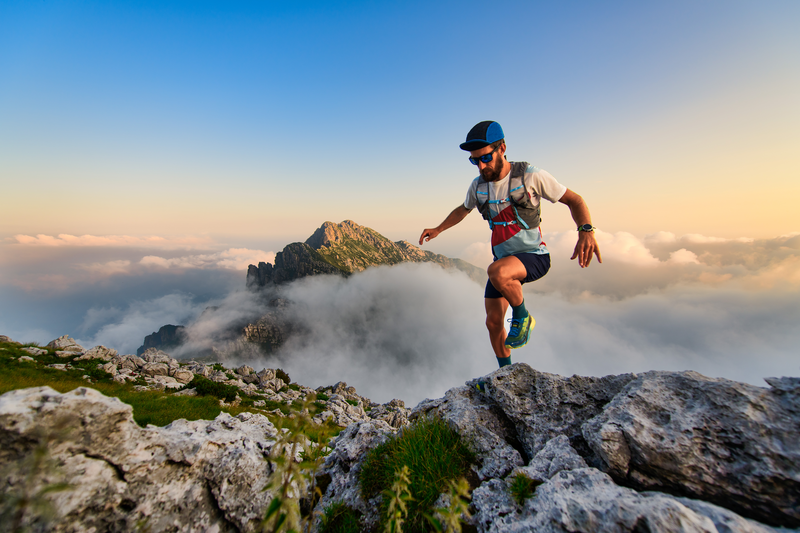
95% of researchers rate our articles as excellent or good
Learn more about the work of our research integrity team to safeguard the quality of each article we publish.
Find out more
REVIEW article
Front. Pharmacol. , 07 March 2024
Sec. Ethnopharmacology
Volume 15 - 2024 | https://doi.org/10.3389/fphar.2024.1294755
Hyperuricemia (HUA), a severe metabolic disease derived from purine metabolism disorder, will lead to abnormally increased serum uric acid (SUA) levels in the body. Studies have shown that HUA is highly related to gout, hypertension, diabetes, coronary heart disease, chronic kidney diseases, and so on. Traditional Chinese medicine (TCM) shows excellent results in treating HUA because of its unique advantages of multi-metabolites and multi-targets. This article reports on the use of TCM components for uric acid (UA)-lowering activity with excellent efficacy and low side effects based on established HUA models. This work summarizes the advantages and limitations of various HUA disease models for efficacy evaluation. Applications of TCM in HUA treatment have also been discussed in detail. This paper reveals recent research progress on HUA in constructing evaluation models and systematic TCM interventions. It will provide a scientific reference for establishing the HUA model and suggest future TCM-related HUA studies.
Hyperuricemia (HUA) is a metabolic disease with a high morbidity worldwide that is recognized as the “fourth leading cause of hypertension, hyperglycemia, and hyperlipidemia” (Zhang, 2015). HUA is diagnosed if the serum uric acid (SUA) concentration is higher than 417 μmol/L for men or 357 μmol/L for women (Jiang et al., 2020). Studies have shown that other diseases, such as gout, hypertension, diabetes, atherosclerosis, cardiovascular and cerebrovascular diseases, and kidney diseases, are associated with a high risk of long-term high uric acid (UA) status in the body (Figure 1) (Han et al., 2017; Kimura et al., 2021; Nishizawa et al., 2022; Kuwabara et al., 2023). Therefore, the study and treatment of HUA are of great practical significance for human health.
HUA is mainly treated by improving lifestyle or pharmacotherapy to reduce SUA levels (Alvarez-Lario and Alonso-Valdivielso, 2014). In terms of improving lifestyle, the intake of low-purine foods, stopping smoking and drinking alcohol, exercising, and controlling weight have proven to be effective measures for attenuating HUA (Morgan and Singh, 2021). In pharmacotherapy, UA-lowering drugs are classified into three categories: drugs that inhibit UA production (allopurinol, febuxostat, etc.), drugs that promote UA excretion (probenecid, benzbromarone, etc.), and drugs that promote UA decomposition (pegloticase, rasburicase, etc.) (Pacher et al., 2006; Terkeltaub, 2006; Cortes et al., 2010). Although these drugs can somewhat treat HUA, they have unavoidable side effects and clinical limitations (Saag et al., 2022). Therefore, developing safer and more effective therapeutic strategies for HUA is necessary (Flores et al., 2023).
Traditional Chinese medicine (TCM) refers to traditional medicine developed in China for thousands of years, containing natural or processed plants, animals, and minerals from nature. It is used for clinical therapy with the guidance of TCM theory and modern science (Ji et al., 2021; Cao et al., 2023). Compared to Eastern medicine, TCM has high safety characteristics, multiple metabolites, and multiple targets, indicating that it is suitable for HUA- and UA-level regulation. This review summarizes the pathogenesis, evaluation model of drug efficacy, and intervention treatment progress for HUA using TCM.
HUA results from an imbalance of SUA in the human body, which results from excessive production or impaired renal excretion of UA during purine metabolism. UA is the end product of purine metabolism in the human body and is mainly synthesized in the liver, intestines, and other tissues (Lima et al., 2015). Two typical biosynthesis pathways of UA from purines are shown in Figure 2 (Mandal and Mount, 2015): 1) adenosine monophosphate (AMP) is degraded into adenosine and phosphates with the catalysis of 5′-nucleotidase (5′-NT). Subsequently, adenosine is deaminated by adenosine deaminase (ADA) to form inosine, which is subsequently transformed into hypoxanthine by purine nucleoside phosphorylase (PNPase). Finally, hypoxanthine is transformed into xanthine and then into UA by xanthine oxidase (XO). 2) Guanosine monophosphate (GMP) is dephosphorylated to form guanosine by 5′-NT, which is then transformed into guanine via PNPas catalysis. Guanine is converted into xanthine by guanine deaminase (GDA), which is further converted into UA via XO catalysis. UA exists in the body in an anionic state and cannot autonomously cross the cell membranes. In most mammals, including rats and mice, UA can be enzymatically converted to allantoin, which is more soluble and easily excreted in the urine (shown in the empty box in Figure 2). Approximately two-thirds of UA is excreted from the body through the kidneys, while one-third is excreted through the gut (Fathallah-Shaykh and Cramer, 2014). UA mainly depends on UA transporters to complete its reabsorption and excretion, which include influx and efflux transporters to maintain UA homeostasis, such as SLC and ABC transporters expressed in human renal proximal tubule cells (Figure 3) (Ichida et al., 2012). The abnormal expression of UA transporters results in the accumulation of UA in the body and the occurrence of HUA.
FIGURE 3. Schematic diagram of the UA excretion process in the human body through the intestines and kidneys.
The symptoms of HUA are assigned to “gout ,” “lijie,” “rebi,” and so on in the TCM field. Skilled TCM practitioners believe that HUA is caused by inborn weakness and kidney qi deficiency, six exogenous pathogenic factors, internal damage because of seven emotions, emotional frustration, dietary intemperance, etc. (Feng et al., 2022). The pathogenesis of HUA includes kidney qi deficiency, zang–fu disorders, visceral dysfunction, phlegm, and blood stasis (Jianmin et al., 2022). This qi cannot push the flow of body fluids and blood, resulting in endogenous congestion because of the deficiency of the liver and kidneys. HUA results from a deficiency in origin and excess superficiality and is diagnosed according to the differentiation of zang and fu, mainly in the spleen and kidneys. In short, HUA is closely related to the five zang–fu organs, especially the spleen, liver, and kidneys.
An important function of the spleen is to transport and transform nutrients into the human body (Yang et al., 2020; Huang et al., 2022; Jin et al., 2022; Chen et al., 2023). If the spleen runs unhealthily, dampness and turbidity are endogenously generated, and dampness, heat and phlegm turbidity accumulate, leading to excessive UA production in the blood (Wang et al., 2023). The liver controls the conveyance and dispersion. If liver function is disrupted, the kidneys become dysfunctional. Dampness-heat and phlegm turbidity decrease, reducing UA excretion in the blood (Li and Bao, 2020). Both excessive UA production and reduced UA excretion can form HUA. According to TCM theory, HUA results from dampness, phlegm, and blood stasis because of an intemperate diet or liver–kidney qi deficiency. Clinically, the symptoms of HUA can be classified into a phlegm-dampness block, yang qi deficiency, yin blood deficiency, dampness-heat accumulation, qi stagnation and blood stasis, cold condensation and qi junction, and so on (Zhang et al., 2022a), which can be regulated and treated by tonifying the kidney and spleen, tonifying the liver and kidney, eliminating dampness and turbidity, clearing dampness and heat, promoting blood circulation, removing blood stasis, and so on.
The HUA cell model has the advantages of simple operation, small dosage, and high screening efficiency. Establishing an efficient and stable cell model for studying HUA will be conducive to screening UA-lowering drugs and the corresponding mechanics studies. UA is usually produced in the liver and excreted through the kidneys and intestines. Thus, kidney, liver, and intestinal cell lines are primarily used to construct HUA cell models (Table 1). The most commonly used cell lines include human proximal convoluted human kidney-2 (HK-2 cells), human tubular epithelial cells (HKC), human embryonic kidney cells 293 (HEK293 cells), human normal liver cells (LO2 cells), human liver cancer cells (HepG2 cells), and human colorectal adenocarcinoma cells (Caco-2 cells) (Wu et al., 2021a).
The HUA research model of HUA is established by directly adding UA or urate to the culture medium when culturing the cells. For example, Zang et al. (2011) added 1,500 μmol/L UA into the culture medium to mimic the excessive UA intake of renal proximal tubular epithelial cells (RTECs). The result showed that the UA absorption rate reached its maximum at 30 min and plateaued after 60 min. The cells were then maintained in a high-UA environment to establish the HUA research model. This research also proved that the absorption process of UA is closely related to URAT1, which could be used for pharmacodynamic screening and molecular mechanism research. Similarly, Yan et al. (2019) incubated osteoblasts and vascular smooth muscle cells (VSMCs) with 5 mg/dL urate crystals for 48 h to establish an HUA model. Therefore, UA can be a common and direct inducer for developing HUA cell models.
Increasing adenosine concentration promotes UA synthesis (Figure 2). Liu et al. (2017) used LO2 cells as target cells and adenine and adenosine as inducers to construct an in vitro HUA model. The cells were treated with 2.5 mmol/L adenosine and 0.005 U/mg XO for the final production of UA. Hou et al. (2019) used HK-2 cells and adenosine to construct an HUA research model using XO. XO, an important enzyme involved in UA production in human cells, can be used as a drug target in UA-lowering strategies. The level of UA in the cell supernatant can be measured by HPLC, significantly realizing the real-time monitoring of the UA concentration. Cell models of HUA established using this method require small doses of reagents and have low experimental costs. They can also be used to screen UA-lowering drugs. However, these cells are mostly immortal cell lines, a potential limitation because of the different epigenetics of natural cells in the human body.
