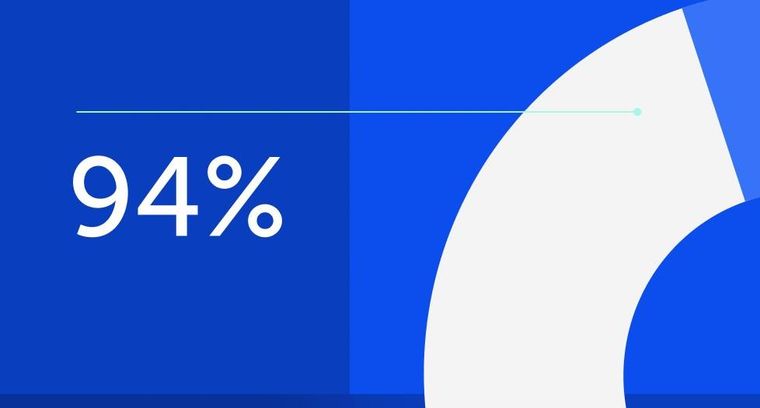
94% of researchers rate our articles as excellent or good
Learn more about the work of our research integrity team to safeguard the quality of each article we publish.
Find out more
REVIEW article
Front. Pharmacol., 10 January 2024
Sec. Ethnopharmacology
Volume 14 - 2023 | https://doi.org/10.3389/fphar.2023.1231006
This article is part of the Research TopicChemistry, Toxicity, Synthesis, Biological and Pharmacological Activities of Coumarins and their Derivatives: Recent Advances and Future PerspectivesView all 8 articles
Introduction: Coumarins are naturally occuring metabolites from plants and a few micro-organisms. They have been widely used in the food and drug industry in their natural or synthetic forms. Numerous coumarins possess several biological activities such as anti-inflammatory, anti-ulcers, anti-tumour, anti-microbial, anti-coagulant. The aim of this study was to assess the bioactivity, and toxicity of coumarins from African medicinal plants.
Methods: We searched online databases and search engines such as PubMed, Google Scholar and Web of Science for key terms such as coumarins, toxicity, bioavailability, bioactivity with appropriate Boolean operators. Only full-length research articles published in English between 1956 to 2023 were reviewed.
Results: We recorded 22 coumarins from 15 plant species from Africa. Most of the plant species (33%) were from North Africa. These were followed by East Africa at 21%, then West, and Central Africa at 18.2% each. Most of the coumarins (21.3%) were isolated from the entire plant and the leaves (19.1%) and most of them (46.7%) had some antimicrobial activity. Five coumarins viz osthole, pseudocordatolide C & calanolide, chartreusin and esculetin had either antitumor or anticancer activity. Six coumarins had varying levels and types of toxicity ranging from inhibiting blood clotting as anticoagulants, to cytotoxic effects, causing hyperventilation, tremor, & photophobia, pulmonary haemorrhage, carcinogenic activity, severe neurotoxicity, hepato- and phototoxicity.
Conclusion: Several African medicinal plants are sources of various coumarins that possess several biological activities as well as toxicities. This calls for more research into their safety and efficacy because of their wide spread applications as therapeutic agents.
Coumarins are naturally occurring metabolites in a variety of plants, micro-organisms and in some animal species (Perone, 2016). Coumarin (1,2-benzopyrone; 2H-1-benzopyran-2-one; cis-o-coumarinic acid lactone) is also known as benzopyrone or coumarinic anhydride and tonka bean camphor. It is a white crystalline solid, belonging to the class of lactones. It consists of an aromatic ring fused to a condensed lactone ring (Venugopala et al., 2013).
Coumarins usually occur in plants as glycosides and esters but mostly occur in free form. Coumaric metabolites are lactones of 2-coumaric acid (2-hydroxy-Z-cinnamic acid) and are constructed by a benzene ring fused to an α-pyrone ring (Figure 1) (Matos et al., 2015). Coumarins are very complex and diverse metabolites. Naturally occurring coumarins may be categorized as coumarins, isocoumarins, furanocoumarins, pyranocoumarins, biscoumarins, and phenylcoumarins (Lacy and O’kennedy, 2004; Zhu and Jiang, 2018) and synthetic pyrone-substituted coumarins (Lacy and O’kennedy, 2004).
The chemical formula of coumarins is C9H6O2, and they can exist in various isomeric forms due to differences in the substitution pattern on the benzene and the pyrone ring (Wu et al., 2009). The presence or absence of particular functional groups confers the coumarin its name and properties, such as simple coumarins which are the basic coumarin metabolites with no additional substituents. These include hydroxycoumarins like scopoletin and umbelliferone, methoxycoumarins like esculetin and 7-methoxycoumarin and alkyl coumarins like daphnetin and aesculetin (Wu et al., 2009). Coumarins are variably soluble in most organic solvents or their combinations (Huang et al., 2015) but are freely soluble in ethanol, chloroform, diethyl ether and oils (Jung and Oh, 2011).
Coumarin occurs naturally in many plants and confers a pleasant spice aroma akin to vanilla (Egan et al., 1990). Coumarins were first isolated from Dipteryx odorata (Aubl.) Forsyth f (tonka beans) by Vogel in 1820 (Doctor et al., 2020). They also occur in high concentrations in some essential oils, such as Cinnamomum aromaticum (cinnamon bark oil) and Cassia fistula L. (cassia leaf oil). Coumarins have been identified in various plant species from many different families including the fruits of Vaccinium myrtillus L. (bilberry), Rubus chamaemorus L (cloudberry), Camellia sinensis (L.) Kuntze (green tea), Melilotus albus Medik. and Melilotus officinalis (L.) Lam (sweet clover), Galium odoratum (L.) Scop(sweet woodruff), Vanilla planifolia Andrews (vanilla leaves and beans), Lavendula officinalis subsp. angustifolium (lavender) and Myroxylon pereirae (L.) Harms (balsam of Peru) (Kostova et al., 2011; Kicel and Wolbis, 2012). A broad spectrum of coumarins present in both free state and as glucosides have also been found in many other plant species. Several coumarins have been identified, principally as secondary metabolites in green plants, but the exact number is hard to determine due to synthetic coumarins created regularly (Kostova, 2005).
