- 1Hubei Key Laboratory of Diabetes and Angiopathy, Medicine Research Institute, Xianning Medical College, Hubei University of Science and Technology, Xianning, China
- 2School of Basic Medical Sciences, Xianning Medical College, Hubei University of Science and Technology, Xianning, China
- 3School of Mathematics and Statistics, Hubei University of Science and Technology, Xianning, China
Myocardial fibrosis, which is a common pathological manifestation of many cardiovascular diseases, is characterized by excessive proliferation, collagen deposition and abnormal distribution of extracellular matrix fibroblasts. In clinical practice, modern medicines, such as diuretic and β receptor blockers, and traditional Chinese medicines, such as salvia miltiorrhiza and safflower extract, have certain therapeutic effects on myocardial fibrosis. We reviewed some representative modern medicines and traditional Chinese medicines (TCMs) and their related molecular mechanisms for the treatment of myocardial fibrosis. These drugs alleviate myocardial fibrosis by affecting related signaling pathways and inhibiting myocardial fibrosis-related protein synthesis. This review will provide more references and help for the research and treatment of myocardial fibrosis.
1 Introduction
Myocardial fibrosis (MF) is a common pathological change in various heart diseases such as atherosclerosis and coronary disease, and the main pathological feature of myocardial infarction. An injury in the vessel wall during atherosclerosis can promote transforming growth factor-β (TGF-β) production by vascular and inflammatory cells, which can mediate fibrotic and inflammatory components in the lesion and lead to MF (Goumans and Ten Dijke, 2018). Coronary disease leads to MF by increasing the levels of type I and type III collagen (Ismail et al., 1999). In myocardial infarction, the persistent activated myofibroblasts in the infarct scar can continuously produce profibrotic factors that translocate to remote areas of the myocardium, which can result in the activation and proliferation of local fibroblasts and promote interstitial and perivascular fibrosis (Talman and Ruskoaho, 2016). In addition, mechanical stress in the undamaged left ventricular wall may be a risk factor for MF (Talman and Ruskoaho, 2016). According to an epidemiological survey, more than 800,000 people worldwide die of fibrous diseases, especially pulmonary and cardiac fibrosis, every year. MF is the response mechanism of myocardial injury. Its main pathological features are the proliferation and activation of cardiac fibroblasts (CFs) (Cheng et al., 2023), the deposition of extracellular matrix (ECM), the formation of scar tissue, a decrease in tissue compliance and a decline in cardiac function (Ren et al., 2022). MF is not only the pathological reaction of cardiovascular diseases including myocardial infarction, myocardial ischemia and sudden cardiac death, but also the risk factor for their further deterioration (Talman and Ruskoaho, 2016; Ambrose, 2006; Torrisi et al., 2020) (Figure 1). In recent years, studies on modern and traditional Chinese medicine (TCM) for the treatment of MF have been carried out, and a series of achievements in drug treatment have been obtained (González et al., 2018) (Table 1).
There is a long history of using modern medicine to treat MF. The development of anti-myocardial fibrosis drugs has decreased the case fatality rate for MF (Ghionzoli et al., 2022). The occurrence and development of MF are closely linked with the renin-angiotensin-aldosterone system (RAAS), oxidative stress, immune inflammation, the matrix metalloproteinase system, fibroblast proliferation and the TGF-β1/Smad3 signaling pathway [5]. Some drugs that target these pathways, such as RAAS inhibitors, have been proven to be effective in reducing ECM deposition in the myocardium (Friedman, 2022). However, MF is characterized by rapid onset, high mortality and complicated mechanisms, and effective treatments for MF via modern medicine are lacking. To date, no primarily antifibrotic drugs have been approved for the treatment of cardiovascular disease (Morfino et al., 2023). Although many prospective targets in the treatment of MF have been discovered, there is still no evidence of clinical benefits [11].
TCM has a long history of active ingredients, extracts, and herbal formulas that are produced by boiling, frying and other processing methods to treat human diseases (Li et al., 2021). Emerging evidence has demonstrated that bioactive ingredients in TCM have multiple antifibrotic effects; thus, TCM is recognized as an important and effective treatment strategy for MF (Li et al., 2021). TCM has good therapeutic potential for treating MF with low costs and side effects (Li et al., 2023). In addition, due to its multicomponent, multitarget and multilevel characteristics, TCM can also be used to treat different fibrotic and cardiovascular diseases in different stages (Li et al., 2023). However, it has some shortcomings. The specific effective ingredients in TCM and related molecular mechanisms are not clear, and there is a relatively weak theoretical and scientific basis for the use of TCM. Although there are various TCM methods for the treatment of MF, comparative pharmacological studies are lacking (Zhang et al., 2023). Further study is needed to elucidate the molecular mechanisms underlying the prevention and treatment of MF by TCM (Zhang et al., 2023). Clinically, a unified TCM syndrome differentiation system is also lacking because symptoms and manifestations vary with each individual (Ren et al., 2022). In addition, the production of TCM needs stricter and more standard regulation and quality control to improve the quality, purity and potency of TCM drugs.
Here, we reviewed some representative drugs and their molecular mechanisms. This article will provide more references and lay a foundation for further research on the treatment of MF.
A normal heart can progressively develop and transform into a fibrotic heart. MF occurs during the pathological process of myocardial infarction, myocardial ischemia and sudden cardiac death. Severe MF can accelerate and worsen myocardial infarction, myocardial ischemia, and sudden cardiac death. Myocardial infarction is mainly caused by heart tumors, coronary artery stenosis and myocardial injury. Myocardial ischemia is mainly caused by coronary artery spasm and hypoxia. Sudden cardiac death is mainly caused by atherosclerosis.
Modern medicine is divided into three categories: angiotensin converting enzyme inhibitors (ACEIs), diuretics, and β-receiver blockers. The typical representative ACEIs include perindopril and captopril; typical representative diuretics include eplerenone, tolasemide, and canrenoate potassium; and typical representative β-receiver blockers include propranolol and carvedilol. TCM is divided into three categories: Salvia miltiorrhiza and Carthamus tinctorius extract, astragaloside, and angesica. The typical Salvia miltiorrhiza and Carthamus tinctorius extracts include danshinone, safflower yellow and Salvia miltiorrhiza safflower. The typical representative astragalosides include astragaloside Ⅳ and astragalus saponins. The typical representative Angesica drugs include angelica sinensis and polysaccharide.
