- 1School of Elderly Health, Chengdu Medical College, Chengdu, Sichuan, China
- 2School of Nursing, Chengdu Medical College, Chengdu, Sichuan, China
- 3Chengdu Eighth People’s Hospital (Geriatric Hospital of Chengdu Medical College), Chengdu, Sichuan, China
- 4Sichuan Collaborative Innovation Center for Elderly Care and Health, Chengdu, Sichuan, China
Introduction: Berberine is an isoquinoline alkaloid extracted from Berberis vulgaris, which possesses a variety of pharmacological activities. Alzheimer’s disease (AD) is a complex disease with multiple pathologic factors, with cognitive decline being the main manifestation of AD. The neuroprotective effects of berberine in animal models of Alzheimer’s disease (AD) have been widely reported, exhibiting protective effects against risk factors associated with AD. In this study, we summarize and evaluate the effects of berberine on cognitive function and β-amyloid precursor protein in animal models of AD.
Material and methods: Eligible studies were retrieved from PubMed, MEDLINE, EMBASE, Web of Science, and Cochrane Library databases up to 1 June 2023. Risk of bias was assessed by the Systematic Review Center for Laboratory Animal Experiments (SYRCLE). Statistical analyses were performed using STATA 14.0 and Review Manger 5.4 software to calculate weighted standardized mean difference (SMD) and 95% confidence intervals (CI), Morris water maze (MWM) test and β-amyloid precursor protein as outcome measures. Heterogeneity was tested using the I2 test. Sensitivity analysis and publication bias were also assessed.
Results: 19 studies involving 360 animals met the inclusion criteria, and the results of the meta-analysis showed that berberine decreased escape latency (SMD = −2.19, 95% CI: (−2.50, −1.88), p < 0.00001), increased the number of platform crossings (SMD = 4.27, 95% CI (3.38, 5.17), p < 0.00001), time in the target quadrant (SMD = 5.92, 95% CI (4.43, 7.41), p < 0.00001) and APP expression (SMD = 0.73, 95% CI: (0.25, 1.21), p = 0.003).
Conclusion: Berberine can regulate APP expression and improve cognitive function in animal models of AD, and the mechanism may be related to the involvement of berberine in APP processing and influence the expression of its related factors.
Systematic review registration: PROSPERO, CRD42023437445
1 Introduction
Alzheimer’s disease (AD) is a neurodegenerative condition characterized by memory loss and cognitive decline. Currently, over 55 million individuals globally experience dementia, and this number is projected to surpass 78 million by 2030 due to population aging, with AD contributing to 60%–70% of dementia cases (Leng and Edison, 2021; Leng and Edison, 2021). It stands as the primary cause of disability among individuals aged 65 and above worldwide (Jia et al., 2020). It is swiftly emerging as one of this century’s most burdensome, costly, and lethal diseases (Scheltens et al., 2021). As the population aged 65 and above grows, so does the prevalence of AD among older adults. In summary, AD poses a significant global health threat (Dauphinot et al., 2022).
There are many typical pathological features of AD including accumulation of tau neurogenic fiber tangles (Busche and Hyman, 2020), synaptic degeneration (Griffiths and Grant, 2023), mitochondrial dysfunction (Morton et al., 2021) neuroinflammation and oxidative stress (Tonnies and Trushina, 2017) among others. One of the characteristic hallmarks of AD is the deposition of senile plaques containing β-amyloid (Aβ). Aβ is produced from the amyloid precursor protein (APP) by sequential proteolytic cleavage by β-secretase and γ-secretase. The aggregation of Aβ into amyloid plaques is considered a pivotal pathogenic event in AD. APP is a transmembrane precursor protein widely expressed in the central nervous system, peripheral tissues of the liver and pancreas, adipose tissue, and myotubes. APP is first cleaved by β-site cleaving enzyme 1 (BACE 1) in vivo, releasing soluble APPβ (sAPPβ) peptide (Kim and Tsai, 2009). APP peptides produced by non-amyloidogenic pathways (e.g., sAPPα) are metabolically beneficial in the central nervous system and peripheral tissues. However, amyloidogenesis triggered by APP cleavage not only exacerbates AD progression but also adversely affects metabolic conditions. Therefore, pharmacological modulation to increase non-amyloid pathway peptide production and reduce amyloidogenic pathway peptides, specifically targeting BACE1-mediated APP cleavage without impacting other substrates, might prove effective in combating AD (Guo et al., 2021b). Understanding the role of APP in AD pathogenesis concerning Aβ production and abnormal aggregation is crucial. However, current therapeutic strategies against β-amyloid have almost always ended in failure in clinical trials. The first new AD drug, aducanumab (Aduhelm), approved by the U.S. FDA using the accelerated approval pathway since 2003, has also generated much controversy (Liu and Howard, 2021), so it is necessary to search for better drugs for AD.
Donepezil, carboplatin, galantamine and memantine are routinely used in clinical practice for the treatment of AD. However, these medications are single-target drugs focusing on specific mechanisms, exhibiting temporary and modest symptomatic improvements but hardly preventing or reversing AD progression (Silva et al., 2014). Traditional Chinese medicine (TCM) is characterized by multi-targets, multi-systems, multi-links and multi-pathways in the treatment of dementia, which shows the unique advantages of TCM (Chen et al., 2020a). Berberine, a natural alkaloid, has become a hot research topic for its role in central nervous system diseases (Cheng et al., 2022). Berberine inhibits neuroinflammation, oxidation, and endoplasmic reticulum stress production, exhibiting neuroprotective, antioxidant, and anti-inflammatory properties, thereby reducing neuronal damage and apoptosis (Zhang et al., 2020). Multiple studies emphasize berberine’s efficacy in enhancing conditions related to cognitive impairment (Fang et al., 2020; Zhang et al., 2021a; Yao et al., 2023). Research indicates that berberine (BBR) curbs Aβ-induced microglia activity by modulating suppressor of cytokine signaling 1 (SOCS 1) (Guo et al., 2021a). It has also been shown that endoplasmic reticulum stress is central to signaling by the mechanism of phosphorylation of tau protein by hyperactivation of glycogen synthase kinase 3β (GSK 3 β) and phosphorylation of eukaryotic translation initiation factor-2α (eIF2α) by activation of PRKR-like endoplasmic reticulum kinase (PERK). Berberine ameliorates endoplasmic reticulum stress and thus cognitive deficits in APP/PS1 mice (Wu et al., 2021). While various experiments have assessed berberine’s anti-AD properties, there has not been a systematic review of its effects on cognitive function and β-amyloid precursor protein. Therefore, it is necessary to investigate the effects of berberine on cognitive function and β-amyloid precursor protein to clarify the therapeutic potential of berberine in AD.
Based on the limitations of current studies and systematic evaluations, the purpose of this study was to systematically review the current literature in evaluating the effects of berberine on the MWM test and in β-amyloid precursor proteins in AD models. Behavioral indicators were analyzed to determine whether berberine improves cognitive function in animal models, to further evaluate the efficacy of berberine on β-amyloid expression in AD models and the potential mechanisms, and to explore the different effects of different intervention times, modes of intervention, and dosages and routes of administration in mice with AD models.
