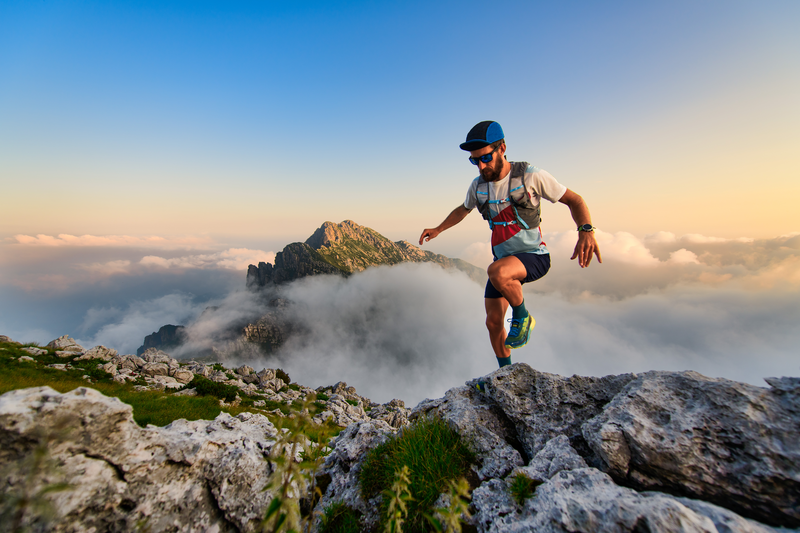
94% of researchers rate our articles as excellent or good
Learn more about the work of our research integrity team to safeguard the quality of each article we publish.
Find out more
ORIGINAL RESEARCH article
Front. Pharmacol. , 11 April 2024
Sec. Pharmacology of Infectious Diseases
Volume 15 - 2024 | https://doi.org/10.3389/fphar.2024.1282480
This article is part of the Research Topic Antimicrobial agents against multi-resistant strains View all 4 articles
Objective: FL058 is a novel beta-lactamase inhibitor with a broad spectrum of activity and a favorable safety profile. The objective of this study was to evaluate pharmacokinetic/pharmacodynamic (PK/PD) relationships for the combination of FL058 and meropenem in an in vitro infection model.
Methods: By simulating human concentration-time profiles in the in vitro model, meropenem combined with FL058 when administered 1 g/0.5 g, 1 g/1 g, 2 g/1 g, and 2 g/2 g q8h by 3-h infusion achieved approximately 2- and 4-log10 kill to KPC/OXA-producing Klebsiella pneumoniae and Escherichia coli; the combination therapy could not inhibit NDM-producing K. pneumoniae but could maintain NDM-producing E. coli around a baseline.
Results: The PK/PD indexes that best described the bacterial killing from baseline in log10 CFU/mL at 24 h were the percent time of free drug above the minimal inhibitory concentration (MIC) (%fT > MIC, MIC with FL058 at 4 mg/L) for meropenem and the percent time of free drug above 1 mg/L (%fT > 1 mg/L) for FL058. The targets for achieving a static effect and the 1- and 2-log10 kill were 74, 83, and 99 for %fT > MIC of meropenem and 40, 48, and 64 for %fT > 1 mg/L of FL058, respectively. The PK/PD index of %fT > 1 mg/L can provide a basis for evaluating clinical dosing regimens for FL058 combined with meropenem.
Conclusion: FL058 combined with meropenem might be a potential treatment for KPC- and/or OXA-48-producing Enterobacterales infection.
The extensive use of antibacterial agents has resulted in rapidly increasing drug-resistant bacteria. Against this backdrop, the detection rate of multidrug-resistant bacteria represented by Gram-negative bacilli is increasing, posing a huge challenge to anti-infective therapy in clinical practice. According to the data from CHINET (www.chinets.com), an antimicrobial surveillance network, the resistance rate of Klebsiella pneumoniae to meropenem in tertiary hospitals has increased from 2.9% in 2005 to 24.4% in 2021. For Escherichia coli, the resistance rate to meropenem reaches 1.4%–2.1%. The primary mechanism of Enterobacterales resistance to beta-lactam antibiotics is the production of beta-lactamase. Beta-lactamases are grouped into four classes according to the Ambler classification system: Class A (e.g., extended-spectrum beta-lactamases, ESBLs; and K. pneumoniae carbapenemases, KPCs), Class B (e.g., New Delhi metallo-beta-lactamases, NDMs), Class C (e.g., AmpC cephalosporinases), and Class D (e.g., oxacillinases, OXAs). A large investigational survey of carbapenem-resistant Enterobacterales (CRE) revealed that KPCs are the most prevalent beta-lactamases and NDMs are the second most prevalent beta-lactamases in K. pneumoniae (Wang et al., 2018). In recent years, OXAs have become more common in carbapenem-resistant K. pneumoniae (Tangden and Giske, 2015; Yin et al., 2017).
