- 1Department of Oncology, Xiangya Hospital, Central South University, Changsha, China
- 2Changsha Stomatological Hospital, Hunan University of Traditional Chinese Medicine, Changsha, China
- 3Department of Pharmacy, Xiangya Hospital, Changsha, China
- 4Department of Radiation Oncology and Department of Head and Neck Oncology, Cancer Center, West China Hospital, Sichuan University, Chengdu, Sichuan, China
- 5Xiangya Lung Cancer Center, Xiangya Hospital, Central South University, Changsha, China
- 6National Clinical Research Center for Geriatric Disorders, Xiangya Hospital, Central South University, Changsha, Hunan, China
Background: Tumor treating fields (TTF) was first approved for treatment of glioblastoma. Recently, the LUNAR study demonstrated that TTF + standard therapy (ST) extended survival in patients with advanced non-small cell lung cancer (NSCLC). This primary objective of this study is to analyze the cost-effectiveness of this treatment from the United States healthcare payers’ perspective.
Methods: A 3-health-state Markov model was established to compare the cost-effectiveness of TTF + ST and that of ST alone. Clinical data were extracted from the LUNAR study, supplemented by additional cost and utility data obtained from publications or online sources. One-way sensitivity analysis, probabilistic sensitivity analysis, and scenario analysis were conducted. The willingness-to-pay (WTP) threshold per quality-adjusted life-years (QALYs) gained was set to $150,000. The main results include total costs, QALYs, incremental cost-effectiveness ratio (ICER) and incremental net monetary benefit (INMB). Subgroup analyses were conducted for two types of ST, including immune checkpoint inhibitor, and docetaxel.
Results: During a 10-year time horizon, the costs of TTF + ST and ST alone were $431,207.0 and $128,125.9, and the QALYs were 1.809 and 1.124, respectively. The ICER of TTF + ST compared to ST was $442,732.7 per QALY, and the INMB was -$200,395.7 at the WTP threshold. The cost of TTF per month was the most influential factor in cost-effectiveness, and TTF + ST had a 0% probability of being cost-effective at the WTP threshold compared with ST alone.
Conclusion: TTF + ST is not a cost-effective treatment for advanced NSCLC patients who progressed after platinum-based therapy from the perspective of the United States healthcare payers.
Introduction
Non-small cell lung cancer (NSCLC) stands as the leading cause of cancer-related death, with a 5-year survival rate of approximately 23% (Siegel et al., 2023). Despite advancement in imaging technology and the widespread adoption of CT screening, over 55% of patients are still diagnosed at an advanced stage (Siegel et al., 2020). For individuals with metastatic NSCLC, the prospect of surgical cure diminishes. Systemic treatments such as targeted therapy, immunotherapy, radiotherapy, and chemotherapy serve to prolong their survival and improve the quality of life, (Chen et al., 2020). The predominant approach for patients with driver gene-negative advanced NSCLC is platinum-based chemotherapy (Clegg et al., 2001), but the inevitability of subsequent resistance poses a substantial challenge (Bluthgen and Besse, 2015; Barnfield and Ellis, 2016). Thus, finding new treatments is an urgent problem that needs to be solved.
Tumor treating fields (TTF) is a non-invasive tumor physical therapy, demonstrating significant therapeutic efficacy, convenience, and minimal adverse reactions in recurrent glioblastoma (Kirson et al., 2004). Further, the National Comprehensive Cancer Network (NCCN) recommended TTF for treating recurrent and newly diagnosed glioblastoma in 2013 and 2016, respectively (Petoukhova et al., 2023; Swartz et al., 2023). Preclinical research focusing on NSCLC suggests that TTF induces immunogenic death of tumor cell, enhances antigen presentation of dendritic cells and leukocyte chemotaxis, and synergizes with PD-1/PD-L1 inhibitors to inhibit tumor growth (Giladi et al., 2014; Giladi et al., 2015; Karanam et al., 2017; Shteingauz et al., 2018; Voloshin et al., 2020). Meanwhile, the combination of TTF with chemotherapy agents, such as cisplatin, paclitaxel, and pemetrexed has demonstrated inhibitory effects on NSCLC growth (Giladi et al., 2014). In terms of clinical applications for NSCLC patients, the phase 1/2 EF-15 (NCT00749346) clinical trial revealed that second-line TTF, in combination with chemotherapy, extended the median overall survival (mOS) of 42 patients with stage IIIB (with pleural effusion) or IV NSCLC to 13.8 months (Pless et al., 2013). Recently, the LUNAR (NCT02973789) trial confirmed the favorable efficacy and safety of TTF in combination with standard therapy (ST), including immune checkpoint inhibitor (ICI) or docetaxel, for metastatic NSCLC that has progressed after platinum-based therapy.
The LUNAR trial was a randomized, open-label, pivotal phase 3 study that included 276 patients from 130 regions in 19 countries (Leal et al., 2023). The mOS of the TTF + ST group and that of the ST group were 13.2 months and 9.5 months, respectively. Subgroup analysis indicated that the mOS of the TTF + docetaxel group was 11.1 month, while that of the docetaxel alone group was 8.7 months. Similarly, the TTF + ICI group exhibited a mOS of 18.5 months, whereas the ICI group had a mOS of 10.8 months. The median progression-free survival (mPFS) was 8 months and 4.1 months respectively in the TTF + ST group and the ST group. The mPFS was 4.4 months in the TTF + docetaxel group and 4.2 months in the docetaxel alone group. Similarly, the TTF + ICI group displayed a mPFS of 5.9 months, compared to 4.0 months in the ICI alone group (Leal et al., 2023).
Here, we aimed to investigate the cost-effectiveness of TTF + ST in the treatment of advanced NSCLC which had progressed after platinum-based chemotherapy, and explore the cost-effectiveness within subgroups by ST type, including ICI and docetaxel.
Materials and methods
This study strictly follows the Consolidated Health Economic Evaluation Reporting Standards 2022 (CHEERS 2022). We used R (version 4.2.1) for analyzation and visualization.
Model construction
We utilized the “heemod” package to construct a Markov model featuring 21-day cycles for simulating the health status transitions of the patients in the LUNAR study. The model included three states, PFS, progressive disease (PD), and death, with death as the absorbing state (Kevin Zarca et al., 2023; Leal et al., 2023). The time horizon was set to 10 years, considering that over 95% of patients were assumed to be dead after 10 years of treatment. The transition probabilities from PFS to PD and from PD to death was determined based on the PFS and OS data and/or natural modality rate. Additionally, the transition probability from PFS to death was estimated to be equal to the natural modality rate automatically obtained from the “heemod” package (Kevin Zarca et al., 2023) (Figure 1).
