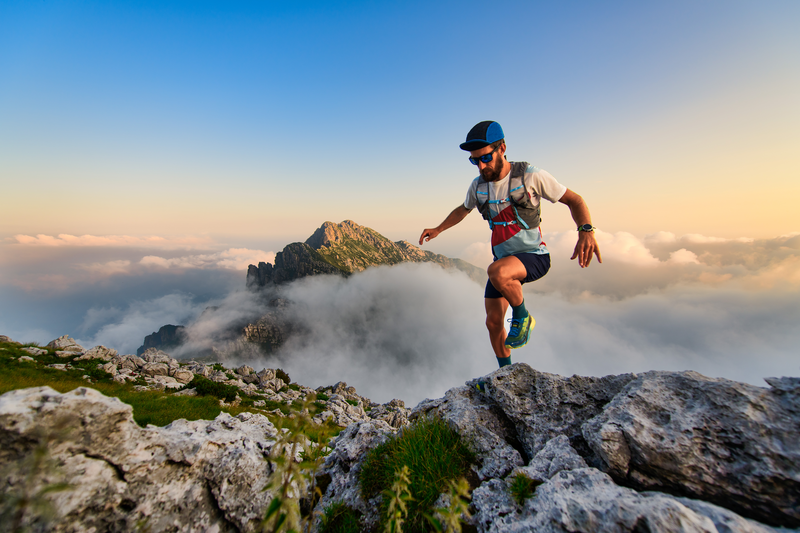
94% of researchers rate our articles as excellent or good
Learn more about the work of our research integrity team to safeguard the quality of each article we publish.
Find out more
REVIEW article
Front. Pharmacol. , 15 August 2023
Sec. Pharmacology of Anti-Cancer Drugs
Volume 14 - 2023 | https://doi.org/10.3389/fphar.2023.1265172
A correction has been applied to this article in:
Corrigendum: Advances in the roles of glycyrrhizic acid in cancer therapy
Since the first 70 years of reporting cancer chemotherapy, malignant tumors have been the second most common cause of death in children and adults. Currently, the commonly used anti-cancer methods include surgery, chemotherapy, radiotherapy, and immunotherapy. Although these treatment methods could alleviate cancer, they lead to different forms of side effects and have no particularly significant effect on prolonging the patients’ life span. Glycyrrhizic acid (GL), a native Chinese herbal extract, has a wide range of pharmacological effects, such as anti-cancer, anti-inflammatory, antioxidant, and immune regulation. In this review, the anti-cancer effects and mechanisms of GL are summarized in various cancers. The inhibition of GL on chemotherapy-induced side effects, including hepatotoxicity, nephrotoxicity, genotoxicity, neurotoxicity and pulmonary toxicity, is highlighted. Therefore, GL may be a promising and ideal drug for cancer therapy.
Cancer has afflicted the multicellular organism for more than 200 million years, and the ancestors of modern humans were plagued by cancer more than a million years ago (Hausman, 2019). As the second most natural cause of death in children and adults (Gilbertson, 2011), each type of cancer has its particular age and form of occurrence, occurring in different proportions and in distinct genders. Until now, people have expended gigantic efforts to understand the origin of cancer cells, the formation of cancer tissue, and the mechanisms of their spread and recurrence, but this disease remains a mystery. A growing body of research suggests that the change in the incidence rate of cancer may be caused by more subtle alterations in the cell hierarchy (Lim et al., 2009; Molyneux et al., 2010). The formation of cancer probably involves three pathways: 1) spreading from the origin of growth to adjacent cells or tissues; 2) spreading from lymph nodes to local lymph nodes; 3) spreading to various tissues and organs throughout the body through blood circulation (Wong and Hynes, 2006).
Although the medical field has made some progress in cancer treatment after more than half a century of research, cancer is still deemed as a human tragedy. There are many treatment methods for cancer based on its type and progression stage. The traditional treatment methods for cancer include surgery, chemotherapy and radiotherapy. With the development of the times and technology, new cancer treatment methods, such as target-specific therapies and immunotherapy, are increasingly applied in clinical practice (Liu et al., 2021). However, these treatment methods all have certain limitations. Surgical treatment is highly traumatic and it cannot completely eliminate cancer cells. The effect of radiotherapy will be not ideal when tumors are not sensitive to radiation and tumors are of systemic metastasis. Among them, chemotherapy for malignant tumors has the strongest cancer killing effect (Safarzadeh et al., 2014; Jain et al., 2016). However, its toxic effects on healthy cells, multiple drug resistance (MDR) after permanent treatment and low bioavailability have enormously limited its clinical application (Rastogi et al., 2014). After these treatments, the survival time of cancer patients has been prolonged, but a great proportion of patients still experience cancer recurrence and cannot achieve permanently survival (Yin et al., 2021). Therefore, seeking novel methods for cancer treatment remains a crucial aspect of medical research.
Glycyrrhizic acid (GL), a triterpenoid saponin, is the main active component and sweet component of the extract from the root of Glycyrrhiza uralensis Fisch. GL is structurally composed of two molecules of glucuronic acid and one molecule of glycyrrhetinic acid (GA) (Figure 1). GL is metabolized to GA under the action of gut bacteria. In addition, GL is metabolized in the intestine or be transformed via enzymolysis to GA-3-O-mono-β-d-glucuronide (GAMG), a distal glucuronic acid hydrolysate of GL with higher bioavailability and stronger physiological functions (Zuo et al., 2023).
Accumulating studies have demonstrated that GL shows multiple pharmacological activities, such as anti-inflammatory (Liu et al., 2018; Maione et al., 2019), anti-tumor (Zhang et al., 2015; Chang et al., 2019; Tsai et al., 2020), anti-viral (Cinatl et al., 2003; Fu et al., 2016), antioxidant (Xu et al., 2018), immunoregulation (Bernela et al., 2016; Han et al., 2017) and liver protection (Huo et al., 2020) etc. GL mainly inhibits the expression of nuclear factor kappa-B (NF-κB) pathway, thereupon then restraining the encoding of important genes such as inflammatory cytokines, anti-apoptotic factors and cyclooxygenase-2 (COX2) (Li S. et al., 2014), achieving anti-inflammatory and anti-tumor effects. Thus, GL is commonly used in treating various cancers in clinical practice (Stecanella et al., 2021; Zuo et al., 2022), as well as in alleviating liver, lung, and kidney damage caused by cancer chemotherapy (Orazizadeh et al., 2014; Qu et al., 2017). Meanwhile, it also has a protective effect on brain tissue in cases of global cerebral ischemia, brain injury caused by cerebral hemorrhage, and focal ischemia (Xiong et al., 2016).
In addition, GL could facilitate the entry of other drugs into cells through interaction with cell membranes due to its strong hydrophilicity and lipophilicity (Selyutina and Polyakov, 2019). Studies have shown that even at high concentrations, GL still has pharmacological tolerance in rats and humans, and long-term administration has no significant toxic or side effects (van Rossum et al., 1999; Bi et al., 2023; Li et al., 2023). Therefore, it is often used as a blending agent for other drugs to diminish toxicity and enhance drug efficacy (Radwant and Aboul-Enein, 2002; Chen et al., 2009).
GL inhibits the occurrence and development of cancer by inducing apoptosis pathways in cancer cells. It shows a series of anti-cancer related pharmacological activities, such as broad-spectrum anti-cancer ability, anti-chemotherapy and radiotherapy induced tissue toxicity, absorption enhancement and anti-multiple drug resistance (MDR) mechanism (Wakamatsu et al., 2007; Ajazuddin et al., 2014). Multiple studies have shown that GL acts as an inhibitor of cell signal transduction molecules, angiogenesis inhibitors, tumor related cytokine inhibitors, efficiently and low-toxicity inhibiting the migration and invasion of several types of cancer cells (Kohlschutter et al., 2008; Smolarczyk et al., 2012). The following sections will specifically highlight the anti-cancer mechanism of GL and its inhibition on the side effects of cancer treatment.
GL has been reported to have inhibitory effects on various cancers, such as leukemia (Chueh et al., 2012; Bruserud et al., 2015; Shustik et al., 2017), malignant glioma (Li S. et al., 2014), colon cancer (Yang et al., 2015; Jiang et al., 2016), lung cancer (Khan et al., 2013; Huang et al., 2014) etc. The application of GL in various cancers was summarized in Table 1.