In consideration of the diversity of the above-mentioned beta-lactamases, researchers have paid close attention to the development of novel broad-spectrum beta-lactamase inhibitors (Shlaes, 2013; Bush, 2015; Vanscoy et al., 2016; Bhagwat et al., 2019). Presently, novel beta-lactamase inhibitors of the non-beta-lactam structure have been marketed, including avibactam, relebactam, and vaborbactam. Neither relebactam nor vaborbactam can inhibit Class D beta-lactamases. FL058 is a novel diazabicyclooctane (DBO) beta-lactamase inhibitor with a structure and activity similar to avibactam. It mainly inhibits Class A, Class C, and some Class D beta-lactamases but does not inhibit NDMs (Sharma et al., 2016). An in vitro susceptibility study (to be published) showed that, unlike avibactam, FL058 alone had certain inhibitory activity on E. coli. Meropenem combined with 4 μg/mL FL058 had a significantly lower minimal inhibitory concentration (MIC) for NDM-producing E. coli (MIC90 = 0.5 mg/L) and partial inhibitory action on NDM-producing K. pneumoniae (MIC50 = 0.25 mg/L, MIC90 = 4 mg/L). A completed phase I clinical trial showed FL058 had good safety, tolerance, and pharmacokinetic (PK) characteristics (Huang et al., 2023).
In vitro pharmacokinetic/pharmacodynamic (PK/PD) models have become important tools for screening dosing regimens for beta-lactam antibiotic/beta-lactamase inhibitor therapies (Macgowan et al., 2016; Vanscoy et al., 2016; Macgowan et al., 2017; Sabet et al., 2018). They can also be used to assess the correlation between exposure to a beta-lactam antibiotic/beta-lactamase inhibitor and changes in the colony count. The subsequent analysis of the exposure-response relationship, in turn, can support dosage selection. In light of this, this study simulated the clinical dosing regimens for FL058 combined with meropenem in an in vitro model to find the best component ratio of the two drugs and the best PK/PD index and targets for the two-drug combination therapy.
The study used eight strains of KPC-2-, NDM-1-, or OXA-48-producing Enterobacterales (six strains of K. pneumoniae and two strains of E. coli), which were provided by Microbiology Division, Institute of Antibiotics, Huashan Hospital, Fudan University.
FL058 (Qilu Pharmaceutical Co., Ltd.; Batch No.: B0220E01; purity: 98.9%) and meropenem (Sumitomo Dainippon Pharma; Batch Nos.: 2329C, 2407C, and 2408C).
The MICs of meropenem alone, FL058 alone, and two-drug combination therapy (FL058 concentration: fixed concentration of 4 μg/mL; concentration ratio of meropenem: FL058 = 1:1, 2:1, and 4:1) were determined by broth microdilution in accordance with the standard of Clinical and Laboratory Standards Institute (CLSI). Briefly, 50 μL of broth containing 5 × 105 CFU/mL bacteria were mixed with 50 μL of the drug solution in a 96-well plate in duplicate. The drug solution was either subjected to gradient dilution by a factor of two, or the dosage of FL058 was fixed at 4 μg/mL. MIC values were read with the naked eye within 16–20 h.
An in vitro PK/PD model was established to simulate drug concentration in human plasma (Liang et al., 2011; Liu et al., 2016; Bian et al., 2019). Drug-containing or blank culture media were pumped into the central compartment with a peristaltic pump under segmented control by WinLIN 3.2 (Cole-Parmer) to simulate the intravenous instillation of the drug or drug elimination process. In the study, the following PK characteristics of meropenem in healthy Chinese subjects were simulated: distribution-phase half-life (t1/2,α), 0.37 h; elimination-phase half-life (t1/2,β), 1.3 h; maximum concentration (Cmax), 23.0 mg/L (1 g of meropenem, infusion 2 h); and peak time (Tmax) at the end of infusion (Zhao et al., 2004). A Phase I clinical trial showed that FL058 in healthy Chinese subjects had an elimination half-life close to that of meropenem (Huang et al., 2003). Therefore, the concentration–time curve of FL058 was simulated similarly to that of the meropenem dosing. In the study, the Cmax of FL058 was 42.7 mg/L (1 g of FL058, infusion 2 h). The simulation of the dosing regimens of FL058 combined with meropenem based on the in vitro PK/PD model is shown in Table 1. Culture media samples were collected before dosing and after 0.5 h, 1 h, 1.5 h, 2 h (immediately at the end of the instillation), 2.5 h, 3 h, 4 h, 6 h, 8 h, 10 h, 16 h, 18 h, and 24 h and stored at −70°C. The concentrations of meropenem and FL058 were determined by liquid chromatography-tandem mass spectrometry (LC-MS/MS) with an Atlantis®T3, 3 μm, 2.1 × 100 mm column, using 0.1% formic acid-8 mM sodium acetate in water and 0.1% formic acid–8 mM sodium acetate in 90% acetonitrile as mobile phases. The flow rate of the mobile phases was 0.6 mL/min. Both meropenem and FL058 had a linearity range of 0.05–50.0 mg/L.