In the LUNAR study, 137 patients underwent TTF (150 kHz, 18 h per day, NovoTTF device system) + ST, while 139 patients received ST [ICIs: nivolumab, pembrolizumab, or atezolizumab; or docetaxel]. Among patients who received ICIs, 2/3 were treated with nivolumab, 1/6 with pembrolizumab, and 1/6 with atezolizumab (Leal et al., 2023). To resemble the design of the LUNAR study, we defined the docetaxel treatment as receiving 75 mg/m2 of docetaxel over 1 h every 3 weeks, the ICI treatment as taking pembrolizumab (200 mg every 3 weeks), atezolizumab (1,200 mg every 3 weeks), or nivolumab (240 mg every 2 weeks) in a 1:1:2 ratio. The TTF treatment was defined as receiving TTF every day. Furthermore, the TTF + ST was defined as undergoing TTF + ICI or TTF + docetaxel in a 66:71 ratio, and the ST was defined as taking ICI or docetaxel alone in a 71:68 ratio (Leal et al., 2023).
We set a relatively high willingness-to-pay (WTP) threshold of $150,000 per quality-adjusted life-years (QALYs) gained because of the high inflation rate in the United States in recent years and an increasing popularity of this WTP threshold in cost-effectiveness analyses nowadays (Neumann et al., 2014; Neumann and Kim, 2023). As for cost-effectiveness results, we focused on overall costs, QALYs, ICERs, and incremental net monetary benefit (INMB) to evaluate cost-effectiveness.
Clinical data
OS and PFS data for the TTF + ST, ST, TTF + ICI, IC, TTF + docetaxel, and docetaxel groups were extracted from the LUNAR study using the GetData Graph Digitizer (version 2.26). Subsequently, the “IPDfromKM” package (Liu et al., 2021) was employed to reconstruct individual patient data, which were then adopted to recreate Kaplan-Meier Curves to ensure the accuracy (Supplementary Figure S1).
To obtain long-term OS and PFS survival curves, we applied a variety of distributions in the “flexsurv” package (including exponential, Weibull, log-logistic, log-normal, generalized gamma, gamma, Gompertz, and Roystone/Parmar distributions) to fit the individual patient data. The Akaike Information Criterion (AIC) and Bayesian Information Criterion (BIC) values were calculated to identify the best distribution for each dataset (Supplementary Table S1). Additionally, we collected the incidences of various adverse events, discontinuation rates, and subsequent treatments after progression for the main analysis (Table 1) and subgroup analyses (Supplementary Table S2), from the LUNAR study.
Cost and utility data
In this study, we evaluated the cost-effectiveness from the perspective of the United States healthcare payers. We incorporated a wide range of cost sources, including cancer treatment, supportive care, laboratory tests and scans, managements of adverse events, intravenous administration, and hospitalization. To estimate the dose of agents, we assumed a typical 65-year-old patient with 70 kg in weight and 1.8 m2 in body surface area (BSA) (Liu et al., 2021). Drug costs were sourced from the Centers for Medicare and Medicaid Services or based on published articles, with adjustments made for inflation (Insinga et al., 2018; Wong et al., 2018; Criss et al., 2019; Ondhia et al., 2019; De Marzi et al., 2020; Dutt et al., 2020; Zhang et al., 2020; Rothwell et al., 2021; Gong et al., 2022; Li et al., 2022; Shen et al., 2022). Utilities for PFS and PD, scaling from 0 (death) to 1 (perfect health), and disutility of adverse events were derived from previously published studies (Chouaid et al., 2013; Amdahl et al., 2016). Costs and utilities were discounted at an annual rate of 5% (Smith and Gravelle, 2001) (Table 1).
Sensitivity analysis
We performed one-way sensitivity analysis and probabilistic sensitivity analysis to evaluate the model’s robustness. In one-way sensitivity analysis, we varied the body weight, body surface area, cost, and proportion values by ±25%. Discount rates were varied between 0% and 8%, and utility/disutility values were adjusted by ±10%. For proportions with a baseline value of 0%, the lower and upper boundary were set to 0% and 1%, respectively. In probabilistic sensitivity analysis, we repeated 1,000 Monte Carlo iterations for all distributions assigned to costs (γ distribution), proportions (β distribution), utilities (β distribution), body surface area (normal distribution), body weight (normal distribution), and the discount rate (uniform distribution). The mean of the distributions was set to the baseline value, with the standard deviations set to make the boundaries of the 95% confidence intervals to approach the boundary values used in the one-way sensitivity analysis.
Scenario analysis
Considering the costs and effects may vary greatly over time from the initiation of treatment, we further estimated cost-effectiveness of all the 6 treatments setting the time horizons to 5 years, 8 years, and 15 years, respectively.
Results
Base-case results
We constructed a Markov model and predicted the 10-year survival and treatment status of patients in the LUNAR study (Table 2). For the base case scenario, 10-year average costs of TTF + ST and ST alone were $434,969.3 and $130,571.3 per patient, while the corresponding QALYs were 1.809 and 1.124, respectively. The incremental costs and QALYs for TTF + ST compared with ST alone were $304,398.0 and 0.685, respectively, resulting in an ICER of $444,656.4 per QALY. Meanwhile, at a WTP threshold of $150,000 per QALY, the INMB of TTF + ST compared with ST alone was -$201,712.6.
Furthermore, the subgroup analysis involving TTF + ICI, ICI alone, TTF + docetaxel, and docetaxel alone revealed that 10-year average costs for these treatments were $897,027.2, $238,404.7, $221,648.7, $92,599.1, respectively. The corresponding QALYs were 2.318, 1.399, 1.289, 1.035, respectively. Compared with the docetaxel group, the incremental costs for the TTF + ICI, ICI alone, and TTF + docetaxel group were $804,428.1, $145,805.6, and $129,049.6, and the incremental QALYs were 1.283, 0.364, and 0.253, which yielded ICERs of $627,315.5, $401,107.9, and $509,139.1 per QALY gained, and INMBs of -$612,078.0, -$91,279.5, and -$91,029.7, respectively. Moreover, compared with TTF + docetaxel, the incremental costs of TTF + ICI and ICI alone were $675,378.5 and $16,756.0, with the incremental QALYs of 1.029 and 0.11, respectively, which generated ICERs of $656,428.8 and $152,207.6 per QALY gained and INMBs of -$521,048.3 and -$249.9, respectively. Last, the incremental cost and incremental QALY of TTF + ICI compared with ICI alone were $658,622.5 and 0.919, yielding an ICER of $716,807.9 per QALY gained and an INMB of -$520,798.4 (Table 3).
