- 1Department of Botany, Faculty of Science, University of Khartoum, Khartoum, Sudan
- 2Université de Lorraine, INRAE, LAE, Nancy, France
- 3Physiology and Biochemistry Research Laborotory, Department of Biology, Science Faculty, Selcuk University, Konya, Türkiye
- 4Department of Pharmacy, Botanic Garden “Giardino dei Semplici” “Gabriele d’Annunzio” University, Chieti, Italy
- 5Department of Molecular Biology and Genetics, Istanbul AREL University, Istanbul, Türkiye
- 6School of Pharmacy, University of Camerino, Camerino, Italy
- 7Department of Medical Biochemistry, Faculty of Medicine, Harran University, Sanliurfa, Türkiye
- 8Department of Biology, Science Arts Faculty, Harran University, Sanliurfa, Türkiye
- 9Department of Landscape Architecture, Faculty of Agriculture, Bingol University, Bingöl, Türkiye
- 10Department of Genetic Engineering and Biotechnology, University of Rajshahi, Rajshahi, Bangladesh
- 11Department of Biology, College of Science, United Arab Emirates University, Al-Ain, United Arab Emirates
- 12Department of Basic Medical Sciences, College of Medicine, QU Health, Doha, Qatar
The present study was designed to evaluate the chemical composition, antioxidant, enzyme inhibition and cytotoxic properties of different extracts from aerial parts of V. diversifolium (family Scrophulariaceae), a plant that is native to Lebanon, Syria and Turkey. Six extracts, namely, hexane, dichloromethane (DCM), ethyl acetate (EtOAc), ethanol (EtOH), 70% EtOH, and water (aqueous) were prepared by maceration. The EtOH extract was predominated by the presence of rutin (4280.20 μg g−1) and p-coumaric acid (3044.01 μg g−1) while the highest accumulation of kaempferol-3-glucoside (1537.38 μg g−1), caffeic acid (130.13 μg g−1) and 4-hydroxy benzoic acid (465.93 μg g−1) was recorded in the 70% EtOH, aqueous, and EtOAc extracts, respectively. The EtOH (46.86 mg TE/g) and 70% EtOH (46.33 mg TE/g) extracts displayed the highest DPPH radical scavenging result. Both these extracts, along with the aqueous one, exerted the highest ABTS radical scavenging result (73.03–73.56 mg TE/g). The EtOH and 70% EtOH extracts revealed the most potent anti-AChE (2.66 and 2.64 mg GALAE/g) and anti-glucosidase (1.07 and 1.09 mmol ACAE/g) activities. The aqueous extract was the most efficacious in inhibiting the proliferation of prostate cancer (DU-145) cells with an IC50 of 8.71 μg/mL and a Selectivity Index of 3.7. In conclusion, this study appraised the use of V. diversifolium aerial parts as a potential therapeutic source for future development of phytopharmaceuticals that target specific oxidative stress-linked diseases including diabetes, cancer, cardiovascular disease, and Alzheimer’s disease among others.
1 Introduction
Oxidative stress, particularly reactive oxygen species (ROS), is a major contributor to the pathogenesis of several diseases (Shaito et al., 2022; Aramouni et al., 2023). Oxidative stress could mediate most chronic diseases by activating several transcription factors that can lead to the expression of many genes like those associated to inflammatory cytokines, chemokines, cell cycle regulatory molecules and anti-inflammatory molecules (Reuter et al., 2010). Living organisms employ well-controlled antioxidant defense systems to mitigate damages instigated by ROS (Badran et al., 2020). Many risk factors like aging or unhealthy lifestyles abate the effectiveness of these protective systems, and hence increase ROS accumulation, further precipitating or accentuating pathologies like Alzheimer’s, skin pigmentation, cardiovascular disease, diabetes, and cancer among others (Mesmar et al., 2021; Slika et al., 2022; Kıran et al., 2023).
Despite the tremendous efforts invested to prevent or treat these diseases, the overall incidence and associated morbidity remain alarmingly high (Kim et al., 2018). For instance, estimates suggest that over 45 million people are already affected by Alzheimer’s disease worldwide and this is expected to double every 20 years until 2050 (Santos et al., 2018). Diabetes mellitus is another disease with a rise of about 3% deaths between the year 2000–2019 and it was estimated that more than 1.31 billion people could be living with diabetes by 2050 worldwide (WHO, 2023a; Lancet, 2023). Similarly, cancer remained the second leading cause of global mortality, accounting for an estimated 19.3 million new cases and almost 10.0 million deaths in 2020 (Sung et al., 2021). Therefore, there is an ever-increasing need for concerted efforts to explore new targets and identify new molecules possessing a therapeutic value. One resource that is bountiful in such compounds is plants. Indeed, natural products from medicinal plants and their constituents serve as a crucial source of bioactives that appear promising to prevent or manage various diseases (Anwar et al., 2016; Anwar et al., 2017; Anwar et al., 2018; Kim et al., 2018; Samaha et al., 2019; Fardoun et al., 2020; AlKahlout et al., 2022). For example, three plants belonging to the genus Verbascum (family Scrophulariaceae), namely, V. cheiranthifolium V. myriocarpum and V. pyroliforme, are rich in phenolics and possess significant antioxidant and enzyme inhibitory activities (Zengin et al., 2023). Metabolites like verbascoside and chlorogenic acid in many Verbascum species activate endogenous antioxidant systems to defend against and scavenge free radicals, and hence ameliorate oxidative damage (Bender et al., 2021; Zengin et al., 2023).
Verbascum diversifolium (Synonyms: V. mesopotamicum Boiss.; V. nitidulum Freyn & Sint.) is a biennial plant that grows mainly in the temperate biome and mainly native to Lebanon, Syria and Turkey. Morphologically, V. diversifolium is characterized by deep tap roots, long stalked glandular hairs indumentum, lanceolate to linear-lanceolate, crenate-dentate basal leaves, cyme inflorescence (clusters of 1-4 flowers), triangular-lanceolate acutish calyx lobes, broadly ovate to subglobose capsules, as well as brown, oblong, and reticulate seeds (Karavelioğullari et al., 2011). In Turkish and Romanian traditional medicine, V. diversifolium is used to manage cough, inflammation including laryngitis, swelling, scars, and wounds, as well as an emollient or expectorant (Tita et al., 2009; Cakilcioglu et al., 2011). Chemically, Verbascum plants are characterized by the presence of iridoid and neolignan type glycosides, oleanan type terpenes, flavonoids, polysaccharides, saponins, steroids and alkaloids (Küçük et al., 2016; Blanco-Salas et al., 2021; Zengin et al., 2021; Zengin et al., 2023). They possessed versatile biological properties such as antioxidant, cytotoxic, antimicrobial and anti-inflammatory activities (Tatli and Akdemir, 2006a; Kahraman et al., 2012; Blanco-Salas et al., 2021; Donn et al., 2023). Moreover, bioactives of Verbascum species, including apigenin, verbascoside and chlorogenic acid were found to possess antioxidant, anticancer, anti-Alzheimer activities (Bender et al., 2021; Zengin et al., 2023). Despite the medicinal use of V. diversifolium, the literature is devoid of reports describing its chemical constituents or biological activity and hence, it was hypothesized that V. diversifolium could have a promising pharmacological potential like other species from this genus. The present study was undertaken to evaluate the chemical composition, antioxidant, enzyme inhibition and cytotoxic properties of different extracts from the aerial parts of V. diversifolium.
2 Materials and methods
2.1 Plant material
V. diversifolium plant materials were obtained from a field investigation (Kurudere Village, Bingol Turkey). Taxonomical identification was carried out by Dr. Rıdvan Polat and a specimen was deposited in the herbarium of Bingol University (Voucher Number: RP-1067). The aerial parts were carefully separated and dried in shade at room temperature, then ground into powder using a laboratory mill and stored in the dark.
2.2 Extraction
The extracts were prepared using the six solvents n-hexane, dicholoromethane, ethyl acetate, ethanol, ethanol/water (70%) and water. Each plant material (10 g) was macerated with 200 mL of the respective solvent at room temperature overnight. For water extract, the plant material (10 g) was soaked in boiled water for 15 min (as infusion method). The organic solvents were evaporated to remove solvents. The water extract was dried by a freeze drier.
2.3 Assay for total phenolic and flavonoid contents
The total content of phenols and flavonoids were assessed following the procedures described in the paper (Slinkard and Singleton, 1977).
2.4 HPLC-ESI-MS/MS
HPLC-MS/MS studies were performed using an Agilent 1290 Infinity series and a Triple Quadrupole 6420 from Agilent Technology (Santa Clara, CA) equipped with an electrospray ionization (ESI) source operating in negative and positive ionization modes. All details are given in Supplementary Material.
2.5 Antioxidant tests
In vitro antioxidant assays were conducted using the previously reported methods (Grochowski et al., 2017). For the 2,2-diphenyl-1-picrylhydrazyl (DPPH), 2,2′-azino-bis(3-ethylbenzothiazoline-6-sulfonic acid) (ABTS) radical scavenging, Cupric reducing antioxidant capacity (CUPRAC), and ferric reducing antioxidant power (FRAP) tests, the results were expressed as mg of Trolox equivalents (TE) per g extract. In the phosphomolybdenum (PBD) assay, the antioxidant potential was quantified in terms of mmol of Trolox equivalents (TE) per g extract. Metal chelating activity (MCA) was expressed as mg of disodium edetate equivalents (EDTAE) per g extract (Grochowski et al., 2017).
2.6 Enzyme inhibitory tests
The enzyme inhibition experiments for the samples were conducted following previously established protocols (Grochowski et al., 2017). Inhibition of amylase and glucosidase activities were expressed as mmol of acarbose equivalents (ACAE) per g extract, while inhibition of acetylcholinesterase (AChE) and butyrylcholinesterase (BChE) activities were expressed as mg of galanthamine equivalents (GALAE) per g extract. Tyrosinase inhibition was quantified as mg of kojic acid equivalents (KAE) per g of the tested extracts.
2.7 Cell analysis
2.7.1 Materials
The following materials were used in this study: Cell culture medium (DMEM-F12/RPMI 1640; Sigma-Aldrich Cat No: D0697/R8758, United States), fetal bovine serum (FBS; Sigma-Aldrich Cat No: F7524, United States), %1 penicillin/streptomycin (Sigma-Aldrich Cat No: P4333, United States), L-glutamine (Sigma-Aldrich Cat No: 59202C, United States), trypsin-EDTA solution (Sigma-Aldrich Cat No: 59417C, United States), dimethyl sulfoxide (DMSO) (Sigma-Aldrich Cat No: PHR1309, United States), MTT (3-(4,5-Dimethylthiazol-2-yl)-2,5-Diphenyltetrazolium Bromide; Sigma-Aldrich Cat No: M2128, United States). Culture plates (96 well) were purchased from Nunc (Brand products, Denmark).
2.7.2 Cell culture
Cancer and normal cell lines were obtained from ATCC and stored in liquid nitrogen: DU-145 (Prostate Carcinoma), MDA-MB-231 (Breast Adenocarcinoma), HELA (Cervix Adenocarcinoma), HT-29 (Colon Adenocarcinoma), HGC-27 (Gastric Cancer), and HEK-293 (Normal Human Embryonic Kidney) cells. These cells were cultured in DMEM-F12/RPMI-1640 media supplemented with 10% FBS and 100 μg/mL of streptomycin/100 IU/mL of penicillin in incubators at 37 °C under humid conditions containing 5% CO2.
