- 1State Key Laboratory of Dampness Syndrome of Chinese Medicine, The Second Affiliated Hospital of Guangzhou University of Chinese Medicine, Guangzhou, China
- 2State Key Laboratory of Integration and Innovation of Classical Formula and Modern Chinese Medicines, National Chinmedomics Research Center, Heilongjiang University of Chinese Medicine, Harbin, China
Gout represents a metabolic ailment resulting from the accumulation of monosodium urate crystals within joints, causing both inflammation and, harm to tissues. The primary contributor to gout’s emergence is an elevated presence of serum urate, which is under the regulation of kidney and, gut urate transporters. Mitigating this risk factor is crucial for averting gout’s onset. Several treatments rooted in TCM and related active compounds have demonstrated efficacy in managing gout, skillfully regulating serum uric acid (UA) levels and curbing inflammation’s progression. This analysis compiles key foundational research concerning the molecular signaling pathways and UA transporters linked to gout, under the regulation of TCM. The focus includes individual botanical drug, active compounds, and TCM formulations, which have been consolidated and examined in this overview. The primary keywords chosen were “gout, hyperuricemia, gouty arthritis, traditional Chinese medicine, Chinese botanical drug, medicinal botanical drug, and natural plant”. Various relevant literature published within the last 5 years were gathered from electronic databases, including PubMed, Web of Science, CNKI, and others. The findings revealed that TCM has the capacity to modulate various signaling pathways, including MAPK, NF-κB, PI3K/Akt, NLRP3 and JAK/STAT. Additionally, it impacts UA transporters like URAT1, GLUT9, ABCG2, as well as OATs and OCTs, thereby contributing to gout treatment. TCM helps maintain a balanced inflammatory interaction and facilitates UA excretion. This study enhances our understanding of TCM’s anti-gout mechanisms and introduces novel perspectives for establishing the clinical significance and future prospects of TCM-based gout treatment.
1 Introduction
Gout represents a painful type of arthritis triggered by the accumulation of monosodium urate (MSU) crystals within synovial fluid and other bodily tissues in people with hyperuricaemia. Intermittent flaring condition of gout often bring along unforeseeable discomfort, swelling of joints, reddening, and damage to joints as a result of recurrent flare-ups (Richette et al., 2020). The enhancement of people’s material living conditions and the escalating consumption of purine-rich foods have driven a year-by-year surge in the prevalence of gout. At present, many the global populace grapples with gout, transforming it into a significant quandary for public health, inflicting immense anguish and imposing substantial financial burdens on sufferers (Shields and Beard, 2015). Currently, the acute manifestation of gout has been addressed through the utilization of corticosteroids, colchicines, and non-steroidal anti-inflammatory medications (Qaseem et al., 2017). However, these accessible treatment options merely mitigate symptoms or delay the onset of gout, and even entail significant adverse effects, such as gastrointestinal responses, skin eruptions, systemic gaps, and the potential for renal dysfunction (Perez-Ruiz et al., 2015). Therefore, the extended management of gout necessitates the swift development of novel and more dependable therapeutic strategies.
Gout presents a multifaceted pathological sequence, encompassing diverse underlying factors. These include urate accumulation (Maiuolo et al., 2016), hindrances to uric acid elimination (Méndez-Salazar and Martínez-Nava, 2022), and inflammatory response triggered by deposited urate (Shin et al., 2019), as well as the interactions among these factors (Merriman, 2015; Major et al., 2018). UA forms as a byproduct of human purine metabolism. Purines undergo a metabolic process leading from hypoxanthine to xanthine through the action of xanthine dehydrogenase, and finally to UA. Furthermore, excessive purine intake arises from the consumption of items rich in purine, including foods abundant in both purine and high sugar or alcohol content. These dietary choices prompt the assimilation of substantial adenosine triphosphate (ATP), resulting in the generation of adenosine diphosphate (ADP) and adenosine monophosphate (AMP). This, in turn, elevates AMP deaminase activity, facilitating the conversion of AMP into inosine monophosphate (IMP), thus expediting UA production (Li et al., 2020c; Zhang et al., 2020). On a different note, the excretion of UA is under the regulation of both the renal and intestinal systems. Inadequate UA elimination gives rise to an elevation in serum UA levels (Li et al., 2023b). The kidney is responsible for excreting UA through a process involving glomerular filtration, proximal tubule reabsorption, as well as secretion and subsequent reabsorption (García-Arroyo et al., 2018). The proper uptake and release of UA rely on transport proteins located in the apical and basolateral membranes of the epithelial cells in the proximal tubules. These proteins could potentially give rise to irregularities, culminating in anomalous UA elimination and consequently elevating the concentration of UA in the bloodstream (Joosten et al., 2020). Moreover, UA holds the capacity to trigger numerous intracellular signaling pathways, culminating in the generation of inflammatory cytokines (Zhang et al., 2021a; Cobo et al., 2022; Zaninelli et al., 2022). This, in turn, culminates in an inflammatory response and tissue deterioration, where inflammatory cells and factors synergistically incite each other, contributing to the progression of gout (Zhang et al., 2021c; Zhu et al., 2021). Collectively, these consequences of urate are believed to underlie a significant portion of the physiological implications of hyperuricemia and gout, highlighting that this biologically active molecule surpasses its mere status as a ‘byproduct’ of purine metabolism (Figure 1).
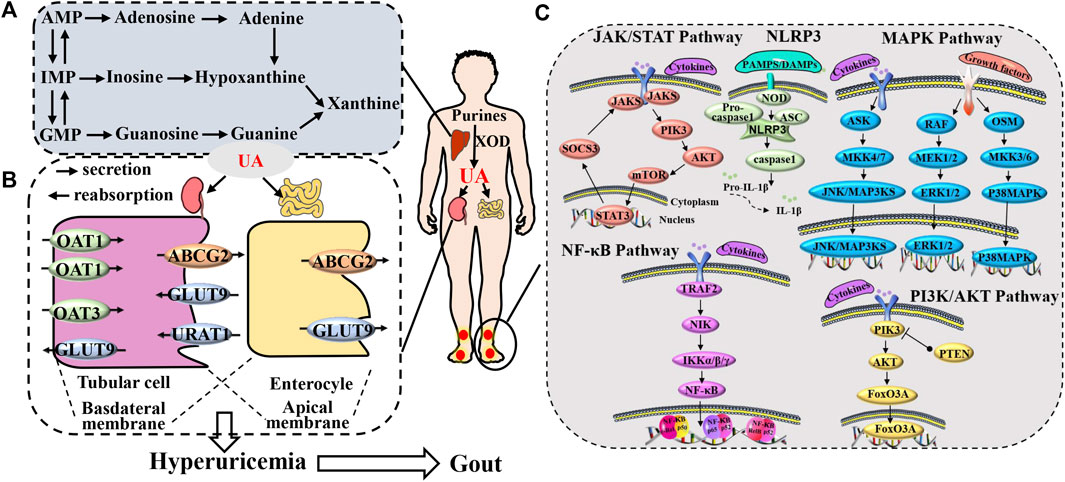
FIGURE 1. Gout pathogenesis. (A) The production process of uric acid. (B) process of uric acid excretion mediated by uric acid transporters and (C) the gout inflammation.
TCM boasts a legacy of millennia in China (Zhang et al., 2018a). A wealth of clinical and laboratory research endeavors have been undertaken to validate the efficacy of TCM in addressing gout (Xiao et al., 2018; Liu et al., 2021; Xiao et al., 2021). Certain Chinese medicinal botanical drugs or TCM formulations demonstrate multifaceted targeting and low toxicity. As a result, they present favorable characteristics and hold promising potential for both preventing and treating gout (Table 1). For instance, extracts like Phellodendron chinense C.K.Schneid. (Lin et al., 2021), Coptis chinensis Franch. (Li et al., 2021a), Atractylodes lancea (Thunb.) DC. (Fu et al., 2021), Achyranthes bidentata Blume. (Wu et al., 2018), Smilax glabra Roxb. (Liang et al., 2019), Eucommia ulmoides Oliv. (Fang et al., 2019), Ermiao Wan (Gu et al., 2023), Simiao Wan (Lin et al., 2020a), Sanmiao Wan (Wu et al., 2018), Wuling San (Huang et al., 2023) and Erding Formula (Zuo et al., 2018) demonstrated efficacy in enhancing gout-induced pathological damage and reducing inflammatory responses.
In clinical practice, the core strategy for effective treatment can be considered as two aspects in early-onset and intermittent of gout: 1) in early-onset of gout: urate-lowering therapy to hyperuricemia, which relate to dissolute MSU crystals and prevent long-term of gout flares; and 2) in intermittent of gout: anti-inflammatory therapy to intermittent episodes of severely painful arthritis (gout flares) caused by deposited MSU crystals (Keller and Mandell, 2021). Thus, this review encompasses two distinct research domains: the present investigations into TCM’s impact on anti-gout molecular signaling pathways (Tables 2, 3), and the exploration of UA transporters (Table 4). The amalgamation of these findings establishes a foundational knowledge, serving as a stepping stone for the prospective creation of efficacious gout treatments.
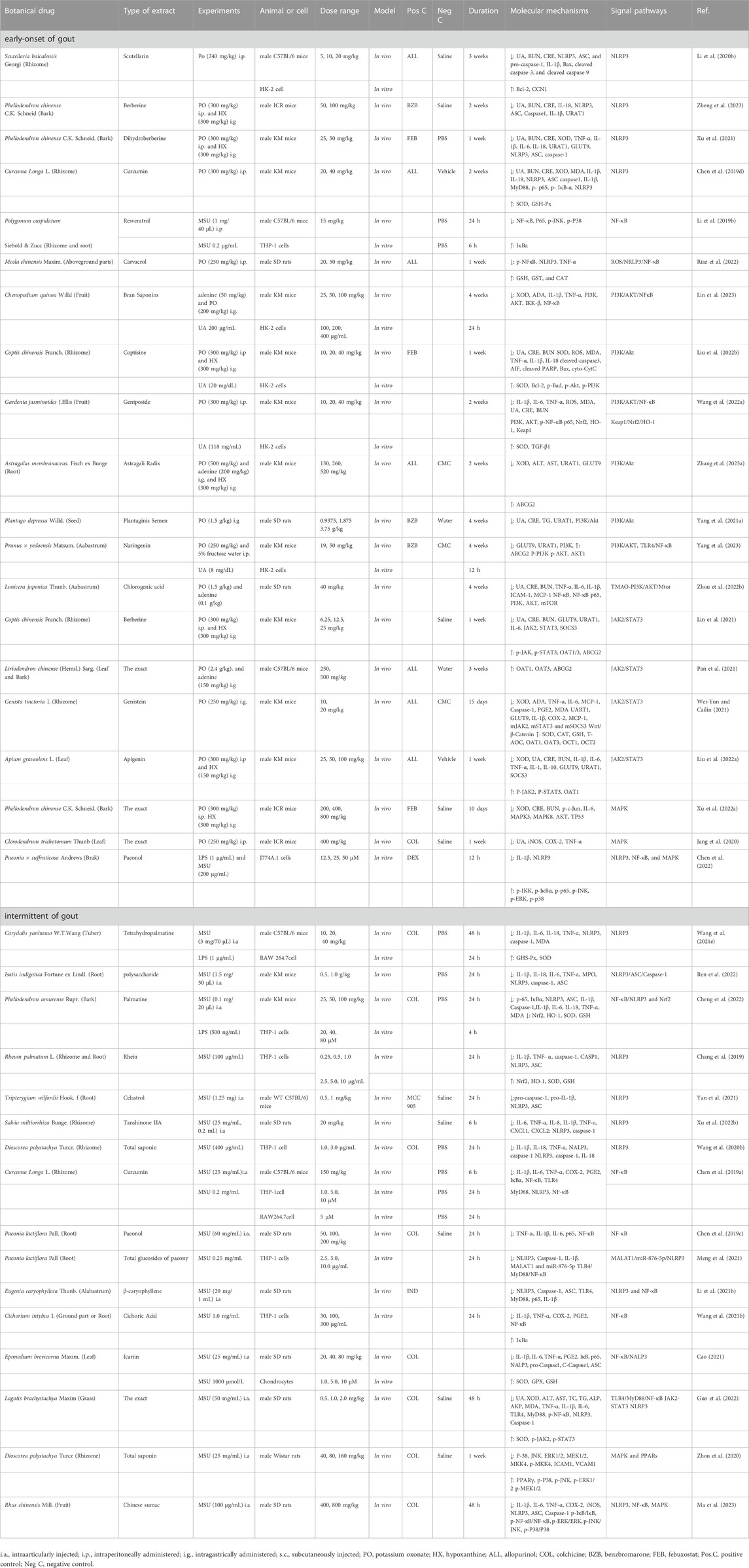
TABLE 2. Regulation effect of botanical drug on signaling pathways in early-onset and intermittent of gout.
