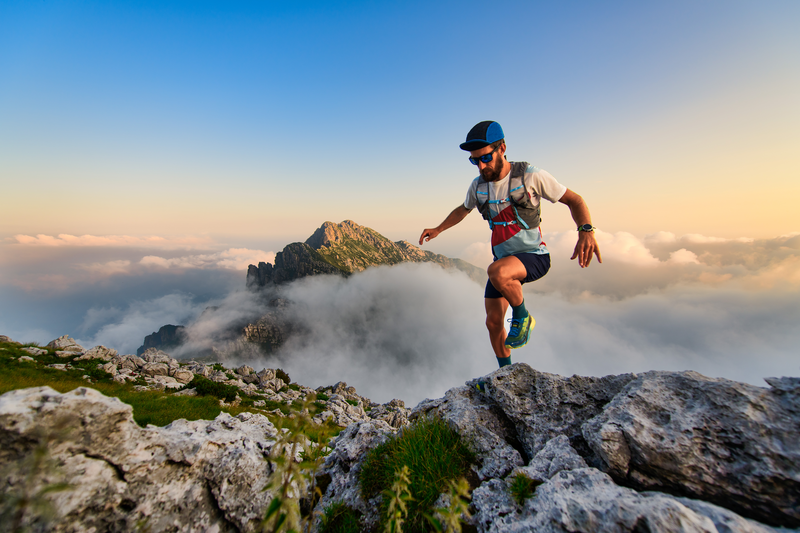
94% of researchers rate our articles as excellent or good
Learn more about the work of our research integrity team to safeguard the quality of each article we publish.
Find out more
ORIGINAL RESEARCH article
Front. Pharmacol. , 25 April 2024
Sec. Ethnopharmacology
Volume 15 - 2024 | https://doi.org/10.3389/fphar.2024.1355650
This article is part of the Research Topic Modulation of the Crosstalk Between Tumor Cells and Microenvironmental Cells by Herbal Medicine View all articles
Distant metastasis is a major cause of treatment failure in cancer patients and a key challenge to improving cancer care today. We hypothesized that enhancing anti-cancer immune response and inhibiting circulating tumor cells (CTCs) adhesion and transendothelial migration through synergistic multi-target approaches may effectively prevent cancer metastasis. “Fuyuan Decoction” (FYD) is a traditional Chinese medicine compound that is widely used to prevent postoperative metastasis in cancer patients, but its underlying mechanism remains unclear. In this work, we systematically elucidated the underlying molecular mechanism by which FYD prevents cancer metastasis through multi-compound and multi-target synergies in vitro and in vivo. FYD significantly prevented cancer metastasis at non-cytotoxic concentrations by suppressing the adhesion of CTCs to endothelial cells and their subsequent transendothelial migration, as well as enhancing anti-cancer immune response. Mechanistically, FYD interrupts adhesion of CTCs to vascular endothelium by inhibiting TNF-α-induced CAMs expression via regulation of the NF-κB signaling pathway in endothelial cells. FYD inhibits invasion and migration of CTCs by suppressing EMT, PI3K/AKT and FAK signaling pathways. Moreover, FYD enhances the anti-cancer immune response by significantly increasing the population of Tc and NK cells in the peripheral immune system. In addition, the chemical composition of FYD was determined by UPLC-HRMS, and the results indicated that multiple compounds in FYD prevents cancer metastasis through multi-target synergistic treatment. This study provides a modern medical basis for the application of FYD in the prevention of cancer metastasis, and suggesting that multi-drug and multi-target synergistic therapy may be one of the most effective ways to prevent cancer metastasis.
Cancer is one of the deadliest diseases, and it is estimated that 30.2 million new cancer diagnoses and 16.3 million cancer-related deaths in the word in 2040 (Sung et al., 2021). Metastasis is a critical element leading to the failure of clinical cancer treatment, and more than 90% of cancer-related deaths are related to metastasis (Ferlay et al., 2019). The nasopharyngeal carcinoma (NPC) is a malignant tumor originating from the epithelial cells of the nasopharynx and represents the most prevalent head and neck cancer in South China and Southeast Asia (Chua et al., 2016; Zhang et al., 2022). The incidence rate of NPC has exhibited a significant increase in recent years, with men experiencing notably higher rates of both incidence and mortality compared to women. (Yu et al., 2022). The primary therapeutic approach for patients with advanced NPC involves the utilization of radiotherapy or chemotherapy in combination with radiotherapy; however, the current survival rates remain unsatisfactory due to the emergence of acquired drug resistance and metastasis (Zhong et al., 2018; Zhang et al., 2021). Therefore, it is imperative to explore novel therapeutic and chemopreventive strategies in order to enhance the prognosis of patients afflicted with NPC.
Cancer metastasis is a multi-stage process, mainly including cancer cells shedding from the primary site, entering the vascular microenvironment and migrating to distant tissues and organs (Paul et al., 2017). Cancer cells that enter the blood circulation system are called circulating tumor cells (CTCs). However, the survival of circulating tumor cells (CTCs) in the bloodstream is limited to a small fraction due to mechanical shear stress and immune cell-mediated clearance (Leblanc and Peyruchaud, 2016). Studies have shown that surviving CTCs can establish new tumor lesions and form metastases by adhering to the vascular endothelium and through vascular infiltration (Gay and Felding-Habermann, 2011). Therefore, the adhesion of CTCs to endothelium in the bloodstream microenvironment is a critical step in facilitating distant metastasis (Stott et al., 2010). Cell adhesion molecules (CAMs, including VCAM-1, ICAM-1 and E-selectin), that are involved in the adhesion of CTCs to endothelial cells and are regulated by the NF-κB signaling pathway induced by inflammatory cytokines (Lu et al., 2014; Lu et al., 2019; Liu et al., 2021). The transendothelial migration of CTCs is another critical process facilitating successful metastasis, which is tightly regulated by the epithelial-to-mesenchymal transition (EMT), PI3K/AKT, and FAK signaling pathways (Wang et al., 2022). Furthermore, previous studies have shown that tumor infiltrating cells (TIMs, TILs), along with immunosuppressive checkpoints, play a significant role in immune evasion of NPC cells, ultimately leading to increased growth and metastasis (Al-Rajhi et al., 2020; Liu et al., 2022; Yang et al., 2022). Therefore, based on a comprehensive understanding of the intricate processes underlying cancer metastasis, we proposed that enhancing anti-cancer immune response and inhibiting CTCs adhesion and transendothelial migration through multi-target synergies may effectively prevent cancer metastasis.
Traditional Chinese medicine (TCM) is widely accepted as an effective strategy for the treatment of cancers due to its high efficiency, low toxicity and multiple targets (Yuan et al., 2017; Xiang et al., 2019). In recent years, many herbal monomers have been found to be beneficial in suppressing cancer invasion and metastasis (Wang et al., 2022; Zhong et al., 2023). However, Chinese herbal compounds possessing multi-components and multi-target characteristics may present a more suitable approach for chemoprevention of cancer metastasis, as they aim to enhance the overall therapeutic efficacy in individuals with cancer (Jiang and Liu, 2011; Sarwar et al., 2018; Wang et al., 2020; Yang et al. 2021; Xiangqi Ye and Ye 2021). Fuyuan Decoction (FYD), a traditional Chinese formula comprised of nine herbs (the specific components are presented in the Methods section), has been utilized for years to prevent metastasis of cancer patients after surgery, and has shown favorable clinical response (Xiangqi Ye and Ye 2021). The herbs that make up FYD exhibits many anti-cancer activities. The triterpenoids of Ganoderma lucidum can inhibit telomerase activity and prevent the development of NPC (Zheng and Chen, 2017). We recently found that G. lucidum extract promotes tumor cell pyroptosis and inhibits metastasis in breast cancer (Zhong et al., 2023). Ginsenoside Rg3, a component of Chinese ginseng, can decrease NPC migration and invasion (Wang et al., 2019), and can cause apoptosis in NPC by activating apoptosis-inducing factors (Xie et al., 2013; Law et al., 2014). Astragalus polysaccharide triggered apoptosis in nasopharyngeal cancer cells and altered the Bax/bcl-2 ratio and cell cycle (Zhou et al., 2017; Yang et al., 2021). A network correlation analysis revealed that the Hedyotis diffusa and Scutellaria barbata might improve the survival rate of patients with advanced nasopharyngeal cancer (Han et al., 2020; Wang et al., 2020). Despite numerous studies demonstrating the anti-tumor properties of a single herb in FYD, the precise mechanism underlying the inhibitory effects of FYD compound on cancer metastasis remains elusive.