2.7.3 Cell viability assay
The cytotoxic effects of V. diversifolium (ethanol, ethanol/water and water) were assessed using the MTT (3-(4,5-Dimethylthiazol-2-yl)-2,5-Diphenyltetrazolium Bromide) assay. Cells (DU-145, MDA-MB-231, HELA, HT-29, HGC-27, and HEK-293) were seeded at 1 × 104 cells per well in a 96-well sterile plate and incubated for 24 h. The media were then replaced with extracts at varying doses (0, 2.5, 5, 10, 25, 50, 100, and 200 μg/mL) for another 24 h. Subsequently, 10 μL of MTT solution (0.5 mg/mL) was added to each well as a reaction agent, followed by a 4-h incubation period. After removing the media, 100 μL of DMSO was added, and measurements were taken at OD570-OD690 nm using a plate reader (Thermo Multiskan GO, Thermo, United States). Finally, plots were generated, and the IC50 value was calculated.
2.7.4 KEGG pathway analysis for CDK6 gene
KEGG (Kyoto Encyclopedia of Genes and Genomes) pathway analysis is a valuable approach in bioinformatics and systems biology for make sense of complex biological data, gain insights into cellular processes, and discover potential applications in fields such as drug discovery, disease research, and personalized medicine. In this study we used KEGG PATHWAY Database online server for analysis the involvement of the cyclin dependent kinase 6 gene in different cellular pathway (Chen et al., 2017).
2.8 In silico analysis
2.8.1 Ligand preparation
The phytochemicals from V. diversifolium were retrieved in sdf format from the Pub-Chem database (Kim et al., 2021). These compounds underwent a purification process and were subjected to energy minimization using the Avogadro software, employing the mmf94 force field methodology (Hanwell et al., 2012).
2.8.2 Protein preparation
The 3D structure of target proteins, namely, cyclin-dependent kinase 6 (CDK6, PDB ID: 1xo2), tyrosinase (PDB ID: 3awu), alpha-amylase (PDB ID: 3baj), alpha-glucosidase (PDB ID: 3w37), AChE (PDB ID: 4bdt), and BChE (PDB ID: 6qab) were retrieved from protein data bank (Bouley et al., 2015). The protein structure was initially cleaned and heteroatoms were removed by using Discovery Studio software (Versions: 21.1.0.0). Then, using the GROMOS96 43b1 force field and the SwissPDB Viewer software, the energy of the cleaned protein was minimized and optimized (Guex and Peitsch, 1997).
2.8.3 Molecular docking
Utilizing the PyRx software and its Autodock Wizard feature, a molecular docking study was performed between phytochemicals from V. diversifolium and the target proteins (Dallakyan and Olson, 2015). Firstly, the protein’s structural configuration was converted into a macromolecular representation, and the ligands were transformed into PDBQT format. For the subsequent docking process, specific parameters were established: the center coordinates and a grid box size defined were set on the binding site shown in Table 1. The final docking calculations were executed using PyRx, with the selection of top-ranking molecules based on their lower binding energy values. To explore the resultant binding interactions and poses, Discovery Studio software was employed.
2.8.4 Statistical analysis
Experiments were performed in triplicate, and differences between the extracts were compared using one way ANOVA and Tukey’s test. Graph Pad Prism (version 9.2) was used for the analysis. A p-value of <0.05 was considered significant.
3 Results and discussion
3.1 Bioactive constituents
The total phenolic (TPC) and flavonoids (TFC) contents of V. diversifolium aerial parts extracts were determined and results are presented in Table 2. The TPC of extracts was in the range of 14.09–45.69 mg GAE/g and it was in the following decreasing order; EtOH > EtOAc >70% EtOH > H2OH > DCM > Hexane. The TFC of extracts was in the range of 1.01–21.57 mg RE/g and it was in the following decreasing order; EtOH >70% EtOH > H2OH > EtOAc > DCM > Hexane. Hence, polar solvents recovered higher amount of TPC and TFC than the non-polar solvents. It was also observed that EtOH extract recovered higher TPC and TFC than hydroethanol and water extracts. This may be attributed to the presence of more nonphenolic compounds like carbohydrates and terpenes in aqueous than in other extracts. It may also be due to the possible recovery of some phenolic compounds in EtOH which may possess more phenol groups or have higher molecular weights than the phenolics in the aqueous or hydroalcoholic extracts (Do et al., 2014). In fact, recovery of phenolics from plants materials is affected by the type of solvent extraction and extraction process (Alara et al., 2021). Thus, based on results of TPC and TFC, EtOH was the most efficient extracting solvent. Furthermore, a quantitative analysis of 32 bioactive compounds of the different extracts of V. diversifolium was performed by HPLC-MS/MS. Results are depicted in Table 3.
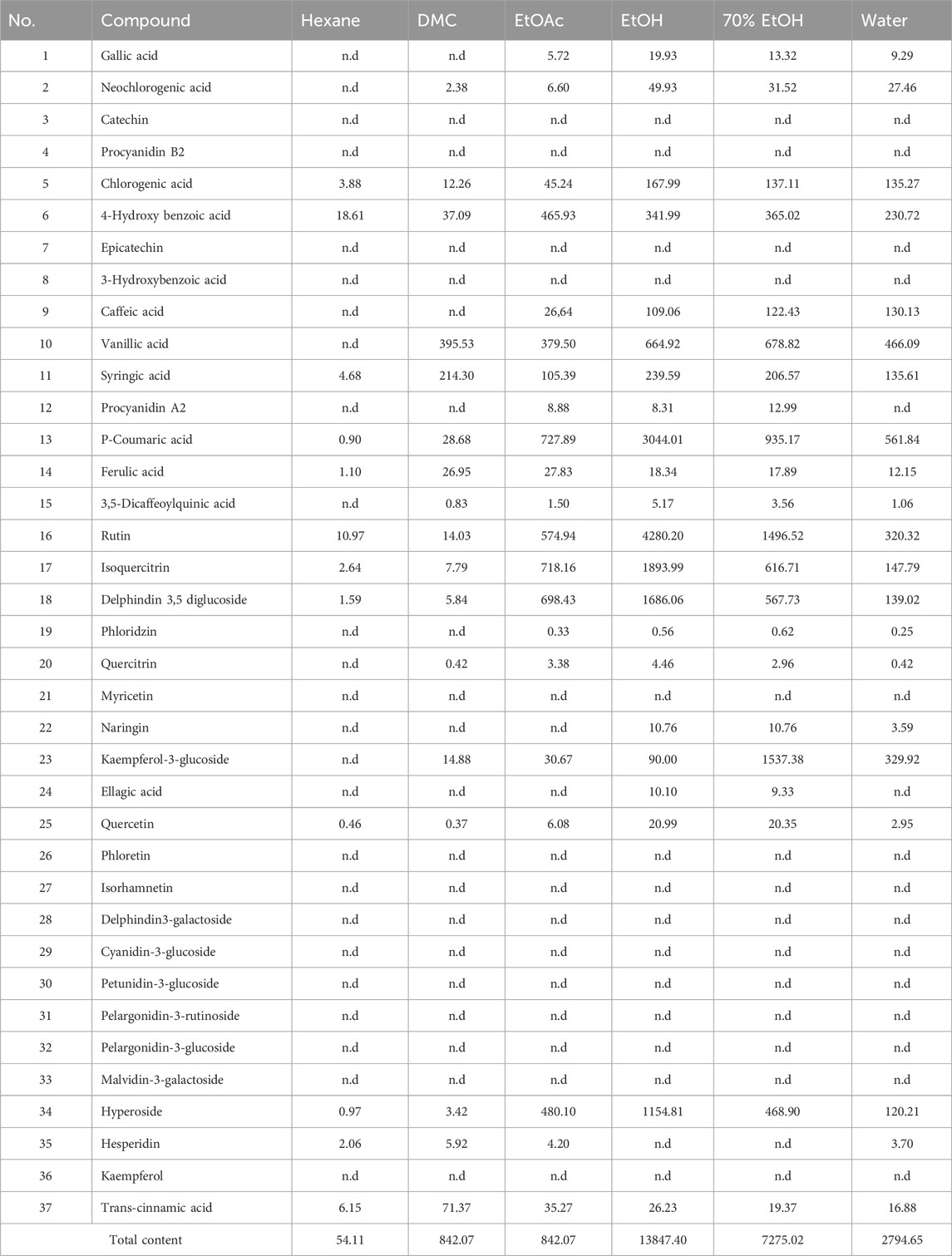
TABLE 3. Content (µg g−1 of dried extract) of bioactive compounds in Verbascum diversifolium aerial parts extracts.
The highest number of compounds was obtained from the polar extracts and the least number in the hexane and DCM extracts in agreement with the pattern obtained for TPC and TFC. The concentration of total identified compounds was in the following decreasing order; EtOH (13847.40 μg g−1) > 70% EtOH (7275.02 μg g−1) > EtOAc (4352.67 μg g−1) > H2OH (2794.65 μg g−1) > DCM (842.07 μg g−1) > Hexane (54.11 μg g−1). The EtOH extract predominated by the presence of rutin (4280.20 μg g−1), p-coumaric acid (3044.01 μg g−1), isoquercitrin (1893.99 μg g−1), delphindin 3,5 diglucoside (1686.06 μg g−1) and hyperoside (1154.81 μg g−1). The 70% EtOH extract was characterized by high accumulation of kaempferol-3-glucoside (1537.38 μg g−1), rutin (1496.52 μg g−1) and p-coumaric acid (935.17 μg g−1). The highest content of 4-hydroxy benzoic acid (465.93 μg g−1) and ferulic acid (27.83 μg g−1) were identified in the EtOAc extract. Highest accumulation of caffeic acid (130.13 μg g−1) and trans-cinnamic acid (71.37 μg g−1) was observed in the aqueous and DCM extracts respectively. However, most of these compounds which are known for their beneficial effect are previously identified in other Verbascum species (El Gizawy et al., 2019; Angeloni et al., 2021; Gökmen et al., 2021; Zengin et al., 2021; Zengin et al., 2023). Besides, bioactives like rutin, isoquercitrin and kaempferol are known to exhibit antioxidant potential while phenolic acids like vanillic, caffeic, cinnamic, 4-Hydroxy benzoic and gallic acids are effective anticancer agents and enzyme inhibitors (Abotaleb et al., 2020; Aleixandre et al., 2022; Budryn et al., 2022).
3.2. Chemical antioxidant assays
Six complementary assays including radical scavenging (DPPH and ABTS), reducing (CUPRAC and FRAP), metal chelating and total antioxidant assays were performed to evaluate the antioxidant property of V. diversifolium aerial parts and results are presented in Table 4. Extracts showed similar pattern in their scavenging towards the DPPH and ABTS radicals but recorded higher values in ABTS assay. The DPPH radical scavenging of different extracts was in the following decreasing order; EtOH (46.86 mg TE/g) ≂ 70% EtOH (46.33 mg TE/g) (p ≥ 0.05) > EtOAc (38.49 mg TE/g) > H2OH (37.25 mg TE/g) > DCM (1.67 mg TE/g) > Hexane (0.54 mg TE/g) (p < 0.05). The EtOH, 70% EtOH and aqueous extracts had relatively the same effect to scavenge the ABTS radical (73.56–73.03 mg TE/g) (p ≥ 0.05) followed respectively by the EtOAc (53.33 mg TE/g), DCM (22.56 mg TE/g) and Hexane (8.10 mg TE/g) extracts (p < 0.05). The highest result to reduce the Cu++ and Fe+++ ions was exerted respectively by the EtOH (174.84 and 79.24 mg TE/g respectively), EtOAc (128.66 and 60.83 mg TE/g respectively) and 70% EtOH (116.08 and 57.47 mg TE/g) extracts (p < 0.05). For the DCM extract, it significantly (p < 0.05) displayed higher Cu++ ions reducing effect (89.85 mg TE/g) than the aqueous extract (77.52 mg TE/g) while the opposite was true for their effect to reduce the Fe+++ ions (30.22 and 51.40 mg TE/g respectively). The hexane extract showed the least values in both assays. Although the EtOH extract exhibited the highest antioxidant result in the 4 previous assays, it nonetheless displayed the least metal chelating power (13.79 mg EDTAE/g). The aqueous extract (54.63 mg EDTAE/g) exerted significantly (p < 0.05) the highest value followed by the DCM (42.92 mg EDTAE/g) extract. The 70% EtOH (35.67 mg EDTAE/g) and hexane (35.12 mg EDTAE/g) extracts showed relatively the same effect (p ≥ 0.05).