A single colony on an agar plate was selected and inoculated into the broth for culture overnight on the shaker, and then the bacterial culture liquid was diluted to ca. 0.5 McFarland Standard (ca. 108 CFU/mL); 1.8 mL of the dilution was injected into the central compartment (180 mL) and precultured for 1 h before being inoculated into the in vitro model. A 1 mL aliquot of the bacterial culture fluid was sampled from the central compartment at preset time points (before dosing and 1 h, 2 h, 4 h, 6 h, 8 h, 10 h, 16 h, 18 h, and 24 h after dosing) with a sterile injector; 10 μL of the diluted solution or the bulk bacterial culture fluid was placed on agar plates for 18–24 h of culture, then subjected to colony counting. The lower limit of colony count was 100 CFU/mL.
The measured concentration data were fitted to targeted concentration data by linear fitting (weighed 1/Y) with the Linear module in Phoenix WinNonlin (version 8.1, Certara) to determine their relationship. The PK parameters of meropenem and FL058 in the in vitro model were calculated using the NCA and PK modules in Phoenix WinNonlin, including area under the concentration–time curve from 0 to 24 h (fAUC24, f denotes free drug), maximum concentration (fCmax), f%T > CT (threshold concentration, CT: 0.25, 1, and 4 mg/L), and f%T > MIC. The correlation between the PK/PD indexes and the change from colony count baseline at 24 h was analyzed with the sigmoid Emax model in the PD module in Phoenix WinNonlin. The PK/PD indexes selected for FL058 were fAUC24 and f%T > CT in a fixed dosage regimen of meropenem (q8h by 2-h infusion). The PK/PD indexes selected for meropenem were fAUC24/MIC, fCmax/MIC, and f%T > MIC (MIC for FL058 at a fixed concentration of 4 mg/L) in a meropenem-to-FL058 dose ratio of 2:1 (Lepak et al., 2019). Dosing regimens of FL058 and meropenem for PK/PD analysis are shown in Supplementary Table S1. A larger R2 and a smaller coefficient of variation indicated a better PK/PD index.
The MICs for FL058 and meropenem alone and in combination are shown in Table 2; all strains were resistant to meropenem (MICs ≥8 mg/L). FL058 restored the susceptibility of K. pneumoniae and E. coli to meropenem, and FL058 monotherapy had certain activity against ATCC BAA-1705 and ATCC BAA-2452. The MICs were apparently lower when the concentration of FL058 was fixed at 4 μg/mL, at which concentration the MIC of meropenem decreased by a factor of 8–512.
Table 2. Susceptibility testing results of meropenem alone, FL058 alone, or meropenem combined with FL058 against K. pneumoniae and E. coli.
Figure 1 shows the linear fitting of the observed concentrations to the targeted concentrations of the in vitro PK model. Meropenem’s linear fitting equation was y = 0.988x+0.0, and weighed R = 0.994. The linear fitting equation for FL058 was y = 1.13x+0.0, and weighed R = 0.996, suggesting that the PK profiles in the in vitro model can satisfactorily match the PK profiles of meropenem and FL058 in humans.
Figure 1. Accuracy of observed concentration of (A) meropenem and (B) FL058 vs. targeted concentration (open blue circles denote observed concentration points, solid red line denotes the fitted straight line, dashed black line denotes the 45° line).
