- 1School of Biology and Basic Medical Sciences, Soochow University, Suzhou, China
- 2Guangxi Subtropical Crops Research Institute, Nanning, China
- 3Key Laboratory of Quality and Safety Control for Subtropical Fruit and Vegetable, Ministry of Agriculture and Rural Affairs, Nanning, China
The importance of adequate sleep for good health cannot be overstated. Excessive light exposure at night disrupts sleep, therefore, it is important to find more healthy drinks that can promote sleep under sleep-disturbed conditions. The present study investigated the use of A. sinensis (Lour.) Spreng leaf tea, a natural product, to reduce the adverse effects of nighttime light on sleep. Here, Aquilaria sinensis leaf tea at 1.0 and 1.5 g/L significantly increased sleep time in zebrafish larvae (5–7 dpf) with light-induced sleep disturbance. Transcriptome sequencing and qRT-PCR analysis revealed a decrease in the immune-related genes, such as nfkbiab, tnfrsf1a, nfkbiaa, il1b, traf3, and cd40 in the 1.5 g/L Aquilaria sinensis leaf tea treatment group. In addition, a gene associated with sleep, bhlhe41, showed a significant decrease. Moreover, Aquilaria sinensis leaf tea suppressed the increase in neutrophils of Tg(mpo:GFP) zebrafish under sleep-disturbed conditions, indicating its ability to improve the immune response. Widely targeted metabolic profiling of the Aquilaria sinensis tea using ultra-performance liquid chromatography coupled with electrospray tandem mass spectrometry (UPLC-ESI-MS/MS) revealed flavonoids as the predominant component. Network pharmacological and molecular docking analyses suggested that the flavonoids quercetin and eupatilin in Aquilaria sinensis leaf tea improved the sleep of zebrafish by interacting with il1b and cd40 genes under light exposure at night. Therefore, the results of the study provide evidence supporting the notion that Aquilaria sinensis leaf tea has a positive impact on sleep patterns in zebrafish subjected to disrupted sleep due to nighttime light exposure. This suggests that the utilization of Aquilaria sinensis leaf tea as a potential therapeutic intervention for sleep disturbances induced by light may yield advantageous outcomes.
1 Introduction
Aquilaria sinensis (Lour.) Spreng, or Agarwood is a traditional spice and valuable Chinese medicine. It belongs to the Thymelaeaceae tree family. For religious and medicinal purposes, Aquilaria sinensis resin and oil are used in perfumes and incense (Naziz et al., 2019). Traditional medicine uses A. sinensis resin or oil to treat inflammation, diarrhea, joint pain, cardiovascular disease, insomnia, and stimulate the body (Pang et al., 2023a). A. sinensis leaves are secondary agricultural products before the wood can be harvested. There are many health benefits associated with A. sinensis leaves, and traditionally, these leaves have been consumed as tea, especially in Asia and Southeast Asia (Han and Li, 2012; Adam et al., 2017; Surjanto et al., 2019). Studying the biological activity of A. sinensis leaf extract will help expand the plant’s therapeutic and medicinal potential and realize the comprehensive utilization of resources. The extracts from agarwood leaves exhibit a variety of biological activities, including antimicrobial, anticancer, and anti-inflammatory properties (Cheng et al., 2013; Kamonwannasit et al., 2013; Eissa et al., 2021). Additionally, A. sinensis leaf extracts may help improve the immune response of macrophages by regulating pro-inflammatory cytokines and also enhance innate immunity against infection on RAW264.7 murine macrophages. Investigating these aspects using animal models will validate.
Sleep is a physiological phenomenon that has been hypothesized to possess rejuvenating and regulatory attributes (Benington and Craig Heller, 1995; Mackiewicz et al., 2007). Despite the lack of precise understanding regarding its specific purpose, sleep has garnered significant attention in recent times owing to its potential impact on the immune system. Numerous investigations have provided evidence that sleep disturbance alters diverse elements of the immune system, including the proportions of cell subpopulations (such as CD4+ and NK) and levels of cytokines (such as IFN-g, TNF-a, and IL-1) (Dimitrov et al., 2007; Zager et al., 2007; Yehuda et al., 2009). Earlier we mentioned that A. sinensis leaf extracts may help improve the immune response, so this study aims to investigate the effect of tea on sleep.
Zebrafish is one of the best model organisms used in research due to its tremendously large spawning capacity, in vitro development, transparent embryos, and highly homologous to humans. Exposing zebrafish larvae to chemical treatments is considerably simpler and easier than exposing mammals. Thus, it is an ideal vertebrate model for evaluating the efficacy of natural products, food, and drugs and studying sleep and immune responses (Prober et al., 2006; Appelbaum et al., 2009; Rihel et al., 2010; Varela et al., 2017; Tran and Prober, 2022). Furthermore, akin to humans, zebrafish exhibit a comparable sleep-wake cycle, and the presence of nighttime illumination can greatly impede zebrafish sleep, resulting in sleep disturbances (Yokogawa et al., 2007; Hirayama et al., 2019). In this study, to investigate the new efficacy of A. sinensis leaf tea, using behavior, transcriptomics, metabolomics, and network pharmacology, we found the improved effects of sleep-disturbed and immune functions of A. sinensis leaf tea in zebrafish.
2 Material and methods
2.1 Zebrafish culture
Adults of AB and Tg(mpo:GFP) (Renshaw et al., 2006) zebrafish (Danio rerio) lines were cultured in a circulating water system under a 14 h/10 h light-dark cycle and a constant temperature of 28°C; these adults were fed three times per day. The male and female zebrafish were paired at night, and eggs were laid the following day within 1 h after turning on the lights. These embryos were placed in 10 cm dishes containing 1 × E3 medium (The pH was adjusted to 7.0–7.2 using 5 mM NaCl, 0.17 mM KCl, 0.33 mM CaCl2 and 0.33 mM MgSO4) with methylene blue (0.3 ppm) and reared in a light-controlled incubator (14 h/10 h light-dark cycle, ZT 0–ZT 14, light condition; ZT 14–ZT 24, dark condition; ZT: Zeitgeber Time) at 28°C. All experimental procedures of this study were approved by the Animal Care and Use Committee of Soochow University and performed following Chinese government regulations.
2.2 Boiling water extraction of A. sinensis leaf tea
Aquilaria sinensis leaf tea was from commercially available A. sinensis leaf tea (Yishen Tea Co., Ltd. Lianjiang City, China) and processed following the regional standard “technical specification” for the production of organic oolong tea in Guangdong Province (https://www.sdtdata.com/fx/fmoa/tsLibCard.doView). Then, the guidelines outlined in the “Technical Guidelines for Toxicological Examination and Evaluation of Health Food and Its Raw Materials Safety (2020 edition)" https://www.samr.gov.cn/tssps/tzgg/zjwh/202010/t20201031_322804.html) were followed to obtain the leaf extract. Here, the A. sinensis leaf tea were weighed, then put into a 50 mL conical flask, and 25 mL of 1 × E3 medium (100°C) was added; after 30 min, the extract was transferred to a new 50 mL flask from the conical flask, this process was repeated twice and the extracts combined. The extract was then centrifuged at 1000 rpm and 28°C for 5 min, and the supernatant obtained was used as the leaf tea for subsequent experiments.
2.3 Determination of semi-lethal concentration of A. sinensis leaf tea in zebrafish
Zebrafish larvae at 5 dpf (days post fertilization) were housed in 24-well plates (ten fish per well) containing 2 mL of maintaining a well without tea as the negative control and three replicate wells per concentration under 14 h/10 h light-dark cycle and 28°C conditions. The number of dead zebrafish larvae was recorded during daily processing and LC50 was calculated using Probit (assumes normal distribution) analysis (Finney, 1971) by GraphPad (version 8.0).
2.4 Effects of A. sinensis leaf tea, eupatilin, and quercetin on the sleep patterns of zebrafish with light-induced sleep disturbance
One zebrafish larva (5 dpf) was placed in each well of a 48-well plate and treated with 1 mL of A. sinensis leaf tea (0.5, 0.75, 1.00, and 1.50 g/L), eupatilin (2, 4, 10 μM) (TargetMol, Boston, MA, United States), and quercetin (40, 80, 160 μM) (Shanghai Yuanye Bio-Technology CO., Ltd.) at varying concentrations; eight wells were maintained per treatment concentration. Sleep disturbance was induced by providing 300 lux illumination every night from 23:00–03:00, using a zebrafish behavior trajectory tracking system (ZebraBox, ViewPoint, France) which also recorded the behavior based on light exposure, and the sleep data were recorded using ZebraLab software (Version 2.3.1, ViewPoint, France) with the following parameters: detection threshold, 20; burst, 25; freeze, 4; bin size, 60 s. Then, the sleep and wake behaviors of each zebrafish were analyzed using MATLAB (Version R2021a) scripts as described in David Prober’s lab (Lee et al., 2017).
