- Third Hospital of Shanxi Medical University, Shanxi Bethune Hospital, Shanxi Academy of Medical Sciences, Tongji Shanxi Hospital, Taiyuan, China
Systemic sclerosis (SSc) is a connective tissue disease with an unknown etiology. Clinically, it is characterized by localized or diffuse skin thickening and fibrosis. The pathogenesis of SSc includes microvascular injury, autoimmune-mediated inflammation, and fibroblast activation. These processes interact and contribute to the diverse clinicopathology and presentation of SSc. Given the limited effectiveness and substantial side effects of traditional treatments, the treatment strategy for SSc has several disadvantages. Mesenchymal stem cells (MSCs) are expected to serve as effective treatment options owing to their significant immunomodulatory, antifibrotic, and pro-angiogenic effects. Exosomes, secreted by MSCs via paracrine signaling, mirror the effect of MSCs as well as offer the benefit of targeted delivery, minimal immunogenicity, robust reparability, good safety and stability, and easy storage and transport. This enables them to circumvent the limitations of the MSCs. When using exosomes, it is crucial to consider preparation methods, quality standards, and suitable drug delivery systems, among other technical issues. Therefore, this review aims to summarize the latest research progress on MSCs and exosomes in SSc, offering novel ideas for treating SSc.
1 Introduction
Systemic sclerosis (SSc) is a type of autoimmune connective tissue disease involving multiple organs and characterized by skin fibrosis. In this disease condition, autoantibodies and collagen accumulation compromise the normal tissue architecture, leading to skin fibrosis and multiple organ dysfunction (Tamby et al., 2003; Denton and Black, 2004; Servettaz et al., 2006). Furthermore, despite a prevalence ranging from 50 to 300 cases per million and a predilection for individuals aged 30–50 years, the standardized mortality rate of SSc has shown insignificant change over the last 40 years, with one-third of patients dying within 10 years of disease diagnosis (Rubio-Rivas et al., 2014). Interstitial lung disease is the most common cause of death in patients with SSc. Previously, numerous therapy alternatives, including glucocorticoids, immunosuppressants, and antifibrotic medicines, have been utilized for symptom management and disease progression inhibition, yielding unsatisfactory outcomes. Autologous hematopoietic stem cell transplantation (AHSCT) emerged for treating refractory SSc in the past decade. This approach improves the degree of skin sclerosis and visceral lesions and significantly augments the overall survival of patients with SSc. The requirement for immunosuppressive medications before AHSCT, with concerns about transplantation-related mortality, introduces risks of infection, making broad adoption of AHSCT challenging (Turse et al., 2018). Recent studies have found a trend toward enhanced Forced Vital Capacity in patients with SSc treated with the biological drug Romilkimab compared with those not treated with it. However, phase III trials are needed to confirm these findings (Allanore et al., 2020). Consequently, there is an urgent need for medications with high efficacy and minimal adverse effects.
When activated by various inflammatory stimuli and cells, mesenchymal stem cells (MSCs) can migrate to the damaged location through blood arteries. They can perform anti-inflammatory, antifibrotic, and tissue repair functions by secreting soluble substances and extracellular vesicles. MSCs and their exosomes also have immunomodulatory properties. Exosomes, which are extracellular vesicles, serve a crucial function in parental cells and are carriers of a number of bioactive substances, making them more effective therapeutically (Cras et al., 2015). The potential of MSCs to treat diseases is being substantiated as researchers focus more on them. Furthermore, they are becoming increasingly essential for tissue engineering, tissue organ transplantation, gene therapy, and immunotherapy. Therefore, this review aims to outline the recent advancements in MSCs and their exosomes in systemic sclerosis. The findings of this study serve to provide a reference point for guiding future clinical research in treating patients with SSc.
2 Overview of MSCs and their exosomes
MSCs are adnexal, heterogeneous cells obtained from multiple organs with multidirectional differentiation potential (Friedenstein et al., 1976; Pittenger et al., 1999; Reinders et al., 2010; Ding et al., 2011; Shi et al., 2012). MSCs exhibit positive surface expression of CD73, CD90, and CD105 but negative surface expression of CD11b, CD14, CD19, CD79a, CD34, and CD45 (Dominici et al., 2006). MSCs are commonly used to treat immune system illnesses due to their non-rejection, self-healing, and immunomodulatory properties.
Exosomes are membrane nanovesicles derived from endosomes and range from 30 to 150 nm in size. They are secreted by all living cells and typically contain different biomolecules, such as proteins, lipids, cytokines, and RNAs (Cras et al., 2015; van Rhijn-Brouwer et al., 2016), which facilitate cell-to-cell signaling. MSC-derived exosomes include mRNAs, miRNAs, cytokines, and growth factors (Soundara et al., 2017). They have garnered much attention due to their lower immunogenicity than MSCs, coupled with their reduced risk of tumor formation (Moghadasi et al., 2021).
3 Pathogenesis of SSc
The pathogenesis of SSc primarily resolves around immune dysfunction (Dumoitier et al., 2014; Ganesan et al., 2023). This dysfunction disrupts B-cell homeostasis, thereby triggering autoantibody production and profibrotic cytokine secretion, such as interleukin-6 (IL-6) and interleukin-12 (IL-21) (FForestier et al., 2018). Furthermore, T-cell and specific helper T (Th cells) cells are essential for SSc pathophysiology. Profibrotic cytokines, specifically interleukin-13 and interleukin-4 (IL-4), are generated by Th2 cells, which are important in fibroblast activation and differentiation to myofibroblasts (Postlethwaite et al., 1992; Berlow et al., 2018). Additionally, Th17 cells exert pro-inflammatory effects. Nevertheless, the underlying mechanism through which they promote fibrosis remains partially understood (Xing et al., 2013; Liu et al., 2014; Liu et al., 2020; Xing et al., 2020). The pro-inflammatory cytokine chemokine (C-X-C motif) ligand 2 (CXCL 2) and collagen deposition have been associated with the profibrotic action of IL-17 A on fibroblasts (Brembilla et al., 2013; Vettori et al., 2020). Conversely, another study showed an inverse correlation between IL-17 A levels and disease activity, having a protective effect in SSc. IL-17 A inhibits fibroblast-to-myofibroblast transformation via transforming growth factor β (TGF-β), hindering collagen production by SSc fibroblasts in vitro (Truchetet et al., 2013). In a study conducted by Chizzolini et al. using 3D organotypic skin analogs, IL-17 A stimulates the expression of pro-inflammatory genes without affecting collagen formation (Dufour et al., 2020). T follicular helper cells (TFH cells), a subset of CD4+ T-cell, can localize in B-cell follicles through elevated expression of the chemokine receptor type 5 (CXCR5), thereby promoting B-cell immunoglobulin production (Breitfeld et al., 2000; Schaerli et al., 2000). Furthermore, TFH cells can improve immunoglobulin secretion by secreting IL-21 and stimulating B-cell proliferation. Circulating T follicular helper cells (cTfh cells) also function as TFH cells. Elevated IL-21 levels were observed in the serum of patients with SSc than in healthy individuals. This elevation is correlated with the number of plasmablasts, suggesting that abnormal circulating TFH cell expression in patients with SSc could contribute to B-cell changes. Cultivating TFH cells with their B-cell from patients with SSc stimulates plasma cell development and leads to significant levels of immunoglobulin synthesis in vitro (Ricard et al., 2019). Taken together, circulating TFH cells exhibit dysregulation in SSc. The resulting immunological defects generated by cTfh cells foster aberrant B-cell activation and differentiation, primarily through excessive production of IL-21. Histologically, several studies have revealed an association between T lymphocytes and skin fibrosis (Lei et al., 2016; Huang et al., 2020), including the infiltration of TFH-like cells in skin lesions of patients with SSc (Taylor et al., 2018; Maehara et al., 2020; Ly et al., 2021). The frequency of TFH-like cell occurrence in the fibrotic lesions of patients with SSc remains uncertain despite their identification of these lesions. Other T lymphocyte subpopulations, such as Cyclin-CD8+ T lymphocytes, have been shown to be responsible for the skin tissue fibrotic process (Francois et al., 2013; Dumoitier et al., 2017; Li, 2018; Maehara et al., 2020).