Sensitivity analyses
Figure 2 displayed the 20 most influential factors identified in the one-way sensitivity analysis. The cost of TTF per month emerged as the top factor influencing the ICER. As the cost varied between its lower boundary ($20,410.86) and upper boundary ($34,018.1), the ICER fluctuated from $351,099.2 to $538,213.6 per QALY gained. The ICER was also sensitive to the utility of survival with disease progression (ICER ranging from $486,052.7 to $409,757.0 per QALY gained), discount rate (ICER ranging from $407,087.6 to $467,814.7 per QALY gained), the cost of best supportive care per cycle (ICER ranging from $433,225.9 to $456,086.9 per QALY gained), and the utility of progression-free survival (ICER ranging from $451,341.0 to $438,166.9 per QALY gained). Furthermore, we assigned a range of $0 to $30,000 for the cost of TTF per month to evaluate the corresponding INMB of TTF + ST versus ST alone at the WTP threshold of $150,000 per QALY gained, and the results showed that the INMB became positive when the cost of TTF per month was below $5,787 (Supplementary Figure S5A). According to the probabilistic sensitivity analysis (Figure 3), the total cost of TTF + ST ranged from $333,554.6 to $589,389.5, while that of ST alone ranged from $96,268.2 to $186,130.7. The QALYs of them ranged from 1.513 to 2.173 and from 0.947 to 1.299, respectively. Accordingly, all the resamples yielded positive ICER, ranging from $292,215.0 to $638,461.3 per QALY gained, which indicated that the probability of TTF + ST being cost-effective was 0% at the WTP threshold of $150,000 per QALY gained.
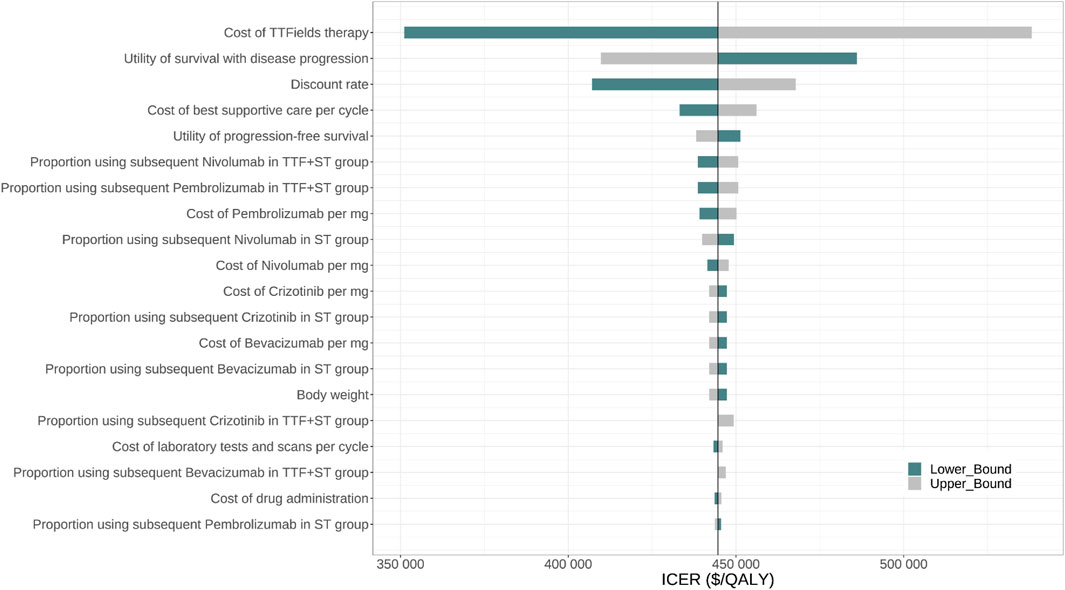
FIGURE 2. One-way sensitivity analysis for TTF + Standard therapy vs. Standard therapy. Abbr. TTF, tumor treating fields; ST, standard therapy; ICER, incremental cost-effectiveness ratio; QALY, quality-adjusted life-year.
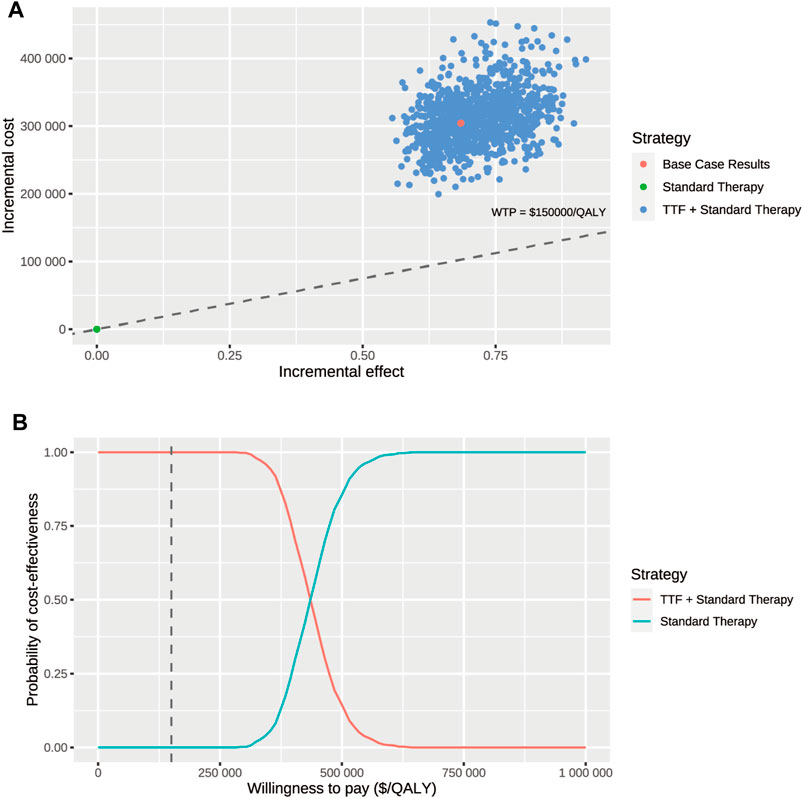
FIGURE 3. Probabilistic sensitivity analysis. (A) Incremental cost ($) and incremental effect (QALY) incurred by 1,000 probabilistic resamplings per strategy. (B) Probability of cost-effectiveness at varying willingness-to-pay. The dashed line represents the willing-to-pay threshold of $150,000 per QALY gained. Abbr. TTF, tumor-treating fields; WTP, willingness-to-pay.
