- Center for Addiction Sciences and Therapeutics and Department of Pharmacology and Toxicology, John Sealy School of Medicine, University of Texas Medical Branch, Galveston, TX, United States
Opioid misuse and opioid-involved overdose deaths are a massive public health problem involving the intertwined misuse of prescription opioids for pain management with the emergence of extremely potent fentanyl derivatives, sold as standalone products or adulterants in counterfeit prescription opioids or heroin. The incidence of repeated opioid overdose events indicates a problematic use pattern consistent with the development of the medical condition of opioid use disorder (OUD). Prescription and illicit opioids reduce pain perception by activating µ-opioid receptors (MOR) localized to the central nervous system (CNS). Dysregulation of meso-corticolimbic circuitry that subserves reward and adaptive behaviors is fundamentally involved in the progressive behavioral changes that promote and are consequent to OUD. Although opioid-induced analgesia and the rewarding effects of abused opioids are primarily mediated through MOR activation, serotonin (5-HT) is an important contributor to the pharmacology of opioid abused drugs (including heroin and prescription opioids) and OUD. There is a recent resurgence of interest into psychedelic compounds that act primarily through the 5-HT2A receptor (5-HT2AR) as a new frontier in combatting such diseases (e.g., depression, anxiety, and substance use disorders). Emerging data suggest that the MOR and 5-HT2AR crosstalk at the cellular level and within key nodes of OUD circuitry, highlighting a major opportunity for novel pharmacological intervention for OUD. There is an important gap in the preclinical profiling of psychedelic 5-HT2AR agonists in OUD models. Further, as these molecules carry risks, additional analyses of the profiles of non-hallucinogenic 5-HT2AR agonists and/or 5-HT2AR positive allosteric modulators may provide a new pathway for 5-HT2AR therapeutics. In this review, we discuss the opportunities and challenges associated with utilizing 5-HT2AR agonists as therapeutics for OUD.
1 Introduction
Opioid-involved overdose deaths remain at crisis levels in the United States (Ciccarone and Shoptaw, 2022; Ahmad et al., 2023). Initially attributable to overprescription of opioid analgesics for pain control, the overdose crisis is now amplified by deaths involving synthetic opioids in the illicit drug supply (Humphreys et al., 2022; Humphreys and Shover, 2023). Pain management practices evolved to reduce opioid-involved overdoses (Bruera and Del Fabbro, 2018; Friedman et al., 2020), however, a substantial proportion of the population remains at risk for overdose and the evolution to opioid use disorder (OUD) (Vowles et al., 2015; Volkow and McLellan, 2016). A debilitating condition with significant health consequences (Hasin et al., 2013), ∼2.7 million people aged 12 or older in the United States were diagnosed with OUD in 2020 (SAMHSA, 2021). The diagnosis of substance use disorders (SUDs), including OUD, in the United States is currently based upon the Diagnostic and Statistical Manual of Mental Disorders-5 (DSM-5) which defines symptoms (e.g., taking more drug than intended, inability to stop, intense craving, withdrawal/tolerance, unsuccessful efforts to control use, etc.) on a continuum from mild (2–3 symptoms) to moderate (4–5 symptoms) to severe (6–11 symptoms) (Hasin et al., 2013). The International Statistical Classification of Diseases and Related Health Problems-10 (ICD-10) criteria align in general with DSM-5 (Hasin et al., 2013). OUD may develop at opioid doses employed in prescription pain management (McAuliffe, 2013; Minozzi et al., 2013; Campbell et al., 2015; Degenhardt et al., 2021), although the burden of prescription OUD in pain patients is difficult to estimate (Guillou-Landreat et al., 2021; Hasin et al., 2022; Sullivan and Ballantyne, 2022).
The multitude of opioid compounds include opioid poppy alkaloids (e.g., morphine), semi-synthetic (e.g., heroin) and fully synthetic opioids (e.g., fentanyl and fentanyl analogs) which bind to the µ-opioid receptor (MOR), a G protein-coupled receptor (GPCR). Agonist-induced activation of the MOR mitigates pain and evokes intense and pleasurable euphoria, which in turn contributes to the evolution of OUD (Le Merrer et al., 2009; Baldo, 2016; Higginbotham et al., 2022). The activation of MOR triggers G protein coupling to intracellular signal transduction mechanisms in cells of the peripheral and central nervous system (CNS); for instance, MOR-activated Gαi/o inhibits adenylyl cyclase release, calcium- and voltage-gated channels to hyperpolarize and suppress neuronal activity (Higginbotham et al., 2022). The MOR also signals from both membrane and intracellular localizations to bias distinct downstream effector pathways which may differentially drive on- and off-target effects (Stoeber et al., 2018; Pineyro and Nagi, 2021). Chronic opioid exposure results in a myriad of cellular adaptations and plasticity in neural systems that contribute to the development and maintenance of OUD and chronic pain syndromes (Christie et al., 1992; Williams et al., 2001; Christie, 2008; O'Brien, 2009; Puretic and Demarin, 2012; Pitchers et al., 2014).
The MOR is also the target for medications for OUD (MOUD) which are effective in treating OUD, managing craving and withdrawal, as well as reducing overdoses (Volkow and McLellan, 2016; Wakeman et al., 2020; Brandt et al., 2023). However, the MOUD medications buprenorphine and methadone are partial and full MOR agonists, respectively, and are subject to abuse, misuse and diversion (Koob and Volkow, 2016; Chilcoat et al., 2019; Strang et al., 2020), prompting the field to mine mechanistic targets outside of the opioid system for novel medications for OUD (Coussens et al., 2019; Marek et al., 2019; Rasmussen et al., 2019). An area of intense interest is the prospect that psychedelics may be harnessed for the treatment of OUD (DiVito and Leger, 2020; Koslowski et al., 2021; Barnett and Weleff, 2022; Urban et al., 2023) and pain conditions (Zia et al., 2023).
Psychedelics include a large group of natural and synthetic compounds (Nichols, 2016) that preferentially bind to the serotonin (5-hydroxytryptamine; 5-HT) 5-HT2A receptor (5-HT2AR) to alter sensory perceptions and cognitive processes in humans (Quednow et al., 2012; Kometer et al., 2013; Holze et al., 2021a; Becker et al., 2023). Intriguingly, the “classical” psychedelic d-lysergic acid diethylamide (LSD) was reported to evoke effective analgesia, likely linked to altered pain perception (Kast and Collins, 1964; Ramaekers et al., 2021). Furthermore, analyses of anecdotal and self-reported data support the consideration of psychedelics for treatment of OUD (Savage and McCabe, 1973; Garcia-Romeu et al., 2019; Argento et al., 2022; Jones et al., 2022), and several clinical trials with psilocybin are ongoing for OUD (e.g., NCT0416066, NCT0542029, NCT06005662) and pain conditions (e.g., NCT06001749, NCT05224336, NCT05068791). MOR function is fundamentally integrated with 5-HT neurotransmission (Tao et al., 1998; Tao and Auerbach, 2002b; Tao et al., 2003; Ozdemir, 2017) with a prominent role for the 5-HT2AR (Aira et al., 2012; Heijmans et al., 2021; Li et al., 2021; Sierra et al., 2022), from the 5-HT2R family (5-HT2AR, 5-HT2BR, 5-HT2CR) (Barnes et al., 2021). As opposed to the MOR, the coupling of the 5-HT2AR to Gαq/11 heterotrimeric G proteins triggers phosphatidylinositol-4,5-bisphosphate hydrolysis and subsequent release of inositol triphosphate, diacylglycerol, and other effectors (Barnes et al., 2021). Both the 5-HT2AR (Barnes et al., 2021) and MOR (Pineyro and Nagi, 2021) interact directly with intracellular β-arrestins to control signaling and membrane trafficking which is driven by a diversity of cellular signaling factors. The MOR and 5-HT2AR co-express in individual neurons that mediate aspects of nociception and OUD processes (Lopez-Gimenez et al., 2008; Aira et al., 2012). Co-activation of the 5-HT2AR in heterologous cells is permissive in evoking MOR cellular localization and function (Lopez-Gimenez et al., 2008), actions which may contribute to 5-HT-mediated outcomes upon opioid exposure in vivo (Tao et al., 1998; Harris and Aston-Jones, 2001; Singh et al., 2003).
Much remains to be learned about cellular and circuit interactions between MOR and 5-HT2AR, and the renewed interest in psychedelics for their potential therapeutic efficacy in neuropsychiatric disorders (McClure-Begley and Roth, 2022; Slocum et al., 2022) premises the goal to explore the engagement of 5-HT2AR processes in OUD. In the current quest to develop non-opioid medications for OUD, psychedelics may provide value as medication candidates. The goal of the present review is to explore interactions between opioids and psychedelics as well as the opportunities and challenges for pain and OUD therapeutics.
2 Psychedelic pharmacology
Psychedelics derived from plants and fungi have served significant spiritual and medical purposes since ancient times (Griffiths and Grob, 2010; Doblin et al., 2019; Bouso and Sanchez-Aviles, 2020). Three main structural classes of serotonergic hallucinogens include ergolines (e.g., LSD), tryptamines (e.g., psilocybin, converted by the body to the active molecule psilocin) and phenethylamines [e.g., 2,5-dimethoxy-4-methylamphetamine (DOM), 2,5-dimethoxy-4-iodophenyl]-2-aminopropane (DOI)]. These compounds share close structural similarity to the endogenous tryptamine 5-HT (Gaddum and Hameed, 1954) which spurred the discovery that 5-HT2AR agonist efficacy is a key mechanism of action for this class of compounds to evoke overlapping and powerful subjective effects in humans (Nichols and Walter, 2021), although psychedelic pharmacology is complex and nuanced (Howell and Cunningham, 2015; Castellanos et al., 2020; McClure-Begley and Roth, 2022; Wulff et al., 2023). While the presumed abuse liability and limited medical value historically restricted clinical studies of psychedelics (Henningfield et al., 2022; Hendricks et al., 2023), 5-HT2AR neurotransmission was established to mediate hallucinations in schizophrenia and Parkinson’s disease psychosis (Schmidt et al., 1995; Ballanger et al., 2010; Hacksell et al., 2014; Cho et al., 2017). With nanomolar potency as a 5-HT2AR inverse agonist/antagonist and selectivity for the 5-HT2AR over other GPCRs (Vanover et al., 2006), pimavanserin blocked hallucinations and delusions in refractory schizophrenia (Nasrallah et al., 2019) and Parkinson’s disease patients (Meltzer et al., 2010), and is now approved for treatment for the latter indication (Hacksell et al., 2014; Davis et al., 2021). Although the efficacy of pimavanserin to suppress psychedelic-induced hallucinations is not yet reported, the 5-HT2R antagonist ketanserin (Brogden and Sorkin, 1990) reversed the subjective effects (e.g., visual/auditory alterations) of LSD (Preller et al., 2017; Preller et al., 2019; Pokorny et al., 2020; Holze et al., 2021b; Becker et al., 2023) and psilocybin in humans (Quednow et al., 2012; Kometer et al., 2013). It is also important to recognize that the functional network mechanisms underlying psychedelic-vs. disease-evoked hallucinations are distinguishable (Rolland et al., 2014; Leptourgos et al., 2020; Silverstein and Lai, 2021).
Preclinical model systems are essential in linking 5-HT2AR functionality with hallucinogenesis, particularly the rodent head twitch response (HTR). The HTR is a rapid, rotational head movement evoked by LSD and other hallucinogens which is eliminated in constitutive 5-HT2AR knockout mice (Gonzalez-Maeso et al., 2007; Halberstadt and Geyer, 2010). The 5-HT2AR antagonist M100907 (MDL100907; volinanserin) exhibits ∼100-fold selectivity at the 5-HT2AR versus other monoamine GPCRs (Palfreyman et al., 1993; Kehne et al., 1996; Casey et al., 2022) and blocks psychedelic-evoked HTRs (Canal et al., 2013; Fink et al., 2015; Halberstadt et al., 2020). Importantly, the potency of LSD to evoke the HTR correlates positively with its potency to evoke psychoactivity in humans (Corne and Pickering, 1967), providing a rodent “proxy” for hallucinogenic actions. Direct cortical 5-HT2AR activation induces the HTR in rats (Willins and Meltzer, 1997) while the subjective response to psilocybin positively correlated with neocortical 5-HT2AR occupancy (Madsen et al., 2019), supporting a critical role of cortical 5-HT2AR in psychedelic mechanisms of action.
The case for the role of the 5-HT2AR in the mechanisms of action of psychedelics is strong, but we would be remiss to overlook the fact that distinct in vitro and in vivo profiles are recognized across psychedelic molecules which variably bind to multiple 5-HT receptors, dopamine D1 receptor (D1R) and D2R, as well as other brain-localized targets (Howell and Cunningham, 2015; Castellanos et al., 2020; McClure-Begley and Roth, 2022; Wulff et al., 2023). For instance, LSD potently binds to human 5-HT2AR but also 5-HT1AR, 5-HT2CR, D2R and with some affinity for α adrenergic, D1R and D3R (Rickli et al., 2015; Rickli et al., 2016). LSD exhibits distinct stimulus effects mediated by the 5-HT2AR (Cunningham et al., 1985; Nielsen et al., 1985; Cunningham and Appel, 1987; Cunningham and Appel, 1988; Baker, 2018), while lisuride, non-hallucinogenic congener of LSD, only partially substituted for LSD or DOI in drug discrimination analyses and is proposed to engage dopaminergic D2R and 5-HT1AR signaling (Callahan and Appel, 1990; Marona-Lewicka et al., 2002). Further, the recent discovery that LSD and psilocybin bind to the tropomyosin receptor kinase B receptor for brain-derived neurotrophic factor suggests new complexity to the actions of these intriguing compounds (Moliner et al., 2023).
Some serotonergic hallucinogens exhibit high affinity for both the 5-HT2AR and 5-HT2CR which colocalize in many of the same brain regions and are reported to interact at the cellular level in vitro (Moutkine et al., 2017; Felsing et al., 2018), in rat medial prefrontal cortex (mPFC) ex vivo (Price et al., 2019), and in vivo preclinical models (Pockros et al., 2012; Burton et al., 2013; Cunningham et al., 2013; Anastasio et al., 2015; Bazovkina et al., 2015; Moutkine et al., 2017) to control neural bases for several behaviors (Cunningham and Anastasio, 2014; Howell and Cunningham, 2015). A modulatory role for the 5-HT2CR over psychedelic actions is suggested to contribute to some behavioral outcomes in animals (Krebs-Thomson et al., 1998; Smith et al., 2003; Krebs-Thomson et al., 2006), while a functional interaction between the 5-HT2AR and 5-HT2CR is proposed to mediate in vivo effects of psilocybin in mice (Erkizia-Santamaria et al., 2022). Of note, endogenous 5-HT binds to the transmembrane domains that comprise the 5-HT2AR orthosteric site which shares ∼80% homology with the 5-HT2BR and 5-HT2CR (Barnes et al., 2021). Agonist actions at the 5-HT2BR cause cardiac valvulopathy and pulmonary hypertension, as seen with the anti-obesity medication fenfluramine (Rothman and Baumann, 2009; Barnes et al., 2021). LSD and psilocin, the active metabolite of psilocybin, exhibit similar affinity at the 5-HT2AR and 5-HT2BR in vitro (Porter et al., 1999), but neither are projected to pose risk for valvulopathy, particularly on acute dosing (Tagen et al., 2023). However, given the critical liability of off-target 5-HT2BR agonist actions (Horvath et al., 2004; Hutcheson et al., 2011), current efforts to identify 5-HT2AR-selective agonists as novel chemical entities require 5-HT2BR affinity and efficacy screening early in molecule discovery (Cao et al., 2022; Chen et al., 2023; Cunningham et al., 2023).