Time–killing curves of FL058 combined with meropenem (q8h) and no-treatment control groups against K. pneumoniae are shown in Figure 2. All dose regimens quickly killed the bacteria in 0–2 h, but at 8 h (the trough concentration point), rebound growth of bacteria was frequently observed. This was most evident with the 1 g/0.125g, 1 g/0.25 g, and 2 g/0.25 g (meropenem/FL058) combinations. Curves showing the killing of two strains of NDM-producing K. pneumoniae and one strain of NDM-producing E. coli by FL058 combined with meropenem are shown in Figure 3. Four dosage combinations for meropenem/FL058 (1 g/0.5 g, 1 g/1 g, 2 g/1 g, and 2 g/2 g) had a killing effect on two strains of NDM-producing K. pneumoniae (17-R1-95 and 17-R2-27) in 2 h, but the bacteria resumed growth starting from 3 to 4 h postdose, and the growth continued. By 24 h, the bacterial load exceeded the baseline. In 3 h, all six dosage regimens for FL058 combined with meropenem killed approximately 2-log10 CFU/mL of NDM-producing E. coli ATCC BAA-2452. The bacterium resumed growth starting from 4 to 8 h, but these combinations maintained an inhibitory effect in 8–24 h. Time–killing curves of FL058 combined with meropenem against KPC- or OXA-producing K. pneumoniae and KPC-producing E. coli are shown in Supplementary Figure S1.
Figure 2. Time–killing curves of meropenem in combination with FL058 q8h by a 2-h infusion against three KPC-producing K. pneumoniae. (A) K. pneumoniae ATCC BAA-1705, (B) K. pneumoniae 17-R1-16, and (C) K. pneumoniae 17-R1-38. Tick marks on the x-axis are the start of each infusion. KPN: K. pneumoniae.
Figure 3. Time–killing curves of meropenem in combination with FL058 q8h by a 2-h infusion against NDM-producing Enterobacterales: (A) E. coli ATCC BAA-2452, (B) K. pneumoniae 17-R1-95, and (C) K. pneumoniae 17-R2-27. Tick marks on the x-axis are the start of each infusion. KPN: Klebsiella pneumoniae, ECO: Escherichia coli.
FL058 cannot inhibit NDMs, which can hydrolyze meropenem rapidly (Ma et al., 2019). Therefore, the three strains of NDM-producing bacteria (ATCC BAA-2452, 17-R1-95, and 17-R2-27) were excluded from the PK/PD analysis. Sigmoid Emax model fitting results of the correlation between the PK/PD indexes of FL058 and change from baseline in colony count at 24 h in the in vitro model are shown in Figure 4. The estimated values of model parameters are shown in Table 3. The percent time of free drug above 1 mg/L (% fT > 1 mg/L), %fT > 4 mg/L, and area under the concentration–time curve from 0 to 24 h (fAUC) of FL058 showed a good fit (R = 0.896). With the smallest coefficient of variation (CV) of EC50 (the concentration of the drug that gives half-maximal response), %fT > 1 mg/L is the most efficacy-related PK/PD index of FL058. The PK/PD targets of FL058 are shown in Table 4. FL058 %fT >1 mg/L combined with meropenem achieved a static effect, and the 1- and 2-log10 kills in the colony count were 40, 48, and 64, respectively.
Figure 4. Relationships between the PK/PD indexes of FL058 in combination with meropenem and the change from baseline in colony count at 24 h. (A) %f T > 0.25 mg/L, percent time of the dosing interval that the free drug concentration is above 0.25 mg/L; (B) %f T > 1 mg/L; (C) %f T > 4 mg/L; (D) f AUC, area under the concentration–time curve from time 0 to 24 h, f denotes free drug. Open red circles denote observations; solid blue lines denote Emax model predictions.
Sigmoid Emax model fitting results of the correlation between the PK/PD indexes of meropenem and change from baseline in colony count at 24 h in the in vitro model are shown in Figure 5. The estimated model parameter values are shown in Table 3. The best-fitting result was the percent time of free drug above MIC (%fT > MIC, MIC with FL058 at 4 mg/L) (R = 0.931), suggesting that the PK/PD index of meropenem remained %fT > MIC in combination with FL058 (Abdul-Aziz et al., 2015). The PK/PD targets of meropenem are shown in Table 4. %fT > MIC for meropenem combined with FL058 at a dose ratio of 2:1 achieved a static effect, and the 1- and 2-log10 kill in colony count values were 74, 83, and 99, respectively.
Figure 5. Relationships between the PK/PD indexes of meropenem in combination with FL058 and the change from baseline in colony count at 24 h. (A) f AUC/MIC, area under the concentration–time curve from 0 to 24 h over MIC; (B) f Cmax/MIC, maximum concentration over MIC; (C) %f T > MIC, percent time of the dosing interval that the free drug concentration is above MIC. f denotes free drug. Open red circles denote observations; solid blue lines denote Emax model predictions.