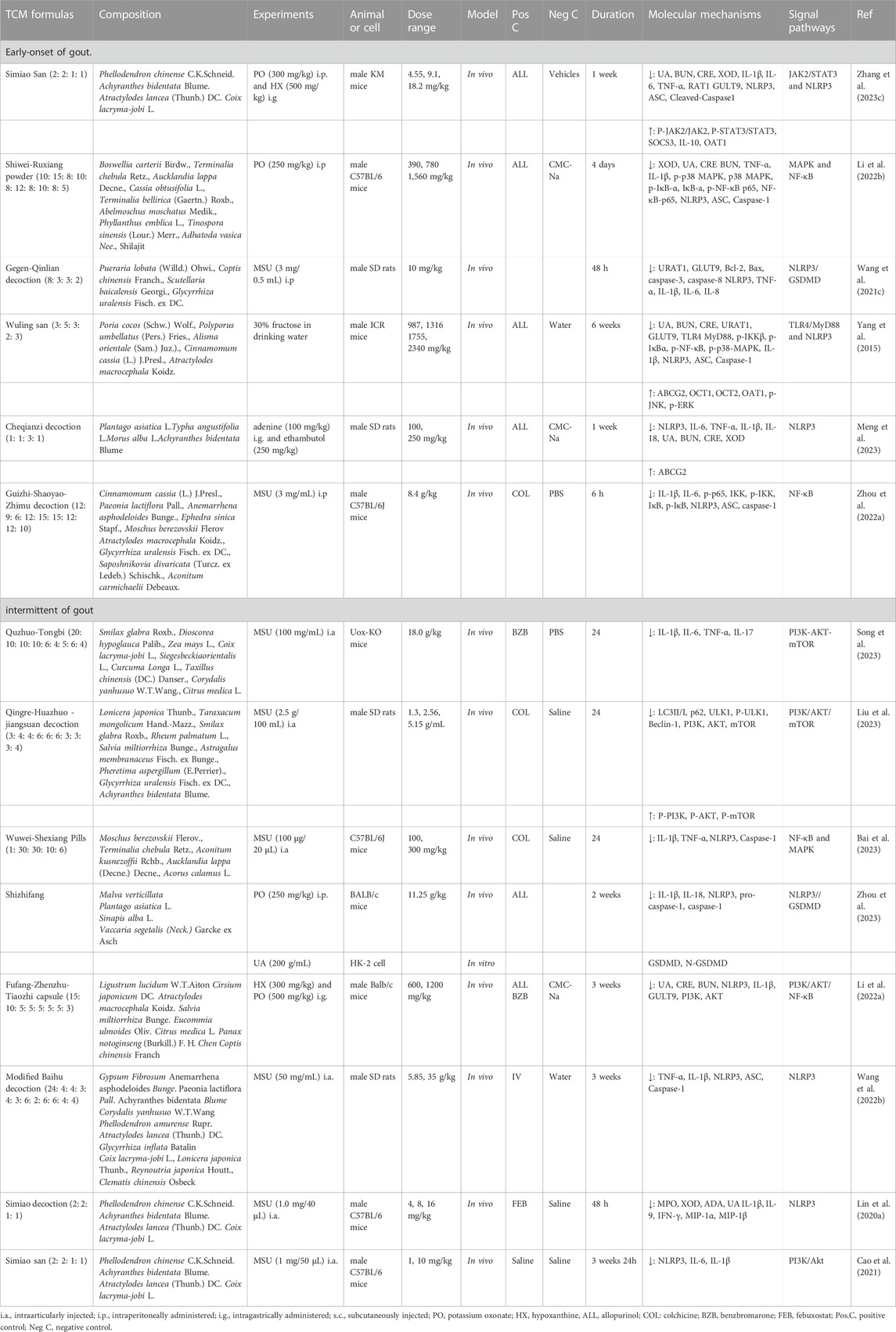
TABLE 3. Regulation effect of TCM formulas on signaling pathways in early-onset and intermittent of gout.
2 Signal pathways of TCM modulating gout pathological progression
2.1 NLRP3 signal pathway
One of the most extensively studied and vital inflammasomes is the NOD-like receptor thermal protein domain-associated protein 3 (NLRP3). This vital component comprises the sensing molecule NLRP3, coupled with apoptosis-associated speck-like (ASC) adaptors, caspase activating recruitment domain, and recruitment caspase-1. Activation of nuclear factor κ-light-chain-enhancer of activated B cells (NF-κB) prompts the upregulation of NLRP3 expression. Following this, it combines and forms an intricate configuration with an adapting protein, ASC, and procaspase-1. Caspase-1 is subsequently activated through the autolysis of procaspase-1. This sequential process subsequently triggers the handling and liberation of interleukin (IL)-1β, IL-18, along with alarm signals. Additionally, it leads to the initiation of pyroptotic cell demise (Yang et al., 2019; de Almeida et al., 2022). Pathogen-associated molecular patterns (PAMPs), including bacterial toxins, microorganisms, and lipopolysaccharides (LPS), contribute to the activation of NLRP3. Additionally, NLRP3 displays responsiveness to various damage-associated molecular patterns (DAMPs), such as MSU, alum, silica, ATP, and others (Biasizzo and Kopitar-Jerala, 2020). Since NLRP3 recognizes a wide range of PAMPs and DAMPs, it is often involved in the pathogenesis of various human diseases as a central sensor of these inflammatory conditions (Zhan et al., 2022). The NLRP3 inflammasome has been established as a primary mechanism by which MSU crystals initiate the inflammatory response (So and Martinon, 2017) through the assembly of NLRP3 and the activation of caspase-1 (Goldberg et al., 2017). Activation of IL-1β triggers the production of IL-6, IL-18, and tumor necrosis factor-α (TNF-α), prompting the migration of neutrophils and other cells into the synovial fluid and synovium, ultimately inciting the onset of inflammation (Hu et al., 2019). Research has demonstrated an elevation in the expression of NLRP3, ASC, IL-1β, and Caspase-1 in joint tissues during the progression of gout (Lin et al., 2020b). Concurrently, additional researchers have also investigated how the inflammatory response initiated by MSU crystals decreases due to the decrease in proteins linked with NLRP3, consequently alleviating both pain and swelling (Yin et al., 2020; Wang et al., 2021a).
Scutellarin is a bioactive flavonoid extracted from the traditional Chinese botanical drug Scutellaria baicalensis Georgi. This compound exhibits neuroprotective, antioxidant, anti-inflammatory, and anti-tumor properties (Chen et al., 2015; Baluchnejadmojarad et al., 2018; Li et al., 2019a). It has the capacity to notably decrease the expression of cleaved-Caspase-3, cleaved-Caspase-9, protein B-cell lymphoma-2 (Bcl-2) associated X protein (BAX), while elevating the levels of the antiapoptotic Bcl-2. Furthermore, scutellarin mitigates the expression of proteins linked to the NLRP3 pathway (NLRP3, ASC, Caspase1, and IL-1β). Through its modulation of the NLRP3 signaling pathway, scutellarin can enhance renal damage caused by UA (Li et al., 2020b). Tetrahydropalmatine, a primary bioactive compound with analgesic, anti-addictive, anti-inflammatory, neuroprotection, and anticancer activities (Du et al., 2022), found in Corydalis yanhusuo (Y.H.Chou & Chun C.Hsu) W.T.Wang, has demonstrated a noteworthy ability to reduce the levels of NLRP3, caspase-1, and IL-1β in the paw tissues of mice with MSU-induced acute gout. It also diminishes proinflammatory cytokines such as TNF-α, IL-1β, IL-6, and IL-18, and thereby alleviating gout-related pain and swelling. This compound acts therapeutically by inhibiting the activation of the NLRP3 inflammasome and the subsequent caspase-1 formation (Wang et al., 2021e). The utilization of Isatis indigotica Fortune ex Lindl. polysaccharide (can lead to a reduction in the levels of NLRP3, ASC, and caspase-1. This modulation aids in alleviating symptoms linked to MSU-induced gouty arthritis, such as neutrophil infiltration in knee joints, joint circumference and the production of TNF-α, IL-1β, IL-6, and IL-18. This effective inhibition of the NLRP3 signaling pathway is facilitated by this approach (Ren et al., 2022). Furthermore, palmatine (Cheng et al., 2022), rhein (Chang et al., 2019), berberine (Zheng et al., 2023), dihydroberberine (Xu et al., 2021), celastrol (Yan et al., 2021), tanshinone IIA (Xu et al., 2022b), total saponin of Dioscorea polystachya Turcz. (Wang et al., 2020b), isoorientin (An et al., 2021), urcumin (Li et al., 2019c) and curcumin (Chen et al., 2019d) have been found to possess anti-inflammatory properties by impeding the NLRP3 signaling pathway. Consequently, these compounds contribute to the improvement of gout-associated conditions.
In a study by Lin et al. (2020a), it was revealed that Simiao decoction effectively eased gouty arthritis symptoms in mice. This decoction led to a decrease the levels of IL-1β, IL-6, and TNF-a within colon tissue, and it hindered the growth of harmful gut bacteria while also suppressing the levels of Caspase-1, ASC, and NLRP3. These observations suggest that Simiao decoction has the potential to ease gouty arthritis by blocking the NLRP3 signaling pathway and opposing inflammation in the gastrointestinal tract. An additional study demonstrated a reduction in miRNA levels of Bax, caspase-3, caspase-8, and Bcl-2 within the renal tissue of hyperuricemic rats treated with Gegen-Qinlian decoction. Furthermore, it decreased the expression of Bcl-2 and caspase gene family proteins in an in vitro UA-stimulated model. These results indicate that Gegen-Qinlian decoction could potentially serve as a favorable approach for gout treatment, functioning by inhibiting apoptosis and renal inflammation (Wang et al., 2021c).
Furthermore, the Chinese Formula Wuling san (Yang et al., 2015), Cheqianzi decoction (Meng et al., 2023), Baihu decoction (Wang et al., 2022b), Sanhuang- Xiexin decoction (Wu et al., 2019) and Shizhi fang (Zhou et al., 2023) have exhibited significant effects in gout prevention by operating through pathways linked to the inhibition of the NLRP3 signaling cascade and the mitigation of renal damage.
2.2 NF-κB signal pathway
Recognized as the key intermediary in inflammation and energy insufficiency, NF-κB governs the synthesis of pro-inflammatory cytokines (Saito and Tanaka, 2017). The family of NF-κB transcription factors encompasses five structurally akin proteins: p50, p52, p65, RelB, and c-Rel (Zhang et al., 2019a). IκBα, which serves as the suppressor of NF-κB, is regulated by the IκB kinase (IKK) complex. Upon stimulation, the NF-κB pathway is activated by degradation of the IκB or ubiquitination of p100 (Mitchell et al., 2016), subsequently orchestrating an inflammatory role by governing the expression of downstream genes, including proinflammatory cytokines (TNF-α, IL-1, and IL-6) (Lepetsos et al., 2019). Studies have indicated that MSU crystals facilitate the nuclear translocation of proteins associated with the NF-κB signaling cascade and the expression of inflammatory factors, finally exacerbating the severity of gout (Chen et al., 2019a; Cao, 2021).
Obtained from Paeonia × suffruticosa Andrews, paeonol exhibits anti-inflammatory characteristics by decreasing the concentrations of proinflammatory cytokines (TNF-α, IL-1β, and IL-6) and reducing p65 within the nucleus. Additionally, it lessens NF-κB DNA-binding activity in synovial tissues of rats experiencing inflammation induced by MSU (Chen et al., 2019c). Icariin, an active compound derived from the botanical drug Epimedium brevicornu Maxim. has demonstrated the ability to diminish inflammation and safeguard the functionality of ankle joints. This is achieved by decreasing the levels of TNF-α, IL-1β, and IL-6, along with Prostaglandin E2 (PGE2). Furthermore, it inhibits the nuclear translocation of p65 and IκB, thus effectively mitigating the symptoms of acute gout arthritis (Cao, 2021). Curcumin, the primary bioactive compound in Curcuma Longa L. hinders the degradation of IκBα and phosphorylation of NF-κB subunits (p50 and p65). This dual effect leads to decreased expression of pro-inflammatory cytokines such as TNF-α, IL-1β, and IL-6, as well as cyclooxygenase-2 (COX-2) and PGE2. As a consequence, a protective impact of curcumin is exerted against gout by alleviating the inflammatory response via modulation of the NF-κB signaling pathway (Chen et al., 2019a). The active biological component found in the roots of Paeonia lactiflora Pall., known as total glucosides of P. lactiflora Pall. demonstrated the ability to lower the expression of toll-like receptor 4 (TLR4), myeloid differentiation factor 88 (MyD88), as well as several key proteins involved in the NF-κB pathway, including phospho-IKBα, IKBα, phospho-p65, and p65. This led to the inhibition of the TLR4/MyD88/NF-κB pathway’s activation triggered by MSU, ultimately providing relief from gout symptoms (Meng et al., 2021). Furthermore, isovitexin (Sun et al., 2021), β-caryophyllene (Li et al., 2021b), cichoric acid (Wang et al., 2021b), resveratrol (Li et al., 2019b), carvacrol (Riaz et al., 2022) and bran saponins (Lin et al., 2023) can offer a safeguarding impact against gout by suppressing the NF-κB signaling pathway.
Several TCM formulations have been observed to decelerate the progression of gout. For instance, Guizhi-Shaoyao-Zhimu decoction, traditionally utilized in TCM for managing “Bi Zheng” exhibited the ability to diminish neutrophil recruitment and lower the concentrations of IL-6, IL-1β, and monocyte chemoattractant protein-1 (MCP-1) (Zhang et al., 2019b). It also hindered the activation of NF-κB signaling pathways by reducing p-IKKβ and p-p65 levels, augmenting IκBα levels, and mitigating NF-κB’s binding to DNA (Wei et al., 2021). Consequently, this formulation effectively shielded mice induced by MSU from inflammation (Zhou et al., 2022a). Moreover, adapted Sanmiao pills, widely utilized in China for the management of gouty arthritis, have demonstrated capability to mitigate the harm caused to ankle joints by MSU and hinder the release of inflammatory cytokines (such as TNF-α, IL-6, and IL-1β), alongside reducing the levels of pivotal proteins engaged in the TLRs/MyD88/NF-κB signaling pathway (Chen et al., 2023).
2.3 PI3K/AKT signal pathway
Phosphatidylinositol 3 kinase (PI3K) and protein kinase B (AKT) are upstream elements of the NF-κB signaling cascade, pivotal in the initiation and release of various inflammatory factors (Wang et al., 2020a). Elevated levels of UA have the capacity to attach to receptors situated on the cell membrane, engaging with receptors present on the membrane’s surface. This interaction triggers the activation of PI3K and AKT, thereby initiating a sequence of subsequent reactions in mice with hyperuricemia (Wang et al., 2022a). Moreover, the process of autophagy is governed by PI3K/AKT pathway andmammalian target of rapamycin (mTOR) (Evangelisti et al., 2020), and it is implicated in the inflammatory response of gouty arthritis (Lou et al., 2022). The MSU crystal has also been found to activate autophagy with subsequent cell death by inhibiting the phosphorylation of the AKT/mTOR signaling cascade (Hwang et al., 2015), thereby playing a crucial role in the promotion of gout (Xiao et al., 2023). PI3K enzymes are grouped into three primary categories (Class I, II, and III) according to their structural characteristics and substrate specificity. Among these, PI3KI, which contributes to various biological activities, has garnered the most research attention (Guo et al., 2015). Phosphoinositide-associated kinase-1 (PDK1) and AKT are brought to the inner layer of the cellular membrane via pleckstrin homology regions. AKT reveals two principals phosphorylation sites (Thr308 and Ser473), which are phosphorylated by PDK1 and mTORC2, respectively (Manning and Toker, 2017). Following full activation, AKT is orchestrating distinct subsequent cascades and carrying out diverse functions like governing cell viability, metabolism, and growth by impacting the activation statuses of numerous downstream effectors (Chen et al., 2018).
