- 1School of Pharmacy, Yancheng Teachers University, Yancheng, China
- 2Sheyang County Comprehensive Inspection and Testing Center, Yancheng, China
- 3Department of Immunology, Medical School, Nantong University, Nantong, China
Albumin is derived from human or animal blood, and its ability to bind to a large number of endogenous or exogenous biomolecules makes it an ideal drug carrier. As a result, albumin-based drug delivery systems are increasingly being studied. With these in mind, detailed studies of the transport mechanism of albumin-based drug carriers are particularly important. As albumin receptors, glycoprotein 60 (GP60) and secreted protein acidic and rich in cysteine (SPARC) play a crucial role in the delivery of albumin-based drug carriers. GP60 is expressed on vascular endothelial cells and enables albumin to cross the vascular endothelial cell layer, and SPARC is overexpressed in many types of tumor cells, while it is minimally expressed in normal tissue cells. Thus, this review supplements existing articles by detailing the research history and specific biological functions of GP60 or SPARC and research advances in the delivery of antitumor drugs using albumin as a carrier. Meanwhile, the deficiencies and future perspectives in the study of the interaction of albumin with GP60 and SPARC are also pointed out.
1 Introduction
Albumin is derived from blood plasma and is the most abundant protein in the blood, accounting for approximately 50% of the total protein in the plasma (Forsthuber et al., 2020; Jagdish et al., 2021). The common types of albumin include human serum albumin (HSA), bovine serum albumin (BSA), equine serum albumin (ESA), and murine serum albumin (MSA). Although there are many types of albumin, HSA and BSA are the most common albumin types used in biomedical research works and applications. The crystal structures of the proteins of HSA and BSA are similar, and both show a heart-shaped structure. HSA consists of 585 amino acids, and the molecular weight of HSA is approximately 69.367 kDa. BSA consists of 583 amino acids, and the molecular weight of BSA is approximately 69.293 kDa. The isoelectric points of both native HSA and BSA are between 4.7 and 4.9. Because albumin has the advantages of non-immunogenicity, non-cytotoxicity, and multiple drug-binding sites, it has a wide range of applications in the biomedical field (Hu et al., 2022), especially in drug delivery systems. There are many albumin drug-binding sites in the human body, such as GP60, GP30, GP18, secreted protein acidic and rich in cysteine (SPARC), FcRn, cubilin, and megalin (Molitoris et al., 2022). Among these albumin receptors, GP60 and SPARC play key roles in the delivery of antitumor drugs based on albumin drug carriers.
To date, there are some reviews that summarize advances in albumin-based drug delivery. However, most of these reviews focus more on describing the preparation methods of albumin nanoparticles, drug-loading types, and the current research progress of albumin nanoparticles, and few reviews detailing GP60 and SPARC are available (Spada et al., 2021; Ishima et al., 2022; Paul et al., 2022). As a supplement, this review details the research history, biological functions, and current research advances of GP60 and SPARC and the existing problems and future perspectives about albumin-based drug carriers constructed by the GP60- and SPARC-mediated pathway.
2 The research history of GP60 and SPARC
GP60, also called albondin, is a 60-kDa microvascular endothelial glycoprotein. In 1978, Rohde et al. described a kind of glycoprotein whose molecular weight is 60 kDa (Rohde et al., 1978). Yagi et al. explored the functions of GP60 based on a mouse mammary tumor in 1980, and they found that the presence of GP60 in mature B-type virions is related to the environment of the MJY-alpha cells (Yagi et al., 1980). Then, in 1984, GP60 was purified by Schultz et al. from bovine leukemia virus (BLV) using controlled pore glass and reverse-phase liquid chromatography (RPLC), and they also analyzed the amino acid sequence of the purified GP60 (Schultz et al., 1984). Around the 1990s, Schnitzer et al. found that GP60 is expressed on vascular endothelial cells and that it can interact with albumin to allow albumin to be transported across the endothelium (Schnitzer et al., 1988; Schnitzer, 1992). Milici and Tiruppathi also found that GP60 not only binds natural albumin but also facilitates its internalization and transcytosis (Milici et al., 1987; Tiruppathi et al., 1997). It has been shown that approximately 50% of albumin crossing the vascular endothelium is dependent on the GP60 receptor, and the rest of albumin crosses this barrier mainly through intercellular junctions and the fluid-phase mechanism (Schnitzer, 1993; Schnitzer and Oh, 1994). Iancu, Schnitzer, and Tiruppathi found that albumin cellular internalization occurs mainly through the caveolin-dependent endocytotic process (Schnitzer et al., 1995; Tiruppathi et al., 1997; Iancu et al., 2011). In 1996, the GP60 receptor from vascular endothelial cells was isolated and characterized by Tiruppathi et al., and their findings also indicated that the GP60 receptor on the surface of endothelial cells mediates the specific binding of native albumin to endothelial cells and, thus, may regulate the uptake of albumin and its transcytosis (Tiruppathi et al., 1996). Based on the albumin–GP60 interaction, a drug called ABI-007 was constructed successfully, and it could deliver paclitaxel to the tumor through GP60 receptors on the surface of vascular endothelial cells (Nyman et al., 2005). Shortly thereafter, the famous antitumor drug named Abraxane® was approved for marketing by the U.S. Food and Drug Administration. In the last decade, a lot of research studies based on the albumin–GP60 interaction started to emerge. For example, albumin-consolidated AIEgens were constructed for boosting glioma and cerebrovascular NIR-II fluorescence imaging, and these albumin-based AIE nanoprobes enable the limited fluorescence imaging-guided surgery of brain tumor and cerebral ischemia (Gao et al., 2023). Also, protein nanoparticles constructed via the albumin–GP60 interaction demonstrated a strong ability to overcome cancer drug resistance, and it was expected to be further used in clinical practice (Hassanin and Elzoghby, 2020). Cisplatin-loaded albumin–gold nanoparticles could interact with glycans of the GP60 receptor, and the mechanisms of this interaction were explored at the molecular and cellular levels by Jaiswal et al.; thus, this finding could be effectively used for in vivo or in vitro targeted drug delivery applications to cure cancer (Jaiswal et al., 2023). Kumari et al. used BSA to modify metal nanoparticles in order to make these metal nanoparticles interact with glycans of the GP60 receptor on endothelial cells for targeted drug delivery, and they also explored in detail the mechanism of interaction between albumin and glycans of the GP60 receptor; thus, these findings could form a promising platform to investigate the interaction of albumin nanoparticles with the GP60 receptor in both in vitro and in vivo applications for targeted drug delivery therapy (Kumari et al., 2022). A brief research history of the GP60 receptor is shown in Figure 1.
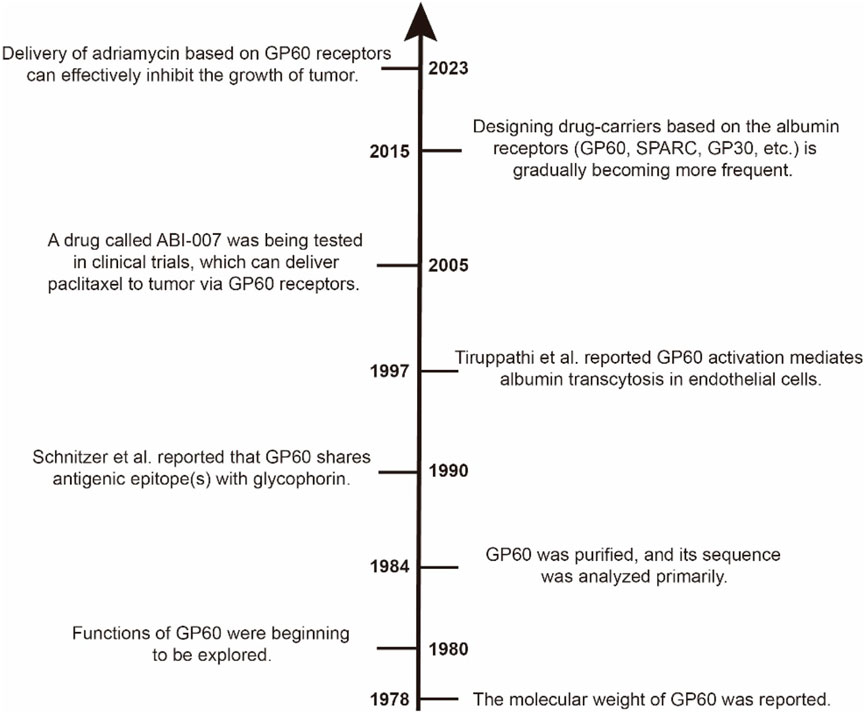
FIGURE 1. Timeline of the research about GP60 receptors. In the 1980s, GP60 was purified and characterized. In the 1990s, the functions of GP60 were reported one after another. Since the beginning of the 21st century, albumin–GP60 interaction and albumin–SPARC interaction have been successively applied to studies of drug delivery, especially in the last decade.
SPARC is also known as osteonectin or BM-40 (basement membrane 40). SPARC is a single-copy gene that is highly conserved, with over 70% amino acid sequence homology in various sequences. The length of amino acids of SPARC derived from humans is 303, and its molecular weight is approximately 34.632 kDa (UniProt entry: P09486). Termine et al. first identified SPARC as a major non-collagenous component of bone in 1981 and found that SPARC is a cysteine-rich, low-molecular-weight glycoprotein (Termine et al., 1981). In 1988, the sequence of complete amino acids of SPARC was reported (Lankat-Buttgereit et al., 1988). Lane and Sage conducted a series of experiments about SPARC. Their results showed that SPARC could inhibit cell cycle progression in vitro, in part through a cationic, disulfide-bonded region. Moreover, SPARC could bind to the B chain of the platelet-derived growth factor and alter the response of cells to several cytokines (Lane and Sage, 1994). In 1996, Jendraschak et al. investigated how SPARC regulates angiogenesis. The results showed that SPARC might act pleiotropically during angiogenesis in conjunction with other known angiogenic factors (Jendraschak and Sage, 1996). Since 1999, SPARC has been consistently reported to be overexpressed in various types of tumors, such as breast cancer, liver cancer, neuroblastoma, and glioma (Le Bail et al., 1999; Gorantla et al., 2013; Lin et al., 2016; Gao et al., 2021). In 2008, a review by Tai and Tang introduced in detail the role of SPARC in cancer progression and its potential for cancer therapy, and the role of SPARC in sensitizing therapy-resistant cancer types was also discussed (Tai and Tang, 2008). Over the past decade, more and more biomedical applications focused on drug delivery systems based on the albumin–SPARC interaction have been developed. Applications of SPARC in the biomedical field include the construction of drug delivery systems and the preparation of imaging agents and diagnostic reagents (Nagaraju and El-Rayes, 2013; Hu et al., 2022; Jiang et al., 2023). A brief research history of SPARC is shown in Figure 2.