Furthermore, macrophages are crucial in scleroderma (Christmann and Lafyatis, 2010). Monocytes enter tissues and, depending on their surroundings, differentiate into macrophages. M1 macrophages phagocytose foreign pathogens, releasing pro-inflammatory molecules, including tumor necrosis factor α, IL-6, and IL-12, activating other immune cells, and triggering the inflammatory response. Conversely, M2 macrophages reduce the inflammatory response by generating interleukin 4, 10, and 13 to repair and remodel tissues, ultimately promoting fibrosis (Figure 1). Therefore, M1 polarization to the M2 type is one of the main causes of M1/M2 macrophage disproportion, leading to SSc fibrosis (Christmann and Lafyatis, 2010). In summary, macrophages trigger the immune system through various pathways, releasing pro-inflammatory and profibrotic mediators. This process spurs fibroblast activation into myofibroblasts, thereby producing large amounts of extracellular matrix, ultimately causing fibrosis.
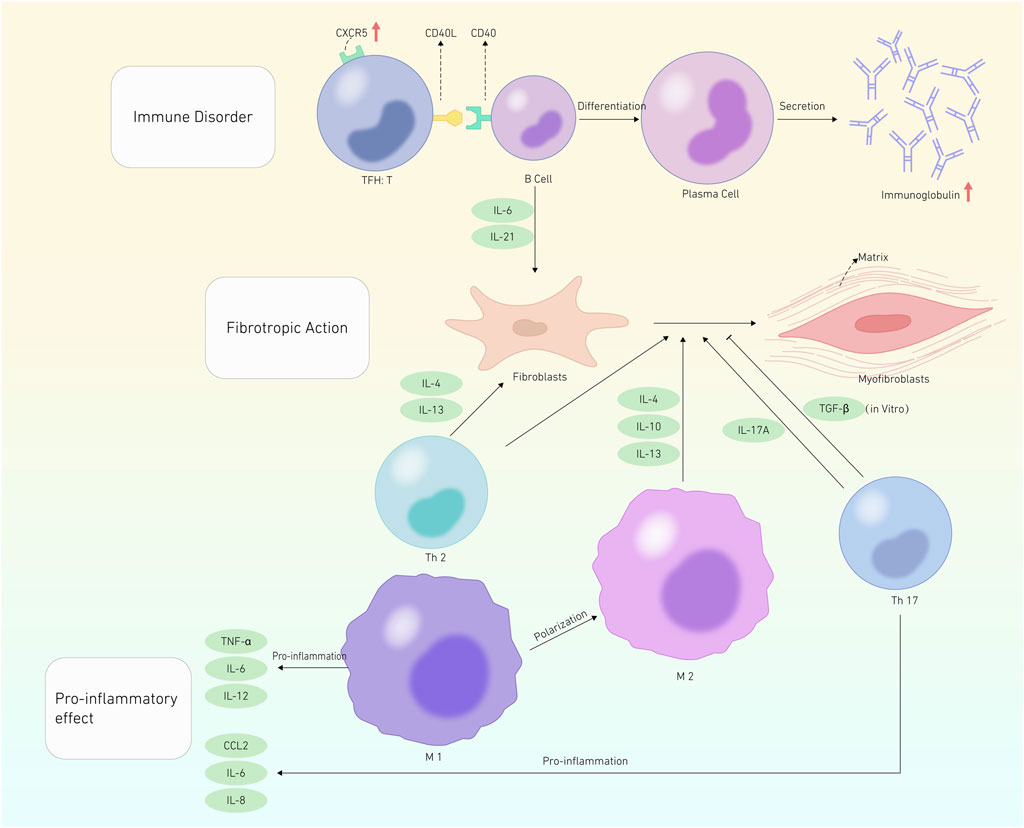
FIGURE 1. Pathogenesis of SSc. This graphic depicts systemic sclerosis pathogenesis in terms of Immune disoder, Fibrotropic action, and Pro-inflammatory effects. The red cut head represents an increase in the substance’s quantity. The promotion effect is represented by a black shear head, whereas the inhibitory effect is represented by a flat shear head.
4 MSCs and their exosomes in SSc
4.1 MSCs and their exosomes in SSc-associated interstitial lung disease
Interstitial lung disease (ILD) is one of the most common manifestations of SSc. Currently, SSc-associated ILD has a mere 10-year survival rate of only 40%–50% and is the leading cause of SSc-related death (Kawano and Chung, 2021).
4.1.1 Pathogenesis of SSc-associated ILD
Collagen is the main component of the extracellular matrix in lung tissue. It is crucial in maintaining the structural integrity of lung tissue. The occurrence of pulmonary interstitial fibrosis is ultimately caused by abnormal extracellular matrix metabolism, which promotes increased fibrotic factor production or relatively insufficient antifibrotic factor production, culminating in the progression of pulmonary fibrosis. Alveolitis frequently occurs in the early stages of pulmonary fibrosis. It is marked by inflammation in the alveoli and the accumulation of serous fluid and cellular components. The inflammatory process is spurred by numerous monocytes, lymphocytes, plasma cells, alveolar macrophages, and other inflammatory cells infiltrating the pulmonary interstitium. However, the integrity of the alveolar structure remains unaffected. During the advanced stages, the alveoli are replaced by firm collagen, leading to the destruction of the alveolar walls. This process culminates in dilated honeycomb-shaped spaces within the lungs, all of which are pathological changes (Li et al., 2018).
The pathogenesis of pulmonary interstitial fibrosis induced by SSc remains unclear. However, it is established that an abnormal repair process is initiated upon recurrent minor injuries to the alveolar epithelium. This process occurs when immune cells attack the lungs, triggering the epithelial-mesenchymal transition. Consequently, fibroblasts are stimulated and secrete enormous amounts of extracellular matrix. This process promotes the production of cytokines, such as TGF-β and IL-1, by activating the nuclear factor kB and mitogen-activated protein kinase (MAPK) pathways. The cytokines, via autocrine and paracrine effects, mediate the movement of inflammatory cells, including macrophages, toward the stimulated site and promote the proliferation and differentiation of fibroblastic cells (Li et al., 2018). In contrast, RNA-binding protein 7 exhibited increased levels in injured lung epithelial cells in a mouse bleomycin-induced pulmonary fibrosis model. It then interfered with the repair and regeneration process by promoting apoptosis. Moreover, it triggered the degradation of nuclear-enriched abundant transcript 1, resulting in the apoptosis of epithelial cells. This sequence of events subsequently stimulated the production of chemokine (C-X-C motif) ligand 12 (CXCL 12). This led to the recruitment of fibrotic monocyte populations to the damaged areas by segregated-nucleus-containing atypical monocytes (SatM), thereby promoting pulmonary fibrosis (Fukushima and Akira, 2021). Furthermore, activated leukocyte cell adhesion molecules, specifically CD166 (ALCAM; CD166), can block TGF-β and extracellular signal-regulated protein kinase (ERK) signaling pathways. This consequently curtails TGF-β and ERK signaling pathway activation within lung tissues, reducing apoptosis, inhibiting lung tissue repair, and escalating lung fibrosis (Kim et al., 2022).