2 Materials and methods
2.1 Search strategy
Qualified studies published through June 2023 were searched in PubMed, Embase, Web of Science, and the Cochrane Library. The strategy was developed based on a combination of medical subject headings (MeSH) and free-text terms, and the database search strategy was based on PubMed as an example, with the search formula shown below: (Alzheimer Disease [MeSH Terms]) OR (Dementia [MeSH Terms]) OR (Cognition [MeSH Terms]) OR (Alzheimer) OR (AD) OR (cognitive) AND “Berberine” (MeSH Terms) OR “Berberine” [Title/Abstract] OR “Umbellatine” [Title/Abstract]. The detailed search strategy was provided in the Supplementary Material.
2.2 Inclusion and exclusion criteria
2.2.1 Inclusion criteria
Two investigators (JY-L and YX-H) jointly developed the inclusion and exclusion criteria for this review. Studies were considered if all of the following criteria were met: 1) an animal model with Alzheimer’s disease, induced by genetic variants (transgenics) or drugs; 2) berberine dosing was the only intervention, with no restriction on the duration of the intervention, dosage of the drug, mode of dosing, or type of mice; 3) availability of the Morris Water Maze (MWM) test or the β-amyloid precursor protein (APP) indicator; 4) randomized controlled trials; 5) published in English.
2.2.2 Exclusion criteria
1) Therapies other than interventions using berberine; 2) studies of cytokines; 3) duplicate literature or literature without a control group; 4) lack of full text, literature review studies, course-completion theses, dissertations, theses, and abstracts for yearbooks; 5) literature that did not meet the inclusion criteria after manual screening was also excluded.
2.3 Data extraction
Two researchers (J-YL and Y-XH) independently extracted the following data and resolved differences by consulting with a third reviewer: 1) year of publication and name of the first author, 2) animal information, including species, sex, age, weight, and sample size of each group, etc. 3) intervention regimen, including duration of the intervention, dosage of medication, and mode of medication administration, etc. 4) assessment of the outcome, including the escape latency, the number of platform crossings, time in the target quadrant selected in the MWM test, and β-amyloid precursor protein, with the fourth day or more extracted as the final outcome if the outcome was presented at different time points. If the data were presented only graphically, the values in the graphs were estimated using the GetData Graph Digitizer 2.26.
2.4 Quality evaluation and risk of bias assessment
Two evaluators independently assessed the quality of the study using the 10-item scale introduced by the SYRCLE Risk of Bias (RoB) Tool for Animal Research and resolved differences by consulting a third reviewer. The evaluation criteria of the tool are: random allocation sequence; similar baseline characteristics; allocation concealment; randomized housing; blinded interventions; random selection for outcome assessment; blinded assessment of outcomes; incomplete outcome data; selective outcome reporting; and bias from other sources (Hooijmans et al., 2014). A “Y” response indicates a low risk of bias, an “N” response indicates a high risk of bias, and an “NC” response indicates an uncertain level of bias due to insufficient data. One point was awarded for each “Y” response.
2.5 Statistical analysis
Data were analyzed using RevMan 5.4 and Stata 14.0 software. A random effects model was used to calculate mean ± SD, 95% confidence intervals (CI) and standardized mean difference (SMD) to account for potential heterogeneity. The heterogeneity of the included data was determined by calculating the I2 value; if I2 ≤ 50%, p ≥ 0.1, it was considered that there was no significant heterogeneity among the studies, and the fixed-effects model was used for the analysis; if I2 > 50%, p < 0.1, it was considered that there was significant heterogeneity among the studies, and the random-effects model was used for the analysis; when the heterogeneity was higher, regression analysis, subgroup analysis, and sensitivity analysis were used to explore the source of heterogeneity, and funnel plot and Egger’s test were used to evaluate publication bias.
3 Results
In accordance with the developed literature search, a total of 2,741 articles were found in the initial search for the item study strategy, and 1,096 duplicates were excluded through Notexpress literature management software, leaving 1,645 articles to be screened. A total of 1,602 articles were excluded by reading the title and abstract, 23 articles were excluded by reading the full text, and 23 studies were excluded due to the following reasons: incomplete study data; absence of the primary endpoints of our study; cellular experiments rather than animal experiments, and 19 articles were finally included in the final literature (Zhu and Qian, 2006; Bhutada et al., 2011; Durairajan et al., 2012; Mangrulkar et al., 2013; Patil et al., 2015; de Oliveira et al., 2016; Kumar et al., 2016; Chen et al., 2017; He et al., 2017; Huang et al., 2017; Cai et al., 2018; Hussien et al., 2018; Chen et al., 2020b; Lin et al., 2020; Wang et al., 2021b; Liang et al., 2021; Wu et al., 2021; Ye et al., 2021; Yang and Wang, 2022). The flow diagram of the selection process is shown in (Figure 1).
3.1 Characterization of the study
A total of 19 papers were included with a total of 360 mice, including 175 animals in the control group and 175 in the exercise group. AD models included both transgenic and non-transgenic models. Five studies used the APP/PS1 mouse model (n = 5). Four studies used the 3 × Tg AD mouse model (n = 4), five studies used Wistar rats (n = 5), two studies used Sprague-Dawley rats (n = 2), one study used TgCRND8 mice, one study used Swiss Albino mice and one study used B6C3-Tg mice. Thirteen studies used only male animals, one study used only female animals, two studies used animals of both sexes, and three did not describe the sex of the animals used in the experiment. The duration of the intervention ranged from half a month to 4 months. A total of 14 out of 19 studies mentioned the age of the animals ranging from 1 month to 8 months of age. Seventeen studies used dosing doses of 50 mg–100 mg/kg, one study used a dose of 40 mg/kg, and one study used a dose of 187.5 mg/kg. Outcome indicators: 17 studies mentioned escape latency, 14 studies mentioned duration in the platform quadrant, 13 studies mentioned the number of platform crossings, 8 studies mentioned APP. All these data can be found in Table 1.
3.2 Study quality evaluation and risk of bias assessment
Study Quality Assessment Table 2 shows the methodological quality assessment of the 19 included studies, with study quality scores ranging from 3 - 6 out of 10. No studies were considered to be at low risk of sequence generation, allocation concealment (selection bias), blinded intervention and randomized outcome assessment (detection bias).15 studies were considered to be at low risk of baseline characteristics (selection bias). 15 studies were judged to be at low risk of randomized housing (performance bias). 15 studies were judged to have a low risk of incomplete outcome data (attrition bias). However, only 6 studies were considered to have a low risk of blinding assessment of outcome (detection bias). All 19 studies were considered to be at low risk of selective outcome reporting and other sources of bias.
3.3 Results of the meta-analysis
3.3.1 Effect of berberine on cognitive function in AD models: Escape latency of MWM
Seventeen studies with a total of 332 animals used escape latency as an outcome indicator and all reported a positive effect of berberine in reducing escape latency. However, there was a high heterogeneity between studies (p < 0.00001, I2 = 80%), so a random effects model was performed for analysis. According to the results, in terms of reducing escape latency, the intervention group had a significant effect in reducing escape latency compared to the control group (SMD = −2.92, 95% CI: 3.68, −2.17, p < 0.00001) (Figure 2).
Due to heterogeneity, we further performed subgroup analyses based on animal species (mice, rats), intervention dose (<100 mg/kg, ≥100 mg/kg), duration of intervention (<14, 14–30, >30 days), and route of administration (oral administration and gavage administration). The results are shown below Table 3.
