- 1Department of Clinical Oncology, Maria Skłodowska-Curie National Research Institute of Oncology, Kraków, Poland
- 2Wrocław University of Science and Technology, Faculty of Chemistry, Department of Advanced Material Technologies, Wrocław, Poland
Breast cancer (BC) is a heterogenous disease, with prognosis and treatment options depending on Estrogen, Progesterone receptor, and Human Epidermal Growth Factor Receptor-2 (HER-2) status. HER-2 negative, endocrine-independent BC presents a significant clinical challenge with limited treatment options. To date, promising strategies like immune checkpoint inhibitors have not yielded breakthroughs in patient prognosis. Despite being considered archaic, agents derived from natural sources, mainly plants, remain backbone of current treatment. In this context, we critically analyze novel naturally-derived drug candidates, elucidate their intricate mechanisms of action, and evaluate their pre-clinical in vitro and in vivo activity in endocrine-independent HER-2 negative BC. Since pre-clinical research success often does not directly correlate with drug approval, we focus on ongoing clinical trials to uncover current trends. Finally, we demonstrate the potential of combining cutting-edge technologies, such as antibody-drug conjugates or nanomedicine, with naturally-derived agents, offering new opportunities that utilize both traditional cytotoxic agents and new metabolites.
1 Introduction
Breast cancer (BC) in females has emerged as the most frequently diagnosed cancer, comprising almost 12% of all new cases. Among women alone, BC resulted in nearly 650,000 deaths globally in 2020 (Caswell-Jin et al., 2018; Sung et al., 2021). BC subtypes differ in terms of prognosis and treatment strategies. Depending on the expression of the estrogen receptor (ER), progesterone receptor (PR), Ki67 antigen, and Human epidermal growth factor receptor-2 (HER-2), BC can be divided into Luminal A&B (70% for HER-2 negative cases), HER-2 positive (around 15% including ER/PR + HER-2+ BC), and triple negative (TNBC) (10%–15%). Around 30% of BC patients experience metastatic disease during follow up (20%–30%) or de novo (5%–10%). BC mortality rates vary by subtype, with TNBC at around 30%, luminal B at 20%–25%, HER-2-positive at 20%, and luminal A at 10%–15% (Hennigs et al., 2016; André et al., 2019; Gnant et al., 2022; Wynn and Tang, 2022; Hassan and Ates-Alagoz, 2023). Patients with TNBC have the shortest survival. In contrary, HER-2 negative Luminal BC carries a good prognosis. Patients with Luminal BC, who have developed resistance to hormonal agents face an unfavorable situation and the course of such endocrine-resistant BC is more like TNBC (André et al., 2019; Hassan and Ates-Alagoz, 2023). This cluster of endocrine-independent HER-2 negative BCs represents an unresolved clinical challenge, characterized by a dearth of treatment alternatives based on chemotherapy agents (Cardoso et al., 2020; Cortes et al., 2020; Modi et al., 2020; Bardia et al., 2021; Corti et al., 2021; Michalak and Püsküllüoğlu, 2022; Püsküllüoğlu and Michalak, 2022; Siddiqui et al., 2022). Majority of currently used drugs serving as a backbone to the most effective approved options are naturally derived (Table 1, based on product characteristics, accessed on 5th September 2023) (Cortes et al., 2020; Modi et al., 2020; Pullaiah and Raveendran, 2020; Rugo et al., 2020; Bardia et al., 2021; Corti et al., 2021; Ibrahim, 2021; Michalak and Püsküllüoğlu, 2022; Püsküllüoğlu and Michalak, 2022; Sekar et al., 2022; Siddiqui et al., 2022; Aloss and Hamar, 2023; Dogra and Kumar, 2023; Kciuk et al., 2023).
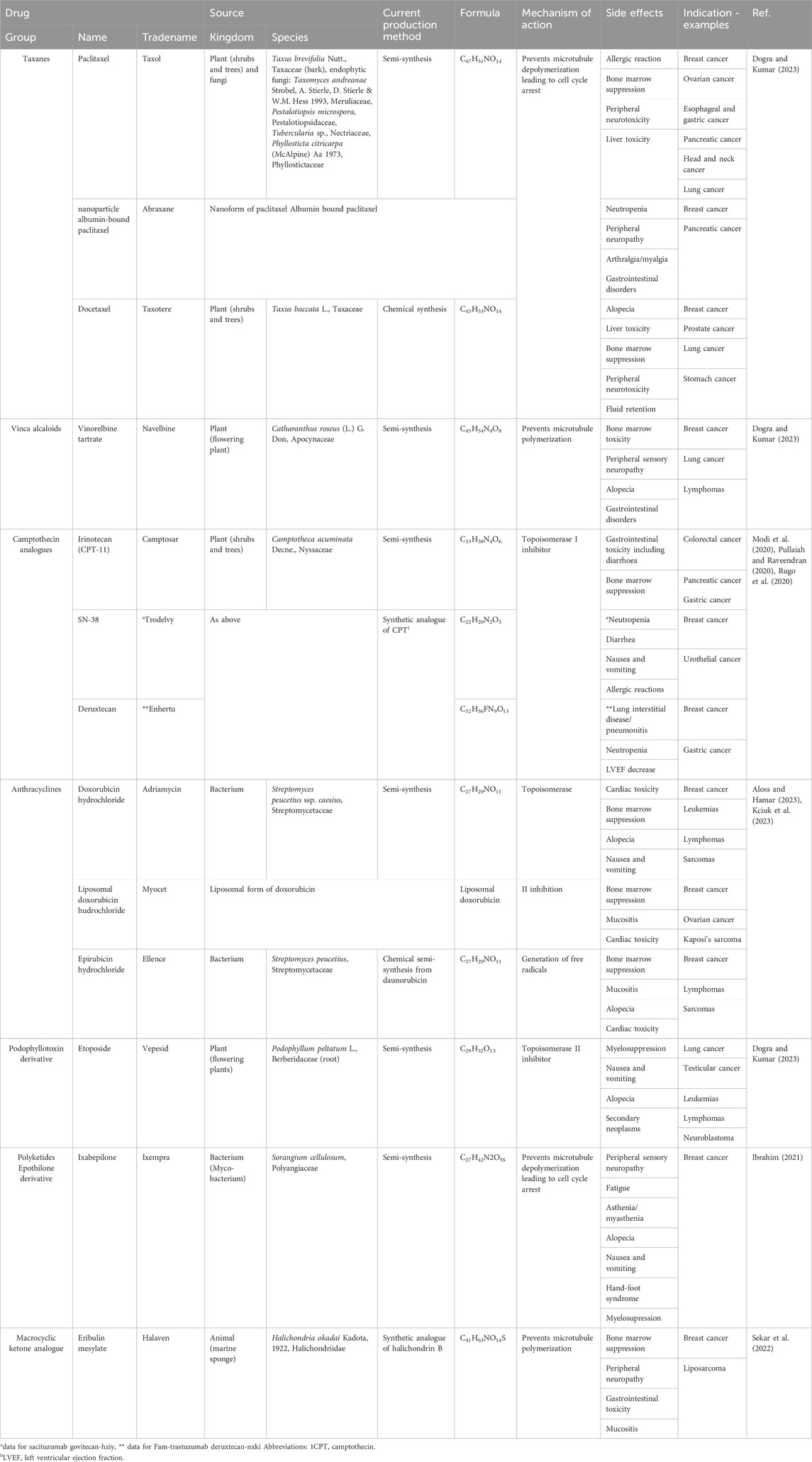
TABLE 1. Drugs derived from natural resources used in the treatment of TNBC and hormonal-resistant HER-2 negative BC.
Medications such as check point inhibitors or targeted drugs have not yielded the anticipated breakthrough in therapy, while newly registered antibody-drug conjugates (ADC) still rely on chemotherapy agents derived from living organisms (Cortes et al., 2020; Modi et al., 2020; Pullaiah and Raveendran, 2020; Rugo et al., 2020; Bardia et al., 2021; Corti et al., 2021; Ibrahim, 2021; Michalak and Püsküllüoğlu, 2022; Püsküllüoğlu and Michalak, 2022; Sekar et al., 2022; Siddiqui et al., 2022; Aloss and Hamar, 2023; Dogra and Kumar, 2023; Kciuk et al., 2023).
In the recent years, there has been a significant advancement in the development of novel natural-derived drug candidates aimed at tackling the unmet challenges in treating TNBC and HER-2 negative, endocrine-resistant BC. The pathway from metabolite identification to drug registration (Figure 1) is a multistep and long process. Majority of metabolites showing potential in pre-clinical setting will fail to meet endpoints in clinical trials. In this review we aim to show that the recent development in this field can be attributed to each step of this translational process: from bench to bedside (Berdigaliyev and Aljofan, 2020; Choudhari et al., 2020; Kaushik et al., 2021). We present natural resources that can be a source of biologically active metabolites and the significant pre-clinical activities exhibited by these metabolites, offering insights into their intricate mechanisms suggested in recently published studies. Moreover, we identify trends in ongoing clinical trials that incorporate naturally-derived drugs. We also shed light on the potential role of cutting-edge technologies like antibody-drug conjugates or nanomedicine in diminishing the toxicity and improving efficacy of old and newly discovered naturally-derived agents making predictions about future directions.
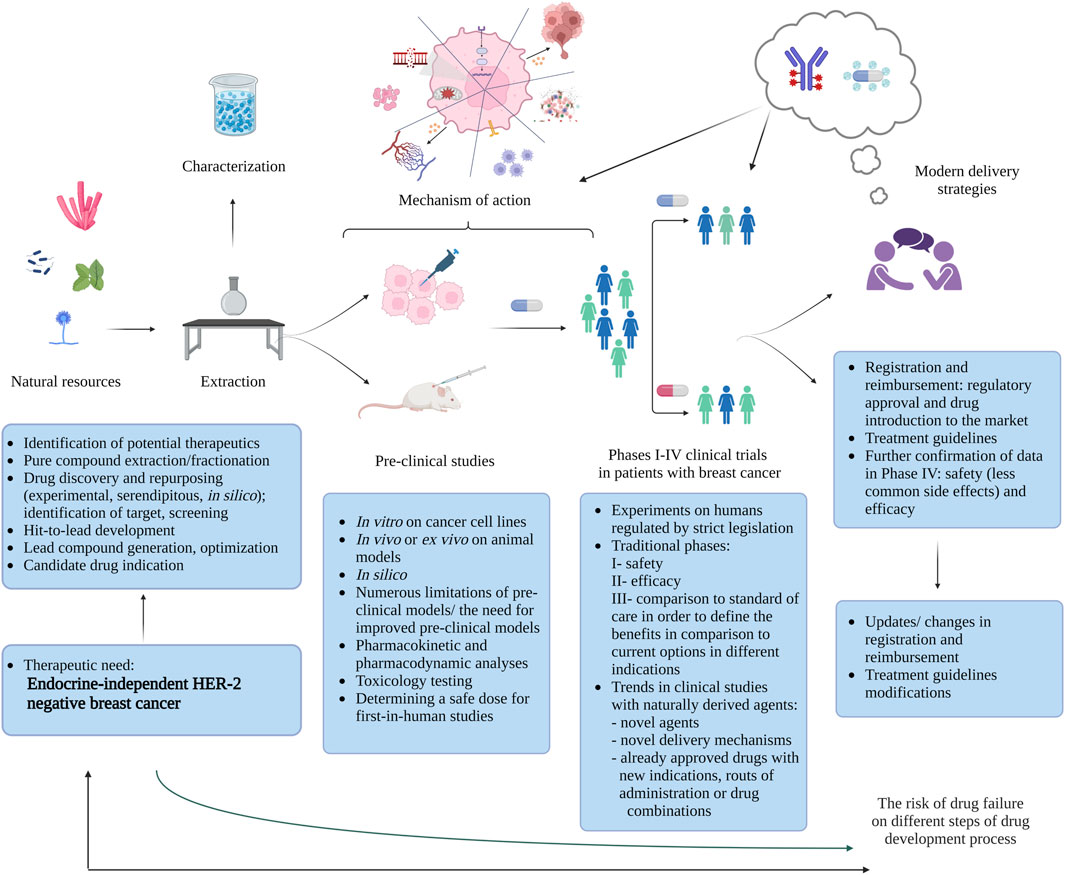
FIGURE 1. Bench-to-bedside journey: natural metabolites for endocrine-independent HER-2 negative breast cancer treatment (Berdigaliyev and Aljofan, 2020; Choudhari et al., 2020; Kaushik et al., 2021) (created with BioRender).
2 The current paradigms in the treatment
2.1 General treatment guidelines
Systemic treatment in TNBC radical setting is recommended for tumors larger than 5 mm or node-positive disease. Preferred regimens involve anthracycline-based and taxane-based therapies, capecitabine and, in some cases, novel agents in the (neo)adjuvant setting (NCCN Clinical Practice Guidelines in Oncology, 2023; Triple-negative Breast Cancer, 2023).
2.2 Single agent schemes
In patients with metastatic endocrine-independent HER-2-negative BC with minor cancer-related symptoms and with a limited tumor burden, sequentially used single-agent schemes are preferred (Cardoso et al., 2020; NCCN Clinical Practice Guidelines in Oncology, 2023). The order of agents is not established and depends on numerous factors, for example, previous treatment received in adjuvant and metastatic setting, the risk of cross-resistance, Breast Cancer gene 1/2 (BRCA1/2) mutation status, or comorbidities. Typical options include (Cardoso et al., 2020; NCCN Clinical Practice Guidelines in Oncology, 2023; ER-positive HER2-negative, Breast Cancer, 2023; Triple-negative Breast Cancer, 2023):
• Taxanes: docetaxel, paclitaxel or nanoparticle albumin-bound (nab)paclitaxel.
• Anthracyclines: doxorubicin hydrochloride (doxorubicin) or its pegylated, liposomal form; epirubicin hydrochloride (epirubicin).
• Capecitabine (an oral prodrug of fluorouracil).
• Vinca alkaloids: vinorelbine tartrate (vinorelbine) as intravenous infusion or oral capsules.
• Gemcitabine hydrochloride (gemcitabine).
• Eribulin mesylate.
• Etoposide as oral form.
• Platinum agents: cisplatin, carboplatin.
• Ixabepilone, not available in Europe.
Other agents used include:
• The Poly (ADP-ribose) polymerase (PARP) inhibitor olaparib in BRCA1/2 mutated patients.
• ADC Fam-trastuzumab deruxtecan-nxki (T-DXd) for pretreated patients with HER-2 low status (1+ or 2+ in immunohistochemistry and in situ hybridization negative status) as per the Destiny-Breast04 study in ER/PR-positive and ER/PR-negative BC (Modi et al., 2020).