4.1.2 Mechanism and efficacy of MSCs and their exosomes in treating SSc-ILD
MSCs can induce immunosuppression by bolstering the regulatory T-cell (Treg cells) and IL-10-secreting regulatory B-cell (IL-10+ Breg cells) levels. In addition, MSCs contribute to tissue repair by secreting numerous growth factors (Cahill et al., 2016; Li et al., 2017; Tseng and Hoon, 2021). Furthermore, MSCs can use intracellular channels or extracellular vesicles (EVs), mediated by gap junction proteins (EVs), to facilitate the transport of mitochondria from MSCs to impaired cells. This process restores ATP accumulation and function (Morrison et al., 2017; Paliwal et al., 2018). MSCs can mitigate inflammation by secreting inflammatory factors such as IL-4 and prostaglandin E2 (PGE-2) (Shirjang et al., 2017; Harrell et al., 2020). When intercellular contact molecules or soluble factors secreted by MSCs (such as CD45, CD44, CD86, and CD163) interact with T-cell, they can inhibit their maturation. Moreover, these soluble factors can diminish dendritic cell activation and proliferation while inhibiting natural killer cell cytotoxicity (Ni et al., 2018; de Castro et al., 2019; He et al., 2020). MSCs and their exosomes also exhibit antifibrotic attributes by reducing the amount of profibrotic M2 phenotype macrophages (Willis et al., 2018; Luo et al., 2019). Furthermore, they directly counteract fibrotic processes by regulating the ratio of metalloproteinases/tissue inhibitors of metalloproteinases (Xu et al., 2017; Chu et al., 2019). Finally, MSCs can hinder the epithelial-mesenchymal transition, promoting angiogenesis and alveolar repair.
Numerous animal studies have shown that MSCs are effective in treating pulmonary fibrosis (Yang et al., 2021). According to Pereira-Simon et al., intervention in a bleomycin-induced pulmonary fibrosis model revealed that adipose-derived MSCs (AD-MSCs) and umbilical cord-derived MSCs (UC-MSCs) had a palliative impact on pulmonary fibrosis (Periera-Simon et al., 2021). Therefore, this suggests that MSCs from various sources have a therapeutic effect on pulmonary fibrosis. Furthermore, Tashiro et al. investigated the differences between young and old male mice through intratracheal injections of AD-MSCs following bleomycin administration. They found that mice treated with bleomycin from young donor AD-MSCs exhibited significantly lower pulmonary fibrosis and other inflammatory markers than those treated with bleomycin from older donors (Caplan, 2017). This highlights the fact that donor-related factors influence the efficacy of MSCs. In 2017, Zhang et al. conducted a clinical experiment where UC-MSCs were injected into 14 patients with diffuse cutaneous SSc (injection site not reported). They found decreased skin thickness, improved lung function, and adverse reactions of upper respiratory tract infection and diarrhea (Zhang et al., 2017). Furthermore, Zhang et al. found that following intravenous administration of allogeneic UC-MSCs (1 × 106 cells/kg) to patients with interstitial lung disease, lung function, and CT images displayed improvement in all three patients after undergoing 12 months of comprehensive treatment (Ferreli et al., 2017). Farge et al. found that after a single intravenous infusion of allogeneic bone marrow-derived MSCs (BM-MSCs) to 20 patients, their pulmonary function remained stable after 1 year of follow-up. These findings indicate that MSCs have exhibited safe usage and minimal adverse reactions in clinical scenarios, thus offering a promising therapeutic outlook for SSc-ILD.
MSCs serve as one source of exosomes for treating SSc-associated pulmonary fibrosis. In a previous study, we discovered that exosome-derived MSCs can affect lung fibrosis and control cytokine expression linked to this condition. Mansouri et al. found that BM-MSC-derived exosomes significantly reduced bleomycin-induced pulmonary fibrosis 7 days after creating an animal model. These exosomes also altered the inflammatory response of lung tissue. In addition, they found that prior administration of BM-MSC-derived exosomes before bone marrow mononuclear cell transplantation prevented pulmonary fibrosis (Mansouri et al., 2019). Furthermore, UC-MSC-derived exosomes can reduce collagen deposition in lung tissue and alleviate bleomycin-induced pulmonary fibrosis by inhibiting the epithelial-mesenchymal transition process. This process is activated by TGF-β1/mothers against decapentaplegic homolog 2/3 (Smad2/3) signaling pathway (Yang J. et al., 2020).
4.2 Research advancement in MSCs and their exosomes in treating SSc-associated skin fibrosis
With modern advancements in science and technology, MSC transplantation provides a promising therapeutic option for treating skin fibrosis in SSc. Results from animal experimental studies revealed that MSCs were effective in treating skin fibrosis in an SSc-mouse model. MSC also reduced collagen synthesis and collagen-Iα1, collagen-Iα2, fibronectin 1, and α-smooth muscle actin gene expression levels. These combined effects underscore their antifibrotic role. In addition, MSC treatment decreased TGF-β, interferon-γ, IL-10, IL-1b, and IL-6 mRNAs expression, indicating the anti-inflammatory effect of MSCs (Yu et al., 2022). The National Institutes of Health (NIH) published a study in 2017 showing that subcutaneous injection of AD-MSCs reduced skin fibrosis in mice and significantly decreased the amount of hydroxyproline in subcutaneous tissue. UC-MSCs have garnered more attention than BM-MSCs due to their simpler collection process, ethical considerations, and safety factors (Arutyunyan et al., 2016). Besides sharing characteristics with BM-MSCs, our previous research highlighted that UC-MSCs also exhibited various immunomodulatory molecules, including TGF-β1, indoleamine2,3-dioxygenase (IDO), tumor necrosis factor-alpha stimulated gene-6, and PGE-2 (Sabapathy et al., 2014). Based on the specific benefits of UC-MSCs, Yang, Yuan, et al. (2020) revealed that UC-MSCs suppressed the inflammatory response at the lesions and inhibited bleomycin-induced collagen production at the lesions, thus lowering fibrosis (Rad et al., 2019; Yang Y. et al., 2020). The animal trials conducted previously demonstrated that MSCs can markedly improve skin fibrosis, a breakthrough that opens up a new avenue for using MSCs in treating SSc-related skin fibrosis, presenting a novel treatment alternative. These findings further imply that MSCs can ameliorate the fibrotic condition of the skin, thereby resulting in skin regeneration. A recent study revealed that UC-MSC-derived exosomes reduced bleomycin-induced skin fibrosis for at least 3 weeks (Yu et al., 2022). In addition, we discovered that UC-MSC-derived exosomes inhibited the conversion of vascular endothelial cells to mesenchymal cells (Alexander and Greco, 2022). In a clinical trial, Khanna et al. (2018) injected AD-MSCs subcutaneously into all fingers of patients with impaired function, including diffuse and localized sclerosis cases. The findings of the study revealed several improvements: the Cochin Hand Function Score improved, the Range of Motion Score decreased, and the Sheffield Hand Assessment Questionnaire score indicated an increase. Additionally, the EQ(5D) 5-level assessment showed improvement in patients with diffuse, and the global assessment of SSc activity for diffuse patients improved compared with the corresponding parameters before the intervention. However, the intervention was associated with sequelae, such as upper respiratory tract infection, arthralgia, cellulitis, limb pain, hypoesthesia, and other related symptoms (Magalon et al., 2019). Guiducci et al. found that intravenous infusion of MSCs into patients with SSc led to decreased necrotic skin area, microscopic tubular cell populations on skin section analysis, and a robust expression of angiogenic factors (Table 1) (Guiducci et al., 2010). Therefore, MSCs are considered safe for studying SSc in humans; however, their efficacy requires further confirmation.
4.3 MSCs and their exosomes in SSc-related vascular injury
4.3.1 Pathogenesis of SSc-related vascular injury
Vascular injury represents an early event in SSc pathogenesis, significantly influencing the onset of pulmonary hypertension, finger-end ulcers, and Raynaud’s phenomenon (Kahaleh, 2004; Abraham and Distler, 2007; Cipriani et al., 2011; Matucci-Cerinic et al., 2013; Asano and Sato, 2015; Odonwodo et al., 2023). However, the underlying cause of chronic inflammation is the combination of autoimmunity, vascular injury, and fibrosis. Among these factors, vascular lesions and the autoimmune inflammatory response serve as the pathological foundation of the disease and are two key drivers of its progression (Abraham and Varga, 2005; Senecal et al., 2020; Shimizu et al., 2022).