Chelation of metal ions is considered a good strategy to avoid ROS generation that is associated with redox active metal catalysis. The transition metal ion, Fe2+, has the ability to move single electrons, stimulate lipid peroxidation by the Fenton reaction and thus can allow for the formation and propagation of many radical reactions (Kumar et al., 2013a; Kumar et al., 2013b). Ferrozine can quantitatively form complexes with Fe2+; however, in the presence of a chelating milieu, the formation of Fe2+-ferrozine complex is decreased, suggesting chelation of iron by phytochemicals present in the plant (Sunday Joel et al., 2017). Phytochemicals present in V. diversifolium extracts interfered with the formation of ferrous-ferrozine complex, suggesting that they had chelating activity and captured ferrous ion before ferrozine (Mishra et al., 2013). It was postulated that the reason that extracts showed higher reducing values for copper ions than for iron ions can be explained in terms of the standard reduction potentials of the metals. The standard reduction potential of the Cu2+/Cu+ couple (+0.15 V) is much lower than that for the Fe3+/Fe2+ couple (+0.77 V) (Lomozová et al., 2022).
The total antioxidant results of extracts was in the range of 0.97 and 2.58 mmol TE/g with least and highest significant (p < 0.05) activity recorded from the hexane and EtOAc extracts respectively. It is clear that extracts showing high antioxidant results are rich in TPC supporting previous studies that correlating the antioxidant activity of extracts to their phenolic content (Alara et al., 2021; Molole et al., 2022). Furthermore, phenolic compounds like chlorogenic, 4-hydroxy benzoic, vanillic, syringic, p-coumaric, caffeic, and cinnamic acids, rutin, isoquercitrin, kaempferol-3-glucoside and hyperoside, detected in considerable amount in polar extracts, are well known for their antioxidant properties (Brewer, 2011; Rababah et al., 2011; Spiegel et al., 2020). Furthermore, the antioxidant effect of phenolics is highly associated with their structure, as structures with the most hydroxyl groups usually exert the greatest antioxidant activity (Rice-Evans et al., 1996). It is known that hydroxylated cinnamates are more effective than benzoate counterparts. Insertion of an ethylenic group between a phenyl ring carrying a p-hydroxyl group and the carboxylate group, as in p-coumaric acid, has a highly favourable effect on the reducing properties of the OH group compared with cinnamic acid. Incorporation of a hydroxyl group into p-coumaric acid adjacent to that in the para position as in caffeic acid increase its antioxidant property. Substitution of the 3-hydroxyl group of caffeic acid by a methoxy group (ferulic acid) considerably enhances its antioxidant effectiveness (Rice-Evans et al., 1996). Also, it has been established that the position and degree of hydroxylation is fundamental to the antioxidant activity of flavonoids, particularly in terms of the o-dihydroxylation of the B ring, the carbonyl at position 4, and a free hydroxyl group at positions 3 and/or 5 in the C and A tings, respectively (Rice-Evans et al., 1996). Eventually, these compounds may act synergistically and the highest antioxidant effect displayed by polar extracts, mainly the EtOH extract, could be attributed to relatively high accumulation of these compounds.
Overall, it was noted that phytoconstituents with highest antiradical and ion reducing results were mainly recovered from the EtOH extract, while those with best chelating and total antioxidant results were mainly accumulated in the aqueous and EtOAc extracts, respectively. This indicates that V. diversifolium contain antioxidant molecules with variable mechanisms of action. However, these results supported those obtained for V. nubicum (El Gizawy et al., 2019), V. bugulifolium (Gökmen et al., 2021), V. bombyciferu (Angeloni et al., 2021), V. euphraticum, V. oocarpum, V. cheiranthifolium, V. myriocarpum and V. pyroliforme (Zengin et al., 2021; Zengin et al., 2023) where the highest antioxidant activity was mainly exerted by polar extracts (MeOH, hydromethanolic or aqueous). Also, the two compounds, verbascoside and luteolin, isolated from the aerial parts of V. bugulifolium were found to possess potent antioxidant activity in the DPPH (IC50 = 5 μg/mL for luteolin) and CUPRAC (IC50 = 10 µg/mLfor verbascoside) assays (Gökmen et al., 2021). Hence this genus can be considered as a promising source of antioxidants.
3.3 Enzyme inhibition activity
Enzymes are currently receiving increased attention due to their potential therapeutic alternative for several diseases like Alzheimer’s disease, diabetes and some skin disorders. In the present study, extracts of V. diversifolium aerial parts were evaluated for their capacity to inhibit the AChE, BChE, Tyrosinase, α-amylase and α-glucosidase enzymes. Results are shown in Table 5.
The AChE inhibition activity of different extracts was in the range of 1.12–2.66 mg GALAE/g with highest activity displayed by the EtOH and 70% EtOH extracts (p > 0.05) followed by the hexane (2.38 mg GALAE/g) and DCM (2.28 mg GALAE/g) extracts (p > 0.05). The aqueous and EtOAc extracts revealed the least activity. With the exception of the aqueous extract, all extracts exerted good anti-BChE activity in the range of 2.54–5.21 mg GALAE/g. The highest significant (p < 0.05) effect was displayed by the DCM extract followed respectively by the EtOH (4.64 mg GALAE/g) and EtOAc (3.09 mg GALAE/g) extracts. It was noted that most extracts showed higher anti-BChE activity than anti-AChE one.
Some of the identified phenolics in the present study were shown to possess significant enzyme inhibition property. For example, the anti-AChE activity of phenolic acids was reported in the following order; rosmarinic acid > caffeic acid > gallic acid = chlorogenic acid > homovanillic acid > sinapic acid (Szwajgier, 2015). Additionally, syringic, vanillic, 4-Hydroxy benzoic and gallic acids were reported to exhibit anti-AChE activity (Srinivasulu et al., 2018; Budryn et al., 2022). The flavonoids, quercetin and kaempferol were also reported as efficient AChE inhibitors (Szwajgier, 2015). All extracts, except the aqueous one, exhibited considerable anti-Tyrosinase activity in the range of 41.40–58.39 mg KAE/g with the EtOH and hexane extracts revealed the highest and least activity. This activity could be partly due the presence of p-coumaric acid and caffeic acid (Crespo et al., 2019). Although these compounds were also detected in the aqueous extract it devoid of anti-Tyrosinase activity suggesting an antagonistic effect by other molecules. Targeting the α-amylase and α-glucosidase enzymes is believed an important approach in the development of novel anti-diabetic agents where by postprandial hyperglycemia can be better controlled (Alam et al., 2019). The α-amylase inhibition activity was in the range of 0.04–0.51 mmol ACAE/g with highest and least activity exerted by the DCM and aqueous extracts. The α-glucosidase inhibition activity was in the range of not active to 1.09 mmol ACAE/g where the EtOH and 70% EtOH extracts revealed the highest activity and the aqueous extract was not effective. A recent study showed that syringic and vanillic acids were effective against the α-amylase while caffeic acid was effective against the α-glucosidase (Aleixandre et al., 2022). Overall, these results revealed that V. diversifolium possessed significant enzyme inhibition properties against the tested enzyme in agreement with previous studies on other Verbascum species (Angeloni et al., 2021; Zengin et al., 2021; Kızıltaş et al., 2023; Zengin et al., 2023).
3.4 Cytotoxicity
The search for new natural anticancer drugs is a main objective of scientific research (El Hasasna et al., 2015; El Hasasna et al., 2016; Athamneh et al., 2017; Alsamri et al., 2019; Athamneh et al., 2020). In the present study, the cytotoxic effect of the polar (EtOH, 70% EtOH and H2OH) extracts of V. diversifolium aerial parts was examined against 5 cancer cells; DU-145, MDA-MB-231, HELA, HT-29 and HGC-27 in addition to the normal cell HEK-293. Results are presented in Table 6 and Figure 1. According to the US National Cancer Institute (NCI) plant screening program, a crude plant extract is generally considered to have acceptable in vitro cytotoxic activity if the IC50 value is less than 20 μg/mL after 48- and 72-h incubation with cancer cell lines (Boik, 2001). Based on the present results, the three extracts displayed cytotoxic effect against DU-145 cell (IC50 8.71–17.85 μg/mL) with the highest effect obtained from the aqueous extract. Results also revealed cytotoxicity against HGC-27 (IC50 7.051–16.09 μg/mL) with the EtOH extract exerting the highest effect. Additionally, the 70% EtOH (IC50 7.65 μg/mL) and aqueous (IC50 13.89 μg/mL) extracts showed cytotoxicity against HELA cells. All the three extracts were less toxic towards the MDA-MB-231 and HT-29 cells (IC50 > 20 μg/mL). On the other hand, the EtOH (IC50 14.95 μg/mL) and 70% EtOH (IC50 13.09 μg/mL) extract were toxic against the normal HEK-293 cell while the aqueous extract was significantly less toxic (IC50 32.54 μg/mL). Besides, the selectivity index (SI) was calculated and SI > 3 is considered as indicative for safer and more effective therapy in cancer therapy (Segun et al., 2019). Accordingly, the aqueous extract was found to be the most selective to inhibit the DU-145 cell with SI = 3.7 while the 70% EtOH (SI = 1.7) and EtOH (SI = 2.1) extracts were less selective for HELA and HGC-27 cell. However, assessing the clinical relevance of these findings is still warranted.
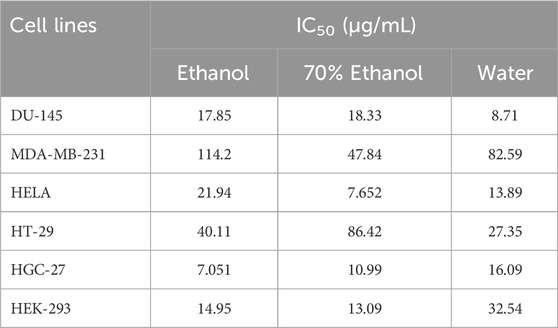
TABLE 6. Cytotoxic effects of Verbascum diversifolium polar extracts on cancer and normal cell lines.
