- Department of Neurology and Neurosurgery, Alan Edwards Centre for Research on Pain, Montréal Neurological Institute, McGill University, Montréal, QC, Canada
Chronic pain is a significant global socioeconomic burden with limited long-term treatment options. The intractable nature of chronic pain stems from two primary factors: the multifaceted nature of pain itself and an insufficient understanding of the diverse physiological mechanisms that underlie its initiation and maintenance, in both the peripheral and central nervous systems. The development of novel non-opioidergic analgesic approaches is contingent on our ability to normalize the dysregulated nociceptive pathways involved in pathological pain processing. The anterior cingulate cortex (ACC) stands out due to its involvement in top-down modulation of pain perception, its abnormal activity in chronic pain conditions, and its contribution to cognitive functions frequently impaired in chronic pain states. Here, we review the roles of the monoamines dopamine (DA), norepinephrine (NE), serotonin (5-HT), and other neuromodulators in controlling the activity of the ACC and how chronic pain alters their signaling in ACC circuits to promote pathological hyperexcitability. Additionally, we discuss the potential of targeting these monoaminergic pathways as a therapeutic strategy for treating the cognitive and affective symptoms associated with chronic pain.
Introduction
Chronic pain remains a significant global socioeconomic burden, afflicting millions of individuals worldwide, and effective long-term treatment options remain elusive (Holmes, 2016; Dahlhamer et al., 2018; Nahin et al., 2023). The complexity of chronic pain arises from its multifaceted nature, encompassing sensory and emotional components that involve distinct regions of the central and peripheral nervous systems. While there have been notable advancements in identifying neuronal pathways associated with chronic pain, the precise pathophysiological mechanisms inducing their dysfunction are diverse and not well established. As a result, the development of effective non-opioidergic pharmacological treatments to alleviate chronic pain symptoms has been hindered.
Recent technological advances have provided unprecedented opportunities for investigating the cortical networks involved in nociception, as well as those dysregulated by chronic pain. Significant progress has been made in identifying key regions of the “pain matrix,” a network of supraspinal regions involved in nociceptive signaling that participate in promoting pathological pain processing (Kuner and Flor, 2017; Kuner and Tan, 2020; Mercer et al., 2021).
Within the pain matrix, the anterior cingulate cortex (ACC), a region of the medial prefrontal cortex (mPFC), is particularly relevant due to its role in top-down pain modulation and its ability to regulate executive functions commonly disrupted in chronic pain (Rainville et al., 1997; Kummer et al., 2020; Phelps et al., 2021). Several studies have shown that, following neuropathic injury, pyramidal neurons in the ACC become hyperexcitable, and functional imaging studies have identified the ACC as one of the most constitutively active brain regions in chronic pain patients (Cordeiro Matos et al., 2015a; Jensen et al., 2016; Ferraro et al., 2021; Lançon et al., 2021).
Emerging evidence suggest a link between alterations in the cortical function of neuromodulators such as the monoamines dopamine (DA), norepinephrine (NE), serotonin (5-HT), and chronic pain-related cortical hyperexcitability (Ong et a, 2019; Kuner and Tan, 2020; Yang et al., 2020). DA and NE appear particularly important due to their molecularly antagonistic, yet functionally synergistic role in modulating pyramidal activity in the ACC. These neuromodulators and others, such as acetylcholine (ACh) and the neuropeptide oxytocin, are crucial in adjusting the activity of ACC circuits by balancing excitatory and inhibitory inputs on pyramidal neurons and thus play a major role in tuning pain perception. Additionally, chronic pain is linked to a high prevalence of cognitive disabilities also observed in other disorders that impact the release of neuromodulators (Hart et al., 2003; Cools and Arnsten, 2022). Affect, attention, memory, executive planning, spatial awareness, sensory discrimination, and other cognitive functions heavily influenced by cortical monoamines are common issues in chronic pain patients (Jarcho et al., 2012; Bushnell et al., 2013; Markovic et al., 2021). Our current treatments for chronic pain can be viable in the short term but lose effectiveness and create tolerance and addiction in the long term (Varrassi et al., 2010; Speed et al., 2018). Furthermore, current pain treatments do not effectively alleviate the cognitive deficits associated with chronic pain (Chapman et al., 2002; Schiltenwolf et al., 2014).
Here we discuss the involvement of the three major monoamines (DA, NE, and 5-HT), as well as ACh and oxytocin, in promoting hyperexcitability in the ACC, leading to pathological pain processing and cognitive deficits in chronic pain conditions. We will review the complex interplay between neuromodulation of ACC circuits and pain processing, ultimately fostering better understanding of the cortical causes of chronic pain at the cellular and molecular level, to aid the development of novel and more effective interventions for chronic pain management.
Dysregulated ACC major contributor to pathological pain processing
The mPFC, and especially the ACC, is a critical hub for attention, emotional regulation, and pain modulation (Rainville et al., 1997; Stevens et al., 2011; Arnsten and Katya, 2012). Neuroimaging studies consistently report ACC activation following pain stimulation (Casey, 1999; Bastuji et al., 2016). Afferents from the medial thalamus and the somatosensory cortex relay noxious signals to the ACC, which then filters them and relays them back down to other cortical or subcortical regions involved in pain perception. These include, but are not limited to, the periaqueductal grey (PAG), the midbrain, the parabrachial nucleus (PBN), the dorsal horn of the spinal cord (DHSC), and other cortical areas such as the prelimbic, somatosensory, and infralimbic cortices (Kuner et al., 2020).
While the ACC predominantly mediates the affective dimension of nociception, it has also been shown to modulate its sensory component (Rainville et al., 1997; Chen et al., 2018; Singh et al., 2020). If the output of the ACC becomes dysregulated, the downstream signals processed by its target regions will be disrupted and will promote abnormal nociception. For example, experimental in vivo stimulation of pyramidal neurons in the ACC through opto- and chemogenetic actuators consistently leads to a decrease in mechanical withdrawal thresholds as well as the induction of conditioned place aversion (CPA), established readouts for the sensory and affective aspects of pain, respectively (Johansen et al., 2001; Kang et al., 2015). The ACC is pathologically hyperactive in the case of patients with nerve injuries or other chronic illnesses known to cause prolonged painful states (Jensen et al., 2016; Tan and Kuner, 2021). Decreasing this hyperactivity in animal models of chronic pain reduces both allodynia (abnormal sensitivity to innocuous stimuli) and hyperalgesia (increased sensitivity to noxious stimuli), thereby demonstrating that dysregulated ACC circuits contribute to abnormal pain processing (Kang et al., 2015; Sellmeiher et al., 2018; Juarez-Salinas et al., 2019).
This increase in excitability is caused by changes in the balance of excitatory and inhibitory inputs (E/I balance) as well as intrinsic changes in the excitability of pyramidal neurons. Following nerve injury, the E/I balance in the ACC is shifted due to enhanced excitatory glutamate release and decreased inhibitory gamma-aminobutyric acid (GABA) release. The increase in glutamate release induces a robust facilitation of long-term potentiation (LTP) that is correlated with increases in NMDA-R currents and an upregulation of the GluN2B subunit (Kuner and Flor, 2017; Zhuo, 2020; Chen et al., 2021). For GABA release, although neuropathic pain does not appear to decrease the excitability of GABAergic interneurons, there is evidence of disinhibition due to loss of GABAergic synapses (Blom et al., 2014). The intrinsic excitability of synaptically isolated pyramidal neurons in the ACC is also potentiated following nerve injury. Hyperpolarization-activated cyclic nucleotide-gated (HCN) channels, dendritic cation channels known to tune the excitability of neurons by controlling their input resistance, are dysfunctional in neuropathic states (Cordeiro-Matos et al., 2015b; Santello and Nevian, 2015; Santello et al., 2017; Lançon et al., 2021). Since HCN channel activity is controlled by the ratio of Gs to Gi-linked metabotropic signaling, up and down regulators of adenyl cyclase respectively, this strongly suggests that G protein-coupled receptor activation is altered in the ACC in neuropathic states.
Identifying the factors that promote abnormal GPCR signaling in chronic pain conditions could be key to the development of novel analgesics or treatments that impede the instigation or maintenance of the pathological phenotype following nerve injury.
Dopamine
The role of DA in modulating mPFC function is relatively well studied in cognitive and attentional disorders, and more recently, in pain (Figure 1) (Vijayraghavan et al., 2007; Huang et al., 2019a). A genetically defined subset of VGluT2/DAT+ projection neurons located in the medial ventral tegmental area (VTA) has been reported to innervate the ACC and this mesocortical pathway is hypothesized to underlie salience and tune attention (Horvitz, 2000; Arnsten and Pliszka, 2011; Poulin et al., 2018). This pathway is now being studied further in its response to pain states and whether it is dysfunctional in chronic pain conditions. A recent study indicates that acute pain induces an inhibition of VTA neurons indirectly via the PBN and the substantia nigra (SNr) (Yang et al., 2021). In line with this, the VTA is hypoactive in chronic pain conditions and HPLC analysis demonstrates that DA levels are reduced in the ACC 3 days following CFA injection to evoke inflammatory pain (Narita et al., 2003; Ko et al., 2018; Huang et al., 2019b; Darvish-Ghane et al., 2020). Human imaging studies have also indicated a reduced activity of the VTA following salient stimulation in multiple forms of chronic pain (Loggia et al., 2014; Martikainen et al., 2015). Given these findings, it becomes critical to understand the role of DA in modulating ACC circuits and the impact that decreased cortical DAergic signaling would have on cortical output in chronic pain states.
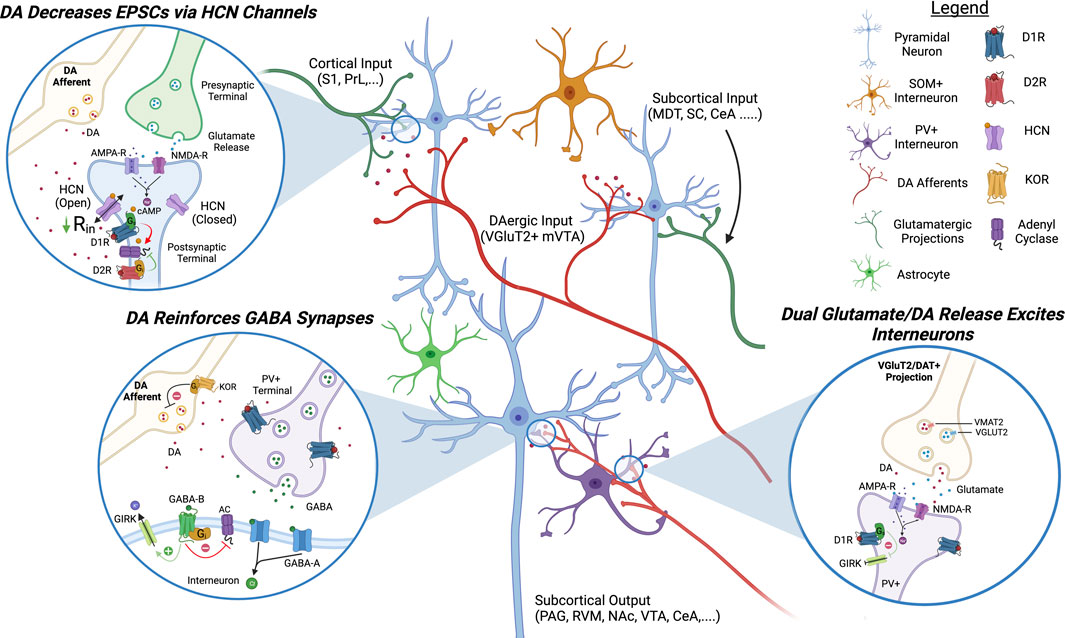
FIGURE 1. Proposed Model of DAergic Modulation of ACC Circuits. DAergic signaling in the ACC inhibits pyramidal neurons and activates inhibitory interneurons. D1R activation decreases EPSCs on pyramidal neurons by increasing the open channel probability of HCN channels and activates GABAergic interneurons via glutamatergic signaling and inhibition of K+ channels. DA, dopamine; HCN, hyperpolarization-activated cyclic nucleotide-gated channels; AC, adenyl cyclase; GIRK, G-protein coupled inward rectifying potassium channels; KOR, kappa opioid receptor; PAG, periaqueductal gray; S1, somatosensory cortex 1; PrL, prelimbic cortex; MDT, medial dorsal thalamus; SC, spinal cord; CeA, central nucleus of amygdala; RVM, rostral ventromedial medulla; NAc, nucleus accumbens; VTA, ventral tegmental area.