2 Modern medicine
2.1 Angiotensin converting enzyme inhibitors (ACEIs)
ACEIs are one of the most studied and effective drug types in the treatment of MF (Jessup et al., 2009). Blocking the renin angiotensin system (RAS) with ACEIs can prevent fibrosis development (Table 1) (Liu et al., 2021). Perindopril is a powerful and long-lasting ACEI (Li et al., 2019). Recent studies have shown that perindopril can alleviate MF by reducing the levels of galectin-3 (Gal-3) (Figure 2) (Li et al., 2019; Hu et al., 2022; Blanda et al., 2020; Slack et al., 2021). Captopril is a classic drug for the clinical treatment of MF (Yoshimura et al., 1989). It inhibits MF in two ways (Zhang et al., 2019; Plosker and McTavish, 1995; Schalekamp et al., 1980; Akhtar et al., 2022; Rha et al., 2021; Li et al., 2022). First, it can hinder the conversion of angiotensin I to angiotensin II by inhibiting ACE (Zhang et al., 2019; Plosker and McTavish, 1995; Schalekamp et al., 1980). It can improve ventricular remodeling and inhibit the occurrence of MF (Figure 2) (Akhtar et al., 2022). Second, captopril can inhibit MF by downregulating α-smooth muscle actin and vimentin expression (Rha et al., 2021; Li et al., 2022).
MF can result from cardiovascular diseases such as myocardial infarction and atherosclerosis. Although ACEI is the most commonly used antihypertensive drug, it is underutilized in the treatment of heart failure and left ventricular dysfunction after myocardial infarction and its application in treating atherosclerosis is limited (Howes and Christie, 1998; Dendorfer et al., 2005). In addition, the molecular mechanisms through which perindopril can alleviate interstitial collagen deposition in the myocardium and inhibit MF have not been elucidated, which hinders its application in the treatment of MF (Li et al., 2019).
2.2 Diuretics
It has been proven that the phenomenon of “aldosterone escape” determines the irreplaceable role of aldosterone antagonists in the treatment of MF (Table 1) (Buffolo et al., 2022; Schimmel et al., 2022; Rossier, 2021). It has been shown that eplerenone’s high affinity for Kv1.3 channels, potassium channel proteins on the T-lymphocyte (Treg) membrane, enables it to antagonize Kv1.3 channels directly to suppress the proliferation of Tregs that can secrete TGF-β (Shao et al., 2018; Chen et al., 2016). Therefore, eplerenone can alleviate MF (Shao et al., 2018; Chen et al., 2016). Canrenoate potassium, a new aldosterone receptor antagonist, has fewer side effects related to sex hormones than spironolactone (Bos et al., 2005). It alleviates isoproterenol-induced MF in rats by inhibiting the RAAS (Bos et al., 2004). The long-acting loop diuretic torsemide can antagonize aldosterone, antagonize the physiological effect of aldosterone on water sodium retention, inhibit the activation of the RAAS (Figure 2), and expel excess water from between tissues, which avoids the side effects of hyperactivity of the RAAS system caused by long-term use of diuretics (Kurlykina et al., 2017; Ghionzoli et al., 2022). Therefore, torsemide has a good cardioprotective effect that delays or reverses MF. In addition, inhibiting aldosterone synthase (CYP11B2) with torsemide prevents atrial fibrosis and atrial fibrillation in mice (Adam et al., 2015).
Diuretics, a type of the most frequently used medications, are well tolerated (Diuretics, 2021). Their common side effects resulting from the diuresis and mineral loss include weakness, dizziness, electrolyte imbalance, low sodium and low potassium (Diuretics, 2021). Aldosterone receptor antagonists have therapeutic effects on MF without increasing aldosterone or inducing sodium retention, but under other physiological conditions, it is unknown whether they also have the same potency (Rossier, 2021). Dose-dependent blood potassium elevation is an adverse reaction of eplerenone (Struthers et al., 2008). In addition, eplerenone is also associated with sexual side effects (Struthers et al., 2008). Torsemide has significant advantages in terms of diuretic effects and high bioavailability (Greene et al., 2021). Previous studies have proven that torsemide can inhibit MF and reverse ventricular remodeling (López et al., 2004; López et al., 2007). However, its detailed mechanisms remain unclear.
2.3 β receptor blockers
β receptor blockers can treat MF and other cardiovascular diseases (Ke et al., 2023). Hyperactivation of the sympathetic nerve is one of the common pathophysiological mechanisms of MF and many cardiovascular diseases (Table 1) (Czuriga and Edes, 2004; Perlini et al., 2005). β receptor blockers can inhibit the effects of epinephrine and norepinephrine on multiple tissues and systems, thereby inhibiting MF (Oliver et al., 2019).
Propranolol is a type of β receptor blocker (Tsai et al., 2022). It can cut off the β2 receptor and inhibit the cyclic adenosine phosphate (CAMP)/protein kinase A (PAK) nitric oxide signaling pathway to alleviate MF (Nuamnaichati et al., 2018). Propranolol inhibits MF by inhibiting the expression of fibroblast growth factor-23 (FGF-23) (Figure 2) (Tsai et al., 2022).
Carvedilol is the most effective way to improve the survival rate after myocardial infarction (Zheng et al., 2019). Carvedilol, a blocker of β1 and β2 adrenergic receptors (ARs), ameliorates MF induced by a high-fructose/high-fat diet in mice by enhancing cardiac β-arrestin 2 signaling (Figure 2) (Ibrahim et al., 2020; Ibrahim et al., 2021).
β receptor blockers are one of the most commonly used drugs and are usually well tolerated (Diuretics, 2018). However, β-adrenergic blockade can lead to common side effects, including bradycardia, fatigue, dizziness, depression, memory loss, insomnia, impotence and chills in the limbs (Diuretics, 2018). The mechanism by which propranolol can compensate for local nitric oxide deficiency in arterial circulation is still unclear, which limits its application (Pacca et al., 2002). Although carvedilol can inhibit MF, its exact mechanism remains unknown (Zhu et al., 2013).
2.4 Other modern medicines
Other modern medicines, such as irisin and clopidogrel, have therapeutic effects on MF (Pan et al., 2021; Jia et al., 2013). Irisin inhibits MF by inhibiting the levels of reactive oxygen species (ROS) and the NF-KB-Snail Signaling Pathway (Pan et al., 2021). Clopidogrel inhibits platelet activation, inhibits platelet leukocyte binding, and causes inflammatory cells to secrete cytokines (IL-1) into the heart, thus inhibiting MF (Table 2) (Jia et al., 2013; Liu et al., 2020).
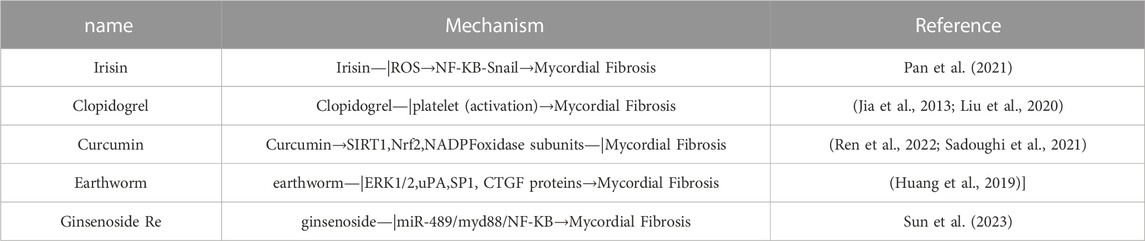
TABLE 2. Molecular mechanisms by which other modern medicines and traditional Chinese medicines treat MF.