In this study, we investigated the molecular mechanisms by which FYD prevents NPC metastasis in vitro and in vivo. In vitro experiments, we characterized the chemical composition of FYD and examined its impact on CNE1 cell viability, adhesion, invasion ability, as well as elucidated its underlying molecular mechanism. Additionally, we conducted in vivo studies to evaluate the immunomodulatory properties of FYD and its efficacy in preventing cancer metastasis using syngeneic mouse models.
The FYD consists of nine different herbs: Actindia arguta (Siebold & Zucc.) Planch. ex Miq. [Actinidiaceae; Actindia arguta root] 25 g, S. barbata D.Don [Lamiaceae; Scutellaria barbata whole plant] 20 g, Scleromitrion diffusum (Willd.) R.J.Wang [Rubiaceae; Scleromitrion diffusum whole plant] 40 g, Iphigenia indica (L.) A.Gray ex Kunth [Colchicaceae; Iphigenia indica pseudobulb] 4 g, Salvia miltiorrhiza Bunge [Lamiaceae; Salviae miltiorrhizae radix et rhizome] 20 g, G. lucidum (Curtis) P. Karst. 15 g, Panax ginseng C.A.Mey. [Araliaceae; Panax ginseng radix et rhizome] 12 g, Lycium chinense Mill. [Solanaceae; Lycium chinense fruit] 12 g, and Astragalus mongholicus Bunge [Fabaceae; Astragalus mongholicus root] 20 g. All herbs were purchased from Jiangxi Kangqingtang Herbal decoction Pieces Co., Ltd. (Zhangshu, China). FYD standard decoction was prepared according to “Management standard of traditional Chinese medicine (TCM) decoction in medical institutions” (Wang et al., 2019). The water-mixed raw materials were soak for 30 min, then decocted for another 45 min and filtered for collection of the filtrate. The residue was decocted again and filtered twice. The filtrates were then freeze-dried to obtain FYD powder.
Compound identification of FYD, cell culturing, cell viability assay, cell cycle assay, cell apoptosis assay, hetero-adhesion assay, migration assay, invasion assay, flow cytometry, western blot, RNA extraction and qRT-PCR assay, in vivo pulmonary metastasis assays are described in the online Supplementary Material. The primer sequences for qPCR were presented in Supplementary Table S1.
Specific pathogen-free female BALB/c mice (20–22 g, 6–8 weeks old) were procured from the Shanghai SLAC Laboratory Animal Co., Ltd (Lu et al., 2019). The mice were housed in a controlled environment with no exposure to pathogens and provided ad libitum access to pelleted food and water. All animal experiments adhered to the guidelines outlined in accordance with the Guide for the Care and Use of Laboratory Animals (National Research Council, 1996). All animal experimental procedures were thoroughly evaluated and approved by the Laboratory Animal Ethics Committee of Minjiang University.
The results were expressed as means and standard deviations. Statistical analyses were conducted using the SPSS statistical software package. Differences in experimental data were assessed through analysis of variance (ANOVA), with Dunnett’s method employed for adjusting the ANOVA for multiple comparisons. A significance level of p < 0.05 was considered statistically significant, while a significance level of p < 0.01 was considered extremely statistically significant.
UPLC-HRMS analysis revealed that FYD comprises a total of 1430 chemical components, which can be classified and identified into 107 categories (Supplementary Table S2). The categories of the top 6 compounds and their subclasses were shown in Supplementary Table S3, and the proportion of the number of the top 6 compounds and their subclasses was shown in Supplementary Figure S1. The peaks exhibiting higher abundance in the positive and negative ion base peak chromatograms (BPC) underwent confirmation of their peak shape and secondary spectral examination prior to being assigned with numerical labels. Sequential numbering was applied to both the positive and negative ion chromatograms (Figures 1A,B). The 40 peaks identified in the positive and negative ion BPC diagram for FYD composition analysis mainly comprise carboxylic acids, purine nucleosides, coumarins, flavonoids, organic oxides, hydroxy acids, cinnamic acids, lipids and other classes of compounds. The detailed information of the compounds was shown in Supplementary Table S4.
Figure 1. Compound identification and cytotoxicity assessment of Fuyuan Decoction (FYD). (A, B) UHPLC-HRMS analysis was performed in positive (A) and negative (B) ion modes, generating base peak chromatograms (BPC plots) of FYD with the top 40 compounds annotated. (C, D) Cell apoptosis was evaluated using flow cytometry after incubating CNE1 cells with FYD for 24 h (C), and the results were presented as a histogram depicting the distribution of apoptotic cells (D). (E, F) Cell cycle distribution analysis was carried out using flow cytometry after treating CNE1 cells with FYD for 24 h (E), and the findings were illustrated as a histogram (F). The data are presented as mean ± SD (n = 3–6); * indicates p < 0.05 compared to the Control.
The cytotoxicity of FYD was evaluated in CNE1 cells, revealing a concentration-dependent reduction in cell viability with IC50 and IC10 (Lu et al., 2019) values of 4.695 mg/mL and 4 mg/mL, respectively (Supplementary Figure S2). We also examined the effects of FYD on the proliferative activity of normal cell lines human nasal epithelial cells HNEPC and human umbilical vein endothelial cells (HUVECs), with IC50 values of 5.218 mg/mL and 4.789 mg/mL, respectively (Supplementary Figures S3, S4). Furthermore, based on the results of cell cycle and apoptosis assays, it was observed that medication concentrations equal to or below 4 mg/mL did not exhibit any discernible effect on CNE1 cells, and apoptosis was not produced (Figures 1C–F). These findings suggest that FYD possesses weak cytotoxicity at concentrations less than 4 mg/mL. Therefore, in order to elucidate the mechanism underlying the cancer metastasis preventive effect of FYD, subsequent experiments were conducted using FYD concentrations at or below 4 mg/mL, aiming to demonstrate its inhibitory effect on CTCs adhesion and immune enhancement rather than cytotoxicity-mediated CTCs elimination.
The adhesion of CTCs to endothelial cells is a critical step in the metastasis of cancer cells to distant sites (Stott et al., 2010). Here, we investigated the impact of FYD on the heterogeneous adherence between TNF-α-induced cancer cell CNE1 and HUVECs. The results of the adhesion assay between CNE1 cells and HUVECs showed that FYD effectively inhibited adhesion with a concentration-dependent trend. In the presence of FYD doses of 0.5, 1.0, 2.0, and 4.0 mg/mL, the adhesion rates between CNE1 and HUVECs were observed to be 77.1% ± 12.8%, 30.5% ± 1.8%, 21.5% ± 3.7%, and 7.4% ± 1.1%, respectively, as compared to the control group (induced by TNF-α) (Figures 2A,B). The present study demonstrated that FYD effectively suppressed TNF-α-induced adhesion of CNE1 to HUVECs at non-toxic concentrations.
Figure 2. FYD interrupts the adhesion of CNE1 cells to HUVECs by suppressing TNF-α-induced expression of CAMs. (A) Fluorescence microscopy was used to capture images of CNE1 cells (labeled with Rhodamine 123, shown in green) adhering to HUVECs. (B) FYD significantly attenuated the adhesion of CNE1 cells to HUVECs stimulated by TNF-α. The relative adhesion percentage was calculated based on control conditions (TNF-α only). (C) HUVECs were pretreated with FYD for 24 h, followed by incubation with TNF-α (10 ng/mL) for an additional 4 h. The expression of CD106 (VCAM-1), CD54 (ICAM-1), and CD62E (E-selectin) on HUVECs was analyzed using flow cytometry. (D) FYD significantly suppressed the expression of VCAM-1, ICAM-1, and E-selectin in TNF-α-stimulated HUVECs. The relative expression of CD106, CD54, and CD62E was quantified as a percentage of the mean fluorescence intensity observed in the control group treated with TNF-α alone. NC, representing the negative control group. The data are presented as mean ± SD (n = 3–6); ** indicates p < 0.01 compared to the Control (treated with TNF-α alone); ## indicate p < 0.01 compared to the Negative control (without TNF-α).