New beta-lactam antibiotic/beta-lactamase inhibitor combinations are being developed for clinical trials in response to the rapid global increase of beta-lactamase-producing Enterobacterales. It is necessary to fully understand the PK/PD characteristics of these combinations. The results from this study showed that for FL058 combined with meropenem, the best PK/PD index was %fT > CT, coinciding with avibactam. For relebactam and CB-618, which are structurally similar to FL058, the best PK/PD index was fAUC/MIC (Mavridou et al., 2015; Crass and Pai, 2019). The PK/PD index is %fT > CT for beta-lactamase inhibitors of other structures such as tazobactam and enmetazobactam (Melchers et al., 2016; Bernhard et al., 2020), AUC for ANT2681 (Das et al., 2020), and fAUC/MIC for vaborbactam and taniborbactam (Griffith et al., 2019; Abdelraouf et al., 2020). More in-depth studies are needed to determine the best PK/PD index for FL058 in animal infection models or human patients.
In the in vitro model, FL058 0.5 g q8h infusion 2-h dose regimen had a %f T > 1 mg/L of 70%, which was greater than the target value of 64% needed for a 2-log10 kill. At an FL058 dosage greater than 0.5 g, the bacteria’s rebound growth at the trough concentration would be suppressed (Figure 2), suggesting the lowest effective dose (LED) of FL058 might be 0.5 g q8h infusion 2 h. This LED is on par with avibactam, a drug in the same class as FL058. The study data from meropenem administered at a daily dose of 3 g or 6 g (q8h) were subjected to pooled analysis to get the best PK/PD index and targets for FL058 because the grouped analysis of meropenem administered at a daily dose of 3 g was much closer to that of 6 g (Supplementary Figure S2). This finding coincides with the result that meropenem showed a comparable bactericidal effect at daily doses of 3 g and 6 g (Figure 2; Figure 3).
None of the currently market-available beta-lactam antibiotic/beta-lactamase inhibitor combinations can effectively treat NDM-producing Enterobacterales. Although ceftazidime/avibactam may be used in combination with aztreonam, the combination of these three drugs is of lower cost-effectiveness and higher safety concern. In contrast to avibactam and relebactam, FL058 alone in an in vitro susceptibility test showed some inhibitory activity against NDM-producing E. coli and, when used in combination with meropenem, had little inhibitory activity against NDM-producing K. pneumoniae (pending publication). In the in vitro model studies, however, FL058, in combination with meropenem, failed to suppress NDM-producing K. pneumoniae but did partially suppress NDM-producing E. coli. The possible reasons might be as follows: ① FL058 alone had some activity against E. coli, and its diazo heterocyclic ring structure was not hydrolyzed by NDMs, as shown in Supplementary Figure S3B. When E. coli ATCC BAA-2452 was cultured with FL058, the concentration of FL058 in the central compartment was consistent with the predicted concentration. ② Meropenem could be hydrolyzed by NDMs, but its concentration remained at a certain level in the central compartment where E. coli ATCC BAA-2452 was cultured, as shown in Supplementary Figure S3A. Moreover, the hydrolysis rate of beta-lactam antibiotics under the action of beta-lactamase had a positive correlation with the bacterial load (Kristoffersson et al., 2019). ③ When the two drugs were co-administered, their MIC against E. coli was low. ④ In the central compartment where NDM-producing K. pneumoniae was cultured, meropenem was almost completely hydrolyzed by NDMs, and its peak concentration was below the lower limit of detection. A current study of a mouse thigh infection model showed that FL058, in combination with meropenem at a level equivalent to 1 g/1 g q8h in humans, had close to a 2-log10 kill against NDM-producing K. pneumoniae. Meropenem has a broad antibacterial spectrum that covers multiple bacterial infections. Moreover, its bactericide activity against aerobic Gram-positive bacteria and anaerobic bacteria is significantly higher than that of aztreonam. Therefore, it is expected that this combination has a higher potential than the combination of aztreonam/avibactam, which is under development.
There were some limitations in this study. The killing curves of meropenem alone and FL058 alone against carbapenemase-producing Enterobacterales (CPE) were not assessed in the study because we believed it is unlikely that either of these monotherapies could be used for the treatment of an infection caused by CPE in clinical practice. The PK/PD index of meropenem was assessed when co-administered with FL058 in the ratio of 2:1, not in the ratio of 1:1. Subsequent studies will be carried out to further investigate the bactericide activity of FL058 in combination with meropenem against NDM-producing E. coli.