In terms of the subgroups, we conducted one-way sensitivity analyses for all 6 pairwise comparisons, including TTF + ICI vs. docetaxel alone, TTF + docetaxel vs. docetaxel alone, TTF + ICI vs. TTF + docetaxel, ICI alone vs. TTF + docetaxel, and ICI alone vs. docetaxel alone, the results of which were demonstrated in the Supplementary Figures S4A–F. In the first five comparisons, the cost of TTF per month remained the most influential factor in determining the ICERs, while in the comparison of ICI alone vs. docetaxel alone, the most influential factor was the cost of nivolumab per mg (Supplementary Figure S4F). We also conducted a probabilistic sensitivity analysis for the 4 treatments. The results showed that the probabilities of TTF + ICI, TTF + docetaxel, and ICI alone being cost-effective were 0% at a WTP threshold of $150,000 per QALY gained (Supplementary Figures S4G, H). Similarly, we explored the influences of the cost of TTF per month on the INMBs of TTF + ICI versus docetaxel, TTF + ICI versus ICI, TTF + docetaxel versus docetaxel, and ICI versus TTF + docetaxel. The results showed that the INMB of TTF + docetaxel versus docetaxel became positive when the cost of TTF per month was below $8,381, and the INMB of ICI versus TTF + docetaxel became negative when the cost was below $27,266 per month (Supplementary Figure S4B). Notably, the INMB of TTF + ICI versus docetaxel and that of TTF + ICI versus ICI stayed negative even if the cost of TTF was $0 per month (Supplementary Figure S4B).
Scenario analysis
The base case results were based on a 10-year time frame. To investigate the effect of setting the time horizon in affecting cost-effectiveness. We further assessed the cost-effectiveness of all the 6 treatments in 5-year, 8-year, and 15-year time horizons. Specifically, the ICER of TTF + ST compared with ST alone decreased from $559933.7 per QALY gained (5 years) to $467513.0 per QALY gained (8 years) and then to $420263.5 per QALY gained (15 years). Besides, the ICER of TTF + ICI vs. docetaxel, the ICER of TTF + ICI vs. TTF + docetaxel, the ICER of ICI vs. docetaxel, and the ICER of TTF + docetaxel vs. docetaxel also decreased but were still above the WTP threshold of $150,000 per QALY gained, as the time horizon extended. It was also noteworthy that ICI stayed cost-effective compared with TTF + docetaxel in 5-year, and 8-year time horizons.
Discussion
First-line therapies for patients with driver gene-negative metastatic NSCLC include ICI monotherapy (high PD-L1 expression) (Mok et al., 2019; Herbst et al., 2020; Papadimitrakopoulou et al., 2020; Akinboro et al., 2022), ICI + platinum-based chemotherapy (regardless of PD-L1 expression) (Reck et al., 2022), and a four-drug combination of bevacizumab and atezolizumab plus chemotherapy (Socinski et al., 2018). While platinum-based first-line treatment has shown certain benefits, disease progression remains a common occurrence (Ramlau et al., 2012). Therefore, finding new therapy is crucial to improve patient outcomes. The groundbreaking LUNAR study marks the first instance where TTF + ST has exhibited extended survival in patients with metastatic NSCLC who have previously experienced failure with platinum-based therapy. However, the cost-effectiveness of this treatment, which is essential in its clinical application, is yet to be explored. Our study demonstrates that although TTF + ST leads to an average gain of 0.685 QALY compared with ST alone, it is not a cost-effective treatment for patients with NSCLC after platinum-based therapy in the United States, and the cost of TTF per month is the primary factor influencing its cost-effectiveness.
The lack of cost-effectiveness in combining TTF with standard therapy is attributed to the high cost of TTF rather than the limited health benefits. The high cost of TTF treatment is a primary concern in its cost-effectiveness, and several studies have assessed the cost-effectiveness of TTF + temozolomide (TMZ) in newly diagnosed glioblastoma (Bernard-Arnoux et al., 2016; Connock et al., 2019; Guzauskas et al., 2019). From the perspective of French health insurance, Bernard-Arnoux et al. (2016) demonstrated that the ICER of TTF + TMZ versus TMZ was €596 411 per life-year gained (LYG), and the probabilistic sensitivity analysis indicated that TTF + TMZ had 0% chance to be cost-effective at a WTP threshold of €100,000/LYG. Connock et al. (2019) reached a similar conclusion through a partitioned survival model in French. The ICER of TTF + TMZ compared with TMZ alone was €510,273/LYG, which widely exceeded the WTP threshold of €100,000/LYG. To achieve an ICER of less than €100,000/LYG, the cost of TTF device needs to be reduced by approximately 85%. However, from the perspective of the United States health insurance with a 5-year time horizon, Guzauskas et al. (2019) demonstrated that TTF + TMZ, with an ICER of $197,336 per QALY gained was cost-effective within the WTP threshold of $200,000 per QALY gained in the United States. These studies collectively emphasize that the high cost of TTF significantly impacts the ICER (Bernard-Arnoux et al., 2016; Connock et al., 2019; Guzauskas et al., 2019). Similarly, our analysis reveals that, in the treatment of advanced NSCLC, the TTF cost has the most substantial influence on the ICER of TTF + ST versus ST alone and the ICERs of subgroup comparisons involving TTF. Further analysis demonstrates that TTF + ST may only be cost-effective when the price of TTF per month is significantly reduced to less than $5,787, which represents about 21.3% of the estimated cost, $27,214,48 per month, in the base case analysis.
