kg/min for levosimendan is reasonable. The improvement in CI by levosimendan is mainly mediated through its calcium sensitization effect while selectively inhibiting phosphodiesterase III (PDE III) isoform and activating potassium channels. During septic shock, there is a significant downregulation of L-type calcium channels in myocardial cell membranes, leading to decreased sensitivity of myofilaments to calcium ions (Lew et al., 1996; Tavernier et al., 2001; Hobai et al., 2015). On the one hand, levosimendan and its metabolite OR-1896 directly bind to troponin C in cardiomyocytes, improving the stability of myocardial fibrin spatial configuration and calcium myofilament reactivity, thus increasing myocardial contractility (Sorsa et al., 2004; Zhang et al., 2015). Levosimendan, on the other hand, selectively inhibits PDEⅢ isoform activity, increases cAMP levels, promotes voltage-gated calcium channel phosphorylation, and allows calcium ions to enter the cell, showing additional positive inotropic effects (Szilágyi et al., 2004). Finally, levosimendan can also increase the outward potassium (K+) current by activating the adenosine triphosphate-dependent K+ channels, leading to membrane hyperpolarization. This causes the closure of voltage-dependent calcium (Ca2+) channels on the cellular membrane, effectively inhibiting the influx of Ca2+. Additionally, it activates the sodium-calcium exchange channels, promoting the efflux of Ca2+ and consequently reducing the intracellular Ca2+ concentration (Yokoshiki et al., 1997). At the same time, it opens the potassium channel of vascular smooth muscle, thus dilating blood vessels, reducing cardiac preload, increasing myocardial contractility and coronary blood supply, and improving heart pump function (Zangrillo et al., 2015). While levosimendan increases the CI, it does not increase myocardial oxygen consumption (Zhang et al., 2015). This may be attributed to its positive inotropic effect, which is not dependent on membrane depolarization-induced intracellular calcium influx, thereby avoiding intracellular calcium overload (Kaheinen et al., 2004). Maack et al. (Maack et al., 2019) proposed that the positive inotropic effect of levosimendan was a result of the synergistic action of PDEⅢ inhibition and calcium sensitization, with the latter being more important. This also explains why levosimendan, when exerting its positive inotropic effect through PDEⅢ inhibition, does not lead to intracellular calcium overload. However, there is currently limited research to demonstrate whether different dosing regimens of levosimendan have varying effects on CI levels and blood lactate levels. Therefore, multicenter, prospective RCTs are required to determine the optimal dosing regimen, efficacy, and safety of levosimendan in sepsis.
This study demonstrated that levosimendan could reduce Lac levels in patients with sepsis by improving microcirculation. The mechanisms of the improved microcirculation may be mainly related to vasodilation, anti-inflammatory, and anti-oxidative effects (Parissis et al., 2004; Wang et al., 2015; Hajjej et al., 2017; Heringlake et al., 2021). Levosimendan induces vasodilation by promoting the opening of ATP-sensitive potassium channels (K+ATP channels) in vascular smooth muscle cells, thereby dilating afferent arterioles in the kidney, increasing renal blood flow, and improving lactate clearance in septic patients with liver and kidney dysfunction (Heringlake et al., 2021; Vincent and Bakker, 2021). Some studies have found that levosimendan can downregulate NF-κB-dependent transcription, inhibit inducible nitric oxide (NO) synthase promoter activity, and reduce NO expression (Sareila et al., 2008), thereby reducing the levels of inflammatory factors and oxidative products such as brain natriuretic peptide (BNP), interleukin-6 (IL-6), tumor necrosis factor-alpha (TNF-α), and C-reactive protein (CRP) in patients with sepsis (Farmakis et al., 2016). This suggests that levosimendan exerts anti-inflammatory and antioxidant effects (Parissis et al., 2004; Wang et al., 2015; Hajjej et al., 2017). These findings indirectly demonstrate that levosimendan can improve tissue microcirculatory ischemia and hypoxia, alleviate mitochondrial dysfunction, reduce lactate levels, improve organ microcirculatory perfusion (Morelli et al., 2006), and further validate the effectiveness of levosimendan in improving hemodynamics in septic patients (Memiş et al., 2012).
This study found that, like other drugs analyzed, levosimendan did not reduce patient mortality, and there is currently no consensus on this phenomenon. A meta-analysis conducted by Zangrillo et al. (2015) on the effect of levosimendan on mortality in septic shock patients showed a reduction in mortality. However, studies by Bhattacharjee et al. (2017) and Chang et al. (2018) both indicated that levosimendan did not reduce mortality in sepsis and septic shock patients. In this study, none of the interventions, including levosimendan, were able to reduce the 28-day mortality rate, and the use of dopamine and norepinephrine increased the risk of 28-day mortality. The inability of levosimendan to reduce mortality rates may be related to various complex factors influencing mortality. Although we found that levosimendan can increase cardiac index and reduce blood lactate levels, these benefits did not translate into clinical endpoints. Some studies suggest that levosimendan can increase heart rate, thereby increasing the possibility of tachyarrhythmias, which is unfavorable for septic patients (Barraud et al., 2007). The main reasons for levosimendan-induced tachycardia are twofold. On one hand, it is due to the vasodilatory effects of levosimendan. On the other hand, Gordon et al. found that patients on levosimendan had a higher requirement for norepinephrine, which may contribute to tachycardia (Barraud et al., 2007; Torraco et al., 2014). Therefore, the use of rate-control medications in sepsis patients with co-existing tachycardia may be beneficial (Fang and Dong, 2014). Although guidelines suggest the use of dobutamine in sepsis patients with inadequate perfusion, there is a lack of definitive evidence supporting this suggestion (Sato and Nasu, 2015). As a result, it remains unclear whether positive inotropes offer any additional benefits to sepsis compared to norepinephrine.