Coptisine, a prominent bioactive compound found in Coptis chinensis Franch. diminishes the levels of cleaved poly ADP-ribose polymerase (PARP), cleaved-caspase-3, Bax, cytochrome C (cytC), and apoptosis-inducing factor (AIF). Concurrently, it elevates the protein levels of Bcl-2 and p-Bad, thereby offering protection against oxidative stress, mitochondrialapoptosis, and renal inflammatory harm triggered by hyperuricemia. This safeguarding effect is achieved by modulating the PI3K/AKT signaling pathway (Liu et al., 2022b). From the fruit of Gardenia jasminoides J.Ellis., it was found that an iridoid glycoside, called geniposide, can be extracted, which has antiarthritic properties (Wang et al., 2021d; Wang et al., 2022c; Ke et al., 2022). The geniposide-phospholipid complex reduces levels of inflammatory cytokines (TNF-α, IL-6, and IL-1β) in hyperuricemic mice and regulates the PI3K/AKT/NF-κB signaling cascade to improve post-hyperuricemia chronic kidney disease (Wang et al., 2022a). Naringenin, a flavonoid found in citrus fruits known for its diverse biological effects, offers renal protection by reducing elevated IL-6 and TNF-α level. Additionally, it improves hyperuricemia conditions by boosting UA excretion through the inhibition of the PI3K/AKT signaling cascade in the kidney (Yang et al., 2023). Chlorogenic acid, present in ample amounts within Lonicera japonica Thunb. and Eucommia ulmoides Oliv., has been observed to lower the protein levels of phosphorylated AKT (P-AKT), PI3K, and mTOR. It effectively safeguards against hyperuricemia-induced kidney damage by activating the PI3K/AKT signaling cascade (Zhou et al., 2022b).
Gout falls under the “arthralgia syndrome” classification in TCM, arising from the accumulation of turbid toxins, heat, andblood stasis. A formulation known as Qingre-Huazhuo-Jiangsuan decoction, designed to eliminate turbidity, dispel heat, decrease acidity, and combat inflammation, exhibits the ability to mitigate the inflammatory state of acute gouty arthritis (wang et al., 2018). This is achieved by activating autophagy genes and suppressing the PI3K/AKT/mTOR pathway in knee synovial tissue (Liu et al., 2023). Furthermore, Quzhuo-Tongbi decoction effectively enhances the presence of allobaculum and candidatussaccharimonas in the gut microbiome, decrease concentration of inflammatory cytokines (TNF-α, IL-6, IL-17, and IL-1β), and restores the equilibrium between Th17 and Treg cells. This comprehensive approach regulates the differentiation of CD4+ T cells through the PI3K/AKT/mTOR cascade and remodels the gut microbiome, offering a strategy for gout treatment (Song et al., 2023).
Furthermore, certain herbal remedies have demonstrated gout-protective effects, including Huangqin-Qingre-Chubi capsule (Zhang et al., 2023b), Fufang -Zhenzhu-Tiaozhi capsule (Li et al., 2022a) and Simiao san (Cao et al., 2021), and some herbal medicines, such as Astragalus membranaceus Fisch. ex Bunge (Zhang et al., 2023a), Phellodendron chinense C.K.Schneid (Xu et al., 2022a). and Plantago depressa Willd (Yang et al., 2021a). These interventions have been linked to anti-inflammatory effects and the modulation of the PI3K/AKT signaling pathway.
2.4 JAK/STAT signal pathway
The Janus Kinase (JAK), and the signal transducer and activator of transcription (STAT) signaling pathway is composed of tyrosine kinase-related receptors called JAK, and STAT (Li et al., 2015), This pathway is interconnected with various bodily functions and is involved in significant biological processes such as immune regulation, cell proliferation, apoptosis, and differentiation (Xin et al., 2020). STAT assumes a critical role in both signal activation and transcription. In the cytoplasmic, the STAT group functions as a downstream recipient for JAKs, which are intracellular tyrosine kinases linked to the internal domains of receptors situated on the cell membrane (Chapman et al., 2022). In a general sense, external signals like IL-2 to IL-7, epidermal growth factor, and interferon (IFN) (O'Shea et al., 2015) attach to their respective receptors located on the cell membrane. This interaction leads to the proximity of receptor-associated JAK kinases, resulting in their mutual phosphorylation and activation. Subsequently, the activated receptors phosphorylate STAT proteins, prompting their migration from the inner cellular surroundings to the nucleus (Morris et al., 2018), Once in the nucleus, these STAT proteins bind to DNA and orchestrate the regulation of gene transcription. Depending on the tissue specificity and the receptors engaged in the signaling process, diverse JAKs and STATs become enlisted in this mechanism (Savage et al., 2020), which exhibits a strong connection with the onset and progression of different diseases (Zhang et al., 2022a). During gout flare-ups, the activation of JAK2/STAT3 signaling results in an elevation of cytokine expression, including IL-6, IL-1β, and TNF-α, within the kidneys and joints. Consequently, this provokes an inflammatory reaction towards the accumulation of crystals (Xin et al., 2020; Ren et al., 2021). Simultaneously, the reduction of the JAK/STAT signaling cascade can curtail the deposition of monosodium urate crystals and lower serum urate levels, effectively suppressing joint pain and inflammation (Cunningham et al., 2016).
Berberine, the primary constituent of Phellodendron chinense C.K.Schneid., has exhibited the capacity to diminish the expression of phospho-JAK2, phospho -STAT3, and suppressor of cytokine signaling 3 (SOCS3). Moreover, it lessens renal concentrations of TNF-α, IL-1β, and IL-6 in mouse models of hyperuricemia, thereby mitigating renal impairment. This is achieved through the inhibition of the JAK-STAT signaling pathway’s activation and the attenuation of the inflammatory response triggered by UA (Lin et al., 2021). Apigenin, a plant-derived flavone compound, is acknowledged as a biologically active flavonoid known for its anti-inflammatory properties. It exhibits a robust affinity for JAK2 proteins and, UA transporters effectively enhancing UA metabolism and mitigating renal damage (Liu et al., 2017b). This is accomplished by curtailing UA production, enhancing excretion, and dampening the activity of the JAK2/STAT3 signaling pathway in hyperuricemia mice (Liu et al., 2022a). Lagotis brachystachya Maxim., primarily employed for treating yellow water disease (gouty arthritis) and hepatitis, is capable of reducing the expression of phospho-JAK2 and phospho-STAT3. It also operates to hinder the JAK2/STAT3 signaling cascade within synovial tissues, thereby alleviating ankle joint swelling and gout-induced inflammation in rats (Guo et al., 2022). Liriodendron chinense (Hemsl.) Sarg., a component of TCM employed to expel “wind and dampness”, has been observed to impede the advancement of hyperuricemic nephropathy. This is achieved by curbing the activation of the JAK2/STAT3 signaling cascade, lessening the influx of inflammatory agents, and diminishing the buildup of UA within the kidney (Pan et al., 2021). Genistein, an isoflavone initially extracted from the flowering plant Genista tinctoria L., has been identified for its ability to diminish the levels of renal mJAK2, mSTAT3, and mSOCS3. This action leads to a reduction in renal inflammation and show cases a safeguarding effect against potassium oxonate-induced hyperuricemia in mice (Wei-Yun and Cailin, 2021).
Furthermore, both in vivo and in vitro studies have demonstrated that Simiao wan can effectively lower the concentrations of TNF-α, IL-6, and IL-1β. It achieves this by suppressing the expressions of phospho-JAK2/JAK2, phospho-STAT3/STAT3, and SOCS3, thereby constraining the activity of the JAK2/STAT3 signaling pathways. This comprehensive approach contributes to the alleviation of hyperuricemia and renal inflammation (Yang et al., 2021b; Zhang et al., 2023c).
2.5 MAPK signal pathway
Mitogen-activated protein kinases (MAPKs), comprising extracellular signal-regulated kinases (ERK1/2), Jun N-terminal kinase (JNK), and P38, play crucial roles in the onset of gouty arthritis episodes. Serving as precursor kinases, mitogen-activated protein kinase kinase 4/7 (MKK4/7), MKK3/6 andmitogen-activated extracellular signal-regulated kinase 1/2 (MEK1/2) govern the activation of JNK, P38, and ERK1/2, respectively (Zhang et al., 2018b; Stramucci et al., 2018; Wang et al., 2019). Recent studies have indicated that UA has the potential to induce the promotion and migration of vascular smooth muscle cells (VSMCs) through the activation of the p38 and p44/42 MAPK pathways in individuals with hyperuricemia (Doğru et al., 2022), Ultimately, this process contributes to vascular remodeling and the development of other associated conditions (Canepa et al., 2017; Oğuz et al., 2017). MAPKs, which form part of an extensive group of serine-threonine kinases, create signaling pathways that extend from the cellular membrane to the nucleus, governing oxidative stress, cell proliferation, apoptosis, differentiation, innate immunity, and inflammation (Guo et al., 2020). The MAPK pathway consists of MKK, MAPK kinase, and MAPK components. Recently, emerging members of the MAPK family, such as ERK1/2, ERK3/4, ERK5, ERK7/8, JNK 1/2/3, and p38 α/β/γ (ERK6)/δ), have been elucidated (Chen et al., 2019b). Within multicellular organisms, protein kinases participate in modulating cellular functions by adding phosphate groups to distinct serine and threonine positions on target protein substrates (Oh et al., 2019; Guo et al., 2020). Increasing evidence suggests that the activation of the MAPK cascade is intricately linked with the regulation of autophagy, which is associated with renal tubular injury and inflammation in the context of hyperuricemia (Bao et al., 2018; Wu et al., 2021). UA triggers apoptosis by disrupting the equilibrium between pro-apoptosis and anti-apoptosis proteins via the MAPK pathway. This leads to oxidative stress within kidney cells and contributes to inflammation (Jang et al., 2020). Consequently, there is an indication that genes responsible for mediating the positive regulation of the MAPK pathway might potentially contribute to hypouricemic effects (Jang et al., 2020).
Extracted from Dioscorea polystachya Turcz., the total saponin fraction exhibits the ability to ameliorate synovial tissue conditions. Additionally, it diminishes the protein expressions of phospho-MEK1/2, phospho-ERK1/2, phospho-JNK, and MKK4 in rats induced with MSU crystals. This process effectively mitigates inflammation in gout arthritis by inhibiting the MAPK signaling pathway (Zhou et al., 2020). Derived from the root bark of Paeonia × suffruticosa Andrews., paeonol (a phenolic compound), has been found to widespread use in TCM for various forms of arthritis (Liu et al., 2017a). Paeonol effectively hinders the expression of IL-1β induced by MSU and reduces the levels of phospho-MAPK (p-ERK, p-p38, and p-JNK), as well as phospho-IKKβ and phospho-IκBα. These findings suggest that paeonol curtails the activation of p-IKKβ through the MAPK pathway and prevents the degradation of p-IκBα. Consequently, paeonol functions as an anti-gout agent by regulating the MAPK signaling cascade (Chen et al., 2022). Astaxanthin, a xanthophyll carotenoid, exhibits the capability to lower the expression of phosphor-p38, phosphor-JNK, and phosphor-ERK1/2 within J774A.1 cells, synovial fibroblasts, and articular chondrocytes. As a consequence, this action inhibits the production of COX-2 proteins and NLRP3 inflammasomes, thereby enhancing its potential to mitigate MSU crystal-mediated arthritis through the deactivation of the MAPK pathway (Peng et al., 2020). Phellodendron chinense C.K.Schneid., a frequently employed herbal remedy for treating gout or hyperuricemia, effectively restores the over-expression of IL-6 and p-c-Jun proteins, along with mRNA levels of AKT, MAPK3, MAPK8, and TP53 within the renal tissues of hyperuricemia-afflicted mice. It further curbs UA production, exerting a nephroprotective effect through the inhibition of inflammatory and cell death, including MAPK (Xu et al., 2022a). Moreover, conventional Chinese herbal substances like Clerodendrum trichotomum Thunb. and Rhus chinensis Mill. manifest notable anti-inflammatory properties in cases of gout through their modulation of the MAPK signaling pathway (Jang et al., 2020; Ma et al., 2023).
Shiwei-Ruxiang powder is a customary remedy frequently employed in addressing gout and has demonstrated efficacy in clinical trials for preventing hyperuricemia and gout (Yu et al., 2022). Notably, Shiwei-Ruxiang powder has been observed to ameliorate renal impairment by diminishing CRE and BUN levels, while also counteracting the concentrations of pro-inflammatory agents like TNF-α and IL-1β, along with the expression of phospho-p38 MAPK, within hyperuricemia mice. This process is inherently connected to the MAPK signaling cascade (Li et al., 2022b). Wuwei-Shexiang pills, formulated with Acorus calamus L., Moschus berezovskii Flerov, Aconitum carmichaelii Debeaux., Aucklandia lappa (Decne) Decne., and Terminalia chebula Retz., exhibits the ability to alleviate ankle joint swelling and diminish TNF-α and IL-1β concentrations within MSU-induced mice air pouch lavage fluid. This is accomplished by restraining the levels of p-p38/p38 through the modulation of the MAPK signaling cascade, thus effectively mitigating gout-related inflammation (Bai et al., 2023).
3 The uric acid transporter of TCM in the treatment of gout
It has been documented that around 90% of individuals with hyperuricemia experience underexcretion-type hyperuricemia (Ota-Kontani et al., 2020). Both the intestine and the kidney contribute significantly to the elimination process of UA, with approximately 70%–75% being expelled through the renal pathway, while 20%–25% is excreted through the gastrointestinal tract. Importantly, UA is characterized by its polarity and does not readily traverse membranes. Consequently, the excretion of UA hinges on transporter proteins situated within renal proximal convoluted tubules and the intestinal environment (Dalbeth et al., 2021).