2.5 Transcriptome sequencing and data analysis
Thirty zebrafish larvae (5 dpf) were placed in each well of a six-well plate and treated with 3 mL of 1.5 g/L A. sinensis leaf tea, maintaining wells without the tea as a control and three wells per treatment as replicates. The zebrafish larvae were subjected to sleep disturbance by exposure to 300 lux light and collected at ZT 18 on the third day after treatment. RNA was extracted from these larval samples via Trizol reagent (Invitrogen, United States). Transcriptome sequencing was performed by BioMarker (Biomarker Technologies Corporation, Beijing, China), and the KEGG (Kyoto Encyclopedia of Genes and Genomes), COG (Clusters of Orthologous Groups of proteins), Ortholog database, and GO (Gene Ontology) database. The reads from each sample were assembled using the StringTie software, and the computation of FPKM (Fragments per kilobase of transcript per million mapped reads) was performed by both StringTie and edgeR in order to evaluate the expression levels of all mRNAs. Subsequently, the mRNAs and genes exhibiting differential expression levels were identified using the R package, with a criterion of log2 (fold change) > 1.2 or < −1.2 and a significance level of p < 0.05. The Biomarker cloud platform (Biomarker Biotechnology Co., Beijing, China) was used to prepare the transcriptome sequencing data statistics and a few tables.
2.6 Quantitative real-time PCR
As described previously, total RNA was extracted using TRIzol Reagent® (Invitrogen), and cDNA was prepared by reverse transcription of 3 μg of DNAse I-treated total RNA. Then, qRT-PCR was performed using the cDNA on a StepOnePlus ABI instrument using SYBR green detection kit (Invitrogen) and the following thermal profile: 40 cycles of 10 s at 95°C and 30 s at 60°C. The relative expression levels of the nfkbiab, cd40, traf3, il1b, nfkbiaa, tnfrsf1a, bhlhe41 were calculated following the 2−△△CT method (VanGuilder et al., 2008). The list of primers used in this study is shown in Supplementary Table S1.
2.7 Sleep disturbance and images of neutrophil-specific Tg(mpo:GFP) zebrafish
Ten Tg(mpo:GFP) larvae (5 dpf) were placed in each well of a 12-well plate in embryo culture medium with and without A. sinensis leaf tea (1.5 g/L). Light-induced sleep disturbance experiments were carried out on the zebrafish behavior trajectory tracking system (ZebraBox, ViewPoint, France) by providing 300 lux illumination every night from ZT 14 to ZT 18. On the third day of sleep disturbance, Tg(mpo:GFP) larvae were anesthetized at ZT 17–ZT 18, and images were captured after being treated with a fluorescence microscope (Axio Imager M2, Zeiss).
2.8 Widely targeted metabolomic profiling
The chemical constituents of 1.5 g/L A. sinensis leaf tea by the Wuhan MetWare Biotechnology Co., Ltd. via widely-targeted metabolome analyses (www.metware.cn). Standard procedures (Yuan et al., 2018) were followed for extraction, metabolite identification, and quantification. Briefly, after freeze drying sample, 70% methanol internal standard was added at a 30:1 ratio. After centrifugation, the supernatant was removed and stored in an injection vial for liquid chromatography-tandem mass spectrometry (LC-MS/MS). The ultra-performance liquid chromatography coupled with electrospray tandem mass spectrometry (UPLC-ESI-MS/MS; ExionLC™ AD, Sciex, Darmstadt, Germany) and the tandem mass spectrometry system (Sciex, Darmstadt, Germany) were used to analyze the metabolites of A. sinensis leaf tea extracts. Metabolites were quantified using the LC-ESI-Q TRAP-MS/MS (4000Q TRAP, ABI, United States) for multiple reaction monitoring (MRM).
The detected metabolites were classified into Class I and Class II based on the number of each metabolite, and their proportion in the total substance was analyzed by Microsoft Excel software (Version Microsoft 365, Microsoft Corporation, United States). The relative content of the primary metabolite was determined based on the peak map area of Class I. Then, the ratio of the relative content of each primary substance to the total content was also screened by Microsoft Excel software (Version Microsoft 365, Microsoft Corporation, United States).
2.9 Prediction of target genes of flavonoids in A. sinensis leaf tea and construction of the network
The Traditional Chinese Medicine Systems Pharmacology Database and Analysis Platform (https://old.tcmsp-e.com/tcmsp.php) was used to predict the target genes corresponding to the flavonoid metabolites. The compound-target network was generated using Cytoscape 3.9.1 based on transcriptomic and metabolomics data (Li et al., 2022).
2.10 Molecular docking
The structure of the metabolites (quercetin and eupatilin) was obtained from the PubChem database, and the SWISS-MODEL platform (https://swissmodel.expasy.org/) was used to generate the 3D structure of the target protein (Il1b and Cd40). Finally, AutoDock Tools (Version 1.5.7) was used for molecular docking, and as a calculation method, molecular docking was used to predict receptor-ligand binding modes, and PyMol 4.6.0 was used to visualize the docking results (Morris et al., 2009; Seeliger and de Groot, 2010).
2.11 Statistical analysis
The normality of the data was assessed through the application of the Kolmogorov–Smirnov test. In the event that the data exhibited a normal distribution, One-way ANOVA was employed, with adjustments made for multiple comparisons using Tukey’s test. Conversely, if the data did not meet the assumptions of parametric analysis, the Kruskal–Wallis test was utilized, with corrections for multiple comparisons made using Dunn–Sidak. The LC50 of A. sinensis leaf tea on zebrafish and the mortality rate of zebrafish larvae were determined using the Probit (assumes normal distribution) function in GraphPad 8.0 software. Additionally, Student’s t-test with Origin 2021 software (OriginLab, Northampton, Massachusetts, United States) was used for comparing two-group data. A significance level of p < 0.05 was considered statistically significant for all tests.
3 Results
3.1 Semi-lethal concentration of A. sinensis leaf tea on zebrafish
First, to determine the semi-lethal concentration (LC50) of A. sinensis leaf tea, 5 dpf zebrafish larvae were treated with six different concentrations (0.0 as a control, 2.0, 4.0, 8.0, 16.0, and 32.0 g/L; Figures 1A, B), and the number of dead larvae was recorded daily for 5 days after treatment (from 6 to 10 dpf). The survival curve showed that all the larvae treated at 5 dpf with A. sinensis leaf tea at 8.0, 16.0, and 32.0 g/L concentrations died at 6 dpf (the first day after treatment), while a few (16.67% and 40%) larvae treated with 2.0 and 4.0 g/L died. No new dead larvae were found in all the concentrations on 7 and 8 dpf (days 2–3 after treatment). At 9 dpf (the 4th day after treatment), more larvae (20% and 50%) treated with 2.0 g/L and 4.0 g/L died, while no new deaths were observed in the other concentrations. No new larval deaths were recorded in any treatment groups on the 5th day after treatment (10 dpf) (Figure 1C). Further analysis found that on the 4th day after treatment, the LC50 of the A. sinensis leaf tea on zebrafish larvae was 3.707 g/L, with a 0.003–0.004 g/L 95% confidence interval (Figure 1D).
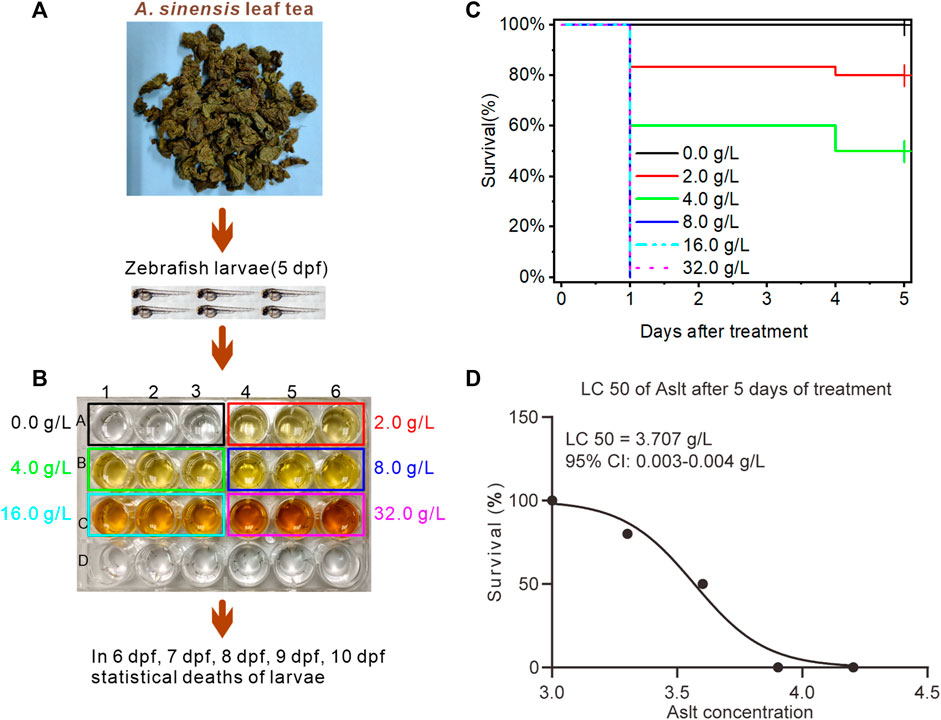
FIGURE 1. Effect of Aquilaria sinensis (Lour.) Spreng leaf tea extract on zebrafish larvae. (A) Treatment of zebrafish larvae at 5 dpf with A. sinensis leaf tea extract. (B) Zebrafish larvae exposed to six concentrations: 0.0 g/L (control), 2.0 g/L, 4.0 g/L, 8.0 g/L, 16.0 g/L, and 32.0 g/L (n = 90). The number of dead larvae was counted between 6 and 10 dpf. (C) The survival ratio of zebrafish larvae was calculated and plotted as Kaplan-Meier curves (n = 90). (D) LC50 of A. sinensis leaf extract (n = 90). Here, Aslt represents the A. sinensis leaf extract.