In early SSc stages, vascular remodeling occurs, characterized by intimal hyperplasia, capillary dilatation, and microvascular intracellular sugar aggregation following endothelial cell injury. Subsequently, avascular zones develop due to damage to capillaries and small arteries (Koch and Distler, 2007; Hughes and Herrick, 2019). Larger arteries can become occluded and form thrombi due to endothelial proliferation and fibroproliferation, fibrin deposition, and smooth muscle cell hypertrophy (Koch and Distler, 2007; Kavian and Batteux, 2015; Hughes and Herrick, 2019). One common clinical SSc symptom, Reynold’s disease, is characterized by alternating episodes of ischemia-reperfusion and persistent arterial vasospasm, with elevated junctional adhesion molecule (JAM) expression (Koch and Distler, 2007). In a recent study, we discovered that JAMs were substantially upregulated in the vascular endothelium, leading to increased binding of macrophages and platelets to the endothelium. Endothelial cell damage is caused by increased superoxide anion generation by neutrophils and platelets (Hughes and Herrick, 2019). Therefore, in the early stages of SSc, endothelial cell destruction might be accompanied by impaired neurological function, resulting in vascular damage (Kahaleh, 2004; Abraham and Distler, 2007; Hughes and Herrick, 2019). Thus, while the precise pathophysiology of endothelial cell damage remains unknown, autoimmunity appears to be connected with the production of cytokines and adhesion molecules by epithelial cells, eventually culminating in endothelial cell apoptosis (Lunardi et al., 2000; Ahmed et al., 2006; Mulligan-Kehoe and Simons, 2008; Hughes and Herrick, 2019).
4.3.2 Mechanism and efficacy of MSCs and their exosomes in treating SSc-related vascular injury
Recent research has revealed that local or systemic autologous stem cell transplantation in SSc can be used to efficiently treat peripheral vascular lesions such as finger ulcers and limb necrosis (Nevskaya et al., 2009; Ishigatsubo et al., 2010; Yun, 2011). For example, in SSc patients with acute limb gangrene, the peripheral vascular network can be regenerated through intravenous injection, culture, and amplification of autologous bone marrow mesenchymal stem cells, consistent with findings from previous studies (Yun, 2011). Guiducci S. et al. Discovered that MSCs can secrete pro-angiogenic factors such as TGF-β1, which promotes vascular regeneration and allows pericytes, vascular smooth muscle cells, and other cells to play a significant role in the vascular regeneration process. Concurrently, MSCs drive their differentiation into pericytes and smooth muscle cells during neovascularization, thereby enhancing neovascularization stabilization. However, whether SSc-MSCs can be guided effectively to develop into epithelial cells within the vessel wall remains to be seen. Komaki et al. used a mouse ear ischemia injury model to investigate the pro-neovascularization effect of human-term placental tissue-derived MSC exosomes (PlaMSC-exos). They discovered that the pro-neovascularization effect was mediated by exosomes released from placental tissue-derived MSCs. Therefore, PlaMSC-exo is being considered as a potential treatment strategy (Komaki et al., 2017).
4.4 Research progress on MSCs and their exosomes in other organ involvement associated with SSc
4.4.1 Pathogenesis of gastrointestinal involvement in systemic sclerosis
Gastrointestinal (GI) tract involvement is prevalent in 90% of patients with SSc, marked by lesions spanning from the oral cavity to the anus, causing symptoms such as GI dysmotility, esophagitis, gastritis, gastroesophageal reflux, and mucosal ulcers. In severe cases, this can lead to challenges in swallowing and nutrient malabsorption. It significantly affects the quality of life of patients with SSc (Gyger and Baron, 2012). Therefore, comprehending the underlying mechanism of these diseases is essential for developing an appropriate treatment program for GI diseases. Two main theories exist regarding the GI tract lesion mechanism (Emmanuel, 2016). The first is related to autonomic nerve axonal damage (i.e., GI motility dysfunction caused by sympathetic overactivity). The second is associated with vascular and autoimmune phenomena (i.e., progressive fibrotic changes in the GI tract due to impaired collagen and other extracellular matrix component deposition). However, regardless of the explanation, GI involvement results from a chain of responses rather than a single component.
4.4.2 Pathogenesis of renal involvement in systemic sclerosis
Scleroderma renal crisis (SRC), a primary cause of death in diffuse scleroderma, is frequently associated with SSc. SRC is estimated to affect 5%–10% of patients with systemic sclerosis (Woodworth et al., 2016; Hudson et al., 2021). Most patients experience malignant hypertension and increased renal impairment. SRC is linked to the renin-angiotensin-aldosterone system activation, upregulation of endothelin-B receptors, and activation of anti-endothelial cell antibodies. These factors cause renal vasoconstriction and ischemia (Scheen et al., 2023).
4.4.3 Pathogenesis of cardiac involvement in systemic sclerosis
In diffuse cutaneous SSc, the heart is among the initial organs to be affected in patients with SSc. The most prevalent symptoms include myocardial fibrosis, coronary artery disease, pericarditis, and heart failure. Heart disease accounts for 27.2% of SSc-related fatalities (Wangkaew et al., 2017). Therefore, early detection is crucial. Furthermore, since the heart of patients with SSc may exhibit transitory vasospasm and functional changes in the early stages of the disease, distinguishing symptoms are usually absent in the early stage. The fibrotic changes in the coronary arteries result in permanent damage to heart function resulting in systemic blood circulation anomalies (Kahan et al., 1985).
4.4.4 Pathogenesis of musculoskeletal involvement in systemic sclerosis
Musculoskeletal involvement is the leading cause of reduced mobility and disability in patients with SSc. Arthralgia is the most common symptom, and poor prognosis is linked to tendon friction. Recent research has revealed a link between elevated blood YKL-40 levels and chondrocyte and/or fibroblast activity in patients with SSc (Lorand et al., 2014).
4.4.5 Mesenchymal stem cells and their exosomes for various organs related to systemic sclerosis
Inflammation and the autoimmune response play a pivotal role in organ damage in patients with SSc. MSCs and their exosomes reduce inflammation and fibrosis in the heart, kidney, digestive tract, and musculoskeletal system by modulating the immune system and reducing the release of inflammatory mediators and immune cell activation. Simultaneously, a sufficient amount of pro-angiogenic molecules, such as vascular endothelial growth factor, and basic fibroblast growth factor is released. These molecules stimulate angiogenesis, repair, and enhance blood circulation and oxygenation. Furthermore, MSCs and their exosomes can prevent the production of fibrosis-related cytokines and collagen, thereby lowering the extent of organ fibrosis. Finally, their high regenerative potential can facilitate tissue healing and functional recovery in damaged areas.
5 Brief summary and outlook
In summary, MSCs and exosomes have been proven to improve lung function, reduce cutaneous fibrosis, promote neovascularization, and mitigate fibrosis in the heart, kidneys, digestive tract, and musculoskeletal system to varying degrees. However, it is undeniable that the research and utilization of MSCs and their exosomes have certain limitations due to their early stage. The limitations are as follows: the aging and functional degradation of MSCs, distribution and local differentiation in vivo, and the potential tumorigenic risk, which have hindered the development of MSCs in clinical research and application to a certain extent, whereas exosomes, due to their low extracellular yield, high demand, and limited therapeutic capacity, have prompted researchers to urgently search for methods that can improve the therapeutic effects. Furthermore, to optimize the therapeutic efficacy of MSCs and exosomes, it is essential to conduct comprehensive research on dosage, frequency, administration methods, timing (early or late stage of the disease), and donor status (age and health). Additionally, MSCs hold potential for diverse applications in various sectors, such as their chemical modification for use as drug carriers for tracer effects. In conclusion, while MSCs and their exosomes have favorable and extensive benefits in treating SSc, further clinical trials are required to address some potential concerns.
Author contributions
YZ: Writing–original draft. YY: Writing–original draft, Supervision, Writing–review and editing. XG: Writing–review and editing. WG: Writing–review and editing. LZ: Writing–original draft, Writing–review and editing, Supervision.
Funding
The author(s) declare financial support was received for the research, authorship, and/or publication of this article. This work is supported by Natural Science Foundation of Shanxi Province (grant number 202203021211074). The funding body played no role in the design of the study and collection, analysis, and interpretation of data and in writing the manuscript.