• ADC sacituzumab govitecan-hziy (SG) for metastatic and pretreated HER-2 negative ER/PR-positive BC as per the TROPiCS-02 study (Rugo et al., 2020) and for TNBC as per the ASCENT trial (Bardia et al., 2021).
• Pembrolizumab, anti-Programmed cell death protein 1 (PD1) monoclonal antibody (mAb) as per the KEYNOTE 355 study in TNBC in combination with chemotherapy (Cortes et al., 2020).
2.3 Combination regiments
For patients who require combination regimens due to rapidly progressing disease, symptoms or large tumor burden, there are numerous drug combinations available (Cardoso et al., 2020; NCCN Clinical Practice Guidelines in Oncology, 2023; ER-positive HER2-negative, Breast Cancer, 2023; Triple-negative Breast Cancer, 2023):
• Anthracycline-based schemes (with taxanes, cyclophosphamide and fluorouracil).
• Taxane-based regimens (with gemcitabine, capecitabine).
• Platinum-based regimens (with taxanes, gemcitabine or vinorelbine).
• Ixabepilone with capecitabine.
• An old scheme known as CMF (cyclophosphamide, methotrexate and fluorouracil).
2.4 Naturally-derived agents
Numerous of the above-mentioned drugs have been derived from natural sources as secondary metabolites (Michalak and Püsküllüoğlu, 2022; Püsküllüoğlu and Michalak, 2022; Siddiqui et al., 2022). ADC such as T-DXd and SG, used in the discussed indication, can also be included in this group. The drugs conjugated with mAbs (trastuzumab directed against HER-2 for T-DXd and sacituzumab directed against Trophoblast cell-surface antigen 2 [TROP-2] for SG) are camptothecin derivatives (deruxtecan for T-DXd and SN-38 for SG) (Corti et al., 2021).
There is a long history of folk medicine with the usage of species being a source for anticancer naturally-derived agents. For example, the Himalayan yew (Taxus wallichiana Zucc., Taxaceae) bark and leaves are used in traditional medicine for steam baths (rheumatism), paste application (fractures, headaches), and medicinal hair oils. In Pakistan, stem decoction treats tuberculosis. Unani medicine uses them for Zarnab, addressing various conditions, while Ayurveda employs young shoots for a tincture treating headache, feeble pulse, giddiness, diarrhea, and severe biliousness (Juyal et al., 2014). Within the Ayurvedic medicinal system, various metabolites of periwinkle (Catharanthus roseus (L.) G. Don, Apocynaceae) are employed in traditional herbal medicine to address a range of health conditions, including diabetes, cancer, stomach disorders, liver, kidney and cardiovascular diseases (Kumar et al., 2022). The extract containing podophyllotoxin has been historically noted for its effectiveness as a laxative and as a treatment for diverse medical conditions, including tuberculosis, gonorrhea, menstrual disorders, dropsy, psoriasis, syphilis or venereal warts (Shah et al., 2021).
3 Secondary metabolites as anticancer agents
Research into natural metabolites with anticancer properties began in the 1960s and is still ongoing (Michalak and Püsküllüoğlu, 2022; Püsküllüoğlu and Michalak, 2022). Extracts obtained from plants, algae, fungi and lichens are a challenge because they are multi-component mixtures of active, partially active and inactive substances. Their composition may vary depending on the plant raw materials used; parts used (aerial parts, roots, bark, etc.); form of the biomass–fresh, dried, fermented; area, season, date, time of harvesting, etc., and the method of preparing these extracts (Heinrich et al., 2022) imitations can also include restricted availability of natural raw materials; structural complexity of secondary metabolites; stability concerns; purification, isolation and characterization difficulties; synthetic limitations or formulation hurdles. In order to ensure the reproducibility and interpretation of the results of pharmacological and clinical tests, Heinrich et al. (2022) proposed recommendations regarding the reporting of plant material and its method (stages) of processing.
3.1 The main groups of secondary metabolites
The main groups of secondary metabolites reported to have anticancer properties in endocrine-independent HER-2-negative breast cancer are phenolics, alkaloids, and terpenoids (Raman et al., 2018; Ding et al., 2020; Lee et al., 2020; Mazzei et al., 2020; Maungchanburi et al., 2021; Noh et al., 2021; Lin et al., 2022; Qi et al., 2022; Sancha et al., 2022; Youssef et al., 2022; Thilagavathi et al., 2023). Phenolics include simple phenols (phenolic acids and coumarins) and polyphenols (flavonoids and non-flavonoids such as tannins, lignans, and stilbenes). Examples of alkaloids, low-molecular-weight nitrogenous metabolites, are atropine, caffeine, capsaicin, cocaine, daturin, hiosciamin, lysergic acid, nicotine, strychnine, quinine (Alamgir, 2018; Thilagavathi et al., 2023). Terpenoids or terpenes, the major metabolites of essential oils, are classified as hemiterpenes (C5), monoterpenes (C10), sesquiterpenes (C15), diterpenes (C20), sesterterpenes (C25), triterpenes (C30), and tetraterpenes/carotenoids. This group of secondary metabolites includes aromatic oils, carotenoids, resins, steroids, waxes, and others (Alamgir, 2018; Thilagavathi et al., 2023).
3.2 Optimizing anticancer metabolite extraction
The key stage of research is the selection of biomass, which is a potential source of these anticancer metabolites. Subsequently, appropriate extraction techniques should be used to isolate metabolites of interest. In addition to simple extraction techniques, such as mixing/shaking, maceration or continuous extraction in the Soxhlet apparatus, novel extraction techniques, such as supercritical fluid extraction, sonication, ultrasound- and microwave-assisted extraction (Shin et al., 2017; Raman et al., 2018; Ding et al., 2020; Lee et al., 2020; Mazzei et al., 2020; Noh et al., 2021; Youssef et al., 2022) are in demand. The extraction process is usually carried out at room temperature in order to protect biologically active metabolites from degradation. Extracts derived from the biomass typically provide low yields of bioactive chemicals, which makes their isolation and purification time-consuming and expensive (Mazzei et al., 2020; Lin et al., 2022). Therefore, the selection of the appropriate extraction process, experimental conditions, and solvent seems to be of paramount importance. Finally, a detailed characterization of the obtained extract, which is a concentrate of biologically active metabolites, is required, or the isolation of metabolites from the extract showing desired anticancer properties specific to a given cancer type. The overriding goal is to search for extracts rich in bioactive substances that have an inhibitory effect on the proliferation and viability of cancer cells, while being comparatively safe for normal cells (Raman et al., 2018). Therefore, further fractionation of the extract is needed using the column chromatography separation. The purity of the active metabolites can be confirmed by a High-Performance Liquid Chromatography (HPLC). For the identification of different metabolites within an obtained fraction, gas chromatography coupled with mass analysis (GC-MS) can be applied. The functional groups in the isolated metabolites can be determined by Fourier Transform Infrared (FT-IR) Spectroscopy and the structure can be identified using Proton Nuclear Magnetic Resonance Spectroscopy (1H NMR) and other spectroscopic data (Maungchanburi et al., 2021; Heinrich et al., 2022; Qi et al., 2022; Sancha et al., 2022; Youssef et al., 2022).
The conducted research shows that not all extracts/extracted metabolites are cytotoxic to tested BC cell lines (Supplementary Table S1). For example, alliacane sesquiterpenes–purpuracolide B and purpuracolide C extracted from edible fungus Gomphus purpuraceus (Iwade) K. Yokoy. 1989 are inactive against MDA-MB-231 cell line (He et al., 2022). This shows how time-consuming and tedious it can be to work on the selection, extraction and testing of a metabolite that may have cytotoxic properties against cancer cells. Another challenge is the selectivity of the natural extracts/extracted metabolites produced in relation to the tested cancer cell lines. For example, Alsaraf et al. (2019) showed that the methanolic extract produced from plantain (Plantago lanceolata L., Plantaginaceae) leaves significantly inhibits the proliferation of TNBC CAL51 cells but demonstrate minor effect on the MDA-MB cells (Alsaraf et al., 2019). It also indicates that the heterogenicity of BC or even BC subtypes like TNBC cannot be neglected (Marra et al., 2020).
In the future, it is crucial to conduct comprehensive characterization of the obtained extracts to determine their chemical composition and to identify specific metabolites possessing anticancer properties. Some studies have already conducted fractionation of natural extracts and identified metabolites (e.g., pancratistatin (Youssef et al., 2022), lupeol (Sánchez-Valdeolívar et al., 2020), oleuropein aglycone (Zwartsen et al., 2019; Mazzei et al., 2020;), pyoluteorin (Ding et al., 2020), atranorin (Harikrishnan et al., 2021)) showing potential activity against endocrine-independent HER-2 negative hormone-pretreated BC.
4 Pre-clinical data
While clinical guidelines recognize the overlap in features and treatment of TNBC and endocrine-resistant BC (Moy et al., 2021), studies in molecular biology and pharmacology often overlook the issue of endocrine-resistant BC. Consequently, there is a scarcity of pre-clinical models and studies exploring these subtypes, with a predominant focus on TNBC in other available reviews.
In this chapter we provide a perspective on recent advances in the primary literature showing limitations of pre-clinical models. We also stress the significance of investigating endocrine-independent HER-2-negative BC subtypes collectively similarly to the approach in clinical guidelines.
4.1 In vitro studies
Last years have yielded numerous publications regarding in vitro studies on natural metabolite derivatives in TNBC, but also a certain amount of research in HER-2 negative endocrine-pretreated BC. Currently, the majority of promising metabolites are derived from plants (e.g., phytochemicals), but fungi and algae/seaweeds are tempting sources as well (Supplementary Table S1).
4.1.1 Defining cell line models
Defining a proper in vitro cell line model for both: TNBC and HER-2 negative endocrine-resistant BC is a challenging and neglected task (Cheng et al., 2022; Soosainathan et al., 2022). Attempts to create hormone-resistant model usually involve growing a cell line (originally from the Luminal BC cell line) that has developed resistance to a particular type of hormone therapy, such as tamoxifen (selective estrogen receptor modulator), fulvestrant (ER antagonist) or aromatase inhibitors. Authors usually focus on inducting hormonal resistance in the commonly used endocrine-sensitive MCF-7 cell line, which only partially mimics real heterogenous clinical scenario (Cardoso et al., 2020; Soosainathan et al., 2022) (Supplementary Table S1). What is more, in case of in vitro studies with hormone-pretreated BC cell line models, the efforts are commonly directed at reversing the hormone-resistance using natural metabolites, not at showing potential effects of new chemotherapeutics (Wang et al., 2021). Regarding TNBC cell lines: MDA-MB-231, MDA-MB-468, and CAL51 are most commonly used. Among the cell lines mentioned, MDA-MB-231 are the most frequently applied TNBC model in in vitro studies (Supplementary Table S1). Considering high heterogenicity in TNBC (Marra et al., 2020) using a cell line characterized by mutation in V-Raf murine sarcoma viral oncogene homolog B1 (BRAF) and Neurofibromatosis 1 genes does not reflect potential response to the treatment in all TNBC subtypes (Wagner, 2022).
When metabolites are evaluated in conventional cell line models, the scenario parallels the topical application of a ‘candidate medication’, as these compounds do not undergo the full spectrum of Absorption, Distribution, Metabolism, and Excretion (ADME) pharmacokinetic processes.
4.1.2 Improving cell line models
Overall, these in vitro models possess numerous limitations. Improved in vitro pre-clinical models, such as organoids, organ-on-a-chip, tissue-on-a-chip, bioengineered tissue models and multi-cellular spheroids may hold superiority over simpler patient-derived cell lines for identifying novel classes of drugs. Nevertheless, even with these advancements, these models exhibit significant limitations, including restricted physiological relevance, challenges in standardization and reproducibility and high costs (Xu et al., 2021b; Yadav et al., 2021).
In vitro metabolism techniques, which utilize liver fractions or other metabolically capable systems such as primary hepatocytes or recombinant enzymes, represent a significant advancement. These innovative approaches not only facilitate the dissection of biological processes but also accelerate drug development and enhance the efficiency of toxicology studies (Ooka et al., 2020).
What is more, the potential benefit of using novel delivery techniques, e.g., drug nanoform is difficult to be examined in in vitro studies as potential benefits of these drugs can result from improved solubility or obtaining more comfortable route of administration.
4.2 In vivo studies
4.2.1 Currently applied in vivo models
In vivo studies in mice with anticancer metabolites face numerous limitations in terms of translatability to humans, capturing the complexity of tumor heterogeneity, and accurately reflecting human immune responses. The application of the natural products in mice injected with MDA-MB-231 cells results in decrease in the tumor size/volume and tumor weight (Utage et al., 2018; Collard et al., 2020; Luo et al., 2022). Recently published data from in vivo researches suggest that natural products derived from various sources have the potential to inhibit tumor growth, reduce angiogenesis, proliferation, and modulate the tumor microenvironment in TNBC and HER-2 negative hormone-pretreated BC. However, the efficacy and interactions of these natural products may vary. Further research is needed to elucidate the underlying mechanisms and optimize their therapeutic application (Supplementary Table S2).
4.2.2 Improving in vitro models
Advanced in vivo studies (e.g., patient-derived xenograft models or humanized mouse models) can improve data quality but also come with ethical concerns, limited predictive power and high costs (Xu et al., 2021b; Yadav et al., 2021).
While number of the presented studies can be considered cutting edge research in pre-clinical setting the effectiveness of these chemotherapies as new and highly efficient therapeutic options should be further examined in clinical trials.
5 Mechanistic findings for novel naturally-derived drugs
5.1 The main mechanisms responsible for the anticancer activity of naturally-derived metabolites
An overview of numerous effects of natural metabolites in TNBC and HER-2 negative endocrine-resistant BC indication is presented in Figure 2. Most registered chemotherapeutic agents used in BC treatment act through inhibition of mitosis, lead to cell cycle arrest, influence deoxyribonucleic acid (DNA) replication processes, and thus induce apoptosis through well-known mechanisms (see Table 1).