The inability of levosimendan to reduce mortality rates may be attributable to its adverse reactions. Common adverse effects of levosimendan include hypotension, arrhythmias, and decreased red blood cell volume (Wang and Wang, 2019). Levosimendan increases positive inotropy by increasing calcium sensitivity without increasing myocardial oxygen consumption, differing in pharmacological mechanism from catecholamine-based positive inotropic drugs that act through beta-adrenergic receptors. Therefore, theoretically, the incidence of arrhythmias with levosimendan in clinical use should be relatively reduced compared to other positive inotropic drugs. However, in practical application, levosimendan’s adverse reactions related to arrhythmias do not show a significant decrease (Maack et al., 2019). Basic research suggested that levosimendan induced atrial fibrillation by shortening action potential duration and refractoriness, leading to atrial tissue activation and enhanced electrical circuit activity (Frommeyer et al., 2017). Follath et al. (2002) indicated that the decrease in red blood cell volume was attributable to the vasodilatory effect of levosimendan, leading to blood dilution. Sepsis patients often have unstable hemodynamics and are at risk of hypotension; the vasodilatory effect of levosimendan further increases this risk. Therefore, levosimendan is recommended for patients who have undergone adequate fluid resuscitation and have achieved the target mean arterial pressure (Society of Critical Care Medicine and Chinese Medical Association, 2015).
This study has several limitations. Firstly, although we have included all relevant studies to date, the sample size is still relatively small. Apart from the studies by Gordon et al. (2016) and Janssens (2017), which had over 500 cases, all other studies included fewer than 150 cases. Secondly, this study treated severe sepsis and septic shock patients as a whole, which may lead to heterogeneity. Owing to the limited number of studies available, we were unable to conduct subgroup analysis on different patient groups. Thirdly, some studies reported varying loading doses of levosimendan, and the duration of drug use varied among studies. However, due to the limited number of studies, subgroup analyses could not be carried out. Lastly, none of the included studies provided information stratified by sex; therefore, we did not explore the influence of sex-related factors. Future research should explore the impact of factors such as sex and age on the efficacy of levosimendan in clinical use.
5 Conclusion
Based on existing evidence, it can be concluded that levosimendan does not reduce mortality but can improve the CI and reduce blood Lac levels in sepsis patients. Levosimendan at 0.1 µg/kg/min is recommended. Hence, levosimendan has certain clinical value in the management of sepsis. Nevertheless, given the overall low quality and insufficient sample size of the included studies, these findings are insufficient to guide clinical practice, and physicians should exercise caution when interpreting these findings. Furthermore, more high-quality research is needed to further explore the efficacy of levosimendan in the treatment of sepsis and related conditions.
Data availability statement
The original contributions presented in the study are included in the article/Supplementary Material, further inquiries can be directed to the corresponding author.
Author contributions
RT: Conceptualization, Methodology, Resources, Writing–original draft, Writing–review and editing. HG: Writing–original draft. ZY: Formal Analysis, Investigation, Writing–review and editing. HY: Conceptualization, Writing–review and editing. QL: Methodology, Writing–review and editing. QZ: Supervision, Writing–review and editing. QD: Funding acquisition, Resources, Writing–original draft.
Funding
The author(s) declare financial support was received for the research, authorship, and/or publication of this article. This study was supported by Hebei Natural Science Foundation (H2020307040), the Government Funded Clinical Medical Excellence Training Program (2024008), and the Government Funded Clinical Medical Excellence Training Program (2020003).
Conflict of interest
The authors declare that the research was conducted in the absence of any commercial or financial relationships that could be construed as a potential conflict of interest.
Publisher’s note
All claims expressed in this article are solely those of the authors and do not necessarily represent those of their affiliated organizations, or those of the publisher, the editors and the reviewers. Any product that may be evaluated in this article, or claim that may be made by its manufacturer, is not guaranteed or endorsed by the publisher.