3.2 Aquilaria sinensis leaf tea improves sleep in zebrafish with sleep disturbance
Further, to explore the effects of A. sinensis leaf tea extract on zebrafish with sleep disturbed (Figure 2A), 5 dpf larvae were exposed to six concentrations (0.00 as a control, 0.50, 0.75, 1.00, 1.25, 1.50 g/L, which less than half of LC50) of A. sinensis leaf tea extract (Figure 2B). Light exposure resulted in zebrafish sleep disturbance (Yokogawa et al., 2007). Only 1.50 g/L of A. sinensis leaf tea-treated larvae slept more compared to the other groups under day conditions, and no difference was observed among all groups at night time (without sleep disturbed time, 03:00–9:00). However, under sleep-disturbed conditions (23:00–03:00), the sleep time of zebrafish treated with 0.75, 1.00, 1.25, and 1.50 g/L of A. sinensis leaf tea was significantly increased compared with control (0.0 g/L) (Figures 2C–E) but no influence for the sleep bout (Figure 2F). These results suggest that A. sinensis leaf tea could improve the sleep of zebrafish under sleep disturbance conditions but could not change the sleep bout.
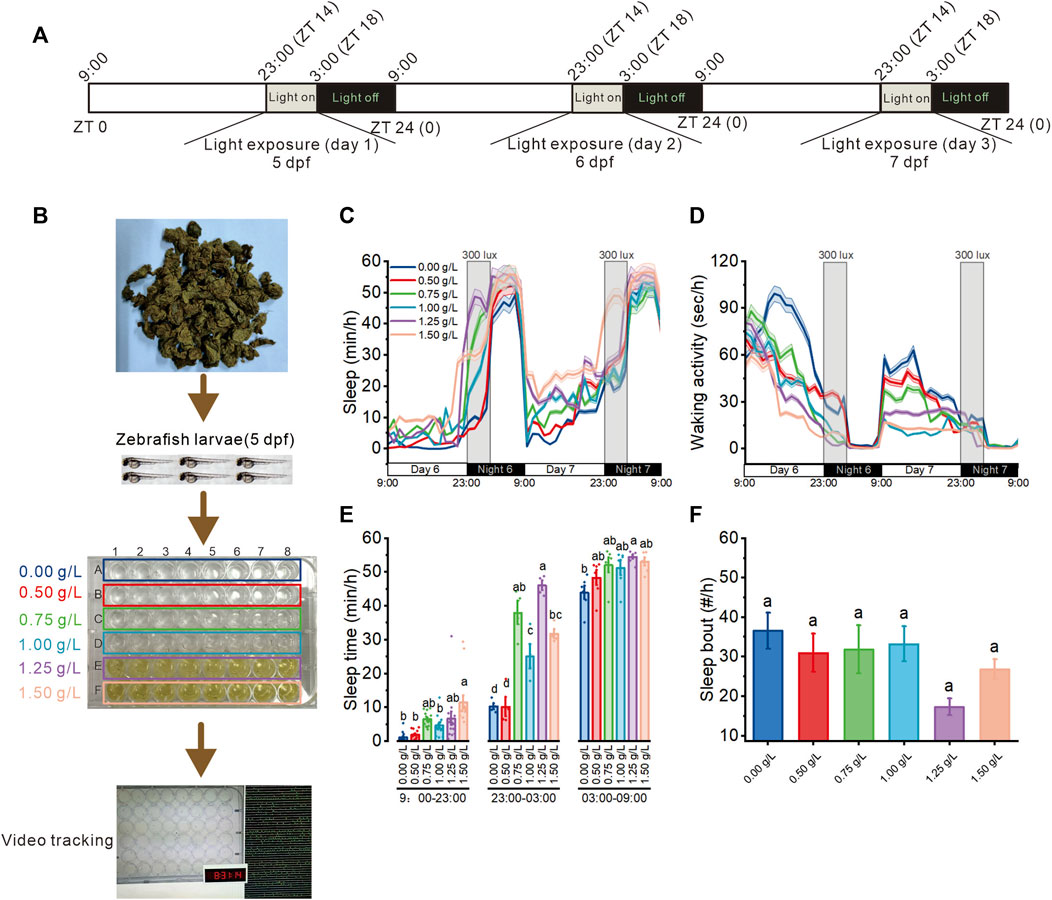
FIGURE 2. Effect of Aquilaria sinensis leaf tea on the sleep of zebrafish with sleep disturbance. (A) The schematic diagram shows sleep disturbance treatment. Sleep disturbance was performed for three consecutive days between 23:00 and 03:00 (ZT14-ZT18) from 5 dpf to 8 dpf. (B) Zebrafish larvae (5 dpf) were extract treated with six concentrations of A. sinensis leaf tea (0.00, 0.50, 0.75, 1.00, 1.25, and 1.50 g/L; n = 48) and placed in a behavior-tracking system. (C,D) Sleep and waking activity traces (±SEM) of zebrafish larvae treated with (A) sinensis leaf tea. The gray box indicates sleep disturbance time. (300 lux light on 23:00–03:00) (n = 48). (E) Quantification of total sleep across day time (9:00–23:00), sleep disturbance time (23:00–03:00), and night time (03:00–09:00) on 6 dpf (n = 48). (F) Sleep bout number between 23:00–03:00 (n = 48). (one-way ANOVA, Tukey’s post hoc test, Different lowercase letters indicate significant differences between various concentrations).
3.3 Transcriptome sequencing of A. sinensis leaf tea treated on sleep-disturbed zebrafish
This study found that 0.75–1.50 g/L concentrations of A. sinensis leaf tea significantly improved the sleep of zebrafish under sleep-disturbed conditions. Zebrafish treated with 1.50 g/L after 3 days of treatment were sequenced using 0.00 g/L as the control (Figure 3A). Transcriptome sequencing identified 1643 differentially expressed genes (DEG, p-value ≤0.05, log2FC ≥ 1.2), of which 762 were upregulated, and 881 were downregulated (Figures 3B, C). Further, GO enrichment analysis revealed that the DEGs were associated with inflammatory response (Figures 3D, F), and KEGG enrichment revealed that more DEGs were associated with the NOD-like receptor signaling pathway (Figure 3E). These observations suggest that A. sinensis leaf tea affects the inflammatory response of zebrafish under light-induced sleep disturbance conditions to improve sleep.
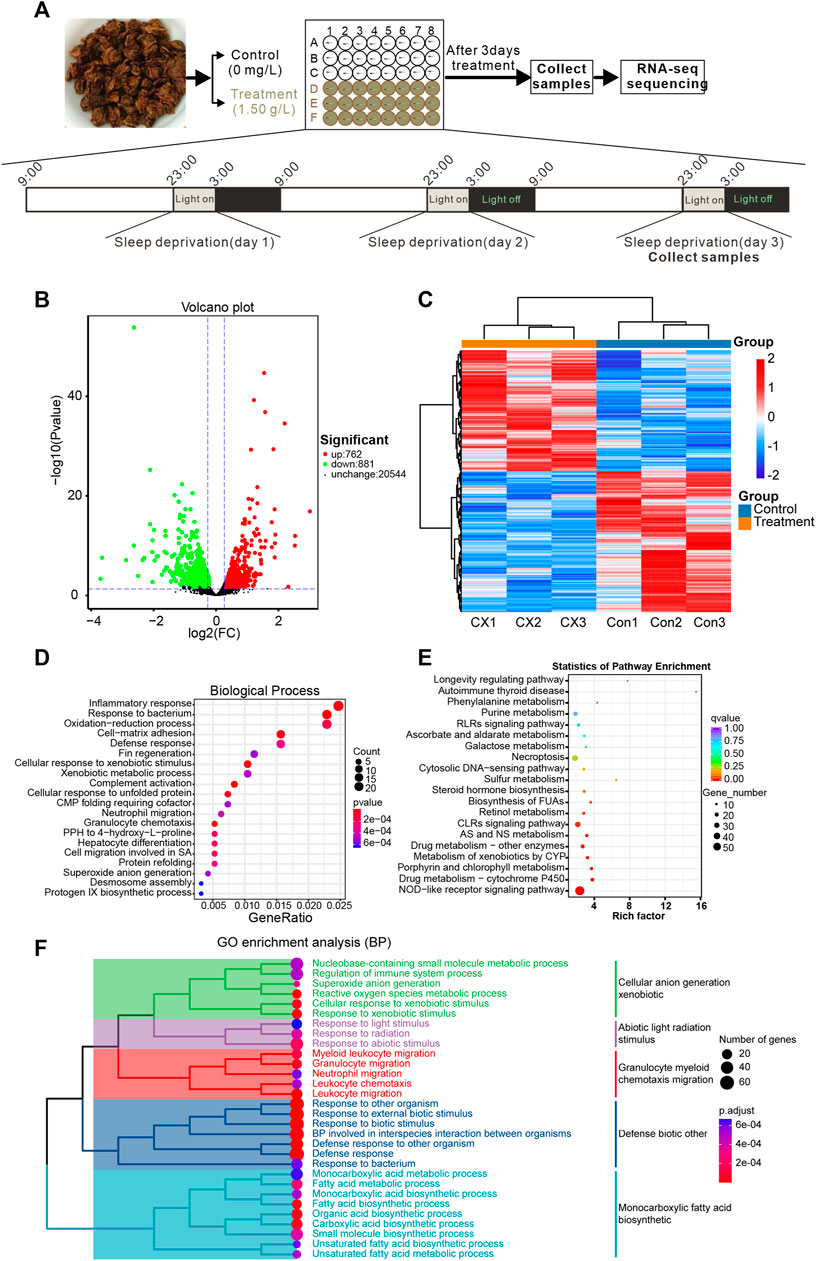
FIGURE 3. Transcriptome sequencing reveals changes in gene expression in zebrafish larvae exposed to Aquilaria sinensis leaf tea with sleep disturbance. (A) The schematic diagram shows the treatment and sample collection time of A. sinensis leaf tea-treated larvae under sleep disturbance conditions. The transcriptome of untreated larvae (0.00 g/L; Control) and larvae treated with 1.50 g/L tea (Treatment) and collected at 3:00 (ZT 18) on the third day of treatment. (B) The Volcano map displays the transcriptome sequencing results. (C) Heatmap of 1643 differentially expressed genes (p-value ≤0.05, log2FC ≥ 1.2). (D) GO enrichment analysis of the DEGs. (E) KEGG enrichment analysis showed that many genes were enriched in the NOD-like receptor. (F) The clustered heatmap of GO enrichment analysis, such as the regulation of the immune system process, myeloid leukocyte migration, granulocyte migration, neutrophil migration, leukocyte chemotaxis, and leukocyte migration.