Colon cancer, a disease with high incidence, easy migration and difficult cure, constantly develops drug resistance during chemotherapy, increasing the difficulty of treatment (Liang et al., 2020). Khan et al. (2018) found that GL administration inhibited the 1,2-dimethylhydrazine-induced colon tumorigenesis in Wistar rats through reducing the expression of Ki-67, proliferating cell nuclear antigen (PCNA), NF-kB, cyclooxygenase-2(COX-2), inducible nitric oxide synthase (iNOS), and vascular endothelial growth factor (VEGF) and enhancing the expression of p53, connexin-43, B-cell lymphoma-2 (Bcl-2), survivin, and caspase-3, suggesting the chemopreventive potential of GL against colon cancer. Simultaneously, upregulation of the expression of tumor suppressor protein p53 activates casepase-3, thereby inducing tumor cell apoptosis (Yi et al., 2016). In addition, GL reduced drug resistance by combining with first-line anti-cancer drugs. Some theories suggest that GL may enhance the absorption of paclitaxel-loaded GL micelles in the jejunum and colon by inhibiting p-glycoprotein (Yang et al., 2015). Interestingly, Afkhami-Poostchi et al. (2020) applied bacteria-directed enzyme prodrug therapy to convert GL to glycyrrhetinic acid and found that combined treatment of bacteria overexpressing β-glucuronidase and GL more greatly inhibited tumor growth when compared with sole GL treatment.
Gastric cancer is the fifth leading cause of cancer-related deaths worldwide, with approximately half of cases occurring in developing countries (Ang and Fock, 2014; Karimi et al., 2014; den Hoed and Kuipers, 2016). GL could inhibit the growth of stomach cancer KATO III cells and induce the fragmentation of DNA to oligonucleosomal-sized fragments, suggesting that GL induced apoptosis (Hibasami et al., 2005). Wang et al. (2020) reported that GL induced apoptosis MGC-803 cells of inhibiting phosphorylation of the PI3K/AKT pathway, downregulating the expression of Bcl-1, survivin and p65, and upregulating the expression of Bax and the cleavage of poly (ADP-ribose) polymerase (PARP). Cell cycle arrest could cause inhibition of cell proliferation. A study has shown that GL inhibited the proliferation of MGC-803 cells, a kind of gastric cancer cells, by inducing G1/s phase arrest because GL treatment downregulated the levels of several G1 phase-related proteins (cyclin D1, D2, D3, E1, and E2) (Wang et al., 2020). In a recent study, Khan et al. (2023) reported that GL treatment inhibited helicobacter pylori infection in AGS cells (gastric cancer cells) via inhibiting high mobility group box1 (HMGB1) and inducing autolysosomal degradation function.
Leukemia is one of the causes of cancer-related deaths in humans, and is an invasive malignant tumor, that is, produced by the rapid growth of abnormal white blood cells (Lee et al., 2007). GL treatment increased the levels of reactive oxygen species (ROS) and the activity of caspase-3 and decreased the mitochondrial membrane potential (ΔΨm) in WEHI-3 cells, as well as led to G0/G1 phase arrest, DNA damage and breakage in a dose-dependent manner (Chueh et al., 2012). GL could inhibit TF-1 cells proliferation in vitro and reduce the volume of TF-1 tumor via inhibiting the activation of AKT/mTOR/signal transducer and activator of transcription 3 (STAT3) signaling pathway, attenuating the expression of cyclin D1 and survivin and increasing the cleavage of caspase-3 and PARP (He et al., 2015). In addition, GL reversed multidrug resistance in human leukemia cell line CEM/ADR 5000 (Zhou and Wink, 2018). Based on these anti-leukemia capabilities, researches on the rationality of GL combination therapy are gradually being carried out. A study has shown that co-treatment with GL and imatinib (a first-line drug) not only enhanced the levels of apoptosis greatly in K562 cells (chronic myeloid leukemia), but also decreased the tumor burden significantly in EL-4 lymphoma-bearing C57BL/6J mice (Hostetler et al., 2017).
Gliomas are the most common brain tumor of the central nervous system, approximately accounting for 35%–50% of adult intracranial tumors. It is worth noting that malignant gliomas account for about 60% of gliomas (Penas-Prado et al., 2012). Research has shown that GL inhibited the proliferation of human glioblastoma U251 cells in a time- and dose-dependent manners via down-regulating the expression of p65 (Li S. et al., 2014). NF-κB is a key transcription factor involved in the pathological processes of various human diseases, controlling multiple genes involved in the development of diffuse gliomas and promoting the growth of high-grade gliomas (Kanzawa et al., 2003; Wang et al., 2004). GL inhibited NF-κB pathway via downregulating the expression of p65 protein, encoding of important genes such as anti-inflammatory cytokines, COX-2 and iNOS (Li S. et al., 2014). Dipotassium glycyrrhizinate, a dipotassium salt of GL, also showed an anti-proliferative effect via inducing apoptosis and an anti-migratory effect in U251 and U138MG cells through upregulating the levels of miR-4443 and miR-3620, which are responsible for the post-transcriptional inhibition of NF-κB (Bonafe et al., 2022a).
Lung cancer is the most common malignant tumor worldwide and the main cause of death for cancer patients. Huang et al. (2014) found that GL inhibited the growth of A549 cells by induction of apoptosis, but had no effects on NCI-H23 cells, another lung adenocarcinoma cell line. They further reported that GL suppressed the expression and activity of thromboxane synthase (TxAS) in A549 cells and clarified the anti-tumor effect of GL in lung adenocarcinoma cells is dependent on inhibition of TxAS (Huang et al., 2014). Importantly, treatment with 50 mg/kg GL for 8 weeks significantly inhibited the growth of xenograft of lung adenocarcinoma cells in vivo (Huang et al., 2014). Deng et al. (2017) reported that the effect of GL treatment alone is comparable to combination of GL and cisplatin in a mouse lung adenocarcinoma model, suggesting the clinical application of GL may decrease the dosage of cisplatin, thereby reducing the side effects of chemotherapy. In HCC827 cells, a non-small cell lung cancer cell line, GL inhibited the migration and invasion of cancer cells via targeting JAK/STAT/HMGB1 signaling (Wu et al., 2018).
Liver cancer is a common malignant tumor with extremely high mortality rate, ranking third among global cancer mortality rates (Sung et al., 2021). Through the research on the prevalence of liver cancer, it is found that the incidence rate of liver cancer in most countries around the world is still rising year by year. Hepatocellular carcinoma (HCC) has poor differentiation ability and proliferate indefinitely. The dedifferentiation of HCC contributes to malignant progression, characterized by significant morphological changes and loss of liver function (Sun et al., 2011). A recent study has shown that GL administration led to a decrease in stem cell pluripotency and induced the differentiation in HCC in vitro and in vivo by targeting c-Jun N-terminal kinase 1 (JNK1) (Cai et al., 2019); Importantly, blockage of JNK1 mitigate the degree of malignancy of HCC (Cai et al., 2019). In addition, GL combination effectively enhanced the anti-tumor effects of sorafenib, an inhibitor of multi kinases, in HCC treatment (Cai et al., 2019). Tsai et al. (2020) reported that GL not only inhibited dramatically the tumor cell growth and invasion, as well as the phosphorylation of extracellular-signal-regulated kinase (ERK), Akt (Ser473), epidermal growth factor receptor (EGFR) and the anti-apoptotic and metastatic proteins, but also triggered markedly caspase-8/9-mediated apoptosis in HCC in vitro and in vivo. The combination of GL could reverse the resistance of cisplatin in hepatocellular carcinoma cells via inhibiting of MDR-associated proteins (Wakamatsu et al., 2007). Covalent conjugation of GL with polyethyleneimine increased significantly the gene transfection efficiency and superior selectivity for HepG2 cells, suggesting the potential clinical application in vivo (Cao et al., 2019). Several studies reported that co-delivery of GL enhanced the therapeutic efficacy of doxorubicin for hepatocellular carcinoma in vitro and in vivo (Wang et al., 2019; Yang et al., 2019), suggesting the significant clinical application value.
Melanoma is a highly malignant tumor with pigmented cell, melanocyte, accounting for less than 5% of all skin cancers but 80% of skin cancer-related deaths (Bertolotto, 2013). The incidence of malignant melanoma has been steadily increasing globally over the past few decades (Godar, 2011). The immunosuppressive tumor microenvironment (TME) has been identified as a major barrier to evoke an anti-tumor response in melanoma. Moreover, immunosuppressive TME is directly connected with the high activation of T-regulatory cells (Tregs) and myeloid-derived suppressor cells (MDSCs) function. GL downregulated the expression of the anti-apoptotic factor Bcl 2, upregulated the expression of the proinflammatory factor Bax and enhanced the activity of caspase-9 and caspase-3, indicating that GL inhibited the proliferation of melanoma cells by inducing apoptosis (Juin et al., 2020). Meanwhile, GL incubation effectively reduced the expression of Treg specific markers (Forkhead Box P3, glucocorticoid-induced TNFR-related protein and cytotoxic T lymphocyte antigen 4), phospho-STAT3, COX-2 and prostaglandin E2 in melanoma cells, finally limiting the progression of melanoma (Juin et al., 2020). Furthermore, studies showed that GL inhibited the metastasis of melanoma cells by regulating T helper type 2 (Th2) cell resistance, interfering with further the dissemination of melanoma (Kobayashi et al., 2002; Su et al., 2017). Dipotassium of GL inhibited the metastases of melanoma Cells into brain (Bonafe et al., 2022b).
