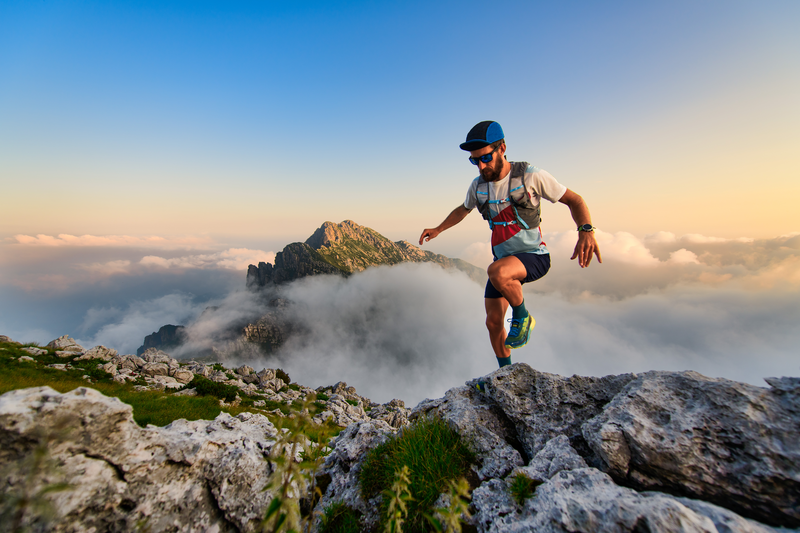
95% of researchers rate our articles as excellent or good
Learn more about the work of our research integrity team to safeguard the quality of each article we publish.
Find out more
REVIEW article
Front. Pharmacol. , 15 May 2024
Sec. Ethnopharmacology
Volume 15 - 2024 | https://doi.org/10.3389/fphar.2024.1347234
Peritoneal dialysis is one of the renal replacement treatments for patients with end-stage renal disease. Peritoneal dialysis-related peritoneal fibrosis is a pathological change in peritoneal tissue of peritoneal dialysis patients with progressive, non-suppurative inflammation accompanied by fibrous tissue hyperplasia, resulting in damage to the original structure and function, leading to peritoneal function failure. Currently, there is no specific drug in the clinic. Therefore, it is necessary to find a drug with good effects and few adverse reactions. Astragalus membranaceus (AMS) is the dried root of the Astragalus membranaceus (Fisch.) Bge. AMS and its active ingredients play a significant role in anti-inflammation, anti-fibrosis, regulation of immune function and regulation of blood pressure. Studies have shown that it can alleviate peritoneal fibrosis by reducing inflammatory response, inhibiting oxidative stress, degrading extracellular matrix deposition, regulating apoptosis, and regulating Transforming Growth Factor-β. The author summarized the relationship between AMS and its active ingredients by referring to relevant literature at home and abroad, in order to provide some theoretical basis for further clinical research.
Peritoneal dialysis (PD) is an effective kidney replacement therapy for patients with end stage renal disease. Compared with hemodialysis, PD has advantages such as low cost, preservation of residual renal function, and stable hemodynamics (P. K.-T. Li et al., 2017). However, long-term PD treatment will lead to peritoneal structure and function disorders, leading to peritoneal fibrosis (PF) and peritoneal failure, resulting in the termination of PD (Zhou et al., 2016). PF has two interacting parts, including the fibrotic process itself and inflammatory progression, in other words, the occurrence process of PF is closely related to peritoneal inflammation (Hu et al., 2023), epithelial-mesenchymal transition (EMT) (Giri et al., 2023; Kunoki, 2023), oxidative stress (Zhao, Shi, et al., 2020), apoptosis (B. Wang et al., 2018), and micro RNA (Gohlke et al., 2022). At present, there are no specific drugs for PF in clinic, mainly glucocorticoids and/or immunosuppressants, whose purpose is to alleviate symptoms and delay the progression of fibrosis as much as possible (Gong et al., 2022; Zhu et al., 2022). Therefore, it is imperative to actively explore the mechanism of PD-induced PF, seek mature and effective targets for the prevention and treatment of PF, and develop new PF treatment methods.
According to the theory of traditional Chinese medicine, PF belongs to the category of “micro syndrome”, the formation of syndrome is mainly caused by Qi accumulation and blood stasis. The treatment should be based on the method of promoting Qi and promoting blood circulation, and the long-term disease consumption of Qi should be supplemented by the prescription of tonifying Qi. Modern pharmacology believes that AMS contains many active ingredients such as polysaccharides, saponins, flavonoids, organic acids and trace elements, and plays a significant role in anti-inflammation, anti-fibrosis, regulation of immune function and regulation of blood pressure (Lau et al., 2012; Kai et al., 2015). At the same time, AMS and its active ingredients have low toxicity and few adverse reactions, and play a positive role in the prevention and treatment of PD-related PF (Agyemang et al., 2013). Therefore, in order to clarify the potential application of AMS and its active components in the prevention and treatment of PF, this paper will review its anti-PD-related PF action and its mechanism, in order to provide scientific support for future PF treatment research direction. Date information was mainly collected from PubMed, Web of Science and CNKI databases (up to April 2024). The anti-peritoneal fibrosis effects of Astragalus membranaceus (Fisch.) Bge and its active components were reviewed in this paper. Search keywords for this type of work include: " Astragalus membranaceus ", “Astragaloside”, “Astragalus saponin”, “total Astragalus saponin”, “Astragalus polysaccharide” and them corresponding subject terms, and “peritoneal fibrosis” or “retroperitoneal fibrosis".
