- Department of Gastroenterology, The Fourth Affiliated Hospital of China Medical University, Shenyang, Liaoning, China
Prostate cancer (PCa) is one of the most common cancers in males, exhibiting a wide spectrum of clinical manifestations that pose challenges in its diagnosis and treatment. The Wnt signaling pathway, a conserved and complex pathway, is crucial for embryonic development, tissue homeostasis, and various physiological processes. Apart from the classical Wnt/β-catenin signaling pathway, there exist multiple non-classical Wnt signaling pathways, including the Wnt/PCP and Wnt/Ca2+ pathways. Non-coding RNAs (ncRNAs) are involved in the occurrence and development of PCa and the response to PCa treatment. ncRNAs are known to execute diverse regulatory roles in cellular processes, despite their inability to encode proteins. Among them, microRNAs, long non-coding RNAs, and circular RNAs play key roles in the regulation of the Wnt signaling pathway in PCa. Aberrant expression of these ncRNAs and dysregulation of the Wnt signaling pathway are one of the causes of cell proliferation, apoptosis, invasion, migration, and angiogenesis in PCa. Moreover, these ncRNAs affect the characteristics of PCa cells and hold promise as diagnostic and prognostic biomarkers. Herein, we summarize the role of ncRNAs in the regulation of the Wnt signaling pathway during the development of PCa. Additionally, we present an overview of the current progress in research on the correlation between these molecules and clinical features of the disease to provide novel insights and strategies for the treatment of PCa.
1 Introduction
In males, prostate cancer (PCa) is one of the most common cancers and the fifth leading cause of cancer-related deaths (Sung et al., 2021). According to estimates for 2020, the worldwide incidence of PCa was 1.4 million new cases, with more than 375,000 males dying owing to the disease (Sandhu et al., 2021). PCa is a complex and heterogeneous disease, exhibiting a wide range of clinical manifestations, ranging from indolent to aggressive (He et al., 2022). Thus, investigating the mechanisms of the occurrence and development of PCa is crucial for its diagnosis and treatment. Although the molecular mechanisms underlying PCa progression remain unclear, genetic alterations and signaling pathways have been found to play key roles (Wang et al., 2022b).
The Wnt signaling pathway is a conserved pathway that plays crucial roles in embryonic development, tissue homeostasis, and stem cell maintenance (Zhou et al., 2022a). The Wnt signaling pathway can be categorized into three classes: 1) Wnt/β-catenin signal transduction, 2) Wnt/PCP signal transduction, 3) Wnt/Ca2+ signal transduction (Asano et al., 2022). Dysregulation of the Wnt signaling pathway is associated with many diseases, including cancer (Yeh et al., 2019). In PCa, aberrant activation of the Wnt signaling pathway leads to dysregulation of cell proliferation, apoptosis, invasion, and angiogenesis (Koushyar et al., 2022). Understanding the mechanisms underlying Wnt signaling dysregulation can provide valuable insights into the pathogenesis of PCa and elucidate potential therapeutic targets.
Non-coding RNAs (ncRNAs) have been recognized as critical regulatory factors in gene expression and signal transduction in both normal physiology and disease pathogenesis, particularly in PCa (Ferri et al., 2022; Szaflik et al., 2022). ncRNAs are known to execute diverse regulatory roles in cellular processes, despite their inability to encode proteins (Wang et al., 2022a). Recent studies have elucidated several types of ncRNAs, such as microRNAs (miRNAs), long non-coding RNAs (lncRNAs), and circular RNAs (circRNAs), which play key roles in the regulation of the Wnt signaling pathway in PCa (Goodall and Wickramasinghe, 2021; Xue et al., 2022). The alterations in the expression patterns of these ncRNAs at distinct stages of PCa progression indicate their potential as diagnostic and prognostic biomarkers (He et al., 2020; Song et al., 2020). For example, the high expression of the lncRNA CCAT2 and SOX2-OT is associated with the diagnosis and prognosis of PCa, indicating their potential utility as biomarkers (He et al., 2020; Song et al., 2020). Moreover, these ncRNAs affect the characteristics of PCa cells such as proliferation, invasion, migration, and apoptosis; additionally, these ncRNAs can influence the therapeutic response of cancer cells by regulating the Wnt signaling pathway (He et al., 2020; Song et al., 2020; Wang et al., 2021). Therefore, Wnt signaling pathway-related ncRNAs are promising prospects as therapeutic targets for PCa.
In conclusion, our review presents a comprehensive elucidation of the intricate interplay between ncRNAs and the Wnt signaling pathway in the context of PCa occurrence, progression, and therapeutic approaches. Significantly, we underscore the profound implications of ncRNAs as promising diagnostic and prognostic biomarkers, accentuating their pivotal role in modulating PCa aggressiveness and therapeutic response. Moreover, we delve deep into the immense potential of targeting ncRNAs as therapeutic interventions for PCa, exploring a plethora of strategic avenues. Finally, we address the contemporary challenges encountered in this ever-evolving field.
2 Wnt signaling pathway and its role in PCa development
The Wnt signaling pathway is a complex intracellular signaling network that is involved in the regulation of cell fate, proliferation, and differentiation in many tissues (Figure 1) (Asano et al., 2022; Zhou et al., 2022a). Dysregulation of this pathway is associated with the occurrence and progression of many cancers, including PCa (Nusse and Clevers, 2017; Pisano et al., 2021). The Wnt signaling pathway can be categorized into the following two types: canonical and non-canonical (Rim et al., 2022). The canonical Wnt/β-catenin pathway operates through a mechanism whereby the binding of the Wnt protein to its receptor Frizzled triggers the activation of the Disheveled protein, leading to the inactivation of Axin protein and ultimately reducing the degradation of β-catenin (Rim et al., 2022). Consequently, β-catenin gradually accumulates in the cytoplasm and subsequently translocates into the nucleus, where it binds to TCF/LEF transcription factors to induce downstream gene expression (Rim et al., 2022). The non-canonical pathway includes the Wnt/PCP and Wnt/Ca2+ signaling pathways (Menck et al., 2021; Sarabia-Sanchez et al., 2023; VanderVorst et al., 2023). The Wnt/PCP signaling pathway is primarily involved in the regulation of cell polarity and tissue morphogenesis (VanderVorst et al., 2023). Additionally, it regulates cell adhesion and directional migration by activating the JNK and Rho GTPase signaling pathways (VanderVorst et al., 2023). The Wnt/Ca2+ signaling pathway primarily modulates gene expression and cell behavior by regulating intracellular Ca2+ levels (Sarabia-Sanchez et al., 2023). Moreover, it plays a critical role in embryonic nervous system development, cell polarity, and glial cell differentiation (Sarabia-Sanchez et al., 2023). The Wnt/Ror signaling pathway was recently discovered, and its function remains to be comprehensively elucidated (Menck et al., 2021). However, it has been shown to induce the formation and repair of synapses in neurons through certain Wnt ligands (Menck et al., 2021).
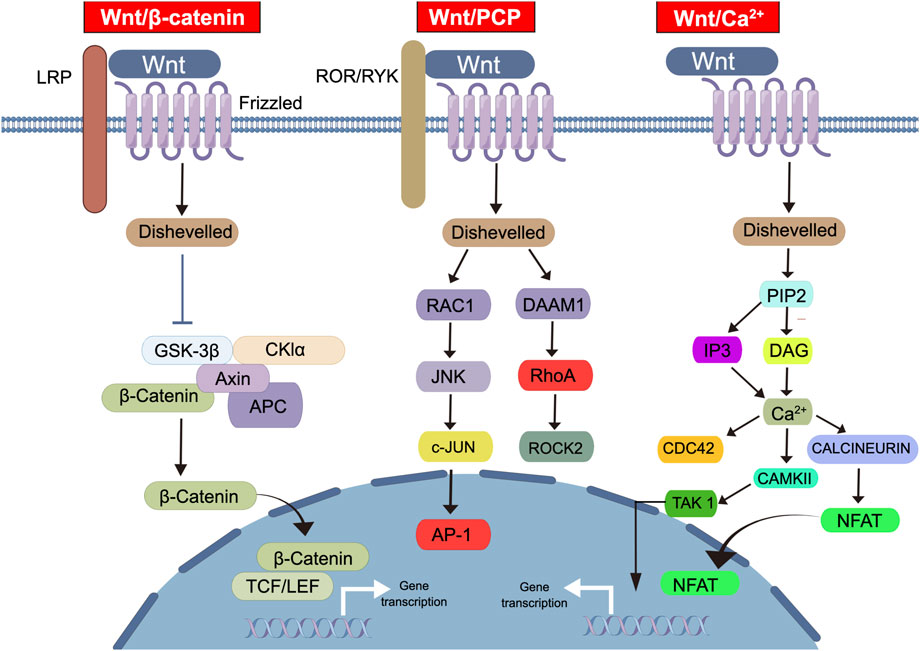
FIGURE 1. The diverse types and molecular mechanisms of the Wnt signaling pathway. This diagram illustrates the classification and members of the Wnt signaling pathway. The Wnt signaling pathway can be categorized into three classes: (1) Wnt/β-catenin signal transduction, (2) Wnt/PCP signal transduction, (3) Wnt/Ca2+ signal transduction. In the Wnt/β-catenin pathway, Wnt ligands bind to Frizzled receptors and LRP co-receptors, leading to the activation of the disheveled protein and subsequent inactivation of Axin protein. This results in the accumulation of β-catenin in the cytoplasm, its translocation into the nucleus, and binding to TCF/LEF transcription factors, ultimately inducing downstream gene expression. Wnt/PCP signaling pathway involved in cell polarity, tissue morphogenesis, cell adhesion, and directional migration through JNK and Rho GTPase signaling pathways. The Wnt/Ca2+ signaling pathway modulates gene expression and cell behavior by regulating intracellular Ca2+ levels. Abbreviations: Wnt, Wingless-related integration site; LRP, Low-density lipoprotein receptor-related protein; GSK3β, Glycogen Synthase Kinase 3 beta; CKIα, Casein kinase I alpha; Axin, Axis inhibitor; APC, Adenomatous Polyposis Coli; TCF, T-cell factor; LEF, Lymphoid enhancer-binding factor; RAC1, Ras-related C3 botulinum toxin substrate 1; DAMM1, Disheveled-associated activator of morphogenesis 1; JNK, c-Jun N-terminal kinase; RhoA, Ras homolog family member A; c-JUN, Cellular Jun oncogene; ROCK2, Rho-associated coiled-coil kinase 2; AP-1, Activator protein 1; PIP2, Phosphatidylinositol 4,5-bisphosphate; IP3, Inositol trisphosphate; DAG, Diacylglycerol; CDC42, Cell division control protein 42 homolog; TAK1, Transforming growth factor-beta-activated kinase 1; CAMKII, Ca2+/calmodulim-dependent protein kinase II; NFAT, Nuclear factor of activated T-cells.
The Wnt signaling pathway is activated through the interaction of ligands and receptors on cell surfaces, leading to subsequent signal transduction through intracellular signaling molecules such as β-catenin and TCF/LEF transcription factors (Zhou et al., 2022b). In PCa, aberrant activation of the Wnt signaling pathway leads to dysregulation of cell proliferation, apoptosis, invasion, and angiogenesis (Khurana and Sikka, 2019; Lin et al., 2020). This dysregulation often results from mutations or alterations in key components of the Wnt signaling pathway, such as adenomatous polyposis coli (APC), Axin, and β-catenin, which promote cell cycle progression by activating cyclin D1 and c-myc and enhancing the induction effect exerted by TGF-β signaling (Reya and Clevers, 2005; Zhong et al., 2020; Yu et al., 2021). In addition, these components possibly promote neovascularization and tumor invasion by increasing the expression of VEGF, MMP-9, and IL-8 (Vallee and Lecarpentier, 2018).
Aberrant activation of the Wnt signaling pathway can serve as a biomarker for PCa (Yeh et al., 2019; Pisano et al., 2021). The expression of β-catenin protein in PCa tissue is significantly increased, and mutations and dysregulation of Wnt signaling pathway-related genes can also lead to the aberrant activation of this pathway (Yeh et al., 2019; Pisano et al., 2021). Distinct mutations in the Wnt signaling pathway genes are associated with specific subtypes of PCa. For example, APC mutations are frequently observed in early-stage and low-grade PCa, whereas β-catenin mutations are observed in advanced-stage and high-grade PCa (Desai et al., 2022; Mangolini et al., 2022). Furthermore, in metastatic PCa, an increased proportion of activation mutations in the Wnt/β-catenin signaling pathway-related genes is observed (Desai et al., 2022; Mangolini et al., 2022). The aberrant activation of the Wnt signaling pathway is also associated with the staging and metastasis of PCa (Yeh et al., 2019; Pisano et al., 2021). The classical Wnt/β-catenin signaling pathway is activated in late-stage PCa and bone metastatic PCa, facilitating cell proliferation and drug resistance (Yeh et al., 2019; Pisano et al., 2021).
