- 1Department of Pharmacy, the First Affiliated Hospital of Army Medical University (Third Military Medical University), Chongqing, China
- 2Chongqing Public Health Medical Center, Southwest University Public Health Hospital, Chongqing, China
- 3Department of Infectious Diseases, the First Affiliated Hospital of Army Medical University (Third Military Medical University), Chongqing, China
Background: The plasma concentrations of the four most commonly used first-line anti-tuberculosis (TB) drugs, isoniazid (INH), rifampicin (RMP), ethambutol (EMB), and pyrazinamide (PZA), are often not within the therapeutic range. Insufficient drug exposure could lead to drug resistance and treatment failure, while excessive drug levels may lead to adverse reactions. The purpose of this study was to identify the physiological parameters influencing anti-TB drug concentrations.
Methods: A retrospective cohort study was conducted. The 2-h plasma concentrations of the four drugs were measured by using the high-performance liquid chromatography-tandem mass spectrometry method.
Results: A total of 317 patients were included in the study. The proportions of patients with INH, RMP, EMB, and PZA concentrations within the therapeutic range were 24.3%, 31.5%, 27.8%, and 18.6%, respectively. There were positive associations between the concentrations of INH and PZA and RMP and EMB, but negative associations were observed between the concentrations of INH and RMP, INH and EMB, RMP and PZA, and EMB and PZA. In the multivariate analysis, the influencing factors of the INH concentration were the PZA concentration, total bile acid (TBA), serum potassium, dose, direct bilirubin, prealbumin (PA), and albumin; those of the RMP concentration were PZA and EMB concentrations, weight, α-l-fucosidase (AFU), drinking, and dose; those of the EMB concentration were the RMP and PZA concentrations, creatinine, TBA and indirect bilirubin; and those of the PZA concentration were INH, RMP and EMB concentrations, sex, weight, uric acid and drinking.
Conclusion: The complex correlations between the concentrations of the four first-line anti-TB drugs lead to a major challenge in dose adjustment to maintain all drugs within the therapeutic window. Levels of TBA, PA, AFU, and serum potassium should also be considered when adjusting the dose of the four drugs.
Introduction
`Tuberculosis (TB) is a major global public health problem, and China is one of the countries with a heavy burden of TB (Chakaya et al., 2021). Drug therapy is an important method for tuberculosis prevention and control, and the combined application of multiple drugs is characteristic of anti-TB therapy, which has a long-term therapeutic course and is associated with many adverse reactions. Currently, the recommended dose of anti-TB drugs is based on the body mass of patients. Due to individual differences in pharmacokinetics, drug concentrations vary widely among patients (Kumar et al., 2018). Insufficient drug exposure could lead to drug resistance and treatment failure (Weiner et al., 2003; Dheda et al., 2018), while excessive drug levels may lead to adverse reactions (Martson et al., 2021). Therefore, therapeutic drug monitoring (TDM) is recommended for anti-TB drugs (Alsultan and Peloquin, 2014). Isoniazid (INH), rifampicin (RMP), ethambutol (EMB), and pyrazinamide (PZA) are the most commonly used first-line anti-TB drugs in clinical practice (Furin et al., 2019). Previous studies showed that low blood concentrations of INH and RMP were present in more than 70% of patients treated with these drugs (Park et al., 2016; Stott et al., 2018). The month 2 culture conversion TB patients had lower concentrations of INH, EMB, and PZA than the month 1 TB patients (Lei et al., 2019). Therefore, it is urgent to investigate the factors influencing the plasma concentrations of first-line anti-TB drugs (Prahl et al., 2014; Park et al., 2016) to optimize individualized dosage regimens, improve drug efficacy, and reduce related adverse reactions.
Evidence from a previous study suggested that INH, RMP, EMB, and PZA all reach peak serum concentrations approximately 2 h after administration (Alsultan and Peloquin, 2014). In Chinese patients with TB, the recommended therapeutic windows of the four drugs are defined as follows: INH, 3–6 μg/ml; RMP, 8–24 μg/ml; PZA, 20–60 μg/ml; and EMB, 2–6 μg/ml (Lu and Zhu, 2021). Therefore, investigating the influencing factors of the serum concentration of the four drugs at 2 h after administration may provide a reference for the rational use of the drugs (Sharma et al., 2018; Zhao et al., 2022). Patients’ baseline characteristics, drug administration route and dose, blood uric acid (UA) level, serum albumin level, serum creatinine level, total bilirubin (TBIL) level, and blood urea nitrogen level have been identified as influencing factors of the four anti-TB drug plasma concentrations (Zhao et al., 2022). However, they did not consider the drug‒drug interactions (DDIs) among the four drugs, and the predictive value of indicators was not determined.
INH is mainly acetylated to acetyl isoniazid and isoniazid acid by N-acetyltransferase 2 in the liver (Metushi et al., 2016). INH can elevate plasma concentrations of companion drugs mainly by inhibiting CYP3A4 or 2C19 (Peloquin and Davies, 2021). RMP is also involved in numerous DDIs since it is a strong inducer of multiple CYP isoforms, including CYP3A4, 2A6, 2B6, 2C9 and 2C19, and the majority of prescription drugs are metabolized by one or more of these isoforms (Niemi et al., 2003; Nahid et al., 2016). PZA is mainly metabolized in the liver by microsomal dehydrogenase and xanthine oxidase, hydrolyzed into pyrazinoic acid, and then hydroxylated into inactive metabolites, which are excreted by glomerular filtration (Stemkens et al., 2019). Most EMB is excreted in its original form through the kidney, and kidney function has a certain effect on the EMB plasma concentration (Denti et al., 2015). Therefore, impaired liver function and renal function may influence the concentrations of the four drugs, and there may be some correlations of drug exposure among the four drugs.
