- 1INSERM U1151, Institut Necker Enfants Malades, Paris, France
- 2Université de Paris-Cité, Paris, France
- 3Centre de Référence Maladie Rare Pour La Mucoviscidose et Maladies de CFTR, Hôpital Necker Enfants Malades, Assistance Publique Hôpitaux de Paris, Paris, France
- 4Department of Physics and Biophysics, Institute of Biology, Warsaw University of Life Sciences, Warsaw, Poland
- 5Telethon Institute of Genetics and Medicine, Pozzuoli, Italy
- 6U1138/CNRS ERL 8228, Centre de Recherche des Cordeliers, Paris, France
- 7Service de Médecine Génomique des Maladies de Système et d’Organe, Hôpital Cochin, Paris, France
- 8European Reference Network for Rare Diseases, Frankfurt, Belgium
Introduction: Cystic fibrosis (CF) is caused by defective Cystic Fibrosis Transmembrane Conductance Regulator (CFTR) proteins. CFTR controls chloride (Cl−) and bicarbonate (HCO3−) transport into the Airway Surface Liquid (ASL). We investigated the impact of F508del-CFTR correction on HCO3− secretion by studying transepithelial HCO3− fluxes.
Methods: HCO3− secretion was measured by pH-stat technique in primary human respiratory epithelial cells from healthy subjects (WT) and people with CF (pwCF) carrying at least one F508del variant. Its changes after CFTR modulation by the triple combination VX445/661/770 and in the context of TNF-α+IL-17 induced inflammation were correlated to ASL pH and transcriptional levels of CFTR and other HCO3− transporters of airway epithelia such as SLC26A4 (Pendrin), SLC26A9 and NBCe1.
Results: CFTR-mediated HCO3− secretion was not detected in F508del primary human respiratory epithelial cells. It was rescued up to ∼ 80% of the WT level by VX-445/661/770. In contrast, TNF-α+IL-17 normalized transepithelial HCO3− transport and increased ASL pH. This was related to an increase in SLC26A4 and CFTR transcript levels. VX-445/661/770 induced an increase in pH only in the context of inflammation. Effects on HCO3− transport were not different between F508del homozygous and F508del compound heterozygous CF airway epithelia.
Conclusion: Our studies show that correction of F508del-CFTR HCO3− is not sufficient to buffer acidic ASL and inflammation is a key regulator of HCO3− secretion in CF airways. Prediction of the response to CFTR modulators by theratyping should take into account airway inflammation.
1 Introduction
The airway epithelium interfaces between the internal milieu and the external environment. It is lined by a thin layer of fluid called the airway surface liquid (ASL), whose composition is finely tuned. There is increasing evidence that defects in ASL pH regulation are associated with various respiratory diseases, including cystic fibrosis (CF) (Coakley et al., 2003). CF is a life-limiting disease caused by mutations in the cystic fibrosis transmembrane conductance regulator (CFTR) gene. The most frequent mutation, p. Phe508del, F508del thereafter, is carried at least on one allele by around 80% of people with CF (pwCF) worldwide and results in defective CFTR protein trafficking due to protein misfolding, reduced stability at the cell surface, and dysfunctional channel gating (Pranke et al., 2019).
CFTR transports two of the most abundant and physiologically important anions, namely, chloride (Cl−) and bicarbonate (HCO3−) into the ASL (Quinton, 2008). HCO3− plays a key role in epithelial surface homeostasis by ensuring adequate water content, controlling the volume and pH of the periciliary layer, and regulating mucin biophysical properties such as hydration. All these factors are necessary for efficient mucociliary clearance (MCC) (Quinton, 2008; Bridges, 2012; Gorrieri et al., 2016; Tang et al., 2016; Ferrera et al., 2021). Mutations of the CFTR channel have mainly been characterized by their disruption of Cl− transport; however, they also display defective HCO3− transport, which is intimately related to CF disease (Quinton, 2001). At the pulmonary level, deficient HCO3− secretion was shown to decrease ASL hydration and reduce its pH, which further compromises mucin formation and mucociliary clearance and impairs the airway host defenses (Garland et al., 2013; Hoegger et al., 2014; Shah et al., 2016; Simonin et al., 2019). The addition of HCO3− onto the CF epithelium was shown to increase ASL height and pH (Garland et al., 2013), improve mucus viscoelastic properties (Gomez et al., 2020; Ferrera et al., 2021), restore bacterial killing (Pezzulo et al., 2012), and impede the growth and biofilm formation of pulmonary bacterial pathogens (Dobay et al., 2018).
Highly effective CFTR modulator therapy (HEMT) enables partial F508del-CFTR functional restoration. Triple combination HEMT combines two corrector molecules, namely, elexacaftor (VX-445) and tezacaftor (VX-661), to process misfolded F508del-CFTR protein to the cell membrane and a potentiator, ivacaftor (VX-770), to increase channel opening. Phase 3 clinical trials with elexacaftor/tezacaftor/ivacaftor (ETI) have shown dramatic improvement in lung disease and a strong positive impact on the quality of life for patients carrying at least one F508del-CFTR allele (Middleton et al., 2019). This involves an improvement in CFTR-dependent Cl− transport, resulting in better hydration of airway secretions (Morrison et al., 2022). Interestingly, Rehman et al. showed that ETI induced an increase in pH, but this was observed only in the context of inflammation with the addition of TNF-α+IL-17 to the CF epithelium (Rehman et al., 2020; Rehman et al., 2021). According to the authors, the failure of F508del-CFTR correction to improve pH value in the absence of inflammation was unexpected and suggested that CFTR-dependent HCO3− secretion and its role in ASL pH control were dependent on the inflammatory status.
These studies, however, relied on ASL pH variation, which involves both transepithelial HCO3− and H+ flux. To dissect the role of CFTR on pH homeostasis and the potential impact of CFTR correction in the CF lung environment, we characterized HCO3− transepithelial flux and ASL pH changes in healthy and CF epithelium and evaluated the impact of the F508del-CFTR rescue in the context of inflammation.
2 Materials and methods
2.1 Patients
Respiratory airway cell sampling was performed in CF patients and healthy subjects (WT) (Clinical Trials: NCT02965326). The study was approved by the Ile de France 2 Ethics Committee, and written informed consent was obtained from each adult and both parents for participants below 18 years of age (AFSSAPS (ANSM) B1005423-40, EudraCT no. 2010-A00392-37; CPP IDF2: 2010-05-03-3).
2.2 Cell culture
Human bronchial epithelial cells were isolated from bronchial explants (one to two bifurcations) by enzymatic digestion as previously described (Golec et al., 2022). Cells were first grown in T75 cm2 flasks in DMEM/F-12 cell culture medium supplemented with 10% newborn calf serum, Rho kinase inhibitor Y-27632 (10 μM), piperacillin/tazobactam 90µg/10 μg/mL, colimycin 16 μg/mL, and amphotericin B 5 μg/mL, and additional growth factors. For air–liquid interface (ALI) culture, 350,000 human respiratory airway expanded cells suspended in an amplification medium were seeded on type IV collagen-coated porous filter with a 0.33 cm2 surface (6.5 mm diameter, 0.4 μm pore size, Corning, Tewksbury, MA). UG2% medium (DMEM/F-12, supplemented with 2% Ultroser G) containing antibiotics, as mentioned above, was added to the basal side of the filters. After 72 h of liquid/liquid interface, apical media were removed, and cells were cultured in ALI with UG2% basal medium changed every 48–72 h for 3–4 weeks to establish a differentiated epithelium. The transepithelial electrical resistance (RT) of cultures was measured using a chopstick voltmeter (Millicell ERS).
Prior to functional studies, cells were incubated at the basolateral side for 48 h with DMSO 0.03% (referred to as the control condition) or VX-770 (100 nM), VX-445 (3 µM), and VX-661 (3 µM), referred to as VX-445/661/770 (Selleckchem), all dissolved in DMSO. To assess inflammation-induced responses, epithelia were treated on the basolateral side with 10 ng/mL TNF-α (R&D Systems) and 20 ng/mL IL-17 (R&D Systems) for 48 h.