3.4 Aquilaria sinensis leaf tea influences immune response and sleep-related genes
Further, the Cytoscape software performed a string protein-protein interaction analysis of DEGs enriched in the inflammatory response and NOD-like receptor signaling pathway (Figure 4A). This analysis identified six hub immune-related proteins from the inflammatory response and NOD-like receptor signaling pathway by protein-protein interaction of DEGs, including Nfkbiab, Tnfrsf1a, Nfkbiaa, Il1b, Traf3, and Cd40 (Figure 4B). A heatmap based on transcriptome data with the expression revealed the downregulation of genes encoding these six proteins in A. sinensis leaf tea zebrafish after treatment (Figure 4C). Further analysis of the expression levels of three sleep-related genes from transcriptome data, bhlhe41, sik3, and aanat2, revealed a downregulation of bhlhe41 in zebrafish treated 3 days with A. sinensis leaf tea compared to the control under sleep disturbance conditions (Figure 4D). No significant changes were observed in sik3 and aanat2, suggesting that A. sinensis leaf tea promotes the sleep of zebrafish under sleep-disturbed conditions by inhibiting the activity of bhlhe41.
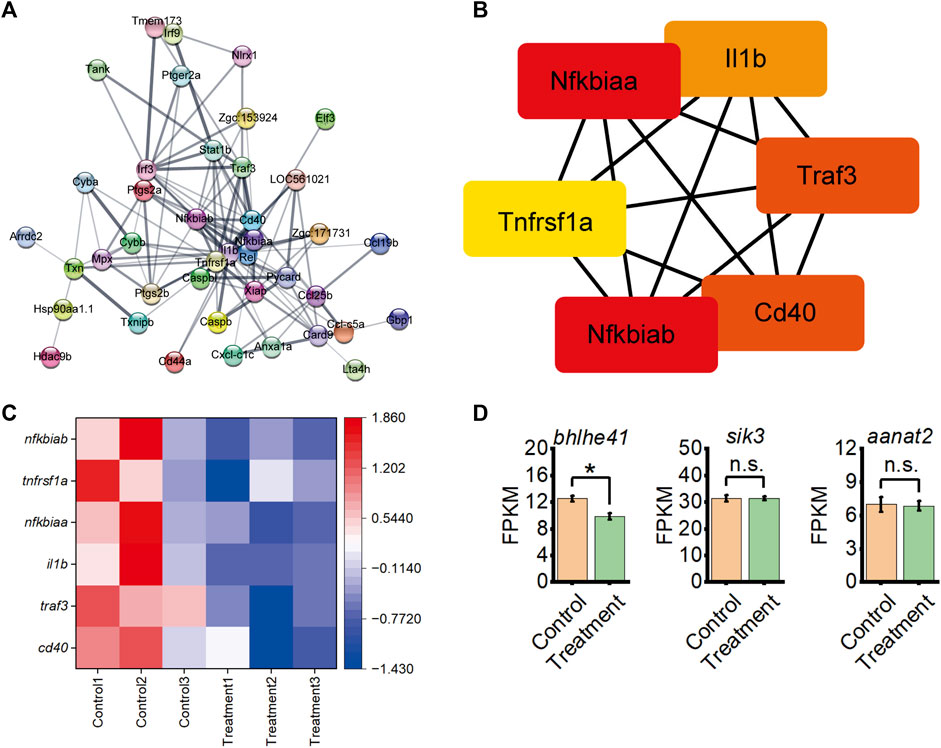
FIGURE 4. Inflammatory response interacts with the NOD-like receptor signaling pathway from DEG between control and 1.50 g/L Aquilaria sinensis leaf tea treatment groups and expression levels of sleep-related genes. (A) String analysis of DEGs associated with immune response. The thicker the lines between the proteins, the stronger the interaction. (B) Six immune-related genes were identified from String analysis. (C) Heatmap shows the expression of six immune-related genes under sleep disturbance conditions in DEGs. (D) FPKM of sleep-related genes between control and treatment group (one-way ANOVA, Tukey’s post hoc test. Data are shown as mean ± standard deviations. n.s. indicates no significance, * indicates significance at p < 0.05).
3.5 Aquilaria sinensis leaf tea affects immune-related genes and expression
Transcriptome sequencing identified that the expression levels of six immune-related genes and one sleep-related gene were significantly downregulated in larvae treated with A. sinensis leaf tea during sleep disturbance. Subsequent qRT-PCR validated these results (Figures 5A–G), indicating the reliability and repeatability of the sequencing data.
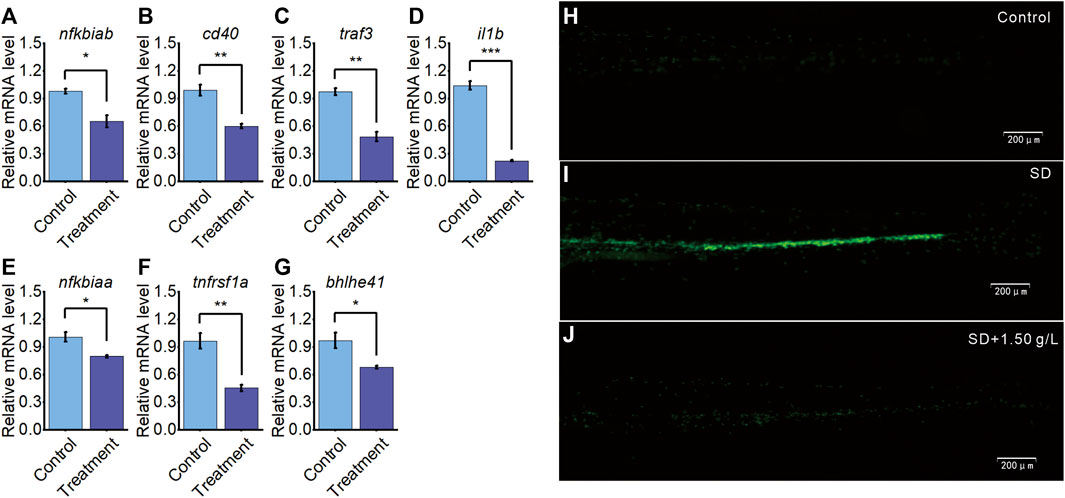
FIGURE 5. A. sinensis tea influences immune response and sleep gene on zebrafish sleep disturbed. (A–G) qRT-PCR validation of seven differentially expressed genes between the treatment group and the control group. (H) The neutrophil image of 5 dpf Tg(mpo:GFP) under normal condition (n = 30), (I) sleep disturbed condition (SD; n = 30), and (J) sleep disturbed condition with 1.50 g/L A. sinensis leaf tea treatment (n = 30) (one-way ANOVA, Tukey’s post hoc test. Data are shown as mean ± standard deviations. *: p < 0.05, **: p < 0.01, and ***: p < 0.001).
To further validate the previous findings that A. sinensis leaf tea improves immune under zebrafish sleep disturbed, the 5dpf neutrophil-specific transgenic fish, Tg(mpo:GFP), were divided into control group (no light exposed at 23:00–03:00), SD group (sleep disturbance, light exposed induced zebrafish sleep disturbed at 23:00–03:00) and SD + 1.50 g/L (sleep disturbance with 1.50 g/L of A. sinensis leaf tea). After 3 days, the SD group significantly induced neutrophil response compared with the control, and the SD + 1.50 g/L group improved neutrophils response compared with the SD group, suggesting that neutrophils migrated and aggregated significantly under sleep disturbance, A. sinensis leaf tea treated inhibit neutrophils aggregated through improves immune response (Figures 5H–J). These results further suggest that A. sinensis leaf tea improves the immune system of zebrafish under sleep disturbance conditions.