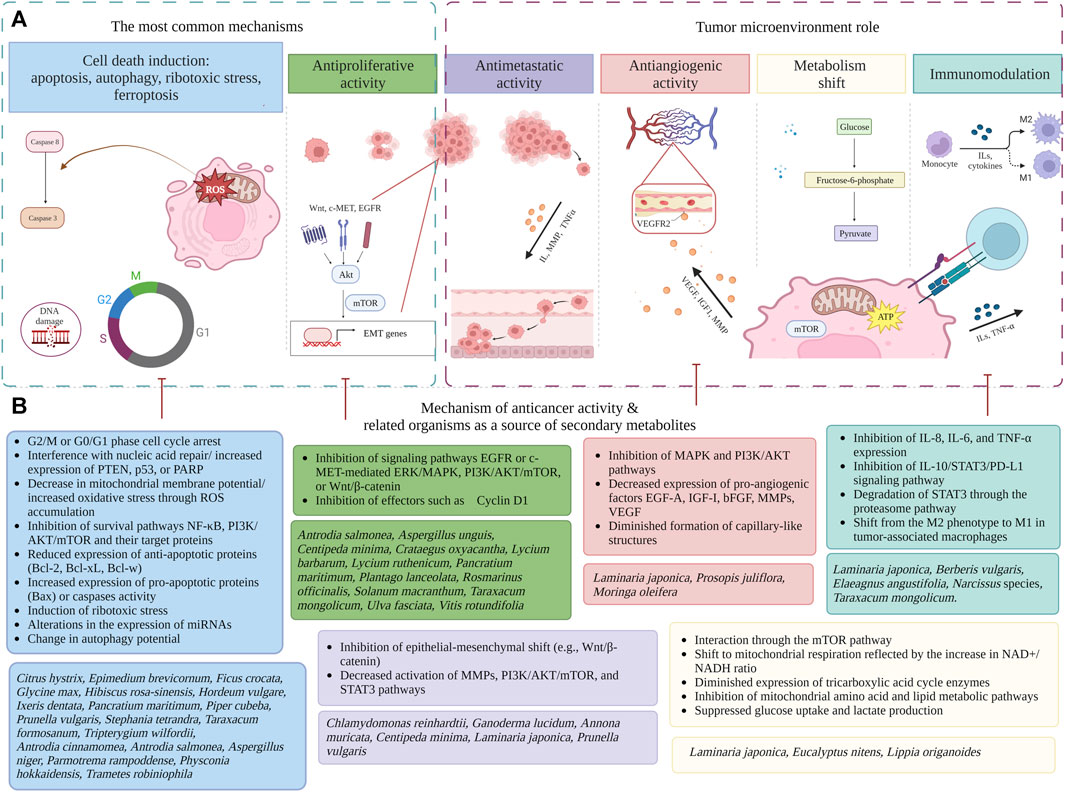
FIGURE 2. An overview of mechanistic effects of natural metabolites in endocrine-independent HER-2 negative breast cancer (created with BioRender). (A) Diagrammatic representation of the mechanistic findings: cell death induction (Cheng et al., 2019; Guo and Pei, 2019; Nguyen et al., 2019; Zhang et al., 2019; Ding et al., 2020; Ho et al., 2020; Liu et al., 2020; Sánchez-Valdeolívar et al., 2020; Harikrishnan et al., 2021; Maungchanburi et al., 2021; Noh et al., 2021; Fouzat et al., 2022; Lin et al., 2022; Luo et al., 2022; Sancha et al., 2022; Sangpairoj et al., 2023), antiproliferative activity (Alsaraf et al., 2019; Zwartsen et al., 2019; Collard et al., 2020; Jaglanian and Tsiani, 2020; Kalebar et al., 2020; Lee et al., 2020; Mazzei et al., 2020; Yang et al., 2021; Lin et al., 2022; Youssef et al., 2022; Kombiyil and Sivasithamparam, 2023) antimetastatic activity (Hsu et al., 2020; Lee et al., 2020; Chen et al., 2022; Luo et al., 2022); antiangiogenic activity (Utage et al., 2018; Hsu et al., 2020; Zunica et al., 2021), disrupted cancer cell metabolism (Raman et al., 2018; Guerra et al., 2021; Yang et al., 2021; Chen et al., 2022; Ke et al., 2022), immunomodulation (Kim et al., 2018; Zhao et al., 2020; 2021; Deng et al., 2021; Chen et al., 2022; Fouzat et al., 2022; Lv et al., 2022). (B) Each mechanism of anticancer activity is described in details and recently studied organisms as a source of secondary metabolites are presented (full species names including authorities and family are presented in Table 1; Supplementary Table S1. Abbreviations: AKT, protein kinase B; Bax, Bcl-2-associated X protein; Bcl-2, B-cell lymphoma 2; Bcl-w, B-cell lymphoma 2-like protein; Bcl-xL, B-cell lymphoma-extra-large; BC, Breast cancer; bFGF, basic fibroblast growth factor; CDK, cyclin-dependent kinase; EGFR, epidermal growth factor receptor; EGF-A, epidermal growth factor A; EMT, epithelial mesenchymal transition; ERK, extracellular signal-regulated kinase; HER-2, human epidermal growth factor receptor-2; IGF-I, insulin-like growth factor-I; IL, interleukin; MAPK, mitogen-activated protein kinase; mTOR, mammalian target of rapamycin; miRNA, microRNA; MMP, matrix metalloproteinase; NAD+, nicotinamide adenine dinucleotide (oxidized form); NADH, nicotinamide adenine dinucleotide (reduced form); NF-κB, nuclear factor-kappa B; PARP, poly (ADP-ribose) polymerase; PI3K, phosphatidylinositol-3-kinase; PD-L1, programmed death-ligand 1; p53, tumor protein 53; PTEN, phosphatase and tensin homolog; ROS, reactive oxygen species; STAT3, signal transducer and activator of transcription 3; TNF-α, tumor necrosis factor-alpha; VEGF, vascular endothelial growth factor; Wnt, wingless-related integration site.
5.1.1 Cell death induction
Programmed cell death is a broad and intricate concept encompassing a diverse array of mechanisms, including apoptosis, which occurs as a consequence of numerous changes in cancer cells. Some of these mechanisms have been identified for naturally-derived drugs (Alsaraf et al., 2019; Cheng et al., 2019; Guo and Pei, 2019; Mendonca et al., 2019; Nguyen et al., 2019; Zhang et al., 2019; Ding et al., 2020; Ho et al., 2020; Jaglanian and Tsiani, 2020; Lee et al., 2020; Mazzei et al., 2020; Sánchez-Valdeolívar et al., 2020; Harikrishnan et al., 2021; Maungchanburi et al., 2021; Noh et al., 2021; Yang et al., 2021; Fouzat et al., 2022; Ke et al., 2022; Lin et al., 2022; Luo et al., 2022; Sancha et al., 2022; Sangpairoj et al., 2023) (Figure 2). The exact mechanism leading to cell death is essential to be defined as it can predict drugs’ toxicity, efficacy or suggest potential effects of combining with other medications such as synergistic or complementary effect. In a study aimed at assessing the cytotoxic properties of secondary metabolites isolated from Aspergillus niger soil fungus (Aspergillaceae), pyoluteorin was observed to arrest the cell cycle of TNBC cells at the G2/M phase. Further experiments suggested that pyoluteorin’s apoptotic effect was linked to a decrease in mitochondrial membrane potential and an accumulation of reactive oxygen species (ROS). (Ding et al., 2020). ROS can react with a variety of biological molecules like DNA, proteins, lipids inducing their damage and initiate apoptosis of cancer cells (Alsaraf et al., 2019; Mendonca et al., 2019). Increased oxidative stress and decreased mitochondrial membrane potential were also observed in in vitro and in vivo HER-2 negative BC models for the following extracts: the common hibiscus (Hibiscus rosa-sinensis L., Malvaceae) (Nguyen et al., 2019), sea daffodil (Pancratium maritimum L., Amaryllidaceae) (Sancha et al., 2022), lichen Parmotrema rampoddense (Nyl.) Hale 1974, Parmeliaceae (Harikrishnan et al., 2021).
Interfering with the ability to repair nucleic acids is another mechanism leading to the death of cancer cells. Combining cisplatin with triptolide, a natural metabolite derived from a Chinese herb–Tripterygium wilfordii Hook. f. (Celastraceae) for treating TNBC caused DNA damage and arrested TNBC cells in the S phase of the cell cycle, making them more sensitive to cisplatin treatment. Triptolide decreased the levels of The Poly (ADP-ribose) polymerase (PARP1) and X-ray repair cross complementing protein 1 (XRCC1), which are involved in repairing single-strand breaks and base excision, on protein level (Zhang et al., 2019).
Inhibition of survival pathways is one of the key mechanisms by which natural metabolites provoke apoptosis. Among these signaling pathways: phosphatidylinositol-4,5-bisphosphate 3-kinase catalytic subunit alpha/protein kinase B (PI3K/AKT) and nuclear factor-kappa B (NF-κB) can be named (Yang et al., 2021; Ke et al., 2022).
The inhibition of anti-apoptotic proteins B-cell lymphoma 2 (Bcl-2) and B-cell lymphoma 2 (Bcl-xL) and activation of pro-apoptotic proteins Bcl-2-associated death promoter (Bad) and Bcl-2-associated X protein (Bax) that regulate mitochondrial pathway and lead to altered caspase pathway expression is the next mechanism. Extract from Kaffir lime (Citrus hystrix DC., Rutaceae) leaves contain citronellol and citronellal. These metabolites have been shown to inhibit the expression of Bcl-2 and activate pathway dependent on caspase-3 in TNBC in vivo model (Ho et al., 2020). Similar results for caspase-3 activation were observed for Prunella vulgaris L. (Lamiaceae) extract (Luo et al., 2022). Another plant extract obtained from red guava (Psidium guajava L., Myrtaceae) induced apoptosis through caspase-3, but also through PARP signaling (Liu et al., 2020). Induction of numerous caspases activity was obtained in vivo on BC lines with Piper cubeba L. f. (Piperaceae) seeds extract (Maungchanburi et al., 2021). The Elaeagnus angustifolia L. (Elaeagnaceae) flower extract induced pro-apoptotic proteins such as: Bax and cleaved caspase-8 and reducing the anti-apoptotic Bcl-2 (Fouzat et al., 2022). Alkaloids obtained from sea daffodil (Pancratium maritimum L.) also increased Bax expression, and decreased Bcl-xL expression (Sancha et al., 2022). The dichloromethane extract from a tree Ficus crocata (Miq.) Mart. ex Miq. (Moraceae) was found to have apoptotic effects on MDA-MB-231 cells, leading to increased expression of tumor suppressor protein p53, as well as procaspase-8, and procaspase-3 (Sánchez-Valdeolívar et al., 2020). It has been found that the treatment with lichen (Physconia hokkaidensis Kashiw. 1975; Physciaceae) extract resulted in the downregulation of Bcl-2, p-AKT and adenosine monophosphate-activated protein kinase (AMPK) and while significantly upregulating the levels of cleaved caspase-9, cleaved caspase-3, and cleaved-PARP. Physconia hokkaidensis Kashiw. 1975 extract showed selective cytotoxicity toward TNBC lines, but not luminal BC lines (Noh et al., 2021). The extract obtained from common selfheal (Prunella vulgaris L., Lamiaceae) triggered cell apoptosis by enhancing nuclear DNA damage and augmenting the expression of cleaved caspase-3 (Luo et al., 2022). The lichen Parmotrema rampoddense (Nyl.) Hale 1974 contains a metabolite called atranorin, which has antimicrobial properties, but a study of Harikrishnan et al. (2021) found that atranorin can also have anticancer properties–it significantly decreased the levels of anti-apoptotic proteins such as AKT, Bcl-2, Bcl-xL, and B-cell lymphoma 2-like protein (Bcl-w), while increasing the levels of pro-apoptotic protein Bax and caspases-3 activity in breast cancer cells. This effect was even greater than that of the AKT inhibitor ipatasertib (Harikrishnan et al., 2021). Resveratrol, a polyphenol derived from numerous fruit plants, induced apoptosis in TNBC cells by reducing DNA polymerase delta 1 (POLD1) expression. This mechanism involved decreased levels of anti-apoptotic proteins like Proliferating cell nuclear antigen and BCL-2, increased expression of Cleaved-PARP1 and Cleaved-Caspase3, and potential binding of resveratrol to POLD1 functional domains (Liang et al., 2021).
The metabolite known as oleuropein aglycone was created by using enzymes to break down oleuropein found in olive leaves. This substance was found to have pro-apoptotic effects on two different types of cancer cells: MDA-MB-231 and Tamoxifen-resistant MCF-7 (Mazzei et al., 2020). Icariin, a major metabolite extracted from a Chinese herb Epimedium brevicornum Maxim. (Berberidaceae) showed potential to overcome tamoxifen resistance in MCF-7/TAM cells. It induced cell cycle arrest in the G0/G1 phase, apoptosis, and inhibited autophagy. At the molecular level, icariin treatment resulted in the downregulation of CDK2, CDK4, Cyclin D1, Bcl-2, Microtubule-associated protein light chain 3 (LC3-1, LC3-II), Autophagy-related protein 5 (AGT5), and Beclin-1, but upregulated the expression of caspase-3, PARP, and p62 (Cheng et al., 2019). Tetrandrine, an alkaloid extracted from the Chinese herbal medicine–Stephania tetrandra S. Moore (Menispermaceae) root targeted autophagy genes LC3-II/LC3-I, p62/SQSTM1, and Beclin-1, inhibited PI3K/AKT/mTOR signaling and increased Phosphatase and tensin homolog (PTEN) expression in TNBC cell lines (Guo and Pei, 2019). The extract obtained from red alga Halymenia durvillei Bory de Saint-Vincent (Halymeniaceae) was also found to increase the expression of LC3 (an indicator of autophagic cell death) (Sangpairoj et al., 2023). Extract from dandelion (Taraxacum formosanum Kitam., Asteraceae) induced another interesting mechanism that can lead to cell death in BC cell lines: ribotoxic stress. Ribotoxic stress refers to a cellular pathway that reacts to errors in translation, triggering the activation of p38 and c-Jun N-terminal kinases. This response leads to cell cycle arrest, the generation of inflammatory cytokines, and the initiation of apoptotic signaling (Lin et al., 2022). Ferroptosis is an iron-dependent programmed cell death, distinct from apoptosis. It involves the metabolism of unsaturated fatty acids, leading to lipid peroxidation and eventual cell death. This mechanism seems to be caused by both well-known drugs (such as doxorubicin), but also by plant-derived metabolites, e.g., curcumin (Cao et al., 2022; Kciuk et al., 2023).