Most commercially used coumarins were synthesized from salicylaldehyde (DeGarmo and Raizman, 1967). High-grade coumarin is still isolated from tonka beans using various methods such as hot water steeping and sub critical water extraction (Doctor et al., 2020). Coumarins are extremely variable in structure leading to various pharmacological activities (Lacy and O’kennedy, 2004). The simplicity and versatility of the coumarin scaffold makes it a convenient starting-point for a wide range of applications (Stefanachi et al., 2018; Annunziata et al., 2020).
Although coumarins are widely distributed in the plant kingdom, most of the commercially used ones have been synthetically produced for many years. Coumarins have several applications such as in the perfumery, cosmetic and related industries (Givel, 2003). Large quantities of coumarin, mostly associated with vanillin, are used in the food industry for flavouring chocolates, baked goods, and in flavoured beverages (Givel, 2003). However, since 1954, synthetic vanillin use as a direct additive in tobacco products has been suspended in the United States by the FDA due to the production of hazardous compounds when burnt (Riveiro et al., 2010; Sarker and Nahar, 2017; Prusty and Kumar, 2020). Because of their unique sweet note and stability, coumarins have long been recognized as an important raw material in the fragrance industry as odour-enhancers. They help to achieve a long-lasting effect when combined with natural essential oils (Doctor et al., 2020). As such, they are widely used in hand soaps, detergents, lotions and perfumes, in tobacco to enhance its natural aroma, in toothpastes, antiperspirant deodorants, creams, hair sprays, shampoos and many other household products (Perone, 2016). Large quantities of coumarins are used in rubber and plastic materials and in paints and sprays to neutralize unpleasant odours (Kirsch et al., 2016). Coumarins also have significant uses in electroplating, mostly in the automotive industry, to provide high polished quality chrome-plated steel. However, this use has been on the decline (Kirsch et al., 2016).
Coumarins have several pharmacological properties with applications in the medical field (Skalicka-Woźniak et al., 2016), as a cardiovascular targeting drug (Najmanova et al., 2015), and as a cancer screening molecule and therapy (Lacy and O'Kennedy, 2004). Coumarins possess both immunomodulatory and antitumor activity (Riveiro et al., 2010). They have been recommended for treatment of a number of clinical conditions, including high protein oedema and brucellosis (Luszczki et al., 2009; Riveiro et al., 2010; Raja et al., 2011). Coumarins such as osthole have undergone clinical trials for treatment of lymphoedema (Farinola and Piller, 2005), following breast cancer treatment and in treatment of lung and kidney cancer and melanoma alone or in combination with cimetidine (Riveiro et al., 2010; Prusty and Kumar, 2020). Coumarins have also been used for prevention of dental caries (Perone, 2016). Coumarins have been tested for treatment of schizophrenia, microcirculation disorders and angiopathic ulcers (Skalicka-Woźniak et al., 2016). The vitamin K epoxide reductase (VKORC1), the key enzyme of the vitamin K cycle is the molecular target of coumarins (Oldenburg et al., 2007). Thus, the objective of this study was to assess the bioactivity, and toxicity of coumarins from African medicinal plants.
We performed a literature search using PubMed, Google Scholar and Web of Science. We searched for terms such as coumarins, toxicity, bioavialability, bioactivity with appropriate Boolean operators. Only full-length articles published in English between 1956–2023 were reviewed. The review was limited to African medicinal plant species only. We used only original research articles or review papers and excluded results from in silico studies.
We recorded 22 coumarins that were isolated from 15 plant species (Table 1). Most of the plant species (33%) were from North Africa, followed by East Africa (21%), then West, and Central Africa at 18.2% each. Most of these coumarins (21.3%) were isolated from the whole plant. These were followed by the leaves (19.1%), then seeds, fruits and roots each at 12.8%. However, only two plant species Mammea africana Sabine and Tetrapleura tetraptera (Schumach. & Thonn.) Taub. had a more restricted native range to Africa. The rest of the plant species were more widely distributed in different parts of the world. The distribution of the plant species was checked with the Kew database at https://powo.science.kew.org. All the coumarins showed multiple bioactivities. For instance, esculin had more than six bioactivities. Ten different coumarins had antimicrobial activity including antibacterial, antiviral, antifungal or anti-parasitic activity. Bicoumarin/Biscoumarin and dicoumarol had anticoagulant activity while three coumarins: esculetin, scopoletin, dicoumarol had anti-inflammatory activity. Five coumarins: osthole, pseudocordatolide C and calanolide, chartreusin and esculetin had either antitumor or anticancer activity.
Six coumarins (dicoumarol, coumarin, osthole, esculetin, psoralen, bergapten, Figures 2A–F) had varying levels and types of toxicity ranging from excessive bleeding due to inhibition of blood clotting (anticoagulants), cytotoxic effects causing hyperventilation, tremor, & photophobia, pulmonary haemorrhage, carcinogenic activity, severe neurotoxicity, hepatotoxicity and phototoxicity (Table 2). Osthole, a natural coumarin isolated from dried fruits of Cnidium monnieri (L.) Cusson, had anti-allergic activity.
FIGURE 2. (A) Dicoumarol (4-Hyroxycoumarin) (B) Coumarin (C) Osthenol (D) Esculetin (E) Psoralen (F) Bergapten (Ulrich et al. 1999).