The objectives of this study were to investigate the correlations of 2-h plasma concentrations among the four first-line anti-TB drugs, identify physiological parameters influencing the plasma concentrations of the four drugs through a stepwise multivariable linear regression model, and estimate the predictive value of indicators by using receiver operating characteristic (ROC) curve analysis.
Materials and methods
Ethics statement
The study was conducted in accordance with the Declaration of Helsinki, and the protocol of the study was approved by the Ethics Committee of Chongqing Public Health Medical Center (2023–005-02-KY). All methods were carried out in accordance with relevant guidelines and regulations. The Ethics Committee of Chongqing Public Health Medical Center approved this study to be exempt from individual patient consent for publication as existing data were collected and de-identified.
Patients and study design
Adult patients with TB hospitalized in Chongqing Public Health Medical Center from September 2021 to July 2022, undergoing 2-h plasma concentration measurements of INH, RMP, EMB, and PZA, were included in the current retrospective study. Patients receiving the treatment for the first time or patients on chronic treatment were both included. Patients who were coinfected with human immunodeficiency virus or who had diseases or symptoms that affect drug metabolism were excluded.
Data collection
The following demographic and medical details, several indicators of liver function and renal function and electrolytes within 3 days of drug concentration measurement of each eligible patient, were collected: a) sex, age, weight, underlying diseases, drinking history, and drug dose; b) prealbumin (PA), α-l-fucosidase (AFU), glutamic-pyruvic transaminase (ALT), glutamic-oxaloacetic transaminase (AST), AST/ALT, γ-glutamyl transferase (γ-GT), alkaline phosphatase (ALP), total bile acid (TBA), TBIL, direct bilirubin (DBIL), indirect bilirubin (IBIL), albumin, and cholinesterase (CHE); c) serum urea and creatinine levels, UA, cystatin C (CysC) and estimated glomerular filtration rate (eGFR); and d) serum potassium, sodium and magnesium.
Measurement of drug concentrations
Venous blood was drawn 2 h after drug ingestion under fasting conditions. Plasma was separated after centrifugation at 3,500 rpm for 10 min at 4 °C. An aliquot of 100 μL of plasma was placed into tubes containing 400 μL of 50% methanol. After 2 min of vortexing, the mixtures were centrifuged at 4 °C and 15,000 rpm for 10 min. The supernatant (20 μL) was transferred into a new tube containing 180 µL of 100% methanol, and these mixtures were then centrifuged at 4 °C and 15,000 rpm for 3 min. The supernatant (2 μL) was injected into the LC‒MS/MS system for analysis (Song et al., 2007). The method was validated over the concentration range of 0.25–50 μg/ml for INH, RMP, and EMB and 1.0–200 μg/ml for PZA. The lower limit of quantification was 0.25 μg/ml for INH, RMP, and EMB and 1.0 μg/ml for PZA.
Statistical analysis
The statistical analysis was performed by using IBM SPSS 19.0 (IBM Corp., Armonk, NY, United States). The correlations among drug concentrations and indicators were evaluated by Pearson’s correlation analysis. To investigate the independent influencing factors of plasma concentrations of INH, RMP, EMB, and PZA, 25 factors, including sex, age, dose, drinking history, and biochemical indicators, were included in the stepwise multivariable linear regression model. The predictive effect of UA and TBA for the concentrations of the four drugs was determined by receiver operating characteristic (ROC) curve analysis. A p-value of <0.05 was considered statistically significant.
Results
Patient characteristics
A total of 317 samples from 317 patients were included in the current study. The percentage of treatment-naïve patients was 55.5% (176/317). The demographic and clinical data of the patients are shown in Table 1 and Table 2. The proportion of males was 68.5%, with ages of 18–91 years and weights of 31–105 kg, and 50.8% of patients did not have a drinking history. The primary administered doses of first-line drugs were as follows: INH, 300 mg/d; RMP, 450 mg/d; EMB, 750 mg/d; and PZA, 1,500 mg/d. The proportions of patients with INH, RMP, EMB, and PZA concentrations within the therapeutic range were 24.3%, 31.5%, 27.8%, and 18.6%, respectively.
Correlations between drug concentrations and indicators
The correlations between variables and the plasma concentration of first-line anti-TB drugs are shown in Table 3. Except for albumin, γ-GT and eGFR, other indicators showed correlations with the concentration of at least one of the drugs. Levels of UA were positively associated with concentrations of INH and PZA (r = 0.230, p < 0.001; r = 0.343, p < 0.001) but negatively associated with concentrations of RMP and EMB (r = -0.332, p < 0.001; r = -0.258, p < 0.001). The concentration of INH was negatively associated with the concentrations of RMP and EMB (r = -0.361, p < 0.001; r = -0.274, p < 0.001) but positively associated with the concentration of PZA (r = 0.606, p < 0.001); the concentration of RMP was positively associated with the concentration of EMB (r = 0.543, p < 0.001) but negatively associated with the concentration of PZA (r = -0.558, p < 0.001); and the concentration of EMB was negatively associated with the concentration of PZA (r = -0.470, p < 0.001) (Figure 1).