Multiple components of the Wnt signaling pathway are potential targets in PCa therapeutic interventions (Koushyar et al., 2022). The inhibition of the Wnt signaling pathway in PCa may be crucial for its prevention and treatment. Few studies have investigated methods for inhibiting the Wnt/β-catenin signaling pathway (Brown, 2005; Park and Kim, 2023). For example, researchers have developed and evaluated the efficacy of Protac/molecular glue, antibody-drug conjugates, and anti-sense oligonucleotides in inhibiting the Wnt signaling pathway in preclinical models and clinical trials (Park and Kim, 2023). Additionally, RNA interference technology has shown promising results in preclinical models (Brown, 2005). However, the investigation of these methods is at its nascent stages, and further investigation is necessary to ascertain their efficacy in clinical treatment. Epigenetic alterations also regulate the activity of the Wnt signaling pathway in PCa (Xiong et al., 2009; Eismann et al., 2023). Alterations in DNA methylation and histone modification patterns frequently occur in PCa cells, leading to alterations in gene expression and the activity of the Wnt signaling pathway (Xiong et al., 2009; Eismann et al., 2023). Therefore, directing therapeutic interventions toward these epigenetic modifications may be a viable approach.
The crosstalk between the Wnt signaling pathway and other signaling pathways, such as the androgen receptor (AR) signaling pathway, is gaining recognition as a key factor in the pathogenesis of PCa (Pisano et al., 2021). This presents a challenge in the targeting of the Wnt signaling pathway in PCa treatment (Pisano et al., 2021). The critical role of AR signaling in PCa progression is well-known, and recent studies indicate that Wnt and AR signals interact in complex ways, regulating each other’s activities (Pisano et al., 2021). Thus, disrupting this crosstalk may be necessary to achieve desired treatment outcomes. Overall, a comprehensive examination of the Wnt/β-catenin signaling pathway may provide novel insights and avenues for the diagnosis, treatment, and prevention of PCa.
3 The involvement of NcRNAs in modulating the Wnt signaling pathway in PCa
The dysregulation of ncRNAs has been associated with the occurrence and progression of PCa (Ramnarine et al., 2019). Recent studies have elucidated several types of ncRNAs, such as miRNAs, lncRNAs, and circRNAs, which play crucial roles in PCa by regulating the Wnt signaling pathway (Figure 2). Various types of ncRNAs interact with each other and with other regulatory factors, such as transcription factors, and induce epigenetic modifications, to activate the Wnt signaling pathway (Ramnarine et al., 2019; Orafidiya et al., 2022). For example, the lncRNA SOX2-OT downregulates transcription factor 7-like (TCF7L), a negative regulator of the Wnt signaling pathway, thereby activating the pathway (Song et al., 2020). Similarly, miR-182 regulates the Wnt signaling pathway by targeting APC and glycogen synthase kinase 3 beta (GSK3β), which are two key components of the destruction complex that regulates β-catenin stability (Wang et al., 2018). ncRNAs also activate the Wnt signaling pathway by directly regulating the expression of Wnt signaling pathway-related genes. For example, the lncRNA TUG1 is highly expressed in PCa tissues and cells and promotes cell proliferation, migration, and invasion through the miR-496/Wnt/β-catenin axis (Xiu et al., 2020). miRNAs, such as miR-653-5p, miR-182, miR-1301-3p, and miR-454, also activate the Wnt/β-catenin signaling pathway and promote the malignant progression of PCa cells (Guan et al., 2017; Fu et al., 2018; Wang et al., 2018; Fu et al., 2019). Although many ncRNAs activate the Wnt signaling pathway to promote PCa progression, certain ncRNAs inhibit the Wnt signaling pathway to exert an anti-cancer effect on PCa (Dong et al., 2020). For example, the well-characterized tumor suppressor miR-34a targets multiple Wnt signaling pathway-related genes, including those of Wnt1, LEF1, and β-catenin (Dong et al., 2020).
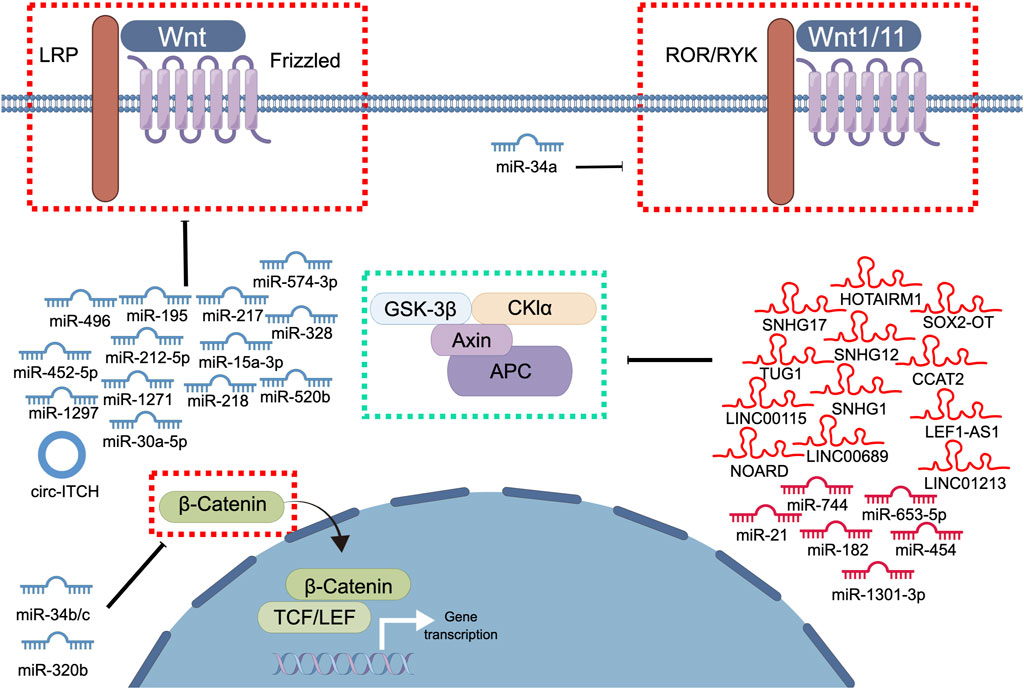
FIGURE 2. The involvement of ncRNAs in modulating the Wnt signaling pathway in prostate cancer. This diagram illustrates the involvement of ncRNAs in modulating the Wnt signaling pathway in PCa. Blue represents the role of suppressing the Wnt signaling pathway. Red represents roles that facilitate the Wnt signaling pathway.
Despite the aforementioned findings, the molecular mechanism of the regulation of the Wnt signaling pathway by ncRNAs in PCa is not entirely understood, and additional investigations are required to elucidate the complex interplay between various types of ncRNAs and other regulatory factors that activate or inhibit the Wnt signaling pathway in PCa. Furthermore, the investigation of ncRNAs poses technical challenges, owing to their low abundance and high sequence variability (Huang et al., 2023). Advances in next-generation sequencing technologies and bioinformatics tools have improved our ability to identify and validate ncRNA targets and their interactions with the Wnt signaling pathway (Mattick et al., 2023).
4 Type and role of Wnt signaling pathway-related NcRNAs in PCa progression
The Wnt signaling pathway plays a key role in the progression of PCa, with ncRNAs as crucial regulatory factors of this pathway (Pakula et al., 2017; Sonawala et al., 2022). Recent studies have elucidated several types of Wnt signaling pathway-related ncRNAs, including miRNAs, lncRNAs, and circRNAs, which exhibit abnormal expression in PCa and are closely linked to its progression (Table 1).
4.1 miRNAs
miRNAs are RNA molecules that are 22 nucleotides in length and regulate gene expression by binding to complementary target messenger RNAs (mRNAs) (Lagos-Quintana et al., 2001). The tissue-specific expression of miRNAs provides a premise for their clinical application as diagnostic and prognostic markers in cancer (Kim and Croce, 2021). For example, miR-574-3p is significantly downregulated in PCa tissues, and low expression of miR-574-3p is associated with an advanced tumor stage and a high Gleason score (Chiyomaru et al., 2013). The interactions between miRNAs and the Wnt signaling pathway in PCa are of two types: direct targeting of key genes associated with the Wnt signaling pathway and indirect targeting of the pathway via other genes. Based on their function, miRNAs can be categorized as oncogenic miRNAs or tumor suppressor miRNAs. Most miRNAs, including miR-34a, miR-34b/c, miR-1297, miR-1271, miR-218, miR-520b, miR-138, miR-574-3p, miR-15a-3p, and miR-320, exert tumor-suppressive effects by directly or indirectly inhibiting the Wnt signaling pathway (Chiyomaru et al., 2013; Hsieh et al., 2013; Liu et al., 2015; Li et al., 2016b; Liang et al., 2016; Zhong et al., 2017; Ren et al., 2018; Yu et al., 2018; Cui et al., 2019; Dong et al., 2020). However, a few miRNAs, such as miR-653-5p, miR-182, miR-1301-3p, and miR-454, promote malignant progression of PCa cells, such as proliferation, invasion, and migration, by activating the Wnt signaling pathway and targeting tumor suppressor genes (Guan et al., 2017; Fu et al., 2018; Wang et al., 2018; Fu et al., 2019). Simultaneously, miRNAs can also suppress stemness in PCa by inhibiting the Wnt signaling pathway (Hsieh et al., 2013; Zhou et al., 2016; Song et al., 2018). For example, miRNAs, such as miR-320 and miR-1301-3p, inhibit the activation of the Wnt signaling pathway and suppress the proliferation of PCa stem cells (Hsieh et al., 2013; Song et al., 2018). In addition, certain naturally active chemical substances have been found to exert therapeutic effects on PCa by regulating miRNAs and the Wnt signaling pathway. For example, urolithin, an active metabolite produced by human colonic microbiota, has been found to inhibit miR-21 and its downstream Wnt signaling pathway, thereby promoting apoptosis of PCa cells and inhibiting tumor growth (Zhou et al., 2016). Therefore, miRNAs and the Wnt signaling pathway may become notable therapeutic targets in PCa treatment, and their in-depth examination may reveal the pathogenesis of PCa and aid in the development of novel drugs against PCa.
4.2 LncRNAs
LncRNAs are RNA molecules that are 200 nucleotides in length and do not encode proteins (Szaflik et al., 2022). Although most of the genome is comprised of ncRNAs that are encoded by “junk DNA,” they were originally considered to lack physiological functions (Huang et al., 2023). However, as the potential roles of lncRNAs in biological processes have been unveiled, the aforementioned notion has gradually changed (Wang et al., 2022a). LncRNAs regulate gene expression at various levels, i.e., chromatin, transcriptional, and post-transcriptional levels (Bhattacharjee et al., 2023; Petrone et al., 2023). Mounting evidence suggests that lncRNAs play crucial roles in PCa cell invasion, migration, and apoptosis and in castration-resistant PCa (CRPC), thereby affecting the proliferation, migration, and response of cancer cells to treatment (Li et al., 2020d; Song et al., 2020; Liu et al., 2021; Tan et al., 2021; Wu et al., 2021). LncRNAs can serve as signals, baits, or scaffolds to modulate cellular functions (Yang et al., 2020; Yan and Bu, 2021). Similar to miRNAs, lncRNAs may exert tumor-suppressive or oncogenic effects, contingent upon their category and mode of regulation. For example, lncRNAs such as TUG1, SOX2-OT, LINC04080, LINC00115, LINC00689, NORAD, SNHG1, and LEF1-AS1 activate the Wnt signaling pathway and promote tumor growth and metastasis by regulating other ncRNAs or proteins (Wang et al., 2019; Li et al., 2020a; Chen et al., 2020; Meng et al., 2020; Song et al., 2020; Zhang and Li, 2020; Peng et al., 2021). Conversely, lncRNAs such as lncRNA625 and HOTAIRM1 inhibit the Wnt signaling pathway to suppress cancer, promote cell apoptosis, and inhibit cell proliferation in PCa (Li et al., 2017; Wang et al., 2021). Investigation of the role of lncRNA625 in cancers has revealed that it promotes tumor development in esophageal carcinoma; however, it exerts a significant tumor-suppressive effect on PCa, suggesting that lncRNA625 could potentially serve as a therapeutic target for PCa (Li et al., 2017; Guo-Wei et al., 2019). Given the lack of effective PCa treatments, the investigation of treatments based on Wnt signaling pathway-related lncRNAs is of great significance for developing novel therapeutic strategies for PCa. Therefore, in-depth research on lncRNAs is expected to yield novel treatment options for patients with PCa. In addition, Wnt signaling pathway-related lncRNAs may have notable implications for prognosis evaluation and diagnostic positioning for PCa, with diverse potential applications.
4.3 CircRNAs
CircRNAs were first discovered in plant viruses and the Sendai virus through electron microscopy in 1976 (Kolakofsky, 1976). However, it is a widely held belief that circRNAs are the result of splicing errors and exhibit low expression levels (Memczak et al., 2013). With the advancement of bioinformatics and sequencing technologies, various types of circRNAs have been implicated in tumors (Zhou et al., 2021). For example, cir-znf215 has been found to promote the growth and metastasis of cholangiocarcinoma by inhibiting the AKT pathway (Liao et al., 2023). circRNAs also exhibit tissue- and cell-specific expression (Wang et al., 2023). Therefore, circRNAs could potentially serve as diagnostic and prognostic markers as well as therapeutic targets for PCa. cir-ITCH is typically downregulated in PCa tissues and cell lines compared with normal adjacent tissues and normal RWPE-1 cells, indicating the potential of cir-ITCH as a diagnostic and prognostic marker for PCa (Li et al., 2020c). Moreover, cir-ITCH exerts an anti-cancer effect on PCa by inhibiting the Wnt signaling pathway (Li et al., 2020c). In addition, circRNAs interact with ncRNAs and regulate each other’s expression levels (Li et al., 2020c; Ghafouri-Fard et al., 2021). For example, mutual inhibition of expression has been observed between cir-ITCH and miR-17 in PCa (Li et al., 2020c; Ghafouri-Fard et al., 2021).