2.5 Combined treatment of MF
Compared with single drug treatment of MF, the combined use of different types of drugs to treat MF is superior (Cohn, 2003). After myocardial infarction in rats, the therapeutic effect of the combination of eplerenone and the angiotensin Ⅱ receptor antagonist (ARB) candesartan was better than that of single drug therapy (Fraccarollo et al., 2003). The combination of β receptor blockers and ACEIs can provide a comprehensive neuroendocrine blocking effect, especially in the heart and blood vessels (Demkes et al., 2021).
Modern medicine inhibits MF by downregulating certain signaling pathways (TGF-β/Smad, cAMP-PAK-NO, RAAS), protein levels (factor-23, Gal-3, ACE) and receptors (β2 receptor). Gal-3: galectin-3; RAAS: renin-angiotensin-aldosterone system; Ang Ⅰ: angiotensin Ⅰ; Ang Ⅱ: angiotensin Ⅱ; TGF-β: transforming growth factor-β; β-Arrestin 2: a key protein regulating endothelial nitric oxide synthase activity; CYP11B2: aldosterone synthase; KV1.3: the Kv1.3 channel, a potassium channel protein on the membrane of T lymphocytes; cAMP-PAK-NO: cyclic adenosine phosphate (CAMP)/protein kinase A (PAK) nitric oxide signaling pathway; FGF-23: fibroblast growth factor-23; Smad: a TGF-β intracellular signaling molecules in the cytokine superfamily; α-SMA: α-smooth muscle actin; vimentin: a fibroblast marker; ACE: angiotensin converting enzyme.
3 Traditional Chinese medicine
3.1 Salvia miltiorrhiza and Carthamus tinctorius extract
Salvia miltiorrhiza and Carthamus tinctorius extracts (SCE) have been widely used in clinical practice and have achieved good effects in the treatment of myocardial ischemia and MF (Table 1) (Wang et al., 2020). The chalcone pigment safflower yellow (SY) is the main effective component of Carthamus tinctorius (Bai et al., 2020). SY can be used to treat atherosclerosis by reducing blood lipid levels and improving antioxidant capacity (Bai et al., 2020). Danshinone, an active component of Salvia miltiorrhiza, can ameliorate MF (Jiang et al., 2019). It has two mechanisms. First, danshinone has been suggested to reverse the increase in the levels of collagen type 1 (Col1), collagen type 3 (Col3) and α-smooth muscle actin (α-SMA) in HF rats induced by ligation of the left anterior descending branch (LAD) of the coronary artery through upregulating miR-205-3p, miR-29b, or miR-618 (Yang et al., 2023). Second, MF is achieved by downregulating the TGF-β/SMAD2/3 signaling pathway (Ren et al., 2022).
A mixture of active ingredients from SCE can exert a synergistic effect (Orgah et al., 2020). This combined medication is better than individual medicines in terms of the therapeutic effects on cardiovascular diseases (Orgah et al., 2020). It was reported that the effect of SCE on attenuating fibrosis was closely linked with the downregulation of TGF-β/Smad3 signaling and that SCE can inhibit the increase in the levels of H3K4me3 and H3k36me3 in the Smad3 promoter region induced by TGF-β in CFs (Figure 3) (Yang et al., 2019). In addition, when the levels of water-soluble effective components (salvianolic acid B and hydroxysafflower yellow A) of SCE are increased, they can protect the damaged heart by inhibiting tissue oxidation, inflammatory cell infiltration and platelet aggregation (Orgah et al., 2020).
The results of experimental research and clinical practice have proven that SCE can exert a good therapeutic effect on MF, but potential targets and molecular mechanisms of its inhibitory effects on MF need to be further studied.
3.2 Astragalus
Astragalus is a promising antifibrotic drug (Ren et al., 2023). Its main antifibrotic components are calycosin, astragaloside IV, astragalus polysaccharides and formononetin (Tan et al., 2020) (Table 1). It has been confirmed that astragalus membranaceus and its effective components can inhibit MF (Pan and Zhu, 2018). Astragalus saponins can inhibit the TGF-β/Smad pathway and P38 MAPK/NF-KB pathway, alleviating MF (Figure 3) (Zhu et al., 2022). Astragaloside IV has four mechanisms (Pan et al., 2021; Li et al., 2023; Zhang et al., 2022; Wan et al., 2018; Wei et al., 2020; Li et al., 2021; Lu et al., 2017). First, astragaloside IV inhibits the TGF-β-smad signaling pathway to inhibit MF (Pan et al., 2021; Li et al., 2023). Second, astragaloside IV alleviates MF by suppressing the ROS/caspase1/gasdermin D (GSDMD) signaling pathway in mice (Figure 3) (Zhang et al., 2022). Third, astragaloside IV exerts antifibrotic effects by inhibiting the NOD-like receptor family pyrin domain-containing 3 (NLRP3)/caspase I/IL-18 pathway in mice with isoproterenol-induced cardiac fibrosis (Figure 3) (Wan et al., 2018). Fourth, astragaloside IV significantly downregulates the transient receptor potential melastatin 7 (TRPM7) channel to inhibit hypoxia-induced cardiac fibrosis. Astragaloside IV can alleviate cardiac fibrosis by targeting the mir-135a-TRPM7-TGF-β/Smad pathway (Figure 3) (Wei et al., 2020; Li et al., 2021; Lu et al., 2017).
It has been reported that astragalus membranaceus has therapeutic effects on MF, but its material basis remains unclear (Ren et al., 2023). Moreover, there are many research limitations. Technical limitations suggest that the exact components of astragalus mongholicus Bunge or the compound medicine cannot be fully determined. In addition, the exact therapeutic effect of astragalus mongholicus Bunge on MF cannot be accurately determined by a multicomponent study, and the results of randomized controlled trials demonstrating its efficacy are not sufficient (Ren et al., 2023).
3.3 Angelica
Angelica contains a variety of components that have a wide range of biological activities, such as immune regulation, liver protection, and antiatherosclerotic, antitumor, anti-inflammatory and analgesic activities (Table 1) (Chang et al., 2021).