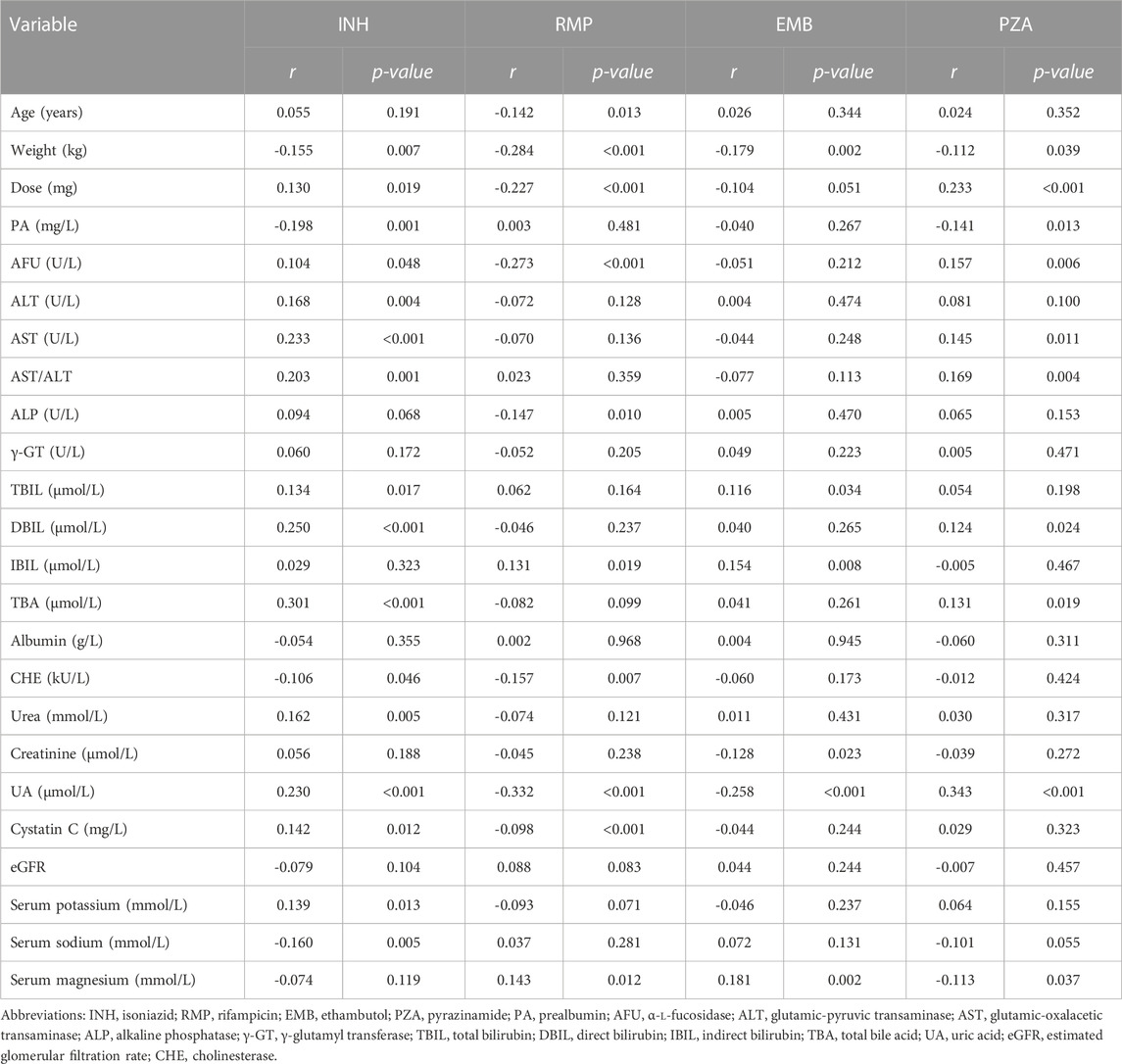
TABLE 3. Correlations between variables and the plasma concentration of first-line anti-tuberculosis drugs.