Table 3 demonstrated that different animal species, dose, duration of intervention and route of administration significantly shortened the escape latency (p < 0.05) compared to controls.
The subgroup analysis observed that mice had slightly reduced heterogeneity (I2 = 71%), while rats did not (I2 = 84%). For dose, medication doses ≥100 mg/kg had mildly reduced heterogeneity (I2 = 71%), whereas <100 mg/kg did not (I2 = 94%). Heterogeneity was significantly reduced for berberine interventions <14 days (I2 = 0), slightly reduced for >30 heterogeneity (I2 = 64%), no reduction in heterogeneity for 14–30 days (I2 = 84%). For route of administration, a slight decrease in heterogeneity with oral administration of berberine (I2 = 71%) and no decrease with gavage administration (I2 = 84%).
3.3.2 Effects of berberine on cognitive function in AD models: Time spent in the MWM platform quadrant
Fourteen studies used time spent in the platform quadrant as an outcome measure, totaling 280 mice. There was a high degree of heterogeneity between studies (I2 = 87%, p < 0.00001), so a random-effects model was performed for analysis. Meta-analysis showed a significant difference between the intervention and control groups in terms of time spent in the platform quadrant. (SMD = 5.92, 95% CI (4.43, 7.41), p < 0.00001) (Figure 3).
Due to heterogeneity, we further performed subgroup analyses based on animal type (mice and rats), duration of intervention (<14, 14–30, >30 days), and route of administration (oral administration and gavage administration). The results are shown below Table 4.
Table 4 demonstrated that different animal species, duration of intervention and route of administration significantly increased time spent in the platform quadrant (p < 0.05) compared to controls.
The subgroup analysis observed that a significant decrease in heterogeneity in rats (I2 = 0%), and no decrease in heterogeneity in mice (I2 = 87%). For duration of intervention, a significant reduction in heterogeneity for berberine interventions <14 days and interventions 14–30 days (I2 = 0) and a slight reduction in heterogeneity >30 (I2 = 71%). For route of administration, subgroup analyses found no decrease in heterogeneity for both oral (I2 = 81%) and gavage administration of berberine (I2 = 87%).
3.3.3 Effects of berberine on cognitive function in AD models: Number of MWM platform crossings
Thirteen studies used the number of platform crossings as an outcome measure, totaling 258 mice. There was a high degree of heterogeneity between the studies (I2 = 70%, p < 0.00001), so a random effects model was performed for analysis. Meta-analysis showed a significant difference between the intervention and control groups in terms of the number of platform crossings compared to the control group (SMD = 4.27, 95% CI (3.38, 5.17); p < 0.00001). (Figure 4).
Due to the heterogeneity, we further performed subgroup analyses based on animal type (mice and rats), intervention dose (<100 mg/kg, ≥100 mg/kg), duration of intervention (<14, 14–30, >30 days), and route of administration (oral administration and gavage administration). The results are shown below Table 5.
Table 5 demonstrated that different animal species, dose, duration of intervention and route of administration significantly increased the number of platform crossings compared to the control group (p < 0.05).
The subgroup analysis observed that a slight decrease in heterogeneity in mice (I2 = 53%). No change in heterogeneity in rats (I2 = 87%). For dose, no heterogeneity reduction with doses <100 mg/kg (I2 = 84%) or ≥100 mg/kg (I2 = 78%). For duration of intervention, no reduction in heterogeneity for berberine interventions <14 days (I2 = 75%) and 14–30 days (I2 = 80%), with a slight reduction in heterogeneity for berberine interventions >30 days (I2 = 56%). For route of administration, a slight decrease in heterogeneity with berberine gavage administration (I2 = 57%) and no decrease with gavage administration (I2 = 81%).
3.3.4 Effect of berberine on β-amyloid precursor protein in AD models
There were eight studies using β-amyloid precursor protein as an outcome Indicator, totaling 160 mice. There was a high heterogeneity among the studies (I2 = 90%, p < 0.0001), and therefore a random-effects model was performed for the analysis. Meta-analysis showed that there was a significant difference between the intervention and control groups compared to the control group in terms of APP (SMD = −3.28, 95% CI (−4.89, −1.68); p < 0.0001). (Figure 5).
Due to heterogeneity, we further performed subgroup analyses based on animal type (mice and rats), duration of intervention (≤30, >30 days), and route of administration (oral administration and gavage administration). The results are shown below Table 6.
Table 6 demonstrated that different animal species significantly reduced APP levels compared to the controls (p < 0.05), whereas there was no difference for berberine at ≤30 days (p = 0.05) of dosing and oral administration (p = 0.05).
The subgroup analysis observed that heterogeneity was significantly reduced in rats (I2 = 0%) but unchanged in mice (I2 = 92%). For duration of intervention, no heterogeneous reduction in berberine intervention ≤30 days (I2 = 85%) or berberine intervention >30 days (I2 = 95%). For route of administration, there was no decrease in heterogeneity for berberine administered by gavage (I2 = 94%) or by oral administration (I2 = 81%).
3.4 Publication bias
We performed publication bias tests for the four outcomes using funnel plots (Figure 6) and Egger’s test. The results show that scatters such as APP, escape latency, platform quadrant duration, and number of cross-platforms are largely outside the scope of the funnel plot, demonstrating asymmetry. Egger’s test showed potential evidence of publication bias in escape latency (p = 0.000), duration of plateau quadrant (p = 0.000), number of plateau crossings (p = 0.000), and APP (p = 0.009), suggesting the presence of publication bias. To assess the effect of publication bias for the outcomes (APP, escape latency, platform quadrant duration, and number of platform crossings, APP), we used a trim-and-fill approach. The results showed that the robustness of these outcomes was not significantly affected by publication bias (Table 7).
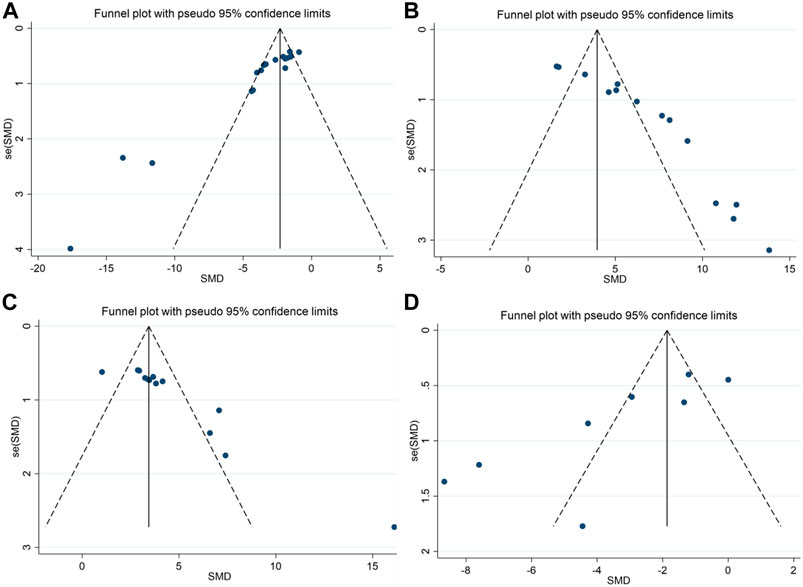
FIGURE 6. Funnel plots showing the effect of berberine on cognitive function and APP in AD models; (A) escape latency; (B) the duration in platform quadrant; (C) platform crossing number; (D) APP.