It has been reported that butylphthalide, the active ingredient of angelica, can activate the P13K/STAT3 pathway by downregulating miR-22, reducing H9c2 cell damage and inhibiting MF during myocardial infarction under hypoxia in vitro (Figure 3) (Lin et al., 2019). Angelica sinensis polysaccharide (ASP), a major bioactive component extracted from the roots of angelica, has antioxidative activity and can treat multiple diseases resulting from oxidative stress (Song et al., 2021). ASP can decrease ROS levels in a dose-dependent manner (Song et al., 2021; Pan and Zhu, 2018). It has been shown that ASP can alleviate cardiac fibrosis by inhibiting oxidative stress (Song et al., 2021; Pan and Zhu, 2018). The underlying and detailed mechanisms by which ASP prevents MF are worthy of further study.
3.4 Other TCM
Other TCMs, such as curcumin, earthworms and ginsenoside, have therapeutic effects on MF (Yu et al., 2019; Lai et al., 2015; Wang et al., 2021). Curcumin decreases cardiac fibrogenesis by activating SIRT1, increasing Nrf2, and increasing NADPH oxidase subunits (Sadoughi et al., 2021; Ren et al., 2022). Earthworms can inhibit MF by inhibiting the levels of MF-related proteins in H9c2 cells (Huang et al., 2019). Ginsenoside Re, the active ingredient of ginsenoside, can alleviate MF by inhibiting the miR-489/MyD88/NF-ΚB signaling pathway (Table 2) (Sun et al., 2023).
Traditional Chinese medicine inhibits MF by downregulating some related signaling pathways (P13K/STAT3, TGF-β/Smad, NF-KB), related molecules (miR-22, H3K36, H3K4me3, miR-618), and levels of reactive oxygen species. ROS: reactive oxygen species; NF-kB: K gene binding nuclear factor; TRPM7: transient receptor potential melastatin 7; P38: an important stress activating member of the MAPK family; STAT3: signal transduction and activator of transcription; P13K: phosphoinositide 3 kinase; NLRP3: nucleotide binding oligomerization domain-like receptor protein 3; IL-18: interleukin-18; caspase 1: cysteinyl aspartate specific proteinase 1; Col1: collagen type 1; Col3: collagen type 3; H3K4me3: trimethylation of lysine in the third subunit of histone 4; H3K36me3: histone 3 lysine 36 trimethylation.
Other modern medicine and traditional Chinese medicine inhibit MF through relevant molecular mechanisms (ROS/NF-KB-Snail pathway, ERK1/2, uPA, SP1, CTGF proteins). SIRT1: Silencing regulatory protein 1; Nrf2: encoded by the NFE2L2 gene, regulating approximately 250 genes involving in cellular homeostasis; H9C2 cells: Embryonic rat cardiomyocytes; uPA: a multifunctional serine protease with a relative molecular weight of 55,000 kDa, which can be synthesized by fibroblasts, monocyte, neutrophils, epithelial cells, and tumor cells; SP1: sequence-specific DNA binding proteins that regulates the transcription of cellular and viral genes rich in GC sequences in certain promoters. It is an important and essential transcription factor. CTGF protein: connective tissue growth factor protein.
4 Conclusion and perspective
To date, increasing attention has been focused on MF. MF is closely linked with the occurrence and development of various heart diseases, including atherosclerosis, coronary disease and myocardial infarction. Modern medicine and TCM, two different medical systems, are important for treating MF and have their own advantages and disadvantages. Compared with TCM, modern medicine has experienced rigorous scientific testing and regulation, but it has potential side effects and high treatment costs. In addition, TCM is effective in treating MF, but because of its unclear composition and targeting site, it is difficult to elucidate the detailed mechanisms (Liu et al., 2022). Fundamental scientific studies, rigorous clinical trials, standard production regulation and quality control can promote the development and credibility of TCM worldwide. These processes can contribute to a better understanding of TCM and develop its clinical value in the treatment of MF in the future (Yang et al., 2022).
Collocation of TCM and modern medicine in treating MF has attracted more attention. The combination of Guanxinning injection (GXNI) and modern medical techniques can better treat MF (Fan et al., 2023). It is confirmed that GXNI can substantially alleviate MF via H&E and masson staining methods (Fan et al., 2023). In addtion, the relationship between transforming growth factor-beta receptor 1 (TGFBR1) and calycosin has been revealed through using modern medical techniques including molecular docking, molecular dynamics (MD) simulation and surface plasmon resonance imaging (SPRi) (Chen et al., 2022). It is revealed that calycosin can attenuate MF by downregualting the TGFBR1 signaling pathway (Chen et al., 2022). Modern medical technology can promote the application of TCM in the prevention and treatment of MF. The rapid development of modern medicine is both challenge and opportunity for the integration of TCM and modern medicine. Modern medical technology and achievements are beneficial for complementary advantages of TCM and modern medicine (Xu and Chen, 2007).
We reviewed and summarized the research progress of modern medicine and TCM to treat MF, which can provide more references and be beneficial for further study of the development of drugs to treat MF.
Author contributions
All authors contributed to the manuscript. ZZ, LL, and XL edited this manuscript. ZR and XW conceived, edited, and finalized the manuscript. All authors contributed to the article and approved the submitted version.
Funding
This study was supported by the Natural Science Foundation of Hubei Province (Grant No. 2022CFB843), Hubei Provincial Science and Technology Plan Project (Grant No. 2021DFE025), the Xianning Science and Technology Plan Project (Grant No. 2021ZRKX024), Hubei University of Science and Technology School-level Fund (No. BK202121, and BK202220), Special Project on Diabetes and Angiopathy (No. 2022TNB04) and the Scientific Research and Innovation Team of Hubei University of Science and Technology (No. 2022T01).
Acknowledgments
We thank ZR and XW for contributing ideas and manuscript editing, ZZ, LL, and XL for manuscript editing.
Conflict of interest
The authors declare that the research was conducted in the absence of any commercial or financial relationships that could be construed as a potential conflict of interest.
Publisher’s note
All claims expressed in this article are solely those of the authors and do not necessarily represent those of their affiliated organizations, or those of the publisher, the editors and the reviewers. Any product that may be evaluated in this article, or claim that may be made by its manufacturer, is not guaranteed or endorsed by the publisher.