5 Molecular mechanisms and functions of Wnt signaling pathway-related NcRNAs in PCa
5.1 Invasion and migration
Metastasis is the primary cause of most cancer-related deaths (Fares et al., 2020). Invasion and migration are critical steps in the cascade of tumor metastasis (Fares et al., 2020). miR-34a is a key regulatory factor in tumor suppression, modulating the expression of numerous target proteins involved in cell cycle, differentiation, epithelial-to-mesenchymal transition (EMT), and apoptosis, among others, and antagonizing processes such as cancer cell activity, stemness, metastasis, and chemoresistance (Misso et al., 2014). The expression of miR-34a is significantly lower in PCa tissues than in normal tissues (Lichner et al., 2015). The overexpression of miR-34a significantly reduces the proliferation and migration abilities of PCa cell lines, sush as PC3 cells (Lichner et al., 2015) (Figure 3). These effects are achieved by inhibiting the Wnt signaling pathway through the regulation of Wnt1 transcriptional activity (Dong et al., 2020). Notably, bones are the most common site of metastasis in PCa (Coleman et al., 2020). In PCa exhibiting activated Ras signaling, bone metastasis associated with low expression of miR-34a has been observed (Chen et al., 2015). miR-34a knockdown has been observed to induce the expression of TCF7 and BIRC5 by activating the Wnt signaling pathway, thereby promoting cell survival (Chen et al., 2015). These findings suggest that miR-34a could serve as a potential target in the treatment of metastatic PCa.
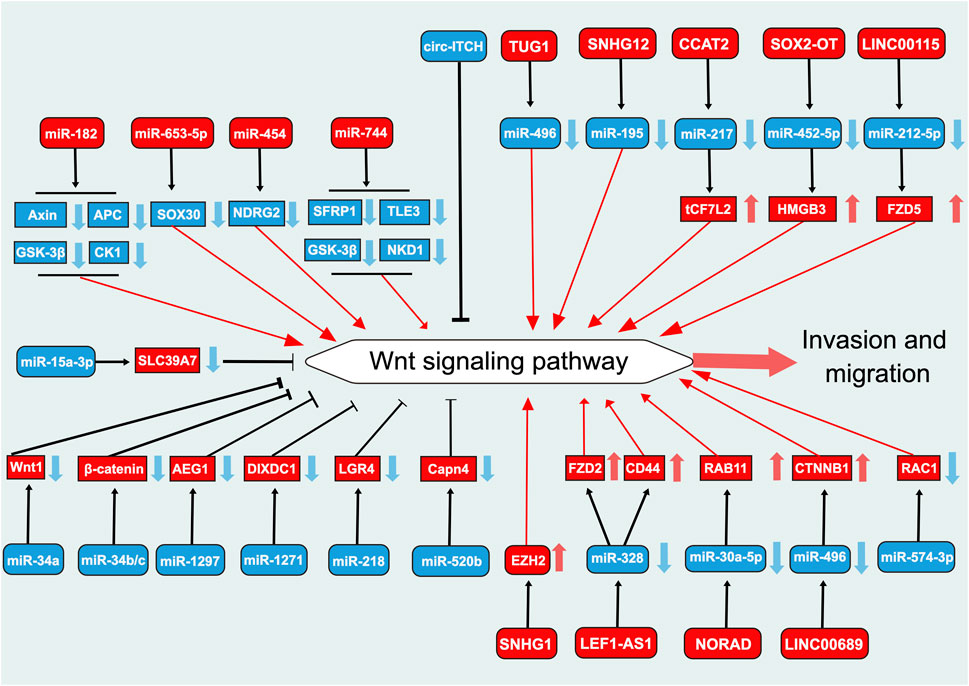
FIGURE 3. Role of Wnt signaling pathway-related ncRNAs in the regulation of invasion and migration in prostate cancer. This diagram illustrates the role of Wnt signaling pathway-related ncRNAs in the regulation of invasion and migration in PCa. Blue represents the role of suppressing the Wnt signaling pathway. Red represents roles that facilitate the Wnt signaling pathway.
Li et al. demonstrated that miR-1297 directly targets the 3′-untranslated region of AEG-1 and regulates its mRNA and protein expression levels (Liang et al., 2016). In addition, they found that miR-1297 inhibits the Wnt signaling pathway by targeting AEG-1 in PCa, thereby suppressing cell proliferation and invasion (Liang et al., 2016). DIXDC1 is involved in the regulation of the proliferation and invasion of various tumors (Zhong et al., 2017; Xin et al., 2018). In PCa, it can be directly targeted and inhibited by miR-1271 (Zhong et al., 2017). MiR-1271 exhibits low expression in PCa and inhibits cell proliferation, invasion, and Wnt signal transduction by targeting DIXDC1 (Zhong et al., 2017).
Epidemiological and histopathological evidence suggests a correlation between inflammation and PCa incidence (De Nunzio et al., 2011). LGR4, which is induced by IL-6 during cancer progression, has been recently identified as a response gene associated with PCa progression (Liu et al., 2013). Yang et al. found that miR-218 directly targets LGR4 to inhibit the Wnt signaling pathway in LNCaP-IL-6+ cells during IL-6-induced PCa cell progression, thereby suppressing cell proliferation, cell cycle progression, and invasion (Li et al., 2016b). CapnS1 has been found to have a negative correlation with disease progression in various solid tumors (Zheng et al., 2020). In PCa, CapnS1 expression is regulated by miR-520b, and it exerts an oncogenic effect by promoting Wnt signal transduction (Ren et al., 2018). miR-520b is significantly downregulated in PCa (Ren et al., 2018). Inhibition of CapnS1 by miR-520b suppresses the growth and invasion of PCa cells associated with the downregulation of Wnt signal transduction (Ren et al., 2018). miR-138 has been observed to be downregulated in invasive PCa cell lines and promote PCa cell invasion and migration through the Wnt signaling pathway, whereas its overexpression has been observed to suppress these functions (Yu et al., 2018). miR-574-3p is significantly downregulated in PCa, and its low expression is associated with advanced tumor stage and a high Gleason score (Chiyomaru et al., 2013). The overexpression of miR-574-3p significantly inhibits the proliferation, migration, and invasion of PCa cells, which is associated with the inhibition of the Wnt signaling pathway via RAC1 (Chiyomaru et al., 2013). SOX30 is a recently identified cancer-related member of the SOX family that has a significant role in various types of cancer (Fu et al., 2019). miR-653-5p is highly expressed in PCa tissues and promotes the proliferation and invasion of PCa cells by targeting and upregulating β-catenin expression via SOX30 and activating the Wnt signaling pathway (Fu et al., 2019). miR-182 expression is higher in PCa tissues than in non-cancerous tissues, and miR-182 significantly activates the Wnt signaling pathway by targeting multiple negative regulators of Wnt signaling, thereby promoting cell proliferation, colony formation, migration, and invasion (Wang et al., 2018). miR-454 is highly expressed in PCa tissues and cell lines and promotes PCa cell proliferation and invasion by upregulating the Wnt signaling pathway, which is achieved by inhibiting NDRG2 expression (Wei et al., 2020). miR-744 significantly activates the Wnt signaling pathway by targeting multiple negative regulators of the pathway and promotes PCa cell proliferation, migration, and invasion (Guan et al., 2017).
TUG1, a 7.1 kb lncRNA, was first discovered to be upregulated in mouse retinal cells in response to taurine treatment (Young et al., 2005). TUG1 is highly expressed in PCa tissues and cells and promotes cell proliferation, migration, and invasion through the miR-496/Wnt/β-catenin axis (Li et al., 2020b; Xiu et al., 2020). LncRNA SOX2-OT plays crucial roles in psychiatric disorders, cancer, and diabetic complications (Li et al., 2020c). In PCa tissues and cells as well, SOX2-OT is highly expressed. Regulation of the miR-452-5p/HMGB3 axis and inactivation of the Wnt signaling pathway have been shown to inhibit PCa cell proliferation and metastasis, thereby suppressing tumor growth in vivo (Song et al., 2020). SNHG12, also known as LINC04080, is a lncRNA spanning approximately 1867 nucleotides and is located in the 1p35.3 region (Lan et al., 2017). In PCa, SNHG12 expression is upregulated in serum and tissues and is associated with RFS, biochemical recurrence, and Gleason scores of 8–10 in patients (Wang et al., 2019). This lncRNA activates the Wnt signaling pathway through the sponging effect of miR-195, thereby promoting cell proliferation, invasion, and migration in PCa (Song et al., 2019). LINC00115 was first identified as a notable pro-cancer lncRNA in lung cancer (Li et al., 2016a). In PCa, it is highly expressed in tissues and closely associated with a poor prognosis (Peng et al., 2021). LINC00115 promotes PCa cell proliferation and invasion by targeting the miR-212-5p/FZD5/Wnt axis (Peng et al., 2021). LINC00689, first found to be associated with obesity susceptibility genes in the Han Chinese population of northern China, exerts a pro-cancer effect in multiple solid tumors, including gastric cancer, breast cancer, and liver cancer (Liu et al., 2019b; Du et al., 2020; Lu et al., 2020). This lncRNA activates the Wnt signaling pathway by regulating miR-496/CTNNB1, thereby promoting PCa cell proliferation, migration, and invasion (Meng et al., 2020). LEF1 is a key component of the Wnt/β-catenin signaling pathway. It is highly expressed in PCa and is associated with its malignant progression (Fakhr et al., 2021). The recently identified lncRNA LEF1-AS1 is encoded by the LEF1 locus and is associated with poor prognosis in multiple cancer types (Li et al., 2020d). LEF1-AS1 promotes PCa metastasis and serves as a competing endogenous RNA (ceRNA) for miR-328, thereby modulating Wnt/β-catenin pathway activity by regulating FZD2 and CD44, ultimately promoting androgen-independent PCa (AIPC) cell proliferation, migration, invasion, angiogenic ability, and tumor growth (Li et al., 2020d). In addition, lncRNAs also play crucial roles in PCa metastasis by directly binding to EZH2 (Chen et al., 2020). For example, both the lncRNAs SNHG1 and EZH2 are highly expressed in PCa tissues and cells, and their expression is positively correlated. SNHG1 regulates the Wnt signaling pathway through the EZH2 gene, modulating PCa cell proliferation, invasion, and migration (Chen et al., 2020). These findings further enrich our understanding of the mechanism of action of Wnt signaling pathway-related lncRNAs in PCa. The aforementioned lncRNAs are associated with the Wnt signaling pathway and play vital roles in the growth and progression of PCa, thereby presenting as novel targets for PCa treatment.
In addition to the aforementioned Wnt signaling pathway-related lncRNAs, the role of the lncRNA CCAT2 in PCa metastasis should be considered (Zheng et al., 2016; He et al., 2020). Studies have shown that complex feedback loops exist between CCAT2 and the Wnt signaling pathway (Zheng et al., 2016; He et al., 2020). Moreover, CCAT2 plays a key role in the invasion and migration of PCa (Zheng et al., 2016; He et al., 2020). Notably, CCAT2 is not only aberrantly expressed in PCa but also exhibits similar expression patterns in many other cancers (Ling et al., 2013). In colorectal cancer, it is a downstream target of the Wnt signaling pathway, indicating that TCF7L2 is also involved in this feedback loop (Ling et al., 2013). Therefore, an in-depth investigation of the role of lncRNAs in the Wnt signaling pathway is of great significance for the prognosis and treatment of cancer as well as the recovery from cancer. In summary, the key role of the lncRNA CCAT2 in PCa metastasis indicates its potential as a therapeutic target.
In contrast to miRNAs and lncRNAs, circRNAs associated with the Wnt signaling pathway in PCa have been the subject of comparatively less investigation. The expression of cir-ITCH is downregulated in PCa tissues and cell lines (Li et al., 2020c), and its overexpression significantly inhibits the proliferation, migration, and invasion of human PCa cells. Cir-ITCH and miR-17 function as mutual expression inhibitory factors (Li et al., 2020c). Cir-ITCH contributes to the suppression of PCa progression by inhibiting the Wnt/β-catenin signaling pathway, which may be achieved through the inhibition of miR-17 (Li et al., 2020c).
5.2 EMT
The metastasis of solid tumors is also influenced by the characteristics and plasticity of cancer cells, such as EMT (Babaei et al., 2021). During EMT, epithelial cells transform into highly mobile mesenchymal cells, thereby increasing the migration ability of cancer cells (Hao et al., 2019). Inhibiting EMT is key to preventing cancer metastasis and improving prognosis (Fedele et al., 2022). miR-15a-3p is downregulated in PCa tissues and cell lines, whereas its overexpression inhibits cell proliferation, invasion, and EMT by downregulating the Wnt signaling pathway, with SLC39A7 as its direct downstream target (Cui et al., 2019) (Figure 4). LEF1 is a key transcription factor in the Wnt signaling pathway that regulates cell proliferation and invasion (Fakhr et al., 2021). miR-34a can regulate the level of LEF1 to inhibit EMT in PCa cells (Liang et al., 2015). Additionally, two other members of the miR-34 family, namely miR-34b/c, when overexpressed, can target β-catenin mRNA expression, thereby inhibiting cell migration and EMT in PCa (Liu et al., 2015).