Osthole, esculin, dihydroxycoumarin and many other coumarins have shown beneficial biochemical profiles in relation to pathophysiological processes dependent upon reactive oxygen species due to the possession of amine, and hydroxy groups (Kostova et al., 2011). A study by Abdizadeh et al. (2020) evaluated the photoprotective activity of some hydroxycoumarins as compared with p-aminobenzoic acid (PABA) as a model sun screen. The activity could be related to their antioxidant actions which could minimize skin photoaging (Abdizadeh et al., 2020). Another study by Mitra et al. (2013) compared the antioxidative activities of seven hydrocoumarins with those of α-tocopherol for the oxidation of tetralin and linoleic acid in a homogeneous solution. Hydrocoumarins exhibited a higher induction period than α-tocopherol in both systems. However, the rate of oxygen absorption during the induction period for the latter was slower than that of the hydrocoumarins in both systems. In addition, 6,7-dihydroxy-4,4-dimethylhydrocoumarin showed less cytotoxicity toward human fibroblasts than 2, 6-di-t-butyl4-methylphenol (Kostova et al., 2011).
Although different studies have confirmed the antimicrobial activities of various coumarins from plants (Vaou et al., 2021; Ojala et al., 1999; Karakaya et al., 2019), such studies are lacking of African medicinal plants. Some coumarin antibiotics of natural origin, although not necessarily from African plant species have been developed into modern antibiotics. These include NOVOBIOCIN™ and CLOROBIOCIN™ which are inhibitors of DNA gyrase. They both have a broad spectrum towards Gram-positive bacteria, including methicilin resistant strains of staphylococci species (Raja et al., 2011; Matos et al., 2012). This shows the potential of African plant species as a source of coumarin based drugs. Due to some limitations of these metabolites particularly with regard to solubility, toxicity and development of resistance, a novel series of coumarin analogues has been synthesized (Kirsch et al., 2016). The ester or carboxylic acid on the coumarin ring was found to be important for the inhibitory activity against Gram-positive and Gram-negative bacteria (Chiang et al., 2010; Annunziata et al., 2020). The presence of phenolic hydroxyl groups and or carboxylic acid was found necessary for enhanced activity against Helicobacter pylori (Venugopala et al., 2013).
Several coumarins like esculetin, dicoumarol isolated from plants or of synthetic origin possess significant anti-inflammatory and or analgesic activities (Kirsch et al., 2016). In a Quantitative Structure-Activity Relationship (QSAR) study for lead optimization in the design of coumarins as potent non-steroidal anti-inflammatory agents, the coumarin positions C4- and C7- contributed to the high activity (Poumale et al., 2013). Natural products such as esculetin, fraxetin, inhibit lipoxygenase and cyclooxygenase enzymic systems and neutrophil-dependent superoxide anion generation (Kontogiorgis and Hadjipavlou-Litina, 2005).
This activity focuses essentially on the inhibition of HIV-1 protease (HIV-PR) and HIV-1 integrase (Sancho et al., 2004). PHENPROCOUMON™, warfarin and substituted 4-hydroxy-2-pyrone derivatives are actually referred to as the first generation of HIV-PR inhibitors (Hassan et al., 2016). Certain coumarin dimers, particularly those containing hydrophobic moieties on the linker, display potent inhibitory activity against HIV-1 integrase (Sancho et al., 2004). Calanolide A and B are semi-synthetic coumarins on market used as combination therapies for HIV. Psoralen was found to be a very potent anti-HIV drug during research but was discontinued for its high hepatotoxicity in mice (Sancho et al., 2004; Guo et al., 2020). Phenprocoumon emerged as a novel lead template possessing weak HIV protease inhibitory activity (Hassan et al., 2016). TRIOXSALEN™ was then developed and marketed as chemical derivative of psoralen despite its photosensitization of the skin (Wishart et al., 2018).
Osthole, umbelliferon, esculetin and geiparvarin all showed anti-tumor activity. Hydroxycoumarin might be a useful adjuvant for melanoma therapy because of its selective anti-proliferative agents that mediate apoptosis in renal carcinoma cells, through modulation of mitogen-activated protein kinases attributed to a nitro group in the aromatic ring (Kostova, 2005; Jung and Oh, 2011). In an attempt by Zhang et al. (2021) to develop novel antitumor candidates, a series of coumarin sulfonamides and amides derivatives were designed and synthetized. The majority of these metabolites showed good cytotoxic activity against MDA-MB-231 and kB cell lines (Zhang et al., 2021). Menezes and Diederich, (2019) conducted an extensive review on the role of natural coumarins as anticancer agents. They showed how coumarins modulate several targets such as carbonic anhydrase, Hsp90, histone deacetylase and topoisomerase enzyme in cancer cells leading to apoptosis.
Some natural and synthetic coumarins are cholinesterase inhibitors, which are a promising approach for the treatment of Alzheimer´s disease and possible therapeutic applications in the treatment of Parkinsons´s disease (Anand et al., 2012). A series of umbelliferon metabolites and synthetic coumarins were evaluated as inhibitors of monoaminooxigenase (MAO) and steroid 5α reductase type I in cell culture systems (Anand et al., 2012). Ether derivatives demonstrate MAO-B selectivity, and sulfonic ester derivatives demonstrate MAO-A selectivity by QSAR studies (Koyiparambath et al., 2021). Calanolide-A drugs are marketed for their ability to block activity of cytochrome P450 3A4, while ethyl biscoumacetate is a glutamine synthase inhibitor (Wishart et al., 2018; Sai et al., 1999).
Many coumarins have different effects on blood. Most notable is warfarin, which has anti-coagulant properties (Hosseini et al., 2015). Warfarin drugs are used for the management of thromboembolism and pulmonary embolism with atrial fibrillation (Wishart et al., 2018). Ethyl biscoumacetate is a semi-synthetic compound used as anti-coagulant alternative to warfarin due to fewer side effects (Sun et al., 2020). Esculin and its metabolites are used as vasoprotective agents (Kostova, 2005). Moreover, other coumarins like biscoumarin/bicoumarin have similar activity to ethyl biscoumacetate and esculin (Sun et al., 2020; Najmanova et al., 2015).