References
Adam, O., Zimmer, C., Hanke, N., Hartmann, R., Klemmer, B., Böhm, M., et al. (2015). Inhibition of aldosterone synthase (CYP11B2) by torasemide prevents atrial fibrosis and atrial fibrillation in mice. J. Mol. Cell Cardiol. 85, 140–150. doi:10.1016/j.yjmcc.2015.05.019
Akhtar, H., Al Sudani, H., Hussein, M., Mun, Farhan, and Elkholy, K. (2022). Effects of renin-angiotensin-aldosterone system inhibition on left ventricular hypertrophy, diastolic function, and functional status in patients with hypertrophic cardiomyopathy: A systematic review. Cureus 14 (7), e26642. doi:10.7759/cureus.26642
Ambrose, J. A. (2006). Myocardial ischemia and infarction. J. Am. Coll. Cardiol. 47 (11), D13–D17. doi:10.1016/j.jacc.2006.04.013
Bai, X., Wang, W. X., Fu, R. J., Yue, S. J., Gao, H., Chen, Y. Y., et al. (2020). Therapeutic potential of hydroxysafflor yellow A on cardio-cerebrovascular diseases. Front. Pharmacol. 11, 01265. doi:10.3389/fphar.2020.01265
Blanda, V., Bracale, U. M., Di Taranto, M. D., and Fortunato, G. (2020). Galectin-3 in cardiovascular diseases. Int. J. Mol. Sci. 21 (23), 9232. doi:10.3390/ijms21239232
Bos, R., Mougenot, N., Findji, L., Médiani, O., Vanhoutte, P. M., and Lechat, P. (2005). Inhibition of catecholamine-induced cardiac fibrosis by an aldosterone antagonist. J. Cardiovasc Pharmacol. 45 (1), 8–13. doi:10.1097/00005344-200501000-00003
Bos, R., Mougenot, N., Médiani, O., Vanhoutte, P., and Lechat, P. (2004). Potassium canrenoate, an aldosterone receptor antagonist, reduces isoprenaline induced cardiac fibrosis in the rat. J. Pharmacol. Exp. Ther. 309 (3), 1160–1166. doi:10.1124/jpet.103.063388
Buffolo, F., Tetti, M., Mulatero, P., and Monticone, S. (2022). Aldosterone as a mediator of cardiovascular damage. Hypertension 79 (9), 1899–1911. doi:10.1161/HYPERTENSIONAHA.122.17964
Chang, J., Ma, C., Guo, H., Ran, H., Chen, G., and Li, Y. (2021). Ultrafiltration extract of radix angelica sinensis and radix hedysari attenuates risk of low-dose X-ray radiation-induced myocardial fibrosis in vitro. Evid. Based Complement. Altern. Med. 2021, 5580828. doi:10.1155/2021/5580828
Chen, G., Xu, H., Xu, T., Ding, W., Zhang, G., Hua, Y., et al. (2022). Calycosin reduces myocardial fibrosis and improves cardiac function in post-myocardial infarction mice by suppressing TGFBR1 signaling pathways. Phytomedicine 104, 154277. doi:10.1016/j.phymed.2022.154277
Chen, X., Zhang, W., Wang, Q., Du, L., Yi, Y., Liu, Y., et al. (2016). Eplerenone inhibits atrial fibrosis in mutant TGF-β1 transgenic mice. Sci. China Life Sci. 59 (10), 1042–1047. doi:10.1007/s11427-016-0037-y
Cheng, Y., Wang, Y., Yin, R., Xu, Y., Zhang, L., Zhang, Y., et al. (2023). Central role of cardiac fibroblasts in myocardial fibrosis of diabetic cardiomyopathy. Front. Endocrinol. 14, 1162754. doi:10.3389/fendo.2023.1162754
Cohn, J. N. (2003). Interaction of beta-blockers and angiotensin receptor blockers/ACE inhibitors in heart failure. J. Renin Angiotensin Aldosterone Syst. 4 (3), 137–139. doi:10.3317/jraas.2003.021
Czuriga, I., and Edes, I. (2004). Beta-adrenergic receptor blockers in clinical practice. Orv. Hetil. 145 (38), 1951–1960.
Demkes, E. J., Wenker, S., Silvis, M. J. M., van, N. M. M. J., Visser, M. J., Jansen, M. S., et al. (2021). Neutral effects of combined treatment with GLP-1R agonist exenatide and MR antagonist potassium canrenoate on cardiac function in porcine and murine chronic heart failure models. Front. Pharmacol. 12, 702326. doi:10.3389/fphar.2021.702326
Dendorfer, A., Dominiak, P., and Schunkert, H. (2005). ACE inhibitors and angiotensin II receptor antagonists. Handb. Exp. Pharmacol. 170, 407–442. doi:10.1007/3-540-27661-0_15
Diuretics, (2018). “Beta adrenergic blocking agents,” in LiverTox: Clinical and research information on drug-induced liver injury (Bethesda, MD, USA: National Institute of Diabetes and Digestive and Kidney Diseases).
Diuretics, (2021). LiverTox: Clinical and research information on drug-induced liver injury. Bethesda, MD, USA: National Institute of Diabetes and Digestive and Kidney Diseases.
Fan, S., Xiao, G., Ni, J., Zhao, Y., Du, H., Liang, Y., et al. (2023). Guanxinning injection ameliorates cardiac remodeling in HF mouse and 3D heart spheroid models via p38/FOS/MMP1-mediated inhibition of myocardial hypertrophy and fibrosis. Biomed. Pharmacother. 162, 114642. doi:10.1016/j.biopha.2023.114642
Fraccarollo, D., Galuppo, P., Hildemann, S., Christ, M., Ertl, G., and Bauersachs, J. (2003). Additive improvement of left ventricular remodeling and neurohormonal activation by aldosterone receptor blockade with eplerenone and ACE inhibition in rats with myocardial infarction. J. Am. Coll. Cardiol. 42 (9), 1666–1673. doi:10.1016/j.jacc.2003.05.003
Friedman, S. L. (2022). Fighting cardiac fibrosis with CAR T cells. N. Engl. J. Med. 386 (16), 1576–1578. doi:10.1056/NEJMcibr2201182
Ghionzoli, N., Gentile, F., Del Franco, A. M., Castiglione, V., Aimo, A., Giannoni, A., et al. (2022). Current and emerging drug targets in heart failure treatment. Heart Fail Rev. 27 (4), 1119–1136. doi:10.1007/s10741-021-10137-2
González, A., Schelbert, E. B., Díez, J., and Butler, J. (2018). Myocardial interstitial fibrosis in heart failure: Biological and translational perspectives. J. Am. Coll. Cardiol. 71 (15), 1696–1706. doi:10.1016/j.jacc.2018.02.021
Goumans, M. J., and Ten Dijke, P. (2018). TGF-Β signaling in control of cardiovascular function. Cold Spring Harb. Perspect. Biol. 10 (2), a022210. doi:10.1101/cshperspect.a022210
Greene, S. J., Velazquez, E. J., Anstrom, K. J., Eisenstein, E. L., Sapp, S., Morgan, S., et al. (2021). Pragmatic design of randomized clinical trials for heart failure: Rationale and design of the TRANSFORM-HF trial. JACC Heart Fail 9 (5), 325–335. doi:10.1016/j.jchf.2021.01.013
Howes, L. G., and Christie, N. (1998). Angiotensin receptor antagonists and ACE inhibitors. Aust. Fam. Physician 27 (10), 914–917, 919-921.