In summary, the best PK/PD index for FL058 in combination with meropenem in vitro model was %f T > 1 mg/L. The target of this index for achieving 2-log10 kill was 64%, which could provide a basis for assessment of the clinical dosing regimens of FL058 combined with meropenem. The lowest effective dose of meropenem/FL058 against KPC-/OXA-producing K. pneumoniae and E. coli was probably 1 g/0.5 g q8h infusion 2 h. Moreover, FL058 and meropenem might be a potential treatment for KPC- and/or OXA-48-producing Enterobacterales infection.
The raw data supporting the conclusions of this article will be made available by the authors, without undue reservation.
ZH: writing–original draft, visualization, software, methodology, investigation, formal analysis, and data curation. XB: writing–original draft, methodology, formal analysis, and data curation. YL: writing–original draft, investigation, formal analysis, and data curation. JH: writing–original draft, investigation, and data curation. BG: writing–review and editing, supervision, project administration, and conceptualization. XY: writing–original draft, resources, and data curation. YJ: writing–original draft, resources, and data curation. SZ: writing–review and editing, supervision, funding acquisition, and conceptualization. XW: writing–review and editing, and conceptualization. CG: writing–review and editing, and conceptualization. JZ: writing–review and editing, supervision, resources, and conceptualization. XW: writing–review and editing, supervision, resources, project administration, and conceptualization.
The author(s) declare financial support was received for the research, authorship, and/or publication of this article. This study was funded by the Major Research and Development Project of Innovative Drugs, Ministry of Science and Technology of China (2017ZX09304005). The authors declare that this study received funding from Qilu Pharmaceutical Co., Ltd. The funder was not involved in the study design, collection, analysis, interpretation of data, the writing of this article, or the decision to submit it for publication.
We thank Wang Liang, Luyue JZ, Kai Ma, and other staff of Shanghai Junji Medical Laboratory Co., Ltd., for their assistance with in vitro experiments.
Authors SZ, XW, and CG were employed by Qilu Pharmaceutical Co Ltd.
The remaining authors declare that the research was conducted in the absence of any commercial or financial relationships that could be construed as a potential conflict of interest.
All claims expressed in this article are solely those of the authors and do not necessarily represent those of their affiliated organizations, or those of the publisher, the editors, and the reviewers. Any product that may be evaluated in this article, or claim that may be made by its manufacturer, is not guaranteed or endorsed by the publisher.
The Supplementary Material for this article can be found online at: https://www.frontiersin.org/articles/10.3389/fphar.2024.1282480/full#supplementary-material
Abdelraouf, K., Almarzoky, A. S., and Nicolau, D. P. (2020). In vivo pharmacodynamics of new-generation β-lactamase inhibitor taniborbactam (formerly VNRX-5133) in combination with cefepime against serine-β-lactamase-producing Gram-negative bacteria. J. Antimicrob. Chemother. 75 (12), 3601–3610. doi:10.1093/jac/dkaa373
Abdul-Aziz, M. H., Lipman, J., Mouton, J. W., Hope, W. W., and Roberts, J. A. (2015). Applying pharmacokinetic/pharmacodynamic principles in critically ill patients: optimizing efficacy and reducing resistance development. Semin. Respir. Crit. Care Med. 36 (1), 136–153. doi:10.1055/s-0034-1398490
Bernhard, F., Odedra, R., Sordello, S., Cardin, R., Franzoni, S., Charrier, C., et al. (2020). Pharmacokinetics-pharmacodynamics of enmetazobactam combined with cefepime in a neutropenic murine thigh infection model. Antimicrob. Agents Chemother. 64 (6), e00078-20. doi:10.1128/AAC.00078-20