3.5 Meta-regression analysis
Publication bias was measured by escape latency as an outcome measure, and we performed univariate meta-regression to explore the sources of heterogeneity, and multivariate meta-regression with year of publication, study sample size, sex of animals, animal species (mice and rats), method of administration (oral and gavage), and duration of administration as covariates. Regression to look for sources of heterogeneity.
In the meta-regression analysis, heterogeneity in escape latency could not be determined using year of publication (β = 0.14, I2 = 83.55%, r2 = −18.16%, p = 0.531), study sample size (β = 0.52, I2 = 81.87%, r2 = 10.28%, p = 0.135), animal species (β = −0.73, I2 = 82.60%, and r2 = −25.34%, p = 0.329), animal sex (β = −0.94, I2 = 83.58%, r2 = −20.34%, p = 0.417), method of administration (β = −1.05, I2 = 83.52%, r2 = −11.67%, p = 0.583) and duration of administration (β = 0.02, I2 = 82.83%, r2 = 1.33%, p = 0.182) were explained. These covariates were not associated with heterogeneity (Supplementary Figures S1–S6).
3.6 Sensitivity analysis
We performed sensitivity analyses to test the stability of the meta and to identify sources of heterogeneity. We performed sensitivity analyses based on escape latency, number of platform crossings, time in the target quadrant, and APP outcome expression. After consecutively removing each study, the effects of the remaining studies were within the 95% CI of the total effect. This indicates that the sensitivity level of the meta-analysis was low and the results were stable and reliable (Figure 7).
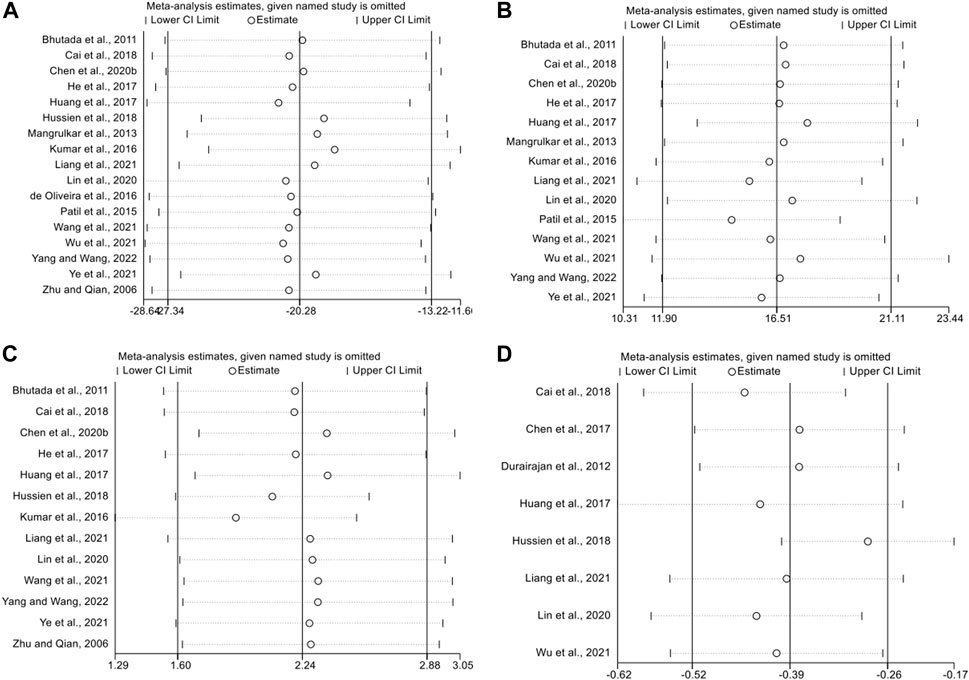
FIGURE 7. The sensitivity analysis of included studies; (A) escape latency; (B) the duration in platform quadrant; (C) platform crossing number; (D) APP.
4 Discussion
To our knowledge, this is the inaugural systematic evaluation and meta-analysis investigating the impact of berberine on cognitive function and APP in an AD animal model, employing the MWM water maze test and APP as outcome indicators. Our findings suggest that berberine has a potential impact on improving cognitive function and APP expression in AD animal models. Berberine holds promise in enhancing cognitive function and reducing APP expression in AD animal models.
Yuan et al. published the first review on the effects of berberine on AD in animal studies in 2019 (Yuan et al., 2019). The review highlighted consistent positive effects of berberine on memory deficits across various animal models, indicating its therapeutic potential for AD. However, Yuan’s review had limitations, including the inclusion of studies without experimental and animal behavioral assessments. While the overall effects were evident, the microscopic mechanisms remain unclear, necessitating further research to elucidate biochemical details and specific drug targets. Additionally, the review lacked a meta-analysis, a gap addressed by our study.
Our studies exhibited a significant degree of heterogeneity. Animal studies are typically designed to be more exploratory and heterogeneous than clinical trials. Subgroup analyses were conducted to identify the sources of heterogeneity. The subgroup analyses considered animal species, intervention duration, administration route, and dose. Following subgroup analysis, berberine’s positive effects on AD animals persisted, and some heterogeneity was effectively explained. For instance, compared to controls, berberine intervention for <14 days (SMD = 1.62, 95% CI (0.89, 2.36), p < 0.0001), I2 = 0%) and 14–28 days (SMD = 9.76, 95% CI (7.61, 11.92), p < 0.00001), I2 = 0%) significantly extended the duration in the platform quadrant, indicating that intervention time might contribute to heterogeneity. However, despite further sensitivity analyses and meta-regression analyses, the heterogeneity between studies based on escape latency and APP could not be fully elucidated, and more studies are still needed to provide more precise evidence. Eight AD models were included in this study: four transgenic animal models and four drug-induced animal models. APP/PS1 double transgenic mice were generated from two vectors. One vector encodes the APP gene carrying the Swedish (KM 670/671 NL) mutation, and the other encodes the FAD-linked PSEN 1 gene without exon 9 (dE 9) (Yokoyama et al., 2022). These mice exhibit Aβ deposition starting at 6 months, escalating at 9 months, and display impairment by 12 months, marked by an increase in neurogenic and reactive astrocytes (Kamphuis et al., 2012) with plaque deposition. APP/PS1/tau 3 × Tg-AD mice might surpass APP/PS1 double transgenic mice due to the latter’s comparatively limited pathology in hyperphosphorylated tau proteins, a significant feature associated with AD dementia severity (Yang et al., 2018). These two types of mice constituted the most utilized AD models in this study. Activated microglia in TgCRND 8 mice exhibit early accumulation of amyloid plaques at 3 months, followed by a strong astrocyte response shortly thereafter (Yokoyama et al., 2022). The B6C3-Tg transgenic mouse is an "amyloid-only" strain generating viable brain amyloid deposits and cognitive deficits (Finnie et al., 2017). While injections of Aβ, streptozotocin, colchicine, or other drugs are relatively simple and stable, the evidence of neurodegeneration in these models varies, presenting mixed research outcomes. There exists a disparity between drug-induced AD models and the intricate, multimodal pathology of human AD. Strategies divergent from the current approach need consideration to bridge this gap (Nazem et al., 2015). In essence, the existence of diverse AD models may engender substantial result heterogeneity. Experimental biases in water maze tests (Othman et al., 2022) and differences in study protocol designs, among other dissimilarities, could also contribute to this heterogeneity.