3 Abuse liability of MOR agonists vs. psychedelics
The effectiveness of MOR agonists for pain management is complicated by its powerful efficacy to evoke euphoric and pleasurable effects which are at the core of their abuse liability. The FDA guidelines define abuse liability as the probability that a psychoactive drug will sustain patterns of non-medical self-administration, drug-seeking and craving, a triad likely to result in undesirable health consequences (Carter and Griffiths, 2009; Leiderman, 2009; Comer et al., 2012; Calderon et al., 2015). The capacity of a drug to maintain self-administration under experimental conditions in animals aligns well with the likelihood of abuse by humans (Panlilio et al., 2005; Sughondhabirom et al., 2005; Calderon et al., 2015; Calderon et al., 2023). This model system is based upon the operant construct that a psychoactive drug serves as a positive rewarding stimulus which increases the prospect of a behavioral response (e.g., lever press) to gain drug access (Morse and Skinner, 1958). This assay has high predictive validity in assessing the abuse liability of novel drugs and in aligning molecular and neurobiological adaptations associated with repeated drug self-intake in rodents (O'Connor et al., 2011; Belin-Rauscent and Belin, 2012; Cunningham and Anastasio, 2014; Howell and Cunningham, 2015; Neelakantan et al., 2017; Spanagel, 2017; Ezeomah et al., 2020; Merritt et al., 2022) and humans (Jones and Comer, 2013; Stoops, 2022). Self-administration models are widely deployed to identify the primary role for MOR mechanisms in the rewarding properties of opioid agonists (e.g., morphine, heroin, fentanyl) which are prevented by MOR antagonists naloxone or naltrexone (Koob et al., 1984; Beardsley et al., 2004; Leri and Burns, 2005; Vendruscolo et al., 2018). In contrast to MOR agonists, psychedelics [e.g., DOI, DOM, N,N-dimethyltryptamine (DMT), mescaline, psilocybin] exhibit limited reinforcing effects. At best, psychedelics support transient or sporadic drug-taking in self-administration assays (Fantegrossi et al., 2004a; Fantegrossi et al., 2004b; Maguire et al., 2013; Goodwin, 2016; Johnson et al., 2018), although intermittent-access self-administration may be useful to further interrogate psychedelic abuse liability (Goodwin, 2016). Of note, near complete tolerance to psychedelics (e.g., LSD) occurs under several dosing paradigms in humans (Belleville et al., 1956; Rosenberg et al., 1963) and animals (Trulson and Jacobs, 1977; Schlemmer et al., 1986; Buchborn et al., 2015), perhaps accounting for the limited repetitive abuse liability of psychedelics (Belleville et al., 1956).
Only a handful of studies explored the efficacy of psychedelics to alter self-administration of opioid analgesics. The psychedelic DOM attenuated heroin self-administration, resulting in a downward shift of the heroin dose-response curve in one study (Maguire et al., 2013); however, in a more recent study deployed in a sucrose versus drug choice procedure, DOM did not impact fentanyl intake, but did decrease completed choice trials and response rates (Maguire, 2023). Employing a behavioral economics variant of the self-administration paradigm which estimates unique descriptors of opioid demand as a function of its “price,” DOI decreased the motivation to consume fentanyl and total fentanyl consumption in a 5-HT2AR-dependent manner (i.e., blocked by M100907) (Martin et al., 2021). While initial research may support the potential usage of psychedelics to reduce the abuse liability and intake of opioids, future studies are necessary to disentangle the key mechanistic facets of psychedelics that impact opioid self-administration. That said, 5-HT2AR agonist psychedelics are thought to maintain a large margin of safety and a low level of toxicity (Nichols, 2016; Sakloth et al., 2019), particularly in contrast to opioid agonists (Von Korff et al., 2011; Jeffery et al., 2020; Preuss et al., 2023).
A complementary model to assess abuse liability in keeping with FDA guidelines (Calderon et al., 2015; FDA, 2017; Calderon et al., 2023) is the drug discrimination paradigm which is robust, sensitive and exhibits predictive validity (Appel et al., 1982; McMahon, 2015; Bolin et al., 2018). This assay involves training subjects through differential reinforcement to recognize the stimulus effects of a psychoactive drug versus no (or another) drug. Useful in establishing whether novel CNS-active compounds are predicted to have abuse liability (Soto et al., 2019; Wild et al., 2019; Wold et al., 2020; Stoops, 2022), this assay is an indirect measure of reinforcing efficacy with remarkable pharmacological specificity and utility in assessing in vivo pharmacology in humans and animals (Jones and Comer, 2013; McMahon, 2015; Bolin et al., 2018; Stoops, 2022). Drug discrimination analyses established deep knowledge of the neural mechanisms underlying the interoceptive effects of MOR agonists (Beardsley et al., 2004; Meert and Vermeirsch, 2005; Schwienteck et al., 2019; Walker et al., 2021), which are blocked by naloxone or naltrexone (Beardsley et al., 2004; Flynn and France, 2021), albeit larger doses are required to block the long-acting MOR agonist carfentanil (Flynn and France, 2021). Drug discrimination assays also detailed a complex interaction between MOR agonists with kappa and delta opioid receptor agonists and mixed agonists (Picker, 1997; Yadlapalli et al., 2018).
In contrast to the limited psychedelic intake seen in self-administration models, animals readily learn to discriminate the interoceptive effects of hallucinogens (e.g., LSD, DOM, DOI, and others) from no drug (e.g., saline) and cross-substitution occurs across this class of drugs (Appel et al., 1982; Appel and Cunningham, 1986; Fiorella et al., 1995b; a;Baker, 2018; Bolin et al., 2018; Heal et al., 2018). The effective doses of hallucinogens in drug discrimination analyses correlate significantly with their potency to evoke hallucinations in humans, HTRs in rodents as well as affinity as 5-HT2AR agonists (Glennon et al., 1983; Sadzot et al., 1989; Nelson et al., 1999; Halberstadt et al., 2020). Notably, for a series of 5-HT2R antagonists tested, the dose that blocked 50% of the stimulus effects of LSD correlated significantly with the affinity for the 5-HT2AR, but not the 5-HT2CR (Nielsen et al., 1985; Fiorella et al., 1995b). This 5-HT2AR-dominant mechanism occurs within the 30-min pretreatment timeframe with 5-HT2AR antagonists, while a late-stage (90 min) of the stimulus effects of LSD involve dopamine D2R properties (Marona-Lewicka et al., 2002). There is conjecture that these time-dependent effects in drug discrimination may align with the transition from the psychedelic effects to aversive aspects of the experience (Marona-Lewicka et al., 2002; Zamberlan et al., 2018). Interestingly, a recent analysis speculated that opioid receptors may be involved in the reported subjective effects of psychedelics (Zamberlan et al., 2018).
4 The 5-HT2AR as a target for opioid use disorder
Opioid signaling, particularly through the MOR, is mechanistically implicated in the rewarding effects of abused opioids (Koob et al., 1984; Negus et al., 1993) as well as pain relief in rodents (King et al., 2011; Okun et al., 2011; Navratilova et al., 2012) and humans (Becerra and Borsook, 2008), effects mediated by meso-corticolimbic circuitry (Strang et al., 2020). These actions in circuitry are ultimately dependent upon the selectivity of opioid compounds for MOR, potency, half-life, pharmacokinetics and specific pharmacological profiles (Vearrier and Grundmann, 2021). With continuing abuse in vulnerable individuals, initially impulsive opioid use evolves to compulsive use which reflects direct and indirect actions and adaptations within neurocircuitry (Madden et al., 1997; Bakhshipour-Rudsari and Karimpour-Vazifehkhorani, 2021; Tolomeo et al., 2021; Peck et al., 2022). The evolution from initial opioid use to diagnosis of OUD involves altered biological homeostasis, rendering behavior increasingly resistant to change, even in the face of the negative consequences (Koob and Volkow, 2016; Strang et al., 2020). An overactive incentive-motivational system centered in ventral tegmental area (VTA) dopamine (DA) neurons and its neural projections to the nucleus accumbens (NAc) serves as the “accelerator” (reward, persistent drug-induced behaviors) (Figure 1). Subregions of the prefrontal cortex (PFC) play both direct and subtle regulatory roles as a “behavioral brake” over drug use (inhibitory control, executive function) and is vulnerable to disruption by chronic opioid exposure leading to heightened preoccupation and anticipation of drug acquisition (Ujcikova et al., 2021; Ceceli et al., 2023). A well-characterized role for the 5-HT2AR in meso-corticolimbic neurocircuitry serves to illustrate the power and complexity of this system (Figure 1).
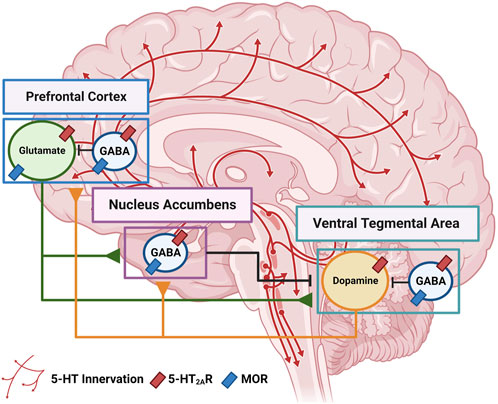
FIGURE 1. Cellular distribution of 5-HT2AR and MOR within meso-corticolimbic circuitry derived primarily from rodent studies. Serotonergic axons from DRN (red arrows) innervate the circuit. The VTA consists of locally projecting GABA interneurons (blue) and dopaminergic neurons (orange) that project to the NAc and PFC. In the PFC, GABAergic interneurons (blue) project locally while pyramidal glutamatergic neurons in the PFC (green) project to NAc and VTA. The NAc is comprised of GABA medium spiny neurons (blue) that project to VTA and other structures not shown. Figure created with BioRender.com.
A large population (50%–66%) of PFC pyramidal neurons express the 5-HT2AR (Blue et al., 1988; Miner et al., 2003; Santana et al., 2004) within layer V pyramidal neurons (Pompeiano et al., 1994; Lopez-Gimenez et al., 2001; Amargos-Bosch et al., 2004; Price et al., 2019) which affords stimulatory glutamate drive to the VTA DA neurons (Vazquez-Borsetti et al., 2009; Vazquez-Borsetti et al., 2011) and NAc (Mocci et al., 2014), indirectly enhancing mesolimbic DA neurotransmission. Intriguingly, PFC axons preferentially contact VTA DA neurons that project back to the mPFC (Carr and Sesack, 2000). Systemic administration or direct infusion of the 5-HT2AR agonist and hallucinogen DOI into the medial PFC (mPFC) not only evokes the HTR (Willins and Meltzer, 1997), but also increases the rate of firing of VTA DA neurons (Bortolozzi et al., 2005) with both outcomes blocked by the selective 5-HT2AR antagonist M100907 (Willins and Meltzer, 1997; Bortolozzi et al., 2005). Intra-mPFC infusion of M100907 blocked DOI-evoked mesocortical DA release in the VTA and DA release in the mPFC of the same rats, supporting the clarification that 5-HT2AR activation of corticotegmental glutamate projections synapse on mesocortical DA neurons (Pehek et al., 2006). Also, systemic DOI increased glutamate release in the VTA, which was blocked by intracortical administration of M100907 (Pehek et al., 2006). Thus, the 5-HT2AR in PFC projections increases stimulatory drive on the VTA, explaining the observed 5-HT2AR-stimulated DA release in the PFC (Bortolozzi et al., 2005; Pehek et al., 2006). Of note, this multi-synaptic template is likely to have additional elements, since approximately 50% of the 5-HT2AR-expressing pyramidal neurons that synapse in the VTA also synapse in the dorsal raphe nucleus (DRN), the major contributor to serotonergic innervation of PFC (Vazquez-Borsetti et al., 2009) with broad opportunities for modified 5-HT2AR control of meso-corticolimbic pathways involved in opioid actions. Further, activation of the 5-HT2AR is essential for induction of cortical neuroplasticity, giving rise to the hypothesis that 5-HT2AR agonists hold promise to “liberate” cortical activity as a therapeutic approach (Ly et al., 2018). Future studies should explore the nature of “corrective” neuroplasticity (as may occur with psychedelics) vs. “deranged” neuroplasticity (noted as decreased function of normal reward and cognitive function in OUD) to understand vulnerability to continued drug use and relapse vulnerability (Koob and Le Moal, 2005).
Opioid and 5-HT2AR biology intersect at multiple circuit and cellular levels (Barnes et al., 2021; Heijmans et al., 2021; Sasaki et al., 2021). Systemic administration of the MOR agonists morphine and fentanyl acutely raises extracellular 5-HT levels in the DRN and areas of the forebrain that receive innervation from 5-HT DRN efferents (i.e., PFC, striatum, amygdala, etc.) (Tao and Auerbach, 1995; 2002a; Tao et al., 2003) (Figure 1). Interestingly, this effect wanes upon repeated exposure to opioid agonists indicating a reduced responsivity of 5-HT signaling with chronic opioid use (Tao et al., 1998). Extracellular 5-HT in the raphe is also significantly diminished during opioid withdrawal which likely contributes to abstinence evoked phenomena (Tao et al., 1998). Further, functional crosstalk between the MOR and 5-HT2AR was suggested to regulate opioid-mediated abuse liability (Marek, 2003; Lopez-Gimenez et al., 2008; Mohammadi et al., 2016; Pang et al., 2016; Neelakantan et al., 2017; Sierra et al., 2022). Morphine and other opioids suppress LSD-evoked HTRs, most likely through 5-HT2AR agonist actions (Corne et al., 1963; Vetulani et al., 1980). The selective 5-HT2AR antagonist M100907 augments the antinociceptive effects of the MOR agonist oxycodone in a sex-specific manner in mice supporting a role for 5-HT2AR mechanisms in MOR evoked analgesia (Sierra et al., 2022). The psychedelics DOM and quipazine (de la Fuente Revenga et al., 2021) decreased the discriminative stimulus effects of morphine in monkeys in a 5-HT2AR-dependent manner (Li et al., 2011). Nonetheless, the exact mechanistic nature of this interaction between 5-HT2AR and MOR systems is difficult to disentangle based upon current knowledge, but we propose the convergent effects of the 5-HT2AR and opioid systems may occur at the level of the mPFC (Figure 1) (Willins and Meltzer, 1997).
Signaling through MOR VTA and NAc are well studied (Trigo et al., 2010; Fields and Margolis, 2015), and the MOR also localizes in high density to the PFC (Tempel and Zukin, 1987; Mansour et al., 1988; Mansour et al., 1995) (Figure 1) which is highlighted as a “hot spot” for dysregulated motivational function and opioid-mediated behaviors (Baldo, 2016). The MOR localizes in γ-aminobutyric acid (GABA) interneurons in the neocortex and is proposed to disinhibit glutamate-driven outflow to its terminal fields (Taki et al., 2000; Ferezou et al., 2007). A subset of cortical glutamate neurons is also reported to express MOR (Birdsong et al., 2019; Zhang et al., 2020; Du et al., 2022). Opportunistically, the 5-HT2AR localizes within these key regions (Figure 1) (Liu et al., 2014; Howell and Cunningham, 2015; Browne et al., 2019; Cunningham et al., 2020; Nagai et al., 2020). Intriguingly, repeated morphine treatment of rats elevates 5-HT2AR protein levels within the PFC as assessed ex vivo (Pang et al., 2016), while altered 5-HT2AR-mediated Gαq/11 activation was observed in the dorsolateral PFC dissected from postmortem brains of OUD patients (Odagaki et al., 2021), suggesting that the 5-HT2AR in PFC plays a role in the modulation of processes involved in OUD.
Cellular colocalization of 5-HT2AR and MOR transcripts was observed in layer V of rat neocortex (Lopez-Gimenez et al., 2008), although the resident cell type remains to be described. MOR agonists suppress 5-HT2AR agonist-mediated excitation of PFC pyramidal neurons (Marek and Aghajanian, 1998). Along these lines, in the presence of 5-HT2AR expression, upregulation of MOR levels was observed, and co-addition of 5-HT plus morphine resulted in rapid desensitization, internalization and downregulation of MOR in cellular models; these effects were not observed in the absence of 5-HT2AR transfection (Lopez-Gimenez et al., 2008). As morphine-induced MOR desensitization was demonstrated to maintain potent analgesic efficacy without leading to antinociceptive tolerance (Kim et al., 2008), co-activation of 5-HT2AR and MOR may provide an opportunity for opioid-sparing therapy for conditions of chronic opioid pain management or to reduce the likelihood of OUD development. Taken together, these behavioral and cellular observations putatively support a cooperative MOR and 5-HT2AR regulation of outflow of the PFC (Marek and Aghajanian, 1998) to control output of OUD neurocircuitry, and premise future studies to further clarify this hypothesis.