To summarize, GL exerts the anti-tumor activity via inhibiting cell proliferation, inducing apoptosis and resulting in cell cycle arrest in various tumors (Figure 2).
FIGURE 2. Anti-cancer mechanism diagram of GL. GL mainly plays an important role in various cancers by inhibiting the proliferation of cancer cells, inducing cell apoptosis through its anti-inflammatory, antioxidant, and immune regulatory effects, and inducing cell cycle arrest.
Most anti-cancer drugs could cause inevitable damage to normal cells during treatment. Therefore, the protection of normal tissues and organs becomes the primary goal of improving the life quality of patients. An increasing number of studies have shown that GL has a strong therapeutic effect on liver, lung and kidney injury caused by chemotherapy or radiotherapy. In addition to the above anti-cancer capabilities, GL could significantly reduce side effects occurring during chemotherapy, especially hepatotoxicity (Li J. Y. et al., 2014). In addition, GL also has better therapeutic effects on nephrotoxicity (Ju et al., 2017), genotoxicity (Arjumand and Sultana, 2011), neurotoxicity (Klein et al., 2023), pulmonary toxicity (Zhu et al., 2021) and other events occurring during cancer treatment. In the following section, we will review the inhibition and potential mechanisms of GL on these side effects (Figure 3).
FIGURE 3. Mechanism diagram of GL reducing toxic side effects. GL could inhibit a series of side effects such as nephrotoxicity, hepatotoxicity, genotoxicity, neurotoxicity and lung toxicity in cancer treatment by inhibiting the release of inflammatory factors, inhibiting apoptosis and clearing ROS. Fundamentally speaking, GL mainly reduces a series of tissue damage in the process of tumor treatment through its anti-inflammatory and antioxidant properties, thus effectively reducing the side effects of tumor treatment.
Liver is the most important organ for the metabolism of chemical drugs. After metabolized in the liver, a large number of metabolites accumulates in the liver and lead to liver damage and hepatotoxicity. The action mechanism is by inducing the liver lipid peroxidation, resulting in imbalance in redox status, at the same time high levels of ROS-mediated oxidative stress reaction cause liver tissue cell apoptosis and inflammation damage, eventually lead to hepatotoxicity (Waseem et al., 2015). GL administration for 3 h before high-dose methotrexate administration significantly increased hepatic enzyme levels (Mano et al., 2023), suggesting that optimal administration of GL could avoid pharmacokinetic interactions with methotrexate and exerts a hepatoprotective effects. Hepatotoxicity is a common toxic side effect of many chemotherapeutic drugs. Kishimoto et al. (2021) reported that 39 of 118 patients with acute leukemia (33%) developed grade 3–4 hepatotoxicity after combined administration of intravenous monoammonium glycyrrhizinate and methotrexate. As one of the hepatoprotective compounds, GL is commonly used in the treatment of acute and chronic liver injury, viral hepatitis, hepatic steatosis, liver fibrosis, liver cancer and other diseases. Moreover, GL has various pharmacological effects, such as anti-inflammatory, neuroprotection, antiviral, anti-tumor, antioxidant, and hepatoprotective activities (Li J. Y. et al., 2014). It could reduce the content of ROS by reducing lipid peroxidation (Kiso et al., 1984) and increasing the activity of superoxide dismutase (SOD), glutathione peroxidase (GPx) and catalase (Kao et al., 2009). Furthermore, GL inhibits tripterygium-glycoside-tablet-induced acute liver injury by regulating pyruvate kinase M2 and reducing oxidative, inflammation stress and apoptosis (Wang et al., 2022). In addition, GL could significantly reduce the steatosis and necrosis of hepatocytes, inhibit interstitial inflammation and liver fibrosis, and promote cell regeneration (Li J. Y. et al., 2014).
Chemotherapy is one of the main cancer treatments. In cancer treatment, traditional chemotherapeutic drugs are the first-line drugs for the treatment of several malignancies, but they also cause kidney toxicity. In addition to traditional cytotoxic drugs, molecular targeted drugs could also affect kidney function and cause nephrotoxicity, which limits the efficacy of treatment and affects the life quality and overall survival of patients (Santos et al., 2020). Studies have shown that the nephrotoxicity induced by some anti-cancer drugs may be closely related to the oxidative stress and inflammatory responses. GL has antioxidant and anti-inflammatory properties, which could effectively inhibit pro-inflammatory substances (NF-κB, TNF-α, IL-1β, IL-6, and HMGB 1), increase levels of nuclear factor erythroid 2-related factor 2 (Nrf 2) and heme oxygenase-1 (HO-1), and restore the activity of antioxidant enzyme and GSH/GSSG ratio (Wu et al., 2015), reduce lipid peroxidation (Arjumand and Sultana, 2011), suggesting that GL effectively inhibits renal inflammation and oxidative stress, and relieve chemical kidney injury. In gentamicin-induced renal injury, GL may inhibit gentamicin-induced ROS generation or scavenge ROS before it reaches the cellular target tissue, while GL also restores the expression of aquaporin 2, thus improving renal defects in rats with gentamicin-induced acute renal failure (Sohn et al., 2003). Moreover, GL could also ameliorate cisplatin-induced renal injury and inhibit nephrotoxicity through reducing ROS-mediated p53 activation and promoting p21 expression in HK-2 cells (Ju et al., 2017). The renal protective effects of GL may be related to upregulation of Nrf2 and downregulation of NF-κB in the kidney of BALB/c mice (Wu et al., 2015).
Most of the anti-tumor drugs have genotoxic effects that contribute to growth inhibition. Exposure to genotoxins causes an increased risk of carcinogenic and teratogenicity (Arjumand and Sultana, 2011). These genotoxic and oncogenic potential may lead to the formation of secondary cancers (Said Salem et al., 2017). Some studies have indicated that many drug-induced genotoxicity and chromosomal instability are closely related to the parameters of oxidative stress. ROS act directly on intracellular components, including lipids, proteins and DNA, and disrupt their structures. The chemoprotective agents could exert their antigenotoxic effects through one or variety of mechanisms, such as inhibiting the formation of reactive carcinogenic metabolites, inducing enzymes that detoxify carcinogens, scavenging ROS, inhibiting cell proliferation and regulating cell apoptosis (Arjumand and Sultana, 2011). GL has anti-inflammatory, anti-oxidant, anti-cancer, and immunomodulatory effects (Akamatsu et al., 1991; Kelloff et al., 1994; Wakamatsu et al., 2007) and is considered as a possible chemopreventive agent. Increasing genotoxic researches have demonstrated that GL is not only nonteratogenic and nonmutagenic, but also have anti-genotoxic property (Isbrucker and Burdock, 2006). Arjumand and Sultana (2011) reported that GL administration significantly reversed the genotoxicity induced by cisplatin, including a decrease in DNA fragmentation and increases in the content of glutathione and activities of the anti-oxidant enzymes (catalase, glutathione peroxidase, glutathione reductase, quinone reductase and glutathione-S-transferase).
Cancer therapies could cause a wide range of neurologic adverse effects and may cause a significant increase in morbidity and mortality of cancer patients (Dietrich, 2020). Some chemotherapeutic drugs, such as taxanes (paclitaxel and docetaxel), platinum compounds (cisplatin, oxaliplatin and carboplatin), and vinca alkaloids (vinblastine and vincristine), have strong neurotoxicity and could easily induce a peripheral neuropathy (CIPN) (Klein and Lehmann, 2021). CIPN is associated with a length-dependent axonal sensory neuropathy in taxol-induced neuropathy (Staff et al., 2017). Paclitaxel easy access to and accumulate in the dorsal root ganglia, which will cause numbness and pain in hands and feet (Cavaletti et al., 2000). Paclitaxel induces disruption of axonal transport through microtubule stabilization, changes in mitochondrial morphology and function, and inflammatory responses, leading to axonal symmetry damage and loss of nerve fibers, and subsequent neurotoxicity (Niznansky et al., 2022). Increasing studies have suggested that GL could provide neuroprotection in nerve system due to its strong anti-inflammatory, anti-apoptosis, antioxidant and autophagy regulation properties (Kim et al., 2012; Yang et al., 2018; Gendy et al., 2023; Shan et al., 2023). A recent study has shown that GL alleviated paclitaxel-induced neurotoxicity in vitro and in vivo by inhibiting the neuronal uptake mediated by organic anion transport peptides (OATPs), which are the main neuronal transporters of paclitaxel (Klein et al., 2023).
