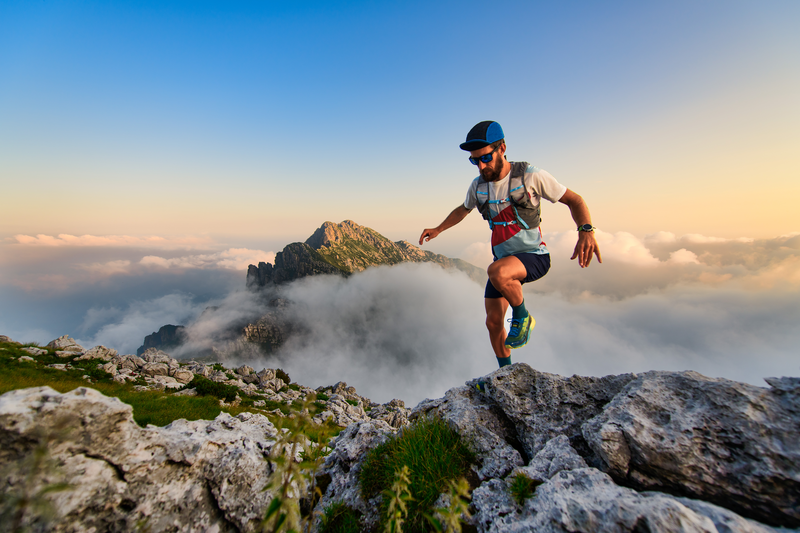
94% of researchers rate our articles as excellent or good
Learn more about the work of our research integrity team to safeguard the quality of each article we publish.
Find out more
REVIEW article
Front. Pharmacol. , 07 August 2023
Sec. Pharmacology of Anti-Cancer Drugs
Volume 14 - 2023 | https://doi.org/10.3389/fphar.2023.1225821
This article is part of the Research Topic The Role of Tumor Microenvironment in the Development, Treatment and Prognosis of Hepatocellular Carcinoma View all 30 articles
Hepatocellular carcinoma (HCC) is the most common primary liver cancer and the fourth leading cause of cancer-related death worldwide. HCC often occurs in the setting of chronic liver disease or cirrhosis. Recent evidence has highlighted the importance of the immune microenvironment in the development and progression of HCC, as well as its role in the potential response to therapy. Liver disease such as viral hepatitis, alcohol induced liver disease, and non-alcoholic fatty liver disease is a major risk factor for the development of HCC and has been demonstrated to alter the immune microenvironment. Alterations in the immune microenvironment may markedly influence the response to different therapeutic strategies. As such, research has focused on understanding the complex relationship among tumor cells, immune cells, and the surrounding liver parenchyma to treat HCC more effectively. We herein review the immune microenvironment, as well as the relative effect of liver disease on the immune microenvironment. In addition, we review how changes in the immune microenvironment can lead to therapeutic resistance, as well as highlight future strategies aimed at developing the next-generation of therapies for HCC.
Hepatocellular carcinoma (HCC) is the most common primary liver cancer and the fourth leading cause of cancer-related death worldwide (Forner et al., 2018). Chronic liver disease due to various etiologies such as viral hepatitis, alcohol induced liver disease (ALD), non-alcoholic fatty liver disease (NAFLD), and non-alcoholic steatohepatitis (NASH) is a major risk factor for the development of HCC (Llovet et al., 2016). The severity of the underlying liver disease is often a major factor in determining treatment strategy as it is often a driving factor related to therapeutic morbidity. To this point, patients with advanced tumors or severe underlying liver disease are often not candidates for curative treatment options and these patients are treated with locoregional or systemic therapies (Llovet et al., 2002; Maluccio et al., 2005; Maluccio et al., 2008; Raoul et al., 2019). Recently, there has been increased interest in the use of immune checkpoint inhibitors (ICIs) to treat patient with advanced HCC. To date, response rates and survival related to ICI treatment remain varied and often not durable. As such, there has been increased efforts to understand mechanisms of resistance to ICI therapy.
Recent evidence has highlighted the importance of the liver immune microenvironment in the development and progression of HCC, as well as the potential response to therapy. Research has focused on understanding the complex interactions among tumor cells, immune cells, and the liver tissue. Moreover, there is an emerging understanding as to how the immune microenvironment may change relative to different liver disease etiologies. In addition, there are ongoing efforts to investigate the effect of liver disease on the immune microenvironment, as well as to characterize the impact of liver disease on response to therapy. We herein review the liver immune microenvironment, as well as the impact of liver disease on the immune microenvironment. In addition, we review how changes in the immune microenvironment can lead to therapeutic resistance, as well as highlight future strategies aimed at developing the next-generation of therapies for HCC.
The liver is naturally exposed to a large influx of antigens from the gastrointestinal tract. As such, the liver is uniquely immune tolerant having developed intrinsic tolerogenic mechanisms in the innate and adaptive immune responses (Figure 1). Thus, the liver protects itself from autoimmune damage secondary to large antigen presentation from the gastrointestinal tract (Jenne and Kubes, 2013; Brown et al., 2019). However, the liver also provides a unique proinflammatory microenvironment composed of Kupffer cells, antigen-presenting cells (APCs), T cells, and hepatic stellate cells (HSCs) (Stauffer et al., 2012; Agosti et al., 2018; Koo et al., 2020). During liver injury and disease states, a wide range of liver cells participate in a complex proinflammatory response that can result in hepatocyte death and disease progression (Figure 2). (Koo et al., 2020)
FIGURE 1. Mechanisms involved in hepatocellular carcinoma immune evasion. In physiological conditions, liver has the ability to induce immunotolerance against antigen from gastrointestinal tract. These mechanisms have a detrimental role during hepatocellular carcinoma development and progression. Upregulation of inhibitory programmed death-ligand 1 molecule from tumor cells, Kupffer cells, liver sinusoidal endothelial cells and antigen presenting cells, together with the release of interleukin-10 and transforming growth factor beta, lead to an exhausted phenotype of CD8+ cells and prevent tumor cells from immune damage. HCC: Hepatocellular carcinoma; PD-L1: Programmed death-ligand 1; CTL4A: Cytotoxic T lymphocyte antigen 4; PD-1: Programmed cell death protein 1; TGFβ: Transforming growth factor beta; IL-10: Interleukin-10. From: Polidoro et al. Tumor microenvironment in primary liver tumors: A challenging role of natural killer cells. World J Gastroenterol 2020. PMID 32952338.
FIGURE 2. Liver disease from various etiologies such as non-alcoholic fatty liver disease/non-alcoholic steatohepatitis, alcohol induced liver disease, viral hepatitis, and cirrhosis/fibrosis result in alterations in the tumor immune microenvironment subsequently leading to development and progression of hepatocellular carcinoma.
In the liver, the innate immune system consists of multiple cell types that act as the first line of defense against pathogens. Kupffer cells (KC) are resident macrophages within the liver, which are in constant contact with antigens arriving to the liver from the gastrointestinal tract (Racanelli and Rehermann, 2006; Nakamoto and Kanai, 2014; Tacke, 2017). In turn, the KC serve as the first line of immune defense. Additionally, a large population of peripheral monocytes are often recruited to the liver. Kupffer cells can be distinguished from monocyte derived macrophages as KCs have low levels of CD11b and CCR2, and high F4/80 expression (Holt et al., 2008; Obstfeld et al., 2010; Stienstra et al., 2010). Furthermore, Bleriot et al. identified two distict populations of KCs which shared a core molecular signature while expressing different genes and proteins (Blériot et al., 2021). Similarly, macrophages exists in multiple subtypes such as the M1 phenotype with antitumor inflammatory reactions and the M2 phenotype characterized by tumor promoting capabilities with immune suppression (Liu et al., 2021).
Natural killer (NK) cells are another subset of the innate immune system that have cytolytic activity against stressed cells, virally infected cells, and malignant cells (Abul et al., 2007; Kahraman et al., 2010). Unlike CD8+ T-cells, which require costimulation for cytotoxic activity, NK cells have the unique ability to kill targeted cells without a need for secondary activation. Neutrophils, the most abundant population of circulating white blood cells, activate early phases of the inflammatory response in the innate immune system (Abul et al., 2007). Dendritic cells (DCs), a type of APC, are innate immune cells that present antigens to T-cells thus initiating the adaptive immune response (Abul et al., 2007).
Recent evidence has highlighted the changes in the adaptive immune system in the immune microenvironment secondary to liver disease. T-cells are abundant in healthy livers and exist in several subsets: CD4+ helper T (Th) cells, CD8+ cytotoxic T cells, and regulatory T-cells (Tregs) (Ramadori et al., 2022). CD4+ T cells are essential for tumor control to prevent tumor initiation and facilitate clearance of premalignant and malignant cells (Rakhra et al., 2010; Kang et al., 2011; Heinrich et al., 2021). CD4+ T cells are often initiators of an anti-tumor response and are associated with a favorable response to immunotherapy. CD8+ cytotoxic T-cells are the main effector cells of the cellular immune system and eliminate infected or malignant cells through recognition of presented antigens (Van Herck et al., 2019). Additionally, there is a population of CD8+ tissue-resident memory (TRM) cells that reside in the liver and act as local immune sentinels (MacParland et al., 2018; Hirsova et al., 2021).
