- 1Department of Pharmacognosy, School of Pharmacy, Mount Kenya University, Thika, Kenya
- 2Department of Medical Biochemistry, Mount Kenya University, Thika, Kenya
- 3Department of Biochemistry, Microbiology, and Biotechnology, Kenyatta University, Nairobi, Kenya
- 4School of Pharmacy, Kabarak University, Nakuru, Kenya
- 5Department of Pharmacy, Kisii University, Kisii, Kenya
Although medicinal plants have been used by ethnic communities since ancient times to prevent and treat various diseases, only a few have been scientifically documented. Therefore, due to their rare availability and lack of comprehensive scientific information, we reviewed the ethnomedicinal uses, phytochemistry, and pharmacological activities of plants within the genus Sarcophyte. To do this, we used specific search terms and phrases to retrieve relevant information from online sources published in English from 2000 to July 2023. The results showed that there are only two plants in the genus Sarcophyte (Sarcophyte sanguinea Sparrm. and Sarcophyte piriei Hutch.), which are traditionally used to treat a wide range of diseases, especially cancer, and skin, gastrointestinal, and urinogenital tract ailments in humans, and to cure animals in ethnoveterinary practices. It was noted that 13 secondary metabolites have been isolated from the two plants, the most prominent of which are flavonoids (diinsininol, diinsinin, and naringenin). The antioxidant activity of S. piriei is reported based on the scavenging of 2,2-diphenyl-1-picrylhydrazyl (DPPH) (IC50: 4.26 ± 0.22 μg/mL) and 2 -2′-Azino-di-[3-ethylbenzthiazoline sulfonate (ABTS) radicals (IC50: 4.62 ± 0.14 μg/mL), chelating iron (IC50: 1.82 ± 0.01 μg/mL, 3.50 ± 0.09 μg/mL), and nitric oxide (IC50: 9.97 ± 0.88 μg/mL, 9.09 ± 0.11 μg/mL). The methanolic stem extracts of S. piriei possess antimicrobial activity against Staphylococcus aureus, Escherichia coli, Klebsiella pneumoniae, Vibrio fluvialis, and Enterococcus avium, with minimum inhibitory concentration (MIC) values ranging from 0.16 to 0.625 mg/mL, and a minimum bactericidal concentration (MBC) of 1.25 to 5 mg/mL. Cytotoxic effects of the extracts from the two plant species were also demonstrated. Sarcophyte piriei possesses therapeutic potential as evidenced by the inhibitory effects of the aqueous rhizome extract on edema (1,000 mg/kg) and prostaglandin synthesis (IC50 = 0.2 mg/mL). In addition, diinsininol and diinsinin were isolated from S. sanguinea inhibited prostaglandin synthesis (IC50: 9.20 µM, 13.14 µM) and platelet-activating factor-induced exocytosis. Therefore, based on this review, further scientific research is needed to demystify the links between traditional medicinal uses, various secondary metabolites, and the pharmacology of the two plants.
1 Background
The use of medicinal plants as therapeutic agents for human and animal diseases is an extensive and diverse phenomenon, demonstrating their beneficial role in meeting primary healthcare needs (Jamaddar et al., 2023). Moreover, medicinal plants play a significant role in the conventional healthcare system as they are a valuable source of various allopathic drugs currently used to treat diseases (Dey et al., 2021). Considering the high cost of conventional healthcare, especially in low- and middle-income countries (LIMCs), the higher efficacy in managing various diseases (Ekor, 2014; Patrício et al., 2022), and the cultural acceptability of botanical drugs, traditional medicine still plays an important role worldwide (Dey et al., 2021; Siddique et al., 2021; Ndhlovu et al., 2023).
The vast therapeutic potential of medicinal plants has attracted immense research interest from the scientific community to unearth alternative effective lead compounds for drug development, especially against devastating diseases (Abdalla and Mühling, 2019; Swamy, 2020; Salama et al., 2021). It is now well recognized that the pharmacological effects of medicinal plants are mediated by various bioactive secondary metabolites (Swamy, 2020). Consequently, research has demystified the pharmacological role of various phytochemicals in preventing, slowing down, or averting the pathogenesis of many diseases (Howes and Perry, 2011; Gaikwad et al., 2014; Howes, 2017; Ahmad et al., 2020; Moriasi et al., 2020a; Moriasi et al., 2021a). For instance, antioxidant-associated phytochemicals quench oxidative stress in the body, thereby preventing undesirable sequelae (Moriasi et al., 2020b). Amelioration of oxidative stress is key to preventing and reversing associated diseases such as diabetes mellitus, neurodegeneration, cancer, and metabolic syndrome, among others (Moriasi et al., 2020b; Moriasi et al., 2021b). Therefore, certain phytochemicals in medicinal plants can help predict the efficacy and potency of that plant against specific or a range of diseases.
The existence of traditional medicine depends primarily on the diversity of medicinal plants and the associated ethnomedicinal information on their preparation and use (Josephine Ozioma and Antoinette Nwamaka Chinwe, 2019). Thus, medicinal plants are an indispensable resource for maintaining the health and overall wellbeing of people and animals in various ethnic groups worldwide (WHO, 2013; Josephine Ozioma and Antoinette Nwamaka Chinwe, 2019; Okot et al., 2020). The popularity and high dependency on traditional medicine, especially in sub-Saharan Africa (James et al., 2018), denote its significant role in healthcare. However, rapidly increasing human populations, urbanization, climate change, and habitat destruction are harming essential medicinal plants and their resources (Okello and Kiringe, 2004; Kamau et al., 2016; Razgour et al., 2020). To address this, it is imperative to carefully investigate and document the ethnomedicinal information, pharmacological activities, and phytochemistry of promising plants to preserve the knowledge and facilitate further research aimed at valorizing their efficacy as potential sources of new drugs and to support conservation programs (Kamau et al., 2016; Kigen et al., 2014; Haque et al., 2022; Hmidouche et al., 2023). Accordingly, we reviewed the local and traditional medicinal uses, pharmacological activities, and phytochemistry of plants within the genus Sarcophyte because of the lack of comprehensive scientific information and because they are rarely encountered.
The Sarcophyte genus belongs to the plant family Balanophoraceae and the order Santalales (sandalwood), according to the Angiosperm Phylogeny Group IV classification system (Angiosperm Phylogeny Group, 2016; Nickrent, 2020). The family comprises 18 genera with over 100 species of root holoparasitic geophytes with a pantropical distribution (Dennis et al., 2023). The genus Sarcophyte comprises a single species of two plants: Sarcophyte sanguinea Sparrm.) and Sarcophyte piriei (Hutch.). The two plants are native to eastern and southern Africa, but they are rarely encountered due to their patchy distribution, leading to inadequate sampling, poor preservation, and insufficient research (Maroyi, 2017).
The two plants are used in traditional medicine to treat cancer, snake bites, and disorders of the respiratory, gastrointestinal, integumentary, reproductive, and nervous systems (Ogundaini et al., 1996; Muriuki, 2011; De Wet et al., 2013; Naidoo et al., 2013; Maroyi, 2017). However, there is a paucity of comprehensive scientific literature on the ethnomedicinal uses, phytochemistry, and pharmacological activities of plants of the genus Sarcophyte. Thus, sufficient scientific reports on the genus Sarcophyte are needed to help appraise its ethnomedicinal, pharmacological, and phytochemical value.
Therefore, this review provides a comprehensive account of the plants’ ethnomedicinal uses, phytochemistry, and pharmacological activities of the two plants of the genus Sarcophyte. This review further highlights the existing research gaps and potential scientific opportunities that may significantly contribute to the valorization of the investigated plants as sources of alternative therapeutic agents for the treatment of various diseases.