3.1 Renal uric acid transporter
Urate transport across the apical surface of the kidney, initiating in the lumen and reaching the cell, is mediated by specific transporters such as glucose transporter 9 (GLUT9/SLC2A9), organic anion transporter 4 (OAT4/SLC22A11), OAT10/SLC22A13, and urate anion transporter 1 (URAT1/SLC22A12) (Lu et al., 2019). ATP Binding Cassette Transporter C4 (ABCC4), ATP binding cassette transporter G2 (ABCG2), phosphate cotransporter type 1 (NPT1/SLC17A1), OAT1/SLC22A6, OAT2/SLC22A7, and OAT3/SLC22A8 function as secretory transporters (Dong and Dong, 2023). URAT1, characterized by the presence of 12 transmembrane structural domains, displays exclusive expression on the luminal membrane within the proximal renal tubule (Tan et al., 2017) and it is recognized as the primary apical urate/anion exchanger in humans, facilitating urate reabsorption. The deletion of the URAT1 gene results in a notable decrease in the reabsorption and excretion of UA, consequently precipitating renal hyperuricemia and the onset of gout (Kuo et al., 2017). GLUT9, found in both the basal and apical membranes of the renal proximal tubule, also accounts for the reabsorption of UA (Auberson et al., 2018), playing a crucial role in the renal process of UA excretion (Lüscher et al., 2022). Moreover, knockout mice of SLC2A9 demonstrate impaired enterocyte urate transport kinetics coupled with elevated serum urate concentration (Novikov et al., 2019). Present within the basolateral membrane of cells, OAT1 and OAT3 aid in the transportation of urate into proximal tubule cells, thereby contributing to the process of UA elimination (García-Nieto et al., 2022). This significance is highlighted by the observation of elevated serum UA levels in mice with knockout of OAT1 and OAT3, emphasizing the role of organic anion transporters in hyperuricemia pathogenesis (Wu et al., 2017). ABCG2, arising from a gene located at the chromosomal region linked to gout susceptibility on chromosome 4q, is widely recognized for its role as a potent urate secretion transporter with high capacity (Woodward, 2015). Its presence is notable on the apical membrane of proximal tubules in the human kidney (Lin et al., 2019), and it is similarly found in both the intestine and liver (Eckenstaler and Benndorf, 2021). The expression profile of ABCG2 within the kidney exhibits partial similarity to that of URAT1, implying a functional interplay between these two transporters in the handling of renal UA (Hoque et al., 2020). Research findings indicate that mice lacking the ABCG2 gene experience increased serum levels of UA, linked to escalated renal UA excretion and reduced UA excretion within the intestines (Toyoda et al., 2019).
3.2 Intestinal uric acid transporter
An additional path for UA elimination occurs within the intestines. ABCG2 is a pivotal regulator of urate excretion primarily in the gastrointestinal tract (Bhatnagar et al., 2016), signifying its crucial involvement in this physiological process (Yin et al., 2022). Impaired ABCG2 function notably raises the susceptibility to hyperuricemia and potentially gout, particularly among the younger population (Ye et al., 2022). In a well-established hyperuricemia mouse model achieved through the application of the uricase inhibitor potassium oxonate, there is a consistent elevation in the serum UA concentration within ABCG2-knockout mice (Kuo et al., 2017). Furthermore, studies have indicated that heightened ABCG2 expression was noticed in the intestines of nephrectomized rats, accompanied by reduced UA excretion. Surprisingly, even with this decrease, serum UA concentration showed no elevation, suggesting the potential involvement of the intestinal tract as an “extra-renal” route for urate elimination. This highlights the potential of intestinal urate excretion as a novel approach for gout and hyperuricemia treatment, offering the advantage of avoiding potential side effects like exacerbating kidney injury and urinary calculus formation.
Currently, it has been widely proven that TCM can effectively reduce UA in clinical practice. The multi-target function of TCM simultaneously affects multiple UA transporters, achieving the reduction of UA and the protection of the kidneys. Various herbal extracts used in TCM and natural bioactive compounds have been demonstrated to be beneficial in treating gout (Table 4).
4 Discussions and perspective
This comprehensive analysis has substantiated that Chinese medicine monomers, individual herbal remedies from Chinese tradition, and formulations of TCM offer significant assistance in gout management by impacting the expression and function of urate transporters. These encompass GLUT9, URAT1, OATs, and ABCG2, as well as their associated regulatory cascades like NLRP3, NF-κB, JAK/STAT, PI3K/AKT, and MAPK pathways. However, there are still several limitations must be considered in the gout therapy using TCM.
As we reviewed, these botanical drugs have shown positive anti-gout effects. Yet it is worth noting that the therapeutic effect of inhibiting inflammatory signaling pathways and uric acid transporters on gout has only been experimentally validated in cells and animals. Due to the differences between species, the therapeutic effects of some plant drugs with anti-inflammatory and reducing uric acid effects at the cellular and animal levels may not be satisfactory in the human, so more in-depth research is needed.
Another point to consider is that more studies are needed to confirm whether TCM can be effective targets for gout treatment. In recent literature, a compelling association between UA transporters and signaling pathways has come to light. UA drives excretion through the activation of signaling cascades involving NLRP3, PI3K/Akt and MAPK, leading to the augmentation of PDZK1 and ABCG2 expression, thereby mitigating the effects of hyperuricemia (Chen et al., 2018). The PI3K/Akt pathway stands out as a significant signaling route linked to transporter expression, capable of enhancing the levels of GLUT9 and URAT1 (Zhang et al., 2023a; Yang et al., 2023). Moreover, the depletion of GLUT9 led to the suppression of JAK2/STAT3 signaling, resulting in decreased release of pro-inflammatory molecules (Nie et al., 2021). Taken together, the mutual modulation between UA transporters and this signaling pathway may accomplish the dual objectives of diminishing UA level and safeguarding renal health and offer innovative avenues for advancing targeted gout therapy using TCM.
Notably, studies on the regulatory mechanisms the therapeutic applications of small molecule metabolites in gout by regulating protein functions are particularly lacking, which may be a weak direction for understanding the role in the pathogenesis and treatment of gout. Utilizing a metabolomics approach, researchers have employed a strategy to unveil the underlying metabolic mechanisms and plausible targets associated with TCM. Likewise, the interplay between metabolites and receptors offers an avenue to investigate potential therapeutic targets and the regulatory function of TCM within signaling pathways, facilitating internal signaling communication for addressing gout. A prime illustration lies in the distinctive involvement of purine metabolism in both inflammatory responses and immune system modulation in the context of gout. Notably, purinergic receptors, including P2X1, P2X7, P2Y2, and P2Y14, are found expressed across a wide spectrum of immune cells (Wang and Chen, 2018). ATP stimulation triggers immune cell activation, serving as a secondary pathogenic indicator in gout (Li et al., 2021c). Typically, altered ATP levels can trigger the P2X7R-NLRP3 signaling cascade, leading to the secretion of IL-1β linked to gout attacks (Tao et al., 2017). In contrast, ATP has the potential to convert into adenosine, resulting in the shift from the pro-inflammatory reaction through the P2Y and P2X receptor signaling routes to a more prevalent anti-inflammatory impact facilitated by the P1Y receptor signaling cascade. Hence, utilizing metabolomics strategy, a comprehensive exploration into distinct governing mechanisms within purine metabolism and the incorporation of diverse factors influencing both upstream and downstream processes can precisely ascertain the contribution of purine metabolism to gout flares. This endeavor offers viable avenues for strategic clinical interventions.
At present, we are engaged in clinical experimental investigations focusing on the role of biomarkers influenced by protein targets in the initiation of gout. Simultaneously, we are exploring the potential of utilizing TCM-regulated proteins to modulate metabolic pathways for gout management in mouse models. Furthermore, by combining and synthesizing the results of multiple studies that investigate TCM’s inhibitory effects on signaling pathways like NLRP3, along with its positive effects on gout, we are optimistic about substantial progress in the field of anti-gout TCM. This advancement involves a direct emphasis on proteins and biomarkers in the approaching phase, potentially leading to a smooth transition into clinical application and improving the current scenario of gout detection, prediction, and therapy.
Author contributions
XS: Writing–original draft. LY: Writing–review and editing. HS: Writing–review and editing. YS: Writing–review and editing. SW: Writing–review and editing. YH: Writing–review and editing. WW: Writing–review and editing. LK: Writing–review and editing. XW: Conceptualization, Project administration, Supervision, Writing–review and editing.
Funding
The authors declare financial support was received for the research, authorship, and/or publication of this article. This work was supported by grants from Key research and development project of states key laboratory of dampness syndrome of Chinese medicine (SZ2021ZZ49).
Conflict of interest
The authors declare that the research was conducted in the absence of any commercial or financial relationships that could be construed as a potential conflict of interest.
Publisher’s note
All claims expressed in this article are solely those of the authors and do not necessarily represent those of their affiliated organizations, or those of the publisher, the editors and the reviewers. Any product that may be evaluated in this article, or claim that may be made by its manufacturer, is not guaranteed or endorsed by the publisher.
References
Ai, G., Huang, R., Xie, J., Zhong, L., Wu, X., Qin, Z., et al. (2023). Hypouricemic and nephroprotective effects of palmatine from Cortex Phellodendri Amurensis: a uric acid modulator targeting Keap1-Nrf2/NLRP3 axis. J. Ethnopharmacol. 301, 115775. doi:10.1016/j.jep.2022.115775
An, M. F., Shen, C., Zhang, S. S., Wang, M. Y., Sun, Z. R., Fan, M. S., et al. (2023). Anti-hyperuricemia effect of hesperetin is mediated by inhibiting the activity of xanthine oxidase and promoting excretion of uric acid. Front. Pharmacol. 14, 1128699. doi:10.3389/fphar.2023.1128699
An, M. F., Wang, M. Y., Shen, C., Sun, Z. R., Zhao, Y. L., Wang, X. J., et al. (2021). Isoorientin exerts a urate-lowering effect through inhibition of xanthine oxidase and regulation of the TLR4-NLRP3 inflammasome signaling pathway. J. Nat. Med. 75, 129–141. doi:10.1007/s11418-020-01464-z
Auberson, M., Stadelmann, S., Stoudmann, C., Seuwen, K., Koesters, R., Thorens, B., et al. (2018). SLC2A9 (GLUT9) mediates urate reabsorption in the mouse kidney. Pflugers Arch. 470, 1739–1751. doi:10.1007/s00424-018-2190-4
Bai, L., Wu, C., Lei, S., Zou, M., Wang, S., Zhang, Z., et al. (2023). Potential anti-gout properties of Wuwei Shexiang pills based on network pharmacology and pharmacological verification. J. Ethnopharmacol. 305, 116147. doi:10.1016/j.jep.2023.116147
Baluchnejadmojarad, T., Zeinali, H., and Roghani, M. (2018). Scutellarin alleviates lipopolysaccharide-induced cognitive deficits in the rat: insights into underlying mechanisms. Int. Immunopharmacol. 54, 311–319. doi:10.1016/j.intimp.2017.11.033
Bao, J., Shi, Y., Tao, M., Liu, N., Zhuang, S., and Yuan, W. (2018). Pharmacological inhibition of autophagy by 3-MA attenuates hyperuricemic nephropathy. Clin. Sci. (Lond) 132, 2299–2322. doi:10.1042/cs20180563
Bao, R., Chen, Q., Li, Z., Wang, D., Wu, Y., Liu, M., et al. (2022). Eurycomanol alleviates hyperuricemia by promoting uric acid excretion and reducing purine synthesis. Phytomedicine 96, 153850. doi:10.1016/j.phymed.2021.153850
Bhatnagar, V., Richard, E. L., Wu, W., Nievergelt, C. M., Lipkowitz, M. S., Jeff, J., et al. (2016). Analysis of ABCG2 and other urate transporters in uric acid homeostasis in chronic kidney disease: potential role of remote sensing and signaling. Clin. Kidney J. 9, 444–453. doi:10.1093/ckj/sfw010
Biasizzo, M., and Kopitar-Jerala, N. (2020). Interplay between NLRP3 inflammasome and autophagy. Front. Immunol. 11, 591803. doi:10.3389/fimmu.2020.591803
Canepa, M., Viazzi, F., Strait, J. B., Ameri, P., Pontremoli, R., Brunelli, C., et al. (2017). Longitudinal association between serum uric acid and arterial stiffness: results from the Baltimore longitudinal study of aging. Hypertension 69, 228–235. doi:10.1161/hypertensionaha.116.08114
Cao, L., Zhao, T., Xue, Y., Xue, L., Chen, Y., Quan, F., et al. (2021). The anti-inflammatory and uric acid lowering effects of Si-Miao-San on gout. Front. Immunol. 12, 777522. doi:10.3389/fimmu.2021.777522
Cao, Y. (2021). Icariin alleviates MSU-induced rat GA models through NF-κB/NALP3 pathway. Cell Biochem. Funct. 39, 357–366. doi:10.1002/cbf.3598
Chang, W. C., Chu, M. T., Hsu, C. Y., Wu, Y. J., Lee, J. Y., Chen, T. J., et al. (2019). Rhein, an anthraquinone drug, suppresses the NLRP3 inflammasome and macrophage activation in urate crystal-induced gouty inflammation. Am. J. Chin. Med. 47, 135–151. doi:10.1142/s0192415x19500071
Chang, Y. H., Chiang, Y. F., Chen, H. Y., Huang, Y. J., Wang, K. L., Hong, Y. H., et al. (2021). Anti-inflammatory and anti-hyperuricemic effects of chrysin on a high fructose corn syrup-induced hyperuricemia rat model via the amelioration of urate transporters and inhibition of NLRP3 inflammasome signaling pathway. Antioxidants (Basel) 10, 564. doi:10.3390/antiox10040564
Chapman, S., Kwa, M., Gold, L. S., and Lim, H. W. (2022). Janus kinase inhibitors in dermatology: Part I. A comprehensive review. J. Am. Acad. Dermatol 86, 406–413. doi:10.1016/j.jaad.2021.07.002