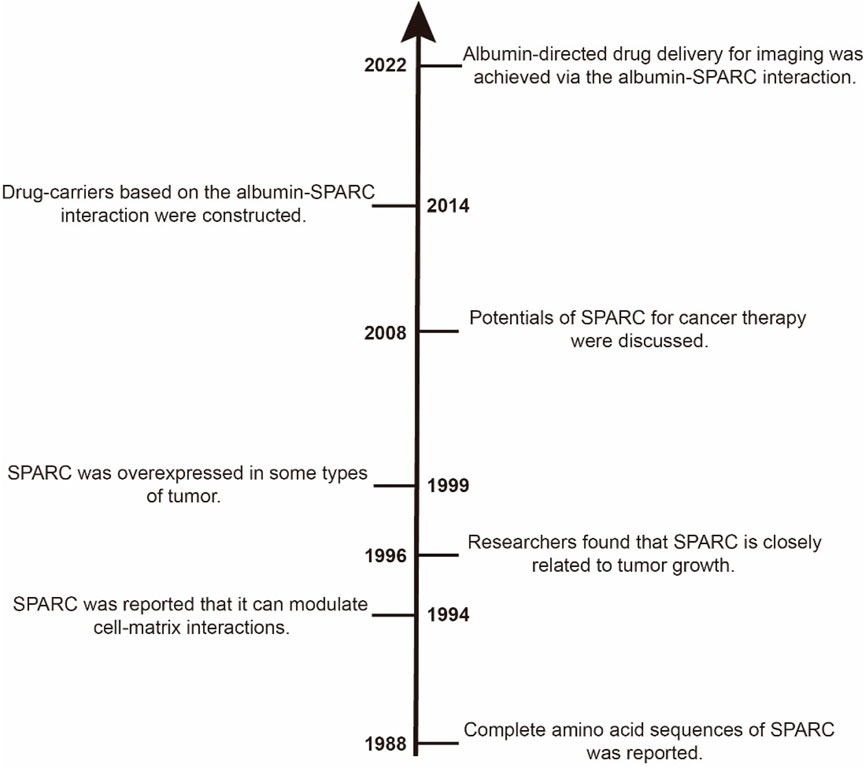
FIGURE 2. Timeline of the research about SPARC. In the 1980s, the physical and chemical characteristics of SPARC were successively identified. Then, SPARC was found to be similar to GP60 receptors, and its functions were successively reported in the 1990s. In the first decade of the 21st century, the potentials of SPARC were primarily discussed and explored. In the last decade, more and more research studies reported applications of the albumin–SPARC interaction.
3 Specific biological functions of GP60 and SPARC
In this review, we focused on the function of GP60 and SPARC as albumin receptors. However, GP60 or SPARC carries out many biological functions in addition to its role as an albumin receptor. Thus, in order to provide a comprehensive understanding of GP60 and SPARC, we also provide a comprehensive overview of their other biological functions.
3.1 GP60
GP60 receptors are expressed in vascular endothelial cells except in brain tissues, and they enable the transport of albumin from the inside to the outside of blood vessels in 13 s (Stewart, 2000; Hama et al., 2021). It is important to note that the distribution of HSA regulated by SPARC depends on blood volume and protein status in vivo. Albumin first binds to the GP60 receptor, which in turn binds further to an intracellular protein called caveolin-1. Then, the cell membrane invaginates to produce transcellular vesicles, which eventually allows albumin to cross the vascular endothelium (John et al., 2001). So, specific biological functions or characteristics of GP60 as an albumin receptor include overexpression in tumor tissues and mediating albumin transcytosis in endothelial cells. These functions and features of GP60 provide a rationale for its use as a therapeutic target for tumors, as well as the theoretical basis for the design of various types of drug carriers based on the albumin–GP60 interaction. Table 1 details the known functions of GP60 and the reported years.
3.2 SPARC
The biological functions of SPARC that are now well defined include its role as a protein that interacts with albumin, regulating the cellular secretion rates of fibronectin and laminin extracellular matrix proteins, regulating cell proliferation, preventing cellular adhesion, promoting cellular deformation, regulating cell differentiation, inhibiting cellular response to some growth factors, and regulating the production of the extracellular matrix and metalloproteinase. Compared to the GP60 receptor, SPARC exhibits more biological functions in vivo (Rivera et al., 2011; Bradshaw, 2016; Chen et al., 2020). Here, the role of SPARC in the occurrence and development of tumors is highlighted. To date, there are at least four functions of SPARC identified as relevant to tumors. First, SPARC has an anti-adhesive effect. This function is different from most of the extracellular matrix (ECM) constituents, and the function shows some concentration dependence (Volmer et al., 2004). SPARC interferes with cell surface binding to ECM components and interacts with growth factors to change the cell shape. The separation of cells is the beginning of tumor invasion of surrounding tissues and distant metastasis, which has a key role in the progression of malignant tumors. Second, SPARC can degrade the ECM. SPARC induces the synthesis of collagenase, gelatinase, the mesenchymal degrading enzyme, etc., to degrade the matrix and impair the function of the matrix barrier, which ultimately promotes the metastasis of tumor cells. Third, SPARC is involved in the regulation of multiple signaling pathways. These pathways include the PI3K–Akt–mTOR pathway, mitogen-activated protein kinase (MAPK)/extracellular signal-regulated pathway, WNT/beta-catenin signaling pathway, and endothelial paracellular pathway via protein tyrosine phosphorylation. Finally, SPARC can modulate angiogenesis. As early as 1996, Jendraschak and Sage summarized the findings that SPARC can modulate angiogenesis. The findings showed that SPARC can promote the lysis of the basement membrane and the movement of endothelial cells. The hydrolysis product of SPARC, named calcium-binding peptide, can stimulate angiogenesis and cell growth. Therefore, in the early stages of malignant transformation, SPARC plays a crucial role in tumor cell proliferation and metastasis, angiogenesis, and the remodeling of the extracellular matrix. SPARC is overexpressed in progressively aggressive tumors, which may be indicative of a failure of homeostatic repair between the tissue and the microenvironment. More functions or characteristics of SPARC are summarized in Table 1.
4 Antitumor drug delivery targeting GP60 and SPARC
With the development and advancement of science and technology over time, more and more researchers are focusing on drug-targeted delivery, especially the construction of drug carriers with biological activities. Over the past decade, researchers have attempted to construct a number of drug delivery systems with biological activities, such as ferritin nanocarriers, cell membrane-coated drug carriers, and activated protein nanoparticles (Liang et al., 2014; Lee et al., 2016; He et al., 2019; Fang et al., 2023). In this section, protein drug carriers constructed based on the albumin–GP60 interaction and albumin–SPARC interaction are highlighted. The drug delivery system constructed on the basis of albumin first crosses the barrier of vascular endothelial cells into the interstitial space of tumor tissues through the mediation of GP60, and then, drug carriers bind to SPARC expressed by tumor cells, ultimately achieving the goal of targeting tumor cells (Figure 3).
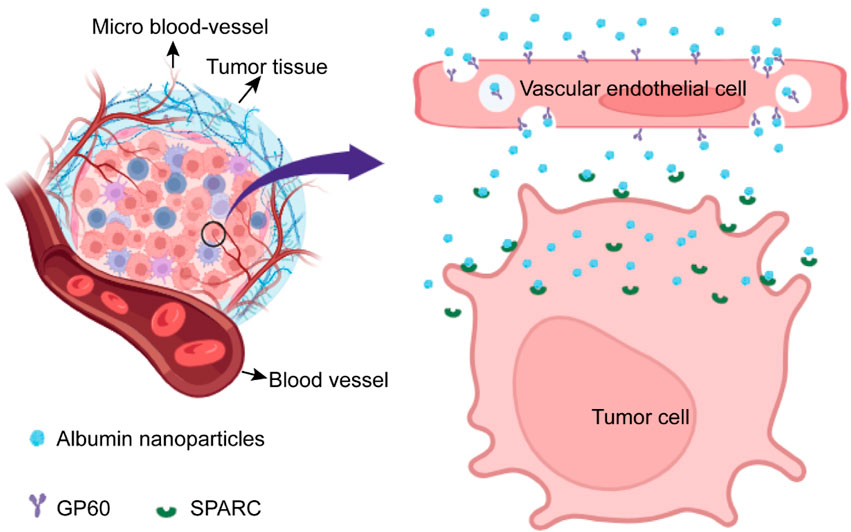
FIGURE 3. Schematic diagram of albumin nanoparticles targeting tumor cells via the GP60 and SPARC pathways. When albumin nanoparticles are circulated to tumor tissues via intravenous injection, albumin nanoparticles will rely on GP60 receptors to penetrate vascular endothelial cells and enter the tumor microenvironment. Then, these albumin nanoparticles will interact with the SPARC protein secreted by tumor cells, ultimately achieving the effect of targeting tumor cells.