2.3 Measurement of HCO3− fluxes
To investigate HCO3− transepithelial transport, HCO3− secretion rates were monitored in Ussing chambers under open-circuit conditions by the pH-stat method. A mini pH electrode connected to an automated titration workstation, a temperature sensor, and a burette (all from Radiometer Analytical) were introduced to the mucosal chamber to automatically titrate 5 mM HCl to maintain the pH of the solution at 7.000 ± 0.005. TitraMaster 85 software was used to control the rate of titration, amount of titrant added, and continuous measurement of solution pH. The rate of HCO3− secretion [µEq∙h-1∙cm-2] was calculated in 5-min intervals by noting the amount of titrant added, its concentration, and the cell surface area. HCO3− secretion rates were calculated 20–40 min after the addition of each activator/inhibitor and considered stable when remaining constant for at least 15 min. The solution composition used for pH-stat measurements is provided in Supplementary Table S1. The apical solution was vigorously bubbled with pure O2, while the basolateral solution was bubbled with carbogen (95% O2 and 5% CO2). The HCO3− secretion rates were monitored in the presence of the ENaC channel blocker amiloride (100µM, Spectrum Chemical) (amiloride secretion rate) and after the subsequent addition of activators or inhibitors of ion-transporting proteins to determine their involvement in HCO3− transport across the epithelium. This included cAMP agonists forskolin (Fsk) (10µM, Sigma-Aldrich), M3-isobutyl-1-methylxanthine (IBMX) (100µM, Sigma-Aldrich Merck), and genistein (10µM, Sigma-Aldrich Merck) to activate and potentiate CFTR activity, the specific CFTR inhibitor Inh-172 (10µM, Sigma-Aldrich Merck), and in the case of remaining HCO3− flux, the SLC26A4 inhibitor YS-01 (5µM, AOBIOUS) and GlyH-101 (10µM, Sigma-Aldrich Merck). This allowed evaluation of the cAMP-activated HCO3− flux (Fsk/IBMX + genistein) and its inhibition by Inh-172, GlyH-101, and YS-01. As CFTR may be partially activated at the basal state, the change after CFTR inhibition was considered the index of CFTR activity.
2.4 Measurement of ASL pH
In order to measure ASL pH under physiological conditions, we designed a system with a controlled atmosphere enclosure enabling us to maintain humidity, pCO2 at 5%, and temperature at 37°C as previously described (Simonin et al., 2019). ASL pH measurements were performed in the presence of the cAMP agonist Fsk (10µM, Sigma-Aldrich), and IBMX (100µM, Sigma-Aldrich Merck) was added to the basolateral solution to stimulate CFTR. As the ASL layer was too thin to allow direct and reproducible measurement of pH without risking disruption, the pH was measured after the addition of the 50 µL solution at the cell monolayer’s apical side. The solution composition was used for physiological conditions, and Cl−-free conditions are provided in Supplementary Table S2. This 50 µL solution, representing diluted ASL, was harvested after 8 h of incubation, and the pH was measured immediately, directly in the controlled atmosphere enclosure with a micro-combination pH electrode (Thermo Fisher Scientific Orion 9810BN, Illkirch, Grand Est, France). Prior to each experiment, the pH microelectrode was calibrated with pH 4 and pH 7 buffers. Experimental solutions were equilibrated at pH 7.4. We previously showed that in this setup, the measured pH value did not differ by more than 0.03 pH units from the theoretical one calculated according to the Henderson–Hasselbalch equation (Simonin et al., 2019).
2.5 RT–PCR genetic analysis
A measure of 200 ng of total RNA was reverse-transcribed (Thermo Fischer Scientific). Real-time quantitative PCR was performed on a LightCycler (Roche Diagnostics) using the LightCyclerFastStart DNA Master SYBR Green 1 kit (Roche Diagnostics) according to the manufacturer’s protocols, except that the reaction volume was reduced 2.5-fold. Specific primers were designed using Primer 3 (free online software; Supplementary Table S3). In each run, a standard curve was obtained using a serial dilution of stock cDNA prepared from a mix of different samples of total RNA. The expression of the CFTR, SLC26A4, NBCE1, and SLC26A9 transcripts were normalized to cyclophilin-A (PPIA) expression (the mean threshold cycle for PPIA = 27.5 ± 0.1).
2.6 Statistical analysis
Statistical analysis was performed using GraphPad Prism® software. Data are presented as mean ± (SD), and the “n\” value represents one filter. Comparisons were performed with the Wilcoxon matched-pair signed-rank test or Mann–Whitney test as appropriate. Correlations were assessed with Spearman’s test. A significant value was retained if p < 0.05.
3 Results
3.1 VX-445/661/770 increases the CFTR HCO3− flux
pH-stat experiments enabled us to quantify the HCO3− secretion rate. Reference HCO3− secretion rates in the wild-type (WT) respiratory epithelium are shown in Figure 1 and Supplementary Table S4. Amiloride secretion rate across WT respiratory cells (0.24 ± 0.04 µEq∙h-1∙cm-2) was increased by Fsk/IBMX + genistein (up to 0.51 ± 0.04µEq∙h-1∙cm-2) with subsequent complete inhibition by Inh-172.
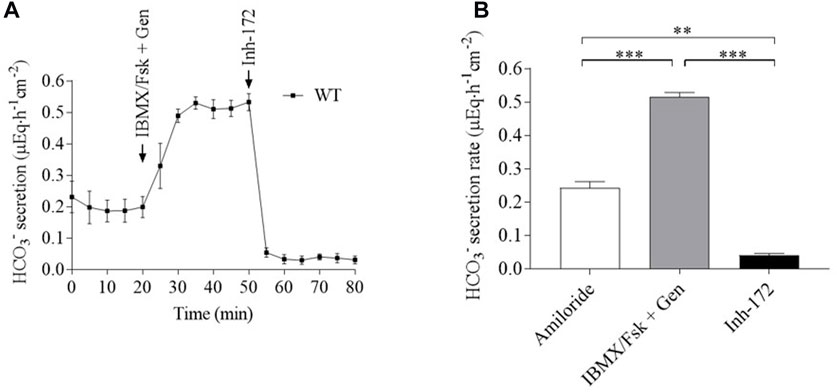
FIGURE 1. Bicarbonate secretion in the respiratory epithelium expressing WT CFTR. HCO3− flux is measured in the WT human respiratory epithelium by pH-stat in the presence of amiloride and after the successive addition of Fsk/IBMX (10µM/100 µM) + genistein (Gen) (100 µM) and Inh-172 (10 µM). (A) Representative tracing and (B) summary of five experiments. Results are presented as mean ± SD (n = 5). A comparison was carried out by the Wilcoxon matched-pair signed-rank test.**p < 0.01; ***p < 0.001.
Representative tracings of HCO3− fluxes in F508del human respiratory epithelial cells under DMSO and VX-445/661/770 conditions are shown in Figure 2A. Control DMSO-treated airway epithelia displayed no HCO3− secretion either in the presence of amiloride or after CFTR activation by Fsk/IBMX + genistein. In contrast, VX-445/661/770 treatment elevated HCO3− secretion, reaching 78% ± 12% (0.18 ± 0.03 µEq∙h-1∙cm-2) of the secretion level observed in WT human respiratory epithelial cells (Figures 2A, B). The addition of Fsk/IBMX + genistein increased CFTR-dependent HCO3− secretion up to 70% ± 29% of the WT level (Figures 2A, C, and Supplementary Table S5). Inh-172 completely inhibited this HCO3− secretion, providing evidence that it was due to CFTR (Figures 2A, D, and Supplementary Table S5). There was no significant difference in HCO3− secretion rates between homozygous and heterozygous F508del cells (Figures 2B, C, D, and Supplementary Table S4).