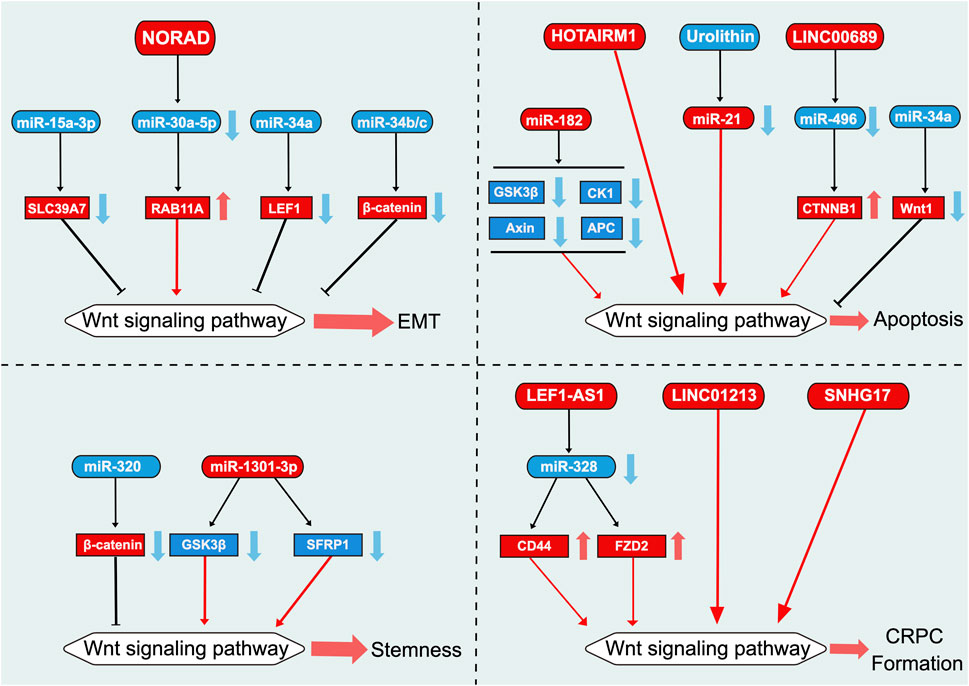
FIGURE 4. Role of Wnt signaling pathway-related ncRNAs in the regulation of EMT, apoptosis, stemness, and CRPC formation in prostate cancer. This diagram illustrates the role of Wnt signaling pathway-related ncRNAs in the regulation of EMT, apoptosis, stemness, and CRPC formation in PCa. Blue represents the role of suppressing the Wnt signaling pathway. Red represents roles that facilitate the Wnt signaling pathway.
The lncRNA NORAD exerts a pro-cancer effect in melanoma, pancreatic cancer, and glioblastoma (Soghli et al., 2021). In PCa as well, NORAD is highly expressed in cells and tissues and promotes cell proliferation, invasion, and EMT (Zhang and Li, 2020; Hu et al., 2021; Fletcher et al., 2022). miR-30a-5p attenuates NORAD-mediated promotion of cell proliferation, invasion, and EMT by targeting RAB11A (Zhang and Li, 2020).
5.3 Apoptosis
Cell apoptosis is a key self-regulation mechanism in multicellular organisms, serving to eliminate unwanted or abnormal cells (Kerr et al., 1972). Dysregulation of cell apoptosis has been implicated in various diseases, including cancer, autoimmune diseases, cardiovascular diseases, and neurological diseases (Chen et al., 2021). In recent years, a growing body of evidence has shown that ncRNAs play a crucial role in PCa cell apoptosis (Tamtaji et al., 2021). The Wnt signaling pathway is associated with PCa cell apoptosis, and ncRNAs associated with this pathway also play key roles in PCa cell apoptosis (Tamtaji et al., 2021). The overexpression of miR-34a inhibits the Wnt signaling pathway by regulating the transcriptional activity of Wnt1, thereby significantly increasing the rate of cell apoptosis (Dong et al., 2020) (Figure 4). Compared with non-cancerous tissues, PCa tissues exhibit upregulated expression of miR-182 (Wang et al., 2018). The upregulation of miR-182 activates the Wnt signaling pathway by targeting negative regulatory factors of the pathway, such as GSK3β, APC, CK1, and Axin, ultimately inhibiting cell apoptosis (Wang et al., 2018). Urolithin, a bioactive metabolite derived from ellagic acid, has been observed to inhibit miR-21 and its downstream Wnt/β-catenin signaling pathway to reduce cell viability and promote caspase-dependent cell apoptosis in DU145 cells (Zhou et al., 2016; Singh et al., 2019).
LINC0689 exerts a pro-oncogenic effect in multiple parenchymal tumors, where its expression is elevated (Liu et al., 2019b; Du et al., 2020). LINC00689 is upregulated in end-stage PCa tissues and inhibits apoptosis through miR-496/CTNNB1 (Meng et al., 2020). In addition, the lncRNA HOTAIRM1 is highly expressed in mature bone marrow cells (Zhang et al., 2014). Silencing HOTAIRM1 in PC3 cells promotes PCa cell apoptosis by downregulating the Wnt/β-catenin signaling pathway; however, the exact mechanism remains unknown (Wang et al., 2021).
5.4 Stemness
Cancer stem cells play a crucial role in the survival, proliferation, metastasis, and recurrence of tumors (Murota et al., 2022). miR-320 inhibits the activation of the Wnt/β-catenin signaling pathway by targeting β-catenin mRNA expression, thereby suppressing PCa stem cell characteristics such as tumor sphere formation, chemoresistance, and tumorigenicity (Hsieh et al., 2013) (Figure 4). In contrast, miR-320 knockdown significantly enhances the aforementioned characteristics (Hsieh et al., 2013). miR-1301-3p is significantly upregulated in PCa cells and tissues and targets inhibitors of the Wnt signaling pathway, namely GSK3β and SFRP1, thereby promoting the proliferation of PCa stem cells by activating the Wnt/β-catenin signaling pathway (Song et al., 2018).
5.5 CRPC formation
Targeting the Wnt/β-catenin signaling pathway may be an attractive therapeutic strategy for treating CRPC (Shafi et al., 2013). The potential of Wnt signaling pathway-related lncRNAs in the treatment of CRPC is currently gaining increasing attention (Yap et al., 2011) (Figure 4). Androgen deprivation therapy has become the mainstay for the treatment of patients with advanced PCa (Shafi et al., 2013). However, most patients eventually progress to CRPC, leading to poor prognosis (Yap et al., 2011). Bone metastasis is a notable issue in patients with CRPC (Beltran et al., 2016; Lin et al., 2020). Luo et al. elucidated that crosstalk between the AR and Wnt/β-catenin signals promotes the androgen-independent transformation of PCa (Luo et al., 2020). Although androgens can inhibit the Wnt/β-catenin signaling pathway in androgen-dependent PCa cells, this inhibitory effect is not observed in AIPC cells (Luo et al., 2020). Moreover, LEF1-AS1 has been observed to promote PCa metastasis through the Wnt/β-catenin signaling pathway and function as a ceRNA for miR-328, thereby regulating the activity of the Wnt/β-catenin signaling pathway by regulating FZD2 and CD44, ultimately enhancing proliferation, migration, invasion, and angiogenic ability of AIPC cells and tumor growth (Li et al., 2020d). The Wnt/β-catenin signaling pathway inhibits AIPC cell proliferation by promoting the cell cycle process and inhibiting apoptosis (Luo et al., 2020). Therefore, targeting the Wnt/β-catenin signaling pathway may be a viable strategy for the treatment of CRPC.
Exosomes are small vesicles that measure approximately 40–160 nm (typically approximately 100 nm) in diameter and originate from endosomes (Pegtel and Gould, 2019). Many studies have demonstrated the importance of several lncRNAs in exosomes across various cancers (Kalluri and LeBleu, 2020). For example, the exosomal lncRNA HOXD-AS1 has been observed to promote the metastasis of PCa through the miR-361-5p/FOXM1 axis (Jiang et al., 2021). Wnt signaling pathway-related lncRNAs loaded in exosomes could potentially serve as diagnostic and therapeutic tools for the treatment of CRPC (Guo et al., 2022). For example, LINC01213 plays a role in the transition of PCa cells from an androgen-dependent to an androgen-independent state (Guo et al., 2022). Additionally, it induces androgen deprivation tolerance by activating the Wnt signaling pathway through exosome-mediated intercellular communication in PCa (Guo et al., 2022). The lncRNA SNHG17 is one of the four significantly upregulated lncRNAs in metastatic PCa and AIPC cells, wherein it promotes tumor cell proliferation, survival, invasion, and resistance to chemotherapy by upregulating the Wnt/β-catenin signaling pathway (Bai et al., 2020; Zhao et al., 2021).
6 Wnt signaling pathway-related NcRNAs in the diagnosis and treatment of PCa
Early detection of PCa is crucial for effective treatment and improved survival rates (Sung et al., 2021). For example, in the event of early detection, the five-year survival rate of patients with localized PCa is nearly 100% (Sandhu et al., 2021). In contrast, the median survival duration for patients with metastatic PCa is approximately 3 years (Sandhu et al., 2021). Therefore, early diagnosis of PCa is essential. Additionally, because PCa is prone to metastasis and chemoresistance, it has become one of the leading causes of cancer-related mortality worldwide (He et al., 2022). Due to the lack of symptoms in the early stages of PCa, despite technological advancements, the discovery of novel tumor biomarkers remains crucial (Wang et al., 2022b). This is necessary to address the challenges associated with the diagnosis and treatment of prostate cancer. NcRNAs exhibit tissue-specific expression and are detectable at all stages of PCa development, making them potential biomarkers and therapeutic targets (Mugoni et al., 2022). Mounting evidence suggests that Wnt signaling pathway-related ncRNAs are closely associated with PCa progression (Doghish et al., 2022), rendering them promising biomarkers for the diagnosis, prognosis, and treatment of PCa (Table 2). Therefore, investigating Wnt signaling pathway-related ncRNAs in the context of early diagnosis, prognosis prediction, cancer treatment, and resolution of treatment resistance is an effective strategy to improve the survival of PCa patients.
6.1 Potential PCa diagnostic biomarkers
Early screening and diagnosis of cancer are crucial for patient survival (Sung et al., 2021). The identification of suitable biomarkers has consistently posed a notable challenge in the field of cancer research (Movahedpour et al., 2022; Xie et al., 2022). Wnt signaling pathway-related ncRNAs aid in the early diagnosis of PCa. In patients with PCa, certain Wnt signaling pathway-related ncRNAs, such as SNHG17 and LINC00115, are upregulated (Peng et al., 2021), whereas other ncRNAs, such as miR-34a, are downregulated (Chiyomaru et al., 2013). Additionally, certain ncRNAs are aberrantly expressed in various stages or special subtypes of PCa (Li et al., 2020d; Meng et al., 2020). For instance, LINC00689 is upregulated in end-stage PCa tissues and LEF1-AS1 is significantly overexpressed in AIPC (Li et al., 2020d; Meng et al., 2020). These findings suggest that Wnt signaling pathway-related ncRNAs could potentially serve as diagnostic biomarkers for PCa. Notably, ncRNAs present in plasma will be relatively non-invasive and more convenient as diagnostic tools. Wnt signaling pathway-related lncRNAs loaded in exosomes could also potentially serve as diagnostic and therapeutic tools for PCa (Guo et al., 2022). For example, exosomal LINC01213 can serve as a diagnostic biomarker for CRPC (Guo et al., 2022).
6.2 Potential PCa prognostic biomarkers
Patient prognostic information is essential in the process of making informed treatment decisions (Lin and Farooqi, 2021). Mounting evidence suggests that the Wnt signaling pathway-related ncRNAs may hold significant potential for predicting patient prognosis (Chiyomaru et al., 2013; Dong et al., 2020; Xiu et al., 2020; Peng et al., 2021; Zhao et al., 2021). These ncRNAs are closely associated with overall survival, disease-free survival, recurrence-free survival, five-year survival rates, and progression-free survival of patients with PCa (Peng et al., 2021). For example, high expression of LINC00115 is associated with shorter overall survival and recurrence-free survival in patients with PCa (Peng et al., 2021). Additionally, Wnt signaling pathway-related ncRNAs are also associated with other prognostic factors (Chiyomaru et al., 2013; Dong et al., 2020; Xiu et al., 2020; Zhao et al., 2021). For instance, miR-34a is associated with bone metastasis of Ras-activated PCa cells (Dong et al., 2020). Furthermore, miR-574-3p is associated with advanced tumor stage and higher Gleason scores (Chiyomaru et al., 2013). In contrast, high expression of SNHG17 is associated with grade, stage, and metastasis (Zhao et al., 2021). Additionally, TUG1 is associated with Gleason score, clinical stage, preoperative PSA level, and lymph node metastasis (Xiu et al., 2020). These findings have crucial implications for the prognostic assessment and treatment selection in PCa. Therefore, Wnt signaling pathway-related ncRNAs could potentially serve as vital indicators for the prognostic evaluation and treatment selection in PCa.