Hypoxanthine and xanthine are the precursors of UA during purine metabolism (Figure 2). XO can oxidize hypoxanthine to xanthine and subsequently oxidizes xanthine into UA. Adachi et al. (2017) established a cell model of HUA by stimulating BRL3A cells with 4 mol/L xanthine for 48 h. A higher concentration of UA was observed than that in the control experiment by monitoring XO activity and UA levels in the cell supernatant. Based on these results, an in vitro HUA cell model was developed using xanthine as an inducer.
Bisphenol A can increase the reaction efficiency of transforming xanthine to UA by enhancing the activity of XO, giving the possibility of high UA with the presence of bisphenol A. For example, Ma et al. (2018) incubated primary mouse liver cells and 200 M xanthine for hours. After the intervention of 24 h with 0.1 μM or 1 μM bisphenol A, the synthesis of UA in primary hepatocytes was significantly increased. During the experiment, it was necessary to add 500 μM potassium oxonate (PO) to inhibit the decomposition of UA by uricase because of the presence of uricase in primary hepatocytes. This study used primary cells for modeling; however, primary cells have several intrinsic shortcomings, including a difficult separation process, a high proportion of heterozygotic cells, and a highly differentiated tendency.
Human organic anion transporters OAT1 (hOAT1) and OAT3 (hOAT3) are expressed on the basolateral side of proximal tubule cells, facilitating the uptake of organic anions into the cell. The rate absorption transporter hOAT4 can reabsorb urate from the renal tubule into the blood. Hagos et al. (2007) modified HEK293 cells to stably express hOAT1, hOAT3, and OAT4. After incubation with torasemide, hOAT4 expression increased, and simultaneously, the activities of hOAT1 and hOAT3 were inhibited, leading to the inhibition of urate excretion and the occurrence of HUA.
An artificial HUA cell model, established by increasing the expression of human URAT1 (hURAT1), promoted UA reabsorption and inhibited UA excretion. hURAT1 reabsorbs UA into epithelial cells and re-enters blood circulation. For example, Chen et al. (2016a) generated Madin–Darby canine kidney (MDCK) cell lines that persistently expressed hURAT1 in vitro. This cell line (MDCK-hURAT1) significantly enhanced the ability of cells to transport UA, making it suitable for screening urate-lowering drugs.
Among the inducers of HUA, fructose is a special substance that can both promote the synthesis of UA and interfere with the excretion of UA, leading to an increase in SUA content (Perez-Pozo et al., 2010). Fructose is phosphorylated by fructokinase to produce adenosine diphosphate (ADP) in the cytoplasm, which is then metabolized to UA (Figure 2). For example, Chen et al. (2016b) and Wang et al. (2018a) added 5 mmol/L and 10 mmol/L fructose to the medium and incubated the fructose with HK-2 cells for 48 h and 72 h. Compared to the control group, the concentration of UA in cells treated with fructose increased significantly. In addition to the increase in UA, fructose can promote cell inflammation and kidney damage and decrease the clearance efficiency of UA. In vitro studies confirmed that fructose stimulates the expression of monocyte chemoattractant protein (MCP-1) and upregulates the expression of the endothelial pro-inflammatory mediator intercellular adhesion molecule (ICAM-1) in HK-2 cells, which promotes inflammatory response and inhibits UA excretion (Glushakova et al., 2008). HK-2 cells are proximal convoluted tubule cells derived from normal kidneys that were immortalized by incorporating the HPV-16 E6/E7 gene. Thus, these cells have the advantages of immortality, a lack of ethical controversy, and reliable reproducibility. This method provides a pathway for establishing cell models of HUA and a reference for the large-scale screening of UA-lowering drugs in vitro.
The animal models used in HUA research mainly include poultry species, rodents, and primates (Figure 4). Chickens and quails are common model animals used in HUA studies because they have the same UA metabolic pathways as humans. For example, Bian et al. (2020) successfully used quail to establish a research model for HUA by feeding them yeast solutions. However, divergence between poultry species and humans limits their application in HUA studies because of differences in other metabolic processes.
Rodents, especially rats and mice, are the most commonly used animal models in the laboratory. They are more physiologically similar to humans than poultry species. Moreover, they have a smaller body size and are easier to feed than other laboratory animals. However, rodents have urate oxidase in their bodies, which can convert UA into a more soluble allantoin that is excreted from the body. This enzyme activity indicates that rodents cannot maintain a stable HUA status for a prolonged period without external interference.
Primates, with physiological processes similar to humans, have almost the same UA metabolic pathway and can theoretically be considered perfect animal models for HUA studies. However, because of their high cost, tedious operation, and specific metabolic differences, only a few studies have adopted primates as animal models for HUA research.
Zebrafish, as a vertebrate model, have recently become a new animal model for studying human diseases and drug therapies, with the advantages of low price, small size, and short experimental period. However, species differences still exist between zebrafish and humans, and zebrafish-based HUA research models are still in the preliminary exploration stage (Zhang et al., 2019).
No ideal animal model can substitute for human beings in HUA studies. However, as common laboratory animals, mice and rats still play a vital role in studying HUA despite urate oxidase in the body, especially regarding HUA formation and organ damage. Therefore, we will emphasize recent HUA studies using mice or rats as animal models in the section on animal studies.
Animal models can be established by gavage or intraperitoneal injection of UA. For example, Chen et al. (2001) administered an intraperitoneal injection of 250 mg/kg UA and obtained a good HUA disease model. SUA levels in mice peaked 10 min after injection, were maintained for 4 h, and then returned to baseline after 5 h. This method can be used as a fast HUA study tool, such as the selection of UA-lowering drugs, but it is unsuitable for an in-depth study of the specific mechanism of HUA disease.
Adenine can be converted into AMP by adenine phosphoribosyltransferase (APRT), which simultaneously increases the activity of XO, promoting synthesis UA as mentioned above (Figure 2). Yang et al. (2011) intragastrically administered adenine (200 mg/kg, in sodium carboxymethyl cellulose) consecutively for 30 days to establish a rat model of HUA. After 10 days, the SUA level of the rats increased significantly, and the rats showed symptoms of emaciation, kidney injury (kidneys were covered with greyish-yellow particles), and severe renal tubule injury. In short, adenine significantly increased SUA levels and induced HUA in a rat model. The model has good stability, but the accompanying organ injuries must be considered.
Hypoxanthine metabolizes into xanthine via XO catalysis, which eventually generates UA. Zheng and Huang (2011) established an HUA mouse model by intraperitoneal injection of hypoxanthine (1,000 mg/kg). The results showed that the UA level in the mice dramatically increased 30 min after injection but significantly decreased 1 h later. The duration of high UA levels induced by this method is efficient but short, making it suitable for establishing animal models of acute HUA.
Yeast can increase the activity of XO, which promotes the production of UA, disorders of purine metabolism, and, ultimately, leads to the occurrence of HUA. Chen et al. (2003) attempted to add different concentrations of yeast paste to mice by intragastric administration, and the results showed that the UA levels of mice increased continuously when the feed dosage of yeast paste was 15–30 g/kg for 1 to 2 consecutive weeks. A higher concentration of 60 g/kg yeast paste led to the death of the mice. This finding is consistent with previously published experimental phenomena, which indicated that yeast powder could sometimes cause rats to exhibit severe weight loss and poor physical condition. In summary, yeast, as an inducer of HUA in animals, was concentration-dependent and was always added with other induction drugs for disease modeling. Therefore, using yeast as an inducer of HUA is feasible, but a detailed experimental design needs to be explored.
The antituberculosis drug ethambutol competitively inhibits the excretion of UA in the kidney, leading to an increase in SUA levels. Ethambutol and niacin alone are rarely used as modeling agents to establish HUA models. These drugs are often administered in combination with other drugs. In principle, the HUA model induced by anti-TB drugs is identified as secondary HUA, which is not suitable for disease modeling of primary HUA in animals.
Potassium oxazinate (PO) is a drug commonly used to model HUA in mice and rats. It has a purine-analogous structure and can bind to uricase to inhibit UA oxidase activity, leading to the accumulation of UA in the animal body. Wu et al. (2021b) established a mouse model of HUA by intraperitoneal injection of PO for 7 days. The UA level was higher in the model group than in the control group, indicating that the model construction of HUA was successfully achieved. In addition, Wang et al. (2018b) established a rat model of HUA by intraperitoneal injection of PO into Wistar rats. The results showed that 300 mg/kg was the optimal concentration of PO that could maintain clinically defined HUA concentrations for 2 h. The PO-induced animal model of HUA has the advantages of simplicity, convenience, and sensitivity and is suitable for establishing short-term acute animal models of HUA. However, PO inhibits the activity of UA oxidase and other enzymes, such as orotate phosphoribosyl transferase, which sometimes results in uncertain interference with experimental results.
Combination modeling combines several metabolites as inducers for HUA modeling (as mentioned above in the section Yeast as an inducer). A combination of substances is the most common situation, such as a combination of two UA precursors, drugs that decrease UA excretion, and drugs that inhibit uricase activity. This method has the advantages of synergistically increasing the SUA level, elongating the maintenance time of the high SUA level, increasing the stability of the model, and reducing kidney damage. Thus, it is popular in animal HUA modeling.
Shen et al. (2019) used 100 mg/kg adenine and 250 mg/kg ethambutol administered by intragastric administration to prepare a rat model of HUA. They investigated the mechanism of Dendrobium ferruginosa Four Myriad Formula in lowering UA. The UA level of the model group increased gradually after 1, 2, and 3 weeks compared with that of the control group, indicating that the rat HUA model was successfully established. Unfortunately, the rats showed symptoms of kidney injury, such as glomerular atrophy. Therefore, despite its ability to induce animal models, the combination of adenine and ethambutol has a limited application range because of organ damage.
Studies have shown that combining PO and hypoxanthine has the advantages of a fast response and long maintenance time for high SUA levels. He et al. (2021) established a mouse model of HUA by intragastrically administering different concentrations of hypoxanthine and PO to KM male mice consecutively for 7 days. The results showed that the combination of 500 mg/kg hypoxanthine and 1,000 mg/kg PO was an appropriate experimental condition for successfully establishing a mouse model for HUA studies. The treated mice displayed various symptoms, including poor physical conditions, reduced diet, and mental malaise.
Li et al. (2021a) administered a combination of PO and adenine to SD rats intragastrically consecutively for 5 days to investigate the optimal experimental conditions for HUA modeling. After 1 week, the SUA level in the model group increased significantly compared to that in the control group, and an optimized result was achieved at a dosage of 1,500 mg/kg PO and 50 mg/kg adenine. This approach promoted the synthesis of UA and effectively inhibited the activity of uricase, which resulted in a rapid increase in UA levels in a short time. However, kidney injury caused by adenine nephrotoxicity must be considered when adenine is used to establish animal models for HUA studies.
Di (2013) fed male SD rats different doses of yeast paste and PO by intragastric administration and intraperitoneal injection to establish a chronic HUA model. SUA levels in the rats were significantly increased, and the glomeruli, interstitia, and tubules displayed tiny pathological lesions after 28 days. Urate crystal deposition has also been observed in certain animals. SUA levels increased rapidly and were maintained for a long time when yeast paste was combined with 200 mg/kg PO extract. In addition, the degree of renal injury was low, and almost no rats died during the experiment. This approach also affects the heart, large and small arteries, and other tissues and organs, satisfying the symptoms of caused secondary HUA. This method provides an excellent drug screening platform for HUA in animals and a perfect pathway for studying the interaction between HUA and cardiovascular disease.
