- 1Centre for Quality of Health and Living, Faculty of Health and Environmental Sciences, Central University of Technology, Bloemfontein, South Africa
- 2Department of Paraclinical Sciences, Faculty of Veterinary Science, University of Pretoria, Pretoria, South Africa
- 3Department of Biochemistry, Faculty of Science, University of Yaoundé I, Yaoundé, Cameroon
- 4University Institute of Technology of Wood Technology, Mbalmayo, Cameroon
- 5Department of Biochemistry, Federal University Oye-Ekiti, Oye, Nigeria
- 6Department of Organic Chemistry, Faculty of Science, University of Yaoundé I, Yaound, Cameroon
Background: Sarcocephalus pobeguinii (Hua ex Pobég) is used in folk medicine to treat oxidative-stress related diseases, thereby warranting the investigation of its anticancer and anti-inflammatory properties. In our previous study, the leaf extract of S. pobeguinii induced significant cytotoxic effect against several cancerous cells with high selectivity indexes towards non-cancerous cells.
Aim: The current study aims to isolate natural compounds from S. pobeguinii, and to evaluate their cytotoxicity, selectivity and anti-inflammatory effects as well as searching for potential target proteins of bioactive compounds.
Methods: Natural compounds were isolated from leaf, fruit and bark extracts of S. pobeguinii and their chemical structures were elucidated using appropriate spectroscopic methods. The antiproliferative effect of isolated compounds was determined on four human cancerous cells (MCF-7, HepG2, Caco-2 and A549 cells) and non-cancerous Vero cells. Additionally, the anti-inflammatory activity of these compounds was determined by evaluating the nitric oxide (NO) production inhibitory potential and the 15-lipoxygenase (15-LOX) inhibitory activity. Furthermore, molecular docking studies were carried out on six putative target proteins found in common signaling pathways of inflammation and cancer.
Results: Hederagenin (2), quinovic acid 3-O-[α-D-quinovopyranoside] (6) and quinovic acid 3-O-[β-D-quinovopyranoside] (9) exhibited significant cytotoxic effect against all cancerous cells, and they induced apoptosis in MCF-7 cells by increasing caspase-3/-7 activity. (6) showed the highest efficacy against all cancerous cells with poor selectivity (except for A549 cells) towards non-cancerous Vero cells; while (2) showed the highest selectivity warranting its potential safety as a chemotherapeutic agent. Moreover, (6) and (9) significantly inhibited NO production in LPS-stimulated RAW 264.7 cells which could mainly be attributed to their high cytotoxic effect. Besides, the mixture nauclealatifoline G and naucleofficine D (1), hederagenin (2) and chletric acid (3) were active against 15-LOX as compared to quercetin. Docking results showed that JAK2 and COX-2, with the highest binding scores, are the potential molecular targets involved in the antiproliferative and anti-inflammatory effects of bioactive compounds.
Conclusion: Overall, hederagenin (2), which selectively killed cancer cells with additional anti-inflammatory effect, is the most prominent lead compound which may be further investigated as a drug candidate to tackle cancer progression.