Gs-coupled DA receptor subtype 1 (D1R) is the most studied DA receptor in the ACC and is expressed on pyramidal neurons in layers 2/3 and L5/6 as well as GABAergic interneurons (Muly et al., 1998; Clarkson et al., 2017). In pyramidal neurons, D1Rs are co-localized with HCN channels on dendritic spines and electrophysiological studies have demonstrated that their activation increases the open channel probability of HCN channels, decreasing the input resistance (Paspalas et al., 2012; Lançon et al., 2021). Functionally, activation of D1R expressed on pyramidal ACC inhibits excitatory post synaptic currents (EPSCs), AMPA-R currents, and causes general inhibition by increasing the rheobase of pyramidal neurons (Darvish-Ghane et al., 2016; Lançon et al., 2021). D1Rs are also expressed on parvalbumin (PV+) fast-spiking GABAergic interneurons in the ACC (Le Moine and Gaspar, 1998; Glausier et al., 2009; Santana et al., 2009). Activation of D1Rs expressed on GABAergic interneurons inhibits leak K+ channels and inward-rectifying K+ channels to induce a robust excitation and increase inhibitory post synaptic currents (IPSCs) on pyramidal neurons (Seamans et al., 2001; Gorelova et al., 2002; Kröner et al., 2007; Ren et al., 2018; Satoh et al., 2018). In chronic pain conditions, overall D1R activation in the ACC appears to play a critical role in controlling pain perception and pain relief. Not only is microinjection of D1R agonists in the ACC analgesic but D1R signaling in the ACC is required for effective pain relief in neuropathic mice (Lançon et al., 2021). Conversely, conditional KO of D1Rs in the ACC using a crisper/cas9 construct is hyperalgesic (Darvish-Ghane et al., 2020).
Gi-coupled DA receptor subtype 2 (D2Rs) is also expressed on PV+ interneurons but its expression decreases with age and is thought to play a role in the development of cortical circuits and in cognitive disorders such as schizophrenia in adulthood (Le Moine and Gaspar, 1998; Porter et al., 1999; Santana et al., 2009; Graham et al., 2015). Activation of D2Rs on interneurons has been shown to decrease IPSCs on pyramidal neurons (Seamans et al., 2001). D2Rs are also expressed on pyramidal neurons and their activation appears to play an excitatory role but the effect appears less robust than D1R activation (Green et al., 2020; Lançon et al., 2021). DA receptor subtype 3 (D3Rs) are also present in L2/3 ACC and although less is known about their direct effect on neuron excitability, they appear to play a role in gating the release of DA, NE, and ACh (Lacroix et al., 2003). The DA receptor subtype 5 (D5R) is expressed on pyramidal and might play a similar role as the D1R (Sarinana and Tonegawa, 2016).
In chronic pain D1R mRNA is decreased whereas D2R mRNA is increased in the ACC (Ortega-Legaspi et al., 2011). In line with this, D1R-mediated inhibition of AMPA-R currents is reduced in inflammatory pain models and other evidence indicates that D1R-evoked IPSCs are reduced in high-stress models (Satoh et al., 2018; Darvish-Ghane et al., 2022). All data converge to conclude that chronic pain is inducing a hypodopaminergic state in the ACC. Given that D2Rs have a higher sensitivity for DA than D1Rs, this hypodopaminergic state likely leads to a decrease in Gs-coupled signaling and an increase in Gi-coupled signaling. Activating DAergic projections in the mPFC, or activating DA neurons in the VTA directly, is analgesic in chronic pain models, thereby demonstrating that increasing cortical DA signaling is sufficient to occlude chronic pain symptoms (López-Avila et al., 2004; Huang et al., 2020).
What is causing a hypoactive VTA in chronic pain remains unclear but could be due to recruitment of the endogenous opioid system, with both the kappa opioid receptors (KORs) and the mu opioid receptors (MORs) playing a role. Dynorphin, the endogenous agonist for the Gi-coupled KOR, is well known for its role in gating DA release (Xi et al., 1998). The KOR is highly expressed on DA terminals within the mesolimbic pathway where its activation inhibits the release of DA. In line with this, selective KOR agonists have been shown to be highly aversive in both humans and rodents and this aversion is dependent on KOR expression in DA neurons (Liu et al., 2019). In the ACC, prodynorphin mRNA is increased following nerve injury, suggesting KOR over-activation could play a role in decreasing mesocortical DA release in chronic pain conditions (Palmisano et al., 2019). Actually, a recent study demonstrates that microinjection of a KOR antagonist in the ACC is analgesic in neuropathic mice while microinjection of a KOR agonist can produce a neuropathic-like phenotype in naïve mice (Navratilova et al., 2023). MORs, other Gi-coupled opioidergic receptors, are expressed on GABAergic neurons in the VTA and their activation inhibits GABA release, causing disinhibition of DAergic projections (Johnson and North, 1992; Narita et al., 2001). In chronic pain, MOR activation is decreased in the VTA, possibly mediated by MOR internalization, causing an elevation of synaptic GABA levels (Ozaki et al., 2002; Zuo, 2005). It is also possible that increased activity of the locus coeruleus (LC), the main source of central NE, is contributing to a hypoactive VTA in chronic pain. There is evidence of LC projections to the VTA and NE application on VTA neurons decreases the firing activity of DA neurons (Guiard et al., 2008).
Interestingly, there is a high comorbidity between several hypodopaminergic disorders, including Parkinson’s disease and major depressive disorder, and chronic pain (Miller and Cano, 2009; Ford, 2010). Parkinsonian patients have an exceptionally high incidence of non-myogenic chronic pain and fMRI studies demonstrate their ACC is hyperactive (Schestatsky et al., 2007; Blanchet and Brefel-Courbon, 2018). Supplementing supraspinal DA with L-DOPA decreases the sensory symptoms of chronic pain alongside ACC activity in Parkinsonian patients (Brefel-Courbon et al., 2005). In line with this, supplementing neuropathic mice with L-DOPA decreases chronic pain symptoms and ACC excitability (Lançon et al., 2021). As a proof of concept, it has also recently been shown that low doses of L-DOPA can reduce symptoms of chronic back pain in humans as well (Reckziegal et al., 2019). However, due to the known effects of long-term L-DOPA treatment on fine motor control, a more restrained approach to increasing cortical DA will be needed to reduce side effects.
Norepinephrine
NE is another monoamine with a robust effect on supraspinal pain circuits and it appears dysregulated in chronic pain conditions (Figure 2). Similar to the VTA, the LC has high cellular heterogeneity with projection-specific neuronal subsets. Whereas spinal-projecting LC neurons are antinociceptive (see the analgesic effects of α2 agonist clonidine injected intrathecally), cortically-projecting LC neurons are pro-nociceptive (Filos et al., 1992; De Kock et al., 2005; Hirschberg et al., 2017; Koga et al., 2020). Although the LC has a less defined topographic organization than the VTA, transcriptomic studies have shown that LC projections to the mPFC express high levels of both VMAT2 and the sodium channel accessory subunit Navβ3, allowing them to fire at high frequencies for prolonged periods (Kole et al., 2008; Chandler, 2016). In chronic pain, the LC displays increased excitability and seems to play a role in pathological pain processing as stimulation of LC projections to the ACC increases glutamatergic transmission and induces sensitization to pain and itch (Hirschberg et al., 2017; Koga et al., 2020; Camarena-Delgado et al., 2022; Iqbal et al., 2023).
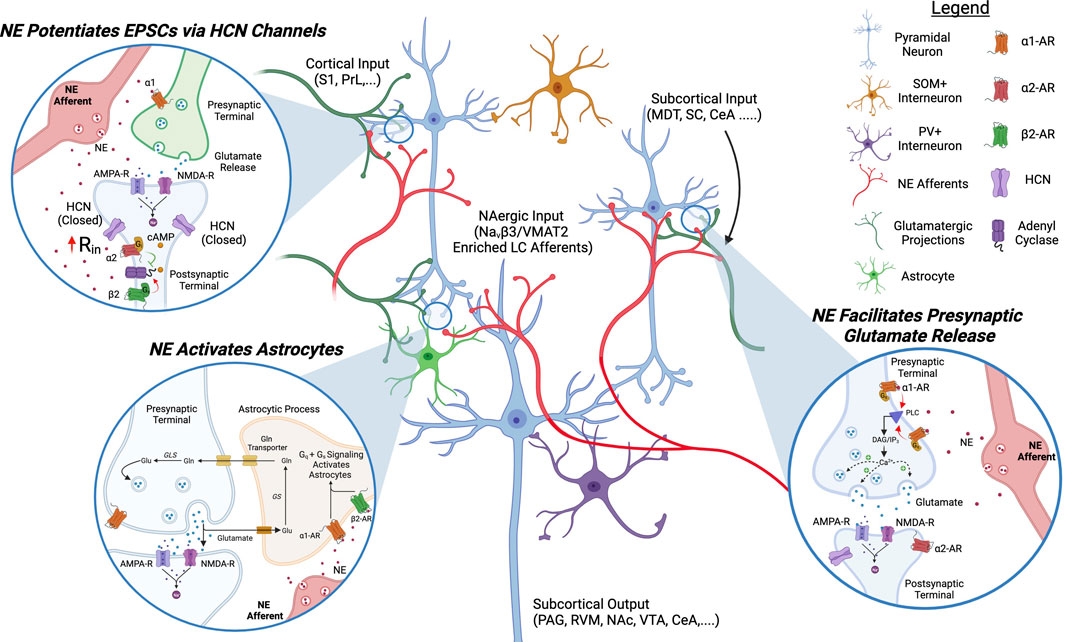
FIGURE 2. Proposed Model of NAergic Modulation of ACC Circuits. NAergic signaling in the ACC excites pyramidal neurons and activates astrocytes. α2 and α1 receptor activation increases EPSCs on pyramidal neurons by decreasing the open channel probability of HCN channels and promoting presynaptic glutamate release, respectively. α1 and β2 signaling activate astrocytes. NE, norepinephrine; HCN, hyperpolarization-activated cyclic nucleotide-gated channels; AC, adenyl cyclase; Gln, glutamine; PLC, phospholipase C; PAG, periaqueductal gray; S1, somatosensory cortex 1; PrL, prelimbic cortex; MDT, medial dorsal thalamus; SC, spinal cord; CeA, central nucleus of amygdala; RVM, rostral ventromedial medulla; NAc, nucleus accumbens; VTA, ventral tegmental area.
The predominant adrenergic receptors in the ACC are the GPCRs β2, α1, and α2. The Gq-coupled α1 receptor is expressed in L2/3/5/6 on glutamatergic terminals where its activation promotes the release of glutamate, increasing EPSCs (Santana et al., 2013; Zhang et al., 2013). The Gi-coupled α2 receptor is found on pyramidal neurons in L2/3/5/6 of the ACC and its activation has the opposite effect of D1R on HCN channels, i.e., closing them (Wang et al., 2007). α2 agonists inhibit HCN channels, increase input resistance, and increase the excitability of pyramidal neurons in the ACC (Zhang et al., 2013). The synergistic role of α1 and α2 activation leads NE to have a robust excitatory drive on ACC pyramidal and consequently on pain perception (Zhang et al., 2013; Kaushal et al., 2016).
Astrocytes and microglia play a key role in synapse reinforcement and pruning in cortical circuits and these non-neuronal cell types are also modulated by NE in the ACC and have been shown to play a role in tuning pain-induced aversion (Romanos et al., 2020; Iqbal et al., 2023). β2 and α1 receptors are expressed on astrocytes and their activation induces aversion: conditional KD of β2 receptors using micro-RNA based interference (miRNAi) reduces pain-induced CPA while their opto-activation on astrocytes promotes aversive memory formation (Iqbal et al., 2023). This is particularly interesting as it has been reported that overactivation of microglia in the SC is involved in pruning inhibitory synapses and promoting pain sensitization (Yousefpour et al., 2023). Given the ACC also exhibits a reduction in GABAergic signaling in neuropathic conditions, NAergic overactivation of microglia could play a similar role in cortical circuits (Blom et al., 2014). There is evidence that DA, by chemical similarity with NE, can also bind to α1 noradrenergic receptors expressed on astrocytes in the ACC and this α1-mediated catecholaminergic pathway may play a role in cognition. (Pittolo et al., 2022).
Due to the excitatory effect of NE on pyramidal excitability, activation of PFC-projecting LC fibers causes aversion and exacerbates pain perception whereas microinjection of an α1 antagonist in the ACC is analgesic (Kaushal et al., 2016; Hirschberg et al., 2017; Koga et al., 2020). In chronic pain states, the LC displays hyperexcitable characteristics and there is anatomical evidence of NE fiber sprouting in the ACC in neuropathic rodents (Cordeiro Matos et al., 2018; Camarena-Delgado et al., 2022). What causes the increase in cortical adrenergic activity is not established yet, but similarly to the situation in the VTA, ineffective MOR activation by endogenous opioids in ACC-projecting LC neurons may be causing a disinhibition in chronic pain states (Guajardo et al., 2017). The pathway leading to an increased NEergic excitation of pyramidal neurons in the ACC in chronic pain conditions could become a therapeutic target. However, due to the analgesic effects of spinal NE, designing a clinical approach that exclusively targets NE signaling in the mPFC remains a challenge.