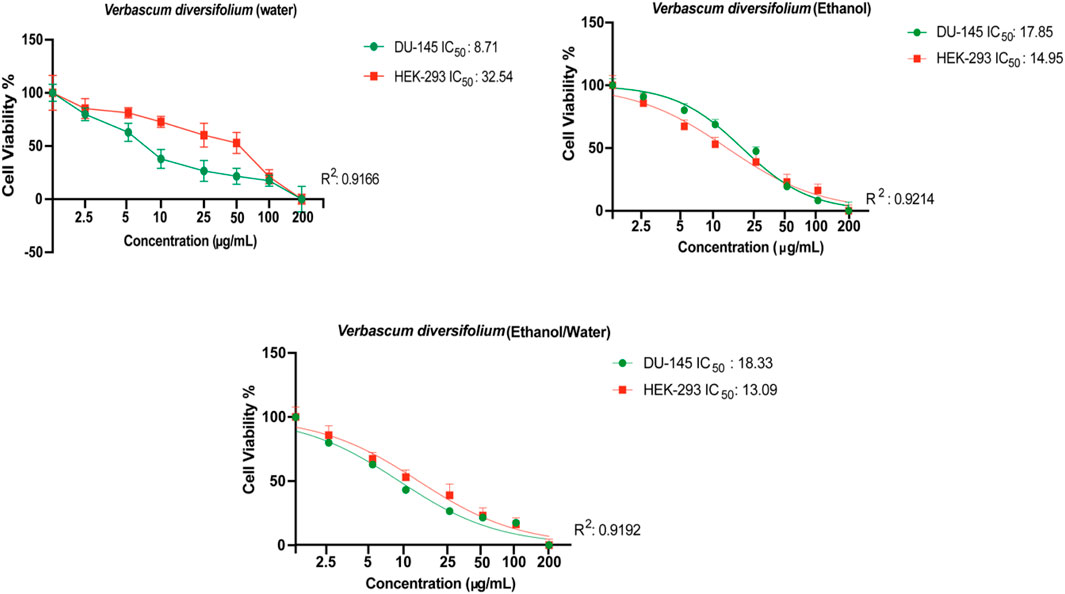
FIGURE 1. Evaluation of cytotoxic effects of Verbascum diversifolium (ethanol, ethanol/water and water) extracts on DU-145 and HEK-293 cell lines by MTT assay. All data are expressed as the mean ± SD from three independent experiments. The IC50 value was calculated using the GraphPad Prism 9 program.
Phenolic compounds exert a wide variety of anticancer effects. They modulate ROS-scavenging enzyme activities, participate in arresting the cell cycle, induce apoptosis, autophagy, and suppress cancer cell proliferation and invasiveness. The anticancer activity of phenolic acids and flavonoids is highly associated with their chemical structure. For example, an increase in the number of the OH ring substituents in the phenolic acids leads to higher antiproliferative and cytotoxic activities (Abotaleb et al., 2020). For flavonoids, the cytotoxic activity is also affected by the number of hydroxyl groups that the compounds carry; and the saturation of the C2 = C3 double bond reduces the activity (Gomes et al., 2003). However, some of the compounds identified in the present study are known to exert cytotoxic effects against cancer cell lines. For instance, coumaric acid exerted cytotoxic effect against human colon HT-29 and HCT-15, human lung A549 and prostate PC-3 cells (Sharma et al., 2017; Sharma et al., 2018; Sharma et al., 2019). Vanillic acid was active against human colon cancer HCT116 cell (Gong et al., 2019). Syringic acid possesses cytotoxic effect in human colorectal cancer SW1116 and SW837 cells (Abaza et al., 2013). Gallic and caffeic acids were effective against HeLa cells (Gomes et al., 2003). The latter also exhibited cytotoxic effect towards Ht-29 cell line (Anantharaju et al., 2016). Quercetin has antitumor activity against colon carcinoma CT-26 cells, prostate adenocarcinoma LNCaP cells, PC3 cells, pheochromocytoma PC12 cells, estrogen receptor-positive breast cancer MCF-7 cells, acute lymphoblastic leukemia MOLT-4 T-cells, human myeloma U266B1 cells, human lymphoid Raji cells, and ovarian cancer CHO cells (Hashemzaei et al., 2017). Moreover, many Verbascum species were reported in the literature to exert significant cytotoxic effect against many cancer cell lines like V. sinaiticum against P-388 cells (Afifi et al., 1993), MCF-7, and HepG2 cells (Tauchen et al., 2015), V. pterocalycinum var. mutense against SK-MEL cells (Tatli and Akdemir, 2006b), V. sinaiticum against Hep-2 and MCF-7 cells. (Talib and Mahasneh, 2010), V. thapsus against A549 cells (Zhao et al., 2011), V. ovalifolium against SK-MEL-2 cell (Luca et al., 2019), V. nigrum against A431 cells (Iliescu et al., 2020), V. alceoides against HeLa and HUVEC cells (Dinani et al., 2020), and V. lasianthum against A549 cells (Hazman et al., 2021).
3.5 Selection of target protein and its Kyoto Encyclopedia of genes and genomes pathway analysis
Cyclin dependent kinase 6 (CDK6) assumes a central position in the regulation of the cell cycle, particularly within the G1 phase, where it engages in partnerships with cyclins to propel the progression of the cell cycle (Figure 2). Conducting a KEGG pathway analysis on CDK6 offers an opportunity to gain valuable insights into the biological mechanisms and pathways where this protein plays a crucial role. To perform the in silico anticancer activity, CDK6 (PDB ID: 1XO2) was selected as a potential drug target and its KEGG pathway analyzed. The KEGG identifier of this protein is hsa:1021. This target gene plays a significant role in 23 different pathways as shown in Supplementary Table S1.
CDK6 holds promise as a viable target for cancer treatment, as indicated in Figure 3. Employing KEGG pathway analysis can shed light on the pathways linked to CDK6’s function, which could have significance in the realm of drug development. This analysis has the potential to assist in the discovery of new drug targets, the exploration of possible combination therapies, and the comprehension of how currently available drugs targeting CDK6 may influence other pathways.
3.6 Molecular docking targeting cyclin dependent kinase 6
After the comprehensive KEGG pathway analysis, we confirmed that CDK6 is involved in different cancer pathways that emerge it as potential anticancer drug target. Therefore, in this study, we sought to determine if phytochemicals of V. diversifolium can act as potential inhibitors of this target. Our results revealed that cyanidin-3-O-glucoside showed the highest binding affinity with −10.2 kcal/mol compared to the control compounds. Fisetin with −8.87 kcal/mol, where it interacts the target protein 1xo2 with six hydrogen bonds at Glu18, Ile19, Val101, Asp104, Thr107, and Gln149 (Figure 4A). Similarly, the complex between 1xo2 and ellagic acid exhibited two hydrogen bond exactly the same position as cyanidin-3-O-glucoside at Val101 and Asp104 (Figure 4B) with binding energy −9.1 kcal/mol (Supplementary Table S2).
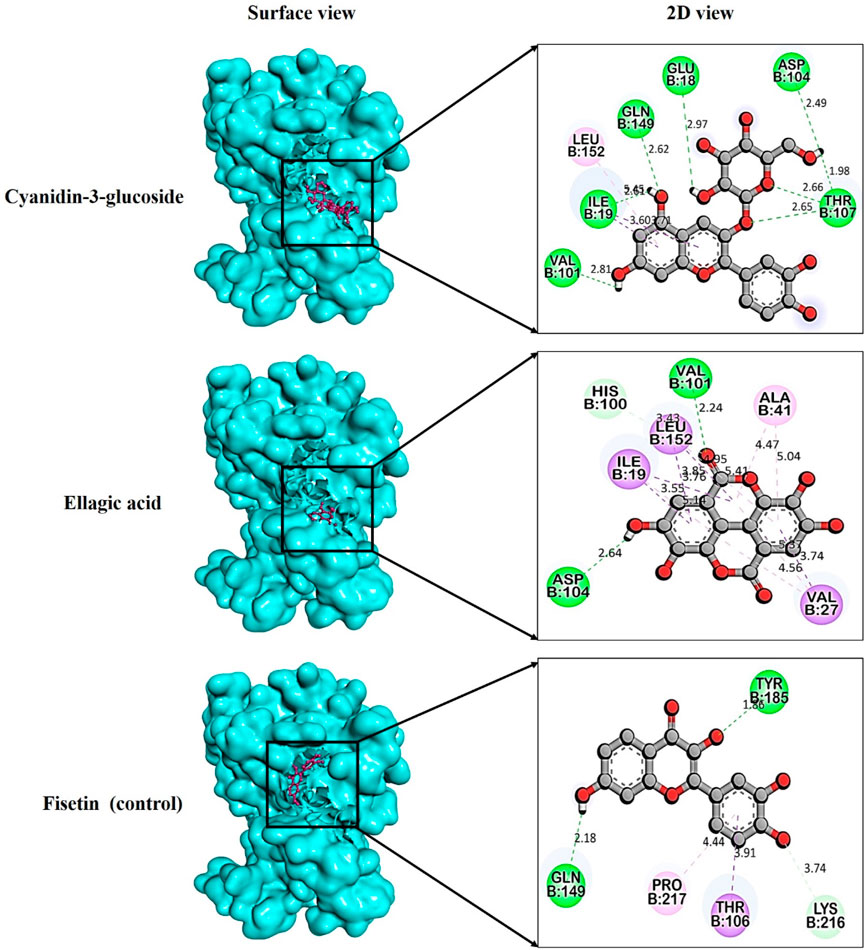
FIGURE 4. Docking pose targeting cyclin dependent kinase 6 (PDB ID: 1xo2) with phytochemicals from Verbascum diversifolium.
3.7 Molecular docking targeting tyrosinase
Tyrosinase and its associated proteins play a key role in melanin production, and inhibiting tyrosinase is a well-established approach for addressing hyperpigmentation issues. Many natural and synthetic antimelanogenic agents have shown promise in research, but often present with side effects like depigmentation, dermatitis, thyroid cancer, nephrotoxicity and genotoxicity (Maeda and Fukuda, 1996; Westerhof and Kooyers, 2005; Parvez et al., 2006; Chen et al., 2015; Ogiwara et al., 2015). Consequently, identifying new melanogenesis inhibitors is very important in medicine and cosmetics.
In this study, specific phytochemicals derived from V. diversifolium were docked as ligands targeting tyrosinase (PDB ID: 3awu) for assessing the inhibitory activity. Among the three complexes, 3awu + Isoquercetin exhibited the strongest binding affinity of −7.6 kcal/mol followed by 3awu + Rutin and 3awu + Neochlorogenic acid with binding affinity of −7.5 and −7.3 kcal/mol (Supplementary Table S3), respectively. Moreover, a total of four strong hydrogen bonds were identified for complex 3awu + Isoquercetin at Arg30, Asp69, Gln72, and Trp254 residues with bond length of approximately 1.25, 3.04, 2.84, and 2.59 Å (Figure 5A), correspondingly. The complex 3awu + Rutin revealed two hydrogen bonds at Arg30 (2.74Å) and Asp69 (2.57Å) residues (Figure 5B), respectively. Lastly, with a slightly lower binding affinity than other complexes, 3awu + Neochlorogenic acid displayed three hydrogen bonds at Arg30 (2.63Å), Asp69 (2.46Å) and Trp254 (2.86Å) residues (Figure 5C), in that order. The numerous hydrogen bonds identified in this molecular docking study provide valuable insights into the binding interactions between the ligand and the target protein, supporting the potential therapeutic relevance of the studied compounds.
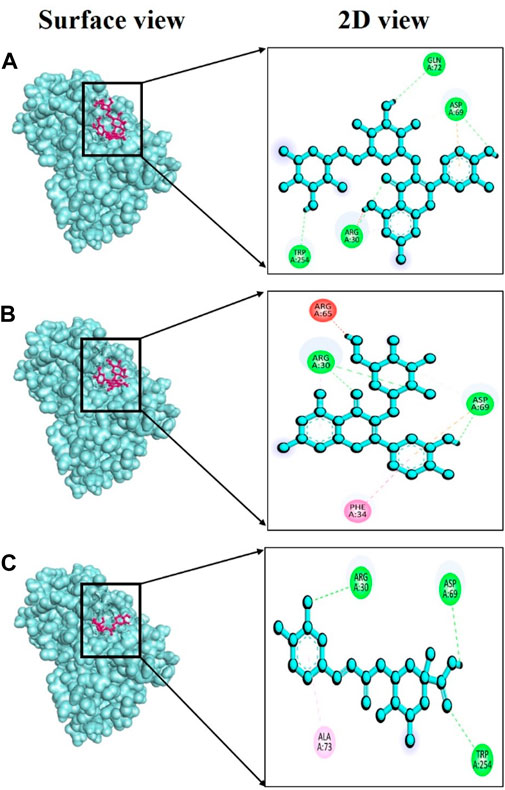
FIGURE 5. Docking interaction targeting tyrosinase (PDB ID: 3awu), where (A) Isoquercetin, (B) Rutin, (C) Neochlorogenic acid.