In 2005, Abraxane®, constructed based on HSA, first utilized the GP60 and SPARC pathways to enhance the therapeutic effects of paclitaxel. In addition, this drug has achieved more favorable therapeutic results. To this day, Abraxane® still maintains a high market share. Since 2005, more and more studies have begun to try to construct albumin drug carriers to treat various types of tumors. In 2011, Iancu et al. constructed multi-walled carbon nanotubes functionalized with HSA, and their findings demonstrated good targeting effects on HepG2 cells (Lee et al., 2016). In 2016, Lee et al. prepared a kind of albumin nanoparticles that accumulated in the tumor site of an HCT116 cell-xenograft mouse model, and the results demonstrated excellent tumor targetability via a GP60-mediated transcytosis mechanism (Lee et al., 2016). In 2018, a kind of HSA nanoparticles loaded with paclitaxel was constructed for the targeted therapy of glioma by Ruan et al., and this kind of albumin nanoparticles can effectively cross the blood–brain barrier to target brain capillary endothelial cells and U87 cells. All results in the research showed a satisfactory antitumor effect and could serve as a novel strategy for the treatment of glioma (et al., 2018). One year later, a kind of albumin nanoparticles loaded with pirarubicin was constructed to treat the occurrence of cancer and the metastasis of tumors (Zhou et al., 2019). In 2021, Hama et al. investigated evidence for the delivery of Abraxane® via the denatured albumin transport system in detail, with data indicating that Abraxane-derived HSA was taken up into endothelial cells or tumor cells by a mechanism different from normal endogenous albumin. These data thus provided new scientific rationale for the development of a novel albumin drug delivery strategy via a denatured albumin receptor (Hama et al., 2021). Our previous work showed that biologically active albumin nanoparticles can effectively target solid tumors via GP60 and SPARC pathways and can effectively inhibit tumor growth and prolong the survival time of tumor-bearing mice (Meng et al., 2023). Albumin drug delivery systems constructed based on the GP60 or SPARC pathway are numerous. Table 2 summarizes in detail the type of albumin used, the receptor pathway, the type of application, and the level of experimentation.
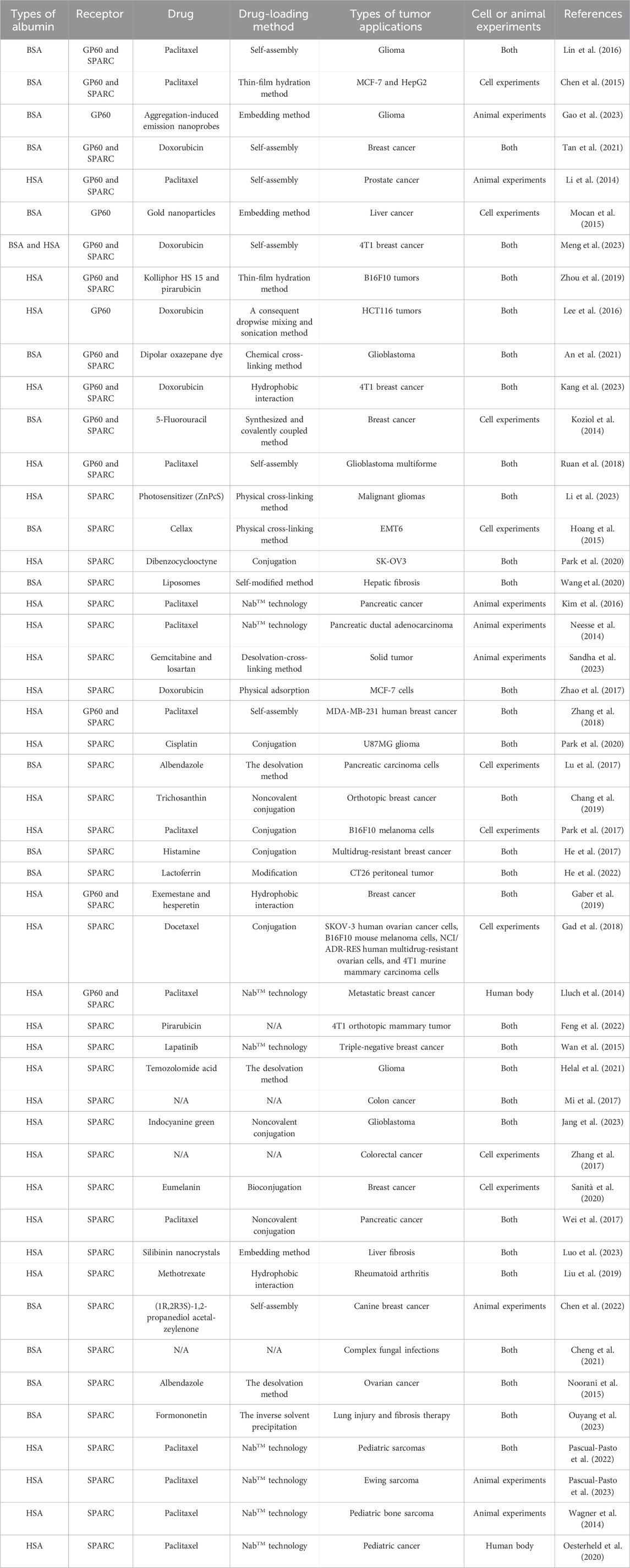
TABLE 2. Study of drug delivery systems constructed on the basis of albumin–GP60 or albumin–SPARC interaction in the last decade.
As shown in Table 2, BSA is significantly more frequently used than HSA, which may be due to several reasons, including the low price of BSA, easy availability of raw materials, and low cost of production (Liu et al., 2022; Zhao et al., 2022). For GP60 receptors and SPARC, SPARC was more frequently used to construct albumin-based drug carriers, which might be due to the reason that SPARC is directly expressed and secreted by tumor cells. Drug carriers constructed based on the albumin–SPARC interaction can reach the tumor tissue or cells more efficiently. Compared to conducting the targeting experiment of GP60 or SPARC at the cellular level only, most research studies about the albumin-based drug delivery system performed both cell experiments and animal experiments, which may be due to the fact that it is more convincing to conduct the targeting experiment of GP60 and SPARC based on the animal experiment level.
In 2016, an article published in ACS Nano reported that albumin nanoparticles could penetrate the blood–brain barrier for biomimetic drug delivery. The main principle is to achieve targeted drug delivery through SPARC- and GP60-mediated biomimetic transport. The constructed albumin nanoparticles exhibited enhanced BBB penetration, intra-tumoral infiltration, and cellular uptake, and this research provided a facile method for dual drug-loaded albumin nanoparticle preparation and a promising avenue for biomimetic delivery targeting brain tumors based on combination therapy (Lin et al., 2016). Tan et al. used albumin to prepare chondroitin sulfate-mediated nanoparticles, and the nanoparticles led to greater drug accumulation at the tumor site than with DOX nanoparticles (no albumin modified) or free DOX. The main reason for this effect was that albumin nanoparticles utilized the GP60- and SPARC-mediated pathway for targeted DOX delivery, and the effect resulted in significant inhibition of tumor growth and lower exposure of major organs to DOX (Tan et al., 2021). Li et al. thought nanoparticles using albumin as a particle matrix had entered the mainstream of drug delivery because albumin can interact with its receptors or binding proteins (Li et al., 2014). They concluded that the non-crosslinked formulation was more advantageous for the delivery of paclitaxel by a comparative study. Zhou et al. used the thin-film hydration method to obtain an albumin-bound complex of albumin–pirarubicin (Zhou et al., 2019). In their research, the lack of any chemical reactions preserved albumin bioactivities, and the albumin–pirarubicin complex showed greater tumor accumulation and tumor penetration through GP60- and SPARC-mediated biomimetic transport than pirarubicin and denatured albumin–pirarubicin. An et al. constructed a noble triple-receptor-targeting fluorescent complex based on the GP60- and SPARC-mediated pathway (An et al., 2021). In the study, the imaging of glioblastoma (GBM) cell lines and human clinical GBM tissues was successfully demonstrated, and the study presented great promise for the application of these albumin complexes for GBM identification and surgery at clinical sites. Other research studies of albumin nanoparticles based on the GP60- and SPARC-mediated pathway are shown in Table 2.
Albumin nanoparticles can be enriched in tumor tissue in two ways. The first way is to achieve enrichment in tumor tissue through the receptor protein pathway, and the second way is to achieve enrichment in tumor tissue via an enhanced permeability and retention effect (EPR effect). Why do some studies achieve targeted drug delivery using just one receptor? First, this is related to the choice of enrichment. The use of both GP60 and SPARC receptors together places greater emphasis on targeted drug delivery through the receptor protein pathway. While just one receptor was used, the study placed more emphasis on achieving targeted drug delivery through the combined use of both modalities (the receptor protein pathway and EPR effect). Second, the different delivery modes of albumin nanoparticles also determine whether they can select a single receptor for targeted delivery. For example, delivery by in situ injection at the tumor site allows the selection of only the SPARC receptor for targeted drug delivery. If the albumin nanoparticles are administered via intravenous injection, it may be necessary to utilize both GP60 and SPARC receptors to achieve a targeted delivery effect. Third, experimental studies of albumin nanoparticles carried out solely at the cellular experimental level can also achieve targeting effects using only a single receptor.
In 2009, Knauer et al. revealed that the albumin-binding domain of SPARC was located at its C-terminus (Knauer et al., 2009). However, there are no articles on the binding regions of albumin to GP60 and SPARC. In 2017, Mi et al. showed interactions between HSA and SPARC via molecular dynamic simulation; however, the result is also only a visualization of the picture and still does not guide the specific domain of interaction (Mi et al., 2017). In our ongoing research, we tentatively found that the IIAIIB region of albumin may be its binding region to GP60 and SPARC, but this still needs to be supported by more detailed research data.
5 Discussion
The denaturation of albumin affects its ability to bind to the GP60 receptor and SPARC, so maintaining the activity of albumin as much as possible helps to strengthen its interaction with the GP60 receptor and SPARC. However, albumin-based drug carriers are often denatured by exposure to organic solvents during preparation, which reduces their ability to bind to the GP60 receptor or SPARC and can even lead to the loss of their ability to bind to the GP60 receptor or SPARC. In order to overcome the problem, strategies on how to make albumin self-assemble into nanoparticles under conditions of invariance must be proposed as soon as possible. We proposed a strategy to make albumin self-assemble into nanoparticles without the addition of organic reagents using the technology of reverse QTY code several months ago. The experimental results showed that albumin nanoparticles modified by the technology of reverse QTY code can maintain its biological activities and target tumor tissue or cells efficiently via the GP60 receptor and SPARC pathway (Meng et al., 2023). Next, we will continue to conduct research to show better effects of tumor treatment in pigs, monkeys, and even human applications as well.