Serotonin
5-HT projections from the medial dorsal raphe nucleus (DRN) to the ACC have also been shown to modulate E/I balance and pain sensitivity. Although DRN projections to the ACC have been identified using retrograde labeling, little is known about their response to both acute pain stimulation and whether they are dysregulated in chronic pain conditions (O’Hearn and Molliver, 1984; Sarter and Markowitsch, 1984; Waselus et al., 2011). Fortunately, due to the established role of 5-HT in psychosis, the impact of 5-HT on ACC circuits is well characterized (Figure 3).
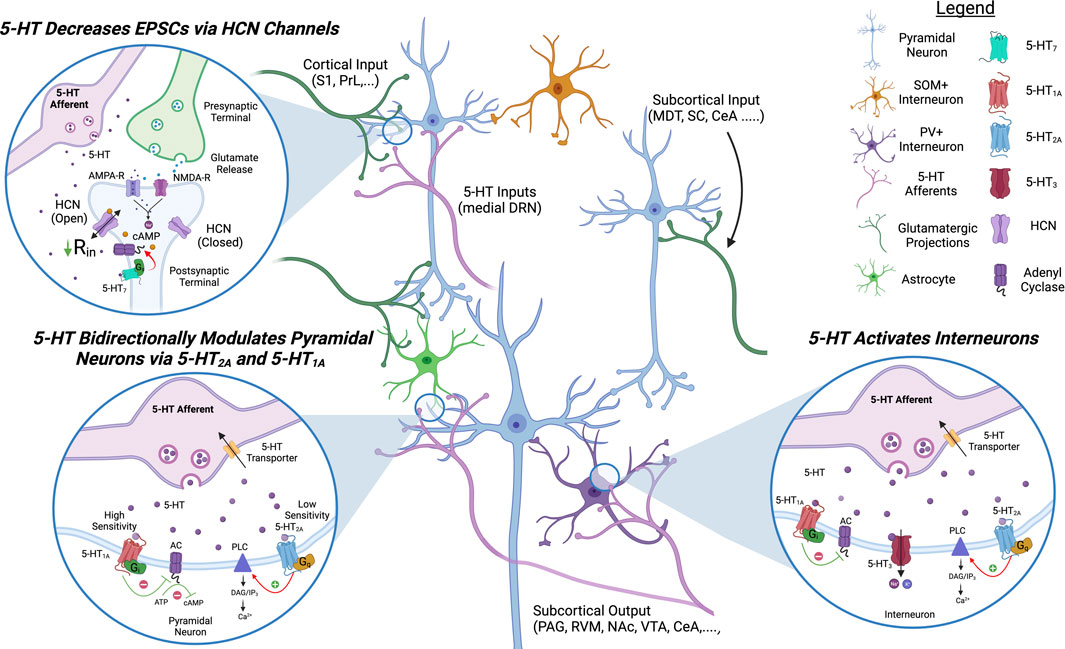
FIGURE 3. Proposed Model of Serotonergic Modulation of ACC Circuits. 5-HT signaling in the ACC inhibits pyramidal neurons and activates inhibitory interneurons. 5-HT7 decreases EPSCs on pyramidal neurons by increasing the open channel probability of HCN channels. 5-HT1 and 5-HT2 bidirectionally modulate pyramidal activity via Gi- and Gq-signaling, respectively. 5-HT2 and 5-HT3 signaling activates inhibitory interneurons. 5-HT, serotonin; HCN, hyperpolarization-activated cyclic nucleotide-gated channels; AC, adenyl cyclase; PLC, phospholipaseC; PAG, periaqueductal gray; S1, somatosensory cortex 1; PrL, prelimbic cortex; MDT, medial dorsal thalamus; SC, spinal cord; CeA, central nucleus of amygdala; RVM, rostral ventromedial medulla; NAc, nucleus accumbens; VTA, ventral tegmental area.
Pyramidal neurons have been shown to express the receptors 5-HT1A/B, 5-HT2A, 5-HT7, and 5-HT4; and 5-HT application on acute brain slices is generally inhibitory in synaptically isolated pyramidal neurons (Tanaka and Alan North, 1993; Santana et al., 2004; Santello et al., 2017; Tian et al., 2017). However, selective activation of postsynaptic 5-HT1A or 5-HT2A receptors appears to have opposite effects: agonists of Gi-coupled 5-HT1A hyperpolarize pyramidal cells, reduce EPSCs and NMDA currents, while selective activation of Gq-coupled 5-HT2A depolarizes pyramidal cells (Araneda and Andrade, 1991; Tanaka and Alan North, 1993; Avesar and Gulledge, 2012; Tian et al., 2017). As the 5-HT2A receptor has a lower affinity for 5-HT than 5-HT1A, an increase in 5-HT release could turn an inhibitory response into an excitatory response. The Gs-coupled 5-HT7 receptor, located on apical dendrites in L5, has also been demonstrated to modulate HCN channels similarly to D1R. Selective 5-HT7 agonists inhibit pyramidal neurons via opening of HCN channels and treatment with the 5-HT7 agonist LP-211, permeable to the blood-brain barrier, alleviates symptoms of chronic pain in animal models (Santello and Nevian, 2015; Santello et al., 2017). Less is known about the overall effects of Gs-coupled 5-HT4 receptor in the ACC circuits as its activation has mixed effects of pyramidal excitability (Yan, 2002).
The 5-HT1A, 5-HT2A, and 5-HT3 receptors are also expressed on GABAergic interneurons (Zhou and Hablitz, 1999; Yan, 2002; Santana et al., 2004; Puig et al., 2010). Roughly 30% of PV+ interneurons express 5-HT1A or 5-HT2A and the 5-HT2A receptor is expressed on somatostatin (SOM+) interneurons in neighboring cortical regions (Puig et al., 2010; De Filippo et al., 2021). 5-HT1A activation on fast spiking interneurons in the ACC decreases interneuron activity while 5-HT2A activation increases it (Puig et al., 2010). The 5-HT-gated cation channel 5-HT3 depolarizes GABAergic interneurons and increases IPSCs on pyramidal neurons (Zhou and Hablitz, 1999; Férézou et al., 2002; Puig et al., 2004). The role of cortical 5-HT on pain responses is less characterized than DA or NE but there is evidence the 5-HT2A and 5-HT7 receptors play a role in sensory discrimination and pain sensitization (Gresch et al., 2007; Santello et al., 2017).
At the source of cortical 5-HT projections, it has been reported that the DRN can be inhibited but also activated by noxious stimulation (Sanders et al., 1980; Porro et al., 1991). A study using microdialysis indicates increased 5-HT concentrations in the PFC of neuropathic mice following DRN stimulation (Ito et al., 2013) Modulating GABAergic interneurons in the DRN can bidirectionally modulate nociception: activation of DRN-GABA+ neurons is anti-nociceptive while inhibition of DRN-GABA+ is pro-nociceptive (Xie et al., 2022). Additionally, there is a high degree of crosstalk between the DRN and VTA, likely affecting cortical DA release (Wang et al., 2023). Recent findings indicate that VGluT3+ DRN projections to the VTA play a role in tuning pain perception by modulating DA release in the striatum and that this pathway is inhibited in neuropathic conditions, decreasing glutamatergic drive on DAT+ VTA neurons (Wang et al., 2023). Further research is required to determine how the DRN and its projections to the ACC are affected by the onset of chronic pain.
Acetylcholine
ACh, originating from the basal forebrain, is another neuromodulator that has a strong impact on mPFC circuitry and is well studied for its role in sustained attention and working memory (Passetti et al., 2000; Howe et al., 2017). The ACC expresses both nicotinic ACh receptors (nAChRs), consisting mostly of the α7, α4, and β2 subunits, and muscarinic receptors (mAChRs), mainly the subtypes M1, M2, and M4 (Albuquerque et al., 2009). Cholinergic projections to the mPFC can be separated into 4 distinct pathways, each innervating different regions of the mPFC with different modulatory effects (Bloem et al., 2014; Oswald et al., 2022). Due to the expression of a wide range of cholinergic receptors and distinct cholinergic inputs, the effect of ACh on ACC circuitry is situationally dependent as it can both inhibit or excite neurons depending on the situation, and whether ACh is release phasically or tonically (Gulledge and Stuart, 2005; Aracri et al., 2010). nAChRs are ACh-gated cation channels expressed on both GABAergic interneurons and pyramidal neurons in the ACC and their activation impacts ACC circuitry differently depending on the cortical layer. In L2/3 nAChR activation is inhibitory to pyramidal neurons due to the expression of nAChRs on GABAergic somata and terminals whereas in L5/6 nAChR activation induces pyramidal firing due to a higher expression on pyramidal neurons (Araci et al., 2010; Poorthuis et al., 2013). Although nAChRs are also expressed on GABAergic interneurons in L5/6, their effect is dependent on glutamatergic transmission and their activation can be either excitatory or inhibitory on interneurons (Vidal and Changeux, 1989; Gulledge and Stuart, 2005; Aracri et al., 2010).
The mAChRs are GPCRs expressed on pyramidal neurons in L2/3/5/6 and are generally excitatory to L5/6 but can induce inhibition in L2/3 due to dual expression on PV+ GABAergic interneurons (Disney and Aoki, 2008; Shirey et al., 2009). Selective M1 agonists increase EPSCs in the mPFC L5/6 and can induce persistent firing, the neuronal substrate for working memory (Zhang and Philippe, 2010; Kamiński et al., 2017). Due to this, mAChRs play a major role in modulating sustained memory tasks in rodents and primates (Shirey et al., 2009; Galvin et al., 2020).
In pain, cholinergic projections from the medial septal nucleus (MSN) to the ACC is pronociceptive and stimulation of these cholinergic projections is hyperalgesic in inflammatory models of chronic pain (Jiang et al., 2018). Conversely, chemogenetic inhibition of the MSN to ACC pathway is analgesic and produces conditioned place preference (CPP) in chronic pain (Jiang et al., 2018). Scopolamine, a nonspecific muscarinic antagonist, is commonly used for its anti-depressive effects and microinjection into the ACC produces anti-nociceptive effects, possibly by inhibiting nociception-related attention (Ortega-Legaspi et al., 2003). However, due to M1 expression on GABAergic interneurons and the differential effect of ACh depending on physiological state, increased ACh release can be analgesic: microinjection of the M1 agonist McN-A-343 into the ACC increases mechanical nociceptive thresholds via promoting GABA release (Koga et al., 2017).
Although it is unknown if chronic pain states disrupt the release of ACh in the ACC, the monoamines DA and NE play an active role in modulating mAChR-induced persistent firing (Zhang et al., 2013; Lançon et al., 2021). Therefore, we can safely infer that dysregulation of their release affects the functionality of cholinergic signaling in the ACC. In the striatum, ACh has also recently been shown to generate action potentials in distal DA axons via activation of nAChRs, promoting the local release of DA (Liu et al., 2022). Therefore, alterations in the release of ACh could be affecting the local release of DA in the ACC through a similar crosstalk, and this merits further investigation.
Oxytocin
Neuropeptides and other intercellular mediators have also been shown to significantly affect ACC circuitry and are affected by the onset of chronic pain. The neuropeptide oxytocin (OXT) specifically appears to play a key role in modulating ACC outputs as oxytocin microinjection in the ACC increases mechanical withdrawal thresholds (Li Anna et al, 2021). Physiologically, OXT is released in the ACC via projections from the paraventricular nucleus (PVN) in hypothalamus. In agreement with the analgesic effects of OXT microinjection in the ACC, optogenetic stimulation of the PVN to ACC pathway is also anti-nociceptive (Li Xu-Hui et al, 2021). This effect appears mediated by both the modulation of pyramidal neurons and GABAergic interneurons via the Gq-coupled oxytocin receptor (OXTR) and vasopressin 1A receptor (Schorscher-Petcu et al., 2010). OXT application in acute brain slices significantly decreases E/I ratio by decreasing EPSCs and increasing IPSCs on pyramidal neurons (Li Anna et al, 2021). This is mainly mediated by activation of GABAergic interneurons: oxytocin increases the resting membrane potential and decreases the rheobase of interneurons but not pyramidal cells. In chronic pain, OXT levels in the ACC are unchanged but the OXTR expression is significantly increased (Li Xu-Hui et al, 2021).
Connecting chronic pain with attentional dysfunction
Chronic pain patients commonly report deficits in working memory, emotional regulation, attention, and anxiety; all of which have been shown to be processed by the ACC (Rudy et al., 1988; Hart et al., 2003; Apkarian et al., 2004; Grégoire et al., 2012; Guerreiro et al., 2022). There is an overwhelming overlap between the cognitive deficits commonly seen in chronic pain patients and the cognitive roles associated with the ACC, hinting that a dysregulated ACC could be a central hub for chronic pain-induced cognitive deficits (Kummer et al., 2020). This link works in the other direction as well, as patients with pathological deficits in attention control and working memory, such as ADHD and PTSD, display a high prevalence of chronic pain (Brennstuhl et al., 2015; Kind and Otis, 2019; Kasahara et al., 2020; Battison et al., 2023). Pain, being one crucial survival signal from an evolutionary standpoint, demands our immediate attention; thus, it is understandable why it is so deeply integrated into our cognitive processes, and why chronic pain can exert such a profound influence on our attention.