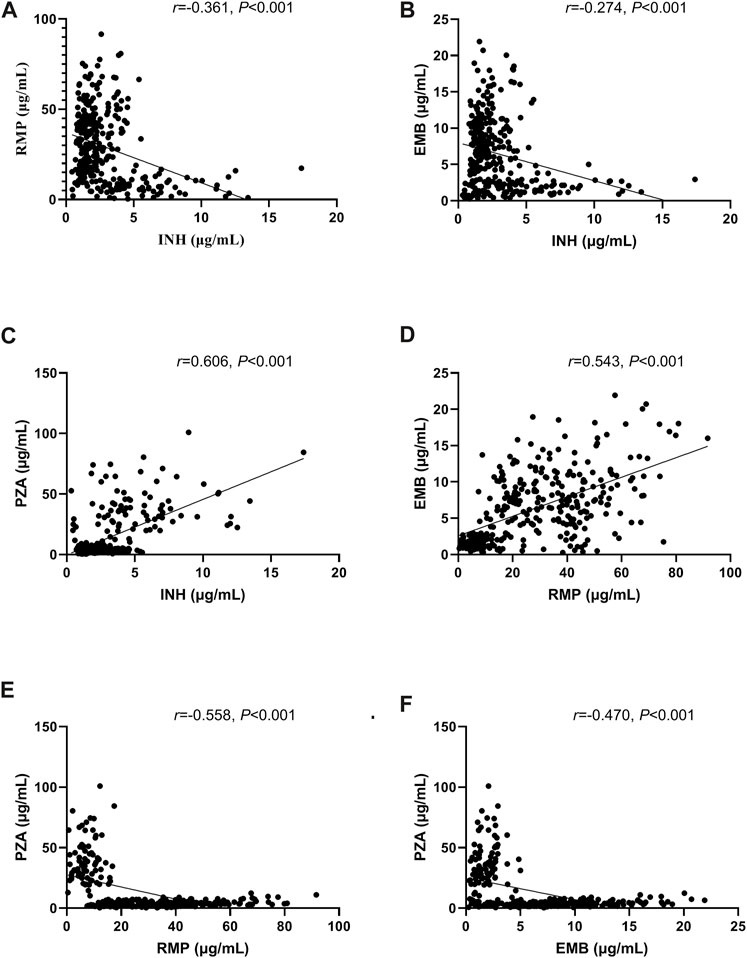
FIGURE 1. Correlations of concentrations between the first-line anti-tuberculosis (TB) drugs isoniazid (INH) and rifampicin (RMP) (A), INH and ethambutol (EMB) (B), INH and pyrazinamide (PZA) (C), RMP and EMB (D), RMP and PZA (E), and EMB and PZA (F).
Factors influencing drug concentrations
In the multivariate analysis, the PZA concentration; levels of TBA, DBIL, PA and albumin; dose; and serum potassium were the independent influencing factors of the INH concentration. PZA and EMB concentrations, weight, levels of UA, drinking and dose were the independent influencing factors of the RMP concentration. RMP and PZA concentrations, levels of creatinine, TBA and IBIL were the independent influencing factors of the EMB concentration. INH, RMP, and EMB concentrations, levels of UA, weight and drinking were the independent influencing factors of the PZA concentration (Table 4).
Exposure cutoff values for drug concentrations
A ROC curve analysis was subsequently performed to calculate the cutoff points of drug concentrations. The cutoff value of UA with the largest Youden index for INH concentrations higher than 6 μg/ml was 562.5 μmol/L, the cutoff value of UA with the largest Youden index for RMP concentrations <8 μg/ml was 486.5 μmol/L, the cutoff value of UA with the largest Youden index for EMB concentrations between 2 and 6 μg/ml was 549.5 μmol/L, and the cutoff value of UA with the largest Youden index for PZA concentrations between 20 and 60 μg/ml was 462.5 μmol/L (Figure 2). The cutoff value of TBA with the largest Youden index for INH concentrations higher than 6 μg/ml was 5.05 μmol/L (Figure 3).
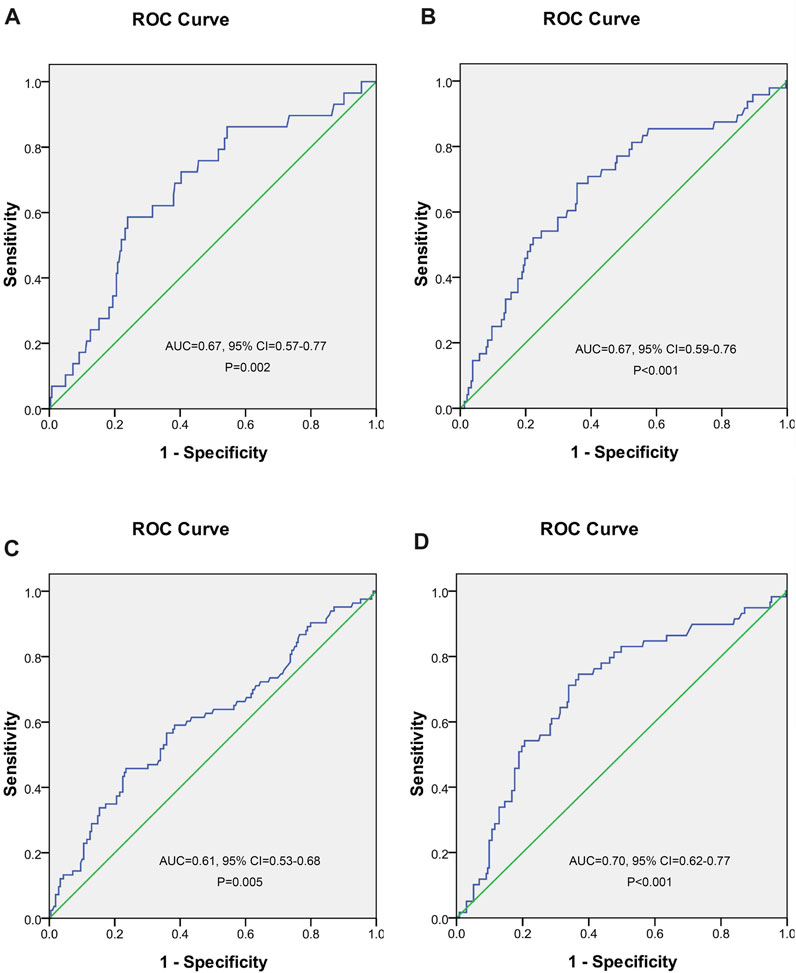
FIGURE 2. Receiver operating characteristic curve of blood uric acid for predicting concentrations of isoniazid (A), rifampicin (B), ethambutol (C), and pyrazinamide (D).