In recent years, a number of studies have demonstrated the importance of maintaining albumin activities for the targeting delivery of albumin-based drug carriers. In 2022, Nisar et al. investigated the interaction and structural modifications of native albumin (BSA) with iron oxide nanoparticles, and the results provided an understanding of the interaction and structural modifications of native albumin (BSA), which has the potential to provide fundamental repercussions in future studies (Nisar et al., 2022). In 2018, Hyun et al. used native albumin to modify polymer nanoparticles for enhancing drug delivery to solid tumors (Hyun et al., 2018). The results showed a surface layer formed with native albumin facilitated nanoparticle transport and drug delivery into tumors via the interaction with albumin-binding proteins, and the study demonstrated that native albumin can enhance the penetration of nanoparticles through the binding receptor or protein pathway. Back in 2016, Miranda et al. investigated the influence of albumin structure and gold speciation on the synthesis of gold nanoparticles (Miranda É et al., 2016). Their results presented that the denaturation of albumin would expose hydrophobic groups to the solvent, and the result would weaken the ability of the albumin–receptor interaction. This research also confirmed maintaining the biological activities of albumin as key for the targeting of albumin-based carriers. In addition, Wu et al. found albumin with biological activities could enhance the transport of copolymer nanoparticles, and this study confirmed similarly that maintaining the biological activities of albumin is important for targeting the delivery of albumin-based carriers (Wu et al., 2013).
Native albumin has numerous drug-binding sites. If researchers do not need to self-assemble albumin into nanoparticles but just use a single molecule of albumin for drug delivery, it will reduce the chance of denaturing albumin because there is no need to add organic solvents to induce albumin to self-assemble into nanoparticles. Thus, the use of single-molecule albumin to load antitumor drugs may be an effective way to strengthen the efficiency of the albumin–GP60 and albumin–SPARC interactions. In addition, if albumin can be analyzed by rational bioinformatics methods and modified to show amphiphilicity, then the amphiphilic albumin obtained from the modification can also self-assemble into nanoparticles without the addition of chemically induced reagents, and thus, its biological activities can be well preserved.
6 Conclusion
GP60 is overexpressed in vascular endothelial cells, and SPARC is overexpressed in most tumor cells, such as breast cancer, glioma, melanoma, and liver cancer. GP60 and SPARC act as receptor proteins of albumin, so they can interact with albumin closely. Therefore, GP60 and SPARC can be key targets for tumor therapy. Albumin drug delivery systems constructed on the basis of the albumin–GP60 interaction and albumin–SPARC interaction can target tumor tissue or cells via GP60 and SPARC pathways. However, denatured albumin has a diminished ability to bind to GP60 or SPARC, so how to maximize the preservation of albumin activity is the key to the effective use of GP60 or SPARC for cancer therapy.
Author contributions
QJ: conceptualization, data curation, funding acquisition, investigation, writing–original draft, and writing–review and editing. HZ: conceptualization, investigation, methodology, writing–original draft, and writing–review and editing. YQ: writing–original draft. RZ: writing–original draft. LW: writing–original draft. EZ: writing–original draft. XZ: conceptualization, funding acquisition, writing–original draft, and writing–review and editing. RM: conceptualization, formal analysis, funding acquisition, investigation, project administration, software, supervision, writing–original draft, and writing–review and editing.
Funding
The authors declare that financial support was received for the research, authorship, and/or publication of this article. This review was supported by the National Natural Science Foundation of China (No. 32170915 and 82172931), the Natural Science Foundation of Jiangsu Province (No. BK20210947), and the Scientific Research Program of Nantong (No. JC12022091).
Conflict of interest
The authors declare that the research was conducted in the absence of any commercial or financial relationships that could be construed as a potential conflict of interest.
Publisher’s note
All claims expressed in this article are solely those of the authors and do not necessarily represent those of their affiliated organizations, or those of the publisher, the editors, and the reviewers. Any product that may be evaluated in this article, or claim that may be made by its manufacturer, is not guaranteed or endorsed by the publisher.
References
Alachkar, H., Santhanam, R., Maharry, K., Metzeler, K. H., Huang, X., Kohlschmidt, J., et al. (2014). SPARC promotes leukemic cell growth and predicts acute myeloid leukemia outcome. J. Clin. investigation 124, 1512–1524. Epub 2014/03/05. doi:10.1172/JCI70921
An, J. M., Moon, H., Verwilst, P., Shin, J., Kim, B. M., Park, C. K., et al. (2021). Human glioblastoma visualization: triple receptor-targeting fluorescent complex of dye, SIWV tetra-peptide, and serum albumin protein. ACS Sensors. 25 (6), 2270–2280. Epub 2021/06/09. doi:10.1021/acssensors.1c00320
Atorrasagasti, C., Aquino, J. B., Hofman, L., Alaniz, L., Malvicini, M., Garcia, M., et al. (2011). SPARC downregulation attenuates the profibrogenic response of hepatic stellate cells induced by TGF-β1 and PDGF. Am. J. physiology Gastrointest. liver physiology 300, G739–G748. Epub 2011/02/12. doi:10.1152/ajpgi.00316.2010
Atorrasagasti, C., Onorato, A. M., and Mazzolini, G. (2022). The role of SPARC (secreted protein acidic and rich in cysteine) in the pathogenesis of obesity, type 2 diabetes, and non-alcoholic fatty liver disease. J. physiology Biochem. 79, 815–831. Epub 2022/08/27. doi:10.1007/s13105-022-00913-5
Bao, J. M., Dang, Q., Lin, C. J., Lo, U. G., Feldkoren, B., Dang, A., et al. (2021). SPARC is a key mediator of TGF-β-induced renal cancer metastasis. J. Cell. physiology 236, 1926–1938. doi:10.1002/jcp.29975
Bhoopathi, P., Chetty, C., Dontula, R., Gujrati, M., Dinh, D. H., Rao, J. S., et al. (2011). SPARC stimulates neuronal differentiation of medulloblastoma cells via the Notch1/STAT3 pathway. Cancer Res. 71, 4908–4919. Epub 2011/05/27. doi:10.1158/0008-5472.CAN-10-3395
Bhoopathi, P., Gondi, C. S., Gujrati, M., Dinh, D. H., and Lakka, S. S. (2011). SPARC mediates Src-induced disruption of actin cytoskeleton via inactivation of small GTPases Rho-Rac-Cdc42. Cell. Signal. 23, 1978–1987. Epub 2011/07/30. doi:10.1016/j.cellsig.2011.07.008
Bradshaw, A. D. (2016). The role of secreted protein acidic and rich in cysteine (SPARC) in cardiac repair and fibrosis: does expression of SPARC by macrophages influence outcomes? J. Mol. Cell. Cardiol. 93, 156–161. Epub 2015/11/20. doi:10.1016/j.yjmcc.2015.11.014
Chang, Y., Yao, S., Chen, Y., Huang, J., Wu, A., Zhang, M., et al. (2019). Genetically-engineered protein prodrug-like nanoconjugates for tumor-targeting biomimetic delivery via a SHEATH strategy. Nanoscale 11, 611–621. Epub 2018/12/18. doi:10.1039/c8nr08951e
Chen, C., Hu, H., Qiao, M., Zhao, X., Wang, Y., Chen, K., et al. (2015). Anti-tumor activity of paclitaxel through dual-targeting lipoprotein-mimicking nanocarrier. J. drug Target. 23, 311–322. Epub 2014/12/30. doi:10.3109/1061186X.2014.994182
Chen, J., Wang, M., Xi, B., Xue, J., He, D., Zhang, J., et al. (2012). SPARC is a key regulator of proliferation, apoptosis and invasion in human ovarian cancer. PloS one 7, e42413. Epub 2012/08/11. doi:10.1371/journal.pone.0042413
Chen, Q., Xu, C., Sun, Z., Yang, J., Chen, F., Lin, Z., et al. (2022). Development of S4A-BSA-Au NPs for enhanced anti-tumor therapy of canine breast cancer. Nanoscale Adv. 4, 1808–1814. Epub 2022/09/23. doi:10.1039/d1na00640a
Chen, S., Zou, Q., Chen, Y., Kuang, X., Wu, W., Guo, M., et al. (2020). Regulation of SPARC family proteins in disorders of the central nervous system. Brain Res. Bull. 163, 178–189. Epub 2020/05/18. doi:10.1016/j.brainresbull.2020.05.005
Cheng, L., Niu, M. M., Yan, T., Ma, Z., Huang, K., Yang, L., et al. (2021). Bioresponsive micro-to-nano albumin-based systems for targeted drug delivery against complex fungal infections. Acta Pharm. Sin. B 11, 3220–3230. Epub 2021/11/04. doi:10.1016/j.apsb.2021.04.020