There is a large body of data on the toxicity of coumarin in experimental laboratory animals. Coumarins have hepatotoxic and carcinogenic properties in vivo in rodents and other mammalian species. These included adenomas and carcinomas of the liver, lungs and bile ducts and adenomas of the kidney and liver in rats and mice respectively. Carcinomas were found only at doses higher than 100 mg/kg body weight per day (Lake, 1999; EFSA, 2004). In humans, coumarins were approved for clinical use since the 1970s for treating various venous and lymphatic oedemas, as well as tumours including renal cell carcinoma (Marshall et al., 1994). However, their use was eventually discontinued and they were recalled from the market following the development of severe hepatotoxicity in patients after treatment (Cox et al., 1989; WHO, 1995). Coumarins are rapidly absorbed from the gastrointestinal tract after oral administration and extensively metabolized by the liver in the first pass, with only 2%–6% reaching the systemic circulation intact (Guo et al., 2020). Coumarins such as furanocoumarins are toxic to humans. Methoxypsoralen derivatives are potent photosensitizers. They can be activated by near-UV light becoming mutagenic, phototoxic and carcinogenic (Ojala et al., 1999; Ceska et al., 1997). Osthole had an acute intraperitoneal LD50 in mice of 710 mg/kg bw manifesting toxicity as hyperventilation, tremors, and photophobia. In subchronic studies, orally administered osthole in Wistar rats at 5–50 mg/kg bw for 45 days, caused pulmonary haemorrhage and mild reno and hepato-inflammation (Shokoohinia et al., 2017). Esculetin showed a low acute toxicity with an oral LD50 of over 2000 mg/kg bw, the intraperitoneal LD50 being 1,450 mg/kg bw (Tubaro et al., 1988). Orally administered psoralen in rats (80 mg/kg bw) caused cholestatic liver injuries (Wang et al., 2019).
Several African medicinal plants are sources of various coumarins which possess several biological activities. Coumarins generally have several therapeutic applications. Most of the coumarins in this review (46.7%) had some antimicrobial activity. Six coumarins: osthole, imperatorin/umbelliferon, pseudocordatolide C & calanolide, chartreusin and esculetin had either antitumor or anticancer activity. They had varying levels and types of toxicity ranging from inhibiting blood clotting as anticoagulants, cytotoxic effects, causing hyperventilation, tremor, and photophobia. Such plants need to be conserved because of their potential as sources of coumarins and other drugs. This calls for more research into their safety and efficacy because of their wide spread applications as therapeutic agents.
AG conceptualised the study. EM collected data and drafted the first manuscript. AG conducted data analysis and drafted the final manuscript. All authors contributed to the article and approved the submitted version..
The authors declare that the research was conducted in the absence of any commercial or financial relationships that could be construed as a potential conflict of interest.
All claims expressed in this article are solely those of the authors and do not necessarily represent those of their affiliated organizations, or those of the publisher, the editors and the reviewers. Any product that may be evaluated in this article, or claim that may be made by its manufacturer, is not guaranteed or endorsed by the publisher.
Abdizadeh, R., Hadizadeh, F., and Abdizadeh, T. (2020). QSAR analysis of coumarin-based benzamides as histone deacetylase inhibitors using CoMFA, CoMSIA and HQSAR methods. J. Mol. Struct. 1199, 126961. doi:10.1016/j.molstruc.2019.126961
Ahmadi, F. S. V., Sajjadi, S. E., Shokoohinia, Y., Azizian, H., and Taheripak, G. (2016). Grandivittin as a natural minor groove binder extracted from ferulago macrocarpa to ct-DNA, experimental and in silico analysis. Chemico-Biological Interact. 258, 89–101. doi:10.1016/j.cbi.2016.08.020
Anand, P., Singh, B., and Singh, N. (2012). A review on coumarins as acetylcholinesterase inhibitors for Alzheimer’s disease. Bioorg. Med. Chem. 20 (3), 1175–1180. doi:10.1016/j.bmc.2011.12.042
Annunziata, F., Pinna, C., Dallavalle, S., Tamborini, L., and Pinto, A. (2020). An overview of coumarin as a versatile and readily accessible scaffold with broad-ranging biological activities. Int. J. Mol. Sci. 21 (13), 4618. doi:10.3390/ijms21134618
Basile, A., Sorbo, S., Spadaro, V., Bruno, M., Maggio, A., Faraone, N., et al. (2009). Antimicrobial and antioxidant activities of coumarins from the roots of Ferulago campestris (Apiaceae). Mol. (Basel, Switz. 14 (3), 939–952. doi:10.3390/molecules14030939
Boyer, P. L., Currens, M., McMahon, J., Boyd, M., and Hughes, S. (1993). Analysis of nonnucleoside drug-resistant variants of human immunodeficiency virus type 1 reverse transcriptase. J. Virology 67 (4), 2412–2420. doi:10.1128/JVI.67.4.2412-2420.1993
Buckheit, R. W., White, E. L., Fliakas-Boltz, V., Russell, J., Stup, T. L., Kinjerski, T. L., et al. (1999). Unique anti-human immunodeficiency virus activities of the nonnucleoside reverse transcriptase inhibitors calanolide A, costatolide, and dihydrocostatolide. Antimicrob. agents Chemother. 43 (8), 1827–1834. doi:10.1128/AAC.43.8.1827
Chakthong, S., Weaaryee, P., Puangphet, P., Mahabusarakam, W., Plodpai, P., Voravuthikunchai, S. P., et al. (2012). Alkaloid and coumarins from the green fruits of Aegle marmelos. Phytochemistry, 75, 108–113. doi:10.1016/j.phytochem.2011.11.018
Chiang, C. C., Cheng, M. J., Peng, C. F., Huang, H. Y., and Chen, I. S. (2010). A novel dimeric coumarin analog and antimycobacterial constituents from Fatoua pilosa. Chem. Biodivers. 7 (7), 1728–1736. doi:10.1002/cbdv.200900326
Cox, D., O'kennedy, R., and Thornes, R. D. (1989). The rarity of liver toxicity in patients treated with coumarin (1, 2-benzopyrone). Hum. Toxicol. 8 (6), 501–506. doi:10.1177/096032718900800612
Crichton, E. G., and Waterman, P. G. (1978). Dihydromammea c/ob: a New Coumarin from the seed of Mammea africana. Phytochemistry 17 (10), 1783–1786. doi:10.1016/s0031-9422(00)88695-1
Dabur, R., Sharma, B., and Mittal, A. (2018). Mechanistic approach of anti-diabetic compounds identified from natural sources. Chem. Biol. Lett. 5 (2), 63–99.