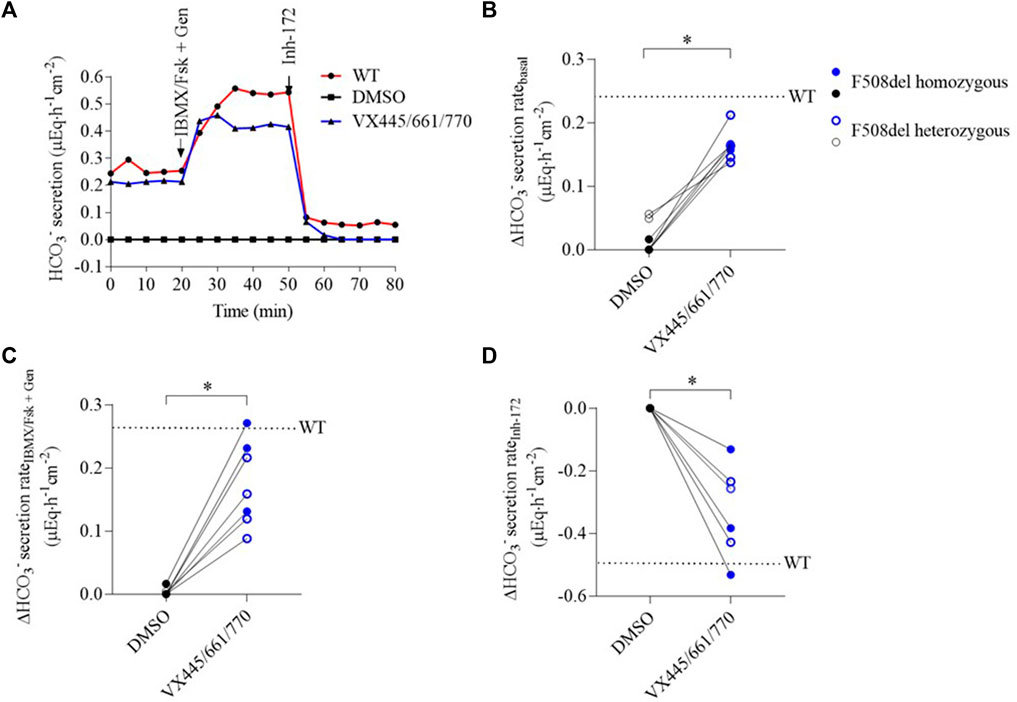
FIGURE 2. VX-445/661/770 increases F508del-CFTR bicarbonate secretion in respiratory epithelial cells. HCO3− flux is measured in WT and F508del airway epithelia by pH-stat at baseline in the presence of amiloride and after the successive addition of Fsk/IBMX (10µM/100 µM) + Gen (100 µM) and Inh-172 (10 µM). Tracings were recorded in WT cells (red) and CF cells after a 48-h incubation with DMSO (black) or VX-445 (3 µM)/VX-661 (3 µM)/VX-770 (100 nM) (blue). (A) Representative tracing of the pH-stat experiment of human respiratory epithelial cells from healthy control and a patient carrying a homozygous F508del mutation. (B) Individual HCO3− secretion rate changes in the presence of amiloride (100 µM) between DMSO- and VX-445/661/770-treated human respiratory epithelial cells (n = 8). The dotted line represents the mean baseline secretion rate of WT cells. F508del homozygous human respiratory epithelial cells are represented as full circles and heterozygous cells as open circles. (C) Individual HCO3− secretion rate changes in response to Fsk/IBMX + genistein after a 48-h incubation of human respiratory epithelial cells (n = 7) with DMSO or VX-445/661/770. The dotted line represents the mean of WT secretion rate change. (D) Individual HCO3− secretion rate changes in response to Inh-172 after a 48-h incubation of human respiratory epithelial cells (n = 7) with DMSO or VX-445/661/770. The dotted line represents the mean of the WT secretion rate change. A comparison was carried out by the Wilcoxon matched-pair signed-rank test.*p < 0.05.
3.2 Inflammation enhances CFTR HCO3− transport correction in F508del human respiratory epithelial cells
We next investigated the effect of inflammation alone and in combination with VX-445/661/770 on HCO3− secretion (Figure 3, Supplementary Table S5). Incubation with IL-17/TNF-α increased HCO3− secretion above the WT level in the presence of amiloride (comparison with WT and NS) (Figures 3A, B, Supplementary Table S5). The change after stimulation by Fsk/IBMX + genistein was up to 87% ± 41% of the WT (Figures 3A, C, Supplementary Table S5). HCO3− flux was partially inhibited by Inh-172, and complete inhibition was achieved by the subsequent addition of YS-01 (SLC26A4 inhibitor) and GlyH-101 (respective part in the inhibition of Inh-172, YS-01, and GlyH-101: 37% ± 2%; 33% ± 1.5%; and 29% ± 1%) (Figures 3A, D, and Supplementary Table S5).
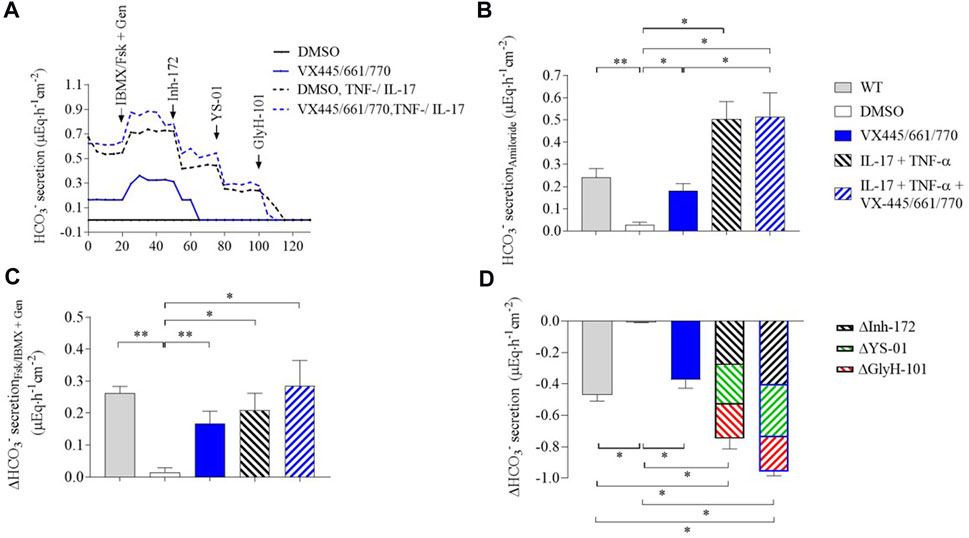
FIGURE 3. Inflammation increases bicarbonate transport in human respiratory epithelial cells. HCO3− flux is measured in WT and F508del human respiratory epithelial cells by pH-stat at baseline in the presence of amiloride and after the successive addition of Fsk//IBMX (10µM/100 µM) + genistein (100 µM) and Inh-172 (10 µM), YS-01 (5 µM), and GlyH-101 (10 µM). CF epithelia were from two F508del homozygous patients and two F508del patients in trans with a minimal function mutation (R560K and 1717 + 1G>T). Tracings were recorded after 48-h incubation with DMSO (black line), VX-445 (3 µM)/VX-661 (3 µM)/VX-770 (100 nM) (blue plain line), TNF-α(10 ng/mL)/IL-17 (20 ng/mL) (black dashed line), and TNF-α/IL-17 + VX-445/661/770 (blue dashed line). Representative tracing of the pH-stat experiment of human respiratory epithelial cells from an F508del homozygous patient. HCO3− secretion in the presence of amiloride in WT and CF human respiratory epithelial cells after a 48-h incubation with DMSO, VX-445/661/770, TNF-α/IL-17, and TNF-α/IL-17 + VX-445/661/770. HCO3− secretion rate changes in response to Fsk/IBMX + genistein in WT and CF human respiratory epithelial cells after a 48-h incubation in DMSO, VX-445/661/770, TNF-α/IL-17, and TNF-α/IL-17 + VX-445/661/770. (D) HCO3− secretion rate changes after Fsk/IBMX + genistein activation in response to Inh-172 (black lines), YS-01 (green lines), and GlyH-101 (red lines) in WT and CF human respiratory epithelial cells after a 48-h incubation in DMSO, VX-445/661/770, TNF-α/IL-17, and TNF-α/IL-17 + VX-445/661/770. A comparison was carried out by the Mann–Whitney signed-rank test for WT versus CF cells and the Wilcoxon matched-pair signed-rank test for comparisons between CF cells. p-values are as follows: *p < 0.05; **p < 0.01; other comparisons were not significant.
The combination of VX-445/661/770 with TNF-α and IL-17 did not modify the amiloride HCO3− secretion rate or the cAMP-activated HCO3− flux, as compared to TNF-α/IL-17 alone. In contrast, HCO3− flux inhibition was increased, mainly the Inh-172 response (p < 0.05) (respective part in the inhibition of Inh-172, YS-01, and GlyH-101: 45% ± 2%; 29% ± 6%; and 26% ± 1.5%).
3.3 Inflammation, but not VX-445/661/770 alone, induces ASL pH alkalinization
VX-445/661/770 did not significantly change ASL pH in comparison to DMSO control conditions (from 7.12 (0.11) to 7.14 (0.11)) (Figure 4A). In contrast, TNF-α and IL-17 significantly increased ASL pH to 7.37 (0.04) (p = 0.009), as shown in Figure 4A. The combination of VX-445/661/770 with TNF-α/IL-17 induced a further small but significant change in ASL pH to 7.39 (0.01) (p = 0.03), similar to values observed in WT cells (Figure 4A).