Some chemotherapeutic agents, such as bleomycin, could cause severe pulmonary fibrosis and produce pulmonary toxicity (van der Schoot et al., 2016). GL could alleviate benzo(a)pyrene exposure-induced lung injury in rats via ameliorating the detoxification and antioxidant function of lung (Qamar et al., 2012). HMGB1 is a cytokine-like protein found in the nucleus of all cells and has multiple functions in inflammation, infection, tissue damage, cell apoptosis, and immune response (Qu et al., 2019). Furthermore, HMGB1 could not only act as a proinflammatory factor to directly involve in tissue damage (Qin et al., 2006), but also induce lung fibrosis through NF-κB-mediated release of transforming growth factor beta1 (TGF-β1) (Wang et al., 2017). As an inhibitor of HMGB 1, GL treatment reduced the inflammation and fibrosis by inhibiting the mitogen-activated protein kinase (MAPK) inflammatory signaling and Smad3 fibrotic signaling pathway (Zhu et al., 2021), thereby alleviating the lung toxicity induced by bleomycin, which is clinically used to treated various tumors (Gederaas et al., 2023).
With the development of modern science and technology, the discovery of plant pharmacological components was promoted. GL, as the main active ingredient of the licorice extract, has a wide range of pharmacological activities. Notably, increasing literature have demonstrated that GL shows inhibitory effects on various cancers by inhibiting cell proliferation, inducing apoptosis and resulting in cell cycle arrest through multitudinous mechanisms. What’s more, GL could reduce effectively the side effects of cancer treatment via inhibition of chemotherapy-induced renal toxicity, liver toxicity, genotoxicity, neurotoxicity and pulmonary toxicity. Given the important roles of GL in cancer treatment, the application of GL alone in the cancer chemotherapy or combined use with other anti-tumor drugs will have very bright application prospects.
Besides above side effects induced in the chemotherapy of cancer, ototoxicity is another common side effect which limits the clinical use of chemotherapeutics (Basirat et al., 2023). Many chemotherapeutics could affect the inner ear or auditory nerve, leading to hearing loss. For example, Cisplatin is widely used as a chemotherapeutic drug with a high rate of ototoxicity (an average incidence of more than 60%) (Arwanda et al., 2023). Inflammation and oxidative stress may be closely related to the ototoxicity of Cisplatin (Ramkumar et al., 2021). However, the role of GL in combating ototoxicity induced by chemotherapy is largely unclear. Therefore, it is expected that GL will help solving the hearing loss caused by ototoxic drugs in the future. What’s more, the mechanisms for GL inhibiting various side effects are not presently clarified accurately. Thus, more studies should be performed to elucidate the protective mechanisms.
GL shows stronger actions in cancer treatment, but some factors may limit its clinical application. The human body has multiple reactions to GL and there are significant individual differences. However, the reasons for the differences are not yet clear, and the proportion of sensitive populations has not been determined. On the basis of different administration methods and drug concentrations, electrolyte imbalance, edema, elevated blood pressure and false aldosterone symptoms may occur (Gomez-Sanchez and Gomez-Sanchez, 1992; Johns, 2009; Celik et al., 2012). Studies have shown that the risk of toxicity after oral administration is much lower than intravenous or intraperitoneal administration, but we should still be particularly careful to avoid high doses or long-term ingestion of GL (Wang and Nixon, 2001). What’s more, people with diseases such as heart and kidney problems, hypertension may be more susceptible to the adverse effects of GL (Ruszymah et al., 1995). In addition, the content of GL in different varieties of liquorice varies greatly, and the yield depends on the source of plants, which also limits greatly its development and application. Han et al. (2021) recommended that regulating biosynthesis pathway of GL through environmental stimuli would provide a new idea for obtaining high-quality GL. Although side effects of GL have been reported, their incidence can be avoided with reasonable medication. The most important thing is that its potential for treating various diseases is worthy of recognition.
YZ: Writing–original draft. ZS: Writing–original draft. JX: Resources, Software, Writing–original draft. YL: Resources, Software, Writing–original draft. JH: Resources, Software, Writing–original draft. JJ: Writing–review and editing. XZ: Conceptualization, Writing–review and editing. LL: Conceptualization, Writing–review and editing.
The author(s) declare financial support was received for the research, authorship, and/or publication of this article. This study is financially supported by National College Student Innovation Training Program of China (202310354023) and Jiaxing University Student Research and Training Program (8517221143).
The authors declare that the research was conducted in the absence of any commercial or financial relationships that could be construed as a potential conflict of interest.
All claims expressed in this article are solely those of the authors and do not necessarily represent those of their affiliated organizations, or those of the publisher, the editors and the reviewers. Any product that may be evaluated in this article, or claim that may be made by its manufacturer, is not guaranteed or endorsed by the publisher.
Afkhami-Poostchi, A., Mashreghi, M., Iranshahi, M., and Matin, M. M. (2020). Use of a genetically engineered E. coli overexpressing ÿ-glucuronidase accompanied by glycyrrhizic acid, a natural and anti-inflammatory agent, for directed treatment of colon carcinoma in a mouse model. Int. J. Pharm. 579, 119159. doi:10.1016/j.ijpharm.2020.119159
Ajazuddin, , , Alexander, A., Qureshi, A., Kumari, L., Vaishnav, P., Sharma, M., et al. (2014). Role of herbal bioactives as a potential bioavailability enhancer for Active Pharmaceutical Ingredients. Fitoterapia 97, 1–14. doi:10.1016/j.fitote.2014.05.005
Akamatsu, H., Komura, J., Asada, Y., and Niwa, Y. (1991). Mechanism of anti-inflammatory action of glycyrrhizin: effect on neutrophil functions including reactive oxygen species generation. Planta Med. 57 (2), 119–121. doi:10.1055/s-2006-960045
Ang, T. L., and Fock, K. M. (2014). Clinical epidemiology of gastric cancer. Singap. Med. J. 55 (12), 621–628. doi:10.11622/smedj.2014174
Arjumand, W., and Sultana, S. (2011). Glycyrrhizic acid: a phytochemical with a protective role against cisplatin-induced genotoxicity and nephrotoxicity. Life Sci. 89 (13-14), 422–429. doi:10.1016/j.lfs.2011.06.016
Arwanda, M. D., Haryuna, T. S., Adriztina, I., and Khalid, K. (2023). Curcumin prevents ototoxicity induced by cisplatin as evaluated with OAE. Iran. J. Otorhinolaryngol. 35 (129), 189–197. doi:10.22038/IJORL.2023.71786.3452
Basirat, U., Bin Tariq, U., Moeen, N., Jawhar, Z. H., Shoja, S. J., Kareem, A. K., et al. (2023). A systematic review of the chemo/radioprotective effects of melatonin against ototoxic adverse effects induced by chemotherapy and radiotherapy. Curr. Pharm. Des. 29 (15), 1218–1229. doi:10.2174/1381612829666230503145707
Bernela, M., Ahuja, M., and Thakur, R. (2016). Enhancement of anti-inflammatory activity of glycyrrhizic acid by encapsulation in chitosan-katira gum nanoparticles. Eur. J. Pharm. Biopharm. 105, 141–147. doi:10.1016/j.ejpb.2016.06.003
Bertolotto, C. (2013). Melanoma: from melanocyte to genetic alterations and clinical options. Sci. (Cairo) 2013, 635203. doi:10.1155/2013/635203
Bi, X., Yang, L., Lin, Y., Deng, W., Jiang, T., Zhang, L., et al. (2023). Efficacy and safety of glycyrrhizic acid in treatment of autoimmune hepatitis. Am. J. Chin. Med. 51 (2), 391–405. doi:10.1142/S0192415X23500209
Bonafe, G. A., Dos Santos, J. S., Fernandes, A., Ziegler, J. V., Marson, F. A. L., Rocha, T., et al. (2022a). Anti-migratory effect of dipotassium glycyrrhizinate on glioblastoma cell lines: microarray data for the identification of key MicroRNA signatures. Front. Oncol. 12, 819599. doi:10.3389/fonc.2022.819599
Bonafe, G. A., Dos Santos, J. S., Ziegler, J. V., Marson, F. A. L., Rocha, T., and Ortega, M. M. (2022b). Dipotassium glycyrrhizinate on melanoma cell line: inhibition of cerebral metastases formation by targeting NF-kB genes-mediating MicroRNA-4443 and MicroRNA-3620-dipotassium glycyrrhizinate effect on melanoma. Int. J. Mol. Sci. 23 (13), 7251. doi:10.3390/ijms23137251
Bruserud, O., Nepstad, I., Hauge, M., Hatfield, K. J., and Reikvam, H. (2015). STAT3 as a possible therapeutic target in human malignancies: lessons from acute myeloid leukemia. Expert Rev. Hematol. 8 (1), 29–41. doi:10.1586/17474086.2015.971005
Cai, S., Bi, Z., Bai, Y., Zhang, H., Zhai, D., Xiao, C., et al. (2019). Glycyrrhizic acid-induced differentiation repressed stemness in hepatocellular carcinoma by targeting c-jun N-terminal kinase 1. Front. Oncol. 9, 1431. doi:10.3389/fonc.2019.01431
Cao, M., Gao, Y., Zhan, M., Qiu, N., Piao, Y., Zhou, Z., et al. (2019). Glycyrrhizin acid and glycyrrhetinic acid modified polyethyleneimine for targeted DNA delivery to hepatocellular carcinoma. Int. J. Mol. Sci. 20 (20), 5074. doi:10.3390/ijms20205074
Cavaletti, G., Cavalletti, E., Oggioni, N., Sottani, C., Minoia, C., D'Incalci, M., et al. (2000). Distribution of paclitaxel within the nervous system of the rat after repeated intravenous administration. Neurotoxicology 21 (3), 389–393.