6.3 Potential therapeutic targets
Cancer treatment has long been regarded as one of the most formidable challenges worldwide, despite the progress made in treatment modalities (Sung et al., 2021). Targeted therapy strategies based on ncRNAs have yielded novel insights into cancer treatment (Paunovska et al., 2022). NcRNAs regulate cell proliferation, invasion, migration, apoptosis, and stemness in PCa and conversion of PCa to CRPC by directly or indirectly interacting with the Wnt signaling pathway (Guan et al., 2017; Wang et al., 2018). Therefore, modulating the expression of Wnt signaling pathway-related ncRNAs could be an effective strategy for treating PCa and improving patient prognosis. For example, silencing miR-182 using inhibitors has been observed to significantly reduce the growth of PCa xenograft tumors, whereas silencing miR-744 using short hairpin RNA (shRNA) has been observed to significantly reduce the growth of PCa xenograft tumors (Guan et al., 2017; Wang et al., 2018). However, identifying targeted drugs that modulate ncRNA expression and stably transmit this effect remains a challenge, and necessites an enhanced understanding of the structure and function of Wnt signaling pathway-related ncRNAs. Most lncRNAs and circRNAs function as “sponges” for miRNAs to activate or deactivate the Wnt signaling pathway (Li et al., 2020d). Therefore, the regulation of target miRNA of Wnt signaling pathway-related lncRNA and circRNA or the interfering with upstream lncRNA and circRNA associated with Wnt signaling pathway-related miRNA could also be a viable treatment strategy. For example, miR-496 intervention has been observed to effectively reverse the growth-promoting effect of TUG1 on PCa xenografts (Li et al., 2020d).
Targeting ncRNAs has been considered an attractive strategy for cancer treatment (Damase et al., 2021; Garbo et al., 2022; Zogg et al., 2022). In addition to using the above approach, there are other methods that can be employed to intervene in the expression of ncRNAs involved in the Wnt pathway, which may provide therapeutic benefits for patients (Damase et al., 2021; Garbo et al., 2022; Zogg et al., 2022). In the field of Wnt pathway-related miRNAs, miRNA mimics (miRNA-like dsRNA) have been found to enhance the expression and function of certain miRNAs; meanwhile, antagomiRs can serve as tools to inhibit oncogenic miRNAs associated with the Wnt pathway, thereby blocking the specific functions of these miRNAs (Neumeier and Meister, 2020; Xu et al., 2023). In recent years, strategies based on lncRNAs for cancer treatment have gained widespread recognition. Currently, the main therapeutic approaches for managing lncRNAs involve modulating their expression levels to decrease oncogenic lncRNAs (through RNA interference methods) or increase tumor-suppressive lncRNAs (Garbo et al., 2022). It is worth noting that targeting strategies for lncRNAs need to take into account their cellular localization. Antisense oligonucleotides (ASOs) are the most effective method for targeting nuclear lncRNAs (Adewunmi et al., 2023). However, small interfering RNAs (siRNAs) are preferred for cytoplasmic lncRNAs (Li et al., 2022). Additionally, other strategies such as aptamers, nucleases, and miRNAs can be developed to disrupt lncRNA activit (Damase et al., 2021; Zogg et al., 2022). Due to their wide biological activity and stability, circRNAs have emerged as a potential and powerful therapeutic strategy that can significantly impact cancer occurrence and progression (Zong et al., 2023). However, limiting off-target effects remains a challenge in this field (Loan Young et al., 2023). Addressing this issue, specific carriers for synthetic circRNAs or siRNAs targeting junction sequences could offer substantial benefits to patients. All in all, targeted therapeutic strategies based on ncRNAs hold promise as a novel approach for PCa treatment.
6.4 Potential chemoresistance targets
Chemoresistance is a notable concern in cancer treatment, and enhancing chemosensitivity in PCa through ncRNA intervention has become a strategy that is increasingly being recognized and investigated (Chen et al., 2022). Targeting Wnt signaling pathway-related ncRNAs may help reverse chemoresistance in PCa (Liu et al., 2019a). Cisplatin, a platinum-based chemotherapeutic drug commonly used in the treatment of PCa, works by forming covalent bonds with DNA, leading to the formation of DNA cross-links and inhibiting DNA replication and transcription (Li et al., 2021). In PCa, cisplatin plays a role in inhibiting tumor growth by damaging the DNA of cancer cells and triggering apoptosis (programmed cell death) (Dhar et al., 2011). However, the development of resistance to cisplatin remains a major challenge in PCa treatment. Mechanisms underlying cisplatin resistance in PCa include enhanced DNA repair mechanisms, altered drug uptake and efflux, increased drug inactivation, and alterations in cell death pathways (Kalathil et al., 2023). miR-425-5p is downregulated in PCa and is further downregulated in cisplatin-resistant PCa (Liu et al., 2019a). Therefore, upregulating miR-425-5p by targeting the Wnt signaling pathway could potentially enhance the sensitivity of PCa to cisplatin (Liu et al., 2019a). Additionally, HOTTIP, a known oncogene, is upregulated in patients with PCa and PCa cell lines, promoting PCa cell proliferation and reducing sensitivity to cisplatin by activating the Wnt signaling pathway (Jiang et al., 2019). As a member of a class of chemotherapy drugs known as taxanes, docetaxel acts by disrupting microtubule dynamics, ultimately inhibiting cell division and inducing cell death (Sanchez-Hernandez et al., 2023). Docetaxel exerts its anti-cancer effects by targeting rapidly dividing cancer cells, inhibiting tumor growth, and promoting cancer cell death (Sanchez-Hernandez et al., 2023). In the treatment of PCa, docetaxel is particularly effective in advanced or metastatic CRPC (Gebrael et al., 2023). However, similar to cisplatin, resistance to docetaxel of PCa can develop over time. Mechanisms of docetaxel resistance in PCa involve alterations in microtubule dynamics, activation of cell survival pathways, and increased drug efflux (Gebrael et al., 2023). SNHG17 promotes chemotherapeutic resistance to docetaxel in PCa tumor cells by upregulating the Wnt signaling pathway, thereby leading to increased chemoresistance (Zhao et al., 2021). Therefore, modulation of Wnt signaling pathway-related ncRNAs may be an effective strategy to reverse chemoresistance in PCa. However, identifying targeted drugs that can regulate ncRNA expression and stably transmit this effect remains a challenge (Jaiswal et al., 2023). Therefore, an enhanced understanding of the structure and function of Wnt signaling pathway-related ncRNAs can aid the development of novel treatment strategies to reverse chemoresistance in PCa.
7 Conclusion
This review provided a comprehensive overview of the role of the Wnt signaling pathway and related ncRNAs (miRNAs, lncRNAs, and circRNAs) in PCa. MiRNAs affect the expression of target genes associated with the Wnt signaling pathway. Additionally, the Wnt signaling pathway establishes feedback mechanisms and functions as an upstream mediator of miRNAs. In most cases, lncRNAs regulate the expression of proteins in the Wnt signaling pathway by serving as sponges for miRNAs. CircRNAs also regulate the expression of the Wnt signaling pathway; however, similar to lncRNAs, they primarily regulate the expression of the Wnt signaling pathway by targeting miRNAs. We also discussed the novel avenues for the development of siRNAs and shRNAs that target the Wnt signaling pathway and their potential clinical applications. However, the limited efficacy of siRNAs and shRNAs in vivo has hindered their potential clinical application, necessitating the exploration of more reliable strategies to target ncRNAs.
Although the regulation of the Wnt signaling pathway through ncRNA intervention is a promising avenue for the treatment of PCa, certain issues need to be addressed. First, the Wnt signaling pathway is highly intricate, consisting of 19 distinct types of Wnt-secreted glycoproteins and over 15 types of Wnt receptors in humans, which activate various downstream pathways. Second, the differences and balance between the classical and non-classical Wnt signals are difficult to capture, making targeting the Wnt signaling pathway even more challenging. Third, the Wnt signaling pathway plays a fundamental role in the dynamic balance of systems, such as the digestive and hematopoietic systems; therefore, blocking the Wnt signaling pathway may lead to systemic toxicity. Hence, modulation of the Wnt signaling pathway as a therapeutic strategy for PCa is both an opportunity and a challenge, warranting further research. In the future, it will be necessary to conduct additional investigations of the interplay between the Wnt signaling pathway and ncRNAs and develop more reliable methods for targeting ncRNAs for their clinical application. Simultaneously, alternative therapeutic strategies for PCa should also be explored to improve the efficacy of treatment and the quality of life of patients.
Author contributions
TB: Writing–original draft. LL: Writing—review & editing. JT: Writing–review and editing, Supervision.
Funding
The author(s) declare that no financial support was received for the research, authorship, and/or publication of this article.
Conflict of interest
The authors declare that the research was conducted in the absence of any commercial or financial relationships that could be construed as a potential conflict of interest.
Publisher’s note
All claims expressed in this article are solely those of the authors and do not necessarily represent those of their affiliated organizations, or those of the publisher, the editors and the reviewers. Any product that may be evaluated in this article, or claim that may be made by its manufacturer, is not guaranteed or endorsed by the publisher.
References
Adewunmi, O., Shen, Y., Zhang, X. H., and Rosen, J. M. (2023). Targeted inhibition of lncRNA Malat1 alters the tumor immune microenvironment in preclinical syngeneic mouse models of triple negative breast cancer. Cancer Immunol. Res. 23, 23–45. doi:10.1158/2326-6066.CIR-23-0045
Asano, N., Takeuchi, A., Imatani, A., Saito, M., Jin, X., Hatta, W., et al. (2022). Wnt signaling and aging of the gastrointestinal tract. Int. J. Mol. Sci. 23 (20), 12210. doi:10.3390/ijms232012210
Babaei, G., Aziz, S. G., and Jaghi, N. Z. Z. (2021). EMT, cancer stem cells and autophagy; the three main axes of metastasis. Biomed. Pharmacother. 133, 110909. doi:10.1016/j.biopha.2020.110909
Bai, M., Lei, Y., Wang, M., Ma, J., Yang, P., Mou, X., et al. (2020). Long non-coding RNA SNHG17 promotes cell proliferation and invasion in castration-resistant prostate cancer by targeting the miR-144/CD51 Axis. Front. Genet. 11, 274. doi:10.3389/fgene.2020.00274
Beltran, H., Prandi, D., Mosquera, J. M., Benelli, M., Puca, L., Cyrta, J., et al. (2016). Divergent clonal evolution of castration-resistant neuroendocrine prostate cancer. Nat. Med. 22 (3), 298–305. doi:10.1038/nm.4045
Bhattacharjee, R., Prabhakar, N., Kumar, L., Bhattacharjee, A., Kar, S., Malik, S., et al. (2023). Crosstalk between long noncoding RNA and microRNA in Cancer. Cell Oncol. (Dordr) 46, 885–908. doi:10.1007/s13402-023-00806-9
Brown, A. M. (2005). Canonical Wnt signaling: high-throughput RNAi widens the path. Genome Biol. 6 (9), 231. doi:10.1186/gb-2005-6-9-231