While CD4+ and CD8+ T cells promote an anti-tumor inflammatory response, Tregs are an immunosuppressive subset of CD4+ T-cells and are essential to maintain homeostasis and immune tolerance (Togashi et al., 2019; Wang et al., 2021). The accumulation of Tregs has been recognized as promoting immune evasion and hepatocarcinogenesis (Togashi et al., 2019; Wang et al., 2021). Natural killer T cells (NKT) are considered a bridge between innate and adaptive immunity via expression of NK cell surface markers as well as antigen receptor characteristics of T-cells (Abul et al., 2007; Arrese et al., 2016). NKT cells are located in the sinusoids of the liver to provide intravascular immune surveillance (Geissmann et al., 2005; Abul et al., 2007). NKT cells are both proinflammatory mediated through the type I NKT cell subtype, as well as immune suppressive protecting against liver injury via Type II NKT cells (Kumar, 2013).
Non-alcoholic fatty liver disease (NAFLD) and its severe form non-alcoholic steatohepatitis (NASH) are characterized by the accumulation of triglycerides within hepatocytes with approximately 10%–20% of patients progressing to cirrhosis (Cusi, 2012). NAFLD and NASH are a manifestation of metabolic syndrome, which is generally characterized as a constellation of type 2 diabetes mellitus, dyslipidemia, obesity, and cardiovascular disease (Cusi, 2012). In the United States, the prevalence of NASH is increasing and is becoming a significant risk factor for the development of HCC (Sheka et al., 2020).
NAFLD/NASH have multiple effects on the immune microenvironment. In the innate immune system, CCR2 macrophages are increased in the liver correlating with levels of CCL2 found in steatotic hepatocytes (Obstfeld et al., 2010; Stienstra et al., 2010). In preclinical studies, drugs targeting the CCL2/CCR2 axis impaired macrophage recruitment to the liver and reduced hepatosteatosis, inflammation, and fibrosis (Baeck et al., 2012; Lefebvre et al., 2016). In addition, there is often a large influx of neutrophils among patients with NASH (Feng et al., 2011). Myeloperoxidase (MPO) is used by neutrophils to create reactive oxygen species (ROS) in order to kill microbes. In patients with NASH, MPO is often increased suggesting that accumulation of MPO and ROS contribute to the development of NASH (Rensen et al., 2009). Neutrophils also exacerbate liver inflammation through the recruitment of macrophages and APCs (Arrese et al., 2016). Additionally, neutrophils release neutrophil extracellular traps (NETs), which are long chromatin fibers embedded with inflammatory proteins and neutrophil proteases (van der Windt et al., 2018; Wang et al., 2021). Preclinical studies suggest NET formation in the early stages NAFLD and increases with the progress to NASH (Wang et al., 2021).
Relative to the adaptive immune response, liver biopsies among patients with NASH have demonstrated increased infiltrating clusters of B cells and T cells that correlate with increased levels of oxidative stress-derived epitopes released from damaged hepatocytes (Garnelo et al., 2017; Sutti and Albano, 2020). Additionally, preclinical studies indicate ROS-dependent cell death of hepatic CD4+ T cells can occur leading to impaired anti-tumor surveillance (Ma et al., 2016; Brown et al., 2018). Furthermore, in early stages of NASH, there is an obesity-induced hepatic type I interferon (INF-1) response that has been associated with increased pathogenic CD8+ T-cell production of proinflammatory cytokines, which contributes to hepatocyte damage (Ghazarian et al., 2017). Of note, several investigators have reported improvement of NASH and restored hepatic insulin sensitivity and reduced fibrosis using experimental models with deletion of CD8+ T cells (Ghazarian et al., 2017; Van Herck et al., 2019). NKT cells also contribute to both the development and progression of NASH (Syn et al., 2010). Patients with NASH cirrhosis have four times as many NKT cells than individuals with healthy livers (Syn et al., 2012). Wolf et al. reported cross-talk between CD8 T-cells, NKT cells, and hepatocytes in the setting of NASH development and transition to HCC (Wolf et al., 2014). These investigators reported that experimental reduction of NKT cells, despite elevated CD8+ T cells, prevented liver damage. In turn, the data suggested that CD8 T-cells alone are not sufficient to cause liver damage in the absence of NKT cells (Wolf et al., 2014).
In addition to changes in pro-inflammatory cells within the immune microenvironment, alterations in immunosuppressive Tregs have been noted. In one experimental mouse model, Tregs were noted to be increased in NASH-livers with a lower concentration of CD4+ T cells (Wang et al., 2021). When Tregs were depleted, HCC initiation and progression of NASH was drastically inhibited (Wang et al., 2021). Furthermore, an imbalance between helper T cells and Tregs can promote progression of NAFLD along with higher expression of inflammatory cytokines (He et al., 2017; Zhang CY. et al., 2022).
Viral hepatitis is the leading cause of HCC worldwide (D'Souza et al., 2020). Similar to NAFLD/NASH, chronic liver disease caused by viral hepatitis has an effect on the immune microenvironment. A study by DeBattista et al. demonstrated that hepatitis B viral (HBV) and hepatitis C virus (HCV) have distinct molecular signatures and immune landscapes within the liver (De Battista et al., 2021). For example, among patients with HBV-HCC, there was a lower proportion of differentially expressed genes related to the immune response, yet a higher number of upregulated genes versus patients with HCV-HCC. In addition, HCV-HCC was characterized by downregulation of immune genes within the tumor especially related to T-cells, as well as upregulation of oxidative stress genes (De Battista et al., 2021). In contrast, the molecular signature of HBV-HCC was characterized by the upregulation of genes related to cell cycle control and monocyte/macrophage activation (De Battista et al., 2021).
Non-viral related HCC and HBV-HCC immune microenvironment appear to be composed of distinct immune subsets. Lim et al. utilized cytometry by time of flight (CyTOF) to perform in-depth immunoprofiling and reported that the HBV-HCC immune microenvironment was more immunosuppressive and exhausted with increased Tregs and CD8+ resident memory T cells (Lim et al., 2019). Increased Treg were associated with a poor prognosis, while CD8+ resident memory T cells were associated with a favorable prognosis (Lim et al., 2019). In a separate study, Li et al. reported that patients with higher levels of Tregs in the peripheral blood and/or tumor sites had a worse prognosis (Li et al., 2016). In pre-clinical mouse models, depleting Tregs was potentially therapeutic for HBV-related liver diseases through induction of antiviral and antitumor immunity. In turn, the data suggested that Tregs play a role in the development of cirrhosis, the transformation of cirrhosis to HCC, and the progression and metastasis of HCC (Li et al., 2016). In yet another study, Zhang et al. demonstrated that HBV-HCC, HCV-HCC and non-viral HCC had similar molecular phenotypes with inhibition of immune pathways. In the immune microenvironment associated with virus induced HCC there was, however, excessive M2-type macrophage polarization associated with immune suppression (Zhang YZ. et al., 2022). Similarly, Ding et al. performed a meta-analysis of 1,520 patients and noted that infiltration of immune cells in the tumor microenvironment for viral associated HCC versus non-viral associated HCC differed relative to M0 macrophages, M2 macrophages, Tregs, naive B cells, follicular helper T cells, activated dendritic cells, activated mast cells, and plasma cells (Ding et al., 2021).
The influence of viral hepatitis on the development and progression of HCC is complex and may initially be benefitial recruiting immune cells to protect against HCC development (Zamor et al., 2017). Among patients with HCV, medications such as direct acting antivirals (DAAs) are used to eradicate the virus from infected individuals. Recent investigators have focused on the effect that DAAs may have on HCC tumorigenesis after eradication of viral hepatitis. Reports have described early occurance and recurrence of HCC in patients who where successfully treated with DAAs (Conti et al., 2016; Reig et al., 2016). With DAA therapies for HCV infection, it is common to see a sustained virological response (SVR). However, reactivation of HBV in patients with co-infection and development of HCC among patients who achieved SVR has been observed (Borgia et al., 2021). It has been hypothesized that changes occur in intrahepatic immune surveillance following a SVR. Amaddeo et al. evaluate changes in the immune microenvironment after HCV eradication by comparing patients with HCC treated with DAA who had a SVR versus untreated controls (Amaddeo et al., 2020). Interestingly, there was no difference in immune profiles between the two groups, but there was a down regulation of interferon related genes after DAA treatment (Amaddeo et al., 2020). More studies are required to understand the effect of DAAs on the immune microenvironment, as well as the pathogenesis of HCC development of HCC among patients with a SVR.
Most HCC tumors arise in the setting of chronic liver disease and liver cirrhosis/fibrosis, which has a dramatic effect on the immune microenvironment. Ke et al. investigated the role of liver fibrosis to regulate tumor-infiltrating lymphocytes (TILs) and induce immunosuppression (Ke et al., 2021). Among patients with HCC, high CD8+ T cell infiltration was correlated with prolonged survival (Ke et al., 2021). Indeed, in mouse models with CCl4-induced liver fibrosis, as well as fibrotic human livers, elevated expression of immune checkpoints and decreased antitumor immunity was noted versus the control group (Ke et al., 2021). In addition, compared with patients who had low fibrosis scores, patients with high fibrosis scores had a significant reduction in tumor-cell-killing capacity of NK cells (Amer et al., 2018). Furthermore, in preclinical studies, Brandt et al. investigated the chemokine CXCL10 during fibrosis-associated hepatocarcinogenesis (Brandt et al., 2022). Of note, mice with Cxcl10 deficiency exhibited attenuated hepatocarcinogenesis. When fibrosis was induced, there was a pro-inflammatory tumor microenvironment, an accumulation of anti-tumoral immune cells in the tissue, and an accumulation of anti-tumoral T cells in the invasive tumor margin (Brandt et al., 2022).