Conflict of interest
The authors declare that the research was conducted in the absence of any commercial or financial relationships that could be construed as a potential conflict of interest.
Publisher’s note
All claims expressed in this article are solely those of the authors and do not necessarily represent those of their affiliated organizations, or those of the publisher, the editors and the reviewers. Any product that may be evaluated in this article, or claim that may be made by its manufacturer, is not guaranteed or endorsed by the publisher.
References
Abraham, D., and Distler, O. (2007). How does endothelial cell injury start? The role of endothelin in systemic sclerosis. Arthritis Res. Ther. 9 (Suppl. 2), S2. doi:10.1186/ar2186
Abraham, D. J., and Varga, J. (2005). Scleroderma: from cell and molecular mechanisms to disease models. Trends Immunol. 26 (11), 587–595. doi:10.1016/j.it.2005.09.004
Ahmed, S. S., Tan, F. K., Arnett, F. C., Jin, L., and Geng, Y. J. (2006). Induction of apoptosis and fibrillin 1 expression in human dermal endothelial cells by scleroderma sera containing anti-endothelial cell antibodies. Arthritis Rheum. 54 (7), 2250–2262. doi:10.1002/art.21952
Alexander, T., and Greco, R. (2022). Hematopoietic stem cell transplantation and cellular therapies for autoimmune diseases: overview and future considerations from the autoimmune diseases working party (ADWP) of the European society for blood and marrow transplantation (EBMT). Bone Marrow Transpl. 57 (7), 1055–1062. doi:10.1038/s41409-022-01702-w
Allanore, Y., Wung, P., Soubrane, C., Esperet, C., Marrache, F., Bejuit, R., et al. (2020). A randomised, double-blind, placebo-controlled, 24-week, phase II, proof-of-concept study of romilkimab (SAR156597) in early diffuse cutaneous systemic sclerosis. Ann. Rheum. Dis. 79 (12), 1600–1607. doi:10.1136/annrheumdis-2020-218447
Arutyunyan, I., Elchaninov, A., Makarov, A., and Fatkhudinov, T. (2016). Umbilical cord as prospective source for mesenchymal stem cell-based therapy. Stem Cells Int. 2016, 6901286. doi:10.1155/2016/6901286
Asano, Y., and Sato, S. (2015). Vasculopathy in scleroderma. Semin. Immunopathol. 37 (5), 489–500. doi:10.1007/s00281-015-0505-5
Berlow, N. E., Svalina, M. N., Quist, M. J., Settelmeyer, T. P., Zherebitskiy, V., Kogiso, M., et al. (2018). IL-13 receptors as possible therapeutic targets in diffuse intrinsic pontine glioma. PLoS One 13 (4), e0193565. doi:10.1371/journal.pone.0193565
Breitfeld, D., Ohl, L., Kremmer, E., Ellwart, J., Sallusto, F., Lipp, M., et al. (2000). Follicular B helper T cells express CXC chemokine receptor 5, localize to B cell follicles, and support immunoglobulin production. J. Exp. Med. 192 (11), 1545–1552. doi:10.1084/jem.192.11.1545
Brembilla, N. C., Montanari, E., Truchetet, M. E., Raschi, E., Meroni, P., and Chizzolini, C. (2013). Th17 cells favor inflammatory responses while inhibiting type I collagen deposition by dermal fibroblasts: differential effects in healthy and systemic sclerosis fibroblasts. Arthritis Res. Ther. 15 (5), R151. doi:10.1186/ar4334
Cahill, E. F., Kennelly, H., Carty, F., Mahon, B. P., and English, K. (2016). Hepatocyte growth factor is required for mesenchymal stromal cell protection against bleomycin-induced pulmonary fibrosis. Stem Cells Transl. Med. 5 (10), 1307–1318. doi:10.5966/sctm.2015-0337
Caplan, A. I. (2017). Mesenchymal stem cells: time to change the name. Stem Cells Transl. Med. 6 (6), 1445–1451. doi:10.1002/sctm.17-0051
Christmann, R. B., and Lafyatis, R. (2010). The cytokine language of monocytes and macrophages in systemic sclerosis. Arthritis Res. Ther. 12 (5), 146. doi:10.1186/ar3167
Chu, K. A., Wang, S. Y., Yeh, C. C., Fu, T. W., Fu, Y. Y., Ko, T. L., et al. (2019). Reversal of bleomycin-induced rat pulmonary fibrosis by a xenograft of human umbilical mesenchymal stem cells from Wharton's jelly. Theranostics 9 (22), 6646–6664. doi:10.7150/thno.33741
Cipriani, P., Marrelli, A., Liakouli, V., Di Benedetto, P., and Giacomelli, R. (2011). Cellular players in angiogenesis during the course of systemic sclerosis. Autoimmun. Rev. 10 (10), 641–646. doi:10.1016/j.autrev.2011.04.016
Cras, A., Farge, D., Carmoi, T., Lataillade, J. J., Wang, D. D., and Sun, L. (2015). Update on mesenchymal stem cell-based therapy in lupus and scleroderma. Arthritis Res. Ther. 17, 301. doi:10.1186/s13075-015-0819-7
de Castro, L. L., Lopes-Pacheco, M., Weiss, D. J., Cruz, F. F., and Rocco, P. (2019). Current understanding of the immunosuppressive properties of mesenchymal stromal cells. J. Mol. Med. Berl. 97 (5), 605–618. doi:10.1007/s00109-019-01776-y
Denton, C. P., and Black, C. M. (2004). Scleroderma-clinical and pathological advances. Best. Pract. Res. Clin. Rheumatol. 18 (3), 271–290. doi:10.1016/j.berh.2004.03.001
Ding, D. C., Shyu, W. C., and Lin, S. Z. (2011). Mesenchymal stem cells. Cell Transpl. 20 (1), 5–14. doi:10.3727/096368910X
Dominici, M., Le Blanc, K., Mueller, I., Slaper-Cortenbach, I., Marini, F., Krause, D., et al. (2006). Minimal criteria for defining multipotent mesenchymal stromal cells. The International Society for Cellular Therapy position statement. Cytotherapy 8 (4), 315–317. doi:10.1080/14653240600855905
Dufour, A. M., Borowczyk-Michalowska, J., Alvarez, M., Truchetet, M. E., Modarressi, A., Brembilla, N. C., et al. (2020). IL-17A dissociates inflammation from fibrogenesis in systemic sclerosis. J. Invest. Dermatol. 140 (1), 103–112. doi:10.1016/j.jid.2019.05.026
Dumoitier, N., Chaigne, B., Regent, A., Lofek, S., Mhibik, M., Dorfmuller, P., et al. (2017). Scleroderma peripheral B lymphocytes secrete interleukin-6 and transforming growth factor beta and activate fibroblasts. Arthritis Rheumatol. 69 (5), 1078–1089. doi:10.1002/art.40016
Dumoitier, N., Lofek, S., and Mouthon, L. (2014). Pathophysiology of systemic sclerosis: state of the art in 2014. Presse Med. 43 (10 Pt 2), e267–e278. doi:10.1016/j.lpm.2014.08.001
Emmanuel, A. (2016). Current management of the gastrointestinal complications of systemic sclerosis. Nat. Rev. Gastroenterol. Hepatol. 13 (8), 461–472. doi:10.1038/nrgastro.2016.99
Ferreli, C., Gasparini, G., Parodi, A., Cozzani, E., Rongioletti, F., and Atzori, L. (2017). Cutaneous manifestations of scleroderma and scleroderma-like disorders: a comprehensive review. Clin. Rev. Allergy Immunol. 53 (3), 306–336. doi:10.1007/s12016-017-8625-4
Fforestier, A., Guerrier, T., Jouvray, M., Giovannelli, J., Lefevre, G., Sobanski, V., et al. (2018). Altered B lymphocyte homeostasis and functions in systemic sclerosis. Autoimmun. Rev. 17 (3), 244–255. doi:10.1016/j.autrev.2017.10.015
Francois, A., Chatelus, E., Wachsmann, D., Sibilia, J., Bahram, S., Alsaleh, G., et al. (2013). B lymphocytes and B-cell activating factor promote collagen and profibrotic markers expression by dermal fibroblasts in systemic sclerosis. Arthritis Res. Ther. 15 (5), R168. doi:10.1186/ar4352
Friedenstein, A. J., Gorskaja, J. F., and Kulagina, N. N. (1976). Fibroblast precursors in normal and irradiated mouse hematopoietic organs. Exp. Hematol. 4 (5), 267–274.