Chen, Y. J., Wang, L., Zhou, G. Y., Yu, X. L., Zhang, Y. H., Hu, N., et al. (2015). Scutellarin attenuates endothelium-dependent aasodilation impairment induced by hypoxia reoxygenation, through regulating the PKG signaling pathway in rat coronary artery. Chin. J. Nat. Med. 13, 264–273. doi:10.1016/s1875-5364(15)30013-3
Chen, B., Li, H., Ou, G., Ren, L., Yang, X., and Zeng, M. (2019a). Curcumin attenuates MSU crystal-induced inflammation by inhibiting the degradation of IκBα and blocking mitochondrial damage. Arthritis Res. Ther. 21, 193. doi:10.1186/s13075-019-1974-z
Chen, C., Nelson, L. J., Ávila, M. A., and Cubero, F. J. (2019b). Mitogen-activated protein kinases (MAPKs) and cholangiocarcinoma: the missing link. Cells 8, 1172. doi:10.3390/cells8101172
Chen, G., Guo, T., and Yang, L. (2022). Paeonol reduces IL-β production by inhibiting the activation of nucleotide oligomerization domain-like receptor protein-3 inflammasome and nuclear factor-κB in macrophages. Biochem. Cell Biol. 100, 28–36. doi:10.1139/bcb-2021-0255
Chen, G., Jia, P., Yin, Z. Y., Kong, S. Z., Xiang, Z. B., and Zheng, X. X. (2019c). Paeonol ameliorates monosodium urate-induced arthritis in rats through inhibiting nuclear factor-κB-mediated proinflammatory cytokine production. Phytother. Res. 33, 2971–2978. doi:10.1002/ptr.6472
Cheng, J. J., Ma, X. D., Ai, G. X., Yu, Q. X., Chen, X. Y., Yan, F., et al. (2022). Palmatine protects against MSU-induced gouty arthritis via regulating the NF-κB/NLRP3 and Nrf2 pathways. Drug Des. Devel Ther. 16, 2119–2132. doi:10.2147/dddt.S356307
Chen, M., Lu, X., Lu, C., Shen, N., Jiang, Y., Chen, M., et al. (2018). Soluble uric acid increases PDZK1 and ABCG2 expression in human intestinal cell lines via the TLR4-NLRP3 inflammasome and PI3K/Akt signaling pathway. Arthritis Res. Ther. 20, 20. doi:10.1186/s13075-018-1512-4
Chen, M., Ye, C., Zhu, J., Zhang, P., Jiang, Y., Lu, X., et al. (2020). Bergenin as a novel urate-lowering therapeutic strategy for hyperuricemia. Front. Cell Dev. Biol. 8, 703. doi:10.3389/fcell.2020.00703
Chen, R., Li, F., Zhou, K., Xing, M., Zhang, X., Zhao, X., et al. (2023). Component identification of modified sanmiao pills by UPLC-Xevo G2-XS QTOF and its anti-gouty arthritis mechanism based on network pharmacology and experimental verification. J. Ethnopharmacol. 311, 116394. doi:10.1016/j.jep.2023.116394
Chen, Y., Li, C., Duan, S., Yuan, X., Liang, J., and Hou, S. (2019d). Curcumin attenuates potassium oxonate-induced hyperuricemia and kidney inflammation in mice. Biomed. Pharmacother. 118, 109195. doi:10.1016/j.biopha.2019.109195
Chen, Y., Zhao, Z., Li, Y., Yang, Y., Li, L., Jiang, Y., et al. (2021). Baicalein alleviates hyperuricemia by promoting uric acid excretion and inhibiting xanthine oxidase. Phytomedicine 80, 153374. doi:10.1016/j.phymed.2020.153374
Cobo, I., Cheng, A., Murillo-Saich, J., Coras, R., Torres, A., Abe, Y., et al. (2022). Monosodium urate crystals regulate a unique JNK-dependent macrophage metabolic and inflammatory response. Cell Rep. 38, 110489. doi:10.1016/j.celrep.2022.110489
Cui, D., Liu, S., Tang, M., Lu, Y., Zhao, M., Mao, R., et al. (2020). Phloretin ameliorates hyperuricemia-induced chronic renal dysfunction through inhibiting NLRP3 inflammasome and uric acid reabsorption. Phytomedicine 66, 153111. doi:10.1016/j.phymed.2019.153111
Cunningham, C. C., Corr, E. M., Mccarthy, G. M., and Dunne, A. (2016). Intra-articular basic calcium phosphate and monosodium urate crystals inhibit anti-osteoclastogenic cytokine signalling. Osteoarthr. Cartil. 24, 2141–2152. doi:10.1016/j.joca.2016.07.001
Dalbeth, N., Gosling, A. L., Gaffo, A., and Abhishek, A. (2021). Gout. Lancet 397, 1843–1855. doi:10.1016/s0140-6736(21)00569-9
De Almeida, L., Devi, S., Indramohan, M., Huang, Q. Q., Ratsimandresy, R. A., Pope, R. M., et al. (2022). POP1 inhibits MSU-induced inflammasome activation and ameliorates gout. Front. Immunol. 13, 912069. doi:10.3389/fimmu.2022.912069
Doğru, S., Yaşar, E., and Yeşilkaya, A. (2022). Uric acid can enhance MAPK pathway-mediated proliferation in rat primary vascular smooth muscle cells via controlling of mitochondria and caspase-dependent cell death. J. Recept Signal Transduct. Res. 42, 293–301. doi:10.1080/10799893.2021.1931320
Dong, F. J., and Dong, G. J. (2023). Research progress of traditional Chinesemedicine in improving hyperuricemia by regulating uric acid transporter. Shanghai J. Traditional Chin. Med. 57, 14–20. doi:10.16305/j.1007-1334.2023.22110341034
Du, Q., Meng, X., and Wang, S. (2022). A comprehensive review on the chemical properties, plant sources, pharmacological activities, pharmacokinetic and toxicological characteristics of tetrahydropalmatine. Front. Pharmacol. 13, 890078. doi:10.3389/fphar.2022.890078
Eckenstaler, R., and Benndorf, R. A. (2021). The role of ABCG2 in the pathogenesis of primary hyperuricemia and gout-an update. Int. J. Mol. Sci. 22, 6678. doi:10.3390/ijms22136678
Evangelisti, C., Chiarini, F., Cappellini, A., Paganelli, F., Fini, M., Santi, S., et al. (2020). Targeting Wnt/β-catenin and PI3K/Akt/mTOR pathways in T-cell acute lymphoblastic leukemia. J. Cell Physiol. 235, 5413–5428. doi:10.1002/jcp.29429
Fang, C., Chen, L., He, M., Luo, Y., Zhou, M., Zhang, N., et al. (2019). Molecular mechanistic insight into the anti-hyperuricemic effect of Eucommia ulmoides in mice and rats. Pharm. Biol. 57, 112–119. doi:10.1080/13880209.2019.1568510
Fu, X. L., Zhou, J., Tang, W. W., Liu, Y., Li, Z. L., Li, P., et al. (2021). Study on the compatibility effect and active constituents of atractylodis rhizoma in Ermiao wan against acute gouty arthritis. J. Ethnopharmacol. 279, 114353. doi:10.1016/j.jep.2021.114353
GarcíA-Arroyo, F. E., Gonzaga, G., MuñOZ-JiméNEZ, I., Blas-Marron, M. G., Silverio, O., Tapia, E., et al. (2018). Probiotic supplements prevented oxonic acid-induced hyperuricemia and renal damage. PLoS One 13, e0202901. doi:10.1371/journal.pone.0202901
GarcíA-Nieto, V. M., Claverie-MartíN, F., Moraleda-Mesa, T., Perdomo-RamíREZ, A., Tejera-CarreñO, P., Cordoba-Lanus, E., et al. (2022). Gout associated with reduced renal excretion of uric acid. Renal tubular disorder that nephrologists do not treat. Nefrol. Engl. Ed. 42, 273–279. doi:10.1016/j.nefroe.2022.05.007
Goldberg, E. L., Asher, J. L., Molony, R. D., Shaw, A. C., Zeiss, C. J., Wang, C., et al. (2017). β-Hydroxybutyrate deactivates neutrophil NLRP3 inflammasome to relieve gout flares. Cell Rep. 18, 2077–2087. doi:10.1016/j.celrep.2017.02.004
Gong, M., Zhang, H., Liu, X., Li, Q., Zhang, Y., Zhang, W., et al. (2022). Effect of Eucommia ulmoides leaves on hyperuricemia and kidney injury induced by a high-fat/high-fructose diet in rats. Iran. J. Basic Med. Sci. 25, 527–535. doi:10.22038/ijbms.2022.62681.13867
Gu, C., Hu, X., Shan, B., Wu, X., and Chen, J. (2023). Targeted and non-targeted metabolomics uncovering the effects of Er-Miao-Wan formula on rats with hyperuricemia. J. Pharm. Biomed. Anal. 226, 115246. doi:10.1016/j.jpba.2023.115246
Guo, M. X., Zhang, M. M., Yang, H. Y., Zhang, C. L., Cheng, H. Y., Li, N. Z., et al. (2022). Lagotis brachystachya maxim attenuates chronic alcoholic liver injury combined with gouty arthritis in rats via its anti-inflammatory activity. Front. Pharmacol. 13, 995777. doi:10.3389/fphar.2022.995777
Guo, X. L., Gao, Y. Y., Yang, Y. X., Zhu, Q. F., Guan, H. Y., He, X., et al. (2023). Amelioration effects of α-viniferin on hyperuricemia and hyperuricemia-induced kidney injury in mice. Phytomedicine 116, 154868. doi:10.1016/j.phymed.2023.154868
Guo, Y. J., Pan, W. W., Liu, S. B., Shen, Z. F., Xu, Y., and Hu, L. L. (2020). ERK/MAPK signalling pathway and tumorigenesis. Exp. Ther. Med. 19, 1997–2007. doi:10.3892/etm.2020.8454
Guo, H., German, P., Bai, S., Barnes, S., Guo, W., Qi, X., et al. (2015). The PI3K/AKT pathway and renal cell carcinoma. J. Genet. Genomics 42, 343–353. doi:10.1016/j.jgg.2015.03.003
Han, S., Wei, R., Han, D., Zhu, J., Luo, W., Ao, W., et al. (2020). Hypouricemic effects of extracts from urtica hyperborea jacq. Ex wedd. In hyperuricemia mice through XOD, URAT1, and OAT1. Biomed. Res. Int. 2020, 2968135. doi:10.1155/2020/2968135
Hoque, K. M., Dixon, E. E., Lewis, R. M., Allan, J., Gamble, G. D., Phipps-Green, A. J., et al. (2020). The ABCG2 Q141K hyperuricemia and gout associated variant illuminates the physiology of human urate excretion. Nat. Commun. 11, 2767. doi:10.1038/s41467-020-16525-w
Hua, L., Zhang, S., Yang, J., Zhou, X., and Shi, S. (2023). Sanghuangporus vaninii ethanol extract alleviates hyperuricemic renal injury by regulating the uric acid transporters and inhibiting HK-2 apoptosis. Biomed. Pharmacother. 164, 114970. doi:10.1016/j.biopha.2023.114970
Huang, J., Lin, Z., Wang, Y., Ding, X., and Zhang, B. (2023). Wuling san based on network pharmacology and in vivo evidence against hyperuricemia via improving oxidative stress and inhibiting inflammation. Drug Des. Devel Ther. 17, 675–690. doi:10.2147/dddt.S398625
Huang, L., Deng, J., Chen, G., Zhou, M., Liang, J., Yan, B., et al. (2019). The anti-hyperuricemic effect of four astilbin stereoisomers in Smilax glabra on hyperuricemic mice. J. Ethnopharmacol. 238, 111777. doi:10.1016/j.jep.2019.03.004
Hu, Y., Gui, Z., Zhou, Y., Xia, L., Lin, K., and Xu, Y. (2019). Quercetin alleviates rat osteoarthritis by inhibiting inflammation and apoptosis of chondrocytes, modulating synovial macrophages polarization to M2 macrophages. Free Radic. Biol. Med. 145, 146–160. doi:10.1016/j.freeradbiomed.2019.09.024
Hwang, H. S., Yang, C. M., Park, S. J., and Kim, H. A. (2015). Monosodium urate crystal-induced chondrocyte death via autophagic process. Int. J. Mol. Sci. 16, 29265–29277. doi:10.3390/ijms161226164
Jang, M. G., Song, H., Kim, J. H., Oh, J. M., Park, J. Y., Ko, H. C., et al. (2020). Prevention of hyperuricemia by Clerodendrum trichotomum leaf extract in potassium oxonate-induced mice. Dev. Reprod. 24, 89–100. doi:10.12717/dr.2020.24.2.89
Jeong, J., Lim, M. K., Han, E. H., Lee, S. H., Kang, S., and Lee, S. (2022). Extract of Aster glehni ameliorates potassium oxonate-induced hyperuricemia by modulating renal urate transporters and renal inflammation by suppressing TLR4/MyD88 signaling. Food Sci. Biotechnol. 31, 1729–1739. doi:10.1007/s10068-022-01153-5
Joosten, L. A. B., Crişan, T. O., Bjornstad, P., and Johnson, R. J. (2020). Asymptomatic hyperuricaemia: a silent activator of the innate immune system. Nat. Rev. Rheumatol. 16, 75–86. doi:10.1038/s41584-019-0334-3
Ke, J. T., Zhang, H., Bu, Y. H., Gan, P. R., Chen, F. Y., Dong, X. T., et al. (2022). Metabonomic analysis of abnormal sphingolipid metabolism in rheumatoid arthritis synovial fibroblasts in hypoxia microenvironment and intervention of geniposide. Front. Pharmacol. 13, 969408. doi:10.3389/fphar.2022.969408
Keller, S. F., and Mandell, B. F. (2021). Management and cure of gouty arthritis. Med. Clin. North Am. 105, 297–310. doi:10.1016/j.mcna.2020.09.013
Kim, O. K., Yun, J. M., Lee, M., Kim, D., and Lee, J. (2021). Hypouricemic effects of Chrysanthemum indicum L. And cornus officinalis on hyperuricemia-induced HepG2 cells, renal cells, and mice. Plants (Basel) 10, 1668. doi:10.3390/plants10081668
Kuo, T. M., Huang, C. M., Tu, H. P., Min-Shan Ko, A., Wang, S. J., Lee, C. P., et al. (2017). URAT1 inhibition by ALPK1 is associated with uric acid homeostasis. Rheumatol. Oxf. 56, 654–659. doi:10.1093/rheumatology/kew463
Lee, Y. S., Sung, Y. Y., Yuk, H. J., Son, E., Lee, S., Kim, J. S., et al. (2019). Anti-hyperuricemic effect of Alpinia oxyphylla seed extract by enhancing uric acid excretion in the kidney. Phytomedicine 62, 152975. doi:10.1016/j.phymed.2019.152975
Lepetsos, P., Papavassiliou, K. A., and Papavassiliou, A. G. (2019). Redox and NF-κB signaling in osteoarthritis. Free Radic. Biol. Med. 132, 90–100. doi:10.1016/j.freeradbiomed.2018.09.025
Li, C. Y., Wang, Q., Wang, X., Li, G., Shen, S., and Wei, X. (2019a). Scutellarin inhibits the invasive potential of malignant melanoma cells through the suppression epithelial-mesenchymal transition and angiogenesis via the PI3K/Akt/mTOR signaling pathway. Eur. J. Pharmacol. 858, 172463. doi:10.1016/j.ejphar.2019.172463
Li, H. X., Zhao, W., Shi, Y., Li, Y. N., Zhang, L. S., Zhang, H. Q., et al. (2015). Retinoic acid amide inhibits JAK/STAT pathway in lung cancer which leads to apoptosis. Tumour Biol. 36, 8671–8678. doi:10.1007/s13277-015-3534-8
Li, L. Z., Wang, X. M., Feng, X. J., Liu, K., Li, B., Zhu, L. J., et al. (2023a). Effects of a macroporous resin extract of dendrobium officinale leaves in rats with hyperuricemia induced by anthropomorphic unhealthy lifestyle. Evid. Based Complement. Altern. Med. 2023, 9990843. doi:10.1155/2023/9990843
Li, M. H., Guan, J., Chen, Z., Mo, J. X., Wu, K. R., Hu, X. G., et al. (2022a). Fufang Zhenzhu Tiaozhi capsule ameliorates hyperuricemic nephropathy by inhibition of PI3K/AKT/NF-κB pathway. J. Ethnopharmacol. 298, 115644. doi:10.1016/j.jep.2022.115644
Li, W. Y., Yang, F., Chen, J. H., and Ren, G. F. (2021b). β-Caryophyllene ameliorates MSU-induced gouty arthritis and inflammation through inhibiting NLRP3 and NF-κB signal pathway: in silico and in vivo. Front. Pharmacol. 12, 651305. doi:10.3389/fphar.2021.651305
Liang, D., Yong, T., Diao, X., Chen, S., Chen, D., Xiao, C., et al. (2021). Hypouricaemic and nephroprotective effects of Poria cocos in hyperuricemic mice by up-regulating ATP-binding cassette super-family G member 2. Pharm. Biol. 59, 275–286. doi:10.1080/13880209.2021.1885450
Liang, G., Nie, Y., Chang, Y., Zeng, S., Liang, C., Zheng, X., et al. (2019). Protective effects of Rhizoma smilacis glabrae extracts on potassium oxonate- and monosodium urate-induced hyperuricemia and gout in mice. Phytomedicine 59, 152772. doi:10.1016/j.phymed.2018.11.032
Li, F., Liu, Y., Xie, Y., Liu, Z., and Zou, G. (2020a). Epigallocatechin gallate reduces uric acid levels by regulating xanthine oxidase activity and uric acid excretion in vitro and in vivo. Ann. Palliat. Med. 9, 331–338. doi:10.21037/apm.2019.11.28
Li, G., Guan, C., Xu, L., Wang, L., Yang, C., Zhao, L., et al. (2020b). Scutellarin ameliorates renal injury via increasing CCN1 expression and suppressing NLRP3 inflammasome activation in hyperuricemic mice. Front. Pharmacol. 11, 584942. doi:10.3389/fphar.2020.584942
Li, H., Ou, G., He, Y., Ren, L., Yang, X., and Zeng, M. (2019b). Resveratrol attenuates the MSU crystal-induced inflammatory response through the inhibition of TAK1 activity. Int. Immunopharmacol. 67, 62–68. doi:10.1016/j.intimp.2018.12.004
Li, L., Zhang, Y., and Zeng, C. (2020c). Update on the epidemiology, genetics, and therapeutic options of hyperuricemia. Am. J. Transl. Res. 12, 3167–3181.