The traditional peritoneal dialysate used lactate as buffer, supplemented with different concentrations of glucose, low pH, high glucose and hyperosmolar are its characteristics (Nakano et al., 2020). The solution will produce a large number of glucose degradation products (GDPs) and advanced glycation end products (AGEs) under high temperature. It leads to inflammation and oxidative stress (Khalid et al., 2022), so the inflammatory reaction runs through PF. On the one hand, peritoneal contact with peritoneal permeate with poor biocompatibility causes micro-inflammation in the peritoneum (Alqaedi et al., 2021); on the other hand, inflammatory injury also stimulates peritoneal mesodermal cells to release inflammatory factors, such as tumor necrosis factor-α (TNF-α), interleukins (ILs), interferons, etc., cause inflammatory response and induce inflammatory cells (such as monocytes, macrophages, neutrophils, etc.) to gather at the damaged site, further releasing inflammatory mediators and reactive oxygen species (ROS),etc., leading to increased intraperitoneal inflammatory response (Henderson et al., 2020). At the same time, the excessive release of inflammatory factors will also cause changes in the extracellular matrix (ECM), leading to ECM deposition, which will stimulate the activation of fibroblasts and further induce peritoneal fibrosis (R. Wang et al., 2022). Oxidative stress is a series of pathophysiological processes caused by the excessive production of ROS and other oxides in cells, which involves the generation, damage, repair, and other aspects of intracellular molecules. AGEs produced by peritoneal permeation can induce a series of chain reactions after binding with RAGE, which promotes the production of mitochondria and endoplasmic reticulum ROS, causing damage to peritoneal mesothelial cells. On the contrary, ROS can also increase the production of AGEs in vivo, and the cycle repeats and becomes vicious. In order to maintain ROS at a low level, the abundant antioxidant system in the body plays an important role, among which nicotinamide-adenine dinucleotide phosphate (NADPH) plays a regulatory role in ROS(Li and Shah, 2003; Lennicke and Cochemé, 2021). Most ROS also comes from mitochondria, which participate in cell metabolism through oxidative phosphorylation (Wang et al., 2011).
EMT is a dynamic structure composed of interstitial matrix and basement membrane, and its composition and proportion will change with different physiological and pathological states (Sutherland et al., 2023; Yuan et al., 2023), that is, when EMT occurs, epithelial cells lose cell polarity, intercellular adhesion, and the support of basement membrane, and at the same time acquire the characteristics of mesenchymal cells (Masola et al., 2022). In the process of fibrosis, transforming growth factor-β (TGF-β) is its main effector, which plays an important role in the occurrence and development of many diseases (such as liver fibrosis (Song et al., 2023), kidney lesions (Tang et al., 2022), pulmonary fibrosis (N. Zhang et al., 2023), etc.). TGF-β is a strong fibrogenic factor (Bottinger and Bitzer, 2002) and plays an important role in the production of fibrosis.
AMS, the root of legumes, was first recorded in Shennong’s Herbal Classic. AMS is the dried root of the perennial herbs Astragalus membranaceus (Fisch.) Bge. At present, Mongolian Astragalus is widely used. Its chemical composition is complex but consistent (T. Chen et al., 2021), (S. Li et al., 2020), containing polysaccharides, glycosides, flavonoids, proteins, amino acids, alkaloids, trace elements, and other active substances (Sheng et al., 2018; Sheik et al., 2021). At present, the extraction methods of saponins include traditional decoctions, reflux extraction, ultrasonic or microwave assisted extraction, bionic extraction, enzyme assisted extraction and so on (Sun et al. , 2023), 160 saponins have been found, triterpenoid saponins are the most studied secondary metabolites in this group of plants, of which Astragaloside IV (AS-IV) is the most common (Su et al. , 2021). At the same time, the results showed that the content of secondary metabolites of flavonoids varied greatly in different parts of the plant, and the root contained the most active components (Zhang et al., 2012; Sun et al., 2023), more than 60 kinds of flavonoids have been identified from AMS, including isoflavones, onononin, and vermus isoflavones (Liu et al. , 2017). Among them, saponins, flavonoids and Astragalus polysaccharide (APS) are the main active components. APS, as one of the main active components in AMS, has anti-inflammatory and immunomodulatory effects, which are important for the regulation of multiple systems and the treatment of multiple diseases (Cai et al., 2023). Due to the complex chemical structure of APS, its components are difficult to separate and identify (Shan et al. , 2019), So it was not studied in depth (Figure 1).
It has been found that AMS and its active ingredients can improve the clinical manifestations of peritoneal permeation-induced PF. The biological activities of Astragalus and its active ingredients are shown in Table 1.
The improvement effect of AMS on PF has been proved. Huang et al. (Huang et al., 2003) cultured HMrSV5 cells in vitro and found that a high concentration of AMS not only did not cause cytotoxicity, but also had a significant protective effect in reducing cell destruction, indicating that AMS could reverse the damage caused by high glucose abdominal permeate solution and inflammatory factors, and promote the proliferation of mesothelial cells, with an effect similar to that of steroid drugs. These results suggest that AMS is important for the maintenance of normal peritoneal function. One study randomly assigned 56 patients with continuous ambulatory peritoneal dialysis. By comparing abdominal dialysis before and after treatment, it was found that using abdominal dialysis containing AMS could antagonize the inhibition of abdominal dialysis on macrophage activity and phagocytosis function. At the same time, AMS can increase the secretion of TNF-α and nitrogen oxide, indicating the immune defense function of AMS in PF treatment (M. Zhang et al., 2007). In the process of TGF-β signaling, Smads protein family is a key mediator. When active TGF-β binds to the TGF-β receptor on the surface, it induces phosphorylation of the C-terminal of downstream Smad protein, phospho-Smad2 and Smad3 form heterodimeric complexes with Smad4, and transported to the nucleus (Roberts and Derynck, 2021). High glucose peritoneal dialysis solution can lead to excessive secretion of TGF-β1, and the use of a conventional dose of peritoneal dialysis solution containing AMS injection (10 mg/mL concentration) can reverse the overexpression of TGF-β1, suggesting that AMS plays a certain role in delaying PF through TGF-β (H. Xu et al., 2005). In this process, Smad7 acts as a TGF-β/Smad signaling antagonist to inhibit TGF-β-mediated phosphorylation of Smad2 and Smad3 (Silva et al., 2019). In the rat PF model induced by standard abdominal permeation solution, it was found that AMS injection (contained 2 g/mL crude drug) can alleviate PF, and the mechanism is to inhibit the action of monocyte chemotactic protein-1, thereby reducing the recruitment and activation of monocytes/macrophages. In addition, the expression of TNF-α and TGF-β1 in the peritoneum was reduced after AMS treatment at a dose of 4000 mg/kg, and the reduction of phosphorylated SMAD2/3 also confirmed the inhibitory effect of AMS on TGF-β/Smad pathway (Z. Li et al., 2014), and it was also found that the role of macrophages in peritoneal fibrosis is bidirectional and complex.
