- 1Department of Botany, Pir Mehr Ali Shah Arid Agriculture University, Rawalpindi, Pakistan
- 2Department of Veterinary Pathology, Pir Mehr Ali Shah Arid Agriculture University, Rawalpindi, Pakistan
- 3Department of Clinical Pharmacy, College of Pharmacy, King Saud University, Riyadh, Saudi Arabia
- 4Department of Vegetable Science, Chaudhary Charan Singh Haryana Agricultural University, Hisar, India
Background: Liver disease is a serious health concern in today’s world, posing a challenge to both healthcare providers and pharmaceutical companies. Most synthetic drugs and chemicals cause liver damage accounting for approximately 10% of acute hepatitis and 50% of acute liver failure.
Purpose: The present study aimed to evaluate the hepato-protective activity of an extract of chicory formulation assisted by silver nanoparticles against carbon tetra chloride (CCl4)-induced hepatic damage in rat’s liver.
Methods: Rats of the Wistar strain (Rattus norvegicus) were used to test the in vivo hepato-protective efficacy at various doses. Rats were randomly divided into nine groups, each containing six rats. The groups were as follows: first group (control), second group (CCl4), third group, silymarin (20 mg/kg of body weight), fourth group (CCl4+chicory) (1.75 mg/kg of b. wt), fifth group (CCl4 + chicory at the dose of 2.35 mg/kg), sixth group (CCl4 + chicory of 3.25 mg/kg), seventh group (CCl4 +AgNPs 1.75 mg/kg of b. wt.), eighth group (CCl4 + AgNPs 2.35 mg/kg of body weight), and ninth group (CCl4 + AgNPs 3.25 mg/kg of b. wt.). Blood samples were taken 24 h after the last administration (i.e., 30th day). The blood samples were analyzed for different serum enzymes such as ALP (alkaline phosphatase), ALT (alanine transaminase), bilirubin (Blr), triglyceride, and cholesterol. Histology liver sections were performed.
Results: Treatment with AgNPs and chicory extract showed significant hepato-protective activity in a dose-dependent manner. In three doses, the chicory extract at a rate of 3.25 mg/kg of body weight significantly reduced elevated levels of biochemical markers in comparison to CCl4-intoxicated rats. Histology of the liver sections from CCl4-treated rats revealed inflammation of hepatocytes, necrosis, cytoplasmic degeneration, vacuolization, and a deformed central vein. The chicory formulation extract exhibited a remarkable recovery percentage in the liver architecture that was higher than the drug (i.e., silymarin). While treatment with AgNPs also repaired the degenerative changes and restored the normal form of the liver, chicory formulation extract possessed more hepato-protective potential as compared to AgNPs by regulating biochemical and histo-pathological parameters.
Conclusion: This study can be used as confirmation of the hepato-protective potential of chicory compounds for possible use in the development programs of drugs to treat liver diseases.
Introduction
The liver is the largest glandular organ of the body whose functions are greater than any other organ (Sanjiv, 2002). It regulates a variety of physiological functions such as metabolism, bile secretion, and vitamin storage. In addition, this organ detoxifies various toxic substances and is involved in the synthesis of useful compounds (Latief et al., 2023). It is important in the maintenance of body functions in addition to the regulation of homeostasis, as this organ regulates various metabolic processes such as growth, disease resistance, nutrition delivery, energy flow, and reproduction. Being a detoxifying organ, it is vulnerable to constant attack by foreign molecules, resulting in liver failure (Kumar et al., 2021). Therefore, the maintenance of the liver’s health is important for the wellbeing of an individual. Liver disease is a serious health concern in today’s world, posing a challenge to both healthcare providers and pharmaceutical companies (Smuckler, 1975). Liver disorders are among the most serious health problems in the world (Malhotra and Singh, 2001). These can be classified as acute and chronic hepatitis (inflammatory liver disorders), hepatosis, and cirrhosis. Different toxic chemicals such as carbon tetrachloride, thioacetamide, certain chemotherapeutic agents, high alcohol consumption, and some microbes result in liver cell injury (Bharathi et al., 2023). CCl4 is a xenobiotic that is introduced into water as waste from different industries, causing hepatotoxicity in living creatures. It is frequently used to cause liver disease in various models to test hepato-protective medicines (Malhotra and Singh, 2001).
Synthetic medications available on the market for liver treatment cause many issues (Vairetti et al., 2021). Even the Food and Drug Administration (FDA) of the US has withdrawn or not approved drugs that cause liver ailments (Ostapowicz et al., 2002; Pandit et al., 2012). Over 1,000 drugs and chemicals have been found to be a cause of liver damage (Upadhyay et al., 2010; Porceddu et al., 2012), responsible for 10% of acute hepatitis and 50% of acute liver failure (Pandit et al., 2012). There is increasing concern about drug-induced hepatotoxicity, which also results in malnutrition, malfunctioning of organs, and mortality (Porceddu et al., 2012).
Liver treatment can be conducted with phytomedicines, as they are harmless, easily accessible, cost-effective, and minimally toxic with fewer side effects compared to conventional medicines (Agarwal et al., 2006; Nair and CHANDA, 2007; Doudach et al., 2022). Therefore, there is increasing interest in herbal medicines (Hussain et al., 2010). For thousands of years, medicinal plants have been used by local communities all over the world for the treatment of numerous ailments. Historically, Egypt, China, and Greece are the oldest civilizations with a rich history of herbal formulations.
Chicory (Cichorium intybus L.) is popularly used for liver disorders (Faraji et al., 2020). A poly-herbal compound is available in the market with the name Sharbat-e-Bazori (Khan et al., 2024), obtained from its seeds and roots, along with other herbs such as Foeniculum vulgare seeds and root, and seeds of Cucumis melo var. utilissimus, C. sativa, and Tribulus terrestris (Hayat et al., 2008). This compound is a famous Unani product that is used in various liver disorders such as hepatic cirrhosis, fat accumulation, hepatic steatosis, and end-stage liver disease (Faraji et al., 2020). It also helps with urinary tract infections and possesses lithotriptic, diuretic, and alkalinizing effects (Aziz et al., 2011).
Nanomedicine is an emerging field of science that is popularly used in various disciplines, including medicine, to provide cost-effective and novel therapies. Various researchers have used metal nanoparticles because of their broad application in medicine, biology, and physics (Caruthers et al., 2007). Among all metallic nanoparticles, silver nanoparticles (AgNPs) possess unique properties and are commonly used in experiments (Rai et al., 2009). AgNPs have antifungal, antibacterial, and antioxidant activities and are anti-inflammatory (Gurunathan et al., 2009; Wong et al., 2009). Silver nanoparticles have been studied for several therapeutic purposes, as they are less toxic and have increased biodegradability and bioavailability. Due to their small size, they can easily interact with biological systems.
However, scientific literature data supporting the folkloric use of the chicory formulation in liver diseases is not available, and its tentative mechanism(s) are still unknown. The present study therefore aimed to evaluate the potential in vivo hepatoprotective activity of aqueous extracts of chicory formulation against CCl4-induced hepatotoxicity.