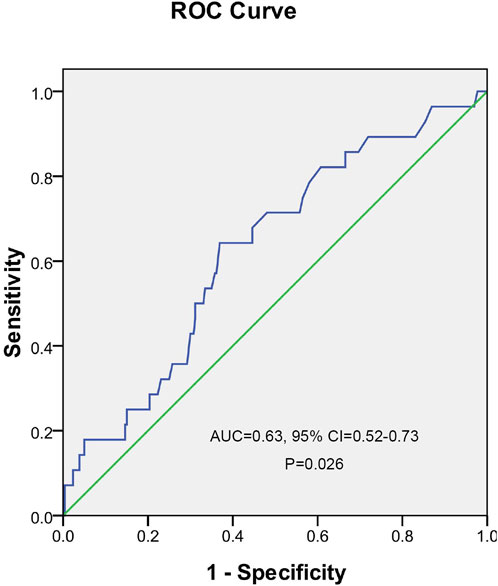
FIGURE 3. Receiver operating characteristic curve of total bile acid for predicting the concentration of isoniazid.
Discussion
In the clinic, doctors often encounter the phenomenon of an inability of a drug to reach therapeutic blood concentration levels, even after adjusting to the maximum dose, but the reason is not clear. Currently, doctors are still using the traditional standard dosing method and consider changing the drug dose when resistance or adverse events appear. In the current work, a total of 317 patients were included, and the relationships of patient demographic characteristics and major biochemical indicators prior to drug administration with 2-h plasma concentrations of anti-TB drugs were analyzed by a stepwise multivariable linear regression model. The results showed that the proportion of patients with anti-TB drug concentrations in therapeutic ranges was very low: 66.6% of patients had lower INH concentrations, 50.8% of patients had higher RMP concentrations, 47.9% of patients had higher EMB concentrations, and 77.3% of patients had lower PZA concentrations than the therapeutic concentration ranges. Low serum INH may play a role in recurrence and in acquired drug resistance (Park et al., 2016). The microbiological efficacy of PZA increases with increasing drug concentrations; however, optimizing the PZA dose alone is unlikely to be sufficient to allow TB treatment shortening; rather, the RMP dose would need to be increased in parallel (Zhang et al., 2021). Based on our results, there was a negative correlation between the concentrations of PZA and RMP. The complex correlations will pose a severe challenge in the dose adjustment to maintain all drugs within the therapeutic window. INH is a weak inhibitor of CYP3A4 or 2C19, while RMP is a strong inducer of CYP3A4, 2A6, 2B6, 2C9 and 2C19, and most EMB is excreted in its original form through the kidney. Therefore, weak correlations were found between INH and RMP and INH and EMB in our work.
A large number of previous studies have shown that anti-TB drugs can affect UA metabolism in humans, which could result in elevated blood UA (Louthrenoo et al., 2015; Thumamo Pokam et al., 2018; Ha et al., 2019). We also found that UA was an independent influencing factor of the PZA concentration. However, the association of UA with anti-TB drug concentrations and the predictive value of UA for the concentrations were complex. When the concentration of UA was elevated, the possibilities of INH concentrations higher than 6 μg/ml and RMP concentrations below 8 μg/ml were increased, but EMB and PZA concentrations were prone to be maintained in the normal range. The mechanisms of the interaction between blood UA and anti-TB drug metabolism need to be further investigated.
Low-level INH may result in INH monoresistant and multidrug resistant (MDR) TB, ultimately affecting the treatment outcome (Nagel et al., 2017). In the current work, the proportion of patients receiving 300 mg INH was 90.2%, but 66.6% of patients had INH concentrations that were lower than the therapeutic range. Previous studies showed that drug dose was positively correlated with the plasma concentration of INH (Um et al., 2007; Zhao et al., 2022), which is consistent with the results of the present study, indicating that an insufficient dose is an important factor that leads to subtherapeutic concentrations. Data showed that 80% of Asian patients presented a fast-acetylation genotype of INH, leading to low exposure to INH, and higher INH doses should be used in fast acetylators (Peloquin and Davies, 2021). The patients included in our study were not genotyped for their acetylation status, but we suspected that the proportion of the fast-acetylation genotype of INH would be high. Using INH 900 mg, 3 times weekly, may be a choice for this patient group.
TBA can effectively reflect liver cell injury and the secretion and synthesis function of liver cells, and it is elevated before bilirubin increases. PA is synthesized mainly by liver cells and has a shorter half-life than albumin, which decreases before albumin decreases and can sensitively reflect the degree of early liver injury. In addition to UA, the liver function indicators TBA, PA, and DBIL were also found to be associated with the INH concentration. INH may induce hypokalemia (Molie et al., 2019). Our results also showed that serum potassium was significantly associated with the INH concentration. Therefore, except for previously well-known influencing factors, levels of TBA, PA, and serum potassium should also be considered when adjusting the dose of INH.
A positive correlation between RMP plasma concentrations and serum albumin levels has been reported (Tappero et al., 2005; Abulfathi et al., 2019; Lei et al., 2019), which may be attributed to the albumin binding rate of the drug. In our results, we did not find marked effects of albumin on the RMP concentration, possibly because most patients had lower albumin levels (65.8%). Weight showed a significant effect on the RMP concentration, which indicated that the dose of RMP should be adjusted individually. In the current work, 50.8% of patients had RMP concentrations higher than the therapeutic range. This phenomenon may be attributed to the fact that 31% of patients received 600 mg/d RMP. Previous studies showed that higher RMP doses were associated with more rapid sputum sterilization but with similar toxicity (Velasquez et al., 2018). The level of AFU may be elevated in approximately 40% of patients with hepatitis and 30% of patients with cirrhosis (Johnson, 2001). We also found that AFU was an independent influencing factor of the RMP concentration. The level of AFU should be considered when setting the dose of RMP.