Supplementary material
The Supplementary Material for this article can be found online at: https://www.frontiersin.org/articles/10.3389/fphar.2024.1358735/full#supplementary-material
References
Antcliffe, D. B., Santhakumaran, S., Orme, R. M. L., Ward, J. K., Al-Beidh, F., O'dea, K., et al. (2019). Levosimendan in septic shock in patients with biochemical evidence of cardiac dysfunction: a subgroup analysis of the LeoPARDS randomised trial. Intensive Care Med. 45, 1392–1400. doi:10.1007/s00134-019-05731-w
Banfor, P. N., Preusser, L. C., Campbell, T. J., Marsh, K. C., Polakowski, J. S., Reinhart, G. A., et al. (2008). Comparative effects of levosimendan, OR-1896, OR-1855, dobutamine, and milrinone on vascular resistance, indexes of cardiac function, and O2 consumption in dogs. Am. J. Physiol. Heart Circ. Physiol. 294, H238–H248. doi:10.1152/ajpheart.01181.2007
Barraud, D., Faivre, V., Damy, T., Welschbillig, S., Gayat, E., Heymes, C., et al. (2007). Levosimendan restores both systolic and diastolic cardiac performance in lipopolysaccharide-treated rabbits: comparison with dobutamine and milrinone. Crit. Care Med. 35, 1376–1382. doi:10.1097/01.CCM.0000261889.18102.84
Bhattacharjee, S., Soni, K. D., Maitra, S., and Baidya, D. K. (2017). Levosimendan does not provide mortality benefit over dobutamine in adult patients with septic shock: a meta-analysis of randomized controlled trials. J. Clin. Anesth. 39, 67–72. doi:10.1016/j.jclinane.2017.03.011
Bian, X., Liu, L., Xia, W., and Qin, Y. (2020). Protective effect of levosimendan on renal function in patients with septic shock. Clin. Misdiagn Misther 33, 48–54. doi:10.3969/j.issn.1002-3429.2020.05.011
Chang, Q., Chen, D., Wu, W., and Zhang, J. (2020). Effects of ulinastatin combined with Danhong injection on the level of STREM-1and HBP,Th17/Treg and clinical efficacy in sepsis patients. J. Hebei Med. Univ. 41, 899–904. doi:10.3969/j.issn.1007-3205.2020.08.008
Chang, W., Xie, J. F., Xu, J. Y., and Yang, Y. (2018). Effect of levosimendan on mortality in severe sepsis and septic shock: a meta-analysis of randomised trials. BMJ Open 8, e019338. doi:10.1136/bmjopen-2017-019338
Chen, C., and Fu, H. (2023). Evaluation of efficacy of levosimendan in septic shock based on cardiac function and hemodynamic parameters. Mod. Med. Health Res. Electron J. 7, 13–15. doi:10.3969/j.issn.2096-3718.2023.11.005
Doorduin, J., Sinderby, C. A., Beck, J., Stegeman, D. F., Van Hees, H. W., Van Der Hoeven, J. G., et al. (2012). The calcium sensitizer levosimendan improves human diaphragm function. Am. J. Respir. Crit. Care Med. 185, 90–95. doi:10.1164/rccm.201107-1268OC
Dugar, S., Choudhary, C., and Duggal, A. (2020). Sepsis and septic shock: guideline-based management. Cleve Clin. J. Med. 87, 53–64. doi:10.3949/ccjm.87a.18143
Dünser, M. W., Ruokonen, E., Pettilä, V., Ulmer, H., Torgersen, C., Schmittinger, C. A., et al. (2009). Association of arterial blood pressure and vasopressor load with septic shock mortality: a post hoc analysis of a multicenter trial. Crit. Care 13, R181. doi:10.1186/cc8167
Fan, Z., Chen, H., Yan, J., Le, J., Zhou, X., and Zhu, J. (2019). Levosimendan's influence on myocardial depression and patients with septic shock. Acta Medica Mediterr. 35, 1103–1108. doi:10.19193/0393-6384_2019_2_170
Fang, H., Jin, X., and Xiong, L. (2021). Effect of Ginkgo leaf extract and dipyridamole injection on serum tumor necrosis factor-α, procalcitonin, bacterial lipopolysaccharide and 28-day mortality in patients with sepsis. Chin. J. Prim. Med. Pharm. 28, 330–334. doi:10.3760/cma.j.issn.1008-6706.2021.03.003
Fang, M., and Dong, S. (2014). Effects of levosimendan on hemodynamics and cardiac function in patients with septic shock. Zhonghua Wei Zhong Bing Ji Jiu Yi Xue 26, 692–696. doi:10.3760/cma.j.issn.2095-4352.2014.10.002
Farmakis, D., Alvarez, J., Gal, T. B., Brito, D., Fedele, F., Fonseca, C., et al. (2016). Levosimendan beyond inotropy and acute heart failure: evidence of pleiotropic effects on the heart and other organs: an expert panel position paper. Int. J. Cardiol. 222, 303–312. doi:10.1016/j.ijcard.2016.07.202
Fleischmann, C., Scherag, A., Adhikari, N. K., Hartog, C. S., Tsaganos, T., Schlattmann, P., et al. (2016). Assessment of global incidence and mortality of hospital-treated sepsis. Current estimates and limitations. Am. J. Respir. Crit. Care Med. 193, 259–272. doi:10.1164/rccm.201504-0781OC