5.1.2 Antiproliferative and antimetastatic activity
Other important mechanisms include inhibition of cells’ proliferation (Alsaraf et al., 2019; Zwartsen et al., 2019; Collard et al., 2020; Jaglanian and Tsiani, 2020; Kalebar et al., 2020; Lee et al., 2020; Mazzei et al., 2020; Yang et al., 2021; Lin et al., 2022; Youssef et al., 2022; Kombiyil and Sivasithamparam, 2023) and metastatic potential (Avila-Carrasco et al., 2019; Hsu et al., 2020; Lee et al., 2020; Chen et al., 2022; Luo et al., 2022) (Figure 2). Ability to inhibit the growth and division of cancer cells is a hallmark of anti-cancer treatment. By inhibiting cancer cell growth mainly through interfering with signaling pathways, such as the Epidermal growth factor receptor (EGFR)-Mediated Extracellular signal-regulated kinases/Mitogen-activated protein kinases (ERK/MAPK), Wnt/β-catenin or PI3K/AKT/mTOR (partially overlapping with these responsible for proapoptotic, antiangiogenic or antimetastatic potential) natural metaboliteshave the potential to prevent the cancer progression and improve the efficacy of cancer treatments (Cumaoglu et al., 2018; Yang et al., 2021; Kombiyil and Sivasithamparam, 2023). For instance, pre-treatment with extracts derived from shrubs’ Lycium barbarum L. and Lycium ruthenicum Murray (Solanaceae) fruit has been shown to inhibit the phosphorylation of EGFR and ERK in MDA-MB-231 cells stimulated with epidermal growth factor (Cumaoglu et al., 2018). The methanolic extract of hawthorn (Crataegus oxyacantha L., Rosaceae) berry, which is rich in polyphenols, was observed to have toxic effects on TNBC cell lines by exhibiting a significant downregulation of the transcriptional and translational expression of Wnt pathway agonists, as well as an upregulation of Wnt antagonists (Kombiyil and Sivasithamparam, 2023). Seaweed–Ulva fasciata Delile (Ulvaceae) has been found to exhibit inhibitory effects on the signaling pathway involving EGFR/PI3K/AKT, resulting in the induction of cytotoxicity in TNBC cells (Pragna Lakshmi et al., 2017).
Muscadine grapes extract has showed its antiproliferative activity in vitro and in vivo (was given to nude mice with human TNBC tumors for 4 weeks). It decreased TNBC size and reduced the markers Ki67 and cyclin D1 in in vivo studies on nude mice and reduced c-Met and inhibited ERK/MAPK and AKT signaling, leading to decreased cyclin D1 and cell cycle arrest in in vitro experiments (Collard et al., 2020). Another study has demonstrated that the aqueous extract of Solanum macranthum Dunal fruit (the potato tree from Solanaceae) could potentially hinder the growth of MDA-MB-231 cells when it was dissolved in water (Kalebar et al., 2020). The use of Plantago lanceolata L. leaf extract on BC cells showed a significant ability to inhibit the growth of CAL51 cells, which are classified as TNBC. However, the extract had only a minor effect on other types of breast cancer cells. Moreover, at higher doses, it caused visible morphological changes to the cells (Alsaraf et al., 2019). Another investigation involved analysis of sea daffodil (Pancratium maritimum L.), a member of the Amaryllidaceae, as a potential anticancer agent. Pancratistatin, a bioactive metabolite, was isolated and its growth inhibitory effects were assessed on MDA-MB-231 cells (Youssef et al., 2022). Oleuropein aglycone derived from olive leaves displayed antiproliferative activity against both TNBC MDA-MB-231 and Tamoxifen-resistant MCF-7 call lies (Mazzei et al., 2020). Other natural metabolites that reduced proliferation of TNBC cell lines in vivo and in vitro were derived from: dandelion (Taraxacum mongolicum Hand.-Mazz., Asteraceae) (Lin et al., 2022), fungus Aspergillus sp. (Zwartsen et al., 2019), rosemary (Rosmarinus officinalis L., Lamiaceae) (Jaglanian and Tsiani, 2020), herb–Centipeda minima (L.) A. Braun & Asch., Asteraceae (Lee et al., 2020), or mushroom Antrodia salmonea T.T. Chang & W.N. Chou, Polyporaceae (Chang et al., 2017).
The ability to create metastatic disease is one of later steps in cancer development. It requires additional molecular alterations such as epithelial-mesenchymal shift in the metastatic cascade to allow cancer cell to move and develop as metastasis. Among the mechanisms already described in Section 7.4, increased expression of MMPs and disrupted expression of adhesive molecules (e.g., increased N-Catherin, β-Catenin and decreased E-Catherin) are noted (Wilusz and Majka, 2008; Avila-Carrasco et al., 2019). Ebushicao (Centipeda minima (L.) A. Braun & Asch.) extract in TNBC cell line led to decreased MMP-9, PI3K/AKT/mTOR and STAT3 pathways activation, resulting in an inhibited metastatic process (Lee et al., 2020). Another extract from medicinal fungus Ganoderma lucidum (Curtis) P. Karst (Ganodermataceae) inhibited the release of Interleukins (IL8, IL6), MMP-6 and MMP-9 and reduced TNBC cell migration (Barbieri et al., 2017). Fucoidan from brown seaweed Laminaria japonica Areschoug, 1851 (Laminariaceae) can also significantly reduce the ability of TNBC cells to metastasize (Hsu et al., 2020). Antimetastatic activity in this BC setting was also noted for extract derived from: common selfheal (Prunella vulgaris L.), green alga Chlamydomonas reinhardtii (CC-124) from Chlamydomonadaceae, and soursop (Annona muricata Linn) from Annonaceae (Syed Najmuddin et al., 2016; Kamble et al., 2018; Chen et al., 2022; Luo et al., 2022).
5.2 Tumor microenvironment role
The tumor microenvironment holds significant importance in pre-clinical research on naturally-derived agents. It consists of a complex interplay of cellular and non-cellular components surrounding the tumor, including immune cells, extracellular matrix, signaling molecules or blood vessels. These factors profoundly influence tumor growth, invasion, and how the tumor responds to various agents.
When exploring mechanisms related to tumor microenvironment role in endocrine-independent HER-2 negative breast cancer antiangiogenic activity (Hsu et al., 2020; Zunica et al., 2021), metabolism shift (Lin et al., 2019; Zhao et al., 2020; 2021; Deng et al., 2021; Chen et al., 2022; Fouzat et al., 2022; Lv et al., 2022) and immunomodulation (Guerra et al., 2021; Yang et al., 2021; Chen et al., 2022; Ke et al., 2022) (Figure 2) are presented.
5.2.1 Antiangiogenic activity
As an example, fucoidan, extracted from brown seaweed Laminaria japonica Areschoug, 1851, has been shown to inhibit Mitogen-activated protein kinase and PI3K/AKT pathways, as well as the expression of several pro-angiogenic factors including: Epidermal growth factor A (EGF-A), Insulin-like growth factor I (IGF-I), Basic fibroblast growth factor (bFGF), Metalloproteinases 2 and 9 (MMP-2 and 9) and Vascular endothelial growth factor (VEGF) leading to diminished formation of capillary-like structures (Hsu et al., 2020).
5.2.2 Immunomodulation
Berberine is a plant derivative that influences BC immunity through several mechanisms, including reduced expression of IL-6 and Tumor necrosis factor-α (TNF-α) weakening the inflammatory processes induced by TNBC cell lines (Zhao et al., 2020). Another example is a flowering plant–dandelion (Taraxacum mongolicum Hand.-Mazz.) extract that inhibited IL-10/Signal transducer and activator of transcription 3 (STAT3)/PD-L1 signaling pathway as well as shifted from the tumor-associated macrophages phenotype M2 to M1 (Deng et al., 2021). Narciclasine, an antimitotic metabolite derived from daffodil (Narcissus L.) bulbs from Amaryllidaceae was able to degrade STAT3 in a specific manner in tamoxifen-resistant MCF-7 cells through the proteasome pathway (Lv et al., 2022).
5.2.3 Metabolism shift
Eucalyptus bark extract used in TNBC cell lines resulted in hydrolysis of cholesterol esters and triglycerides, leading to shift to mitochondrial respiration, which was reflected by increase in Nicotinamide adenine dinucleotide (NAD+, oxidized form) to Nicotinamide adenine dinucleotide (NADH, reduced form) ratio (Guerra et al., 2021). This provides cancer cells with a more efficient energy source and lowers ROS production (Guerra et al., 2021). The extract from the aromatic shrub–Lippia origanoides Kunth (Verbenaceae) was found to hinder the metabolic activity of cells by reducing the expression of tricarboxylic acid cycle enzymes and inhibiting mitochondrial amino acid and lipid metabolic pathways. It also disrupted the process of mitochondrial oxidative phosphorylation by inhibiting the expression of multiple subunits of Complex I (Raman et al., 2018).
Further investigation is required to understand the role of the tumor microenvironment and its longitudinal changes during drug treatment, particularly in relation to drug resistance.
5.3 Other mechanisms
Natural metabolites effects on cellular or molecular level in BC treatment are also explored in many other aspects than simply using them as potential drugs, e.g., for chemoprotection. One example is protection of hematopoiesis by extract derived from a perennial plant–Polygonatum sibiricum Redouté (Liliaceae) (Xie et al., 2021). Polysaccharides contained in this herb are claimed to inhibit activity of myeloid cells in immune tumor infiltrating cells and in spleen (in in vivo studies with mice with TNBC) at the same time protecting bone-marrow hematopoiesis (Xie et al., 2021). Other effects include: deactivating the stromal microenvironment by reducing tumor associated fibroblasts (Xu et al., 2020), reversing resistance to chemotherapy (Wang et al., 2020; Yi et al., 2021), and acting on cancer stem cells (Wang et al., 2020; Ke et al., 2022). Some natural metabolites do not act as medications by themselves, but increase sensitivity to currently available treatment options. In the case of hormone-pretreated tumors the efforts are directed at overcoming the endocrine resistance (e.g., Wang et al., 2021). Resveratrol overcomes endocrine resistance in tamoxifen-resistant MCF-7/TR cells by influencing the transforming growth factor-beta (TGF-β)/Smad signaling pathway (Shi et al., 2013). or TNBC tumors studies are aimed at chemotherapy resistance mechanisms (e.g., (Suarez-Arroyo and Martinez-Montemayor, 2018)). Natural raw materials are also known to serve as carriers of many secondary metabolites that have been reported to have anticancer properties in both in vitro and in vivo experiments, especially metabolites belonging to phenolics, alkaloids, and terpenoids (Püsküllüoğlu and Michalak, 2022).
With all the enthusiasm accompanying these metabolites there is always a risk of increased toxicity or drugs interaction, e.g., in research by Zunica et al. (2021) moringa seed extract (Moringa oleifera Lam., Moringaceae) used in in vivo experiment on mice with TNBC and obesity has shown a negative interaction when used as a concurrent systemic treatment (Zunica et al., 2021).
6 Advancing drug delivery: novel technologies
A rapid advancement within the domain of targeted delivery of cytotoxic agents is observed. Examples of clinically applied strategies in endocrine-independent HER-2-negative breast cancer include nanotechnology and ADCs.
The composition of ADCs presents distinctive hurdles in the characterization of pharmacokinetics and pharmacodynamics. This arises from the necessity for a comprehensive grasp of these processes’ attributes across various molecular entities (such as the conjugate, unbound payload and antibody) within diverse tissues (Li et al., 2020).
6.1 Nanotechnology
Nanocarriers offer enhanced drug activity and utility through controlled release, extended circulation, and targeted delivery to cancer cells. Encapsulating drugs in nanoparticles can enable alternative administration routes. These nanocarriers utilize passive and active targeting mechanisms. Among others, nanoforms of taxanes, anthracyclines, camptothecins or eribulin mesylate have recently been tested (Yap et al., 2021; Miguel et al., 2022; Püsküllüoğlu and Michalak, 2022). Nanoparticles can be obtained from natural sources by themselves acting as immunomodulators, drugs, or in cancer prevention or cytoprotection (Püsküllüoğlu and Michalak, 2022). Next to clinically applied nanoforms of drugs new options are tested in pre-clinical and clinical setting (see below). Example of recently published pre-clinical study involves doxorubicin-loaded magnetite nanoparticles in TNBC in vitro cell line model (Markhulia et al., 2023).
6.2 Antibody-drug conjugates
Currently, the greatest group of targeting agents seems to be ADCs with SG and T-DXd being already approved in this indication (Cardoso et al., 2020; Püsküllüoğlu et al., 2023). ADC selectively recognizes and binds to specific cancer cells. The linker acts as a bridge, connecting the antibody to the cytotoxic payload, which exerts its effect by inducing cell death in the targeted cancer cells. The phenomenon where neighbouring cancer cells, not directly targeted by the drug, are still affected by the cytotoxic payload is called bystander effect. Ideal ADC payloads should have high cytotoxicity, low immunogenicity, and high stability. They should also possess modifiable functional groups for conjugation, promote bystander killing effects, have proper water solubility, and target intracellular sites for effective tumor cell penetration (Püsküllüoğlu et al., 2023; Wang et al., 2023). ADCs including naturally-derived agents that are tested in clinical trials in endocrine-independent HER-2-negative BC are presented in the next chapter. For pre-clinical setting a good example is ADC consisting of humanized MUC1 antibody linked to monomethyl auristatin (MMAE) and showing activity in cell line model (Li et al., 2023). MMAE is an antimitotic agent derived from sea hare (Dolabella auricularia [Lightfoot], 1786) from Aplysiidae that is too toxic to be used on its own, but linking to mAb allows toxicity to be limited (Püsküllüoğlu and Michalak, 2022).
It is expected that both: new receptors and new natural metabolites linked to targeting part will be further explored in the nearest future. The emphasis will be placed on the exploration of more specific targets for these BC populations. Exosome-based carriers are another alternative for modern drug delivery system (Song et al., 2021). However, the implementation of exosomes in BC clinical settings has not yet been realized, resulting in an uncertain assessment of their usefulness. Examples of other options used to target cytotoxic agents include targeted small molecules (Sun et al., 2021).
Figure 3 presents an overview of already applied and potential delivery strategies for natural metabolites in endocrine-independent HER-2 negative BC.