The pro-inflammatory cytokine TNF-α plays a pivotal role in cancer metastasis by inducing the expression of CAMs on HUVECs (Lian et al., 2016). We employed flow cytometry to assess the effect of FYD on the expression of E-selectin (CD62E), ICAM-1 (CD54), and VCAM-1 (CD106) in HUVECs. The experimental results showed that FYD effectively inhibited the expression of VCAM-1, ICAM-1, and E-selectin in HUVECs with a concentration-dependent trend (Figures 2C,D). This finding prompted us to further investigate the molecular mechanism underlying FYD mediated disruption of CNE1 cells adhesion to endothelial cells in subsequent investigations.
The NF-κB signaling pathway has been extensively demonstrated to play a pivotal role in the regulation of expression of CAMs (Jayakumar et al., 2014; Zeng et al., 2021). Here, we employed western blot analysis to investigate the effect of FYD on the expression of CAMs in HUVECs via modulation of the NF-κB signaling pathway. As shown in Figures 3A–F, the NF-кB signaling pathway is activated by TNF-α through upregulation of NF-кB p65 expression and phosphorylation of p-IкBα (Ser 32), p-IKKα/β (Ser 176/180) and p-NF-кB p65 (Ser 536). Treatment with FYD in HUVECs resulted in a significant concentration-dependent reduction in the total protein level of IкBα and NF-кB p65, as well as the phosphorylation levels of p-IкBα (Ser 32), p-IKKα/β (Ser 176/180) and p-NF-кB p65 (Ser 536), compared to the TNF-α stimulated HUVECs. Additionally, the p-IKK/IKK ratio exhibited a significant reduction in comparison to HUVECs stimulated with TNF-α. We performed additional analysis to investigate the impact of FYD on NF-кB p65 nuclear translocation using immunofluorescence staining. The results revealed a significant dose-dependent inhibition of NF-кB p65 nuclear translocation in HUVECs by FYD (Figures 3G,H). These findings suggest that FYD exerts inhibitory effects on the NF-κB signaling pathway, leading to the degradation of NF-кB p65 through blockade of its nuclear translocation. Consequently, the downregulation of CAMs expression on HUVECs by FYD is closely associated with modulation of the NF-κB signaling pathway.
Figure 3. The expression of CAMs in HUVECs is downregulated by FYD through modulation of the NF-κB signaling pathway. (A–F) The HUVECs were pretreated with FYD at different concentrations (0, 0.5, 1.0, and 2.0 mg/mL) for a duration of 24 h followed by stimulation with TNF-α (10 ng/mL) for an additional 4 h. Western blot analysis was performed to assess the expression levels of IκBα, p-IκBα (Ser32), IKKα, IKKβ, p-IKKα/β (Ser176/180), NF-κB p65, and p-NF-κB p65 (Ser536). GAPDH was used as an internal control. Band intensity quantification was conducted using Image Lab software and expressed as a percentage relative to the control group treated with TNF-α alone. (G) The impact of FYD on the nuclear translocation of NF-κB p65 was assessed through immunofluorescence staining and visualized using a confocal microscope. NF-κB p65 was labeled with Alexa Fluor 594 (red), while the cell nucleus was labeled with DAPI (blue). (H) The nuclear/total fluorescence intensity ratio of NF-κB p65 (labeled with Alexa Fluor 594) was quantified using Fiji ImageJ software and expressed as a percentage of the control (treated with TNF-α alone). The data are presented as mean ± SD (n = 3–6); **indicate p < 0.01 compared to the Control (treated with TNF-α alone); ## indicate p < 0.01 compared to the Negative control (without TNF-α).
Another crucial step in the metastatic process involves the transmigration of CTCs across endothelial cells lining blood vessels, with cancer cell aggressiveness playing a pivotal role in this intricate mechanism (Wang et al., 2022). Here, we investigated the effect of FYD on the migratory and invasive behavior of CNE1 cells in vitro. The wound-healing assays revealed a concentration-dependent inhibition of CNE1 cell migration by FYD, with noticeable effects observed at concentrations of 3 mg/mL and 4 mg/mL after 48 h (Figures 4A–C). Furthermore, transwell assays demonstrated that FYD effectively suppressed CNE1 cell invasion in a concentration-dependent manner, with the group treated with 4 mg/mL showing only 6% invasion compared to the control group (Figures 4D,E). These results indicate that FYD exerts a significant inhibitory effect on the migration and invasion of CNE1 cells.
Figure 4. Repression of migration and invasion in CNE1 cells is observed upon FYD treatment. (A) The cell migration ability was assessed using a wound-healing assay in CNE1 cells treated with various concentrations of FYD for 24 and 48 h (B, C) The relative migration area was quantified using the Fiji ImageJ software and expressed as a percentage of control (FYD free). The regions of migration were delineated by yellow solid lines. (D, E) The cell invasion ability was assessed using the transwell assay in CNE1 cells treated with various concentrations of FYD for 24 h. The relative invasion rate was calculated as a percentage of the control group (without FYD treatment). The data are presented as mean ± SD (n = 3–6); **indicate p < 0.01 compared to the Control (without FYD treatment).
The EMT process is strongly associated with cancer cell migration and invasion (Cheng et al., 2018; Liu et al., 2022). Therefore, we performed western blotting analysis to determine the effect of FYD on expression of EMT-related proteins in CNE1 cells. The results are shown in Figures 5A–C, FYD produced concentration-dependent inhibition of ZEB1, N-cadherin, β-catenin and MMP2 proteins expression, compared with the control group. We also performed qRT-PCR analysis to investigate the mRNA levels of EMT transcription-related factors, and found that the transcription levels of transcription factors ZEB1, FN1, and SNAIL2 was significantly reduced by FYD (Supplementary Figure S5).
Figure 5. The effects of FYD on EMT, PI3K/AKT and FAK signaling pathways in CNE1 cells. Following a 24-h treatment with FYD, western blot analysis was performed to determine the expression levels of proteins associated with EMT, PI3K/AKT, and FAK pathways. GAPDH served as an internal control. Band intensity quantification was conducted using Image Lab software and expressed as a percentage relative to the control group (without FYD treatment). (A–C) Western blot analysis revealed that FYD effectively suppressed the expression of N-cadherin, β-catenin, ZEB1, and MMP2. (D–F) FYD dose-dependently inhibited both total and phosphorylation levels of PI3K and AKT proteins in CNE1 cells. (G,H) Dose-dependent treatment with FYD significantly attenuated the total and phosphorylation levels of FAK proteins in CNE cells. The data are presented as mean ± SD (n = 3); **indicate p < 0.01 compared to the Control (without FYD treatment).
The phosphatidylinositol-3-kinase (PI3K)/AKT signaling pathway participates in migration process of metastatic cancer cells, including the regulation of cytoskeleton-remodeling proteins and EMT–activating proteins that specifically regulate cell motility (Xue et al., 2012; Xue and Hemmings, 2013). We investigated the effect of FYD on the PI3K/AKT signaling pathway through western blot assay. The results demonstrated a significant concentration-dependent inhibition of p-PI3K (Tyr 458), AKT 1/2/3, and p-AKT 1/2/3 (AKT1-Tyr 315/AKT 2-Tyr 316/AKT 3-Tyr 312) expression by FYD, as compared to the control group (Figures 5D–F). Additionally, the p-PI3K/PI3K ratio exhibited a significant reduction. These findings suggest that FYD may inhibit the PI3K/AKT signaling pathway by inducing phosphorylation and total protein degradation. Moreover, the FAK signaling axis plays a pivotal role in facilitating cancer cell migration and invasion (Zhang et al., 2022). According to our findings, FYD exhibited a dose-dependent inhibition on the expression of FAK, p-FAK (Tyr 397), and p-PTK2 (Tyr 576/577) (Figures 5G,H). As a result of these findings, FYD suppresses the invasion and migration of cancer cell by regulating the EMT, PI3K/AKT, and FAK signaling cascades.