Liu et al. (2018) created an animal model of HUA by administering subcutaneous injections of PO (100 mg/kg) and fructose (5% and 10%). The results showed that PO and fructose increased SUA levels quickly and maintained them for some time, indicating that the HUA model was successfully established. The combination of PO and fructose can be used to quickly establish an animal model of HUA, with the advantages of higher efficiency, greater stability, and longer sustainability than a single substance. PO combined with 5% fructose did not cause kidney damage and could be used to establish an animal model of asymptomatic HUA in the human body for mechanistic investigations and drug therapies. PO combined with 10% fructose caused obvious renal pathological lesions, which were an indicative of chronic kidney injury in the HUA study.
Bie (2017) used a 0.2 (w/w) yeast diet and 10% fructose to induce HUA in a rat model. The results showed that the SUA concentration remained constant at 2–4 weeks. Compared to yeast and PO, yeast and fructose induced a rapid and continuous increase in SUA levels and reduced kidney damage simultaneously. The combination of yeast and fructose is simple and inexpensive and can be used to establish a stable and reliable animal model of HUA.
Excessive fructose levels lead to elevated SUA levels. Excessive fructose can be phosphorylated by fructokinase to increase the level of AMP and the activity of XO, which both result in a higher UA concentration in the serum. However, excessive fructose will cause kidney injury and disrupt the UA transport system, inhibiting UA excretion and promoting UA reabsorption. Therefore, fructose increased UA production and decreased UA excretion. Li et al. fed SD rats 10% fructose daily and found that SUA levels dramatically increased from 20 to 40 days. Simultaneously, the expression of GLUT9 protein was upregulated, strengthening the UA reabsorption process by the renal tubules. Essawy et al. (2014) continuously fed rats with 60% fructose and found that the levels of both SUA and insulin increased significantly after 4 weeks. Based on these experiments, we speculate that fructose-induced animal modeling of HUA is more suitable for studying HUA accompanied by multiple complications such as diabetes or hyperlipidemia.
Genetic engineering for modeling HUA refers mainly to the deletion of urate oxidase or urate transporter genes to better mimic the pathogenesis of HUA in the human body.
The urate oxidase gene encodes uricase, which is not expressed in humans but is highly expressed in mice. Uricase catalyzes the conversion of UA into allantoin, a substance easily excreted in urine (Figure 2). Knocking out the urate oxidase gene in rats or mice can eliminate the disturbance in allantoin caused by urate oxidase and further simulate UA metabolism in the human body. Lu et al. (2018) knocked out the urate oxidase gene in C57BL/6J mice and found that approximately 40% of the mice survived for 62 weeks. All surviving mice had high SUA levels, indicating the possibility of modeling e HUA. However, mice must be raised in a sterile environment for this type of experiment model, and the mortality of the model animals must be considered in practice.
GLUT9, a type of urate transporter, is expressed in the human kidneys and is responsible for UA reabsorption. However, GLUT9 is mainly expressed in the liver of mice. Studies have demonstrated that while systemic GLUT9 gene knockout leads to high mortality in mouse embryos, hepato-specific GLUT9 gene knockout can result in high survival and UA levels in mice (Zhang et al., 2022b).
ABCG2 is a UA transporter involved in UA secretion in the renal tubules and small intestine. Takada et al. (Faulds et al., 2012) demonstrated that ABCG2 knockout mice had higher SUA levels than those in the control group. In addition, renal UA excretion increased, whereas intestinal UA excretion decreased.
Laboratory animals cannot completely recapitulate the physiology and pathology of UA metabolism in humans because of the species divergence between humans and other animals. Therefore, developing novel, simple, and effective animal models is crucial for investigating HUA.
Lin (2022) intragastrically administered 37.5 mg/kg adenine and 37.5 mg/kg PO to male C57BL/6 mice once a day for 3 weeks to construct a mouse model of HUA with kidney yang deficiency. The treated mice showed reduced weight, increased water intake, increased urine excretion, clear urine, difficult defecation, reduced grip strength, vertical fur, arching back, huddling, and weakened irritability. These symptoms indicated that the model of HUA with kidney yang deficiency was successfully established and could be used in subsequent mechanism exploration or drug screening.
Zhan et al. (Zhan, 2018) successfully established a rat model of damp-heat HUA using a high-fat and high-sugar diet, 10 mL/kg liquor gavage, and 200 g/L honey water ad libitum for 14 days. From the eighth day, the rats were fed a mixture of yeast extract (20 g/kg) and a high-fat diet and intraperitoneally injected with 300 mg/kg PO. Simultaneously, from the 10th day, the rats were kept in an artificial climate chamber with humidity and heat (relative humidity 89%–95%, temperature 30°C–34°C) for 4 days. The UA levels of the model group were significantly higher than those of the control group. In addition, the model group was tired and curled up with reduced activity, yellowed hair, and messy.
A damp-heat rat model of HUA was constructed by feeding rats fat and sweet food (5% lard, 3% egg yolk, and 5% honey added to the fodder) and administering Escherichia coli endotoxin (2 μg/kg) intravenously twice weekly for 6 weeks. The damp-heat HUA rat model could also be established by hypodermic injection of PO (200 mg/kg) and intragastric administration of ethambuterol (250 mg/kg) once daily for 6 weeks (Tang and He, 2019).
A rat model of HUA with spleen deficiency was established by feeding rats PO and high-purine supplements in a small-cage environment (Shang et al., 2020). High-purine supplements were administered during the entire process, and PO was intraperitoneally injected from the third week. Four weeks in total were required for the successful modeling of HUA with spleen deficiency. From the second week, the treated rats showed symptoms of reduced activity, yellow and less luster fur, reduced diet, and soft stools.
Shi et al. (2016) used a high-fat diet, hypoxanthine (500 mg/kg), and PO (100 mg/kg) to induce phlegm-dampness in a rat model of HUA. The rats were treated once daily for 30 days. During this process, the rats showed symptoms of mental sluggishness, rough and yellow fur, less shiny fur, inappetence, and thin and smelly excreta. These symptoms were consistent with the general manifestations of phlegm-dampness syndrome, indicating the success of modeling HUA with phlegm-dampness.
Presently, standard biomedical treatment for HUA mainly depends on drugs with active compounds such as benzbromarone (Zhao et al., 2022), probenecid (Granados, 2022), allopurinol (Rodevand et al., 2004), febuxostat (Chinchilla et al., 2016), and topiroxostat (Kario et al., 2021). These drugs have proven to be effective but are accompanied by side effects such as poor medication compliance and SUA rebound (Strilchuk, 2019). Therefore, clinicians should consider non-pharmacological dietary interventions before using these drugs (Liska, 2021). In addition to dietary interventions, TCM has become a choice for HUA treatment because of its mild but efficient therapeutic effect.
Based on dialectical thinking, TCM is guided by the “holistic view” and “prevention of disease” theory. It regulates all aspects of UA metabolism, highlighting the advantages of effects and multiple targets in the treatment of HUA. According to the TCM literature, HUA is treated by removing dampness and turbidity, strengthening the spleen and kidney, promoting blood circulation, eliminating blood stasis, etc. Therefore, high SUA levels are mainly regulated by damp-clearing, heat-clearing, rheumatism-clearing, and blood circulation-promoting drugs. Recent studies have shown that TCM plays a vital role in treating HUA by decreasing UA production, promoting UA excretion, inhibiting urate oxidase activity, regulating the expression of inflammatory factors, and improving the body’s self-regulation ability.
This study used a single-botanical drug as the research object. The approach involved systematically sorting its mechanism of action on UA metabolism and providing new ideas for drug screening, prescription compatibility, and target determination in the treatment of HUA. The study further explored the therapeutic effect of single-botanical drugs on HUA and provided a theoretical basis for experimental research and clinical treatment of HUA (see Table 2).
Cromidon plantaginis (L.f.) Hilliard [Scrophulariaceae] (CPH)
Plantaginis is identified as a dry or fresh whole botanical drug of Plantago asiatica L. or Plantago depressa Willd. because of its functions of clearing heat, diuretic, expelling phlegm, cooling blood, and removing toxins. Its extracts Plantaginis inhibit the production of reactive oxide species (ROS), a kind of substance that can induce kidney injury, and downregulate the expression of URAT1 mRNA in the kidney, which improves kidney function and promotes UA excretion in mice (Zeng et al., 2013). CPH Plantaginis can also downregulate the expression of NLRP3, an apoptosis-related point-like protein (ASC), caspase-1, and the inflammatory factor IL-12 in renal tissue, thereby attenuating the inflammatory response (Zou et al., 2021). In addition, plantamajoside in CPH Plantaginis can decrease UA levels in PO-induced acute HUA mice by regulating renal UA transporter expression, TLR/MyD88/NF-κB pathways, and NLRP3 inflammatory pathways (Tingting, 2020).
Polygonum cuspidatum Siebold & Zucc. [Polygonaceae](PCSZ)
Polygoni Cuspidati Polygonum cuspidatum Sieb. is a traditional botanical drug with the functions of clearing heat, detoxifying, dispersing stasis, relieving pain, removing dampness, and eliminating jaundice. Based on a rat HUA model established using PO and adenine, Ye (2019) found that PCSZ significantly reduced SUA levels and protected the kidney. The possible mechanism of lowering SUA levels is inhibiting the reabsorption of UA by renal tubules by inhibiting the expression of GLUT9 and URAT1 mRNA and promoting UA excretion in the kidney by increasing the expression of OAT3 mRNA. In addition, polydatin can reduce the expression of inflammatory factors TNF-α, IL-1β, and IL-6 in serum, playing an anti-inflammatory role and alleviating inflammation-caused kidney injury (Ma et al., 2019).
Dioscorea collettii var. hypoglauca (Palib.) S.J.Pei & C.T.Ting [Dioscoreaceae] (DCVH)
Dioscoreae Hypoglaucae, Dioscorea septemloba, and Dioscorea hypoglauca, plays an important role in eliminating dampness and turbidity, dispelling pathogenic wind, and dredging channel blockage. In a rat model of HUA induced by PO and ethambutol, Chen et al. (2016a) found that the total saponins of Bixie could reduce SUA levels in a dose-dependent manner. DCVH could reduce the expression of URAT1 and decrease UA reabsorption. In contrast, it can upregulate the expression of OAT1, OAT3, and ABCG2 and promote the excretion of UA in the kidney. The ability of DCVH to decrease SUA by promoting UA excretion could be reflected by UA excretion indexes, such as 24 h UA excretion, UA excretion fraction, UA clearance rate, creatinine clearance rate, unit glomerular filtration UA excretion, and urinary UA content (Zhang et al., 2018).