Follath, F., Cleland, J. G., Just, H., Papp, J. G., Scholz, H., Peuhkurinen, K., et al. (2002). Efficacy and safety of intravenous levosimendan compared with dobutamine in severe low-output heart failure (the LIDO study): a randomised double-blind trial. Lancet 360, 196–202. doi:10.1016/s0140-6736(02)09455-2
Frommeyer, G., Kohnke, A., Ellermann, C., Dechering, D. G., Kochhäuser, S., Reinke, F., et al. (2017). Acute infusion of levosimendan enhances atrial fibrillation in an experimental whole-heart model. Int. J. Cardiol. 236, 423–426. doi:10.1016/j.ijcard.2017.01.106
Gordon, A. C., Perkins, G. D., Singer, M., Mcauley, D. F., Orme, R. M., Santhakumaran, S., et al. (2016). Levosimendan for the prevention of acute organ dysfunction in sepsis. N. Engl. J. Med. 375, 1638–1648. doi:10.1056/NEJMoa1609409
Grossini, E., Farruggio, S., Pierelli, D., Bolzani, V., Rossi, L., Pollesello, P., et al. (2020). Levosimendan improves oxidative balance in cardiogenic shock/low cardiac output patients. J. Clin. Med. 9, 373. doi:10.3390/jcm9020373
Grossini, E., Molinari, C., Caimmi, P. P., Uberti, F., and Vacca, G. (2009). Levosimendan induces NO production through p38 MAPK, ERK and Akt in porcine coronary endothelial cells: role for mitochondrial K(ATP) channel. Br. J. Pharmacol. 156, 250–261. doi:10.1111/j.1476-5381.2008.00024.x
Hajjej, Z., Meddeb, B., Sellami, W., Labbene, I., Morelli, A., and Ferjani, M. (2017). Effects of levosimendan on cellular metabolic alterations in patients with septic shock: a randomized controlled pilot study. Shock 48, 307–312. doi:10.1097/SHK.0000000000000851
Heringlake, M., Alvarez, J., Bettex, D., Bouchez, S., Fruhwald, S., Girardis, M., et al. (2021). An update on levosimendan in acute cardiac care: applications and recommendations for optimal efficacy and safety. Expert Rev. Cardiovasc Ther. 19, 325–335. doi:10.1080/14779072.2021.1905520
Herpain, A., Bouchez, S., Girardis, M., Guarracino, F., Knotzer, J., Levy, B., et al. (2019). Use of levosimendan in intensive care unit settings: an opinion paper. J. Cardiovasc Pharmacol. 73, 3–14. doi:10.1097/FJC.0000000000000636
Hobai, I. A., Edgecomb, J., Labarge, K., and Colucci, W. S. (2015). Dysregulation of intracellular calcium transporters in animal models of sepsis-induced cardiomyopathy. Shock 43, 3–15. doi:10.1097/SHK.0000000000000261
Hua, X., Cao, L., and Wang, Y. (2022). Efficacy and safety of rh-BNP combined with levosimendan in treatment of elderly patients with sepsis-induced myocardial dysfunction. J. Tongji Univ. Med. Sci. 43, 100–106. doi:10.12289/j.issn.1008-0392.21332
Huang, H., Sun, X., Mak, X., Tong, L., and Hu, G. (2015). The clinical effects of levosimendan in treatment of septic shock and cardiac depression. Sichuan Med. J. 36, 831–834. doi:10.16252/j.cnki.issn1004-0501-2015.06.020
Huang, Y., Wu, G., Yuan, M., and Huo, B. (2017). Comparative observation of effects of levosimendan and dobutamine on myocardial inhibition in patients with septic shock. Lingnan J. Emerg. Med. 22, 4–6. doi:10.3969/j.issn.1671-301X.2017.01.002
Hutton, B., Salanti, G., Caldwell, D. M., Chaimani, A., Schmid, C. H., Cameron, C., et al. (2015). The PRISMA extension statement for reporting of systematic reviews incorporating network meta-analyses of health care interventions: checklist and explanations. Ann. Intern Med. 162, 777–784. doi:10.7326/M14-2385
Janssens, U. (2017). Levosimendan-no effect on multiorgan failure in septic shock. Med. Klin. Intensivmed. Notfmed 112, 254–257. doi:10.1007/s00063-016-0248-7
Kaheinen, P., Pollesello, P., Levijoki, J., and Haikala, H. (2004). Effects of levosimendan and milrinone on oxygen consumption in isolated Guinea-pig heart. J. Cardiovasc Pharmacol. 43, 555–561. doi:10.1097/00005344-200404000-00011
Kern, H., Schröder, T., Kaulfuss, M., Martin, M., Kox, W. J., and Spies, C. D. (2001). Enoximone in contrast to dobutamine improves hepatosplanchnic function in fluid-optimized septic shock patients. Crit. Care Med. 29, 1519–1525. doi:10.1097/00003246-200108000-00004
Koya, H. H., and Paul, M. (2023). “Shock,” in StatPearls (Treasure Island, FL: StatPearls Publishing).