The Arnsten group has repeatedly shown that the homeostatic balance of DA and NE in the mPFC is key to tuning attention in primates and changes in the cortical release of these neuromodulators is associated with attention disorders such as ADHD and PTSD (Arnsten, 2000; Vijayraghavan et al., 2007). Since chronic pain also influences the ratio of NE/DA release in the ACC, it may explain why chronic pain patients have a high prevalence of attention deficits and why there is a high comorbidity between attention disorders and chronic pain. Both ADHD and chronic pain states could result in a similar shift in the differential release of NE and DA, explaining the significant overlap in their symptoms. Similarly, depressive and Parkinsonian patients have decreased DAergic signaling and commonly report attention deficits as well as chronic pain (Mehler-Wex et al., 2006; Zhou et al., 2012).
ACC pyramidal neurons are capable of glutamatergic and cholinergic persistent firing, a non-synaptic cellular substrate for working memory, and it has been demonstrated that DA and NE bidirectional control the duration of this persistent activity: DA decreases it while NE increases it (Zhang et al., 2013; Kamiński et al., 2017; Lançon et al., 2021). Therefore, in neuropathic conditions, the disrupted homeostatic ratio in DA/NE release leads to a dysfunction in how persistent firing is initiated and maintained, likely contributing to deficits in sustained attention and working memory.
Furthermore, decreased levels of DA in the ACC, together with increased levels of NE, decreases the rheobase of pyramidal neurons, making them easier to fire ectopically. Chronic pain-induced increase in the likelihood of pyramidal activation with any given stimulus leads to disruptions in what we pay attention to. Recent endoscopic imaging studies conclude that the proportion of nociception-sensitive neurons in the ACC is increased in neuropathic conditions (Li Anna et al, 2021; Acuña et al., 2023). Behaviorally, a disruption in the filtering process required for sensory discrimination might explain why a noxious stimulus specifically receives our attention in normal conditions and why an innocuous stimulus can be perceived as painful in neuropathic conditions (Vander Weele et al., 2018). This filtering mechanism in the ACC could underlie a key cortical mechanism sensitive to modulation by monoamines that can underlie allodynia and spontaneous pain in chronic pain patients. Persistent pain pathologically sensitizes our attention, increasing both pain-related and innocuous attention signals, shifting the homeostatic balance of attention to innocuous somatosensory, auditory, and visual stimuli. In this regard, chronic pain can be considered an attentional disorder.
Given this, the analgesic effects of monoamine signaling in the ACC likely stems from their influence not only on the emotional valence of pain, but also through their role in tuning our pain awareness. This might explain the role of the ACC in modulating the sensory aspect of pain since pain thresholds, commonly assayed via withdrawal and threshold tests such as the von Frey and the Hargreaves assays, are a function of both peripheral and central sensitivity to external stimuli. Schizophrenic patients commonly report pain insensitivity and have increased DAergic and serotonergic signaling in the brain (Dworkin, 1994; Stahl, 2018). As their peripheral nociceptive nerves are functional and they do not exhibit any somatosensory deficits apart from abnormal perception, this pain insensitivity is potentially mediated by the PFC (Chang and Lenzenweger, 2005). This is supported by EEG studies, which show that peripheral nerve stimulation in schizophrenic patients leads to reduced response amplitudes in the dlPFC and ACC, but not in somatosensory cortex (Huang et al., 2010; Daskalakis et al., 2020). Therefore, analgesia induced by microinjection of DA or 5-HT in the ACC is plausibly functioning by decreasing pain awareness rather than pain sensation.
In light of this, current treatments aimed at treating chronic pain can be somewhat effective at decreasing the sensory symptoms, but because they are mainly peripherally acting, they do not alleviate the cognitive deficits (Schiltenwolf et al., 2014). Systemic gabapentin, a commonly prescribed analgesic, leads to even greater cognitive dysfunction in chronic pain patients (Grégoire et al., 2012; Shem et al., 2018). Centrally acting non-selective monoamine reuptake inhibitors show some efficacy at treating the cognitive deficits in chronic pain. The non-selective NE and 5-HT reuptake inhibitor duloxetine, a commonly prescribed analgesic for cancer treatments, alleviates chronic pain-induced cognitive, but not emotional, symptoms (Grégoire et al., 2012; Chu et al., 2015).
Next steps and conclusion
Dysregulation of monoamines and other neuromodulators in the ACC in chronic pain as a main contributor to pathological cortical hyperexcitability and chronic pain symptoms is now a widely accepted working hypothesis in the pain research community (Ong et al., 2019; Tan and Kuner, 2021; Kasanetz et al., 2022). The controlled balance of Gs/Gi-signaling in specific ACC neurons, as well as in their astrocytic and microglial partners, is key for the healthy integration of innocuous and nociceptive inputs reaching the pain matrix (Figure 4). Disruptions in this balance in one direction leads to hypersensitivity for both nociceptive and innocuous signals while disruptions in the other direction can induce dissociation and pain insensitivity (Dworkin, 1994). Although key neuromodulatory pathways have been identified as instigators of pathological pain signaling, there are still holes in the literature that need to be addressed.
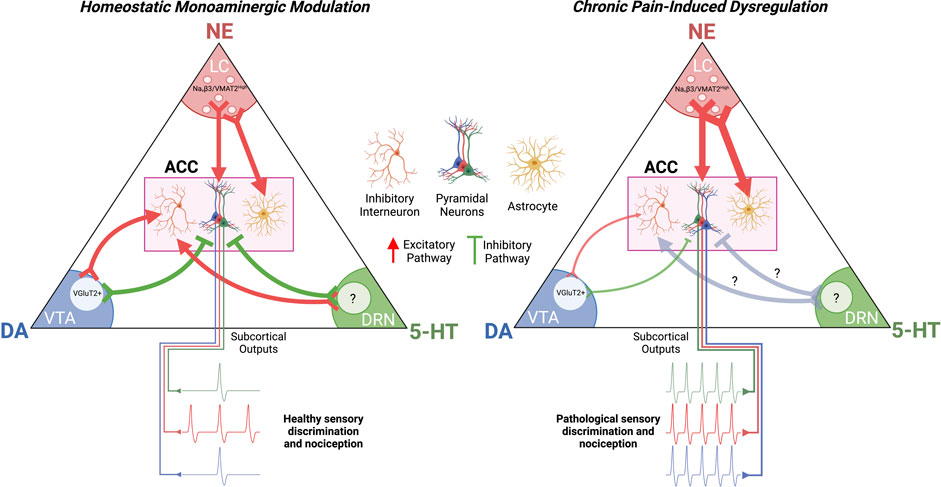
FIGURE 4. Chronic Pain-Induced Dysregulation of Cortical Monoamine Signaling. Chronic pain potentiates NAergic signaling in the ACC while decreasing DAergic signaling. This increases excitatory drive on pyramidal neurons while decreasing excitatory drive on inhibitory interneurons. The effect of chronic pain on 5-HT signaling in the ACC is not established. The resultant effect of dysregulated monoaminergic signaling in the ACC is pathological nociception and a decrease in sensory discrimination. ACC, anterior cingulate cortex; LC, locus coeruleus; VTA, ventral tegmental area; DRN, dorsal raphe nucleus.
For instance, how chronic pain influences ACh and 5-HT inputs in the ACC has yet to be investigated and could provide insight into their roles in modulating normal and pathological pain perception. In the LC and VTA, it remains unclear what is prompting the changes in their activity and these midbrain regions should be further studied in neuropathic conditions. Additionally, what causes a loss of inhibitory synapses in the neuropathic ACC has yet to be identified. This could be mediated by overactivation of astrocytes and/or microglia but could also be mediated by abnormal corelease of DA with the classical neurotransmitter glutamate. There is precedence for glutamate originating for DAT+ projections forming asymmetrical synapses on PV+ interneurons and dopamine can form hub synapses with both glutamatergic and GABAergic synapses (Qi et al., 2016; Paget-Blanc et al., 2022). As the D1R was found to be expressed on the presynaptic GABA terminals innervating pyramidal cells, it is possible DA is reinforcing these synapses as well as activating PV+ interneurons with glutamate (Muly et al., 1998). The role of KORs in modulating DA release in the ACC also deserves serious investigation.
Moreover, it is intriguing, and apparently contrary to expectation, that Gs activation via DA/NE/5-HT signaling on pyramidal neurons in the ACC results in inhibition, whereas Gi activation leads to excitation. This phenomenon seems to arise from the modulation of HCN channels through cAMP and their consequent impact on input resistance. Nevertheless, further research is required to determine all the downstream effects of PKA activation.
Central monoaminergic pathways also exhibit a high degree of sexual dimorphism and should be studied further in this regard. For example, the female hormone estradiol has a robust excitatory effect on VTA neurons and could explain why L-DOPA is more successful in females than males at decreasing symptoms of lower back pain (Yoest et al., 2018; Reckziegel et al., 2019). The LC also exhibits prominent sex differences: MOR activation on LC neurons causes a dramatic reduction in NE release in males, but not females (Bangasser et al., 2011; Guajardo et al., 2017).
In addition, this review focuses on the ACC but more attention should be given to how monoamines affect other cortical regions of the pain matrix dysregulated in chronic pain states. The neighboring prelimbic (PrL) cortex is particularly intriguing due to the opposite effect chronic pain induces relative to the ACC: PrL neurons are hypoactive in neuropathic conditions and their activation is analgesic (Wang et al., 2015). Since the PrL cortex also receives dense monoaminergic inputs, it would be interesting to understand how alterations in the release of monoamines induces hypoactivity rather than hyperexcitability. Additionally, the insular cortex appears to play a larger role in modulating pain perception in primates than in rodents. It deserves more investigation as it has also been identified as one of the most active brain regions in neuropathic patients (Jensen et al., 2016; Lu et al., 2016; Ferraro et al., 2021; Tan and Kuner, 2021).
Lastly, although ACC activity is positively correlated with pain sensitization, this is not always the case. Recent reports indicate that gabapentin and nitrous oxide can in fact induce an increase in ACC activity alongside analgesia (Acuña et al., 2023; Weinrich et al., 2023). Given this, the role of the ACC in modulating attention and pain perception is more nuanced than it appears and it may be possible to reinstate proper somatosensory filtering by increasing the activity of specific ACC circuits.
Although further studies are warranted to determine the impact of chronic pain on supraspinal regions involved in the processing of nociceptive information, tremendous progress has been made in the past decade. Our current model remains incomplete however and finding a treatment that selectively corrects cortical monoaminergic function in chronic pain might be key to the development of novel therapeutics.
Author contributions
KL: Conceptualization, Writing–original draft, Writing–review and editing. PS: Conceptualization, Writing–review and editing.
Funding
The author(s) declare financial support was received for the research, authorship, and/or publication of this article. CIHR grant PJT-153098 (PS), Louise and Alan Edwards Foundation pilot grant (PS) and postdoctoral fellowship (KL).
Acknowledgments
All figures were produced with BioRender.
Conflict of interest
The authors declare that the research was conducted in the absence of any commercial or financial relationships that could be construed as a potential conflict of interest.
The author(s) declared that they were an editorial board member of Frontiers, at the time of submission. This had no impact on the peer review process and the final decision.
Publisher’s note
All claims expressed in this article are solely those of the authors and do not necessarily represent those of their affiliated organizations, or those of the publisher, the editors and the reviewers. Any product that may be evaluated in this article, or claim that may be made by its manufacturer, is not guaranteed or endorsed by the publisher.