Chen, J., Wang, F., Xu, H., Xu, L., Chen, D., Wang, J., et al. (2020). Long non-coding RNA SNHG1 regulates the wnt/β-catenin and PI3K/AKT/mTOR signaling pathways via EZH2 to affect the proliferation, apoptosis, and autophagy of prostate cancer cell. Front. Oncol. 10, 552907. doi:10.3389/fonc.2020.552907
Chen, L., Song, Y., Hou, T., Li, X., Cheng, L., Li, Y., et al. (2022). Circ_0004087 interaction with SND1 promotes docetaxel resistance in prostate cancer by boosting the mitosis error correction mechanism. J. Exp. Clin. Cancer Res. 41 (1), 194. doi:10.1186/s13046-022-02404-3
Chen, W. Y., Liu, S. Y., Chang, Y. S., Yin, J. J., Yeh, H. L., Mouhieddine, T. H., et al. (2015). MicroRNA-34a regulates WNT/TCF7 signaling and inhibits bone metastasis in Ras-activated prostate cancer. Oncotarget 6 (1), 441–457. doi:10.18632/oncotarget.2690
Chen, X., Zeh, H. J., Kang, R., Kroemer, G., and Tang, D. (2021). Cell death in pancreatic cancer: from pathogenesis to therapy. Nat. Rev. Gastroenterol. Hepatol. 18 (11), 804–823. doi:10.1038/s41575-021-00486-6
Chiyomaru, T., Yamamura, S., Fukuhara, S., Hidaka, H., Majid, S., Saini, S., et al. (2013). Genistein up-regulates tumor suppressor microRNA-574-3p in prostate cancer. PLoS One 8 (3), e58929. doi:10.1371/journal.pone.0058929
Coleman, R. E., Croucher, P. I., Padhani, A. R., Clezardin, P., Chow, E., Fallon, M., et al. (2020). Bone metastases. Nat. Rev. Dis. Prim. 6 (1), 83. doi:10.1038/s41572-020-00216-3
Cui, Y., Yang, Y., Ren, L., Yang, J., Wang, B., Xing, T., et al. (2019). miR-15a-3p suppresses prostate cancer cell proliferation and invasion by targeting SLC39A7 via downregulating wnt/β-catenin signaling pathway. Cancer Biother Radiopharm. 34 (7), 472–479. doi:10.1089/cbr.2018.2722
Damase, T. R., Sukhovershin, R., Boada, C., Taraballi, F., Pettigrew, R. I., and Cooke, J. P. (2021). The limitless future of RNA therapeutics. Front. Bioeng. Biotechnol. 9, 628137. doi:10.3389/fbioe.2021.628137
De Nunzio, C., Kramer, G., Marberger, M., Montironi, R., Nelson, W., Schroder, F., et al. (2011). The controversial relationship between benign prostatic hyperplasia and prostate cancer: the role of inflammation. Eur. Urol. 60 (1), 106–117. doi:10.1016/j.eururo.2011.03.055
Desai, M. M., Cacciamani, G. E., Gill, K., Zhang, J., Liu, L., Abreu, A., et al. (2022). Trends in incidence of metastatic prostate cancer in the US. JAMA Netw. Open 5 (3), e222246. doi:10.1001/jamanetworkopen.2022.2246
Dhar, S., Kolishetti, N., Lippard, S. J., and Farokhzad, O. C. (2011). Targeted delivery of a cisplatin prodrug for safer and more effective prostate cancer therapy in vivo. Proc. Natl. Acad. Sci. U. S. A. 108 (5), 1850–1855. doi:10.1073/pnas.1011379108
Doghish, A. S., Ismail, A., El-Mahdy, H. A., Elkady, M. A., Elrebehy, M. A., and Sallam, A. M. (2022). A review of the biological role of miRNAs in prostate cancer suppression and progression. Int. J. Biol. Macromol. 197, 141–156. doi:10.1016/j.ijbiomac.2021.12.141
Dong, B., Xu, G. C., Liu, S. T., Liu, T., and Geng, B. (2020). MiR-34a affects G2 arrest in prostate cancer PC3 cells via Wnt pathway and inhibits cell growth and migration. Eur. Rev. Med. Pharmacol. Sci. 24 (16), 8349–8358. doi:10.26355/eurrev_202008_22631
Du, W., Chen, W., Shu, Z., Xiang, D., Bi, K., Lu, Y., et al. (2020). Identification of prognostic biomarkers of hepatocellular carcinoma via long noncoding RNA expression and copy number alterations. Epigenomics 12 (15), 1303–1315. doi:10.2217/epi-2019-0385
Eismann, L., von Walter, P., Jung, A., Chaloupka, M., Rodler, S., Westhofen, T., et al. (2023). Methylation status of various gene loci in localized prostate cancer: novel biomarkers for diagnostics and biochemical recurrence. Urol. Oncol. 41, 325.e1–325325.e8. doi:10.1016/j.urolonc.2023.04.009
Fakhr, E., Zare, F., Azadmanesh, K., and Teimoori-Toolabi, L. (2021). LEF1 silencing sensitizes colorectal cancer cells to oxaliplatin, 5-FU, and irinotecan. Biomed. Pharmacother. 143, 112091. doi:10.1016/j.biopha.2021.112091
Fares, J., Fares, M. Y., Khachfe, H. H., Salhab, H. A., and Fares, Y. (2020). Molecular principles of metastasis: a hallmark of cancer revisited. Signal Transduct. Target Ther. 5 (1), 28. doi:10.1038/s41392-020-0134-x
Fedele, M., Sgarra, R., Battista, S., Cerchia, L., and Manfioletti, G. (2022). The epithelial-mesenchymal transition at the crossroads between metabolism and tumor progression. Int. J. Mol. Sci. 23 (2), 800. doi:10.3390/ijms23020800
Ferri, C., Di Biase, A., Bocchetti, M., Zappavigna, S., Wagner, S., Le Vu, P., et al. (2022). MiR-423-5p prevents MALAT1-mediated proliferation and metastasis in prostate cancer. J. Exp. Clin. Cancer Res. 41 (1), 20. doi:10.1186/s13046-021-02233-w
Fletcher, C. E., Deng, L., Orafidiya, F., Yuan, W., Lorentzen, M., Cyran, O. W., et al. (2022). A non-coding RNA balancing act: miR-346-induced DNA damage is limited by the long non-coding RNA NORAD in prostate cancer. Mol. Cancer 21 (1), 82. doi:10.1186/s12943-022-01540-w
Fu, Q., Gao, Y., Yang, F., Mao, T., Sun, Z., Wang, H., et al. (2018). Suppression of microRNA-454 impedes the proliferation and invasion of prostate cancer cells by promoting N-myc downstream-regulated gene 2 and inhibiting WNT/β-catenin signaling. Biomed. Pharmacother. 97, 120–127. doi:10.1016/j.biopha.2017.10.115
Fu, Q., Sun, Z., Yang, F., Mao, T., Gao, Y., and Wang, H. (2019). SOX30, a target gene of miR-653-5p, represses the proliferation and invasion of prostate cancer cells through inhibition of Wnt/β-catenin signaling. Cell Mol. Biol. Lett. 24, 71. doi:10.1186/s11658-019-0195-4
Garbo, S., Maione, R., Tripodi, M., and Battistelli, C. (2022). Next RNA therapeutics: the mine of non-coding. Int. J. Mol. Sci. 23 (13), 7471. doi:10.3390/ijms23137471
Gebrael, G., Fortuna, G. G., Sayegh, N., Swami, U., and Agarwal, N. (2023). Advances in the treatment of metastatic prostate cancer. Trends Cancer 23, 2405–8033. doi:10.1016/j.trecan.2023.06.009
Ghafouri-Fard, S., Dinger, M. E., Maleki, P., Taheri, M., and Hajiesmaeili, M. (2021). Emerging role of circular RNAs in the pathobiology of lung cancer. Biomed. Pharmacother. 141, 111805. doi:10.1016/j.biopha.2021.111805
Goodall, G. J., and Wickramasinghe, V. O. (2021). RNA in cancer. Nat. Rev. Cancer 21 (1), 22–36. doi:10.1038/s41568-020-00306-0
Guan, H., Liu, C., Fang, F., Huang, Y., Tao, T., Ling, Z., et al. (2017). MicroRNA-744 promotes prostate cancer progression through aberrantly activating Wnt/β-catenin signaling. Oncotarget 8 (9), 14693–14707. doi:10.18632/oncotarget.14711
Guo, Z., Lu, X., Yang, F., He, C., Qin, L., Yang, N., et al. (2022). Exosomal LINC01213 plays a role in the transition of androgen-dependent prostate cancer cells into androgen-independent manners. J. Oncol. 2022, 8058770. doi:10.1155/2022/8058770
Guo-Wei, H., Chun-Quan, L., Lian-Di, L., Ji-Wei, J., Lin, L., Ji-Yu, D., et al. (2019). LncRNA625 inhibits STAT1-mediated transactivation potential in esophageal cancer cells. Int. J. Biochem. Cell Biol. 117, 105626. doi:10.1016/j.biocel.2019.105626
Hao, Y., Baker, D., and Ten Dijke, P. (2019). TGF-beta-Mediated epithelial-mesenchymal transition and cancer metastasis. Int. J. Mol. Sci. 20 (11), 2767. doi:10.3390/ijms20112767
He, P., Xiong, G., Guo, W., Jiang, G., Li, Y., and Li, H. (2020). Long non-coding RNA CCAT2 promotes prostate cancer cell proliferation and invasion by regulating the Wnt/β-catenin signaling pathway. Oncol. Lett. 20 (4), 97. doi:10.3892/ol.2020.11958
He, Y., Xu, W., Xiao, Y. T., Huang, H., Gu, D., and Ren, S. (2022). Targeting signaling pathways in prostate cancer: mechanisms and clinical trials. Signal Transduct. Target Ther. 7 (1), 198. doi:10.1038/s41392-022-01042-7
Hsieh, I. S., Chang, K. C., Tsai, Y. T., Ke, J. Y., Lu, P. J., Lee, K. H., et al. (2013). MicroRNA-320 suppresses the stem cell-like characteristics of prostate cancer cells by downregulating the Wnt/beta-catenin signaling pathway. Carcinogenesis 34 (3), 530–538. doi:10.1093/carcin/bgs371
Hu, C. Y., Chen, J., Qin, X. H., You, P., Ma, J., Zhang, J., et al. (2021). Long non-coding RNA NORAD promotes the prostate cancer cell extracellular vesicle release via microRNA-541-3p-regulated PKM2 to induce bone metastasis of prostate cancer. J. Exp. Clin. Cancer Res. 40 (1), 98. doi:10.1186/s13046-021-01891-0
Huang, L. A., Lin, C., and Yang, L. (2023). Plumbing mysterious RNAs in "dark genome" for the conquest of human diseases. Mol. Ther. 31, 1577–1595. doi:10.1016/j.ymthe.2023.05.003
Jaiswal, A., Kaushik, N., Choi, E. H., and Kaushik, N. K. (2023). Functional impact of non-coding RNAs in high-grade breast carcinoma: Moving from resistance to clinical applications: A comprehensive review. Biochim. Biophys. Acta Rev. Cancer 1878 (4), 188915. doi:10.1016/j.bbcan.2023.188915
Jiang, H., Xiong, W., Chen, L., Lv, Z., Yang, C., and Li, Y. (2019). Knockdown of the long noncoding RNA HOTTIP inhibits cell proliferation and enhances cell sensitivity to cisplatin by suppressing the Wnt/β-catenin pathway in prostate cancer. J. Cell Biochem. 120 (6), 8965–8974. doi:10.1002/jcb.27851
Jiang, Y., Zhao, H., Chen, Y., Li, K., Li, T., Chen, J., et al. (2021). Exosomal long noncoding RNA HOXD-AS1 promotes prostate cancer metastasis via miR-361-5p/FOXM1 axis. Cell Death Dis. 12 (12), 1129. doi:10.1038/s41419-021-04421-0
Kalathil, A. A., Guin, S., Ashokan, A., Basu, U., Surnar, B., Delma, K. S., et al. (2023). New pathway for cisplatin prodrug to utilize metabolic substrate preference to overcome cancer intrinsic resistance. ACS Cent. Sci. 9 (7), 1297–1312. doi:10.1021/acscentsci.3c00286
Kalluri, R., and LeBleu, V. S. (2020). The biology, function, and biomedical applications of exosomes. Science 367 (6478), eaau6977. doi:10.1126/science.aau6977
Kerr, J. F., Wyllie, A. H., and Currie, A. R. (1972). Apoptosis: a basic biological phenomenon with wide-ranging implications in tissue kinetics. Br. J. Cancer 26 (4), 239–257. doi:10.1038/bjc.1972.33
Khurana, N., and Sikka, S. C. (2019). Interplay between SOX9, wnt/β-catenin and androgen receptor signaling in castration-resistant prostate cancer. Int. J. Mol. Sci. 20 (9), 2066. doi:10.3390/ijms20092066
Kim, T., and Croce, C. M. (2021). MicroRNA and ER stress in cancer. Semin. Cancer Biol. 75, 3–14. doi:10.1016/j.semcancer.2020.12.025
Kolakofsky, D. (1976). Isolation and characterization of Sendai virus DI-RNAs. Cell 8 (4), 547–555. doi:10.1016/0092-8674(76)90223-3
Koushyar, S., Meniel, V. S., Phesse, T. J., and Pearson, H. B. (2022). Exploring the Wnt pathway as a therapeutic target for prostate cancer. Biomolecules 12 (2), 309. doi:10.3390/biom12020309
Lagos-Quintana, M., Rauhut, R., Lendeckel, W., and Tuschl, T. (2001). Identification of novel genes coding for small expressed RNAs. Science 294 (5543), 853–858. doi:10.1126/science.1064921
Lan, T., Ma, W., Hong, Z., Wu, L., Chen, X., and Yuan, Y. (2017). Long non-coding RNA small nucleolar RNA host gene 12 (SNHG12) promotes tumorigenesis and metastasis by targeting miR-199a/b-5p in hepatocellular carcinoma. J. Exp. Clin. Cancer Res. 36 (1), 11. doi:10.1186/s13046-016-0486-9
Li, F., Ainiwaer, J. L., Sheyhiding, I., Zhang, Z., and Zhang, L. W. (2016a). Identification of key long non-coding RNAs as competing endogenous RNAs for miRNA-mRNA in lung adenocarcinoma. Eur. Rev. Med. Pharmacol. Sci. 20 (11), 2285–2295.
Li, F., Gu, C., Tian, F., Jia, Z., Meng, Z., Ding, Y., et al. (2016b). MiR-218 impedes IL-6-induced prostate cancer cell proliferation and invasion via suppression of LGR4 expression. Oncol. Rep. 35 (5), 2859–2865. doi:10.3892/or.2016.4663
Li, J. B., Liu, F., Zhang, B. P., Bai, W. K., Cheng, W., Zhang, Y. H., et al. (2017). LncRNA625 modulates prostate cancer cells proliferation and apoptosis through regulating the Wnt/β-catenin pathway by targeting miR-432. Eur. Rev. Med. Pharmacol. Sci. 21 (11), 2586–2595.