Materials and methods
Preparation of chicory compound
The raw material used for the preparation of chicory formulation as defined in Unani Pharmacopoeia was obtained from a local market in Rawalpindi, Pakistan, identified in the taxonomy lab of the Department of Botany, Pir Mehr Ali Shah Arid Agriculture University Rawalpindi, Pakistan. To prepare the chicory compound, dead and decaying parts were removed from samples and washed in running tap water for the removal of dust. The plant parts (Table 1) were dried in the open air at room temperature and ground to a fine powder. Each ingredient of the chicory formulation was mixed in proportion, i.e., C. intybus (seed and root), F. vulgare (seed and root), and melon seeds (C. melo), according to Unani Pharmacopoeia (Shailajan et al., 2012).
Preparation of extracts
The plant extract was prepared with distilled water. For this purpose, 20 g of chicory formulation powder was added to 400 mL of distilled water in a separate flask. The flask was covered with foil paper and kept in a shaker for a day. The mixture was filtered using filter paper. After filtration, the dried extract was separated by the use of a needle and put in an Eppendorf tube. The residue powder was saved for future use (Figure 1).
Synthesis of silver nanoparticles
For the synthesis of AgNPs, 0.34 g of AgNO3 was added to 1,000 mL of water to prepare a 5 mM solution of silver nitrate. Then 100 mL of plant extract was added to 900 mL of silver nitrate solution. The plant extract was added drop-wise with constant shaking in a conical flask. The synthesis of nanoparticles was carried out with a microwave oven to speed up the process of AgNP formation. The microwave was set at 600 W, and the solution was observed every 30 s until a color change appeared. The color change demonstrated the formation of silver nanoparticles. After this, the mixture was centrifuged at 10,000 rpm for 20 min to separate the impurities. After centrifugation, precipitated AgNPs were washed twice with ethanol to remove impurities. The pellet formed inside the centrifuge tube was collected carefully and dried (Figure 1) (Roy et al., 2015; Haryani et al., 2018).
Characterization of silver nanoparticles
The formation of silver nanoparticles was marked by a change in color. A UV visible spectrophotometer (V-750 UV-Visible Spectrophotometer), Fourier transform infrared spectrophotometer (NICOLET 6700, Thermo, Waltham, MA, United States), energy dispersive X-Ray analysis (SIGMA model), and a scanning electron microscope (SEM, JSM5910 JEOL, Tokyo, Japan) were used for the characterization of synthesized silver nanoparticles.
In vivo hepato-protective activity
Male albino rats of the Wistar strain (Rattus norvegicus), with weight of 175–250 g), were obtained from the animal house of the Department of Veterinary and Animal Sciences, Arid Agriculture University Rawalpindi, and used for the experimental study. Rats were kept in clean plastic cages in the laboratory on a standard pellet diet and tap water. They were allowed to adapt to the laboratory conditions for a week before treatment.
Preparation of doses and treatments
The solution of AgNPs was made in distilled water, and different doses of plant chicory formulation and AgNPs were administered to the animals. Silymarin (20 mg/kg of body weight) was administered as standard treatment (Nirala and Bhadauria, 2008).
Experimental procedure
After 1 week of acclimatization, rats were randomly divided into nine groups, each containing six rats. The detailed treatment layout is provided in Table 2. The first group was kept as normal rats (control), the second group was the CCl4-treated group (1 mg/kg of body weight), the third group, silymarin (20 mg/kg of body weight), the fourth group (CCl4 (1 mg/kg)+chicory (1.75 mg/kg of b. wt), the fifth group (CCl4 (1 mg/kg) + chicory at a dose of 2.35 mg/kg), the sixth group (CCl4 (1 mg/kg) + chicory of 3.25 mg/kg), the seventh group (CCl4 (1 mg/kg) +AgNPs 1.75 mg/kg of b. wt.), the eighth group (CCl4 (1 mg/kg) + AgNPs 2.35 mg/kg of body weight), and the ninth group (CCl4 (1 mg/kg) + AgNPs 3.25 mg/kg of b. wt.).
All plant extracts were made in distilled water and administered through an oral route for four successive weeks. The amount of each extract was freshly prepared, weekly according to body weight changes, and plant extract was injected daily for 30 days. The CCl4-treated groups received oral administration of CCl4 (1 mL/kg per body weight) three times weekly for two consecutive weeks (Elshater et al., 2013).
The rats were anesthetized by using a cotton-wool ball soaked in diethyl ether and placed in a closed container. Rats were humanely sacrificed, and blood samples were collected to obtain the serum. Within half an hour of blood collection, the sera samples were obtained from the blood. The sera were kept in a deep freezer until analyzed.
Blood biochemistry
The serum was used for the determination of markers of liver function tests (LFTs) such as alkaline phosphatase (ALP) and alanine transaminase (ALT) (Jalili et al., 2022). Cholesterol, triglyceride, and bilirubin were measured using diagnostic kits, and an auto-analyzer (Micro Lab 200, Merck) was used for the measurement.
Histopathological examination
Small pieces of liver tissue in each group were collected in 10% neutral-buffered formalin. These tissues were processed and embedded in paraffin wax. Sections of 3–5 μm thickness were cut and stained with hematoxylin and eosin (H&E). The sections were examined microscopically for the evaluation of histopathological changes (Bancroft et al., 1994).
Statistical analysis
The results were expressed as the mean ± S.D. The data were analyzed statistically by one-way ANOVA; p < 0.05 was considered significant in all cases. A post-hoc test was performed for multiple statistical comparisons between parametric data. The software Statistix 8.1 was used for the analysis of the data.
Results
Characterization of nanoparticles
UV-visible spectroscopy
This study carried out the characterization of silver nanoparticles synthesized from chicory compounds using this technique. During the synthesis, formation of silver nanoparticles was detected through color change from light yellow to brown. The wavelength was fixed between 200 and 700 nm. Absorption band was obtained at 432 nm (Figure 2), which corresponds to the surface plasmon resonance of AgNPs. The shape and position of the characteristic absorption band depended on the size and shape of nanoparticles.
Fourier transform infrared spectroscopy
In the present work, FTIR analysis was performed to detect the presence of functional groups involved in capping and stabilization of silver nanoparticles synthesized from chicory compounds. Figure 3 shows FTIR spectra of silver nanoparticles that exhibited a number of absorption peaks at different wavelengths: 3,441 cm−1, 2,958 cm−1, 2,922 cm−1, and 2,850 cm−1; 2,362 cm−1 and 1734 cm−1; 1718 cm−1, 1,647 cm−1, and 1,560 cm−1; 1,541 cm−1, 1,458 cm−1, and 1,384 cm−1; 1,093 cm−1, 800 cm−1, 669 cm−1, and 536 cm−1; and 1261 cm−1 (Figure 3).
EDX analysis of AgNPs
Energy dispersive X-Ray analysis (EDX) was carried out to determine the elemental composition of synthesized silver nanoparticles. An EDX spectrum of AgNPs shows a strong peak at 3 KeV that correspond to the silver element along with weak Cl, S, P, O, C, and Pb peaks, which may be due to the presence of biomolecules on the surface of nanoparticles, which are involved in the reduction of silver ion to elemental silver (Figure 4).