3.6 Chemical constituents of A. sinensis leaf tea and the target sites of flavonoids
The UPLC-MS/MS analysis of 1.50 g/L A. sinensis leaf tea identified 1800 chemical metabolites (Supplementary Table S2), of which flavonoids were the most abundant (335 metabolites) and accounted for 18.5% of the total components (Figure 6A). Additionally, the relative content of flavonoids was found to be the highest (21.54%; Figure 6B). Further analysis based on network pharmacology identified 70 target genes for the top 77 flavonoid metabolites in A. sinensis leaf tea. Interaction analysis showed that two out of all target genes screened from transcriptome data were the targets of flavonoids. Here, il1b was found to be the target gene of pachypodol, quercetin, isorhamnetin, cirsilineol, ayanin, tangeretin, and eupatilin, while cd40 was the target gene of myricetin, quercetin, glycitin, eupatilin, and wogonin (Figure 6C). Among these, il1b and cd40 were the common target genes of quercetin and eupatilin.
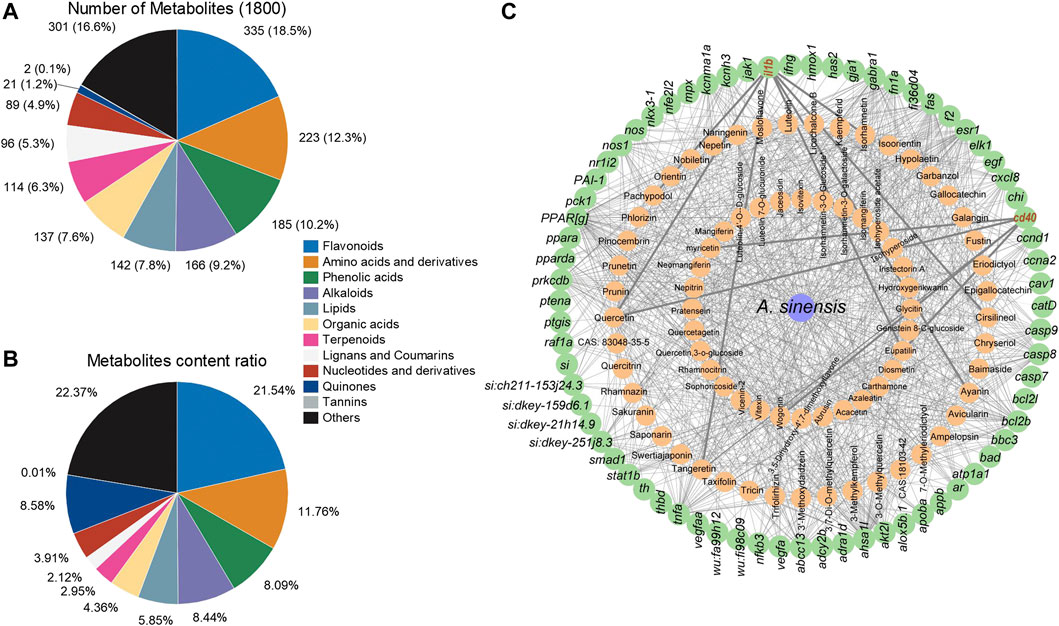
FIGURE 6. Analysis of metabolites in Aquilaria sinensis leaf tea and the gene targets of flavonoids. (A) Among 1800 metabolites were detected, 335 types of flavonoids accounted for the highest proportion. (B) The content of flavonoids obtained accounted for 21.54% in all metabolites, higher than other substances. (C) Flavonoid and target gene network (Green represents target sites and orange represents metabolites of A. sinensis).
3.7 Molecular docking of two active components from A. sinensis leaf tea
Based on the UPLC-MS/MS and network results, we screened eupatilin and quercetin (Figures 7A, G) and performed molecular docking with Cd40 and Il1b proteins that these two proteins modulate sleep. Molecular docking showed that eupatilin formed one hydrogen bond with PRO-102 in Cd40 (Figure 7B), while quercetin formed four hydrogen bonds with VAL-233, SER-234, and GLU-249 in Il1b (Figure 7H).
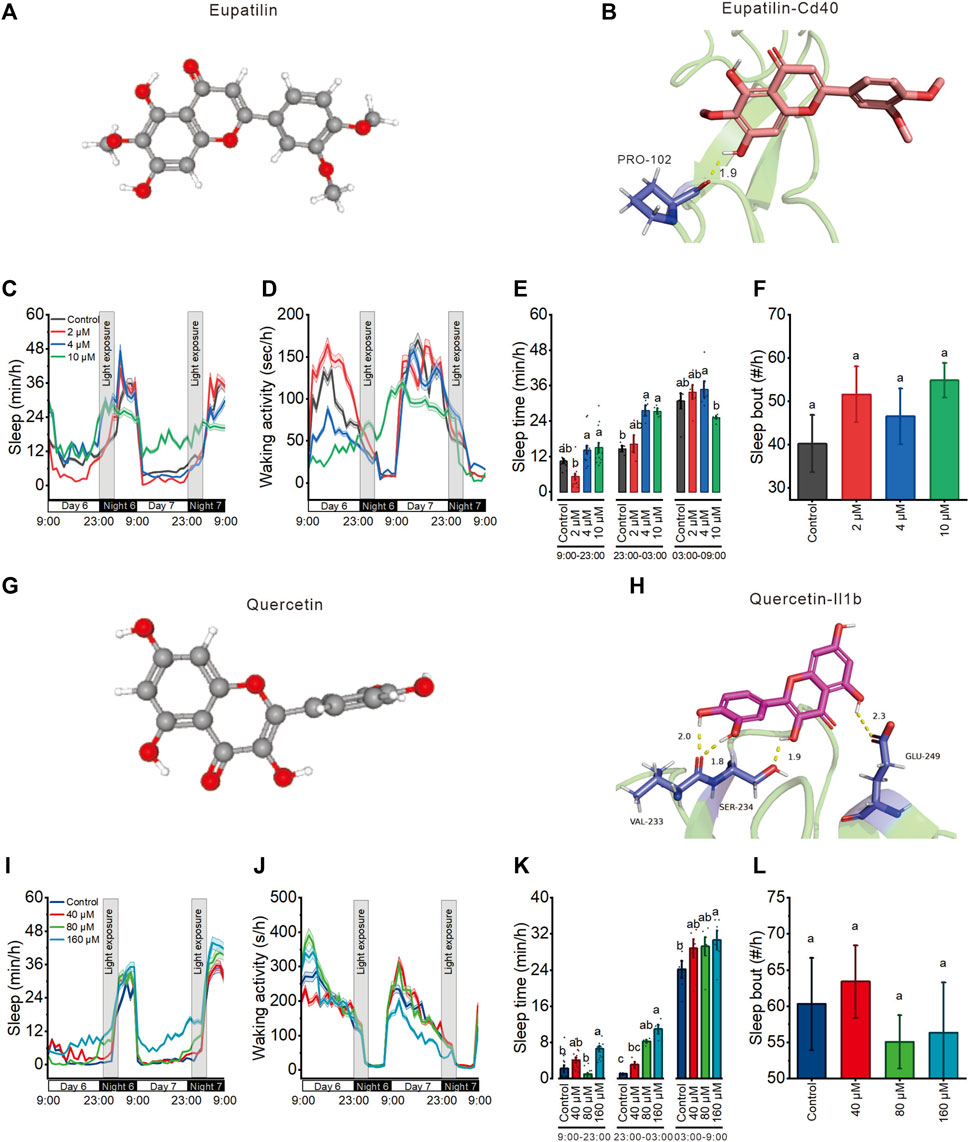
FIGURE 7. Eupatilin and quercetin, the potential active ingredients of Aquilaria sinensis leaf tea, improve the sleep of zebrafish with light-induced sleep disturbance. (A) 3D chemical structure of eupatilin based on PubChem database. (B) Molecular docking of eupatilin with Cd40. (C–D) Sleep and waking activity traces (±SEM) of zebrafish larvae treated with eupatilin. The gray box indicates sleep disturbance time (300 lux light on 23:00–03:00) (n = 48). (E) Quantification of total sleep across day time (9:00–23:00), sleep disturbance time (23:00 to 03:00), and night without sleep disturbance time (03:00–09:00) on 6 dpf in eupatilin treated and control groups (n = 48). (F) Quantification of sleep bout on sleep disturbance time between control and eupatilin treated groups (n = 48). (G) 3D chemical structure of quercetin based on PubChem database. (H) Molecular docking of quercetin with Il1b. (I–J) Sleep and waking activity traces (±SEM) of zebrafish larvae treated with quercetin (n = 48). (K) Quantification of total sleep across day time (9:00–23:00), sleep disturbance time (23:00 to 03:00), and night without sleep disturbance time (03:00–09:00) in quercetin treated and control groups on 6 dpf (n = 48). (L) Quantification of sleep bout on sleep disturbance time between control and quercetin-treated groups (n = 48). Lowercase letters indicate significant differences (one-way ANOVA, Tukey’s post hoc test. Data are shown as mean ± standard deviations. p < 0.05).