Due to the challenges in modeling nasopharyngeal carcinoma metastasis in immunocompetent syngeneic mice, the mechanisms of lung metastasis based on CTCs are similar for both breast cancer and nasopharyngeal cancer. In addition, in vitro CCK8 and transwell assays also showed that FYD could concentration-dependently inhibit the survival and invasion of mouse breast cancer 4T1 cells (Figures 6A–C), which was consistent with the results obtained in human nasopharyngeal carcinoma CNE1 cells (Figure 4D). Therefore, in this study, we utilized 4T1 breast cancer cells to examine the potential preventive effect of FYD on pulmonary metastasis in vivo. In this experiment, BALB/c mice were pretreated with FYD (0, 400, 800, and 1600 mg/kg) for a duration of 3 days, and then the mice received an intravenous injection of 4T1 cells via the tail vein. The doses administered to BALB/c mice were converted from clinical doses and in vitro 4T1 cell experiments (Bae et al., 2015; Nair et al., 2018). The administration of FYD continued to observe lung metastasis and survival rates. The results as shown in Figures 6D,E, revealed that FYD at doses of 800 mg/kg and 1600 mg/kg significantly reduced the formation of lung metastatic nodules induced by 4T1 cells. Histological hematoxylin and eosin (H&E) staining in Figure 6F demonstrated that the FYD-treated group had significantly fewer lung metastases compared to the control group. Moreover, FYD treatment extended the lifespan of mice with lung metastases, as depicted in Figure 6G. Immunohistochemical staining also showed that the expression of Ki67 was reduced in the lung metastasis tissue after FYD treatment, indicating that FYD treatment led to a weakened proliferation ability of 4T1 cells in lung tissue (Supplementary Figure S6). Furthermore, we further evaluated the effect of FYD for the PI3K/AKT and NF-κB signaling pathways in the lungs of mice through immunohistochemical staining. Interestingly, lung tumor metastases exhibited higher expression levels of PI3K, AKT and NF-κB p65 compared to healthy lung tissues; however, their expression significantly decreased following FYD treatment (Supplementary Figure S7). These findings suggest that FYD also effectively inhibits the PI3K/AKT and NF-κB signaling pathways in vivo. Importantly, no noticeable side effects were observed in the mice after 3 weeks of FYD treatment. The FYD treatment did not induce any significant changes in the body weight of the mice, while histological examination using H&E staining revealed that the FYD-treated mice exhibited normal morphological characteristics in their heart, liver, spleen, and kidney tissues (Supplementary Figure S8). The in vivo data revealed that FYD, even at weakly toxic doses, exhibits a remarkable inhibitory effect on the pulmonary metastasis.
Figure 6. In vivo impact of FYD on cancer pulmonary metastasis in immunocompetent BALB/c mice. (A) The cytotoxicity of FYD to 4T1 cells were determined by CCK8 assay, and relative survibility was expressed as percentage of control. (B) The cell invasion ability was assessed using the transwell assay in 4T1 cells treated with various concentrations of FYD for 24 h. (C) The relative invasion rate was calculated as a percentage of the control group (without FYD treatment). (D) The formation of lung cancer nodules was observed in vivo in mice. Prior to inoculation with 4T1 cells (5 × 104 cells/mouse, tail vein injection), the mice were pre-treated with FYD for 3 days at doses of 0, 400, 800, and 1600 mg/kg (100 μL volume per mouse, i.g., q.d.). The treatment was continued for a duration of 21 days. Representative images of the lungs displaying surface metastasis in mice are shown, with red arrows indicating the presence of breast cancer pulmonary nodules. (E) FYD significantly reduced the number of pulmonary metastatic nodules in a dose-dependent manner. (F) Representative images of lung sections stained with H&E from FYD-treated mice are shown. The black arrows indicate the area of breast cancer metastasis. (G) Evaluation of the survival period in mice treated with FYD. (H) The percentage of cytotoxic T lymphocytes (Tc cells, CD45+CD3+CD8+) and natural killer (NK) cells (CD45+CD49b+ cells) in peripheral blood of mice were assessed using flow cytometry. (I) Quantitative analysis revealed that FYD significantly elevated the proportions of Tc and NK cells in the peripheral blood, bringing them closer to the normal range. The data are presented as mean ± SD (n = 6); * indicate p < 0.05, ** indicate p < 0.01 compared to the Control (Pulmonary metastatic mice); # indicate p < 0.05, ## indicate p < 0.01 compared to the Normal (Healthy mice).
It has been proposed that enhancing the immune system’s function and boosting immunity can help prevent cancer metastasis in the body (Lian et al., 2019). To investigate the effect of FYD on cellular immune function, flow cytometry was employed to measure the proportion of cytotoxic T lymphocytes (Tc cells) and natural killer cells (NK cells) in the peripheral blood. As shown in Figures 6H,I, FYD treatment significantly augmented the proportion of Tc cells (CD45+CD3+CD8+ cells) and NK cells (CD45+CD49b+ cells) in the peripheral blood, approaching physiological levels. These findings suggest that FYD reduces cancer metastasis and strengthens the anti-cancer immune response by promoting the release of Tc and NK cells by the immune system.
The present studies confirmed for the first time that the FYD has a significant preventive effect on cancer metastasis due to its multifunctionality, and its effect is comprehensive and safe. At the molecular and cellular level, FYD inhibits CTCs adhesion to the vascular endothelial cells by down-regulating the expression of CAMs on the surface of endothelial cells induced by the inflammatory factor. Further mechanism studies showed that FYD reduced CAMs expression in endothelial cells by down-regulating the phosphorylation levels of IKK-α/β, IκB-α and NF-κB p65, as well as the nuclear translocation of NF-кB p65, thereby inhibiting the NF-κB signaling pathway activated by inflammatory factors. Furthermore, FYD inhibits cancer cells migration and invasion by inhibiting the EMT, PI3K/AKT, and FAK signal cascades. In addition, at the animal level, we also showed that FYD enhances anti-cancer immune responses by substantially increasing the subsets of Tc and NK cells in the peripheral immune system. A series of the beneficial effects of FYD collectively resulted in the failure of CTCs to adhere to the endothelium and transendothelial migration, prompting CTCs to continue to be exposed to the hostile bloodstream microenvironment and die under the attack of immune cells and the action of anoikis, thus effectively preventing cancer metastasis (Figure 7).
Figure 7. Mechanism of action of multifunctional FYD in preventing cancer metastasis. FYD interrupts adhesion of CTCs to vascular endothelium by suppressing CAMs through inhibition of the TNF-α-stimulated NF-кB signal pathways in endothelial cells. Additionally, FYD reduces motility of CTCs by regulating EMT, PI3K/AKT and FAK signaling pathways, thereby the inhibiting transendothelial migration (extravasation) of CTCs. Furthermore, FYD enhances anti-cancer immune responses by substantially increasing the subsets of Tc and NK cells in the peripheral immune system. The combined effects of FYD resulted in the continuous exposure of CTCs to the hostile microenvironment of the bloodstream, rendering them susceptible to death induced by anoikis, shear stress, and immune elimination.
Our previous studies have demonstrated that inflammatory factors (including IL-1β, TNF-α and TNF-β, et al.) induce the expression of CAMs (such as ICAM-1, VCAM-1, and E-selectin) on the surface of endothelial cells by activating the NF-κB signaling pathway, which is crucial for CTCs to adhere to the vascular endothelial cells of the distant metastatic tissues (the most important and first step of metastatic cascade) (Lu et al., 2014; Lian et al., 2016; Wang et al., 2022). It is clear now that NF-κB plays an essential role as a dimeric transcription factor in the expression of CAMs activated by inflammatory cytokines (Hoesel and Schmid, 2013). In the resting state, NF-κB binds to its repressor protein IκB in the cytoplasm, blocking the entry transcription of NF-κB target genes into the nucleus. However, in the presence of inflammatory factors, IKK complex (IKKβ and IKKα) is activated and causes the phosphorylation and degradation of IκB protein, allowing NF-κB to enter the nucleus to initiate transcription of target genes (Perkins, 2006; Hayden and Ghosh, 2008). Because of the presence of at least one κB binding site in the gene promoters of ICAM-1, VCAM-1, and E-selectin, activation of the NF-κB signaling pathway rapidly promotes their expression (Lian et al., 2016). In the current study, we found that FYD significant interrupts adhesion of CNE1 cells to HUVECs by suppressing CAMs via inhibition of the NF-κB signaling pathway in endothelial cells (Figure 2; Figure 3). These encouraging results may be due to the fact that FYD contains a variety of anti-inflammatory compounds, including Fraxetin (Deng et al., 2022), Scutellarin (Wang et al., 2019; Peng et al., 2020; Mei et al., 2022), Ganoderic acid A (Jia et al., 2021; Zheng et al., 2022), Succinic acid (Krajka-Kuzniak et al., 2019), Ursolic acid (Yuan et al., 2021), Coumaric acid (Yoon et al., 2014), Rosmarinic acid (Han et al., 2017; Jin et al., 2021), Salvianolic acid B (Wang et al., 2022; Zhao et al., 2023), Luteolin (Aziz et al., 2018; Li et al., 2019) (Supplementary Table S2), which not only inhibit the NF-κB signaling pathway, but also inhibit the levels of inflammatory factors (e.g., IL-1β, IL-6, TNF-α). Therefore, the synergistic effect of multiple compounds prompted FYD to show a more significant anti-inflammatory effect compared with single agents.