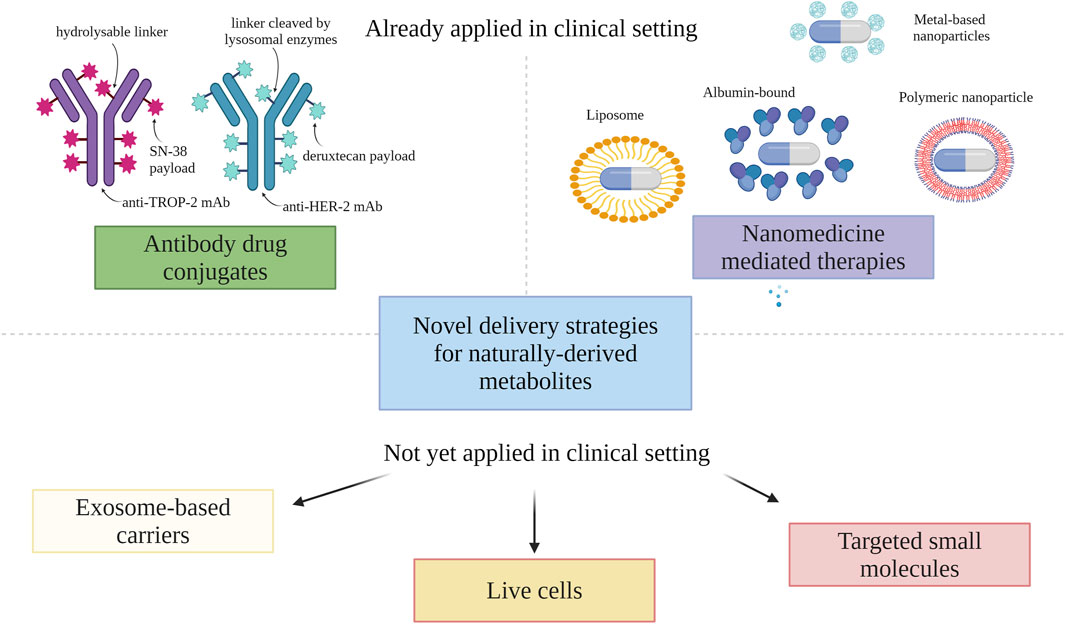
FIGURE 3. Novel mechanisms for drug delivery (created with BioRender). Abbreviations: HER-2, human epidermal growth factor receptor 2; mAb, monoclonal antibody; TROP2, trophoblast cell surface antigen 2.
7 Trends in clinical trials
Clinical trials are crucial in drug development, even for effective drugs in pre-clinical studies. They verify safety, efficacy, optimal dosage, and long-term effects in diverse human populations. Due to the pharmacokinetic and pharmacodynamic processes of naturally-derived compounds administered orally or parenterally, their biological effects on breast cancer cells and observed toxicity in the human body can be associated with the activity of their metabolites. Predicting such outcomes in pre-clinical trials is sometimes impracticable. Ethical and regulatory compliance mandates their use before widespread adoption. This section gives authors perspective on trends in ongoing clinical trials in endocrine-independent HER-2 negative breast cancer together with comments regarding trials’ limitations and challenges. Currently, studies are organized around four aspects: checking the safety and efficacy of new naturally-derived metabolites; exploring the potential of drugs of natural origin nanoforms or targeting them through systems such as ADC; investigating new schemes, routes of administration or treatment sequences of already approved drugs with natural origin. In contrast to the pre-clinical scenery, it is easier to select and define hormone-resistant setting in clinical trials as patients’ populations are clearly defined in inclusion and exclusion criteria within the study protocols. Clinical trials, viewed as experiments involving human subjects, are subject to a significantly higher degree of regulations and legal safeguards compared to pre-clinical studies. These include obligations related to their registration (all trials discussed in this section were registered in ClinicalTrials.gov and accessed on 4th November 2023). Clinical trials are conducted in pre-defined phases, during which safety, efficacy, and efficacy in comparison to the existing standard of care are evaluated in a given indication. Despite these regulations ensuring high standards and confidence in the quality of results, the interpretation of clinical trial outcomes can be challenging. For example, unplanned subgroup analyses may provide a misleading sense of excessive certainty regarding the quality of the obtained data. Furthermore, each type of study poses specific challenges, as discussed in each section below.
7.1 Trials regarding novel metabolites
Undoubtedly, the highest expectations are placed upon this particular group of studies. The pursuit of novel pharmaceutical agents exhibiting no cross-resistance with previously employed treatments and enhancing overall survival stands as the paramount hope in the realm of clinical research. Challenges may include lack of production standardization, limited access to natural sources, and thus, cost of the treatment. Some naturally-derived medications even when tested in clinical trials have no clear definition of their components or the solvent. Efforts to better control this issue are imperative, as it can lead to challenges in reproducing clinical data, as well as pose a significant risk of drug interactions. NCT04403529is the phase 3 randomized, double-blind trial checking the efficacy of Traditional Chinese Medicine in TNBC adjuvant setting. Primary endpoints are disease free survival (DFS) and quality of life. Unfortunately, clinicaltrial.gov web page devoted to that study does not explain which twelve herbal metabolites are incorporated into that medicine. That issue is a certain limitation in few ongoing clinical trials. In the phase 4 NCT02615457 trial, Huaier granules are being evaluated for their efficacy in radically treated TNBC. Huaier granules are a traditional Chinese medication extracted from a mushroom Trametes robiniophila Murrill 1907 (Polyporaceae) applied in colitis, nephrosis, tuberous sclerosis, and various cancers (Narayanan et al., 2023). Following international guidelines all tumors greater than 5 mm should receive preoperative systemic treatment (NCCN Clinical Practice Guidelines in Oncology, 2023). As per protocol patients who completed neoadjuvant treatment are excluded. As a result, a very low recruitment rate or comparison to control arm receiving the treatment inferior to current standard of care can be expected. This is another limitation of these clinical trials. The phase 1/2 NCT03387085 trial in metastatic TNBC setting tests metronomic chemotherapy of numerous naturally derived agents in terms of safety and ORR. Drugs such as: albumin-binding prodrug of doxorubicin; a set of vaccines derived from recombinant Saccharomyces cerevisiae yeast; nab-paclitaxel and other chemotherapeutics and biological agents are included in that trial. The phase 2 NCT05007444 study with P2Et (a standardized extract of tara (Caesalpinia spinosa (Molina) Kuntze, Fabaceae) in addition to standard neoadjuvant therapy is being performed on BC patients (not eligible for anti-HER-2 treatment) in order to assess optimal biological dose of P2Et based on its toxicological profile and other parameters as secondary endpoints. The phase 2 NCT05403333 trial is examining the efficacy (PFS) of weekly utidelone in HER-2 negative inoperable or metastatic BC (both TNBC and hormone-pretreated). This metabolite, a modified epothilone (see Table 1 for ixabepilone source) analog has already been tested in BC setting and although positive, the previous study did not result in drug approval (Xu et al., 2021a). An intriguing study, yet one that raises many unanswered questions and concerns (e.g., about the broad study population, which includes DCIS, and dosage, staging of the disease), is the phase 1 trial (NCT05680662) examining herbal titled “The Study of Quadruple Therapy Quercetin, Zinc, Metformin, and EGCG as Adjuvant Therapy for Early, Metastatic Breast Cancer, and Triple-negative Breast Cancer”. Quercetin, present in foods like kale, berries, onions, cherries, red grapes, broccoli, tea, and red wine, is traditionally used to prevent diseases such as osteoporosis, lung and cardiovascular diseases, and cancer. Epigallocatechin gallate (EGCG) is a polyphenol present in green tea has been tested for its chemopreventive activity (Du et al., 2012; Xu et al., 2019). Natural metabolites have been also tested in other contexts such as primary or secondary BC prevention and management of side effects. Epidiferphane, a claimed neuroregenerative dietary supplement (Steindler and Reynolds, 2017), is being evaluated alongside taxanes in BC to prevent peripheral neuropathy in the phase 1/2 NCT05074290 trial. Pentoxifylline, a synthetic xanthine derivative originally derived from coffee beans and tea leaves (Dastgheib et al., 2022). Unfortunately, most studies in this context are expected to yield negative results, as seen with soy protein for BC prevention in the NCT00204477 trial or PSC 833 (a cyclosporine derivative from the fungus Tolypocladium inflatum W. Gams 1971, Ophiocordycipitaceae) for multidrug-resistance inhibition in BC patients receiving taxanes (NCT00002826).
7.2 Trials regarding drugs’ nanoforms
Studies regarding nanoforms of existing medications and drugs that are active, but too toxic to be used on their own are costly and more likely to fail than traditional ones. What is more, the benefits of introducing drug nanoform are difficult to prove in pre-clinical studies. Nonetheless, that direction is constantly proving to be effective in terms of obtaining better treatment outcomes, diminishing toxicity, offers better solubility or a new route for administration (Püsküllüoğlu and Michalak, 2022). In a single arm phase 2 the PHENOMENAL study (NCT03328884) nanoliposomal irinotecan is being tested for central nervous system (CNS) ORR in HER-2 negative BC patients with brain metastases. This drug is also being evaluated in combination with pembrolizumab in TNBC brain metastatic population in phase 2 NCT05255666 trial with CNS Disease Control Rate (DCR) as the primary endpoint. While nanoforms of irinotecan are already approved for use in other indications such as pancreatic cancer (Wang-Gillam et al., 2019) docetaxel’s nanoforms have no registration as of now. In phase 3 NCT03671044 study, nanosomal docetaxel lipid suspension and a standard docetaxel are compared for ORRs in later lines of palliative TNBC treatment. Paclitaxel’s nanoforms have been approved for use in few indications including metastatic BC or pancreatic cancer (Samaan et al., 2019). In phase 3 NCT04137653 study, nab-paclitaxel is being tested in radical treatment of TNBC.
7.3 Trials regarding drugs linked to targeting part
Among potential benefits of ADC: selective toxicity, increased potency, reduced drug resistance can be named. The limitations in these trials are an industry-sponsored bias and a risk of setting endpoints that is not optimal, but easier to achieve. Additionally, endocrine-independent HER-2-negative breast cancer lacks specific therapeutic targets tailored to this subtype, potentially limiting the efficacy of tested ADCs. Patritumab Deruxtecan is a camptothecin derivative linked to anti-HER-3 mAb. In the phase 2 NCT04699630 trial, ORR and 6-month PFS are being assessed in metastatic BC including two arms for HER-2 negative endocrine-resistant patients. In another phase 2 NCT04965766 trial, the same drug is being tested for ORR in the metastatic HER-2 negative endocrine-resistant BC population. The phase 3 ANGLeD trial (NCT03613181) covers HER-2 negative metastatic BC patients with leptomeningeal disease and extremely unfavorable prognosis. Paclitaxel trevatide (paclitaxel-Angiopep-2 conjugate) is compared to other agents (including eribulin mesylate) to check for OS. In the phase 2 NCT04742153 trial MR002, an anti-HER2 mAb conjugated MMAE is being assessed for ORR in HER-2 low metastatic BC. The phase 1/2 NCT04441099 trial assesses the dose and anti-tumor activity of anthracycline-based ADC targeting Receptor tyrosine kinase-like orphan receptor 1 in few indications including metastatic TNBC (for the phase 2 part). The TROPION-PanTumor02 phase 1/2 (NCT05460273) trial explores the anti-TROP2 mAb linked to deruxtecan ADC (Datopotamab- DXd) in terms of ORR in different BC cohorts.
7.4 Trials with currently approved agents
In number of trials, currently approved drugs are being tested to search for better administration scheme or set standards in their combination with other medicines or determine optimal therapy sequences. The main limitation is the retrospective observational design of these studies, driven by challenges in organizing randomized controlled trials (RCTs) for non-patented drugs, primarily due to financial constraints. Metronomic chemotherapy uses low doses of drugs at regular short intervals, with antiangiogenic and antineoplastic effects (Cazzaniga et al., 2021). For example, the phase 2 NCT03071926 trial explores the outcomes of metronomic pegylated doxorubicin in endocrine-resistant advanced BC, while phase 2 NCT05747326 study checks 1-year performance free survival (PFS) of capecitabine and vinorelbine in HER-2 negative BC. An example of study that tries to set the place for commonly used drugs in TNBC radical treatment setting is phase 4 NCT04136782 trial with carboplatin, nab-paclitaxel, epirubicin and docetaxel.
Among the studies exploring new combinations of known drugs, there are numerous examples: the KEYDOX phase 1/2 trial (NCT03591276) studies the efficacy–objective response rate (ORR) and safety of pembrolizumab (an anti-PD-1 mAb) with pegylated liposomal doxorubicin in endocrine-resistant BC. In NCT04039230 phase 1/2 study SG is tested in combination with talazoparib (a PARP inhibitor) in metastatic TNBC. The same ADC is tested together with PI3K inhibitor alpelisib in metastatic, pretreated HER-2 negative BC in the phase 1 ASSET trial (NCT05143229), and other ADC T-DXd is checked for its safety when administered with nivolumab (an anti-PD1 mAb) in phase 1 NCT03523572 study including patients with BC.
Improving clinical trials involving natural-source medications is crucial due to challenges like lack of standardization and limited access to natural sources. Ambiguity in drug components and solvents can hinder data reproducibility and pose drug interaction risks. Addressing these issues through rigorous quality control, standardized protocols, and drug characterization is imperative for more reliable and effective clinical trial outcomes. Improving clinical trials for nanoforms of existing medications and toxic drugs requires addressing cost challenges and optimizing pre-clinical data to enhance the chances of developing safe and effective nanoform treatments. Enhancing clinical studies for drugs composed of natural-source-derived metabolites attached to targeting part requires addressing industry-sponsored bias and carefully selecting clinically relevant endpoints. Transparent trial design, independent oversight, and adaptive approaches can enhance scientific validity and yield more reliable results. Collaborative funding, innovative trial designs, and real-world data utilization are potential approaches to address limitations in clinical trials for currently approved drugs when searching for better therapy sequence, administration scheme or drugs’ combinations.
8 Future directions
Endocrine-independent HER-2-negative breast cancer is a condition known for its unfavorable treatment outcomes. Despite the introduction of innovative therapies like immune checkpoint inhibitors, the improvement in patients’ survival remains unsatisfactory. As a result, chemotherapy, primarily based on naturally-derived metabolites, remains the cornerstone of treatment. There are numerous novel natural agents with suggested pre-clinical and currently tested clinical activity in this BC subtype.
• Pre-clinical in vitro and in vivo models used to assess efficacy of the tested metabolites are far from perfection. The issue lies in the usage of archaic models, such as patient-derived cell lines. Additionally, it would be an overstatement to consider currently available cell lines as good representatives for all TNBCs or for endocrine-resistant HER-2-negative BCs. Regarding TNBC, data are primarily based on 1 cell line, MDA-MB-231. Cell lines obtained from endocrine-pretreated samples also do not adequately reflect numerous possible clinical scenarios. It is essential to employ contemporary pre-clinical models to accurately study the mechanisms, especially in the context of tumor microenvironment and therapeutic effects of naturally-derived agents. Advanced three-dimensional cell culture models or organoid cultures offer relevant platforms for examining how these agents interact with the organism and how they affect disease progression. These advanced models closely mimic the tumor microenvironment and provide a more precise reflection of how individual patients may respond to these treatments.