Ginkgo L. [Ginkgoaceae], the dry leaves of Ginkgo biloba L., promote blood circulation, remove blood stasis, smooth collaterals, relieve pain, gather lungs and relieve asthma, remove turbidity, and reduce lipids. In addition, GF extract effectively reduced ICAM-1 and MCP-1 mRNA expression, decreased inflammatory factor release, and maintained glomerular endothelial cell stability (Cheng and Zhu, 2013). In summary, GF decreases SUA levels mainly by alleviating the inflammatory damage to the kidneys.
Cichorium intybus L. [Asteraceae]
Cichorium intybus is a perennial botanical drug belonging to the Cichorium genus of the Asteraceae family that causes diuresis detumescence and purging of the gallbladder and liver. It can significantly reduce UA levels through multiple pathways and target effects. Chicory reduces UA production by inhibiting the activities of key enzymes involved in UA metabolism. However, it can also increase UA excretion by inhibiting UA reabsorption in the kidney and regulating intestinal flora. Wang et al. (2017a) and Jin et al. (2018) used fructose-induced HUA in rats to study the uric-lowering mechanism of chicory. They found that decreased UA was associated with the downregulation of GLUT9 and ABCG2 and the upregulation of OAT1, OAT1-LIKE, and OAT3-LIKE (Wang et al., 2017b). Bian et al. (2020) chose quail fed with a high-purine diet as their research target. They found that chicory could recover intestinal flora distribution, reduce bacterial endotoxin levels in the cecal contents and peripheral blood, and decrease SUA levels.
Poria is a medicinal and edible fungus important in promoting water infiltration, strengthening the spleen, and soothing the heart. In HUA mice induced by hypoxanthine and PO, Liang et al. (2021) found that a poria extract decreased SUA levels and upregulated ABCG2 content. This protein lowers UA levels and protects the kidney. Wang et al. (2022) established an HUA rat model using adenine and PO to evaluate the anti-HUA efficacy of poria powder. They found that poria powder significantly improved renal function, glomerular atrophy, and renal tubule dilation in HUA rats. Poria powder also significantly improved the relative abundance of Bacteroidetes, Alistipes, and Parabacteroides in the intestinal tract and reduced the relative abundance of Firmicutes to regulate the intestinal flora. It was speculated that poria powder might promote UA excretion by regulating intestinal flora to lower UA and alleviate kidney injury.
Smilax glabra Roxb. [Smilacaceae]
Smilacis Glabrae is a rhizome of Smilax glabra Roxb. Studies have shown that SGR can effectively reduce SUA levels and protect kidneys through multi-target and multi-pathway synergistic functions in a PO-induced HUA mouse model. The mechanism has three aspects: one is that SGR can inhibit the activity of XO in the liver and subsequently inhibit the production of UA; one is that SGR can downregulate renal URAT1 and GLUT9 mRNA expression to promote renal UA excretion (Huang et al., 2019); the last one is that SGR can decrease the expression of IL-1β and TNF-α and alleviate the kidney injury caused by inflammation (Wang et al., 2019a).
Gentiana quadrifaria Blume [Gentianaceae]
Gentianae is a valuable TCM with functions such as clearing heat and dampness and purging the liver and gall. Gentiopicrin, the main active metabolite of gentian, significantly improved UA levels in PO-induced HUA mice. It regulates the expression of renal UA transporters, reduces the expression of GLUT9 and URAT1, and increases the expression of OAT1 and OAT3, thereby decreasing UA levels. Simultaneously, gentian inhibited the activation of NLRP3 inflammasomes produced because of excessive UA accumulation and relieved kidney inflammation (Au et al., 2021).
Pueraria montana var. lobata (Willd.) Maesen & S. M. Almeida ex Sanjappa & Predeep [Fabaceae]
Puerariae Lobatae dispels wind and dampness, activates blood circulation and collaterals, and invigorates the spleen with aromatics. In rats with HUA induced by yeast paste and PO, its extract showed anti-gouty arthritis and decreased SUA level function by inhibiting UA production and promoting UA excretion. Kudarin could significantly increase the activity of alkaline phosphatase and promote the expression of ABCG2 in HK-2 cells, thereby promoting UA excretion and reducing UA (Zhang et al., 2016).
Lonicera sempervirens L. [Caprifoliaceae]
Lonicerae Japonicae, because of its sweet taste, cold nature, and lung/stomach/heart tropism, has the functions of clearing heat and toxins, dispelling wind and heat, etc. Studies showed that honeysuckle can significantly inhibit XO activity and reduce SUA levels effectively in HUA mice induced by PO (Chien et al., 2009). Molecular docking showed that the binding energies of isochlorogenic acid B, isochlorogenic acid A, and chlorogenic acid or esculin with XO were higher than those with febuxostat, indicating higher inhibitory effects on XO. Both quercetin and isochlorogenic acid B have significant urate-lowering effects and inhibit XO activity in fructose-induced HAU rats (Peng, 2020).
Portulaca quadrifida L. [Portulacaceae]
Portulaca oleracea is a wild medicinal and edible plant important in clearing heat and dampness and detoxification and has anti-inflammatory, thirst relieving, and diuretic properties. Lv et al. (2022) established an HUA mouse model using hypoxanthine and PO stimulation. They found that purslane extract effectively reduced SUA levels in a dose-dependent manner in HUA mice but had little effect on urinary UA levels. The mechanism may be that the purslane extract reduces the activity of XO in the liver and further inhibits the production of UA in the body.
Taraxacum sect. Taraxacum F.H. Wigg. [Asteraceae]
Taraxaci is an Asteraceae plant that can clear heat and toxins, relieve swelling and eliminate mass, induce diuresis, and free strangury. Zou et al. (2020) established a rat model of acute HUA using a combination of PO and hypoxanthine. They administered dandelion extract intragastrically after 1 week. They found that different doses (60 g/kg, 30 g/kg, and 15 g/kg, respectively) of water, n-butanol, ethyl acetate, and petroleum ether extract significantly reduced SUA levels.
Gardenia thunbergia Thunb. [Rubiaceae]
Gardenia jasminoides protects the liver, benefits the gallbladder, relieves hypertension, maintains sedation, prevents bleeding, and reduces swelling. Hu et al. (2013) reported that geniposide, the main active metabolite in gardenia, could significantly reduce SUA levels and increase UA excretion to lower UA levels in the body by inhibiting the activity of XO in the liver and regulating the expression of mURAT1, mGLUT9, mOAT1, mOAT3, and mABCG2 in the kidneys of PO-induced HUA mice. In addition, gardenia extract reduced serum creatinine and urea levels and upregulated the expression of organic cationic/carnitine transporters, thereby repairing renal dysfunction in an HUA model (Meng et al., 2014).
Perilla frutescens (L.) Britton [Lamiaceae]
Perilla frutescens has analgesic, sedative, and detoxification properties. It can regulate SUA levels, promote renal UA excretion, and protect the renal tissue. Zhang et al. (2020) established an HUA mouse model by intragastrically administering yeast paste and PO. They found that the decrease in kidney index, UA, creatinine, and UA levels depended on the dose of Perilla frutescens.
Chrysanthemum × morifolium (Ramat.) Hemsl. [Asteraceae](CM) is an ornamental plant with medicinal value in dispelling wind-heat, tranquilizing the liver, improving eyesight, and clearing heat toxins. Shen (2019) reported that the inhibition of XO by the CM alcohol extract depended on the concentration of the CM alcohol extract, and the inhibition percentage of a high concentration of the CM alcohol extract on XO could reach 50%. Li et al. (2021b) screened three potential XO inhibitors from CM, genistein, luteolin, and apigenin, using surface plasmon resonance technology. Peng et al. (2019) established an HUA rat model by intragastric PO administration. They found that extracts of “Boju,” a kind of CM that is well-known in China, had an obvious UA-lowing effect and renal function protection ability by inhibiting XO activity and regulating the expression of renal UA transport-related proteins (ABCG2, URAT1, and GLUT9) and lipids. The extract also interfered with the pathological process of HUA by regulating biomarkers associated with the metabolism of tryptophan, sphingomyelin, glycerolipids, and arachidonic acid.
Zingiber officinale Roscoe [Zingiberaceae] (ZOR) relieves the exterior and dispels cold, warms the spleen and stomach, stops vomiting, warms the lungs, arrests cough, and removes toxins. Qi et al. (2011) found that a crude methanol extract of ZOR had an inhibitory effect on XO activity in vitro. However, only a dosage greater than 600 mg/kg demonstrated SUA-lowering ability and significantly inhibited XO activity in the liver.
Glycyrrhiza Tourn. ex L. [Fabaceae]
Glycyrrhizae invigorates the spleen, replenishes qi, moistens the lung, arrests cough, clears heat toxins, and reconciles multiple medications. Studies have shown that polysaccharides significantly inhibit XO activity at different doses, reducing SUA levels in rats (Guo, 2018). Wang et al. (2019b) found that glycyrrhizin, the main metabolite of licorice, significantly reduced blood UA levels and increased UA excretion in HUA mice. Glycyrrhizin downregulates the expression of OAT4 and URAT1 in the kidney tissues of HUA mice, thereby affecting UA metabolism and playing a role in UA reduction. Glycyrrhizin flavanol had a good ability to lower UA in vitro. It could improve recovery from oxidative stress injury in NRK-52E cells caused by high UA levels, possibly because of the inhibition of XO activity (Cao et al., 2022).
Sun et al. (2017) found that Lycium barbarum var. aurantiicarpum K.F. Ching [Solanaceae] extract at a medium dose (5 g/kg) and high dose (10 g/kg) significantly reduced the content of SUA and XO in HUA mice, indicating that the extract of Lycium barbarum maintained the efficacy of HUA treatment by reducing XO content to decrease UA production. Yu et al. (2020) established an HUA mouse model using PO. After treatment with Lycium barbarum polysaccharide, SUA level significantly decreased, whereas urinary UA level significantly increased. Correspondingly, they observed that OAT1 and OAT3 levels increased, and GLUT9 levels decreased in the kidney. Meanwhile, serum XO and liver XO activity decreased after treatment with Lycium barbarum polysaccharide.
Gynostemma pentaphyllum (Thunb.) Makino [Cucurbitaceae] is a cold-natured and bitter-tasting botanical drug that clears heat and toxins, arrests cough, clears lung heat, nourishes the heart for tranquilization, benefits qi, produces essence, and so on. Wu et al. (2020) found that gypenoside extract effectively inhibits the increase in SUA levels by inhibiting UA production and increasing UA solubility in body fluids, thereby protecting kidney function and promoting UA excretion. Pang et al. (2017) established an HUA animal model using a lipid emulsion; they found that the SUA, XOD, ADA, and XDH levels significantly decreased after gypenoside treatment. Simultaneously, URAT1 and GLUT9 expressions were downregulated, OAT1 expression was upregulated in the kidney, and the renal index and UA excretion fractions were increased.
Modern pharmacological studies have shown that Dioscorea polystachya Turcz. [Dioscoreaceae] (DPT) Dioscoreae Nipponice has anti-inflammatory and analgesic effects and is now widely used to treat inflammatory diseases. The total saponins in DPT can decrease UA production by inhibiting the activities of XO and ADA. Liu (2019) reported that total pangolin saponin could effectively adjust the mRNA and protein levels of URAT1, GLUT9, OAT1, and OAT3 and promote renal urate excretion in PO-induced HUA mice.