Alcohol induced liver disease remains a major risk factor for the development of HCC contributing to nearly 30% of cases (McKillop and Schrum, 2009; Akinyemiju et al., 2017). Alcohol induced liver disease is a spectrum encompassing fatty liver, alcoholic hepatitis, and cirrhosis (Singal et al., 2014; Jinjuvadia et al., 2015). With chronic alcohol consumption, there is induction of the enzyme CYP2E1 which becomes the primary pathway of alcohol metabolism rather than alcohol dehydrogenase (Lu and Cederbaum, 2008). As a result of altered metabolism, there is increased acetaldehyde which carries metagenic and carcinogenic properties (Brooks and Theruvathu, 2005; McKillop and Schrum, 2009). Alcohol consumption has also been shown to cause alterations in the gut microbiome with increased absorption of endotoxin leading to activation of KCs (Bajaj et al., 2014). This activation of KCs results in the release of inflammatory cytokines causing increased collagen deposition, scarring and ultimately fibrosis (Thurman, 1998; Bode and Bode, 2005; Nagata et al., 2007). Furthermore, preclinical studies suggest chronic alcohol consumption reduces Tregs and causes an increase in helper T cells (Chen et al., 2016). The molecular mechanisms and full impact of changes in immune subsets on the progression of alcoholic liver disease has not yet been fully elucidated (Zhang CY. et al., 2022).
ICIs are now a therapeutic option for many malignancies and indications continue to expand (Le et al., 2015; Le et al., 2017; Eso et al., 2020). Inflammation plays a central role in the development of HCC as it drives carcinogenesis and therefore immunotherapies, including ICIs, have been proposed as part of an ideal treatment strategy for patients with HCC (Jenne and Kubes, 2013; Makarova-Rusher et al., 2015). With chronic antigen exposure, programmed cell death-1 (PD-1) is unregulated on immune cells including CD4+ and CD8+ T cells, NK cells, B cells, monocytes, DC, as well as immunosuppressive cells such as Tregs and myeloid-derived suppressor cells (MDSCs) (Prieto et al., 2015). When PD-1 binds with its ligand, PD-L1 and PD-L2, T cell receptor signaling is inhibited and thereby creates an exhausted dysfunctional T cell phenotype (Prieto et al., 2015) (Figure 3). Cancer cells have utilized this mechanism to form an immunosuppressive microenvironment allowing tumors cells to be unchecked by the immune system (Prieto et al., 2015). In addition, activation of T cells upregulates the immunosuppressive receptor, cytotoxic T lymphocyte associated protein-4 (CTLA-4). CTLA-4 acts as a check on the adaptive immune response by taking away the necessary costimulatory signal for T cell activation (Figure 3). CTLA-4 is present on activated T cells, DCs, and constitutively expressed on Tregs (Prieto et al., 2015; Inarrairaegui et al., 2017). Drugs targeting the PD-1/PD-L1 and CTLA-4/CD80/CD86 axes alone or in combination have been reported to be safe and effective among patients with advanced HCC (Figure 3). In addition, new and novel combination therapies are being tested (Table 1).
FIGURE 3. Under physiologic conditions, tumor antigens are recognized and presented to CD4+ T cells, which in turn further activate CD8+ T cells to initiate immune attack. T cell activation causes upregulation of CTLA-4 and PD-1 to prevent overactivation of the immune response. Immune checkpoint inhibitors block these inhibitory signals to increase the anti-tumor immune response. From: Brown ZJ et al. Safety, efficacy, and tolerability of immune checkpoint inhibitors in the treatment of hepatocellular carcinoma. Surgical Oncology June 2022. PMID 35395582.
TABLE 1. Ongoing studies of combination therapies with immune checkpoint inhibitors for patients with hepatocellular carcinoma.
Atezolizumab is a PD-L1 inhibitor that is now standard first line therapy in combination with bevacizumab (atezo-bev) for patients with advanced HCC based on the IMbrave150 trial (Finn et al., 2020a). Among patients with advanced HCC, atezo-bev demonstrated a 12 month overall survival (OS) of 67.2% versus 54.6% for patients in the sorafenib cohort; median progression free survival (PFS) was 6.8 versus 4.3 months in the atezo-bev and sorafenib cohorts, respectively. Of note, this trial only included patients with preserved liver function and therefore may not be applicable to the large population of patients in which HCC arises in the setting of liver dysfunction. Real world retrospective studies have compared atezo-bev to sorafenib or lenvatinib among patients with advanced HCC and liver dysfunction (Kim et al., 2022; Hiraoka et al., 2023; Jost-Brinkmann et al., 2023; Lee et al., 2023). These studies have demonstrated a similar survival advantage in the atezo-bev cohort (Kim et al., 2022; Hiraoka et al., 2023; Jost-Brinkmann et al., 2023; Lee et al., 2023). Currently, the IMbrave050 trial is evaluating the efficacy of adjuvant atezo-bev versus surveillance among patients with resected or ablated HCC (NCT04102098).
Tremelimumab, a CTLA-4 inhibitor, has had limited efficacy as monotherapy in preliminary clinical trials; in turn, combination therapy with the PD-L1 inhibitor, durvalumab, has been investigated (Sangro et al., 2013). In a phase II randomized trial, patients with advanced HCC received various combinations of tremelimumab and durvalumab or either drug as monotherapy (Kelley et al., 2021). The greatest efficacy was noted among patients treated with a tremelimumab priming dose and 4 weeks of durvalumab, resulting in an ORR of 24% and median OS of 18 months (Abou-Alfa et al., 2022). The follow-up phase III HIMALAYA trial compared durvalumab monotherapy, sorafenib, or a priming dose of tremelimumab with weekly durvalumab among patients who were treatment naïve with advanced HCC (Abou-Alfa et al., 2022). This study demonstrated that combination tremelimumab/durvalumab resulted in a median OS of 16.4 months versus 13.8 months among patients in the sorafenib cohort. As a result of this trial, combination tremelimumab/durvalumab was approved for patients with unresectable HCC in the United States and Europe (Keam, 2023). There is currently an ongoing phase III trial (EMERALD-3, NCT05301842) for patients with locally advanced HCC not amenable to curative transplant, ablation, or surgery. Patients are randomized to receive either the combination of transarterial chemoembolization (TACE), durvalumab, and tremelimumab with or without lenvatinib versus TACE alone.
Nivolumab is a PD-1 inhibitor first approved as second-line therapy for HCC. The Checkmate 040 trial evaluated nivolumab among patients with advanced HCC (up to Child-Pugh B) who may or may not have been treated with sorafenib (Kudo et al., 2021). The median duration of response was 9.9 months with an ORR of 12% and disease control rate of 55%. Nivolumab had an acceptable safety profile, including patients with underlying liver disease. The Checkmate 459 trial compared nivolumab with sorafenib among patients with advanced HCC in the first line setting (Yau et al., 2022). While there was no significant difference in OS between the two treatment arms, the results are difficult to interpret because several patients crossed over to the nivolumab arm after progressing on sorafenib. Retrospective studies have demonstrated similar findings with no survival advantage seen with nivolumab over sorafenib (Chapin et al., 2023).
As single agent, nivolumab demonstrated no improvement is survival compared with sorafenib; the combination of nivolumab and ipilimumab (CTLA-4 inhibitor) was administered at different doses and intervals to patients with advanced HCC previously treated with sorafenib (Yau et al., 2022). At 24 months, the OS for the combination nivolumab/ipilimumab cohort was 40%. Currently, a phase II randomized trial evaluating neoadjuvant nivolumab versus nivolumab/ipilimumab for patients with resectable HCC is in process (NCT03222076). Pre-liminary data has demonstrated a median PFS of 19.5 months for the nivolumab/ipilimumab cohort versus 9.4 months in the nivolumab monotherapy cohort (Kaseb et al., 2022). Several other ongoing trials are investigating the use of ICIs in the neoadjuvant and adjuvant setting (NCT 03682276, NCT 03299946, Checkmate 9DX, NCT 03383458).
Pembrolizumab is a PD-1 inhibitor and has had limited success in clinical trials as a single agent therapy for HCC (Zhu et al., 2018; Finn et al., 2020b). These data have resulted in other trials investigating the combination of pembrolizumab and lenvatinib (tyrosine kinase inhibitor), which has demonstrated a median PFS of 9.3 months and median OS of 22 months in phase I trial of patients with advanced HCC (Finn et al., 2020c). In a different study, Chen et al. reported on 170 treatment-naïve patients with unresectable HCC treated with the combination of pembrolizumab and lenvatinib with or without a hepatic artery infusion pump (HAIP) (Chen et al., 2021). Median OS was 17.7 months was in the HAIP/pembrolizumab/lenvatinib cohort versus 12.6 months among patients in the pembrolizumab/lenvatinib cohort (Chen et al., 2021). Currently, the LEAP-012 phase III randomized clinical trial is evaluating the use of TACE with or without pembrolizumab/lenvatinib for patients with intermediate stage HCC (NCT04246177).