5 Novel perspectives in 5-HT2AR molecule discovery
Largely based on the historical bias that all psychedelics evoke hallucinations and have abuse potential (and remain in Schedule I), there is an important gap in the preclinical profiling of 5-HT2AR agonists in OUD models. New chemical biology initiatives are underway to identify novel 5-HT2AR agonist-targeted molecules with distinctive profiles, perhaps lacking hallucinogenic effects, as seen with structural congeners the hallucinogen LSD and non-hallucinogen lisuride (White and Appel, 1982; White, 1986; Gonzalez-Maeso et al., 2003). In particular, there is intense investment in characterizing the promise of hallucinogenic and non-hallucinogenic 5-HT2AR agonists for medications development for OUD and other SUDs (DiVito and Leger, 2020; Koslowski et al., 2021; Barnett and Weleff, 2022; Jones and Nock, 2022; Urban et al., 2023). One such effort focused on ibogaine, an indole alkaloid derived from the evergreen shrub Tabernanthe iboga (Jenks, 2002). Ibogaine is a molecule with affinity for opioid receptors which is reported to decrease opioid withdrawal symptoms (Parke et al., 2002; Mash, 2023) and alleviate drug cravings (Mash et al., 2016; Mash et al., 2018; Noller et al., 2018; Mash, 2023). The hallucinogen ibogaine has a complex mechanism of action (Mash et al., 1995a; Mash et al., 1995b) and an unacceptable safety profile (Koenig et al., 2014; Thurner et al., 2014), but recently served as a promising starting point for new chemical entities (Cameron et al., 2021). The synthesis of ibogaine analogs resulted in identification of tabernanthalog (TBG) which exhibits a better preclinical safety profile than ibogaine (Cameron et al., 2021). The TBG binding profile is, however, complex as multiple receptor systems are involved in its pharmacology, including the 5-HT2AR, 5-HT2CR as well as other monoamine receptors, the 5-HT transporter and monoamine oxidase (Cameron et al., 2021). This unique molecule did not evoke HTRs in rodents, suggesting the possibility that TBG serves an example of a “non-hallucinogenic” derivative which is effective in reducing alcohol and heroin intake in rodents, but not sucrose ingestion, effects blocked by ketanserin (Cameron et al., 2021; Heinsbroek et al., 2023).
An historically interesting compound is BOL-148 (2-bromo-LSD) (Troxler and Hofmann, 1957; Lewis et al., 2023) which was initially identified in the 1950s as lacking hallucinogenic effects (Turner et al., 1959; Karst et al., 2010). BOL-148, long recognized for its inability to evoke HTRs (Corne and Pickering, 1967), blocked the psychological effects of LSD in a small human study (Ginzel and Mayer-Gross, 1956). More recent studies validated that BOL-148 lacks efficacy to evoke HTRs but does exhibit an antidepressant-like profile in mice, and 5-HT2AR-dependent neuronal plasticity (Lewis et al., 2023). An additional molecule, Ariadne (4-methyl-2,5-dimethoxy-alpha-ethylphenethylamine) did not evoke classical psychedelic effects in humans (Shulgin and Shulgin, 1991) nor the HTR in mice (Cunningham et al., 2023), but drug discrimination analyses demonstrated substitution for the empathogen 3,4-methylenedioxymethamphetamine (Glennon, 1993) and LSD (Winter, 1980). Thus, Ariadne exhibits an interesting behavioral profile as a unique non-hallucinogenic molecule possibly distinguished from classical hallucinogens at the level of restricted 5-HT2AR signaling efficacy (Cunningham et al., 2023).
These advances are key steps toward our future understanding of 5-HT2AR signaling specificity as well as the requirements for maximizing psychedelics for therapeutic efficacy and rationally designing such analogs (Ly et al., 2018; Olson et al., 2018; Cameron et al., 2021; Dong et al., 2021; Cao et al., 2022; Cunningham et al., 2023). Additionally, molecular studies of ligand-activated GPCRs and GPCR-transducer complexes are expanding our understanding of how 5-HT2AR activation leads to biased and unbiased signal transduction (Hilger et al., 2018; Kim et al., 2020). As the science of 5-HT2AR molecular signaling advances to define hallucinogenic versus non-hallucinogenic 5-HT2AR agonists, future studies will allow interrogation of how 5-HT2AR agonists with distinct profiles of biased signaling efficacy may impact opioid-evoked behaviors and their relative propensity to correct opioid-mediated neuronal dysfunction.
Selective 5-HT2AR activation may be useful in combating impaired serotonergic control that contributes to vulnerability to pain conditions and OUD. As noted, selective agonist targeting of the 5-HT2AR and/or 5-HT2CR is challenged by the high sequence homology with the 5-HT2BR at the 5-HT orthosteric binding pocket at which repeated activation can cause valvulopathy and pulmonary hypertension (Fitzgerald et al., 2000). While activation of the 5-HT2CR may be a benefit for pain management and/or treatment of OUD (Nakai et al., 2010; Neelakantan et al., 2017; Ma et al., 2019; Rasmussen et al., 2019; Higgins et al., 2020), there are on-target side effects such as hypophagia and anhedonia (Lam et al., 2008; Yamamoto et al., 2017) as is the case for 5-HT2AR agonists (hallucinations) that may limit their clinical practicality (Shalit et al., 2019; Olson, 2021). In addition to direct agonist actions at GPCRs, allosteric modulation is an alternate strategy to design ligands that do not directly engage the orthosteric binding pocket, but rather modulate the function of the GPCR. A positive allosteric modulator (PAM) can increase receptor functional responses and/or the intrinsic affinity for the orthosteric ligand through binding to sites on GPCRs that are topographically distinct from the orthosteric site targeted by endogenous (i.e., 5-HT) and synthetic agonists (Lindsley et al., 2016; Wold et al., 2019a). Several medicinal chemistry campaigns reported 5-HT2CR PAMs with pharmacological profiles different from agonists (Garcia-Carceles et al., 2017; Wold et al., 2019b; Singh et al., 2019; Wild et al., 2019; Wold et al., 2020; Chen et al., 2023). In this light, oleamide is an intriguing example of an endogenous lipid in mammals (Arafat et al., 1989; Cravatt et al., 1995) that controls behavior (Hedlund et al., 2003; Soria-Gomez et al., 2010; Prospero-Garcia et al., 2016; Mendez-Diaz et al., 2019) and activates 5-HT2AR signaling (Cao et al., 2022), but non-selectively (Thomas et al., 1997; Boger et al., 1998; Cheer et al., 1999; Hedlund et al., 1999; Fedorova et al., 2001). A recent synthetic campaign resulted in a series of oleamide-based analogues characterized as selective 5-HT2CR PAMs or dual 5-HT2CR/5-HT2AR PAMs (Chen et al., 2023), as seen with another the natural product derivative PNU-69176E (Im et al., 2003; Ding et al., 2012). In the process, CTW0404 and CTW0419 were identified as potential 5-HT2AR PAMs which exhibit distinct pharmacological profiles relative to 5-HT2AR agonists (WO2023023287A1) (Zhou et al., 2023). These new discoveries allow for future studies to explore the possibility that 5-HT2AR PAMs may be an alternative strategy to engage a subset of 5-HT2AR-mediated biological actions distinct from psychedelic 5-HT2AR agonists.
6 Conclusion
Medications that are FDA-approved for OUD are available, however even when utilized, OUD patients maintain a high propensity for relapse and overdose death (Brandt et al., 2023) and suffer adverse side effects on current MOUD (FDA, 2018). Here, we reviewed evidence for 5-HT2AR agonists as novel therapeutics for OUD and propose potential next steps in the elucidation of the complex interactions occurring between 5-HT2AR and MOR systems. The behavioral evidence implicates the potential of functional MOR and 5-HT2AR interactions in regions controlling opioid-evoked behavioral outcomes and MOR and 5-HT2AR are known to colocalize within nodes of OUD circuitry. However, the degree to which cellular and signaling dynamics mediate crosstalk between the MOR and 5-HT2AR in the mPFC and how these may be modulated by opioids or exploited by psychedelics remains unresolved. Notably, we focus here on MOR to the exclusion of kappa, delta and nociceptin receptors which are involved in pain (Chu Sin Chung and Kieffer, 2013; Zaveri, 2016; Snyder et al., 2018; Quirion et al., 2020) and OUD (Butelman et al., 2012; Chu Sin Chung and Kieffer, 2013; Valentino and Volkow, 2018; Ubaldi et al., 2021) and could play a role in the psychomimetic effects of psychedelics (Clark and Abi-Dargham, 2019). Further, while classical psychedelics that act as 5-HT2AR agonists are showing promise for treatment of facets of OUD, these molecules carry risks (abuse, side effects) and may not be a viable option for many patients. Thus, further analyses of the profiles of non-hallucinogenic 5-HT2AR agonists and/or 5-HT2AR PAMs provides a new frontier to further characterize the therapeutic potential of the 5-HT2AR. Finally, as discussed above psychedelics exhibit a promiscuous pharmacology (e.g., 5-HT2CR affinity and efficacy) and how these “off-target” effects contribute to therapeutic efficacy is an important question for the field at large as is a greater knowledge of the interactions between psychedelics and the opioid system.
Author contributions
All authors listed have made a substantial, direct, and intellectual contribution to the work and approved it for publication.
Funding
This work was supported in part by R01DA038446, T32DA07287, UG3DA052282, and UG3DA050317 from the National Institutes of Health, the Chauncey Leake Distinguished Professor Endowment and the Center for Addiction Sciences and Therapeutics at UTMB.
Conflict of interest
The authors declare that the research was conducted in the absence of any commercial or financial relationships that could be construed as a potential conflict of interest.
Publisher’s note
All claims expressed in this article are solely those of the authors and do not necessarily represent those of their affiliated organizations, or those of the publisher, the editors and the reviewers. Any product that may be evaluated in this article, or claim that may be made by its manufacturer, is not guaranteed or endorsed by the publisher.
Abbreviations
5-HT, serotonin; 5-HT2AR, 5-HT2A receptor; BOL-148, 2-bromo-LSD; CNS, central nervous system; D1R, dopamine 1 receptor; D2R, dopamine 2 receptor; DA, dopamine; DMT, N,N-dimethyltryptamine; DOI, 1-[2,5-dimethoxy-4-iodophenyl]-2-aminopropane; DOM, 1-(2,5-dimethoxy-4-methylphenyl)-2-aminopropane; DRN, dorsal raphe nucleus; GABA, γ-aminobutyric acid; GPCR, G protein-coupled receptor; HTR, head twitch response; LSD, d-lysergic acid diethylamide; MOR, µ-opioid receptor; MOUD, medication for opioid use disorder; mPFC, medial prefrontal cortex; NAc, nucleus accumbens; OUD, opioid use disorder; PFC, prefrontal cortex; TBG, tabernanthalog; VTA, ventral tegmental area.
References
Ahmad, F. B., Cisewski, J. A., Rossen, L. M., and Sutton, P. (2023). Provisional drug overdose death counts. Available: https://www.cdc.gov/nchs/nvss/vsrr/drug-overdose-data.htm (Accessed April 2023.
Aira, Z., Buesa, I., Del Cano, G. G., Salgueiro, M., Mendiable, N., Mingo, J., et al. (2012). Selective impairment of spinal mu-opioid receptor mechanism by plasticity of serotonergic facilitation mediated by 5-HT2A and 5-HT2B receptors. Pain 153, 1418–1425. doi:10.1016/j.pain.2012.03.017
Amargos-Bosch, M., Bortolozzi, A., Puig, M. V., Serrats, J., Adell, A., Celada, P., et al. (2004). Co-expression and in vivo interaction of serotonin1A and serotonin2A receptors in pyramidal neurons of prefrontal cortex. Cereb. Cortex. 14, 281–299. doi:10.1093/cercor/bhg128
Anastasio, N. C., Stutz, S. J., Fink, L. H., Swinford-Jackson, S. E., Sears, R. M., Dileone, R. J., et al. (2015). Serotonin (5-HT) 5-HT2A receptor (5-HT2AR):5-HT2CR imbalance in medial prefrontal cortex associates with motor impulsivity. ACS Chem. Neurosci. 6, 1248–1258. doi:10.1021/acschemneuro.5b00094
Appel, J. B., and Cunningham, K. A. (1986). The use of drug discrimination procedures to characterize hallucinogenic drug actions. Psychopharmacol. Bull. 22, 959–967.
Appel, J. B., White, F. J., and Holohean, A. M. (1982). Analyzing mechanism(s) of hallucinogenic drug action with drug discrimination procedures. Neurosci. Biobehav Rev. 6, 529–536. doi:10.1016/0149-7634(82)90036-7
Arafat, E. S., Trimble, J. W., Andersen, R. N., Dass, C., and Desiderio, D. M. (1989). Identification of fatty acid amides in human plasma. Life Sci. 45, 1679–1687. doi:10.1016/0024-3205(89)90278-6
Argento, E., Socias, M. E., Hayashi, K., Choi, J., Mackay, L., Christie, D., et al. (2022). Psychedelic use is associated with reduced daily opioid use among people who use illicit drugs in a Canadian setting. Int. J. Drug Policy 100, 103518. doi:10.1016/j.drugpo.2021.103518
Baker, L. E. (2018). Hallucinogens in drug discrimination. Curr. Top. Behav. Neurosci. 36, 201–219. doi:10.1007/7854_2017_476
Bakhshipour-Rudsari, A., and Karimpour-Vazifehkhorani, A. (2021). The role of impulsivity and sensitivity to reward in dropout of addiction treatment in heroin addicts. Addict. Health 13, 45–51. doi:10.22122/ahj.v13i1.290
Baldo, B. A. (2016). Prefrontal cortical opioids and dysregulated motivation: A network hypothesis. Trends Neurosci. 39, 366–377. doi:10.1016/j.tins.2016.03.004
Ballanger, B., Strafella, A. P., Van Eimeren, T., Zurowski, M., Rusjan, P. M., Houle, S., et al. (2010). Serotonin 2A receptors and visual hallucinations in Parkinson disease. Arch. Neurol. 67, 416–421. doi:10.1001/archneurol.2010.35
Barnes, N. M., Ahern, G. P., Becamel, C., Bockaert, J., Camilleri, M., Chaumont-Dubel, S., et al. (2021). International Union of Basic and Clinical Pharmacology. CX. Classification of Receptors for 5-hydroxytryptamine; Pharmacology and Function. Pharmacol. Rev. 73, 310–520. doi:10.1124/pr.118.015552
Barnett, B. S., and Weleff, J. (2022). Psychedelics in the treatment of substance use disorders. Psychiatr. Ann. 52, 365–370. doi:10.3928/00485713-20220804-01
Bazovkina, D. V., Kondaurova, E. M., Naumenko, V. S., and Ponimaskin, E. (2015). Genotype-dependent difference in 5-HT2C receptor-induced hypolocomotion: Comparison with 5-HT2A receptor functional activity. Neural Plast. 2015, 846589. doi:10.1155/2015/846589
Beardsley, P. M., Aceto, M. D., Cook, C. D., Bowman, E. R., Newman, J. L., and Harris, L. S. (2004). Discriminative stimulus, reinforcing, physical dependence, and antinociceptive effects of oxycodone in mice, rats, and rhesus monkeys. Exp. Clin. Psychopharmacol. 12, 163–172. doi:10.1037/1064-1297.12.3.163
Becerra, L., and Borsook, D. (2008). Signal valence in the nucleus accumbens to pain onset and offset. Eur. J. Pain 12, 866–869. doi:10.1016/j.ejpain.2007.12.007
Becker, A. M., Klaiber, A., Holze, F., Istampoulouoglou, I., Duthaler, U., Varghese, N., et al. (2023). Ketanserin reverses the acute response to LSD in a randomized, double-blind, placebo-controlled, crossover study in healthy participants. Int. J. Neuropsychopharmacol. 26, 97–106. doi:10.1093/ijnp/pyac075
Belin-Rauscent, A., and Belin, D. (2012). “Animal models of drug addiction,” in Addictions. Editor D Belin. IntechOpen.
Belleville, R. E., Fraser, H. F., Isbell, H., Logan, C. R., and Wikler, A. (1956). Studies on lysergic acid diethylamide (LSD-25). I. Effects in former morphine addicts and development of tolerance during chronic intoxication. AMA Arch. Neurol. Psychiatry 76, 468–478.
Birdsong, W. T., Jongbloets, B. C., Engeln, K. A., Wang, D., Scherrer, G., and Mao, T. (2019). Synapse-specific opioid modulation of thalamo-cortico-striatal circuits. Elife 8, e45146. doi:10.7554/eLife.45146
Blue, M. E., Yagaloff, K. A., Mamounas, L. A., Hartig, P. R., and Molliver, M. E. (1988). Correspondence between 5-HT2 receptors and serotonergic axons in rat neocortex. Brain Res. 453, 315–328. doi:10.1016/0006-8993(88)90172-2
Boger, D. L., Patterson, J. E., and Jin, Q. (1998). Structural requirements for 5-HT2A and 5-HT1A serotonin receptor potentiation by the biologically active lipid oleamide. Proc. Natl. Acad. Sci. U. S. A. 95, 4102–4107. doi:10.1073/pnas.95.8.4102
Bolin, B. L., Alcorn, J. L., Reynolds, A. R., Lile, J. A., Stoops, W. W., and Rush, C. R. (2018). Human drug discrimination: elucidating the neuropharmacology of commonly abused illicit drugs. Curr. Top. Behav. Neurosci. 39, 261–295. doi:10.1007/7854_2016_10
Bortolozzi, A., Az-Mataix, L., Scorza, M. C., Celada, P., and Artigas, F. (2005). The activation of 5-HT receptors in prefrontal cortex enhances dopaminergic activity. J. Neurochem. 95, 1597–1607. doi:10.1111/j.1471-4159.2005.03485.x
Bouso, J. C., and Sanchez-Aviles, C. (2020). Traditional healing practices involving psychoactive plants and the global mental health agenda: Opportunities, pitfalls, and challenges in the "Right to Science" framework. Health Hum. Rights 22, 145–150.