Scanning electron microscopy
The morphology of silver nanoparticles observed by SEM analysis by the University of Peshawar showed spherical-shaped particles with an average size of about 47 ± 0.009 nm. The majority of the particles were ∼35 nm and ∼49 nm in diameter, and the minimum and maximum particle sizes observed were 25 nm and 64 nm in diameter respectively (Figure 5). Silver nanoparticles were not in direct contact with each other even in aggregated form: this shows the stabilization of AgNPs by the capping agents.
Serum biochemical parameters of liver function
This study was undertaken to investigate, in vivo, the hepto-protective activity of silver nanoparticles (AgNPs) and chicory formulation extract (CCE) by using a rat model. Firstly, hepatic damage was induced by applying CCl4, and the damage reveals an increase in the level of different liver enzymes. Administration of CCl4 expresses the damaging effects in the liver in terms of an increase in serum level of ALT, ALP, cholesterol, bilirubin, and triglycerides (Table 3).
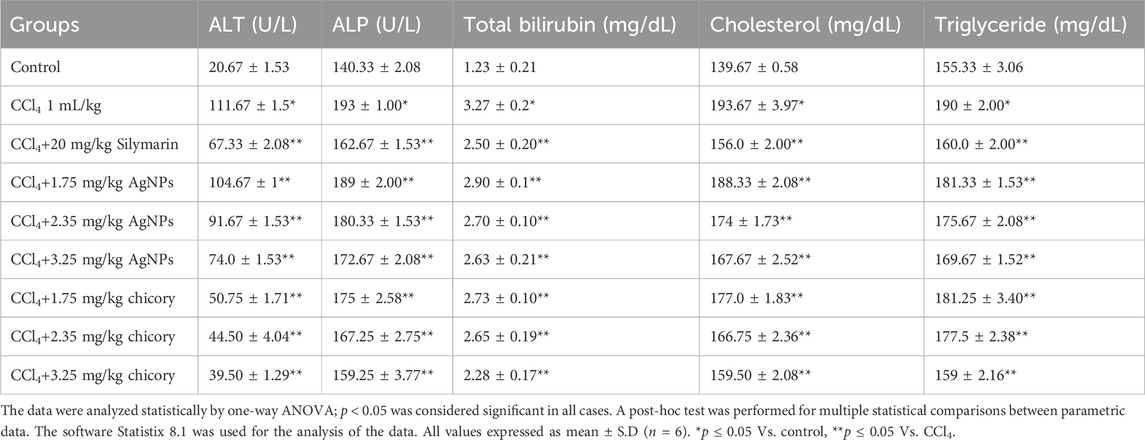
TABLE 3. Effect of AgNPs and aqueous extract of chicory on serum biochemical parameters of liver function in CCl4 intoxicated rats.
The AgNPs and chicory formulation extract possessed remarkable hepato-protective potential in a dose-dependent manner (Table 3). The AgNPs and CCE showed significant hepato-protective activity in a dose dependent manner (Table 3). An increase in the level of ALT and ALP was observed in the CCl4-treated group as compared to the normal group. Treatment with AgNPs at a dose of 3.25 mg/kg showed a significant reduction in levels of ALT and ALP as compared to the CCl4 group. On the other hand, AgNPs at the dose of 1.75 mg/kg failed to ameliorate the hepatic injury significantly.
The hepato-protective activity of AgNPs at a dose of 3.25 mg/kg was comparable with standard silymarin. As compared to the normal control group, the level of total bilirubin, cholesterol, and triglycerides was higher in CCl4-intoxicated rats. However, the treatment with silver nanoparticles (3.25 mg/kg) restored the normal level of cholesterol, bilirubin, and triglycerides. AgNPs at the doses of 1.75 mg/kg and 2.35 mg/kg reduced the level of these biochemical parameters but not significantly. The colloidal solution of silver nanoparticles showed maximum hepato-protective activity at a dose of 3.25 mg/kg in terms of a decrease in the level of liver enzymes (Table 3). Post treatment of rats with aqueous extracts of chicory compounds showed remarkable protection against CCl4-induced toxicity. Restoration of the biochemical parameters towards the normal value was observed in the group treated with 1.75 mg/kg and 2.35 mg/kg doses of chicory extract. However, the treatment of CCl4-intoxicated rats with chicory extract at a dose of 3.25 mg/kg was shown to be highly significant in terms of a decrease in the values of ALT and ALP.
The administration of CCl4 resulted in an increase in total bilirubin, cholesterol, and triglycerides, which indicated the liver dysfunction. However, treatment with a 3.25 mg/kg dose of chicory extract showed significant amelioration by reducing the level of total bilirubin, triglycerides, and cholesterol (Table 3).
Recovery percentage of serum and biochemical parameters of hepatotoxicity induced by CCL4
The recovery percentage of the induced hepatic toxicity caused by CCL4 was calculated through damaged and control values obtained from the blood analysis for ALT, ALP, total bilirubin, cholesterol, and total glyceride in line with the given doses. A detailed analysis indicated that the activity recorded was concentration dependent for both chicory formulation extracts (CCE) as well as silver nanoparticles (AgNPs); however, the activity of CCE was higher than the control in all the studied parameters (Figure 6).
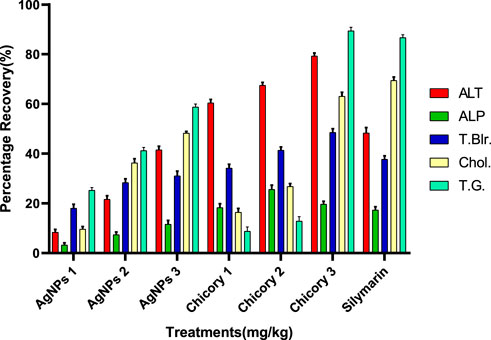
FIGURE 6. Summary of the recovery percentage of chicory formulation extract (CCE) and silver nanoparticles (AgNPs). Silymarin (standard drug), AgNPs 1 (AgNPs 1.75 mg/kg), AgNPs 2 (AgNPs 2.35 mg/kg), AgNPs 3 (AgNPs 3.25 mg/kg), Chicory 1 (1.75 mg/kg), Chicory 2 (2.35 mg/kg), Chicory 3 (3.25 mg/kg).
Recovery percentage of chicory formulation extract (CCE)
All the treatments showed significant recovery in a dose-dependent manner (Figure 6). Maximum recovery percentages for ALT, total bilirubin, and total glyceride recorded at a dose of 3.25 mg/kg were 79.30%, 48.93%, and 89.42%, respectively, higher than the drug (Figure 7). The optimum recovery percentage for ALP was recorded in a dose of 2.35 mg/kg higher than the standard, and for the cholesterol, a maximum recovery percent was noted at 3.25 mg/kg closer to the drug.
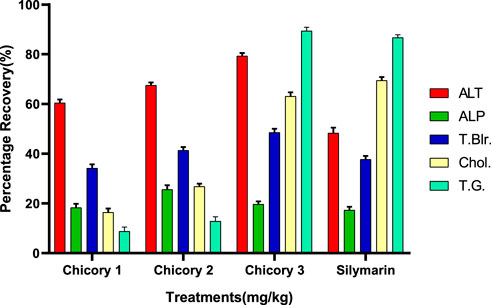
FIGURE 7. Recovery percentage of chicory formulation extract at the various concentrations. Silymarin (standard drug), Chicory 1 (1.75 mg/kg), Chicory 2 (2.35 mg/kg), Chicory 3 (3.25 mg/kg).