2 Methodology
We retrieved the relevant literature on the ethnobotanical uses, phytochemistry, and pharmacological activities of the genus Sarcophyte published from 2000 to July 2023 from Google Scholar, Wiley Online Library, Web of Science, PubMed, SCOPUS, SpringerLink, SciFinder, and Science Direct, using specific search terms and phrases such as “Sarcophyte,” “Pharmacologic activity of Sarcophyte,” “Taxonomy of Sarcophyte,” “distribution/diversity of Sarcophyte,” “phytochemistry of Sarcophyte,” and “ethnomedicinal uses of Sarcophyte.” Additionally, an extensive search and analysis of traditional medicinal uses, phytochemistry, and pharmacological activities of plant species belonging to the genus Sarcophyte was performed using published articles, journals, Ph.D. and MSc. dissertations, conference papers, available data from herbaria, and books published in English. The materials were carefully screened, and only complete and relevant information was further evaluated and included in this review. Information on the worldwide distribution of plant species in the genus Sarcophyte was obtained from online databases, including JSTOR Global Plants (JGP) (JSTOR, 2022a), World Flora Online (WFO) (World Flora Database, 2022), Prelude Medicinal Plants (PMP) (Africamuseum, 2022), African Plant Database (APD) (APD, 2022), and Global Biodiversity Information Facility (GBIF) (GBIF, 2022). Species details recorded included collector, accepted name, species number, occurrence, and the herbarium. Species names were confirmed using the International Plant Name Index (IPNI) (IPNI, 2022) and the taxonomic data in the WFO database (IPNI, 2022). The PubChem database was used to verify the IUPAC names of secondary metabolites isolated from plants of the genus Sarcophyte, and their chemical structures were drawn using ChemBio Draw Ultra, version 14.0.
3 Botanical description
Plants of the Sarcophyte genus are characterized by their unique botanical features, as currently described in the World Flora Online (WFO) database (World Flora Online, 2022). Plants of this genus are perennial polyparasitic herbs that grow up to 40 cm long and attach themselves to the roots of various host plants, especially those of the Mimosaceae family. They form a large, warty, irregularly lobed tuber measuring 5–15 cm x 5–11 cm, and short stems surrounded by three to four lobed sheaths, 8–25 cm long. Some of the host plants for Sarcophyte include Acacia, Hyphaene spp., Commiphora spp., Ficus spp., Faidherbia albida (Delile) A. Chev., and Mimusops obtusifolia Lam.
Moreover, plants of the genus Sarcophyte possess numerous spirally arranged, scale-like, ovate-lanceolate leaves up to 3.5 cm long. Their inflorescence is fleshy, with a colored to bright red panicle measuring 5–10 cm in diameter, 2–6 cm long branches, and subtended by a bract. The bracts are ovate-lanceolate, measuring 0.8–2 cm x 0.5–1.2 cm, and are only scaly at the base. The male inflorescence has many secondary flowering branches in groups of two to three, unisexual, usually regular, and 3 (-4)- merous. The pedicel is short, and the perianth segments are elliptic to oblong, measuring 1.5–3.5 mm x 1.5–2 m, with a blunt to acute apex, and flesh stamens measuring 1.5–2.5 mm. The female inflorescence has many secondary branches, each with 5–12 almost globular, spadix-like clusters, 0.4–1.4 cm in diameter, with up to 200 flowers completely sunk in a common receptacle. They do not have a perianth, and their ovary is inferior and 3-celled, while the stigma is disc-shaped, the style reduced, and one-seeded. The fruits are pseudo-berry, aggregated in a rounded and reddish infructescence, with a fleshy epicarp, a hard endocarp, and crowded carpels of the separate flowers that are not consolidated as in a compound fruit (Schmelzer and Gurib-Fakim, 2013). The stamens of S. sanguinea are more than half of the perianth segments, and the flowers have a stinky, unpleasant odor, whereas the stamens of S. piriei are less than half the length of the perianth segments, and the flowers are odorless or have a fruity odor (Schmelzer and Gurib-Fakim, 2013).
4 Origin and geographic distribution
The genus Sarcophyte is native to Africa, and its distribution ranges from Ethiopia to South Africa (Wikimedia Foundation, 2022). Sarcophyte comprises a single species with only two plants, whose accepted names are S. sanguinea and S. piriei. Sarcophyte sanguinea is widespread in eastern and southern Africa, from Ethiopia and Somalia in the north and south to South Africa, while S. piriei is widespread in Zambia, Malawi, Mozambique, Zimbabwe, and East Africa, from Somalia and Ethiopia to Mozambique and Zimbabwe (Mduduzi and Aluwani, 2017).
Sarcophyte piriei has been encountered in several parts of Kenya, including Ruwenzori (Kibwezi) (JSTOR, 2022b), Nairobi National Park, Kajiado, Mau Highlands, Tinderet Highlands (Luke, 2017), and Mbeere South (Muriuki, 2011; Onyancha, 2021). Figure 1 shows the distribution of the genus Sarcophyte in Africa.
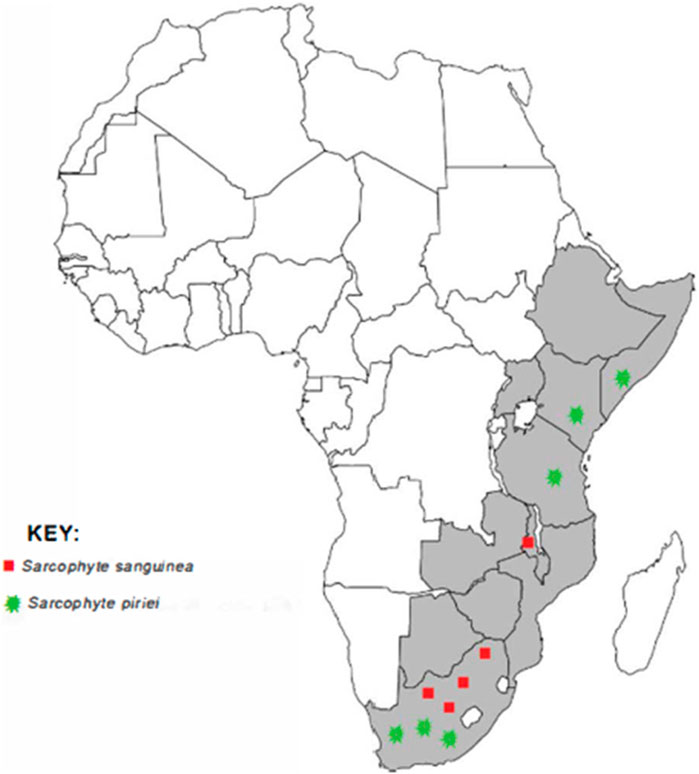
FIGURE 1. A map of Africa showing the distribution of the two plants of the genus Sarcophyte (source: authors).
5 Ethnomedicinal uses
This review observed that S. sanguinea is used ethnomedicinally to treat cervical cancer, pimples, dysentery, amenorrhea, swollen glands, gonorrhea, HIV/AIDS, genital warts, sores, shingles, hypertension, and vomiting in humans in various African ethnic communities (Table 1). In addition, it is used to treat black quarter disease and to control lice infestations in pigs (Table 1).
In addition, S. piriei is used to treat cancer, acne, skin sores, skin eruptions, burns, wounds, shingles, snakebites, sore throat, diarrhea, abdominal pain, menstrual pain, skin bruises, and toothache in traditional medicine (Table 1). The extensive application of these plants underscores their perceived therapeutic versatility in traditional medicine. However, the lack of quantitative data or prevalence rates for each application diminishes the accuracy of these claims.
This study also revealed that S. sanguinea has many diverse applications in South Africa, but only one use was reported in Malawi (Table 1). This regional divergence raises intriguing questions about the cultural, ecological, or historical factors influencing the use of the plant. Conversely, two uses were documented for S. piriei in Kenya, and only one was documented in South Africa, Somalia, and Tanzania (Table 1). The observed discrepancies in use reports necessitate a deeper exploration of the factors that contribute to these variations, such as cultural practices, ecological contexts, or methodological differences in ethnomedicinal and ethnobotanical data collection.
6 Phytochemistry of Sarcophyte sparrm
6.1 Identified compounds
Plants of the genus Sarcophyte contain various secondary metabolites responsible for various biological activities. Qualitative phytochemical screening of the ethanol and methanol extracts of S. piriei indicated the presence of alkaloids, flavonoids, tannins, phenolics, saponins, and terpenoids (Mahammed et al., 2020). In addition, quantitative phytochemical analysis of S. piriei using gas chromatography-mass spectrometry (GC-MS) revealed the presence of various phytochemicals, including phthalic acid, di (October 3-yl) ester (Figure 2), 3-O-methyl-D-glucose (Figure 2), 5-aminoimidazole-4-carboxamide-1-α-D-ribofuranosyl 5' -monophosphate (Figure 2), monomethyl phthalate (Figure 2), hexasiloxane, 1,1,3,3,5,5,7,7,9,9,11,11-dodecamethyl-(Figure 2), and thiophene, 2-nitro-(Figure 2) as the main compounds (Mbakazi et al., 2022).