Chetty, C., Dontula, R., Ganji, P. N., Gujrati, M., and Lakka, S. S. (2012). SPARC expression induces cell cycle arrest via STAT3 signaling pathway in medulloblastoma cells. Biochem. biophysical Res. Commun. 417, 874–879. Epub 2011/12/31. doi:10.1016/j.bbrc.2011.12.065
Fang, R. H., Gao, W., and Zhang, L. (2023). Targeting drugs to tumours using cell membrane-coated nanoparticles. Nat. Rev. Clin. Oncol. 20, 33–48. Epub 2022/10/29. doi:10.1038/s41571-022-00699-x
Feng, J., and Tang, L. (2014). SPARC in tumor pathophysiology and as a potential therapeutic target. Curr. Pharm. Des. 20, 6182–6190. Epub 2014/06/21. doi:10.2174/1381612820666140619123255
Feng, J., Xiang, L., Fang, C., Tan, Y., Li, Y., Gong, T., et al. (2022). Dual-targeting of tumor cells and tumor-associated macrophages by palmitic acid modified albumin nanoparticles for antitumor and antimetastasis therapy. ACS Appl. Mater. interfaces 14, 14887–14902. Epub 2022/03/29. doi:10.1021/acsami.1c23274
Forsthuber, M., Kaiser, A. M., Granitzer, S., Hassl, I., Hengstschläger, M., Stangl, H., et al. (2020). Albumin is the major carrier protein for PFOS, PFOA, PFHxS, PFNA and PFDA in human plasma. Environ. Int. 137, 105324. Epub 2020/02/29. doi:10.1016/j.envint.2019.105324
Funk, S. E., and Sage, E. H. (1991). The Ca2(+)-binding glycoprotein SPARC modulates cell cycle progression in bovine aortic endothelial cells. Proc. Natl. Acad. Sci. U. S. A. 88, 2648–2652. Epub 1991/04/01. doi:10.1073/pnas.88.7.2648
Gaber, M., Hany, M., Mokhtar, S., Helmy, M. W., Elkodairy, K. A., and Elzoghby, A. O. (2019). Boronic-targeted albumin-shell oily-core nanocapsules for synergistic aromatase inhibitor/herbal breast cancer therapy. Mater. Sci. Eng. C, Mater. Biol. Appl. 105, 110099. Epub 2019/09/25. doi:10.1016/j.msec.2019.110099
Gad, S. F., Park, J., Park, J. E., Fetih, G. N., Tous, S. S., Lee, W., et al. (2018). Enhancing docetaxel delivery to multidrug-resistant cancer cells with albumin-coated nanocrystals. Mol. Pharm. 15, 871–881. Epub 2018/01/18. doi:10.1021/acs.molpharmaceut.7b00783
Gao, D., Li, Y., Wu, Y., Liu, Y., Hu, D., Liang, S., et al. (2023). Albumin-consolidated AIEgens for boosting glioma and cerebrovascular NIR-II fluorescence imaging. ACS Appl. Mater. interfaces 15, 3–13. Epub 2022/01/08. doi:10.1021/acsami.1c22700
Gao, Z. W., Liu, C., Yang, L., He, T., Wu, X. N., Zhang, H. Z., et al. (2021). SPARC overexpression promotes liver cancer cell proliferation and tumor growth. Front. Mol. Biosci. 8, 775743. Epub 2021/12/17. doi:10.3389/fmolb.2021.775743
Girotti, M. R., Fernández, M., López, J. A., Camafeita, E., Fernández, E. A., Albar, J. P., et al. (2011). SPARC promotes cathepsin B-mediated melanoma invasiveness through a collagen I/α2β1 integrin axis. J. investigative dermatology 131, 2438–2447. Epub 2011/08/19. doi:10.1038/jid.2011.239
Goldblum, S. E., Ding, X., Funk, S. E., and Sage, E. H. (1994). SPARC (secreted protein acidic and rich in cysteine) regulates endothelial cell shape and barrier function. Proc. Natl. Acad. Sci. U. S. A. 91, 3448–3452. Epub 1994/04/12. doi:10.1073/pnas.91.8.3448
Golembieski, W. A., Ge, S., Nelson, K., Mikkelsen, T., and Rempel, S. A. (1999). Increased SPARC expression promotes U87 glioblastoma invasion in vitro. Int. J. Dev. Neurosci. official J. Int. Soc. Dev. Neurosci. 17, 463–472. Epub 1999/11/26. doi:10.1016/s0736-5748(99)00009-x
Gorantla, B., Bhoopathi, P., Chetty, C., Gogineni, V. R., Sailaja, G. S., Gondi, C. S., et al. (2013). Notch signaling regulates tumor-induced angiogenesis in SPARC-overexpressed neuroblastoma. Angiogenesis 16, 85–100. Epub 2012/09/08. doi:10.1007/s10456-012-9301-1
Hama, M., Ishima, Y., Chuang, V. T. G., Ando, H., Shimizu, T., and Ishida, T. (2021). Evidence for delivery of Abraxane via a denatured-albumin transport system. ACS Appl. Mater. interfaces 13, 19736–19744. Epub 2021/04/22. doi:10.1021/acsami.1c03065
Harris, B. S., Zhang, Y., Card, L., Rivera, L. B., Brekken, R. A., and Bradshaw, A. D. (2011). SPARC regulates collagen interaction with cardiac fibroblast cell surfaces. Am. J. physiology Heart circulatory physiology 301, H841–H847. Epub 2011/06/15. doi:10.1152/ajpheart.01247.2010
Hassanin, I., and Elzoghby, A. (2020). Albumin-based nanoparticles: a promising strategy to overcome cancer drug resistance. Cancer drug Resist. (Alhambra, Calif. 3, 930–946. Epub 2020/11/03. doi:10.20517/cdr.2020.68
He, J., Fan, K., and Yan, X. (2019). Ferritin drug carrier (FDC) for tumor targeting therapy. J. Control. Release 311-312, 288–300. doi:10.1016/j.jconrel.2019.09.002
He, Y., Fang, Y., Zhang, M., Zhao, Y., Tu, B., Shi, M., et al. (2022). Remodeling "cold" tumor immune microenvironment via epigenetic-based therapy using targeted liposomes with in situ formed albumin corona. Acta Pharm. Sin. B 12, 2057–2073. doi:10.1016/j.apsb.2021.09.022
He, Y. J., Xing, L., Cui, P. F., Zhang, J. L., Zhu, Y., Qiao, J. B., et al. (2017). Transferrin-inspired vehicles based on pH-responsive coordination bond to combat multidrug-resistant breast cancer. Biomaterials. 113, 266–278. Epub 2016/11/15. doi:10.1016/j.biomaterials.2016.11.001
Helal, D. O., Rouatbi, N., Han, S., Tzu-Wen Wang, J., Walters, A. A., Abdel-Mottaleb, M. M. A., et al. (2021). A natural protein based platform for the delivery of Temozolomide acid to glioma cells. Eur. J. Pharm. Biopharm. official J. Arbeitsgemeinschaft fur Pharmazeutische Verfahrenstechnik eV. 169, 297–308. Epub 2021/10/23. doi:10.1016/j.ejpb.2021.10.007
Hoang, B., Ernsting, M. J., Roy, A., Murakami, M., Undzys, E., and Li, S. D. (2015). Docetaxel-carboxymethylcellulose nanoparticles target cells via a SPARC and albumin dependent mechanism. Biomaterials. 59, 66–76. Epub 2015/05/10. doi:10.1016/j.biomaterials.2015.04.032
Hu, H., Quintana, J., Weissleder, R., Parangi, S., and Miller, M. (2022). Deciphering albumin-directed drug delivery by imaging. Adv. drug Deliv. Rev. 185, 114237. Epub 2022/04/02. doi:10.1016/j.addr.2022.114237
Hua, H. W., Jiang, H. S., Jia, L., Jia, Y. P., Yao, Y. L., Chen, Y. W., et al. (2021). SPARC regulates ferroptosis induced by sorafenib in human hepatocellular carcinoma. Cancer biomarkers Sect. A Dis. markers 32, 425–433. Epub 2021/04/13. doi:10.3233/CBM-200101
Hyun, H., Park, J., Willis, K., Park, J. E., Lyle, L. T., Lee, W., et al. (2018). Surface modification of polymer nanoparticles with native albumin for enhancing drug delivery to solid tumors. Biomaterials 180, 206–224. Epub 2018/07/27. doi:10.1016/j.biomaterials.2018.07.024
Iancu, C., Mocan, L., Bele, C., Orza, A. I., Tabaran, F. A., Catoi, C., et al. (2011). Enhanced laser thermal ablation for the in vitro treatment of liver ca ncer by specific delivery of multiwalled carbon nanotubes functionaliz ed with human serum albumin. Int. J. nanomedicine 6, 129–141. doi:10.2147/IJN.S15841
Ishima, Y., Maruyama, T., Otagiri, M., Chuang, V. T. G., and Ishida, T. (2022). The new delivery strategy of albumin carrier utilizing the interaction with albumin receptors. Chem. Pharm. Bull. 70, 330–333. Epub 2022/05/02. doi:10.1248/cpb.c21-01024
Jagdish, R. K., Maras, J. S., and Sarin, S. K. (2021). Albumin in advanced liver diseases: the good and bad of a drug!. Hepatology 74, 2848–2862. Epub 2021/03/28. doi:10.1002/hep.31836
Jaiswal, V. D., Pangam, D. S., and Dongre, P. M. (2023). Biophysical study of cisplatin loaded albumin-gold nanoparticle and its interaction with glycans of gp60 receptor. Int. J. Biol. Macromol. 231, 123368. Epub 2023/01/23. doi:10.1016/j.ijbiomac.2023.123368
Jang, H. J., Song, M. G., Park, C. R., Youn, H., Lee, Y. S., Cheon, G. J., et al. (2023). Genome-Engineered mpkCCDc14 cells as a new resource for studying AQP2. Int. J. Mol. Sci. 24, 1684. Epub 2023/01/09. doi:10.3390/ijms24021684
Jendraschak, E., and Sage, E. H. (1996). Regulation of angiogenesis by SPARC and angiostatin: implications for tumor cell biology. Seminars cancer Biol. 7, 139–146. Epub 1996/06/01. doi:10.1006/scbi.1996.0019
Jiang, S., Sun, H. F., Li, S., Zhang, N., Chen, J. S., and Liu, J. X. (2023). SPARC: a potential target for functional nanomaterials and drugs. Front. Mol. Biosci. 10, 1235428. Epub 2023/08/14. doi:10.3389/fmolb.2023.1235428
John, T. A., Vogel, S. M., Minshall, R. D., Ridge, K., Tiruppathi, C., and Malik, A. B. (2001). Evidence for the role of alveolar epithelial gp60 in active transalveolar albumin transport in the rat lung. J. Physiol. 1 (533), 547–559. Epub 2001/06/05. doi:10.1111/j.1469-7793.2001.0547a.x