Although there has been success in treatment of patient with advanced HCC using ICIs, response rates remain variable, sometimes poor, and often not durable. Mechanisms of resistance to immune therapies are becoming increasingly understood and this information may lead to improvement in outcomes through better patient selection or more targeted combination therapies. In general, there are two types of resistance to ICIs: primary and secondary/acquired. Primary resistance is characterized by failure of the HCC tumor to respond initially to ICIs. As evidenced in clinical trials, ICIs are only effective in about 30%–40% of patients with HCC, likely due to primary resistance (De Lorenzo et al., 2022). There are several mechanisms of primary resistance. One theory is related to the tumor mutational burden (TMB). A high TMB results in more neoantigens and possibly increased immune recognition, thereby making the tumor more immunogenic. Data from several studies have compared patients with low versus high TMB, have noted improved OS with ICIs in the latter group of individuals (Rizvi et al., 2015; Van Allen et al., 2015; Hugo et al., 2016; Ang et al., 2019). Another mechanism of primary resistance is dysfunctional neo-antigen presentation either through acquired genetic mutations that alter antigen presentation or decrease neo-antigen expression (McGranahan et al., 2016; McGranahan et al., 2017; Chowell et al., 2018; Ichinokawa et al., 2019). HCC tumors often contain a high copy number alteration burden and commonly have chromosome instability leading to a loss of genes needed for antigen presentation (Bassaganyas et al., 2020). To support this theory, Haber et al. demonstrated that patients with HCC who had upregulation of MHC-II molecules and increased neo-antigen presentation had a better response to ICIs (Haber et al., 2023).
Recent efforts have focused on the impact of liver disease on the immune microenvironment and subsequent response to therapy. For example, in a subgroup analysis of patients from IMBrave150 that evaluated atezo-bev, the ORR among patients with NASH-related HCC was 27% versus 35% among patients with HCC due to other etiologies (Ducreux et al., 2021). Pre-clinical studies have demonstrated loss of CD4+ T-cells in association with NASH suggesting immunotherapy may be impaired in the setting of NASH related hepatic tumors. Additionally, steatohepatitis was noted to reduce the ability of immunotherapeutic agents thereby inhibiting hepatic tumor growth through reduction of tumor infiltration by CD4+ T cells and effector memory cells (Ma et al., 2016; Brown et al., 2018; Heinrich et al., 2021).
Secondary or acquired resistance is characterized by patients who have disease recurrence or progression after initially responding to ICIs (De Lorenzo et al., 2022). These mechanisms are poorly understood, but are likely driven by tumor heterogeneity. While PD-1/PD-L1 and CTLA-4 are the more commonly targeted immune checkpoints, other immune checkpoints exist and their presence in the immune microenvironment may impact response to therapy. Targeting additional immune checkpoints, like TIM-3 or LAG-3, using combination therapy may help overcome immune exhaustion and secondary resistance (Zhou et al., 2017). In addition, tumor heterogeneity often results in ICI-sensitive and ICI-resistant cells. In theory, these resistant cells can survive after ICI therapy and clone themselves to become the majority population within the tumor. This process may explain why some patients respond to ICIs, but then ultimately progress (Weiss and Sznol, 2021). Profiling the tumor and using combination therapy may allow us to overcome tumor heterogeneity.
Epigenetics regulate gene expression without altering the DNA sequence. Alterations of epigenomic drivers can promote cancer onset, progression, and influence response to chemotherapy (Hogg et al., 2020; Wu et al., 2021). A study by Wu et al. demonstrated that patients with high epigenetic related genes (ERGs) benefited more from ICIs whereas patients with low ERGs had more T cell dysfunction and subsequentlyless clinical benefit from ICIs (Wu et al., 2021). In addition, the use of next-generation sequencing (NGS) has been utilized to determine predictive and prognostic information. Using NGS, Harding et al. found that patients with HCC tumors harboring Wnt/CTNNB1 mutations were refractory to ICIs with an associated shorter disease control rate, PFS, and OS (Harding et al., 2019).
Liver transplantation (LT) is the preferred treatment strategy for patients with liver cirrhosis and HCC as LT treats both the malignancy, as well as the underlying liver disease (Brown et al., 2023). Traditional LT criteria limit the potential pool of candidates based on strict HCC size and number (Milan criteria: 1 tumor >5 cm; 3 or fewer > 3 cm) (Adam et al., 2018). More recent data have demonstrated that patients successfully down-staged to within Milan LT criteria have post-transplant results similar to patients who initially present within Milan criteria (Yao et al., 2015; Kardashian et al., 2020). While ICIs have changed the treatment paradigm for patients with HCC, ICIs have only been sparingly used in the field of LT due to the potentially fatal complication of allograft rejection (Takamoto et al., 2023). Of note, graft rejection has been reported to be as high as 45% when ICIs are given prior to LT, especially if ICIs are administered within 90 days of LT (Qiao et al., 2021; Schnickel et al., 2022). Other studies have reported using ICIs for downstaging prior to LT, noting it to be relatively safe with a rejection rate of approximately 25% (Tabrizian et al., 2021; Gu et al., 2023). Kuo et al. investigated the washout period between last ICI dose and LT and noted a 42 days washout period for atezolizumab, nivolumab, or pembrolizumab (Kuo et al., 2023).
Several studies have also reported using ICIs following LT to prevent tumor recurrence with a rejection rate of 18.5% (Gu et al., 2023). Interestingly, Rudolph et al. noted that receipt of ICIs 3 months prior to LT may be safer than post-LT ICI administration (Rudolph et al., 2023). A current clinical trial (NCT0518550) is investigating atezo/bev in combinaton with TACE prior to LT among patients with HCC beyond Milan Crietria. The goal of the study is to assess the possibility to downstage patients and not increase the risk of 1-year post-transplant rejection. More data are needed to define the role of ICIs among patient undergoing LT patients. In particular, the competing mechanisms of anti-rejection medications and ICIs on the immune microenviroment require further elucidation.
Immune checkpoint inhibitors have been adopted as first line therapy for patients with advanced HCC. However, response rates remain variable and a majority of patients do not receive clinical benefit from ICI therapy. Recent efforts have focused on mechanisms of resistance to understand better why patients fail to response to ICIs. The immune microenvironment is frequently altered by liver disease, which can influence patient response to ICI treatment. A better understanding of the influence liver disease has on the immune microenvironment combined with knowledge gained from NGS and epigenetic alterations may improve patient selection, as well as provide novel targeted therapies to improve tumor response. In particular, the ability to understand and successfully target escape pathways may lead to improved outcomes for patients with advanced HCC.
All authors listed have made a substantial, direct, and intellectual contribution to the work and approved it for publication.
The authors declare that the research was conducted in the absence of any commercial or financial relationships that could be construed as a potential conflict of interest.
All claims expressed in this article are solely those of the authors and do not necessarily represent those of their affiliated organizations, or those of the publisher, the editors and the reviewers. Any product that may be evaluated in this article, or claim that may be made by its manufacturer, is not guaranteed or endorsed by the publisher.
Abou-Alfa, G. K., Lau, G., Kudo, M., Chan, S. L., Kelley, R. K., Furuse, J., et al. (2022). Tremelimumab plus durvalumab in unresectable hepatocellular carcinoma. NEJM Evid. 1 (8), EVIDoa2100070. doi:10.1056/evidoa2100070
Abul, K., Abbas, A. H. L., and Pillai, Shiv (2007). Cellular and molecular immunology. Philadelphia, PA: Saunders Elsevier.