• Defining the composition of medications, especially if they are mixtures of different ingredients, including the material in which they are dissolved. Some traditional medications even when tested in clinical trials have no clear definition of their components. this can result not only in difficult pre-clinical or clinical data reproducibility, but also generates a risk of drug interactions. Such risk is very high in cancer patients due to the numerous other agents they take, including other anticancer treatment, side effects prevention and treatment, comorbidities treatment or supplements intake (van Leeuwen et al., 2011).
• Biomarkers are quantifiable indicators, including genetic mutations or other molecular features, which furnish valuable insights into the treatment response of a disease. Biomarkers aid in identifying disease subtypes and forecasting individual patient responses to particular naturally-derived therapies. Their pivotal role lies in customizing personalized treatment approaches, empowering healthcare providers to make well-informed decisions and enhance therapeutic outcomes. Investigating predictive factors for new anticancer drugs enhances treatment effectiveness. In pre-clinical research, biomarkers help assess treatment effectiveness and safety in cell and animal studies. For instance, monitoring changes in tumor growth-related biomarkers provides insights into the agents’ mechanisms of action. Each successful pre-clinical concept should be followed by clinical trials, preferably incorporating biomarker analysis in the trial design or planning additional trials to explore specific patients’ groups, rather than conducting post-hoc analyses. The final stage should involve validating biomarkers/predictive factors through appropriate tests applicable in clinical practice (Hughes et al., 2011; Califf, 2018; Berdigaliyev and Aljofan, 2020; Choudhari et al., 2020; Kaushik et al., 2021). Currently, the available treatment options for endocrine-independent, HER-2-negative breast cancer, except for immune checkpoint inhibitors in metastatic TNBC and HER-2 low status for T-DXd lack validated predictive factors similar to those for anti-HER2 treatment. The absence of specific therapeutic targets for this breast cancer subtype may limit the efficacy, even if an agent is associated with targeting mechanism.
• Performing clinical trials and patenting natural metabolites with anticancer activity requires extensive and time-consuming research. Numerous trials are ongoing, but successful studies in this area are relatively few, and patenting issues can further complicate financing for costly clinical trials (Sahoo et al., 2022). Therefore, we advocate for the promotion of increased support for non-sponsored trials.
In summary, current treatment options offer insufficient outcomes for patients with endocrine-independent HER-2-negative breast cancer. There are favorable prospects for the advancement of new naturally-derived drugs in this setting. Nature is still an inexhaustible source of medications, their prototypes, as well as an inspiration for medicines created in the laboratory. Naturally-derived metabolites that have already been rejected due to solubility issues or toxicity profile can come back into play by using new methods of their targeting and delivery such as ADCs or creating their nanoforms. Furthermore, certain studies have shown the synergistic impacts of combining naturally-derived agents with standard therapies, suggesting new approaches to overcome drug resistance and improve treatment outcomes in this complex subgroup of breast cancer. Considerable efforts are yet to be undertaken in unraveling the intricacies of how effectively bring naturally-derived metabolites from bench to bedside.
Author contributions
MP: Conceptualization, Writing–original draft, Writing–review and editing. IM: Conceptualization, Writing–original draft, Writing–review and editing.
Funding
The author(s) declare that no financial support was received for the research, authorship, and/or publication of this article.
Conflict of interest
MP reports a relationship with Gilead, AstraZeneca, Roche, Janssen, Pfizer, Amgen that includes: speaking and lecture fees and travel reimbursement.
The remaining author declares that the research was conducted in the absence of any commercial or financial relationships that could be construed as a potential conflict of interest.
Publisher’s note
All claims expressed in this article are solely those of the authors and do not necessarily represent those of their affiliated organizations, or those of the publisher, the editors and the reviewers. Any product that may be evaluated in this article, or claim that may be made by its manufacturer, is not guaranteed or endorsed by the publisher.
Supplementary material
The Supplementary Material for this article can be found online at: https://www.frontiersin.org/articles/10.3389/fphar.2024.1349242/full#supplementary-material
References
Alamgir, A. N. M. (2018). Phytoconstituents—active and inert constituents, metabolic pathways, chemistry and application of phytoconstituents, primary metabolic products, and bioactive compounds of primary metabolic origin. Prog. Drug Res. 74, 25–164. doi:10.1007/978-3-319-92387-1_2
Aloss, K., and Hamar, P. (2023). Recent preclinical and clinical progress in liposomal doxorubicin. Pharmaceutics 15, 893. doi:10.3390/PHARMACEUTICS15030893
Alsaraf, K. M., Mohammad, M. H., Al-Shammari, A. M., and Abbas, I. S. (2019). Selective cytotoxic effect of Plantago lanceolata L. against breast cancer cells. J. Egypt Natl. Canc Inst. 31, 10–17. doi:10.1186/s43046-019-0010-3
André, F., Ciruelos, E., Rubovszky, G., Campone, M., Loibl, S., Rugo, H. S., et al. (2019). Alpelisib for PIK3CA-mutated, hormone receptor-positive advanced breast cancer. N. Engl. J. Med. 380, 1929–1940. doi:10.1056/NEJMOA1813904
Avila-Carrasco, L., Majano, P., Sánchez-Toméro, J. A., Selgas, R., López-Cabrera, M., Aguilera, A., et al. (2019). Natural plants compounds as modulators of epithelial-to-mesenchymal transition. Front. Pharmacol. 10, 715. doi:10.3389/fphar.2019.00715
Barbieri, A., Quagliariello, V., Del Vecchio, V., Falco, M., Luciano, A., Amruthraj, N. J., et al. (2017). Anticancer and anti-inflammatory properties of Ganoderma lucidum extract effects on melanoma and triple-negative breast cancer treatment. Nutrients 9, 210. doi:10.3390/NU9030210
Bardia, A., Hurvitz, S. A., Tolaney, S. M., Loirat, D., Punie, K., Oliveira, M., et al. (2021). Sacituzumab govitecan in metastatic triple-negative breast cancer. N. Engl. J. Med. 384, 1529–1541. doi:10.1056/NEJMOA2028485
Berdigaliyev, N., and Aljofan, M. (2020). An overview of drug discovery and development. Future Med. Chem. 12, 939–947. doi:10.4155/fmc-2019-0307
Califf, R. M. (2018). Biomarker definitions and their applications. Exp. Biol. Med. 243, 213–221. doi:10.1177/1535370217750088
Cao, X., Li, Y., Wang, Y., Yu, T., Zhu, C., Zhang, X., et al. (2022). Curcumin suppresses tumorigenesis by ferroptosis in breast cancer. PLoS One 17, e0261370. doi:10.1371/JOURNAL.PONE.0261370
Cardoso, F., Paluch-Shimon, S., Senkus, E., Curigliano, G., Aapro, M. S., André, F., et al. (2020). 5th ESO-ESMO international consensus guidelines for advanced breast cancer (ABC 5). Ann. Oncol. 31, 1623–1649. doi:10.1016/J.ANNONC.2020.09.010
Caswell-Jin, J. L., Plevritis, S. K., Tian, L., Cadham, C. J., Xu, C., Stout, N. K., et al. (2018). Change in survival in metastatic breast cancer with treatment advances: meta-analysis and systematic review. JNCI Cancer Spectr. 2, pky062. doi:10.1093/JNCICS/PKY062
Cazzaniga, M. E., Cordani, N., Capici, S., Cogliati, V., Riva, F., and Cerrito, M. G. (2021). Metronomic chemotherapy. Cancers (Basel) 13, 2236. doi:10.3390/CANCERS13092236
Chang, C. T., Korivi, M., Huang, H. C., Thiyagarajan, V., Lin, K. Y., Huang, P. J., et al. (2017). Inhibition of ROS production, autophagy or apoptosis signaling reversed the anticancer properties of Antrodia salmonea in triple-negative breast cancer (MDA-MB-231) cells. Food Chem. Toxicol. 103, 1–17. doi:10.1016/J.FCT.2017.02.019
Chen, L. M., Yang, P. P., Al Haq, A. T., Hwang, P. A., Lai, Y. C., Weng, Y. S., et al. (2022). Oligo-Fucoidan supplementation enhances the effect of Olaparib on preventing metastasis and recurrence of triple-negative breast cancer in mice. J. Biomed. Sci. 29, 70. doi:10.1186/S12929-022-00855-6
Cheng, G. J., Leung, E. Y., and Singleton, D. C. (2022). In vitro breast cancer models for studying mechanisms of resistance to endocrine therapy. Explor Target Antitumor Ther. 3, 297–320. doi:10.37349/ETAT.2022.00084
Cheng, X., Tan, S., Duan, F., Yuan, Q., Li, Q., and Deng, G. (2019). Icariin induces apoptosis by suppressing autophagy in tamoxifen-resistant breast cancer cell line MCF-7/TAM. Breast Cancer 26, 766–775. doi:10.1007/S12282-019-00980-5
Choudhari, A. S., Mandave, P. C., Deshpande, M., Ranjekar, P., and Prakash, O. (2020). Phytochemicals in cancer treatment: from preclinical studies to clinical practice. Front. Pharmacol. 10, 1614. doi:10.3389/fphar.2019.01614
Collard, M., Gallagher, P. E., and Tallant, E. A. (2020). A polyphenol-rich extract from muscadine grapes inhibits triple-negative breast tumor growth. Integr. Cancer Ther. 19, 1534735420917444. doi:10.1177/1534735420917444
Cortes, J., Cescon, D. W., Rugo, H. S., Nowecki, Z., Im, S. A., Yusof, M. M., et al. (2020). Pembrolizumab plus chemotherapy versus placebo plus chemotherapy for previously untreated locally recurrent inoperable or metastatic triple-negative breast cancer (KEYNOTE-355): a randomised, placebo-controlled, double-blind, phase 3 clinical trial. Lancet 396, 1817–1828. doi:10.1016/S0140-6736(20)32531-9
Corti, C., Giugliano, F., Nicolò, E., Ascione, L., and Curigliano, G. (2021). Antibody–drug conjugates for the treatment of breast cancer. Cancers (Basel) 13, 2898. doi:10.3390/CANCERS13122898
Cumaoglu, A., Bekci, H., Ozturk, E., Yerer, M. B., Baldemir, A., and Bishayee, A. (2018). Goji berry fruit extracts suppress proliferation of triple-negative breast cancer cells by inhibiting EGFR-mediated ERK/MAPK and PI3K/Akt signaling pathways. Nat. Prod. Commun. 13, 1934578X1801300. doi:10.1177/1934578X1801300613
Dastgheib, M., Shetab-Boushehri, S. V., Baeeri, M., Gholami, M., Karimi, M. Y., and Hosseini, A. (2022). Rolipram and pentoxifylline combination ameliorates experimental diabetic neuropathy through inhibition of oxidative stress and inflammatory pathways in the dorsal root ganglion neurons. Metab. Brain Dis. 37, 2615–2627. doi:10.1007/S11011-022-01060-Y
Deng, X. X., Jiao, Y. N., Hao, H. F., Xue, D., Bai, C. C., and Han, S. Y. (2021). Taraxacum mongolicum extract inhibited malignant phenotype of triple-negative breast cancer cells in tumor-associated macrophages microenvironment through suppressing IL-10/STAT3/PD-L1 signaling pathways. J. Ethnopharmacol. 274, 113978. doi:10.1016/J.JEP.2021.113978
Ding, T., Yang, L. J., Zhang, W. D., and Shen, Y. H. (2020). Pyoluteorin induces cell cycle arrest and apoptosis in human triple-negative breast cancer cells MDA-MB-231. J. Pharm. Pharmacol. 72, 969–978. doi:10.1111/JPHP.13262
Dogra, A., and Kumar, J. (2023). Biosynthesis of anticancer phytochemical compounds and their chemistry. Front. Pharmacol. 14, 1136779. doi:10.3389/fphar.2023.1136779
Du, G. J., Zhang, Z., Wen, X. D., Yu, C., Calway, T., Yuan, C. S., et al. (2012). Epigallocatechin Gallate (EGCG) is the most effective cancer chemopreventive polyphenol in green tea. Nutrients 4, 1679–1691. doi:10.3390/NU4111679
ER-positive HER2-negative, Breast Cancer (2023). ESMO metastatic breast cancer living guideline. Available at: https://www.esmo.org/living-guidelines/esmo-metastatic-breast-cancer-living-guideline/er-positive-her2-negative-breast-cancer (Accessed September 28, 2023).