The activation marker of the Wnt/β-catenin signaling pathway is the upregulated expression and nuclear translocation of the key protein β-catenin, and Snail is its transcription factor (Schunk et al., 2021). EMT of HMrSV5 cells was induced by TGF-β, and β-catenin and Snail were reduced. After AMS intervention, EMT was further inhibited. The results suggested that AMS could inhibit TGF-β-induced EMT of HMrSV5 cells to a certain extent. Part of the mechanism is through activation of Wnt/β-catenin signaling pathway, inhibition of β-catenin protein expression and inhibition of Snail transcription factor. In addition, Glycogen Synthase Kinase-3 (GSK-3β) can exist in complex form with β-catenin. Some scholars further detected the expression and phosphorylation of GSK-3β and β-catenin proteins in the cytoplasm and nucleus of peritoneal mesodermal cells after AMS intervention, and found that AMS could stabilize GSK-3β and β-catenin complex and degrade β-catenin. The specific mechanism of AMS regulating Wnt/β-catenin signaling pathway was reconfirmed and further discovered (Yu et al., 2018). Wnt/β-catenin signaling pathway can crosstalk with TGF-β signaling pathway (Guo et al., 2017). Based on this, further experiments found that β-catenin can bind to Smad7 protein. It was found that β-catenin may compete with Smad7 after the construction of Smad7 cell lines, and at the same time reduce the inhibitory effect of AMS on EMT to a certain extent, and the same operation was performed in the rat PF model induced by intrapitoneal injection of 4.25% high glucose peritoneal permeate solution, and the results were also similar. It is suggested that AMS can upregulate Smad7 and competitively inhibit β-catenin through both classical Wnt/β-catenin pathway and crosspoilers with TGF-β, and finally reduce β-catenin expression and interfere with PF(Yu et al., 2018). Some studies have found that stimulation of rat peritoneal mesothelial cells with AMS medium can antagonize the upregulation of ROS and NADPH oxidase subunit p47phox expression caused by Angiotensin Ⅱ, and not only reduce ROS production and p47phox expression. It can also inhibit the expression and activity of NADPH oxidase, so it can be inferred that AMS can reduce the production of ROS by inhibiting the activity of NADPH oxidase, thus blocking its signal transduction, inhibiting the overexpression of TGF-β1 downstream, reducing the occurrence of EMT, and delaying PF (J. Xu et al., 2010). In addition, the experiments of Liu et al. (X. Liu et al., 2014) showed that TGF-β significantly induced the expression of NADPH oxidase subunit p67phoxmRNA and protein in rat peritoneal mesothelial cells, and induced intracellular ROS production, which could be significantly improved by AMS stimulation.
In conclusion, a certain concentration of AMS not only does not cause damage to the body, but also has a protective effect on PF, which is specifically reflected in the fact that AMS can act on the target of TGF-β, interfere with EMT through the associated cytokine network, and improve the oxidative stress in the body to balance the production of ROS, Further, regulate the downstream TGF-β related signaling pathway. However, the research direction of AMS is too simple and not deep enough, only stays in the upstream medium. At the same time, for other cytokines, such as connective tissue growth factor and other downstream mediators, these studies are not clear. Therefore, the mechanism of AMS regulating PF still needs to be further studied (Figure 2).
Figure 2. Effect of Astragalus memeranaceus. AMS not only antagonizes the effect of peritoneal dialysate by restoring macrophage activity, but also inhibits MCP-1 to reduce macrophage recruitment and activation. At the same time, it can inhibit TGF-β pathway and Wnt/β-catenin-related pathway. It also reduces the production of ROS. MCP-1: monocyte chemotactic protein-1; TGF-β: transforming growth factor-β; ROS: reactive oxygen species.
AS-IV, isoastragalus Ⅰ, acetoflavin Ⅰ, Fusarium flavin A, Fusarium flavin B and soybean saponin Ⅰ were isolated from Astragalus memeranaceus. In TGF-β-induced EMT models, AS-IV decreased the expression of vimentin and phospho-Smad2/3 in a dose-dependent manner and increased the expression of Smad7. Therefore, it is speculated that AS-IV can alleviate PF by up-regulating Smad7 in TGF-β/Smad signaling pathway (Z. Li et al., 2013). In addition to the classical Smad pathway, TGF-β can also promote cell survival, proliferation, and collagen synthesis by activating the PI3K/Akt pathway (Y. E. Zhang, 2009). Yu et al. found that TGF-β1 upregulates β-catenin when inducing EMT in HMrSV5 cells, while AS-IV can degrade β-catenin to a certain extent. β-catenin is not only involved in stabilizing cell adhesion, but also a key signaling molecule in the Wnt signaling pathway. At the same time, AS-IV can inhibit the high expression of p-Akt, p-GSK-3β and p-mTOR. Compared with Akt inhibitor MK226, mTOR inhibitor rapamycin and Akt pathway agonist Insulin, it is found that AS-IV is likely to delay PF through the AKt pathway (Yu et al., 2019).