Celik, M. M., Karakus, A., Zeren, C., Demir, M., Bayarogullari, H., Duru, M., et al. (2012). Licorice induced hypokalemia, edema, and thrombocytopenia. Hum. Exp. Toxicol. 31 (12), 1295–1298. doi:10.1177/0960327112446843
Chang, H. Y., Chen, S. Y., Wu, C. H., Lu, C. C., and Yen, G. C. (2019). Glycyrrhizin attenuates the process of epithelial-to-mesenchymal transition by modulating HMGB1 initiated novel signaling pathway in prostate cancer cells. J. Agric. Food Chem. 67 (12), 3323–3332. doi:10.1021/acs.jafc.9b00251
Chen, L., Yang, J., Davey, A. K., Chen, Y. X., Wang, J. P., and Liu, X. Q. (2009). Effects of diammonium glycyrrhizinate on the pharmacokinetics of aconitine in rats and the potential mechanism. Xenobiotica 39 (12), 955–963. doi:10.3109/00498250903271997
Chueh, F. S., Hsiao, Y. T., Chang, S. J., Wu, P. P., Yang, J. S., Lin, J. J., et al. (2012). Glycyrrhizic acid induces apoptosis in WEHI-3 mouse leukemia cells through the caspase- and mitochondria-dependent pathways. Oncol. Rep. 28 (6), 2069–2076. doi:10.3892/or.2012.2029
Cinatl, J., Morgenstern, B., Bauer, G., Chandra, P., Rabenau, H., and Doerr, H. W. (2003). Glycyrrhizin, an active component of liquorice roots, and replication of SARS-associated coronavirus. Lancet 361 (9374), 2045–2046. doi:10.1016/s0140-6736(03)13615-x
den Hoed, C. M., and Kuipers, E. J. (2016). Gastric cancer: how can we reduce the incidence of this disease? Curr. Gastroenterol. Rep. 18 (7), 34. doi:10.1007/s11894-016-0506-0
Deng, Q. P., Wang, M. J., Zeng, X., Chen, G. G., and Huang, R. Y. (2017). Effects of glycyrrhizin in a mouse model of lung adenocarcinoma. Cell Physiol. Biochem. 41 (4), 1383–1392. doi:10.1159/000467897
Dietrich, J. (2020). Neurotoxicity of cancer therapies. Contin. (Minneap Minn) 26 (6), 1646–1672. doi:10.1212/CON.0000000000000943
Fu, X., Wang, Z., Li, L., Dong, S., Li, Z., Jiang, Z., et al. (2016). Novel chemical ligands to ebola virus and marburg virus nucleoproteins identified by combining affinity mass spectrometry and metabolomics approaches. Sci. Rep. 6, 29680. doi:10.1038/srep29680
Gederaas, O. A., Sharma, A., Mbarak, S., Sporsheim, B., Hogset, A., Bogoeva, V., et al. (2023). Proteomic analysis reveals mechanisms underlying increased efficacy of bleomycin by photochemical internalization in bladder cancer cells. Mol. Omics 2023. doi:10.1039/d2mo00337f
Gendy, A. M., El-Sadek, H. M., Amin, M. M., Ahmed, K. A., El-Sayed, M. K., El-Haddad, A. E., et al. (2023). Glycyrrhizin prevents 3-nitropropionic acid-induced neurotoxicity by downregulating HMGB1/TLR4/NF-κB p65 signaling, and attenuating oxidative stress, inflammation, and apoptosis in rats. Life Sci. 314, 121317. doi:10.1016/j.lfs.2022.121317
Gilbertson, R. J. (2011). Mapping cancer origins. Cell 145 (1), 25–29. doi:10.1016/j.cell.2011.03.019
Godar, D. E. (2011). Worldwide increasing incidences of cutaneous malignant melanoma. J. Skin. Cancer 2011, 858425. doi:10.1155/2011/858425
Gomez-Sanchez, E. P., and Gomez-Sanchez, C. E. (1992). Central hypertensinogenic effects of glycyrrhizic acid and carbenoxolone. Am. J. Physiol. 263 (6), E1125–E1130. doi:10.1152/ajpendo.2006.263.6.E1125
Han, S., Sun, L., He, F., and Che, H. (2017). Anti-allergic activity of glycyrrhizic acid on IgE-mediated allergic reaction by regulation of allergy-related immune cells. Sci. Rep. 7 (1), 7222. doi:10.1038/s41598-017-07833-1
Han, Y. X., Jia, Q. J., Yang, D. F., Chai, W. G., Zhang, X. M., He, Q. L., et al. (2021). Current advances in environmental stimuli regulating the glycyrrhizic acid biosynthesis pathway. Fitoterapia 151, 104860. doi:10.1016/j.fitote.2021.104860
Hausman, D. M. (2019). What is cancer? Perspect. Biol. Med. 62 (4), 778–784. doi:10.1353/pbm.2019.0046
He, S. Q., Gao, M., Fu, Y. F., and Zhang, Y. N. (2015). Glycyrrhizic acid inhibits leukemia cell growth and migration via blocking AKT/mTOR/STAT3 signaling. Int. J. Clin. Exp. Pathol. 8 (5), 5175–5181.
Hibasami, H., Iwase, H., Yoshioka, K., and Takahashi, H. (2005). Glycyrrhizin induces apoptosis in human stomach cancer KATO III and human promyelotic leukemia HL-60 cells. Int. J. Mol. Med. 16 (2), 233–236. doi:10.3892/ijmm.16.2.233
Hostetler, B. J., Uchakina, O. N., Ban, H., and McKallip, R. J. (2017). Treatment of hematological malignancies with glycyrrhizic acid. Anticancer Res. 37 (3), 997–1004. doi:10.21873/anticanres.11409
Huang, R. Y., Chu, Y. L., Jiang, Z. B., Chen, X. M., Zhang, X., and Zeng, X. (2014). Glycyrrhizin suppresses lung adenocarcinoma cell growth through inhibition of thromboxane synthase. Cell Physiol. Biochem. 33 (2), 375–388. doi:10.1159/000356677
Huo, X., Meng, X., Zhang, J., and Zhao, Y. (2020). Hepatoprotective effect of different combinations of 18α-and 18β-Glycyrrhizic acid against CCl4-induced liver injury in rats. Biomed. Pharmacother. 122, 109354. doi:10.1016/j.biopha.2019.109354
Isbrucker, R. A., and Burdock, G. A. (2006). Risk and safety assessment on the consumption of Licorice root (Glycyrrhiza sp), its extract and powder as a food ingredient, with emphasis on the pharmacology and toxicology of glycyrrhizin. Regul. Toxicol. Pharmacol. 46 (3), 167–192. doi:10.1016/j.yrtph.2006.06.002
Jain, A. S., Dhawan, V. V., Sarmento, B., and Nagarsenker, M. S. (2016). In vitro and ex vivo evaluations of lipid anti-cancer nanoformulations: insights and assessment of bioavailability enhancement. AAPS PharmSciTech 17 (3), 553–571. doi:10.1208/s12249-016-0522-2
Jiang, L., Cheng, Q., Zhang, B., and Zhang, M. (2016). IL-13 induces the expression of 11βHSD2 in IL-13Rα2 dependent manner and promotes the malignancy of colorectal cancer. Am. J. Transl. Res. 8 (2), 1064–1072.