Kamihagi, K., Katayama, M., Ouchi, R., and Kato, I. (1994). Osteonectin/SPARC regulates cellular secretion rates of fibronectin and laminin extracellular matrix proteins. Biochem. biophysical Res. Commun. 200, 423–428. Epub 1994/04/15. doi:10.1006/bbrc.1994.1466
Kang, W., Shi, Y., Yang, Z., Yin, X., Zhao, Y., Weng, L., et al. (2023). Flexible human serum albumin nanocapsules to enhance drug delivery and cellular uptake for photodynamic/chemo cancer therapy. RSC Adv. 13, 5609–5618. Epub 2023/02/18. doi:10.1039/d2ra06859a
Kim, H., Samuel, S., Lopez-Casas, P., Grizzle, W., Hidalgo, M., Kovar, J., et al. (2016). SPARC-independent delivery of nab-paclitaxel without depleting tumor stroma in patient-derived pancreatic cancer xenografts. Mol. cancer Ther. 15, 680–688. Epub 2016/02/03. doi:10.1158/1535-7163.MCT-15-0764
Knauer, D., Hwang, L., Lowe, C., Hwang, J., Norng, M., Trieu, V., et al. (2009). Albumin-binding and angiogenic domains of SPARC located at its C-terminus. Cancer Res. 69, 2144. doi:10.1158/0008-5472.sabcs-09-2144
Koziol, M. J., Sievers, T. K., Smuda, K., Xiong, Y., Müller, A., Wojcik, F., et al. (2014). Kinetics and efficiency of a methyl-carboxylated 5-Fluorouracil-bovine serum albumin adduct for targeted delivery. Macromol. Biosci. 14, 428–439. Epub 2014/05/14. doi:10.1002/mabi.201300363
Kumari, N., Mathe, V. L., and Dongre, P. M. (2019). Albumin nanoparticles conjugates binding with glycan - a strategic approach for targeted drug delivery. Int. J. Biol. Macromol. 126, 74–90. Epub 2018/12/26. doi:10.1016/j.ijbiomac.2018.12.184
Kumari, N., Mathe, V. L., Krishna, C. M., and Dongre, P. M. (2022). BSA-drug-ZnO-PEI conjugates interaction with glycans of gp60 endothelial cell receptor protein for targeted drug delivery: a comprehensive spectroscopic study. J. Biomol. Struct. Dyn. 40, 9253–9269. Epub 2021/05/22. doi:10.1080/07391102.2021.1925155
Lane, T. F., and Sage, E. H. (1994). The biology of SPARC, a protein that modulates cell-matrix interactions. FASEB J. official Publ. Fed. Am. Soc. Exp. Biol. 8, 163–173. Epub 1994/02/01. doi:10.1096/fasebj.8.2.8119487
Lankat-Buttgereit, B., Mann, K., Deutzmann, R., Timpl, R., and Krieg, T. (1988). Cloning and complete amino acid sequences of human and murine basement membrane protein BM-40 (SPARC, osteonectin). FEBS Lett. 236, 352–356. Epub 1988/08/29. doi:10.1016/0014-5793(88)80054-1
Le Bail, B., Faouzi, S., Boussarie, L., Guirouilh, J., Blanc, J. F., Carles, J., et al. (1999). Osteonectin/SPARC is overexpressed in human hepatocellular carcinoma. J. pathology 189, 46–52. Epub 1999/08/19. doi:10.1002/(SICI)1096-9896(199909)189:1<46::AID-PATH392>3.0.CO;2-X
Lee, E. J., Lee, N. K., and Kim, I. S. (2016). Bioengineered protein-based nanocage for drug delivery. Adv. drug Deliv. Rev. 106, 157–171. Epub 2016/10/31. doi:10.1016/j.addr.2016.03.002
Lee, S., Lee, C., Kim, B., Thao, L. Q., Lee, E. S., Kim, J. O., et al. (2016). A novel prototype of albumin nanoparticles fabricated by supramolecular cyclodextrin-adamantane association. Colloids surfaces B, Biointerfaces. 147, 281–290. Epub 2016/08/16. doi:10.1016/j.colsurfb.2016.08.009
Li, C., Li, Y., Gao, Y., Wei, N., Zhao, X., Wang, C., et al. (2014). Direct comparison of two albumin-based paclitaxel-loaded nanoparticle formulations: is the crosslinked version more advantageous? Int. J. Pharm. 468, 15–25. Epub 2014/04/09. doi:10.1016/j.ijpharm.2014.04.010
Li, X., Oh, J. S., Lee, Y., Lee, E. C., Yang, M., Kwon, N., et al. (2023). Albumin-binding photosensitizer capable of targeting glioma via the SPARC pathway. Biomaterials Res. 27, 23. Epub 2023/03/23. doi:10.1186/s40824-023-00360-3
Li, X. L., Li, J. L., Qiu, D. J., and Ma, L. (2022). Methylation-mediated expression of SPARC is correlated with tumor progression and poor prognosis of breast cancer. Neoplasma. 69, 794–806. Epub 2022/04/12. doi:10.4149/neo_2022_211002N1401
Liang, M., Fan, K., Zhou, M., Duan, D., Zheng, J., Yang, D., et al. (2014). H-ferritin-nanocaged doxorubicin nanoparticles specifically target and kill tumors with a single-dose injection. Proc. Natl. Acad. Sci. U. S. A. 111, 14900–14905. Epub 2014/10/01. doi:10.1073/pnas.1407808111
Lin, T., Zhao, P., Jiang, Y., Tang, Y., Jin, H., Pan, Z., et al. (2016). Blood-brain-barrier-penetrating albumin nanoparticles for biomimetic drug delivery via albumin-binding protein pathways for antiglioma therapy. ACS Nano. 22 (10), 9999–10012. Epub 2016/12/10. doi:10.1021/acsnano.6b04268
Liu, B., Lv, T., Zhao, X., Zhou, M., Song, C., Zeng, C., et al. (2022). Fluorescence discrimination of HSA from BSA: a close look at the albumin-induced restricted intramolecular rotation of flavonoid probe. Spectrochimica acta Part A, Mol. Biomol. Spectrosc. 264, 120306. Epub 2021/08/31. doi:10.1016/j.saa.2021.120306
Liu, L., Hu, F., Wang, H., Wu, X., Eltahan, A. S., Stanford, S., et al. (2019). Secreted protein acidic and rich in cysteine mediated biomimetic delivery of methotrexate by albumin-based nanomedicines for rheumatoid arthritis therapy. ACS Nano 28 (13), 5036–5048. Epub 2019/04/13. doi:10.1021/acsnano.9b01710
Lluch, A., Alvarez, I., Muñoz, M., Seguí, M., Tusquets, I., and García-Estévez, L. (2014). Treatment innovations for metastatic breast cancer: nanoparticle albumin-bound (NAB) technology targeted to tumors. Crit. Rev. oncology/hematology 89, 62–72. Epub 2013/09/28. doi:10.1016/j.critrevonc.2013.08.001
López-Murcia, F. J., Terni, B., and Llobet, A. (2015). SPARC triggers a cell-autonomous program of synapse elimination. Proc. Natl. Acad. Sci. U. S. A. 112, 13366–13371. Epub 2015/10/01. doi:10.1073/pnas.1512202112
Lu, H., Noorani, L., Jiang, Y., Du, A. W., and Stenzel, M. H. (2017). Penetration and drug delivery of albumin nanoparticles into pancreatic multicellular tumor spheroids. J. Mater. Chem. B 5, 9591–9599. Epub 2017/12/28. doi:10.1039/c7tb02902k
Luo, S., Yang, Y., Zhao, T., Zhang, R., Fang, C., Li, Y., et al. (2023). Albumin-based silibinin nanocrystals targeting activated hepatic stellate cells for liver fibrosis therapy. ACS Appl. Mater. interfaces 15, 7747–7758. Epub 2023/02/01. doi:10.1021/acsami.2c19269
Luo, Z., Zhou, Y., Luo, P., Zhao, Q., Xiao, N., Yu, Y., et al. (2014). SPARC deficiency affects bone marrow stromal function, resulting in impaired B lymphopoiesis. J. Leukoc. Biol. 96, 73–82. Epub 2014/03/07. doi:10.1189/jlb.1A0713-415RR
Maniatis, N. A., Brovkovych, V., Allen, S. E., John, T. A., Shajahan, A. N., Tiruppathi, C., et al. (2006). Novel mechanism of endothelial nitric oxide synthase activation mediated by caveolae internalization in endothelial cells. Circulation Res. 99, 870–877. Epub 2006/09/16. doi:10.1161/01.RES.0000245187.08026.47
Meng, R., Hao, S., Sun, C., Hou, Z., Hou, Y., Wang, L., et al. (2023). Reverse-QTY code design of active human serum albumin self-assembled amphiphilic nanoparticles for effective anti-tumor drug doxorubicin release in mice. Proc. Natl. Acad. Sci. U. S. A. 120, e2220173120. Epub 2023/05/15. doi:10.1073/pnas.2220173120
Mi, F. L., Burnouf, T., Lu, S. Y., Lu, Y. J., Lu, K. Y., Ho, Y. C., et al. (2017). Self-targeting, immune transparent plasma protein coated nanocomplex for noninvasive photothermal anticancer therapy. Adv. Healthc. Mater. 6. Epub 2017/07/20. doi:10.1002/adhm.201700181
Milici, A. J., Watrous, N. E., Stukenbrok, H., and Palade, G. E. (1987). Transcytosis of albumin in capillary endothelium. J. Cell Biol. 105(6 Pt 1), 2603–2612. doi:10.1083/jcb.105.6.2603
Minshall, R. D., Tiruppathi, C., Vogel, S. M., Niles, W. D., Gilchrist, A., Hamm, H. E., et al. (2000). Endothelial cell-surface gp60 activates vesicle formation and trafficking via G(i)-coupled Src kinase signaling pathway. J. Cell Biol. 150, 1057–1070. Epub 2000/09/07. doi:10.1083/jcb.150.5.1057
Miranda É, G., Tofanello, A., Brito, A. M., Lopes, D. M., Albuquerque, L. J., de Castro, C. E., et al. (2016). Effects of gold salt speciation and structure of human and bovine serum albumins on the synthesis and stability of gold nanostructures. Front. Chem. 4, 13. Epub 2016/04/12. doi:10.3389/fchem.2016.00013
Mocan, L., Matea, C., Tabaran, F. A., Mosteanu, O., Pop, T., Mocan, T., et al. (2015). Photothermal treatment of liver cancer with albumin-conjugated gold nanoparticles initiates Golgi Apparatus-ER dysfunction and caspase-3 apoptotic pathway activation by selective targeting of Gp60 receptor. Int. J. nanomedicine 10, 5435–5445. Epub 2015/09/09. doi:10.2147/IJN.S86495