Doctor, N., Parker, G., Vang, K., Smith, M., Kayan, B., and Yang, Y. (2020). Stability and extraction of vanillin and coumarin under subcritical water conditions. Molecules 25 (5), 1061. doi:10.3390/molecules25051061
Eason, C., and Spurr, E. (1995). Review of the toxicity and impacts of brodifacoum on non-target wildlife in New Zealand. N. Z. J. zoology 22 (4), 371–379. doi:10.1080/03014223.1995.9518055
EFSA (2004). Opinion of the scientific panel on food additives, flavourings, processing aids and materials in contacts with food (AFC) on a request from the commission related to. EFSA J. 104, 1–36. Coumarin; adopted on 6 October 2004.
Egan, D., O’Kennedy, R., Moran, E., Cox, D., Prosser, E., and Thornes, R. D. (1990). The pharmacology, metabolism, analysis, and applications of coumarin and coumarin-related compounds. Drug Metab. Rev. 22, 503–529. doi:10.3109/03602539008991449
Farinola, N., and Piller, N. (2005). Pharmacogenomics: its role in re-establishing coumarin as treatment for lymphedema. Lymphatic Res. Biol. 3 (2), 81–86. doi:10.1089/lrb.2005.3.81
Givel, M. (2003). A comparison of US and Norwegian regulation of coumarin in tobacco products. Tob. Control 12 (4), 401–405. doi:10.1136/tc.12.4.401
Guo, P.-J., Lin, Z.-J., Zhang, X.-M., Zou, L.-N., Guo, F.-F., and Zhang, B. (2020). Toxicological research and safety consideration of coumarins. Zhongguo Zhong Yao Za Zhi. 45 (3), 518–522. doi:10.19540/j.cnki.cjcmm.20190827.401
Hassan, M. Z., Osman, H., Ali, M. A., and Ahsan, M. J. (2016). Therapeutic potential of coumarins as antiviral agents. Eur. J. Med. Chem. 123, 236–255. doi:10.1016/j.ejmech.2016.07.056
Hazleton, L., Tusing, T., Zeitlin, B., Thiessen, R., and Murer, H. (1956). Toxicity of coumarin. J. Pharmacol. Exp. Ther. 118 (3), 348–358.
Hirsch, A.-M., Longeon, A., and Guyot, M. (2002). Fraxin and esculin: two coumarins specific to Actinidia chinensis and A. deliciosa (kiwifruit). Biochem. Syst. Ecol. 30 (1), 55–60. doi:10.1016/s0305-1978(01)00064-3
Hirsh, J., Dalen, J. E., Anderson, D. R., Poller, L., Bussey, H., Ansell, J., et al. (2001). Oral anticoagulants: mechanism of action, clinical effectiveness, and optimal therapeutic range. Chest 119 (1), 8S–21S. doi:10.1378/chest.119.1_suppl.8s
Hosseini, M., Hosseinzadeh, A., Raufian, K., and Hedjazi, A. (2015). Nontraumatic retroperitoneal hematoma after warfarin administration: fatal case report and review of the literature. Am. J. forensic Med. pathology 36 (4), 245–248. doi:10.1097/PAF.0000000000000190
Hsia, C. W., Lin, K. C., Lee, T. Y., Hsia, C. H., Chou, D. S., Jayakumar, T., et al. (2019). Esculetin, a coumarin derivative, prevents thrombosis: inhibitory signaling on PLCγ2-PKC-AKT activation in human platelets. Int. J. Mol. Sci. 20 (11), 2731. doi:10.3390/ijms20112731
Huang, X., Wang, J., Hao, H., Ouyang, J., Gao, Y., Bao, Y., et al. (2015). Determination and correlation of solubility and solution thermodynamics of coumarin in different pure solvents. Fluid Phase Equilibria 394, 148–155. doi:10.1016/j.fluid.2015.03.022
Jadhav, S. B., Fatema, S., Patel, M. A. I., and Farooqui, M. (2017). Novel pyrazoles: M. Tuberculosis growth inhibition and synergistic study. Chem. Biol. Interface 7 (2).
Jung, J.-C., and Oh, S. (2011). Practical synthesis of hydroxychromenes and evaluation of their biological activity. Molecules 17 (1), 240–247. doi:10.3390/molecules17010240
Karakaya, S., Şimşek, D., Özbek, H., Güvenalp, Z., Altanlar, N., Cavit, C., et al. (2019). Antimicrobial activities of extracts and isolated coumarins from the roots of four ferulago species growing in Turkey. Iran. J. Pharm. Res. IJPR 18 (3), 1516–1529. doi:10.22037/ijpr.2019.1100718
Khalil, N., Bishr, M., Desouky, S., and Salama, O. (2020). Ammi visnaga L., a potential medicinal plant: a review. Molecules 25 (2), 301. doi:10.3390/molecules25020301
Kicel, A., and Wolbis, M. (2012). Coumarins from the flowers of Trifolium repens. Chem. Nat. Compd. 48 (1), 130–132. doi:10.1007/s10600-012-0179-3
Kirsch, G., Abdelwahab, A. B., and Chaimbault, P. (2016). Natural and synthetic coumarins with effects on inflammation. Molecules 21 (10), 1322. doi:10.3390/molecules21101322
Kontogiorgis, C. A., and Hadjipavlou-Litina, D. J. (2005). Synthesis and antiinflammatory activity of coumarin derivatives. J. Med. Chem. 48 (20), 6400–6408. doi:10.1021/jm0580149
Kostova, I. (2005). Synthetic and natural coumarins as cytotoxic agents. Curr. Med. Chemistry-Anti-Cancer Agents 5 (1), 29–46. doi:10.2174/1568011053352550
Kostova, I., Bhatia, S., Grigorov, P., Balkansky, S., S Parmar, V., K Prasad, A., et al. (2011). Coumarins as antioxidants. Curr. Med. Chem. 18 (25), 3929–3951. doi:10.2174/092986711803414395
Koyiparambath, V. P., Prayaga Rajappan, K., Rangarajan, T., Al-Sehemi, A. G., Pannipara, M., Bhaskar, V., et al. (2021). Deciphering the detailed structure–activity relationship of coumarins as Monoamine oxidase enzyme inhibitors—an updated review. Chem. Biol. Drug Des. 98 (4), 655–673. doi:10.1111/cbdd.13919
Kubrak, T., Podgórski, R., and Stompor, M. (2017). Natural and synthetic coumarins and their pharmacological activity.