3.8 Molecular docking targeting alpha-amylase
Over time, various phenolic compounds have gained recognition for their strong antioxidant properties and their potential in managing diabetes mellitus. Examples of these plant phenolics include esculetin, umbelliferone, bergapten, emodin, and kaempferol, all of which have previously been studied for their ability to regulate hyperglycemia (Lin et al., 2016). Despite their promising potential, there have been fewer studies on the effects of these compounds from V. diversifolium. Therefore, in this study, we conducted in silico molecular docking assays to assess the alpha-amylase (PDB ID: 3baj) inhibitory activities of compounds isolated from V. diversifolium. Among the three complexes, 3baj + Rutin showed exceptionally strong binding energy of −9.4 kcal/mol (Supplementary Table S4) which indicates a robust interaction between the two molecules, and suggesting a potentially promising ligand-protein complex. Furthermore, this complex presented five hydrogen bonds at Thr163, Glu233, His299, Asp300 and His305 amino acid residues with bonding distance of 2.55, 2.83, 2.34, 2.19, and 2.13 Å (Figure 6A), correspondingly. The complex 3baj + Isoquercetin showed a binding affinity of −8.7 kcal/mol (Supplementary Table S4) revealing four hydrogen bonds at Arg195 (2.85 Å), Glu233 (2.67Å), His299 (2.60Å) and His305 (2.76Å) residues (Figure 6B) which also signifies a significant and favorable interaction between the two molecules. Lastly, 3baj + Neochlorogenic acid complex gave −7.9 kcal/mol binding affinity. Within this complex, three hydrogen bonds were identified at Gln63 (2.59Å), Thr163 (2.60Å), and Asp187 (2.56Å) residues (Figure 6C), respectively.
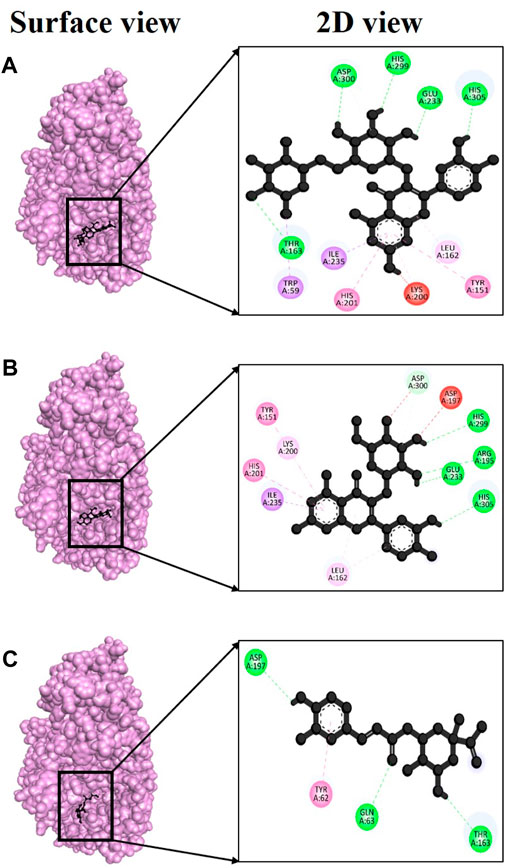
FIGURE 6. Docking interaction targeting alpha-amylase (PDB ID: 3baj), where (A) Isoquercetin, (B) Rutin, (C) Neochlorogenic acid.
3.9 Molecular docking targeting Alpha-glucosidase
Alpha-glucosidase (PDB ID: 3w37) is another critical enzyme involved in diabetes mellitus. Compounds capable of inhibiting alpha-glucosidase can slow down the digestion and absorption of carbohydrates. Consequently, they can help regulate postprandial hyperglycemia independently of insulin (Kazeem et al., 2013). Several types of synthetic medications like metformin, sulfonylureas, thiazolidinediones, biguanides, meglitinides, and dipeptidyl peptidase-IV inhibitors have already been developed to treat type 2 diabetes mellitus (Stein et al., 2013; Quan et al., 2019). However, prolonged use of these therapeutic agents may lead to severe side effects, including liver complications, hypoglycemia, and diarrhea, among others. Given these concerns, it has become essential to develop new inhibitors with high effectiveness and minimal toxicity. In this context, we assessed, using in silico docking approaches, whether phytochemicals from V. diversifolium possess inhibitory properties of Alpha-glucosidase. Among the three complexes studied, the interaction between 3w37 and rutin exhibited an exceptionally strong binding energy of −9.5 kcal/mol (Supplementary Table S5) indicating a dynamic interaction between the two molecules. This suggests a potentially promising ligand-protein complex. Additionally, in this complex, four hydrogen bonds were observed at Asp357, Met470, Ser474, and Arg552 amino acid residues, with bonding distances of 2.11, 2.94, 2.36, and 3.05 Å (Figure 7A), respectively. The complex involving 3w37 and kaempferol-3-glucoside showed a binding affinity of −7.4 kcal/mol (Supplementary Table S5), demonstrating a favorable interaction between the two molecules. This complex featured three hydrogen bonds at Glu105 (2.19 Å), Asn108 (2.30 Å), and Arg113 (2.55 Å) residues (Figure 7B), further emphasizing the significance of their interaction. Finally, the complex 3w37+ Isoquercetin had a binding affinity of −7.2 kcal/mol (Supplementary Table S5). Within this complex, two hydrogen bonds were identified at Asp232 (2.52 Å) and Asp568 (1.93 Å) residues (Figure 7C), contributing to the overall stability of the complex.
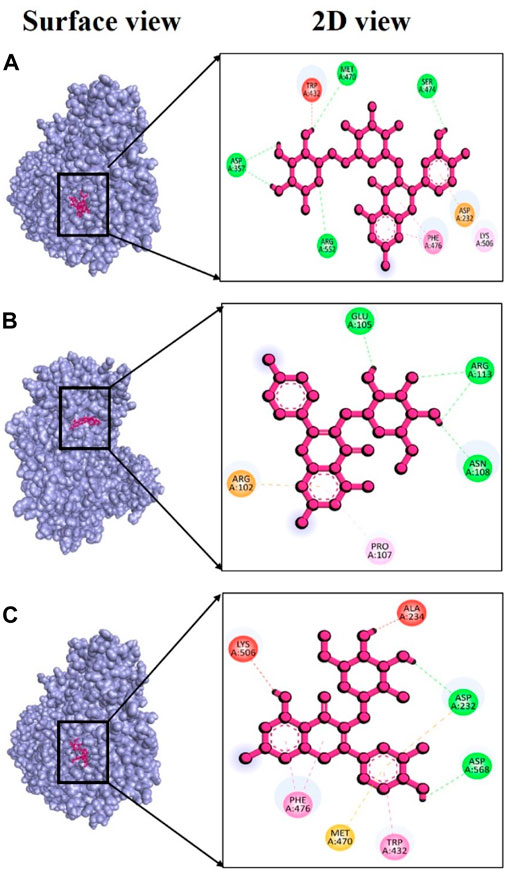
FIGURE 7. Docking interaction targeting alpha-glucosidase (PDB ID: 3w37), where (A) Rutin, (B) kaempferol-3-glucoside, (C) Isoquercetin.
3.10 Molecular docking targeting AChE and BChE
Alzheimer’s disease (AD) is a progressive and neurodegenerative condition associated with reduced levels of acetylcholine (Ach). In the brain, acetylcholine is primarily broken down by cholinesterase (ChE), making the inhibition of ChE an effective approach for treatment of AD. There are two types of ChE enzymes that exist in human nervous system: acetylcholinesterase (AChE) and butyrylcholinesterase (BChE). Currently, four anti- AChE agents—tacrine, rivastigmine, donepezil, and galantamine—are employed as drugs for Alzheimer’s disease (Hosen et al., 2023). However, their use is not without many severe side effects. Research findings suggest that as AD advances, there is an increase in the level and activity of butyrylcholinesterase (BChE), while conversely, the activity of acetylcholinesterase (AChE) decreases. Consequently, scientific research has shifted its focus towards the cholinergic system, particularly in the search for compounds that can inhibit AChE and BChE. Our ongoing research primarily centers on the identification of AChE (PDB ID: 4bdt) and BChE (PDB ID: 6qab) inhibitors using computer-based molecular docking method, leveraging phytochemicals derived from V. diversifolium plant.
In the case of AChE, the complex 4bdt + hyperoside displayed a notably strong binding energy of −9.0 kcal/mol (Supplementary Table S6), indicating a highly dynamic interaction between these two molecules. Notably, within this complex, four hydrogen bonds were identified at amino acid residues Asp74, Gln291, Ser293, and Tyr337, with bond distances of 2.50, 1.85, 2.83, and 2.27Å (Figure 8A), respectively. In the case of the complex involving 4bdt and isoquercetin, a binding affinity of −8.5 kcal/mol (Supplementary Table S6) was observed, signifying a favorable interaction between these molecules. This complex featured three hydrogen bonds at Pro235 (2.68 Å), Arg296 (2.79 Å), and Gln369 (2.33 Å) residues (Figure 8B), underscoring the significance of their interaction. The complex formed by 4bdt and neochlorogenic acid exhibited a binding affinity of −8.4 kcal/mol (Supplementary Table S6). Within this complex, four hydrogen bonds were detected at Asp74, Trp286, Arg296, and Tyr341 residues, with bond distances of 2.44, 1.87, 3.01, and 2.63Å (Figure 8C), respectively, contributing to the overall stability of the complex.
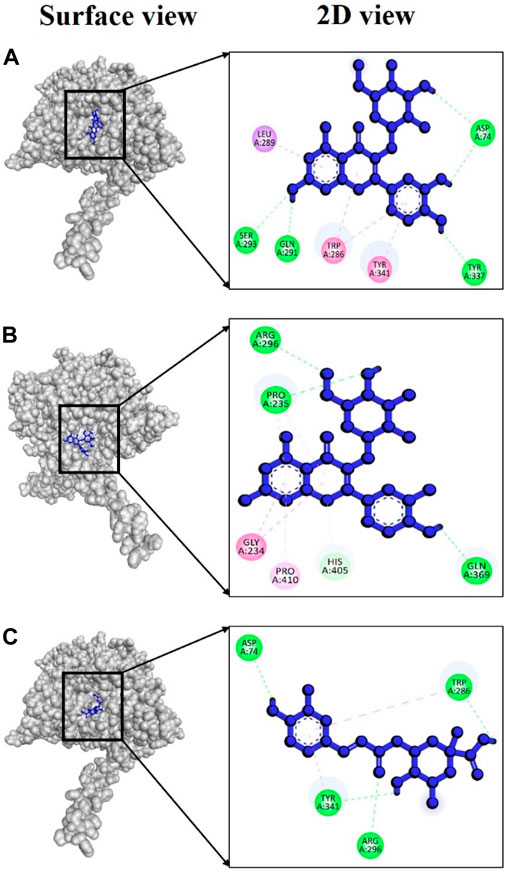
FIGURE 8. Docking interaction targeting AChE (PDB ID: 4bdt), where (A) Hyperoside, (B) Isoquercetin, (C) Neochlorogenic acid.