Molitoris, B. A., Sandoval, R. M., Yadav, S. P. S., and Wagner, M. C. (2022). Albumin uptake and processing by the proximal tubule: physiological, pathological, and therapeutic implications. Physiol. Rev. 102, 1625–1667. Epub 2022/04/06. doi:10.1152/physrev.00014.2021
Nagaraju, G. P., and El-Rayes, B. F. (2013). SPARC and DNA methylation: possible diagnostic and therapeutic implications in gastrointestinal cancers. Cancer Lett. 328, 10–17. Epub 2012/09/04. doi:10.1016/j.canlet.2012.08.028
Neesse, A., Frese, K. K., Chan, D. S., Bapiro, T. E., Howat, W. J., Richards, F. M., et al. (2014). SPARC independent drug delivery and antitumour effects of nab-paclitaxel in genetically engineered mice. Gut 63, 974–983. Epub 2013/09/27. doi:10.1136/gutjnl-2013-305559
Nie, J., and Sage, E. H. (2009). SPARC inhibits adipogenesis by its enhancement of beta-catenin signaling. J. Biol. Chem. 284, 1279–1290. Epub 2008/11/08. doi:10.1074/jbc.M808285200
Nisar, A., Ajabia, D. K., Agrawal, S. B., Varma, S., Chaudhari, B. P., and Tupe, R. S. (2022). Mechanistic insight into differential interactions of iron oxide nanoparticles with native, glycated albumin and their effect on erythrocytes parameters. Int. J. Biol. Macromol. 212, 232–247. Epub 2022/05/22. doi:10.1016/j.ijbiomac.2022.05.106
Noorani, L., Stenzel, M., Liang, R., Pourgholami, M. H., and Morris, D. L. (2015). Albumin nanoparticles increase the anticancer efficacy of albendazole in ovarian cancer xenograft model. J. nanobiotechnology 13, 25. Epub 2015/04/19. doi:10.1186/s12951-015-0082-8
Nyman, D. W., Campbell, K. J., Hersh, E., Long, K., Richardson, K., Trieu, V., et al. (2005). Phase I and pharmacokinetics trial of ABI-007, a novel nanoparticle formulation of paclitaxel in patients with advanced nonhematologic malignancies. J. Clin. Oncol. official J. Am. Soc. Clin. Oncol. 23, 7785–7793. Epub 2005/11/01. doi:10.1200/JCO.2004.00.6148
Oesterheld, J. E., Reed, D. R., Setty, B. A., Isakoff, M. S., Thompson, P., Yin, H., et al. (2020). Phase II trial of gemcitabine and nab-paclitaxel in patients with recurrent Ewing sarcoma: a report from the National Pediatric Cancer Foundation. Pediatr. blood cancer 67, e28370. Epub 2020/05/10. doi:10.1002/pbc.28370
Ouyang, B., Deng, L., Yang, F., Shi, H., Wang, N., Tang, W., et al. (2023). Albumin-based formononetin nanomedicines for lung injury and fibrosis therapy via blocking macrophage pyroptosis. Mater. today Bio 20, 100643. Epub 2023/05/22. doi:10.1016/j.mtbio.2023.100643
Park, C. R., Kim, H. Y., Song, M. G., Lee, Y. S., Youn, H., Chung, J. K., et al. (2020). Efficacy and safety of human serum albumin-cisplatin complex in U87MG xenograft mouse models. Int. J. Mol. Sci. 21, 7932. Epub 2020/10/30. doi:10.3390/ijms21217932
Park, C. R., Song, M. G., Park, J. Y., Youn, H., Chung, J. K., Jeong, J. M., et al. (2020). Conjugation of arginylglycylaspartic acid to human serum albumin decreases the tumor-targeting effect of albumin by hindering its secreted protein acidic and rich in cysteine-mediated accumulation in tumors. Am. J. Transl. Res. 12, 2488–2498. Epub 2020/07/14.
Park, J., Sun, B., and Yeo, Y. (2017). Albumin-coated nanocrystals for carrier-free delivery of paclitaxel. J. Control. release official J. Control. Release Soc. 263, 90–101. Epub 2017/01/04. doi:10.1016/j.jconrel.2016.12.040
Pascual-Pasto, G., Castillo-Ecija, H., Unceta, N., Aschero, R., Resa-Pares, C., Gómez-Caballero, A., et al. (2022). SPARC-mediated long-term retention of nab-paclitaxel in pediatric sarcomas. J. Control. release official J. Control. Release Soc. 342, 81–92. Epub 2022/01/03. doi:10.1016/j.jconrel.2021.12.035
Pascual-Pasto, G., Resa-Pares, C., Castillo-Ecija, H., Aschero, R., Baulenas-Farres, M., Vila-Ubach, M., et al. (2023). Low Bcl-2 is a robust biomarker of sensitivity to nab-paclitaxel in Ewing sarcoma. Biochem. Pharmacol. 208, 115408. Epub 2023/01/06. doi:10.1016/j.bcp.2022.115408
Paul, M., Itoo, A. M., Ghosh, B., and Biswas, S. (2022). Current trends in the use of human serum albumin for drug delivery in cancer. Expert Opin. drug Deliv. 19, 1449–1470. Epub 2022/10/19. doi:10.1080/17425247.2022.2134341
Petersson, S. J., Jørgensen, L. H., Andersen, D. C., Nørgaard, R. C., Jensen, C. H., and Schrøder, H. D. (2013). SPARC is up-regulated during skeletal muscle regeneration and inhibits myoblast differentiation. Histology Histopathol. 28, 1451–1460. Epub 2013/05/15. doi:10.14670/HH-28.1451
Pichler, R. H., Hugo, C., Shankland, S. J., Reed, M. J., Bassuk, J. A., Andoh, T. F., et al. (1996). SPARC is expressed in renal interstitial fibrosis and in renal vascular injury. Kidney Int. 50, 1978–1989. Epub 1996/12/01. doi:10.1038/ki.1996.520
Rempel, S. A., Golembieski, W. A., Fisher, J. L., Maile, M., and Nakeff, A. (2001). SPARC modulates cell growth, attachment and migration of U87 glioma cells on brain extracellular matrix proteins. J. neuro-oncology 53, 149–160. Epub 2001/11/22. doi:10.1023/a:1012201300188
Rivera, L. B., Bradshaw, A. D., and Brekken, R. A. (2011). The regulatory function of SPARC in vascular biology. Cell. Mol. life Sci. CMLS 68, 3165–3173. Epub 2011/08/09. doi:10.1007/s00018-011-0781-8
Rivera, L. B., and Brekken, R. A. (2011). SPARC promotes pericyte recruitment via inhibition of endoglin-dependent TGF-β1 activity. J. Cell Biol. 193, 1305–1319. Epub 2011/06/29. doi:10.1083/jcb.201011143
Rohde, W., Pauli, G., Paulsen, J., Harms, E., and Bauer, H. (1978). Bovine and ovine leukemia viruses. I. Characterization of viral antigens. J. virology. 26, 159–164. Epub 1978/04/01. doi:10.1128/JVI.26.1.159-164.1978
Ruan, C., Liu, L., Lu, Y., Zhang, Y., He, X., Chen, X., et al. (2018). Substance P-modified human serum albumin nanoparticles loaded with paclitaxel for targeted therapy of glioma. Acta Pharm. Sin. B 8, 85–96. Epub 2018/06/07. doi:10.1016/j.apsb.2017.09.008
Ryu, S., Sidorov, S., Ravussin, E., Artyomov, M., Iwasaki, A., Wang, A., et al. (2022). The matricellular protein SPARC induces inflammatory interferon-response in macrophages during aging. Immunity 55, 1609–1626.e7. Epub 2022/08/14. doi:10.1016/j.immuni.2022.07.007
Sage, H., Decker, J., Funk, S., and Chow, M. (1989). SPARC: a Ca2+-binding extracellular protein associated with endothelial cell injury and proliferation. J. Mol. Cell. Cardiol. 21 (1), 13–22. Epub 1989/02/01. doi:10.1016/0022-2828(89)90833-x
Sage, H., Vernon, R. B., Decker, J., Funk, S., and Iruela-Arispe, M. L. (1989). Distribution of the calcium-binding protein SPARC in tissues of embryonic and adult mice. J. Histochem. Cytochem. official J. Histochem. Soc. 37, 819–829. Epub 1989/06/01. doi:10.1177/37.6.2723400
Sandha, K. K., Behera, C., Chibber, P., Kumar, R., Kumar, A., Mondhe, D. M., et al. (2023). Antifibrotic agent mediated tumor microenvironment modulation and improved nanomedicine delivery in solid tumor. Mol. Pharm. 20, 2927–2941. Epub 2023/05/17. doi:10.1021/acs.molpharmaceut.2c01081
Sanità, G., Armanetti, P., Silvestri, B., Carrese, B., Calì, G., Pota, G., et al. (2020). Albumin-modified melanin-silica hybrid nanoparticles target breast cancer cells via a SPARC-dependent mechanism. Front. Bioeng. Biotechnol. 8, 765. Epub 2020/08/01. doi:10.3389/fbioe.2020.00765
Schnitzer, J. E. (1992). gp60 is an albumin-binding glycoprotein expressed by continuous endothelium involved in albumin transcytosis. Am. J. physiology. 262, H246–H254. Epub 1992/01/01. doi:10.1152/ajpheart.1992.262.1.H246
Schnitzer, J. E. (1993). Update on the cellular and molecular basis of capillary permeability. Trends Cardiovasc Med. 3, 124–130. doi:10.1016/1050-1738(93)90012-U
Schnitzer, J. E., Allard, J., and Oh, P. (1995). NEM inhibits transcytosis, endocytosis, and capillary permeability: implication of caveolae fusion in endothelia. Am. J. Physiol. 268, H48–H55. Epub 1995/01/01. doi:10.1152/ajpheart.1995.268.1.H48
Schnitzer, J. E., Carley, W. W., and Palade, G. E. (1988). Albumin interacts specifically with a 60-kDa microvascular endothelial glycoprotein. Proc. Natl. Acad. Sci. U. S. A. 85, 6773–6777. Epub 1988/09/01. doi:10.1073/pnas.85.18.6773
Schnitzer, J. E., and Oh, P. (1994). Albondin-mediated capillary permeability to albumin Differential role of receptors in endothelial transcytosis and endocytosis of native and modified albumins. J. Biol. Chem. 25, 6072-6082. doi:10.1016/S0021-9258(17)37571-3
Schnitzer, J. E., Shen, C. P., and Palade, G. E. (1990). Lectin analysis of common glycoproteins detected on the surface of continuous microvascular endothelium in situ and in culture: identification of sialoglycoproteins. Eur. J. Cell Biol. 52, 241–251. Epub 1990/08/01.