Lacy, A., and O'Kennedy, R. (2004). Studies on coumarins and coumarin-related compounds to determine their therapeutic role in the treatment of cancer. Curr. Pharm. Des. 10 (30), 3797–3811. doi:10.2174/1381612043382693
Lake, B. (1999). Coumarin metabolism, toxicity and carcinogenicity: relevance for human risk assessment. Food Chem. Toxicol. 37 (4), 423–453. doi:10.1016/s0278-6915(99)00010-1
Liu, C. W., Chien, M. W., Su, C. Y., Chen, H. Y., Li, L. J., and Lai, C. C. (2012). Analysis of flavonoids by graphene-based surface-assisted laser desorption/ionization time-of-flight mass spectrometry. Analyst 137 (24), 5809–5816.
Luszczki, J. J., Wojda, E., Andres-Mach, M., Cisowski, W., Glensk, M., Glowniak, K., et al. (2009). Anticonvulsant and acute neurotoxic effects of imperatorin, osthole and valproate in the maximal electroshock seizure and chimney tests in mice: a comparative study. Epilepsy Res. 85 (2-3), 293–299. doi:10.1016/j.eplepsyres.2009.03.027
Marealle, A. I., Moyo, A. A., Machumi, F., Qwarse, M., Chenyambuga, Y. M., Heydenreich, M., et al. (2023). Antimycobacterial activity of scopoletin from ethanolic extract of Hymenodictyon floribundum (Hochst. & Steud.) B.L.Rob. Stem bark. Sci. Afr., 21, e01778. doi:10.1016/j.sciaf.2023.e01778
Marshall, M. E., Kervin, K., Benefield, C., Umerani, A., Albainy-Jenei, S., Zhao, Q., et al. (1994). Growth-inhibitory effects of coumarin (1, 2-benzopyrone) and 7-hydroxycoumarin on human malignant cell lines in vitro. J. cancer Res. Clin. Oncol. 120, S3–S10. doi:10.1007/BF01377114
Matos, J. M., Vazquez-Rodriguez, S., Santana, L., Uriarte, E., Fuentes-Edfuf, C., Santos, Y., et al. (2012). Looking for new targets: simple coumarins as antibacterial agents. Med. Chem. 8 (6), 1140–1145. doi:10.2174/1573406411208061140
Matos, M. J., Santana, L., Uriarte, E., Abreu, O. A., Molina, E., and Yordi, E. G. (2015). “Coumarins—an important class of phytochemicals,” in Phytochemicals - isolation, characterisation and role in human health. Editors A. Venket Rao, and L. G. Rao (InTech).
McKee, T. C., Fuller, R. W., Covington, C. D., Cardellina, J. H., Gulakowski, R. J., Krepps, B. L., et al. (1996). New pyranocoumarins isolated from Calophyllum lanigerum and Calophyllum teysmannii. J. Nat. Prod. 59 (8), 754–758. doi:10.1021/np9603784
Menezes, J. C., and Diederich, M. (2019). Translational role of natural coumarins and their derivatives as anticancer agents. Future Med. Chem. 11 (9), 1057–1082. doi:10.4155/fmc-2018-0375
Mitra, I., Saha, A., and Roy, K. (2013). Quantification of contributions of different molecular fragments for antioxidant activity of coumarin derivatives based on QSAR analyses. Can. J. Chem. 91 (6), 428–441. doi:10.1139/cjc-2012-0527
Mustafa, Y., Mohammed, E., and Khalil, R. (2021). Synthesis, characterization, and anticoagulant activity of new functionalized biscoumarins. Egypt. J. Chem. 64 (8), 4461–4468.
Nahar, L., Talukdar, A. D., Nath, D., Nath, S., Mehan, A., Ismail, F. M., et al. (2020). Naturally occurring calanolides: occurrence, biosynthesis, and pharmacological properties including therapeutic potential. Molecules 25 (21), 4983. doi:10.3390/molecules25214983
Najmanova, I., Dosedel, M., Hrdina, R., Anzenbacher, P., Filipsky, T., Riha, M., et al. (2015). Cardiovascular effects of coumarins besides their antioxidant activity. Curr. Med. Chem. 15 (9), 830–849. doi:10.2174/1568026615666150220112437
Nguelefack-Mbuyo, P., Nguelefack, T., Dongmo, A., Afkir, S., Azebaze, A., Dimo, T., et al. (2008). Anti-hypertensive effects of the methanol/methylene chloride stem bark extract of Mammea africana in L-NAME-induced hypertensive rats. J. Ethnopharmacol. 117 (3), 446–450. doi:10.1016/j.jep.2008.02.028
Ojala, T., Vuorela, P., Kiviranta, J., Vuorela, H., and Hiltunen, R. (1999). A bioassay using Artemia salina for detecting phototoxicity of plant coumarins. Planta Medica 65 (08), 715–718. doi:10.1055/s-1999-14049
Ojewole, J. A., and Adewunmi, C. O. (2004). Anti-inflammatory and hypoglycaemic effects of Tetrapleura tetraptera (Taub)[fabaceae] fruit aqueous extract in rats. J. Ethnopharmacol. 95 (2-3), 177–182. doi:10.1016/j.jep.2004.06.026
Oldenburg, J., Watzka, M., Rost, S., and Müller, C. R. (2007). VKORC1: molecular target of coumarins. J. Thrombosis Haemostasis 5, 1–6. doi:10.1111/j.1538-7836.2007.02549.x
Panno, M., Giordano, F., Palma, M., Bartella, V., Rago, V., Maggiolini, M., et al. (2009). Evidence that bergapten, independently of its photoactivation, enhances p53 gene expression and induces apoptosis in human breast cancer cells. Curr. cancer drug targets 9 (4), 469–481. doi:10.2174/156800909788486786
Perone, V. B. (2016). in The natural occurrence and uses of toxic coumarins. Microbial Toxins. Editors S. Kadis, A. Ciegler, and S. J. Ajl, 67–88.