References
Acuña, M. A., Kasanetz, F., De Luna, P., Falkowska, M., and Nevian, T. (2023). Principles of nociceptive coding in the anterior cingulate cortex. Proc. Natl. Acad. Sci. 120 (23), e2212394120. doi:10.1073/pnas.2212394120
Aghajanian, G. K., and Marek., G. J. (1999). Serotonin, via 5-HT2A receptors, increases EPSCs in layer V pyramidal cells of prefrontal cortex by an asynchronous mode of glutamate release. Brain Res. 825 (1-2), 161–171. doi:10.1016/s0006-8993(99)01224-x
Albuquerque, E. X., Pereira, E. F. R., Alkondon, M., and Rogers, S. W. (2009). Mammalian nicotinic acetylcholine receptors: from structure to function. Physiol. Rev. 89, 73–120. doi:10.1152/physrev.00015.2008
Apkarian, V. A., Yamaya Sosa, , Krauss, B. R., Thomas, P. S., Fredrickson, B. E., Levy, R. E., et al. (2004). Chronic pain patients are impaired on an emotional decision-making task. Pain 108 (1-2), 129–136. doi:10.1016/j.pain.2003.12.015
Aracri, P., Consonni, S., Morini, R., Perrella, M., Rodighiero, S., Amadeo, A., et al. (2010). Tonic modulation of GABA release by nicotinic acetylcholine receptors in layer V of the murine prefrontal cortex. Cereb. Cortex 20 (7), 1539–1555. doi:10.1093/cercor/bhp214
Araneda, R., and Andrade, R. (1991). 5-Hydroxytryptamine2 and 5-hydroxytryptamine1A receptors mediate opposing responses on membrane excitability in rat association cortex. Neuroscience 40 (2), 399–412. doi:10.1016/0306-4522(91)90128-b
Arnsten, A. F. T., and Katya, R. (2012). Neurobiological circuits regulating attention, cognitive control, motivation, and emotion: disruptions in neurodevelopmental psychiatric disorders. J. Am. Acad. Child Adolesc. Psychiatry 51 (4), 356–367. doi:10.1016/j.jaac.2012.01.008
Arnsten, A. F. T., and Pliszka, S. R. (2011). Catecholamine influences on prefrontal cortical function: relevance to treatment of attention deficit/hyperactivity disorder and related disorders. Pharmacol. Biochem. Behav. 99 (2), 211–216. doi:10.1016/j.pbb.2011.01.020
Arnsten, A. F. T. (2000). Stress impairs prefrontal cortical function in rats and monkeys: role of dopamine D1 and norepinephrine alpha-1 receptor mechanisms. Prog. Brain Res. 126, 183–192. doi:10.1016/S0079-6123(00)26014-7
Avesar, D., and Gulledge, A. T. (2012). Selective serotonergic excitation of callosal projection neurons. Front. neural circuits 6, 12. doi:10.3389/fncir.2012.00012
Bangasser, D. A., Zhang, X., Garachh, V., Hanhauser, E., and Valentino, R. J. (2011). Sexual dimorphism in locus coeruleus dendritic morphology: a structural basis for sex differences in emotional arousal. Physiology Behav. 103 (3-4), 342–351. doi:10.1016/j.physbeh.2011.02.037
Bastuji, H., Frot, M., Perchet, C., Magnin, M., and Garcia-Larrea, L. (2016). Pain networks from the inside: spatiotemporal analysis of brain responses leading from nociception to conscious perception. Hum. Brain Mapp. 37 (12), 4301–4315. doi:10.1002/hbm.23310
Battison, E. A. J., Brown, P. C. M., Holley, A. L., and Wilson, A. C. (2023). Associations between chronic pain and attention-deficit hyperactivity disorder (ADHD) in youth: a scoping review. Children 10 (1), 142. doi:10.3390/children10010142
Bernard, B., Schoppink, L., Rotaru, D. C., Faiz, A., Hendriks, P., Mansvelder, H. D., et al. (2014). Topographic mapping between basal forebrain cholinergic neurons and the medial prefrontal cortex in mice. J. Neurosci. 34 (49), 16234–16246. doi:10.1523/JNEUROSCI.3011-14.2014
Blanchet, P. J., and Brefel-Courbon, C. (2018). Chronic pain and pain processing in Parkinson's disease. Prog. Neuro-Psychopharmacol. Biol. Psychiatry 87, 200–206. doi:10.1016/j.pnpbp.2017.10.010
Blom, S. M., Pfister, J.-P., Santello, M., Senn, W., and Nevian, T. (2014). Nerve injury-induced neuropathic pain causes disinhibition of the anterior cingulate cortex. J. Neurosci. 34 (17), 5754–5764. doi:10.1523/JNEUROSCI.3667-13.2014
Brefel-Courbon, C., Payoux, P., Thalamas, C., Ory, F., Quelven, I., Chollet, F., et al. (2005). Effect of levodopa on pain threshold in Parkinson's disease: a clinical and positron emission tomography study. Mov. Disord. official J. Mov. Disord. Soc. 20, 1557–1563. doi:10.1002/mds.20629
Brennstuhl, M.-Jo, Tarquinio, C., and Montel, S. (2015). Chronic pain and PTSD: evolving views on their comorbidity. Perspect. psychiatric care 51, 295–304. doi:10.1111/ppc.12093
BushnellCatherine, M. M. Č., Low, L. A., and Low, L. A. (2013). Cognitive and emotional control of pain and its disruption in chronic pain. Nat. Rev. Neurosci. 14 (7), 502–511. doi:10.1038/nrn3516
Camarena-Delgado, , Carmen, M.L.-T., Suárez-Pereira, I., Bravo, L., López-Martín, C., Garcia-Partida, J. A., et al. (2022). Nerve injury induces transient locus coeruleus activation over time: role of the locus coeruleus–dorsal reticular nucleus pathway. Pain 163 (5), 943–954. doi:10.1097/j.pain.0000000000002457
Casey, K. L. (1999). Forebrain mechanisms of nociception and pain: analysis through imaging. Proc. Natl. Acad. Sci. 96 (14), 7668–7674. doi:10.1073/pnas.96.14.7668
Chandler, D. J. (2016). Evidence for a specialized role of the locus coeruleus noradrenergic system in cortical circuitries and behavioral operations. Brain Res. 1641, 197–206. doi:10.1016/j.brainres.2015.11.022
Chang, B. P., and Lenzenweger, M. F. (2005). Somatosensory processing and schizophrenia liability: proprioception, exteroceptive sensitivity, and graphesthesia performance in the biological relatives of schizophrenia patients. J. Abnorm. Psychol. 114 (1), 85–95. doi:10.1037/0021-843X.114.1.85
Chapman, S. L., Byas-Smith, M. G., and Reed, B. A. (2002). Effects of intermediate- and long-term use of opioids on cognition in patients with chronic pain. Clin. J. Pain 18 (4), S83–S90. doi:10.1097/00002508-200207001-00010
Chen, Q.-Yu, Li, X.-H., and Zhuo, M. (2021). NMDA receptors and synaptic plasticity in the anterior cingulate cortex. Neuropharmacology 197, 108749. doi:10.1016/j.neuropharm.2021.108749
Chen, T., Taniguchi, W., Chen, Q.-Yu, Tozaki-Saitoh, H., Song, Q., Liu, R.-H., et al. (2018). Top-down descending facilitation of spinal sensory excitatory transmission from the anterior cingulate cortex. Nat. Commun. 9 (1), 1886–1917. doi:10.1038/s41467-018-04309-2
Chu, K. L., Xu, J., Frost, J., Li, L., Gomez, E., Dart, M. J., et al. (2015). A selective α2 B adrenoceptor agonist (A-1262543) and duloxetine modulate nociceptive neurones in the medial prefrontal cortex, but not in the spinal cord of neuropathic rats. Eur. J. Pain 19 (5), 649–660. doi:10.1002/ejp.586
Clarkson, R. L., Liptak, A. T., Gee, S. M., Sohal, V. S., and Bender, K. J. (2017). D3 receptors regulate excitability in a unique class of prefrontal pyramidal cells. J. Neurosci. 37, 5846–5860. doi:10.1523/JNEUROSCI.0310-17.2017
Cools, R., and Arnsten, A. F. T. (2022). Neuromodulation of prefrontal cortex cognitive function in primates: the powerful roles of monoamines and acetylcholine. Neuropsychopharmacology 47 (1), 309–328. doi:10.1038/s41386-021-01100-8
Cordeiro Matos, S., Zamfir, M., Longo, G., Ribeiro-da-Silva, A., and Philippe, S. (2018). Noradrenergic fiber sprouting and altered transduction in neuropathic prefrontal cortex. Brain Struct. Funct. 223, 1149–1164. doi:10.1007/s00429-017-1543-7
Cordeiro Matos, S., Zhang, Z., and Philippe, S. (2015a). Peripheral neuropathy induces HCN channel dysfunction in pyramidal neurons of the medial prefrontal cortex. J. Neurosci. 35, 13244–13256. doi:10.1523/JNEUROSCI.0799-15.2015
Cordeiro Matos, S., Zhang, Z., and Philippe, S. (2015b). Peripheral neuropathy induces HCN channel dysfunction in pyramidal neurons of the medial prefrontal cortex. J. Neurosci. 35 (38), 13244–13256. doi:10.1523/JNEUROSCI.0799-15.2015
Dahlhamer, J., Lucas, J., Zelaya, C., Nahin, R., Mackey, S., Lynn, D. B., et al. (2018). Prevalence of chronic pain and high-impact chronic pain among adults—United States. Morb. Mortal. Wkly. Rep. 67, 1001–1006. doi:10.15585/mmwr.mm6736a2
Darvish-Ghane, S., Brendan, L., Facciol, A., Chatterjee, D., and Loren, J. (2022). Inflammatory pain alters dopaminergic modulation of excitatory synapses in the anterior cingulate cortex of mice. Neuroscience 498, 249–259. doi:10.1016/j.neuroscience.2022.07.010
Darvish-Ghane, S., Manabu, Y., and Zhuo, M. (2016). Dopaminergic modulation of excitatory transmission in the anterior cingulate cortex of adult mice. Mol. Pain 12, 1744806916648153. doi:10.1177/1744806916648153
Darvish-Ghane, S., Quintana, C., Beaulieu, J.-M., and LorenMartin, J. (2020). D1 receptors in the anterior cingulate cortex modulate basal mechanical sensitivity threshold and glutamatergic synaptic transmission. Mol. Brain 13 (1), 121–215. doi:10.1186/s13041-020-00661-x
Daskalakis, A. A., Zomorrodi, R., Blumberger, D. M., and Rajji, T. K. (2020). Evidence for prefrontal cortex hypofunctioning in schizophrenia through somatosensory evoked potentials. Schizophrenia Res. 215, 197–203. doi:10.1016/j.schres.2019.10.030
De Filippo, , Roberto, B. R. R., Alexander, S., Cooper, C., Tukker, J. J., Harms, C., et al. (2021). Somatostatin interneurons activated by 5-HT2A receptor suppress slow oscillations in medial entorhinal cortex. Elife 10, e66960. doi:10.7554/eLife.66960
De Kock, M., Lavand’homme, P., and Waterloos, H. (2005). The short-lasting analgesia and long-term antihyperalgesic effect of intrathecal clonidine in patients undergoing colonic surgery. Anesth. Analgesia 101, 566–572. doi:10.1213/01.ANE.0000157121.71808.04
Disney, A. A., and Aoki, C. (2008). Muscarinic acetylcholine receptors in macaque V1 are most frequently expressed by parvalbumin-immunoreactive neurons. J. Comp. Neurology 507 (5), 1748–1762. doi:10.1002/cne.21616
Dworkin, H. R. (1994). Pain insensitivity in schizophrenia: a neglected phenomenon and some implications. Schizophr. Bull. 20 (2), 235–248. doi:10.1093/schbul/20.2.235
Férézou, I., Bruno, C., Hill, E. L., Rossier, J., Hamel, E., and Lambolez, B. (2002). 5-HT3 receptors mediate serotonergic fast synaptic excitation of neocortical vasoactive intestinal peptide/cholecystokinin interneurons. J. Neurosci. 22 (17), 7389–7397. doi:10.1523/JNEUROSCI.22-17-07389.2002
Ferraro, S., Klugah-Brown, B., Tench, C. R., Yao, S., Anna, N., Demichelis, G., et al. (2021). Dysregulated anterior insula reactivity as robust functional biomarker for chronic pain–convergent evidence from neuroimaging meta-analysis. https://www.medrxiv.org/content/10.1101/2021.03.24.21254023v1.full.