3.8 Eupatilin and quercetin are the major components of A. sinensis leaf tea that improve sleep in zebrafish with light-induced sleep disturbance
To finally investigate the effects of eupatilin and quercetin on zebrafish sleep with light-induced sleep disturbance, we treated 5 dpf larvae with these two components at three concentrations before the light-induced sleep disturbance. 4 μM and 10 μM eupatilin respectively increased sleep time during sleep disturbance time but had no influence during both day time and night time (Figures 7C–E). 80 μM and 160 μM quercetin respectively increased sleep time during sleep disturbance time but had no influence during day time, and 160 μM quercetin increased sleep at night time (Figures 7I–K) Moreover, both eupatilin and quercetin treated groups larvae exhibited no significant change in sleep bout (Figures 7F, L). These results indicate that eupatilin and quercetin are the potential active ingredients of A. sinensis leaf tea that improve zebrafish sleep under light-induced sleep disturbance.
4 Discussion
Aquilaria sinensis leaves are rich in bioactive substances (Nie et al., 2009; Qi et al., 2009; Feng and Yang, 2011; Yu et al., 2013; Yang et al., 2018) with potential biological effects. Specific studies have demonstrated that A. sinensis leaves possess anti-inflammatory, sedative, antioxidant, antimicrobial, laxative, and hypnotic effects (Hara et al., 2008; Zhou et al., 2008; Kakino et al., 2010; Li; Li et al., 2021; Liu et al., 2021). The present study investigated the effects of A. sinensis leaf tea on the sleep of zebrafish under sleep-disturbed conditions. Preliminary experiments found 3.707 g/L as the LC50 of A. sinensis leaf tea on 5 dpf zebrafish, which indicates non-toxicity according to the dose classification of acute toxicity (Huang et al., 2021). Based on this observation, a control and five concentrations of A. sinensis leaf tea were used to investigate the effects on zebrafish with disturbed sleep. Our results showed that A. sinensis leaf tea significantly promoted the sleep of zebrafish under sleep-disturbed conditions.
Sleep disturbance causes “a systemic low-grade inflammation” which is characterized by the release of cytokines and chemokines (Hurtado-Alvarado et al., 2016). Besides, animal studies have demonstrated the role of cytokines in regulating sleep (Kubota et al., 2001a; Kubota et al., 2001b; Marshall and Born, 2002; Opp, 2005; Clinton et al., 2011; Besedovsky et al., 2019). The transcriptome analysis of the present study revealed that 762 differentially expressed genes were upregulated, and 881 genes were significantly downregulated in zebrafish after A. sinensis leaf tea treatment. Most of these DEGs were associated with inflammatory response and NOD-like receptor signaling pathway. The String functional protein association network also displayed close interactions with 41 proteins, including six immune-related proteins (Nfkbiab, Tnfrsf1a, Nfkbiaa, Il1b, Traf3, and Cd40). Nfkbiab and Nfkbiaa are members of the nuclear factor of kappa light polypeptide gene enhancer in the B-cells inhibitor family, belonging to the NF-κB signaling pathway. The NF-κB signaling pathway has long been considered a typical pro-inflammatory signaling pathway (Pugaz et al., 2013). Besides, Leukin 1β (il1b) is one of the major mediators of the innate immune response and many studies found that Il1b levels were increased in human and animal models under sleep disturbance (Dinges et al., 1995; Krueger et al., 1999; Hu et al., 2003; Frey et al., 2007; Irwin et al., 2015; Lee et al., 2018). Cd40 regulation sleep through modulates genes associated with sleep homeostasis (such as Homer1a, Early growth response 2) (Gast et al., 2013; Karrer et al., 2015). Thus, the study indicates that A. sinensis leaf tea may improve sleep by affecting the inflammatory response of zebrafish under sleep disturbance.
In humans, the immune system is greatly affected by lack of sleep, a sufficient amount of sleep is associated with a reduction in neutrophils in the blood, and a lack of sleep affects the population diversity and function of circulating neutrophils in healthy adults (Christoffersson et al., 2014). The present study used the neutrophil-specific transgenic zebrafish Tg (mpo: GFP) to explore whether A. sinensis leaf tea can decrease neutrophil levels under sleep disturbance and found that neutrophils migrated and aggregated significantly under sleep disturbance. However, no significant migration of neutrophils was observed in zebrafish after A. sinensis leaf tea treatment during sleep disturbance. These results support the hypothesis that A. sinensis leaf tea could improve the immune system of zebrafish under sleep disturbance by modulating the expression of immune genes and neutrophils levels.
Metabolite profiling identified 1800 bioactive metabolites in A. sinensis leaf tea, of which flavonoids were the most abundant. Flavonoids are known to improve the immune system (Martinez et al., 2019). Min et al. also found that flavonoids constitute most of the ingredients in A. sinensis leaf extracts; however, using the UPLC-MS/MS method, they only identified 418 bioactive metabolites (Min et al., 2023). Hence, the present work identified more active ingredients of A. sinensis leaf tea using the same method. Further network and molecular docking analyses revealed that Il1b binds to quercetin and Cd40 to eupatilin. Research has shown that quercetin and quercetin 3-O-glucuronide regulate the sleep-wake cycle by activating the GABA(A) receptor and improving sleep duration and quality of insomnia mice (Kambe et al., 2010; Kim et al., 2021). Eupatilin has been shown to inhibit pancreatic cancer cells, the growth of kidney cancer cells, and the metastasis of prostate cancer cells while improving the immune system (Zhong et al., 2019; Serttas et al., 2021; Park and Kim, 2022). Our study first reports that eupatilin may improve sleep of a sleep-disturbed zebrafish, but it requires further investigation. Moreover, behavioral experiments showed that quercetin and eupatilin significantly improved sleep in zebrafish with light-induced sleep disturbance. Based on these findings, we speculate that quercetin and eupatilin play a crucial role in improving sleep in sleep-disturbance zebrafish; however, this hypothesis needs to be confirmed by further animal experiments.
5 Conclusion
Our study proved that A. sinensis leaf tea significantly improves the sleep quality of zebrafish with sleep disturbance due to light exposure. Transcriptome sequencing, qRT-PCR, and neutrophil transgenic fish imaging revealed that A. sinensis leaf tea promotes sleep through an improved immune response. Eupatilin and quercetin were identified as the potential active ingredients in A. sinensis leaf tea responsible for this effect. However, it requires further investigation and the development of natural products from leaves containing these two metabolites.
In summary, this study demonstrated that A. sinensis leaf tea promotes sleep and improves the immunity of zebrafish under light-induced sleep disturbance. These findings present a potential avenue for enhancing the sleep of individuals with sleep disturbance.
Data availability statement
The data presented in the study are deposited in the NCBI repository, accession number PRJNA1025141.
Ethics statement
The animal study was approved by the Animal Care and Use Committee of Soochow University. The study was conducted in accordance with the local legislation and institutional requirements.
Author contributions
XT: Light exposure, Sampling, Methodology (LC50), Data analysis, Writing; LW: Exposure, Sampling, Funding acquisition, Data analysis; WS: Methodology (RNA extraction), Writing; HS: Methodology (Sleep behavior); LL: Conceptualization, Review; LM and QH: Methodology (Imaging of transgenic fish); HH: Conceptualization, Review; ZZ: Conceptualization, Writing, Manuscript Review. All authors contributed to the article and approved the submitted version.
Funding
This research was funded by the Special fund project of Guangxi Subtropical Crops Research Institute (Guireyan 201408), the Special project for basic scientific research of Guangxi Academy of Agricultural Sciences (Guinongke 2024YP135 and Guinongke 2021YT143), the National Natural Science Foundation of China (81701347), the Priority Academic Program Development (PAPD) of Jiangsu Higher Education Institutions, and the Science and Technology Development Fund of Guangxi Academy of Agricultural Sciences (Guinongke 2022JM04), the Special fund project of Guangxi Subtropical Crops Research Institute (Guireyan 201304). Local science and technology development fund guided by the Central Government (GuikeZY20111007).
Acknowledgments
We thank Professor Han Wang for the helpful and constructive discussion and Ms. Ji Zhang and Ms. Jinchun Yang for raising zebrafish.
Conflict of interest
The authors declare that the research was conducted in the absence of any commercial or financial relationships that could be construed as a potential conflict of interest.
Publisher’s note
All claims expressed in this article are solely those of the authors and do not necessarily represent those of their affiliated organizations, or those of the publisher, the editors and the reviewers. Any product that may be evaluated in this article, or claim that may be made by its manufacturer, is not guaranteed or endorsed by the publisher.