Fukushima, K., and Akira, S. (2021). Novel insights into the pathogenesis of lung fibrosis: the RBM7-NEAT1-CXCL12-SatM axis at fibrosis onset. Int. Immunol. 33 (12), 659–663. doi:10.1093/intimm/dxab034
Ganesan, N., Chang, Y. D., Hung, S. C., Lan, J. L., Liao, J. W., Fu, S. T., et al. (2023). Mesenchymal stem cells suppressed skin and lung inflammation and fibrosis in topoisomerase I-induced systemic sclerosis associated with lung disease mouse model. Cell Tissue Res. 391 (2), 323–337. doi:10.1007/s00441-022-03716-8
Guiducci, S., Porta, F., Saccardi, R., Guidi, S., Ibba-Manneschi, L., Manetti, M., et al. (2010). Autologous mesenchymal stem cells foster revascularization of ischemic limbs in systemic sclerosis: a case report. Ann. Intern. Med. 153 (10), 650–654. doi:10.7326/0003-4819-153-10-201011160-00007
Gyger, G., and Baron, M. (2012). Gastrointestinal manifestations of scleroderma: recent progress in evaluation, pathogenesis, and management. Curr. Rheumatol. Rep. 14 (1), 22–29. doi:10.1007/s11926-011-0217-3
Harrell, C. R., Markovic, B. S., Fellabaum, C., Arsenijevic, N., Djonov, V., and Volarevic, V. (2020). The role of Interleukin 1 receptor antagonist in mesenchymal stem cell-based tissue repair and regeneration. Biofactors 46 (2), 263–275. doi:10.1002/biof.1587
He, F., Wang, Y., Li, Y., and Yu, L. (2020). Human amniotic mesenchymal stem cells alleviate paraquat-induced pulmonary fibrosis in rats by inhibiting the inflammatory response. Life Sci. 243, 117290. doi:10.1016/j.lfs.2020.117290
Huang, E., Peng, N., Xiao, F., Hu, D., Wang, X., and Lu, L. (2020). The roles of immune cells in the pathogenesis of fibrosis. Int. J. Mol. Sci. 21 (15), 5203. doi:10.3390/ijms21155203
Hudson, M., Ghossein, C., and Steen, V. (2021). Scleroderma renal crisis. Presse Med. 50 (1), 104063. doi:10.1016/j.lpm.2021.104063
Hughes, M., and Herrick, A. L. (2019). Systemic sclerosis. Br. J. Hosp. Med. (Lond). 80 (9), 530–536. doi:10.12968/hmed.2019.80.9.530
Ishigatsubo, Y., Ihata, A., Kobayashi, H., Hama, M., Kirino, Y., Ueda, A., et al. (2010). Therapeutic angiogenesis in patients with systemic sclerosis by autologous transplantation of bone-marrow-derived cells. Mod. Rheumatol. 20 (3), 263–272. doi:10.1007/s10165-010-0274-x
Kahaleh, M. B. (2004). Vascular involvement in systemic sclerosis (SSc). Clin. Exp. Rheumatol. 22 (3 33), S19–S23.
Kahan, A., Nitenberg, A., Foult, J. M., Amor, B., Menkes, C. J., Devaux, J. Y., et al. (1985). Decreased coronary reserve in primary scleroderma myocardial disease. Arthritis Rheum. 28 (6), 637–646. doi:10.1002/art.1780280607
Kavian, N., and Batteux, F. (2015). Macro- and microvascular disease in systemic sclerosis. Vasc. Pharmacol. 71, 16–23. doi:10.1016/j.vph.2015.05.015
Kawano, Y., and Chung, L. (2021). Promise and challenge of systemic sclerosis therapies. Nat. Rev. Rheumatol. 17 (10), 581–582. doi:10.1038/s41584-021-00678-z
Kim, M. N., Hong, J. Y., Kim, E. G., Lee, J. W., Lee, S. Y., Kim, K. W., et al. (2022). A novel regulatory role of activated leukocyte cell-adhesion molecule in the pathogenesis of pulmonary fibrosis. Am. J. Respir. Cell Mol. Biol. 66 (4), 415–427. doi:10.1165/rcmb.2020-0581OC
Koch, A. E., and Distler, O. (2007). Vasculopathy and disordered angiogenesis in selected rheumatic diseases: rheumatoid arthritis and systemic sclerosis. Arthritis Res. Ther. 9 (Suppl. 2), S3. doi:10.1186/ar2187
Komaki, M., Numata, Y., Morioka, C., Honda, I., Tooi, M., Yokoyama, N., et al. (2017). Exosomes of human placenta-derived mesenchymal stem cells stimulate angiogenesis. Stem Cell Res. Ther. 8 (1), 219. doi:10.1186/s13287-017-0660-9
Lei, L., He, Z. Y., Zhao, C., Sun, X. J., and Zhong, X. N. (2016). Elevated frequencies of CD4(+) IL-21(+) T, CD4(+) IL-21R(+) T and IL-21(+) Th17 cells, and increased levels of IL-21 in bleomycin-induced mice may be associated with dermal and pulmonary inflammation and fibrosis. Int. J. Rheum. Dis. 19 (4), 392–404. doi:10.1111/1756-185X.12522
Li, S. C. (2018). Scleroderma in children and adolescents: localized scleroderma and systemic sclerosis. Pediatr. Clin. North Am. 65 (4), 757–781. doi:10.1016/j.pcl.2018.04.002
Li, X., Wang, Y., An, G., Liang, D., Zhu, Z., Lian, X., et al. (2017). Bone marrow mesenchymal stem cells attenuate silica-induced pulmonary fibrosis via paracrine mechanisms. Toxicol. Lett. 270, 96–107. doi:10.1016/j.toxlet.2017.02.016
Li, Y., Gao, Q., Xu, K., Peng, X., Yuan, X., Jiang, W., et al. (2018). Interleukin-37 attenuates bleomycin-induced pulmonary inflammation and fibrosis in mice. Inflammation 41 (5), 1772–1779. doi:10.1007/s10753-018-0820-9
Liu, M., Yang, J., Xing, X., Cui, X., and Li, M. (2014). Interleukin-17A promotes functional activation of systemic sclerosis patient-derived dermal vascular smooth muscle cells by extracellular-regulated protein kinases signalling pathway. Arthritis Res. Ther. 16 (6), 4223. doi:10.1186/s13075-014-0512-2
Liu, T., Li, S., Ying, S., Tang, S., Ding, Y., Li, Y., et al. (2020). The IL-23/IL-17 pathway in inflammatory skin diseases: from bench to bedside. Front. Immunol. 11, 594735. doi:10.3389/fimmu.2020.594735
Lorand, V., Czirjak, L., and Minier, T. (2014). Musculoskeletal involvement in systemic sclerosis. Presse Med. 43 (10 Pt 2), e315–e328. doi:10.1016/j.lpm.2014.03.027
Lunardi, C., Bason, C., Navone, R., Millo, E., Damonte, G., Corrocher, R., et al. (2000). Systemic sclerosis immunoglobulin G autoantibodies bind the human cytomegalovirus late protein UL94 and induce apoptosis in human endothelial cells. Nat. Med. 6 (10), 1183–1186. doi:10.1038/80533
Luo, X. Y., Meng, X. J., Cao, D. C., Wang, W., Zhou, K., Li, L., et al. (2019). Transplantation of bone marrow mesenchymal stromal cells attenuates liver fibrosis in mice by regulating macrophage subtypes. Stem Cell Res. Ther. 10 (1), 16. doi:10.1186/s13287-018-1122-8
Ly, N., Ueda-Hayakawa, I., Nguyen, C., Huynh, T., Kishimoto, I., Fujimoto, M., et al. (2021). Imbalance toward TFH 1 cells playing a role in aberrant B cell differentiation in systemic sclerosis. Rheumatol. Oxf. 60 (3), 1553–1562. doi:10.1093/rheumatology/keaa669