Recovery percentage of silver nanoparticles (AGNPs)
Compared to the hepato-protective drug (silymarin), the recovery percentile of AgNPs for each parameter was found in a dose-dependent manner (Figure 8). For ALT, the maximum recovery percentage was recorded at the dose of 3.25 mg/kg (41.39%), which was comparable to the standard (48.72%). The same trend was observed for ALP, total bilirubin, cholesterol, and total glyceride, with optimum levels at the highest dose closer to the drug (Figure 8).
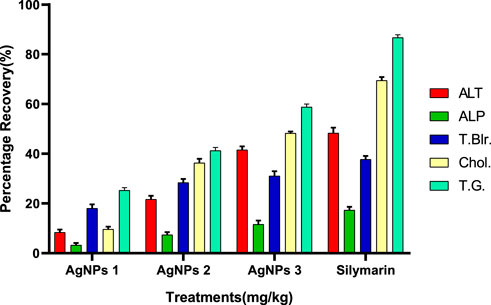
FIGURE 8. Recovery percentage of silver nanoparticles (AgNPs) at the various concentrations. Silymarin (standard drug), AgNPs 1 (AgNPs 1.75 mg/kg), AgNPs 2 (AgNPs 2.35 mg/kg), AgNPs 3 (AgNPs 3.25 mg/kg).
Histopathological examination
Histopathological studies were carried out to examine the degree of damage caused by oral administration of CCl4 and recovery through administration of different doses of AgNPs and chicory extract. Microscopic analysis of liver tissue taken from each group was done, histopathology of CCl4-intoxicated rats was compared with control, and comparison of remaining treatments was done with the CCl4 group. The hepato-protective activity of chicory extract and AgNPs was confirmed by histopathological examination (Figure 9).
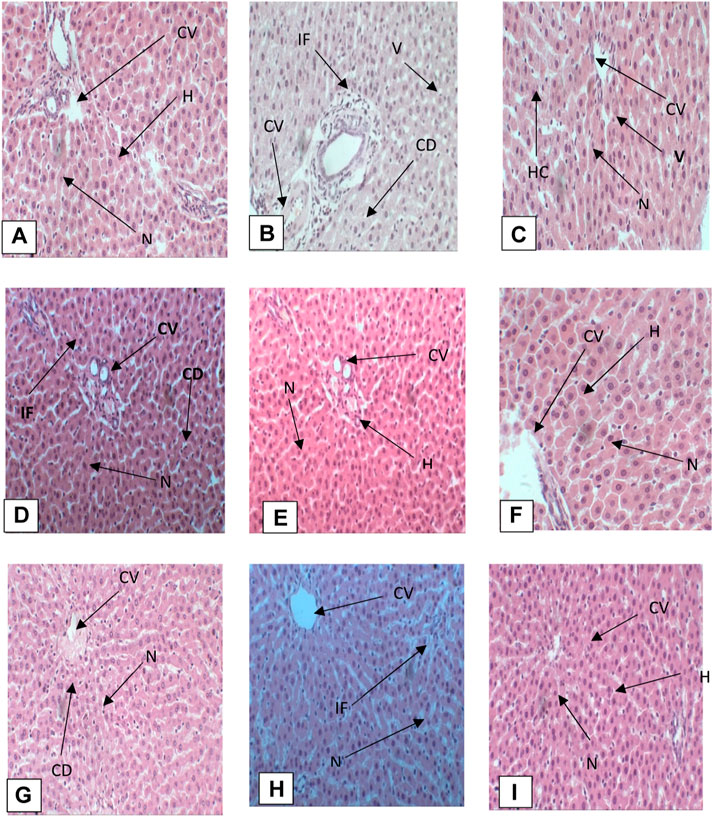
FIGURE 9. (A) Photomicrograph of liver section of control group, (B) CCl4 group, (C) silymarin group, (D) CCl4 + chicory (1.75 mg/kg) group, (E) CCl4 + chicory (2.35 mg/kg) group, (F) CCl4 + chicory (3.25 mg/kg), (G) group CCl4 + AgNPs (1.75 mg/kg) group, (H) CCl4 + AgNPs (2.35 mg/kg) group, (I) CCl4 + AgNPs (3.25 mg/kg) group. Abbreviations: (N) nucleus, (S) sinusoid, (V) vacuolization, (H) hepatocyte, (BD) bile duct, (IF) infiltration, (CV) central vein (McDonald et al., 2020), cytoplasmic degeneration (CD), (PT) portal triad.
Histopathology of control group
Histopathology of the control group showed normal architecture of hepatocytes when compared with the CCl4 group. There was a normal arrangement of hepatocytes, with intact structure, devoid of degenerative changes, well preserved cytoplasm, and prominent nucleus. There was a distinct central vein (Figure 9A).
CCl4 group
Histopathology of the CCl4 group showed vacuolization, nuclear degeneration, infiltration, distal sinusoid, loss of cellular boundaries, necrosis, ballooning degeneration, deformation of central vein, and disruption of normal hepatic cords. Kupffer cells at various points were also observed (Figure 9B).
CCl4 + silymarin 20 mg/kg
Histopathological analysis of liver sections of rats treated with silymarin showed recovery of hepatocytes with restoration of normal architecture. There was reduced vacuolization, normal central vein, prominent nucleus, absence of necrosis, and reduced sinusoid (Figure 9C).
CCl4 + chicory extract (1.75 mg/kg)
Histopathological analysis of photomicrograph of rats treated with chicory extract at the dose of 1.75 mg/kg showed mild recovery of hepatocytes toward normal architecture. There was less vacuolization and a prominent nucleus, but at some places cytoplasmic degeneration was also observed (Figure 9D).
CCl4 + chicory (2.35 mg/kg)
Histopathology of rats treated with CCl4 + chicory extract (2.35 mg/kg) showed remarkable recovery. A prominent nucleus, intact central vein, reduced vacuolization, well preserved cytoplasm, infiltration at few places, and a less dilated sinusoid were observed. Hepatic architecture was just near to normal (Figure 9E).
CCl4 + chicory extract (3.25 mg/kg)
Histopathology of live section of rats treated with chicory extract at the dose of 3.25 mg/kg of body weight revealed a marked decrease in inflammation, normal arrangement of hepatocytes, intact hepatic cord, mild inflammation, prominent nucleus, intact central vein, and well preserved cytoplasm (Figure 9F).
CCl4 + AgNPs (1.75 mg/kg)
Histopathological analysis of photomicrograph of rats treated with AgNPs at the dose of 1.75 mg/kg showed mild recovery of hepatocytes toward normal architecture. Less vacuolization and prominent nucleus were observed, but at some places cytoplasmic degeneration was also observed (Figure 9G).
CCl4 + AgNPs (2.35 mg/kg)
Histopathology of rats treated with CCl4 + AgNPs (2.35 mg/kg) showed remarkable recovery. Prominent nucleus, intact central vein, reduced vacuolization, well preserved cytoplasm, infiltration at few places, and less dilated sinusoid were observed. Hepatic architecture was just near to normal (Figure 9H).