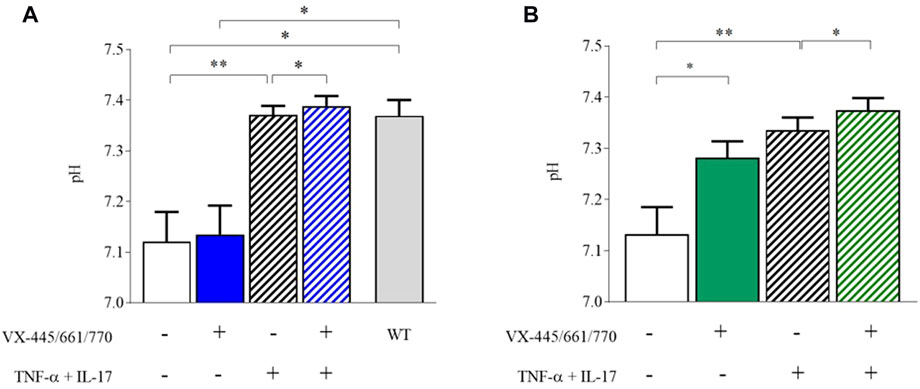
FIGURE 4. ASL pH of F508del human respiratory epithelial cells is abnormally acidic and is increased by the VX-445/661/770 and TNF-α+IL-17 combination. ASL pH of human respiratory epithelial cells was measured under physiological conditions (37°C, 5% CO2) after an 8-h apical incubation of 50 µL physiological Ringer’s solution (A) or Cl−-free Ringer’s solution (B). The measurements were performed in the presence of Fsk//IBMX (10µM/100 µM) added basolaterally before the 8-h incubation. ASL pH was assessed under physiological conditions in human respiratory epithelial cells from F508del homozygous patients under control DMSO conditions (n = 4) versus VX-445/661/770 (n = 4), IL-17+TNF-α (n = 6), IL-17+TNF-α + VX-445/661/770 (n = 6), and WT (n = 5). (B) ASL pH was assessed in Cl−-free conditions in human respiratory epithelial cells from F508del homozygous patients under control DMSO conditions (n = 9) versus VX-445/661/770 (n = 15), IL-17+TNF-α (n = 9), and IL-17+TNF-α + VX-445/661/770 (n = 8). Data are presented as mean ± SD. A comparison was carried out by the Mann–Whitney signed-rank test for WT versus CF cells and the Wilcoxon matched-pair signed-rank test. p-values are as follows: *p < 0.05; **p < 0.01.
To investigate the respective roles of CFTR and SLC26A4 in pH homeostasis, we performed ASL pH measurements in Cl−-free solutions where SLC26A4 activity was abrogated. Under these conditions, the ASL pH of DMSO-treated F508del airway cells was not significantly modified (7.12 vs. 7.14 in Cl− conditions), while it significantly increased from 7.14 (0.11) to 7.28 (0.12) when the cells were incubated with VX-445/661/770, thus unmasking a CFTR-dependent pH correction (Figure 4B). Under pro-inflammatory conditions, ASL pH was significantly increased up to 7.34 (0.08) (p = 0.0023 versus DMSO but NS versus VX-445/661/770), with an additional effect of VX-445/661/770 reaching values similar to those obtained under physiological conditions (7.38 (0.07)).
3.4 Inflammation increases CFTR and SLC26A4 expression
VX-445/661/770 did not modify the transcript levels of CFTR, SLC26A4, SLC26A9, or NBCe1 (Figure 5). TNF-α/IL-17 incubation increased SLC26A4 transcript levels by more than 40-fold (p < 0.001), but in contrast, CFTR transcript levels only increased by 1.5-fold (p < 0.01), while it did not significantly change NBCe1 transcript levels. For SLC26A9, there was a trend for an increase in the transcript levels upon TNF-α/IL-17; however, this did not reach a significant level (Figure 5).
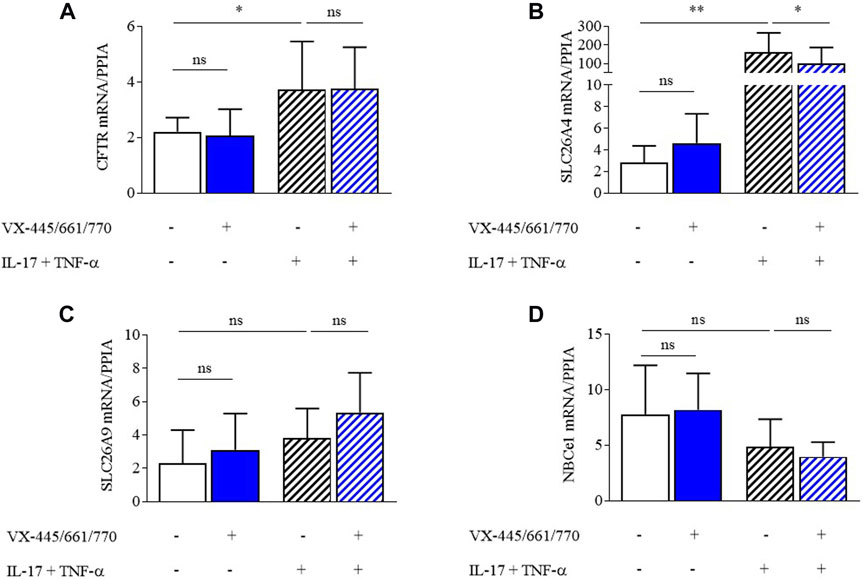
FIGURE 5. Main base transporter expression in primary F508del/F508del human respiratory epithelial cells by RT-qPCR. Transcript expression levels of CFTR (A), SLC26A4 (Pendrin) (B), SLC26A9 (C), and NBCe1 (D) were analyzed using real-time RT–PCR in ALI-differentiated primary human bronchial epithelial cells from F508del homozygous patients. Transcript levels were analyzed in the control DMSO condition (n = 8, full white bars), VX-445/661/770 (n = 4, full blue bars), IL-17+TNF-α (n = 8, stripped white bars), and IL-17+TNF-α + VX-445/661/770 (n = 8, stripped blue bars) and normalized with cyclophilin transcript (PPIA) as an internal control. When indicated, cells were treated with IL-17 (20 ng/mL) and TNF-α (10 ng/mL) and/or VX-770 (100 nM), VX-445 (3 µM), and VX-661 (3 µM) for 48 h. Data are presented as mean ± SD, and statistical analysis is performed with the Wilcoxon matched-pair signed-rank test (*p < 0.05, **p < 0.01; ns: non-significant).
4 Discussion
There are still few pharmacological data on the rescue of HCO3− secretion by CFTR correction in the CF airway epithelium. Our results show that F508del-CFTR correction by VX-445/661/770 rescues HCO3− transport up to 80% of the normal level in primary cultures of the airway epithelium. Inflammation, e.g., the TNFα/IL-17 cocktail, massively increases the HCO3− transport, involving both CFTR and SLC26A4. CFTR correction in the context of inflammation further increased HCO3− secretion by CFTR. Strikingly, the increase in CFTR-mediated HCO3− secretion had a marginal impact on ASL pH in contrast to TNF-α/IL-17-induced inflammation.
4.1 F508del-CFTR correction restores HCO3− transport but not ASL pH
Similar observations were recently reported in intestinal F508del organoids where VX-445/661/770 induced an increase in CFTR-dependent HCO3− transport (Bijvelds et al., 2022; Ciciriello et al., 2022) and in D1152H primary respiratory cells where ivacaftor improved selective HCO3− transport defects (Laselva et al., 2020). Interestingly, in these reports, as shown in our study, carrying F508del on both alleles did not increase the level of HCO3− transport correction in comparison to carrying F508del in combination with another mutation. All these results were obtained from short-circuit currents in the Cl−-free medium, which enables indirectly assessing HCO3− transport by assessing the transepithelial potential difference. Here, by using the pH-stat titration system, an indirect method for assessing HCO3− secretion, we demonstrate that VX-445/661/770 treatment increases net transepithelial HCO3− fluxes. The increase in secretion in response to Fsk + genistein and its complete inhibition by Inh-172 indicate that this effect is due to CFTR.
Nevertheless, despite this massive increase in CFTR-dependent HCO3− transepithelial transport, the effect of F508del-CFTR rescue on the ASL pH of cultured airway epithelia was marginal. This confirms the observations by Rehman et al. (2021) and Morrison et al. (2022) and provides evidence that the correction of the CFTR channel defect alone is not sufficient to buffer the acidic ASL of CF airways. In contrast, Ludovico et al. showed that VX445/661/770 alkalinized the pH to WT levels (Ludovico et al., 2022). The reason for this discrepancy is unclear but may be related to differences in the media culture and differential expression of HCO3− transporters (Saint-Criq et al., 2020; Livnat et al., 2023).