Bhagwat, S. S., Periasamy, H., Takalkar, S. S., Palwe, S. R., Khande, H. N., and Patel, M. V. (2019). The novel β-lactam enhancer zidebactam augments the in vivo pharmacodynamic activity of cefepime in a neutropenic mouse lung acinetobacter baumannii infection model. Antimicrob. Agents Chemother. 63 (4), e02146-18. doi:10.1128/AAC.02146-18
Bian, X., Liu, X., Chen, Y., Chen, D., Li, J., and Zhang, J. (2019). Dose optimization of colistin combinations against carbapenem-resistant acinetobacter baumannii from patients with hospital-acquired pneumonia in China by using an in vitro pharmacokinetic/pharmacodynamic model. Antimicrob. Agents Chemother. 63 (4), e01989-18. doi:10.1128/AAC.01989-18
Bush, K. (2015). A resurgence of β-lactamase inhibitor combinations effective against multidrug-resistant Gram-negative pathogens. Int. J. Antimicrob. Agents. 46 (5), 483–493. doi:10.1016/j.ijantimicag.2015.08.011
Crass, R. L., and Pai, M. P. (2019). Pharmacokinetics and pharmacodynamics of β-lactamase inhibitors. Pharmacotherapy 39 (2), 182–195. doi:10.1002/phar.2210
Das, S., Johnson, A., Mcentee, L., Farrington, N., Kirby, A., Unsworth, J., et al. (2020). Pharmacodynamics of the novel metallo-β-lactamase inhibitor ANT2681 in combination with meropenem for the treatment of infections caused by NDM-producing enterobacteriaceae. Antimicrob. Agents Chemother. 64 (11), e01076-20. doi:10.1128/AAC.01076-20
Griffith, D. C., Sabet, M., Tarazi, Z., Lomovskaya, O., and Dudley, M. N. (2019). Pharmacokinetics/pharmacodynamics of vaborbactam, a novel beta-lactamase inhibitor, in combination with meropenem. Antimicrob. Agents Chemother. 63 (1), e01659-18. doi:10.1128/AAC.01659-18
Huang, Z., Yang, X., Jin, Y., Yu, J., Cao, G., Wang, J., et al. (2023). First-in-human study to evaluate the safety, tolerability, and population pharmacokinetic/pharmacodynamic target attainment analysis of FL058 alone and in combination with meropenem in healthy subjects. Antimicrob. Agents Chemother. 68, e0133023. doi:10.1128/aac.01330-23
Kristoffersson, A. N., Bissantz, C., Okujava, R., Haldimann, A., Walter, I., Shi, T., et al. (2019). A novel mechanism-based pharmacokinetic-pharmacodynamic (PKPD) model describing ceftazidime/avibactam efficacy against β-lactamase-producing Gram-negative bacteria. J. Antimicrob. Chemother. 75, 400–408. doi:10.1093/jac/dkz440
Lepak, A. J., Zhao, M., and Andes, D. R. (2019). WCK 5222 (Cefepime/Zidebactam) pharmacodynamic target analysis against metallo-β-lactamase producing enterobacteriaceae in the neutropenic mouse pneumonia model. Antimicrob. Agents Chemother. 63, 01648–1719. doi:10.1128/AAC.01648-19
Liang, W., Liu, X. F., Huang, J., Zhu, D. M., Li, J., and Zhang, J. (2011). Activities of colistin- and minocycline-based combinations against extensive drug resistant Acinetobacter baumannii isolates from intensive care unit patients. BMC Infect. Dis. 11, 109. doi:10.1186/1471-2334-11-109
Liu, X., Zhao, M., Chen, Y., Bian, X., Li, Y., Shi, J., et al. (2016). Synergistic killing by meropenem and colistin combination of carbapenem-resistant Acinetobacter baumannii isolates from Chinese patients in an in vitro pharmacokinetic/pharmacodynamic model. Int. J. Antimicrob. Agents. 48 (5), 559–563. doi:10.1016/j.ijantimicag.2016.07.018
Ma, B., Fang, C., Lu, L., Wang, M., Xue, X., Zhou, Y., et al. (2019). The antimicrobial peptide thanatin disrupts the bacterial outer membrane and inactivates the NDM-1 metallo-β-lactamase. Nat. Commun. 10 (1), 3517. doi:10.1038/s41467-019-11503-3
Macgowan, A., Tomaselli, S., Noel, A., and Bowker, K. (2017). The pharmacodynamics of avibactam in combination with ceftaroline or ceftazidime against β-lactamase-producing Enterobacteriaceae studied in an in vitro model of infection. J. Antimicrob. Chemother. 72 (3), 762–769. doi:10.1093/jac/dkw480
Macgowan, A. P., Noel, A. R., Tomaselli, S. G., Nicholls, D., and Bowker, K. E. (2016). Pharmacodynamics of ceftolozane plus tazobactam studied in an in vitro pharmacokinetic model of infection. Antimicrob. Agents Chemother. 60 (1), 515–521. doi:10.1128/AAC.00727-15