Looking into the two types of ST, ICI and docetaxel, our subgroup analyses showed that neither TTF + ICI nor TTF + docetaxel is cost-effective compared with either ICI alone or docetaxel alone, and ICI alone is cost-effective compared with TTF + docetaxel. The sensitivity analysis also identified the cost of TTF per month as the primary target to enhance the cost-effectiveness of TTF + ICI and TTF + docetaxel. It is noteworthy that the substantial increase in patients’ average QALY (2.318) in the TTF + ICI group compared to that of the ICI group (1.399), the TTF + docetaxel group (1.289), and the docetaxel group (1.035) emphasizes that TTF + ICI is an effective strategy for patients with advanced NSCLC. Moreover, in the comparison of TTF + ICI versus TTF + docetaxel and the comparison of TTF + ICI versus docetaxel alone, the costs of the ICIs (nivolumab, atezolizumab, and pembrolizumab) are among the top 6 most influential factors determining the ICERs, indicating that, besides the cost of TTF, the high costs of ICIs are key factors impairing the cost-effectiveness of TTF + ICI. Previous studies have consistently indicated a lack of cost-effectiveness of several ICIs in second-line treatment of advanced NSCLC in Swiss, the United States, Australia, or China, and suggested that the cost of these ICIs was essential in affecting the cost-effectiveness (Matter-Walstra et al., 2016; Aguiar et al., 2017; Gao and Li, 2019; Liu et al., 2020). Therefore, cutting down the prices of both TTF and immune checkpoint inhibitors is a possible way to generate economic benefits (Qiao et al., 2021; Borghini et al., 2022; Liu et al., 2022; Wang et al., 2022; Zheng et al., 2022; Cheng et al., 2023; Liang et al., 2023).
Our study also has certain limitations. Firstly, the use of published K-M curves instead of individual patient data from the LUNAR study introduces a potential source of inaccuracy when reconstructing the clinical data, but K-M curves were recreated to ensure the accuracy on the image level. Additionally, given the lack of manufacturer pricing data, the estimated price of TTF was based on inflation from previously published literature, which may be overestimated. However, the impact of TTF cost on the cost-effectiveness was intendedly explored in our study by adopting a wide range of TTF cost per month. Furthermore, our study tried to resemble the design of LUNAR study to ensure the coherence of clinical parameters, but real-life therapeutic situation may differ from the trial, such as different proportion of patients receiving ICI versus patients receiving docetaxel and different dosage of agents, which may lead to a lack of generalizability of the results. Finally, we used disutility from a study on renal cell carcinoma due to a lack of disutility data source of grade 1–2 or grade 3–5 adverse events, which can lead to inaccuracies in QALYs (Amdahl et al., 2016).
Conclusion
From the perspective of the United States insurance payers, TTF + ST does not appear to be a cost-effective therapeutic approach for metastatic NSCLC. The predominant factor affecting the potential of financial benefit is the cost of TTF. Therefore, strict regulation of TTF pricing by health administrations is essential to increase the accessibility and affordability for more patients with advanced NSCLC.
Data availability statement
The original contributions presented in the study are included in the article/Supplementary Material, further inquiries can be directed to the corresponding authors.
Author contributions
WT: Conceptualization, Methodology, Software, Writing–original draft. JN: Data curation, Investigation, Methodology, Software, Writing–original draft. LC: Writing–review and editing. YZ: Writing–review and editing. YS: Writing–review and editing. GX: Writing–review and editing. SH: Writing–review and editing. GT: Conceptualization, Investigation, Supervision, Writing–original draft. RZ: Conceptualization, Funding acquisition, Project administration, Writing–review and editing.
Funding
The author(s) declare financial support was received for the research, authorship, and/or publication of this article. This work was supported by the National Multidisciplinary Cooperative Diagnosis and Treatment Capacity (lung cancer z027002), National Clinical Research Center for Geriatric Disorders, Xiangya Hospital, Central South University (No. 2022LNJJ10), Advanced Lung Cancer Targeted Therapy Research Foundation of China (CTONG-YC20210303), and Chen Xiao-Ping Foundation for the Development of Science and Technology of Hubei Province (CXPJJH121005-01).
Conflict of interest
The authors declare that the research was conducted in the absence of any commercial or financial relationships that could be construed as a potential conflict of interest.
Publisher’s note
All claims expressed in this article are solely those of the authors and do not necessarily represent those of their affiliated organizations, or those of the publisher, the editors and the reviewers. Any product that may be evaluated in this article, or claim that may be made by its manufacturer, is not guaranteed or endorsed by the publisher.
Supplementary material
The Supplementary Material for this article can be found online at: https://www.frontiersin.org/articles/10.3389/fphar.2024.1333128/full#supplementary-material
Abbreviations
QALYs, quality-adjusted life-years; ICER, incremental cost-effectiveness ratio; WTP, willingness-to-pay; NMB, net monetary benefit.