Brandt, L., Hu, M. C., Liu, Y., Castillo, F., Odom, G. J., Balise, R. R., et al. (2023). Risk of experiencing an overdose event for patients undergoing treatment with medication for opioid use disorder. Am. J. Psychiatry 180, 386–394. doi:10.1176/appi.ajp.20220312
Brogden, R. N., and Sorkin, E. M. (1990). Ketanserin. A review of its pharmacodynamic and pharmacokinetic properties, and therapeutic potential in hypertension and peripheral vascular disease. Drugs 40, 903–949. doi:10.2165/00003495-199040060-00010
Browne, C. J., Abela, A. R., Chu, D., Li, Z., Ji, X., Lambe, E. K., et al. (2019). Dorsal raphe serotonin neurons inhibit operant responding for reward via inputs to the ventral tegmental area but not the nucleus accumbens: evidence from studies combining optogenetic stimulation and serotonin reuptake inhibition. Neuropsychopharmacology 44, 793–804. doi:10.1038/s41386-018-0271-x
Bruera, E., and Del Fabbro, E. (2018). Pain management in the era of the opioid crisis. Am. Soc. Clin. Oncol. Educ. Book 38, 807–812. doi:10.1200/EDBK_208563
Buchborn, T., Schroder, H., Dieterich, D. C., Grecksch, G., and Hollt, V. (2015). Tolerance to LSD and DOB induced shaking behaviour: differential adaptations of frontocortical 5-HT(2A) and glutamate receptor binding sites. Behav. Brain Res. 281, 62–68. doi:10.1016/j.bbr.2014.12.014
Burton, C. L., Rizos, Z., Diwan, M., Nobrega, J. N., and Fletcher, P. J. (2013). Antagonizing 5-HT₂A receptors with M100907 and stimulating 5-HT₂C receptors with Ro60-0175 blocks cocaine-induced locomotion and zif268 mRNA expression in Sprague-Dawley rats. Behav. Brain Res. 240, 171–181. doi:10.1016/j.bbr.2012.11.030
Butelman, E. R., Yuferov, V., and Kreek, M. J. (2012). κ-opioid receptor/dynorphin system: genetic and pharmacotherapeutic implications for addiction. Trends Neurosci. 35, 587–596. doi:10.1016/j.tins.2012.05.005
Calderon, S., Giarola, A., and Heal, D. (2015). “Regulatory framework and guidance to the evaluation of the abuse liability of drugs in the United States and Europe,” in Nonclinical assessment of abuse potential for new pharmaceuticals. Editors C. G. Markgraf, T. J. Hudzik, and D. R. Compton (Boston: Academic Press), 245–268.
Ciccarone, D., and Shoptaw, S. (2022). Understanding stimulant use and use disorders in a new era. Med. Clin. North Am. 106, 81–97. doi:10.1016/j.mcna.2021.08.010
Calderon, S. N., Bonson, K. R., Reissig, C. J., Lloyd, J. M., Galati, S., and Chiapperino, D. (2023). Considerations in assessing the abuse potential of psychedelics during drug development. Neuropharmacology 224, 109352. doi:10.1016/j.neuropharm.2022.109352
Callahan, P. M., and Appel, J. B. (1990). Differentiation between the stimulus effects of (+)-lysergic acid diethylamide and lisuride using a three-choice, drug discrimination procedure. Psychopharmacology 100, 13–18. doi:10.1007/BF02245782
Cameron, L. P., Tombari, R. J., Lu, J., Pell, A. J., Hurley, Z. Q., Ehinger, Y., et al. (2021). A non-hallucinogenic psychedelic analogue with therapeutic potential. Nature 589, 474–479. doi:10.1038/s41586-020-3008-z
Campbell, G., Nielsen, S., Larance, B., Bruno, R., Mattick, R., Hall, W., et al. (2015). Pharmaceutical opioid use and dependence among people living with chronic pain: Associations observed within the pain and opioids in treatment (POINT) cohort. Pain Med. 16, 1745–1758. doi:10.1111/pme.12773
Canal, C. E., Booth, R. G., and Morgan, D. (2013). Support for 5-HT2C receptor functional selectivity in vivo utilizing structurally diverse, selective 5-HT2C receptor ligands and the 2,5-dimethoxy-4-iodoamphetamine elicited head-twitch response model. Neuropharmacology 70, 112–121. doi:10.1016/j.neuropharm.2013.01.007
Cao, D., Yu, J., Wang, H., Luo, Z., Liu, X., He, L., et al. (2022). Structure-based discovery of nonhallucinogenic psychedelic analogs. Science 375, 403–411. doi:10.1126/science.abl8615
Carr, D. B., and Sesack, S. R. (2000). Dopamine terminals synapse on callosal projection neurons in the rat prefrontal cortex. J. Comp. Neurol. 425, 275–283. doi:10.1002/1096-9861(20000918)425:2<275::aid-cne9>3.0.co;2-z
Carter, L. P., and Griffiths, R. R. (2009). Principles of laboratory assessment of drug abuse liability and implications for clinical development. Drug Alcohol Depend. 105 (1), S14–S25. doi:10.1016/j.drugalcdep.2009.04.003
Casey, A. B., Cui, M., Booth, R. G., and Canal, C. E. (2022). Selective" serotonin 5-HT2A receptor antagonists. Biochem. Pharmacol. 200, 115028. doi:10.1016/j.bcp.2022.115028
Castellanos, J. P., Woolley, C., Bruno, K. A., Zeidan, F., Halberstadt, A., and Furnish, T. (2020). Chronic pain and psychedelics: a review and proposed mechanism of action. Reg. Anesth. Pain Med. 45, 486–494. doi:10.1136/rapm-2020-101273
Ceceli, A. O., Huang, Y., Kronberg, G., Malaker, P., Miller, P., King, S. G., et al. (2023). Common and distinct fronto-striatal volumetric changes in heroin and cocaine use disorders. Brain 146, 1662–1671. doi:10.1093/brain/awac366
Cheer, J. F., Cadogan, A. K., Marsden, C. A., Fone, K. C., and Kendall, D. A. (1999). Modification of 5-HT2 receptor mediated behaviour in the rat by oleamide and the role of cannabinoid receptors. Neuropharmacology 38, 533–541. doi:10.1016/s0028-3908(98)00208-1
Chen, J., Garcia, E. J., Merritt, C. R., Zamora, J. C., Bolinger, A. A., Pazdrak, K., et al. (2023). Discovery of novel oleamide analogues as brain-penetrant positive allosteric serotonin 5-HT2C receptor and dual 5-HT2C/5-HT2A receptor modulators. J. Med. Chem. 66, 9992–10009. doi:10.1021/acs.jmedchem.3c00908
Chilcoat, H. D., Amick, H. R., Sherwood, M. R., and Dunn, K. E. (2019). Buprenorphine in the United States: Motives for abuse, misuse, and diversion. J. Subst. Abuse Treat. 104, 148–157. doi:10.1016/j.jsat.2019.07.005
Cho, S. S., Strafella, A. P., Duff-Canning, S., Zurowski, M., Vijverman, A. C., Bruno, V., et al. (2017). The relationship between serotonin-2A receptor and cognitive functions in nondemented Parkinson's disease patients with visual hallucinations. Mov. Disord. Clin. Pract. 4, 698–709. doi:10.1002/mdc3.12466
Christie, M. J. (2008). Cellular neuroadaptations to chronic opioids: tolerance, withdrawal and addiction. Br. J. Pharmacol. 154, 384–396. doi:10.1038/bjp.2008.100
Christie, M. J., Williams, J. T., and North, R. A. (1992). Cellular mechanisms of opioid tolerance: studies in single brain neurons. Mol. Pharmacol. 32, 633–638.
Chu Sin Chung, P., and Kieffer, B. L. (2013). Delta opioid receptors in brain function and diseases. Pharmacol. Ther. 140, 112–120. doi:10.1016/j.pharmthera.2013.06.003
Clark, S. D., and Abi-Dargham, A. (2019). The role of dynorphin and the kappa opioid receptor in the symptomatology of schizophrenia: A review of the evidence. Biol. Psychiatry 86, 502–511. doi:10.1016/j.biopsych.2019.05.012
Comer, S. D., Zacny, J. P., Dworkin, R. H., Turk, D. C., Bigelow, G. E., Foltin, R. W., et al. (2012). Core outcome measures for opioid abuse liability laboratory assessment studies in humans: IMMPACT recommendations. Pain 153, 2315–2324. doi:10.1016/j.pain.2012.07.035
Corne, S. J., and Pickering, R. W. (1967). A possible correlation between drug-induced hallucinations in man and a behavioural response in mice. Psychopharmacologia 11, 65–78. doi:10.1007/BF00401509
Corne, S. J., Pickering, R. W., and Warner, B. T. (1963). A method for assessing the effects of drugs on the central actions of 5-hydroxytryptamine. Br. J. Pharmacol. Chemother. 20, 106–120. doi:10.1111/j.1476-5381.1963.tb01302.x
Coussens, N. P., Sittampalam, G. S., Jonson, S. G., Hall, M. D., Gorby, H. E., Tamiz, A. P., et al. (2019). The opioid crisis and the future of addiction and pain therapeutics. J. Pharmacol. Exp. Ther. 371, 396–408. doi:10.1124/jpet.119.259408
Cravatt, B. F., Prospero-Garcia, O., Siuzdak, G., Gilula, N. B., Henriksen, S. J., Boger, D. L., et al. (1995). Chemical characterization of a family of brain lipids that induce sleep. Science 268, 1506–1509. doi:10.1126/science.7770779
Cunningham, K. A., Anastasio, N. C., Fox, R. G., Stutz, S. J., Bubar, M. J., Swinford, S. E., et al. (2013). Synergism between a serotonin 5-HT2A receptor (5-HT2AR) antagonist and 5-HT2CR agonist suggests new pharmacotherapeutics for cocaine addiction. ACS Chem. Neurosci. 4, 110–121. doi:10.1021/cn300072u
Cunningham, K. A., and Anastasio, N. C. (2014). Serotonin at the nexus of impulsivity and cue reactivity in cocaine addiction. Neuropharmacology 76 Pt B, 460–478. doi:10.1016/j.neuropharm.2013.06.030
Cunningham, K. A., and Appel, J. B. (1988). “Hallucinogenic and non-hallucinogenic 5-HT agonists: Differences in subjective effects parallel differences in receptor dynamics,” in 5-HT agonists as psychoactive drugs. Editors R. H. Rech, and G. A. Gudelsky (Ann Arbor, MI: NPP Press), 217–238.
Cunningham, K. A., and Appel, J. B. (1987). Neuropharmacological reassessment of the discriminative stimulus properties of d-lysergic acid diethylamide (LSD). Psychopharmacol. Berl. 91, 67–73. doi:10.1007/BF00690929
Cunningham, K. A., Callahan, P. M., and Appel, J. B. (1985). Differentiation between the stimulus effects of l-5-hydroxytryptophan and LSD. Eur. J. Pharmacol. 108, 179–186. doi:10.1016/0014-2999(85)90723-x
Cunningham, K. A., Howell, L. L., and Anastasio, N. C. (2020). “Serotonin neurobiology in cocaine use disorder,” in Handbook of the behavioral neurobiology of serotonin. Editors C. P. Muller, and K. A Cunningham. 2nd Edition ((United Kingdom: Academic Press), 745–802.
Cunningham, M. J., Bock, H. A., Serrano, I. C., Bechand, B., Vidyadhara, D. J., Bonniwell, E. M., et al. (2023). Pharmacological mechanism of the non-hallucinogenic 5-HT(2A) agonist ariadne and analogs. ACS Chem. Neurosci. 14, 119–135. doi:10.1021/acschemneuro.2c00597
Davis, J., Zamora, D., Horowitz, M., and Leucht, S. (2021). Evaluating pimavanserin as a treatment for psychiatric disorders: A pharmacological property in search of an indication. Expert Opin. Pharmacother. 22, 1651–1660. doi:10.1080/14656566.2021.1942455
De La Fuente Revenga, M., Shah, U. H., Nassehi, N., Jaster, A. M., Hemanth, P., Sierra, S., et al. (2021). Psychedelic-like properties of quipazine and its structural analogues in mice. ACS Chem. Neurosci. 12, 831–844. doi:10.1021/acschemneuro.0c00291
Degenhardt, L., Hungerford, P., Nielsen, S., Bruno, R., Larance, B., Clare, P. J., et al. (2021). Pharmaceutical opioid use patterns and indicators of extramedical use and harm in adults with chronic noncancer pain, 2012-2018. JAMA Netw. Open 4, e213059. doi:10.1001/jamanetworkopen.2021.3059
Ding, C., Bremer, N. M., Smith, T. D., Seitz, P. K., Anastasio, N. C., Cunningham, K. A., et al. (2012). Exploration of synthetic approaches and pharmacological evaluation of PNU-69176E and its stereoisomer as 5-HT2C receptor allosteric modulators. ACS Chem. Neurosci. 3, 538–545. doi:10.1021/cn300020x
Divito, A. J., and Leger, R. F. (2020). Psychedelics as an emerging novel intervention in the treatment of substance use disorder: a review. Mol. Biol. Rep. 47, 9791–9799. doi:10.1007/s11033-020-06009-x
Dong, C., Ly, C., Dunlap, L. E., Vargas, M. V., Sun, J., Hwang, I. W., et al. (2021). Psychedelic-inspired drug discovery using an engineered biosensor. Cell 184, 2779–2792.e18. doi:10.1016/j.cell.2021.03.043
Doblin, R. E., Christiansen, M., Jerome, L., and Burge, B. (2019). The past and future of psychedelic science: An introduction to this issue. J. Psychoact. Drugs 51, 93–97. doi:10.1080/02791072.2019.1606472
Du, Y., Yu, K., Yan, C., Wei, C., Zheng, Q., Qiao, Y., et al. (2022). The contributions of mu-opioid receptors on glutamatergic and GABAergic neurons to analgesia induced by various stress intensities. eNeuro 9, 0487. doi:10.1523/ENEURO.0487-21.2022
Erkizia-Santamaria, I., Alles-Pascual, R., Horrillo, I., Meana, J. J., and Ortega, J. E. (2022). Serotonin 5-HT2A, 5-HT2C and 5-HT1A receptor involvement in the acute effects of psilocybin in mice. In vitro pharmacological profile and modulation of thermoregulation and head-twich response. Biomed. Pharmacother. 154, 113612. doi:10.1016/j.biopha.2022.113612
Ezeomah, C., Cunningham, K. A., Stutz, S. J., Fox, R. G., Bukreyeva, N., Dineley, K. T., et al. (2020). Fentanyl self-administration impacts brain immune responses in male Sprague-Dawley rats. Brain Behav. Immun. 87, 725–738. doi:10.1016/j.bbi.2020.03.003
Fda (2018). Public meeting on patient-focused drug development for opioid use disorder (Voice of the Patient). Available: https://www.fda.gov/industry/prescription-drug-user-fee-amendments/public-meeting-patient-focused-drug-development-opioid-use-disorder (Accessed 5/7/23.
Fantegrossi, W. E., Woods, J. H., and Winger, G. (2004a). Transient reinforcing effects of phenylisopropylamine and indolealkylamine hallucinogens in rhesus monkeys. Behav. Pharmacol. 15, 149–157. doi:10.1097/00008877-200403000-00007
Fantegrossi, W. E., Woolverton, W. L., Kilbourn, M., Sherman, P., Yuan, J., Hatzidimitriou, G., et al. (2004b). Behavioral and neurochemical consequences of long-term intravenous self-administration of MDMA and its enantiomers by rhesus monkeys. Neuropsychopharmacology 29, 1270–1281. doi:10.1038/sj.npp.1300442
Fda, (2017). Assessment of abuse potential of drugs: guidance for industry. Center for Drug Evaluation and Research.