A substantial portion of the research on AD treatment with Traditional Chinese Medicine (TCM) has focused on herbs like Panax ginseng (Razgonova et al., 2019), curcumin (Bhat et al., 2019), active constituents of herbs such as polysaccharides (Zhang et al., 2022), epimedium glycoside Icariin (Yu et al., 2022), evodiamine (Pang et al., 2022) and specific therapies such as Tai Chi (Park et al., 2023), acupuncture (Cai et al., 2019), aiming to ameliorate memory and cognitive deficits in AD models. These approaches operate through diverse mechanisms, including regulation of β-amyloid and tau proteins, amelioration of cognitive deficits by preventing synaptic degeneration and neuroinflammation in AD, and modulation of AD pathology via mechanisms like antioxidant activity, anti-neuroapoptosis, or autophagy. Other nonpharmacologic interventions play an important role in improving AD development. Studies have shown that exercise increases AMPK activity and upregulation of the PGC-1α/FNDC5/BDNF pathway, which are involved in mediating the beneficial effects of exercise on Aβ-induced learning and memory deficits in mice (Azimi et al., 2018). It also prevents the decline in hippocampus-dependent cognitive function and Aβ deposition in early AD progression by modulating microglia-mediated neuroinflammation and oxidative stress (Zhang et al., 2019). Exercise programs combining aerobic (swimming) and resistance exercise, or using moderate intensity running have better results. This places greater demands on the intensity, duration, and construction of study protocols, and the exact mechanisms by which exercise training improves cognitive activity are not fully understood. There is also an acupuncture therapy that has a higher overall benefit in combination with conventional medications or other therapies (Jia et al., 2017; Wang et al., 2021a; WuLi et al., 2021). However, methodological problems from clinical trials and animal experiments affect the strength of the evidence. Therefore, more randomized clinical trials and better study designs are needed. Compared with other pharmacologic interventions, berberine has a variety of potential multi-targeted therapeutic effects for the treatment of AD, including anti-neuroinflammation, inhibition of endoplasmic reticulum stress, and improvement of the cholinergic system (Tian et al., 2023). The unique advantage of safranin is that it has been used as an anti-inflammatory and bacteriostatic drug for many years in the clinic, with effectiveness and safety (Song et al., 2020). It has been found that safranin can cross the blood-brain barrier (Wu et al., 2022b), and play a therapeutic role in central nervous system diseases. This provides a theoretical basis for the study of flavosol intervention in the treatment of AD.
AD pathology, observed microscopically in brain tissue, presents two abnormal structures: extracellular amyloid plaques and intraneuronal neurofibrillary tangles. Aβ precedes tau in the pathogenesis of AD, initiating tau’s conversion from a normal to a toxic state. Thus, early detection of plaques, tangles, and cognitive deficits, coupled with intervention to disrupt the biochemical pathways they trigger, could facilitate successful AD therapy (Bloom, 2014). The transmembrane glycoprotein, Amyloid Precursor Protein (APP), widely present in cell membranes across various tissues, significantly contributes to the Aβ pathogenic pathway. Tau strongly promotes BACE1 expression and Aβ production when interacting with APP (Zhang et al., 2021b). Aβ is derived from amyloid by sequential cleavage of β and γ-secretases (Crews et al., 2010). In the non-amyloidogenic pathway, α-secretase cleaves APP, generating N-terminal secreted APP (sAPPα) and an 83-amino-acid C-terminal fragment (CTF) (C83), which, upon further cleavage by γ-secretase, produces the 3 kDa product (P3) and the APP intracellular domain (AICD) (Wang et al., 2017). A small amount of APP is cleaved by β-secretase at Asp 1 (β-site) and Glu 11 (Crews et al., 2010). Glu 11 is the major β-cleavage site that produces the 89-amino acid CTF (C89), which is further cleaved by γ-secretase to produce the truncated Aβ1-40/42. While β-site APP cleavage enzyme 2 (BACE2) shares homology with BACE1, its role differs; it acts as a θ-secretase, cleaving APP within the Aβ structural domain, impeding Aβ production (Liu et al., 2013). Our study showed that berberine reduced APP expression in an animal model of AD, suggesting that berberine can process APP to reduce Aβ. The mechanism may be to affect the hyperphosphorylation of BACE1, Tau protein, or the terminal fragment of APP (C99) to regulate Aβ. Berberine is involved in regulating APP modification, which may inhibit Aβ production through BACE1 inhibition and regulation of γ-secretase substrates. This can adjust the preference of cleavage sites that favor Aβ reduction. However, the precise downstream mechanisms of berberine-induced APP downregulation necessitate further exploration. Additionally, in the Morris water maze, berberine treatment exhibited favorable effects on cognitive function in an AD animal model, manifesting in reduced escape latencies, prolonged platform quadrant durations, and increased platform crossings. Aβ levels in the hippocampus seemed to correlate with spatial cognitive deficits in AD (Wu et al., 2022a). The observed enhancement in spatial cognitive function due to berberine may primarily result from attenuated hippocampal Aβ. Protein phosphatase 2A (PP-2A) modulates Aβ levels by regulating APP phosphorylation and β- and γ-secretase activities (Zhang et al., 2015). It suggesting that berberine may be a good multi-targeted drug that can modulate AD related substances tau, PP-2A, Aβ, APP, or BACE-2. However, identifying the specific target among multiple targets that plays a pivotal role in berberine modulation remains a focal point for future research.
This study exhibits several limitations. First, the included studies used a variety of AD models, and the total number of studies and total sample size were relatively small, which may have affected the effectiveness of the berberine intervention. Second, in terms of study quality, the method of randomized allocation was unclear and none of the studies clearly described the methods used for generation of allocation sequences, hiding of allocation sequences, blinded interventions, and assessment of randomized outcomes. Subsequent studies should aim for more refined and comprehensive approaches in terms of high-quality research. Third, although we attempted to use pre-designed subgroup analyses, meta-regression to explore sources of heterogeneity, it seems to be ineffective. Various experimental details of different study protocols and intervention processes in animal experiments may also be the key potential factors leading to a high degree of heterogeneity. More attention should be paid to the rigor of study design and the provision of sufficient experimental information in future studies. Fourth, we did not conduct further meta-analysis on the relevant indicators because the available data for some indicators only existed in individual studies, and more attention needs to be paid to these indicators in the future.
5 Conclusion
Berberine improved cognitive dysfunction and decline of β-amyloid precursor protein in AD animals. Berberine showed significant memory-improving activity in several animal models of memory deficits by mechanisms including downregulation of APP-associated protein expression, modulation of Aβ, and effects on BACE1 and tau phosphorylation, but the validity of the findings may be affected by heterogeneity and publication bias. More rigorous experimental designs and more comprehensive studies are needed to test the protective effects of berberine in animal models of AD in the future.
Data availability statement
The original contributions presented in the study are included in the article/Supplementary Materials, further inquiries can be directed to the corresponding author.
Author contributions
J-YL: Writing–original draft, Writing–review and editing. YD: Writing–original draft, Writing–review and editing. Y-XH: Writing–review and editing. LL: Writing–original draft, Writing–review and editing.
Funding
The author(s) declare financial support was received for the research, authorship, and/or publication of this article. This work was supported partially by the Sichuan Provincial Administration of Traditional Chinese Medicine (2023MS100). This study was supported by Chengdu Medical College and Chengdu Eighth People’s Hospital.