NLRP3 receptor protein 3 inflammasome (NLRP3 inflammasome) is a multiprotein complex, located in the cytoplasm and is named by NOD-like receptor protein 3 (NOD-like receptorprotein 3, NLRP3 inflammasome). NLRP3, apoptosis-associated speck-like potein containing a CARD, and cysteinyl aspartate specific proteinase-1 (Caspase-1), and the activation of NLRP3 inflammasome on the one hand will lead to the release of pro-inflammatory mediators such as interleukin1-β (IL-1β) and interleukin-18, this can induce the progression of inflammation (Lamouille et al., 2014), on the other hand, also can induce the scorch death of damaged cells (J. Chen and Chen, 2018). Zhang et al. (L. Zhang et al., 2015) found that high-glucose peritoneal dialysis solution could activate NLRP3 inflammasome-related parts in a time-dose-dependent manner, such as NLRP3, pro-caspase-1 precursor containing cysteine, IL-1β precursor and IL-1β. AS-IV can partially inhibit peritoneal mesothelial-to-mesenchymal transition by blocking its activation in a concentration-dependent manner. At the same time, the inhibition of the NLRP3 inflamome by AS-IV is not the same as the complete block of other small molecule compounds (such as dopamine receptor-1), but is more likely to partially restore the homeostasis of the low level of NLRP3 activation.
By establishing an in vitro HPMCs/HMrSV5 cell model, Li et al. (Z. Li et al., 2019) confirmed that AS-IV can reduce mitochondrial membrane lipid peroxidation caused by high glucose dialysis solution by reducing the production of ROS, thus alleviating the disorder of charge distribution inside and outside mitochondria, protecting mitochondrial function, improving oxidative stress, and ultimately inhibiting apoptosis induced by mitochondrial damage. On the other hand, AS-IV can control the expression of the translocator of the outer mitochondrial membrane (Tom), although it has no significant effect on the expression of Tom20. However, Tom70 can be upregulated to reverse the damage caused by high glucose dialysate and protect the mitochondrial translocase system. It has also been observed that AS-IV could reduce the expression levels of dynamin related protein-1 and recombinant human mitochondrial fission protein. It also promoted the expression of optic atrophy rotein, a mitochondrial fusion protein. Therefore, it is speculated that AS-IV can improve HPMCs injury and protect peritoneal structure and function through the above pathways. In addition, experiments have shown that ROS may act AS a second messenger to regulate the Smads signaling pathway and mediate the occurrence of EMT, and AS-IV can reduce the production of ROS to inhibit the phosphorylation of Smad2/3, thus achieving the purpose of inhibiting and reverting EMT, similar to the ROS scavenger n-acetylcysteine (NAC) (Shi et al., 2016).
Ferroptosis is a cell death mode different from apoptosis, necrosis, and autophagy, and its main feature is induced lipid peroxidation (Stockwell et al., 2017). Cao Huimin et al. (Cao et al., 2021) found that there was iron death in the HMrSV5 model, and AS-IV could protect cells by inhibiting iron death. In this study, it was found that after the intervention of AS-IV, the level of Fe2+ and ROS decreased, the content of MDA decreased, and the content of GSH increased. The expression of cell tumor antigen p53 and Acyl-CoA synthetase long-chain family member 4 was downregulated. The expression of solute carrier family 7 member 11 (SLC7A11,xCT)and glutathione peroxidase 4 (GPX4) was upregulated. It is suggested that AS-IV may protect peritoneal cells by reducing Fe2+ content, inhibiting ROS production and enhancing xCT and GPX4 activity through cystine/glutamate reverse transporter (xCT)/GPX4 pathway.
Proliferator-activated receptor coactivator-1α (PGC-1α) is an important transcription coactivator of cell kernel receptors. Its primary function is to regulate the synthesis of mitochondrial proteins, including subunits of the respiratory chain complex, by activating nucleus respiratory factor 1 (NRF-1). In addition, PGC-1α binds to transcription factor mitochondrial A, TFAM, thereby initiating the replication and transcription of mitochondrial DNA (Feng et al., 2016). Some studies (Z.-H. Li et al., 2022) suggested that the expression of Bcl-2 in rat stromal cells with fibrosis induced by high glucose was inhibited, and similar changes were also observed in the expression of PGC-1α, NRF-1 and TFAM. However, after Astragalus total saponins (ATS) treatment, the expression of these proteins increased, and after interfering with PGC-1α treatment, the expression of ATS on cell viability and mitochondrial number, including related proteins, showed a downward trend. Therefore, it is speculated that the intervention mechanism of ATS is to promote the expression of PGC-1α and increase mitochondrial synthesis, thereby inhibiting apoptosis and fibrosis-related proteins. Reduce fiber collagen synthesis and fiber tissue proliferation, thereby reducing the degree of PF (Figure 3).
Figure 3. Effect of Astragaloside IV and Astragalus total saponins. The inhibition of AS-IV involves several pathways, including TGF-β pathway, PI3K pathway, Akt pathway, MAPK pathway, etc. It can inhibit Ferroptosis and the production of ROS and inhibit the NLRP3 Inflammasome. TGF-β: Transforming Growth Factor-β; ROS: reactive oxygen species; NLRP3: NOD-like receptor protein 3.
In conclusion, Astragalus saponins (including AS-IV), AS-IV, the main active ingredient of AMS, can delay fibrosis by regulating oxidative stress, apoptosis and inhibiting inflammatory response.
It has been shown that high glucose peritoneal permeate decreases the mitochondrial membrane potential of HMrSV5, and APS can reverse this process. In addition, APS can also downregulate the protein expression of Bcl-2-associated X protein and cleaved Caspase3 and upregulate Bcl-2, antagonizing cell apoptosis and protecting peritoneal tissue, and it is confirmed that APS is associated with the expression level of proteins related to mitochondrial apoptosis of HMrSV5 (Zhao, Shan, et al., 2020). Vascular endothelial growth factor (VEGF) can induce endothelial cell proliferation and promote angiogenesis. Appropriate angiogenesis can promote tissue repair, but when the degree of angiogenesis exceeds a certain limit, the peritoneal glucose absorption rate will increase, resulting in the disappearance of peritoneal osmotic concentration gradient and the reduction of peritoneal ultrafiltration. On this basis, some scholars have found that APS can inhibit the expression of VEGF protein level, thereby inhibiting angiogenesis and reversing the occurrence of fibroperitoneal maintenance in PD rats (Feng et al. , 2024) In conclusion, APS protects peritoneal tissue mainly by regulating mitochondria-related proteins and VEGF. Recently, it has been reported in the literature that APS can inhibit the TLR4/NF-κB signalling pathway and reduce apoptosis, thus ameliorating the occurrence of idiopathic pulmonary fibrosis in mice. Surprisingly, the authors also found that the gut microbiota can also affect lung disease through the gut-lung axis, and APS can increase the proportion of intestinal probiotics, reduce harmful bacteria, and balance the gut microbiota by regulating the metabolic pathway, which ultimately leads to therapeutic effects. This exists as an inspiration for us to study the treatment of PF (Wei et al., 2023).