Li, M., Chen, X., Wang, X., Wei, X., Wang, D., Liu, X., et al. (2021). RSL3 enhances the antitumor effect of cisplatin on prostate cancer cells via causing glycolysis dysfunction. Biochem. Pharmacol. 192, 114741. doi:10.1016/j.bcp.2021.114741
Li, P. Y., Yang, J., Chong, T., Huang, Y., Liu, Y., and Li, H. (2020a). TUG1 knockdown inhibits the tumorigenesis and progression of prostate cancer by regulating microRNA-496/Wnt/β-catenin pathway. Anticancer Drugs 31 (6), 592–600. doi:10.1097/CAD.0000000000000882
Li, P. Y., Wang, P., Gao, S. G., and Dong, D. Y. (2020b). Long noncoding RNA SOX2-OT: regulations, functions, and roles on mental illnesses, cancers, and diabetic complications. Biomed. Res. Int. 2020, 2901589. doi:10.1155/2020/2901589
Li, P. Y., Yang, G., Yang, D., Li, D., and Sun, Q. (2020d). LncRNA LEF1-AS1 promotes metastasis of prostatic carcinoma via the Wnt/β-catenin pathway. Cancer Cell Int. 20 (1), 543. doi:10.1186/s12935-020-01624-x
Li, P. Y., Yu, C., Zhang, Y., Liu, J., Jia, Y., Sun, F., et al. (2020c). Circular RNA cir-ITCH is a potential therapeutic target for the treatment of castration-resistant prostate cancer. Biomed. Res. Int. 2020, 7586521. doi:10.1155/2020/7586521
Li, Y., Li, F., Pan, H., Huang, X., Yu, J., Liu, X., et al. (2022). Targeted OUM1/PTPRZ1 silencing and synergetic CDT/enhanced chemical therapy toward uveal melanoma based on a dual-modal imaging-guided manganese metal-organic framework nanoparticles. J. Nanobiotechnology 20 (1), 472. doi:10.1186/s12951-022-01643-y
Liang, J., Li, Y., Daniels, G., Sfanos, K., De Marzo, A., Wei, J., et al. (2015). LEF1 targeting EMT in prostate cancer invasion is regulated by miR-34a. Mol. Cancer Res. 13 (4), 681–688. doi:10.1158/1541-7786.MCR-14-0503
Liang, X., Li, H., Fu, D., Chong, T., Wang, Z., and Li, Z. (2016). MicroRNA-1297 inhibits prostate cancer cell proliferation and invasion by targeting the AEG-1/Wnt signaling pathway. Biochem. Biophys. Res. Commun. 480 (2), 208–214. doi:10.1016/j.bbrc.2016.10.029
Liao, W., Du, J., Li, L., Wu, X., Chen, X., Feng, Q., et al. (2023). CircZNF215 promotes tumor growth and metastasis through inactivation of the PTEN/AKT pathway in intrahepatic cholangiocarcinoma. J. Exp. Clin. Cancer Res. 42 (1), 125. doi:10.1186/s13046-023-02699-w
Lichner, Z., Ding, Q., Samaan, S., Saleh, C., Nasser, A., Al-Haddad, S., et al. (2015). miRNAs dysregulated in association with Gleason grade regulate extracellular matrix, cytoskeleton and androgen receptor pathways. J. Pathol. 237 (2), 226–237. doi:10.1002/path.4568
Lin, S. R., Mokgautsi, N., and Liu, Y. N. (2020). Ras and Wnt interaction contribute in prostate cancer bone metastasis. Molecules 25 (10), 2380. doi:10.3390/molecules25102380
Lin, X., and Farooqi, A. A. (2021). Cucurbitacin mediated regulation of deregulated oncogenic signaling cascades and non-coding RNAs in different cancers: spotlight on JAK/STAT, wnt/β-catenin, mTOR, TRAIL-mediated pathways. Semin. Cancer Biol. 73, 302–309. doi:10.1016/j.semcancer.2020.10.012
Ling, H., Spizzo, R., Atlasi, Y., Nicoloso, M., Shimizu, M., Redis, R. S., et al. (2013). CCAT2, a novel noncoding RNA mapping to 8q24, underlies metastatic progression and chromosomal instability in colon cancer. Genome Res. 23 (9), 1446–1461. doi:10.1101/gr.152942.112
Liu, H., Yin, J., Wang, H., Jiang, G., Deng, M., Zhang, G., et al. (2015). FOXO3a modulates WNT/β-catenin signaling and suppresses epithelial-to-mesenchymal transition in prostate cancer cells. Cell Signal 27 (3), 510–518. doi:10.1016/j.cellsig.2015.01.001
Liu, J., Wei, W., Guo, C. A., Han, N., Pan, J. F., Fei, T., et al. (2013). Stat3 upregulates leucine-rich repeat-containing g protein-coupled receptor 4 expression in osteosarcoma cells. Biomed. Res. Int. 2013, 310691. doi:10.1155/2013/310691
Liu, S. J., Dang, H. X., Lim, D. A., Feng, F. Y., and Maher, C. A. (2021). Long noncoding RNAs in cancer metastasis. Nat. Rev. Cancer 21 (7), 446–460. doi:10.1038/s41568-021-00353-1
Liu, S., Wang, Q., Liu, Y., and Xia, Z. Y. (2019a). miR-425-5p suppresses tumorigenesis and DDP resistance in human-prostate cancer by targeting GSK3β and inactivating the Wnt/β-catenin signaling pathway. J. Biosci. 44 (4), 102. doi:10.1007/s12038-019-9920-4
Liu, S., Zhu, Q., Guo, Y., Xiao, Z., Hu, L., and Xu, Q. (2019b). LncRNA LINC00689 promotes the growth, metastasis and glycolysis of glioma cells by targeting miR-338-3p/PKM2 axis. Biomed. Pharmacother. 117, 109069. doi:10.1016/j.biopha.2019.109069
Loan Young, T., Chang Wang, K., James Varley, A., and Li, B. (2023). Clinical delivery of circular RNA: lessons learned from RNA drug development. Adv. Drug Deliv. Rev. 197, 114826. doi:10.1016/j.addr.2023.114826
Lu, H., Zhang, Q., Sun, Y., Wu, D., and Liu, L. (2020). LINC00689 induces gastric cancer progression via modulating the miR-338-3p/HOXA3 axis. J. Gene Med. 22 (12), e3275. doi:10.1002/jgm.3275
Luo, J., Wang, D., Wan, X., Xu, Y., Lu, Y., Kong, Z., et al. (2020). Crosstalk between AR and Wnt signaling promotes castration-resistant prostate cancer growth. Onco Targets Ther. 13, 9257–9267. doi:10.2147/OTT.S245861
Mangolini, A., Rocca, C., Bassi, C., Ippolito, C., Negrini, M., Dell'Atti, L., et al. (2022). Detection of disease-causing mutations in prostate cancer by NGS sequencing. Cell Biol. Int. 46 (7), 1047–1061. doi:10.1002/cbin.11803
Mattick, J. S., Amaral, P. P., Carninci, P., Carpenter, S., Chang, H. Y., Chen, L. L., et al. (2023). Long non-coding RNAs: definitions, functions, challenges and recommendations. Nat. Rev. Mol. Cell Biol. 24 (6), 430–447. doi:10.1038/s41580-022-00566-8
Memczak, S., Jens, M., Elefsinioti, A., Torti, F., Krueger, J., Rybak, A., et al. (2013). Circular RNAs are a large class of animal RNAs with regulatory potency. Nature 495 (7441), 333–338. doi:10.1038/nature11928
Menck, K., Heinrichs, S., Baden, C., and Bleckmann, A. (2021). The WNT/ROR pathway in cancer: from signaling to therapeutic intervention. Cells 10 (1), 142. doi:10.3390/cells10010142
Meng, L., Li, Z., Chen, Y., Liu, D., and Liu, Z. (2020). LINC00689 promotes prostate cancer progression via regulating miR-496/CTNNB1 to activate Wnt pathway. Cancer Cell Int. 20, 215. doi:10.1186/s12935-020-01280-1
Misso, G., Di Martino, M. T., De Rosa, G., Farooqi, A. A., Lombardi, A., Campani, V., et al. (2014). Mir-34: a new weapon against cancer? Mol. Ther. Nucleic Acids 3, e194. doi:10.1038/mtna.2014.47
Movahedpour, A., Khatami, S. H., Karami, N., Vakili, O., Naeli, P., Jamali, Z., et al. (2022). Exosomal noncoding RNAs in prostate cancer. Clin. Chim. Acta 537, 127–132. doi:10.1016/j.cca.2022.10.018
Mugoni, V., Ciani, Y., Nardella, C., and Demichelis, F. (2022). Circulating RNAs in prostate cancer patients. Cancer Lett. 524, 57–69. doi:10.1016/j.canlet.2021.10.011
Murota, Y., Tabu, K., and Taga, T. (2022). Cancer stem cell-associated immune microenvironment in recurrent glioblastomas. Cells 11 (13), 2054. doi:10.3390/cells11132054
Neumeier, J., and Meister, G. (2020). siRNA specificity: RNAi mechanisms and strategies to reduce off-target effects. Front. Plant Sci. 11, 526455. doi:10.3389/fpls.2020.526455
Nusse, R., and Clevers, H. (2017). Wnt/β-Catenin signaling, disease, and emerging therapeutic modalities. Cell 169 (6), 985–999. doi:10.1016/j.cell.2017.05.016
Orafidiya, F., Deng, L., Bevan, C. L., and Fletcher, C. E. (2022). Crosstalk between long non coding RNAs, microRNAs and DNA damage repair in prostate cancer: new therapeutic opportunities? Cancers (Basel) 14 (3), 755. doi:10.3390/cancers14030755
Pakula, H., Xiang, D., and Li, Z. (2017). A tale of two signals: AR and WNT in development and tumorigenesis of prostate and mammary gland. Cancers (Basel) 9 (2), 14. doi:10.3390/cancers9020014
Park, W. J., and Kim, M. J. (2023). A new wave of targeting 'undruggable' Wnt signaling for cancer therapy: challenges and opportunities. Cells 12 (8), 1110. doi:10.3390/cells12081110
Paunovska, K., Loughrey, D., and Dahlman, J. E. (2022). Drug delivery systems for RNA therapeutics. Nat. Rev. Genet. 23 (5), 265–280. doi:10.1038/s41576-021-00439-4
Pegtel, D. M., and Gould, S. J. (2019). Exosomes. Annu. Rev. Biochem. 88, 487–514. doi:10.1146/annurev-biochem-013118-111902
Peng, N., Zhang, Z., Wang, Y., Yang, M., Fan, J., Wang, Q., et al. (2021). Down-regulated LINC00115 inhibits prostate cancer cell proliferation and invasion via targeting miR-212-5p/FZD5/Wnt/β-catenin axis. J. Cell Mol. Med. 25 (22), 10627–10637. doi:10.1111/jcmm.17000
Petrone, I., Santos, E. C. D., Binato, R., and Abdelhay, E. (2023). Epigenetic alterations in DCIS progression: what can lncRNAs teach us? Int. J. Mol. Sci. 24 (10), 8733. doi:10.3390/ijms24108733
Pisano, C., Tucci, M., Di Stefano, R. F., Turco, F., Scagliotti, G. V., Di Maio, M., et al. (2021). Interactions between androgen receptor signaling and other molecular pathways in prostate cancer progression: current and future clinical implications. Crit. Rev. Oncol. Hematol. 157, 103185. doi:10.1016/j.critrevonc.2020.103185
Ramnarine, V. R., Kobelev, M., Gibb, E. A., Nouri, M., Lin, D., Wang, Y., et al. (2019). The evolution of long noncoding RNA acceptance in prostate cancer initiation, progression, and its clinical utility in disease management. Eur. Urol. 76 (5), 546–559. doi:10.1016/j.eururo.2019.07.040
Ren, W., Wang, D., Li, C., Shu, T., Zhang, W., and Fu, X. (2018). Capn4 expression is modulated by microRNA-520b and exerts an oncogenic role in prostate cancer cells by promoting Wnt/β-catenin signaling. Biomed. Pharmacother. 108, 467–475. doi:10.1016/j.biopha.2018.09.019
Reya, T., and Clevers, H. (2005). Wnt signalling in stem cells and cancer. Nature 434 (7035), 843–850. doi:10.1038/nature03319
Rim, E. Y., Clevers, H., and Nusse, R. (2022). The Wnt pathway: from signaling mechanisms to synthetic modulators. Annu. Rev. Biochem. 91, 571–598. doi:10.1146/annurev-biochem-040320-103615
Sanchez-Hernandez, E. S., Ochoa, P. T., Suzuki, T., Ortiz-Hernandez, G. L., Unternaehrer, J. J., Alkashgari, H. R., et al. (2023). Glucocorticoid receptor regulates and interacts with LEDGF/p75 to promote docetaxel resistance in prostate cancer cells. Cells 12 (16), 2046. doi:10.3390/cells12162046
Sandhu, S., Moore, C. M., Chiong, E., Beltran, H., Bristow, R. G., and Williams, S. G. (2021). Prostate cancer. Lancet 398 (10305), 1075–1090. doi:10.1016/S0140-6736(21)00950-8
Sarabia-Sanchez, M. A., Moreno-Londono, A. P., Castaneda-Patlan, M. C., Alvarado-Ortiz, E., Martinez-Morales, J. C., and Robles-Flores, M. (2023). Non-canonical Wnt/Ca2+ signaling is essential to promote self-renewal and proliferation in colon cancer stem cells. Front. Oncol. 13, 1121787. doi:10.3389/fonc.2023.1121787
Shafi, A. A., Yen, A. E., and Weigel, N. L. (2013). Androgen receptors in hormone-dependent and castration-resistant prostate cancer. Pharmacol. Ther. 140 (3), 223–238. doi:10.1016/j.pharmthera.2013.07.003
Singh, R., Chandrashekharappa, S., Bodduluri, S. R., Baby, B. V., Hegde, B., Kotla, N. G., et al. (2019). Enhancement of the gut barrier integrity by a microbial metabolite through the Nrf2 pathway. Nat. Commun. 10 (1), 89. doi:10.1038/s41467-018-07859-7
Soghli, N., Yousefi, T., Abolghasemi, M., and Qujeq, D. (2021). NORAD, a critical long non-coding RNA in human cancers. Life Sci. 264, 118665. doi:10.1016/j.lfs.2020.118665