Filos, K. S., Goudas, L. C., Patroni, O., and Polyzou, V. (1992). Intrathecal clonidine as a sole analgesic for pain relief after cesarean section. J. Am. Soc. Anesthesiol. 77 (2), 267–274. doi:10.1097/00000542-199208000-00008
Galvin, C. V., Sheng, T. Y., Paspalas, C. D., Yang, Y., Jin, Lu E., Datta, D., et al. (2020). Muscarinic M1 receptors modulate working memory performance and activity via KCNQ potassium channels in the primate prefrontal cortex. Neuron 106 (4), 649–661. doi:10.1016/j.neuron.2020.02.030
Glausier, R. J., Khan, Z. U., and Chris Muly, E. (2009). Dopamine D1 and D5 receptors are localized to discrete populations of interneurons in primate prefrontal cortex. Cereb. cortex 19 (8), 1820–1834. doi:10.1093/cercor/bhn212
Gorelova, N., Seamans, J. K., and Yang, C. R. (2002). Mechanisms of dopamine activation of fast-spiking interneurons that exert inhibition in rat prefrontal cortex. J. neurophysiology 88 (6), 3150–3166. doi:10.1152/jn.00335.2002
Graham, L. D., Durai, H. H., Garden, J. D., Cohen, E. L., Echevarria, F. D., and Stanwood, G. D. (2015). Loss of dopamine D2 receptors increases parvalbumin-positive interneurons in the anterior cingulate cortex. ACS Chem. Neurosci. 6 (2), 297–305. doi:10.1021/cn500235m
Green, M. S., Nathani, S., Zimmerman, J., Fireman, D., and Urs, N. M. (2020). Retrograde labeling illuminates distinct topographical organization of D1 and D2 receptor-positive pyramidal neurons in the prefrontal cortex of mice. Eneuro 7, ENEURO.0194–20.2020. doi:10.1523/ENEURO.0194-20.2020
Grégoire, S., Michaud, V., Chapuy, E., Eschalier, A., and Ardid, D. (2012). Study of emotional and cognitive impairments in mononeuropathic rats: effect of duloxetine and gabapentin. Pain® 153 (8), 1657–1663. doi:10.1016/j.pain.2012.04.023
Gresch, J. P., Barrett, R. J., Sanders-Bush, E., and Smith, R. L. (2007). 5-Hydroxytryptamine (serotonin) 2A receptors in rat anterior cingulate cortex mediate the discriminative stimulus properties of d-lysergic acid diethylamide. J. Pharmacol. Exp. Ther. 320 (2), 662–669. doi:10.1124/jpet.106.112946
Guajardo, M. H., Snyder, K., Ho, A., and RitaValentino, J. (2017). Sex differences in μ-opioid receptor regulation of the rat locus coeruleus and their cognitive consequences. Neuropsychopharmacology 42 (6), 1295–1304. doi:10.1038/npp.2016.252
Guerreiro, R. S., Guimaraes, M. R., Silva, J. M., Dioli, C., Vamvaka-Iakovou, A., Sousa, R., et al. (2022). Chronic pain causes Tau-mediated hippocampal pathology and memory deficits. Mol. Psychiatry 27 (11), 4385–4393. doi:10.1038/s41380-022-01707-3
Guiard, B. P., El Mansari, M., and Blier, P. (2008). Cross-talk between dopaminergic and noradrenergic systems in the rat ventral tegmental area, locus ceruleus, and dorsal hippocampus. Mol. Pharmacol. 74 (5), 1463–1475. doi:10.1124/mol.108.048033
Gulledge, T. A., and Stuart, G. J. (2005). Cholinergic inhibition of neocortical pyramidal neurons. J. Neurosci. 25 (44), 10308–10320. doi:10.1523/JNEUROSCI.2697-05.2005
Hart, P. R., Wade, J. B., and Martelli, M. F. (2003). Cognitive impairment in patients with chronic pain: the significance of stress. Curr. pain headache Rep. 7, 116–126. doi:10.1007/s11916-003-0021-5
Hirschberg, S., Li, Y., Randall, A., Kremer, E. J., and Pickering, A. E. (2017). Functional dichotomy in spinal-vs prefrontal-projecting locus coeruleus modules splits descending noradrenergic analgesia from ascending aversion and anxiety in rats. Elife 6, e29808. doi:10.7554/eLife.29808
Horvitz, C. J. (2000). Mesolimbocortical and nigrostriatal dopamine responses to salient non-reward events. Neuroscience 96 (4), 651–656. doi:10.1016/s0306-4522(00)00019-1
Howe, M. W., Gritton, H. J., Lusk, N. A., Roberts, E. A., Hetrick, V. L., Berke, J. D., et al. (2017). Acetylcholine release in prefrontal cortex promotes gamma oscillations and theta–gamma coupling during cue detection. J. Neurosci. 37 (12), 3215–3230. doi:10.1523/JNEUROSCI.2737-16.2017
Huang, M.-X., Lee, R. R., Gaa, K. M., Song, T., Harrington, D. L., Loh, C., et al. (2010). Somatosensory system deficits in schizophrenia revealed by MEG during a median-nerve oddball task. Brain Topogr. 23, 82–104. doi:10.1007/s10548-009-0122-5
Huang, S., Borgland, S. L., and Zamponi, G. W. (2019b). Dopaminergic modulation of pain signals in the medial prefrontal cortex: challenges and perspectives. Neurosci. Lett. 702, 71–76. doi:10.1016/j.neulet.2018.11.043
Huang, S., Borgland, S. L., and Zamponi, G. W. (2019a). Peripheral nerve injury-induced alterations in VTA neuron firing properties. Mol. Brain 12, 89–10. doi:10.1186/s13041-019-0511-y
Huang, S., Zhang, Z., Gambeta, E., Shi, C., Thomas, C., Godfrey, N., et al. (2020). Dopamine inputs from the ventral tegmental area into the medial prefrontal cortex modulate neuropathic pain-associated behaviors in mice. Cell Rep. 31, 107812–112020. doi:10.1016/j.celrep.2020.107812
Iqbal, Z., Lei, Z., Ramkrishnan, A. S., Liu, S., Hasan, M., Akter, M., et al. (2023). Adrenergic signalling to astrocytes in anterior cingulate cortex contributes to pain-related aversive memory in rats. Commun. Biol. 6 (1), 10. doi:10.1038/s42003-022-04405-6
Ito, H., Yanase, M., Yamashita, A., Kitabatake, C., Hamada, A., Suhara, Y., et al. (2013). Analysis of sleep disorders under pain using an optogenetic tool: possible involvement of the activation of dorsal raphe nucleus-serotonergic neurons. Mol. Brain 6, 59–10. doi:10.1186/1756-6606-6-59
Jarcho, M. J., Emeran, A. M., Ziyue, K. J., Feier, N. A., and London, E. D. (2012). Pain, affective symptoms, and cognitive deficits in patients with cerebral dopamine dysfunction. PAIN® 153 (4), 744–754. doi:10.1016/j.pain.2012.01.002
Jensen, K. B., Regenbogen, C., Ohse, M. C., Frasnelli, J., Freiherr, J., and Lundström, J. N. (2016). Brain activations during pain: a neuroimaging meta-analysis of patients with pain and healthy controls. Pain 157, 1279–1286. doi:10.1097/j.pain.0000000000000517
Jiang, Y.-Y., Zhang, Yu, Cui, S., Liu, F.-Yu, Yi, M., and Wan, Y. (2018). Cholinergic neurons in medial septum maintain anxiety-like behaviors induced by chronic inflammatory pain. Neurosci. Lett. 671, 7–12. doi:10.1016/j.neulet.2018.01.041
Johansen, P. J., Fields, H. L., and Manning, B. H. (2001). The affective component of pain in rodents: direct evidence for a contribution of the anterior cingulate cortex. Proc. Natl. Acad. Sci. 98 (14), 8077–8082. doi:10.1073/pnas.141218998
Johnson, S. W., and North, R. A. (1992). Opioids excite dopamine neurons by hyperpolarization of local interneurons. J. Neurosci. 12 (2), 483–488. doi:10.1523/JNEUROSCI.12-02-00483.1992
Juarez-Salinas, , Dina, L., Braz, J. M., Alexander, E., Gee, S., Sohal, V., et al. (2019). GABAergic cell transplants in the anterior cingulate cortex reduce neuropathic pain aversiveness. Brain 142 (9), 2655–2669. doi:10.1093/brain/awz203
Kamiński, J., Sullivan, S., Chung, J. M., Ross, I. B., Mamelak, A. N., and Rutishauser, U. (2017). Persistently active neurons in human medial frontal and medial temporal lobe support working memory. Nat. Neurosci. 20, 590–601. doi:10.1038/nn.4509
Kang, S. J., Kwak, C., Lee, J., Sim, S.-E., Shim, J., Choi, T., et al. (2015). Bidirectional modulation of hyperalgesia via the specific control of excitatory and inhibitory neuronal activity in the ACC. Mol. Brain 8, 81. doi:10.1186/s13041-015-0170-6
Kasahara, S., Niwa, S.-I., Ko, M., Sato, N., Oka, H., and Yamada, Y. (2020). Attention-deficit/hyperactivity disorder and chronic pain. Psychosom. Med. 82 (3), 346–347. doi:10.1097/PSY.0000000000000789
Kasanetz, F., Acuña, M. A., and Nevian, T. (2022). “Anterior cingulate cortex, pain perception, and pathological neuronal plasticity during chronic pain,” in The neurobiology, physiology, and psychology of pain (Cambridge, Massachusetts, United States: Academic Press), 193–202.
Kaushal, R., Taylor, B. K., Jamal, A. B., Zhang, L., Ma, F., Donahue, R., et al. (2016). GABA-A receptor activity in the noradrenergic locus coeruleus drives trigeminal neuropathic pain in the rat; contribution of NAα1 receptors in the medial prefrontal cortex. Neuroscience 334, 148–159. doi:10.1016/j.neuroscience.2016.08.005
Kind, S., and Otis, J. D. (2019). The interaction between chronic pain and PTSD. Curr. pain headache Rep. 23, 91–97. doi:10.1007/s11916-019-0828-3
Ko, M.Yi, Eun, Y. J., June, Y. L., Soo, P. K., Sung, H. W., Lee, B. H., Kim, H. Y., Yang, C.Ha, Cho, H. J., et al. (2018). The role of ventral tegmental area gamma-aminobutyric acid in chronic neuropathic pain after spinal cord injury in rats. J. Neurotrauma 35, 1755–1764. doi:10.1089/neu.2017.5381
Koga, K., Yamada, A., Song, Q., Li, X.-H., Chen, Q.-Yu, Liu, R.-H., et al. (2020). Ascending noradrenergic excitation from the locus coeruleus to the anterior cingulate cortex. Mol. Brain 13, 49–14. doi:10.1186/s13041-020-00586-5
Koga, K., Yu, M., Honda, K., Eto, F., Furukawa, T., Migita, K., et al. (2017). Activations of muscarinic M1 receptors in the anterior cingulate cortex contribute to the antinociceptive effect via GABAergic transmission. Mol. pain 13, 1744806917692330. doi:10.1177/1744806917692330
Kole, H. P., Maarten, S., Kampa, B. M., Williams, S. R., Ruben, P. C., and Stuart, G. J. (2008). Action potential generation requires a high sodium channel density in the axon initial segment. Nat. Neurosci. 11 (2), 178–186. doi:10.1038/nn2040
Kröner, S., Krimer, L. S., Lewis, D. A., and Barrionuevo, G. (2007). Dopamine increases inhibition in the monkey dorsolateral prefrontal cortex through cell type–specific modulation of interneurons. Cereb. Cortex 17 (5), 1020–1032. doi:10.1093/cercor/bhl012
Kummer, K. K., Mitrić, M., Kalpachidou, T., and Kress, M. (2020). The medial prefrontal cortex as a central hub for mental comorbidities associated with chronic pain. Int. J. Mol. Sci. 21 (10), 3440. doi:10.3390/ijms21103440
Kuner, R., and Flor, H. (2017). Structural plasticity and reorganisation in chronic pain. Nat. Rev. Neurosci. 18, 113–130. doi:10.1038/nrn.2017.5
Kuner, R., and Tan, K. (2020). Cellular circuits in the brain and their modulation in acute and chronic pain. Physiol. Rev. 101, 213–258. doi:10.1152/physrev.00040.2019