Hu, G., Wu, J., Gu, H., Deng, X., Xu, W., Feng, S., et al. (2022). Galectin-3-centered paracrine network mediates cardiac inflammation and fibrosis upon β-adrenergic insult. Sci. China Life Sci. 66, 1067–1078. doi:10.1007/s11427-022-2189-x
Huang, P., Shibu, M. A., Kuo, C., Han, C., Chen, Y., Lo, F., et al. (2019). Pheretima aspergillum extract attenuates high-KCl-induced mitochondrial injury and pro-fibrotic events in cardiomyoblast cells. Environ. Toxicol. 34 (8), 921–927. doi:10.1002/tox.22763
Ibrahim, W. S., Ahmed, H. M. S., Mahmoud, A. A. A., Mahmoud, M. F., and Ibrahim, I. A. A. E. (2021). Propranolol and low-dose isoproterenol ameliorate insulin resistance, enhance β-arrestin2 signaling, and reduce cardiac remodeling in high-fructose, high-fat diet-fed mice: Comparative study with metformin. Life Sci. 286, 120055. doi:10.1016/j.lfs.2021.120055
Ibrahim, W. S., Ibrahim, I. A. A. E., Mahmoud, M. F., and Mahmoud, A. A. A. (2020). Carvedilol diminishes cardiac remodeling induced by high-fructose/high-fat diet in mice via enhancing cardiac β-arrestin2 signaling. J. Cardiovasc Pharmacol. Ther. 25 (4), 354–363. doi:10.1177/1074248420905683
Ismail, A., Khosravi, H., and Olson, H. (1999). The role of infection in atherosclerosis and coronary artery disease: A new therapeutic target. Heart Dis. 1 (4), 233–240.
Jessup, J. A., Westwood, B. M., Chappell, M. C., and Groban, L. (2009). Dual ACE-inhibition and AT1 receptor antagonism improves ventricular lusitropy without affecting cardiac fibrosis in the congenic mRen2.Lewis rat. Ther. Adv. Cardiovasc Dis. 3 (4), 245–257. doi:10.1177/1753944709338489
Jia, L., Qi, G., Liu, O., Yang, M., and Cui, W., (2013). Inhibition of platelet activation by clopidogrel prevents hypertension-induced cardiac inflammation and fibrosis. Cardiovasc Drugs Ther. 27 (6), 521–530. doi:10.1007/s10557-013-6471-z
Jiang, Z., Gao, W., and Huang, L. (2019). Tanshinones, critical pharmacological components in Salvia miltiorrhiza. Front. Pharmacol. 10, 202. doi:10.3389/fphar.2019.00202
Ke, F., Kuang, W., Hu, X., Li, C., Ma, W., Shi, D., et al. (2023). A novel vaccine targeting β1-adrenergic receptor. Hypertens. Res. 46 (6), 1582–1595. doi:10.1038/s41440-023-01265-3
Kurlykina, N., Seredenina, E., and Orlova, Y. (2017). Use of loop diuretics in heart failure: Current aspects. Ter. Arkh. 89 (9), 115–119. doi:10.17116/terarkh2017899115-119
Lai, C. H., Han, C. K., Shibu, M. A., Pai, P. Y., Ho, T. J., Day, C. H., et al. (2015). Lumbrokinase from earthworm extract ameliorates second-hand smoke-induced cardiac fibrosis. Environ. Toxicol. 30 (10), 1216–1225. doi:10.1002/tox.21993
Li, C., Meng, X., Wang, L., and Dai, X. (2023). Mechanism of action of non-coding RNAs and traditional Chinese medicine in myocardial fibrosis: Focus on the TGF-β/Smad signaling pathway. Front. Pharmacol. 14, 1092148. doi:10.3389/fphar.2023.1092148
Li, N., Hang, W., Shu, H., and Zhou, N. (2022). Pirfenidone alleviates cardiac fibrosis induced by pressure overload via inhibiting TGF-β1/Smad3 signalling pathway. J. Cell Mol. Med. 26 (16), 4548–4555. doi:10.1111/jcmm.17478
Li, S., Li, S., Hao, X., Zhang, Y., and Deng, W. (2019). Perindopril and a galectin-3 inhibitor improve ischemic heart failure in rabbits by reducing gal-3 expression and myocardial fibrosis. Front. Physiol. 10, 267. doi:10.3389/fphys.2019.00267
Li, X., Li, L., Lei, W., Chua, H. Z., Li, Z., Huang, X., et al. (2021). Traditional Chinese medicine as a therapeutic option for cardiac fibrosis: Pharmacology and mechanisms. Biomed. Pharmacother. 142, 111979. doi:10.1016/j.biopha.2021.111979
Lin, C. C., Chen, S. Y., Lien, H. Y., Lin, S. Z., and Lee, T. M. (2019). Targeting the PI3K/STAT3 axis modulates age-related differences in macrophage phenotype in rats with myocardial infarction. J. Cell Mol. Med. 23 (9), 6378–6392. doi:10.1111/jcmm.14526
Liu, M., Long, X., Xu, J., Chen, M., Yang, H., Guo, X., et al. (2022). Hypertensive heart disease and myocardial fibrosis: How traditional Chinese medicine can help addressing unmet therapeutical needs. Pharmacol. Res. 185, 106515. doi:10.1016/j.phrs.2022.106515
Liu, Y., Fan, Y., Li, J., Chen, M., Chen, A., Yang, D., et al. (2021). Combination of LCZ696 and ACEI further improves heart failure and myocardial fibrosis after acute myocardial infarction in mice. Biomed. Pharmacother. 133, 110824. doi:10.1016/j.biopha.2020.110824
Liu, Y., Lv, H., Tan, R., An, X., Niu, X. H., Liu, Y. J., et al. (2020). Platelets promote Ang II (angiotensin II)-Induced atrial fibrillation by releasing TGF-β1 (transforming growth factor-β1) and interacting with fibroblasts. Hypertension 76 (6), 1856–1867. doi:10.1161/HYPERTENSIONAHA.120.15016