Fedorova, I., Hashimoto, A., Fecik, R. A., Hedrick, M. P., Hanus, L. O., Boger, D. L., et al. (2001). Behavioral evidence for the interaction of oleamide with multiple neurotransmitter systems. J. Pharmacol. Exp. Ther. 299, 332–342.
Felsing, D. E., Anastasio, N. C., Miszkiel, J. M., Gilbertson, S. R., Allen, J. A., and Cunningham, K. A. (2018). Biophysical validation of serotonin 5-HT2A and 5-HT2C receptor interaction. PLoS One 13, e0203137. doi:10.1371/journal.pone.0203137
Ferezou, I., Hill, E. L., Cauli, B., Gibelin, N., Kaneko, T., Rossier, J., et al. (2007). Extensive overlap of mu-opioid and nicotinic sensitivity in cortical interneurons. Cereb. Cortex 17, 1948–1957. doi:10.1093/cercor/bhl104
Fields, H. L., and Margolis, E. B. (2015). Understanding opioid reward. Trends Neurosci. 38, 217–225. doi:10.1016/j.tins.2015.01.002
Fink, L. H., Anastasio, N. C., Fox, R. G., Rice, K. C., Moeller, F. G., and Cunningham, K. A. (2015). Individual differences in impulsive action reflect variation in the cortical serotonin 5-HT2A receptor system. Neuropsychopharmacology 40, 1957–1968. doi:10.1038/npp.2015.46
Fiorella, D., Rabin, R. A., and Winter, J. C. (1995a). Role of 5-HT2A and 5-HT2C receptors in the stimulus effects of hallucinogenic drugs. II: Reassessment of LSD false positives. Psychopharmacol. Berl. 121, 357–363. doi:10.1007/BF02246075
Fiorella, D., Rabin, R. A., and Winter, J. C. (1995b). The role of the 5-HT2A and 5-HT2C receptors in the stimulus effects of hallucinogenic drugs. I: Antagonist correlation analysis. Psychopharmacol. Berl. 121, 347–356. doi:10.1007/BF02246074
Fitzgerald, L. W., Burn, T. C., Brown, B. S., Patterson, J. P., Corjay, M. H., Valentine, P. A., et al. (2000). Possible role of valvular serotonin 5-HT(2B) receptors in the cardiopathy associated with fenfluramine. Mol. Pharmacol. 57, 75–81.
Flynn, S. M., and France, C. P. (2021). Discriminative stimulus effects of carfentanil in rats discriminating fentanyl: Differential antagonism by naltrexone. Drug Alcohol Depend. 221, 108599. doi:10.1016/j.drugalcdep.2021.108599
Friedman, S. R., Krawczyk, N., Perlman, D. C., Mateu-Gelabert, P., Ompad, D. C., Hamilton, L., et al. (2020). The opioid/overdose crisis as a dialectics of pain, despair, and one-sided struggle. Front. Public Health 8, 540423. doi:10.3389/fpubh.2020.540423
Gaddum, J. H., and Hameed, K. A. (1954). Drugs which antagonize 5-hydroxytryptamine. Br. J. Pharmacol. Chemother. 9, 240–248. doi:10.1111/j.1476-5381.1954.tb00848.x
Garcia-Carceles, J., Decara, J. M., Vazquez-Villa, H., Rodriguez, R., Codesido, E., Cruces, J., et al. (2017). A positive allosteric modulator of the serotonin 5-HT(2C) receptor for obesity. J. Med. Chem. 60, 9575–9584. doi:10.1021/acs.jmedchem.7b00994
Garcia-Romeu, A., Davis, A. K., Erowid, E., Erowid, F., Griffiths, R. R., and Johnson, M. W. (2019). Persisting reductions in cannabis, opioid, and stimulant misuse after naturalistic psychedelic use: An online survey. Front. Psychiatry 10, 955. doi:10.3389/fpsyt.2019.00955
Ginzel, K. H., and Mayer-Gross, W. (1956). Prevention of psychological effects of d-lysergic acid diethylamide (LSD 25) by its 2-brom derivative (BOL 148). Nature 178, 210. doi:10.1038/178210a0
Glennon, R. A. (1993). MDMA-like stimulus effects of alpha-ethyltryptamine and the alpha-ethyl homolog of DOM. Pharmacol. Biochem. Behav. 46, 459–462. doi:10.1016/0091-3057(93)90379-8
Glennon, R. A., Young, R., and Jacyno, J. M. (1983). Indolealkylamine and phenalkylamine hallucinogens. Effect of alpha-methyl and N-methyl substituents on behavioral activity. Biochem. Pharmacol. 32, 1267–1273. doi:10.1016/0006-2952(83)90281-2
Gonzalez-Maeso, J., Weisstaub, N. V., Zhou, M., Chan, P., Ivic, L., Ang, R., et al. (2007). Hallucinogens recruit specific cortical 5-HT(2A) receptor-mediated signaling pathways to affect behavior. Neuron 53, 439–452. doi:10.1016/j.neuron.2007.01.008
Gonzalez-Maeso, J., Yuen, T., Ebersole, B. J., Wurmbach, E., Lira, A., Zhou, M., et al. (2003). Transcriptome fingerprints distinguish hallucinogenic and nonhallucinogenic 5-hydroxytryptamine 2A receptor agonist effects in mouse somatosensory cortex. J. Neurosci. 23, 8836–8843. doi:10.1523/JNEUROSCI.23-26-08836.2003
Goodwin, A. K. (2016). An intravenous self-administration procedure for assessing the reinforcing effects of hallucinogens in nonhuman primates. J. Pharmacol. Toxicol. Methods 82, 31–36. doi:10.1016/j.vascn.2016.07.004
Griffiths, R. R., and Grob, C. S. (2010). Hallucinogens as medicine. Sci. Am. 303, 76–79. doi:10.1038/scientificamerican1210-76
Guillou-Landreat, M., Quinio, B., Le Reste, J. Y., Le Goff, D., Fonsecca, J., Grall-Bronnec, M., et al. (2021). Prospective Study on Factors Associated with Referral of Patients with Opioid Maintenance Therapy from Specialized Addictive Disorders Centers to Primary Care. Int. J. Environ. Res. Public Health 18, 5749. doi:10.3390/ijerph18115749
Hacksell, U., Burstein, E. S., Mcfarland, K., Mills, R. G., and Williams, H. (2014). On the discovery and development of pimavanserin: a novel drug candidate for Parkinson's psychosis. Neurochem. Res. 39, 2008–2017. doi:10.1007/s11064-014-1293-3
Halberstadt, A. L., Chatha, M., Klein, A. K., Wallach, J., and Brandt, S. D. (2020). Correlation between the potency of hallucinogens in the mouse head-twitch response assay and their behavioral and subjective effects in other species. Neuropharmacology 167, 107933. doi:10.1016/j.neuropharm.2019.107933
Halberstadt, A. L., and Geyer, M. A. (2010). LSD but not lisuride disrupts prepulse inhibition in rats by activating the 5-HT(2A) receptor. Psychopharmacol. Berl. 208, 179–189. doi:10.1007/s00213-009-1718-x
Harris, G. C., and Aston-Jones, G. (2001). Augmented accumbal serotonin levels decrease the preference for a morphine associated environment during withdrawal. Neuropsychopharmacology 24, 75–85. doi:10.1016/S0893-133X(00)00184-6
Hasin, D. S., O'brien, C. P., Auriacombe, M., Borges, G., Bucholz, K., Budney, A., et al. (2013). DSM-5 criteria for substance use disorders: recommendations and rationale. Am. J. Psychiatry 170, 834–851. doi:10.1176/appi.ajp.2013.12060782
Hasin, D. S., Shmulewitz, D., Stohl, M., Greenstein, E., Aharonovich, E., Petronis, K. R., et al. (2022). Diagnosing prescription opioid use disorder in patients using prescribed opioids for chronic pain. Am. J. Psychiatry 179, 715–725. doi:10.1176/appi.ajp.21070721
Heal, D. J., Gosden, J., and Smith, S. L. (2018). Evaluating the abuse potential of psychedelic drugs as part of the safety pharmacology assessment for medical use in humans. Neuropharmacology 142, 89–115. doi:10.1016/j.neuropharm.2018.01.049
Hedlund, P. B., Carson, M. J., Sutcliffe, J. G., and Thomas, E. A. (1999). Allosteric regulation by oleamide of the binding properties of 5-hydroxytryptamine7 receptors. Biochem. Pharmacol. 58, 1807–1813. doi:10.1016/s0006-2952(99)00274-9
Hedlund, P. B., Danielson, P. E., Thomas, E. A., Slanina, K., Carson, M. J., and Sutcliffe, J. G. (2003). No hypothermic response to serotonin in 5-HT7 receptor knockout mice. Proc. Natl. Acad. Sci. U. S. A. 100, 1375–1380. doi:10.1073/pnas.0337340100
Heijmans, L., Mons, M. R., and Joosten, E. A. (2021). A systematic review on descending serotonergic projections and modulation of spinal nociception in chronic neuropathic pain and after spinal cord stimulation. Mol. Pain 17, 17448069211043965. doi:10.1177/17448069211043965
Heinsbroek, J. A., Giannotti, G., Bonilla, J., Olson, D. E., and Peters, J. (2023). Tabernanthalog reduces motivation for heroin and alcohol in a polydrug use model. Psychedelic Med. 1, 111–119. doi:10.1089/psymed.2023.0009
Hendricks, P. S., Nichols, C. D., Cunningham, K. A., Geyer, M. A., Griffiths, R., and Nichols, D. (2023). Past, Present, and Future of Psychedelics: A Psychedelic Medicine Roundtable Discussion. Psychedelic Med. 1, 2–11. doi:10.1089/psymed.2022.0003
Henningfield, J. E., Coe, M. A., Griffiths, R. R., Belouin, S. J., Berger, A., Coker, A. R., et al. (2022). Psychedelic drug abuse potential assessment research for new drug applications and Controlled Substances Act scheduling. Neuropharmacology 218, 109220. doi:10.1016/j.neuropharm.2022.109220
Higginbotham, J. A., Markovic, T., Massaly, N., and Moron, J. A. (2022). Endogenous opioid systems alterations in pain and opioid use disorder. Front. Syst. Neurosci. 16, 1014768. doi:10.3389/fnsys.2022.1014768
Higgins, G. A., Fletcher, P. J., and Shanahan, W. R. (2020). Lorcaserin: A review of its preclinical and clinical pharmacology and therapeutic potential. Pharmacol. Ther. 205, 107417. doi:10.1016/j.pharmthera.2019.107417
Hilger, D., Masureel, M., and Kobilka, B. K. (2018). Structure and dynamics of GPCR signaling complexes. Nat. Struct. Mol. Biol. 25, 4–12. doi:10.1038/s41594-017-0011-7
Holze, F., Avedisian, I., Varghese, N., Eckert, A., and Liechti, M. E. (2021a). Role of the 5-HT(2A) receptor in acute effects of LSD on empathy and circulating oxytocin. Front. Pharmacol. 12, 711255. doi:10.3389/fphar.2021.711255
Holze, F., Vizeli, P., Ley, L., Muller, F., Dolder, P., Stocker, M., et al. (2021b). Acute dose-dependent effects of lysergic acid diethylamide in a double-blind placebo-controlled study in healthy subjects. Neuropsychopharmacology 46, 537–544. doi:10.1038/s41386-020-00883-6
Horvath, J., Fross, R. D., Kleiner-Fisman, G., Lerch, R., Stalder, H., Liaudat, S., et al. (2004). Severe multivalvular heart disease: a new complication of the ergot derivative dopamine agonists. Mov. Disord. 19, 656–662. doi:10.1002/mds.20201
Howell, L. L., and Cunningham, K. A. (2015). Serotonin 5-HT2 receptor interactions with dopamine function: implications for therapeutics in cocaine use disorder. Pharmacol. Rev. 67, 176–197. doi:10.1124/pr.114.009514
Humphreys, K., Shover, C. L., Andrews, C. M., Bohnert, A. S. B., Brandeau, M. L., Caulkins, J. P., et al. (2022). Responding to the opioid crisis in North America and beyond: recommendations of the Stanford-Lancet Commission. Lancet 399, 555–604. doi:10.1016/S0140-6736(21)02252-2
Humphreys, K., and Shover, C. L. (2023). Twenty-Year Trends in Drug Overdose Fatalities Among Older Adults in the US. JAMA Psychiatry 80, 518–520. doi:10.1001/jamapsychiatry.2022.5159
Hutcheson, J. D., Setola, V., Roth, B. L., and Merryman, W. D. (2011). Serotonin receptors and heart valve disease--it was meant 2B. Pharmacol. Ther. 132, 146–157. doi:10.1016/j.pharmthera.2011.03.008
Im, W. B., Chio, C. L., Alberts, G. L., and Dinh, D. M. (2003). Positive allosteric modulator of the human 5-HT2C receptor. Mol. Pharmacol. 64, 78–84. doi:10.1124/mol.64.1.78
Jeffery, M. M., Chaisson, C. E., Hane, C., Rumanes, L., Tucker, J., Hang, L., et al. (2020). Assessment of potentially inappropriate prescribing of opioid analgesics requiring prior opioid tolerance. JAMA Netw. Open 3, e202875. doi:10.1001/jamanetworkopen.2020.2875
Jenks, C. W. (2002). Extraction studies of Tabernanthe iboga and Voacanga africana. Nat. Prod. Lett. 16, 71–76. doi:10.1080/1057563029001/4881
Johnson, M. W., Griffiths, R. R., Hendricks, P. S., and Henningfield, J. E. (2018). The abuse potential of medical psilocybin according to the 8 factors of the Controlled Substances Act. Neuropharmacology 142, 143–166. doi:10.1016/j.neuropharm.2018.05.012
Jones, G. M., and Nock, M. K. (2022). Exploring protective associations between the use of classic psychedelics and cocaine use disorder: a population-based survey study. Sci. Rep. 12, 2574. doi:10.1038/s41598-022-06580-2
Jones, G., Ricard, J. A., Lipson, J., and Nock, M. K. (2022). Associations between classic psychedelics and opioid use disorder in a nationally-representative U.S. adult sample. Sci. Rep. 12, 4099. doi:10.1038/s41598-022-08085-4
Jones, J. D., and Comer, S. D. (2013). A review of human drug self-administration procedures. Behav. Pharmacol. 24, 384–395. doi:10.1097/FBP.0b013e3283641c3d
Karst, M., Halpern, J. H., Bernateck, M., and Passie, T. (2010). The non-hallucinogen 2-bromo-lysergic acid diethylamide as preventative treatment for cluster headache: an open, non-randomized case series. Cephalalgia 30, 1140–1144. doi:10.1177/0333102410363490
Kast, E. C., and Collins, V. J. (1964). Study of lysergic acid diethylamide as an analgesic agent. Anesth. Analgesia 43, 285–291. doi:10.1213/00000539-196405000-00013
Kehne, J. H., Baron, B. M., Carr, A. A., Chaney, S. F., Elands, J., Feldman, D. J., et al. (1996). Preclinical characterization of the potential of the putative atypical antipsychotic MDL 100,907 as a potent 5-HT2A antagonist with a favorable CNS safety profile. J.Pharmacol Exp.Ther. 277, 968–981.
Kim, J. A., Bartlett, S., He, L., Nielsen, C. K., Chang, A. M., Kharazia, V., et al. (2008). Morphine-induced receptor endocytosis in a novel knockin mouse reduces tolerance and dependence. Curr. Biol. 18, 129–135. doi:10.1016/j.cub.2007.12.057
Kim, K., Che, T., Panova, O., Diberto, J. F., Lyu, J., Krumm, B. E., et al. (2020). Structure of a hallucinogen-activated Gq-coupled 5-HT2A serotonin receptor. Cell 182, 1574–1588. doi:10.1016/j.cell.2020.08.024
King, T., Qu, C., Okun, A., Mercado, R., Ren, J., Brion, T., et al. (2011). Contribution of afferent pathways to nerve injury-induced spontaneous pain and evoked hypersensitivity. Pain 152, 1997–2005. doi:10.1016/j.pain.2011.04.020
Koenig, X., Kovar, M., Boehm, S., Sandtner, W., and Hilber, K. (2014). Anti-addiction drug ibogaine inhibits hERG channels: a cardiac arrhythmia risk. Addict. Biol. 19, 237–239. doi:10.1111/j.1369-1600.2012.00447.x
Kometer, M., Schmidt, A., Jancke, L., and Vollenweider, F. X. (2013). Activation of serotonin 2A receptors underlies the psilocybin-induced effects on alpha oscillations, N170 visual-evoked potentials, and visual hallucinations. J. Neurosci. 33, 10544–10551. doi:10.1523/JNEUROSCI.3007-12.2013
Koob, G. F., and Le Moal, M. (2005). Plasticity of reward neurocircuitry and the 'dark side' of drug addiction. Nat. Neurosci. 8, 1442–1444. doi:10.1038/nn1105-1442
Koob, G. F., Pettit, H. O., Ettenberg, A., and Bloom, F. E. (1984). Effects of opiate antagonists and their quaternary derivatives on heroin self-administration in the rat. J. Pharmacol. Exp. Ther. 229, 481–486.