Lai, Z., Meng, J., Hu, M., Xu, X., Ji, C., and Zhang, G. (2016). Effects of levosimendan on hemodynamics and markers of myocardial damage in patients with septic shock. Chin. J. Crit. Care Med. Electron. Ed. 9, 163–168. doi:10.3877/cma.j.issn.1674-6880.2016.03.005
Lan, Y., Yu, J., Liu, R. A., Lei, Y., Luo, X., and Zeng, F. (2018). Effects of levosimendan on patients with sepsis-induced acute heart failure. Pract. Pharm. Clin. Rem. 21, 1369–1372. doi:10.14053/j.cnki.ppcr.201812011
Lannemyr, L., Ricksten, S. E., Rundqvist, B., Andersson, B., Bartfay, S. E., Ljungman, C., et al. (2018). Differential effects of levosimendan and dobutamine on glomerular filtration rate in patients with heart failure and renal impairment:A randomized double-blind controlled trial. J. Am. Heart Assoc. 7, e008455. doi:10.1161/JAHA.117.008455
Leppikangas, H., Järvelä, K., Sisto, T., Maaranen, P., Virtanen, M., Lehto, P., et al. (2011). Preoperative levosimendan infusion in combined aortic valve and coronary bypass surgery. Br. J. Anaesth. 106, 298–304. doi:10.1093/bja/aeq402
Lew, W. Y., Yasuda, S., Yuan, T., and Hammond, H. K. (1996). Endotoxin-induced cardiac depression is associated with decreased cardiac dihydropyridine receptors in rabbits. J. Mol. Cell Cardiol. 28, 1367–1371. doi:10.1006/jmcc.1996.0127
Liu, D. (2017). What does Sepsis-3 mean beyond definition? Zhonghua Wei Zhong Bing Ji Jiu Yi Xue 29, 97–98. doi:10.3760/cma.j.issn.2095-4352.2017.02.001
Liu, H., Geng, J., He, Z., Lang, Z., Meng, W., and Hu, X. (2020). Effect of levosimendan on myocardial depression and cardiac function in sepsis. J. Clin. Intern Med. 37, 447–448. doi:10.3969/j.issn.1001-9057.2020.06.016
Liu, H., Geng, J., He, Z., Lang, Z., Meng, W., and Hu, X. (2021). Effects of Levosimendan on left ventricular systolic function, hemodynamics and myocardial injury marker levels in patients with septic myocardial depression. Chin. J. Difficult Complicat. Cases 20, 348–352. doi:10.3969/j.issn.1671-6450.2021.04.006
Lu, Z., He, Q., and Cai, J. (2020). Early application of levosimendan on myocardial inhibition and hemodynamic effects in patients with septic shock. Strait Pharm. J. 32, 135–137. doi:10.3969/j.issn.1006-3765.2020.12.049
Maack, C., Eschenhagen, T., Hamdani, N., Heinzel, F. R., Lyon, A. R., Manstein, D. J., et al. (2019). Treatments targeting inotropy. Eur. Heart J. 40, 3626–3644. doi:10.1093/eurheartj/ehy600
Matejovic, M., Krouzecky, A., Radej, J., and Novak, I. (2005). Successful reversal of resistent hypodynamic septic shock with levosimendan. Acta Anaesthesiol. Scand. 49, 127–128. doi:10.1111/j.1399-6576.2005.00541.x
Mehta, R. H., Leimberger, J. D., Van Diepen, S., Meza, J., Wang, A., Jankowich, R., et al. (2017). Levosimendan in patients with left ventricular dysfunction undergoing cardiac surgery. N. Engl. J. Med. 376, 2032–2042. doi:10.1056/NEJMoa1616218
Memiş, D., Inal, M. T., and Sut, N. (2012). The effects of levosimendan vs dobutamine added to dopamine on liver functions assessed with noninvasive liver function monitoring in patients with septic shock. J. Crit. Care 27, e1–e6. doi:10.1016/j.jcrc.2011.06.008
Meng, J. B., Hu, M. H., Lai, Z. Z., Ji, C. L., Xu, X. J., Zhang, G., et al. (2016). Levosimendan versus dobutamine in myocardial injury patients with septic shock: a randomized controlled trial. Med. Sci. Monit. 22, 1486–1496. doi:10.12659/msm.898457
Morelli, A., De Castro, S., Teboul, J. L., Singer, M., Rocco, M., Conti, G., et al. (2005). Effects of levosimendan on systemic and regional hemodynamics in septic myocardial depression. Intensive Care Med. 31, 638–644. doi:10.1007/s00134-005-2619-z
Morelli, A., Donati, A., Ertmer, C., Rehberg, S., Lange, M., Orecchioni, A., et al. (2010). Levosimendan for resuscitating the microcirculation in patients with septic shock: a randomized controlled study. Crit. Care 14, R232. doi:10.1186/cc9387
Morelli, A., Teboul, J. L., Maggiore, S. M., Vieillard-Baron, A., Rocco, M., Conti, G., et al. (2006). Effects of levosimendan on right ventricular afterload in patients with acute respiratory distress syndrome: a pilot study. Crit. Care Med. 34, 2287–2293. doi:10.1097/01.CCM.0000230244.17174.4F