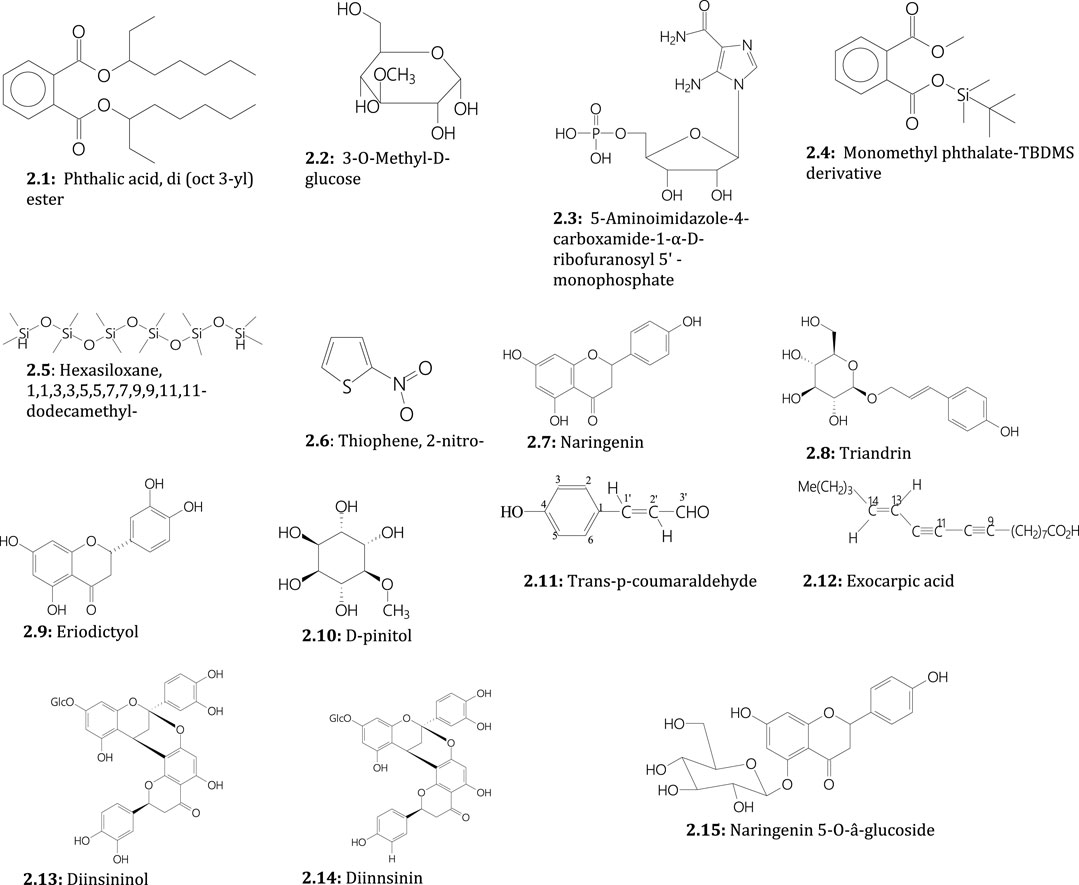
FIGURE 2. Secondary metabolites isolated from S. piriei (2.1–2.6), S. sanguinea (2.7–2.12), and both plants (2.11–2.15)
On the other hand, narigenin (flavonoid) (Figure 2), triandrin (a phenylpropanoid; a 1-O-β-D-glucopyranoside of p-coumaryl alcohol) (Figure 2), eriodictyol (flavonone) (Figure 2), D-pinitol (cyclohexanol) (Figure 2), trans-p-coumaraldehyde (cinnamaldehyde) (Figure 2), and exocarpic acid (polyacetylenic fatty acid) (Figure 2) have been isolated from S. sanguinea (Ogundaini et al., 1996; Schmelzer and Gurib-Fakim, 2013). Notably, three flavonoid glycosides, diinsininol (5,7,3′,4′-tetrahydroxyflavanyl-7-O-â-glucosyl-(4â8:2â-O-7)-eriodictyol) (Figure 2), diinsinin (5,7,3′,4′-tetrahydroxyflavan-7-O-â-glucosyl-(4â8:2â-O-7)-naringenin) (Figure 2) and naringenin 5-O-â-glucoside (Figure 2) have been isolated from tubers/rhizomes of both S. sanguinea and S. piriei and identified using spectroscopic (NMR and U.V.) and spectrometric (FABMS) methods (Ogundaini et al., 1996; Schmelzer and Gurib-Fakim, 2013).
Previous studies have indicated that the flavonoids and fatty acids, among other isolated compounds from the studied plants, possess antioxidant, anticancer, and anti-inflammatory properties (Ríos and Recio, 2005; Adamczak et al., 2020; Moriasi et al., 2021a; Jaradat et al., 2022; Jongrungraungchok et al., 2023; Muruthi et al., 2023).
The identification of these secondary metabolites in S. piriei contributes valuable insights into the chemical composition of these plants and offers a foundation for further empirical exploration of their efficacy. Furthermore, the qualitative and quantitative analyses, along with the isolation and identification of specific compounds from these plants, provide a solid basis for future studies exploring the potential pharmacological and therapeutic applications of these secondary metabolites.
6.2 Chemical tests
The in vitro antioxidant activities of the aqueous and organic extracts of S. piriei were determined using various biochemical assay methods. Available data show that these extracts are potent scavengers of the 2,2-diphenyl-1-picrylhydrazyl (DPPH) radical with IC50 of 4.26 ± 0.22 μg/mL (methanol extract) and 4.62 ± 0.14 μg/mL (dichloromethane extract) compared with ascorbic acid (IC50 = 1.09 ± 0.01 μg/mL) and butylated hydroxytoluene (BHT) (IC50 = 3.96 μg/mL) (Mbakazi et al., 2022).
In the 2 -2′-Azino-di-[3-ethylbenzthiazoline sulfonate (ABTS) assay, the methanolic and dichloromethane extracts of S. piriei had IC50 values of 15.52 ± 0.12 μg/mL and 5.62 ± 0.05 μg/mL, while ascorbic acid and BHT had IC50 values of 1.71 ± 0.00 μg/mL and 3.61 ± 0.08 μg/mL, respectively (Mbakazi et al., 2022). In addition, these extracts demonstrated considerable iron chelating effects with IC50 values of 1.82 ± 0.01 μg/mL and 3.50 ± 0.09 μg/mL for the methanolic and dichloromethane extracts, respectively, against the standards (ascorbic acid = 0.096 ± 0.04 μg/mL; BHT = 1.00 ± 0.01 μg/mL) (Mbakazi et al., 2022). Furthermore, comparable nitric oxide scavenging activity was observed between the methanolic (IC50 = 9.97 ± 0.88 μg/mL) and dichloromethane (IC50 = 9.09 ± 0.11 μg/mL) extracts of this plant; however, ascorbic acid (IC50 = 2.32 ± 0.01 μg/mL) and BHT (3.22 ± 0.04 μg/mL) were more effective in scavenging the nitric oxide in vitro (Mbakazi et al., 2022).
The findings reviewed herein valorize S. piriei as a prospective reservoir of antioxidants, exhibiting a spectrum of mechanisms for antioxidant activity (Moriasi et al., 2020b). A comprehensive scientific approach encompassing pharmacokinetic analyses, bioavailability assessments, and toxicity profile investigations is imperative to facilitate the translation of these findings into clinically applicable therapeutic interventions (Wanjiru et al., 2022; Maina et al., 2023).
Moreover, in pursuing therapeutic applications, it is paramount to establish standardized protocols for the extraction processes and dosage formulations. This standardization will ensure consistency and promote the reproducibility of results, thereby increasing the reliability of therapeutic formulations derived from antioxidant-rich extracts. The findings may also help support their utilization as pharmaceuticals or nutraceuticals, a promising avenue for alleviating conditions associated with oxidative stress and fostering general health (Moriasi et al., 2021a).
7 Pharmacological activities
The extracts and some of the isolated secondary metabolites from plants of the genus Sarcophyte possess biological activities, mainly in vitro antimicrobial and cytotoxic activities and in vivo anti-inflammatory activity (the only in vivo assay reported so far).