4.2 Functional interplay between CFTR and SLC26A4
Airway inflammation is a hallmark of CF disease observed from the first days of life, mainly driven by neutrophilic inflammation and various cytokines such as TNF-α and IL-17 (Lukacs et al., 1995; Tan et al., 2011; Sly et al., 2013). The TNFα/IL-17 inflammatory cytokine combination not only modulates innate anti-infective defense (McAleer and Kolls, 2011) but also our data point to a massive increase in HCO3− secretion both at the basal state and after CFTR activation by cAMP (Fsk/IBMX). In addition, pH-stat experiments enabled us to dissect the participation of other transporters in ASL pH homeostasis. They clearly highlighted the SLC26A4 electroneutral Cl−/HCO3− exchange, as the complete inhibition of HCO3− flux required the subsequent addition of the YS-01 SLC26A4 inhibitor (Lee et al., 2020) and GlyH-101. This pro-inflammatory condition was associated with the pH normalization of F508del ASL. Our results are consistent with Rehman et al.’s observations (Rehman et al., 2020) indicating that SLC26A4 is a key player in CF ASL alkalinization and the recent publication of Guidone et al. showing that YS-01 significantly decreased ASL pH in both CF and non-CF epithelia treated with IL-17/TNF-α (Guidone et al., 2022). However, in contrast to our studies, Guidone et al. observed significant ASL pH alkalinization only after the stimulation of IL-17/TNF-α-treated cells by isoproterenol (Guidone et al., 2022). This discrepancy might be related to cell culture media that impact gene expression and the activity of ion channels (Saint-Criq et al., 2020). As TNF-α/IL-17 increases SLC26A4 and CFTR transcript expression, one could hypothesize that the modification of pH, driven by inflammation, could be related to SLC26A4 activity, both directly by increasing HCO3− secretion by SLC26A4 and CFTR and indirectly via its functional coupling to corrected F508del-CFTR, which would fuel SLC26A4 with Cl− (Garnett et al., 2011; Kim et al., 2019). SLC26A4 appears, therefore, as a key player in pH homeostasis under inflammatory physiological conditions. However, the observation that the correction of CFTR by modulators further increases HCO3− secretion by CFTR under inflammatory conditions and that pH is significantly increased in the Cl−-free medium where SLC26A4 is defective unravels the role of CFTR in ASL pH homeostasis.
The fact that GlyH-101 was necessary to completely inhibit the HCO3− transport in the context of inflammation suggests that additional HCO3− transporters other than CFTR and SLC26A4 may be involved in this inflammation-induced alkalinization (Zajac et al., 2021; Liu et al., 2022). Indeed, while Inh-172 is reported as being very specific to CFTR gating (Caci et al., 2008), GlyH-101 not only occludes the CFTR pore but is also active on anoctamin, bestrophin (Bai et al., 2021), voltage-gated channels (Lin et al., 2023), and SLC26A9 (Bertrand et al., 2009; Liu et al., 2022). It is still debated whether SLC26A9 contributes to HCO3− secretion directly, as suggested by a recent study on perfused mouse bronchioles and nasal cells (Jo et al., 2022; Liu et al., 2022), or via reciprocal regulation with CFTR, as suggested by the observation that the correction of F508del-CFTR also restored SLC26A9 constitutive activity in airway cells (Bertrand et al., 2009; Bertrand et al., 2017; Larsen et al., 2021). Our experiments show that SLC26A9 transcripts are present in the airways and display a trend to increase upon inflammatory triggers, but not at a significant level. This increase may be responsible for the stabilization of corrected F508del-CFTR at the plasma membrane, as shown by Pinto et al. (2021), and thus contribute indirectly to the higher HCO3− fluxes mediated by CFTR observed in pH-stat experiments (Balázs and Mall, 2018).
Paracellular HCO3− flux might also control ASL pH. Very well-designed studies by Thornell et al. (2020) have shown that in human airway epithelia, there is a paracellular pathway permeable to HCO3− ions that may temper the ASL pH changes. Depending on the values of ASL pH, the paracellular flux is absorptive (ASL pH 7.4) or secretory (ASL pH 6.6). Interestingly, cytokine treatment (IL-17/TNF-α) resulted in an increase in paracellular conductance and HCO3− permeability, suggesting that the modulation of paracellular HCO3− transport might represent new therapeutic targets (Parker, 2020). In our pH-stat experiments, we did not observe a paracellular HCO3− flux, as we were able to completely block HCO3− transport by using ion-transporting protein inhibitors. This is consistent with Thornell’s observations (Thornell et al., 2020) showing negligible paracellular HCO3− flux at pH 7.0 (at which we clamp our apical solution).
4.3 Physiopathological relevance of our findings
Altogether, our results suggest that, under physiological conditions, SLC26A4 is the main driver of pH regulation in the bronchial epithelium, with CFTR intervening indirectly by fueling the exchanger with Cl−. Nevertheless, CFTR HCO3− secretion may be required in specific acidic situations, such as gastric acid inhalation, to efficiently and rapidly buffer ASL pH. This has been shown in murine duodenum, where low luminal pH activates the transcellular HCO3− transport that requires CFTR (Hogan et al., 1997). Moreover, the increased levels of HCO3− into the airway microenvironment, due to a proximal paracrine effect, would also favor rapid mucin expansion, resulting in improved mucociliary clearance and decreased airway obstruction and inflammation (Quinton, 2008). Downstream effects could also restore intrinsic immune defenses by augmenting the formation of neutrophil extracellular traps by neutrophils and sensitizing Pseudomonas aeruginosa to the antimicrobial peptide cathelicidin LL-37 (Siew et al., 2022).
These results suggest that the full efficiency of CFTR modulation might require a residual “background” of CFTR inflammation. This is consistent with in vivo studies that showed that nasal mucosa pH was abnormally acidic in CF neonates but normal from 3 months of age after airway inflammation initiation (Abou Alaiwa et al., 2014; Schultz et al., 2017), and a positive correlation between the level of airway inflammation and the respiratory improvement in G551D patients treated with ivacaftor was reported by Rehman et al. (2021). Importantly, several studies report that CFTR modulators abrogate airway inflammation, which may then jeopardize the effect on pH (Lepissier et al., 2023). The physiopathological relevance of these findings, including their long-term remanence, is still unclear (Hisert et al., 2017).
4.4 Study limitation: current knowledge and technical gaps
This study has limitations. First, we measured ASL pH with a pH electrode and assumed that the 50-µL Ringer solution added to the surface of airway cultures for the experiment would represent diluted ASL. However, this ringer contains 25 mM HCO3− and could buffer small pH variations and, thus, underestimate pH changes.
Second, inflammation is a complex status involving different triggers that may behave differently (Roesch et al., 2018; Gentzsch et al., 2021; Rehman and Welsh, 2023). Moreover, the concentration of cytokines used in this experiment might be far above that occurring in vivo. Indeed, we performed a pilot study to assess inflammatory cytokines in sputum and observed that TNF-α levels were 100-fold lower in patients’ sputum than the concentration applied in vitro (Lepissier et al., 2023). Our experimental design is only able to mimic acute inflammation. It could be noted that CFTR rescue may differentially modulate CF epithelia subjected to chronic inflammation. Additionally, we used the novel YS-01 inhibitor as it was shown to potently inhibit pendrin activity with no effects on other ion-transporting proteins involved in ASL regulation such as CFTR or ANO-1; however, its possible off-target effects are still to be discovered (Park et al., 2019; Lee et al., 2020).
Third, we measured the HCO3− transport under conditions aiming to maximize HCO3− fluxes. To do this, we used the ENaC channel inhibitor (amiloride) to hyperpolarize cell membranes and, thus, increase the driving force for HCO3− exit. Solution at the apical side of cell monolayers was unbuffered and therefore generated a large transepithelial (basal to apical) HCO3− gradient with a negligible paracellular HCO3− flux (Thornell et al., 2020). The apical solution pH was clamped to 7.00 to reflect the pH value found in CF patients (Zajac et al., 2021). Finally, we applied a CO2/O2 gradient to the epithelium and used a solution with no HCO3− to be able to monitor the HCO3− fluxes. We agree that the use of an apical pure O2 and HCO3− gradient is not physiological and might impact airway cell physiology. However, we do not think that the O2 level would affect intracellular pH. Indeed, we did not observe any short-term (3–4 h) modification of transepithelial resistance, and Liu et al., under conditions relatively similar to ours, did not report long-term modification of intracellular pH in mouse bronchioles (Liu et al., 2022). Moreover, different groups showed that such an HCO3− gradient did not cause an overestimation of HCO3− secretion (Krouse et al., 2004; Shan et al., 2012).
Finally, our pH-stat experiments show net HCO3− secretion as concurrent H+ transport neutralizes part of the transported HCO3−. Thus, if present, H+ transport may lead to an underestimate of our HCO3− fluxes. This was clearly highlighted in experiments performed in the Calu-3 cell line, showing that HCO3− secretion may be 30% higher than the measured net flux (Krouse et al., 2004; Shan et al., 2012). Our experiments measure global luminal pH and HCO3− transport but may underestimate local pH modification in micro areas around submucosal glands, which express CFTR at a high level and display an acidic pH environment related to CFTR defects (Garnett et al., 2016). Local correction of HCO3− secretion may be very relevant to acting on inflammation-mediated mucus viscosity. ASL acid–base regulation is a very complex phenomenon involving the coordinated activity of all ion-transporting proteins, including H+-transporting proteins present on the apical and basolateral sides of the epithelium (Zajac et al., 2021). The measurement of ASL pH cannot discriminate between the increase in base secretion and the decrease in acid secretion (Shah et al., 2016). The regulation of the ATP12A proton pump by inflammation, as recently reported by Guidone et al., showed that the impact on H+ secretion also needs to be considered (Guidone et al., 2022).