The total flavonoids in Alpinia galanga L.) Willd. [Zingiberaceae] Alpinia officinarum significantly reduced SUA levels by promoting UA excretion and inhibiting UA production in HUA mice. Xue et al. (Xue et al., 2017; Xue et al., 2018) found that galangal extract lowered SUA levels by inhibiting hepatic XO activity in both PO-induced HUA mice and hypoxanthine-induced HUA mice.
Sanghuangporus spp. (SS) are the fruiting bodies of Porophyllaceae. Studies have shown that both the polysaccharide and alcohol extracts of SS have inhibitory effects on the activity of XO to some extent, and the alcohol extract of SS has better UA-lowering ability than the crude polysaccharide of SS. The polysaccharide of SS showed an anti-competitive inhibition effect, whereas the alcohol extract showed non-competitive inhibition when interacting with XO (Li et al., 2022a).
Nelumbo nucifera Gaertn. [Nelumbonaceae] can reduce UA levels and improve renal function by regulating the expression of organic ion transporters in the kidneys during HUA treatment. It can also inhibit the activation of the TLR4/MyD88/NF-κB pathway and the NLRP3 inflammasome, relieving the renal inflammatory response (Wang et al., 2015). In addition, Liu (2019) showed that flavonoids can inhibit XO activity in vitro.
Presently, the ability of TCM to dissolve urate is insufficient to reach a level similar to that of urate oxidase. However, studies have demonstrated that some TCMs (such as Cremastra appendiculata (D.Don) Makino [Orchidaceae], Atractylodes lancea (Thunb.) DC. [Asteraceae], and Rheum palmatum L. [Polygonaceae]), can promote the dissolution of urate better than the 5% sodium bicarbonate solution commonly used in surgical lavage (Lin et al., 2022).
Tufuling granules remove turbidity, eliminate pathogens, remove dampness, and clear heat. HUA was induced with PO (100 mg/kg) by intraperitoneal injection and hypoxanthine (500 mg/kg) by oral gavage. The tufuling granule solution was administered by gavage at doses of 1 g/kg, 2 g/kg, and 4 g/kg, whereas the positive control group was administered febuxostat (4 mg/kg) by gavage. Medium and high doses of granules effectively reduced SUA levels in model rats. Tufuling granules inhibit XDH mRNA expression and reduce XO activity in the liver. Therefore, they can lower UA production directly or indirectly and are s usually used for the clinical treatment of HUA (Zhu, 2015).
An HUA model was established by combining yeast (15 g/kg), adenine (100 mg/kg), and potassium oxonate (300 mg/kg). Fan et al. (2017) found that ermiao pill could reduce UA production by inhibiting the activity of XO and reducing UA levels in HUA rats. In addition, Lv et al. (Lv et al., 2010) found that an ermiao pill and Huangbo water extract significantly reduced the expression of mURAT1 in the kidney of HUA mice, indicating that they could reduce UA levels by inhibiting the function of UA reabsorption transporters.
The classical Guizhi decoction effectively decreases the production of UA by inhibiting the activity of XO in the liver, promoting UA excretion by downregulating the protein levels of URAT1 and GLUT9 and upregulating the protein levels of ABCG2, OCT1, OCT2, OCTN1, and OCTN2 in the kidneys of PO-induced HUA mice. In other words, the Guizhi decoction reduced SUA levels and protected the kidneys (Tan, 2014).
Zuo et al. (2018) established an HUA mouse model (induced by hypoxanthine and PO) to investigate the effect of the erding decoction on reducing UA levels. They found that the erding decoction has anti-inflammatory and analgesic effects. It reduced UA levels by inhibiting URAT1 mRNA expression and increasing OAT3 mRNA expression. They also found that the 50% ethanol extract of erding granules could reduce UA levels and improve the pathological injury of the kidney by inhibiting the expression of inflammatory factors TNF-α, IL-1β, and IL-6 and regulating the expression of GLUT9 and OAT1 proteins.
Qiling formula granule, a clinical prescription, strengthens the spleen by benefitting qi, eliminates blood stasis, resolves turbidity, clears heat, and promotes diuresis. The HUA model was established by feeding mice 10% yeast meal combined with adenine (0.1 g/kg) and PO (0.1 g/kg). Studies have shown that qiling formula granules improve renal function and lipid metabolism in rats with HUA (Zhang and Xu, 2020). A possible mechanism is that qiling formula granules could inhibit the activity of XO in the liver, downregulate the mRNA level of URAT1 in the kidney, and upregulate the mRNA levels of OAT1 and OCT2. Therefore, they promoted UA excretion by reducing the reabsorption of renal urate and increasing its secretion.
Liang et al. (2016) modified Simiao powder and evaluated its efficacy in adenine- (100 mg/kg) and PO-induced (1.5 g/kg) HUA rats. They found that the modified Si-Miao-San powder had an obvious effect on reducing SUA levels and could significantly improve kidney injury caused by HUA, which might be related to the upregulation of URAT1 and GLUT9 protein expression and the downregulation of OAT1 protein expression in the kidney (Cao et al., 2021).
HUA is a metabolic disease caused by multiple factors. Establishing research models plays an important role in recapitulating HUA and developing a deeper understanding of the disease mechanism. In this review, we systematically discuss HUA evaluation model construction methods using cell or animal models by increasing the source of UA, inhibiting the activity of uricase, inhibiting the excretion of UA, knocking out genes, and destroying intestinal flora. However, cell models cannot fully replicate the pathogenesis of HUA in vitro because of the lack of a complex cellular microenvironment, and animal models face problems such as long experimental periods, high experimental costs, and species divergence. Although it is a key tool for studying the mechanism of HUA and drug development, the HUA model still has some problems and limitations regarding the effect stability and mechanism of HUA. Currently, most relatively mature and widely used animal models use a combination of several different modeling drugs that synergistically increase UA. The combination of UA precursors and UOX inhibitors has the advantages of rapidly increasing SUA levels, prolonging maintenance time, and reducing renal damage. Compared to animal models, cell models can be used for efficient, sensitive, and accurate screening of urate-lowering drugs at the cellular and molecular levels, especially for high-throughput drug screening. However, some shortcomings remain in constructing HUA cell models, such as the complex isolation of primary cells, highly differentiated cells, and single drug targets.
In summary, cell models cannot fully replicate the pathogenesis of HUA in vitro because of the lack of a complex cell microenvironment, and animal models face problems with long experimental periods, high experimental costs, and species divergence. Therefore, appropriate animal and cell models and methods should be selected according to the research purpose and experimental conditions. New alternative research techniques, such as organ-on-chips or organoids (Li et al., 2022b; Hou et al., 2022), should be considered to fully recapitulate the physiological and pathological characteristics of HUAs.
As HUA has become a common and high-risk metabolic disease, it is important to develop drugs for the prevention and treatment of HUA. Many studies have confirmed that TCM can treat HUA through multiple pathways and targets, with the advantages of few side effects. TCM can both reduce the level of UA and protect organs from damage, which has great research and development potential in the prevention and treatment of HUA, especially damp-clearing drugs. The pathogenesis of HUA is spleen deficiency and dampness excess. Damp-clearing drugs are based on instilling spleen and removing dampness and treat the spleen and kidney simultaneously, showing unique advantages among many TCMs.
In recent years, the mechanism of action of TCM in the treatment of HUA mainly plays a role in promoting UA excretion, inhibiting UA production, regulating gene expression, and affecting the expression of inflammatory mediators. UA metabolism is closely related to intestinal microflora. Therefore, taking UA intestinal metabolism as the entry point and intestinal microflora as the target, a future research direction is to systematically elaborate the mechanism of the interaction between TCM, intestinal microflora, and HUA. At present, there are many methods to improve intestinal flora disorders, such as probiotics, antibacterial drugs, and fecal microbiota transplantation technology. In the future, TCM intervention can be combined with these methods to improve HUA and promote the internationalization of TCM.
We focused on traditional HUA model construction methods and did not fully present all novel techniques, such as 3D HUA models. In addition, we emphasized the role of TCM in HUA treatment, with few chemical drugs available. This study had some limitations. However, a comprehensive introduction to the recent research progress of HUA in evaluation model construction and TCM intervention will undoubtedly provide a scientific reference for TCM-related HUA studies. In the future, TCM or multiple active compound combinations, together with a reasonable and reliable disease modeling method, will be an efficient pathway for HUA studies and provide a new reference and direction for the clinical treatment of HUA.
HZ: writing–original draft. JY: writing–original draft. XY: writing–original draft. XS: investigation, visualization, and writing–review and editing. XZ: supervision, project administration, and writing–review and editing. TC: visualization and writing–review and editing. JZ: writing–review and editing.
The author(s) declare that financial support was received for the research, authorship, and/or publication of this article. This work was supported by the Taishan Young Scholar Program of Shandong [TSQN202103110], the Qingchuang Talents Induction Program of Shandong Higher Education Institution [10073004], the General Program of National Natural Science Foundation of China [82174039], the General Project of Shandong Natural Science Foundation [ZR2020MH371], and the Key Project of TCM Science and Technology in Shandong Province [Z-2022085].
The authors declare that the research was conducted in the absence of any commercial or financial relationships that could be construed as a potential conflict of interest.
All claims expressed in this article are solely those of the authors and do not necessarily represent those of their affiliated organizations, or those of the publisher, the editors, and the reviewers. Any product that may be evaluated in this article, or claim that may be made by its manufacturer, is not guaranteed or endorsed by the publisher.
The Supplementary Material for this article can be found online at: https://www.frontiersin.org/articles/10.3389/fphar.2024.1294755/full#supplementary-material
Adachi, S. I., Yoshizawa, F., and Yagasaki, K. (2017). Assay systems for screening food and natural substances that have anti-hyperuricemic activity: uric acid production in cultured hepatocytes and purine bodies-induced hyperuricemic model mice. Cytotechnology 69 (3), 435–442. doi:10.1007/s10616-016-0005-z
Alvarez-Lario, B., and Alonso-Valdivielso, J. L. (2014). Hyperuricemia and gout; the role of diet. Nutr. Hosp. 29 (4), 760–770. doi:10.3305/nh.2014.29.4.7196
Au, S., Li, C., Shao, Y., Xie, B., and Hu, R. (2021). Gentiopicroside improves hyperuricemia and renal inflammation in mice via uric acid transporter proteins and NLRP3 inflammasome signaling pathways. Cent. South Pharm. Novemb. 19 (11), 2334–2340.
Bian, M., Wang, J., Wang, Y., Nie, A., Zhu, C., Sun, Z., et al. (2020). Chicory ameliorates hyperuricemia via modulating gut microbiota and alleviating LPS/TLR4 axis in quail. Biomed. Pharmacother. 131, 110719. doi:10.1016/j.biopha.2020.110719
Bie, F. (2017). Fructose combined with yeast induced hyperuricemia model in rats and its mechanism of uric acid metabolism. Qingdao, China: Qingdao University, 49.