CCl4 + AgNPs (3.25 mg/kg)
Histopathology of live section of rats treated with AgNPs at the dose of 3.25 mg/kg revealed a marked decrease in inflammation, mild degeneration, prominent nucleus, intact central vein, and well preserved cytoplasm (Figure 9I).
The histopathological investigation supported the recovery percentage by the application of chicory formulation extract and silver nanoparticles (Figure 9). The chicory formulation has traditionally been used to treat various liver disorders for centuries, and this study revealed that the chicory formulation extract exhibited a remarkable recovery percentage of liver in the liver architecture that was higher than the drug (i.e., silymarin).
Discussion
The current research work was carried out to evaluate the hepato-protective activity of AgNPs and chicory formulation extract against CCl4-induced hepatic damage in a rat model. This study involved the synthesis of AgNPs from a chicory formulation and characterization through UV-visible, SEM, EDX, and FTIR analysis. In the UV-visible spectrum, AgNPs showed an absorption peak at 432 nm that corresponds to the surface plasmon resonance of AgNPs (Figure 2). The EDX spectrum of AgNPs shows a strong peak at 3 KeV that corresponds to silver elements along with weak Cl, S, P, O, C, and Pb peaks. In SEM analysis, the majority of the particles were of size ⁓35 nm and ⁓49 nm in diameter, and the minimum and maximum particle size observed was 25 nm and 64 nm in diameter, respectively (Figure 5).
The FTIR spectrum showed absorption peaks at different wavelengths. For instance, a 3,441 cm−1 peak indicates O-H extending of alcohol and phenols. The absorption peaks at 2,958 cm−1, 2,922 cm−1, and 2,850 cm−1 correspond to alkanes C-H elongating and symmetrical stretching of the methylene group (Syafiuddin et al., 2017). Absorption peaks at 2,362 cm−1 and 1734 cm−1 were assigned to N-H and C=O, respectively. The absorption bands at 1718 cm−1, 1,647 cm−1, and 1,560 cm−1 indicate the presence of C=O, aromatic C=C, and diketones, respectively (Ali et al., 2015). Some of the peaks at 1,541 cm−1, 1,458 cm−1, and 1,384 cm−1 represent alkanes and alkyl groups, C=C stretching of aromatic compounds, and C-O, respectively. Bands at 1,093 cm−1, 800 cm−1, 669 cm−1, and 536 cm−1 indicate the C-O, N-H wagging of amines, C-H bending, and aromatic compounds (Zulfiqar et al., 2019) (Figure 3). The peak observed at 1261 cm−1 denotes the presence of C-H waging of alkyl halides.
The administration of CCl4 expresses the damaging effects in the liver in terms of an increase in serum level, ALT, ALP, cholesterol, bilirubin, and triglycerides. CCl4 reacts with cytochrome P450 and produces trichloromethyl radical that converts into proxy radical in the presence of oxygen. These free radicals attach to macromolecules and initiate lipid peroxidation that causes cellular damage and membrane leakage. It results in an increased level of liver marker enzymes such as ALP and ALT. The level of biochemical markers such as cholesterol, bilirubin, and triglycerides (Chen et al., 2012; Syed et al., 2014). The results of current study demonstrated that AgNPs and chicory formulation extract possessed remarkable hepato-protective potential (Table 3). The hepato-protective activity of a plant depends upon the ability to ameliorate the toxic effect or maintain normal physiological functions (Ouassou et al., 2021).
The present study revealed that treatment with chicory formulation extract and AgNPs restored the normal functioning of the liver and repaired cellular damage, evidenced by a decrease in the level of liver biochemical markers towards the normal values. However, the restorative activity of chicory formulation extract and AgNPs was observed in dose dependent manner (Table 3). These findings are in line with other studies in which AgNPs were used to ameliorate the oxidative damage caused by CCl4 administration (Zhang et al., 2019).
A colloidal solution of silver nanoparticles showed maximum hepato-protective activity at the dose of 3.25 mg/kg in term of a decrease in the level of liver enzymes (Table 3). These results correlate with studies where treatment with nanoparticles was found to be more effective in restoring the level of different liver enzymes in a dose dependent manner. However, more hepato-protective activity was observed at the medium and highest doses (Kumar et al., 2011). Furthermore, AgNPs at the dose of 1.75 mg/kg failed to ameliorate the hepatic injury significantly. The results of this study are correspond to previous studies carried out on silver nanoparticles by using a leaf extract of Rhizophora apiculata. Silver nanoparticles were found more effective in ameliorating hepatic injury at the highest dose (Zhang et al., 2019). Increased levels of ALT, ALP, bilirubin, cholesterol, and triglycerides in the serum suggest a compromised integrity of hepatocellular membranes and cellular leakage. The use of AgNPs showed promise in restoring these enzyme levels to normal, indicating their potential to protect organs from damage. The stabilization of ALT, ALP, bilirubin, cholesterol, and triglycerides through AgNP treatment signified an enhancement in the functional maintenance of hepatocytes. Notably, AgNP treatment led to a reduction in bilirubin levels, moving them closer to the normal range.
The recovery percentage of the induced hepatic toxicity caused by CCL4 was calculated through damaged and control values obtained from the blood analysis for ALT, ALP, total bilirubin, cholesterol, and total glyceride in line with the given doses. The detailed analysis indicated that the activity recorded was concentration dependent for both CCE as well as AgNP; however, the activity of CCE was higher than the control in all the studied parameters due to the presence of more hepatoprotective agents (Figure 6). Maximum recovery percentages for ALT, total bilirubin, and total glyceride recorded at the dose of 3.25 mg/kg were 79.30%, 48.93%, and 89.42%, respectively, higher than the drug (Figure 7). The compounds present in CCE were actually contributed by four different plants, and they exerted their therapeutic potential through their free radicle scavenging activity. The optimum recovery percentage for ALP was recorded at the dose of 2.35 mg/kg higher than the standard, and for the cholesterol, the maximum recovery percentage was noted at 3.25 mg/kg closer to the drug (Figure 7). Detailed analysis of these recovery percentages showed that chicory formulation possesses more hepato-protective activity and was even higher than standard drug. This might be due to the herbal ingredients of chicory formulation, which possess an active constitute responsible for its hepato-protective activity. These results are also supported by another study in which a polyherbal formulation (Aab-e-Murawaqain) showed a significant hepato-protective effect (Amir et al., 2022).
Cichorium intybus is a major component of chicory compound. The hepato-protective activity of its various parts has been reported. The ethanolic extract of roots is reported to contain sesquiterpene lactones, insulin, chicoric acid, pectin, choline, and reducing sugar (Mathur and Mathur, 2016). The hepato-protective activity of methanolic fractions and phenolic compound of seeds of C. intybus has been reported in albino rats of the Wistar strain after the induction of hepato-toxicity by CCl4 administration. Biochemical parameters such as γ-glutamyl transferase (GGT), alanine aminotransferase (ALT), aspartate aminotransferase (AST), and alkaline phosphatase (ALP) were analyzed to evaluate hepato-protective action (Reitman and Frankel, 1957; Elgengaihi et al., 2016).