Pan, C. (2019). Effects of Levosimendan on blood lactate, sTREM-1, sCD14 and sCD163 levels in patients with septic shock. Med. J. Chin. People Health 31, 4–6+9. doi:10.3969/j.issn.1672-0369.2019.14.002
Pan, J., Yang, Y. M., Zhu, J. Y., and Lu, Y. Q. (2019). Multiorgan drug action of levosimendan in critical illnesses. Biomed. Res. Int. 2019, 9731467. doi:10.1155/2019/9731467
Paraskevaidis, I. A., Parissis, J. T., and Th Kremastinos, D. (2005). Anti-inflammatory and anti-apoptotic effects of levosimendan in decompensated heart failure: a novel mechanism of drug-induced improvement in contractile performance of the failing heart. Curr. Med. Chem. Cardiovasc Hematol. Agents 3, 243–247. doi:10.2174/1568016054368232
Parissis, J. T., Adamopoulos, S., Antoniades, C., Kostakis, G., Rigas, A., Kyrzopoulos, S., et al. (2004). Effects of levosimendan on circulating pro-inflammatory cytokines and soluble apoptosis mediators in patients with decompensated advanced heart failure. Am. J. Cardiol. 93, 1309–1312. doi:10.1016/j.amjcard.2004.01.073
Pataricza, J., Krassói, I., Höhn, J., Kun, A., and Papp, J. G. (2003). Functional role of potassium channels in the vasodilating mechanism of levosimendan in porcine isolated coronary artery. Cardiovasc Drugs Ther. 17, 115–121. doi:10.1023/a:1025331617233
Pollesello, P., Papp, Z., and Papp, J. G. (2016). Calcium sensitizers: what have we learned over the last 25 years? Int. J. Cardiol. 203, 543–548. doi:10.1016/j.ijcard.2015.10.240
Ponikowski, P., Voors, A. A., Anker, S. D., Bueno, H., Cleland, J. G. F., Coats, A. J. S., et al. (2016). 2016 ESC Guidelines for the diagnosis and treatment of acute and chronic heart failure: the Task Force for the diagnosis and treatment of acute and chronic heart failure of the European Society of Cardiology (ESC)Developed with the special contribution of the Heart Failure Association (HFA) of the ESC. Eur. Heart J. 37, 2129–2200. doi:10.1093/eurheartj/ehw128
Ravikumar, N., Sayed, M. A., Poonsuph, C. J., Sehgal, R., Shirke, M. M., and Harky, A. (2021). Septic cardiomyopathy: from basics to management choices. Curr. Probl. Cardiol. 46, 100767. doi:10.1016/j.cpcardiol.2020.100767
Saevik, M., Beitnes, J. O., Aaberge, L., and Halvorsen, P. S. (2021). Safety and feasibility of dobutamine stress echocardiography in symptomatic high gradient aortic stenosis patients scheduled for transcatheter aortic valve implantation. J. Clin. Ultrasound 49, 38–48. doi:10.1002/jcu.22915
Sareila, O., Korhonen, R., Auvinen, H., Hämäläinen, M., Kankaanranta, H., Nissinen, E., et al. (2008). Effects of levo- and dextrosimendan on NF-kappaB-mediated transcription, iNOS expression and NO production in response to inflammatory stimuli. Br. J. Pharmacol. 155, 884–895. doi:10.1038/bjp.2008.328
Sato, R., and Nasu, M. (2015). A review of sepsis-induced cardiomyopathy. J. Intensive Care 3, 48. doi:10.1186/s40560-015-0112-5
Schauer, A., Barthel, P., Adams, V., Linke, A., Poitz, D. M., and Weinbrenner, C. (2021). Pharmacological pre- and postconditioning with levosimendan protect H9c2 cardiomyoblasts from anoxia/reoxygenation-induced cell death via PI3K/Akt signaling. J. Cardiovasc Pharmacol. 77, 378–385. doi:10.1097/FJC.0000000000000969
Schellekens, W. J., Van Hees, H. W., Linkels, M., Dekhuijzen, P. N., Scheffer, G. J., Van Der Hoeven, J. G., et al. (2015). Levosimendan affects oxidative and inflammatory pathways in the diaphragm of ventilated endotoxemic mice. Crit. Care 19, 69. doi:10.1186/s13054-015-0798-8
Schmittinger, C. A., Torgersen, C., Luckner, G., Schröder, D. C., Lorenz, I., and Dünser, M. W. (2012). Adverse cardiac events during catecholamine vasopressor therapy: a prospective observational study. Intensive Care Med. 38, 950–958. doi:10.1007/s00134-012-2531-2
Shi, J., and Lin, Y. (2020). Clinical observation of levosimendan on septic shock patients. Shanxi Med. J. 49, 2461–2463. doi:10.3969/j.issn.0253-9926.2020.18.016