AMS belongs to traditional Chinese medicine. Existing literature has shown that AMS and its active components have certain efficacy in delaying PD-induced PF, and its potential mechanism is related to anti-inflammatory response, inhibition of oxidative stress, regulation of apoptosis, etc., involving a variety of signaling pathways, such as TGF-β pathway, Wnt pathway and AKT pathway. In this paper, the role of AMS and its active components in anti-peritoneal dialysis-related peritoneal fibrosis was reviewed in order to provide new drug targets. However, the available research is not comprehensive. Although the toxicity of traditional Chinese medicine is relatively low, the active ingredients of AMS are complex, the bioavailability and conversion rate are low, the pharmacokinetic mechanism is unknown, and its preparations are difficult to promote in clinical practice (Cai et al., 2023). Fortunately, there have been no reports of toxic effects of AMS and its active ingredients. AMS was well tolerated in most patients. Therefore, in a certain dose range, the preparation of concentrated pills may become a method for clinical application. At present, there are many researches on the pharmacology of AMS and its active ingredients, as well as its compound Chinese medicine preparations. Although the researches on the mechanism of action of AMS extract or single physiological active ingredient are extensive, but not in-depth. Perhaps, it is also a method to use AMS in combination with the original drug, or to deliver it to the lesion site through a drug delivery carrier such as hydrogel through loaded monomer to achieve the purpose. At the same time, the research on PD-related PF is not deep enough, and only stays at the level of protein expression, and there are few studies on gene expression. Therefore, the potential targets and binding sites of AMS for PF treatment can be identified through pharmacological research methods and molecular docking techniques, and verified with gene knockout or overexpression techniques, or small molecule inhibitors or agonists.
In summary, AMS and its active components can inhibit the occurrence and development of PD-related PF. In the future, combined with CM theory, serum pharmacology and nanoimaging technology, the pharmacological mechanism of AMS in the treatment of PF will be comprehensively studied.
YH: Writing–review and editing, Writing–original draft. CC: Writing–original draft. YM: Writing–original draft. YZ: Writing–original draft. LC: Writing–original draft. SJ: Writing–original draft. BZ: Writing–original draft. QS: Writing–review and editing.
The author(s) declare that no financial support was received for the research, authorship, and/or publication of this article. This research received no external funding.
The authors declare that the research was conducted in the absence of any commercial or financial relationships that could be construed as a potential conflict of interest.
All claims expressed in this article are solely those of the authors and do not necessarily represent those of their affiliated organizations, or those of the publisher, the editors and the reviewers. Any product that may be evaluated in this article, or claim that may be made by its manufacturer, is not guaranteed or endorsed by the publisher.
Agyemang, K., Han, L., Liu, E., Zhang, Y., Wang, T., and Gao, X. (2013). Recent advances in Astragalus membranaceus anti-diabetic research: pharmacological effects of its phytochemical constituents. Evidence-Based Complementary Altern. Med. 2013, 654643–654649. doi:10.1155/2013/654643
Alqaedi, A., Parameaswari, P., Alnasser, B., Mubaraki, A., Bafaqeeh, M., Alshathri, A., et al. (2021). The prevalence of peritonitis among pediatric peritoneal dialysis patients at large Saudi center. Saudi J. Kidney Dis. Transplant. 32 (4), 973–978. doi:10.4103/1319-2442.338309
Bottinger, E. P., and Bitzer, M. (2002). TGF-beta signaling in renal disease. J. Am. Soc. Nephrol. 13 (10), 2600–2610. doi:10.1097/01.ASN.0000033611.79556.AE
Cai, Y., Si, Z., Jiang, Y., Ye, M., Wang, F., Yang, X., et al. (2023). Structure-activity relationship of low molecular weight Astragalus membranaceus polysaccharides produced by Bacteroides. Carbohydr. Polym. 316, 121036. doi:10.1016/j.carbpol.2023.121036
Cao, H., Shan, Y., Tang, L., and Sheng, M. (2021). Advances in cellular iron death in fibrotic diseases. J. Med. Res. Combat Trauma Care 34 (9), 974–978. doi:10.16571/j.cnki.1008-8199.2021.09.015
Chen, J., and Chen, Z. J. (2018). PtdIns4P on dispersed trans-Golgi network mediates NLRP3 inflammasome activation. Nature 564 (7734), 71–76. doi:10.1038/s41586-018-0761-3
Chen, T., Yang, P., and Jia, Y. (2021). Molecular mechanisms of astragaloside-IV in cancer therapy (Review). Int. J. Mol. Med. 47 (3), 13. doi:10.3892/ijmm.2021.4846
Feng, H., Wang, J.-Y., Zheng, M., Zhang, C.-L., An, Y.-M., Li, L., et al. (2016). CTRP3 promotes energy production by inducing mitochondrial ROS and up-expression of PGC-1α in vascular smooth muscle cells. Exp. Cell. Res. 341 (2), 177–186. doi:10.1016/j.yexcr.2016.02.001
Feng, X., Peng, bin, Feng, L., Zhu, S., Hu, X., Xiong, W., et al. (2024). Effect and mechanism of Astragalus polysaccharide on peritoneal fibrosis and angiogenesis in peritoneal dialysis rats. China Pharm. 35 (6), 712–717.