7.1 Antimicrobial activities
Recently, Mbakazi et al. (Mbakazi et al., 2022), Mohammed et al. (Mahammed et al., 2020), and Dennis et al. (Dennis et al., 2023) reported that the methanol stem extract of S. piriei possessed notable in vitro antibacterial activities against Enterococcus avium (MIC: 0.16 mg/mL; MBC: 1.25 mg/mL), Staphylococcus aureus, Klebsiella pneumonia, and Vibrio fluvialis (MIC: 0.625 mg/mL; MBC: 2.5 mg/mL) based on disk diffusion and serial broth dilution methods. Also, using the same methods, they reported low activity of the methanol stem extract of S. piriei, which was not active against Escherichia coli (MIC: 0.625 mg/mL; MBC: >5 mg/mL) in comparison with the standard drug (streptomycin), whose average MIC was 2.2 × 10−4 mg/mL (Mahammed et al., 2020; Mbakazi et al., 2022).
Previous studies show that plant extracts with high MIC values (>0.625 mg/mL) are ineffective in eradicating microbes (Mwitari et al., 2013; Clinical Laboratory Standards Institute CLSI, 2022). Therefore, this review reveals that the methanol stem extract of S. piriei is more effective against E. avium compared to the other microbial strains studied so far (Mwitari et al., 2013; Clinical Laboratory Standards Institute CLSI, 2022). Thus, there is a need to standardize the extracts before subjecting them to bioassays to demystify their efficacy: this will facilitate further antimicrobial investigations and lead to the isolation and optimization of lead molecules for drug development (Gakuya et al., 2020). However, Serratia marcescens has been reported to be resistant to the methanol and dichloromethane stem extracts of S. piriei, making them of no clinical significance (Mahammed et al., 2020; Mbakazi et al., 2022)
The in vitro evaluation of the methanolic stem tuber extract of S. piriei using the lactate dehydrogenase (LDH) release assay demonstrated putative membrane-damage-induced cytotoxicity effects against Listeria ivanovii, E. avium, and E. coli, as indicated by the percentage of lactate dehydrogenase release (>50%) (Mbakazi et al., 2022).
Aqueous and methanol-dichloromethane stem extracts of S. sanguinea demonstrated lower antibacterial activities against Ureaplasma urealyticum, Oligella ureolytica, Gardnerella vaginalis, Trichomonas vaginalis, and Neisseria gonorrhoeae (MIC: 2.69 mg/mL), indicating negligible antimicrobial efficacy (Naidoo et al., 2013) compared to the standard drug used (ciprofloxacin), whose mean MIC was lower (0.276 μg/mL) (Naidoo et al., 2013) based on microtiter plate dilution method. In addition, the plant extracts studied were inactive against Candida albicans (MIC>16 mg/mL), implying a lack of antifungal efficacy compared with amphotericin B (the reference standard), whose MIC was 2.5 μg/mL (Naidoo et al., 2013). Furthermore, an Ames test indicated that the methanol: dichloromethane (1:1) extract of S. sanguinea is mutagenic, while the aqueous extract is not mutagenic to the TA98 and TA100 strains of Salmonella typhimurium (1,000 and 200 colonies, respectively) when compared with the standard agent (sodium azide) tested on 500 colonies (Ramulondi, 2017).
Considering the variation in antimicrobial efficacy of extracts derived from these plants reported in the reviewed preliminary in vitro studies, there is a need to utilize comprehensive and standardized extraction and assay procedures to increase the reliability and reproducibility of the results (Pandey et al., 2014; Altemimi et al., 2017). In addition, these steps will provide a solid foundation for subsequent stages of drug development by systematically identifying lead molecules with therapeutic potential (Maina et al., 2023). Notably, careful attention to various factors, such as the selection of extraction solvents, extraction techniques, and quality control measures, is necessary to establish standardized procedures and optimize the antimicrobial efficacy of plant extracts (Wanjiru et al., 2022).
Furthermore, in-depth research is needed to unravel the specific mechanisms underlying the observed antibacterial and antiprotozoal activities of plant extracts derived from plants of the genus Sarcophyte. For instance, exploring the molecular and cellular interactions between bioactive secondary metabolites in target microorganisms will provide valuable insights into their mode of action and provide a solid basis for rational drug design and development (Abreu et al., 2012; Bor et al., 2016). Furthermore, it is crucial to determine the potential synergistic effects between different plant extracts and active secondary metabolites to uncover synergies that boost therapeutic efficacy and their potential applications in clinical practice (Abreu et al., 2012).
7.2 Cytotoxicity studies
Various studies utilizing different assay methods indicate variations in the cytotoxic effects of extracts derived from the investigated plants. The aqueous and methanol:dichloromethane (1:1) extracts of S. sanguinea were previously reported to be non-toxic to the normal human embryonic kidney epithelial cell line (Graham, HEK-293), recording 100% cell viability at a concentration of 100 μg/mL using the 3-(4,5-dimethylthiazol-2-yl)-2,5-diphenyltetrazolium bromide (MTT) method (Naidoo et al., 2013). In addition, the methanol and dichloromethane extracts of S. piriei have shown low cytotoxic effects with CC50 ˃ 200 μg/mL (normal human embryonic kidney (HEK293) and human breast endocrine cells (SKBR-3) compared to the standard (doxorubicin) (CC50 = 1.65 μg/mL) in the MTT assay (Mbakazi et al., 2022).
Elsewhere, extracts of S. sanguinea have been demonstrated to be non-toxic to brine shrimp larvae (LC50 ˃ 2000 μg/mL) compared to potassium dichromate (the positive control agent), which was highly toxic to brine shrimp larvae at a concentration of 2 μg/mL, resulting in 100% mortality (Ramulondi, 2017). In vitro toxicity studies for conventional drugs and botanical drug interactions of the aqueous stem extract of S. sanguinea have shown to cause a 15% inhibition of β-glucuronidase at 10 μg/mL (Ramulondi, 2017), demonstrating its potential efficacy in inhibiting colonic genotoxicity, induced by deconjugation of drug and xenobiotic glucuronides in the gastrointestinal (GI) tract (Awolade et al., 2020). However, the same extract inhibits carboxylesterase by 38% and cytochrome P450 3A4 (CYP3A4) by 82% at a concentration of 10 μg/mL (Ramulondi, 2017), which may negatively impact xenobiotic metabolism in the GIT and liver (Sevrioukova and Poulos, 2013; Singh et al., 2021).
This review noted that the aqueous and dichloromethane:methanol extracts of S. sanguinea extracts possess in vitro antiproliferative activity against the human hepatocarcinoma cell line (HepG2/C3A), with approximately 69% cell death at a concentration of 100 μg/ml as compared to the positive control (melphalan), which revealed 100% cell death (Ramulondi, 2017). In addition, S. piriei extracts demonstrated lower in vitro antiproliferative activities against human colorectal carcinoma (Caco-2) and human hepatocellular carcinoma (HepG2) cells (CC50: 221 to >250 μg/mL) than the positive control drug (doxorubicin) (CC50 = 2.27 μg/mL) (Mahmoud et al., 2011; Mbakazi et al., 2022). However, it is arguable that since various medicinal plants are often combined in traditional medicine to treat cancer, among other devastating conditions, the efficacy of these extracts may be improved when combined (Maina et al., 2023), or when another solvent, especially water, which is commonly used in traditional medicine, is used (Sri Widyawati et al., 2014; Truong et al., 2019).
Based on the reported findings, in-depth in vivo studies are necessary to substantiate and validate the observed effects of S. sanguinea extracts against cancer cells using different standardized experimental designs with appropriate animal models and controlled conditions to elucidate their pharmacokinetic and pharmacodynamic aspects in a physiological context. Research shows that the optimization of extraction methodologies represents a critical avenue for refining the therapeutic potential of plant extracts (Handa et al., 2008; Hamuel, 2012; Altemimi et al., 2017; Abubakar and Haque, 2020), such as those from S. sanguinea and S. piriei. This optimization process should consider different extraction solvents, temperature, duration, and pressure to attain maximum yields of the bioactive secondary metabolites while preserving their stability and bioavailability (Dai and Mumper, 2010; Pandey et al., 2014; Altemimi et al., 2017). The use of advanced techniques, such as chromatographic and spectroscopic analyses, can assist in characterizing and quantifying the extracted secondary metabolites, offering valuable insights into their pharmacological activities (Handa et al., 2008).