Previous studies showed the hepato-protective activity of the essential oil of F. vulgare. Its seeds are reported to possess trans-anethole, fenchone, methylchavicol, limonene, camphene, ß-pinene, ß-myrcene, phellandrene, 3-carene, camphor, and cisanethole (Simándi et al., 1999; Heghes et al., 2019). Among these, ß-myrcene and limonene have been shown to affect liver functions in CCl4-induced albino rats, determined from serum biochemical analysis of ALT, AST, ALP, and bilirubin concentration (Reicks and Crankshaw, 1993; Joshi and Soulimani, 2020).
Cucumis melo has been reported to possess hepato-protective activity in CCl4-intoxicated rats, where it increases gluconeogenesis (Milind and Kulwant, 2011). Different phytochemicals from the fresh fruit of Cucumis sativus were reported to possess hepato-protective potential against cumene hydroperoxide (CHP)-induced oxidative stress. The outcomes of this experiment showed that aqueous extract of C. sativus L. acts as a hepatoprotective and antioxidant agent against induced hepatotoxicity, which suggests that antioxidants and radical scavenging components of C. sativus L. fruit extract can easily cross the cell membrane and cope with the intracellular ROS formation (Heidari et al., 2012; Gopalakrishnan et al., 2016). Various previous studies reported that ingredients of chicory formulation individually possess hepato-protective activity. Therefore, the combined synergetic effect of all ingredients may have profound effect in normalizing liver functions.
The administration of CCl4 led to the production of reactive free radicals that bind with macromolecules and induce lipid peroxidation, and result in the disruption of normal liver architecture (Singh et al., 2014). The livers of normal control animals displayed a distinct central vein, well-preserved cytoplasm, and noticeable nucleus and nucleolus (Figure 9). In contrast, liver sections from animals treated with CCl4 exhibited severely toxic cells, including necrotic liver cells, inflammatory cell accumulation, and widespread inflammation throughout the liver parenchyma, with infiltration and necrosis. Having hepatocytes with a preserved cytoplasm suggests that chicory extracts were more effective than AgNPs in mitigating CCl4 toxicity, and silymarin also demonstrated protective effects against CCl4-induced hepatic alterations. The chicory formulation has been traditionally used to treat various liver disorders for centuries, and this study has revealed that the chicory formulation extract exhibited the remarkable recovery percentage of liver in the liver architecture, which was higher than the drug (i.e., silymarin). Nevertheless, the absence of necrosis and the presence of normal hepatic cells in rats treated with chicory extract across various groups indicated significant signals of protection. The histopathological investigation supported the recovery percentage by the application of chicory formulation extract and silver nanoparticles (Figure 9), while treatment with AgNPs also repaired the degenerative changes and restored the form of the liver. Rats treated with high doses of AgNPs showed histology near to the control group. All these results are in agreement with a previous study carried out by Reshi et al. (2017).
This study successfully synthesized and characterized silver nanoparticles from chicory formulation extract. Both the chicory formulation extract and AgNPs were tested for hepato-protective effective in a rat model with CCl4-induced damage. The recovery of CCl4-induced liver damage through the dose dependent application of chicory formulation extract as well as AgNPs signifies the hepato-protective effects. The findings of this study reveal that chicory formulation extract possessed more hepato-protective potential as compared to AgNPs by regulating the biochemical and histo-pathological parameters. This study also validated the traditional use of chicory formulation in traditional systems of medicine to treat liver disorders. However, there is a need for further studies with molecular characterization to find the lead compound with hepato-protective effects from both chicory extract and AgNPs. In addition, there is need to explore the mechanism of action of the compound responsible for this activity.
Study limitations
The findings of the study may be specific to the chosen experimental conditions, and the transferability of the results to broader populations or different settings may be limited.
The study may not have explored a wide range of doses or exposure durations.
Data availability statement
The original contributions presented in the study are included in the article/Supplementary material, further inquiries can be directed to the corresponding authors.
Ethics statement
The animal studies were approved by the Animal house of Department of Veterinary and Animal Sciences, Arid Agriculture University Rawalpindi. The studies were conducted in accordance with the local legislation and institutional requirements. Written informed consent was obtained from the owners for the participation of their animals in this study.
Author contributions
AS: Data curation, Investigation, Methodology, Writing–original draft. RQ: Formal Analysis, Writing–review and editing, Conceptualization, Data curation, Investigation, Project administration, Supervision, Validation. NR: Data curation, Formal Analysis, Writing–review and editing, Software, Visualization. IK: Data curation, Formal Analysis, Visualization, Writing–review and editing. MZ: Data curation, Formal Analysis, Software, Writing–review and editing. SR: Data curation, Formal Analysis, Software, Writing–review and editing. AmA: Formal Analysis, Writing–review and editing. AjA: Data curation, Funding acquisition, Resources, Validation, Visualization, Writing–review and editing. PK: Data curation, Formal Analysis, Funding acquisition, Software, Validation, Visualization, Writing–review and editing.
Funding
The author(s) declare that no financial support was received for the research, authorship, and/or publication of this article.
Acknowledgments
The authors would like to extend their sincere appreciation to the Researchers Supporting Project Number (RSP 2024R350), King Saud University, Riyadh, Saudi Arabia.staff of Department of Botany, and Department of veterinary pathology, PMAS Arid Agriculture University Rawalpindi, and for their always stimulating support to these studies.
Conflict of interest
The authors declare that the research was conducted in the absence of any commercial or financial relationships that could be construed as a potential conflict of interest.
Publisher’s note
All claims expressed in this article are solely those of the authors and do not necessarily represent those of their affiliated organizations, or those of the publisher, the editors and the reviewers. Any product that may be evaluated in this article, or claim that may be made by its manufacturer, is not guaranteed or endorsed by the publisher.
Abbreviations
AgNPs, Silver nanoparticles; ALT, Alanine transaminase; Chol, Cholesterol; T.G, Triglycerides; FTIR, Fourier transform infrared spectroscopy; ALP, Alkaline phosphatase; CCl4, Carbon tetrachloride; Blr, Bilirubin; LFTs, Liver function tests.
References
Agarwal, M., Srivastava, V., Saxena, K., and Kumar, A. (2006). Hepatoprotective activity of Beta vulgaris against CCl4-induced hepatic injury in rats. Fitoterapia 77, 91–93. doi:10.1016/j.fitote.2005.11.004
Ali, K., Ahmed, B., Dwivedi, S., Saquib, Q., Al-Khedhairy, A. A., and Musarrat, J. (2015). Microwave accelerated green synthesis of stable silver nanoparticles with Eucalyptus globulus leaf extract and their antibacterial and antibiofilm activity on clinical isolates. PloS one 10, e0131178. doi:10.1371/journal.pone.0131178
Amir, M., Ahmad, W., Sarafroz, M., Ahmad, A., Ali, A., Ansari, M. A., et al. (2022). Hepatoprotective effect of a polyherbal formulation (Aab-e-Murawaqain) against CCl4 induced liver toxicity in Wistar albino rat model by suppressing proinflammatory cytokines. South Afr. J. Bot. 151, 75–81. doi:10.1016/j.sajb.2021.11.020
Aziz, I., Ismail, A., Zulkifle, M., and Ansari, A. H. (2011). Effects of Sharbat buzoori motadil on citrate excretion, 10, 498–501.