Transendothelial migration is another critical process for successful metastasis of CTCs adhering to vascular endothelial cells, which requires CTCs to acquire enhanced motility (Wang et al., 2022). The motility of CTCs is tightly regulated by multiple signaling pathways, including EMT, PI3K/AKT, and FAK signaling pathways, and their regulatory mechanisms are extremely complex. The EMT process is mediated by transcription factors such as SNAIL, ZEB and Twist, and enhances CTCs motility through activation of mesenchymal markers (e.g., N-cadherin and β-catenin) (Dongre and Weinberg, 2019). Activation of the PI3K/AKT signaling pathway promotes CTCs to undergo EMT and accelerates the malignant progression of cancer metastasis (Xue and Hemmings, 2013; Li et al., 2022). Furthermore, FAK also plays a critical role in cytoskeletal organization, focal adhesion formation, and cell motility (Lietha et al., 2007; Zhang et al., 2022). Therefore, the invasion ability of CTCs may be effectively inhibited through multi-target co-regulation, and Chinese herbal compounds have unique advantages in this respect. In this study, we found that FYD inhibits cancer cells migration and invasion by inhibiting the EMT, PI3K/AKT, and FAK signal cascades (Figure 4; Figure 5), due to the fact that FYD contains multiple active components (including Scutellarin (Li et al., 2019; Wang et al., 2019), Ganoderic Acid A (Cheng and Xie, 2019), Fraxetin (Ma et al., 2022), Butein (Rampogu et al., 2021), et al.) that can act on these signaling pathways simultaneously.
In the present study, we demonstrated that FYD effectively prevents metastasis of nasopharyngeal carcinoma at non-cytotoxic concentrations by inhibiting the adhesion of CTCs to endothelial cells and their subsequent transendothelial migration, as well as enhancing anti-cancer immune response (Figure 7). Mechanistically, FYD blocks adhesion of CTCs to vascular endothelium by suppressing TNF-α-induced expression of CAMs through regulation of the NF-κB signaling pathway in endothelial cells. Moreover, FYD inhibits CTCs invasion and migration by attenuating EMT, PI3K/AKT and FAK signaling cascades. Additionally, FYD enhances the anti-cancer immune response by significantly augmenting the population of Tc and NK cells in the peripheral immune system. Unlike the low effectiveness and harmful side effects associated with standard chemotherapy medication treatment, FYD can safely and efficiently inhibit cancer metastasis at low-risk doses through multi-target and multifunctional properties. This new finding provides a modern medical basis for the application of FYD in the prevention of cancer metastasis, suggesting that multi-drug and multi-target synergistic therapy may be one of the most effective ways to prevent cancer metastasis.
The original contributions presented in the study are publicly available. The data can be found here: https://pan.baidu.com/s/1VqV1p-GqE4XdAdTwECg9qw/ extraction code: 20nu.
The animal study was approved by the Institutional Animal Care and Use Committee (IACUC) of Minjiang University. The study was conducted in accordance with the local legislation and institutional requirements.
YY: Conceptualization, Funding acquisition, Writing–original draft, Data curation, Formal Analysis, Validation. ML: Data curation, Validation, Writing–original draft, Methodology, Visualization. GZ: Data curation, Methodology, Validation, Formal Analysis, Writing–review and editing. WW: Data curation, Methodology, Validation, Writing–review and editing. YY: Data curation, Methodology, Writing–review and editing. YS: Writing–review and editing, Validation. JQ: Validation, Writing–review and editing. YZ: Writing–review and editing, Investigation. CZ: Writing–review and editing, Funding acquisition, Methodology, Project administration, Supervision. XC: Supervision, Writing–review and editing, Investigation, Resources. MH: Resources, Writing–review and editing, Funding acquisition, Project administration. YL: Funding acquisition, Project administration, Writing–review and editing, Conceptualization, Methodology, Supervision, Visualization, Writing–original draft.
The author(s) declare financial support was received for the research, authorship, and/or publication of this article. This research was supported by the National Natural Science Foundation of China (NSFC, grant numbers 81703555, 81973437, and 82274080), the Department of Science and Technology of Fujian Province (grant numbers 2020J01859, 2021J011037, 2021D043, and 2022J01850), the Fujian Provincial Health Commission (grant numbers 2021QNA055), the Fujian Provincial Marine Economic Development Special Fund Project (FJHJF-L-2021-4), the Collaborative Innovation Platform Project of Fuxiaquan National Innovation Demonstration Zone (2021FX02), the Science and Technology Planning Projects of Fuzhou, China (grant number 2021S094), the Science and Technology Planning Projects of Fuzhou Institute of Oceanography, China (grant number 2021F08), the Young and Middle-aged Teacher Education Research Project of Fujian Province (grant number JAT210141).
We express our gratitude to Dr. Xiangqi Ye (from Xiang Qi Integrated Chinese and Western Medicine Clinic, Jiujiang, Jiangxi 332300, China) for his invaluable expertise and support in elucidating the dosing protocols and pharmacological mechanisms of Chinese herbal compounds.
The authors declare that the research was conducted in the absence of any commercial or financial relationships that could be construed as a potential conflict of interest.
All claims expressed in this article are solely those of the authors and do not necessarily represent those of their affiliated organizations, or those of the publisher, the editors and the reviewers. Any product that may be evaluated in this article, or claim that may be made by its manufacturer, is not guaranteed or endorsed by the publisher.
The Supplementary Material for this article can be found online at: https://www.frontiersin.org/articles/10.3389/fphar.2024.1355650/full#supplementary-material
FYD, Fuyuan Decoction; CTCs, Circulating tumor cells; NPC, Nasopharyngeal carcinoma; CAMs, Cell adhesion molecules; EMT, Epithelial-to-mesenchymal transition; TCM, Traditional Chinese medicine; PBS, Phosphate buffer saline; FBS, Fetal bovine serum; ECM, Endothelial cell culture medium; ECGS, endothelial cell growth supplement; CCK8, cell counting kit 8; PI, Propidium iodide; SDS-PAGE, sodium dodecyl sulfate polyacrylamide gel; H&E, Hematoxylin and eosin; NK, Natural killer.