Giri, H., Biswas, I., and Rezaie, A. R. (2023). Activated protein C inhibits mesothelial-to-mesenchymal transition in experimental peritoneal fibrosis. J. Thrombosis Haemostasis 21 (1), 133–144. doi:10.1016/j.jtha.2022.10.012
Gohlke, L., Alahdab, A., Oberhofer, A., Worf, K., Holdenrieder, S., Michaelis, M., et al. (2022) Loss of key EMT-regulating miRNAs highlight the role of ZEB1 in EGFR tyrosine Kinase inhibitor-resistant NSCLC.
Gong, F., Qu, R., Li, Y., Lv, Y., and Dai, J. (2022). Astragalus Mongholicus: a review of its anti-fibrosis properties. Front. Pharmacol. 13, 976561. doi:10.3389/fphar.2022.976561
Guo, Y., Gupte, M., Umbarkar, P., Singh, A. P., Sui, J. Y., Force, T., et al. (2017). Entanglement of GSK-3β, β-catenin and TGF-β1 signaling network to regulate myocardial fibrosis. J. Mol. Cell. Cardiol. 110, 109–120. doi:10.1016/j.yjmcc.2017.07.011
Henderson, N. C., Rieder, F., and Wynn, T. A. (2020). Fibrosis: from mechanisms to medicines. Nature 587 (7835), 555–566. doi:10.1038/s41586-020-2938-9
Hu, W., Li, G., Dong, W., He, P., Liu, W., Wu, Y., et al. (2023). Single-cell sequencing reveals peritoneal environment and insights into fibrosis in CAPD patients. iScience 26 (4), 106336. doi:10.1016/j.isci.2023.106336
Huang, Y., Yi, M., Fu, J., Xue, Y., and Yao, J. (2003). Effects of high glucose and inflammatory factors and Astragalus on human peritoneal mesothelial cytotoxicity. Chin. J. Integr. Traditional West. Nephrol. 5, 262–264.
Kai, Z., Michela, P., Antonio, P., and Annamaria, P. (2015). Biological active ingredients of traditional Chinese herb Astragalus membranaceus on treatment of diabetes: a systematic review. Mini-Reviews Med. Chem. 15 (4), 315–329. doi:10.2174/1389557515666150227113431
Khalid, M., Petroianu, G., and Adem, A. (2022). Advanced glycation end products and diabetes mellitus: mechanisms and perspectives. Biomolecules 12 (4), 542. doi:10.3390/biom12040542
Kunoki, S., Tatsukawa, H., Sakai, Y., Kinashi, H., Kariya, T., Suzuki, Y., et al. (2023). Inhibition of transglutaminase 2 reduces peritoneal injury in a chlorhexidine-induced peritoneal fibrosis model. Lab. Investig. 103, 100050. doi:10.1016/j.labinv.2022.100050
Lamouille, S., Xu, J., and Derynck, R. (2014). Molecular mechanisms of epithelial–mesenchymal transition. Nat. Rev. Mol. Cell. Biol. 15 (3), 178–196. doi:10.1038/nrm3758
Lau, K.-M., Lai, K.-K., Liu, C.-L., Tam, J. C.-W., To, M.-H., Kwok, H.-F., et al. (2012). Synergistic interaction between Astragali Radix and Rehmanniae Radix in a Chinese herbal formula to promote diabetic wound healing. J. Ethnopharmacol. 141 (1), 250–256. doi:10.1016/j.jep.2012.02.025
Lennicke, C., and Cochemé, H. M. (2021). Redox metabolism: ROS as specific molecular regulators of cell signaling and function. Mol. Cell. 81 (18), 3691–3707. doi:10.1016/j.molcel.2021.08.018
Li, J.-M., and Shah, A. M. (2003). ROS generation by nonphagocytic NADPH oxidase: potential relevance in diabetic nephropathy. J. Am. Soc. Nephrol. 14 (Suppl. l_3), S221–S226. doi:10.1097/01.ASN.0000077406.67663.E7
Li, P. K.-T., Chow, K. M., Van De Luijtgaarden, M. W. M., Johnson, D. W., Jager, K. J., Mehrotra, R., et al. (2017). Changes in the worldwide epidemiology of peritoneal dialysis. Nat. Rev. Nephrol. 13 (2), 90–103. doi:10.1038/nrneph.2016.181
Li, S., Sun, Y., Huang, J., Wang, B., Gong, Y., Fang, Y., et al. (2020). Anti-tumor effects and mechanisms of Astragalus membranaceus (AM) and its specific immunopotentiation: status and prospect. J. Ethnopharmacol. 258, 112797. doi:10.1016/j.jep.2020.112797
Li, Z., Sheng, M., Zhang, X., Zhang, L., Zhu, C., and Yang, J. (2013). Effect of Astragalus on TGF-β1/Smads signaling pathway in EMT of rat peritoneal mesothelial cells induced by high-glucose peritoneal fluid. J. Nanjing Univ. Traditional Chin. Med. 29 (3), 227–232+261. doi:10.14148/j.issn.1672-0482.2013.03.012
Li, Z., Shi, J., Zhao, J., Zhang, L., Gao, K., and Sheng, M. (2019). Study on the intervention mechanism of astragaloside on peritoneal mesothelial cell mitochondrial damage induced by high glucose peritoneal fluid. J. Nanjing Univ. Traditional Chin. Med. 35 (4), 436–441. doi:10.14148/j.issn.1672-0482.2019.0436
Li, Z., Zhang, L., He, W., Zhu, C., Yang, J., and Sheng, M. (2014). Astragalus membranaceus inhibits peritoneal fibrosis via monocyte chemoattractant protein (MCP)-1 and the transforming growth factor-β1 (TGF-β1) pathway in rats submitted to peritoneal dialysis. Int. J. Mol. Sci. 15 (7), 12959–12971. doi:10.3390/ijms150712959
Li, Z.-H., Xu, R., Shi, J., Yu, M.-S., Zhong, Y., He, W.-M., et al. (2022). Astragalus total saponins ameliorate peritoneal fibrosis by promoting mitochondrial synthesis and inhibiting apoptosis. Am. J. Chin. Med. 50 (01), 261–274. doi:10.1142/S0192415X22500094