Sonawala, K., Ramalingam, S., and Sellamuthu, I. (2022). Influence of long non-coding RNA in the regulation of cancer stem cell signaling pathways. Cells 11 (21), 3492. doi:10.3390/cells11213492
Song, J., Wu, X., Ma, R., Miao, L., Xiong, L., and Zhao, W. (2019). Long noncoding RNA SNHG12 promotes cell proliferation and activates Wnt/β-catenin signaling in prostate cancer through sponging microRNA-195. J. Cell Biochem. 120 (8), 13066–13075. doi:10.1002/jcb.28578
Song, X. L., Huang, B., Zhou, B. W., Wang, C., Liao, Z. W., Yu, Y., et al. (2018). miR-1301-3p promotes prostate cancer stem cell expansion by targeting SFRP1 and GSK3β. Biomed. Pharmacother. 99, 369–374. doi:10.1016/j.biopha.2018.01.086
Song, X., Wang, H., Wu, J., and Sun, Y. (2020). Long noncoding RNA SOX2-OT knockdown inhibits proliferation and metastasis of prostate cancer cells through modulating the miR-452-5p/HMGB3 Axis and inactivating wnt/β-catenin pathway. Cancer Biother Radiopharm. 35 (9), 682–695. doi:10.1089/cbr.2019.3479
Sung, H., Ferlay, J., Siegel, R. L., Laversanne, M., Soerjomataram, I., Jemal, A., et al. (2021). Global cancer statistics 2020: GLOBOCAN estimates of incidence and mortality worldwide for 36 cancers in 185 countries. CA Cancer J. Clin. 71 (3), 209–249. doi:10.3322/caac.21660
Szaflik, T., Romanowicz, H., Szyllo, K., Kolacinski, R., Michalska, M. M., Samulak, D., et al. (2022). Analysis of long non-coding RNA (lncRNA) UCA1, MALAT1, TC0101441, and H19 expression in endometriosis. Int. J. Mol. Sci. 23 (19), 11583. doi:10.3390/ijms231911583
Tamtaji, O. R., Derakhshan, M., Rashidi Noshabad, F. Z., Razaviyan, J., Hadavi, R., Jafarpour, H., et al. (2021). Non-coding RNAs and brain tumors: insights into their roles in apoptosis. Front. Cell Dev. Biol. 9, 792185. doi:10.3389/fcell.2021.792185
Tan, Y. T., Lin, J. F., Li, T., Li, J. J., Xu, R. H., and Ju, H. Q. (2021). LncRNA-mediated posttranslational modifications and reprogramming of energy metabolism in cancer. Cancer Commun. (Lond) 41 (2), 109–120. doi:10.1002/cac2.12108
Vallee, A., and Lecarpentier, Y. (2018). Crosstalk between peroxisome proliferator-activated receptor gamma and the canonical WNT/β-Catenin pathway in chronic inflammation and oxidative stress during carcinogenesis. Front. Immunol. 9, 745. doi:10.3389/fimmu.2018.00745
VanderVorst, K., Dreyer, C. A., Hatakeyama, J., Bell, G. R. R., Learn, J. A., Berg, A. L., et al. (2023). Vangl-dependent Wnt/planar cell polarity signaling mediates collective breast carcinoma motility and distant metastasis. Breast Cancer Res. 25 (1), 52. doi:10.1186/s13058-023-01651-2
Wang, D., Lu, G., Shao, Y., and Xu, D. (2018). MiR-182 promotes prostate cancer progression through activating Wnt/β-catenin signal pathway. Biomed. Pharmacother. 99, 334–339. doi:10.1016/j.biopha.2018.01.082
Wang, L., Wang, L., Wang, Q., Yosefi, B., Wei, S., Wang, X., et al. (2021). The function of long noncoding RNA HOTAIRM1 in the progression of prostate cancer cells. Andrologia 53 (2), e13897. doi:10.1111/and.13897
Wang, L., Xu, G. E., Spanos, M., Li, G., Lei, Z., Sluijter, J. P. G., et al. (2023). Circular RNAs in cardiovascular diseases: regulation and therapeutic applications. Res. (Wash D C) 6, 0038. doi:10.34133/research.0038
Wang, Q., Cao, L., Zhou, S., Lyu, J., Gao, Y., and Yang, R. (2022b). Construction and validation of a novel pyroptosis-related four-lncRNA prognostic signature related to gastric cancer and immune infiltration. Front. Immunol. 13, 854785. doi:10.3389/fimmu.2022.854785
Wang, Q., Feng, J., and Tang, L. (2022a). Non-coding RNA related to MAPK signaling pathway in liver cancer. Int. J. Mol. Sci. 23 (19), 11908. doi:10.3390/ijms231911908
Wang, X., He, C., Yang, Z., Li, S., Qiao, L., and Fang, L. (2019). Dysregulation of long non-coding RNA SNHG12 alters the viability, apoptosis, and autophagy of prostate cancer cells by regulating miR-195/CCNE1 axis. Int. J. Clin. Exp. Pathol. 12 (4), 1272–1283.
Wei, M., Ma, Y., Shen, L., Xu, Y., Liu, L., Bu, X., et al. (2020). NDRG2 regulates adherens junction integrity to restrict colitis and tumourigenesis. EBioMedicine 61, 103068. doi:10.1016/j.ebiom.2020.103068
Wu, Z., Lu, Z., Li, L., Ma, M., Long, F., Wu, R., et al. (2021). Identification and validation of ferroptosis-related LncRNA signatures as a novel prognostic model for colon cancer. Front. Immunol. 12, 783362. doi:10.3389/fimmu.2021.783362
Xie, Z., Zhong, C., Shen, J., Jia, Y., and Duan, S. (2022). LINC00963: A potential cancer diagnostic and therapeutic target. Biomed. Pharmacother. 150, 113019. doi:10.1016/j.biopha.2022.113019
Xin, H., Li, C., and Wang, M. (2018). DIXDC1 promotes the growth of acute myeloid leukemia cells by upregulating the Wnt/β-catenin signaling pathway. Biomed. Pharmacother. 107, 1548–1555. doi:10.1016/j.biopha.2018.08.144
Xiong, S. D., Yu, K., Liu, X. H., Yin, L. H., Kirschenbaum, A., Yao, S., et al. (2009). Ribosome-inactivating proteins isolated from dietary bitter melon induce apoptosis and inhibit histone deacetylase-1 selectively in premalignant and malignant prostate cancer cells. Int. J. Cancer 125 (4), 774–782. doi:10.1002/ijc.24325
Xiu, D., Liu, L., Cheng, M., Sun, X., and Ma, X. (2020). Knockdown of lncRNA TUG1 enhances radiosensitivity of prostate cancer via the TUG1/miR-139-5p/SMC1A Axis. Onco Targets Ther. 13, 2319–2331. doi:10.2147/OTT.S236860
Xu, M., Lin, J., Yang, S., Yao, J., Chen, M., Feng, J., et al. (2023). Epstein-Barr virus-encoded miR-BART11-3p modulates the DUSP6-MAPK axis to promote gastric cancer cell proliferation and metastasis. J. Virol. 23, e0088123. doi:10.1128/jvi.00881-23
Xue, C., Li, G., Zheng, Q., Gu, X., Bao, Z., Lu, J., et al. (2022). The functional roles of the circRNA/Wnt axis in cancer. Mol. Cancer 21 (1), 108. doi:10.1186/s12943-022-01582-0
Yan, H., and Bu, P. (2021). Non-coding RNA in cancer. Essays Biochem. 65 (4), 625–639. doi:10.1042/EBC20200032
Yang, Y., Yujiao, W., Fang, W., Linhui, Y., Ziqi, G., Zhichen, W., et al. (2020). The roles of miRNA, lncRNA and circRNA in the development of osteoporosis. Biol. Res. 53 (1), 40. doi:10.1186/s40659-020-00309-z
Yap, T. A., Zivi, A., Omlin, A., and de Bono, J. S. (2011). The changing therapeutic landscape of castration-resistant prostate cancer. Nat. Rev. Clin. Oncol. 8 (10), 597–610. doi:10.1038/nrclinonc.2011.117
Yeh, Y., Guo, Q., Connelly, Z., Cheng, S., Yang, S., Prieto-Dominguez, N., et al. (2019). Wnt/beta-catenin signaling and prostate cancer therapy resistance. Adv. Exp. Med. Biol. 1210, 351–378. doi:10.1007/978-3-030-32656-2_16
Young, T. L., Matsuda, T., and Cepko, C. L. (2005). The noncoding RNA taurine upregulated gene 1 is required for differentiation of the murine retina. Curr. Biol. 15 (6), 501–512. doi:10.1016/j.cub.2005.02.027
Yu, F., Yu, C., Li, F., Zuo, Y., Wang, Y., Yao, L., et al. (2021). Wnt/β-catenin signaling in cancers and targeted therapies. Signal Transduct. Target Ther. 6 (1), 307. doi:10.1038/s41392-021-00701-5
Yu, Z., Wang, Z., Li, F., Yang, J., and Tang, L. (2018). miR-138 modulates prostate cancer cell invasion and migration via Wnt/β‑catenin pathway. Mol. Med. Rep. 17 (2), 3140–3145. doi:10.3892/mmr.2017.8273
Zhang, X., Weissman, S. M., and Newburger, P. E. (2014). Long intergenic non-coding RNA HOTAIRM1 regulates cell cycle progression during myeloid maturation in NB4 human promyelocytic leukemia cells. RNA Biol. 11 (6), 777–787. doi:10.4161/rna.28828
Zhang, Y., and Li, Y. (2020). Long non-coding RNA NORAD contributes to the proliferation, invasion and EMT progression of prostate cancer via the miR-30a-5p/RAB11A/WNT/β-catenin pathway. Cancer Cell Int. 20 (1), 571. doi:10.1186/s12935-020-01665-2
Zhao, H., Dong, H., Wang, P., and Zhu, H. (2021). Long non-coding RNA SNHG17 enhances the aggressiveness of C4-2 human prostate cancer cells in association with beta-catenin signaling. Oncol. Lett. 21 (6), 472. doi:10.3892/ol.2021.12733
Zheng, J., Zhao, S., He, X., Zheng, Z., Bai, W., Duan, Y., et al. (2016). The up-regulation of long non-coding RNA CCAT2 indicates a poor prognosis for prostate cancer and promotes metastasis by affecting epithelial-mesenchymal transition. Biochem. Biophys. Res. Commun. 480 (4), 508–514. doi:10.1016/j.bbrc.2016.08.120
Zheng, P., Chen, X., Xie, J., Chen, X., Lin, S., Ye, L., et al. (2020). Capn4 is induced by and required for Epstein-Barr virus latent membrane protein 1 promotion of nasopharyngeal carcinoma metastasis through ERK/AP-1 signaling. Cancer Sci. 111 (1), 72–83. doi:10.1111/cas.14227
Zhong, J., Liu, Y., Xu, Q., Yu, J., and Zhang, M. (2017). Inhibition of DIXDC1 by microRNA-1271 suppresses the proliferation and invasion of prostate cancer cells. Biochem. Biophys. Res. Commun. 484 (4), 794–800. doi:10.1016/j.bbrc.2017.01.169
Zhong, Z., Yu, J., Virshup, D. M., and Madan, B. (2020). Wnts and the hallmarks of cancer. Cancer Metastasis Rev. 39 (3), 625–645. doi:10.1007/s10555-020-09887-6
Zhou, B., Wang, J., Zheng, G., and Qiu, Z. (2016). Methylated urolithin A, the modified ellagitannin-derived metabolite, suppresses cell viability of DU145 human prostate cancer cells via targeting miR-21. Food Chem. Toxicol. 97, 375–384. doi:10.1016/j.fct.2016.10.005
Zhou, H., Zheng, X. D., Lin, C. M., Min, J., Hu, S., Hu, Y., et al. (2021). Advancement and properties of circular RNAs in prostate cancer: an emerging and compelling frontier for discovering. Int. J. Biol. Sci. 17 (2), 651–669. doi:10.7150/ijbs.52266
Zhou, K., Cheong, J. E., Tarakkad Krishnaji, S., Ghalali, A., Fu, H., Sui, L., et al. (2022a). Inhibition of Wnt signaling in colon cancer cells via an oral drug that facilitates TNIK degradation. Mol. Cancer Ther. 22, 25–36. doi:10.1158/1535-7163.MCT-21-0801
Zhou, K., Xu, J., Luo, H., Meng, X., Chen, M., and Zhu, D. (2022b). Wnt signaling pathway in cancer immunotherapy. Cancer Lett. 525, 84–96. doi:10.1016/j.canlet.2021.10.034
Zogg, H., Singh, R., and Ro, S. (2022). Current advances in RNA therapeutics for human diseases. Int. J. Mol. Sci. 23 (5), 2736. doi:10.3390/ijms23052736
Keywords: non-coding RNA, miRNA, lncRNA, circRNA, Wnt signaling pathway, prostate cancer
Citation: Bu T, Li L and Tian J (2023) Unlocking the role of non-coding RNAs in prostate cancer progression: exploring the interplay with the Wnt signaling pathway. Front. Pharmacol. 14:1269233. doi: 10.3389/fphar.2023.1269233
Received: 29 July 2023; Accepted: 12 September 2023;
Published: 27 September 2023.
Edited by:
Sujit Nair, Viridis Biopharma Pvt. Ltd., IndiaReviewed by:
Praful Saha, University of Mumbai, IndiaAniket Mali, Bharati Vidyapeeth Deemed University, India
Copyright © 2023 Bu, Li and Tian. This is an open-access article distributed under the terms of the Creative Commons Attribution License (CC BY). The use, distribution or reproduction in other forums is permitted, provided the original author(s) and the copyright owner(s) are credited and that the original publication in this journal is cited, in accordance with accepted academic practice. No use, distribution or reproduction is permitted which does not comply with these terms.
*Correspondence: Jiyu Tian, jiyu_tian@163.com
†These authors have contributed equally to this work