Al-Rajhi, N., Soudy, H., Ahmed, S. A., Elhassan, T., Mohammed, S. F., Khoja, H. A., et al. (2020). CD3+T-lymphocyte infiltration is an independent prognostic factor for advanced nasopharyngeal carcinoma. BMC Cancer 20, 240. Epub 2020/03/23. doi:10.1186/s12885-020-06757-w
Aziz, N., Kim, M. Y., and Cho, J. Y. (2018). Anti-inflammatory effects of luteolin: a review of in vitro, in vivo, and in silico studies. J. Ethnopharmacol. 225, 342–358. Epub 2018/05/29. doi:10.1016/j.jep.2018.05.019
Bae, J. W., Kim, D. H., Lee, W. W., Kim, H. Y., and Son, C. G. (2015). Characterizing the human equivalent dose of herbal medicines in animal toxicity studies. J. Ethnopharmacol. 162, 1–6. Epub 2014/12/30. doi:10.1016/j.jep.2014.12.023
Cheng, Y., Lu, Y., Zhang, D., Lian, S., Liang, H., Ye, Y., et al. (2018). Metastatic cancer cells compensate for low energy supplies in hostile microenvironments with bioenergetic adaptation and metabolic reprogramming. Int. J. Oncol. 53, 2590–2604. Epub 2018/10/04. doi:10.3892/ijo.2018.4582
Cheng, Y., and Xie, P. (2019). Ganoderic acid A holds promising cytotoxicity on human glioblastoma mediated by incurring apoptosis and autophagy and inactivating PI3K/AKT signaling pathway. J. Biochem. Mol. Toxicol. 33, e22392. Epub 2019/09/11. doi:10.1002/jbt.22392
Chua, M. L. K., Wee, J. T. S., Hui, E. P., and Chan, A. T. C. (2016). Nasopharyngeal carcinoma. Lancet 387, 1012–1024. Epub 2015/09/01. doi:10.1016/S0140-6736(15)00055-0
Deng, S. J., Ge, J. W., Xia, S. N., Zou, X. X., Bao, X. Y., Gu, Y., et al. (2022). Fraxetin alleviates microglia-mediated neuroinflammation after ischemic stroke. Ann. Transl. Med. 10, 439. Epub 2022/05/17. doi:10.21037/atm-21-4636
Dongre, A., and Weinberg, R. A. (2019). New insights into the mechanisms of epithelial-mesenchymal transition and implications for cancer. Nat. Rev. Mol. Cell Biol. 20, 69–84. Epub 2018/11/22. doi:10.1038/s41580-018-0080-4
Ferlay, J., Colombet, M., Soerjomataram, I., Mathers, C., Parkin, D. M., Pineros, M., et al. (2019). Estimating the global cancer incidence and mortality in 2018: GLOBOCAN sources and methods. Int. J. Cancer 144 (144), 1941–1953. Epub 2018/10/24. doi:10.1002/ijc.31937
Gay, L. J., and Felding-Habermann, B. (2011). Contribution of platelets to tumour metastasis. Nat. Rev. Cancer 11, 123–134. Epub 2011/01/25. doi:10.1038/nrc3004
Han, J., Wang, D., Ye, L., Li, P., Hao, W., Chen, X., et al. (2017). Rosmarinic acid protects against inflammation and cardiomyocyte apoptosis during myocardial ischemia/reperfusion injury by activating peroxisome proliferator-activated receptor gamma. Front. Pharmacol. 8, 456. Epub 2017/07/27. doi:10.3389/fphar.2017.00456
Han, X., Zhang, X., Wang, Q., Wang, L., and Yu, S. (2020). Antitumor potential of Hedyotis diffusa Willd: a systematic review of bioactive constituents and underlying molecular mechanisms. Biomed. Pharmacother. 130, 110735. Epub 2021/07/30. doi:10.1016/j.biopha.2020.110735
Hayden, M. S., and Ghosh, S. (2008). Shared principles in NF-kappaB signaling. Cell 132, 344–362. Epub 2008/02/13. doi:10.1016/j.cell.2008.01.020
Hoesel, B., and Schmid, J. A. (2013). The complexity of NF-κB signaling in inflammation and cancer. Mol. Cancer 12, 86. Epub 2013/08/07. doi:10.1186/1476-4598-12-86
Jayakumar, T., Chang, C. C., Lin, S. L., Huang, Y. K., Hu, C. M., Elizebeth, A. R., et al. (2014). Brazilin ameliorates high glucose-induced vascular inflammation via inhibiting ROS and CAMs production in human umbilical vein endothelial cells. Biomed. Res. Int. 2014, 403703. Epub 2014/04/10. doi:10.1155/2014/403703
Jia, Y., Zhang, D., Li, H., Luo, S., Xiao, Y., Han, L., et al. (2021). Activation of FXR by ganoderic acid A promotes remyelination in multiple sclerosis via anti-inflammation and regeneration mechanism. Biochem. Pharmacol. 185, 114422. Epub 2021/01/23. doi:10.1016/j.bcp.2021.114422
Jiang, Y. L., and Liu, Z. P. (2011). Natural products as anti-invasive and anti-metastatic agents. Curr. Med. Chem. 18, 808–829. Epub 2010/12/25. doi:10.2174/092986711794927711
Jin, B. R., Chung, K. S., Hwang, S., Hwang, S. N., Rhee, K. J., Lee, M., et al. (2021). Rosmarinic acid represses colitis-associated colon cancer: a pivotal involvement of the TLR4-mediated NF-κB-STAT3 axis. Neoplasia 23, 561–573. Epub 2021/06/03. doi:10.1016/j.neo.2021.05.002
Krajka-Kuzniak, V., Bednarczyk-Cwynar, B., Narozna, M., Szaefer, H., and Baer-Dubowska, W. (2019). Morpholide derivative of the novel oleanolic oxime and succinic acid conjugate diminish the expression and activity of NF-κB and STATs in human hepatocellular carcinoma cells. Chem. Biol. Interact. 311, 108786. Epub 2019/08/12. doi:10.1016/j.cbi.2019.108786
Law, C. K., Kwok, H. H., Poon, P. Y., Lau, C. C., Jiang, Z. H., Tai, W. C., et al. (2014). Ginsenoside compound K induces apoptosis in nasopharyngeal carcinoma cells via activation of apoptosis-inducing factor. Chin. Med. 9, 11. Epub 2014/04/03. doi:10.1186/1749-8546-9-11
Leblanc, R., and Peyruchaud, O. (2016). Metastasis: new functional implications of platelets and megakaryocytes. Blood 128, 24–31. Epub 2016/05/08. doi:10.1182/blood-2016-01-636399
Li, C. Y., Wang, Q., Wang, X., Li, G., Shen, S., and Wei, X. (2019). Scutellarin inhibits the invasive potential of malignant melanoma cells through the suppression epithelial-mesenchymal transition and angiogenesis via the PI3K/Akt/mTOR signaling pathway. Eur. J. Pharmacol. 858, 172463. Epub 2019/06/19. doi:10.1016/j.ejphar.2019.172463
Li, L., Luo, W., Qian, Y., Zhu, W., Qian, J., Li, J., et al. (2019). Luteolin protects against diabetic cardiomyopathy by inhibiting NF-κB-mediated inflammation and activating the Nrf2-mediated antioxidant responses. Phytomedicine. 59, 152774. Epub 2019/04/23. doi:10.1016/j.phymed.2018.11.034
Li, Y., Yin, Y., He, Y., He, K., and Li, J. (2022). SOS1 regulates HCC cell epithelial-mesenchymal transition via the PI3K/AKT/mTOR pathway. Biochem. Biophys. Res. Commun. 637, 161–169. Epub 2022/11/21. doi:10.1016/j.bbrc.2022.11.015
Lian, S., Lu, Y., Cheng, Y., Yu, T., Xie, X., Liang, H., et al. (2016). S-nitrosocaptopril interrupts adhesion of cancer cells to vascular endothelium by suppressing cell adhesion molecules via inhibition of the NF-кB and JAK/STAT signal pathways in endothelial cells. Eur. J. Pharmacol. 791, 62–71. Epub 2016/10/30. doi:10.1016/j.ejphar.2016.08.018
Lian, S., Xie, R., Ye, Y., Xie, X., Li, S., Lu, Y., et al. (2019). Simultaneous blocking of CD47 and PD-L1 increases innate and adaptive cancer immune responses and cytokine release. EBioMedicine 42, 281–295. Epub 2019/03/18. doi:10.1016/j.ebiom.2019.03.018