Koob, G. F., and Volkow, N. D. (2016). Neurobiology of addiction: A neurocircuitry analysis. Lancet Psychiatry 3, 760–773. doi:10.1016/S2215-0366(16)00104-8
Koslowski, M., Johnson, M. W., Gründer, G., and Betzler, F. (2021). Novel treatment approaches for substance use disorders: Therapeutic use of psychedelics and the role of psychotherapy. Curr. Addict. Rep. 9, 48–58. doi:10.1007/s40429-021-00401-8
Krebs-Thomson, K., Paulus, M. P., and Geyer, M. A. (1998). Effects of hallucinogens on locomotor and investigatory activity and patterns: influence of 5-HT2A and 5-HT2C receptors. Neuropsychopharmacology 18, 339–351. doi:10.1016/S0893-133X(97)00164-4
Krebs-Thomson, K., Ruiz, E. M., Masten, V., Buell, M., and Geyer, M. A. (2006). The roles of 5-HT1A and 5-HT2 receptors in the effects of 5-MeO-DMT on locomotor activity and prepulse inhibition in rats. Psychopharmacol. Berl. 189, 319–329. doi:10.1007/s00213-006-0566-1
Lam, D. D., Przydzial, M. J., Ridley, S. H., Yeo, G. S. H., Rochford, J. J., O'rahilly, S., et al. (2008). Serotonin 5-HT2C receptor agonist promotes hypophagia via downstream activation of melanocortin 4 receptors. Endocrinology 149, 1323–1328. doi:10.1210/en.2007-1321
Le Merrer, J., Becker, J. A., Befort, K., and Kieffer, B. L. (2009). Reward processing by the opioid system in the brain. Physiol. Rev. 89, 1379–1412. doi:10.1152/physrev.00005.2009
Leiderman, D. B. (2009). Risk management of drug products and the U.S. Food and Drug Administration: evolution and context. Drug Alcohol Depend. 105 (1), S9–S13. doi:10.1016/j.drugalcdep.2009.02.007
Leptourgos, P., Fortier-Davy, M., Carhart-Harris, R., Corlett, P. R., Dupuis, D., Halberstadt, A. L., et al. (2020). Hallucinations under psychedelics and in the schizophrenia spectrum: An interdisciplinary and multiscale comparison. Schizophr. Bull. 46, 1396–1408. doi:10.1093/schbul/sbaa117
Leri, F., and Burns, L. H. (2005). Ultra-low-dose naltrexone reduces the rewarding potency of oxycodone and relapse vulnerability in rats. Pharmacol. Biochem. Behav. 82, 252–262. doi:10.1016/j.pbb.2005.08.008
Lewis, V., Bonniwell, E. M., Lanham, J. K., Ghaffari, A., Sheshbaradaran, H., Cao, A. B., et al. (2023). A non-hallucinogenic LSD analog with therapeutic potential for mood disorders. Cell Rep. 42, 112203. doi:10.1016/j.celrep.2023.112203
Li, B., Jiang, J., Zhou, L., Tao, X., Sun, Q., Liu, J., et al. (2021). Blockade of 5-hydroxytryptamine 2A receptor attenuates precipitation of naloxone-induced withdrawal symptoms in opioid-exposed mice. Front. Behav. Neurosci. 15, 797217. doi:10.3389/fnbeh.2021.797217
Li, J. X., Koek, W., Rice, K. C., and France, C. P. (2011). Effects of direct- and indirect-acting serotonin receptor agonists on the antinociceptive and discriminative stimulus effects of morphine in rhesus monkeys. Neuropsychopharmacology 36, 940–949. doi:10.1038/npp.2010.232
Lindsley, C. W., Emmitte, K. A., Hopkins, C. R., Bridges, T. M., Gregory, K. J., Niswender, C. M., et al. (2016). Practical strategies and concepts in GPCR allosteric modulator discovery: Recent advances with metabotropic glutamate receptors. Chem. Rev. 116, 6707–6741. doi:10.1021/acs.chemrev.5b00656
Liu, Z., Zhou, J., Li, Y., Hu, F., Lu, Y., Ma, M., et al. (2014). Dorsal raphe neurons signal reward through 5-HT and glutamate. Neuron 81, 1360–1374. doi:10.1016/j.neuron.2014.02.010
Lopez-Gimenez, J. F., Vilaro, M. T., and Milligan, G. (2008). Morphine desensitization, internalization, and down-regulation of the mu opioid receptor is facilitated by serotonin 5-hydroxytryptamine2A receptor coactivation. Mol. Pharmacol. 74, 1278–1291. doi:10.1124/mol.108.048272
Lopez-Gimenez, J. F., Vilaro, M. T., Palacios, J. M., and Mengod, G. (2001). Mapping of 5-HT2A receptors and their mRNA in monkey brain: [3H]MDL100,907 autoradiography and in situ hybridization studies. J. Comp. Neurol. 429, 571–589. doi:10.1002/1096-9861(20010122)429:4<571::aid-cne5>3.0.co;2-x
Ly, C., Greb, A. C., Cameron, L. P., Wong, J. M., Barragan, E. V., Wilson, P. C., et al. (2018). Psychedelics promote structural and functional neural plasticity. Cell Rep. 23, 3170–3182. doi:10.1016/j.celrep.2018.05.022
Meert, T. F., and Vermeirsch, H. A. (2005). A preclinical comparison between different opioids: antinociceptive versus adverse effects. Pharmacol. Biochem. Behav. 80, 309–326. doi:10.1016/j.pbb.2004.12.002
Ma, L., Steinberg, J. L., Bjork, J. M., Taylor, B. A., Arias, A. J., Terplan, M., et al. (2019). Cingulo-hippocampal effective connectivity positively correlates with drug-cue attentional bias in opioid use disorder. Psychiatry Res. Neuroimaging 294, 110977. doi:10.1016/j.pscychresns.2019.08.005
Madden, G. J., Petry, N. M., Badger, G. J., and Bickel, W. K. (1997). Impulsive and self-control choices in opioid-dependent patients and non-drug-using control participants: drug and monetary rewards. Exp. Clin. Psychopharmacol. 5, 256–262. doi:10.1037//1064-1297.5.3.256
Madsen, M. K., Fisher, P. M., Burmester, D., Dyssegaard, A., Stenbaek, D. S., Kristiansen, S., et al. (2019). Psychedelic effects of psilocybin correlate with serotonin 2A receptor occupancy and plasma psilocin levels. Neuropsychopharmacology 44, 1328–1334. doi:10.1038/s41386-019-0324-9
Maguire, D. R. (2023). Effects of 2,5-dimethoxy-4-methylamphetamine (DOM) and 2-piperazin-1-yl-quinoline (quipazine) on fentanyl versus food choice in rhesus monkeys. J. Pharmacol. Exp. Ther. 384, 155–162. doi:10.1124/jpet.122.001318
Maguire, D. R., Li, J. X., Koek, W., and France, C. P. (2013). Effects of 1-(2,5-dimethoxy-4-methylphenyl)-2-aminopropane (DOM) and quipazine on heroin self-administration in rhesus monkeys. Psychopharmacol. Berl. 225, 173–185. doi:10.1007/s00213-012-2803-0
Mansour, A., Fox, C. A., Akil, H., and Watson, S. J. (1995). Opioid-receptor mRNA expression in the rat CNS: anatomical and functional implications. Trends Neurosci. 18, 22–29. doi:10.1016/0166-2236(95)93946-u
Mansour, A., Khachaturian, H., Lewis, M. E., Akil, H., and Watson, S. J. (1988). Anatomy of CNS opioid receptors. Trends Neurosci. 11, 308–314. doi:10.1016/0166-2236(88)90093-8
Marek, G. J., and Aghajanian, G. K. (1998). 5-Hydroxytryptamine-induced excitatory postsynaptic currents in neocortical layer V pyramidal cells: suppression by mu-opiate receptor activation. Neuroscience 86, 485–497. doi:10.1016/s0306-4522(98)00043-8
Marek, G. J. (2003). Behavioral evidence for mu-opioid and 5-HT2A receptor interactions. Eur. J. Pharmacol. 474, 77–83. doi:10.1016/s0014-2999(03)01971-x
Marek, G. J., Cunningham, K. A., and Rasmussen, K. (2019). Translating pharmacology into therapeutics to fight the opioid crisis. J. Pharmacol. Exp. Ther. 371, 394–395. doi:10.1124/jpet.119.262477
Marona-Lewicka, D., Kurrasch-Orbaugh, D. M., Selken, J. R., Cumbay, M. G., Lisnicchia, J. G., and Nichols, D. E. (2002). Re-evaluation of lisuride pharmacology: 5-hydroxytryptamine1A receptor-mediated behavioral effects overlap its other properties in rats. Psychopharmacol. Berl. 164, 93–107. doi:10.1007/s00213-002-1141-z
Martin, D. A., Gyawali, U., and Calu, D. J. (2021). Effects of 5-HT2A receptor stimulation on economic demand for fentanyl after intermittent and continuous access self-administration in male rats. Addict. Biol. 26, e12926. doi:10.1111/adb.12926
Mash, D. C., Ameer, B., Prou, D., Howes, J. F., and Maillet, E. L. (2016). Oral noribogaine shows high brain uptake and anti-withdrawal effects not associated with place preference in rodents. J. Psychopharmacol. 30, 688–697. doi:10.1177/0269881116641331
Mash, D. C., Duque, L., Page, B., and Allen-Ferdinand, K. (2018). Ibogaine detoxification transitions opioid and cocaine abusers between dependence and abstinence: Clinical observations and treatment outcomes. Front. Pharmacol. 9, 529. doi:10.3389/fphar.2018.00529
Mash, D. C. (2023). IUPHAR - invited review - Ibogaine - A legacy within the current renaissance of psychedelic therapy. Pharmacol. Res. 190, 106620. doi:10.1016/j.phrs.2022.106620
Mash, D. C., Staley, J. K., Baumann, M. H., Rothman, R. B., and Hearn, W. L. (1995a). Identification of a primary metabolite of ibogaine that targets serotonin transporters and elevates serotonin. Life Sci. 57, PL45–PL50. doi:10.1016/0024-3205(95)00273-9
Mash, D. C., Staley, J. K., Pablo, J. P., Holohean, A. M., Hackman, J. C., and Davidoff, R. A. (1995b). Properties of ibogaine and its principal metabolite (12- hydroxyibogamine) at the MK-801 binding site of the NMDA receptor complex. Neurosci. Lett. 192, 53–56. doi:10.1016/0304-3940(95)11608-y
Mcauliffe, W. E. (2013). A critique of Minozzi et al.'s pain relief and dependence systematic review. Addiction 108, 1162–1169. doi:10.1111/add.12181
Mcclure-Begley, T. D., and Roth, B. L. (2022). The promises and perils of psychedelic pharmacology for psychiatry. Nat. Rev. Drug Discov. 21, 463–473. doi:10.1038/s41573-022-00421-7
Mcmahon, L. R. (2015). The rise (and fall?) of drug discrimination research. Drug Alcohol Depend. 151, 284–288. doi:10.1016/j.drugalcdep.2015.04.001
Meltzer, H. Y., Mills, R., Revell, S., Williams, H., Johnson, A., Bahr, D., et al. (2010). Pimavanserin, a serotonin(2A) receptor inverse agonist, for the treatment of Parkinson's disease psychosis. Neuropsychopharmacology 35, 881–892. doi:10.1038/npp.2009.176
Mendez-Diaz, M., Amancio-Belmont, O., Estrada-Gonzalez, V., Ruiz-Contreras, A. E., and Prospero-Garcia, O. (2019). CB1R mediates oleamide's reward while 5HT2cR mediates aversion in the nucleus accumbens shell of rats. Neurosci. Lett. 706, 189–193. doi:10.1016/j.neulet.2019.05.033
Merritt, C. R., Smith, A. E., Khanipov, K., Golovko, G., Dineley, K. T., Anastasio, N. C., et al. (2022). Heightened cocaine-seeking in male rats associates with a distinct transcriptomic profile in the medial prefrontal cortex. Front. Pharmacol. 13, 1022863. doi:10.3389/fphar.2022.1022863
Miner, L. A., Backstrom, J. R., Sanders-Bush, E., and Sesack, S. R. (2003). Ultrastructural localization of serotonin2A receptors in the middle layers of the rat prelimbic prefrontal cortex. Neuroscience 116, 107–117. doi:10.1016/s0306-4522(02)00580-8
Minozzi, S., Amato, L., and Davoli, M. (2013). Development of dependence following treatment with opioid analgesics for pain relief: a systematic review. Addiction 108, 688–698. doi:10.1111/j.1360-0443.2012.04005.x
Mocci, G., Jimenez-Sanchez, L., Adell, A., Cortes, R., and Artigas, F. (2014). Expression of 5-HT2A receptors in prefrontal cortex pyramidal neurons projecting to nucleus accumbens. Potential relevance for atypical antipsychotic action. Neuropharmacology 79, 49–58. doi:10.1016/j.neuropharm.2013.10.021
Mohammadi, R., Jahanshahi, M., and Jameie, S. B. (2016). 5-HT2A serotonin receptor density in adult male rats' hippocampus after morphine-based conditioned place preference. Basic Clin. Neurosci. 7, 249–258. doi:10.15412/J.BCN.03070310
Moliner, R., Girych, M., Brunello, C. A., Kovaleva, V., Biojone, C., Enkavi, G., et al. (2023). Psychedelics promote plasticity by directly binding to BDNF receptor TrkB. Nat. Neurosci. 26, 1032–1041. doi:10.1038/s41593-023-01316-5
Morse, W. H., and Skinner, B. F. (1958). Some factors involved in the stimulus control of operant behavior. J. Exp. Anal. Behav. 1, 103–107. doi:10.1901/jeab.1958.1-103
Moutkine, I., Quentin, E., Guiard, B. P., Maroteaux, L., and Doly, S. (2017). Heterodimers of serotonin receptor subtypes 2 are driven by 5-HT2C protomers. J. Biol. Chem. 292, 6352–6368. doi:10.1074/jbc.M117.779041
Nagai, Y., Takayama, K., Nishitani, N., Andoh, C., Koda, M., Shirakawa, H., et al. (2020). The Role of Dorsal Raphe Serotonin Neurons in the Balance between Reward and Aversion. Int. J. Mol. Sci. 21, 2160. doi:10.3390/ijms21062160
Nakai, K., Nakae, A., Oba, S., Mashimo, T., and Ueda, K. (2010). 5-HT2C receptor agonists attenuate pain-related behaviour in a rat model of trigeminal neuropathic pain. Eur. J. Pain 14, 999–1006. doi:10.1016/j.ejpain.2010.04.008
Nasrallah, H. A., Fedora, R., and Morton, R. (2019). Successful treatment of clozapine-nonresponsive refractory hallucinations and delusions with pimavanserin, a serotonin 5HT-2A receptor inverse agonist. Schizophr. Res. 208, 217–220. doi:10.1016/j.schres.2019.02.018
Navratilova, E., Xie, J. Y., Okun, A., Qu, C., Eyde, N., Ci, S., et al. (2012). Pain relief produces negative reinforcement through activation of mesolimbic reward-valuation circuitry. Proc. Natl. Acad. Sci. U. S. A. 109, 20709–20713. doi:10.1073/pnas.1214605109
Neelakantan, H., Holliday, E. D., Fox, R. G., Stutz, S. J., Comer, S. D., Haney, M., et al. (2017). Lorcaserin suppresses oxycodone self-administration and relapse vulnerability in rats. ACS Chem. Neurosci. 8, 1065–1073. doi:10.1021/acschemneuro.6b00413
Negus, S. S., Henriksen, S. J., Mattox, A., Pasternak, G. W., Portoghese, P. S., Takemori, A. E., et al. (1993). Effect of antagonists selective for mu, delta and kappa opioid receptors on the reinforcing effects of heroin in rats. J. Pharmacol. Exp. Ther. 265, 1245–1252.