Seventy percent of EMB is excreted unchanged in the urine, and elimination is closely related to renal function. In our results, serum creatinine, except for TBA or IBIL, was significantly associated with the EMB concentration, which indicated that serum creatinine should be considered when using EMB.
High exposure to PZA and its metabolites may result in hepatotoxicity, whereas low exposure to PZA has been correlated with the failure of first-line anti-TB therapy (Sundell et al., 2021). Our results showed that most patients’ PZA concentrations were below the therapeutic range (77.3%), which indicated that the patients were at risk of treatment failure. In the process of metabolizing alcohol, the metabolites, ethanol and metabolic derivatives produced by the liver will cause inflammation in the liver, and the inflammation will cause damage to the liver cells and even necrosis, leading to the occurrence of alcoholic hepatitis (Hyun et al., 2021). We found that drinking history was an independent influencing factor of RMP and PZA concentrations. The results highlight the importance of temperance in receiving first-line anti-TB drugs.
The following limitations should be noted. First, this was a single-center retrospective study, and the sample was relatively small. A multicenter, prospective, and large sample study is needed to confirm our results. Second, we analyzed the serum concentration only at 2 h after administration, which may lead to certain bias. Third, in the current study, the sensitivity and specificity observed for the cutoff values of UA and TBA were low. This finding was predictable because of the multiple influencing factors of the concentrations of each drug in the multivariable analysis. However, we could also obtain beneficial findings from the cutoff values to some extent. In addition, it is necessary to analyze the relationship between the drug concentration and drug efficacy and patient prognosis.
In conclusion, the correlations among the four first-line anti-TB drug concentrations were complex, which leads to a major challenge in dose adjustments to maintain all drugs within the therapeutic window. In addition to previously well-known influencing factors, including age, sex, weight, dose, UA, DBIL, and IBIL, the levels of TBA, PA, AFU, and serum potassium should also be considered when adjusting the dose of the four drugs. During long-term administration of the four first-line anti-TB drugs, plasma concentrations of the drugs and relevant indicators should be monitored to optimize the dosing schedule, and the concentration prediction model derived from our multivariate analysis may be referenced.
Data availability statement
The raw data supporting the conclusion of this article will be made available by the authors, without undue reservation.
Ethics statement
The studies involving humans were approved by the Ethics Committee of Chongqing Public Health Medical Center. The studies were conducted in accordance with the local legislation and institutional requirements. The ethics committee/institutional review board waived the requirement of written informed consent for participation from the participants or the participants' legal guardians/next of kin because this is a retrospective study, and existing data were collected and de-identified.
Author contributions
ML, YF, PW, and GZ collected the data. LC, SQ, BW conducted analysis. LC, YG, ZL wrote the manuscript. LC was main originator of the manuscript. PL, SL supervised and corrected the manuscript. PL, ZL, ML, and GZ funded the study. All authors contributed to the article and approved the submitted version.
Funding
This work was supported by the Fund for the Traditional Chinese Medical Research Project of Science and Health of Chongqing, China (2019ZY3483); the Medical Research Project of Science and Health of Chongqing, China (2023MSXM107); Science and Technology Project of Shapingba District, Chongqing, China (Jcd202213, Jcd202214); Chongqing Clinical Pharmacy Key Specialties Construction Project, China. Funding by Senior Medical Talents Program of Chongqing for Young and Middle-aged.
Conflict of interest
The authors declare that the research was conducted in the absence of any commercial or financial relationships that could be construed as a potential conflict of interest.
Publisher’s note
All claims expressed in this article are solely those of the authors and do not necessarily represent those of their affiliated organizations, or those of the publisher, the editors and the reviewers. Any product that may be evaluated in this article, or claim that may be made by its manufacturer, is not guaranteed or endorsed by the publisher.