Supplementary material
The Supplementary Material for this article can be found online at: https://www.frontiersin.org/articles/10.3389/fphar.2023.1246761/full#supplementary-material
References
Adam, A. Z., Lee, S. Y., and Mohamed, R. (2017). Pharmacological properties of agarwood tea derived from Aquilaria (Thymelaeaceae) leaves: an emerging contemporary herbal drink. J. Herb. Med. 10, 37–44. doi:10.1016/j.hermed.2017.06.002
Appelbaum, L., Wang, G. X., Maro, G. S., Mori, R., Tovin, A., Marin, W., et al. (2009). Sleep-wake regulation and hypocretin-melatonin interaction in zebrafish. Proc. Natl. Acad. Sci. U. S. A. 106 (51), 21942–21947. doi:10.1073/pnas.906637106
Benington, J. H., and Craig Heller, H. (1995). Restoration of brain energy metabolism as the function of sleep. Prog. Neurobiol. 45 (4), 347–360. doi:10.1016/0301-0082(94)00057-o
Besedovsky, L., Lange, T., and Haack, M. (2019). The sleep-immune crosstalk in health and disease. Physiol. Rev. 99 (3), 1325–1380. doi:10.1152/physrev.00010.2018
Cheng, J. T., Han, Y. Q., He, J., De Wu, X., Dong, L. B., Peng, L. Y., et al. (2013). Two new tirucallane triterpenoids from the leaves of Aquilaria sinensis. Arch. Pharm. Res. 36 (9), 1084–1089. doi:10.1007/s12272-013-0088-4
Christoffersson, G., Vagesjo, E., Pettersson, U. S., Massena, S., Nilsson, E. K., Broman, J. E., et al. (2014). Acute sleep deprivation in healthy young men: impact on population diversity and function of circulating neutrophils. Brain Behav. Immun. 41, 162–172. doi:10.1016/j.bbi.2014.05.010
Clinton, J. M., Davis, C. J., Zielinski, M. R., Jewett, K. A., and Krueger, J. M. (2011). Biochemical regulation of sleep and sleep biomarkers. J. Clin. Sleep. Med. 7 (5 Suppl. l), S38–S42. doi:10.5664/JCSM.1360
Dimitrov, S., Lange, T., Nohroudi, K., and Born, J. (2007). Number and function of circulating human antigen presenting cells regulated by sleep. Sleep 30 (4), 401–411. doi:10.1093/sleep/30.4.401
Dinges, D. F., Douglas, S. D., Hamarman, S., Zaugg, L., and Kapoor, S. (1995). Sleep deprivation and human immune function. Adv. Neuroimmunol. 5 (2), 97–110. doi:10.1016/0960-5428(95)00002-j
Eissa, M. A., Hashim, YZHY, Mohd Nasir, M. H., Nor, Y. A., Salleh, H. M., Isa, M. L. M., et al. (2021). Fabrication and characterization of Agarwood extract-loaded nanocapsules and evaluation of their toxicity and anti-inflammatory activity on RAW 264.7 cells and in zebrafish embryos. Drug Deliv. 28, 2618–2633. doi:10.1080/10717544.2021.2012307
Feng, J., and Yang, X. (2011). Liposolubility constituents from leaves of Aquilaria sinensis. Zhongguo Zhong Yao Za Zhi 36 (15), 2092–2095. doi:10.4268/cjcmm20111517
Frey, D. J., Fleshner, M., and Wright, K. P. (2007). The effects of 40 hours of total sleep deprivation on inflammatory markers in healthy young adults. Brain Behav. Immun. 21 (8), 1050–1057. doi:10.1016/j.bbi.2007.04.003
Gast, H., Muller, A., Lopez, M., Meier, D., Huber, R., Dechent, F., et al. (2013). CD40 activation induces NREM sleep and modulates genes associated with sleep homeostasis. Brain Behav. Immun. 27 (1), 133–144. doi:10.1016/j.bbi.2012.10.004
Han, W., and Li, X. (2012). Antioxidant activity of aloeswood tea in vitro. Spatula DD - Peer Rev. J. Complementary Med. Drug Discov. 2, 43–50. doi:10.5455/spatula.20120331054309
Hara, H., Ise, Y., Morimoto, N., Shimazawa, M., Ichihashi, K., Ohyama, M., et al. (2008). Laxative effect of agarwood leaves and its mechanism. Biosci. Biotechnol. Biochem. 72 (2), 335–345. doi:10.1271/bbb.70361
Hirayama, J., Alifu, Y., Hamabe, R., Yamaguchi, S., Tomita, J., Maruyama, Y., et al. (2019). The clock components Period2, Cryptochrome1a, and Cryptochrome2a function in establishing light-dependent behavioral rhythms and/or total activity levels in zebrafish. Sci. Rep. 9 (1), 196. doi:10.1038/s41598-018-37879-8
Hu, J., Chen, Z., Gorczynski, C. P., Gorczynski, L. Y., Kai, Y., Lee, L., et al. (2003). Sleep-deprived mice show altered cytokine production manifest by perturbations in serum IL-1ra, TNFa, and IL-6 levels. Brain, Behav. Immun. 17 (6), 498–504. doi:10.1016/j.bbi.2003.03.001
Huang, Z., Liu, F., Lu, Z., and Li, Y. (2021). Zebrafish embryo acute toxicity test method A and method B. Guangdong society of toxicology. T/GDST 1-2021.
Hurtado-Alvarado, G., Dominguez-Salazar, E., Pavon, L., Velazquez-Moctezuma, J., and Gomez-Gonzalez, B. (2016). Blood-Brain barrier disruption induced by chronic sleep loss: low-grade inflammation may Be the link. J. Immunol. Res. 2016, 4576012. doi:10.1155/2016/4576012
Irwin, M. R., Witarama, T., Caudill, M., Olmstead, R., and Breen, E. C. (2015). Sleep loss activates cellular inflammation and signal transducer and activator of transcription (STAT) family proteins in humans. Brain Behav. Immun. 47, 86–92. doi:10.1016/j.bbi.2014.09.017
Kakino, M., Tazawa, S., Maruyama, H., Tsuruma, K., Araki, Y., Shimazawa, M., et al. (2010). Laxative effects of agarwood on low-fiber diet-induced constipation in rats. BMC Complement. Altern. Med. 10, 68. doi:10.1186/1472-6882-10-68
Kambe, D., Kotani, M., Yoshimoto, M., Kaku, S., Chaki, S., and Honda, K. (2010). Effects of quercetin on the sleep-wake cycle in rats: involvement of gamma-aminobutyric acid receptor type A in regulation of rapid eye movement sleep. Brain Res. 1330, 83–88. doi:10.1016/j.brainres.2010.03.033
Kamonwannasit, S., Nantapong, N., Kumkrai, P., Luecha, P., Kupittayanant, S., and Chudapongse, N. (2013). Antibacterial activity of Aquilaria crassna leaf extract against Staphylococcus epidermidis by disruption of cell wall. Ann. Clin. Microbiol. Antimicrob. 12, 20. doi:10.1186/1476-0711-12-20
Karrer, M., Lopez, M. A., Meier, D., Mikhail, C., Ogunshola, O. O., Muller, A. F., et al. (2015). Cytokine-induced sleep: neurons respond to TNF with production of chemokines and increased expression of Homer1a in vitro. Brain Behav. Immun. 47, 186–192. doi:10.1016/j.bbi.2014.11.008
Kim, S., Hong, K. B., Jo, K., Suh, H. J., Kang, D., et al. (2021). Attenuation effect of radiofrequency irradiation on UV-B-induced skin pigmentation by decreasing melanin synthesis and through upregulation of heat shock protein 70. Molecules 26 (10), 7648. doi:10.3390/molecules26247648
Krueger, J. M., Obal, F., and Fang, J. (1999). Why we sleep: a theoretical view of sleep function. Sleep. Med. Rev. 3 (2), 119–129. doi:10.1016/s1087-0792(99)90019-9
Kubota, T., Brown, R. A., Fang, J., and Krueger, J. M. (2001a). Interleukin-15 and interleukin-2 enhance non-REM sleep in rabbits. Am. J. Physiol. Regul. Integr. Comp. Physiol. 281 (3), R1004–R1012. doi:10.1152/ajpregu.2001.281.3.R1004
Kubota, T., Fang, J., Brown, R. A., and Krueger, J. M. (2001b). Interleukin-18 promotes sleep in rabbits and rats. Am. J. Physiol. Regul. Integr. Comp. Physiol. 281 (3), R828–R838. doi:10.1152/ajpregu.2001.281.3.R828
Lee, D. A., Andreev, A., Truong, T. V., Chen, A., Hill, A. J., Oikonomou, G., et al. (2017). Genetic and neuronal regulation of sleep by neuropeptide VF. Elife 6, e25727. doi:10.7554/eLife.25727
Lee, M. K., Park, J. H., Gi, S. H., and Hwang, Y. S. (2018). IL-1β induces fascin expression and increases cancer invasion. Anticancer Res. 38 (11), 6127–6132. doi:10.21873/anticanres.12964
Li, H. (2013). Comparison of the pharmacological effectes between leaves of Aquilaria sinensis(lour.) gilg and the lignum of Aquilaria sinensis(lour.) gilg. Guangzhou university of chinese Medicine, 23–25.