López, B., González, A., Beaumont, J., Querejeta, R., Larman, M., and Díez, J. (2007). Identification of a potential cardiac antifibrotic mechanism of torasemide in patients with chronic heart failure. J. Am. Coll. Cardiol. 50 (9), 859–867. doi:10.1016/j.jacc.2007.04.080
López, B., Querejeta, R., González, A., Sánchez, E., Larman, M., and Díez, J. (2004). Effects of loop diuretics on myocardial fibrosis and collagen type I turnover in chronic heart failure. J. Am. Coll. Cardiol. 43 (11), 2028–2035. doi:10.1016/j.jacc.2003.12.052
Lu, J., Wang, Q. Y., Zhou, Y., Lu, X. C., Liu, Y. H., Wu, Y., et al. (2017). AstragalosideⅣ against cardiac fibrosis by inhibiting TRPM7 channel. Phytomedicine 30, 10–17. doi:10.1016/j.phymed.2017.04.002
Morfino, P., Aimo, A., Castiglione, V., Gálvez-Montón, C., Emdin, M., and Bayes-Genis, A. (2023). Treatment of cardiac fibrosis: From neuro-hormonal inhibitors to CAR-T cell therapy. Heart Fail Rev. 28 (2), 555–569. doi:10.1007/s10741-022-10279-x
Nuamnaichati, N., Sato, V. H., Moongkarndi, P., Parichatikanond, W., and Mangmool, S. (2018). Sustained β-AR stimulation induces synthesis and secretion of growth factors in cardiac myocytes that affect on cardiac fibroblast activation. Life Sci. 193, 257–269. doi:10.1016/j.lfs.2017.10.034
Oliver, E., Mayor, F., and D'Ocon, P. (2019). Beta-blockers: Historical perspective and mechanisms of action. Rev. Esp. Cardiol. 72 (10), 853–862. doi:10.1016/j.rec.2019.04.006
Orgah, J. O., He, S., Wang, Y., Jiang, M., and Orgah, E. A., (2020). Pharmacological potential of the combination of Salvia miltiorrhiza (Danshen) and Carthamus tinctorius (Honghua) for diabetes mellitus and its cardiovascular complications. Pharmacol. Res. 153, 104654. doi:10.1016/j.phrs.2020.104654
Pacca, S. R., de Azevedo, A. P., De Oliveira, C. F., De Luca, I. M., De Nucci, G., and Antunes, E. (2002). Attenuation of hypertension, cardiomyocyte hypertrophy, and myocardial fibrosis by beta-adrenoceptor blockers in rats under long-term blockade of nitric oxide synthesis. J. Cardiovasc Pharmacol. 39 (2), 201–207. doi:10.1097/00005344-200202000-00006
Pan, H., and Zhu, L. (2018). Retracted: Angelica sinensis polysaccharide protects rat cardiomyocytes H9c2 from hypoxia-induced injury by down-regulation of microRNA-22. Biomed. Pharmacother. 106, 225–231. doi:10.1016/j.biopha.2018.06.120
Pan, J. A., Zhang, H., Lin, H., Gao, L., Zhang, H. L., Zhang, J. F., et al. (2021). Irisin ameliorates doxorubicin-induced cardiac perivascular fibrosis through inhibiting endothelial-to-mesenchymal transition by regulating ROS accumulation and autophagy disorder in endothelial cells. Redox Biol. 46, 102120. doi:10.1016/j.redox.2021.102120
Perlini, S., Palladini, G., Ferrero, I., Tozzi, R., Fallarini, S., Facoetti, A., et al. (2005). Sympathectomy or doxazosin, but not propranolol, blunt myocardial interstitial fibrosis in pressure-overload hypertrophy. Hypertension 46 (5), 1213–1218. doi:10.1161/01.HYP.0000185689.65045.4c
Plosker, G. L., and McTavish, D. (1995). Captopril. A review of its pharmacology and therapeutic efficacy after myocardial infarction and in ischaemic heart disease. Drugs Aging 7 (3), 226–253. doi:10.2165/00002512-199507030-00007
Ren, C., Liu, K., Zhao, X., Guo, H., Luo, Y., Chang, J., et al. (2022). Research progress of traditional Chinese medicine in treatment of myocardial fibrosis. Front. Pharmacol. 13, 853289. doi:10.3389/fphar.2022.853289
Ren, C., Zhao, X., Liu, K., Wang, L., Chen, Q., Jiang, H., et al. (2023). Research progress of natural medicine Astragalus mongholicus Bunge in treatment of myocardial fibrosis. J. Ethnopharmacol. 305, 116128. doi:10.1016/j.jep.2022.116128
Rha, E. Y., Kim, J. W., Kim, J. H., and Yoo, G. (2021). Angiotensin-converting enzyme inhibitor, captopril, improves scar healing in hypertensive rats. Int. J. Med. Sci. 18 (4), 975–983. doi:10.7150/ijms.50197
Rossier, M. F. (2021). The cardiac mineralocorticoid receptor (mr): A therapeutic target against ventricular arrhythmias. Front. Endocrinol. (Lausanne). 12, 694758. doi:10.3389/fendo.2021.694758
Sadoughi, F., Hallajzadeh, J., Mirsafaei, L., Asemi, Z., Zahedi, M., Mansournia, M. A., et al. (2021). Cardiac fibrosis and curcumin: A novel perspective on this natural medicine. Mol. Biol. Rep. 48 (11), 7597–7608. doi:10.1007/s11033-021-06768-1
Schalekamp, M. A., de Bruyn, J. H., Wenting, G. J., Man in 't Veld, A. J., and Derkx, F. H. (1980). A new point of attack for the treatment of hypertension; captopril, an orally active inhibitor of the enzymatic conversion of angiotensin I into angiotensin II. Ned. Tijdschr. Geneeskd. 124 (47), 1996–2003.