Mavridou, E., Melchers, R. J., van Mil, A. C., Mangin, E., Motyl, M. R., and Mouton, J. W. (2015). Pharmacodynamics of imipenem in combination with β-lactamase inhibitor MK7655 in a murine thigh model. Antimicrob. Agents Chemother. 59 (2), 790–795. doi:10.1128/AAC.03706-14
Melchers, M. J., Mavridou, E., van Mil, A. C., Lagarde, C., and Mouton, J. W. (2016). Pharmacodynamics of ceftolozane combined with tazobactam against enterobacteriaceae in a neutropenic mouse thigh model. Antimicrob. Agents Chemother. 60 (12), 7272–7279. doi:10.1128/AAC.01580-16
Sabet, M., Tarazi, Z., Rubio-Aparicio, D., Nolan, T. G., Parkinson, J., Lomovskaya, O., et al. (2018). Activity of simulated human dosage regimens of meropenem and vaborbactam against carbapenem-resistant enterobacteriaceae in an in vitro hollow-fiber model. Antimicrob. Agents Chemother. 62 (2), e01969-17. doi:10.1128/AAC.01969-17
Sharma, R., Park, T. E., and Moy, S. (2016). Ceftazidime-avibactam: a novel cephalosporin/β-lactamase inhibitor combination for the treatment of resistant gram-negative organisms. Clin. Ther. 38 (3), 431–444. doi:10.1016/j.clinthera.2016.01.018
Shlaes, D. M. (2013). New β-lactam-β-lactamase inhibitor combinations in clinical development. Ann. N.Y. Acad. Sci. 1277, 105–114. doi:10.1111/nyas.12010
Tangden, T., and Giske, C. G. (2015). Global dissemination of extensively drug-resistant carbapenemase-producing Enterobacteriaceae: clinical perspectives on detection, treatment and infection control. J. Intern. Med. 277 (5), 501–512. doi:10.1111/joim.12342
Vanscoy, B. D., Trang, M., Mccauley, J., Conde, H., Bhavnani, S. M., Friedrich, L. V., et al. (2016). Pharmacokinetics-pharmacodynamics of a novel β-lactamase inhibitor, CB-618, in combination with meropenem in an in vitro infection model. Antimicrob. Agents Chemother. 60 (7), 3891–3896. doi:10.1128/AAC.02943-15
Wang, Q., Wang, X., Wang, J., Ouyang, P., Jin, C., Wang, R., et al. (2018). Phenotypic and genotypic characterization of carbapenem-resistant enterobacteriaceae: data from a longitudinal large-scale CRE study in China (2012-2016). Clin. Infect. Dis. 67 (2), S196–S205. doi:10.1093/cid/ciy660
Yin, D., Dong, D., Li, K., Zhang, L., Liang, J., Yang, Y., et al. (2017). Clonal dissemination of OXA-232 carbapenemase-producing Klebsiella pneumoniae in neonates. Antimicrob. Agents Chemother. 61 (8), e00385-17. doi:10.1128/AAC.00385-17
Keywords: beta-lactamase inhibitor, meropenem, in vitro model, pharmacokinetics/pharmacodynamics, carbapenemase-producing Enterobacterales
Citation: Huang Z, Bian X, Li Y, Hu J, Guo B, Yang X, Jin Y, Zheng S, Wang X, Gao C, Zhang J and Wu X (2024) In vitro pharmacokinetics/pharmacodynamics of FL058 (a novel beta-lactamase inhibitor) combined with meropenem against carbapenemase-producing Enterobacterales. Front. Pharmacol. 15:1282480. doi: 10.3389/fphar.2024.1282480
Received: 24 August 2023; Accepted: 12 March 2024;
Published: 11 April 2024.
Edited by:
Matar Seck, Cheikh Anta Diop University, SenegalReviewed by:
David Paterson, The University of Queensland, AustraliaCopyright © 2024 Huang, Bian, Li, Hu, Guo, Yang, Jin, Zheng, Wang, Gao, Zhang and Wu. This is an open-access article distributed under the terms of the Creative Commons Attribution License (CC BY). The use, distribution or reproduction in other forums is permitted, provided the original author(s) and the copyright owner(s) are credited and that the original publication in this journal is cited, in accordance with accepted academic practice. No use, distribution or reproduction is permitted which does not comply with these terms.
*Correspondence: Xiaojie Wu, d3V4aWFvamllQGZ1ZGFuLmVkdS5jbg==; Jing Zhang, emhhbmdqX2Z1ZGFuQGFsaXl1bi5jb20=
Disclaimer: All claims expressed in this article are solely those of the authors and do not necessarily represent those of their affiliated organizations, or those of the publisher, the editors and the reviewers. Any product that may be evaluated in this article or claim that may be made by its manufacturer is not guaranteed or endorsed by the publisher.
Research integrity at Frontiers
Learn more about the work of our research integrity team to safeguard the quality of each article we publish.