References
Aguiar, P. N., Perry, L. A., Penny-Dimri, J., Babiker, H., Tadokoro, H., de Mello, R. A., et al. (2017). The effect of PD-L1 testing on the cost-effectiveness and economic impact of immune checkpoint inhibitors for the second-line treatment of NSCLC. Ann. Oncol. 28 (9), 2256–2263. doi:10.1093/annonc/mdx305
Akinboro, O., Larkins, E., Pai-Scherf, L. H., Mathieu, L. N., Ren, Y., Cheng, J., et al. (2022). FDA approval summary: pembrolizumab, atezolizumab, and cemiplimab-rwlc as single agents for first-line treatment of advanced/metastatic PD-L1-high NSCLC. Clin. cancer Res. official J. Am. Assoc. Cancer Res. 28 (11), 2221–2228. doi:10.1158/1078-0432.CCR-21-3844
Amdahl, J., Diaz, J., Park, J., Nakhaipour, H. R., and Delea, T. E. (2016). Cost-effectiveness of pazopanib compared with sunitinib in metastatic renal cell carcinoma in Canada. Curr. Oncol. Tor. Ont. 23 (4), e340–e354. doi:10.3747/co.23.2244
Barnfield, P. C., and Ellis, P. M. (2016). Second-line treatment of non-small cell lung cancer: new developments for tumours not harbouring targetable oncogenic driver mutations. Drugs 76 (14), 1321–1336. doi:10.1007/s40265-016-0628-6
Bernard-Arnoux, F., Lamure, M., Ducray, F., Aulagner, G., Honnorat, J., and Armoiry, X. (2016). The cost-effectiveness of tumor-treating fields therapy in patients with newly diagnosed glioblastoma. Neuro-oncology 18 (8), 1129–1136. doi:10.1093/neuonc/now102
Bluthgen, M. V., and Besse, B. (2015). Second-line combination therapies in nonsmall cell lung cancer without known driver mutations. Eur. Respir. Rev. official J. Eur. Respir. Soc. 24 (138), 582–593. doi:10.1183/16000617.00002115
Borghini, A., Vecoli, C., Labate, L., Panetta, D., Andreassi, M. G., and Gizzi, L. A. (2022). FLASH ultra-high dose rates in radiotherapy: preclinical and radiobiological evidence. Int. J. Radiat. Biol. 98 (2), 127–135. doi:10.1080/09553002.2022.2009143
Chen, R., Manochakian, R., James, L., Azzouqa, A. G., Shi, H., Zhang, Y., et al. (2020). Emerging therapeutic agents for advanced non-small cell lung cancer. J. Hematol. Oncol. 13 (1), 58. doi:10.1186/s13045-020-00881-7
Cheng, R., Zhou, Z., and Liu, Q. (2023). The cost-effectiveness of sugemalimab plus chemotherapy as first-line treatment for metastatic squamous and non-squamous NSCLC in China. Adv. Ther. 40 (10), 4298–4309. doi:10.1007/s12325-023-02594-y
Chouaid, C., Agulnik, J., Goker, E., Herder, G. J., Lester, J. F., Vansteenkiste, J., et al. (2013). Health-related quality of life and utility in patients with advanced non-small-cell lung cancer: a prospective cross-sectional patient survey in a real-world setting. J. Thorac. Oncol. official Publ. Int. Assoc. Study Lung Cancer 8 (8), 997–1003. doi:10.1097/JTO.0b013e318299243b
Clegg, A., Scott, D. A., Sidhu, M., Hewitson, P., and Waugh, N. (2001). A rapid and systematic review of the clinical effectiveness and cost-effectiveness of paclitaxel, docetaxel, gemcitabine and vinorelbine in non-small-cell lung cancer. Health Technol. Assess. Winch. Engl. 5 (32), 1–195. doi:10.3310/hta5320
Connock, M., Auguste, P., Dussart, C., Guyotat, J., and Armoiry, X. (2019). Cost-effectiveness of tumor-treating fields added to maintenance temozolomide in patients with glioblastoma: an updated evaluation using a partitioned survival model. J. neuro-oncology 143 (3), 605–611. doi:10.1007/s11060-019-03197-w
Criss, S. D., Mooradian, M. J., Sheehan, D. F., Zubiri, L., Lumish, M. A., Gainor, J. F., et al. (2019). Cost-effectiveness and budgetary consequence analysis of durvalumab consolidation therapy vs No consolidation therapy after chemoradiotherapy in stage III non-small cell lung cancer in the context of the US health care system. JAMA Oncol. 5 (3), 358–365. doi:10.1001/jamaoncol.2018.5449
De Marzi, L., Patriarca, A., Scher, N., Thariat, J., and Vidal, M. (2020). Exploiting the full potential of proton therapy: an update on the specifics and innovations towards spatial or temporal optimisation of dose delivery. Cancer radiotherapie J. de Soc. francaise de radiotherapie Oncol. 24 (6-7), 691–698. doi:10.1016/j.canrad.2020.06.003
Dutt, S., Ahmed, M. M., Loo, B. W., and Strober, S. (2020). Novel radiation therapy paradigms and immunomodulation: heresies and hope. Seminars Radiat. Oncol. 30 (2), 194–200. doi:10.1016/j.semradonc.2019.12.006
Gao, L., and Li, S. C. (2019). Modelled economic evaluation of nivolumab for the treatment of second-line advanced or metastatic squamous non-small-cell lung cancer in Australia using both partition survival and Markov models. Appl. health Econ. health policy 17 (3), 371–380. doi:10.1007/s40258-018-0452-0
Giladi, M., Schneiderman, R. S., Voloshin, T., Porat, Y., Munster, M., Blat, R., et al. (2015). Mitotic spindle disruption by alternating electric fields leads to improper chromosome segregation and mitotic catastrophe in cancer cells. Sci. Rep. 5, 18046. doi:10.1038/srep18046
Giladi, M., Weinberg, U., Schneiderman, R. S., Porat, Y., Munster, M., Voloshin, T., et al. (2014). Alternating electric fields (tumor-treating fields therapy) can improve chemotherapy treatment efficacy in non-small cell lung cancer both in vitro and in vivo. Seminars Oncol. 41 (Suppl. 6), S35–S41. doi:10.1053/j.seminoncol.2014.09.006
Gong, J., Su, D., Shang, J., Xu, S., Tang, L., Sun, Z., et al. (2022). Cost-effectiveness of tislelizumab versus docetaxel for previously treated advanced non-small-cell lung cancer in China. Front. Pharmacol. 13, 830380. doi:10.3389/fphar.2022.830380
Guzauskas, G. F., Pollom, E. L., Stieber, V. W., Wang, B. C. M., and Garrison, L. P. (2019). Tumor treating fields and maintenance temozolomide for newly-diagnosed glioblastoma: a cost-effectiveness study. J. Med. Econ. 22 (10), 1006–1013. doi:10.1080/13696998.2019.1614933
Herbst, R. S., Giaccone, G., de Marinis, F., Reinmuth, N., Vergnenegre, A., Barrios, C. H., et al. (2020). Atezolizumab for first-line treatment of PD-L1-selected patients with NSCLC. N. Engl. J. Med. 383 (14), 1328–1339. doi:10.1056/NEJMoa1917346
Insinga, R. P., Vanness, D. J., Feliciano, J. L., Vandormael, K., Traore, S., and Burke, T. (2018). Cost-effectiveness of pembrolizumab in combination with chemotherapy in the 1st line treatment of non-squamous NSCLC in the US. J. Med. Econ. 21 (12), 1191–1205. doi:10.1080/13696998.2018.1521416
Karanam, N. K., Srinivasan, K., Ding, L., Sishc, B., Saha, D., and Story, M. D. (2017). Tumor-treating fields elicit a conditional vulnerability to ionizing radiation via the downregulation of BRCA1 signaling and reduced DNA double-strand break repair capacity in non-small cell lung cancer cell lines. Cell death Dis. 8 (3), e2711. doi:10.1038/cddis.2017.136
Kevin Zarca, A. F.-P., Wiener, M., Kabat, Z., Filipec, V., Jordan, A., Carranza Alarcon, Y., et al. (2023). Package ‘heemod’: Markov models for health economic evaluations. Available at: https://cran.r-project.org/web/packages/heemod/heemod.pdf.