Schimmel, K., Ichimura, K., Reddy, S., Haddad, F., and Spiekerkoetter, E. (2022). Cardiac fibrosis in the pressure overloaded left and right ventricle as a therapeutic target. Front. Cardiovasc Med. 9, 886553. doi:10.3389/fcvm.2022.886553
Shao, P., Liu, C., Xu, Q., Zhang, B., Li, S., Wu, Y., et al. (2018). Eplerenone reverses cardiac fibrosis via the suppression of tregs by inhibition of Kv1.3 channel. Front. Physiol. 9, 899. doi:10.3389/fphys.2018.00899
Slack, R. J., Mills, R., and Mackinnon, A. C. (2021). The therapeutic potential of galectin-3 inhibition in fibrotic disease. Int. J. Biochem. Cell Biol. 130, 105881. doi:10.1016/j.biocel.2020.105881
Song, X., Kong, J., Song, J., Pan, R., and Wang, L. (2021). Angelica sinensis polysaccharide alleviates myocardial fibrosis and oxidative stress in the heart of hypertensive rats. Comput. Math. Methods Med. 2021, 6710006. doi:10.1155/2021/6710006
Struthers, A., Krum, H., and Williams, G. H. (2008). A comparison of the aldosterone-blocking agents eplerenone and spironolactone. Clin. Cardiol. 31 (4), 153–158. doi:10.1002/clc.20324
Sun, J., Wang, R., Chao, T., Peng, J., Wang, C., and Chen, K. (2023). Ginsenoside Re inhibits myocardial fibrosis by regulating miR-489/myd88/NF-κB pathway. J. Ginseng Res. 47 (2), 218–227. doi:10.1016/j.jgr.2021.11.009
Talman, V., and Ruskoaho, H. (2016). Cardiac fibrosis in myocardial infarction-from repair and remodeling to regeneration. Cell Tissue Res. 365 (3), 563–581. doi:10.1007/s00441-016-2431-9
Tan, Y. Q., Chen, H. W., and Li, J. (2020). Astragaloside IV: An effective drug for the treatment of cardiovascular diseases. Drug Des. Devel Ther. 14, 3731–3746. doi:10.2147/DDDT.S272355
Torrisi, M., Pennisi, G., Russo, I., Amico, F., Esposito, M., Liberto, A., et al. (2020). Sudden cardiac death in anabolic-androgenic steroid users: A literature review. Med. Kaunas. 56 (11), 587. doi:10.3390/medicina56110587
Tsai, C. K., Chen, B. H., Chen, H. H., Hsieh, R. J., Lee, J. C., Chu, Y. T., et al. (2022). Low-dose propranolol prevents functional decline in catecholamine-induced acute heart failure in rats. Toxics 10 (5), 238. doi:10.3390/toxics10050238
Wan, Y., Xu, L., Wang, Y., Tuerdi, N., Ye, M., and Qi, R. (2018). Preventive effects of astragaloside IV and its active sapogenin cycloastragenol on cardiac fibrosis of mice by inhibiting the NLRP3 inflammasome. Eur. J. Pharmacol. 833, 545–554. doi:10.1016/j.ejphar.2018.06.016
Wang, Q., Fu, W., Yu, X., Xu, H., Sui, D., and Wang, Y. (2021). Ginsenoside Rg2 alleviates myocardial fibrosis by regulating TGF-β1/Smad signalling pathway. Pharm. Biol. 59 (1), 106–113. doi:10.1080/13880209.2020.1867197
Wang, Y., Shi, Y., Zou, J., Zhang, X., Liang, Y., Tai, J., et al. (2020). Network pharmacology exploration reveals a common mechanism in the treatment of cardio-cerebrovascular disease with Salvia miltiorrhiza Burge. and Carthamus tinctorius L. BMC Complement. Med. Ther. 20 (1), 351. doi:10.1186/s12906-020-03026-y
Wei, Y., Wu, Y., Feng, K., Zhao, Y., Tao, R., Xu, H., et al. (2020). Astragaloside IV inhibits cardiac fibrosis via mir-135a-TRPM7-TGF-β/smads pathway. J. Ethnopharmacol. 249, 112404. doi:10.1016/j.jep.2019.112404
Yang, H. Y., Liu, M. L., Luo, P., Yao, X. S., and Zhou, H. (2022). Network pharmacology provides a systematic approach to understanding the treatment of ischemic heart diseases with traditional Chinese medicine. Phytomedicine 104, 154268. doi:10.1016/j.phymed.2022.154268
Yang, J., Wang, B., Li, N., Zhou, Q., Zhou, W., and Zhan, Z. (2019). Salvia miltiorrhiza and Carthamus tinctorius extract prevents cardiac fibrosis and dysfunction after myocardial infarction by epigenetically inhibiting smad3 expression. Evid. Based Complement. Altern. Med. 2019, 6479136. doi:10.1155/2019/6479136
Yang, Y., Shao, M., Cheng, W., Yao, J., Ma, L., Wang, Y., et al. (2023). A pharmacological review of tanshinones, naturally occurring monomers from Salvia miltiorrhiza for the treatment of cardiovascular diseases. Oxid. Med. Cell Longev. 2023, 3801908. doi:10.1155/2023/3801908
Yoshimura, S., Imai, K., Hayashi, Y., and Hashimoto, K. (1989). Role of renin-angiotensin system in the pathogenesis of spontaneous myocardial fibrosis in sprague-dawley rats: Effect of long-term administration of captopril. Tohoku J. Exp. Med. 157 (3), 241–249. doi:10.1620/tjem.157.241
Yu, Y., Sun, J., Wang, R., Liu, J., Wang, P., and Wang, C. (2019). Curcumin management of myocardial fibrosis and its mechanisms of action: A review. Am. J. Chin. Med. 47 (8), 1675–1710. doi:10.1142/S0192415X19500861
Zhang, X., Qu, H., Yang, T., Liu, Q., and Zhou, H. (2022). Astragaloside IV attenuate mi-induced myocardial fibrosis and cardiac remodeling by inhibiting ros/caspase-1/gsdmd signaling pathway. Cell Cycle 21 (21), 2309–2322. doi:10.1080/15384101.2022.2093598
Zhang, Y., Zhang, L., Fan, X., Yang, W., Yu, B., Kou, J., et al. (2019). Captopril attenuates TAC-induced heart failure via inhibiting Wnt3a/β-catenin and Jak2/Stat3 pathways. Biomed. Pharmacother. 113, 108780. doi:10.1016/j.biopha.2019.108780
Zhang, Z., Chen, F., Wan, J., and Liu, X. (2023). Potential traditional Chinese medicines with anti-inflammation in the prevention of heart failure following myocardial infarction. Chin. Med. 18 (1), 28. doi:10.1186/s13020-023-00732-w
Zheng, W., Li, D., Gao, X., Zhang, W., and Robinson, B. O. (2019). Carvedilol alleviates diabetic cardiomyopathy in diabetic rats. Exp. Ther. Med. 17 (1), 479–487. doi:10.3892/etm.2018.6954
Zhu, J. N., Chen, R., Fu, Y. H., Lin, Q. X., Huang, S., Guo, L. L., et al. (2013). Smad3 inactivation and MiR-29b upregulation mediate the effect of carvedilol on attenuating the acute myocardium infarction-induced myocardial fibrosis in rat. PLoS One 8 (9), e75557. doi:10.1371/journal.pone.0075557
Keywords: myocardial fibrosis, modern medicine, traditional Chinese medicine, integrated traditional Chinese and modern medicine, mechanism
Citation: Ren Z, Zhang Z, Ling L, Liu X and Wang X (2023) Drugs for treating myocardial fibrosis. Front. Pharmacol. 14:1221881. doi: 10.3389/fphar.2023.1221881
Received: 13 May 2023; Accepted: 29 August 2023;
Published: 12 September 2023.
Edited by:
Prasanth Puthanveetil, Midwestern University, United StatesReviewed by:
Anis Hanna, Albert Einstein College of Medicine, United StatesSarojini Singh, University of Alabama at Birmingham, United States
Copyright © 2023 Ren, Zhang, Ling, Liu and Wang. This is an open-access article distributed under the terms of the Creative Commons Attribution License (CC BY). The use, distribution or reproduction in other forums is permitted, provided the original author(s) and the copyright owner(s) are credited and that the original publication in this journal is cited, in accordance with accepted academic practice. No use, distribution or reproduction is permitted which does not comply with these terms.
*Correspondence: Xin Wang, d2FuZ3hpbjYzMTIyNEAxNjMuY29t
†These authors have contributed equally to this work