Cao, L., Zhao, T., Xue, Y., Xue, L., Chen, Y., Quan, F., et al. (2021). The anti-inflammatory and uric acid lowering effects of Si-Miao-San on gout. Front. Immunol. 12, 777522. doi:10.3389/fimmu.2021.777522
Cao, M., Diao, N., Cai, X., Chen, X., Xiao, Y., Guo, C., et al. (2023). Plant exosome nanovesicles (PENs): green delivery platforms. Mat. Horizons. 10 (10), 3879–3894. doi:10.1039/d3mh01030a
Cao, W., Huang, L., Liu, L., Qian, Y., Yu, H., and Li, F. (2022). Licoflavonol reduces uric acid and the effects on high uric acid-induced oxidative stress in renal tubular epithelial cells. Chin. Mod. Appl. Pharm., 1–7. doi:10.13748/j.cnki.issn1007-7693.2023.04.003
Chen, G., Sun, X., Wang, Q., Zhang, X., and Liu, P. (2001). Study on hyperuricemia model in mice. Chin. Phar macological Bull. (03), 350–352.
Chen, G., Zhang, Q., Ma, X., and Xu, S. (2003). Yeast induced hyperuricemia in mice. Chin. Pharmacol. Bull. (04), 467–469.
Chen, J., Wu, T., Qiu, Y., Cao, Y., and Pang, J. (2016a). A cell line stably expressing lentivirus-mediated h URAT1 as an in vitro model of screening uricosuric agents. Life Sci. Res. 20 (03), 248–254.
Chen, Y., Chen, X. L., Xiang, T., Sun, B. G., Luo, H. X., Liu, M. T., et al. (2016b). Total saponins from dioscorea septemloba thunb reduce serum uric acid levels in rats with hyperuricemia through OATP1A1 up-regulation. J. Huazhong Univ. Sci. Technol. Med. Sci. 36 (2), 237–242. doi:10.1007/s11596-016-1573-z
Chen, Z., Sun, Y., Wang, J., Zhou, X., Kong, X., Meng, J., et al. (2023). Dual-Responsive triple-synergistic Fe-mof for tumor theranostics. ACS Nano 17 (10), 9003–9013. doi:10.1021/acsnano.2c10310
Cheng, H., and Zhu, J. (2013). Study on reducing uric acid and inhibiting Xanthine oxidase from Ginkgo biloba leaf extract. Bull. traditional Chin. Med. 12 (02), 61–63. doi:10.13748/j.cnki.issn1007-7693.2023.04.003
Chien, S. C., Yang, C. W., Tseng, Y. H., Tsay, H. S., Kuo, Y. H., and Wang, S. Y. (2009). Lonicera hypoglauca inhibits xanthine oxidase and reduces serum uric acid in mice. Planta Med. 75 (4), 302–306. doi:10.1055/s-0029-1185300
Chinchilla, S. P., Urionaguena, I., and Perez-Ruiz, F. (2016). Febuxostat for the chronic management of hyperuricemia in patients with gout. Expert Rev. Clin. Pharmacol. 9 (5), 665–673. doi:10.1586/17512433.2016.1162094
Cortes, J., Moore, J. O., Maziarz, R. T., Wetzler, M., Craig, M., Matous, J., et al. (2010). Control of plasma uric acid in adults at risk for tumor Lysis syndrome: efficacy and safety of rasburicase alone and rasburicase followed by allopurinol compared with allopurinol alone--results of a multicenter phase III study. J. Clin. Oncol. 28 (27), 4207–4213. doi:10.1200/JCO.2009.26.8896
Essawy, S. S., Abdel-Sater, K. A., and Elbaz, A. A. (2014). Comparing the effects of inorganic nitrate and allopurinol in renovascular complications of metabolic syndrome in rats: role of nitric oxide and uric acid. Arch. Med. Sci. 10 (3), 537–545. doi:10.5114/aoms.2013.33222
Fan, K., Yan, H., Dai, X., Xing, L., and Wang, Y. (2017). Effects of ermiao pills on hyperuricemia in rats. J. Tianjin Univ. Chin. Med. 36 (01), 43–48.
Fathallah-Shaykh, S. A., and Cramer, M. T. (2014). Uric acid and the kidney. Pediatr. Nephrol. 29 (6), 999–1008. doi:10.1007/s00467-013-2549-x
Faulds, M. H., Zhao, C., Dahlman-Wright, K., and Gustafsson, J. A. (2012). The diversity of sex steroid action: regulation of metabolism by estrogen signaling. J. Endocrinol. 212 (1), 3–12. doi:10.1530/JOE-11-0044
Feng, B., Wang, X., Qi, Q., and Yu, J. (2022). The prevention and treatment of hyperuricemia based on TCM thought of “preventive treatment of disease”. West. J. Traditional Chin. Med. 35 (12), 96–99.
Flores, J., de Ceballos, E., Hernandez-Cruz, B., Munoz, A., Machuca-Aguado, J., Gallardo, S. R., et al. (2023). Refractory multisystemic sarcoidosis, a diagnosis and treatment challenge: a case report. J. Med. Case Rep. 17 (1), 303. doi:10.1186/s13256-023-03996-w
Glushakova, O., Kosugi, T., Roncal, C., Mu, W., Heinig, M., Cirillo, P., et al. (2008). Fructose induces the inflammatory molecule ICAM-1 in endothelial cells. J. Am. Soc. Nephrol. 19 (9), 1712–1720. doi:10.1681/ASN.2007121304
Guo, M. (2018). Study on lowering uric acid of Glycyrrhiza polysaccharides and its granules. Zhenjiang, China: Jiangsu University, 92.
Hagos, Y., Bahn, A., Vormfelde, S. V., Brockmoller, J., and Burckhardt, G. (2007). Torasemide transport by organic anion transporters contributes to hyperuricemia. J. Am. Soc. Nephrol. 18 (12), 3101–3109. doi:10.1681/ASN.2007010106
Han, Y., Zhang, M., Lu, J., Zhang, L., Han, J., Zhao, F., et al. (2017). Hyperuricemia and overexcretion of uric acid increase the risk of simple renal cysts in type 2 diabetes. Sci. Rep. 7 (1), 3802. doi:10.1038/s41598-017-04036-6
He, X., Xu, L., Zhu, C., Pan, X., He, M., Wu, L., et al. (2021). Establishment of a rat model of hyperuricemia combined with hypoxanthine and potassium oxoxazine. Chengdu Univ. Traditional Chin. Med. 34 (04), 541–543. doi:10.13982/j.mfst.1673-9078.2022.5.0956
Hillif, D. (2013). Intervention of Rosuvastatin calcium in rats with high uric acid and its effect mechanism. Ürümqi, China: Xinjiang Medical University, 118.
Hong, Q., Wang, L., Huang, Z., Feng, Z., Cui, S., Fu, B., et al. (2020). High concentrations of uric acid and angiotensin II act additively to produce endothelial injury. Mediat. Inflamm. 2020, 8387654. doi:10.1155/2020/8387654
Hou, C., Hu, Y., Jiang, H., Xu, Z., Sha, W., Liu, J., et al. (2022). Establishment of a 3D hyperuricemia model based on cultured human liver organoids. Free Radic. Biol. Med. 178, 7–17. doi:10.1016/j.freeradbiomed.2021.11.023
Hou, C., Liu, D., Wang, M., Gong, C., Li, Y., Yang, L., et al. (2019). Novel xanthine oxidase-based cell model using HK-2 cell for screening antihyperuricemic functional compounds. Free Radic. Biol. Med. 136, 135–145. doi:10.1016/j.freeradbiomed.2019.04.007
Hu, Q. H., Zhu, J. X., Ji, J., Wei, L. L., Miao, M. X., and Ji, H. (2013). Fructus Gardenia Extract ameliorates oxonate-induced hyperuricemia with renal dysfunction in mice by regulating organic ion transporters and mOIT3. Molecules 18 (8), 8976–8993. doi:10.3390/molecules18088976
Huang, L., Deng, J., Chen, G., Zhou, M., Liang, J., Yan, B., et al. (2019). The anti-hyperuricemic effect of four astilbin stereoisomers in Smilax glabra on hyperuricemic mice. J. Ethnopharmacol. 238, 111777. doi:10.1016/j.jep.2019.03.004
Huang, X., Kong, N., Zhang, X., Cao, Y., Langer, R., and Tao, W. (2022). The landscape of mRNA nanomedicine. Nat. Med. 28 (11), 2273–2287. doi:10.1038/s41591-022-02061-1
Ichida, K., Matsuo, H., Takada, T., Nakayama, A., Murakami, K., Shimizu, T., et al. (2012). Decreased extra-renal urate excretion is a common cause of hyperuricemia. Nat. Commun. 3, 764. doi:10.1038/ncomms1756
Ji, X., Ge, L., Liu, C., Tang, Z., Xiao, Y., Chen, W., et al. (2021). Capturing functional two-dimensional nanosheets from sandwich-structure vermiculite for cancer theranostics. Nat. Commun. 12 (1), 1124. doi:10.1038/s41467-021-21436-5
Jiang, T., Xie, D., Wu, J., He, H., Wang, H., Wang, N., et al. (2020). Association between serum copper levels and prevalence of hyperuricemia: a cross-sectional study. Sci. Rep. 10 (1), 8687. doi:10.1038/s41598-020-65639-0
Jianmin, C., An, L. J., Jing, Z., Ying, W., and Zhen, C. (2022). Pathogenesis analysis of hyperuricemia and treatment strategy of Xuanzhong upper infiltration. TCM Clin. Res. 14 (12), 44–47.
Jin, L., Guo, X., Gao, D., Liu, Y., Ni, J., Zhang, Z., et al. (2022). An NIR photothermal-responsive hybrid hydrogel for enhanced wound healing. Bioact. Mat. 16, 162–172. doi:10.1016/j.bioactmat.2022.03.006
Jin, Y. N., Lin, Z. J., Zhang, B., and Bai, Y. F. (2018). Effects of chicory on serum uric acid, renal function, and GLUT9 expression in hyperuricaemic rats with renal injury and in vitro verification with cells. Med 2018, 1764212. doi:10.1155/2018/1764212
Kario, K., Nishizawa, M., Kiuchi, M., Kiyosue, A., Tomita, F., Ohtani, H., et al. (2021). Comparative effects of topiroxostat and febuxostat on arterial properties in hypertensive patients with hyperuricemia. J. Clin. Hypertens. 23 (2), 334–344. doi:10.1111/jch.14153
Kimura, Y., Tsukui, D., and Kono, H. (2021). Uric acid in inflammation and the pathogenesis of atherosclerosis. Int. J. Mol. Sci. 22 (22), 12394. doi:10.3390/ijms222212394
Kuwabara, M., Kodama, T., Ae, R., Kanbay, M., Andres-Hernando, A., Borghi, C., et al. (2023). The causality between the serum uric acid level and stroke. Hypertens. Res. 43, 354–356. doi:10.1038/s41440-019-0346-z
Li, L., Cheng, D., Yuan, Y., Zhong, L., Zhao, K., An, X., et al. (2021a). Establishment and evaluation of hyperuricemic nephropathy model induced by different doses of potassium oxanate combined with adenine in rats. West China Med. 36 (05), 643–650.