Bancroft, J. D., Cook, H. C., and Stirling, R. W., 1994. Manual of histological techniques and their diagnostic application, Manual of histological techniques and their diagnostic application, pp. 457–457.
Bharathi, M. P., Alagarsamy, V., Prasad, S. S., Krishna, P. V. M., and Vali, C. S. (2023). Hepatoprotective activity of Atylosia rugosa against Carbontetrachloride and Paracetamol induced hepatotoxicity in rats. Curr. Trends Biotechnol. Pharm. 17, 609–620. doi:10.5530/ctbp.2023.1.2
Caruthers, S. D., Wickline, S. A., and Lanza, G. M. (2007). Nanotechnological applications in medicine. Curr. Opin. Biotechnol. 18, 26–30. doi:10.1016/j.copbio.2007.01.006
Chen, Y.-P., Dai, Z.-H., Liu, P.-C., Chuu, J.-J., Lee, K.-Y., Lee, S.-L., et al. (2012). Effects of nanogold on the alleviation of carbon tetrachloride-induced hepatic injury in rats. Chin. J. Physiol. 55, 331–336. doi:10.4077/CJP.2012.BAA064
Doudach, L., Omari, N. E., Mrabti, H. N., Touhami, F., Mrabti, N., Benrahou, K., et al. (2022). Hepatoprotective effect of corrigiola telephiifolia pourr. Root methanolic extracts against ccl4-induced hepatic damage in mice. Biointerface Res. Appl. Chem. 12, 2489–2502. doi:10.33263/BRIAC122.24892502
Elgengaihi, S., Mossa, A.-T. H., Refaie, A. A., and Aboubaker, D. (2016). Hepatoprotective efficacy of Cichorium intybus L. extract against carbon tetrachloride-induced liver damage in rats. J. Diet. Suppl. 13, 570–584. doi:10.3109/19390211.2016.1144230
Elshater, A.-E. A., Salman, M. M. A., and Mohamed, S. A. (2013). The hepato-ameliorating effect of Solanum nigrum against CCL4 induced liver toxicity in Albino rats. Egypt. Acad. J. Biol. Sci. C, physiology Mol. Biol. 5, 59–66. doi:10.21608/eajbsc.2013.16111
Faraji, S., Daneghian, S., and Alizadeh, M. (2020). Effects of chicory (Cichorium intybus L.) on nonalcoholic fatty liver disease. Traditional Med. Res. 5, 476–486. doi:10.53388/tmr20200603192
Gopalakrishnan, S. B., Kalaiarasi, T., and Gnanendra, S. (2016). Evaluation of phytochemical constituents of the fruits of cucumis sativus linn. for their hepatoprotective activity by molecular docking studies. J. Food Technol. Res. 3, 12–22. doi:10.18488/journal.58/2016.3.1/58.1.12.22
Gurunathan, S., Kalishwaralal, K., Vaidyanathan, R., Venkataraman, D., Pandian, S. R. K., Muniyandi, J., et al. (2009). Biosynthesis, purification and characterization of silver nanoparticles using Escherichia coli. Colloids Surfaces B Biointerfaces 74, 328–335. doi:10.1016/j.colsurfb.2009.07.048
Haryani, Y., Nabella, I., Yuharmen, G. F. K., and Kartika, G. F. (2018). Synthesis of silver nanoparticles from cucumis melo L. And assessment of its antimicrobial properties. Synthesis, 3, 46–50. doi:10.15416/pcpr.v3i2.18109
Hayat, M. Q., Khan, M. A., Ahmad, M., Shaheen, N., Yasmin, G., and Akhter, S. (2008). Ethnotaxonomical approach in the identification of useful medicinal flora of tehsil Pindigheb (District Attock) Pakistan.
Heghes, S. C., Vostinaru, O., Rus, L. M., Mogosan, C., Iuga, C. A., and Filip, L. (2019). Antispasmodic effect of essential oils and their constituents: a review. Molecules 24, 1675. doi:10.3390/molecules24091675
Heidari, H., Kamalinejad, M., and Eskandari, M. (2012). Hepatoprotective activity of Cucumis sativus against cumene hydroperoxide induced-oxidative stress. Res. Pharm. Sci. 7, 936.
Hussain, J., Ullah, R., Rehman, N., Khan, A. L., Muhammad, Z., Khan, F. U., et al. (2010). Endogenous transitional metal and proximate analysis of selected medicinal plants from Pakistan. J. Med. Plants Res. 4, 267–270.
Jalili, V., Poorahmadi, Z., Hasanpour Ardekanizadeh, N., Gholamalizadeh, M., Ajami, M., Houshiarrad, A., et al. (2022). The association between obesity with serum levels of liver enzymes, alanine aminotransferase, aspartate aminotransferase, alkaline phosphatase and gamma-glutamyl transferase in adult women. Endocrinol. Diabetes and Metabolism 5, e367. doi:10.1002/edm2.367
Joshi, R. K., and Soulimani, R. (2020). Ethno-medicinal and phytochemical potential of Carum carvi Linn. and Cuminum cyminum: a review. Int. J. Pharmacogn. Life Sci. 1, 33–37. doi:10.33545/27072827.2020.v1.i1a.7
Khan, M. U., Basist, P., Zahiruddin, S., Penumallu, N. R., and Ahmad, S. (2024). Ameliorative effect of traditional polyherbal formulation on TNF-α, IL-1β and Caspase-3 expression in kidneys of wistar rats against sodium fluoride induced oxidative stress. J. Ethnopharmacol. 318, 116900. doi:10.1016/j.jep.2023.116900
Kumar, C. H., Ramesh, A., Kumar, J. S., and Ishaq, B. M. (2011). A review on hepatoprotective activity of medicinal plants. Int. J. Pharm. Sci. Res. 2, 501.
Kumar, M., Ranjan, R., Kumar, A., Sinha, M. P., Srivastava, R., Subarna, S., et al. (2021). Hepatoprotective activity of Silver Nanoparticles synthesized using aqueous leaf extract of Punica granatum against induced hepatotoxicity in rats. Nova Biol. Reper 7, 381–389. doi:10.52547/nbr.7.4.381
Latief, U., Kaur, M., Dar, S. H., Thakur, S., Per, T. S., Tung, G. K., et al. (2023). Preparation and characterisation of liposomes of bergenia ciliata extract and evaluation of their hepatoprotective activity. J. Pharm. Sci. 112, 328–335. doi:10.1016/j.xphs.2022.07.013
Mathur, N., and Mathur, M. (2016). Phyto-pharmacology of Cichorium intybus as hepatoprotective agent. Int. J. Pharm. Sci. Rev. Res. 39, 116–124.
McDonald, M. D., Lewis, K. L., and Ritchie, G. L. (2020). Short term cotton lint yield improvement with cover crop and no-tillage implementation. Agronomy 10, 994. doi:10.3390/agronomy10070994
Nair, R., and Chanda, S. (2007). Antibacterial activities of some medicinal plants of the western region of India. Turkish J. Biol. 31, 231–236.