Adam, R., Karam, V., Cailliez, V., O Grady, J. G., Mirza, D., Cherqui, D., et al. (2018). 2018 annual report of the European liver transplant registry (ELTR) - 50-year evolution of liver transplantation. Transpl. Int. 31 (12), 1293–1317. doi:10.1111/tri.13358
Agosti, P., Sabba, C., and Mazzocca, A. (2018). Emerging metabolic risk factors in hepatocellular carcinoma and their influence on the liver microenvironment. Biochim. Biophys. Acta Mol. Basis Dis. 1864 (2), 607–617. doi:10.1016/j.bbadis.2017.11.026
Akinyemiju, T., Abera, S., Ahmed, M., Alam, N., Alemayohu, M. A., Allen, C., et al. (2017). The burden of primary liver cancer and underlying etiologies from 1990 to 2015 at the global, regional, and national level: Results from the global burden of disease study 2015. JAMA Oncol. 3, 1683–1691. doi:10.1001/jamaoncol.2017.3055
Amaddeo, G., Nguyen, C. T., Maillé, P., Mulé, S., Luciani, A., Machou, C., et al. (2020). Intrahepatic immune changes after hepatitis c virus eradication by direct-acting antiviral therapy. Liver Int. official J. Int. Assoc. Study Liver 40 (1), 74–82. doi:10.1111/liv.14226
Amer, J., Salhab, A., Noureddin, M., Doron, S., Abu-Tair, L., Ghantous, R., et al. (2018). Insulin signaling as a potential natural killer cell checkpoint in fatty liver disease. Hepatol. Commun. 2 (3), 285–298. doi:10.1002/hep4.1146
Ang, C., Klempner, S. J., Ali, S. M., Madison, R., Ross, J. S., Severson, E. A., et al. (2019). Prevalence of established and emerging biomarkers of immune checkpoint inhibitor response in advanced hepatocellular carcinoma. Oncotarget 10 (40), 4018–4025. doi:10.18632/oncotarget.26998
Arrese, M., Cabrera, D., Kalergis, A. M., and Feldstein, A. E. (2016). Innate immunity and inflammation in NAFLD/NASH. Dig. Dis. Sci. 61 (5), 1294–1303. doi:10.1007/s10620-016-4049-x
Baeck, C., Wehr, A., Karlmark, K. R., Heymann, F., Vucur, M., Gassler, N., et al. (2012). Pharmacological inhibition of the chemokine CCL2 (MCP-1) diminishes liver macrophage infiltration and steatohepatitis in chronic hepatic injury. Gut 61 (3), 416–426. doi:10.1136/gutjnl-2011-300304
Bajaj, J. S., Heuman, D. M., Hylemon, P. B., Sanyal, A. J., White, M. B., Monteith, P., et al. (2014). Altered profile of human gut microbiome is associated with cirrhosis and its complications. J. hepatology 60 (5), 940–947. doi:10.1016/j.jhep.2013.12.019
Bassaganyas, L., Pinyol, R., Esteban-Fabró, R., Torrens, L., Torrecilla, S., Willoughby, C. E., et al. (2020). Copy-number alteration burden differentially impacts immune profiles and molecular features of hepatocellular carcinoma. Clin. Cancer Res. 26 (23), 6350–6361. doi:10.1158/1078-0432.CCR-20-1497
Blériot, C., Barreby, E., Dunsmore, G., Ballaire, R., Chakarov, S., Ficht, X., et al. (2021). A subset of Kupffer cells regulates metabolism through the expression of CD36. Immunity 54 (9), 2101–2116.e6. doi:10.1016/j.immuni.2021.08.006
Bode, C., and Bode, J. C. (2005). Activation of the innate immune system and alcoholic liver disease: Effects of ethanol per se or enhanced intestinal translocation of bacterial toxins induced by ethanol? Alcohol. Clin. Exp. Res. 29 (11 Suppl. l), 166S-71S–171s. doi:10.1097/01.alc.0000189280.19073.28
Borgia, M., Dal Bo, M., and Toffoli, G. (2021). Role of virus-related chronic inflammation and mechanisms of cancer immune-suppression in pathogenesis and progression of hepatocellular carcinoma. Cancers (Basel) 13 (17), 4387. doi:10.3390/cancers13174387
Brandt, E. F., Baues, M., Wirtz, T. H., May, J. N., Fischer, P., Beckers, A., et al. (2022). Chemokine CXCL10 modulates the tumor microenvironment of fibrosis-associated hepatocellular carcinoma. Int. J. Mol. Sci. 23 (15), 8112. doi:10.3390/ijms23158112
Brooks, P. J., and Theruvathu, J. A. (2005). DNA adducts from acetaldehyde: Implications for alcohol-related carcinogenesis. AlcoholFayettev. NY) 35 (3), 187–193. doi:10.1016/j.alcohol.2005.03.009
Brown, Z. J., Fu, Q., Ma, C., Kruhlak, M., Zhang, H., Luo, J., et al. (2018). Carnitine palmitoyltransferase gene upregulation by linoleic acid induces CD4(+) T cell apoptosis promoting HCC development. Cell Death Dis. 9 (6), 620. doi:10.1038/s41419-018-0687-6
Brown, Z. J., Greten, T. F., and Heinrich, B. (2019). Adjuvant treatment of hepatocellular carcinoma: Prospect of immunotherapy. Hepatology 70 (4), 1437–1442. doi:10.1002/hep.30633
Brown, Z. J., Tsilimigras, D. I., Ruff, S. M., Mohseni, A., Kamel, I. R., Cloyd, J. M., et al. (2023). Management of hepatocellular carcinoma: A review. JAMA Surg. 158 (4), 410–420. doi:10.1001/jamasurg.2022.7989
Chapin, W. J., Hwang, W. T., Karasic, T. B., McCarthy, A. M., and Kaplan, D. E. (2023). Comparison of nivolumab and sorafenib for first systemic therapy in patients with hepatocellular carcinoma and Child-Pugh B cirrhosis. Cancer Med. 12 (1), 189–199. doi:10.1002/cam4.4906
Chen, R. C., Xu, L. M., Du, S. J., Huang, S. S., Wu, H., Dong, J. J., et al. (2016). Lactobacillus rhamnosus GG supernatant promotes intestinal barrier function, balances Treg and TH17 cells and ameliorates hepatic injury in a mouse model of chronic-binge alcohol feeding. Toxicol. Lett. 241, 103–110. doi:10.1016/j.toxlet.2015.11.019
Chen, S., Xu, B., Wu, Z., Wang, P., Yu, W., Liu, Z., et al. (2021). Pembrolizumab plus lenvatinib with or without hepatic arterial infusion chemotherapy in selected populations of patients with treatment-naive unresectable hepatocellular carcinoma exhibiting PD-L1 staining: A multicenter retrospective study. BMC Cancer 21 (1), 1126. doi:10.1186/s12885-021-08858-6
Chowell, D., Morris, L. G. T., Grigg, C. M., Weber, J. K., Samstein, R. M., Makarov, V., et al. (2018). Patient HLA class I genotype influences cancer response to checkpoint blockade immunotherapy. Science 359 (6375), 582–587. doi:10.1126/science.aao4572
Conti, F., Buonfiglioli, F., Scuteri, A., Crespi, C., Bolondi, L., Caraceni, P., et al. (2016). Early occurrence and recurrence of hepatocellular carcinoma in HCV-related cirrhosis treated with direct-acting antivirals. J. hepatology 65 (4), 727–733. doi:10.1016/j.jhep.2016.06.015
Cusi, K. (2012). Role of obesity and lipotoxicity in the development of nonalcoholic steatohepatitis: Pathophysiology and clinical implications. Gastroenterology 142 (4), 711–725. doi:10.1053/j.gastro.2012.02.003
D'Souza, S., Lau, K. C., Coffin, C. S., and Patel, T. R. (2020). Molecular mechanisms of viral hepatitis induced hepatocellular carcinoma. World J. gastroenterology 26 (38), 5759–5783. doi:10.3748/wjg.v26.i38.5759
De Battista, D., Zamboni, F., Gerstein, H., Sato, S., Markowitz, T. E., Lack, J., et al. (2021). Molecular signature and immune landscape of HCV-associated hepatocellular carcinoma (HCC): Differences and similarities with HBV-HCC. J. Hepatocell. carcinoma 8, 1399–1413. doi:10.2147/JHC.S325959
De Lorenzo, S., Tovoli, F., and Trevisani, F. (2022). Mechanisms of primary and acquired resistance to immune checkpoint inhibitors in patients with hepatocellular carcinoma. Cancers (Basel) 14 (19), 4616. doi:10.3390/cancers14194616
Ding, Z., Dong, Z., Chen, Z., Hong, J., Yan, L., Li, H., et al. (2021). Viral status and efficacy of immunotherapy in hepatocellular carcinoma: A systematic review with meta-analysis. Front. Immunol. 12, 733530. doi:10.3389/fimmu.2021.733530
Ducreux, M., Zhu, A. X., Cheng, A-L., Galle, P. R., Ikeda, M., Nicholas, A., et al. (2021). IMbrave150: Exploratory analysis to examine the association between treatment response and overall survival (OS) in patients (pts) with unresectable hepatocellular carcinoma (HCC) treated with atezolizumab (atezo) + bevacizumab (bev) versus sorafenib (sor). J. Clin. Oncol. 39 (15_Suppl. l), 4071. doi:10.1200/jco.2021.39.15_suppl.4071
Eso, Y., Shimizu, T., Takeda, H., Takai, A., and Marusawa, H. (2020). Microsatellite instability and immune checkpoint inhibitors: Toward precision medicine against gastrointestinal and hepatobiliary cancers. J. gastroenterology 55 (1), 15–26. doi:10.1007/s00535-019-01620-7