Li, S., and Bao, J. (2020). Study on treating gout from liver, spleen and kidney. Emerg. Chin. Traditional Med. 29 (12), 2228–2230.
Li, X. Y., Liu, Y., Liu, F., Chen, H. J., Yang, W. N., Yang, H. Y., et al. (2021b). Study on drug-target binding kinetics profiles of flavonoids in Chrysanthemum morifolium and xanthine oxidase. Zhongguo Zhong Yao Za Zhi 46 (7), 1822–1831. doi:10.19540/j.cnki.cjcmm.20201230.501
Li, Y., Li, H., Wang, R., Yu, Y., Liu, X., and Tian, Z. (2023). Protective effect of sodium butyrate on intestinal barrier damage and uric acid reduction in hyperuricemia mice. Biomed. Pharmacother. 161, 114568. doi:10.1016/j.biopha.2023.114568
Li, Y., Yin, C., Fan, X., Shi, D., Yao, F., and Li, C. (2022a). Antioxidant, hypoglycemic and uric acid activities of Mulberry yellow extract in vitro. Mod. food Sci. Technol. 38 (05), 71–80. doi:10.13982/j.mfst.1673-9078.2022.5.0956
Li, Z., Hui, J., Yang, P., and Mao, H. (2022b). Microfluidic organ-on-a-chip system for disease modeling and drug development. Biosensors-Basel. 12 (6), 370. doi:10.3390/bios12060370
Liang, D., Yong, T., Diao, X., Chen, S., Chen, D., Xiao, C., et al. (2021). Hypouricaemic and nephroprotective effects of Poria cocos in hyperuricemic mice by up-regulating ATP-binding cassette super-family G member 2. Pharm. Biol. 59 (1), 275–286. doi:10.1080/13880209.2021.1885450
Liang, S., Zeng, Y., Yu, Y., Li, Z., Luo, H., and Wu, Z. (2016). Discussion on uric acid lowering effect and mechanism of Simiao SAN Modified Formula based on uric acid transporter. Chin. Herb. Med. 39 (11), 2610–2614. doi:10.13863/j.issn1001-4454.2016.11.044
Lima, W. G., Martins-Santos, M. E., and Chaves, V. E. (2015). Uric acid as a modulator of glucose and lipid metabolism. Biochimie 116, 17–23. doi:10.1016/j.biochi.2015.06.025
Lin, N., Wang, Z., Liao, X., Wang, Y., and Liao, S. (2022). Research progress in the prevention and treatment of gout with Chinese medicine and food. Food drugs 24 (02), 172–177.
Lin, X. (2022). Study on mechanism of Bixiefenzhuangyin Decoction on renal Yang deficiency type hyperuricemia based on intestinal flora. Hubei: Hubei University of Chinese Medicine, 178.
Liska, D. (2021). Non-pharmacological treatment of gout. Vnitr Lek. 67, 25–28. E-2. doi:10.36290/vnl.2021.030
Liu, D., Ren, J., and Liang, M. (2017). Establishment of the cell model of hyperuricemia and its application in screening food-derived anti-hyperuricemic peptides. Mod. Food Sci. Technol. 33 (08), 72–79. doi:10.13982/j.mfst.1673-9078.2017.8.012
Liu, J., Li, Q., Liu, X., and Jiang, X. (2018). Fructose and potassium oxonate were used to establish the rat model of hyperuricemia. Genomics Appl. Biol. 37 (02), 667–674. doi:10.13982/j.mfst.1673-9078.2017.8.012
Liu, Y. (2019). Advances in pharmacology and clinical application of Pangolin in the treatment of chronic urate nephropathy. Shanxi Tradit. Chin. Med. 35 (11), 54–56.
Lu, J., Hou, X., Yuan, X., Cui, L., Liu, Z., Li, X., et al. (2018). Knockout of the urate oxidase gene provides a stable mouse model of hyperuricemia associated with metabolic disorders. Kidney Int. 93 (1), 69–80. doi:10.1016/j.kint.2017.04.031
Lu, L. (2013). Study on Xanthine oxidase inhibitory activity of Chinese herbal extract and its compound beverage in vitro. South China University of Technology, 72.
Lv, Y., Hu, Q., Wang, X., Ou, Y., and Kong, L. (2010). Effects of water extract from Ermiao Pills on uric acid imbalance and its related genes and proteins in hyperuricemia mice. Chin. Herb. Med. 41 (03), 418–423.
Lv, Y., Pan, Y., Sun, M., Yao, H., Wang, M., Du, P., et al. (2022). Effect of portulaca oleracea L. On lowering uric acid and protecting kidney. Sci. Technol. Food Industry 43 (02), 354–359. doi:10.13982/j.mfst.1673-9078.2017.8.012
Ma, L., Hu, J., Li, J., Yang, Y., Zhang, L., Zou, L., et al. (2018). Bisphenol A promotes hyperuricemia via activating xanthine oxidase. Faseb J. 32 (2), 1007–1016. doi:10.1096/fj.201700755R
Ma, T. H., Sheng, T., Tian, C. M., Xing, M. Y., Yan, L. J., and Xia, D. Z. (2019). Effect of ethanolic extract of Polygonum cuspidatum on acute gouty arthritis in mice through NLRP3/ASC/caspase-1 axis. Zhongguo Zhong Yao Za Zhi 44 (3), 546–552. doi:10.19540/j.cnki.cjcmm.20180925.001
Mandal, A. K., and Mount, D. B. (2015). The molecular physiology of uric acid homeostasis. Annu. Rev. Physiol. 77, 323–345. doi:10.1146/annurev-physiol-021113-170343
Meng, Z., Tang, Z., Yan, Y., Ding, G., Xiao, W., and Yang, Z. (2014). Study on lowering uric acid of gardeniside. World Sci. Technol. - Traditional Chin. Med. Mod. 16 (07), 1565–1568.
Morgan, S. L., and Singh, J. A. (2021). How do dietary interventions affect serum urate and gout? Nat. Rev. Rheumatol. 17 (4), 191–192. doi:10.1038/s41584-021-00576-4
Nishizawa, H., Maeda, N., and Shimomura, I. (2022). Impact of hyperuricemia on chronic kidney disease and atherosclerotic cardiovascular disease. Hypertens. Res. 45 (4), 635–640. doi:10.1038/s41440-021-00840-w
Pacher, P., Nivorozhkin, A., and Szabo, C. (2006). Therapeutic effects of xanthine oxidase inhibitors: renaissance half a century after the discovery of allopurinol. Pharmacol. Rev. 58 (1), 87–114. doi:10.1124/pr.58.1.6
Pang, M., Fang, Y., Chen, S., Zhu, X., Shan, C., Su, J., et al. (2017). Gypenosides inhibits xanthine oxidoreductase and ameliorates urate excretion in hyperuricemic rats induced by high cholesterol and high fat food (lipid emulsion). Med. Sci. Monit. 23, 1129–1140. doi:10.12659/msm.903217
Peng, A., Lin, L., Zhao, M., and Sun, B. (2019). Identifying mechanisms underlying the amelioration effect of Chrysanthemum morifolium Ramat. 'Boju' extract on hyperuricemia using biochemical characterization and UPLC-ESI-QTOF/MS-based metabolomics. Food Funct. 10 (12), 8042–8055. doi:10.1039/c9fo01821b
Peng, L. (2020). Virtual screening uric acid - lowering active ingredients of honeysuckle by molecular docking technology. Inf. Traditional Chin. Med. 37 (04), 13–18. doi:10.19656/j.cnki.1002-2406.200091
Perez-Pozo, S. E., Schold, J., Nakagawa, T., Sanchez-Lozada, L. G., Johnson, R. J., and Lillo, J. L. (2010). Excessive fructose intake induces the features of metabolic syndrome in healthy adult men: role of uric acid in the hypertensive response. Int. J. Obes. 34 (3), 454–461. doi:10.1038/ijo.2009.259
Qi, X., Wang, C., Li, F., Chen, M., Wang, Y., Li, Z., et al. (2011). Inhibitory effects of the extracts of some common vegetables on xanthine oxidase. Mod. food Sci. Technol. 27 (05), 511–514. doi:10.13982/j.mfst.1673-9078.2011.05.002
Rodevand, E., Sletvold, O., and Kvande, K. T. (2004). Side effects off allopurinol. Tidsskr. Nor. Laegeforen. 124 (20), 2618–2619.
Saag, K. G., Becker, M. A., White, W. B., Whelton, A., Borer, J. S., Gorelick, P. B., et al. (2022). Evaluation of the relationship between serum urate levels, clinical manifestations of gout, and death from cardiovascular causes in patients receiving febuxostat or allopurinol in an outcomes trial. Arthritis Rheumatol. 74 (9), 1593–1601. doi:10.1002/art.42160
Shang, J., Sun, C., and Liu, H. (2020). Establishment and evaluation of hyperuricemia rat model with spleen deficiency syndrome. J. Basic Chin. Med. 26 (08), 1076–1079.
Shen, Q., Li, H., Lei, S., Jiang, S., Li, B., Zheng, X., et al. (2019). Study on anti-trioxypurine effect and mechanism of Dendrobium candidum simiao prescription on hyperuricemia rats. Chin. J. Mod. Appl. Pharm. 36 (02), 157–163. doi:10.13748/j.cnki.issn1007-7693.2019.02.006
Shi, X., Zhu, M., Jie, L., Zhang, X., Zhang, H., and Sun, W. (2016). Effect of compound tufuling granules on physical and chemical indexes and protein levels in hyperuricemia rats with syndrome of phlegm-dampness. Chin. J. Exp. Traditional Med. Formulae 22 (15), 100–105. doi:10.13422/j.cnki.syfjx.2016150100
Shin, Q. (2015). Screening and inhibition kinetics of Xanthine oxidase inhibitors. Nanchang, China: Nanchang University, 80.
Sun, M., Liu, C., Fu, W., Tang, Y., Liu, X., Sun, J., et al. (2017). Effect and mechanism of Lycium barbarum extract on chronic hyperuricemia. Chin. Natl. Folk. Med. 26 (24), 35–37.
Tan, C. (2014). Experimental study on serum uric acid OAT1 and URAT1 mRNA expression of serum uric acid in hyperuricemia model and mouse kidney uric acid transporter with Beixiewudibi Decoction. Nanjing: Nanjing University of Chinese Medicine, 38.
Tang, S., and He, X. (2019). Effect of jiawei simiao decoction on biochemical indexes of hyperuricemia model rats with damp- heat constitution. China Pharm. 28 (16), 22–24.