Liu, P., Zhao, H., and Luo, Y. (2017). Anti-aging implications of Astragalus membranaceus (huangqi): a well-known Chinese tonic. Aging Dis. 8 (6), 868–886. doi:10.14336/AD.2017.0816
Liu, X., Zhou, H., Cai, L., Zhang, W., Ma, J., Tao, X., et al. (2014). NADPH oxidase-dependent formation of reactive oxygen species contributes to transforming growth factor β1-induced epithelial-mesenchymal transition in rat peritoneal mesothelial cells, and the role of astragalus intervention. Chin. J. Integr. Med. 20 (9), 667–674. doi:10.1007/s11655-012-1176-x
Masola, V., Bonomini, M., Borrelli, S., Di Liberato, L., Vecchi, L., Onisto, M., et al. (2022). Fibrosis of peritoneal membrane as target of new therapies in peritoneal dialysis. Int. J. Mol. Sci. 23 (9), 4831. doi:10.3390/ijms23094831
Nakano, T., Kumiko, T., Mizumasa, T., Kuroki, Y., Tsuruya, K., and Kitazono, T. (2020). The glucose degradation product methylglyoxal induces immature angiogenesis in patients undergoing peritoneal dialysis. Biochem. Biophysical Res. Commun. 525 (3), 767–772. doi:10.1016/j.bbrc.2020.02.048
Schunk, S. J., Floege, J., Fliser, D., and Speer, T. (2021). WNT-β-catenin signalling - a versatile player in kidney injury and repair. Nat. Rev. Nephrol. 17 (3), 172–184. doi:10.1038/s41581-020-00343-w
Shan, Z., and Li, M. (2019). The effects of Astragalus membranaceus active extracts on autophagy-related diseases. Int. J. Mol. Sci. 20 (8), 1904. doi:10.3390/ijms20081904
Sheik, A., Kim, K., Varaprasad, G. L., Lee, H., Kim, S., Kim, E., et al. (2021). The anti-cancerous activity of adaptogenic herb Astragalus membranaceus. Phytomedicine 91, 153698. doi:10.1016/j.phymed.2021.153698
Sheng, J., Zou, X., Cheng, Z., Xiang, Y., Yang, W., Lin, Y., et al. (2018). Recent advances in herbal medicines for digestive system malignancies. Front. Pharmacol. 9, 1249. doi:10.3389/fphar.2018.01249
Shi, J., Yu, M., Yang, J., and Sheng, M. (2016). Experimental study of Astragaloside on inhibiting HMrSV5 oxidative stress and EMT induced by hyperglycemic abdominal permeate Solution. J. Nanjing Univ. Traditional Chin. Med. 32 (4), 337–341. doi:10.14148/j.issn.1672-0482.2016.0337
Silva, F. M. O., Costalonga, E. C., Silva, C., Carreira, A. C. O., Gomes, S. A., Sogayar, M. C., et al. (2019). Tamoxifen and bone morphogenic protein-7 modulate fibrosis and inflammation in the peritoneal fibrosis model developed in uremic rats. Mol. Med. 25 (1), 41. doi:10.1186/s10020-019-0110-5
Song, Y., Wei, J., Li, R., Fu, R., Han, P., Wang, H., et al. (2023). Tyrosine kinase receptor B attenuates liver fibrosis by inhibiting TGF-β/SMAD signaling. Hepatology, Publ. Ahead Print 78, 1433–1447. doi:10.1097/HEP.0000000000000319
Stockwell, B. R., Friedmann Angeli, J. P., Bayir, H., Bush, A. I., Conrad, M., Dixon, S. J., et al. (2017). Ferroptosis: a regulated cell death nexus linking metabolism, redox biology, and disease. Cell. 171 (2), 273–285. doi:10.1016/j.cell.2017.09.021
Su, H., Shaker, S., Kuang, Y., Zhang, M., Ye, M., and Qiao, X. (2021). Phytochemistry and cardiovascular protective effects of huang-qi (astragali radix). Med. Res. Rev. 41 (4), 1999–2038. doi:10.1002/med.21785
Sun, J., Zhou, Y., Sun, Y., Li, C., Zhang, J., and Wang, W. (2023). Research progress on bioactive components of Astragalus membranaceus and their application in food. China Food Addit. 34 (12), 272–276. doi:10.19804/j.issn1006-2513.2023.12.035
Sutherland, T. E., Dyer, D. P., and Allen, J. E. (2023). The extracellular matrix and the immune system: a mutually dependent relationship. Science 379 (6633), eabp8964. doi:10.1126/science.abp8964
Tang, J., Liu, F., Cooper, M. E., and Chai, Z. (2022). Renal fibrosis as a hallmark of diabetic kidney disease: potential role of targeting transforming growth factor-beta (TGF-β) and related molecules. Expert Opin. Ther. Targets 26 (8), 721–738. doi:10.1080/14728222.2022.2133698
Wang, B., Wen, A., Feng, C., Niu, L., Xiao, X., Luo, L., et al. (2018). The in vivo anti-fibrotic function of calcium sensitive receptor (CaSR) modulating poly(p-dioxanone-co-l-phenylalanine) prodrug. Acta Biomater. 73, 180–189. doi:10.1016/j.actbio.2018.04.018
Wang, J., Wang, H., Hao, P., Xue, L., Wei, S., Zhang, Y., et al. (2011). Inhibition of aldehyde dehydrogenase 2 by oxidative stress is associated with cardiac dysfunction in diabetic rats. Mol. Med. 17 (3–4), 172–179. doi:10.2119/molmed.2010.00114
Wang, R., Guo, T., and Li, J. (2022). Mechanisms of peritoneal mesothelial cells in peritoneal adhesion. Biomolecules 12 (10), 1498. doi:10.3390/biom12101498
Xu, H., Zhang, M., and Jiang, C. (2005). Astragalus injection on peritoneal dialysis-related peritoneal mesothelial cell Effect of TGF-β1 Secretion and Expression. Chin. J. Clin. Pharmacol. Ther. 7, 791–794.