References
Abulfathi, A. A., Decloedt, E. H., Svensson, E. M., Diacon, A. H., Donald, P., and Reuter, H. (2019). Clinical pharmacokinetics and pharmacodynamics of rifampicin in human tuberculosis. Clin. Pharmacokinet. 58 (9), 1103–1129. doi:10.1007/s40262-019-00764-2
Alsultan, A., and Peloquin, C. A. (2014). Therapeutic drug monitoring in the treatment of tuberculosis: An update. Drugs 74 (8), 839–854. doi:10.1007/s40265-014-0222-8
Chakaya, J., Khan, M., Ntoumi, F., Aklillu, E., Fatima, R., Mwaba, P., et al. (2021). Global tuberculosis report 2020 - reflections on the global TB burden, treatment and prevention efforts. Int. J. Infect. Dis. 113 (1), S7–S12. Suppl 1. doi:10.1016/j.ijid.2021.02.107
Denti, P., Jeremiah, K., Chigutsa, E., Faurholt-Jepsen, D., PrayGod, G., Range, N., et al. (2015). Pharmacokinetics of isoniazid, pyrazinamide, and ethambutol in newly diagnosed pulmonary TB patients in Tanzania. PLoS One 10 (10), e0141002. doi:10.1371/journal.pone.0141002
Dheda, K., Lenders, L., Magombedze, G., Srivastava, S., Raj, P., Arning, E., et al. (2018). Drug-penetration gradients associated with acquired drug resistance in patients with tuberculosis. Am. J. Respir. Crit. Care Med. 198 (9), 1208–1219. doi:10.1164/rccm.201711-2333OC
Furin, J., Cox, H., and Pai, M. (2019). Tuberculosis. Lancet 393 (10181), 1642–1656. doi:10.1016/S0140-6736(19)30308-3
Ha, Y. J., Chung, S. W., Lee, J. H., Kang, E. H., Lee, Y. J., and Song, Y. W. (2019). Clinical features and risk factors for gout attacks during anti-tuberculosis treatment: A case-control study in South Korea. Int. J. Rheum. Dis. 22 (10), 1905–1911. doi:10.1111/1756-185X.13697
Hyun, J., Han, J., Lee, C., Yoon, M., and Jung, Y. (2021). Pathophysiological aspects of alcohol metabolism in the liver. Int. J. Mol. Sci. 22 (11), 5717. doi:10.3390/ijms22115717
Johnson, P. J. (2001). The role of serum alpha-fetoprotein estimation in the diagnosis and management of hepatocellular carcinoma. Clin. Liver Dis. 5 (1), 145–159. doi:10.1016/s1089-3261(05)70158-6
Kumar, A. K. H., Chandrasekaran, V., Kannan, T., Lavanya, J., Swaminathan, S., and Ramachandran, G. (2018). Intrapatient variability in plasma rifampicin & isoniazid in tuberculosis patients. Indian J. Med. Res. 147 (3), 287–292. doi:10.4103/ijmr.IJMR_1961_16
Lei, Q., Wang, H., Zhao, Y., Dang, L., Zhu, C., Lv, X., et al. (2019). Determinants of serum concentration of first-line anti-tuberculosis drugs from China. Med. Baltim. 98 (41), e17523. doi:10.1097/MD.0000000000017523
Louthrenoo, W., Hongsongkiat, S., Kasitanon, N., Wangkaew, S., and Jatuworapruk, K. (2015). Effect of antituberculous drugs on serum uric acid and urine uric acid excretion. J. Clin. Rheumatol. 21 (7), 346–348. doi:10.1097/RHU.0000000000000297
Lu, Y., and Zhu, H. (2021). Expert consensus on the therapeutic drug monitoring of anti-tuberculosis drugs. Chin. J. Antituberc. 43 (9), 867–873.
Martson, A. G., Burch, G., Ghimire, S., Alffenaar, J. C., and Peloquin, C. A. (2021). Therapeutic drug monitoring in patients with tuberculosis and concurrent medical problems. Expert Opin. Drug Metab. Toxicol. 17 (1), 23–39. doi:10.1080/17425255.2021.1836158
Metushi, I., Uetrecht, J., and Phillips, E. (2016). Mechanism of isoniazid-induced hepatotoxicity: Then and now. Br. J. Clin. Pharmacol. 81 (6), 1030–1036. doi:10.1111/bcp.12885
Molie, T., Teklemariam, Z., Klinkenberg, E., Dessie, Y., Kumsa, A., Mohammed, H., et al. (2019). Intensive phase treatment outcome and associated factors among patients treated for multi drug resistant tuberculosis in Ethiopia: A retrospective cohort study. BMC Infect. Dis. 19 (1), 818. doi:10.1186/s12879-019-4411-7
Nagel, S., Streicher, E. M., Klopper, M., Warren, R. M., and Van Helden, P. D. (2017). Isoniazid resistance and dosage as treatment for patients with tuberculosis. Curr. Drug Metab. 18 (11), 1030–1039. doi:10.2174/1389200218666171031121905
Nahid, P., Dorman, S. E., Alipanah, N., Barry, P. M., Brozek, J. L., Cattamanchi, A., et al. (2016). Official American thoracic society/centers for disease control and prevention/infectious diseases society of America clinical practice guidelines: Treatment of drug-susceptible tuberculosis. Clin. Infect. Dis. 63 (7), e147–e195. doi:10.1093/cid/ciw376
Niemi, M., Backman, J. T., Fromm, M. F., Neuvonen, P. J., and Kivisto, K. T. (2003). Pharmacokinetic interactions with rifampicin: Clinical relevance. Clin. Pharmacokinet. 42 (9), 819–850. doi:10.2165/00003088-200342090-00003
Park, J. S., Lee, J. Y., Lee, Y. J., Kim, S. J., Cho, Y. J., Yoon, H. I., et al. (2016). Serum levels of antituberculosis drugs and their effect on tuberculosis treatment outcome. Antimicrob. Agents Chemother. 60 (1), 92–98. doi:10.1128/AAC.00693-15