1 Introduction
Cancer is one of the leading causes of death worldwide, accounting 19.3 million new cancer cases and about 10 million deaths in 2020 (Sung et al., 2021). This chronic disease is characterized by abnormal and uncontrolled proliferation of cancer cells which later invades normal tissues and organs, and eventually spreading throughout the body. Several reports have proven the cross-talk between inflammation and cancer due to the fact that common signaling pathways are modulated in both deleterious ailments (Hibino et al., 2021; Zhao et al., 2021). Regardless to the cancer type, inflammation conditions have been considered as one of the key factors that promotes all stages of tumorigenesis. Inflammation also helps the survival of malignant cells, therefore impeding the immune surveillance or altering the efficacy of chemotherapeutic agents (Mantovani et al., 2008; Peczek et al., 2022). As one of the most important cancer treatment strategies, chemotherapy uses powerful drugs to kill fast-growing cells, but it induces substantial side effects due to the fact that normal cells are also affected (Amjad et al., 2022). To date, great progress has been made for the discovery and development of effective and safe chemotherapeutic agents, however the number of cancer-related deaths increases every day. This situation urgently requires the search of alternative treatments to improve the quality of life of patients. Natural therapies including medicinal plants constitute a reservoir for the discovery of new anticancer lead compounds. Additionally, as above-mentioned, chronic inflammation accelerates cancer progression, and it has been proven that some anti-inflammatory drugs such as aspirin, celecoxib, diclofenac, etc. are being used against cancer (Rayburn et al., 2009; Zappavigna et al., 2020; Lai et al., 2022). As such, controlling inflammation may represent a valid strategy for cancer prevention and therapy. Thus, developing anticancer agents with additional anti-inflammatory effect is the best approach to tackle cancer progression.
Cameroon has a rich biodiversity, with about 8,620 plants species which are used by local population for the treatment of several ailments (Kuete and Efferth, 2010; Ntie-Kang et al., 2013). Among these plants, Sarcocephalus pobeguinii (Hua ex Pobég) (synonym of Nauclea pobeguinii (Hua ex Pobég) Merr.) is used as infusion or decoction in the treatment of fever, malaria, stomach-ache, sexual and reproductive dysfunctions, epilepsy, diabetes mellitus, hypertension, infectious diseases and jaundice (Mesia et al., 2005; Jiofack et al., 2009; Karou et al., 2011; Kuete et al., 2015; Haudecoeur et al., 2018). In a study investigating the cytotoxicity of selected Cameroonian medicinal plants, the bark extract of S. pobeguinii and its isolated compounds (resveratrol and a glucoside derivative) exhibited antiproliferative potential against multi-factorial drug-resistant cancer cell lines (Kuete et al., 2015). In our previous study, the antiproliferative effect of different plant parts (leaf, fruit, bark and root) extracts of S. pobeguinii were evaluated on several human cancer cell lines, and leaf extract induced significant cytotoxic effect with high selectivity indexes (Mfotie Njoya et al., 2017). So far, phytochemical investigations carried out on bark and root extracts of S. pobeguinii have resulted in the isolation and characterization of compounds such as strictosamide, 5-carboxystrictosidine, methylangustoline, 3-O-β-D-fucosyl-quinovic-acid, 3-keto-quinovic-acid; 19-O-methylangustoline, 3-acetoxy-11-oxo-urs-12-ene, p-coumaric acid, citric acid trimethyl ester, resveratrol, resveratrol β-D-glucopyranoside (Karou et al., 2011; Kuete et al., 2015; Yüce et al., 2019). Despite the use of leaves and fruits of S. pobeguinii in traditional medicine, the isolation of compounds from these plant parts as well as their biological effects have not yet been investigated to the best of our knowledge. Moreover, the use of leaves and fruits for medicinal purposes instead of roots and barks is advantageous for the conservation and sustainable maintenance of medicinal plants. Therefore, the current work aims to find bioactive compounds from leaf and fruit extracts of S. pobeguinii and to evaluate their efficacy, selectivity and anti-inflammatory effects. In addition, this study has been also extended to the isolation of active constituents from roots of S. pobeguinii which might also yield to the discovery of new anticancer lead compounds.
2 Materials and methods
2.1 Plant material
Different parts (leaves, fruits, and barks) of S. pobeguinii were harvested in Ezezan (Nyom II), a neighbouring locality situated at 40 km from Yaoundé (Cameroon). A voucher specimen was prepared and the authentication was done by Mr. Ngansop Eric, a plant taxonomist, after comparison with the specimen number N°32,567 BRF/CAM already available in the library of the National Herbarium of Cameroon. Our plant material was then registered under the number Letouzey R.12493 (YA).