Moreover, exploring potential synergies with other medicinal plants may help to enhance the therapeutic efficacy of the investigated plant extracts by improving their pharmacokinetics and spectrum of therapeutic effects, as reported in previous studies (Zhang et al., 2019; Ndung et al., 2018; Karki et al., 2021; Jouda, 2013). Systematic screening of compatible plant combinations, guided by traditional knowledge and modern pharmacological principles, would be critical in identifying synergistic relationships that amplify the therapeutic benefits of the more promising extracts.
In addition, traditional knowledge systems often incorporate specific solvents, and investigating the implications of such choices on the extraction of bioactive secondary metabolites can offer valuable insights into the efficacy of these preparations in treating the conditions claimed (Do et al., 2014). Thus, collaborative efforts involving traditional practitioners and modern scientists can bridge the gap between empirical knowledge and scientific validation, facilitating a holistic understanding of the therapeutic potential inherent in traditional practices.
7.3 Anti-inflammatory activity
Recent in vivo studies have shown that the aqueous rhizome extract of S. piriei significantly inhibited 50% of carrageenan-induced edema in rodents at a dose of 1,000 mg/kg after 10 h (Ogundaini et al., 1996). In addition, this extract effectively inhibited in vitro prostaglandin synthesis (IC50 = 0.2 mg/mL) comparable to aspirin, suggesting its anti-inflammatory efficacy (Ogundaini et al., 1996). Moreover, diinsininol and diinsinin, isolated from the tuber/rhizome of S. sanguinea, inhibit in vitro prostaglandin synthesis with IC50 values of 9.20 and 13.14 µM, respectively, and platelet-activating factor-induced exocytosis with IC50 values of 49 and 39 μM, respectively (Ogundaini et al., 1996; Attiq et al., 2018).
This review highlights the promising anti-inflammatory properties exhibited by the aqueous rhizome extracts of S. sanguinea and S. piriei, which are mainly attributed to diinsininol and diinsinin. While these findings hold potential for therapeutic applications, a successful translation into clinical practice necessitates a comprehensive approach that includes rigorous pre-clinical investigations, an intricate understanding of pharmacokinetics and pharmacodynamics, and robust clinical trials to establish safety and efficacy in human subjects (Shakya, 2016; Jamshidi-Kia et al., 2018).
Extensive pre-clinical studies are essential to elucidate the safety profile and efficacy in various animal models to ensure reproducibility and consistency of the observed anti-inflammatory effects and to provide a solid foundation for subsequent clinical exploration (Nasri and Shirzad, 2013; Moriasi et al., 2021b). In addition, a nuanced understanding of the pharmacokinetic and pharmacodynamic characteristics of diinsininol and diinsinin, including absorption, distribution, metabolism, and excretion within the biological system, is imperative (Raj and Raveendran, 2019). Furthermore, determining the optimal dosage and route of administration and assessing potential side effects and drug interactions are crucial to determining their safety profile and maximizing their therapeutic benefit (Turner et al., 2011; Kathare et al., 2021).
In addition, well-designed clinical trials involving human subjects are indispensable to validating the anti-inflammatory effects observed in pre-clinical studies. These trials should rigorously adhere to ethical standards, involve diverse participant populations to ensure broader applicability, and employ robust methodologies to yield reliable and generalizable results (Knowles, 2014). The amalgamation of these scientific endeavors is integral to the successful translation of the anti-inflammatory properties of the aqueous rhizome extracts of S. sanguinea and S. piriei and their constituent secondary metabolites into clinically effective therapeutic interventions.
8 Limitations
Our review, although comprehensive, has some limitations. The few reviewed materials may not adequately provide sufficient information on the local and traditional medicinal uses and the pharmacology and phytochemistry of the explored plants. In addition, we did not independently verify the efficacy reported in the reviewed literature; therefore, we could not offer specific applications in clinical practice. Moreover, we observed that only preliminary studies were performed involving in vitro models; therefore, they may not mirror in vivo effects, the reports of which are currently unavailable.
9 Conclusion and future directions
This study summarizes for the first time the traditional uses, phytochemistry, and pharmacology of plants of the genus Sarcophyte. This review noted that extracts of these plants have wide traditional medicinal applications, ranging from the treatment of infectious diseases, disorders of the skin, gastrointestinal and urogenital tracts, and cancer, among others. Also, their extracts possess various in vitro cytotoxic effects against normal cell lines, cancer cell lines, brine shrimp nauplii, and some microbes. In addition, the extracts of these plants possess considerable antioxidant and anti-inflammatory activities. In particular, the available literature revealed that only the anti-inflammatory activity of the pure isolated secondary metabolites had been performed, leaving a significant lack of information on other bioactivities, including those of the other phytochemicals, which should be explored empirically.
Based on the current review, we recommend further empirical investigations to evaluate the toxic effects and pharmacological activities of the extracts and isolated secondary metabolites using a bio-assay-guided approach to decipher their potential. In addition, bioactivity-guided fractionation, identification, and characterization of the secondary metabolites, mainly against pathogenic microbes, oxidative stress, inflammation, and cancer, among others, should be conducted based on the documented ethnomedicinal claims.
Author contributions
OJ: Conceptualization, Resources, Writing–review and editing, Methodology, Supervision. MG: Conceptualization, Resources, Writing–original draft, Writing–review and editing, Methodology, Validation. MJ: Resources, Writing–review and editing, Investigation. WS: Conceptualization, Resources, Writing–review and editing. SG: Writing–review and editing, Conceptualization, Resources. NV: Resources, Writing–review and editing.
Funding
The author(s) declare that no financial support was received for the research, authorship, and/or publication of this article.
Acknowledgments
The authors acknowledge all the authors whose work has been included in this review. Mount Kenya University is also acknowledged for providing an enabling research environment.
Conflict of interest
The authors declare that the research was conducted in the absence of any commercial or financial relationships that could be construed as a potential conflict of interest.
Publisher’s note
All claims expressed in this article are solely those of the authors and do not necessarily represent those of their affiliated organizations, or those of the publisher, the editors and the reviewers. Any product that may be evaluated in this article, or claim that may be made by its manufacturer, is not guaranteed or endorsed by the publisher.
Abbreviations
ABTS, 2 -2′-Azino-di- [3-ethylbenzthiazoline sulfonate; BHT, Butylated hydroxytoluene; BSLT, Brine shrimp lethality test; DPPH, 2,2-diphenyl-1-picrylhydrazyl; EIMS, Electron Ionization Mass Spectrometry; FABMS, Fast Atom Bombardment Mass Spectrometry; FABMS, Fast atom bombardment mass spectrometry; IC50, Median Inhibitory concentration; IR, Infra-red; LC50, Median Lethal Concentration; NMR, Nuclear magnetic resonance; PAF, Platelet-activating factor; TBDMS, tert-Butyldimethylsilyl; UV, Ultraviolet.
References
Abdalla, M. A., and Mühling, K. H. (2019). Plant-derived sulfur containing natural products produced as a response to biotic and abiotic stresses: a review of their structural diversity and medicinal importance. J. Appl. Bot. Food Qual. 92, 204–215. doi:10.5073/JABFQ.2019.092.029
Abreu, A. C., McBain, A. J., and Simões, M. (2012). Plants as sources of new antimicrobials and resistance-modifying agents. Nat. Prod. Rep. 29, 1007–1021. doi:10.1039/c2np20035j
Abubakar, A. R., and Haque, M. (2020). Preparation of medicinal plants: basic extraction and fractionation procedures for experimental purposes. J. Pharm. Bioallied Sci. 12, 1–10. doi:10.4103/jpbs.JPBS_175_19
Adamczak, A., Ożarowski, M., and Karpiński, T. M. (2020). Curcumin, a natural antimicrobial agent with strain-specific activity. Pharmaceuticals 13, 1–12. doi:10.3390/ph13070153
Africamuseum, (2022). Prelude medicinal plants database Prelude medicinal plants database. Available Online: https://www.africamuseum.be/en/research/collections_libraries/biology/prelude/results?keywords=Sarcophyte®ion=0 (accessed on September 12, 2022).