Fouzat, A., Hussein, O. J., Gupta, I., Al-Farsi, H. F., Khalil, A., and Al Moustafa, A. E. (2022). Elaeagnus angustifolia plant extract induces apoptosis via P53 and signal transducer and activator of transcription 3 signaling pathways in triple-negative breast cancer cells. Front. Nutr. 9, 418. doi:10.3389/fnut.2022.871667
Gnant, M., Curigliano, G., Senn, H., Thürlimann, B., and Weber, W. P. (2022). Customizing early breast cancer therapies - the 2021 St. Gallen international breast cancer consensus conference. Breast 62 (1), S2. doi:10.1016/J.BREAST.2022.03.009
Guerra, Â. R., Soares, B. I. G., Freire, C. S. R., Silvestre, A. J. D., Duarte, M. F., and Duarte, I. F. (2021). Metabolic effects of a Eucalyptus bark lipophilic extract on triple negative breast cancer and nontumor breast epithelial cells. J. Proteome Res. 20, 565–575. doi:10.1021/ACS.JPROTEOME.0C00559
Guo, Y., and Pei, X. (2019). Tetrandrine-induced autophagy in MDA-MB-231 triple-negative breast cancer cell through the inhibition of PI3K/AKT/mTOR signaling. Evid. Based Complement. Altern. Med. 2019, 7517431. doi:10.1155/2019/7517431
Harikrishnan, A., Veena, V., Lakshmi, B., Shanmugavalli, R., Theres, S., Prashantha, C. N., et al. (2021). Atranorin, an antimicrobial metabolite from lichen Parmotrema rampoddense exhibited in vitro anti-breast cancer activity through interaction with Akt activity. J. Biomol. Struct. Dyn. 39, 1248–1258. doi:10.1080/07391102.2020.1734482
Hassan, M. A.-K., and Ates-Alagoz, Z. (2023). Cyclin-dependent kinase 4/6 inhibitors against breast cancer. Mini Rev. Med. Chem. 23, 412–428. doi:10.2174/1389557522666220606095540
He, Y., Wan, Y., Zhou, Y., Cai, X., Guo, Z., Hu, F., et al. (2022). Two new alliacane sesquiterpenes from the fruiting bodies of edible mushroom Gomphus purpuraceus. Phytochem. Lett. 50, 85–88. doi:10.1016/J.PHYTOL.2022.06.002
Heinrich, M., Jalil, B., Abdel-Tawab, M., Echeverria, J., Kulić, Ž., McGaw, L. J., et al. (2022). Best Practice in the chemical characterisation of extracts used in pharmacological and toxicological research-The ConPhyMP-Guidelines. Front. Pharmacol. 13, 953205. doi:10.3389/FPHAR.2022.953205
Hennigs, A., Riedel, F., Gondos, A., Sinn, P., Schirmacher, P., Marmé, F., et al. (2016). Prognosis of breast cancer molecular subtypes in routine clinical care: a large prospective cohort study. BMC Cancer 16, 734. doi:10.1186/S12885-016-2766-3
Ho, Y., Suphrom, N., Daowtak, K., Potup, P., Thongsri, Y., and Usuwanthim, K. (2020). Anticancer effect of Citrus hystrix DC. Leaf extract and its bioactive constituents citronellol and, citronellal on the triple negative breast cancer MDA-MB-231 cell line. Pharmaceuticals 13, 476–517. doi:10.3390/PH13120476
Hsu, W. J., Lin, M. H., Kuo, T. C., Chou, C. M., Mi, F. L., Cheng, C. H., et al. (2020). Fucoidan from Laminaria japonica exerts antitumor effects on angiogenesis and micrometastasis in triple-negative breast cancer cells. Int. J. Biol. Macromol. 149, 600–608. doi:10.1016/J.IJBIOMAC.2020.01.256
Hughes, J. P., Rees, S. S., Kalindjian, S. B., and Philpott, K. L. (2011). Principles of early drug discovery. Br. J. Pharmacol. 162, 1239–1249. doi:10.1111/J.1476-5381.2010.01127.X
Ibrahim, N. K. (2021). Ixabepilone: overview of effectiveness, safety, and tolerability in metastatic breast cancer. Front. Oncol. 11, 617874. doi:10.3389/FONC.2021.617874
Jaglanian, A., and Tsiani, E. (2020). Rosemary extract inhibits proliferation, survival, Akt, and mTOR signaling in triple-negative breast cancer cells. Int. J. Mol. Sci. 21, 810. doi:10.3390/IJMS21030810
Juyal, D., Thawani, V., Thaledi, S., and Joshi, M. (2014). Ethnomedical properties of Taxus wallichiana Zucc. (Himalayan yew). J. Tradit. Complement. Med. 4, 159–161. doi:10.4103/2225-4110.136544
Kalebar, V. U., Hoskeri, J. H., Hiremath, S. V., and Hiremath, M. B. (2020). In vitro antiproliferative effect of aqueous extract of Solanum macranthum fruits on MDA-MB-231 tripple negative breast cancer cell line. J. Appl. Biol. Biotechnol. 8, 28–32. doi:10.7324/JABB.2020.80105
Kamble, P., Cheriyamundath, S., Lopus, M., and Sirisha, V. L. (2018). Chemical characteristics, antioxidant and anticancer potential of sulfated polysaccharides from Chlamydomonas reinhardtii. J. Appl. Phycol. 30, 1641–1653. doi:10.1007/S10811-018-1397-2
Kaushik, I., Ramachandran, S., Prasad, S., and Srivastava, S. K. (2021). Drug rechanneling: a novel paradigm for cancer treatment. Semin. Cancer Biol. 68, 279–290. doi:10.1016/j.semcancer.2020.03.011
Kciuk, M., Gielecińska, A., Mujwar, S., Kołat, D., Kałuzińska-Kołat, Ż., Celik, I., et al. (2023). Doxorubicin—an agent with multiple mechanisms of anticancer activity. Cells 12, 659. doi:10.3390/CELLS12040659
Ke, D. Y. J., El-Sahli, S., and Wang, L. (2022). The potential of natural products in the treatment of triple-negative breast cancer. Curr. Cancer Drug Targets 22, 388–403. doi:10.2174/1568009622666211231140623
Kim, S., You, D., Jeong, Y., Yu, J., Kim, S. W., Nam, S. J., et al. (2018). Berberine down-regulates IL-8 expression through inhibition of the EGFR/MEK/ERK pathway in triple-negative breast cancer cells. Phytomedicine 50, 43–49. doi:10.1016/J.PHYMED.2018.08.004
Kombiyil, S., and Sivasithamparam, N. D. (2023). In vitro anti-cancer effect of Crataegus oxyacantha berry extract on hormone receptor positive and triple negative breast cancers via regulation of canonical Wnt signaling pathway. Appl. Biochem. Biotechnol. 195, 2687–2708. doi:10.1007/S12010-021-03724-4
Kumar, S., Singh, B., and Singh, R. (2022). Catharanthus roseus (L.) G. Don: a review of its ethnobotany, phytochemistry, ethnopharmacology and toxicities. J. Ethnopharmacol. 284, 114647. doi:10.1016/J.JEP.2021.114647
Lee, M. M. L., Chan, B. D., Wong, W. Y., Qu, Z., Chan, M. S., Leung, T. W., et al. (2020). Anti-cancer activity of Centipeda minima extract in triple negative breast cancer via inhibition of AKT, NF-κB, and STAT3 signaling pathways. Front. Oncol. 10, 491. doi:10.3389/FONC.2020.00491
Li, C., Chen, S.-C., Chen, Y., Girish, S., Kågedal, M., Lu, D., et al. (2020). Impact of physiologically based pharmacokinetics, population pharmacokinetics and pharmacokinetics/pharmacodynamics in the development of antibody-drug conjugates. Suppl. Article J. Clin. Pharmacol. 2020, S105–S119. doi:10.1002/jcph.1720
Li, L., Cao, J., Chen, C., Qin, Y., He, L., Gu, H., et al. (2023). Antitumor effect of a novel humanized MUC1 antibody-drug conjugate on triple-negative breast cancer. Heliyon 9, e15164. doi:10.1016/J.HELIYON.2023.E15164
Liang, Z. J., Wan, Y., Zhu, D. D., Wang, M. X., Jiang, H. M., Huang, D. L., et al. (2021). Resveratrol mediates the apoptosis of triple negative breast cancer cells by reducing POLD1 expression. Front. Oncol. 11, 569295. doi:10.3389/fonc.2021.569295
Lin, C. J., Chen, J. T., Yeh, L. J., Yang, R. C., Huang, S. M., and Chen, T. W. (2022). Characteristics of the cytotoxicity of Taraxacum mongolicum and Taraxacum formosanum in human breast cancer cells. Int. J. Mol. Sci. 23, 11918. doi:10.3390/IJMS231911918
Lin, Y. S., Chiu, Y. C., Tsai, Y. H., Tsai, Y. F., Wang, J. Y., Tseng, L. M., et al. (2019). Different mechanisms involved in the berberine-induced antiproliferation effects in triple-negative breast cancer cell lines. J. Cell Biochem. 120, 13531–13544. doi:10.1002/JCB.28628
Liu, H. C., Chiang, C. C., Lin, C. H., Chen, C. S., Wei, C. W., Lin, S. Y., et al. (2020). Anti-cancer therapeutic benefit of red guava extracts as a potential therapy in combination with doxorubicin or targeted therapy for triple-negative breast cancer cells. Int. J. Med. Sci. 17, 1015–1022. doi:10.7150/IJMS.40131
Luo, H., Zhao, L., Li, Y., Xia, B., Lin, Y., Xie, J., et al. (2022). An in vivo and in vitro assessment of the anti-breast cancer activity of crude extract and fractions from Prunella vulgaris L. Heliyon 8, e11183. doi:10.1016/J.HELIYON.2022.E11183
Lv, C., Huang, Y., Huang, R., Wang, Q., Zhang, H., Jin, J., et al. (2022). Narciclasine targets STAT3 via distinct mechanisms in tamoxifen-resistant breast cancer cells. Mol. Ther. Oncolytics 24, 340–354. doi:10.1016/J.OMTO.2021.12.025
Markhulia, J., Kekutia, S., Mikelashvili, V., Saneblidze, L., Tsertsvadze, T., Maisuradze, N., et al. (2023). Synthesis, characterization, and in vitro cytotoxicity evaluation of doxorubicin-loaded magnetite nanoparticles on triple-negative breast cancer cell lines. Pharmaceutics 15, 1758. doi:10.3390/PHARMACEUTICS15061758
Marra, A., Trapani, D., Viale, G., Criscitiello, C., and Curigliano, G. (2020). Practical classification of triple-negative breast cancer: intratumoral heterogeneity, mechanisms of drug resistance, and novel therapies. NPJ Breast Cancer 6, 54. doi:10.1038/S41523-020-00197-2
Maungchanburi, S., Rattanaburee, T., Sukpondma, Y., Tedasen, A., Tipmanee, V., and Graidist, P. (2021). Anticancer activity of Piper cubeba L. extract on triple negative breast cancer MDA-MB-231. J. Pharm. Pharmacogn. Res. 10, 39–51. doi:10.56499/jppres21.1160_10.1.39
Mazzei, R., Piacentini, E., Nardi, M., Poerio, T., Bazzarelli, F., Procopio, A., et al. (2020). Production of plant-derived oleuropein aglycone by a combined membrane process and evaluation of its breast anticancer properties. Front. Bioeng. Biotechnol. 8, 908. doi:10.3389/fbioe.2020.00908
Mendonca, P., Darwish, A. G., Tsolova, V., El-Sharkawy, I., and Soliman, K. F. A. (2019). The anticancer and antioxidant effects of muscadine grape extracts on racially different triple-negative breast cancer cells. Anticancer Res. 39, 4043–4053. doi:10.21873/ANTICANRES.13560
Michalak, I., and Püsküllüoğlu, M. (2022). Look into my onco-forest - review of plant natural products with anticancer activity. Curr. Top. Med. Chem. 22, 922–938. doi:10.2174/1568026622666220303112218
Miguel, R. D. A., Hirata, A. S., Jimenez, P. C., Lopes, L. B., and Costa-Lotufo, L. V. (2022). Beyond formulation: contributions of nanotechnology for translation of anticancer natural products into new drugs. Pharmaceutics 14, 1722. doi:10.3390/PHARMACEUTICS14081722
Modi, S., Saura, C., Yamashita, T., Park, Y. H., Kim, S.-B., Tamura, K., et al. (2020). Trastuzumab deruxtecan in previously treated HER2-positive breast cancer. N. Engl. J. Med. 382, 610–621. doi:10.1056/NEJMOA1914510
Moy, B., Rumble, R. B., Come, S. E., Davidson, N. E., Di Leo, A., Gralow, J. R., et al. (2021). Chemotherapy and targeted therapy for patients with human epidermal growth factor receptor 2-negative metastatic breast cancer that is either endocrine-pretreated or hormone receptor-negative: ASCO guideline update. J. Clin. Oncol. 39, 3938–3958. doi:10.1200/JCO.21.01374
Narayanan, S., de Mores, A. R., Cohen, L., Anwar, M. M., Lazar, F., Hicklen, R., et al. (2023). Medicinal mushroom supplements in cancer: a systematic review of clinical studies. Curr. Oncol. Rep. 25, 569–587. doi:10.1007/S11912-023-01408-2
NCCN Clinical Practice Guidelines in Oncology (2023). NCCN clinical practice guidelines in Oncology (NCCN guidelines) version 4.2023 breast cancer. Available at: https://www.nccn.org (Accessed August 27, 2023).