Lietha, D., Cai, X., Ceccarelli, D. F., Li, Y., Schaller, M. D., and Eck, M. J. (2007). Structural basis for the autoinhibition of focal adhesion kinase. Cell 129, 1177–1187. Epub 2007/06/19. doi:10.1016/j.cell.2007.05.041
Liu, W., Chen, G., Zhang, C., Liao, X., Xie, J., Liang, T., et al. (2022). Prognostic significance of tumor-infiltrating lymphocytes and macrophages in nasopharyngeal carcinoma: a systematic review and meta-analysis. Eur. Arch. Otorhinolaryngol. 279, 25–35. Epub 2021/05/25. doi:10.1007/s00405-021-06879-2
Liu, W., Xin, M., Li, Q., Sun, L., Han, X., and Wang, J. (2022). IL-17A promotes the migration, invasion and the EMT process of lung cancer accompanied by NLRP3 activation. Biomed. Res. Int. 2022, 7841279. Epub 2022/11/10. doi:10.1155/2022/7841279
Liu, Y., Zhang, Y., Ding, Y., and Zhuang, R. (2021). Platelet-mediated tumor metastasis mechanism and the role of cell adhesion molecules. Crit. Rev. Oncol. Hematol. 167, 103502. Epub 2021/10/19. doi:10.1016/j.critrevonc.2021.103502
Lu, Y., Lian, S., Ye, Y., Yu, T., Liang, H., Cheng, Y., et al. (2019). S-Nitrosocaptopril prevents cancer metastasis in vivo by creating the hostile bloodstream microenvironment against circulating tumor cells. Pharmacol. Res. 139, 535–549. Epub 2018/10/27. doi:10.1016/j.phrs.2018.10.020
Lu, Y., Yu, T., Liang, H., Wang, J., Xie, J., Shao, J., et al. (2014). Nitric oxide inhibits hetero-adhesion of cancer cells to endothelial cells: restraining circulating tumor cells from initiating metastatic cascade. Sci. Rep. 11 (4), 4344. Epub 2014/03/13. doi:10.1038/srep04344
Ma, Z., Sun, Y., and Peng, W. (2022). Fraxetin down-regulates polo-like kinase 4 (PLK4) to inhibit proliferation, migration and invasion of prostate cancer cells through the phosphatidylinositol 3-kinase (PI3K)/protein kinase B (Akt) pathway. Bioengineered 13, 9345–9356. Epub 2022/04/08. doi:10.1080/21655979.2022.2054195
Mei, X. Y., Zhang, J. N., Jia, W. Y., Lu, B., Wang, M. N., Zhang, T. Y., et al. (2022). Scutellarin suppresses triple-negative breast cancer metastasis by inhibiting TNFα-induced vascular endothelial barrier breakdown. Acta Pharmacol. Sin. 43, 2666–2677. Epub 2022/03/02. doi:10.1038/s41401-022-00873-y
Nair, A., Morsy, M. A., and Jacob, S. (2018). Dose translation between laboratory animals and human in preclinical and clinical phases of drug development. Drug Dev. Res. 79, 373–382. Epub 2018/10/22. doi:10.1002/ddr.21461
Paul, C. D., Mistriotis, P., and Konstantopoulos, K. (2017). Cancer cell motility: lessons from migration in confined spaces. Nat. Rev. Cancer 17, 131–140. Epub 2016/12/03. doi:10.1038/nrc.2016.123
Peng, L., Wen, L., Shi, Q. F., Gao, F., Huang, B., Meng, J., et al. (2020). Scutellarin ameliorates pulmonary fibrosis through inhibiting NF-κB/NLRP3-mediated epithelial-mesenchymal transition and inflammation. Cell Death Dis. 11, 978. Epub 2020/11/15. doi:10.1038/s41419-020-03178-2
Perkins, N. D. (2006). Post-translational modifications regulating the activity and function of the nuclear factor kappa B pathway. Oncogene 25, 6717–6730. Epub 2006/10/31. doi:10.1038/sj.onc.1209937
Rampogu, S., Kim, S. M., Shaik, B., Lee, G., Kim, J. H., Kim, G. S., et al. (2021). Novel Butein derivatives repress DDX3 expression by inhibiting PI3K/AKT signaling pathway in MCF-7 and MDA-MB-231 cell lines. Front. Oncol. 11, 712824. Epub 2021/09/07. doi:10.3389/fonc.2021.712824
Sarwar, M. S., Zhang, H. J., and Tsang, S. W. (2018). Perspectives of plant natural products in inhibition of cancer invasion and metastasis by regulating multiple signaling pathways. Curr. Med. Chem. 25, 5057–5087. Epub 2017/09/20. doi:10.2174/0929867324666170918123413
Stott, S. L., Lee, R. J., Nagrath, S., Yu, M., Miyamoto, D. T., Ulkus, L., et al. (2010). Isolation and characterization of circulating tumor cells from patients with localized and metastatic prostate cancer. Sci. Transl. Med. 2, 25ra23. 25ra23. Epub 2010/04/29. doi:10.1126/scitranslmed.3000403
Sung, H., Ferlay, J., Siegel, R. L., Laversanne, M., Soerjomataram, I., Jemal, A., et al. (2021). Global cancer statistics 2020: GLOBOCAN estimates of incidence and mortality worldwide for 36 cancers in 185 countries. CA Cancer J. Clin. 71, 209–249. Epub 2021/02/05. doi:10.3322/caac.21660
Wang, C. Y., Wang, T. C., Liang, W. M., Hung, C. H., Chiou, J. S., Chen, C. J., et al. (2020). Effect of Chinese herbal medicine therapy on overall and cancer related mortality in patients with advanced nasopharyngeal carcinoma in taiwan. Front. Pharmacol. 11, 607413. Epub 2021/03/13. doi:10.3389/fphar.2020.607413
Wang, D., Li, Q., Liu, R., Xu, H., Yin, Y., Wang, Y., et al. (2019). Quality control of Semen Ziziphi Spinosae standard decoction based on determination of multi-components using TOF-MS/MS and UPLC-PDA technology. J. Pharm. Anal. 9, 406–413. Epub 2020/01/01. doi:10.1016/j.jpha.2019.01.001
Wang, D., Wu, C., Liu, D., Zhang, L., Long, G., Hu, G., et al. (2019). Ginsenoside Rg3 inhibits migration and invasion of nasopharyngeal carcinoma cells and suppresses epithelial mesenchymal transition. Biomed. Res. Int. 2019, 8407683. Epub 2019/03/28. doi:10.1155/2019/8407683
Wang, R., Li, S., Chen, P., Yue, X., Wang, S., Gu, Y., et al. (2022). Salvianolic acid B suppresses hepatic stellate cell activation and liver fibrosis by inhibiting the NF-κB signaling pathway via miR-6499-3p/LncRNA-ROR. Phytomedicine 107, 154435. Epub 2022/09/27. doi:10.1016/j.phymed.2022.154435
Wang, W., Fu, C., Lin, M., Lu, Y., Lian, S., Xie, X., et al. (2022). Fucoxanthin prevents breast cancer metastasis by interrupting circulating tumor cells adhesion and transendothelial migration. Front. Pharmacol. 13, 960375. Epub 2022/09/27. doi:10.3389/fphar.2022.960375
Wang, W., Li, J., Li, F., Peng, J., Xu, M., Shangguan, Y., et al. (2019). Scutellarin suppresses cartilage destruction in osteoarthritis mouse model by inhibiting the NF-κB and PI3K/AKT signaling pathways. Int. Immunopharmacol. 77, 105928. Epub 2019/11/13. doi:10.1016/j.intimp.2019.105928
Wang, Y., Zhang, Q., Chen, Y., Liang, C. L., Liu, H., Qiu, F., et al. (2020). Antitumor effects of immunity-enhancing traditional Chinese medicine. Biomed. Pharmacother. 121, 109570. Epub 2019/11/12. doi:10.1016/j.biopha.2019.109570
Xiang, Y., Guo, Z., Zhu, P., Chen, J., and Huang, Y. (2019). Traditional Chinese medicine as a cancer treatment: modern perspectives of ancient but advanced science. Cancer Med. 8, 1958–1975. Epub 2019/04/05. doi:10.1002/cam4.2108
Xiangqi Ye, Y. L., and Ye, Y. (2021). The present invention discloses a traditional Chinese medicine composition for the treatment of tumors, as well as the prevention of tumor recurrence and metastasis, along with a method for its preparation. Pat. Invent. China.