Ahmad, B., et al. (2020). Mechanisms of action for the anti-obesogenic activities of phytochemicals. Phytochemistry 180. doi:10.1016/j.phytochem.2020.112513
Altemimi, A., Lakhssassi, N., Baharlouei, A., Watson, D. G., and Lightfoot, D. A. (2017). Phytochemicals: extraction, isolation, and identification of bioactive compounds from plant extracts. Plants 6. doi:10.3390/plants6040042
Angiosperm Phylogeny Group (2016). An update of the Angiosperm Phylogeny Group classification for the orders and families of flowering plants: APG IV. Botanical J. Linn. Soc. 181, 1–20. doi:10.1111/boj.12385
APD (2022). African plant database. Available online: https://africanplantdatabase.ch/(accessed on September 12, 2022).
Attiq, A., Jalil, J., Husain, K., and Ahmad, W. (2018). Raging the war against inflammation with natural products. Front. Pharmacol. 9, 1–27. doi:10.3389/fphar.2018.00976
Awolade, P., et al. (2020). Therapeutic significance of β-glucuronidase activity and its inhibitors: a review. Eur. J. Med. Chem. 187. doi:10.1016/j.ejmech.2019.111921
Balogun, F. O., and Ashafa, A. O. T. (2019). A review of plants used in South African traditional medicine for the management and treatment of hypertension. Planta Med. 85, 312–334. doi:10.1055/a-0801-8771
Bor, T., Aljaloud, S. O., Gyawali, R., and Ibrahim, S. A. (2016). “Antimicrobials from herbs, spices, and plants. Fruits, Vegetables, and Herbs,” in Bioactive foods in health promotion (Amsterdam, Netherlands: Elsevier Inc.). doi:10.1016/B978-0-12-802972-5.00026-3
Britannica (2017). Balanophoraceae. Encyclopedia britannica. https://www.britannica.com/plant/Balanophoraceae.
Clinical Laboratory Standards Institute (CLSI) (2022). Analysis and presentation of cumulative antimicrobial susceptibility test data, Wayne PA: Clinical Laboratory Standards Institute.
Dai, J., and Mumper, R. J. (2010). Plant phenolics: extraction, analysis and their antioxidant and anticancer properties. Molecules. doi:10.3390/molecules15107313
Dennis, J. O., Timothy, W., and Joseph, M. N. (2023). A review of phylogeny, medicinal values, phytochemistry and toxicity of Sarcophyte piriei Hutch (Balanophoraceae). Afr. J. Pharm. Pharmacol. 17, 89–99. doi:10.5897/AJPP2023.5353
De Wet, H., Nciki, S., and van Vuuren, S. F. (2013). Medicinal plants used for the treatment of various skin disorders by a rural community in northern Maputaland, South Africa. J. Ethnobiol. Ethnomed 9. doi:10.1186/1746-4269-9-51
De Wet, H., Nkwanyana, M. N., and van Vuuren, S. F. (2010). Medicinal plants used for the treatment of diarrhoea in northern Maputaland, KwaZulu-Natal Province, South Africa. J. Ethnopharmacol. 130, 284–289. doi:10.1016/j.jep.2010.05.004
De Wet, H., Nzama, V. N., and Van Vuuren, S. F. (2012). Medicinal plants used for the treatment of sexually transmitted infections by lay people in northern Maputaland, KwaZulu-Natal Province, South Africa. South Afr. J. Bot. 78, 12–20. doi:10.1016/j.sajb.2011.04.002
De Wet, H., Ramulondi, M., and Ngcobo, Z. N. (2016). The use of indigenous medicine for the treatment of hypertension by a rural community in northern Maputaland, South Africa. South Afr. J. Bot. 103, 78–88. doi:10.1016/j.sajb.2015.08.011
Dey, D., et al. (2021). Ethnomedicinal use, phytochemistry, and pharmacology of xylocarpus granatum J. Koenig. Evidence-based Complementary Altern. Med. 2021. doi:10.1155/2021/8922196
Do, Q. D., et al. (2014). Effect of extraction solvent on total phenol content, total flavonoid content, and antioxidant activity of Limnophila aromatica. J. Food Drug Anal., doi:10.1016/j.jfda.2013.11.001
Dold, A. P., Cocks, M. L., and yesiXhosa, I. (2005). Traditional Xhosa cosmetics. Veld {and} Flora 91, 123–125.
Ekor, M. (2014). The growing use of herbal medicines: issues relating to adverse reactions and challenges in monitoring safety. Frontiers in Neurology 4. doi:10.3389/fphar.2013.00177
Gaikwad, B., Krishna Mohan, G., and Rani, M. S. (2014). Phytochemicals for diabetes management. Pharm Crop 5, 11–28. doi:10.2174/2210290601405010011
Gakuya, D. W., et al. (2020). Traditional medicine in Kenya: past and current status, challenges, and the way forward. Sci Afr 8, e00360. doi:10.1016/j.sciaf.2020.e00360
GBIF (2022). Global biodiversity information facility. Available online: https://www.gbif.org (accessed on September 12, 2022).
Hamuel, J. P. (2012). Extraction methods, basic structures and mode of action as potential chemotherapeutic agents. Phytochemicals - A Global Perspective of Their Role in Nutrition and Health. doi:10.5772/26052
Handa, S. S., Fermeglia, M., Singh, J., Singh, A. K., Tandon, S., Rane, S., et al. (2008). Vasisht. Extraction technologies for medicinal and aromatic pants. International centre for science and high technology.
Haque, E., et al. (2022). An updated and comprehensive review on the ethnomedicinal uses, phytochemistry, pharmacological activity and toxicological profile of Tinospora crispa (L.) Hook. f. and Thomson. Phytochemistry Reviews. doi:10.1007/s11101-022-09843-y
Hmidouche, O., et al. (2023). Ethnomedicinal use, phytochemistry, pharmacology, and toxicology of Euphorbia resinifera O. Berg. (B): a review. Journal of Zoological and Botanical Gardens 4, 364–395. doi:10.3390/jzbg4020029
Howes, M. J. R. (2017). “Phytochemicals as anti-inflammatory nutraceuticals and phytopharmaceuticals,” in Immunity and inflammation in health and disease: emerging roles of nutraceuticals and functional foods in immune support (Amsterdam, Netherlands: Elsevier Inc.). doi:10.1016/B978-0-12-805417-8.00028-7
Howes, M. J. R., and Perry, E. (2011). The role of phytochemicals in the treatment and prevention of dementia. Drugs Aging 28, 439–468. doi:10.2165/11591310-000000000-00000
IPNI (2022). The international plant names Index and World checklist of vascular plants. Published on the Internet at http://www.ipni.org.
Jamaddar, S., et al. (2023). Ethnomedicinal uses, phytochemistry, and therapeutic potentials of litsea glutinosa (lour.) C. B. Robinson: a literature-based review. Pharmaceuticals 16. doi:10.3390/ph16010003
James, P. B., Wardle, J., Steel, A., and Adams, J. (2018). Traditional, complementary and alternative medicine use in Sub-Saharan Africa: a systematic review. BMJ Glob Health 3, e000895. doi:10.1136/bmjgh-2018-000895
Jamshidi-Kia, F., Lorigooini, Z., and Amini-Khoei, H. (2018). Medicinal plants: past history and future perspective. Journal of HerbMed Pharmacology 7, 1–7. doi:10.15171/jhp.2018.01
Jaradat, N., et al. (2022). Antidiabetic, antioxidant, and anti-obesity effects of phenylthio-ethyl benzoate derivatives, and molecular docking study regarding α-amylase enzyme. Sci Rep 12. doi:10.1038/s41598-022-07188-2
Jongrungraungchok, S., et al. (2023). In vitro antioxidant, anti-inflammatory, and anticancer activities of mixture Thai medicinal plants. BMC Complement Med Ther 23. doi:10.1186/s12906-023-03862-8
Josephine Ozioma, E.-O., and Antoinette Nwamaka Chinwe, O. (2019). Herbal medicines in african traditional medicine. Herbal Medicine. doi:10.5772/intechopen.80348
Jouda, M. M. (2013). The antibacterial effect of some medicinal plant extracts and their synergistic effect with antibiotic. Advances in Life Science and Technology.
JSTOR (2022a). Global plants on JSTOR. Available online: https://plants.jstor.org/(accessed on December 16, 2022).