Maehara, T., Kaneko, N., Perugino, C. A., Mattoo, H., Kers, J., Allard-Chamard, H., et al. (2020). Cytotoxic CD4+ T lymphocytes may induce endothelial cell apoptosis in systemic sclerosis. J. Clin. Invest. 130 (5), 2451–2464. doi:10.1172/JCI131700
Magalon, J., Velier, M., Simoncini, S., Francois, P., Bertrand, B., Daumas, A., et al. (2019). Molecular profile and proangiogenic activity of the adipose-derived stromal vascular fraction used as an autologous innovative medicinal product in patients with systemic sclerosis. Ann. Rheum. Dis. 78 (3), 391–398. doi:10.1136/annrheumdis-2018-214218
Mansouri, N., Willis, G. R., Fernandez-Gonzalez, A., Reis, M., Nassiri, S., Mitsialis, S. A., et al. (2019). Mesenchymal stromal cell exosomes prevent and revert experimental pulmonary fibrosis through modulation of monocyte phenotypes. JCI Insight 4 (21), e128060. doi:10.1172/jci.insight.128060
Matucci-Cerinic, M., Kahaleh, B., and Wigley, F. M. (2013). Review: evidence that systemic sclerosis is a vascular disease. Arthritis Rheum. 65 (8), 1953–1962. doi:10.1002/art.37988
Moghadasi, S., Elveny, M., Rahman, H. S., Suksatan, W., Jalil, A. T., Abdelbasset, W. K., et al. (2021). A paradigm shift in cell-free approach: the emerging role of MSCs-derived exosomes in regenerative medicine. J. Transl. Med. 19 (1), 302. doi:10.1186/s12967-021-02980-6
Morrison, T. J., Jackson, M. V., Cunningham, E. K., Kissenpfennig, A., Mcauley, D. F., O'Kane, C. M., et al. (2017). Mesenchymal stromal cells modulate macrophages in clinically relevant lung injury models by extracellular vesicle mitochondrial transfer. Am. J. Respir. Crit. Care Med. 196 (10), 1275–1286. doi:10.1164/rccm.201701-0170OC
Mulligan-Kehoe, M. J., and Simons, M. (2008). Vascular disease in scleroderma: angiogenesis and vascular repair. Rheum. Dis. Clin. North Am. 34 (1), 73–79. vi. doi:10.1016/j.rdc.2007.12.006
Nevskaya, T., Ananieva, L., Bykovskaia, S., Eremin, I., Karandashov, E., Khrennikov, J., et al. (2009). Autologous progenitor cell implantation as a novel therapeutic intervention for ischaemic digits in systemic sclerosis. Rheumatol. Oxf. 48 (1), 61–64. doi:10.1093/rheumatology/ken407
Ni, K., Liu, M., Zheng, J., Wen, L., Chen, Q., Xiang, Z., et al. (2018). PD-1/PD-L1 pathway mediates the alleviation of pulmonary fibrosis by human mesenchymal stem cells in humanized mice. Am. J. Respir. Cell Mol. Biol. 58 (6), 684–695. doi:10.1165/rcmb.2017-0326OC
Paliwal, S., Chaudhuri, R., Agrawal, A., and Mohanty, S. (2018). Regenerative abilities of mesenchymal stem cells through mitochondrial transfer. J. Biomed. Sci. 25 (1), 31. doi:10.1186/s12929-018-0429-1
Periera-Simon, S., Xia, X., Catanuto, P., Coronado, R., Kurtzberg, J., Bellio, M., et al. (2021). Anti-fibrotic effects of different sources of MSC in bleomycin-induced lung fibrosis in C57BL6 male mice. Respirology 26 (2), 161–170. doi:10.1111/resp.13928
Pittenger, M. F., Mackay, A. M., Beck, S. C., Jaiswal, R. K., Douglas, R., Mosca, J. D., et al. (1999). Multilineage potential of adult human mesenchymal stem cells. Science 284 (5411), 143–147. doi:10.1126/science.284.5411.143
Postlethwaite, A. E., Holness, M. A., Katai, H., and Raghow, R. (1992). Human fibroblasts synthesize elevated levels of extracellular matrix proteins in response to interleukin 4. J. Clin. Invest. 90 (4), 1479–1485. doi:10.1172/JCI116015
Rad, F., Ghorbani, M., Mohammadi, R. A., and Habibi, R. M. (2019). Mesenchymal stem cell-based therapy for autoimmune diseases: emerging roles of extracellular vesicles. Mol. Biol. Rep. 46 (1), 1533–1549. doi:10.1007/s11033-019-04588-y
Reinders, M. E., Fibbe, W. E., and Rabelink, T. J. (2010). Multipotent mesenchymal stromal cell therapy in renal disease and kidney transplantation. Nephrol. Dial. Transpl. 25 (1), 17–24. doi:10.1093/ndt/gfp552
Ricard, L., Jachiet, V., Malard, F., Ye, Y., Stocker, N., Riviere, S., et al. (2019). Circulating follicular helper T cells are increased in systemic sclerosis and promote plasmablast differentiation through the IL-21 pathway which can be inhibited by ruxolitinib. Ann. Rheum. Dis. 78 (4), 539–550. doi:10.1136/annrheumdis-2018-214382
Rubio-Rivas, M., Royo, C., Simeon, C. P., Corbella, X., and Fonollosa, V. (2014). Mortality and survival in systemic sclerosis: systematic review and meta-analysis. Semin. Arthritis Rheum. 44 (2), 208–219. doi:10.1016/j.semarthrit.2014.05.010
Sabapathy, V., Sundaram, B., Mankuzhy, P., and Kumar, S. (2014). Human Wharton's Jelly Mesenchymal Stem Cells plasticity augments scar-free skin wound healing with hair growth. PLoS One 9 (4), e93726. doi:10.1371/journal.pone.0093726
Schaerli, P., Willimann, K., Lang, A. B., Lipp, M., Loetscher, P., and Moser, B. (2000). CXC chemokine receptor 5 expression defines follicular homing T cells with B cell helper function. J. Exp. Med. 192 (11), 1553–1562. doi:10.1084/jem.192.11.1553
Scheen, M., Dominati, A., Olivier, V., Nasr, S., De Seigneux, S., Mekinian, A., et al. (2023). Renal involvement in systemic sclerosis. Autoimmun. Rev. 22 (6), 103330. doi:10.1016/j.autrev.2023.103330
Senecal, J. L., Hoa, S., Yang, R., and Koenig, M. (2020). Pathogenic roles of autoantibodies in systemic sclerosis: current understandings in pathogenesis. J. Scleroderma Relat. Disord. 5 (2), 103–129. doi:10.1177/2397198319870667
Servettaz, A., Agard, C., Tamby, M. C., Guilpain, P., Guillevin, L., and Mouthon, L. (2006). Systemic sclerosis: pathophysiology of a multifaceted disease. Presse Med. 35 (12 Pt 2), 1903–1915. doi:10.1016/s0755-4982(06)74924-7
Shi, Y., Su, J., Roberts, A. I., Shou, P., Rabson, A. B., and Ren, G. (2012). How mesenchymal stem cells interact with tissue immune responses. Trends Immunol. 33 (3), 136–143. doi:10.1016/j.it.2011.11.004
Shimizu, M., Takei, S., Mori, M., and Yachie, A. (2022). Pathogenic roles and diagnostic utility of interleukin-18 in autoinflammatory diseases. Front. Immunol. 13, 951535. doi:10.3389/fimmu.2022.951535
Shirjang, S., Mansoori, B., Solali, S., Hagh, M. F., and Shamsasenjan, K. (2017). Toll-like receptors as a key regulator of mesenchymal stem cell function: an up-to-date review. Cell. Immunol. 315, 1–10. doi:10.1016/j.cellimm.2016.12.005