4.5 Bicarbonate transport correction: a path to theratyping
Despite the revolution in CF therapy caused by HEMT, real-world studies have shown high variability in patient responses to modulator therapy (Pranke et al., 2017; Birimberg-Schwartz et al., 2023; Dreano et al., 2023). Theratyping gives the possibility to examine the responses of CFTR modulator drugs in vitro, providing the opportunity to individualize medicine for pwCF (Mall et al., 2020). Our laboratory has implemented theratyping based on HNE cells generated from the patient’s nasal brushes (Bear and Ratjen, 2023; Dreano et al., 2023). Our initial pH-stat studies have shown that the HNE cell monolayers recapitulate the HCO3− transport properties of HBE cultures, and thus, this model is useful in showing patient-specific properties and responses to drug therapy. This could be especially interesting since some CFTR variants were shown to be mainly HCO3− (and not Cl−) defective (LaRusch et al., 2014). It may be that patients carrying genotypes associated with residual Cl− secretion improve because of the rescue of HCO3− secretion. This aspect has been underestimated until now, and bicarbonate rescue now needs to be put on the spot. Moreover, since inflammation is a hallmark of CF, it is mandatory to test CFTR ion transport and, more specifically, HCO3− transport during inflammatory conditions (Gentzsch et al., 2021).
5 Conclusion
Our data show that CFTR correction restores HCO3− secretion, but at a level that is not sufficient to overcome ASL pH acidosis. Inflammation is a key regulator of HCO3− secretion in CF airways and enhances the efficacy of CFTR modulators. The prediction of the response by theratyping should take into account the airway inflammatory phenotype and its effect on ASL pH.
Data availability statement
The raw data supporting the conclusions of this article will be made available by the authors without undue reservation.
Ethics statement
The studies involving humans were approved by the Ile de France 2 Ethics Committee (AFSSAPS (ANSM) B1005423-40, EudraCT no. 2010-A00392-37; CPP IDF2: 2010-05-03-3). The studies were conducted in accordance with local legislation and institutional requirements. The participants provided their written informed consent to participate in this study.
Author contributions
MZ: data curation, formal analysis, investigation, methodology, validation, writing–original draft, and writing–review and editing. AL: investigation and writing–original draft. ED: investigation and writing–review and editing. BC: investigation and writing–review and editing. AH: investigation and writing–review and editing. MK-A: investigation and writing–review and editing. DG: writing–review and editing. GP: funding acquisition, validation, and writing–review and editing. AE: validation and writing–review and editing. EG: writing–review and editing. AH: investigation and writing–review and editing. IP: conceptualization, investigation, supervision, validation, and writing–review and editing. LG: funding acquisition, methodology, validation, and writing–review and editing. IS-G: conceptualization, data curation, formal analysis, funding acquisition, investigation, methodology, resources, validation, visualization, writing–original draft, and writing–review, and editing. GC: writing–review and editing.
Funding
The authors declare that financial support was received for the research, authorship, and/or publication of this article. This research was funded by the Vertex Innovation Award-2017 cycle, The Association ABCF, and the Vaincre La Mucoviscidose - RC20200502648-2020 Award (Correction du transport de chlorure et de bicarbonate au sein de l'épithélium respiratoire par les modulateurs de CFTR). Application aux mutants rares et perspectives thérapeutiques). MZ was financed by the Polish National Agency for Academic Exchange within the Bekker Program no. BPN/BEK/2021/1/00284 and by the National Science Center (NCN), Poland, no. 2019/35/B/NZ1/02546.
Conflict of interest
IS-G reports support for the present manuscript from Vaincre la Mucoviscidose and Mucoviscidose ABCF2. IS-G also reports, outside the submitted work, grants from Agence Nationale pour la Recherche, Assistance Publique– Hôpitaux de Paris and Vertex Innovation Award, and consulting fees and travel support from Vertex therapeutics.
The remaining authors declare that the research was conducted in the absence of any commercial or financial relationships that could be construed as a potential conflict of interest.
Publisher’s note
All claims expressed in this article are solely those of the authors and do not necessarily represent those of their affiliated organizations, or those of the publisher, the editors, and the reviewers. Any product that may be evaluated in this article, or claim that may be made by its manufacturer, is not guaranteed or endorsed by the publisher.
Supplementary material
The Supplementary Material for this article can be found online at: https://www.frontiersin.org/articles/10.3389/fphar.2023.1293578/full#supplementary-material
References
Abou Alaiwa, M. H., Beer, A. M., Pezzulo, A. A., Launspach, J. L., Horan, R. A., Stoltz, D. A., et al. (2014). Neonates with cystic fibrosis have a reduced nasal liquid pH; A small pilot study. J. Cyst. Fibros. 13, 373–377. doi:10.1016/j.jcf.2013.12.006
Bai, W., Liu, M., and Xiao, Q. (2021). The diverse roles of TMEM16A Ca2+-activated Cl− channels in inflammation. J. Adv. Res. 33, 53–68. doi:10.1016/j.jare.2021.01.013
Balázs, A., and Mall, M. A. (2018). Role of the SLC26A9 chloride channel as disease modifier and potential therapeutic target in cystic fibrosis. Front. Pharmacol. 9, 1112. doi:10.3389/fphar.2018.01112
Bear, C., and Ratjen, F. (2023). Charting the path to expanded access for CFTR modulator drugs: the nose knows. Eur. Respir. J. 62, 2301387. doi:10.1183/13993003.01387-2023
Bertrand, C. A., Mitra, S., Mishra, S. K., Wang, X., Zhao, Y., Pilewski, J. M., et al. (2017). The CFTR trafficking mutation F508del inhibits the constitutive activity of SLC26A9. Am. J. Physiol. Cell. Mol. Physiol. 312, L912-L925–L925. doi:10.1152/ajplung.00178.2016
Bertrand, C. A., Zhang, R., Pilewski, J. M., and Frizzell, R. A. (2009). SLC26A9 is a constitutively active, CFTR-regulated anion conductance in human bronchial epithelia. J. Gen. Physiol. 133, 421–438. doi:10.1085/jgp.200810097
Bijvelds, M. J. C., Roos, F. J. M., Meijsen, K. F., Roest, H. P., Verstegen, M. M. A., Janssens, H. M., et al. (2022). Rescue of chloride and bicarbonate transport by elexacaftor-ivacaftor-tezacaftor in organoid-derived CF intestinal and cholangiocyte monolayers. J. Cyst. Fibros. 21, 537–543. doi:10.1016/j.jcf.2021.12.006
Birimberg-Schwartz, L., Ip, W., Bartlett, C., Avolio, J., Vonk, A. M., Gunawardena, T., et al. (2023). Validating organoid-derived human intestinal monolayers for personalized therapy in cystic fibrosis. Life Sci. Alliance 6, e202201857. doi:10.26508/lsa.202201857
Bridges, R. J. (2012). Mechanisms of bicarbonate secretion: lessons from the airways. Cold Spring Harb. Perspect. Med. 2, 015016–a15110. doi:10.1101/cshperspect.a015016
Caci, E., Caputo, A., Hinzpeter, A., Arous, N., Fanen, P., Sonawane, N., et al. (2008). Evidence for direct CFTR inhibition by CFTRinh-172 based on Arg347 mutagenesis. Biochem. J. 413, 135–142. doi:10.1042/BJ20080029
Ciciriello, F., Bijvelds, M. J. C., Alghisi, F., Meijsen, K. F., Cristiani, L., Sorio, C., et al. (2022). Theratyping of the rare CFTR variants E193K and R334W in rectal organoid-derived epithelial monolayers. J. Pers. Med. 12, 632. doi:10.3390/jpm12040632