Kirson, E. D., Gurvich, Z., Schneiderman, R., Dekel, E., Itzhaki, A., Wasserman, Y., et al. (2004). Disruption of cancer cell replication by alternating electric fields. Cancer Res. 64 (9), 3288–3295. doi:10.1158/0008-5472.can-04-0083
Klein, R., Wielage, R., Muehlenbein, C., Liepa, A. M., Babineaux, S., Lawson, A., et al. (2010). Cost-effectiveness of pemetrexed as first-line maintenance therapy for advanced nonsquamous non-small cell lung cancer. J. Thorac. Oncol. official Publ. Int. Assoc. Study Lung Cancer 5 (8), 1263–1272. doi:10.1097/JTO.0b013e3181e15d16
Leal, T., Kotecha, R., Ramlau, R., Zhang, L., Milanowski, J., Cobo, M., et al. (2023). Tumor Treating Fields therapy with standard systemic therapy versus standard systemic therapy alone in metastatic non-small-cell lung cancer following progression on or after platinum-based therapy (LUNAR): a randomised, open-label, pivotal phase 3 study. Lancet Oncol. 24 (9), 1002–1017. doi:10.1016/S1470-2045(23)00344-3
Li, Y., Liang, X., Yang, T., Guo, S., and Chen, X. (2022). Pembrolizumab vs cemiplimab for the treatment of advanced non-small cell lung cancer with PD-L1 expression levels of at least 50%: a network meta-analysis and cost-effectiveness analysis. Front. Oncol. 12, 878054. doi:10.3389/fonc.2022.878054
Liang, X., Chen, X., Li, H., Liu, X., and Li, Y. (2023). Sugemalimab plus chemotherapy vs. chemotherapy for metastatic non-small-cell lung cancer: a cost-effectiveness analysis. Front. public health 11, 1054405. doi:10.3389/fpubh.2023.1054405
Liu, H., Wang, Y., and He, Q. (2022). Cost-effectiveness analysis of sintilimab plus pemetrexed and platinum versus chemotherapy alone as first-line treatment in metastatic non-squamous non-small cell lung cancer in China. Health Econ. Rev. 12 (1), 66. doi:10.1186/s13561-022-00410-x
Liu, N., Zhou, Y., and Lee, J. J. (2021a). IPDfromKM: reconstruct individual patient data from published Kaplan-Meier survival curves. BMC Med. Res. Methodol. 21 (1), 111. doi:10.1186/s12874-021-01308-8
Liu, Q., Luo, X., Peng, L., Yi, L., Wan, X., Zeng, X., et al. (2020). Nivolumab versus docetaxel for previously treated advanced non-small cell lung cancer in China: a cost-effectiveness analysis. Clin. Drug Investig. 40 (2), 129–137. doi:10.1007/s40261-019-00869-3
Liu, Q., Zhou, Z., Luo, X., Yi, L., Peng, L., Wan, X., et al. (2021b). First-line ICI monotherapies for advanced non-small-cell lung cancer patients with PD-L1 of at least 50%: a cost-effectiveness analysis. Front. Pharmacol. 12, 788569. doi:10.3389/fphar.2021.788569
Matter-Walstra, K., Schwenkglenks, M., Aebi, S., Dedes, K., Diebold, J., Pietrini, M., et al. (2016). A cost-effectiveness analysis of nivolumab versus docetaxel for advanced nonsquamous NSCLC including PD-L1 testing. J. Thorac. Oncol. 11 (11), 1846–1855. doi:10.1016/j.jtho.2016.05.032
Mok, T. S. K., Wu, Y. L., Kudaba, I., Kowalski, D. M., Cho, B. C., Turna, H. Z., et al. (2019). Pembrolizumab versus chemotherapy for previously untreated, PD-L1-expressing, locally advanced or metastatic non-small-cell lung cancer (KEYNOTE-042): a randomised, open-label, controlled, phase 3 trial. Lancet (London, Engl. 393 (10183), 1819–1830. doi:10.1016/S0140-6736(18)32409-7
Neumann, P. J., Cohen, J. T., and Weinstein, M. C. (2014). Updating cost-effectiveness--the curious resilience of the $50,000-per-QALY threshold. N. Engl. J. Med. 371 (9), 796–797. doi:10.1056/NEJMp1405158
Neumann, P. J., and Kim, D. D. (2023). Cost-effectiveness thresholds used by study authors, 1990-2021. Jama 329 (15), 1312–1314. doi:10.1001/jama.2023.1792
Ondhia, U., Conter, H. J., Owen, S., Zhou, A., Nam, J., Singh, S., et al. (2019). Cost-effectiveness of second-line atezolizumab in Canada for advanced non-small cell lung cancer (NSCLC). J. Med. Econ. 22 (7), 625–637. doi:10.1080/13696998.2019.1590842
Papadimitrakopoulou, V. A., Mok, T. S., Han, J. Y., Ahn, M. J., Delmonte, A., Ramalingam, S. S., et al. (2020). Osimertinib versus platinum-pemetrexed for patients with EGFR T790M advanced NSCLC and progression on a prior EGFR-tyrosine kinase inhibitor: AURA3 overall survival analysis. Ann. Oncol. official J. Eur. Soc. Med. Oncol. 31 (11), 1536–1544. doi:10.1016/j.annonc.2020.08.2100
Petoukhova, A., Snijder, R., Vissers, T., Ceha, H., and Struikmans, H. (2023). In vivodosimetry in cancer patients undergoing intraoperative radiation therapy. Phys. Med. Biol. 68 (18), 18TR01. doi:10.1088/1361-6560/acf2e4
Pless, M., Droege, C., von Moos, R., Salzberg, M., and Betticher, D. (2013). A phase I/II trial of Tumor Treating Fields (TTFields) therapy in combination with pemetrexed for advanced non-small cell lung cancer. Lung cancer (Amsterdam, Neth. 81 (3), 445–450. doi:10.1016/j.lungcan.2013.06.025
Qiao, L., Zhou, Z., Zeng, X., and Tan, C. (2021). Cost-effectiveness of domestic PD-1 inhibitor camrelizumab combined with chemotherapy in the first-line treatment of advanced nonsquamous non-small-cell lung cancer in China. Front. Pharmacol. 12, 728440. doi:10.3389/fphar.2021.728440