Feng, M., Zhang, J., Anver, M., Hassan, R., and Ho, M. (2011). In vivo imaging of human malignant mesothelioma grown orthotopically in the peritoneal cavity of nude mice. J. Cancer 2, 123–131. doi:10.7150/jca.2.123
Finn, R. S., Ikeda, M., Zhu, A. X., Sung, M. W., Baron, A. D., Kudo, M., et al. (2020c). Phase ib study of lenvatinib plus pembrolizumab in patients with unresectable hepatocellular carcinoma. J. Clin. Oncol. 38 (26), 2960–2970. doi:10.1200/JCO.20.00808
Finn, R. S., Qin, S., Ikeda, M., Galle, P. R., Ducreux, M., Kim, T. Y., et al. (2020a). Atezolizumab plus bevacizumab in unresectable hepatocellular carcinoma. N. Engl. J. Med. 382 (20), 1894–1905. doi:10.1056/NEJMoa1915745
Finn, R. S., Ryoo, B. Y., Merle, P., Kudo, M., Bouattour, M., Lim, H. Y., et al. (2020b). Pembrolizumab as second-line therapy in patients with advanced hepatocellular carcinoma in KEYNOTE-240: A randomized, double-blind, phase III trial. J. Clin. Oncol. 38 (3), 193–202. doi:10.1200/JCO.19.01307
Forner, A., Reig, M., and Bruix, J. (2018). Hepatocellular carcinoma. Lancet (London, Engl. 391 (10127), 1301–1314. doi:10.1016/S0140-6736(18)30010-2
Garnelo, M., Tan, A., Her, Z., Yeong, J., Lim, C. J., Chen, J., et al. (2017). Interaction between tumour-infiltrating B cells and T cells controls the progression of hepatocellular carcinoma. Gut 66 (2), 342–351. doi:10.1136/gutjnl-2015-310814
Geissmann, F., Cameron, T. O., Sidobre, S., Manlongat, N., Kronenberg, M., Briskin, M. J., et al. (2005). Intravascular immune surveillance by CXCR6+ NKT cells patrolling liver sinusoids. PLoS Biol. 3 (4), e113. doi:10.1371/journal.pbio.0030113
Ghazarian, M., Revelo, X. S., Nohr, M. K., Luck, H., Zeng, K., Lei, H., et al. (2017). Type I interferon responses drive intrahepatic T cells to promote metabolic syndrome. Sci. Immunol. 2 (10), eaai7616. doi:10.1126/sciimmunol.aai7616
Gu, Y., Xu, S., Wang, Z., Yang, J., Zheng, S., Wei, Q., et al. (2023). When immunotherapy meets liver transplantation for hepatocellular carcinoma: A bumpy but promising road. Chin. J. Cancer Res. 35 (2), 92–107. doi:10.21147/j.issn.1000-9604.2023.02.02
Haber, P. K., Castet, F., Torres-Martin, M., Andreu-Oller, C., Puigvehí, M., Miho, M., et al. (2023). Molecular markers of response to anti-PD1 therapy in advanced hepatocellular carcinoma. Gastroenterology 164 (1), 72–88.e18. doi:10.1053/j.gastro.2022.09.005
Harding, J. J., Nandakumar, S., Armenia, J., Khalil, D. N., Albano, M., Ly, M., et al. (2019). Prospective genotyping of hepatocellular carcinoma: Clinical implications of next-generation sequencing for matching patients to targeted and immune therapies. Clin. Cancer Res. 25 (7), 2116–2126. doi:10.1158/1078-0432.CCR-18-2293
He, B., Wu, L., Xie, W., Shao, Y., Jiang, J., Zhao, Z., et al. (2017). The imbalance of Th17/Treg cells is involved in the progression of nonalcoholic fatty liver disease in mice. BMC Immunol. 18 (1), 33. doi:10.1186/s12865-017-0215-y
Heinrich, B., Brown, Z. J., Diggs, L. P., Vormehr, M., Ma, C., Subramanyam, V., et al. (2021). Steatohepatitis impairs T-cell-directed immunotherapies against liver tumors in mice. Gastroenterology 160 (1), 331–345.e6. doi:10.1053/j.gastro.2020.09.031
Hiraoka, A., Kumada, T., Tada, T., Hirooka, M., Kariyama, K., Tani, J., et al. (2023). Does first-line treatment have prognostic impact for unresectable HCC? Atezolizumab plus bevacizumab versus lenvatinib. Cancer Med. 12 (1), 325–334. doi:10.1002/cam4.4854
Hirsova, P., Bamidele, A. O., Wang, H., Povero, D., and Revelo, X. S. (2021). Emerging roles of T cells in the pathogenesis of nonalcoholic steatohepatitis and hepatocellular carcinoma. Front. Endocrinol. (Lausanne) 12, 760860. doi:10.3389/fendo.2021.760860
Hogg, S. J., Beavis, P. A., Dawson, M. A., and Johnstone, R. W. (2020). Targeting the epigenetic regulation of antitumour immunity. Nat. Rev. Drug Discov. 19 (11), 776–800. doi:10.1038/s41573-020-0077-5
Holt, M. P., Cheng, L., and Ju, C. (2008). Identification and characterization of infiltrating macrophages in acetaminophen-induced liver injury. J. Leukoc. Biol. 84 (6), 1410–1421. doi:10.1189/jlb.0308173
Hugo, W., Zaretsky, J. M., Sun, L., Song, C., Moreno, B. H., Hu-Lieskovan, S., et al. (2016). Genomic and transcriptomic features of response to anti-PD-1 therapy in metastatic melanoma. Cell 165 (1), 35–44. doi:10.1016/j.cell.2016.02.065
Ichinokawa, K., Nakanishi, Y., Hida, Y., Tsuchikawa, T., Kato, T., Itoh, T., et al. (2019). Downregulated expression of human leukocyte antigen class I heavy chain is associated with poor prognosis in non-small-cell lung cancer. Oncol. Lett. 18 (1), 117–126. doi:10.3892/ol.2019.10293
Inarrairaegui, M., Melero, I., and Sangro, B. (2017). Immunotherapy of hepatocellular carcinoma: Facts and hopes. Clin. cancer Res. official J. Am. Assoc. Cancer Res. 24, 1518–1524. doi:10.1158/1078-0432.CCR-17-0289
Jenne, C. N., and Kubes, P. (2013). Immune surveillance by the liver. Nat. Immunol. 14 (10), 996–1006. doi:10.1038/ni.2691
Jinjuvadia, R., and Liangpunsakul, S. Translational Research and Evolving Alcoholic Hepatitis Treatment Consortium (2015). Trends in alcoholic hepatitis-related hospitalizations, financial burden, and mortality in the United States. J. Clin. gastroenterology 49 (6), 506–511. doi:10.1097/MCG.0000000000000161
Jost-Brinkmann, F., Demir, M., Wree, A., Luedde, T., Loosen, S. H., Müller, T., et al. (2023). Atezolizumab plus bevacizumab in unresectable hepatocellular carcinoma: Results from a German real-world cohort. Aliment. Pharmacol. Ther. 57, 1313–1325. doi:10.1111/apt.17441
Kahraman, A., Schlattjan, M., Kocabayoglu, P., Yildiz-Meziletoglu, S., Schlensak, M., Fingas, C. D., et al. (2010). Major histocompatibility complex class I-related chains A and B (MIC A/B): A novel role in nonalcoholic steatohepatitis. Hepatology 51 (1), 92–102. doi:10.1002/hep.23253
Kang, T. W., Yevsa, T., Woller, N., Hoenicke, L., Wuestefeld, T., Dauch, D., et al. (2011). Senescence surveillance of pre-malignant hepatocytes limits liver cancer development. Nature 479 (7374), 547–551. doi:10.1038/nature10599
Kardashian, A., Florman, S. S., Haydel, B., Ruiz, R. M., Klintmalm, G. B., Lee, D. D., et al. (2020). Liver transplantation outcomes in a U.S. Multicenter cohort of 789 patients with hepatocellular carcinoma presenting beyond milan criteria. Hepatology 72 (6), 2014–2028. doi:10.1002/hep.31210
Kaseb, A. O., Hasanov, E., Cao, H. S. T., Xiao, L., Vauthey, J. N., Lee, S. S., et al. (2022). Perioperative nivolumab monotherapy versus nivolumab plus ipilimumab in resectable hepatocellular carcinoma: A randomised, open-label, phase 2 trial. lancet Gastroenterology hepatology 7 (3), 208–218. doi:10.1016/S2468-1253(21)00427-1
Ke, M. Y., Xu, T., Fang, Y., Ye, Y. P., Li, Z. J., Ren, F. G., et al. (2021). Liver fibrosis promotes immune escape in hepatocellular carcinoma via GOLM1-mediated PD-L1 upregulation. Cancer Lett. 513, 14–25. doi:10.1016/j.canlet.2021.05.007
Keam, S. J. (2023). Tremelimumab: First approval. Drugs 83 (1), 93–102. doi:10.1007/s40265-022-01827-8
Kelley, R. K., Sangro, B., Harris, W., Ikeda, M., Okusaka, T., Kang, Y. K., et al. (2021). Safety, efficacy, and pharmacodynamics of tremelimumab plus durvalumab for patients with unresectable hepatocellular carcinoma: Randomized expansion of a phase I/II study. J. Clin. Oncol. official J. Am. Soc. Clin. Oncol. 39 (27), 2991–3001. doi:10.1200/JCO.20.03555
Kim, B. K., Cheon, J., Kim, H., Kang, B., Ha, Y., Kim, D. Y., et al. (2022). Atezolizumab/bevacizumab vs. Lenvatinib as first-line therapy for unresectable hepatocellular carcinoma: A real-world, multi-center study. Cancers (Basel) 14 (7), 1747. doi:10.3390/cancers14071747
Koo, S. Y., Park, E. J., and Lee, C. W. (2020). Immunological distinctions between nonalcoholic steatohepatitis and hepatocellular carcinoma. Exp. Mol. Med. 52 (8), 1209–1219. doi:10.1038/s12276-020-0480-3
Kudo, M., Matilla, A., Santoro, A., Melero, I., Gracián, A. C., Acosta-Rivera, M., et al. (2021). CheckMate 040 cohort 5: A phase I/II study of nivolumab in patients with advanced hepatocellular carcinoma and child-pugh B cirrhosis. J. Hepatol. 75 (3), 600–609. doi:10.1016/j.jhep.2021.04.047