Johns, C. (2009). Glycyrrhizic acid toxicity caused by consumption of licorice candy cigars. CJEM 11 (1), 94–96. doi:10.1017/s1481803500010988
Ju, S. M., Kim, M. S., Jo, Y. S., Jeon, Y. M., Bae, J. S., Pae, H. O., et al. (2017). Licorice and its active compound glycyrrhizic acid ameliorates cisplatin-induced nephrotoxicity through inactivation of p53 by scavenging ROS and overexpression of p21 in human renal proximal tubular epithelial cells. Eur. Rev. Med. Pharmacol. Sci. 21 (4), 890–899.
Juin, S. K., Ghosh, S., and Majumdar, S. (2020). Glycyrrhizic acid facilitates anti-tumor immunity by attenuating Tregs and MDSCs: an immunotherapeutic approach. Int. Immunopharmacol. 88, 106932. doi:10.1016/j.intimp.2020.106932
Kanzawa, T., Ito, H., Kondo, Y., and Kondo, S. (2003). Current and future gene therapy for malignant gliomas. J. Biomed. Biotechnol. 2003 (1), 25–34. doi:10.1155/S1110724303209013
Kao, T. C., Shyu, M. H., and Yen, G. C. (2009). Neuroprotective effects of glycyrrhizic acid and 18beta-glycyrrhetinic acid in PC12 cells via modulation of the PI3K/Akt pathway. J. Agric. Food Chem. 57 (2), 754–761. doi:10.1021/jf802864k
Karimi, P., Islami, F., Anandasabapathy, S., Freedman, N. D., and Kamangar, F. (2014). Gastric cancer: descriptive epidemiology, risk factors, screening, and prevention. Cancer Epidemiol. Biomarkers Prev. 23 (5), 700–713. doi:10.1158/1055-9965.EPI-13-1057
Kelloff, G. J., Boone, C. W., Crowell, J. A., Steele, V. E., Lubet, R., and Sigman, C. C. (1994). Chemopreventive drug development: perspectives and progress. Cancer Epidemiol. Biomarkers Prev. 3 (1), 85–98.
Khan, R., Khan, A. Q., Lateef, A., Rehman, M. U., Tahir, M., Ali, F., et al. (2013). Glycyrrhizic acid suppresses the development of precancerous lesions via regulating the hyperproliferation, inflammation, angiogenesis and apoptosis in the colon of Wistar rats. PLoS One 8 (2), e56020. doi:10.1371/journal.pone.0056020
Khan, R., Rehman, M. U., Khan, A. Q., Tahir, M., and Sultana, S. (2018). Glycyrrhizic acid suppresses 1,2-dimethylhydrazine-induced colon tumorigenesis in Wistar rats: alleviation of inflammatory, proliferation, angiogenic, and apoptotic markers. Environ. Toxicol. 33 (12), 1272–1283. doi:10.1002/tox.22635
Khan, U., Karmakar, B. C., Basak, P., Paul, S., Gope, A., Sarkar, D., et al. (2023). Glycyrrhizin, an inhibitor of HMGB1 induces autolysosomal degradation function and inhibits Helicobacter pylori infection. Mol. Med. 29 (1), 51. doi:10.1186/s10020-023-00641-6
Kim, S. W., Jin, Y., Shin, J. H., Kim, I. D., Lee, H. K., Park, S., et al. (2012). Glycyrrhizic acid affords robust neuroprotection in the postischemic brain via anti-inflammatory effect by inhibiting HMGB1 phosphorylation and secretion. Neurobiol. Dis. 46 (1), 147–156. doi:10.1016/j.nbd.2011.12.056
Kishimoto, K., Hasegawa, D., Uemura, S., Nakamura, S., Kozaki, A., Saito, A., et al. (2021). Effect of monoammonium glycyrrhizinate on the development of hepatotoxicity after initial intrathecal chemotherapy for leukemia. Anticancer Res. 41 (12), 6231–6236. doi:10.21873/anticanres.15443
Kiso, Y., Tohkin, M., Hikino, H., Hattori, M., Sakamoto, T., and Namba, T. (1984). Mechanism of antihepatotoxic activity of glycyrrhizin. I: effect on free radical generation and lipid peroxidation. Planta Med. 50 (4), 298–302. doi:10.1055/s-2007-969714
Klein, I., Isensee, J., Wiesen, M. H. J., Imhof, T., Wassermann, M. K., Muller, C., et al. (2023). Glycyrrhizic acid prevents paclitaxel-induced neuropathy via inhibition of OATP-mediated neuronal uptake. Cells 12 (9), 1249. doi:10.3390/cells12091249
Klein, I., and Lehmann, H. C. (2021). Pathomechanisms of paclitaxel-induced peripheral neuropathy. Toxics 9 (10), 229. doi:10.3390/toxics9100229
Kobayashi, M., Fujita, K., Katakura, T., Utsunomiya, T., Pollard, R. B., and Suzuki, F. (2002). Inhibitory effect of glycyrrhizin on experimental pulmonary metastasis in mice inoculated with B16 melanoma. Anticancer Res. 22 (6C), 4053–4058.
Kohlschutter, J., Michelfelder, S., and Trepel, M. (2008). Drug delivery in acute myeloid leukemia. Expert Opin. Drug Deliv. 5 (6), 653–663. doi:10.1517/17425247.5.6.653
Lee, S. J., Kim, K. H., Park, J. S., Jung, J. W., Kim, Y. H., Kim, S. K., et al. (2007). Comparative analysis of cell surface proteins in chronic and acute leukemia cell lines. Biochem. Biophys. Res. Commun. 357 (3), 620–626. doi:10.1016/j.bbrc.2007.03.191
Li, J. Y., Cao, H. Y., Liu, P., Cheng, G. H., and Sun, M. Y. (2014a). Glycyrrhizic acid in the treatment of liver diseases: literature review. Biomed. Res. Int. 2014, 872139. doi:10.1155/2014/872139
Li, M., Xiang, L., and Li, Y. (2023). Efficacy and safety of compound glycyrrhizin in the patients with vitiligo: a systematic review and meta-analysis. Expert Rev. Clin. Pharmacol. 16 (6), 601–611. doi:10.1080/17512433.2023.2213887
Li, S., Zhu, J. H., Cao, L. P., Sun, Q., Liu, H. D., Li, W. D., et al. (2014b). Growth inhibitory in vitro effects of glycyrrhizic acid in U251 glioblastoma cell line. Neurol. Sci. 35 (7), 1115–1120. doi:10.1007/s10072-014-1661-4
Liang, G., Zhu, Y., Ali, D. J., Tian, T., Xu, H., Si, K., et al. (2020). Engineered exosomes for targeted co-delivery of miR-21 inhibitor and chemotherapeutics to reverse drug resistance in colon cancer. J. Nanobiotechnology 18 (1), 10. doi:10.1186/s12951-019-0563-2
Lim, E., Vaillant, F., Wu, D., Forrest, N. C., Pal, B., Hart, A. H., et al. (2009). Aberrant luminal progenitors as the candidate target population for basal tumor development in BRCA1 mutation carriers. Nat. Med. 15 (8), 907–913. doi:10.1038/nm.2000
Liu, J., Pandya, P., and Afshar, S. (2021). Therapeutic advances in oncology. Int. J. Mol. Sci. 22 (4), 2008. doi:10.3390/ijms22042008
Liu, W., Huang, S., Li, Y., Li, Y., Li, D., Wu, P., et al. (2018). Glycyrrhizic acid from licorice down-regulates inflammatory responses via blocking MAPK and PI3K/Akt-dependent NF-κB signalling pathways in TPA-induced skin inflammation. Medchemcomm 9 (9), 1502–1510. doi:10.1039/c8md00288f
Maione, F., Minosi, P., Di Giannuario, A., Raucci, F., Chini, M. G., De Vita, S., et al. (2019). Long-lasting anti-inflammatory and antinociceptive effects of acute ammonium glycyrrhizinate administration: pharmacological, biochemical, and docking studies. Molecules 24 (13), 2453. doi:10.3390/molecules24132453
Mano, Y., Abe, K., Takahashi, M., Higurashi, T., Kawano, Y., Miyazaki, S., et al. (2023). Optimal administration of glycyrrhizin avoids pharmacokinetic interactions with high-dose methotrexate and exerts a hepatoprotective effect. Anticancer Res. 43 (4), 1493–1501. doi:10.21873/anticanres.16298
Molyneux, G., Geyer, F. C., Magnay, F. A., McCarthy, A., Kendrick, H., Natrajan, R., et al. (2010). BRCA1 basal-like breast cancers originate from luminal epithelial progenitors and not from basal stem cells. Cell Stem Cell 7 (3), 403–417. doi:10.1016/j.stem.2010.07.010