Coakley, R. D., Grubb, B. R., Paradiso, A. M., Gatzy, J. T., Johnson, L. G., Kreda, S. M., et al. (2003). Abnormal surface liquid pH regulation by cultured cystic fibrosis bronchial epithelium. Proc. Natl. Acad. Sci. 100, 16083–16088. doi:10.1073/pnas.2634339100
Dobay, O., Laub, K., Stercz, B., Kéri, A., Balázs, B., Tóthpál, A., et al. (2018). Bicarbonate inhibits bacterial growth and biofilm formation of prevalent cystic fibrosis pathogens. Front. Microbiol. 9, 2245–2312. doi:10.3389/fmicb.2018.02245
Dreano, E., Burgel, P. R., Hatton, A., Bouazza, N., Chevalier, B., Macey, J., et al. (2023). Theratyping cystic fibrosis patients to guide elexacaftor/tezacaftor/ivacaftor out-of-label prescription. Eur. Respir. J. 62, 2300110. doi:10.1183/13993003.00110-2023
Ferrera, L., Capurro, V., Delpiano, L., Gianotti, A., and Moran, O. (2021). The application of bicarbonate recovers the chemical-physical properties of airway surface liquid in cystic fibrosis epithelia models. Biol. (Basel) 10, 278. doi:10.3390/biology10040278
Garland, A. L., Walton, W. G., Coakley, R. D., Tan, C. D., Gilmore, R. C., Hobbs, C. A., et al. (2013). Molecular basis for pH-dependent mucosal dehydration in cystic fibrosis airways. Proc. Natl. Acad. Sci. U. S. A. 110, 15973–15978. doi:10.1073/pnas.1311999110
Garnett, J. P., Hickman, E., Burrows, R., Hegyi, P., Tiszlavicz, L., Cuthbert, A. W., et al. (2011). Novel role for pendrin in orchestrating bicarbonate secretion in cystic fibrosis transmembrane conductance regulator (CFTR)-expressing airway serous cells. J. Biol. Chem. 286, 41069–41082. doi:10.1074/jbc.M111.266734
Garnett, J. P., Kalsi, K. K., Sobotta, M., Bearham, J., Carr, G., Powell, J., et al. (2016). Hyperglycaemia and Pseudomonas aeruginosa acidify cystic fibrosis airway surface liquid by elevating epithelial monocarboxylate transporter 2 dependent lactate-H+ secretion. Sci. Rep. 6, 37955. doi:10.1038/srep37955
Gentzsch, M., Cholon, D. M., Quinney, N. L., Martino, M. E. B., Minges, J. T., Boyles, S. E., et al. (2021). Airway epithelial inflammation in vitro augments the rescue of mutant CFTR by current CFTR modulator therapies. Front. Pharmacol. 12, 628722. doi:10.3389/fphar.2021.628722
Golec, A., Pranke, I., Scudieri, P., Hayes, K., Dreano, E., Dunlevy, F., et al. (2022). Isolation, cultivation, and application of primary respiratory epithelial cells obtained by nasal brushing, polyp samples, or lung explants. Star. Protoc. 3, 101419. doi:10.1016/j.xpro.2022.101419
Gomez, C. C. S., Parazzi, P. L. F., Clinckspoor, K. J., Mauch, R. M., Pessine, F. B. T., Levy, C. E., et al. (2020). Safety, tolerability, and effects of sodium bicarbonate inhalation in cystic fibrosis. Clin. Drug Investig. 40, 105–117. doi:10.1007/s40261-019-00861-x
Gorrieri, G., Scudieri, P., Caci, E., Schiavon, M., Tomati, V., Sirci, F., et al. (2016). Goblet cell hyperplasia requires high bicarbonate transport to support mucin release. Sci. Rep. 6, 36016–36115. doi:10.1038/srep36016
Guidone, D., Buccirossi, M., Scudieri, P., Genovese, M., Sarnataro, S., De Cegli, R., et al. (2022). Airway surface hyperviscosity and defective mucociliary transport by IL-17/TNF-α are corrected by β-adrenergic stimulus. JCI Insight 7, e164944. doi:10.1172/jci.insight.164944
Hisert, K. B., Heltshe, S. L., Pope, C., Jorth, P., Wu, X., Edwards, R. M., et al. (2017). Restoring cystic fibrosis transmembrane conductance regulator function reduces airway bacteria and inflammation in people with cystic fibrosis and chronic lung infections. Am. J. Respir. Crit. Care Med. 195, 1617–1628. doi:10.1164/rccm.201609-1954OC
Hoegger, M. J., Fischer, A. J., McMenimen, J. D., Ostedgaard, L. S., Tucker, A. J., Awadalla, M. A., et al. (2014). Impaired mucus detachment disrupts mucociliary transport in a piglet model of cystic fibrosis. Science 345, 818–822. doi:10.1126/science.1255825
Hogan, D., Crombie, D., Isenberg, J., Svendsen, P., Schaffalitzky de Muckadell, O., and Ainsworth, M. (1997). Acid-stimulated duodenal bicarbonate secretion involves a CFTR-mediated transport pathway in mice. Gastroenterology 113, 533–541. doi:10.1053/gast.1997.v113.pm9247473
Jo, S., Centeio, R., Park, J., Ousingsawat, J., Jeon, D., Talbi, K., et al. (2022). The SLC26A9 inhibitor S9-A13 provides no evidence for a role of SLC26A9 in airway chloride secretion but suggests a contribution to regulation of ASL pH and gastric proton secretion. FASEB J. 36, e22534. doi:10.1096/fj.202200313RR
Kim, D., Huang, J., Billet, A., Abu-Arish, A., Goepp, J., Matthes, E., et al. (2019). Pendrin mediates bicarbonate secretion and enhances cystic fibrosis transmembrane conductance regulator function in airway surface epithelia. Am. J. Respir. Cell Mol. Biol. 60, 705–716. doi:10.1165/rcmb.2018-0158OC
Krouse, M. E., Talbott, J. F., Lee, M. M., Nam, S. J., and Wine, J. J. (2004). Acid and base secretion in the Calu-3 model of human serous cells. Am. J. Physiol. - Lung Cell. Mol. Physiol. 287, 1274–1283. doi:10.1152/ajplung.00036.2004
Larsen, M. B., Choi, J. J., Wang, X., Myerburg, M. M., Frizzell, R. A., and Bertrand, C. A. (2021). Separating the contributions of SLC26A9 and CFTR to anion secretion in primary human bronchial epithelia. Am. J. Physiol. Cell. Mol. Physiol. 321, L1147–L1160. doi:10.1152/ajplung.00563.2020
LaRusch, J., Jung, J., General, I. J., Lewis, M. D., Park, H. W., Brand, R. E., et al. (2014). Mechanisms of CFTR functional variants that impair regulated bicarbonate permeation and increase risk for pancreatitis but not for cystic fibrosis. PLoS Genet. 10, e1004376. doi:10.1371/journal.pgen.1004376
Laselva, O., Moraes, T. J., He, G., Bartlett, C., Szàrics, I., Ouyang, H., et al. (2020). The CFTR mutation c.3453G > C (D1152H) confers an anion selectivity defect in primary airway tissue that can be rescued by ivacaftor. J. Pers. Med. 10, 40. doi:10.3390/jpm10020040
Lee, E. H., Shin, M. H., Gi, M., Park, J., Song, D., Hyun, Y.-M., et al. (2020). Inhibition of pendrin by a small molecule reduces lipopolysaccharide-induced acute lung injury. Theranostics 10, 9913–9922. doi:10.7150/thno.46417
Lepissier, A., Bonnel, A. S., Wizla, N., Weiss, L., Mittaine, M., Bessaci, K., et al. (2023). Moving the dial on airway inflammation in response to trikafta in adolescents with cystic fibrosis. Am. J. Respir. Crit. Care Med. 207, 792–795. doi:10.1164/rccm.202210-1938LE
Lin, J., Gettings, S. M., Talbi, K., Schreiber, R., Taggart, M. J., Preller, M., et al. (2023). Pharmacological inhibitors of the cystic fibrosis transmembrane conductance regulator exert off-target effects on epithelial cation channels. Pflügers Arch. - Eur. J. Physiol. 475, 167–179. doi:10.1007/s00424-022-02758-9
Liu, L., Yamamoto, A., Yamaguchi, M., Taniguchi, I., Nomura, N., Nakakuki, M., et al. (2022). Bicarbonate transport of airway surface epithelia in luminally perfused mice bronchioles. J. Physiol. Sci. 72, 4. doi:10.1186/s12576-022-00828-2
Livnat, G., Meeker, J. D., Ostmann, A. J., Strecker, L. M., Clancy, J. P., and Brewington, J. J. (2023). Phenotypic alteration of an established human airway cell line by media selection. Int. J. Mol. Sci. 24, 1246. doi:10.3390/ijms24021246
Ludovico, A., Moran, O., and Baroni, D. (2022). Modulator combination improves in vitro the microrheological properties of the airway surface liquid of cystic fibrosis airway epithelia. Int. J. Mol. Sci. 23, 11396. doi:10.3390/ijms231911396