For BChE, the complex 6qab + rutin displayed a remarkably strong binding affinity, measured at −11.2 kcal/mol (Supplementary Table S7), indicating a highly favorable and stable interaction between the molecules involved. Notably, our analysis revealed the formation of six hydrogen bonds within this complex. These hydrogen bonds were established between specific amino acid residues involving Asp70, Gln119, Glu197, Ser198, Ser287 and Tyr332, with bond distances of 2.22, 2.42, 2.64, 2.53, 2.64, and 2.78 Å (Figure 9A), respectively, showcasing the complexity and strength of the molecular interaction. In the case of the complex involving 6qab + isoquercetin, a binding affinity of −10.8 kcal/mol (Supplementary Table S7) was observed. This complex featured seven hydrogen bonds at Gly78, Ser79, Gly115, Gln119, Tyr128, Glu197 and Ser198 with bond distances of 2.17, 2.89, 2.02, 3.00, 2.34, 2.29, and 2.03Å (Figure 9B), respectively, underscoring the significance of their interaction. The complex formed by 6qab + Neochlorogenic acid exhibited a binding affinity of −10.5 kcal/mol (Supplementary Table S7). Within this complex, six hydrogen bonds were detected at Gly78, Gly115, Gly117, Glu197, Ser198 and Pro285 residues, with bond distances of 2.27, 2.93, 2.93, 1.92, 2.03, and 2.85Å (Figure 9C), correspondingly.
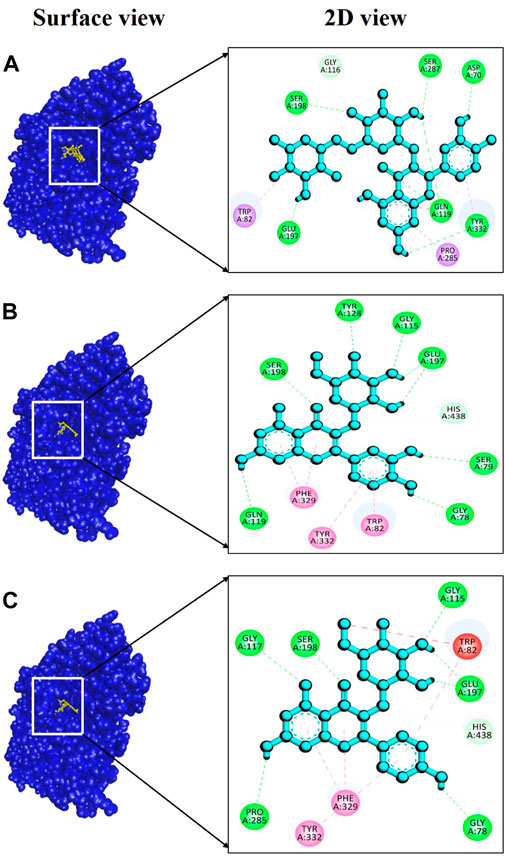
FIGURE 9. Docking interaction targeting BChE, (PDB ID: 6qab), where (A) Rutin, (B) Isoquercetin, (C) Neochlorogenic acid.
4 Conclusion
To the best of our knowledge, this is the first report assessing the chemical compoundss, antioxidant, enzyme inhibitory potentials and cytotoxicity of V. diversifolium. Chemical profile and biological activities varied according to the type of extraction solvent and the results showed that V. diversifolium possessed compounds with interesting biological activities and consequently, it can be a potential source of nutraceutical, pharmacological and cosmetic ingredients. However, evaluation of the morpho-anatomical changes during plant growth and developments and their impact on the chemical composition and biological activity of the plant would be of interest. Also, further research on the isolation of bioactive molecules and illustration their mechanism of action as well as in vivo studies are recommended.
Data availability statement
Data available upon reasonable requests. Requests to access the datasets should be directed to: gokhanzengin@selcuk.edu.tr (GZ) and ali.eid@qu.edu.qa (AE).
Ethics statement
Ethical approval was not required for the studies on humans in accordance with the local legislation and institutional requirements because only commercially available established cell lines were used.
Author contributions
SY: Conceptualization, Formal Analysis, Project administration, Writing–original draft. NN: Conceptualization, Investigation, Writing–original draft, Writing–review and editing. AU: Formal Analysis, Software, Writing–original draft. GC: Data curation, Project administration, Writing–review and editing. AM: Data curation, Investigation, Writing–review and editing. SA: Data curation, Investigation, Writing–review and editing. IK: Data curation, Investigation, Writing–original draft. FS: Data curation, Investigation, Writing–original draft. RP: Funding acquisition, Software, Writing–original draft. SS: Data curation, Investigation, Software, Writing–original draft. FT: Data curation, Investigation, Software, Writing–original draft. YA: Funding acquisition, Writing–review and editing. GZ: Conceptualization, Formal Analysis, Funding acquisition, Project administration, Writing–review and editing. AE: Conceptualization, Formal Analysis, Writing–review and editing.
Funding
The author(s) declare that no financial support was received for the research, authorship, and/or publication of this article.
Conflict of interest
The authors declare that the research was conducted in the absence of any commercial or financial relationships that could be construed as a potential conflict of interest.
The author(s) declared that they were an editorial board member of Frontiers, at the time of submission. This had no impact on the peer review process and the final decision.
Publisher’s note
All claims expressed in this article are solely those of the authors and do not necessarily represent those of their affiliated organizations, or those of the publisher, the editors and the reviewers. Any product that may be evaluated in this article, or claim that may be made by its manufacturer, is not guaranteed or endorsed by the publisher.
Supplementary material
The Supplementary Material for this article can be found online at: https://www.frontiersin.org/articles/10.3389/fphar.2024.1333865/full#supplementary-material
References
Abaza, M. S., Al-Attiyah, R., Bhardwaj, R., Abbadi, G., Koyippally, M., and Afzal, M. (2013). Syringic acid from Tamarix aucheriana possesses antimitogenic and chemo-sensitizing activities in human colorectal cancer cells. Pharm. Biol. 51 (9), 1110–1124. doi:10.3109/13880209.2013.781194
Abotaleb, M., Liskova, A., Kubatka, P., and Büsselberg, D. (2020). Therapeutic potential of plant phenolic acids in the treatment of cancer. Biomolecules 10 (2), 221. doi:10.3390/biom10020221
Afifi, M. A., Ahmed, M. M., Pezzuto, J. M., and Douglas Kinghornt, A. (1993). Cytotoxic flavonolignans and flavones from Verbascum sinaiticum leaves. Phytochemistry 34, 839–841. doi:10.1016/0031-9422(93)85369-3
Alam, F., Shafique, Z., and Amjad, S. (2019). Enzymes inhibitors from natural sources with antidiabetic activity: a review. Phytother. Res. 33, 41–54. doi:10.1002/ptr.6211
Alara, O. R., Abdurahman, N. H., and Ukaegbu, C. I. (2021). Extraction of phenolic compounds: a review. Curr. Res. Food Sci. 4, 200–214. doi:10.1016/j.crfs.2021.03.011
Aleixandre, A., Gil, J. V., Sineiro, J., and Rosell, C. M. (2022). Understanding phenolic acids inhibition of α-amylase and α-glucosidase and influence of reaction conditions. Food Chem. 372, 131231. doi:10.1016/j.foodchem.2021.131231
AlKahlout, A., Fardoun, M., Mesmar, J., Abdallah, R., Badran, A., Nasser, S. A., et al. (2022). Origanum syriacum L. Attenuates the malignant phenotype of MDA-MB231 breast cancer cells. Front. Oncol. 12, 922196. doi:10.3389/fonc.2022.922196
Alsamri, H., El Hasasna, H., Al Dhaheri, Y., Eid, A. H., Attoub, S., and Iratni, R. (2019). Carnosol, a natural polyphenol, inhibits migration, metastasis, and tumor growth of breast cancer via a ROS-dependent proteasome degradation of STAT3. Front. Oncol. 9, 743. doi:10.3389/fonc.2019.00743
Anantharaju, P. G., Gowda, P. C., Vimalambike, M. G., and Madhunapantula, S. V. (2016). An overview on the role of dietary phenolics for the treatment of cancers. Nutr. J. 15 (1), 99. doi:10.1186/s12937-016-0217-2
Angeloni, S., Zengin, G., Sinan, K. I., Ak, G., Maggi, F., Caprioli, G., et al. (2021). An insight into Verbascum bombyciferum extracts: different extraction methodologies, biological abilities and chemical profiles. Industrial Crops Prod. 161, 113201. doi:10.1016/j.indcrop.2020.113201
Anwar, M. A., Al Disi, S. S., and Eid, A. H. (2016). Anti-hypertensive herbs and their mechanisms of action: Part II. Front. Pharmacol. 7, 50. doi:10.3389/fphar.2016.00050
Anwar, M. A., Samaha, A. A., Ballan, S., Saleh, A. I., Iratni, R., and Eid, A. H. (2017). Salvia fruticosa induces vasorelaxation in rat isolated thoracic aorta: role of the PI3K/Akt/eNOS/NO/cGMP signaling pathway. Sci. Rep. 7 (1), 686. doi:10.1038/s41598-017-00790-9
Anwar, M. A., Samaha, A. A., Baydoun, S., Iratni, R., and Eid, A. H. (2018). Rhus coriaria L. (Sumac) evokes endothelium-dependent vasorelaxation of rat aorta: involvement of the cAMP and cGMP pathways. Front. Pharmacol. 9, 688. doi:10.3389/fphar.2018.00688
Aramouni, K., Assaf, R., Shaito, A., Fardoun, M., Al-Asmakh, M., Sahebkar, A., et al. (2023). Biochemical and cellular basis of oxidative stress: implications for disease onset. J. Cell Physiol. 238 (9), 1951–1963. doi:10.1002/jcp.31071
Athamneh, K., Alneyadi, A., Alsamri, H., Alrashedi, A., Palakott, A., El-Tarabily, K. A., et al. (2020). Origanum majorana essential oil triggers p38 MAPK-mediated protective autophagy, apoptosis, and caspase-dependent cleavage of P70S6K in colorectal cancer cells. Biomolecules 10 (3), 412. doi:10.3390/biom10030412
Athamneh, K., Hasasna, H. E., Samri, H. A., Attoub, S., Arafat, K., Benhalilou, N., et al. (2017). Rhus coriaria increases protein ubiquitination, proteasomal degradation and triggers non-canonical Beclin-1-independent autophagy and apoptotic cell death in colon cancer cells. Sci. Rep. 7 (1), 11633. doi:10.1038/s41598-017-11202-3
Badran, A., Nasser, S. A., Mesmar, J., El-Yazbi, A. F., Bitto, A., Fardoun, M. M., et al. (2020). Reactive oxygen species: modulators of phenotypic switch of vascular smooth muscle cells. Int. J. Mol. Sci. 21 (22), 8764. doi:10.3390/ijms21228764
Bender, O., and Atalay, A. (2021). “Chapter 28 - polyphenol chlorogenic acid, antioxidant profile, and breast cancer,” in Cancer. Editors V. R. Preedy, and V. B. Patel Second Edition (San Diego: Academic Press), 311–321.