Acknowledgments
We sincerely thank each researcher and participant for their efforts in this study.
Conflict of interest
The authors declare that the research was conducted in the absence of any commercial or financial relationships that could be construed as a potential conflict of interest.
Publisher’s note
All claims expressed in this article are solely those of the authors and do not necessarily represent those of their affiliated organizations, or those of the publisher, the editors and the reviewers. Any product that may be evaluated in this article, or claim that may be made by its manufacturer, is not guaranteed or endorsed by the publisher.
Supplementary material
The Supplementary Material for this article can be found online at: https://www.frontiersin.org/articles/10.3389/fphar.2023.1301102/full#supplementary-material
References
Azimi, M., Gharakhanlou, R., Naghdi, N., Khodadadi, D., and Heysieattalab, S. (2018). Moderate treadmill exercise ameliorates amyloid-β-induced learning and memory impairment, possibly via increasing AMPK activity and up-regulation of the PGC-1α/FNDC5/BDNF pathway. Peptides 102, 78–88. doi:10.1016/j.peptides.2017.12.027
Bhat, A., Mahalakshmi, A. M., Ray, B., Tuladhar, S., Hediyal, T. A., Manthiannem, E., et al. (2019). Benefits of curcumin in brain disorders. Biofactors 45, 666–689. doi:10.1002/biof.1533
Bhutada, P., Mundhada, Y., Bansod, K., Tawari, S., Patil, S., Dixit, P., et al. (2011). Protection of cholinergic and antioxidant system contributes to the effect of berberine ameliorating memory dysfunction in rat model of streptozotocin-induced diabetes. Behav. Brain Res. 220, 30–41. doi:10.1016/j.bbr.2011.01.022
Bloom, G. S. (2014). Amyloid-beta and tau: the trigger and bullet in Alzheimer disease pathogenesis. JAMA Neurol. 71, 505–508. doi:10.1001/jamaneurol.2013.5847
Busche, M. A., and Hyman, B. T. (2020). Synergy between amyloid-beta and tau in Alzheimer's disease. Nat. Neurosci. 23, 1183–1193. doi:10.1038/s41593-020-0687-6
Cai, M., Lee, J. H., and Yang, E. J. (2019). Electroacupuncture attenuates cognition impairment via anti-neuroinflammation in an Alzheimer's disease animal model. J. Neuroinflammation 16, 264. doi:10.1186/s12974-019-1665-3
Cai, Z., Wang, C., He, W., and Chen, Y. (2018). Berberine alleviates amyloid-beta pathology in the brain of APP/PS1 transgenic mice via inhibiting β/γ-Secretases activity and enhancing α-secretases. Curr. Alzheimer Res. 15, 1045–1052. doi:10.2174/1567205015666180702105740
Chen, S. Y., Gao, Y., Sun, J. Y., Meng, X. L., Yang, D., Fan, L. H., et al. (2020a). Traditional Chinese medicine: role in reducing beta-amyloid, apoptosis, autophagy, neuroinflammation, oxidative stress, and mitochondrial dysfunction of alzheimer's disease. Front. Pharmacol. 11, 497. doi:10.3389/fphar.2020.00497
Cheng, Z., Kang, C., Che, S., Su, J., Sun, Q., Ge, T., et al. (2022). Berberine: a promising treatment for neurodegenerative diseases. Front. Pharmacol. 13, 845591. doi:10.3389/fphar.2022.845591
Chen, Q., Mo, R., Wu, N., Zou, X., Shi, C., Gong, J., et al. (2017). Berberine ameliorates diabetes-associated cognitive decline through modulation of aberrant inflammation response and insulin signaling pathway in DM rats. Front. Pharmacol. 8, 334. doi:10.3389/fphar.2017.00334
Chen, Y., Chen, Y., Liang, Y., Chen, H., Ji, X., and Huang, M. (2020b). Berberine mitigates cognitive decline in an Alzheimer's Disease Mouse Model by targeting both tau hyperphosphorylation and autophagic clearance. Biomed. Pharmacother. 121, 109670. doi:10.1016/j.biopha.2019.109670
Crews, L., Rockenstein, E., and Masliah, E. (2010). APP transgenic modeling of Alzheimer's disease: mechanisms of neurodegeneration and aberrant neurogenesis. Brain Struct. Funct. 214, 111–126. doi:10.1007/s00429-009-0232-6
Dauphinot, V., Potashman, M., Levitchi-Benea, M., Su, R., Rubino, I., and Krolak-Salmon, P. (2022). Economic and caregiver impact of Alzheimer's disease across the disease spectrum: a cohort study. Alzheimers Res. Ther. 14, 34. doi:10.1186/s13195-022-00969-x
de Oliveira, J. S., Abdalla, F. H., Dornelles, G. L., Adefegha, S. A., Palma, T. V., Signor, C., et al. (2016). Berberine protects against memory impairment and anxiogenic-like behavior in rats submitted to sporadic Alzheimer's-like dementia: involvement of acetylcholinesterase and cell death. Neurotoxicology 57, 241–250. doi:10.1016/j.neuro.2016.10.008
Durairajan, S. S., Liu, L. F., Lu, J. H., Chen, L. L., Yuan, Q., Chung, S. K., et al. (2012). Berberine ameliorates beta-amyloid pathology, gliosis, and cognitive impairment in an Alzheimer's disease transgenic mouse model. Neurobiol. Aging 33, 2903–2919. doi:10.1016/j.neurobiolaging.2012.02.016
Fang, Z., Tang, Y., Ying, J., Tang, C., and Wang, Q. (2020). Traditional Chinese medicine for anti-Alzheimer's disease: berberine and evodiamine from Evodia rutaecarpa. Chin. Med. 15, 82. doi:10.1186/s13020-020-00359-1
Finnie, G. S., Gunnarsson, R., Manavis, J., Blumbergs, P. C., Mander, K. A., Edwards, S., et al. (2017). Characterization of an 'amyloid only' transgenic (B6C3-tg(APPswe,PSEN1dE9)85Dbo/mmjax) mouse model of alzheimer's disease. J. Comp. Pathol. 156, 389–399. doi:10.1016/j.jcpa.2017.03.001
Griffiths, J., and Grant, S. G. N. (2023). Synapse pathology in Alzheimer's disease. Semin. Cell. Dev. Biol. 139, 13–23. doi:10.1016/j.semcdb.2022.05.028
Guo, Q., Wang, C., Xue, X., Hu, B., and Bao, H. (2021a). SOCS1 mediates berberine-induced amelioration of microglial activated states in N9 microglia exposed to beta amyloid. Biomed. Res. Int. 2021, 9311855. doi:10.1155/2021/9311855
Guo, Y., Wang, Q., Chen, S., and Xu, C. (2021b). Functions of amyloid precursor protein in metabolic diseases. Metabolism 115, 154454. doi:10.1016/j.metabol.2020.154454