Lin, K. M., Lu, C. L., Hung, K. C., Wu, P. C., Pan, C. F., Wu, C. J., et al. (2019). The paradoxical role of uric acid in osteoporosis. Nutrients 11, 2111. doi:10.3390/nu11092111
Lin, G., Yu, Q., Xu, L., Huang, Z., Mai, L., Jiang, L., et al. (2021). Berberrubine attenuates potassium oxonate- and hypoxanthine-induced hyperuricemia by regulating urate transporters and JAK2/STAT3 signaling pathway. Eur. J. Pharmacol. 912, 174592. doi:10.1016/j.ejphar.2021.174592
Lin, S., Meng, J., Li, F., Yu, H., Lin, D., Lin, S., et al. (2022). Ganoderma lucidum polysaccharide peptide alleviates hyperuricemia by regulating adenosine deaminase and urate transporters. Food Funct. 13, 12619–12631. doi:10.1039/d2fo02431d
Lin, X., Shao, T., Huang, L., Wen, X., Wang, M., Wen, C., et al. (2020a). Simiao decoction alleviates gouty arthritis by modulating proinflammatory cytokines and the gut ecosystem. Front. Pharmacol. 11, 955. doi:10.3389/fphar.2020.00955
Lin, X., Zhou, Q., Zhou, L., Sun, Y., Han, X., Cheng, X., et al. (2023). Quinoa (Chenopodium quinoa Willd) bran saponins alleviate hyperuricemia and inhibit renal injury by regulating the PI3K/AKT/NFκB signaling pathway and uric acid transport. J. Agric. Food Chem. 71, 6635–6649. doi:10.1021/acs.jafc.3c00088
Lin, Y., Luo, T., Weng, A., Huang, X., Yao, Y., Fu, Z., et al. (2020b). Gallic acid alleviates gouty arthritis by inhibiting NLRP3 inflammasome activation and pyroptosis through enhancing Nrf2 signaling. Front. Immunol. 11, 580593. doi:10.3389/fimmu.2020.580593
Li, Q., Huang, Z., Liu, D., Zheng, J., Xie, J., Chen, J., et al. (2021a). Effect of berberine on hyperuricemia and kidney injury: a network pharmacology analysis and experimental validation in a mouse model. Drug Des. Devel Ther. 15, 3241–3254. doi:10.2147/dddt.S317776
Li, Q., Liu, P., Wu, C., Bai, L., Zhang, Z., Bao, Z., et al. (2022b). Integrating network pharmacology and pharmacological validation to explore the effect of Shi Wei Ru Xiang powder on suppressing hyperuricemia. J. Ethnopharmacol. 298, 115679. doi:10.1016/j.jep.2022.115679
Liu, N., Feng, X., Wang, W., Zhao, X., and Li, X. (2017a). Paeonol protects against TNF-α-induced proliferation and cytokine release of rheumatoid arthritis fibroblast-like synoviocytes by upregulating FOXO3 through inhibition of miR-155 expression. Inflamm. Res. 66, 603–610. doi:10.1007/s00011-017-1041-7
Liu, P., Xu, Y., Ye, J., Tan, J., Hou, J., Wang, Y., et al. (2023). Qingre Huazhuo Jiangsuan Decoction promotes autophagy by inhibiting PI3K/AKT/mTOR signaling pathway to relieve acute gouty arthritis. J. Ethnopharmacol. 302, 115875. doi:10.1016/j.jep.2022.115875
Liu, T., Gao, H., Zhang, Y., Wang, S., Lu, M., Dai, X., et al. (2022a). Apigenin ameliorates hyperuricemia and renal injury through regulation of uric acid metabolism and JAK2/STAT3 signaling pathway. Pharm. (Basel) 15, 1442. doi:10.3390/ph15111442
Liu, W., Wu, Y. H., Xue, B., Jin, Y., Zhang, S. M., Li, P. H., et al. (2021). Effect of integrated traditional Chinese and western medicine on gout. J. Tradit. Chin. Med. 41, 806–816. doi:10.19852/j.cnki.jtcm.20210702.001
Liu, Y., Gong, S., Li, K., Wu, G., Zheng, X., Zheng, J., et al. (2022b). Coptisine protects against hyperuricemic nephropathy through alleviating inflammation, oxidative stress and mitochondrial apoptosis via PI3K/Akt signaling pathway. Biomed. Pharmacother. 156, 113941. doi:10.1016/j.biopha.2022.113941
Liu, Y., Wang, L., Du, Y., Chen, Z., Guo, J., Weng, X., et al. (2017b). Effects of apigenin pretreatment against renal ischemia/reperfusion injury via activation of the JAK2/STAT3 pathway. Biomed. Pharmacother. 95, 1799–1808. doi:10.1016/j.biopha.2017.09.091
Li, X., Gao, J., and Tao, J. (2021c). Purinergic signaling in the regulation of gout flare and resolution. Front. Immunol. 12, 785425. doi:10.3389/fimmu.2021.785425
Li, X., Sun, J., Bu, Q., Zhou, B., Li, L., Man, X., et al. (2023b). Association between serum uric acid levels and clinical outcomes in patients with acute kidney injury. Ren. Fail 45, 2169617. doi:10.1080/0886022x.2023.2169617
Li, X., Xu, D. Q., Sun, D. Y., Zhang, T., He, X., and Xiao, D. M. (2019c). Curcumin ameliorates monosodium urate-induced gouty arthritis through Nod-like receptor 3 inflammasome mediation via inhibiting nuclear factor-kappa B signaling. J. Cell Biochem. 120, 6718–6728. doi:10.1002/jcb.27969
Li, X., Yan, Z., CarlströM, M., Tian, J., Zhang, X., Zhang, W., et al. (2020d). Mangiferin ameliorates hyperuricemic nephropathy which is associated with downregulation of AQP2 and increased urinary uric acid excretion. Front. Pharmacol. 11, 49. doi:10.3389/fphar.2020.00049
Lou, D., Zhang, X., Jiang, C., Zhang, F., Xu, C., Fang, S., et al. (2022). 3β,23-Dihydroxy-12-ene-28-ursolic acid isolated from cyclocarya paliurus alleviates NLRP3 inflammasome-mediated gout via PI3K-AKT-mTOR-Dependent autophagy. Evid. Based Complement. Altern. Med. 2022, 5541232. doi:10.1155/2022/5541232
Lu, J., Dalbeth, N., Yin, H., Li, C., Merriman, T. R., and Wei, W. H. (2019). Mouse models for human hyperuricaemia: a critical review. Nat. Rev. Rheumatol. 15, 413–426. doi:10.1038/s41584-019-0222-x
Luo, X., Zhou, L., Wang, S., Yuan, J., Chang, Z., Hu, Q., et al. (2022). The therapeutic effect and the potential mechanism of flavonoids and phenolics of moringa oleifera lam. Leaves against hyperuricemia mice. Molecules 27, 8237. doi:10.3390/molecules27238237
LüSCHER, B. P., Albrecht, C., Stieger, B., Surbek, D. V., and Baumann, M. U. (2022). Glucose transporter 9 (GLUT9) plays an important role in the placental uric acid transport system. Cells 11, 633. doi:10.3390/cells11040633
Maiuolo, J., Oppedisano, F., Gratteri, S., Muscoli, C., and Mollace, V. (2016). Regulation of uric acid metabolism and excretion. Int. J. Cardiol. 213, 8–14. doi:10.1016/j.ijcard.2015.08.109
Major, T. J., Dalbeth, N., Stahl, E. A., and Merriman, T. R. (2018). An update on the genetics of hyperuricaemia and gout. Nat. Rev. Rheumatol. 14, 341–353. doi:10.1038/s41584-018-0004-x
Ma, N., Zhang, Y., Wang, T., Sun, Y., and Cai, S. (2023). The preventive effect of Chinese sumac fruit against monosodium urate-induced gouty arthritis in rats by regulating several inflammatory pathways. Food Funct. 14, 1148–1159. doi:10.1039/d2fo02860c
Manning, B. D., and Toker, A. (2017). AKT/PKB signaling: navigating the network. Cell 169, 381–405. doi:10.1016/j.cell.2017.04.001
MéNDEZ-Salazar, E. O., and MartíNEZ-Nava, G. A. (2022). Uric acid extrarenal excretion: the gut microbiome as an evident yet understated factor in gout development. Rheumatol. Int. 42, 403–412. doi:10.1007/s00296-021-05007-x
Meng, J., Tian, J., Zhao, Y., Li, C., Yi, Y., Zhang, Y., et al. (2023). Ameliorative effect of cheqianzi decoction on hyperuricemia and kidney injury and underlying mechanism in rats. Heliyon 9, e15333. doi:10.1016/j.heliyon.2023.e15333
Meng, Q., Meng, W., Bian, H., Zheng, F., Gu, H., Zuo, R., et al. (2021). Total glucosides of paeony protects THP-1 macrophages against monosodium urate-induced inflammation via MALAT1/miR-876-5p/NLRP3 signaling cascade in gouty arthritis. Biomed. Pharmacother. 138, 111413. doi:10.1016/j.biopha.2021.111413
Merriman, T. R. (2015). An update on the genetic architecture of hyperuricemia and gout. Arthritis Res. Ther. 17, 98. doi:10.1186/s13075-015-0609-2
Mitchell, S., Vargas, J., and Hoffmann, A. (2016). Signaling via the NFκB system. Wiley Interdiscip Rev Syst Biol Med,8, 227–241. doi:10.1002/wsbm.1331
Morris, R., Kershaw, N. J., and Babon, J. J. (2018). The molecular details of cytokine signaling via the JAK/STAT pathway. Protein Sci. 27, 1984–2009. doi:10.1002/pro.3519
Nie, Q., Liu, M., Zhang, Z., Zhang, X., Wang, C., and Song, G. (2021). The effects of hyperuricemia on endothelial cells are mediated via GLUT9 and the JAK2/STAT3 pathway. Mol. Biol. Rep. 48, 8023–8032. doi:10.1007/s11033-021-06840-w
Niu, Y., Li, Q., Tu, C., Li, N., Gao, L., Lin, H., et al. (2023). Hypouricemic actions of the pericarp of mangosteen in vitro and in vivo. J. Nat. Prod. 86, 24–33. doi:10.1021/acs.jnatprod.2c00531
Novikov, A., Fu, Y., Huang, W., Freeman, B., Patel, R., Van Ginkel, C., et al. (2019). SGLT2 inhibition and renal urate excretion: role of luminal glucose, GLUT9, and URAT1. Am. J. Physiol. Ren. Physiol. 316, F173–f185. doi:10.1152/ajprenal.00462.2018
Oğuz, N., KıRçA, M., Çetin, A., and Yeşilkaya, A. (2017). Effect of uric acid on inflammatory COX-2 and ROS pathways in vascular smooth muscle cells. J. Recept Signal Transduct. Res. 37, 500–505. doi:10.1080/10799893.2017.1360350
Oh, C. C., Lee, J., D'Souza, K., Zhang, W., Migrino, R. Q., Thornburg, K., et al. (2019). Activator protein-1 and caspase 8 mediate p38α MAPK-dependent cardiomyocyte apoptosis induced by palmitic acid. Apoptosis 24, 395–403. doi:10.1007/s10495-018-01510-y
O'Shea, J. J., Schwartz, D. M., Villarino, A. V., Gadina, M., Mcinnes, I. B., and Laurence, A. (2015). The JAK-STAT pathway: impact on human disease and therapeutic intervention. Annu. Rev. Med. 66, 311–328. doi:10.1146/annurev-med-051113-024537
Ota-Kontani, A., Hirata, H., Ogura, M., Tsuchiya, Y., and Harada-Shiba, M. (2020). Comprehensive analysis of mechanism underlying hypouricemic effect of glucosyl hesperidin. Biochem. Biophys. Res. Commun. 521, 861–867. doi:10.1016/j.bbrc.2019.10.199
Pan, J., Zhang, C., Shi, M., Guo, F., Liu, J., Li, L., et al. (2021). Ethanol extract of Liriodendron chinense (Hemsl.) Sarg barks attenuates hyperuricemic nephropathy by inhibiting renal fibrosis and inflammation in mice. J. Ethnopharmacol. 264, 113278. doi:10.1016/j.jep.2020.113278
Peng, Y. J., Lu, J. W., Liu, F. C., Lee, C. H., Lee, H. S., Ho, Y. J., et al. (2020). Astaxanthin attenuates joint inflammation induced by monosodium urate crystals. Faseb J. 34, 11215–11226. doi:10.1096/fj.202000558RR
Perez-Ruiz, F., Dalbeth, N., and Bardin, T. (2015). A review of uric acid, crystal deposition disease, and gout. Adv. Ther. 32, 31–41. doi:10.1007/s12325-014-0175-z
Qaseem, A., Harris, R. P., Forciea, M. A., Denberg, T. D., Barry, M. J., Boyd, C., et al. (2017). Management of acute and recurrent gout: a clinical practice guideline from the American college of physicians. Ann. Intern Med. 166, 58–68. doi:10.7326/m16-0570