Ramlau, R., Gorbunova, V., Ciuleanu, T. E., Novello, S., Ozguroglu, M., Goksel, T., et al. (2012). Aflibercept and Docetaxel versus Docetaxel alone after platinum failure in patients with advanced or metastatic non-small-cell lung cancer: a randomized, controlled phase III trial. J. Clin. Oncol. official J. Am. Soc. Clin. Oncol. 30 (29), 3640–3647. doi:10.1200/JCO.2012.42.6932
Reck, M., Remon, J., and Hellmann, M. D. (2022). First-line immunotherapy for non-small-cell lung cancer. J. Clin. Oncol. official J. Am. Soc. Clin. Oncol. 40 (6), 586–597. doi:10.1200/JCO.21.01497
Rothwell, B., Kiff, C., Ling, C., and Brodtkorb, T. H. (2021). Cost effectiveness of nivolumab in patients with advanced, previously treated squamous and non-squamous non-small-cell lung cancer in england. PharmacoEconomics - open 5 (2), 251–260. doi:10.1007/s41669-020-00245-4
Shen, J., Du, Y., Shao, R., and Jiang, R. (2022). First-line sintilimab plus chemotherapy in locally advanced or metastatic esophageal squamous cell carcinoma: a cost-effectiveness analysis from China. Front. Pharmacol. 13, 967182. doi:10.3389/fphar.2022.967182
Shteingauz, A., Porat, Y., Voloshin, T., Schneiderman, R. S., Munster, M., Zeevi, E., et al. (2018). AMPK-dependent autophagy upregulation serves as a survival mechanism in response to Tumor Treating Fields (TTFields). Cell death Dis. 9 (11), 1074. doi:10.1038/s41419-018-1085-9
Shu, Y., Ding, Y., Li, F., and Zhang, Q. (2023). Cost-effectiveness of nivolumab plus ipilimumab versus chemotherapy as first-line therapy in advanced non-small cell lung cancer. Int. Immunopharmacol. 114, 109589. doi:10.1016/j.intimp.2022.109589
Siegel, R. L., Miller, K. D., and Jemal, A. (2020). Cancer statistics, 2020. CA a cancer J. Clin. 70 (1), 7–30. doi:10.3322/caac.21590
Siegel, R. L., Miller, K. D., Wagle, N. S., and Jemal, A. (2023). Cancer statistics, 2023. CA a cancer J. Clin. 73 (1), 17–48. doi:10.3322/caac.21763
Smith, D. H., and Gravelle, H. (2001). The practice of discounting in economic evaluations of healthcare interventions. Int. J. Technol. Assess. health care 17 (2), 236–243. doi:10.1017/s0266462300105094
Socinski, M. A., Jotte, R. M., Cappuzzo, F., Orlandi, F., Stroyakovskiy, D., Nogami, N., et al. (2018). Atezolizumab for first-line treatment of metastatic nonsquamous NSCLC. N. Engl. J. Med. 378 (24), 2288–2301. doi:10.1056/NEJMoa1716948
Swartz, H. M., Vaupel, P., and Flood, A. B. (2023). A critical analysis of possible mechanisms for the oxygen effect in radiation therapy with FLASH. Adv. Exp. Med. Biol. 1438, 127–133. doi:10.1007/978-3-031-42003-0_21
Voloshin, T., Kaynan, N., Davidi, S., Porat, Y., Shteingauz, A., Schneiderman, R. S., et al. (2020). Tumor-treating fields (TTFields) induce immunogenic cell death resulting in enhanced antitumor efficacy when combined with anti-PD-1 therapy. CII 69 (7), 1191–1204. doi:10.1007/s00262-020-02534-7
Wang, H., Liao, L., Xu, Y., Long, Y., Wang, Y., and Zhou, Y. (2022). Economic evaluation of first-line sugemalimab plus chemotherapy for metastatic non-small cell lung cancer in China. Front. Oncol. 12, 1081750. doi:10.3389/fonc.2022.1081750
Wong, W., Yim, Y. M., Kim, A., Cloutier, M., Gauthier-Loiselle, M., Gagnon-Sanschagrin, P., et al. (2018). Assessment of costs associated with adverse events in patients with cancer. PloS one 13 (4), e0196007. doi:10.1371/journal.pone.0196007
Zhang, P. F., Xie, D., and Li, Q. (2020). Cost-effectiveness analysis of nivolumab in the second-line treatment for advanced esophageal squamous cell carcinoma. Future Oncol. 16 (17), 1189–1198. doi:10.2217/fon-2019-0821
Zheng, Z., Zhu, H., Fang, L., and Cai, H. (2022). Cost-effectiveness analysis of sugemalimab vs. chemotherapy as first-line treatment of metastatic nonsquamous non-small cell lung cancer. Front. Pharmacol. 13, 996914. doi:10.3389/fphar.2022.996914
Keywords: advanced non-small cell lung cancer, tumor treating fields, immunotherapy, chemotherapy, cost-effectiveness
Citation: Tian W, Ning J, Chen L, Zeng Y, Shi Y, Xiao G, He S, Tanzhu G and Zhou R (2024) Cost-effectiveness of tumor-treating fields plus standard therapy for advanced non-small cell lung cancer progressed after platinum-based therapy in the United States. Front. Pharmacol. 15:1333128. doi: 10.3389/fphar.2024.1333128
Received: 04 November 2023; Accepted: 26 January 2024;
Published: 05 February 2024.
Edited by:
Ling Chen, People’s Liberation Army General Hospital, ChinaReviewed by:
Li Wei, China Pharmaceutical University, ChinaTao Li, People’s Liberation Army General Hospital, China
Copyright © 2024 Tian, Ning, Chen, Zeng, Shi, Xiao, He, Tanzhu and Zhou. This is an open-access article distributed under the terms of the Creative Commons Attribution License (CC BY). The use, distribution or reproduction in other forums is permitted, provided the original author(s) and the copyright owner(s) are credited and that the original publication in this journal is cited, in accordance with accepted academic practice. No use, distribution or reproduction is permitted which does not comply with these terms.
*Correspondence: Guilong Tanzhu, dHpnbDI3MTZAMTI2LmNvbQ==; Rongrong Zhou, emhvdXJyQGNzdS5lZHUuY24=
†These authors have contributed equally to this work and share first authorship