Peloquin, C. A., and Davies, G. R. (2021). The treatment of tuberculosis. Clin. Pharmacol. Ther. 110 (6), 1455–1466. doi:10.1002/cpt.2261
Prahl, J. B., Johansen, I. S., Cohen, A. S., Frimodt-Moller, N., and Andersen, A. B. (2014). Clinical significance of 2 h plasma concentrations of first-line anti-tuberculosis drugs: A prospective observational study. J. Antimicrob. Chemother. 69 (10), 2841–2847. doi:10.1093/jac/dku210
Sharma, P. K., Bansal, R., Bhardwaj, A. K., Sood, V., Sood, A., and Padwad, Y. (2018). Plasma levels of Rifampicin and Pyrazinamide with pre and post meal administration in tuberculosis patients. Indian J. Tuberc. 65 (1), 35–40. doi:10.1016/j.ijtb.2017.08.004
Song, S. H., Jun, S. H., Park, K. U., Yoon, Y., Lee, J. H., Kim, J. Q., et al. (2007). Simultaneous determination of first-line anti-tuberculosis drugs and their major metabolic ratios by liquid chromatography/tandem mass spectrometry. Rapid Commun. Mass Spectrom. 21 (7), 1331–1338. doi:10.1002/rcm.2961
Stemkens, R., Litjens, C. H. C., Dian, S., Ganiem, A. R., Yunivita, V., van Crevel, R., et al. (2019). Pharmacokinetics of pyrazinamide during the initial phase of tuberculous meningitis treatment. Int. J. Antimicrob. Agents 54 (3), 371–374. doi:10.1016/j.ijantimicag.2019.06.010
Stott, K. E., Pertinez, H., Sturkenboom, M. G. G., Boeree, M. J., Aarnoutse, R., Ramachandran, G., et al. (2018). Pharmacokinetics of rifampicin in adult TB patients and healthy volunteers: A systematic review and meta-analysis. J. Antimicrob. Chemother. 73 (9), 2305–2313. doi:10.1093/jac/dky152
Sundell, J., Wijk, M., Bienvenu, E., Abelo, A., Hoffmann, K. J., and Ashton, M. (2021). Factors affecting the pharmacokinetics of pyrazinamide and its metabolites in patients coinfected with HIV and implications for individualized dosing. Antimicrob. Agents Chemother. 65 (7), e0004621. doi:10.1128/AAC.00046-21
Tappero, J. W., Bradford, W. Z., Agerton, T. B., Hopewell, P., Reingold, A. L., Lockman, S., et al. (2005). Serum concentrations of antimycobacterial drugs in patients with pulmonary tuberculosis in Botswana. Clin. Infect. Dis. 41 (4), 461–469. doi:10.1086/431984
Thumamo Pokam, B. D., Enoh, J. E., Eyo, A. O., Umoh, N. O., and Guemdjom, P. W. (2018). Uric acid levels in patients on antituberculosis drugs in the southwest Region of Cameroon. Int. J. Mycobacteriol 7 (1), 89–91. doi:10.4103/ijmy.ijmy_161_17
Um, S. W., Lee, S. W., Kwon, S. Y., Yoon, H. I., Park, K. U., Song, J., et al. (2007). Low serum concentrations of anti-tuberculosis drugs and determinants of their serum levels. Int. J. Tuberc. Lung Dis. 11 (9), 972–978.
Velasquez, G. E., Brooks, M. B., Coit, J. M., Pertinez, H., Vargas Vasquez, D., Sanchez Garavito, E., et al. (2018). Efficacy and safety of high-dose rifampin in pulmonary tuberculosis. A randomized controlled trial. Am. J. Respir. Crit. Care Med. 198 (5), 657–666. doi:10.1164/rccm.201712-2524OC
Weiner, M., Burman, W., Vernon, A., Benator, D., Peloquin, C. A., Khan, A., et al. (2003). Low isoniazid concentrations and outcome of tuberculosis treatment with once-weekly isoniazid and rifapentine. Am. J. Respir. Crit. Care Med. 167 (10), 1341–1347. doi:10.1164/rccm.200208-951OC
Zhang, N., Savic, R. M., Boeree, M. J., Peloquin, C. A., Weiner, M., Heinrich, N., et al. (2021). Optimising pyrazinamide for the treatment of tuberculosis. Eur. Respir. J. 58 (1), 2002013. doi:10.1183/13993003.02013-2020
Keywords: tuberculosis, isoniazid, rifampicin, ethambutol, pyrazinamide, drug concentration
Citation: Cheng L, Luo M, Guo Y, Fan Y, Wang P, Zhou G, Qin S, Weng B, Li P, Liu Z and Liu S (2023) Correlations among the plasma concentrations of first-line anti-tuberculosis drugs and the physiological parameters influencing concentrations. Front. Pharmacol. 14:1248331. doi: 10.3389/fphar.2023.1248331
Received: 28 June 2023; Accepted: 25 September 2023;
Published: 06 October 2023.
Edited by:
Yurong Lai, Gilead, United StatesReviewed by:
Ingrid Fricke-Galindo, Instituto Nacional de Enfermedades Respiratorias-México (INER), MexicoSandhya Subash, Washington State University Health Sciences Spokane, United States
Copyright © 2023 Cheng, Luo, Guo, Fan, Wang, Zhou, Qin, Weng, Li, Liu and Liu. This is an open-access article distributed under the terms of the Creative Commons Attribution License (CC BY). The use, distribution or reproduction in other forums is permitted, provided the original author(s) and the copyright owner(s) are credited and that the original publication in this journal is cited, in accordance with accepted academic practice. No use, distribution or reproduction is permitted which does not comply with these terms.
*Correspondence: Peibo Li, MTU3MzE4ODUxQHFxLmNvbQ==; Zhirui Liu, emhpcnVpX2xpdTIwMTNAMTYzLmNvbQ==; Songtao Liu, NDE1NzAxNjU5QHFxLmNvbQ==
†These authors have contributed equally to this work