Xu, J., Liu, Z., Ma, S., Lou, D., Wang, Y., and Lu, D. (2010). Effects of Ang II on the expression of ROS and NADPH oxidase subunit p47phox in peritoneal mesothelial cells and the intervention of Astragalus membranaceus. J. Xi’an Jiaot. Univ. Sci. 31 (6), 685–689.
Yu, M., Shi, J., Sheng, M., Gao, K., Zhang, L., Liu, L., et al. (2018). Astragalus inhibits epithelial-to-mesenchymal transition of peritoneal mesothelial cells by down-regulating β-catenin. Cell. Physiology Biochem. 51 (6), 2794–2813. doi:10.1159/000495972
Yu, M., Shi, J., Zhao, J., Zhu, Y., and Sheng, M. (2019). Experimental study on the regulation of Akt signaling pathway by astragaloside to inhibit mesenchymal transition in human peritoneal mesothelial cells. J. Nanjing Univ. Traditional Chin. Med. 35 (1), 53–57. doi:10.14148/j.issn.1672-0482.2019.0053
Yuan, Z., Li, Y., Zhang, S., Wang, X., Dou, H., Yu, X., et al. (2023). Extracellular matrix remodeling in tumor progression and immune escape: from mechanisms to treatments. Mol. Cancer 22 (1), 48. doi:10.1186/s12943-023-01744-8
Zhang, L., Li, Z., He, W., Xu, L., Wang, J., Shi, J., et al. (2015). Effects of astragaloside IV against the TGF-β1-induced epithelial-to-mesenchymal transition in peritoneal mesothelial cells by promoting Smad 7 expression. Cell. Physiology Biochem. 37 (1), 43–54. doi:10.1159/000430332
Zhang, M., Sun, Z., and Jiang, C. (2007). Effect of astragalus injection on peritoneal macrophages in peritoneal dialysis patients. J. Nanjing Univ. Traditional Chin. Med. 5, 319–321.
Zhang, N., Zhao, Z., Zhao, Y., Yang, L., Xue, Y., Feng, Y., et al. (2023). TGF-β changes cyto/mito-ribosome balance to target respiratory chain complex V biogenesis in pulmonary fibrosis therapy. Signal Transduct. Target. Ther. 8 (1), 136. doi:10.1038/s41392-023-01370-2
Zhang, Y., Xu, F., Liang, J., Tang, Ji., Shang, Mi., Wang, X., et al. (2012). Isoflavonoids from roots of Astragalus membranaceus var. mongholicus. China J. Chin. Materia Medica 37 (21), 3243–3248. doi:10.4268/cjcmm20122118
Zhang, Y. E. (2009). Non-Smad pathways in TGF-beta signaling. Cell. Res. 19 (1), 128–139. doi:10.1038/cr.2008.328
Zhao, J., Shan, Y., Zhu, X., Shi, J., Yu, M., Li, Z., et al. (2020). Effect of Astragalus polysaccharides on apoptosis of HMrSV5 cells induced by hyperglycemic abdominal permeate solution. Chin. Archives Traditional Chin. Med. 38 (10), 113-117+280–281. doi:10.13193/j.issn.1673-7717.2020.10.026
Zhao, J., Shi, J., Shan, Y., Yu, M., Zhu, X., Zhu, Y., et al. (2020). Asiaticoside inhibits TGF-β1-induced mesothelial-mesenchymal transition and oxidative stress via the Nrf2/HO-1 signaling pathway in the human peritoneal mesothelial cell line HMrSV5. Cell. Mol. Biol. Lett. 25 (1), 33. doi:10.1186/s11658-020-00226-9
Zhou, Q., Bajo, M.-A., Del Peso, G., Yu, X., and Selgas, R. (2016). Preventing peritoneal membrane fibrosis in peritoneal dialysis patients. Kidney Int. 90 (3), 515–524. doi:10.1016/j.kint.2016.03.040
Keywords: peritoneal fibrosis, Astragalus membranaceus, astragaloside, Astragalus saponin, Astragalus polysaccharide, inflammation peritoneal fibrosis
Citation: Huang Y, Chu C, Mai Y, Zhao Y, Cao L, Ji S, Zhu B and Shen Q (2024) Treatment of peritoneal fibrosis: Therapeutic prospects of bioactive Agents from Astragalus membranaceus. Front. Pharmacol. 15:1347234. doi: 10.3389/fphar.2024.1347234
Received: 08 December 2023; Accepted: 02 May 2024;
Published: 15 May 2024.
Edited by:
Mansour Sobeh, Mohammed VI Polytechnic University, MoroccoReviewed by:
Alex Boye, University of Cape Coast, GhanaCopyright © 2024 Huang, Chu, Mai, Zhao, Cao, Ji, Zhu and Shen. This is an open-access article distributed under the terms of the Creative Commons Attribution License (CC BY). The use, distribution or reproduction in other forums is permitted, provided the original author(s) and the copyright owner(s) are credited and that the original publication in this journal is cited, in accordance with accepted academic practice. No use, distribution or reproduction is permitted which does not comply with these terms.
*Correspondence: Quanquan Shen, c3ByaW5nMTk4NDU3QHNpbmEuY29t
†These authors have contributed equally to this work and share first authorship
Disclaimer: All claims expressed in this article are solely those of the authors and do not necessarily represent those of their affiliated organizations, or those of the publisher, the editors and the reviewers. Any product that may be evaluated in this article or claim that may be made by its manufacturer is not guaranteed or endorsed by the publisher.
Research integrity at Frontiers
Learn more about the work of our research integrity team to safeguard the quality of each article we publish.