Lacroix, P. L., Hows, M. E. P., Shah, J., Hagan, J. J., and Heidbreder, C. A. (2003). Selective antagonism at dopamine D3 receptors enhances monoaminergic and cholinergic neurotransmission in the rat anterior cingulate cortex. Neuropsychopharmacology 28 (5), 839–849. doi:10.1038/sj.npp.1300114
Lançon, K., Qu, C., Navratilova, E., Frank, P., and Philippe, S. (2021). Decreased dopaminergic inhibition of pyramidal neurons in anterior cingulate cortex maintains chronic neuropathic pain. Cell Rep. 37, 109933. doi:10.1016/j.celrep.2021.109933
Le Moine, C., and Gaspar, P. (1998). Subpopulations of cortical GABAergic interneurons differ by their expression of D1 and D2 dopamine receptor subtypes. Mol. Brain Res. 58 (1-2), 231–236. doi:10.1016/s0169-328x(98)00118-1
Li, A., Liu, Y., Zhang, Q., Friesner, I., Jung Jee, H., Chen, Z. S., et al. (2021a). Disrupted population coding in the prefrontal cortex underlies pain aversion. Cell Rep. 37, 109978–109986. doi:10.1016/j.celrep.2021.109978
Li, X.-H., Matsuura, T., Xue, M., Chen, Q.-Yu, Liu, R.-H., Lu, J.-S., et al. (2021b). Oxytocin in the anterior cingulate cortex attenuates neuropathic pain and emotional anxiety by inhibiting presynaptic long-term potentiation. Cell Rep. 36, 109411–109413. doi:10.1016/j.celrep.2021.109411
Liu, C., Cai, X., Ritzau-Jost, A., Kramer, P. F., Li, Y., Khaliq, Z. M., et al. (2022). An action potential initiation mechanism in distal axons for the control of dopamine release. Science 375 (6587), 1378–1385. doi:10.1126/science.abn0532
Liu, S. S., Pickens, S., Nicole, E., Ibarra-Lecue, I., Yang, H., Xue, L., et al. (2019). Kappa opioid receptors drive a tonic aversive component of chronic pain. J. Neurosci. 39 (21), 4162–4178. doi:10.1523/JNEUROSCI.0274-19.2019
Loggia, L. M., Berna, C., Kim, J., Cahalan, C. M., Gollub, R. L., Wasan, A. D., et al. (2014). Disrupted brain circuitry for pain-related reward/punishment in fibromyalgia. Arthritis & rheumatology 66 (1), 203–212. doi:10.1002/art.38191
López-Avila, A., Coffeen, U., Manuel Ortega-Legaspi, J., Del Ángel, R., and Pellicer, F. (2004). Dopamine and NMDA systems modulate long-term nociception in the rat anterior cingulate cortex. Pain 111 (1-2), 136–143. doi:10.1016/j.pain.2004.06.010
Lu, C., Yang, T., Zhao, H., Zhang, M., Meng, F., Fu, H., et al. (2016). Insular cortex is critical for the perception, modulation, and chronification of pain. Neurosci. Bull. 32, 191–201. doi:10.1007/s12264-016-0016-y
Markovic, T., Pedersen, C. E., Massaly, N., Vachez, Y. M., Ruyle, B., Murphy, C. A., et al. (2021). Pain induces adaptations in ventral tegmental area dopamine neurons to drive anhedonia-like behavior. Nat. Neurosci. 24 (11), 1601–1613. doi:10.1038/s41593-021-00924-3
Martikainen, K. I., Emily B, N., Marta, P., Love, T. M., Cummiford, C. M., Carmen, R. G., et al. (2015). Chronic back pain is associated with alterations in dopamine neurotransmission in the ventral striatum. J. Neurosci. 35 (27), 9957–9965. doi:10.1523/JNEUROSCI.4605-14.2015
Mehler-Wex, C., Riederer, P., and Gerlach, M. (2006). Dopaminergic dysbalance in distinct basal ganglia neurocircuits: implications for the pathophysiology of Parkinson’s disease, schizophrenia and attention deficit hyperactivity disorder. Neurotox. Res. 10, 167–179. doi:10.1007/BF03033354
Mercer, N. L., Chen, C., Gilam, G., Mackey, S., and Grégory Scherrer, (2021). Brain circuits for pain and its treatment. Sci. Transl. Med. 13, 619. doi:10.1126/scitranslmed.abj7360
Miller, L. R., and Cano, A. (2009). Comorbid chronic pain and depression: who is at risk? J. pain 10 (6), 619–627. doi:10.1016/j.jpain.2008.12.007
Muly, C. E., Szigeti, K., and Goldman-Rakic, P. S. (1998). D1 receptor in interneurons of macaque prefrontal cortex: distribution and subcellular localization. J. Neurosci. 18 (24), 10553–10565. doi:10.1523/JNEUROSCI.18-24-10553.1998
Nahin, L. R., Feinberg, T., Kapos, F. P., and Terman, G. W. (2023). Estimated rates of incident and persistent chronic pain among US adults. JAMA Netw. Open 6 (5), e2313563. doi:10.1001/jamanetworkopen.2023.13563
Narita, M., Funada, M., and Suzuki, T. (2001). Regulations of opioid dependence by opioid receptor types. Pharmacol. Ther. 89, 1–15. doi:10.1016/S0163-7258(00)00099-1
Narita, M., Ozaki, S., Narita, M., Ise, Y., Yajima, Y., and Suzuki, T. (2003). Change in the expression of c-fos in the rat brain following sciatic nerve ligation. Neurosci. Lett. 352 (3), 231–233. doi:10.1016/j.neulet.2003.08.052
Navratilova, E., Qu, C., Ji, G., Neugebauer, V., Guerrero, M., Rosen, H., et al. (2023). Opposing effects on descending control of nociception by mu and kappa opioid receptors in the anterior cingulate cortex. Anesthesiology. doi:10.1097/ALN.0000000000004773
O'Hearn, E., and Molliver, M. E. (1984). Organization of raphe-cortical projections in rat: a quantitative retrograde study. Brain Res. Bull. 13 (6), 709–726. doi:10.1016/0361-9230(84)90232-6
Ong, W.-Yi, Stohler, C. S., and Herr, D. R. (2019). Role of the prefrontal cortex in pain processing. Mol. Neurobiol. 56, 1137–1166. doi:10.1007/s12035-018-1130-9
Ortega-Legaspi, J. M., de Gortari, P., Garduño-Gutiérrez, R., Isabel Amaya, M., León-Olea, M., Coffeen, U., et al. (2011). Expression of the dopaminergic D1 and D2 receptors in the anterior cingulate cortex in a model of neuropathic pain. Mol. Pain 7, 97. doi:10.1186/1744-8069-7-97
Ortega-Legaspi, J. M., López-Avila, A., Coffeen, U., del Angel, R., and Pellicer, F. (2003). Scopolamine into the anterior cingulate cortex diminishes nociception in a neuropathic pain model in the rat: an interruption of ‘nociception-related memory acquisition’? Eur. J. Pain 7 (5), 425–429. doi:10.1016/s1090-3801(02)00147-7
Oswald, M. J., Yechao, H., Han, Li, Samuel, M., Deniz Nouri, O., Bhavya, O., et al. (2022). Cholinergic basal forebrain nucleus of Meynert regulates chronic pain-like behavior via modulation of the prelimbic cortex. Nat. Commun. 13 (1), 5014. doi:10.1038/s41467-022-32558-9
Ozaki, S., Narita, M., Narita, M., Iino, M., Sugita, J., Matsumura, Y., et al. (2002). Suppression of the morphine-induced rewarding effect in the rat with neuropathic pain: implication of the reduction in µ-opioid receptor functions in the ventral tegmental area. J. Neurochem. 82 (5), 1192–1198. doi:10.1046/j.1471-4159.2002.01071.x
Palmisano, M., Caputi, F. F., Mercatelli, D., Romualdi, P., and Candeletti, S. (2019). Dynorphinergic system alterations in the corticostriatal circuitry of neuropathic mice support its role in the negative affective component of pain. Genes, Brain Behav. 18 (6), e12467. doi:10.1111/gbb.12467
Paget-Blanc, V., Pfeffer, M. E., Marie, P., Paul, L., Maria-Florencia, A., Roman, W., et al. (2022). A synaptomic analysis reveals dopamine hub synapses in the mouse striatum. Nat. Commun. 13 (1), 3102. doi:10.1038/s41467-022-30776-9
Paspalas, C. D., Wang, M., and Amy, F. T. (2012). Constellation of HCN channels and cAMP regulating proteins in dendritic spines of the primate prefrontal cortex: potential substrate for working memory deficits in schizophrenia. Cereb. Cortex 23, 1643–1654. doi:10.1093/cercor/bhs152
Passetti, F., Dalley, J. W., O'Connell, M. T., Everitt, B. J., and Robbins, T. W. (2000). Increased acetylcholine release in the rat medial prefrontal cortex during performance of a visual attentional task. Eur. J. Neurosci. 12 (8), 3051–3058. doi:10.1046/j.1460-9568.2000.00183.x
Phelps, C. E., Navratilova, E., and Frank, P. (2021). Cognition in the chronic pain experience: preclinical insights. Trends Cognitive Sci. 25 (5), 365–376. doi:10.1016/j.tics.2021.01.001
Pittolo, S., Yokoyama, S., Willoughby, D. D., Taylor, C. R., Reitman, M. E., Tse, V., et al. (2022). Dopamine activates astrocytes in prefrontal cortex via α1-adrenergic receptors. Cell Rep. 40 (13), 111426. doi:10.1016/j.celrep.2022.111426
Poorthuis, R. B., Bernard Bloem, , Schak, B., Wester, J., Pj de Kock, C., and HuibertMansvelder, D. (2013). Layer-specific modulation of the prefrontal cortex by nicotinic acetylcholine receptors. Cereb. Cortex 23 (1), 148–161. doi:10.1093/cercor/bhr390
Porro, C. A., Cavazzuti, M., Galetti, A., and Sassatelli, L. (1991). Functional activity mapping of the rat brainstem during formalin-induced noxious stimulation. Neuroscience 41 (2-3), 667–680. doi:10.1016/0306-4522(91)90358-u
Porter, L. L., Rizzo, E., and Hornung, J.-P. (1999). Dopamine affects parvalbumin expression during cortical development in vitro. J. Neurosci. 19 (20), 8990–9003. doi:10.1523/JNEUROSCI.19-20-08990.1999
Poulin, J.-F., Caronia, G., Hofer, C., Cui, Q., Helm, B., Ramakrishnan, C., et al. (2018). Mapping projections of molecularly defined dopamine neuron subtypes using intersectional genetic approaches. Nat. Neurosci. 21 (9), 1260–1271. doi:10.1038/s41593-018-0203-4
Povedano, M., Gascón, J., Gálvez, R., Ruiz, M., and Rejas, J. (2007). Cognitive function impairment in patients with neuropathic pain under standard conditions of care. J. Pain Symptom Manag. 33 (1), 78–89. doi:10.1016/j.jpainsymman.2006.07.012
Puig, M. V., Noemí, S., Celada, P., Guadalupe, M., and Francesc, A. (2004). In vivo excitation of GABA interneurons in the medial prefrontal cortex through 5-HT3 receptors. Cereb. Cortex 14 (12), 1365–1375. doi:10.1093/cercor/bhh097
Puig, M. V., Watakabe, A., Ushimaru, M., Yamamori, T., and Kawaguchi, Y. (2010). Serotonin modulates fast-spiking interneuron and synchronous activity in the rat prefrontal cortex through 5-HT1A and 5-HT2A receptors. J. Neurosci. 30 (6), 2211–2222. doi:10.1523/JNEUROSCI.3335-09.2010
Qi, J., Zhang, S., Wang, H.-L., Barker, D. J., Miranda-Barrientos, J., and Morales, M. (2016). VTA glutamatergic inputs to nucleus accumbens drive aversion by acting on GABAergic interneurons. Nat. Neurosci. 19 (5), 725–733. doi:10.1038/nn.4281
Rainville, P., Duncan, G. H., Price, D. D., Carrier, B., and Catherine Bushnell, M. (1997). Pain affect encoded in human anterior cingulate but not somatosensory cortex. Science 277 (5328), 968–971. doi:10.1126/science.277.5328.968
Reckziegel, D., Pascal, T., Ghantous, M., Wakaizumi, K., Petre, B., Huang, L., et al. (2019). Gender-dependent pharmacotherapy for blocking the transition to chronic back pain: a proof of concept randomized trial. https://www.medrxiv.org/content/10.1101/19006627v1.