Qian, X., Wang, X., Luo, J., Liu, Y., Pang, J., Zhang, H., et al. (2019). Hypouricemic and nephroprotective roles of anthocyanins in hyperuricemic mice. Food Funct. 10, 867–878. doi:10.1039/c8fo02124d
Ren, L., Li, Q., Li, H., Zhan, X., Yang, R., Li, Z., et al. (2022). Polysaccharide extract from Isatidis Radix inhibits multiple inflammasomes activation and alleviate gouty arthritis. Phytother. Res. 36, 3295–3312. doi:10.1002/ptr.7514
Ren, Q., Tao, S., Guo, F., Wang, B., Yang, L., Ma, L., et al. (2021). Natural flavonol fisetin attenuated hyperuricemic nephropathy via inhibiting IL-6/JAK2/STAT3 and TGF-β/SMAD3 signaling. Phytomedicine 87, 153552. doi:10.1016/j.phymed.2021.153552
Riaz, M., Al Kury, L. T., Atzaz, N., Alattar, A., Alshaman, R., Shah, F. A., et al. (2022). Carvacrol alleviates hyperuricemia-induced oxidative stress and inflammation by modulating the NLRP3/NF-κB pathwayt. Drug Des. Devel Ther. 16, 1159–1170. doi:10.2147/dddt.S343978
Richette, P., Doherty, M., Pascual, E., Barskova, V., Becce, F., Castaneda, J., et al. (2020). 2018 updated European League against Rheumatism evidence-based recommendations for the diagnosis of gout. Ann. Rheum. Dis. 79, 31–38. doi:10.1136/annrheumdis-2019-215315
Saito, T., and Tanaka, S. (2017). Molecular mechanisms underlying osteoarthritis development: notch and NF-κB. Arthritis Res. Ther. 19, 94. doi:10.1186/s13075-017-1296-y
Savage, K. T., Santillan, M. R., Flood, K. S., Charrow, A., Porter, M. L., and Kimball, A. B. (2020). Tofacitinib shows benefit in conjunction with other therapies in recalcitrant hidradenitis suppurativa patients. JAAD Case Rep. 6, 99–102. doi:10.1016/j.jdcr.2019.10.010
Shields, G. E., and Beard, S. M. (2015). A systematic review of the economic and humanistic burden of gout. Pharmacoeconomics 33, 1029–1047. doi:10.1007/s40273-015-0288-5
Shin, J. I., Lee, K. H., Joo, Y. H., Lee, J. M., Jeon, J., Jung, H. J., et al. (2019). Inflammasomes and autoimmune and rheumatic diseases: a comprehensive review. J. Autoimmun. 103, 102299. doi:10.1016/j.jaut.2019.06.010
So, A. K., and Martinon, F. (2017). Inflammation in gout: mechanisms and therapeutic targets. Nat. Rev. Rheumatol. 13, 639–647. doi:10.1038/nrrheum.2017.155
Song, S., Fan, M., Wen, X., Shi, X., Lou, Y., He, Z., et al. (2023). Integrated network pharmacology and gut microbiome analysis to reveal the mechanism of Qu-Zhuo-Tong-Bi decoction against hyperuricemia and gout. J. Ethnopharmacol. 316, 116736. doi:10.1016/j.jep.2023.116736
Stramucci, L., Pranteda, A., and Bossi, G. (2018). Insights of crosstalk between p53 protein and the MKK3/MKK6/p38 MAPK signaling pathway in cancer. Cancers (Basel) 10, 131. doi:10.3390/cancers10050131
Sun, X., Li, P., Qu, X., and Liu, W. (2021). Isovitexin alleviates acute gouty arthritis in rats by inhibiting inflammation via the TLR4/MyD88/NF-κB pathway. Pharm. Biol. 59, 1326–1333. doi:10.1080/13880209.2021.1979595
Tan, P. K., Liu, S., Gunic, E., and Miner, J. N. (2017). Discovery and characterization of verinurad, a potent and specific inhibitor of URAT1 for the treatment of hyperuricemia and gout. Sci. Rep. 7, 665. doi:10.1038/s41598-017-00706-7
Tao, J. H., Cheng, M., Tang, J. P., Dai, X. J., Zhang, Y., Li, X. P., et al. (2017). Single nucleotide polymorphisms associated with P2X7R function regulate the onset of gouty arthritis. PLoS One 12, e0181685. doi:10.1371/journal.pone.0181685
Toyoda, Y., MančíKOVá, A., Krylov, V., Morimoto, K., Pavelcová, K., Bohatá, J., et al. (2019). Functional characterization of clinically-relevant rare variants in ABCG2 identified in a gout and hyperuricemia cohort. Cells 8, 363. doi:10.3390/cells8040363
Wang, C. C., Lu, J. W., Peng, Y. J., Lee, C. H., Lee, H. S., Chu, Y. H., et al. (2021a). Ameliorative effects of cardamonin on monosodium urate-induced gouty arthritis through inhibiting NLRP3 inflammasome mediation. Med. Kaunas. 57, 898. doi:10.3390/medicina57090898
Wang, H. B., Huang, S. H., Xu, M., Yang, J., Yang, J., Liu, M. X., et al. (2019). Galangin ameliorates cardiac remodeling via the MEK1/2-ERK1/2 and PI3K-AKT pathways. J. Cell Physiol. 234, 15654–15667. doi:10.1002/jcp.28216
Wang, M. X., Wang, M. M., Liu, C., Chen, J. S., Liu, J. S., Guo, X., et al. (2022a). A geniposide-phospholipid complex ameliorates posthyperuricemia chronic kidney disease induced by inflammatory reactions and oxidative stress. Eur. J. Pharmacol. 930, 175157. doi:10.1016/j.ejphar.2022.175157
Wang, X. J., Qi, Y. D., Guan, H. C., Lin, H. G., He, P. Q., Guan, K. W., et al. (2021c). Gegen qinlian decoction ameliorates hyperuricemia-induced renal tubular injury via blocking the inflammatory signaling pathway. Front. Pharmacol. 12, 665398. doi:10.3389/fphar.2021.665398
Wang, B., Suen, C. W., Ma, H., Wang, Y., Kong, L., Qin, D., et al. (2020a). The roles of H19 in regulating inflammation and aging. Front. Immunol. 11, 579687. doi:10.3389/fimmu.2020.579687
Wang, L., Zhu, L., Duan, C., Li, L., and Chen, G. (2020b). Total saponin of Dioscorea collettii attenuates MSU crystal-induced inflammation via inhibiting the activation of the NALP3 inflammasome and caspase-1 in THP-1 macrophages. Mol. Med. Rep. 21, 2466–2474. doi:10.3892/mmr.2020.11035
Wang, Q., Lin, B., Li, Z., Su, J., and Feng, Y. (2021b). Cichoric acid ameliorates monosodium urate-induced inflammatory response by reducing NLRP3 inflammasome activation via inhibition of NF-kB signaling pathway. Evid. Based Complement. Altern. Med. 2021, 8868527. doi:10.1155/2021/8868527
Wang, S., Liu, W., Li, D., and Wang, Z. (2018). Effects of Qingre hua zhuo jiang acid recipe on serum IL-1β, IL-6 and IL-8 levels in patients with acute gouty arthritis. Shandong Med. J. 58, 93–95. doi:10.3969/j.issn.1002-266X.2018.02.032
Wang, X., and Chen, D. (2018). Purinergic regulation of neutrophil function. Front. Immunol. 9, 399. doi:10.3389/fimmu.2018.00399
Wang, X., Long, H., Chen, M., Zhou, Z., Wu, Q., Xu, S., et al. (2022b). Modified Baihu decoction therapeutically remodels gut microbiota to inhibit acute gouty arthritis. Front. Physiol. 13, 1023453. doi:10.3389/fphys.2022.1023453
Wang, Y., Kong, W., Wang, L., Zhang, T., Huang, B., Meng, J., et al. (2020c). Multiple-purpose connectivity map analysis reveals the benefits of esculetin to hyperuricemia and renal fibrosis. Int. J. Mol. Sci. 21, 7695. doi:10.3390/ijms21207695
Wang, Y., Wu, H., Deng, R., Dai, X. J., Bu, Y. H., Sun, M. H., et al. (2021d). Geniposide downregulates the VEGF/SphK1/S1P pathway and alleviates angiogenesis in rheumatoid arthritis in vivo and in vitro. Phytother. Res. 35, 4347–4362. doi:10.1002/ptr.7130
Wang, Y., Wu, H., Gui, B. J., Liu, J., Rong, G. X., Deng, R., et al. (2022c). Geniposide alleviates VEGF-induced angiogenesis by inhibiting VEGFR2/PKC/ERK1/2-mediated SphK1 translocation. Phytomedicine 100, 154068. doi:10.1016/j.phymed.2022.154068
Wang, Y., Zhu, W., Lu, D., Zhang, C., and Wang, Y. (2021e). Tetrahydropalmatine attenuates MSU crystal-induced gouty arthritis by inhibiting ROS-mediated NLRP3 inflammasome activation. Int. Immunopharmacol. 100, 108107. doi:10.1016/j.intimp.2021.108107
Wei, S. J., Zhang, Q., Xiang, Y. J., Peng, L. Y., Peng, W., Ren, Q., et al. (2021). Guizhi-Shaoyao-Zhimu decoction attenuates bone erosion in rats that have collagen-induced arthritis via modulating NF-κB signalling to suppress osteoclastogenesis. Pharm. Biol. 59, 262–274. doi:10.1080/13880209.2021.1876100
Wei-Yun, B., and Cailin, Z. (2021). Genistein ameliorates hyperuricemia-associated nephropathy in hyperuricemic mice. Food Agric. Immunol. 32, 778–797. doi:10.1080/09540105.2021.1996540
Woodward, O. M. (2015). ABCG2: the molecular mechanisms of urate secretion and gout. Am. J. Physiol. Ren. Physiol. 309, F485–F488. doi:10.1152/ajprenal.00242.2015
Wu, J., Li, J., Li, W., Sun, B., Xie, J., Cheng, W., et al. (2018). Achyranthis bidentatae radix enhanced articular distribution and anti-inflammatory effect of berberine in Sanmiao Wan using an acute gouty arthritis rat model. J. Ethnopharmacol. 221, 100–108. doi:10.1016/j.jep.2018.04.025
Wu, J., Luo, Y., Jiang, Q., Li, S., Huang, W., Xiang, L., et al. (2019). Coptisine from Coptis chinensis blocks NLRP3 inflammasome activation by inhibiting caspase-1. Pharmacol. Res. 147, 104348. doi:10.1016/j.phrs.2019.104348
Wu, M., Ma, Y., Chen, X., Liang, N., Qu, S., and Chen, H. (2021). Hyperuricemia causes kidney damage by promoting autophagy and NLRP3-mediated inflammation in rats with urate oxidase deficiency. Dis. Model Mech. 14, dmm048041. doi:10.1242/dmm.048041
Wu, W., Bush, K. T., and Nigam, S. K. (2017). Key role for the organic anion transporters, OAT1 and OAT3, in the in vivo handling of uremic toxins and solutes. Sci. Rep. 7, 4939. doi:10.1038/s41598-017-04949-2
Xiao, Y. Z., Ye, Z. Z., Liang, Y. T., Chen, X. P., Wang, Y. H., Xu, Q., et al. (2021). Association between Chinese herbal medicine therapy and the risk of chronic kidney disease in gout patients. Front. Pharmacol. 12, 661282. doi:10.3389/fphar.2021.661282
Xiao, L., Lin, S., Xu, W., and Sun, E. (2023). Downregulation of Sox8 mediates monosodium urate crystal-induced autophagic impairment of cartilage in gout arthritis. Cell Death Discov. 9, 95. doi:10.1038/s41420-023-01388-z
Xiao, N., Chen, H., He, S. Y., Xue, C. X., Sui, H., Chen, J., et al. 2018. Evaluating the efficacy and adverse effects of clearing heat and removing dampness method of traditional Chinese medicine by comparison with western medicine in patients with gout. Evid. Based Complement. Altern. Med., 2018, , 8591349.doi:10.1155/2018/8591349
Xin, P., Xu, X., Deng, C., Liu, S., Wang, Y., Zhou, X., et al. (2020). The role of JAK/STAT signaling pathway and its inhibitors in diseases. Int. Immunopharmacol. 80, 106210. doi:10.1016/j.intimp.2020.106210
Xu, L., Cheng, J., Lu, J., Lin, G., Yu, Q., Li, Y., et al. (2022a). Integrating network pharmacology and experimental validation to clarify the anti-hyperuricemia mechanism of cortex phellodendri in mice. Front. Pharmacol. 13, 964593. doi:10.3389/fphar.2022.964593
Xu, L., Lin, G., Yu, Q., Li, Q., Mai, L., Cheng, J., et al. (2021). Anti-hyperuricemic and nephroprotective effects of dihydroberberine in potassium oxonate- and hypoxanthine-induced hyperuricemic mice. Front. Pharmacol. 12, 645879. doi:10.3389/fphar.2021.645879