Kumar, V. (2013). NKT-Cell subsets: Promoters and protectors in inflammatory liver disease. J. Hepatol. 59 (3), 618–620. doi:10.1016/j.jhep.2013.02.032
Kuo, F. C., Chen, C. Y., Lin, N. C., Liu, C., Hsia, C. Y., and Loong, C. C. (2023). Optimizing the safe washout period for liver transplantation following immune checkpoint inhibitors with atezolizumab, nivolumab, or pembrolizumab. Transpl. Proc. 55 (4), 878–883. doi:10.1016/j.transproceed.2023.03.064
Le, D. T., Durham, J. N., Smith, K. N., Wang, H., Bartlett, B. R., Aulakh, L. K., et al. (2017). Mismatch repair deficiency predicts response of solid tumors to PD-1 blockade. Sci. (New York, NY) 357 (6349), 409–413. doi:10.1126/science.aan6733
Le, D. T., Uram, J. N., Wang, H., Bartlett, B. R., Kemberling, H., Eyring, A. D., et al. (2015). PD-1 blockade in tumors with mismatch-repair deficiency. N. Engl. J. Med. 372 (26), 2509–2520. doi:10.1056/NEJMoa1500596
Lee, Y. C., Huang, W. T., Lee, M. Y., Tsao, C. J., and Feng, Y. H. (2023). Bevacizumab and atezolizumab for unresectable hepatocellular carcinoma: Real-world data in taiwan-tainan medical oncology group H01 trial. Vivo 37 (1), 454–460. doi:10.21873/invivo.13099
Lefebvre, E., Moyle, G., Reshef, R., Richman, L. P., Thompson, M., Hong, F., et al. (2016). Antifibrotic effects of the dual CCR2/CCR5 antagonist cenicriviroc in animal models of liver and kidney fibrosis. PLoS One 11 (6), e0158156. doi:10.1371/journal.pone.0158156
Li, W., Han, J., and Wu, H. (2016). Regulatory T-cells promote hepatitis B virus infection and hepatocellular carcinoma progression. Chronic Dis. Transl. Med. 2 (2), 67–80. doi:10.1016/j.cdtm.2016.09.001
Lim, C. J., Lee, Y. H., Pan, L., Lai, L., Chua, C., Wasser, M., et al. (2019). Multidimensional analyses reveal distinct immune microenvironment in hepatitis B virus-related hepatocellular carcinoma. Gut 68 (5), 916–927. doi:10.1136/gutjnl-2018-316510
Liu, J., Geng, X., Hou, J., and Wu, G. (2021). New insights into M1/M2 macrophages: Key modulators in cancer progression. Cancer Cell Int. 21 (1), 389. doi:10.1186/s12935-021-02089-2
Llovet, J. M., Real, M. I., Montana, X., Planas, R., Coll, S., Aponte, J., et al. (2002). Arterial embolisation or chemoembolisation versus symptomatic treatment in patients with unresectable hepatocellular carcinoma: A randomised controlled trial. Lancet (London, Engl. 359 (9319), 1734–1739. doi:10.1016/S0140-6736(02)08649-X
Llovet, J. M., Zucman-Rossi, J., Pikarsky, E., Sangro, B., Schwartz, M., Sherman, M., et al. (2016). Hepatocellular carcinoma. Nat. Rev. Dis. Prim. 2, 16018. doi:10.1038/nrdp.2016.18
Lu, Y., and Cederbaum, A. I. (2008). CYP2E1 and oxidative liver injury by alcohol. Free Radic. Biol. Med. 44 (5), 723–738. doi:10.1016/j.freeradbiomed.2007.11.004
Ma, C., Kesarwala, A. H., Eggert, T., Medina-Echeverz, J., Kleiner, D. E., Jin, P., et al. (2016). NAFLD causes selective CD4(+) T lymphocyte loss and promotes hepatocarcinogenesis. Nature 531 (7593), 253–257. doi:10.1038/nature16969
MacParland, S. A., Liu, J. C., Ma, X. Z., Innes, B. T., Bartczak, A. M., Gage, B. K., et al. (2018). Single cell RNA sequencing of human liver reveals distinct intrahepatic macrophage populations. Nat. Commun. 9 (1), 4383. doi:10.1038/s41467-018-06318-7
Makarova-Rusher, O. V., Medina-Echeverz, J., Duffy, A. G., and Greten, T. F. (2015). The yin and yang of evasion and immune activation in HCC. J. hepatology 62 (6), 1420–1429. doi:10.1016/j.jhep.2015.02.038
Maluccio, M., Covey, A. M., Gandhi, R., Gonen, M., Getrajdman, G. I., Brody, L. A., et al. (2005). Comparison of survival rates after bland arterial embolization and ablation versus surgical resection for treating solitary hepatocellular carcinoma up to 7 cm. J. Vasc. Interv. Radiol. 16 (7), 955–961. doi:10.1097/01.RVI.0000161377.33557.20
Maluccio, M. A., Covey, A. M., Porat, L. B., Schubert, J., Brody, L. A., Sofocleous, C. T., et al. (2008). Transcatheter arterial embolization with only particles for the treatment of unresectable hepatocellular carcinoma. J. Vasc. Interv. Radiol. 19 (6), 862–869. doi:10.1016/j.jvir.2008.02.013
McGranahan, N., Furness, A. J., Rosenthal, R., Ramskov, S., Lyngaa, R., Saini, S. K., et al. (2016). Clonal neoantigens elicit T cell immunoreactivity and sensitivity to immune checkpoint blockade. Science 351 (6280), 1463–1469. doi:10.1126/science.aaf1490
McGranahan, N., Rosenthal, R., Hiley, C. T., Rowan, A. J., Watkins, T. B. K., Wilson, G. A., et al. (2017). Allele-specific HLA loss and immune escape in lung cancer evolution. Cell 171 (6), 1259–1271.e11. doi:10.1016/j.cell.2017.10.001
McKillop, I. H., and Schrum, L. W. (2009). Role of alcohol in liver carcinogenesis. Seminars liver Dis. 29 (2), 222–232. doi:10.1055/s-0029-1214377
Nagata, K., Suzuki, H., and Sakaguchi, S. (2007). Common pathogenic mechanism in development progression of liver injury caused by non-alcoholic or alcoholic steatohepatitis. J. Toxicol. Sci. 32 (5), 453–468. doi:10.2131/jts.32.453
Nakamoto, N., and Kanai, T. (2014). Role of toll-like receptors in immune activation and tolerance in the liver. Front. Immunol. 5, 221. doi:10.3389/fimmu.2014.00221
Obstfeld, A. E., Sugaru, E., Thearle, M., Francisco, A. M., Gayet, C., Ginsberg, H. N., et al. (2010). C-C chemokine receptor 2 (CCR2) regulates the hepatic recruitment of myeloid cells that promote obesity-induced hepatic steatosis. Diabetes 59 (4), 916–925. doi:10.2337/db09-1403
Prieto, J., Melero, I., and Sangro, B. (2015). Immunological landscape and immunotherapy of hepatocellular carcinoma. Nat. Rev. Gastroenterol. Hepatol. 12 (12), 681–700. doi:10.1038/nrgastro.2015.173
Qiao, Z. Y., Zhang, Z. J., Lv, Z. C., Tong, H., Xi, Z. F., Wu, H. X., et al. (2021). Neoadjuvant programmed cell death 1 (PD-1) inhibitor treatment in patients with hepatocellular carcinoma before liver transplant: A cohort study and literature review. Front. Immunol. 12, 653437. doi:10.3389/fimmu.2021.653437
Racanelli, V., and Rehermann, B. (2006). The liver as an immunological organ. Hepatology 43 (2 Suppl. 1), S54–S62. doi:10.1002/hep.21060
Rakhra, K., Bachireddy, P., Zabuawala, T., Zeiser, R., Xu, L., Kopelman, A., et al. (2010). CD4(+) T cells contribute to the remodeling of the microenvironment required for sustained tumor regression upon oncogene inactivation. Cancer Cell 18 (5), 485–498. doi:10.1016/j.ccr.2010.10.002
Ramadori, P., Kam, S., and Heikenwalder, M. (2022). T cells: Friends and foes in NASH pathogenesis and hepatocarcinogenesis. Hepatol. Baltim. Md) 75 (4), 1038–1049. doi:10.1002/hep.32336
Raoul, J. L., Forner, A., Bolondi, L., Cheung, T. T., Kloeckner, R., and de Baere, T. (2019). Updated use of TACE for hepatocellular carcinoma treatment: How and when to use it based on clinical evidence. Cancer Treat. Rev. 72, 28–36. doi:10.1016/j.ctrv.2018.11.002
Reig, M., Mariño, Z., Perelló, C., Iñarrairaegui, M., Ribeiro, A., Lens, S., et al. (2016). Unexpected high rate of early tumor recurrence in patients with HCV-related HCC undergoing interferon-free therapy. J. hepatology 65 (4), 719–726. doi:10.1016/j.jhep.2016.04.008
Rensen, S. S., Slaats, Y., Nijhuis, J., Jans, A., Bieghs, V., Driessen, A., et al. (2009). Increased hepatic myeloperoxidase activity in obese subjects with nonalcoholic steatohepatitis. Am. J. Pathol. 175 (4), 1473–1482. doi:10.2353/ajpath.2009.080999
Rizvi, N. A., Hellmann, M. D., Snyder, A., Kvistborg, P., Makarov, V., Havel, J. J., et al. (2015). Cancer immunology. Mutational landscape determines sensitivity to PD-1 blockade in non-small cell lung cancer. Science 348 (6230), 124–128. doi:10.1126/science.aaa1348
Rudolph, M., Shah, S. A., Quillin, R., Lemon, K., Olowokure, O., Latif, T., et al. (2023). Immune checkpoint inhibitors in liver transplant: A case series. J. Gastrointest. Oncol. 14 (2), 1141–1148. doi:10.21037/jgo-22-922
Sangro, B., Gomez-Martin, C., de la Mata, M., Iñarrairaegui, M., Garralda, E., Barrera, P., et al. (2013). A clinical trial of CTLA-4 blockade with tremelimumab in patients with hepatocellular carcinoma and chronic hepatitis C. J. Hepatol. 59 (1), 81–88. doi:10.1016/j.jhep.2013.02.022