Society of Critical Care Medicine and Chinese Medical Association (2015). Guidelines for the treatment of severe sepsis/septic shock in China (2014). Clin. Educ. General Pract. 13, 365–367. doi:10.3760/cma.j.issn.0578-1426.2015.06.021
Sorsa, T., Pollesello, P., and Solaro, R. J. (2004). The contractile apparatus as a target for drugs against heart failure: interaction of levosimendan, a calcium sensitiser, with cardiac troponin c. Mol. Cell Biochem. 266, 87–107. doi:10.1023/b:mcbi.0000049141.37823.19
Stratton, L., Berlin, D. A., and Arbo, J. E. (2017). Vasopressors and inotropes in sepsis. Emerg. Med. Clin. North Am. 35, 75–91. doi:10.1016/j.emc.2016.09.005
Su, B., Hu, Y., and Fan, H. (2018). Levosimendan as an adjunctive therapy for patients with septic shock. Zhejiang Med. J. 40, 2250–2252+2256. doi:10.12056/j.issn.1006-2785.2018.40.20.2017-2337
Sun, T., Zhang, N., Cui, N., Wang, S. H., Ding, X. X., Li, N., et al. (2023). Efficacy of levosimendan in the treatment of patients with severe septic cardiomyopathy. J. Cardiothorac. Vasc. Anesth. 37, 344–349. doi:10.1053/j.jvca.2022.10.032
Szilágyi, S., Pollesello, P., Levijoki, J., Kaheinen, P., Haikala, H., Edes, I., et al. (2004). The effects of levosimendan and OR-1896 on isolated hearts, myocyte-sized preparations and phosphodiesterase enzymes of the Guinea pig. Eur. J. Pharmacol. 486, 67–74. doi:10.1016/j.ejphar.2003.12.005
Tan, R., Du, Q., and Guo, H. (2023). Levosimendan in sepsis: a systematic review and network meta-analysis. Available at: https://www.crd.york.ac.uk/prospero/display_record.php?ID=CRD42023441220.
Tasouli, A., Papadopoulos, K., Antoniou, T., Kriaras, I., Stavridis, G., Degiannis, D., et al. (2007). Efficacy and safety of perioperative infusion of levosimendan in patients with compromised cardiac function undergoing open-heart surgery: importance of early use. Eur. J. Cardiothorac. Surg. 32, 629–633. doi:10.1016/j.ejcts.2007.07.010
Tavernier, B., Mebazaa, A., Mateo, P., Sys, S., Ventura-Clapier, R., and Veksler, V. (2001). Phosphorylation-dependent alteration in myofilament ca2+ sensitivity but normal mitochondrial function in septic heart. Am. J. Respir. Crit. Care Med. 163, 362–367. doi:10.1164/ajrccm.163.2.2002128
Torraco, A., Carrozzo, R., Piemonte, F., Pastore, A., Tozzi, G., Verrigni, D., et al. (2014). Effects of levosimendan on mitochondrial function in patients with septic shock: a randomized trial. Biochimie 102, 166–173. doi:10.1016/j.biochi.2014.03.006
Vincent, J. L., and Bakker, J. (2021). Blood lactate levels in sepsis: in 8 questions. Curr. Opin. Crit. Care 27, 298–302. doi:10.1097/MCC.0000000000000824
Vincent, J. L., Marshall, J. C., Namendys-Silva, S. A., François, B., Martin-Loeches, I., Lipman, J., et al. (2014). Assessment of the worldwide burden of critical illness: the intensive care over nations (ICON) audit. Lancet Respir. Med. 2, 380–386. doi:10.1016/S2213-2600(14)70061-X
Wang, C., and Wang, Y. (2019). The research progress of levosimendan in the perioperative period of cardiac surgery. J. Clin. Anesthesiol. 35, 719–722. doi:10.12089/jca.2019.07.022
Wang, D., Feng, X., and Wang, S. (2018a). Effects of esmolol combined with levosimendan on cardiac function in patients with severe sepsis. Chin. J. Pract. Med. 45, 87–90. doi:10.3760/cma.j.issn.1674-4756.2018.18.030
Wang, J., Xin, S., Sun, Q., Ma, W., Qi, Y., Wang, Y., et al. (2021). Clinical study of digoxin injection combined with milrinone injection in the treatment of sepsis-induced myocardial dysfunction. Lingnan J. Emerg. Med. 26, 238–241. doi:10.3969/j.issn.1671-301X.2021.03.006
Wang, Q., Yokoo, H., Takashina, M., Sakata, K., Ohashi, W., Abedelzaher, L. A., et al. (2015). Anti-inflammatory profile of levosimendan in cecal ligation-induced septic mice and in lipopolysaccharide-stimulated macrophages. Crit. Care Med. 43, e508–e520. doi:10.1097/CCM.0000000000001269
Wang, W., Hu, F., Dou, H., Ling, L., and Wang, D. (2018b). Effects of levosimendan and dobutamine on serum levels of sTREM-1,sCD14 and sCD163 in treatment of sepsis shock. Hebei Med. J. 40, 3306–3309. doi:10.3969/j.issn.1002-7386.2018.21.026