Xu, L., Liu, X., Zhang, Y., Jia, T., Li, L., Du, Y., et al. (2022b). Tanshinone IIA improves acute gouty arthritis in rats through regulating neutrophil activation and the NLRP3 inflammasome. Dis. Markers 2022, 5851412. doi:10.1155/2022/5851412
Yan, C. Y., Ouyang, S. H., Wang, X., Wu, Y. P., Sun, W. Y., Duan, W. J., et al. (2021). Celastrol ameliorates Propionibacterium acnes/LPS-induced liver damage and MSU-induced gouty arthritis via inhibiting K63 deubiquitination of NLRP3. Phytomedicine 80 153398. doi:10.1016/j.phymed.2020.153398
Yang, B., Xin, M., Liang, S., Huang, Y., Li, J., Wang, C., et al. (2023). Naringenin ameliorates hyperuricemia by regulating renal uric acid excretion via the PI3K/AKT signaling pathway and renal inflammation through the NF-κB signaling pathway. J. Agric. Food Chem. 71, 1434–1446. doi:10.1021/acs.jafc.2c01513
Yang, F., Shi, W., Wang, L., Qin, N., Wang, C., Guo, Y., et al. (2021a). Lipidomics study of the therapeutic mechanism of Plantaginis Semen in potassium oxonate-induced hyperuricemia rat. BMC Complement. Med. Ther. 21, 175. doi:10.1186/s12906-021-03350-x
Yang, J., Chen, G., Guo, T. W., Qin, W. Y., and Jia, P. (2021b). Simiao Wan attenuates monosodium urate crystal-induced arthritis in rats through contributing to macrophage M2 polarization. J. Ethnopharmacol. 275, 114123. doi:10.1016/j.jep.2021.114123
Yang, Y., Wang, H., Kouadir, M., Song, H., and Shi, F. (2019). Recent advances in the mechanisms of NLRP3 inflammasome activation and its inhibitors. Cell Death Dis. 10, 128. doi:10.1038/s41419-019-1413-8
Yang, Y., Zhang, D. M., Liu, J. H., Hu, L. S., Xue, Q. C., Ding, X. Q., et al. (2015). Wuling San protects kidney dysfunction by inhibiting renal TLR4/MyD88 signaling and NLRP3 inflammasome activation in high fructose-induced hyperuricemic mice. J. Ethnopharmacol. 169, 49–59. doi:10.1016/j.jep.2015.04.011
Ye, J., Zeng, Z., Chen, Y., Wu, Z., Yang, Q., and Sun, T. (2022). Examining an association of single nucleotide polymorphisms with hyperuricemia in Chinese flight attendants. Pharmgenomics Pers. Med. 15, 589–602. doi:10.2147/pgpm.S364206
Yin, C., Liu, B., Wang, P., Li, X., Li, Y., Zheng, X., et al. (2020). Eucalyptol alleviates inflammation and pain responses in a mouse model of gout arthritis. Br. J. Pharmacol. 177, 2042–2057. doi:10.1111/bph.14967
Yin, H., Liu, N., and Chen, J. (2022). The role of the intestine in the development of hyperuricemia. Front. Immunol. 13, 845684. doi:10.3389/fimmu.2022.845684
Yu, Z., Bao, X., and Ken, J. (2022). Research status of Tibetan medicine in treatment of gout. Mod. Chin. Med. 24, 1212–1218. doi:10.13313/j.issn.1673-4890.20210623002
Zaninelli, T. H., Fattori, V., Saraiva-Santos, T., Badaro-Garcia, S., Staurengo-Ferrari, L., Andrade, K. C., et al. (2022). RvD1 disrupts nociceptor neuron and macrophage activation and neuroimmune communication, reducing pain and inflammation in gouty arthritis in mice. Br. J. Pharmacol. 179, 4500–4515. doi:10.1111/bph.15897
Zhang, A. H., Yu, J. B., Sun, H., Kong, L., Wang, X. Q., Zhang, Q. Y., et al. (2018a). Identifying quality-markers from Shengmai San protects against transgenic mouse model of Alzheimer's disease using chinmedomics approach. Phytomedicine 45, 84–92. doi:10.1016/j.phymed.2018.04.004
Zhang, M. Q., Sun, K. X., Guo, X., Chen, Y. Y., Feng, C. Y., Chen, J. S., et al. (2023a). The antihyperuricemia activity of Astragali Radix through regulating the expression of uric acid transporters via PI3K/Akt signalling pathway. J. Ethnopharmacol. 317, 116770. doi:10.1016/j.jep.2023.116770
Zhang, Q. B., Zhu, D., Dai, F., Huang, Y. Q., Zheng, J. X., Tang, Y. P., et al. (2021a). MicroRNA-223 suppresses IL-1β and TNF-α production in gouty inflammation by targeting the NLRP3 inflammasome. Front. Pharmacol. 12, 637415. doi:10.3389/fphar.2021.637415
Zhang, Y. K., Chen, J. S., Wang, M. M., Wang, C. Z., Wang, M. X., Wang, Z., et al. (2022b). Synthesis and bioactivity evaluation of novel nuciferine derivatives with antihyperuricemia and nephroprotective effects. Bioorg Chem. 126, 105916. doi:10.1016/j.bioorg.2022.105916
Zhang, C., Li, L., Zhang, Y., and Zeng, C. (2020). Recent advances in fructose intake and risk of hyperuricemia. Biomed. Pharmacother. 131, 110795. doi:10.1016/j.biopha.2020.110795
Zhang, H., Huang, X., Shi, Y., Liu, W., and He, M. (2018b). Identification and analysis of an MKK4 homologue in response to the nucleus grafting operation and antigens in the pearl oyster, Pinctada fucata. Fish. Shellfish Immunol. 73, 279–287. doi:10.1016/j.fsi.2017.12.023
Zhang, L., Xiao, X., Arnold, P. R., and Li, X. C. (2019a). Transcriptional and epigenetic regulation of immune tolerance: roles of the NF-κB family members. Cell Mol. Immunol. 16, 315–323. doi:10.1038/s41423-019-0202-8
Zhang, Q., Peng, W., Wei, S., Wei, D., Li, R., Liu, J., et al. (2019b). Guizhi-Shaoyao-Zhimu decoction possesses anti-arthritic effects on type II collagen-induced arthritis in rats via suppression of inflammatory reactions, inhibition of invasion & migration and induction of apoptosis in synovial fibroblasts. Biomed. Pharmacother. 118, 109367. doi:10.1016/j.biopha.2019.109367
Zhang, Q., Wang, L., Wang, S., Cheng, H., Xu, L., Pei, G., et al. (2022a). Signaling pathways and targeted therapy for myocardial infarction. Signal Transduct. Target Ther. 7, 78. doi:10.1038/s41392-022-00925-z
Zhang, X., Liu, J., Sun, Y., Zhou, Q., Ding, X., and Chen, X. (2023b). Chinese herbal compound Huangqin Qingrechubi capsule reduces lipid metabolism disorder and inflammatory response in gouty arthritis via the LncRNA H19/APN/PI3K/AKT cascade. Pharm. Biol. 61, 541–555. doi:10.1080/13880209.2023.2191641
Zhang, X., Nie, Q., Zhang, Z., Zhao, J., Zhang, F., Wang, C., et al. (2021b). Resveratrol affects the expression of uric acid transporter by improving inflammation. Mol. Med. Rep. 24, 564. doi:10.3892/mmr.2021.12203
Zhang, Y., Tan, X., Lin, Z., Li, F., Yang, C., Zheng, H., et al. (2021c). Fucoidan from laminaria japonica inhibits expression of GLUT9 and URAT1 via PI3K/akt, JNK and NF-κB pathways in uric acid-exposed HK-2 cells. Mar. Drugs 19, 238. doi:10.3390/md19050238
Zhang, Y., Wang, S., Dai, X., Liu, T., Liu, Y., Shi, H., et al. (2023c). Simiao San alleviates hyperuricemia and kidney inflammation by inhibiting NLRP3 inflammasome and JAK2/STAT3 signaling in hyperuricemia mice. J. Ethnopharmacol. 312, 116530. doi:10.1016/j.jep.2023.116530
Zhan, X., Li, Q., Xu, G., Xiao, X., and Bai, Z. (2022). The mechanism of NLRP3 inflammasome activation and its pharmacological inhibitors. Front. Immunol. 13, 1109938. doi:10.3389/fimmu.2022.1109938
Zheng, J., Gong, S., Wu, G., Zheng, X., Li, J., Nie, J., et al. (2023). Berberine attenuates uric acid-induced cell injury by inhibiting NLRP3 signaling pathway in HK-2 cells. Naunyn Schmiedeb. Arch. Pharmacol. 396, 2405–2416. doi:10.1007/s00210-023-02451-3
Zhou, G. Q., Chen, G., Yang, J., Qin, W. Y., and Ping, J. (2022a). Guizhi-Shaoyao-Zhimu decoction attenuates monosodium urate crystal-induced inflammation through inactivation of NF-κB and NLRP3 inflammasome. J. Ethnopharmacol. 283, 114707. doi:10.1016/j.jep.2021.114707
Zhou, J., Wang, C., Zhang, X., Wu, Z., Wu, Y., Li, D., et al. (2023). Shizhifang ameliorates pyroptosis of renal tubular epithelial cells in hyperuricemia through inhibiting NLRP3 inflammasome. J. Ethnopharmacol. 317, 116777. doi:10.1016/j.jep.2023.116777
Zhou, Q., Sun, H. J., Liu, S. M., Jiang, X. H., Wang, Q. Y., Zhang, S., et al. (2020). Anti-inflammation effects of the total saponin fraction from Dioscorea nipponica Makino on rats with gouty arthritis by influencing MAPK signalling pathway. BMC Complement. Med. Ther. 20, 261. doi:10.1186/s12906-020-03055-7
Zhou, X., Zhang, B., Zhao, X., Lin, Y., Wang, J., Wang, X., et al. (2021). Chlorogenic acid supplementation ameliorates hyperuricemia, relieves renal inflammation, and modulates intestinal homeostasis. Food Funct. 12, 5637–5649. doi:10.1039/d0fo03199b
Zhou, X., Zhang, B., Zhao, X., Lin, Y., Zhuang, Y., Guo, J., et al. (2022b). Chlorogenic acid prevents hyperuricemia nephropathy via regulating TMAO-related gut microbes and inhibiting the PI3K/AKT/mTOR pathway. J. Agric. Food Chem. 70, 10182–10193. doi:10.1021/acs.jafc.2c03099
Zhou, Y., Zhang, X., Li, C., Yuan, X., Han, L., Li, Z., et al. (2018). Research on the pharmacodynamics and mechanism of Fraxinus Cortex on hyperuricemia based on the regulation of URAT1 and GLUT9. Biomed. Pharmacother. 106, 434–442. doi:10.1016/j.biopha.2018.06.163
Zhu, J. X., Yang, H. Y., Hu, W. Q., Cheng, J., Liu, Y., Yi, L. T., et al. (2021). Active components from Lagotis brachystachya maintain uric acid homeostasis by inhibiting renal TLR4-NLRP3 signaling in hyperuricemic mice. Inflammopharmacology 29, 1187–1200. doi:10.1007/s10787-021-00844-5
Zuo, J., He, H., Zuo, Z., Bou-Chacra, N., and LöBENBERG, R. (2018). Erding Formula in hyperuricaemia treatment: unfolding traditional Chinese herbal compatibility using modern pharmaceutical approaches. J. Pharm. Pharmacol. 70, 124–132. doi:10.1111/jphp.12840
Glossary
Keywords: gout, TCM, signaling pathway, urate transporter, review
Citation: Sun X, Yang L, Sun H, Sun Y, Wei S, Han Y, Wang W, Kong L and Wang X (2023) TCM and related active compounds in the treatment of gout: the regulation of signaling pathway and urate transporter. Front. Pharmacol. 14:1275974. doi: 10.3389/fphar.2023.1275974
Received: 11 August 2023; Accepted: 12 October 2023;
Published: 29 November 2023.
Edited by:
Abul Kalam Najmi, Jamia Hamdard University, IndiaReviewed by:
Shizhang Wei, Fifth Medical Center of the PLA General Hospital, ChinaShang-Gao Liao, Guizhou Medical University, China
Copyright © 2023 Sun, Yang, Sun, Sun, Wei, Han, Wang, Kong and Wang. This is an open-access article distributed under the terms of the Creative Commons Attribution License (CC BY). The use, distribution or reproduction in other forums is permitted, provided the original author(s) and the copyright owner(s) are credited and that the original publication in this journal is cited, in accordance with accepted academic practice. No use, distribution or reproduction is permitted which does not comply with these terms.
*Correspondence: Xijun Wang, eGlqdW53QHNpbmEuY29t