Schnickel, G. T., Fabbri, K., Hosseini, M., Misel, M., Berumen, J., Parekh, J., et al. (2022). Liver transplantation for hepatocellular carcinoma following checkpoint inhibitor therapy with nivolumab. Am. J. Transpl. 22 (6), 1699–1704. doi:10.1111/ajt.16965
Sheka, A. C., Hameed, B., and Ikramuddin, S. (2020). Nonalcoholic steatohepatitis-reply. JAMA 324 (9), 899–900. doi:10.1001/jama.2020.10437
Singal, A. K., Kamath, P. S., Gores, G. J., and Shah, V. H. (2014). Alcoholic hepatitis: Current challenges and future directions. Clin. gastroenterology hepatology official Clin. Pract. J. Am. Gastroenterological Assoc. 12 (4), 555–564. doi:10.1016/j.cgh.2013.06.013
Stauffer, J. K., Scarzello, A. J., Jiang, Q., and Wiltrout, R. H. (2012). Chronic inflammation, immune escape, and oncogenesis in the liver: A unique neighborhood for novel intersections. Hepatology 56 (4), 1567–1574. doi:10.1002/hep.25674
Stienstra, R., Saudale, F., Duval, C., Keshtkar, S., Groener, J. E. M., van Rooijen, N., et al. (2010). Kupffer cells promote hepatic steatosis via interleukin-1beta-dependent suppression of peroxisome proliferator-activated receptor alpha activity. Hepatology 51 (2), 511–522. doi:10.1002/hep.23337
Sutti, S., and Albano, E. (2020). Adaptive immunity: An emerging player in the progression of NAFLD. Nat. Rev. Gastroenterol. Hepatol. 17 (2), 81–92. doi:10.1038/s41575-019-0210-2
Syn, W. K., Agboola, K. M., Swiderska, M., Michelotti, G. A., Liaskou, E., Pang, H., et al. (2012). NKT-associated hedgehog and osteopontin drive fibrogenesis in non-alcoholic fatty liver disease. Gut 61 (9), 1323–1329. doi:10.1136/gutjnl-2011-301857
Syn, W. K., Oo, Y. H., Pereira, T. A., Karaca, G. F., Jung, Y., Omenetti, A., et al. (2010). Accumulation of natural killer T cells in progressive nonalcoholic fatty liver disease. Hepatology 51 (6), 1998–2007. doi:10.1002/hep.23599
Tabrizian, P., Florman, S. S., and Schwartz, M. E. (2021). PD-1 inhibitor as bridge therapy to liver transplantation? Am. J. Transpl. 21 (5), 1979–1980. doi:10.1111/ajt.16448
Tacke, F. (2017). Targeting hepatic macrophages to treat liver diseases. J. Hepatol. 66 (6), 1300–1312. doi:10.1016/j.jhep.2017.02.026
Takamoto, T., Maruki, Y., and Kondo, S. (2023). Recent updates in the use of pharmacological therapies for downstaging in patients with hepatocellular carcinoma. Expert Opin. Pharmacother., 1–9. doi:10.1080/14656566.2023.2229728
Thurman, R. G. (1998). II. Alcoholic liver injury involves activation of Kupffer cells by endotoxin. Am. J. physiology 275 (4 Pt 1), G605–G611. doi:10.1152/ajpgi.1998.275.4.G605
Togashi, Y., Shitara, K., and Nishikawa, H. (2019). Regulatory T cells in cancer immunosuppression - implications for anticancer therapy. Nat. Rev. Clin. Oncol. 16 (6), 356–371. doi:10.1038/s41571-019-0175-7
Van Allen, E. M., Miao, D., Schilling, B., Shukla, S. A., Blank, C., Zimmer, L., et al. (2015). Genomic correlates of response to CTLA-4 blockade in metastatic melanoma. Science 350 (6257), 207–211. doi:10.1126/science.aad0095
van der Windt, D. J., Sud, V., Zhang, H., Varley, P. R., Goswami, J., Yazdani, H. O., et al. (2018). Neutrophil extracellular traps promote inflammation and development of hepatocellular carcinoma in nonalcoholic steatohepatitis. Hepatology 68 (4), 1347–1360. doi:10.1002/hep.29914
Van Herck, M. A., Weyler, J., Kwanten, W. J., Dirinck, E. L., De Winter, B. Y., Francque, S. M., et al. (2019). The differential roles of T cells in non-alcoholic fatty liver disease and obesity. Front. Immunol. 10, 82. doi:10.3389/fimmu.2019.00082
Wang, H., Zhang, H., Wang, Y., Brown, Z. J., Xia, Y., Huang, Z., et al. (2021). Regulatory T-cell and neutrophil extracellular trap interaction contributes to carcinogenesis in non-alcoholic steatohepatitis. J. Hepatol. 75 (6), 1271–1283. doi:10.1016/j.jhep.2021.07.032
Weiss, S. A., and Sznol, M. (2021). Resistance mechanisms to checkpoint inhibitors. Curr. Opin. Immunol. 69, 47–55. doi:10.1016/j.coi.2021.02.001
Wolf, M. J., Adili, A., Piotrowitz, K., Abdullah, Z., Boege, Y., Stemmer, K., et al. (2014). Metabolic activation of intrahepatic CD8+ T cells and NKT cells causes nonalcoholic steatohepatitis and liver cancer via cross-talk with hepatocytes. Cancer Cell 26 (4), 549–564. doi:10.1016/j.ccell.2014.09.003
Wu, Z. H., Yang, D. L., Wang, L., and Liu, J. (2021). Epigenetic and immune-cell infiltration changes in the tumor microenvironment in hepatocellular carcinoma. Front. Immunol. 12, 793343. doi:10.3389/fimmu.2021.793343
Yao, F. Y., Mehta, N., Flemming, J., Dodge, J., Hameed, B., Fix, O., et al. (2015). Downstaging of hepatocellular cancer before liver transplant: Long-term outcome compared to tumors within milan criteria. Hepatology 61 (6), 1968–1977. doi:10.1002/hep.27752
Yau, T., Park, J. W., Finn, R. S., Cheng, A. L., Mathurin, P., Edeline, J., et al. (2022). Nivolumab versus sorafenib in advanced hepatocellular carcinoma (CheckMate 459): A randomised, multicentre, open-label, phase 3 trial. Lancet Oncol. 23 (1), 77–90. doi:10.1016/S1470-2045(21)00604-5
Zamor, P. J., deLemos, A. S., and Russo, M. W. (2017). Viral hepatitis and hepatocellular carcinoma: Etiology and management. J. Gastrointest. Oncol. 8 (2), 229–242. doi:10.21037/jgo.2017.03.14
Zhang, C. Y., Liu, S., and Yang, M. (2022a). Regulatory T cells and their associated factors in hepatocellular carcinoma development and therapy. World J. gastroenterology 28 (27), 3346–3358. doi:10.3748/wjg.v28.i27.3346
Zhang, Y. Z., Zeb, A., and Cheng, L. F. (2022b). Exploring the molecular mechanism of hepatitis virus inducing hepatocellular carcinoma by microarray data and immune infiltrates analysis. Front. Immunol. 13, 1032819. doi:10.3389/fimmu.2022.1032819
Zhou, G., Sprengers, D., Boor, P. P. C., Doukas, M., Schutz, H., Mancham, S., et al. (2017). Antibodies against immune checkpoint molecules restore functions of tumor-infiltrating T cells in hepatocellular carcinomas. Gastroenterology 153 (4), 1107–1119. doi:10.1053/j.gastro.2017.06.017
Zhu, A. X., Finn, R. S., Edeline, J., Cattan, S., Ogasawara, S., Palmer, D., et al. (2018). Pembrolizumab in patients with advanced hepatocellular carcinoma previously treated with sorafenib (KEYNOTE-224): A non-randomised, open-label phase 2 trial. Lancet Oncol. 19 (7), 940–952. doi:10.1016/S1470-2045(18)30351-6
Keywords: Hepatocellular carcinoma (HCC), immune microenviroment, immune check inhibitor (ICI), liver disase, non-alcocholic fatty liver disease, cirrhosis, alcohol induced liver disease, viral heaptitis
Citation: Brown ZJ, Ruff SM and Pawlik TM (2023) The effect of liver disease on hepatic microenvironment and implications for immune therapy. Front. Pharmacol. 14:1225821. doi: 10.3389/fphar.2023.1225821
Received: 19 May 2023; Accepted: 31 July 2023;
Published: 07 August 2023.
Edited by:
Hui Liu, The University of Hong Kong, Hong Kong SAR, ChinaReviewed by:
Jun-Xi Xiang, The First Affiliated Hospital of Xi’an Jiaotong University, ChinaCopyright © 2023 Brown, Ruff and Pawlik. This is an open-access article distributed under the terms of the Creative Commons Attribution License (CC BY). The use, distribution or reproduction in other forums is permitted, provided the original author(s) and the copyright owner(s) are credited and that the original publication in this journal is cited, in accordance with accepted academic practice. No use, distribution or reproduction is permitted which does not comply with these terms.
*Correspondence: Timothy M. Pawlik, dGltLnBhd2xpa0Bvc3VtYy5lZHU=
Disclaimer: All claims expressed in this article are solely those of the authors and do not necessarily represent those of their affiliated organizations, or those of the publisher, the editors and the reviewers. Any product that may be evaluated in this article or claim that may be made by its manufacturer is not guaranteed or endorsed by the publisher.
Research integrity at Frontiers
Learn more about the work of our research integrity team to safeguard the quality of each article we publish.