JSTOR (2022b). Sarcophyte piriei Hutch. [family balanophoaceae]. Available online: https://plants.jstor.org/stable/10.5555/aL.ap.specimen.k000430580.
Kamau, L. N., Mbaabu, P. M., Mbaria, J. M., Gathumbi, P. K., and Kiama, S. G. (2016). Ethnobotanical survey and threats to medicinal plants traditionally used for the management of human diseases in Nyeri County, Kenya. Tang Humanitas Medicine 6, 1–15. doi:10.5667/tang.2016.0007
Karki, R., et al. (2021). In vitro antimicrobial synergy testing of extensively drug-resistant clinical isolates at an organ transplant center in Nepal. Infect Drug Resist 14, 1669–1677. doi:10.2147/idr.s309531
Kathare, J. M., Mbaria, J. M., Nguta, J. M., Moriasi, G. A., and Mainga, A. O. (2021). Antimicrobial efficacy, cytotoxicity, acute oral toxicity, and phytochemical investigation of the aqueous and methanolic stem bark extracts of bridellia micrantha (hochst.) baill. Pharmacognosy Journal 13, 1248–1256. doi:10.5530/pj.2021.13.158
Kigen, G., et al. (2014). Ethnomedicinal plants traditionally used by the keiyo community in elgeyo marakwet county, Kenya. Journal of Biodiversity, Bioprospecting and Development 1, 1–11. doi:10.4172/ijbbd.1000132
Knowles, R. G. (2014). Development of anti-inflammatory drugs - the research and development process. Basic and Clinical Pharmacology and Toxicology 114, 7–12. doi:10.1111/bcpt.12130
Luke, Q. (2017). Plants of conservation interest of the Nairobi national Park. Nairobi County, Kenya: WRQ, Luke. doi:10.13140/RG.2.2.13794.12487
Mahammed, A., Mitiku, H., and Mohammed, J. (2020). In vitro antibacterial activity of selected medicinal plants extract used in traditional treatment of common human wound infection in Fafan zone, Somali region, Ethiopia. International Journal of Herbal 8, 6–11.
Mahmoud, T. S., et al. (2011). In vitro cytotoxic activity of brazilian middle west plant extracts. Revista Brasileira de Farmacognosia 21, 456–464. doi:10.1590/S0102-695X2011005000061
Maina, M., Mbaria, J., Kamanja, I., and Moriasi, G. (2023). Acute oral toxicity, cognitive-enhancing and anti-lipid peroxidation efficacy, and qualitative phytochemistry of the aqueous aerial part extract of Launaea cornuta (Hochst. ex. Oliv. and Hiern) C. Jeffrey. Heliyon 9, e15487. doi:10.1016/j.heliyon.2023.e15487
Maroyi, A. (2017). Elephantorrhiza elephantina: traditional uses, phytochemistry, and pharmacology of an important medicinal plant species in southern Africa. Evidence-based Complementary and Alternative Medicine 2017. doi:10.1155/2017/6403905
Massimo, M. (2003). Dietary supplements of plant origin: a nutrition and health approach. Oxfordshire: Taylor and Francis Inc. doi:10.4324/9780203351352
Mbakazi, Y., et al. (2022). GC/TOF-MS-based phytochemical analysis, in vitro antiproliferative effects, antioxidant, and antibacterial activity of Sarcophyte sanguinea subsp. piriei (Hutch.) B. Hansen. South African Journal of Botany 150, 752–758. doi:10.1016/j.sajb.2022.08.003
Mduduzi, N., and Aluwani, T. (2017). Sarcophyte sanguinea Sparrm. subsp. sanguinea. Accessed Online at https://pza.sanbi.org/sarcophyte-sanguinea-subsp-sanguineaon26th February, 2023).
Moriasi, G., Ireri, A., and Ngugi, M. (2020a). Cognitive-Enhancing, ex vivo antilipid peroxidation and qualitative phytochemical evaluation of the aqueous and methanolic stem bark extracts of lonchocarpus eriocalyx (harms.). Biochem. Res. Int., 1–16.
Moriasi, G., Ireri, A., and Ngugi, M. P. (2020b). In vitro antioxidant activities of the aqueous and methanolic stem bark extracts of Piliostigma thonningii (Schum.). J Evid Based Integr Med 25. doi:10.1177/2515690x20937988
Moriasi, G. A., Ireri, A. M., Nelson, E. M., and Ngugi, M. P. (2021a). In vivo anti-inflammatory, anti-nociceptive, and in vitro antioxidant efficacy, and acute oral toxicity effects of the aqueous and methanolic stem bark extracts of Lonchocarpus eriocalyx (Harms.). Heliyon 7, e07145. doi:10.1016/j.heliyon.2021.e07145
Moriasi, G., Kibiti, C., and Ngugi, M. (2021b). In vivo antidiabetic efficacy, mineral element composition, and qualitative phytochemistry of the aqueous leaf extracts of pentas zanzibarica (klotzsch.) vatke and olea europaea subspecies africana (mill.). Journal of Advanced Biotechnology and Experimental Therapeutics 4, 334. doi:10.5455/jabet.2021.d134
Muriuki, J. (2011). Medicinal trees in smallholder afroforestry systems: assessing some factors influencing cultivation by some farmers East of Mt. Kenya. Vienna, Austria: University of Natural Resources and Applied Life Sciences Vienna.
Muruthi, C. W., Ngugi, M. P., Runo, S. M., and Mwitari, P. G. (2023). In vitro antiproliferative effects and phytochemical characterization of carissa edulis ((Forssk) vahl) and pappea capensis (eckyl and zeyh) extracts. J Evid Based Integr Med 28. doi:10.1177/2515690x231187711
Mwitari, P. G., Ayeka, P. A., Ondicho, J., Matu, E. N., and Bii, C. C. (2013). Antimicrobial activity and probable mechanisms of action of medicinal plants of Kenya: withania somnifera, warbugia ugandensis, prunus africana and plectrunthus barbatus. PLoS One 8. doi:10.1371/journal.pone.0065619
Naidoo, D., Van Vuuren, S. F., Van Zyl, R. L., and De Wet, H. (2013). Plants traditionally used individually and in combination to treat sexually transmitted infections in northern Maputaland, South Africa: antimicrobial activity and cytotoxicity. J Ethnopharmacol 149, 656–667. doi:10.1016/j.jep.2013.07.018
Nasri, H., and Shirzad, H. (2013). Toxicity and safety of medicinal plants. Journal of HerbMed Pharmacology 2, 21–22.
Ndawonde, B. G., Dlamini, E. T., and Imenda, S. N. (2012). Indigenous knowledge, women and issues of sustainable development. WIT Transactions on Ecology and the Environment 167, 45–54. doi:10.2495/ST110051
Ndawonde, B. G., Zobolo, A. M., Dlamini, E. T., and Siebert, S. J. (2007). A survey of plants sold by traders at Zululand muthi markets, with a view to selecting popular plant species for propagation in communal gardens. Afr J Range Forage Sci 24, 103–107. doi:10.2989/AJRFS.2007.24.2.7.161
Ndhlovu, P. T., Asong, J. A., Omotayo, A. O., Otang-Mbeng, W., and Aremu, A. O. (2023). Ethnobotanical survey of medicinal plants used by indigenous knowledge holders to manage healthcare needs in children. PLoS One 18, e0282113. doi:10.1371/journal.pone.0282113
Ndung, J., et al. (2018). Phytochemical screening and synergistic anti-proliferative activity against selected cancer cell lines of moringa oleifera and Indigofera arrecta leaf extracts. European J Med Plants 23, 1–11. doi:10.9734/EJMP/2018/41021
Nickrent, D. L. (2020). Parasitic angiosperms: how often and how many?. Taxon 69, 5–27. doi:10.1002/tax.12195
Nzue, A. P. M. M. (2009). Use and conservation status of medicinal plants in the cape peninsula, western cape province of South Africa. Msc. Thesis. Stellenbosch, South Africa: University of Stellenbosch. Available at: https://core.ac.uk/download/pdf/37319949.pdf.