Niznansky, L., Osinova, D., Kuruc, R., Hengerics Szabo, A., Szoradova, A., Masar, M., et al. (2022). Natural taxanes: from plant composition to human pharmacology and toxicity. Int. J. Mol. Sci. 23 (24), 15619. doi:10.3390/ijms232415619
Orazizadeh, M., Fakhredini, F., Mansouri, E., and Khorsandi, L. (2014). Effect of glycyrrhizic acid on titanium dioxide nanoparticles-induced hepatotoxicity in rats. Chem. Biol. Interact. 220, 214–221. doi:10.1016/j.cbi.2014.07.001
Penas-Prado, M., Armstrong, T. S., and Gilbert, M. R. (2012). Glioblastoma. Handb. Clin. Neurol. 105, 485–506. doi:10.1016/B978-0-444-53502-3.00004-5
Qamar, W., Khan, R., Khan, A. Q., Rehman, M. U., Lateef, A., Tahir, M., et al. (2012). Alleviation of lung injury by glycyrrhizic acid in benzo(a)pyrene exposed rats: probable role of soluble epoxide hydrolase and thioredoxin reductase. Toxicology 291 (1-3), 25–31. doi:10.1016/j.tox.2011.10.012
Qin, S., Wang, H., Yuan, R., Li, H., Ochani, M., Ochani, K., et al. (2006). Role of HMGB1 in apoptosis-mediated sepsis lethality. J. Exp. Med. 203 (7), 1637–1642. doi:10.1084/jem.20052203
Qu, B., Xing, R., Wang, H., Chen, X., Ge, Q., Peng, D., et al. (2017). Multiple effects of magnesium isoglycyrrhizinate on the disposition of docetaxel in docetaxel-induced liver injury. Xenobiotica 47 (4), 290–296. doi:10.1080/00498254.2016.1185195
Qu, L., Chen, C., Chen, Y., Li, Y., Tang, F., Huang, H., et al. (2019). High-mobility group box 1 (HMGB1) and autophagy in acute lung injury (ALI): a review. Med. Sci. Monit. 25, 1828–1837. doi:10.12659/MSM.912867
Radwant, M. A., and Aboul-Enein, H. Y. (2002). The effect of oral absorption enhancers on the in vivo performance of insulin-loaded poly(ethylcyanoacrylate) nanospheres in diabetic rats. J. Microencapsul. 19 (2), 225–235. doi:10.1080/02652040110081406
Ramkumar, V., Mukherjea, D., Dhukhwa, A., and Rybak, L. P. (2021). Oxidative stress and inflammation caused by cisplatin ototoxicity. Antioxidants (Basel) 10 (12), 1919. doi:10.3390/antiox10121919
Rastogi, V., Yadav, P., Bhattacharya, S. S., Mishra, A. K., Verma, N., Verma, A., et al. (2014). Carbon nanotubes: an emerging drug carrier for targeting cancer cells. J. Drug Deliv. 2014, 670815. doi:10.1155/2014/670815
Ruszymah, B. H., Nabishah, B. M., Aminuddin, S., and Khalid, B. A. (1995). Effects of glycyrrhizic acid on right atrial pressure and pulmonary vasculature in rats. Clin. Exp. Hypertens. 17 (3), 575–591. doi:10.3109/10641969509037425
Safarzadeh, E., Sandoghchian Shotorbani, S., and Baradaran, B. (2014). Herbal medicine as inducers of apoptosis in cancer treatment. Adv. Pharm. Bull. 4 (1), 421–427. doi:10.5681/apb.2014.062
Said Salem, N. I., Noshy, M. M., and Said, A. A. (2017). Modulatory effect of curcumin against genotoxicity and oxidative stress induced by cisplatin and methotrexate in male mice. Food Chem. Toxicol. 105, 370–376. doi:10.1016/j.fct.2017.04.007
Santos, M. L. C., de Brito, B. B., da Silva, F. A. F., Botelho, A., and de Melo, F. F. (2020). Nephrotoxicity in cancer treatment: an overview. World J. Clin. Oncol. 11 (4), 190–204. doi:10.5306/wjco.v11.i4.190
Selyutina, O. Y., and Polyakov, N. E. (2019). Glycyrrhizic acid as a multifunctional drug carrier - from physicochemical properties to biomedical applications: a modern insight on the ancient drug. Int. J. Pharm. 559, 271–279. doi:10.1016/j.ijpharm.2019.01.047
Shan, Y., Liu, P., Zhou, Y., Ding, X., Liu, H., and Yang, J. (2023). Prenatal sevoflurane exposure impairs the learning and memory of rat offspring via HMGB1-induced NLRP3/ASC inflammasome activation. ACS Chem. Neurosci. 14 (4), 699–708. doi:10.1021/acschemneuro.2c00620
Shustik, C., Bence-Bruckler, I., Delage, R., Owen, C. J., Toze, C. L., and Coutre, S. (2017). Advances in the treatment of relapsed/refractory chronic lymphocytic leukemia. Ann. Hematol. 96 (7), 1185–1196. doi:10.1007/s00277-017-2982-1
Smolarczyk, R., Cichon, T., Matuszczak, S., Mitrus, I., Lesiak, M., Kobusinska, M., et al. (2012). The role of Glycyrrhizin, an inhibitor of HMGB1 protein, in anticancer therapy. Arch. Immunol. Ther. Exp. Warsz. 60 (5), 391–399. doi:10.1007/s00005-012-0183-0
Sohn, E. J., Kang, D. G., and Lee, H. S. (2003). Protective effects of glycyrrhizin on gentamicin-induced acute renal failure in rats. Pharmacol. Toxicol. 93 (3), 116–122. doi:10.1034/j.1600-0773.2003.930302.x
Staff, N. P., Grisold, A., Grisold, W., and Windebank, A. J. (2017). Chemotherapy-induced peripheral neuropathy: a current review. Ann. Neurol. 81 (6), 772–781. doi:10.1002/ana.24951
Stecanella, L. A., Bitencourt, A. P. R., Vaz, G. R., Quarta, E., Silva Junior, J. O. C., and Rossi, A. (2021). Glycyrrhizic acid and its hydrolyzed metabolite 18β-glycyrrhetinic acid as specific ligands for targeting nanosystems in the treatment of liver cancer. Pharmaceutics 13 (11), 1792. doi:10.3390/pharmaceutics13111792
Su, X., Wu, L., Hu, M., Dong, W., Xu, M., and Zhang, P. (2017). Glycyrrhizic acid: A promising carrier material for anticancer therapy. Biomed. Pharmacother. 95, 670–678. doi:10.1016/j.biopha.2017.08.123
Sun, W., Ding, J., Wu, K., Ning, B. F., Wen, W., Sun, H. Y., et al. (2011). Gankyrin-mediated dedifferentiation facilitates the tumorigenicity of rat hepatocytes and hepatoma cells. Hepatology 54 (4), 1259–1272. doi:10.1002/hep.24530
Sung, H., Ferlay, J., Siegel, R. L., Laversanne, M., Soerjomataram, I., Jemal, A., et al. (2021). Global cancer statistics 2020: gLOBOCAN estimates of incidence and mortality worldwide for 36 cancers in 185 countries. CA Cancer J. Clin. 71 (3), 209–249. doi:10.3322/caac.21660
Tsai, J. J., Pan, P. J., Hsu, F. T., Chung, J. G., and Chiang, I. T. (2020). Glycyrrhizic acid modulates apoptosis through extrinsic/intrinsic pathways and inhibits protein kinase B- and extracellular signal-regulated kinase-mediated metastatic potential in hepatocellular carcinoma in vitro and in vivo. Am. J. Chin. Med. 48 (1), 223–244. doi:10.1142/S0192415X20500123
van der Schoot, G. G. F., Westerink, N. L., Lubberts, S., Nuver, J., Zwart, N., Walenkamp, A. M. E., et al. (2016). Variation in the HFE gene is associated with the development of bleomycin-induced pulmonary toxicity in testicular cancer patients. Eur. J. Cancer 59, 134–141. doi:10.1016/j.ejca.2016.02.013
van Rossum, T. G., Vulto, A. G., Hop, W. C., Brouwer, J. T., Niesters, H. G., and Schalm, S. W. (1999). Intravenous glycyrrhizin for the treatment of chronic hepatitis C: a double-blind, randomized, placebo-controlled phase I/II trial. J. Gastroenterol. Hepatol. 14 (11), 1093–1099. doi:10.1046/j.1440-1746.1999.02008.x