Werner, H. A., Herbertson, M. J., and Walley, K. R. (1995). Amrinone increases ventricular contractility and diastolic compliance in endotoxemia. Am. J. Respir. Crit. Care Med. 152, 496–503. doi:10.1164/ajrccm.152.2.7633698
Wu, G., and Chang, P. (2016). Levosimendan versus dobutamine in myocardial injury patients with septic shock. Labeled Immunoassays Clin. Med. 23, 1450–1453. doi:10.11748/bjmy.issn.1006-1703.2016.12.026
Xu, C. X., Li, L., Gong, S. J., Yu, Y. H., and Yan, J. (2018). The effects of levosimendan on the cardiac function and prognosis in elderly patients with septic shock and myocardial contractility impairment. Zhonghua Nei Ke Za Zhi 57, 423–428. doi:10.3760/cma.j.issn.0578-1426.2018.06.006
Yan, Z., Li, J., Xia, Z., Han, B., Wu, L., and Pan, Y. (2016). Effect of levosimendan on hemodynamics in patients with sepsis-induced myocardial dysfunction and its mechanism. Contemp. Med. 22, 3–5. doi:10.3969/j.issn.1009-4393.2016.27.002
Yang, F., Zhao, L. N., Sun, Y., and Chen, Z. (2019). Levosimendan as a new force in the treatment of sepsis-induced cardiomyopathy: mechanism and clinical application. J. Int. Med. Res. 47, 1817–1828. doi:10.1177/0300060519837103
Yang, L., and Yi, B. (2021). Effect of levosimendan on myocardial injury in patients with sepsis. Chin. J. Prim. Med. Pharm. 28, 533–537. doi:10.3760/cma.j.issn.1008-6706.2021.04.012
Yang, S., and Li, X. (2019). Observation of clinical efficacy of levosimendan in the treatment of septic shock with myocardial suppression. Chin. J. Clin. Ration. Drug Use 12, 31–32. doi:10.15887/j.cnki.13-1389/r.2019.14.016
Yokoshiki, H., Katsube, Y., Sunagawa, M., and Sperelakis, N. (1997). Levosimendan, a novel Ca2+ sensitizer, activates the glibenclamide-sensitive K+ channel in rat arterial myocytes. Eur. J. Pharmacol. 333, 249–259. doi:10.1016/s0014-2999(97)01108-4
Zangrillo, A., Putzu, A., Monaco, F., Oriani, A., Frau, G., De Luca, M., et al. (2015). Levosimendan reduces mortality in patients with severe sepsis and septic shock: a meta-analysis of randomized trials. J. Crit. Care 30, 908–913. doi:10.1016/j.jcrc.2015.05.017
Zhang, D., Yao, Y., Qian, J., and Huang, J. (2015). Levosimendan improves clinical outcomes of refractory heart failure in elderly Chinese patients. Med. Sci. Monit. 21, 2439–2445. doi:10.12659/MSM.893580
Zhao, F., Liu, Y., and Jiang, Y. (2021). Study on curative effect of short-term use of milrinone in patients with septic myocardial injury. J. Mod. Med. Health 37, 1108–1111. doi:10.3969/j.issn.1009-5519.2021.07.009
Zhou, X. (2023). Effects of levosimendan adjuvant therapy on hemodynamics and disease-related indexes in septic shock patients. Mod. Med. Health Res. Electron J. 7, 67–70. doi:10.3969/j.issn.2096-3718.2023.02.020
Zhuang, C., Wu, W., Bei, Z., Li, J., and Zhong, J. (2023). Effect of milrinone combined with ulinastatin on myocardial inhibition of sepsis. China J. Pharm. Econ. 18, 66–69. doi:10.12010/j.issn.1673-5846.2023.11.014
Keywords: levosimendan, sepsis, systematic review, network meta-analysis, intensive care unit
Citation: Tan R, Guo H, Yang Z, Yang H, Li Q, Zhu Q and Du Q (2024) Efficacy and safety of levosimendan in patients with sepsis: a systematic review and network meta-analysis. Front. Pharmacol. 15:1358735. doi: 10.3389/fphar.2024.1358735
Received: 20 December 2023; Accepted: 21 February 2024;
Published: 08 March 2024.
Edited by:
Mahmoud El-Mas, Alexandria University, EgyptReviewed by:
Elena Grossini, University of Eastern Piedmont, ItalyEman Gohar, Vanderbilt University Medical Center, United States
Copyright © 2024 Tan, Guo, Yang, Yang, Li, Zhu and Du. This is an open-access article distributed under the terms of the Creative Commons Attribution License (CC BY). The use, distribution or reproduction in other forums is permitted, provided the original author(s) and the copyright owner(s) are credited and that the original publication in this journal is cited, in accordance with accepted academic practice. No use, distribution or reproduction is permitted which does not comply with these terms.
*Correspondence: Quansheng Du, ZHFzODg4QDEyNi5jb20=