He, W., Wang, C., Chen, Y., He, Y., and Cai, Z. (2017). Berberine attenuates cognitive impairment and ameliorates tau hyperphosphorylation by limiting the self-perpetuating pathogenic cycle between NF-κB signaling, oxidative stress and neuroinflammation. Pharmacol. Rep. 69, 1341–1348. doi:10.1016/j.pharep.2017.06.006
Hooijmans, C. R., Rovers, M. M., de Vries, R. B., Leenaars, M., Ritskes-Hoitinga, M., and Langendam, M. W. (2014). SYRCLE's risk of bias tool for animal studies. BMC Med. Res. Methodol. 14, 43. doi:10.1186/1471-2288-14-43
Huang, M., Jiang, X., Liang, Y., Liu, Q., Chen, S., and Guo, Y. (2017). Berberine improves cognitive impairment by promoting autophagic clearance and inhibiting production of beta-amyloid in APP/tau/PS1 mouse model of Alzheimer's disease. Exp. Gerontol. 91, 25–33. doi:10.1016/j.exger.2017.02.004
Hussien, H. M., Abd-Elmegied, A., Ghareeb, D. A., Hafez, H. S., Ahmed, H. E. A., and el-Moneam, N. A. (2018). Neuroprotective effect of berberine against environmental heavy metals-induced neurotoxicity and Alzheimer's-like disease in rats. Food Chem. Toxicol. 111, 432–444. doi:10.1016/j.fct.2017.11.025
Jia, L., Quan, M., Fu, Y., Zhao, T., Li, Y., Wei, C., et al. (2020). Dementia in China: epidemiology, clinical management, and research advances. Lancet Neurol. 19, 81–92. doi:10.1016/S1474-4422(19)30290-X
Jia, Y., Zhang, X., Yu, J., Han, J., Yu, T., Shi, J., et al. (2017). Acupuncture for patients with mild to moderate Alzheimer's disease: a randomized controlled trial. BMC Complement. Altern. Med. 17, 556. doi:10.1186/s12906-017-2064-x
Kamphuis, W., Mamber, C., Moeton, M., Kooijman, L., Sluijs, J. A., Jansen, A. H., et al. (2012). GFAP isoforms in adult mouse brain with a focus on neurogenic astrocytes and reactive astrogliosis in mouse models of Alzheimer disease. PLoS One 7, e42823. doi:10.1371/journal.pone.0042823
Kim, D., and Tsai, L. H. (2009). Bridging physiology and pathology in AD. Cell. 137, 997–1000. doi:10.1016/j.cell.2009.05.042
Kumar, A., Ekavali, M. J., Chopra, K., and Dhull, D. K. (2016). Possible role of P-glycoprotein in the neuroprotective mechanism of berberine in intracerebroventricular streptozotocin-induced cognitive dysfunction. Psychopharmacol. Berl. 233, 137–152. doi:10.1007/s00213-015-4095-7
Leng, F., and Edison, P. (2021). Neuroinflammation and microglial activation in Alzheimer disease: where do we go from here? Nat. Rev. Neurol. 17, 157–172. doi:10.1038/s41582-020-00435-y
Liang, Y., Ye, C., Chen, Y., Chen, Y., Diao, S., and Huang, M. (2021). Berberine improves behavioral and cognitive deficits in a mouse model of alzheimer's disease via regulation of beta-amyloid production and endoplasmic reticulum stress. ACS Chem. Neurosci. 12, 1894–1904. doi:10.1021/acschemneuro.0c00808
Lin, L., Li, C., Zhang, D., Yuan, M., Chen, C. H., and Li, M. (2020). Synergic effects of berberine and curcumin on improving cognitive function in an alzheimer's disease mouse model. Neurochem. Res. 45, 1130–1141. doi:10.1007/s11064-020-02992-6
Liu, K. Y., and Howard, R. (2021). Can we learn lessons from the FDA's approval of aducanumab? Nat. Rev. Neurol. 17, 715–722. doi:10.1038/s41582-021-00557-x
Liu, X., Wang, Z., Wu, Y., Wang, J., and Song, W. (2013). BACE2 degradation mediated by the macroautophagy-lysosome pathway. Eur. J. Neurosci. 37, 1970–1977. doi:10.1111/ejn.12204
Mangrulkar, S., Selote, R., Chaple, D., and Chourasia, A. J. (2013). Antiamnesic effect of berberine in colchicines induced experimental alzheimer's disease model. Int. J. Pharma Bio Sci. 4, 618–628.
Morton, H., Kshirsagar, S., Orlov, E., Bunquin, L. E., Sawant, N., Boleng, L., et al. (2021). Defective mitophagy and synaptic degeneration in Alzheimer's disease: focus on aging, mitochondria and synapse. Free Radic. Biol. Med. 172, 652–667. doi:10.1016/j.freeradbiomed.2021.07.013
Nazem, A., Sankowski, R., Bacher, M., and al-Abed, Y. (2015). Rodent models of neuroinflammation for Alzheimer's disease. J. Neuroinflammation 12, 74. doi:10.1186/s12974-015-0291-y
Othman, M. Z., Hassan, Z., and Che Has, A. T. (2022). Morris water maze: a versatile and pertinent tool for assessing spatial learning and memory. Exp. Anim. 71, 264–280. doi:10.1538/expanim.21-0120
Pang, S., Li, S., Cheng, H., Luo, Z., Qi, X., Guan, F., et al. (2022). Discovery of an evodiamine derivative for PI3K/AKT/GSK3β pathway activation and AD pathology improvement in mouse models. Front. Mol. Neurosci. 15, 1025066. doi:10.3389/fnmol.2022.1025066
Park, M., Song, R., Ju, K., Shin, J. C., Seo, J., Fan, X., et al. (2023). Effects of Tai Chi and Qigong on cognitive and physical functions in older adults: systematic review, meta-analysis, and meta-regression of randomized clinical trials. BMC Geriatr. 23, 352. doi:10.1186/s12877-023-04070-2
Patil, S., Tawari, S., Mundhada, D., and Nadeem, S. (2015). Protective effect of berberine, an isoquinoline alkaloid ameliorates ethanol-induced oxidative stress and memory dysfunction in rats. Pharmacol. Biochem. Behav. 136, 13–20. doi:10.1016/j.pbb.2015.07.001
Razgonova, M. P., Veselov, V. V., Zakharenko, A. M., Golokhvast, K. S., Nosyrev, A. E., Cravotto, G., et al. (2019). Panax ginseng components and the pathogenesis of Alzheimer's disease (Review). Mol. Med. Rep. 19, 2975–2998. doi:10.3892/mmr.2019.9972
Scheltens, P., de Strooper, B., Kivipelto, M., Holstege, H., Chetelat, G., Teunissen, C. E., et al. (2021). Alzheimer's disease. Lancet 397, 1577–1590. doi:10.1016/S0140-6736(20)32205-4
Silva, T., Reis, J., Teixeira, J., and Borges, F. (2014). Alzheimer's disease, enzyme targets and drug discovery struggles: from natural products to drug prototypes. Ageing Res. Rev. 15, 116–145. doi:10.1016/j.arr.2014.03.008
Song, D., Hao, J., and Fan, D. (2020). Biological properties and clinical applications of berberine. Front. Med. 14, 564–582. doi:10.1007/s11684-019-0724-6
Tian, E., Sharma, G., and Dai, C. (2023). Neuroprotective properties of berberine: molecular mechanisms and clinical implications, 12. Basel: Antioxidants.