Wakamatsu, T., Nakahashi, Y., Hachimine, D., Seki, T., and Okazaki, K. (2007). The combination of glycyrrhizin and lamivudine can reverse the cisplatin resistance in hepatocellular carcinoma cells through inhibition of multidrug resistance-associated proteins. Int. J. Oncol. 31 (6), 1465–1472. doi:10.3892/ijo.31.6.1465
Wang, H., Ge, X., Qu, H., Wang, N., Zhou, J., Xu, W., et al. (2020). Glycyrrhizic acid inhibits proliferation of gastric cancer cells by inducing cell cycle arrest and apoptosis. Cancer Manag. Res. 12, 2853–2861. doi:10.2147/CMAR.S244481
Wang, H., Wang, H., Zhang, W., Huang, H. J., Liao, W. S., and Fuller, G. N. (2004). Analysis of the activation status of Akt, NFkappaB, and Stat3 in human diffuse gliomas. Lab. Invest. 84 (8), 941–951. doi:10.1038/labinvest.3700123
Wang, Q., Huang, Y., Li, Y., Zhang, L., Tang, H., Zhang, J., et al. (2022). Glycyrrhizic acid mitigates tripterygium-glycoside-tablet-induced acute liver injury via PKM2 regulated oxidative stress. Metabolites 12 (11), 1128. doi:10.3390/metabo12111128
Wang, Q. S., Gao, L. N., Zhu, X. N., Zhang, Y., Zhang, C. N., Xu, D., et al. (2019). Co-delivery of glycyrrhizin and doxorubicin by alginate nanogel particles attenuates the activation of macrophage and enhances the therapeutic efficacy for hepatocellular carcinoma. Theranostics 9 (21), 6239–6255. doi:10.7150/thno.35972
Wang, Q., Wang, J., Wang, J., Hong, S., Han, F., Chen, J., et al. (2017). HMGB1 induces lung fibroblast to myofibroblast differentiation through NF‑κB‑mediated TGF‑β1 release. Mol. Med. Rep. 15 (5), 3062–3068. doi:10.3892/mmr.2017.6364
Wang, Z. Y., and Nixon, D. W. (2001). Licorice and cancer. Nutr. Cancer 39 (1), 1–11. doi:10.1207/S15327914nc391_1
Waseem, M., Bhardwaj, M., Tabassum, H., Raisuddin, S., and Parvez, S. (2015). Cisplatin hepatotoxicity mediated by mitochondrial stress. Drug Chem. Toxicol. 38 (4), 452–459. doi:10.3109/01480545.2014.992437
Wong, S. Y., and Hynes, R. O. (2006). Lymphatic or hematogenous dissemination: how does a metastatic tumor cell decide? Cell Cycle 5 (8), 812–817. doi:10.4161/cc.5.8.2646
Wu, C. H., Chen, A. Z., and Yen, G. C. (2015). Protective effects of glycyrrhizic acid and 18β-glycyrrhetinic acid against cisplatin-induced nephrotoxicity in BALB/c mice. J. Agric. Food Chem. 63 (4), 1200–1209. doi:10.1021/jf505471a
Wu, X., Wang, W., Chen, Y., Liu, X., Wang, J., Qin, X., et al. (2018). Glycyrrhizin suppresses the growth of human NSCLC cell line HCC827 by downregulating HMGB1 level. Biomed. Res. Int. 2018, 6916797. doi:10.1155/2018/6916797
Xiong, X., Gu, L., Wang, Y., Luo, Y., Zhang, H., Lee, J., et al. (2016). Glycyrrhizin protects against focal cerebral ischemia via inhibition of T cell activity and HMGB1-mediated mechanisms. J. Neuroinflammation 13 (1), 241. doi:10.1186/s12974-016-0705-5
Xu, C., Liang, C., Sun, W., Chen, J., and Chen, X. (2018). Glycyrrhizic acid ameliorates myocardial ischemic injury by the regulation of inflammation and oxidative state. Drug Des. Devel Ther. 12, 1311–1319. doi:10.2147/DDDT.S165225
Yang, F. H., Zhang, Q., Liang, Q. Y., Wang, S. Q., Zhao, B. X., Wang, Y. T., et al. (2015). Bioavailability enhancement of paclitaxel via a novel oral drug delivery system: paclitaxel-loaded glycyrrhizic acid micelles. Molecules 20 (3), 4337–4356. doi:10.3390/molecules20034337
Yang, G., Li, J., Cai, Y., Yang, Z., Li, R., and Fu, W. (2018). Glycyrrhizic acid alleviates 6-hydroxydopamine and corticosterone-induced neurotoxicity in SH-SY5Y cells through modulating autophagy. Neurochem. Res. 43 (10), 1914–1926. doi:10.1007/s11064-018-2609-5
Yang, T., Lan, Y., Cao, M., Ma, X., Cao, A., Sun, Y., et al. (2019). Glycyrrhetinic acid-conjugated polymeric prodrug micelles co-delivered with doxorubicin as combination therapy treatment for liver cancer. Colloids Surf. B Biointerfaces 175, 106–115. doi:10.1016/j.colsurfb.2018.11.082
Yi, W., Xiao, E., Ding, R., Luo, P., and Yang, Y. (2016). High expression of fibronectin is associated with poor prognosis, cell proliferation and malignancy via the NF-κB/p53-apoptosis signaling pathway in colorectal cancer. Oncol. Rep. 36 (6), 3145–3153. doi:10.3892/or.2016.5177
Yin, W., Wang, J., Jiang, L., and James Kang, Y. (2021). Cancer and stem cells. Exp. Biol. Med. (Maywood) 246 (16), 1791–1801. doi:10.1177/15353702211005390
Zhang, H., Huang, Q., Zhai, J., Zhao, Y. N., Zhang, L. P., Chen, Y. Y., et al. (2015). Structural basis for 18-beta-glycyrrhetinic acid as a novel non-GSH analog glyoxalase I inhibitor. Acta Pharmacol. Sin. 36 (9), 1145–1150. doi:10.1038/aps.2015.59
Zhou, J. X., and Wink, M. (2018). Reversal of multidrug resistance in human colon cancer and human leukemia cells by three plant extracts and their major secondary metabolites. Med. (Basel) 5 (4), 123. doi:10.3390/medicines5040123
Zhu, Z. H., Li, X., He, L. F., Cai, H. F., Ye, B., and Wu, Z. M. (2021). Glycyrrhizic acid, as an inhibitor of HMGB1, alleviates bleomycin-induced pulmonary toxicity in mice through the MAPK and Smad3 pathways. Immunopharmacol. Immunotoxicol. 43 (4), 461–470. doi:10.1080/08923973.2021.1939371
Zuo, J., Meng, T., Wang, Y., and Tang, W. (2023). A review of the antiviral activities of glycyrrhizic acid, glycyrrhetinic acid and glycyrrhetinic acid monoglucuronide. Pharm. (Basel) 16 (5), 641. doi:10.3390/ph16050641
Keywords: cancer, glycyrrhizic acid, anti-cancer, side effects, mechanisms
Citation: Zhang Y, Sheng Z, Xiao J, Li Y, Huang J, Jia J, Zeng X and Li L (2023) Advances in the roles of glycyrrhizic acid in cancer therapy. Front. Pharmacol. 14:1265172. doi: 10.3389/fphar.2023.1265172
Received: 22 July 2023; Accepted: 07 August 2023;
Published: 15 August 2023.
Edited by:
Zhaofeng Liang, Jiangsu University, ChinaReviewed by:
Yang Ye, Jiangsu University, ChinaCopyright © 2023 Zhang, Sheng, Xiao, Li, Huang, Jia, Zeng and Li. This is an open-access article distributed under the terms of the Creative Commons Attribution License (CC BY). The use, distribution or reproduction in other forums is permitted, provided the original author(s) and the copyright owner(s) are credited and that the original publication in this journal is cited, in accordance with accepted academic practice. No use, distribution or reproduction is permitted which does not comply with these terms.
*Correspondence: Xiansi Zeng, enhzLTIwMDVAdmlwLjE2My5jb20=; Li Li, TGlseTc1ODhAMTYzLmNvbQ==
†These authors have contributed equally to this work
Disclaimer: All claims expressed in this article are solely those of the authors and do not necessarily represent those of their affiliated organizations, or those of the publisher, the editors and the reviewers. Any product that may be evaluated in this article or claim that may be made by its manufacturer is not guaranteed or endorsed by the publisher.
Research integrity at Frontiers
Learn more about the work of our research integrity team to safeguard the quality of each article we publish.