Nelson, D. L., Lucaites, V. L., Wainscott, D. B., and Glennon, R. A. (1999). Comparisons of hallucinogenic phenylisopropylamine binding affinities at cloned human 5-HT2A, -HT(2B) and 5-HT2C receptors. Naunyn Schmiedeb. Arch. Pharmacol. 359, 1–6. doi:10.1007/pl00005315
Nichols, D. E., and Walter, H. (2021). The history of psychedelics in psychiatry. Pharmacopsychiatry 54, 151–166. doi:10.1055/a-1310-3990
Nielsen, E. B., Ginn, S. R., Cunningham, K. A., and Appel, J. B. (1985). Antagonism of the LSD cue by putative serotonin antagonists: relationship to inhibition of in vivo [3H]spiroperidol binding. Behav. Brain Res. 16, 171–176. doi:10.1016/0166-4328(85)90090-7
Noller, G. E., Frampton, C. M., and Yazar-Klosinski, B. (2018). Ibogaine treatment outcomes for opioid dependence from a twelve-month follow-up observational study. Am. J. Drug Alcohol Abuse 44, 37–46. doi:10.1080/00952990.2017.1310218
O'brien, C. P. (2009). Neuroplasticity in addictive disorders. Dialogues Clin. Neurosci. 11, 350–353. doi:10.31887/DCNS.2009.11.3/cpobrien
O'connor, E. C., Chapman, K., Butler, P., and Mead, A. N. (2011). The predictive validity of the rat self-administration model for abuse liability. Neurosci. Biobehav Rev. 35, 912–938. doi:10.1016/j.neubiorev.2010.10.012
Odagaki, Y., Kinoshita, M., Meana, J. J., Callado, L. F., and Garcia-Sevilla, J. A. (2021). 5-HT2A receptor- and M1 muscarinic acetylcholine receptor-mediated activation of Gαq/11 in postmortem dorsolateral prefrontal cortex of opiate addicts. Pharmacol. Rep. 73, 1155–1163. doi:10.1007/s43440-021-00248-w
Okun, A., Defelice, M., Eyde, N., Ren, J., Mercado, R., King, T., et al. (2011). Transient inflammation-induced ongoing pain is driven by TRPV1 sensitive afferents. Mol. Pain 7, 4. doi:10.1186/1744-8069-7-4
Olson, D. E. (2021). The subjective effects of psychedelics may not be necessary for their enduring therapeutic effects. ACS Pharmacol. Transl. Sci. 4, 563–567. doi:10.1021/acsptsci.0c00192
Olson, K. M., Keresztes, A., Tashiro, J. K., Daconta, L. V., Hruby, V. J., and Streicher, J. M. (2018). Synthesis and evaluation of a novel bivalent selective antagonist for the mu-delta opioid receptor heterodimer that reduces morphine withdrawal in mice. J. Med. Chem. 61, 6075–6086. doi:10.1021/acs.jmedchem.8b00403
Ozdemir, E. (2017). The pathophysiological role of serotonin receptor systems in opioid analgesia and tolerance. Int. J. Basic & Clin. Pharmacol. 6, 217–228. doi:10.18203/2319-2003.ijbcp20170312
Palfreyman, M. G., Schmidt, C. J., Sorensen, S. M., Dudley, M. W., Kehne, J. H., Moser, P., et al. (1993). Electrophysiological, biochemical and behavioral evidence for 5-HT2 and 5-HT3 mediated control of dopaminergic function. Psychopharmacol. Berl. 112, S60–S67. doi:10.1007/BF02245008
Pang, G., Wu, X., Tao, X., Mao, R., Liu, X., Zhang, Y. M., et al. (2016). Blockade of serotonin 5-HT2A receptors suppresses behavioral sensitization and naloxone-precipitated withdrawal symptoms in morphine-treated mice. Front. Pharmacol. 7, 514. doi:10.3389/fphar.2016.00514
Panlilio, L. V., Yasar, S., Nemeth-Coslett, R., Katz, J. L., Henningfield, J. E., Solinas, M., et al. (2005). Human cocaine-seeking behavior and its control by drug-associated stimuli in the laboratory. Neuropsychopharmacology 30, 433–443. doi:10.1038/sj.npp.1300599
Parke, L. A., Burton, P., Mcdonald, R. V., Kim, J. A., and Siegel, S. (2002). Ibogaine interferes with motivational and somatic effects of naloxone-precipitated withdrawal from acutely administered morphine. Prog. Neuropsychopharmacol. Biol. Psychiatry 26, 293–297. doi:10.1016/s0278-5846(01)00268-8
Peck, K. R., Nighbor, T. D., and Price, M. (2022). Examining associations between impulsivity, opioid use disorder, and posttraumatic stress disorder: The additive relation between disorders. Exp. Clin. Psychopharmacol. 30, 486–493. doi:10.1037/pha0000507
Pehek, E. A., Nocjar, C., Roth, B. L., Byrd, T. A., and Mabrouk, O. S. (2006). Evidence for the preferential involvement of 5-HT2A serotonin receptors in stress- and drug-induced dopamine release in the rat medial prefrontal cortex. Neuropsychopharmacology 31, 265–277. doi:10.1038/sj.npp.1300819
Picker, M. J. (1997). Discriminative stimulus effects of the mixed-opioid agonist/antagonist dezocine: cross-substitution by mu and delta opioid agonists. J. Pharmacol. Exp. Ther. 283, 1009–1017.
Pineyro, G., and Nagi, K. (2021). Signaling diversity of mu- and delta-opioid receptor ligands: Re-evaluating the benefits of beta-arrestin/G protein signaling bias. Cell Signal 80, 109906. doi:10.1016/j.cellsig.2020.109906
Pitchers, K. K., Coppens, C. M., Beloate, L. N., Fuller, J., Van, S., Frohmader, K. S., et al. (2014). Endogenous opioid-induced neuroplasticity of dopaminergic neurons in the ventral tegmental area influences natural and opiate reward. J. Neurosci. 34, 8825–8836. doi:10.1523/JNEUROSCI.0133-14.2014
Pockros, L. A., Pentkowski, N. S., Conway, S. M., Ullman, T. E., Zwick, K. R., and Neisewander, J. L. (2012). 5-HT(2A) receptor blockade and 5-HT(2C) receptor activation interact to reduce cocaine hyperlocomotion and Fos protein expression in the caudate-putamen. Synapse 66, 989–1001. doi:10.1002/syn.21592
Pokorny, T., Duerler, P., Seifritz, E., Vollenweider, F. X., and Preller, K. H. (2020). LSD acutely impairs working memory, executive functions, and cognitive flexibility, but not risk-based decision-making. Psychol. Med. 50, 2255–2264. doi:10.1017/S0033291719002393
Pompeiano, M., Palacios, J. M., and Mengod, G. (1994). Distribution of the serotonin 5-HT2 receptor family mRNAs: comparison between 5-HT2A and 5-HT2C receptors. Brain Res. Mol. Brain Res. 23, 163–178. doi:10.1016/0169-328x(94)90223-2
Porter, R. H., Benwell, K. R., Lamb, H., Malcolm, C. S., Allen, N. H., Revell, D. F., et al. (1999). Functional characterization of agonists at recombinant human 5-HT2A, 5-HT2B and 5-HT2C receptors in CHO-K1 cells. Br. J. Pharmacol. 128, 13–20. doi:10.1038/sj.bjp.0702751
Preller, K. H., Herdener, M., Pokorny, T., Planzer, A., Kraehenmann, R., Stampfli, P., et al. (2017). The fabric of meaning and subjective effects in LSD-induced states depend on serotonin 2A receptor activation. Curr. Biol. 27, 451–457. doi:10.1016/j.cub.2016.12.030
Preller, K. H., Razi, A., Zeidman, P., Stampfli, P., Friston, K. J., and Vollenweider, F. X. (2019). Effective connectivity changes in LSD-induced altered states of consciousness in humans. Proc. Natl. Acad. Sci. U. S. A. 116, 2743–2748. doi:10.1073/pnas.1815129116
Preuss, C. V., Kalava, A., and King, K. C. (2023). “Prescription of controlled substances: Benefits and risks,” in StatPearls (Treasure Island (FL): StatPearls Publishing LLC.). StatPearls Publishing Copyright © 2023.
Price, A. E., Sholler, D. J., Stutz, S. J., Anastasio, N. C., and Cunningham, K. A. (2019). Endogenous serotonin 5-HT(2A) and 5-HT(2C) receptors associate in the medial prefrontal cortex. ACS Chem. Neurosci. 10, 3241–3248. doi:10.1021/acschemneuro.8b00669
Prospero-Garcia, O., Amancio-Belmont, O., Becerril Melendez, A. L., Ruiz-Contreras, A. E., and Mendez-Diaz, M. (2016). Endocannabinoids and sleep. Neurosci. Biobehav Rev. 71, 671–679. doi:10.1016/j.neubiorev.2016.10.005
Puretic, M. B., and Demarin, V. (2012). Neuroplasticity mechanisms in the pathophysiology of chronic pain. Acta Clin. Croat. 51, 425–429.
Quednow, B. B., Kometer, M., Geyer, M. A., and Vollenweider, F. X. (2012). Psilocybin-induced deficits in automatic and controlled inhibition are attenuated by ketanserin in healthy human volunteers. Neuropsychopharmacology 37, 630–640. doi:10.1038/npp.2011.228
Quirion, B., Bergeron, F., Blais, V., and Gendron, L. (2020). The delta-opioid receptor; a target for the treatment of pain. Front. Mol. Neurosci. 13, 52. doi:10.3389/fnmol.2020.00052
Rosenberg, D. E., Wolbach, A. B., Miner, E. J., and Isbell, H. (1963). Observations on direct and cross tolerance with LSD and d-amphetamine in man. Psychopharmacologia 5, 1–15. doi:10.1007/BF00405570
Ramaekers, J. G., Hutten, N., Mason, N. L., Dolder, P., Theunissen, E. L., Holze, F., et al. (2021). A low dose of lysergic acid diethylamide decreases pain perception in healthy volunteers. J. Psychopharmacol. 35, 398–405. doi:10.1177/0269881120940937
Rasmussen, K., White, D. A., and Acri, J. B. (2019). NIDA's medication development priorities in response to the Opioid Crisis: ten most wanted. Neuropsychopharmacology 44, 657–659. doi:10.1038/s41386-018-0292-5
Rickli, A., Luethi, D., Reinisch, J., Buchy, D., Hoener, M. C., and Liechti, M. E. (2015). Receptor interaction profiles of novel N-2-methoxybenzyl (NBOMe) derivatives of 2,5-dimethoxy-substituted phenethylamines (2C drugs). Neuropharmacology 99, 546–553. doi:10.1016/j.neuropharm.2015.08.034
Rickli, A., Moning, O. D., Hoener, M. C., and Liechti, M. E. (2016). Receptor interaction profiles of novel psychoactive tryptamines compared with classic hallucinogens. Eur. Neuropsychopharmacol. 26, 1327–1337. doi:10.1016/j.euroneuro.2016.05.001
Rolland, B., Jardri, R., Amad, A., Thomas, P., Cottencin, O., and Bordet, R. (2014). Pharmacology of hallucinations: several mechanisms for one single symptom? Biomed. Res. Int. 2014, 307106. doi:10.1155/2014/307106
Rothman, R. B., and Baumann, M. H. (2009). Serotonergic drugs and valvular heart disease. Expert Opin. Drug Saf. 8, 317–329. doi:10.1517/14740330902931524
Sadzot, B., Baraban, J. M., Glennon, R. A., Lyon, R. A., Leonhardt, S., Jan, C. R., et al. (1989). Hallucinogenic drug interactions at human brain 5-HT2 receptors: implications for treating LSD-induced hallucinogenesis. Psychopharmacol. Berl. 98, 495–499. doi:10.1007/BF00441948
Sakloth, F., Leggett, E., Moerke, M. J., Townsend, E. A., Banks, M. L., and Negus, S. S. (2019). Effects of acute and repeated treatment with serotonin 5-HT2A receptor agonist hallucinogens on intracranial self-stimulation in rats. Exp. Clin. Psychopharmacol. 27, 215–226. doi:10.1037/pha0000253
Samhsa (2021). Substance Abuse and Mental Health Services Administration. Key substance use and mental health indicators in the United States: results from the 2020 national survey on drug use and health. HHS Publication No. PEP21-07-01-003, NSDUH Series H-56.
Santana, N., Bortolozzi, A., Serrats, J., Mengod, G., and Artigas, F. (2004). Expression of serotonin1A and serotonin2A receptors in pyramidal and GABAergic neurons of the rat prefrontal cortex. Cereb. Cortex 14, 1100–1109. doi:10.1093/cercor/bhh070
Sasaki, M., Kamiya, Y., Bamba, K., Onishi, T., Matsuda, K., Kohno, T., et al. (2021). Serotonin plays a key role in the development of opioid-induced hyperalgesia in mice. J. Pain 22, 715–729. doi:10.1016/j.jpain.2020.12.008
Savage, C., and Mccabe, O. L. (1973). Residential psychedelic (LSD) therapy for the narcotic addict. A controlled study. Arch. Gen. Psychiatry 28, 808–814. doi:10.1001/archpsyc.1973.01750360040005
Schlemmer, R. F., Nawara, C., Heinze, W. J., Davis, J. M., and Advokat, C. (1986). Influence of environmental context on tolerance to LSD-induced behavior in primates. Biol. Psychiatry 21, 314–317. doi:10.1016/0006-3223(86)90053-3
Schmidt, C. J., Sorensen, S. M., Kehne, J. H., Carr, A. A., and Palfreyman, M. G. (1995). The role of 5-HT2A receptors in antipsychotic activity. Life Sci. 56, 2209–2222. doi:10.1016/0024-3205(95)00210-w
Schwienteck, K. L., Faunce, K. E., Rice, K. C., Obeng, S., Zhang, Y., Blough, B. E., et al. (2019). Effectiveness comparisons of G-protein biased and unbiased mu opioid receptor ligands in warm water tail-withdrawal and drug discrimination in male and female rats. Neuropharmacology 150, 200–209. doi:10.1016/j.neuropharm.2019.01.020
Shalit, N., Rehm, J., and Lev-Ran, S. (2019). Epidemiology of hallucinogen use in the U.S. results from the national epidemiologic survey on alcohol and related conditions III. Addict. Behav. 89, 35–43. doi:10.1016/j.addbeh.2018.09.020
Sierra, S., Muchhala, K. H., Jessup, D. K., Contreras, K. M., Shah, U. H., Stevens, D. L., et al. (2022). Sex-specific role for serotonin 5-HT(2A) receptor in modulation of opioid-induced antinociception and reward in mice. Neuropharmacology 209, 108988. doi:10.1016/j.neuropharm.2022.108988
Silverstein, S. M., and Lai, A. (2021). The phenomenology and neurobiology of visual distortions and hallucinations in schizophrenia: An update. Front. Psychiatry 12, 684720. doi:10.3389/fpsyt.2021.684720
Singh, K., Sona, C., Ojha, V., Singh, M., Mishra, A., Kumar, A., et al. (2019). Identification of dual role of piperazine-linked phenyl cyclopropyl methanone as positive allosteric modulator of 5-HT(2C) and negative allosteric modulator of 5-HT(2B) receptors. Eur. J. Med. Chem. 164, 499–516. doi:10.1016/j.ejmech.2018.12.070
Singh, V. P., Jain, N. K., and Kulkarni, S. K. (2003). Fluoxetine suppresses morphine tolerance and dependence: modulation of NO-cGMP/DA/serotoninergic pathways. Methods Find. Exp. Clin. Pharmacol. 25, 273–280. doi:10.1358/mf.2003.25.4.769675
Slocum, S. T., Diberto, J. F., and Roth, B. L. (2022). Molecular insights into psychedelic drug action. J. Neurochem. 162, 24–38. doi:10.1111/jnc.15540
Smith, R. L., Barrett, R. J., and Sanders-Bush, E. (2003). Discriminative stimulus properties of 1-(2,5-dimethoxy-4-iodophenyl)-2-aminopropane [(+/-)DOI] in C57BL/6J mice. Psychopharmacol. Berl. 166, 61–68. doi:10.1007/s00213-002-1252-6
Snyder, L. M., Chiang, M. C., Loeza-Alcocer, E., Omori, Y., Hachisuka, J., Sheahan, T. D., et al. (2018). Kappa Opioid Receptor Distribution and Function in Primary Afferents. Neuron 99, 1274–1288. doi:10.1016/j.neuron.2018.08.044
Soria-Gomez, E., Marquez-Diosdado, M. I., Montes-Rodriguez, C. J., Estrada-Gonzalez, V., and Prospero-Garcia, O. (2010). Oleamide administered into the nucleus accumbens shell regulates feeding behaviour via CB1 and 5-HT2C receptors. Int. J. Neuropsychopharmacol. 13, 1247–1254. doi:10.1017/S1461145710000702
Soto, C. A., Huang-Chi, D., Fox, R. G., Yang, T., Hooson, J., Anastasio, N. C., et al. (2019). In vivo and in vitro analyses of novel peptidomimetic disruptors for the serotonin 5-HT2C receptor interaction with phosphatase and tensin homolog (PTEN). Front. Pharmacol. 10, 907. doi:10.3389/fphar.2019.00907
Spanagel, R. (2017). Animal models of addiction. Dialogues Clin. Neurosci. 19, 247–258. doi:10.31887/DCNS.2017.19.3/rspanagel
Stoeber, M., Jullie, D., Lobingier, B. T., Laeremans, T., Steyaert, J., Schiller, P. W., et al. (2018). A genetically encoded biosensor reveals location bias of opioid drug action. Neuron 98, 963–976. doi:10.1016/j.neuron.2018.04.021
Stoops, W. W. (2022). A brief introduction to human behavioral pharmacology: Methods, design considerations and ethics. Perspect. Behav. Sci. 45, 361–381. doi:10.1007/s40614-022-00330-5
Strang, J., Volkow, N. D., Degenhardt, L., Hickman, M., Johnson, K., Koob, G. F., et al. (2020). Opioid use disorder. Nat. Rev. Dis. Prim. 6, 3. doi:10.1038/s41572-019-0137-5
Sughondhabirom, A., Jain, D., Gueorguieva, R., Coric, V., Berman, R., Lynch, W. J., et al. (2005). A paradigm to investigate the self-regulation of cocaine administration in humans. Psychopharmacol. Berl. 180, 436–446. doi:10.1007/s00213-005-2192-8
Sullivan, M. D., and Ballantyne, J. C. (2022). Understanding the risks of long-term opioid therapy for chronic pain. Am. J. Psychiatry 179, 696–698. doi:10.1176/appi.ajp.20220592
Tagen, M., Mantuani, D., Van Heerden, L., Holstein, A., Klumpers, L. E., and Knowles, R. (2023). The risk of chronic psychedelic and MDMA microdosing for valvular heart disease. J. Psychopharmacol. 0, 2698811231190865. doi:10.1177/02698811231190865
Taki, K., Kaneko, T., and Mizuno, N. (2000). A group of cortical interneurons expressing mu-opioid receptor-like immunoreactivity: a double immunofluorescence study in the rat cerebral cortex. Neuroscience 98, 221–231. doi:10.1016/s0306-4522(00)00124-x
Tao, R., and Auerbach, S. B. (2002a). GABAergic and glutamatergic afferents in the dorsal raphe nucleus mediate morphine-induced increases in serotonin efflux in the rat central nervous system. J. Pharmacol. Exp. Ther. 303, 704–710. doi:10.1124/jpet.102.038133
Tao, R., and Auerbach, S. B. (1995). Involvement of the dorsal raphe but not median raphe nucleus in morphine-induced increases in serotonin release in the rat forebrain. Neuroscience 68, 553–561. doi:10.1016/0306-4522(95)00154-b
Tao, R., and Auerbach, S. B. (2002b). Opioid receptor subtypes differentially modulate serotonin efflux in the rat central nervous system. J. Pharmacol. Exp. Ther. 303, 549–556. doi:10.1124/jpet.102.037861
Tao, R., Karnik, M., Ma, Z., and Auerbach, S. B. (2003). Effect of fentanyl on 5-HT efflux involves both opioid and 5-HT1A receptors. Br. J. Pharmacol. 139, 1498–1504. doi:10.1038/sj.bjp.0705378
Tao, R., Ma, Z., and Auerbach, S. B. (1998). Alteration in regulation of serotonin release in rat dorsal raphe nucleus after prolonged exposure to morphine. J. Pharmacol. Exp. Ther. 286, 481–488.