Tonnies, E., and Trushina, E. (2017). Oxidative stress, synaptic dysfunction, and alzheimer's disease. J. Alzheimers Dis. 57, 1105–1121. doi:10.3233/JAD-161088
Wang, X. S., Li, J. J., Wang, Y. S., Yu, C. C., He, C., Huang, Z. S., et al. (2021a). Acupuncture and related therapies for the cognitive function of alzheimer's disease: a network meta-analysis. Iran. J. Public Health 50, 2411–2426. doi:10.18502/ijph.v50i12.7924
Wang, Y. Y., Yan, Q., Huang, Z. T., Zou, Q., Li, J., Yuan, M. H., et al. (2021b). Ameliorating ribosylation-induced amyloid-beta pathology by berberine via inhibiting mTOR/p70S6K signaling. J. Alzheimers Dis. 79, 833–844. doi:10.3233/JAD-200995
Wang, X., Zhou, X., Li, G., Zhang, Y., Wu, Y., and Song, W. (2017). Modifications and trafficking of APP in the pathogenesis of alzheimer's disease. Front. Mol. Neurosci. 10, 294. doi:10.3389/fnmol.2017.00294
Wuli, W., Harn, H. J., Chiou, T. W., and Lin, S. Z. (2021). Chinese herbs and acupuncture to improve cognitive function in Alzheimer's disease. Tzu Chi Med. J. 33, 122–127. doi:10.4103/tcmj.tcmj_51_20
Wu, T., Lin, D., Cheng, Y., Jiang, S., Riaz, M. W., Fu, N., et al. (2022a). Amyloid cascade hypothesis for the treatment of alzheimer's disease: progress and challenges. Aging Dis. 13, 1745–1758. doi:10.14336/AD.2022.0412
Wu, X., Liu, X., Yang, L., and Wang, Y. (2022b). Berberine protects against neurological impairments and blood-brain barrier injury in mouse model of intracerebral hemorrhage. Neuroimmunomodulation 29, 317–326. doi:10.1159/000520747
Wu, Y., Chen, Q., Wen, B., Wu, N., He, B., and Chen, J. (2021). Berberine reduces Aβ42 deposition and tau hyperphosphorylation via ameliorating endoplasmic reticulum stress. Front. Pharmacol. 12, 640758. doi:10.3389/fphar.2021.640758
Yang, J. T., Wang, Z. J., Cai, H. Y., Yuan, L., Hu, M. M., Wu, M. N., et al. (2018). Sex differences in neuropathology and cognitive behavior in APP/PS1/tau triple-transgenic mouse model of alzheimer's disease. Neurosci. Bull. 34, 736–746. doi:10.1007/s12264-018-0268-9
Yang, M., and Wang, J. (2022). Berberine ameliorates cognitive disorder via gsk3β/PGC-1α signaling in APP/PS1 mice. J. Nutr. Sci. Vitaminol. (Tokyo) 68, 228–235. doi:10.3177/jnsv.68.228
Yao, J., Wei, W., Wen, J., Cao, Y., and Li, H. (2023). The efficacy and mechanism of berberine in improving aging-related cognitive dysfunction: a study based on network pharmacology. Front. Neurosci. 17, 1093180. doi:10.3389/fnins.2023.1093180
Ye, C., Liang, Y., Chen, Y., Xiong, Y., She, Y., Zhong, X., et al. (2021). Berberine improves cognitive impairment by simultaneously impacting cerebral blood flow and beta-amyloid accumulation in an APP/tau/PS1 mouse model of alzheimer's disease. Cells, 10.
Yokoyama, M., Kobayashi, H., Tatsumi, L., and Tomita, T. (2022). Mouse models of alzheimer's disease. Front. Mol. Neurosci. 15, 912995. doi:10.3389/fnmol.2022.912995
Yuan, N. N., Cai, C. Z., Wu, M. Y., Su, H. X., Li, M., and Lu, J. H. (2019). Neuroprotective effects of berberine in animal models of Alzheimer's disease: a systematic review of pre-clinical studies. BMC Complement. Altern. Med. 19, 109. doi:10.1186/s12906-019-2510-z
Yu, H., Shi, J., Lin, Y., Zhang, Y., Luo, Q., Huang, S., et al. (2022). Icariin ameliorates alzheimer's disease pathology by alleviating myelin injury in 3 × Tg-AD mice. Neurochem. Res. 47, 1049–1059. doi:10.1007/s11064-021-03507-7
Zhang, J. H., Zhang, J. F., Song, J., Bai, Y., Deng, L., Feng, C. P., et al. (2021a). Effects of berberine on diabetes and cognitive impairment in an animal model: the mechanisms of action. Am. J. Chin. Med. 49, 1399–1415. doi:10.1142/S0192415X21500658
Zhang, J. W., Jing, L. J., Jian, G., and Dong, G. C. (2015). Mechanism of protein phosphatase-2Aregulating phosphorylation of amyloid precursor proteosome and Aβ generation. Bratisl. Lek. Listy 116, 184–190. doi:10.4149/bll_2015_037
Zhang, N., Gao, Y., Yu, S., Sun, X., and Shen, K. (2020). Berberine attenuates Aβ42-induced neuronal damage through regulating circHDAC9/miR-142-5p axis in human neuronal cells. Life Sci. 252, 117637. doi:10.1016/j.lfs.2020.117637
Zhang, X., He, Q., Huang, T., Zhao, N., Liang, F., Xu, B., et al. (2019). Treadmill exercise decreases Aβ deposition and counteracts cognitive decline in APP/PS1 mice, possibly via hippocampal microglia modifications. Front. Aging Neurosci. 11, 78. doi:10.3389/fnagi.2019.00078
Zhang, X., Lin, L., Li, H., Xia, W., Liu, Q., Zhou, X., et al. (2022). Update on new trend and progress of the mechanism of polysaccharides in the intervention of Alzheimer's disease, based on the new understanding of relevant theories: a review. Int. J. Biol. Macromol. 218, 720–738. doi:10.1016/j.ijbiomac.2022.07.158
Zhang, Z., Li, X. G., Wang, Z. H., Song, M., Yu, S. P., Kang, S. S., et al. (2021b). δ-Secretase-cleaved Tau stimulates Aβ production via upregulating STAT1-BACE1 signaling in Alzheimer's disease. Mol. Psychiatry 26, 586–603. doi:10.1038/s41380-018-0286-z
Keywords: berberine, cognitive function, β-amyloid precursor protein, Alzheimer’s disease, animal models, meta-analysis
Citation: Liu J-Y, Dai Y, He Y-X and Lin L (2024) Effect of berberine on cognitive function and β-amyloid precursor protein in Alzheimer’s disease models: a systematic review and meta-analysis. Front. Pharmacol. 14:1301102. doi: 10.3389/fphar.2023.1301102
Received: 02 October 2023; Accepted: 15 December 2023;
Published: 16 January 2024.
Edited by:
Arman Zargaran, Tehran University of Medical Sciences, IranReviewed by:
Nazli Namazi, Tabriz University of Medical Sciences, IranArdalan Shariat, Tehran University of Medical Sciences, Iran
Copyright © 2024 Liu, Dai, He and Lin. This is an open-access article distributed under the terms of the Creative Commons Attribution License (CC BY). The use, distribution or reproduction in other forums is permitted, provided the original author(s) and the copyright owner(s) are credited and that the original publication in this journal is cited, in accordance with accepted academic practice. No use, distribution or reproduction is permitted which does not comply with these terms.
*Correspondence: Lin Lin, MTAwMDU2OUBjbWMuZWR1LmNu