Poumale, H. M. P., Hamm, R., Zang, Y., Shiono, Y., and Kuete, V. (2013). “Coumarins and related compounds from the medicinal plants of Africa,” in Medicinal plant research in Africa (Elsevier), 261–300.
Prusty, J. S., and Kumar, A. (2020). Coumarins: antifungal effectiveness and future therapeutic scope. Mol. Divers. 24 (4), 1367–1383. doi:10.1007/s11030-019-09992-x
Qu, L., Li, J., Wang, R., Wang, X., Zhao, T., Chen, Y., et al. (2021). Metabolic and transcriptional profiling of Fraxinus chinensis var. rhynchophylla unravels possible constitutive resistance against Agrilus planipennis. Forests 12 (10), 1373. doi:10.3390/f12101373
Raja, S. B., Murali, M. R., Roopa, K., and Devaraj, S. N. (2011). Imperatorin a furocoumarin inhibits periplasmic Cu-Zn SOD of Shigella dysenteriae their by modulates its resistance towards phagocytosis during host pathogen interaction. Biomed. Pharmacother. 65 (8), 560–568. doi:10.1016/j.biopha.2010.10.010
Reddy, D. S., Kongot, M., and Kumar, A. (2021). Coumarin hybrid derivatives as promising leads to treat tuberculosis: recent developments and critical aspects of structural design to exhibit anti-tubercular activity. Tuberculosis 127, 102050. doi:10.1016/j.tube.2020.102050
Riveiro, M. E., De Kimpe, N., Moglioni, A., Vazquez, R., Monczor, F., Shayo, C., et al. (2010). Coumarins: old compounds with novel promising therapeutic perspectives. Curr. Med. Chem. 17 (13), 1325–1338. doi:10.2174/092986710790936284
Sai, Y., Yang, T. J., Krausz, K. W., Gonzalez, F. J., and Gelboin, H. V. (1999). An inhibitory monoclonal antibody to human cytochrome P450 2A6 defines its role in the metabolism of coumarin, 7-ethoxycoumarin and 4-nitroanisole in human liver. Pharmacogenetics Genomics 9 (2), 229–237.
Sancho, R. o., Márquez, N., Gómez-Gonzalo, M., Calzado, M. A., Bettoni, G., Coiras, M. T., et al. (2004). Imperatorin inhibits HIV-1 replication through an Sp1-dependent pathway. J. Biol. Chem. 279 (36), 37349–37359. doi:10.1074/jbc.M401993200
Santoro, M., Guido, C., De Amicis, F., Sisci, D., Cione, E., Dolce, V., et al. (2016). Bergapten induces metabolic reprogramming in breast cancer cells. Oncol. Rep. 35 (1), 568–576. doi:10.3892/or.2015.4327
Sarker, S. D., and Nahar, L. (2017). Progress in the chemistry of naturally occurring coumarins. Prog. Chem. Nat. Occur. coumarins 106, 241–304. doi:10.1007/978-3-319-59542-9_3
Shokoohinia, Y., Bazargan, S., Miraghaee, S., Javadirad, E., Farahani, F., and Hosseinzadeh, L. (2017). Safety assessment of osthole isolated from Prangos ferulacea: acute and subchronic toxicities and modulation of cytochrome P450. Jundishapur J. Nat. Pharm. Prod. 12 (3). doi:10.5812/jjnpp.63764
Skalicka-Woźniak, K., Orhan, I. E., Cordell, G. A., Nabavi, S. M., and Budzyńska, B. (2016). Implication of coumarins towards central nervous system disorders. Pharmacol. Res. 103, 188–203. doi:10.1016/j.phrs.2015.11.023
Stefanachi, A., Leonetti, F., Pisani, L., Catto, M., and Carotti, A. (2018). Coumarin: a natural, privileged and versatile scaffold for bioactive compounds. Molecules 23 (2), 250. doi:10.3390/molecules23020250
Sun, C., Zhao, W., Wang, X., Sun, Y., and Chen, X. (2020). A pharmacological review of dicoumarol: an old natural anticoagulant agent. Pharmacol. Res. 160, 105193. doi:10.1016/j.phrs.2020.105193
Teng, C.-M., Lin, C.-H., Ko, F.-N., Wu, T.-S., and Huang, T.-F. (1994). The relaxant action of osthole isolated from Angelica pubescens in Guinea-pig trachea. Naunyn-Schmiedeberg's archives Pharmacol. 349, 202–208. doi:10.1007/BF00169838
Tubaro, A., Del Negro, P., Ragazzi, E., Zampiron, S., and Della Loggia, R. (1988). Anti-inflammatory and peripheral analgesic activity of esculetin in vivo. Pharmacol. Res. Commun. 20, 83–85. doi:10.1016/S0031-6989(88)80847-6
Ulrich, M., Lüer, P., and Kreusch, D. (1999). “1.24 - biosynthesis of coumarins.” In: Comprehensive natural products chemistry meth-cohn,Eds: edited by B. Sir Derek, N. Koji, and B. T. Otto - 623–637. Oxford, Pergamon: Elsevier Science. doi:10.1016/B978-0-08-091283-7.00026-6
Vaou, N., Stavropoulou, E., Voidarou, C., Tsigalou, C., and Bezirtzoglou, E. (2021). Towards advances in medicinal plant antimicrobial activity: A review study on challenges and future perspectives. Microorganisms 9 (10), 2041. doi:10.3390/microorganisms9102041
Venugopala, K. N., Rashmi, V., and Odhav, B. (2013). Review on natural coumarin lead compounds for their pharmacological activity. BioMed Res. Int. 2013, 963248. doi:10.1155/2013/963248