Blanco-Salas, J., Hortigón-Vinagre, M. P., Morales-Jadán, D., and Ruiz-Téllez, T. (2021). Searching for scientific explanations for the uses of Spanish folk medicine: a review on the case of mullein (verbascum, Scrophulariaceae). Biology 10 (7), 618. doi:10.3390/biology10070618
Bouley, R., Kumarasiri, M., Peng, Z., Otero, L. H., Song, W., Suckow, M. A., et al. (2015). Discovery of antibiotic (E)-3-(3-carboxyphenyl)-2-(4-cyanostyryl) quinazolin-4 (3 H)-one. J. Am. Chem. Soc. 137 (5), 1738–1741. doi:10.1021/jacs.5b00056
Brewer, M. (2011). Natural antioxidants: sources, compounds, mechanisms of action, and potential applications. Compr. Rev. food Sci. food Saf. 10 (4), 221–247. doi:10.1111/j.1541-4337.2011.00156.x
Budryn, G., Majak, I., Grzelczyk, J., Szwajgier, D., Rodríguez-Martínez, A., and Pérez-Sánchez, H. (2022). Hydroxybenzoic acids as acetylcholinesterase inhibitors: calorimetric and docking simulation studies. Nutrients 14 (12), 2476. doi:10.3390/nu14122476
Cakilcioglu, U., Khatun, S., Turkoglu, I., and Hayta, S. (2011). Ethnopharmacological survey of medicinal plants in Maden (Elazig-Turkey). J. Ethnopharmacol. 137 (1), 469–486. doi:10.1016/j.jep.2011.05.046
Chen, L., Zhang, Y. H., Lu, G., Huang, T., and Cai, Y. D. (2017). Analysis of cancer-related lncRNAs using gene ontology and KEGG pathways. Artif. Intell. Med. 76, 27–36. doi:10.1016/j.artmed.2017.02.001
Chen, W.-C., Tseng, T. S., Hsiao, N. W., Lin, Y. L., Wen, Z. H., Tsai, C. C., et al. (2015). Discovery of highly potent tyrosinase inhibitor, T1, with significant anti-melanogenesis ability by zebrafish in vivo assay and computational molecular modeling. Sci. Rep. 5 (1), 7995. doi:10.1038/srep07995
Crespo, M. I., Chabán, M. F., Lanza, P. A., Joray, M. B., Palacios, S. M., Vera, D. M. A., et al. (2019). Inhibitory effects of compounds isolated from Lepechinia meyenii on tyrosinase. Food Chem. Toxicol. 125, 383–391. doi:10.1016/j.fct.2019.01.019
Dallakyan, S., and Olson, A. J. (2015). Small-molecule library screening by docking with PyRx. Chem. Biol. methods Protoc. 1263, 243–250. doi:10.1007/978-1-4939-2269-7_19
Dinani, M. S., Malakooti, S., and Akbari, V. (2020). In vitro cytotoxic activity of Verbascum alceoides against cervix carcinoma cells. J. Rep. Pharm. Sci. 9 (1), 19. doi:10.4103/jrptps.jrptps_65_19
Do, Q. D., Angkawijaya, A. E., Tran-Nguyen, P. L., Huynh, L. H., Soetaredjo, F. E., Ismadji, S., et al. (2014). Effect of extraction solvent on total phenol content, total flavonoid content, and antioxidant activity of Limnophila aromatica. J. Food Drug Anal. 22 (3), 296–302. doi:10.1016/j.jfda.2013.11.001
Donn, P., Barciela, P., Perez-Vazquez, A., Cassani, L., Simal-Gandara, J., and Prieto, M. A. (2023). Bioactive compounds of verbascum sinuatum L.: health benefits and potential as new ingredients for industrial applications. Biomolecules 13 (3), 427. doi:10.3390/biom13030427
El Gizawy, H. A. E. H., Hussein, M. A., and Abdel-Sattar, E. (2019). Biological activities, isolated compounds and HPLC profile of Verbascum nubicum. Pharm. Biol. 57 (1), 485–497. doi:10.1080/13880209.2019.1643378
El Hasasna, H., Athamneh, K., Al Samri, H., Karuvantevida, N., Al Dhaheri, Y., Hisaindee, S., et al. (2015). Rhus coriaria induces senescence and autophagic cell death in breast cancer cells through a mechanism involving p38 and ERK1/2 activation. Sci. Rep. 5, 13013. doi:10.1038/srep13013
El Hasasna, H., Saleh, A., Al Samri, H., Athamneh, K., Attoub, S., Arafat, K., et al. (2016). Rhus coriaria suppresses angiogenesis, metastasis and tumor growth of breast cancer through inhibition of STAT3, NFκB and nitric oxide pathways. Sci. Rep. 6, 21144. doi:10.1038/srep21144
Fardoun, M. M., Maaliki, D., Halabi, N., Iratni, R., Bitto, A., Baydoun, E., et al. (2020). Flavonoids in adipose tissue inflammation and atherosclerosis: one arrow, two targets. Clin. Sci. (Lond) 134 (12), 1403–1432. doi:10.1042/CS20200356
Gökmen, A., Kúsz, N., Karaca, N., Demirci, F., Hohmann, J., and Kırmızıbekmez, H. (2021). Secondary metabolites from Verbascum bugulifolium Lam. and their bioactivities. Nat. Prod. Res. 35 (23), 5294–5298. doi:10.1080/14786419.2020.1753052
Gomes, C. A., da Cruz, T. G., Andrade, J. L., Milhazes, N., Borges, F., and Marques, M. P. M. (2003). Anticancer activity of phenolic acids of natural or synthetic origin: a structure-activity study. J. Med. Chem. 46 (25), 5395–5401. doi:10.1021/jm030956v
Gong, J., Zhou, S., and Yang, S. (2019). Vanillic acid suppresses HIF-1α expression via inhibition of mTOR/p70S6K/4E-BP1 and raf/MEK/ERK pathways in human colon cancer HCT116 cells. Int. J. Mol. Sci. 20 (3), 465. doi:10.3390/ijms20030465
Grochowski, D. M., Uysal, S., Aktumsek, A., Granica, S., Zengin, G., Ceylan, R., et al. (2017). In vitro enzyme inhibitory properties, antioxidant activities, and phytochemical profile of Potentilla thuringiaca. Phytochem. Lett. 20, 365–372. doi:10.1016/j.phytol.2017.03.005
Guex, N., and Peitsch, M. C. (1997). SWISS-MODEL and the Swiss-Pdb Viewer: an environment for comparative protein modeling. electrophoresis 18 (15), 2714–2723. doi:10.1002/elps.1150181505
Hanwell, M. D., Curtis, D. E., Lonie, D. C., Vandermeersch, T., Zurek, E., and Hutchison, G. R. (2012). Avogadro: an advanced semantic chemical editor, visualization, and analysis platform. J. cheminformatics 4 (1), 17. doi:10.1186/1758-2946-4-17
Hashemzaei, M., Delarami Far, A., Yari, A., Heravi, R. E., Tabrizian, K., Taghdisi, S. M., et al. (2017). Anticancer and apoptosis-inducing effects of quercetin in vitro and in vivo. Oncol. Rep. 38 (2), 819–828. doi:10.3892/or.2017.5766
Hazman, Ö., Aksoy, L., Büyükben, A., Kara, R., Kargioğlu, M., Kumral, Z. B., et al. (2021). Evaluation of antioxidant, cytotoxic, antibacterial effects and mineral levels of Verbascum lasianthum Boiss. ex Bentham. An. Acad. Bras. Ciências 93, e20210865. doi:10.1590/0001-3765202120210865
Hosen, M. E., Rahman, M. S., Faruqe, M. O., Khalekuzzaman, M., Islam, M. A., Acharjee, U. K., et al. (2023). Molecular docking and dynamics simulation approach of Camellia sinensis leaf extract derived compounds as potential cholinesterase inhibitors. Silico Pharmacol. 11 (1), 14. doi:10.1007/s40203-023-00151-7
Iliescu, I. A., Peter, S., Albert, I., Skalicka-Woźniak, K., Miron, A., Luca, S. V., et al. (2020). Verbascum nigrum: cytotoxicity evaluation in A431 epidermoid carcinoma cells and untargeted LC-HR-MS/MS metabolite profiling. Chem. Biodivers. 17 (12), e2000644. doi:10.1002/cbdv.202000644
Kahraman, C., Akdemir, Z. S., and Tatli, I. I. (2012). Promising cytotoxic activity profile, biological activities and phytochemical screening of Verbascum L. species. Med. Aromat. Plant Sci. Biotechnol. 6 (2), 63–75.
Karavelioğullari, F. A., et al. (2011). Verbascum ergin-hamzaoglui (Scrophulariaceae), a new species from south anatolia, Turkey. Turkish J. Bot. 35 (3), 275–283. doi:10.3906/bot-1003-51
Kazeem, M., Adamson, J., and Ogunwande, I. (2013). Modes of inhibition of α-amylase and α-glucosidase by aqueous extract of Morinda lucida Benth leaf. BioMed Res. Int. 2013, 527570. doi:10.1155/2013/527570
Kim, J. H., Kismali, G., and Gupta, S. C. (2018). Natural products for the prevention and treatment of chronic inflammatory diseases: integrating traditional medicine into modern chronic diseases care. Evid. Based Complement. Altern. Med. 2018, 9837863. doi:10.1155/2018/9837863
Kim, S., Chen, J., Cheng, T., Gindulyte, A., He, J., He, S., et al. (2021). PubChem in 2021: new data content and improved web interfaces. Nucleic acids Res. 49 (D1), D1388–D1395. doi:10.1093/nar/gkaa971
Kıran, T. R., Otlu, O., and Karabulut, A. B. (2023). Oxidative stress and antioxidants in health and disease. J. Laboratory Med. 47 (1), 1–11. doi:10.1515/labmed-2022-0108
Kızıltaş, H., Kızıltaş, H., Bingöl, Z., Gören, A. C., and Alwasel, S. H. (2023). Analysis of phenolic compounds by LC-HRMS and determination of antioxidant and enzyme inhibitory properties of verbascum speciousum schrad. Rec. Nat. Prod. 17, 485–500. doi:10.25135/rnp.370.2210.2598
Küçük, S., Özdemir, F., İşcan, G., and İncesu, Z. (2016). Determination of cytotoxic and anticandidal activities of three verbascum L. Species from Turkey: V-cheiranthifolium Boiss. Var. asperulum (Boiss.) murb. Monorg., V-pycnostachyum Boiss. & heldr and V-orgyale Boiss. & heldr. Turkish J. Pharm. Sci. 13 (3), 318–322. doi:10.4274/tjps.2016.06
Kumar, S., Gupta, A., and Pandey, A. K. (2013b). Calotropis procera root extract has the capability to combat free radical mediated damage. ISRN Pharmacol. 2013, 691372. doi:10.1155/2013/691372
Kumar, S., Mishra, A., and Pandey, A. K. (2013a). Antioxidant mediated protective effect of Parthenium hysterophorus against oxidative damage using in vitro models. BMC Complementary Altern. Med. 13 (1), 120. doi:10.1186/1472-6882-13-120
Lancet, T. (2023). Diabetes: a defining disease of the 21st century. Lancet 401 (10394), 2087. doi:10.1016/S0140-6736(23)01296-5
Lin, D., Xiao, M., Zhao, J., Li, Z., Xing, B., Li, X., et al. (2016). An overview of plant phenolic compounds and their importance in human nutrition and management of type 2 diabetes. Molecules 21 (10), 1374. doi:10.3390/molecules21101374
Lomozová, Z., Hrubša, M., Conte, P. F., Papastefanaki, E., Moravcová, M., Catapano, M. C., et al. (2022). The effect of flavonoids on the reduction of cupric ions, the copper-driven Fenton reaction and copper-triggered haemolysis. Food Chem. 394, 133461. doi:10.1016/j.foodchem.2022.133461
Luca, S. V., Miron, A., Aprotosoaie, A. C., Mihai, C. T., Vochita, G., Gherghel, D., et al. (2019). HPLC-DAD-ESI-Q-TOF-MS/MS profiling of Verbascum ovalifolium Donn ex Sims and evaluation of its antioxidant and cytogenotoxic activities. Phytochem. Anal. 30 (1), 34–45. doi:10.1002/pca.2788
Maeda, K., and Fukuda, M. (1996). Arbutin: mechanism of its depigmenting action in human melanocyte culture. J. Pharmacol. Exp. Ther. 276 (2), 765–769.