Soundara, R. T., Giacoppo, S., Diomede, F., Bramanti, P., Trubiani, O., and Mazzon, E. (2017). Human periodontal ligament stem cells secretome from multiple sclerosis patients suppresses NALP3 inflammasome activation in experimental autoimmune encephalomyelitis. Int. J. Immunopathol. Pharmacol. 30 (3), 238–252. doi:10.1177/0394632017722332
Tamby, M. C., Chanseaud, Y., Guillevin, L., and Mouthon, L. (2003). New insights into the pathogenesis of systemic sclerosis. Autoimmun. Rev. 2 (3), 152–157. doi:10.1016/s1568-9972(03)00004-1
Taylor, D. K., Mittereder, N., Kuta, E., Delaney, T., Burwell, T., Dacosta, K., et al. (2018). T follicular helper-like cells contribute to skin fibrosis. Sci. Transl. Med. 10 (431), eaaf5307. doi:10.1126/scitranslmed.aaf5307
Truchetet, M. E., Brembilla, N. C., Montanari, E., Lonati, P., Raschi, E., Zeni, S., et al. (2013). Interleukin-17A+ cell counts are increased in systemic sclerosis skin and their number is inversely correlated with the extent of skin involvement. Arthritis Rheum. 65 (5), 1347–1356. doi:10.1002/art.37860
Tseng, P. Y., and Hoon, M. A. (2021). Oncostatin M can sensitize sensory neurons in inflammatory pruritus. Sci. Transl. Med. 13 (619), eabe3037. doi:10.1126/scitranslmed.abe3037
Turse, E. P., Dailey, F. E., Naseer, M., Partyka, E. K., Bragg, J. D., and Tahan, V. (2018). Stem cells for luminal, fistulizing, and perianal inflammatory bowel disease: a comprehensive updated review of the literature. Stem Cells Cloning 11, 95–113. doi:10.2147/SCCAA.S135414
van Rhijn-Brouwer, F. C., Gremmels, H., Fledderus, J. O., Radstake, T. R., Verhaar, M. C., and van Laar, J. M. (2016). Cellular therapies in systemic sclerosis: recent progress. Curr. Rheumatol. Rep. 18 (2), 12. doi:10.1007/s11926-015-0555-7
Vettori, S., Barra, G., Russo, B., Borgia, A., Pasquale, G., Pellecchia, L., et al. (2020). T-cell proapoptotic and antifibrotic activity against autologous skin fibroblasts in vitro is associated with IL-17a Axis upregulation in systemic sclerosis. Front. Immunol. 11, 220. doi:10.3389/fimmu.2020.00220
Wangkaew, S., Prasertwitayakij, N., Phrommintikul, A., Puntana, S., and Euathrongchit, J. (2017). Causes of death, survival and risk factors of mortality in Thai patients with early systemic sclerosis: inception cohort study. Rheumatol. Int. 37 (12), 2087–2094. doi:10.1007/s00296-017-3846-7
Willis, G. R., Fernandez-Gonzalez, A., Anastas, J., Vitali, S. H., Liu, X., Ericsson, M., et al. (2018). Mesenchymal stromal cell exosomes ameliorate experimental bronchopulmonary dysplasia and restore lung function through macrophage immunomodulation. Am. J. Respir. Crit. Care Med. 197 (1), 104–116. doi:10.1164/rccm.201705-0925OC
Woodworth, T. G., Suliman, Y. A., Li, W., Furst, D. E., and Clements, P. (2016). Scleroderma renal crisis and renal involvement in systemic sclerosis. Nat. Rev. Nephrol. 12 (11), 678–691. doi:10.1038/nrneph.2016.124
Xing, X., Li, A., Tan, H., and Zhou, Y. (2020). IFN-γ+ IL-17+ Th17 cells regulate fibrosis through secreting IL-21 in systemic scleroderma. J. Cell. Mol. Med. 24 (23), 13600–13608. doi:10.1111/jcmm.15266
Xing, X., Yang, J., Yang, X., Wei, Y., Zhu, L., Gao, D., et al. (2013). IL-17A induces endothelial inflammation in systemic sclerosis via the ERK signaling pathway. PLoS One 8 (12), e85032. doi:10.1371/journal.pone.0085032
Xu, L., Ding, L., Wang, L., Cao, Y., Zhu, H., Lu, J., et al. (2017). Umbilical cord-derived mesenchymal stem cells on scaffolds facilitate collagen degradation via upregulation of MMP-9 in rat uterine scars. Stem Cell Res. Ther. 8 (1), 84. doi:10.1186/s13287-017-0535-0
Yang, J., Hu, H., Zhang, S., Jiang, L., Cheng, Y., Xie, H., et al. (2020a). Human umbilical cord mesenchymal stem cell-derived exosomes alleviate pulmonary fibrosis in mice by inhibiting epithelial-mesenchymal transition. Nan Fang. Yi Ke Da Xue Xue Bao 40 (7), 988–994. doi:10.12122/j.issn.1673-4254.2020.07.11
Yang, S., Liu, P., Jiang, Y., Wang, Z., Dai, H., and Wang, C. (2021). Therapeutic applications of mesenchymal stem cells in idiopathic pulmonary fibrosis. Front. Cell Dev. Biol. 9, 639657. doi:10.3389/fcell.2021.639657
Yang, Y., Zhu, S., Li, Y., Lu, Q., Zhang, Q., Su, L., et al. (2020b). Human umbilical cord mesenchymal stem cells ameliorate skin fibrosis development in a mouse model of bleomycin-induced systemic sclerosis. Exp. Ther. Med. 20 (6), 257. doi:10.3892/etm.2020.9387
Yu, Y., Shen, L., Xie, X., Zhao, J., and Jiang, M. (2022). The therapeutic effects of exosomes derived from human umbilical cord mesenchymal stem cells on scleroderma. Tissue Eng. Regen. Med. 19 (1), 141–150. doi:10.1007/s13770-021-00405-5
Yun, J. H. (2011). Autologous mesenchymal stem cells foster revascularization of ischemic limbs in systemic sclerosis. Ann. Intern. Med. 155 (1), 65–66. doi:10.7326/0003-4819-155-1-201107050-00013
Zhang, H., Liang, J., Tang, X., Wang, D., Feng, X., Wang, F., et al. (2017). Sustained benefit from combined plasmapheresis and allogeneic mesenchymal stem cells transplantation therapy in systemic sclerosis. Arthritis Res. Ther. 19 (1), 165. doi:10.1186/s13075-017-1373-2
Glossary
Keywords: mesenchymal stem cells, exosomes, systemic sclerosis, pulmonary interstitial fibrosis, skin fibrosis, vascular injury, other organ involvement
Citation: Zhang Y, Yang Y, Gao X, Gao W and Zhang L (2023) Research progress on mesenchymal stem cells and their exosomes in systemic sclerosis. Front. Pharmacol. 14:1263839. doi: 10.3389/fphar.2023.1263839
Received: 20 July 2023; Accepted: 15 August 2023;
Published: 25 August 2023.
Edited by:
Chao He, University of Alabama at Birmingham, United StatesReviewed by:
Qianjiang Hu, University of Pittsburgh, United StatesJyotsana Pandey, University of Alabama at Birmingham, United States
Copyright © 2023 Zhang, Yang, Gao, Gao and Zhang. This is an open-access article distributed under the terms of the Creative Commons Attribution License (CC BY). The use, distribution or reproduction in other forums is permitted, provided the original author(s) and the copyright owner(s) are credited and that the original publication in this journal is cited, in accordance with accepted academic practice. No use, distribution or reproduction is permitted which does not comply with these terms.
*Correspondence: Liyun Zhang, MTMxNTcxMDIyM0BxcS5jb20=
†These authors have contributed equally to this work