Terkeltaub, R. (2006). Hyperuricemia treatment: is creatinine clearance a safer allopurinol dosing meter than plasma creatinine levels? Nat. Clin. Pract. Rheumatol. 2 (2), 68–69. doi:10.1038/ncprheum0099
Tingting, X. (2020). Development and utilization of plantain herb in hyperuricemia. Huaqiao University, 79. doi:10.27155/d.cnki.ghqiu.2020.000444
Wang, C., Li, Z., Ye, Y., Cai, F., Xiao, H., and Tang, D. (2018a). Dose analysis of acute hyperuricemia induced by potassium oxazinate in Wistar rats. Pharmacol. Clin. Chin. Med. 34 (05), 141–164. doi:10.13412/j.cnki.zyyl.2018.05.035
Wang, C., Lou, X., and Bian, Z. (2023). The scientific connotation of treating hyperuricemia from spleen in Chinese Medicine based on intestinal flora disorder. J. Hunan Univ. Chin. Med. 43 (04), 729–734.
Wang, K., Wu, S., Pan, L., Xiao, N., and Du, B. (2022). Effect of Poria Cocos on renal injury and intestinal flora in hyperuricemia rats. Food Sci. 43 (21), 171–179.
Wang, M. X., Liu, Y. L., Yang, Y., Zhang, D. M., and Kong, L. D. (2015). Nuciferine restores potassium oxonate-induced hyperuricemia and kidney inflammation in mice. Eur. J. Pharmacol. 747, 59–70. doi:10.1016/j.ejphar.2014.11.035
Wang, Q., Chen, X., Ni, Z., Gu, L., Xu, C., and Dai, H. (2018b). Fructose induces HK-2 cells to express monocyte chemoattractant protein-1 through uric acid and reactive oxygen species. J. SHANGHAI JIAO TONG Univ. Med. Sci. 38 (04), 386–393.
Wang, S., Fang, Y., Yu, X., Guo, L., Zhang, X., and Xia, D. (2019a). The flavonoid-rich fraction from rhizomes of Smilax glabra Roxb. ameliorates renal oxidative stress and inflammation in uric acid nephropathy rats through promoting uric acid excretion. Biomed. Pharmacother. 111, 162–168. doi:10.1016/j.biopha.2018.12.050
Wang, Y., Lin, Z., Zhang, B., Nie, A., and Bian, M. (2017b). Cichorium intybus L. promotes intestinal uric acid excretion by modulating ABCG2 in experimental hyperuricemia. Nutr. Metab. 14, 38. doi:10.1186/s12986-017-0190-6
Wang, Y., Lin, Z. J., Nie, A. Z., Li, L. Y., and Zhang, B. (2017a). Effect of Chinese herb chicory extract on expression of renal transporter Glut9 in rats with hyperuricemia. Zhongguo Zhong Yao Za Zhi 42 (5), 958–963. doi:10.19540/j.cnki.cjcmm.2017.0029
Wang, Z., Ci, X., Cui, T., Wei, Z., Zhang, H., Liu, R., et al. (2019b). Effects of different drug components on OAT4 and URAT1 inhibition and blood uric acid level in acute hyperuricemia mice. Chin. Herb. Med. 50 (05), 1157–1163.
Wu, D., Diao, Y., and Xu, X. (2021a). Advances in the study of high uric acid cell model. Clin. Pharmacol. Ther. China 26 (02), 236–240.
Wu, F., Jin, P., Pu, Y., and Yang, H. (2020). Study on chemical constituents of Gynostemma pentaphyllum and its protective effect on kidney. Chin. J. Med. Chem. 30 (03), 153–158. doi:10.14142/j.cnki.cn21-1313/r.2020.03.006
Wu, L., Zhang, X., Cai, Z., Chen, Y., Xiao, T., Zhou, X., et al. (2021b). Protective effects of essential oil from fructus Alpiniae zerumbet on mouse hyperuricemia. J. guangzhou Med. Univ. 46 (12), 1404–1408. doi:10.19367/j.cnki.2096-8388.2021.12.007
Xinlai, C. (2018). Therapeutic effect of Tongfeng-2 prescription on damp-heat hyperuricemia and inflammatory response in rats. Chengdu Physical Education University, 42.
Xue, X., Xu, X., Yin, C., Hu, J., Wang, L., Zou, R., et al. (2017). Experimental study on reducing uric acid and inhibiting Xanthine oxidase from different extracts of Galangal. J. Yangtze Univ. 14 (24), 1–108. doi:10.16772/j.cnki.1673-1409.2017.24.001
Xue, X., Xu, X., Yin, C., Hu, J., Wang, L., Zou, R., et al. (2018). Study on reducing uric acid effect of total flavonoids of Galangal. Hunan J. Chin. Med. 34 (02), 143–145. doi:10.16808/j.cnki.issn1003-7705.2018.02.069
Yan, B., Liu, D., Zhu, J., and Pang, X. (2019). The effects of hyperuricemia on the differentiation and proliferation of osteoblasts and vascular smooth muscle cells are implicated in the elevated risk of osteopenia and vascular calcification in gout: an in vivo and in vitro analysis. J. Cell. Biochem. 120 (12), 19660–19672. doi:10.1002/jcb.29272
Yang, G., Huang, S., Lian, X., Liu, Y., and Xie, Y. (2011). Establishment of hyperuricemia model in rats. Laboratory Animal Sci. 28 (03), 25–28.
Yang, Z., Gao, D., Guo, X., Jin, L., Zheng, J., Wang, Y., et al. (2020). Fighting immune cold and reprogramming immunosuppressive tumor microenvironment with red blood cell membrane-camouflaged nanobullets. ACS Nano 14 (12), 17442–17457. doi:10.1021/acsnano.0c07721
Ye, M. (2019). Effect of Polygonum cuspidatum on expression of uric acid transporter in renal tubules of hyperuricemia rats. Zhejiang J. Traditional Chin. Med. 54 (12), 917–919. doi:10.13633/j.cnki.zjtcm.2019.12.037
Yu, X., Zhang, L., Zhang, P., Zhi, J., Xing, R., and He, L. (2020). Lycium barbarum polysaccharides protect mice from hyperuricaemia through promoting kidney excretion of uric acid and inhibiting liver xanthine oxidase. Pharm. Biol. 58 (1), 944–949. doi:10.1080/13880209.2020.1817951
Zang, L., Liu, Z., and Wu, X. (2011). Effect of total flavones from Xituobi Decoction on uric acid absorption in vitro and its molecular mechanism. J. Chin. Med. Mater. 34 (11), 1763–1765.
Zeng, J., Bi, Y., Wei, J., Zhu, Y., Zhu, J., Wang, X., et al. (2013). The research of Plantago asiatica L herbs extracts reduce the level of uric acid in hyperuricemia mice and it’s mechanism. LISHIZHEN Med. MATERIA MEDICA Res. 24 (09), 2064–2066.
Zhang, H., Zhang, Y., Lu, G., Wang, E., and Chen, X. (2016). The puerarin impact on the expression of ABCG2 in human renal proximal tubule epithelial cells. Shanghai J. Traditional Chin. Med. 50 (03), 74–77. doi:10.16305/j.1007-1334.2016.03.023
Zhang, L., Hao, J., Jing, Y., Wan, C., Chai, J., Zhao, N., et al. (2020). Effect of Perilla leaf on hyperuricemia model mice. Jiangsu Agric. Sci. 48 (12), 156–159. doi:10.15889/j.issn.1002-1302.2020.12.033
Zhang, L., Lin, C., Huang, Q., Wu, T., and Yang, Y. (2022a). Research progress in long-term chronic hyperuricemia modeling methods and their impacts on associated complications. Life Sci. Res. 26 (02), 125–130. doi:10.16605/j.cnki.1007-7847.2021.11.0223
Zhang, Y., Jin, L., Liu, J., Wang, W., Yu, H., Li, J., et al. (2018). Effect and mechanism of dioscin from Dioscorea spongiosa on uric acid excretion in animal model of hyperuricemia. J. Ethnopharmacol. 214, 29–36. doi:10.1016/j.jep.2017.12.004
Zhang, Y., Li, Q., Wang, F., and Xing, C. (2019). A zebrafish (danio rerio) model for high-throughput screening food and drugs with uric acid-lowering activity. Biochem. Biophys. Res. Commun. 508 (2), 494–498. doi:10.1016/j.bbrc.2018.11.050
Zhang, Y., and Xu, H. (2020). Study on the mechanism of compound qiling formula granule in the treatment of hyperuricemia in rats. Chin. Mod. Appl. Pharm. 37 (15), 1825–1829. doi:10.13748/j.cnki.issn1007-7693.2020.15.005
Zhang, Y., Zhang, M., Bai, H., and Yin, X. (2022b). Clinical study on syndrome differentiation and classification of hyperuricemia. Guangming Tradit. Chin. Med. 37 (20), 3645–3649.
Zhang, Z. (2015). The fourth highest after the "three high": high uric acid health risk. Health Nutr. (04), 24–26.
Zheng, Z., and Huang, Y. (2011). Establishment of hyperuricemia model in mice. Channel Pharm. 23 (09), 27–29.
Zhu, M. (2015). Inhibitory effect of compound tuckaia granules on xanthine oxidase in hyperuricemia. Guangzhou: Guangzhou University of Traditional Chinese Medicine, 91.
Zou, C., Wang, H., Li, J., Liu, Z., Li, Y., and Ma, H. (2020). Effect of pugongying on acute hyperuricemia rats. Chin. archives traditional Chin. Med. 38 (04), 170–172. doi:10.13193/j.issn.1673-7717.2020.04.040
Zou, J., Zhang, S., Li, Y., and Wang, H. (2021). Comparative study on lowering uric acid of Psyllium and Psyllium. Gansu Sci. Technol. 50 (08), 109–111.
Keywords: hyperuricemia, traditional Chinese medicine, urate lowering, hyperuricemia models, efficacy evaluation
Citation: Zhou H, Yang J, Yuan X, Song X, Zhang X, Cao T and Zhang J (2024) Hyperuricemia research progress in model construction and traditional Chinese medicine interventions. Front. Pharmacol. 15:1294755. doi: 10.3389/fphar.2024.1294755
Received: 22 September 2023; Accepted: 15 February 2024;
Published: 07 March 2024.
Edited by:
Adolfo Andrade-Cetto, National Autonomous University of Mexico, MexicoReviewed by:
Lu Liu, Yunnan University of Chinese Medicine, ChinaCopyright © 2024 Zhou, Yang, Yuan, Song, Zhang, Cao and Zhang. This is an open-access article distributed under the terms of the Creative Commons Attribution License (CC BY). The use, distribution or reproduction in other forums is permitted, provided the original author(s) and the copyright owner(s) are credited and that the original publication in this journal is cited, in accordance with accepted academic practice. No use, distribution or reproduction is permitted which does not comply with these terms.
*Correspondence: Jiayu Zhang, emhhbmdqaWF5dTA2MTVAMTYzLmNvbQ==; Ting Cao, dGluZ2Nhb0B6anUuZWR1LmNu; Xingcai Zhang, emhhbmd4aW5nY2FpQHd0ZWFvLmNvbQ==
†These authors have contributed equally to this work
Disclaimer: All claims expressed in this article are solely those of the authors and do not necessarily represent those of their affiliated organizations, or those of the publisher, the editors and the reviewers. Any product that may be evaluated in this article or claim that may be made by its manufacturer is not guaranteed or endorsed by the publisher.
Research integrity at Frontiers
Learn more about the work of our research integrity team to safeguard the quality of each article we publish.