Mesmar, J., Fardoun, M. M., Abdallah, R., Al Dhaheri, Y., Yassine, H. M., Iratni, R., et al. (2021). Ziziphus nummularia attenuates the malignant phenotype of human pancreatic cancer cells: role of ROS. Molecules 26 (14), 4295. doi:10.3390/molecules26144295
Mishra, A., Kumar, S., and Pandey, A. K. (2013). Scientific validation of the medicinal efficacy of Tinospora cordifolia. ScientificWorldJournal 2013, 292934. doi:10.1155/2013/292934
Molole, G. J., Gure, A., and Abdissa, N. (2022). Determination of total phenolic content and antioxidant activity of Commiphora mollis (Oliv.) Engl. resin. BMC Chem. 16 (1), 48. doi:10.1186/s13065-022-00841-x
Ogiwara, Y., Sugiura, M., Watanabe, K., Tawara, J., Endo, E., Maruyama, H., et al. (2015). Evaluation of the repeated-dose liver, bone marrow and peripheral blood micronucleus and comet assays using kojic acid. Mutat. Research/Genetic Toxicol. Environ. Mutagen. 780, 111–116. doi:10.1016/j.mrgentox.2015.01.004
Parvez, S., Kang, M., Chung, H. S., Cho, C., Hong, M. C., Shin, M. K., et al. (2006). Survey and mechanism of skin depigmenting and lightening agents. Phytotherapy Res. Int. J. Devoted Pharmacol. Toxicol. Eval. Nat. Prod. Deriv. 20 (11), 921–934. doi:10.1002/ptr.1954
Quan, N. V., Tran, H. D., Xuan, T. D., Ahmad, A., Dat, T. D., Khanh, T. D., et al. (2019). Momilactones A and B are α-amylase and α-glucosidase inhibitors. Molecules 24 (3), 482. doi:10.3390/molecules24030482
Rababah, T. M., Ereifej, K. I., Esoh, R. B., Al-u'datt, M. H., Alrababah, M. A., and Yang, W. (2011). Antioxidant activities, total phenolics and HPLC analyses of the phenolic compounds of extracts from common Mediterranean plants. Nat. Prod. Res. 25 (6), 596–605. doi:10.1080/14786419.2010.488232
Reuter, S., Gupta, S. C., Chaturvedi, M. M., and Aggarwal, B. B. (2010). Oxidative stress, inflammation, and cancer: how are they linked? Free Radic. Biol. Med. 49 (11), 1603–1616. doi:10.1016/j.freeradbiomed.2010.09.006
Rice-Evans, C. A., Miller, N. J., and Paganga, G. (1996). Structure-antioxidant activity relationships of flavonoids and phenolic acids. Free Radic. Biol. Med. 20 (7), 933–956. doi:10.1016/0891-5849(95)02227-9
Samaha, A. A., Fawaz, M., Salami, A., Baydoun, S., and Eid, A. H. (2019). Antihypertensive indigenous Lebanese plants: Ethnopharmacology and a clinical trial. Biomolecules 9 (7), 292. doi:10.3390/biom9070292
Santos, T. C. D., Gomes, T. M., Pinto, B. A. S., Camara, A. L., and de Andrade Paes, A. M. (2018). Naturally occurring acetylcholinesterase inhibitors and their potential use for Alzheimer's disease therapy. Front. Pharmacol. 9, 1192. doi:10.3389/fphar.2018.01192
Segun, P. A., Ogbole, O. O., Ismail, F. M. D., Nahar, L., Evans, A. R., Ajaiyeoba, E. O., et al. (2019). Resveratrol derivatives from Commiphora africana (A. Rich.) Endl. display cytotoxicity and selectivity against several human cancer cell lines. Phytother. Res. 33 (1), 159–166. doi:10.1002/ptr.6209
Shaito, A., Aramouni, K., Assaf, R., Parenti, A., Orekhov, A., Yazbi, A. E., et al. (2022). Oxidative stress-induced endothelial dysfunction in cardiovascular diseases. Front. Biosci. (Landmark Ed. 27 (3), 105. doi:10.31083/j.fbl2703105
Sharma, S. H., Chellappan, D. R., Chinnaswamy, P., and Nagarajan, S. (2017). Protective effect of p-coumaric acid against 1,2 dimethylhydrazine induced colonic preneoplastic lesions in experimental rats. Biomed. Pharmacother. 94, 577–588. doi:10.1016/j.biopha.2017.07.146
Sharma, S. H., Rajamanickam, V., and Nagarajan, S. (2018). Antiproliferative effect of p-Coumaric acid targets UPR activation by downregulating Grp78 in colon cancer. Chem. Biol. Interact. 291, 16–28. doi:10.1016/j.cbi.2018.06.001
Sharma, S. H., Rajamanickam, V., and Nagarajan, S. (2019). Supplementation of p-coumaric acid exhibits chemopreventive effect via induction of Nrf2 in a short-term preclinical model of colon cancer. Eur. J. Cancer Prev. 28 (6), 472–482. doi:10.1097/CEJ.0000000000000496
Slika, H., Mansour, H., Wehbe, N., Nasser, S. A., Iratni, R., Nasrallah, G., et al. (2022). Therapeutic potential of flavonoids in cancer: ROS-mediated mechanisms. Biomed. Pharmacother. 146, 112442. doi:10.1016/j.biopha.2021.112442
Slinkard, K., and Singleton, V. L. (1977). Total phenol analysis: automation and comparison with manual methods. Am. J. enology Vitic. 28 (1), 49–55. doi:10.5344/ajev.1974.28.1.49
Spiegel, M., Kapusta, K., Kołodziejczyk, W., Saloni, J., Żbikowska, B., Hill, G. A., et al. (2020). Antioxidant activity of selected phenolic acids–ferric reducing antioxidant power assay and QSAR analysis of the structural features. Molecules 25 (13), 3088. doi:10.3390/molecules25133088
Srinivasulu, C., Ramgopal, M., Ramanjaneyulu, G., Anuradha, C. M., and Suresh Kumar, C. (2018). Syringic acid (SA)‒a review of its occurrence, biosynthesis, pharmacological and industrial importance. Biomed. Pharmacother. 108, 547–557. doi:10.1016/j.biopha.2018.09.069
Stein, S. A., Lamos, E. M., and Davis, S. N. (2013). A review of the efficacy and safety of oral antidiabetic drugs. Expert Opin. drug Saf. 12 (2), 153–175. doi:10.1517/14740338.2013.752813
Sunday Joel, J., Sheena, O. E., Martins, O. E., Onyemauche, N. S. C., and Emmanuel, A. A. (2017). Comparative antioxidant capacity of aqueous and ethanol fruit extracts of tetrapleura tetraptera. J. Biol. Sci. 17, 185–193. doi:10.3923/jbs.2017.185.193
Sung, H., Ferlay, J., Siegel, R. L., Laversanne, M., Soerjomataram, I., Jemal, A., et al. (2021). Global cancer statistics 2020: GLOBOCAN estimates of incidence and mortality worldwide for 36 cancers in 185 countries. CA Cancer J. Clin. 71 (3), 209–249. doi:10.3322/caac.21660
Szwajgier, D. (2015). Anticholinesterase activity of selected phenolic acids and flavonoids-interaction testing in model solutions. Ann. Agric. Environ. Med. 22 (4), 690–694. doi:10.5604/12321966.1185777
Talib, W. H., and Mahasneh, A. M. (2010). Antiproliferative activity of plant extracts used against cancer in traditional medicine. Sci. Pharm. 78 (1), 33–45. doi:10.3797/scipharm.0912-11
Tatli, I., and Akdemir, Z. (2006a). Traditional uses and biological activities of Verbascum species. Fabad J. Pharm. Sci. 31, 85–96.
Tatli, I. I., and Akdemir, Z. S. (2006b). Cytotoxic activity on some Verbascum species growing in Turkey. Hacettepe Univ. J. Fac. Pharm. (2), 77–86.
Tauchen, J., Doskocil, I., Caffi, C., Lulekal, E., Marsik, P., Havlik, J., et al. (2015). In vitro antioxidant and anti-proliferative activity of Ethiopian medicinal plant extracts. Industrial Crops Prod. 74, 671–679. doi:10.1016/j.indcrop.2015.05.068
Tita, I., Mogosanu, G. D., and Tita, M. G. (2009). Ethnobotanical inventory of medicinal plants from the South-West of Romania. Farmacia 57 (2), 141–156.
Westerhof, W., and Kooyers, T. (2005). Hydroquinone and its analogues in dermatology–a potential health risk. J. Cosmet. dermatology 4 (2), 55–59. doi:10.1111/j.1473-2165.2005.40202.x
WHO (2023a). Diabetes. Geneva, Switzerland: World Health Organization. Available at: https://www.who.int/news-room/fact-sheets/detail/diabetes (Accessed August 27, 2023).
Zengin, G., Mahomoodally, M. F., Sinan, K. I., Sadeer, N., Maggi, F., Caprioli, G., et al. (2021). Evaluation of chemical constituents and biological properties of two endemic Verbascum species. Process Biochem. 108, 110–120. doi:10.1016/j.procbio.2021.06.007
Zengin, G., Yagi, S., Kopjar, M., Ćorković, I., Uba, A. I., Yildiztugay, E., et al. (2023). A comparative study on chemical profiles and biological activities of different extracts of three Verbascum species from Turkey: in vitro, in silico and network pharmacological approaches. Biocatal. Agric. Biotechnol. 52, 102834. doi:10.1016/j.bcab.2023.102834
Keywords: cancer, cardiovascular disease, Herbal medicine, oxidative stress, reactive oxgen species (ROS)
Citation: Yagi S, Nilofar N, Uba AI, Caprioli G, Mustafa AM, Angeloni S, Koyuncu I, Seker F, Polat R, Supti SJ, Tasnim F, Al Dhaheri Y, Zengin G and Eid AH (2024) Elucidating the chemical profile and biological studies of Verbascum diversifolium Hochst. extracts. Front. Pharmacol. 15:1333865. doi: 10.3389/fphar.2024.1333865
Received: 06 November 2023; Accepted: 03 January 2024;
Published: 30 January 2024.
Edited by:
Stalin Antony, University of Electronic Science and Technology of China, ChinaReviewed by:
Songül Karakaya, Atatürk University, TürkiyeMassoud Ranjbar, Bu-Ali Sina University, Iran
Copyright © 2024 Yagi, Nilofar, Uba, Caprioli, Mustafa, Angeloni, Koyuncu, Seker, Polat, Supti, Tasnim, Al Dhaheri, Zengin and Eid. This is an open-access article distributed under the terms of the Creative Commons Attribution License (CC BY). The use, distribution or reproduction in other forums is permitted, provided the original author(s) and the copyright owner(s) are credited and that the original publication in this journal is cited, in accordance with accepted academic practice. No use, distribution or reproduction is permitted which does not comply with these terms.
*Correspondence: Gokhan Zengin, gokhanzengin@selcuk.edu.tr; Ali H. Eid, ali.eid@qu.edu.qa