Nguyen, C., Baskaran, K., Pupulin, A., Ruvinov, I., Zaitoon, O., Grewal, S., et al. (2019). Hibiscus flower extract selectively induces apoptosis in breast cancer cells and positively interacts with common chemotherapeutics. BMC Complement. Altern. Med. 19, 98. doi:10.1186/S12906-019-2505-9
Noh, J. I., Mun, S. K., Lim, E. H., Kim, H., Chang, D. J., Hur, J. S., et al. (2021). Induction of apoptosis in MDA-MB-231 cells treated with the methanol extract of lichen Physconia hokkaidensis. J. Fungi (Basel) 7, 188–210. doi:10.3390/JOF7030188
Ooka, M., Lynch, C., and Xia, M. (2020). Application of in vitro metabolism activation in high-throughput screening. Int. J. Mol. Sci. 21, 8182–8216. doi:10.3390/IJMS21218182
Pragna Lakshmi, T., Vajravijayan, S., Moumita, M., Sakthivel, N., Gunasekaran, K., and Krishna, R. (2017). A novel guaiane sesquiterpene derivative, guai-2-en-10α-ol, from Ulva fasciata Delile inhibits EGFR/PI3K/Akt signaling and induces cytotoxicity in triple-negative breast cancer cells. Mol. Cell Biochem. 1 (2), 123–139. doi:10.1007/S11010-017-3119-5
Pullaiah, T., and Raveendran, V. (2020). “Camptothecin: chemistry, biosynthesis, analogs, and chemical synthesis,” in Camptothecin and camptothecin producing plants: botany, chemistry, anticancer activity and biotechnology (London: Academic Press), 47–103. doi:10.1016/B978-0-12-820499-3.00002-1
Püsküllüoğlu, M., and Michalak, I. (2022). An ocean of possibilities: a review of marine organisms as sources of nanoparticles for cancer care. Nanomedicine (Lond) 17, 1695–1719. doi:10.2217/NNM-2022-0206
Püsküllüoğlu, M., Rudzińska, A., and Pacholczak-Madej, R. (2023). Antibody-drug conjugates in HER-2 negative breast cancers with poor prognosis. Biochim. Biophys. Acta Rev. Cancer 1878, 188991. doi:10.1016/j.bbcan.2023.188991
Qi, R. Y., Guo, C., Peng, X. N., and Tang, J. J. (2022). Sesquiterpenoids from Inula britannica and their potential effects against triple-negative breast cancer cells. Molecules 27, 5230. doi:10.3390/molecules27165230
Raman, V., Aryal, U. K., Hedrick, V., Ferreira, R. M., Fuentes Lorenzo, J. L., Stashenko, E. E., et al. (2018). Proteomic analysis Reveals that an extract of the plant Lippia origanoides suppresses mitochondrial metabolism in triple-negative breast cancer cells. J. Proteome Res. 17, 3370–3383. doi:10.1021/ACS.JPROTEOME.8B00255
Rugo, H. S., Bardia, A., Tolaney, S. M., Arteaga, C., Cortes, J., Sohn, J., et al. (2020). TROPiCS-02: a Phase III study investigating sacituzumab govitecan in the treatment of HR+/HER2-metastatic breast cancer. Future Oncol. 16, 705–715. doi:10.2217/FON-2020-0163
Sahoo, A., Mandal, A. K., Kumar, M., Dwivedi, K., and Singh, D. (2022). Prospective challenges for patenting and clinical trials of anticancer compounds from natural products: coherent review. Recent Pat. Anticancer Drug Discov. 18, 470–494. doi:10.2174/1574892818666221104113703
Samaan, T. M. A., Samec, M., Liskova, A., Kubatka, P., and Büsselberg, D. (2019). Paclitaxel’s mechanistic and clinical effects on breast cancer. Biomolecules 9, 789. doi:10.3390/BIOM9120789
Sancha, S. A. R., Gomes, A. V., Loureiro, J. B., Saraiva, L., and Ferreira, M. J. U. (2022). Amaryllidaceae-type alkaloids from Pancratium maritimum: apoptosis-inducing effect and cell cycle arrest on triple-negative breast cancer cells. Molecules 27, 5759. doi:10.3390/molecules27185759
Sánchez-Valdeolívar, C. A., Alvarez-Fitz, P., Zacapala-Gómez, A. E., Acevedo-Quiroz, M., Cayetano-Salazar, L., Olea-Flores, M., et al. (2020). Phytochemical profile and antiproliferative effect of Ficus crocata extracts on triple-negative breast cancer cells. BMC Complement. Med. Ther. 20, 191. doi:10.1186/S12906-020-02993-6
Sangpairoj, K., Settacomkul, R., Siangcham, T., Meemon, K., Niamnont, N., Sornkaew, N., et al. (2023). Hexadecanoic acid-enriched extract of Halymenia durvillei induces apoptotic and autophagic death of human triple-negative breast cancer cells by upregulating ER stress. Asian Pac J. Trop. Biomed. 12, 132. doi:10.4103/2221-1691.338922
Sekar, P., Ravitchandirane, R., Khanam, S., Muniraj, N., and Cassinadane, A. V. (2022). Novel molecules as the emerging trends in cancer treatment: an update. Med. Oncol. 39, 20. doi:10.1007/s12032-021-01615-6
Shah, Z., Gohar, U. F., Jamshed, I., Mushtaq, A., Mukhtar, H., Zia-Ui-haq, M., et al. (2021). Podophyllotoxin: history, recent advances and future prospects. Biomolecules 11, 603. doi:10.3390/BIOM11040603
Shi, X. P., Miao, S., Wu, Y., Zhang, W., Zhang, X. F., Ma, H. Z., et al. (2013). Resveratrol sensitizes tamoxifen in antiestrogen-resistant breast cancer cells with epithelial-mesenchymal transition features. Int. J. Mol. Sci. 14, 15655–15668. doi:10.3390/IJMS140815655
Shin, S. A., Lee, H. N., Choo, G. S., Kim, H. J., Che, J. H., and Jung, J. Y. (2017). Ixeris dentata (thunb. Ex thunb.) nakai extract inhibits proliferation and induces apoptosis in breast cancer cells through Akt/NF-κB pathways. Int. J. Mol. Sci. 18, 275. doi:10.3390/IJMS18020275
Siddiqui, A. J., Jahan, S., Singh, R., Saxena, J., Ashraf, S. A., Khan, A., et al. (2022). Plants in anticancer drug discovery: from molecular mechanism to chemoprevention. Biomed. Res. Int. 2022, 5425485. doi:10.1155/2022/5425485
Song, H., Liu, B., Dong, B., Xu, J., Zhou, H., Na, S., et al. (2021). Exosome-based delivery of natural products in cancer therapy. Front. Cell Dev. Biol. 9, 650426. doi:10.3389/FCELL.2021.650426
Soosainathan, A., Nikitorowicz-Buniak, J., Pancholi, S., Iravani, M., Alexander, J., Haider, S., et al. (2022). Abstract P4-02-05: exploiting novel models of endocrine-resistant breast cancer to identify new therapeutic targets. Cancer Res. 82, P4, 02-05. doi:10.1158/1538-7445.SABCS21-P4-02-05
Steindler, D. A., and Reynolds, B. A. (2017). Perspective: neuroregenerative nutrition. Adv. Nutr. 8, 546–557. doi:10.3945/AN.117.015388
Suarez-Arroyo, I. J., and Martinez-Montemayor, M. M. (2018). Abstract 2815: Ganoderma lucidum chemosensitizes triple negative breast cancer to carboplatin mediated by DNA damage response. Cancer Res. 78, 2815. doi:10.1158/1538-7445.AM2018-2815
Sun, G., Rong, D., Li, Z., Sun, G., Wu, F., Li, X., et al. (2021). Role of small molecule targeted compounds in cancer: progress, opportunities, and challenges. Front. Cell Dev. Biol. 9, 2043. doi:10.3389/fcell.2021.694363
Sung, H., Ferlay, J., Siegel, R. L., Laversanne, M., Soerjomataram, I., Jemal, A., et al. (2021). Global cancer statistics 2020: GLOBOCAN estimates of incidence and mortality worldwide for 36 cancers in 185 countries. CA Cancer J. Clin. 71, 209–249. doi:10.3322/caac.21660
Syed Najmuddin, S. U. F., Romli, M. F., Hamid, M., Alitheen, N. B., and Abd Rahman, N. M. A. N. (2016). Anti-cancer effect of Annona muricata Linn leaves crude extract (AMCE) on breast cancer cell line. BMC Complement. Altern. Med. 16, 311. doi:10.1186/S12906-016-1290-Y
Thilagavathi, R., Priyankha, S., Kannan, M., Prakash, M., and Selvam, C. (2023). Compounds from diverse natural origin against triple-negative breast cancer: a comprehensive review. Chem. Biol. Drug Des. 101, 218–243. doi:10.1111/CBDD.14172
Triple-negative Breast Cancer (2023). Triple-negative breast cancer, ESMO. Available at: https://www.esmo.org/living-guidelines/esmo-metastatic-breast-cancer-living-guideline/triple-negative-breast-cancer (Accessed September 28, 2023).
Utage, B. G., Shivajirao, P. M., Vasudeo, N. P., Shankar, K. S., and Nivarti, G. R. (2018). Prosopis juliflora (Sw.), DC induces apoptosis and cell cycle arrest in triple negative breast cancer cells: in vitro and in vivo investigations. Oncotarget 9, 30304–30323. doi:10.18632/ONCOTARGET.25717
van Leeuwen, R. W. F., Swart, E. L., Boven, E., Boom, F. A., Schuitenmaker, M. G., and Hugtenburg, J. G. (2011). Potential drug interactions in cancer therapy: a prevalence study using an advanced screening method. Ann. Oncol. 22, 2334–2341. doi:10.1093/ANNONC/MDQ761
Wagner, K. U. (2022). Know thy cells: commonly used triple-negative human breast cancer cell lines carry mutations in RAS and effectors. Breast Cancer Res. 24, 44–52. doi:10.1186/s13058-022-01538-8
Wang, P., Song, D., Wan, D., Li, L., Mei, W., Li, X., et al. (2020). Ginsenoside panaxatriol reverses TNBC paclitaxel resistance by inhibiting the IRAK1/NF-κB and ERK pathways. PeerJ 8, e9281. doi:10.7717/PEERJ.9281
Wang, Y., Yue, W., Lang, H., Ding, X., Chen, X., and Chen, H. (2021). Resuming sensitivity of tamoxifen-resistant breast cancer cells to tamoxifen by tetrandrine. Integr. Cancer Ther. 20, 1534735421996822. doi:10.1177/1534735421996822
Wang, Z., Li, H., Gou, L., Li, W., and Wang, Y. (2023). Antibody–drug conjugates: recent advances in payloads. Acta Pharm. Sin. B 13, 4025–4059. doi:10.1016/J.APSB.2023.06.015
Wang-Gillam, A., Hubner, R. A., Siveke, J. T., Von Hoff, D. D., Belanger, B., de Jong, F. A., et al. (2019). NAPOLI-1 phase 3 study of liposomal irinotecan in metastatic pancreatic cancer: final overall survival analysis and characteristics of long-term survivors. Eur. J. Cancer 108, 78–87. doi:10.1016/j.ejca.2018.12.007
Wilusz, M., and Majka, M. (2008). Role of the Wnt/beta-catenin network in regulating hematopoiesis. Arch. Immunol. Ther. Exp. Warsz. 56, 257–266. doi:10.1007/s00005-008-0029-y
Wynn, C. S., and Tang, S. C. (2022). Anti-HER2 therapy in metastatic breast cancer: many choices and future directions. Cancer Metastasis Rev. 41, 193–209. doi:10.1007/S10555-022-10021-X
Xie, Y., Jiang, Z., Yang, R., Ye, Y., Pei, L., Xiong, S., et al. (2021). Polysaccharide-rich extract from Polygonatum sibiricum protects hematopoiesis in bone marrow suppressed by triple negative breast cancer. Biomed. Pharmacother. 137, 111338. doi:10.1016/J.BIOPHA.2021.111338
Xu, B., Sun, T., Zhang, Q., Zhang, P., Yuan, Z., Jiang, Z., et al. (2021a). Efficacy of utidelone plus capecitabine versus capecitabine for heavily pretreated, anthracycline- and taxane-refractory metastatic breast cancer: final analysis of overall survival in a phase III randomised controlled trial. Ann. Oncol. 32, 218–228. doi:10.1016/J.ANNONC.2020.10.600
Xu, D., Hu, M. J., Wang, Y. Q., and Cui, Y. L. (2019). Antioxidant activities of quercetin and its complexes for medicinal application. Molecules 24, 1123. doi:10.3390/MOLECULES24061123
Xu, H., Hu, M., Liu, M., An, S., Guan, K., Wang, M., et al. (2020). Nano-puerarin regulates tumor microenvironment and facilitates chemo- and immunotherapy in murine triple negative breast cancer model. Biomaterials 235, 119769. doi:10.1016/J.BIOMATERIALS.2020.119769
Xu, Y., Shrestha, N., Préat, V., and Beloqui, A. (2021b). An overview of in vitro, ex vivo and in vivo models for studying the transport of drugs across intestinal barriers. Adv. Drug Deliv. Rev. 175, 113795. doi:10.1016/J.ADDR.2021.05.005
Yadav, J., El Hassani, M., Sodhi, J., Lauschke, V. M., Hartman, J. H., and Russell, L. E. (2021). Recent developments in in vitro and in vivo models for improved translation of preclinical pharmacokinetics and pharmacodynamics data. Drug Metab. Rev. 53, 207–233. doi:10.1080/03602532.2021.1922435
Yang, Z., Zhang, Q., Yu, L., Zhu, J., Cao, Y., and Gao, X. (2021). The signaling pathways and targets of traditional Chinese medicine and natural medicine in triple-negative breast cancer. J. Ethnopharmacol. 264, 113249. doi:10.1016/J.JEP.2020.113249
Yap, K. M., Sekar, M., Fuloria, S., Wu, Y. S., Gan, S. H., Rani, N. N. I. M., et al. (2021). Drug delivery of natural products through nanocarriers for effective breast cancer therapy: a comprehensive review of literature. Int. J. Nanomedicine 16, 7891–7941. doi:10.2147/IJN.S328135
Yi, X., Lou, L., Wang, J., Xiong, J., and Zhou, S. (2021). Honokiol antagonizes doxorubicin resistance in human breast cancer via miR-188-5p/FBXW7/c-Myc pathway. Cancer Chemother. Pharmacol. 87, 647–656. doi:10.1007/S00280-021-04238-W
Youssef, D. T. A., Shaala, L. A., and Altyar, A. E. (2022). Cytotoxic phenylpropanoid derivatives and alkaloids from the flowers of Pancratium maritimum L. Plants 11, 476. doi:10.3390/plants11040476
Zhang, Z., Sun, C., Zhang, L., Chi, X., Ji, J., Gao, X., et al. (2019). Triptolide interferes with XRCC1/PARP1-mediated DNA repair and confers sensitization of triple-negative breast cancer cells to cisplatin. Biomed. Pharmacother. 109, 1541–1546. doi:10.1016/J.BIOPHA.2018.11.008
Zhao, L., Zhang, C., and Nazim, M. (2020). Berberine inhibits MDA-MB-231 cells by attenuating their inflammatory responses. Biomed. Res. Int. 2020, 3617514. doi:10.1155/2020/3617514
Zhao, W., Liu, J., Li, Y., Chen, Z., Qi, D., and Zhang, Z. (2021). Immune effect of active components of traditional Chinese medicine on triple-negative breast cancer. Front. Pharmacol. 12, 731741. doi:10.3389/FPHAR.2021.731741
Zunica, E. R. M., Yang, S., Coulter, A., White, C., Kirwan, J. P., and Gilmore, L. A. (2021). Moringa oleifera seed extract concomitantly supplemented with chemotherapy Worsens tumor progression in mice with triple negative breast cancer and obesity. Nutrients 13, 2923. doi:10.3390/NU13092923
Zwartsen, A., Chottanapund, S., Kittakoop, P., Navasumrit, P., Ruchirawat, M., Van Duursen, M. B. M., et al. (2019). Evaluation of anti-tumour properties of two depsidones - unguinol and Aspergillusidone D - in triple-negative MDA-MB-231 breast tumour cells. Toxicol. Rep. 6, 1216–1222. doi:10.1016/J.TOXREP.2019.10.012
Keywords: HER-2 negative breast cancer, anticancer drugs, natural metabolites, chemotherapy, targeted therapy, nanomedicine
Citation: Püsküllüoğlu M and Michalak I (2024) The therapeutic potential of natural metabolites in targeting endocrine-independent HER-2-negative breast cancer. Front. Pharmacol. 15:1349242. doi: 10.3389/fphar.2024.1349242
Received: 04 December 2023; Accepted: 16 February 2024;
Published: 04 March 2024.
Edited by:
Michał Tomczyk, Medical University of Bialystok, PolandReviewed by:
Magesh Muthu, Wayne State University, United StatesMubashir Mintoo, University of Kansas, United States
Copyright © 2024 Püsküllüoğlu and Michalak. This is an open-access article distributed under the terms of the Creative Commons Attribution License (CC BY). The use, distribution or reproduction in other forums is permitted, provided the original author(s) and the copyright owner(s) are credited and that the original publication in this journal is cited, in accordance with accepted academic practice. No use, distribution or reproduction is permitted which does not comply with these terms.
*Correspondence: Mirosława Püsküllüoğlu, miroslawa.puskulluoglu@onkologia.krakow.pl