Tempel, A., and Zukin, R. S. (1987). Neuroanatomical patterns of the mu, delta, and kappa opioid receptors of rat brain as determined by quantitative in vitro autoradiography. Proc. Natl. Acad. Sci. U. S. A. 84, 4308–4312. doi:10.1073/pnas.84.12.4308
Thomas, E. A., Carson, M. J., Neal, M. J., and Sutcliffe, J. G. (1997). Unique allosteric regulation of 5-hydroxytryptamine receptor-mediated signal transduction by oleamide. Proc. Natl. Acad. Sci. U. S. A. 94, 14115–14119. doi:10.1073/pnas.94.25.14115
Thurner, P., Stary-Weinzinger, A., Gafar, H., Gawali, V. S., Kudlacek, O., Zezula, J., et al. (2014). Mechanism of hERG channel block by the psychoactive indole alkaloid ibogaine. J. Pharmacol. Exp. Ther. 348, 346–358. doi:10.1124/jpet.113.209643
Tolomeo, S., Davey, F., Steele, J. D., and Baldacchino, A. (2021). Compulsivity and impulsivity in opioid dependence. Drug Alcohol Depend. 229, 109018. doi:10.1016/j.drugalcdep.2021.109018
Trigo, J. M., Martin-Garcia, E., Berrendero, F., Robledo, P., and Maldonado, R. (2010). The endogenous opioid system: a common substrate in drug addiction. Drug Alcohol Depend. 108, 183–194. doi:10.1016/j.drugalcdep.2009.10.011
Troxler, F., and Hofmann, A. (1957). Substitutionen am ringsystem der lysergsäure. III. Halogenierung. 45. Mitteilung über Mutterkornalkaloide. Helvetica Chim. Acta 40, 2160–2170. doi:10.1002/hlca.19570400716
Trulson, M. E., and Jacobs, B. L. (1977). Usefulness of an animal behavioral model in studying the duration of action of LSD and the onset and duration of tolerance to LSD in the cat. Brain Res. 132, 315–326. doi:10.1016/0006-8993(77)90424-3
Turner, W. J., Almudevar, M., and Merlis, S. (1959). Chemotherapeutic trials in psychosis. III. Addendum-2-brom-d-lysergic acid diethylamide (BOL). Am. J. Psychiatry 116, 261–262. doi:10.1176/ajp.116.3.261
Ubaldi, M., Cannella, N., Borruto, A. M., Petrella, M., Micioni Di Bonaventura, M. V., Soverchia, L., et al. (2021). Role of nociceptin/orphanin FQ-NOP receptor system in the regulation of stress-related disorders. Int. J. Mol. Sci. 22, 12956. doi:10.3390/ijms222312956
Ujcikova, H., Eckhardt, A., Hejnova, L., Novotny, J., and Svoboda, P. (2021). Alterations in the proteome and phosphoproteome profiles of rat hippocampus after six months of morphine withdrawal: Comparison with the forebrain cortex. Biomedicines 10, 80. doi:10.3390/biomedicines10010080
Urban, M. M., Stingl, M. R., and Meinhardt, M. W. (2023). Mini-review: The neurobiology of treating substance use disorders with classical psychedelics. Front. Neurosci. 17, 1156319. doi:10.3389/fnins.2023.1156319
Vearrier, D., and Grundmann, O. (2021). Clinical pharmacology, toxicity, and abuse potential of opioids. J. Clin. Pharmacol. 61 (2), S70–S88. doi:10.1002/jcph.1923
Valentino, R. J., and Volkow, N. D. (2018). Untangling the complexity of opioid receptor function. Neuropsychopharmacology 43, 2514–2520. doi:10.1038/s41386-018-0225-3
Vanover, K. E., Weiner, D. M., Makhay, M., Veinbergs, I., Gardell, L. R., Lameh, J., et al. (2006). Pharmacological and behavioral profile of N-(4-fluorophenylmethyl)-N-(1-methylpiperidin-4-yl)-N'-(4-(2-methylpropylo xy)phenylmethyl) carbamide (2R,3R)-dihydroxybutanedioate (2:1) (ACP-103), a novel 5-hydroxytryptamine(2A) receptor inverse agonist. J. Pharmacol.Exp.Ther. 317, 910–918. doi:10.1124/jpet.105.097006
Vazquez-Borsetti, P., Celada, P., Cortes, R., and Artigas, F. (2011). Simultaneous projections from prefrontal cortex to dopaminergic and serotonergic nuclei. Int. J. Neuropsychopharmacol. 14, 289–302. doi:10.1017/S1461145710000349
Vazquez-Borsetti, P., Cortes, R., and Artigas, F. (2009). Pyramidal neurons in rat prefrontal cortex projecting to ventral tegmental area and dorsal raphe nucleus express 5-HT2A receptors. Cereb. Cortex 19, 1678–1686. doi:10.1093/cercor/bhn204
Vendruscolo, J. C. M., Tunstall, B. J., Carmack, S. A., Schmeichel, B. E., Lowery-Gionta, E. G., Cole, M., et al. (2018). Compulsive-like sufentanil vapor self-administration in rats. Neuropsychopharmacology 43, 801–809. doi:10.1038/npp.2017.172
Vetulani, J., Bednarczyk, B., Reichenberg, K., and Rokosz, A. (1980). Head twitches induced by LSD and quipazine: Similarities and differences. Neuropharmacology 19, 155–158. doi:10.1016/0028-3908(80)90131-8
Volkow, N. D., and Mclellan, A. T. (2016). Opioid Abuse in Chronic Pain--Misconceptions and Mitigation Strategies. N. Engl. J. Med. 374, 1253–1263. doi:10.1056/NEJMra1507771
Von Korff, M., Kolodny, A., Deyo, R. A., and Chou, R. (2011). Long-term opioid therapy reconsidered. Ann. Intern Med. 155, 325–328. doi:10.7326/0003-4819-155-5-201109060-00011
Vowles, K. E., Mcentee, M. L., Julnes, P. S., Frohe, T., Ney, J. P., and Van Der Goes, D. N. (2015). Rates of opioid misuse, abuse, and addiction in chronic pain: a systematic review and data synthesis. Pain 156, 569–576. doi:10.1097/01.j.pain.0000460357.01998.f1
Wakeman, S. E., Larochelle, M. R., Ameli, O., Chaisson, C. E., Mcpheeters, J. T., Crown, W. H., et al. (2020). Comparative effectiveness of different treatment pathways for opioid use disorder. JAMA Netw. Open 3, e1920622. doi:10.1001/jamanetworkopen.2019.20622
Walker, E. A., Chambers, C., Korber, M. G., Tella, S. R., Prioleau, C., and Fang, L. (2021). Antinociceptive and discriminative stimulus effects of six novel psychoactive opioid substances in male rats. J. Pharmacol. Exp. Ther. 379, 1–11. doi:10.1124/jpet.121.000689
White, F. J., and Appel, J. B. (1982). Lysergic acid diethylamide (LSD) and lisuride: differentiation of their neuropharmacological actions. Science 216, 535–537. doi:10.1126/science.7071600
White, F. J. (1986). Comparative effects of LSD and lisuride: clues to specific hallucinogenic drug actions. Pharmacol. Biochem. Behav. 24, 365–379. doi:10.1016/0091-3057(86)90367-9
Wild, C. T., Miszkiel, J. M., Wold, E. A., Soto, C. A., Ding, C., Hartley, R. M., et al. (2019). Design, synthesis, and characterization of 4-undecylpiperidine-2-carboxamides as positive allosteric modulators of the serotonin (5-HT) 5-HT(2C) receptor. J. Med. Chem. 62, 288–305. doi:10.1021/acs.jmedchem.8b00401
Williams, J. T., Christie, M. J., and Manzoni, O. (2001). Cellular and synaptic adaptations mediating opioid dependence. Physiol. Rev. 81, 299–343. doi:10.1152/physrev.2001.81.1.299
Willins, D. L., and Meltzer, H. Y. (1997). Direct injection of 5-HT2A receptor agonists into the medial prefrontal cortex produces a head-twitch response in rats. J. Pharmacol. Exp. Ther. 282, 699–706.
Winter, J. C. (1980). Effects of the phenethylamine derivatives, BL-3912, fenfluramine, and Sch-12679, in rats trained with LSD as a discriminative stimulus. Psychopharmacol. Berl. 68, 159–162. doi:10.1007/BF00432134
Wold, E. A., Chen, J., Cunningham, K. A., and Zhou, J. (2019a). Allosteric modulation of class A GPCRs: Targets, agents, and emerging concepts. J. Med. Chem. 62, 88–127. doi:10.1021/acs.jmedchem.8b00875
Wold, E. A., Garcia, E. J., Wild, C. T., Miszkiel, J. M., Soto, C. A., Chen, J., et al. (2020). Discovery of 4-phenylpiperidine-2-carboxamide analogues as serotonin 5-HT(2C) receptor-positive allosteric modulators with enhanced drug-like properties. J. Med. Chem. 63, 7529–7544. doi:10.1021/acs.jmedchem.9b01953
Wold, E. A., Wild, C. T., Cunningham, K. A., and Zhou, J. (2019b). Targeting the 5-HT2C receptor in biological context and the current state of 5-HT2C receptor ligand development. Curr. Top. Med. Chem. 19, 1381–1398. doi:10.2174/1568026619666190709101449
Wulff, A. B., Nichols, C. D., and Thompson, S. M. (2023). Preclinical perspectives on the mechanisms underlying the therapeutic actions of psilocybin in psychiatric disorders. Neuropharmacology 231, 109504. doi:10.1016/j.neuropharm.2023.109504
Yadlapalli, J. S. K., Bommagani, S. B., Mahelona, R. D., Wan, A., Gannon, B. M., Penthala, N. R., et al. (2018). Evaluation of morphine-like effects of the mixed mu/delta agonist morphine-6-O-sulfate in rats: Drug discrimination and physical dependence. Pharmacol. Res. Perspect. 6, e00403. doi:10.1002/prp2.403
Yamamoto, H., Kamegaya, E., Hagino, Y., Takamatsu, Y., Sawada, W., Matsuzawa, M., et al. (2017). Loss of GluN2D subunit results in social recognition deficit, social stress, 5-HT2C receptor dysfunction, and anhedonia in mice. Neuropharmacology 112, 188–197. doi:10.1016/j.neuropharm.2016.07.036
Zamberlan, F., Sanz, C., Martinez Vivot, R., Pallavicini, C., Erowid, F., Erowid, E., et al. (2018). The varieties of the psychedelic experience: A preliminary study of the association between the reported subjective effects and the binding affinity profiles of substituted phenethylamines and tryptamines. Front. Integr. Neurosci. 12, 54. doi:10.3389/fnint.2018.00054
Zaveri, N. T. (2016). Nociceptin opioid receptor (NOP) as a therapeutic target: Progress in translation from preclinical research to clinical utility. J. Med. Chem. 59, 7011–7028. doi:10.1021/acs.jmedchem.5b01499
Zhang, X. Y., Dou, Y. N., Yuan, L., Li, Q., Zhu, Y. J., Wang, M., et al. (2020). Different neuronal populations mediate inflammatory pain analgesia by exogenous and endogenous opioids. Elife 9, e55289. doi:10.7554/eLife.55289
Zhou, J., Cunningham, K. A., Bolinger, A. A., and Anastasio, N. C. (2023). Novel heterocyclic compounds as serotonin (5-HT) 5-HT2A and 5-HT2C receptor positive allosteric modulators. United States patent application.
Keywords: opioid use disorder, serotonin, 5-HT2AR, MOR, psychedelics
Citation: Salinsky LM, Merritt CR, Zamora JC, Giacomini JL, Anastasio NC and Cunningham KA (2023) μ-opioid receptor agonists and psychedelics: pharmacological opportunities and challenges. Front. Pharmacol. 14:1239159. doi: 10.3389/fphar.2023.1239159
Received: 12 June 2023; Accepted: 28 September 2023;
Published: 11 October 2023.
Edited by:
Cody Wenthur, University of Wisconsin-Madison, United StatesReviewed by:
Thomas M. Keck, Rowan University, United StatesCopyright © 2023 Salinsky, Merritt, Zamora, Giacomini, Anastasio and Cunningham. This is an open-access article distributed under the terms of the Creative Commons Attribution License (CC BY). The use, distribution or reproduction in other forums is permitted, provided the original author(s) and the copyright owner(s) are credited and that the original publication in this journal is cited, in accordance with accepted academic practice. No use, distribution or reproduction is permitted which does not comply with these terms.
*Correspondence: Noelle C. Anastasio, bmNhbmFzdGFAdXRtYi5lZHU=; Kathryn A. Cunningham, a2N1bm5pbmdAdXRtYi5lZHU=
†These authors share first authorship