Odukoya, J. O., Odukoya, J. O., Mmutlane, E. M., and Ndinteh, D. T. (2022). Ethnopharmacological study of medicinal plants used for the treatment of cardiovascular diseases and their associated risk factors in sub-saharan Africa. Plants 11. doi:10.3390/plants11101387
Ogundaini, A., Farah, M., Perera, P., Samuelsson, G., and Bohlin, L. (1996). Isolation of two new antiinflammatory biflavanoids from Sarcophyte piriei. J Nat Prod 59, 587–590. doi:10.1021/np960386k
Okello, M. M., and Kiringe, J. W. (2004). Threats to biodiversity and their implications in protected and adjacent dispersal areas of Kenya. Journal of Sustainable Tourism 12, 55–69. doi:10.1080/09669580408667224
Okot, D. F., Anywar, G., Namukobe, J., and Byamukama, R. (2020). Medicinal plants species used by herbalists in the treatment of snakebite envenomation in Uganda. Trop Med Health 48. doi:10.1186/s41182-020-00229-4
Olajuyigbe, O. O. (2012). Ethnobotanical survey of medicinal plants used in the treatment of gastrointestinal disorders in the Eastern Cape Province, South Africa. Journal of Medicinal Plants Research 6. doi:10.5897/JMPR11.1707
Omara, T., et al. (2021). Medicinal plants used as snake venom antidotes in east african community: review and assessment of scientific evidences. Journal of Medicinal and Chemical Sciences 4, 107–144. doi:10.26655/JMCHEMSCI.2021.2.4
Onyancha, J. M. (2021). Personal communication with a team of herbalists and traditional medicine practitioners of Mbeere South subcounty in embu county on october. London, UK: Intechopen.
Pandey, A., Tripathi, S., and Pandey, C. A. (2014). Concept of standardization, extraction and pre phytochemical screening strategies for herbal drug. Journal of Pharmacognosy and Phytochemistry JPP 115, 115–119.
Patrício, K. P., et al. (2022). Medicinal plant use in primary health care: an integrative review. Ciencia e Saude Coletiva 27, 677–686. doi:10.1590/1413-81232022272.46312020
Raj, G. M., and Raveendran, R. (2019). “Introduction to basics of pharmacology and toxicology,” in General and molecular pharmacology: principles of drug action (Singapore: Springer Nature Singapore Pte Ltd.), 1.
Ramulondi, M. (2017). Toxicology and herb-drug interaction of selected anti-hypertension plants used by lay persons in Northern Kwazulu-Natal. MSc. Thesis. Richards Bay, South Africa: University of Zululand.
Razgour, O., Kasso, M., Santos, H., and Juste, J. (2020). Up in the air: threats to Afromontane biodiversity from climate change and habitat loss revealed by genetic monitoring of the Ethiopian Highlands bat. Evol Appl, 794–806. doi:10.1111/eva.13161
Ríos, J. L., and Recio, M. C. (2005). Medicinal plants and antimicrobial activity. Journal of Ethnopharmacology. doi:10.1016/j.jep.2005.04.025
Sagbo, I. J., and Mbeng, W. O. (2018). Plants used for cosmetics in the eastern cape province of South Africa: a case study of skin care idowu. Pharmacogn. Rev. 1, 139–156. doi:10.4103/phrev.phrev_9_18
Sagbo, I. J., and Otang-Mbeng, W. (2021). Plants used for the traditional management of cancer in the eastern Cape province of South Africa: a review of ethnobotanical surveys, ethnopharmacological studies and active phytochemicals. Molecules 26. doi:10.3390/molecules26154639
Salama, M. E.-D., et al. (2021). Medicinal plant-derived compounds as potential phytotherapy forCOVID-19: future perspectives. Journal of Pharmacognosy and Phytotherapy 13, 68–81. doi:10.5897/JPP2021.0603
Schmelzer, G. H., and Gurib-Fakim, A. (2013). “Plant resources of tropical africa,” in Medicinal plants (Wageningen, Netherands: PROTA Foundation).
Schmelzer, G. H., and Gurib-Fakim, A. (2013b). “Plant resources of tropical africa,” in Medicinal plants (Wageningen, Netherands: PROTA Foundation).
Sevrioukova, I. F., and Poulos, T. L. (2013). Understanding the mechanism of cytochrome P450 3A4: recent advances and remaining problems. Dalton Transactions 42, 3116–3126. doi:10.1039/c2dt31833d
Shakya, A. K. (2016). Medicinal plants: future source of new drugs Medicinal plants: future source of new drugs. International Journal of Herbal Medicine 4. doi:10.13140/RG.2.1.1395.6085
Siddique, Z., et al. (2021). Exploration of ethnomedicinal plants and their practices in human and livestock healthcare in Haripur District, Khyber Pakhtunkhwa, Pakistan. J Ethnobiol Ethnomed 17. doi:10.1186/s13002-021-00480-x
Singh, A., Gao, M., and Beck, M. W. (2021). Human carboxylesterases and fluorescent probes to image their activity in live cells. RSC Medicinal Chemistry, 12 1142–1153. doi:10.1039/d1md00073j
Sri Widyawati, P., Budianta, T. D. W., Kusuma, F. A., and Wijaya, E. L. (2014). Difference of solvent polarity to phytochemical content and antioxidant activity of Pluchea indicia less leaves extracts. International Journal of Pharmacognosy and Phytochemical Research 6 (4), 850–855.
Stark, T. D., Mtui, D. J., and Balemba, O. B. (2013). Ethnopharmacological survey of plants used in the traditional treatment of gastrointestinal pain, inflammation and diarrhea in Africa: future perspectives for integration into modern medicine. Animals 3. doi:10.3390/ani3010158
Swamy, M. K. (2020). “Plant-derived bioactives: production, properties and therapeutic applications,” in Plant-derived bioactives: production, properties and therapeutic applications (Singapore: Springer). doi:10.1007/978-981-15-1761-7
Truong, D.-H., et al. (2019). Evaluation of the use of different solvents for phytochemical constituents, antioxidants, and in vitro anti-inflammatory activities of severinia buxifolia. J Food Qual 2019, 1–9. doi:10.1155/2019/8178294
Turner, P. V., Brabb, T., Pekow, C., and Vasbinder, M. A. (2011). Administration of substances to laboratory animals: routes of administration and factors to consider. Journal of the American Association for Laboratory Animal Science 50, 600–613.
Wanjiru, J. N., Maitho, T. E., Mbaria, J. M., and Moriasi, G. A. (2022). Subacute toxicity effects of the aqueous shoot extract of yushania alpina (K. Schum.) W.C.lin in sprague dawley rats: an appraisal of its safety in ethnomedicinal usage. J Toxicol 2022. doi:10.1155/2022/6283066
WHO (2013). Traditional medicine: a report by the secretariat. http://apps.who.int/iris/bitstream/10665/92455/1/9789241506090_eng.pdf?ua=1.
Wikimedia Foundation (2022). “Sarcophyte Sparrm. In döring M,” in English wikipedia - species pages (San Francisco, California, United States: Wikimedia Foundation). doi:10.15468/c3kkgh
World Flora Database (2022). World Flora database. Available online: http://www.worldfloraonline.org (accessed on September 12, 2022).
World Flora Online (2022). Sarcophyte Sparrm. http://www.worldfloraonline.org/taxon/wfo-4000034069 (Accessed on September 12, 2022).
Keywords: anticancer, anti-inflammatory, antimicrobial, diinsinin, diinsininol, holoparasite, phytochemicals
Citation: Jared Misonge O, Gervason Apiri M, James Onsinyo M, Samuel Murigi W, Geoffrey Ogeto S and Vincent Obaga N (2024) Ethnomedicinal uses, phytochemistry, and pharmacology of the genus Sarcophyte: a review . Front. Pharmacol. 14:1301672. doi: 10.3389/fphar.2023.1301672
Received: 25 September 2023; Accepted: 13 December 2023;
Published: 08 January 2024.
Edited by:
Thomas Brendler, University of Johannesburg, South AfricaReviewed by:
Verena Spiegler, University of Münster, GermanyLaiba Arshad, Forman Christian College, Pakistan
Copyright © 2024 Jared Misonge, Gervason Apiri, James Onsinyo, Samuel Murigi, Geoffrey Ogeto and Vincent Obaga. This is an open-access article distributed under the terms of the Creative Commons Attribution License (CC BY). The use, distribution or reproduction in other forums is permitted, provided the original author(s) and the copyright owner(s) are credited and that the original publication in this journal is cited, in accordance with accepted academic practice. No use, distribution or reproduction is permitted which does not comply with these terms.
*Correspondence: Moriasi Gervason Apiri, gmoriasi@outlook.com