Xie, J., Shao, J., Lu, Y., Chen, J., Wang, J., Yu, S., et al. (2013). Separation of ginseng active ingredients and their roles in cancer metastasis supplementary therapy. Curr. Drug Metab. 14, 616–623. Epub 2013/05/25. doi:10.2174/13892002113149990003
Xue, G., and Hemmings, B. A. (2013). PKB/Akt-dependent regulation of cell motility. J. Natl. Cancer Inst. 105, 393–404. Epub 2013/01/29. doi:10.1093/jnci/djs648
Xue, G., Restuccia, D. F., Lan, Q., Hynx, D., Dirnhofer, S., Hess, D., et al. (2012). Akt/PKB-mediated phosphorylation of Twist1 promotes tumor metastasis via mediating cross-talk between PI3K/Akt and TGF-β signaling axes. Cancer Discov. 2, 248–259. Epub 2012/05/16. doi:10.1158/2159-8290.CD-11-0270
Yang, J., Chen, J., Liang, H., and Yu, Y. (2022). Nasopharyngeal cancer cell-derived exosomal PD-L1 inhibits CD8+ T-cell activity and promotes immune escape. Cancer Sci. 113, 3044–3054. Epub 2022/05/23. doi:10.1111/cas.15433
Yang, Y. L., Lin, Z. W., He, P. T., Nie, H., Yao, Q. Y., and Zhang, S. Y. (2021). Inhibitory effect of Astragalus polysaccharide combined with cisplatin on cell cycle and migration of nasopharyngeal carcinoma cell lines. Biol. Pharm. Bull. 44, 926–931. Epub 2021/05/07. doi:10.1248/bpb.b20-00959
Yang, Z., Zhang, Q., Yu, L., Zhu, J., Cao, Y., and Gao, X. (2021). The signaling pathways and targets of traditional Chinese medicine and natural medicine in triple-negative breast cancer. J. Ethnopharmacol. 10 (264), 113249. Epub 2020/08/19. doi:10.1016/j.jep.2020.113249
Yoon, J. H., Youn, K., Ho, C. T., Karwe, M. V., Jeong, W. S., and Jun, M. (2014). p-Coumaric acid and ursolic acid from Corni fructus attenuated β-amyloid(25-35)-induced toxicity through regulation of the NF-κB signaling pathway in PC12 cells. J. Agric. Food Chem. 62, 4911–4916. Epub 2014/05/13. doi:10.1021/jf501314g
Yu, H., Yin, X., Mao, Y., Chen, M., Tang, Q., and Yan, S. (2022). The global burden of nasopharyngeal carcinoma from 2009 to 2019: an observational study based on the Global Burden of Disease Study 2019. Eur. Arch. Otorhinolaryngol. 279, 1519–1533. Epub 2021/06/20. doi:10.1007/s00405-021-06922-2
Yuan, L., Zhang, L., Yao, N., Wu, L., Liu, J., Liu, F., et al. (2021). Upregulation of UGT1A1 expression by ursolic acid and oleanolic acid via the inhibition of the PKC/NF-κB signaling pathway. Phytomedicine. 92, 153726. Epub 2021/09/19. doi:10.1016/j.phymed.2021.153726
Yuan, R., Hou, Y., Sun, W., Yu, J., Liu, X., Niu, Y., et al. (2017). Natural products to prevent drug resistance in cancer chemotherapy: a review. Ann. N. Y. Acad. Sci. 1401, 19–27. Epub 2017/09/12. doi:10.1111/nyas.13387
Zeng, W., Sun, Z., Ma, T., Song, X., Li, S., Zhang, Q., et al. (2021). Elevated ZIPK is required for TNF-α-induced cell adhesion molecule expression and leucocyte adhesion in endothelial cells. Acta Biochim. Biophys. Sin. (Shanghai) 53, 567–574. Epub 2021/03/13. doi:10.1093/abbs/gmab019
Zhang, T., Chen, Z., Deng, J., Xu, K., Che, D., Lin, J., et al. (2022). Epstein-Barr virus-encoded microRNA BART22 serves as novel biomarkers and drives malignant transformation of nasopharyngeal carcinoma. Cell Death Dis. 13, 664. Epub 2022/07/31. doi:10.1038/s41419-022-05107-x
Zhang, Y. M., Gong, G. Z., Qiu, Q. T., Han, Y. W., Lu, H. M., and Yin, Y. (2021). Radiomics for diagnosis and radiotherapy of nasopharyngeal carcinoma. Front. Oncol. 11, 767134. Epub 2022/01/25. doi:10.3389/fonc.2021.767134
Zhang, Z., Li, J., Jiao, S., Han, G., Zhu, J., and Liu, T. (2022). Functional and clinical characteristics of focal adhesion kinases in cancer progression. Front. Cell Dev. Biol. 10, 1040311. Epub 2022/11/22. doi:10.3389/fcell.2022.1040311
Zhao, Y., Shao, C., Zhou, H., Yu, L., Bao, Y., Mao, Q., et al. (2023). Salvianolic acid B inhibits atherosclerosis and TNF-α-induced inflammation by regulating NF-κB/NLRP3 signaling pathway. Phytomedicine. 119, 155002. Epub 2023/08/13. doi:10.1016/j.phymed.2023.155002
Zheng, D. S., and Chen, L. S. (2017). Triterpenoids from Ganoderma lucidum inhibit the activation of EBV antigens as telomerase inhibitors. Exp. Ther. Med. 14, 3273–3278. Epub 2017/09/16. doi:10.3892/etm.2017.4883
Zheng, S., Ma, J., Zhao, X., Yu, X., and Ma, Y. (2022). Ganoderic acid A attenuates IL-1β-induced inflammation in human nucleus pulposus cells through inhibiting the NF-κB pathway. Inflammation 45, 851–862. Epub 2021/11/06. doi:10.1007/s10753-021-01590-0
Zhong, C., Li, Y., Li, W., Lian, S., Li, Y., Wu, C., et al. (2023). Ganoderma lucidum extract promotes tumor cell pyroptosis and inhibits metastasis in breast cancer. Food Chem. Toxicol. 174, 113654. Epub 2023/02/10. doi:10.1016/j.fct.2023.113654
Zhong, Q., Liu, Z. H., Lin, Z. R., Hu, Z. D., Yuan, L., Liu, Y. M., et al. (2018). The RARS-mad1l1 fusion gene induces cancer stem cell-like properties and therapeutic resistance in nasopharyngeal carcinoma. Clin. Cancer Res. 24, 659–673. Epub 2017/11/15. doi:10.1158/1078-0432.CCR-17-0352
Keywords: traditional Chinese medicine, Fuyuan decoction, cancer metastasis prevention, cell adhesion, transendothelial migration, anti-cancer immune response
Citation: Ye Y, Lin M, Zhou G, Wang W, Yao Y, Su Y, Qi J, Zheng Y, Zhong C, Chen X, Huang M and Lu Y (2024) Fuyuan decoction prevents nasopharyngeal carcinoma metastasis by inhibiting circulating tumor cells/ endothelial cells interplay and enhancing anti-cancer immune response. Front. Pharmacol. 15:1355650. doi: 10.3389/fphar.2024.1355650
Received: 14 December 2023; Accepted: 09 April 2024;
Published: 25 April 2024.
Edited by:
Huifeng Hao, Peking University, ChinaReviewed by:
Sutapa Mukherjee, Chittaranjan National Cancer Institute (CNCI), IndiaCopyright © 2024 Ye, Lin, Zhou, Wang, Yao, Su, Qi, Zheng, Zhong, Chen, Huang and Lu. This is an open-access article distributed under the terms of the Creative Commons Attribution License (CC BY). The use, distribution or reproduction in other forums is permitted, provided the original author(s) and the copyright owner(s) are credited and that the original publication in this journal is cited, in accordance with accepted academic practice. No use, distribution or reproduction is permitted which does not comply with these terms.
*Correspondence: Chunlian Zhong, emhvbmdjaHVubGlhbjAxMTdAMTI2LmNvbQ==; Xi Chen, MjkyMzk2MjE4QHFxLmNvbQ==; Mingqing Huang, aG1xMTExNUAxMjYuY29t; Yusheng Lu, bHVfeXVfc2hlbmdAMTI2LmNvbQ==
†These authors have contributed equally to this work
Disclaimer: All claims expressed in this article are solely those of the authors and do not necessarily represent those of their affiliated organizations, or those of the publisher, the editors and the reviewers. Any product that may be evaluated in this article or claim that may be made by its manufacturer is not guaranteed or endorsed by the publisher.
Research integrity at Frontiers
Learn more about the work of our research integrity team to safeguard the quality of each article we publish.