Li, W., Chen, H. Q., Wang, H., Mei, W. L., and Dai, H. F. (2021). Natural products in agarwood and Aquilaria plants: chemistry, biological activities and biosynthesis. Nat. Prod. Rep. 38 (3), 528–565. doi:10.1039/d0np00042f
Li, X., Wei, S., Niu, S., Ma, X., Li, H., Jing, M., et al. (2022). Network pharmacology prediction and molecular docking-based strategy to explore the potential mechanism of Huanglian Jiedu Decoction against sepsis. Comput. Biol. Med. 144, 105389. doi:10.1016/j.compbiomed.2022.105389
Liu, W., Ding, H., Li, M., and Lu, S. (2021). Research progress and development of agarwood leaves. Guangdong Chem. Ind. 48 (24), 79–80. doi:10.3969/j.issn.1007-1865.2021.24.032
Mackiewicz, M., Shockley, K. R., Romer, M. A., Galante, R. J., Zimmerman, J. E., Naidoo, N., et al. (2007). Macromolecule biosynthesis: a key function of sleep. Physiol. Genomics 31 (3), 441–457. doi:10.1152/physiolgenomics.00275.2006
Marshall, L., and Born, J. (2002). Brain-immune interactions in sleep. Int. Rev. Neurobiol. 52, 93–131. doi:10.1016/s0074-7742(02)52007-9
Martinez, G., Mijares, M. R., and De Sanctis, J. B. (2019). Effects of flavonoids and its derivatives on immune cell responses. Recent Pat. Inflamm. Allergy Drug Discov. 13 (2), 84–104. doi:10.2174/1872213X13666190426164124
Min, L., Wang, G., Tong, X., Yang, H., Sun, H., Zhang, Z., et al. (2023). Feeding aquilaria sinensis leaves modulates lipid metabolism and improves the meat quality of goats. Foods 12 (3), 560. doi:10.3390/foods12030560
Morris, G. M., Huey, R., Lindstrom, W., Sanner, M. F., Belew, R. K., Goodsell, D. S., et al. (2009). AutoDock4 and AutoDockTools4: automated docking with selective receptor flexibility. J. Comput. Chem. 30 (16), 2785–2791. doi:10.1002/jcc.21256
Naziz, P. S., Das, R., and Sen, S. (2019). The scent of stress: evidence from the unique fragrance of agarwood. Front. Plant Sci. 10, 840. doi:10.3389/fpls.2019.00840
Nie, C., Song, Y., Chen, D., Xue, P., Tu, P., Wang, K., et al. (2009). Studies on chemical constituents of leaves of Aquilaria sinensis. China Journal of Chinese Materia Medica 34 (7), 858–860. doi:10.3321/j.issn:1001-5302.2009.07.014
Opp, M. R. (2005). Cytokines and sleep. Sleep. Med. Rev. 9 (5), 355–364. doi:10.1016/j.smrv.2005.01.002
Pang, K. L., Chin, K. Y., and Nirwana, S. I. (2023). Immunomodulatory effects of agarwood leaf extract on RAW264.7 murine macrophages. Endocr. Metab. Immune Disord. Drug Targets 23 (7), 964–976. doi:10.2174/1871530323666230103153134
Park, T. H., and Kim, H. S. (2022). Eupatilin suppresses pancreatic cancer cells via glucose uptake inhibition, AMPK activation, and cell cycle arrest. Anticancer Res. 42 (1), 483–491. doi:10.21873/anticanres.15506
Prober, D. A., Rihel, J., Onah, A. A., Sung, R. J., and Schier, A. F. (2006). Hypocretin/orexin overexpression induces an insomnia-like phenotype in zebrafish. J. Neurosci. 26 (51), 13400–13410. doi:10.1523/JNEUROSCI.4332-06.2006
Pugazhenthi, S., Zhang, Y., Bouchard, R., and Mahaffey, G. (2013). Induction of an inflammatory loop by interleukin-1β and tumor necrosis factor-α involves NF-kB and STAT-1 in differentiated human neuroprogenitor cells. PLoS One 8 (7), e69585. doi:10.1371/journal.pone.0069585
Qi, J., Lu, J. J., Liu, J. H., and Yu, B. Y. (2009). Flavonoid and a rare benzophenone glycoside from the leaves of Aquilaria sinensis. Chem. Pharm. Bull. (Tokyo) 57 (2), 134–137. doi:10.1248/cpb.57.134
Renshaw, S. A., Loynes, C. A., Trushell, D. M., Elworthy, S., Ingham, P. W., and Whyte, M. K. (2006). A transgenic zebrafish model of neutrophilic inflammation. Blood 108 (13), 3976–3978. doi:10.1182/blood-2006-05-024075
Rihel, J., Prober, D. A., Arvanites, A., Lam, K., Zimmerman, S., Jang, S., et al. (2010). Zebrafish behavioral profiling links drugs to biological targets and rest/wake regulation. Science 327 (5963), 348–351. doi:10.1126/science.1183090
Seeliger, D., and de Groot, B. L. (2010). Ligand docking and binding site analysis with PyMOL and Autodock/Vina. J. Comput. Aided Mol. Des. 24 (5), 417–422. doi:10.1007/s10822-010-9352-6
Serttas, R., Koroglu, C., and Erdogan, S. (2021). Eupatilin inhibits the proliferation and migration of prostate cancer cells through modulation of PTEN and NF-κB signaling. Anticancer Agents Med. Chem. 21 (3), 372–382. doi:10.2174/1871520620666200811113549
Surjanto, S., Batubara, R., and Rangkuti, D. S. (2019). Safety test of agarwood leaves tea (aquilaria malaccencis lamk.) through skin sensitization test on albino rabbit. Open Access Maced. J. Med. Sci. 7 (22), 3896–3899. doi:10.3889/oamjms.2019.528
Tran, S., and Prober, D. A. (2022). Validation of candidate sleep disorder risk genes using zebrafish. Front. Mol. Neurosci. 15, 873520. doi:10.3389/fnmol.2022.873520
VanGuilder, H. D., Vrana, K. E., and Freeman, W. M. (2008). Twenty-five years of quantitative PCR for gene expression analysis. Biotechniques 44 (5), 619–626. doi:10.2144/000112776
Varela, M., Figueras, A., and Novoa, B. (2017). Modelling viral infections using zebrafish: innate immune response and antiviral research. Antivir. Res. 139, 59–68. doi:10.1016/j.antiviral.2016.12.013
Yang, M. X., Liang, Y. G., Chen, H. R., Huang, Y. F., Gong, H. G., Zhang, T. Y., et al. (2018). Isolation of flavonoids from wild aquilaria sinensis leaves by an improved preparative high-speed counter-current chromatography apparatus. J. Chromatogr. Sci. 56 (1), 18–24. doi:10.1093/chromsci/bmx076
Yehuda, S., Sredni, B., Carasso, R. L., and Kenigsbuch-Sredni, D. (2009). REM sleep deprivation in rats results in inflammation and interleukin-17 elevation. J. Interferon Cytokine Res. 29 (7), 393–398. doi:10.1089/jir.2008.0080
Yokogawa, T., Marin, W., Faraco, J., Pezeron, G., Appelbaum, L., Zhang, J., et al. (2007). Characterization of sleep in zebrafish and insomnia in hypocretin receptor mutants. PLoS Biol. 5 (10), e277. doi:10.1371/journal.pbio.0050277
Yu, Q., Qi, J., Yu, H. X., Chen, L. L., Kou, J. P., Liu, S. J., et al. (2013). Qualitative and quantitative analysis of phenolic compounds in the leaves of aquilaria sinensis using liquid chromatography-mass spectrometry. Phytochem. Anal. 24 (4), 349–356. doi:10.1002/pca.2416
Yuan, H., Zeng, X., Shi, J., Xu, Q., Wang, Y., Jabu, D., et al. (2018). Time-course comparative metabolite profiling under osmotic stress in tolerant and sensitive Tibetan hulless barley. Biomed. Res. Int. 2018, 9415409. doi:10.1155/2018/9415409
Zager, A., Andersen, M. L., Ruiz, F. S., Antunes, I. B., and Tufik, S. (2007). Effects of acute and chronic sleep loss on immune modulation of rats. Am. J. Physiol. Regul. Integr. Comp. Physiol. 293 (1), R504–R509. doi:10.1152/ajpregu.00105.2007
Zhong, W., Wu, Z., Chen, N., Zhong, K., Lin, Y., Jiang, H., et al. (2019). Eupatilin inhibits renal cancer growth by downregulating MicroRNA-21 through the activation of YAP1. Biomed. Res. Int. 2019, 5016483. doi:10.1155/2019/5016483
Keywords: light exposure, sleep disturbance, Aquilaria sinensis, zebrafish, immune system
Citation: Tan X, Wang L, Smith WK, Sun H, Long L, Mao L, Huang Q, Huang H and Zhong Z (2023) Aquilaria sinensis leaf tea affects the immune system and increases sleep in zebrafish. Front. Pharmacol. 14:1246761. doi: 10.3389/fphar.2023.1246761
Received: 24 June 2023; Accepted: 02 November 2023;
Published: 16 November 2023.
Edited by:
Giustino Orlando, University of Studies G. d’Annunzio Chieti and Pescara, ItalyReviewed by:
Xizeng Feng, Nankai University, ChinaGrigorios Oikonomou, California Institute of Technology, United States
Copyright © 2023 Tan, Wang, Smith, Sun, Long, Mao, Huang, Huang and Zhong. This is an open-access article distributed under the terms of the Creative Commons Attribution License (CC BY). The use, distribution or reproduction in other forums is permitted, provided the original author(s) and the copyright owner(s) are credited and that the original publication in this journal is cited, in accordance with accepted academic practice. No use, distribution or reproduction is permitted which does not comply with these terms.
*Correspondence: Zhaomin Zhong, emhvbmd6aGFvbWluQHN1ZGEuZWR1LmNu
†These authors have contributed equally to this work