Ren, S.-Q., Wen, Y., Yan, J.-Z., Jin, C., Yin, J.-J., Yuan, J., et al. (2018). Amyloid β causes excitation/inhibition imbalance through dopamine receptor 1-dependent disruption of fast-spiking GABAergic input in anterior cingulate cortex. Sci. Rep. 8 (1), 302. doi:10.1038/s41598-017-18729-5
Romanos, J., Benke, D., Pietrobon, D., Ulrich Zeilhofer, H., and Santello, M. (2020). Astrocyte dysfunction increases cortical dendritic excitability and promotes cranial pain in familial migraine. Sci. Adv. 6 (23), eaaz1584. doi:10.1126/sciadv.aaz1584
Rudy, T. E., Kerns, R. D., and Turk, D. C. (1988). Chronic pain and depression: toward a cognitive-behavioral mediation model. Pain 35 (2), 129–140. doi:10.1016/0304-3959(88)90220-5
Sanders, K. H., Klein, C. E., Mayer, T. E., Heym, C. H., and Handwerker, H. O. (1980). Differential effects of noxious and non-noxious input on neurones according to location in ventral periaqueductal grey or dorsal raphe nucleus. Brain Res. 186 (1), 83–97. doi:10.1016/0006-8993(80)90257-7
Santana, N., Bortolozzi, A., Serrats, J., Mengod, G., and Artigas, F. (2004). Expression of serotonin1A and serotonin2A receptors in pyramidal and GABAergic neurons of the rat prefrontal cortex. Cereb. Cortex 14 (10), 1100–1109. doi:10.1093/cercor/bhh070
Santana, N., Mengod, G., and Artigas, F. (2013). Expression of α(1)-adrenergic receptors in rat prefrontal cortex: cellular co-localization with 5-HT(2A) receptors. Int. J. Neuropsychopharmacol. 16 (5), 1139–1151. doi:10.1017/S1461145712001083
Santana, N., Mengod, G., and Artigas, F. (2009). Quantitative analysis of the expression of dopamine D1 and D2 receptors in pyramidal and GABAergic neurons of the rat prefrontal cortex. Cereb. Cortex 19 (4), 849–860. doi:10.1093/cercor/bhn134
Santello, M., Bisco, A., Natalie Elisabeth, N., Lacivita, E., Leopoldo, M., and Nevian, T. (2017). The brain-penetrant 5-HT7 receptor agonist LP-211 reduces the sensory and affective components of neuropathic pain. Neurobiol. Dis. 106, 214–221. doi:10.1016/j.nbd.2017.07.005
Santello, M., and Nevian, T. (2015). Dysfunction of cortical dendritic integration in neuropathic pain reversed by serotoninergic neuromodulation. Neuron 86 (1), 233–246. doi:10.1016/j.neuron.2015.03.003
Sarinana, J., and Tonegawa, S. (2016). Differentiation of forebrain and hippocampal dopamine 1-class receptors, D 1 R and D 5 R, in spatial learning and memory. Hippocampus 26 (1), 76–86. doi:10.1002/hipo.22492
Sarter, M., and Markowitsch, H. J. (1984). Collateral innervation of the medial and lateral prefrontal cortex by amygdaloid, thalamic, and brain-stem neurons. J. Comp. Neurology 224 (3), 445–460. doi:10.1002/cne.902240312
Satoh, H., Suzuki, H., and Saitow, F. (2018). Downregulation of dopamine D1-like receptor pathways of GABAergic interneurons in the anterior cingulate cortex of spontaneously hypertensive rats. Neuroscience 394, 267–285. doi:10.1016/j.neuroscience.2018.10.039
Schestatsky, P., Kumru, H., Valls-Sole, J., Valldeoriola, F., Marti, M. J., Tolosa, E., et al. (2007). Neurophysiologic study of central pain in patients with Parkinson disease. Neurology 69, 2162–2169. doi:10.1212/01.wnl.0000295669.12443.d3
Schiltenwolf, M., Akbar, M., Hug, A., Pfuller, U., Gantz, S., Neubauer, E., et al. (2014). Evidence of specific cognitive deficits in patients with chronic low back pain under long-term substitution treatment of opioids. Pain physician 17 (1), 9–20. doi:10.36076/ppj.2014/17/9
Schorscher-Petcu, A., Sotocinal, S., Ciura, S., Dupré, A., Ritchie, J., Sorge, R. E., et al. (2010). Oxytocin-induced analgesia and scratching are mediated by the vasopressin-1A receptor in the mouse. J. Neurosci. 30 (24), 8274–8284. doi:10.1523/JNEUROSCI.1594-10.2010
Seamans, J. K., Gorelova, N., Durstewitz, D., and Yang, C. R. (2001). Bidirectional dopamine modulation of GABAergic inhibition in prefrontal cortical pyramidal neurons. J. Neurosci. 21 (10), 3628–3638. doi:10.1523/JNEUROSCI.21-10-03628.2001
Sellmeijer, J., Mathis, V., Hugel, S., Li, X.-H., Song, Q., Chen, Q.-Yu, et al. (2018). Hyperactivity of anterior cingulate cortex areas 24a/24b drives chronic pain-induced anxiodepressive-like consequences. J. Neurosci. 38, 3102–3115. doi:10.1523/JNEUROSCI.3195-17.2018
Shem, K., Barncord, S., Kara, F., and Mohan, M. (2018). Adverse cognitive effect of gabapentin in individuals with spinal cord injury: preliminary findings. Spinal Cord Ser. Cases 4 (1), 9. doi:10.1038/s41394-018-0038-y
Shirey, J. K., Brady, A. E., Jones, P. J., Davis, A. A., Bridges, T. M., Kennedy, J. P., et al. (2009). A selective allosteric potentiator of the M1 muscarinic acetylcholine receptor increases activity of medial prefrontal cortical neurons and restores impairments in reversal learning. J. Neurosci. 29 (45), 14271–14286. doi:10.1523/JNEUROSCI.3930-09.2009
Singh, A., Patel, D., Li, A., Hu, L., Zhang, Q., Liu, Y., et al. (2020). Mapping cortical integration of sensory and affective pain pathways. Curr. Biol. 30 (9), 1703–1715. doi:10.1016/j.cub.2020.02.091
Speed, T. J., Parekh, V., Coe, W., and Antoine, D. (2018). Comorbid chronic pain and opioid use disorder: literature review and potential treatment innovations. Int. Rev. Psychiatry 30 (5), 136–146. doi:10.1080/09540261.2018.1514369
Stahl, S. M. (2018). Beyond the dopamine hypothesis of schizophrenia to three neural networks of psychosis: dopamine, serotonin, and glutamate. CNS spectrums 23 (3), 187–191. doi:10.1017/S1092852918001013
Stevens, F. L., Hurley, R. A., and Taber, K. H. (2011). Anterior cingulate cortex: unique role in cognition and emotion. J. Neuropsychiatry Clin. Neurosci. 23 (2), 121–125. doi:10.1176/jnp.23.2.jnp121
Tan, L. L., and Kuner, R. (2021). Neocortical circuits in pain and pain relief. Nat. Rev. Neurosci. 22 (8), 458–471. doi:10.1038/s41583-021-00468-2
Tanaka, E. I. I. C. H. I. R. O., and Alan North, R. (1993). Actions of 5-hydroxytryptamine on neurons of the rat cingulate cortex. J. Neurophysiology 69 (5), 1749–1757. doi:10.1152/jn.1993.69.5.1749
Tian, Z., Yamanaka, M., Bernabucci, M., Zhao, M.-gao, and Zhuo, M. (2017). Characterization of serotonin-induced inhibition of excitatory synaptic transmission in the anterior cingulate cortex. Mol. Brain 10 (1), 21–10. doi:10.1186/s13041-017-0303-1
Vander Weele, C. M., Siciliano, C. A., Matthews, G. A., Namburi, P., Izadmehr, E. M., Espinel, I. C., et al. (2018). Dopamine enhances signal-to-noise ratio in cortical-brainstem encoding of aversive stimuli. Nature 563 (7731), 397–401. doi:10.1038/s41586-018-0682-1
Varrassi, G., Müller-Schwefe, G., Joseph, P., Orónska, A., Morlion, B., Mavrocordatos, P., et al. (2010). Pharmacological treatment of chronic pain–the need for CHANGE. Curr. Med. Res. Opin. 26 (5), 1231–1245. doi:10.1185/03007991003689175
Vidal, C., and Changeux, J.-P. (1989). Pharmacological profile of nicotinic acetylcholine receptors in the rat prefrontal cortex: an electrophysiological study in a slice preparation. Neuroscience 29 (2), 261–270. doi:10.1016/0306-4522(89)90056-0
Vijayraghavan, S., Wang, M., Birnbaum, S. G., Williams, G. V., and Arnsten, A. F. T. (2007). Inverted-U dopamine D1 receptor actions on prefrontal neurons engaged in working memory. Nat. Neurosci. 10 (3), 376–384. doi:10.1038/nn1846
Wang, G.-Q., Cheng, C., Li, C., Cao, S., Wang, N., Zheng, Z., et al. (2015). Deactivation of excitatory neurons in the prelimbic cortex via Cdk5 promotes pain sensation and anxiety. Nat. Commun. 6 (1), 7660. doi:10.1038/ncomms8660
Wang, M., Ramos, B. P., Paspalas, C. D., Shu, Y., Arthur, S., Duque, A., et al. (2007). Alpha2A-adrenoceptors strengthen working memory networks by inhibiting cAMP-HCN channel signaling in prefrontal cortex. Cell 129 (2), 397–410. doi:10.1016/j.cell.2007.03.015
Wang, X.-Y., Jia, W.-B., Xu, X., Chen, R., Wang, L.-B., Su, X.-J., et al. (2023). A glutamatergic DRN–VTA pathway modulates neuropathic pain and comorbid anhedonia-like behavior in mice. Nat. Commun. 14 (1), 5124. doi:10.1038/s41467-023-40860-3
Waselus, M., Valentino, R. J., and Van Bockstaele, E. J. (2011). Collateralized dorsal raphe nucleus projections: a mechanism for the integration of diverse functions during stress. J. Chem. Neuroanat. 41 (4), 266–280. doi:10.1016/j.jchemneu.2011.05.011
Weinrich, J. A. P., Liu, C. D., Jewell, M. E., Andolina, C. R., Bernstein, M. X., Benitez, J., et al. (2023). Paradoxical increases in anterior cingulate cortex activity during nitrous oxide-induced analgesia reveal a signature of pain affect. bioRxiv, 2023.04.03.534475–04. doi:10.1101/2023.04.03.534475
Xi, Z.-X., Fuller, S. A., and Stein, E. A. (1998). Dopamine release in the nucleus accumbens during heroin self-administration is modulated by kappa opioid receptors: an in vivo fast-cyclic voltammetry study. J. Pharmacol. Exp. Ther. 284 (1), 151–161.
Xie, L., Wu, H., Chen, Q., Xu, F., Li, H., Qi, Xu, et al. (2022). Divergent modulation of pain and anxiety by GABAergic neurons in the ventrolateral periaqueductal gray and dorsal raphe. Neuropsychopharmacology 48, 1509–1519. doi:10.1038/s41386-022-01520-0
Yan, Z. (2002). Regulation of GABAergic inhibition by serotonin signaling in prefrontal cortex: molecular mechanisms and functional implications. Mol. Neurobiol. 26, 203–216. doi:10.1385/MN:26:2-3:203
Yang, H., Johannes, W. de J., Cerniauskas, I., Peck, J. R., Lim, B. K., Gong, H., et al. (2021). Pain modulates dopamine neurons via a spinal–parabrachial–mesencephalic circuit. Nat. Neurosci. 24 (10), 1402–1413. doi:10.1038/s41593-021-00903-8
Yang, S., Boudier-Revéret, M., Jin, C., and Chang, M. C. (2020). Association between chronic pain and alterations in the mesolimbic dopaminergic system. Brain Sci. 10 (10), 701. doi:10.3390/brainsci10100701
Yoest, K. E., Quigley, J. A., and Becker, J. B. (2018). Rapid effects of ovarian hormones in dorsal striatum and nucleus accumbens. Hormones Behav. 104, 119–129. doi:10.1016/j.yhbeh.2018.04.002
Yousefpour, N., Locke, S., Deamond, H., Wang, C., Marques, L., St-Louis, M., et al. (2023). Time-dependent and selective microglia-mediated removal of spinal synapses in neuropathic pain. Cell Rep. 42 (1), 112010. doi:10.1016/j.celrep.2023.112010
Zhang, Z., Matos, S. C., Jego, S., Antoine, A., and Philippe, S. (2013). Norepinephrine drives persistent activity in prefrontal cortex via synergistic α1 and α2 adrenoceptors. PloS One 8 (6), e66122. doi:10.1371/journal.pone.0066122
Zhang, Z., and Philippe, S. (2010). Metabotropic induction of persistent activity in layers II/III of anterior cingulate cortex. Cereb. Cortex 20 (12), 2948–2957. doi:10.1093/cercor/bhq043
Zhou, F.-M., and Hablitz, J. J. (1999). Activation of serotonin receptors modulates synaptic transmission in rat cerebral cortex. J. Neurophysiology 82 (6), 2989–2999. doi:10.1152/jn.1999.82.6.2989
Zhou, S., Chen, X., Wang, C., Yin, C., Hu, P., and Wang, K. (2012). Selective attention deficits in early and moderate stage Parkinson’s disease. Neurosci. Lett. 509 (1), 50–55. doi:10.1016/j.neulet.2011.12.049
Zhuo, M. (2020). Cortical plasticity as synaptic mechanism for chronic pain. J. Neural Transm. 127 (4), 567–573. doi:10.1007/s00702-019-02071-3
Keywords: chronic pain, anterior cingulate cortex, analgesia, dopamine, norepinephrine, serotonin, acetylcholine, monoamines
Citation: Lançon K and Séguéla P (2023) Dysregulated neuromodulation in the anterior cingulate cortex in chronic pain. Front. Pharmacol. 14:1289218. doi: 10.3389/fphar.2023.1289218
Received: 05 September 2023; Accepted: 09 October 2023;
Published: 25 October 2023.
Edited by:
Serena Boccella, University of Campania Luigi Vanvitelli, ItalyReviewed by:
Javier Cuitavi, University of Valencia, SpainDaniel C. Castro, Washington University in St. Louis, United States
Copyright © 2023 Lançon and Séguéla. This is an open-access article distributed under the terms of the Creative Commons Attribution License (CC BY). The use, distribution or reproduction in other forums is permitted, provided the original author(s) and the copyright owner(s) are credited and that the original publication in this journal is cited, in accordance with accepted academic practice. No use, distribution or reproduction is permitted which does not comply with these terms.
*Correspondence: Philippe Séguéla, philippe.seguela@mcgill.ca