Schnitzer, J. E., Ulmer, J. B., and Palade, G. E. (1990). A major endothelial plasmalemmal sialoglycoprotein, gp60, is immunologically related to glycophorin. Proc. Natl. Acad. Sci. U. S. A. 87, 6843–6847. Epub 1990/09/01. doi:10.1073/pnas.87.17.6843
Schultz, A. M., Copeland, T. D., and Oroszlan, S. (1984). The envelope proteins of bovine leukemia virus: purification and sequence analysis. Virology. 135, 417–427. Epub 1984/06/01. doi:10.1016/0042-6822(84)90197-1
Sleep, D. (2015). Albumin and its application in drug delivery. Expert Opin. drug Deliv. 12, 793–812. Epub 2014/12/19. doi:10.1517/17425247.2015.993313
Song, H., Guan, Y., Zhang, L., Li, K., and Dong, C. (2010). SPARC interacts with AMPK and regulates GLUT4 expression. Biochem. biophysical Res. Commun. 396, 961–966. Epub 2010/05/13. doi:10.1016/j.bbrc.2010.05.033
Spada, A., Emami, J., Tuszynski, J. A., and Lavasanifar, A. (2021). The uniqueness of albumin as a carrier in nanodrug delivery. Mol. Pharm. 18, 1862–1894. Epub 2021/04/01. doi:10.1021/acs.molpharmaceut.1c00046
Stewart, P. A. (2000). Endothelial vesicles in the blood-brain barrier: are they related to permeability? Cell Mol. Neurobiol. 20, 149–163. Epub 2000/03/04. doi:10.1023/a:1007026504843
Sussman, A. N., Sun, T., Krofft, R. M., and Durvasula, R. V. (2009). SPARC accelerates disease progression in experimental crescentic glomerulonephritis. Am. J. pathology 174, 1827–1836. Epub 2009/04/04. doi:10.2353/ajpath.2009.080464
Tai, I. T., and Tang, M. J. (2008). SPARC in cancer biology: its role in cancer progression and potential for therapy. Drug Resist. Updat. Rev. Comment. Antimicrob. anticancer Chemother. 11, 231–246. Epub 2008/10/14. doi:10.1016/j.drup.2008.08.005
Tan, T., Yang, Q., Chen, D., Zhao, J., Xiang, L., Feng, J., et al. (2021). Chondroitin sulfate-mediated albumin corona nanoparticles for the treatment of breast cancer. Asian J. Pharm. Sci. 16, 508–518. Epub 2021/10/28. doi:10.1016/j.ajps.2021.03.004
Termine, J. D., Kleinman, H. K., Whitson, S. W., Conn, K. M., McGarvey, M. L., and Martin, G. R. (1981). Osteonectin, a bone-specific protein linking mineral to collagen. Cell 26, 99–105. Epub 1981/10/01. doi:10.1016/0092-8674(81)90037-4
Tiruppathi, C., Finnegan, A., and Malik, A. B. (1996). Isolation and characterization of a cell surface albumin-binding protein from vascular endothelial cells. Proc. Natl. Acad. Sci. U. S. A. 93, 250–254. Epub 1996/01/09. doi:10.1073/pnas.93.1.250
Tiruppathi, C., Song, W., Bergenfeldt, M., Sass, P., and Malik, A. B. (1997). Gp60 activation mediates albumin transcytosis in endothelial cells by tyrosine kinase-dependent pathway*. J. Biol. Chem. 272, 25968–25975. doi:10.1074/jbc.272.41.25968
Volmer, M. W., Radacz, Y., Hahn, S. A., Klein-Scory, S., Stühler, K., Zapatka, M., et al. (2004). Tumor suppressor Smad4 mediates downregulation of the anti-adhesive invasion-promoting matricellular protein SPARC: landscaping activity of Smad4 as revealed by a "secretome" analysis. Proteomics 4, 1324–1334. Epub 2004/06/10. doi:10.1002/pmic.200300703
Wagner, L. M., Yin, H., Eaves, D., Currier, M., and Cripe, T. P. (2014). Preclinical evaluation of nanoparticle albumin-bound paclitaxel for treatment of pediatric bone sarcoma. Pediatr. blood cancer 61, 2096–2098. Epub 2014/04/23. doi:10.1002/pbc.25062
Wan, X., Zheng, X., Pang, X., Zhang, Z., Jing, T., Xu, W., et al. (2015). The potential use of lapatinib-loaded human serum albumin nanoparticles in the treatment of triple-negative breast cancer. Int. J. Pharm. 484, 516-28. doi:10.1016/j.ijpharm.2015.02.037
Wang, J., Ding, Y., and Zhou, W. (2020). Albumin self-modified liposomes for hepatic fibrosis therapy via SPARC-dependent pathways. Int. J. Pharm., 574, 118940. doi:10.1016/j.ijpharm.2019.118940
Wei, Y., Wang, Y., Xia, D., Guo, S., Wang, F., Zhang, X., et al. (2017). Thermosensitive liposomal codelivery of HSA-paclitaxel and HSA-ellagic acid complexes for enhanced drug perfusion and efficacy against pancreatic cancer. ACS Appl. Mater. interfaces 9, 25138–25151. Epub 2017/07/12. doi:10.1021/acsami.7b07132
Wu, Y., Ihme, S., Feuring-Buske, M., Kuan, S. L., Eisele, K., Lamla, M., et al. (2013). A core-shell albumin copolymer nanotransporter for high capacity loading and two-step release of doxorubicin with enhanced anti-leukemia activity. Adv. Healthc. Mater. 2, 884–894. Epub 2012/12/12. doi:10.1002/adhm.201200296
Yagi, M. J., King, N. W., and Bekesi, J. G. (1980). Alterations of a mouse mammary tumor virus glycoprotein with interferon treatment. J. virology 34, 225–233. Epub 1980/04/01. doi:10.1128/JVI.34.1.225-233.1980
Yusuf, N., Inagaki, T., Kusunoki, S., Okabe, H., Yamada, I., Matsumoto, A., et al. (2014). SPARC was overexpressed in human endometrial cancer stem-like cells and promoted migration activity. Gynecol. Oncol. 134, 356–363. Epub 2014/04/29. doi:10.1016/j.ygyno.2014.04.009
Zhang, S., Jin, J., Tian, X., and Wu, L. (2017). hsa-miR-29c-3p regulates biological function of colorectal cancer by targeting SPARC. Oncotarget. 28 (8), 104508–104524. Epub 2017/12/22. doi:10.18632/oncotarget.22356
Zhang, Y., Guo, Z., Cao, Z., Zhou, W., Zhang, Y., Chen, Q., et al. (2018). Endogenous albumin-mediated delivery of redox-responsive paclitaxel-loaded micelles for targeted cancer therapy. Biomaterials 183, 243–257. Epub 2018/09/05. doi:10.1016/j.biomaterials.2018.06.002
Zhao, S., Yu, Q., Pan, J., Zhou, Y., Cao, C., Ouyang, J. M., et al. (2017). Redox-responsive mesoporous selenium delivery of doxorubicin targets MCF-7 cells and synergistically enhances its anti-tumor activity. Acta biomater. 54, 294–306. Epub 2017/03/08. doi:10.1016/j.actbio.2017.02.042
Zhao, Z., Shi, T., Chu, Y., Cao, Y., Cheng, S., Na, R., et al. (2022). Comparison of the interactions of flupyrimin and nitenpyram with serum albumins via multiple analysis methods. Chemosphere. 289, 133139. Epub 2021/12/06. doi:10.1016/j.chemosphere.2021.133139
Zhao, Z. S., Wang, Y. Y., Chu, Y. Q., Ye, Z. Y., and Tao, H. Q. (2010). SPARC is associated with gastric cancer progression and poor survival of patients. Clin. cancer Res. official J. Am. Assoc. Cancer Res. 16, 260–268. Epub 2009/12/24. doi:10.1158/1078-0432.CCR-09-1247
Keywords: GP60, SPARC, albumin, target delivery, drug carriers
Citation: Ji Q, Zhu H, Qin Y, Zhang R, Wang L, Zhang E, Zhou X and Meng R (2024) GP60 and SPARC as albumin receptors: key targeted sites for the delivery of antitumor drugs. Front. Pharmacol. 15:1329636. doi: 10.3389/fphar.2024.1329636
Received: 30 October 2023; Accepted: 08 January 2024;
Published: 23 January 2024.
Edited by:
Donato Cosco, Magna Græcia University, ItalyReviewed by:
Kelong Fan, Chinese Academy of Sciences (CAS), ChinaSilvia Voci, Magna Græcia University, Italy
Rehan Khan, Rutgers University, Newark, United States
Copyright © 2024 Ji, Zhu, Qin, Zhang, Wang, Zhang, Zhou and Meng. This is an open-access article distributed under the terms of the Creative Commons Attribution License (CC BY). The use, distribution or reproduction in other forums is permitted, provided the original author(s) and the copyright owner(s) are credited and that the original publication in this journal is cited, in accordance with accepted academic practice. No use, distribution or reproduction is permitted which does not comply with these terms.
*Correspondence: Run Meng, mengrun_cqu@163.com; Xiaorong Zhou, zhouxiaorong@ntu.edu.cn