Wagstaff, D. J. (1991). Dietary exposure to furocoumarins. Regul. Toxicol. Pharmacol. 14 (3), 261–272. doi:10.1016/0273-2300(91)90029-u
Wang, C., Pei, A., Chen, J., Yu, H., Sun, M.-L., Liu, C.-F., et al. (2012). A natural coumarin derivative esculetin offers neuroprotection on cerebral ischemia/reperfusion injury in mice. J. Neurochem. 121 (6), 1007–1013. doi:10.1111/j.1471-4159.2012.07744.x
Wang, Y., Zhang, H., Jiang, J. M., Zheng, D., Chen, Y. Y., Wan, S. J., et al. (2019). Hepatotoxicity induced by psoralen and isopsoralen from fructus psoraleae: wistar rats are more vulnerable than ICR mice. Food Chem. Toxicol. 125, 133–140. doi:10.1016/J.FCT.2018.12.047
Wang, Y.-H., Avula, B., Nanayakkara, N. D., Zhao, J., and Khan, I. A. (2013). Cassia cinnamon as a source of coumarin in cinnamon-flavored food and food supplements in the United States. J. Agric. food Chem. 61 (18), 4470–4476. doi:10.1021/jf4005862
Wishart, D. S., Feunang, Y. D., Guo, A. C., Lo, E. J., Marcu, A., Grant, J. R., et al. (2018). DrugBank 5.0: a major update to the DrugBank database for 2018. Nucleic acids Res. 46 (D1), D1074–D1082. doi:10.1093/nar/gkx1037
Wu, L., Wang, X., Xu, W., Farzaneh, F., and Xu, R. (2009). The structure and pharmacological functions of coumarins and their derivatives. Curr. Med. Chem. 16 (32), 4236–4260. doi:10.2174/092986709789578187
Wu, T. S., Hsu, M. Y., Kuo, P. C., Sreenivasulu, B., Damu, A. G., Su, C. R., et al. (2003). Constituents from the leaves of Phellodendron amurense var. wilsonii and their bioactivity. J. Nat. Prod. 66 (9), 1207–1211. doi:10.1021/np030034v
Yang, D., Gu, T., Wang, T., Tang, Q., and Ma, C. (2010). Effects of osthole on migration and invasion in breast cancer cells. Biosci. Biotechnol. Biochem. 74 (7), 1430–1434. doi:10.1271/bbb.100110
Yarnell, E., and Abascal, K. (2009). Plant coumarins: myths and realities. Altern. Complementary Ther. 15 (1), 24–30. doi:10.1089/act.2009.15104
Zhang, J., Di, H., Luo, K., Jahufer, Z., Wu, F., Duan, Z., et al. (2018). Coumarin content, morphological variation, and molecular phylogenetics of Melilotus. Mol. 23 (4), 810. doi:10.3390/molecules23040810
Zhang, J., Tan, Y., Li, G., Chen, L., Nie, M., Wang, Z., et al. (2021). Coumarin sulfonamides and amides derivatives: design, synthesis, and antitumor activity in vitro. Molecules 26 (4), 786. doi:10.3390/molecules26040786
Zhang, Z.-R., Leung, W. N., Cheung, H. Y., and Chan, C. W. (2015). Osthole: a review on its bioactivities, pharmacological properties, and potential as alternative medicine. Evidence-Based Complementary Altern. Med. 2015, 919616. doi:10.1155/2015/919616
Zhao, H., Chen, X., Chen, X., Zhu, Y., Kong, Y., Zhang, S., et al. (2020). New peptidendrocins and anticancer chartreusin from an endophytic bacterium of Dendrobium officinale. Ann. Transl. Med. 8 (7), 455. doi:10.21037/atm.2020.03.227
Keywords: coumarins, bioavailability, safety, therapeutic, medicinal plants, drug discovery
Citation: Anywar G and Muhumuza E (2024) Bioactivity and toxicity of coumarins from African medicinal plants. Front. Pharmacol. 14:1231006. doi: 10.3389/fphar.2023.1231006
Received: 29 May 2023; Accepted: 18 December 2023;
Published: 10 January 2024.
Edited by:
Habibu Tijjani, Bauchi State University, NigeriaReviewed by:
Pinarosa Avato, University of Bari Aldo Moro, ItalyCopyright © 2024 Anywar and Muhumuza. This is an open-access article distributed under the terms of the Creative Commons Attribution License (CC BY). The use, distribution or reproduction in other forums is permitted, provided the original author(s) and the copyright owner(s) are credited and that the original publication in this journal is cited, in accordance with accepted academic practice. No use, distribution or reproduction is permitted which does not comply with these terms.
*Correspondence: Godwin Anywar, Z29kd2luYW55d2FyQGdtYWlsLmNvbQ==
Disclaimer: All claims expressed in this article are solely those of the authors and do not necessarily represent those of their affiliated organizations, or those of the publisher, the editors and the reviewers. Any product that may be evaluated in this article or claim that may be made by its manufacturer is not guaranteed or endorsed by the publisher.
Research integrity at Frontiers
Learn more about the work of our research integrity team to safeguard the quality of each article we publish.