Nirala, S. K., and Bhadauria, M. (2008). Propolis reverses acetaminophen induced acute hepatorenal alterations: a biochemical and histopathological approach. Archives Pharmacal Res. 31, 451–461. doi:10.1007/s12272-001-1178-5
Ostapowicz, G., Fontana, R. J., Schiødt, F. V., Larson, A., Davern, T. J., Han, S. H., et al. (2002). Results of a prospective study of acute liver failure at 17 tertiary care centers in the United States. Ann. Intern. Med. 137, 947–954. doi:10.7326/0003-4819-137-12-200212170-00007
Ouassou, H., Bouhrim, M., Daoudi, N. E., Mekhfi, H., Ziyyat, A., Legssyer, A., et al. (2021). Evaluation of hepatoprotective activity of Caralluma europaea stem extract against CCl4-induced hepatic damage in wistar rats. Adv. Pharmacol. Pharm. Sci. 2021, 8883040. doi:10.1155/2021/8883040
Pandit, A., Sachdeva, T., and Bafna, P. (2012). Drug-induced hepatotoxicity: a review. J. Appl. Pharm. Sci. 2, 233–243. doi:10.7324/japs.2012.2541
Porceddu, M., Buron, N., Roussel, C., Labbe, G., Fromenty, B., and Borgne-Sanchez, A. (2012). Prediction of liver injury induced by chemicals in human with a multiparametric assay on isolated mouse liver mitochondria. Toxicol. Sci. 129, 332–345. doi:10.1093/toxsci/kfs197
Rai, M., Yadav, A., and Gade, A. (2009). Silver nanoparticles as a new generation of antimicrobials. Biotechnol. Adv. 27, 76–83. doi:10.1016/j.biotechadv.2008.09.002
Reicks, M., and Crankshaw, D. (1993). Effects of D-limonene on hepatic microsomal monooxygenase activity and paracetamol-induced glutathione depletion in mouse. Xenobiotica 23, 809–819. doi:10.3109/00498259309166786
Reitman, S., and Frankel, S. (1957). A colorimetric method for the determination of serum glutamic oxalacetic and glutamic pyruvic transaminases. Am. J. Clin. pathology 28, 56–63. doi:10.1093/ajcp/28.1.56
Reshi, M. S., Uthra, C., Yadav, D., Sharma, S., Singh, A., Sharma, A., et al. (2017). Silver nanoparticles protect acetaminophen induced acute hepatotoxicity: a biochemical and histopathological approach. Regul. Toxicol. Pharmacol. 90, 36–41. doi:10.1016/j.yrtph.2017.08.011
Roy, K., Sarkar, C., and Ghosh, C. (2015). Single-step novel biosynthesis of silver nanoparticles using cucumis sativus fruit extract and study of its photcatalytic and antibacterial activity. Dig. J. Nanomater. Biostructures (DJNB) 10, 107–115.
Sanjiv, C. (2002). The liver book: a comprehensive guide to diagnosis, treatment and recovery. Atria Jimcafe Co. 415.
Shailajan, S., Sayed, N., and Tiwari, B. (2012). A bioanalytical and multidisciplinary approach for quality assessment of sufoof-e-ziabetes–dulabi: a popular traditional unani antidiabetic medicine. Int. J. Pharm. Sci. Rev. Res. 13, 53–58.
Simándi, B., Deák, A., Rónyai, E., Yanxiang, G., Veress, T., Lemberkovics, É., et al. (1999). Supercritical carbon dioxide extraction and fractionation of fennel oil. J. Agric. food Chem. 47, 1635–1640. doi:10.1021/jf9809535
Singh, D., Arya, P. V., Sharma, A., Aggarwal, V. P., Dobhal, M. P., and Gupta, R. S. (2014). Antioxidant potential of plumieride against CCl₄-Induced peroxidative damage in rats. Antioxidants 3, 798–813. doi:10.3390/antiox3040798
Smuckler, E. (1975). Alcoholic drink, its production and effects. United States: Federation proceedings 34, 2038–2044.
Syafiuddin, A., Hadibarata, T., Beng Hong Kueh, A., and Razman Salim, M. (2017). Novel weed-extracted silver nanoparticles and their antibacterial appraisal against a rare bacterium from river and sewage treatment plan. Nanomaterials 8, 9. doi:10.3390/nano8010009
Syed, S. N., Rizvi, W., Kumar, A., Khan, A. A., Moin, S., and Ahsan, A. (2014). In vitro antioxidant and in vivo hepatoprotective activity of leave extract of Raphanus sativus in rats using CCL4 model. Afr. J. Traditional, Complementary Altern. Med. 11, 102–106. doi:10.4314/ajtcam.v11i3.15
Upadhyay, B., Singh, K., and Kumar, A. (2010). Pharmacognostical and antibacterial studies of different extracts of Euphorbia hirta L. J. phytology 2, 50–60.
Vairetti, M., Di Pasqua, L. G., Cagna, M., Richelmi, P., Ferrigno, A., and Berardo, C. (2021). Changes in glutathione content in liver diseases: an update. Antioxidants 10, 364. doi:10.3390/antiox10030364
Wong, K. K., Cheung, S. O., Huang, L., Niu, J., Tao, C., Ho, C. M., et al. (2009). Further evidence of the anti-inflammatory effects of silver nanoparticles. ChemMedChem Chem. enabling drug Discov. 4, 1129–1135. doi:10.1002/cmdc.200900049
Zhang, H., Jacob, J. A., Jiang, Z., Xu, S., Sun, K., Zhong, Z., et al. (2019). Hepatoprotective effect of silver nanoparticles synthesized using aqueous leaf extract of Rhizophora apiculata. Int. J. Nanomedicine 14, 3517–3524. doi:10.2147/IJN.S198895
Keywords: silver nanoparticles, hepato-protective activity, liver diseases, chicory compound, histopathology
Citation: Siddiqa A, Qureshi R, Raja NI, Khan IA, Ahmad MZ, Rafique S, Ali A, Ahmad A and Kaushik P (2024) Liver-boosting potential: chicory compound-mediated silver nanoparticles for hepatoprotection—biochemical and histopathological insights. Front. Pharmacol. 15:1325359. doi: 10.3389/fphar.2024.1325359
Received: 21 October 2023; Accepted: 23 January 2024;
Published: 21 February 2024.
Edited by:
Ahmed Esmat Abdel Moneim, Helwan University, EgyptReviewed by:
Basma Hamed Marghani, Department of Physiology Faculty of Veterinary Medicine Mansoura University Egypt, EgyptSekena Hassanien Abdel-Aziem, National Research Centre, Egypt
Nancy Safwat Younis, King Faisal University, Saudi Arabia
Copyright © 2024 Siddiqa, Qureshi, Raja, Khan, Ahmad, Rafique, Ali, Ahmad and Kaushik. This is an open-access article distributed under the terms of the Creative Commons Attribution License (CC BY). The use, distribution or reproduction in other forums is permitted, provided the original author(s) and the copyright owner(s) are credited and that the original publication in this journal is cited, in accordance with accepted academic practice. No use, distribution or reproduction is permitted which does not comply with these terms.
*Correspondence: Rahmatullah Qureshi, cmFobWF0dWxsYWhxQHVhYXIuZWR1LnBr; Amir Ali, YW1pcmtoYW4zMTUzMEBnbWFpbC5jb20=