Lukacs, N. W., Strieter, R. M., Chensue, S. W., Widmer, M., and Kunkel, S. L. (1995). TNF-alpha mediates recruitment of neutrophils and eosinophils during airway inflammation. J. Immunol. 154, 5411–5417. doi:10.4049/jimmunol.154.10.5411
Mall, M. A., Mayer-Hamblett, N., and Rowe, S. M. (2020). Cystic fibrosis: emergence of highly effective targeted therapeutics and potential clinical implications. Am. J. Respir. Crit. Care Med. 201, 1193–1208. doi:10.1164/rccm.201910-1943SO
McAleer, J. P., and Kolls, J. K. (2011). Mechanisms controlling Th17 cytokine expression and host defense. J. Leukoc. Biol. 90, 263–270. doi:10.1189/jlb.0211099
Middleton, P. G., Mall, M. A., Dřevínek, P., Lands, L. C., McKone, E. F., Polineni, D., et al. (2019). Elexacaftor–Tezacaftor–Ivacaftor for Cystic Fibrosis with a Single Phe508del Allele. N. Engl. J. Med. 381, 1809–1819. doi:10.1056/NEJMoa1908639
Morrison, C. B., Shaffer, K. M., Araba, K. C., Markovetz, M. R., Wykoff, J. A., Quinney, N. L., et al. (2022). Treatment of cystic fibrosis airway cells with CFTR modulators reverses aberrant mucus properties via hydration. Eur. Respir. J. 59, 2100185. doi:10.1183/13993003.00185-2021
Park, J., Lee, H. J., Song, D., Gi, M., Jo, S., Jeon, D., et al. (2019). Novel pendrin inhibitor attenuates airway hyperresponsiveness and mucin expression in experimental murine asthma. J. Allergy Clin. Immunol. 144, 1425–1428. doi:10.1016/j.jaci.2019.07.016
Parker, M. D. (2020). Soda stream modifies airway fluid. J. Physiol. 598, 4143–4144. doi:10.1113/JP280467
Pezzulo, A. A., Tang, X. X., Hoegger, M. J., Abou Alaiwa, M. H., Ramachandran, S., Moninger, T. O., et al. (2012). Reduced airway surface pH impairs bacterial killing in the porcine cystic fibrosis lung. Nature 487, 109–113. doi:10.1038/nature11130
Pinto, M. C., Quaresma, M. C., Silva, I. A. L., Railean, V., Ramalho, S. S., and Amaral, M. D. (2021). Synergy in cystic fibrosis therapies: targeting SLC26A9. Int. J. Mol. Sci. 22, 13064. doi:10.3390/ijms222313064
Pranke, I., Golec, A., Hinzpeter, A., Edelman, A., and Sermet-Gaudelus, I. (2019). Emerging therapeutic approaches for cystic fibrosis. From gene editing to personalized medicine. Front. Pharmacol. 10, 121. doi:10.3389/fphar.2019.00121
Pranke, I. M., Hatton, A., Simonin, J., Jais, J. P., Le Pimpec-Barthes, F., Carsin, A., et al. (2017). Correction of CFTR function in nasal epithelial cells from cystic fibrosis patients predicts improvement of respiratory function by CFTR modulators. Sci. Rep. 7, 7375. doi:10.1038/s41598-017-07504-1
Quinton, P. M. (2008). Cystic fibrosis: impaired bicarbonate secretion and mucoviscidosis. Lancet 372, 415–417. doi:10.1016/S0140-6736(08)61162-9
Rehman, T., Karp, P. H., Tan, P., Goodell, B. J., Pezzulo, A. A., Thurman, A. L., et al. (2021). Inflammatory cytokines TNF-α and IL-17 enhance the efficacy of cystic fibrosis transmembrane conductance regulator modulators. J. Clin. Invest. 131, e150398. doi:10.1172/JCI150398
Rehman, T., Thornell, I. M., Pezzulo, A. A., Thurman, A. L., Romano Ibarra, G. S., Karp, P. H., et al. (2020). TNFα and IL-17 alkalinize airway surface liquid through CFTR and pendrin. Am. J. Physiol. 319, C331–C344. doi:10.1152/ajpcell.00112.2020
Rehman, T., and Welsh, M. J. (2023). Inflammation as a regulator of the airway surface liquid pH in cystic fibrosis. Cells 12, 1104. doi:10.3390/cells12081104
Roesch, E. A., Nichols, D. P., and Chmiel, J. F. (2018). Inflammation in cystic fibrosis: an update. Pediatr. Pulmonol. 53, S30-S50–S50. doi:10.1002/ppul.24129
Saint-Criq, V., Delpiano, L., Casement, J., Onuora, J. C., Lin, J. H., and Gray, M. A. (2020). Choice of differentiation media significantly impacts cell lineage and response to CFTR modulators in fully differentiated primary cultures of cystic fibrosis human airway epithelial cells. Cells 9, 2137. doi:10.3390/cells9092137
Schultz, A., Puvvadi, R., Borisov, S. M., Shaw, N. C., Klimant, I., Berry, L. J., et al. (2017). Airway surface liquid pH is not acidic in children with cystic fibrosis. Nat. Commun. 8, 1409. doi:10.1038/s41467-017-00532-5
Shah, V. S., Meyerholz, D. K., Tang, X. X., Reznikov, L., Abou Alaiwa, M., Ernst, S. E., et al. (2016). Airway acidification initiates host defense abnormalities in cystic fibrosis mice. Science 351, 503–507. doi:10.1126/science.aad5589
Shan, J., Liao, J., Huang, J., Robert, R., Palmer, M. L., Fahrenkrug, S. C., et al. (2012). Bicarbonate-dependent chloride transport drives fluid secretion by the human airway epithelial cell line Calu-3. J. Physiol. 590, 5273–5297. doi:10.1113/jphysiol.2012.236893
Siew, R., Ou, T.-L., Dahesh, S., Akong, K., and Nizet, V. (2022). Bicarbonate effects on antibacterial immunity and mucus glycobiology in the cystic fibrosis lung: a review with selected experimental observations. Infect. Microbes Dis. 4, 103–110. doi:10.1097/IM9.0000000000000101
Simonin, J., Bille, E., Crambert, G., Noel, S., Dreano, E., Edwards, A., et al. (2019). Airway surface liquid acidification initiates host defense abnormalities in Cystic Fibrosis. Sci. Rep. 9, 6516–6611. doi:10.1038/s41598-019-42751-4
Sly, P. D., Gangell, C. L., Chen, L., Ware, R. S., Ranganathan, S., Mott, L. S., et al. (2013). Risk factors for bronchiectasis in children with cystic fibrosis. N. Engl. J. Med. 368, 1963–1970. doi:10.1056/NEJMoa1301725
Tan, H.-L., Regamey, N., Brown, S., Bush, A., Lloyd, C. M., and Davies, J. C. (2011). The Th17 pathway in cystic fibrosis lung disease. Am. J. Respir. Crit. Care Med. 184, 252–258. doi:10.1164/rccm.201102-0236OC
Tang, X. X., Ostedgaard, L. S., Hoegger, M. J., Moninger, T. O., Karp, P. H., McMenimen, J. D., et al. (2016). Acidic pH increases airway surface liquid viscosity in cystic fibrosis. J. Clin. Invest. 126, 879–891. doi:10.1172/JCI83922
Thornell, I. M., Rehman, T., Pezzulo, A. A., and Welsh, M. J. (2020). Paracellular bicarbonate flux across human cystic fibrosis airway epithelia tempers changes in airway surface liquid pH. J. Physiol. 598, 4307–4320. doi:10.1113/JP280120
Keywords: ion transport across membrane, cystic fibrosis transmembrane conductance regulator, bicarbonate, inflammation, TRIKAFTA
Citation: Zajac M, Lepissier A, Dréano E, Chevalier B, Hatton A, Kelly-Aubert M, Guidone D, Planelles G, Edelman A, Girodon E, Hinzpeter A, Crambert G, Pranke I, Galietta LJV and Sermet-Gaudelus I (2023) Putting bicarbonate on the spot: pharmacological insights for CFTR correction in the airway epithelium. Front. Pharmacol. 14:1293578. doi: 10.3389/fphar.2023.1293578
Received: 13 September 2023; Accepted: 17 November 2023;
Published: 11 December 2023.
Edited by:
Guido Veit, McGill University, CanadaReviewed by:
Anita Balázs, Charité University Medicine Berlin, GermanyIan Michael Thornell, The University of Iowa, United States
Oscar Moran, National Research Council (CNR), Italy
Copyright © 2023 Zajac, Lepissier, Dréano, Chevalier, Hatton, Kelly-Aubert, Guidone, Planelles, Edelman, Girodon, Hinzpeter, Crambert, Pranke, Galietta and Sermet-Gaudelus. This is an open-access article distributed under the terms of the Creative Commons Attribution License (CC BY). The use, distribution or reproduction in other forums is permitted, provided the original author(s) and the copyright owner(s) are credited and that the original publication in this journal is cited, in accordance with accepted academic practice. No use, distribution or reproduction is permitted which does not comply with these terms.
*Correspondence: Isabelle Sermet-Gaudelus, isabelle.sermet@aphp.fr