2.2 Preparation of extracts and isolation of compounds
The powder (907 g) obtained from the air dried and grounded fruits of S. pobeguinii was macerated in the mixture of methanol and methylene chloride (1:1) at room temperature for 48 h with occasional stirring, and the crude extract was obtained after filtration with Whatman N⁰1, followed by evaporation of the solvent to dryness in vacuo. The dried extract (76.5 g) was re-dissolved in MeOH and partitioned with ethyl acetate (EtOAc) to yield the EtOAc fraction (10.2 g) and the MeOH residue (54.7 g). The MeOH soluble fraction was then subjected to silica gel column chromatography (CC) eluted with a gradient mixture of n-hexane-EtOAc (9:1; 7:3; 6:4; 0:1). The collected sub-fractions were pooled based on their thin layer chromatography (TLC) profiles and yielded six sub-fractions (F1-F6) after evaporation. Sub-fraction F3 (8 g) was submitted to silica gel CC, and eluted with the mixture of n-hexane-EtOAc (2:1) to yield the following compounds: (1) (6 mg) identified as a mixture of nauclealatifoline G and naucleofficine D (Agomuoh et al., 2013); (2) (7 mg) known as hederagenin (Joshi et al., 1999); and (3) (6 mg) characterized as chletric acid (Takahashi and Takani, 1978).
The powder of air-dried leaves of S. pobeguinii (1 kg) was extracted in MeOH and heated at 40°C–50°C for 2 h. The methanolic extract was concentrated in vacuo to yield a dark greenish mass (66 g) which was partitioned with n-hexane. The n-hexane soluble part and the methanolic residue were evaporated under pressure to obtain respectively a black residue (26.5 g) and a greenish brown extract (37.3 g). The latter was subjected to silica gel flash chromatography, and eluted respectively with CH2Cl2; CH2Cl2/MeOH (1:1) and MeOH. The n-hexane soluble part and the CH2Cl2 fraction were mixed due to their similar TLC profiles, and this mixed fraction was submitted to silica gel CC eluted with the gradient mixture of n-hexane and EtOAc (9:1; 3:1; 3:2; 1:1; 1:3) to afford compound (4) (5 mg) identified as taraxerol (Yen et al., 2013) collected in n-hexane/EtOAc (3:1); compound (5) (4 mg) known as α-amyrin (3β-hydroxy-urs-12-en-3-ol) (Viet et al., 2021) collected in n-hexane/EtOAc (1:1); and (6) (6 mg) known as quinovic acid 3-O-[α-D-quinovopyranoside] (Mohamed, 1999) collected in n-hexane/EtOAc (1:3).
The powder of dried bark of S. pobeguinii (1 kg) was extracted in MeOH and heated at 40°C–50°C for 2 h. The crude extract was obtained after filtration with Whatman N⁰1, followed by evaporation under reduced pressure to obtain a yellow gummy residue (77 g). This methanolic extract was partitioned with n-hexane to yield the n-hexane fraction (15 g) and a residual MeOH fraction (54 g). The MeOH fraction was then subjected to silica gel CC, and eluted with a gradient of n-hexane/EtOAc yielding the following compounds: (7) (4 mg) identified as erythrodiol and (8) (8 mg) known as quinovic acid (Fatima et al., 2002; Aktar et al., 2009) both collected in the n-hexane/EtOAc (9:1); then compound (9) (8 mg) characterized as quinovic acid 3-O-[β-D-quinovopyranoside] (Yepez et al., 1991) collected in n-hexane/EtOAc (3:1); and finally compound (10) (4 mg) identified as latifoliamide C (Agomuoh et al., 2013) collected in n-hexane/EtOAc (1:9).
2.3 Characterization and structural elucidation of isolated compounds
The chemical constituents of S. pobeguinii were purified using open silica gel column chromatography (Merck, [Darmstadt, Germany]). TLC was done on Alu g R; SIL G/UV254 silica gel plates (Merck, [Darmstadt, Germany]), and visualization of the spots on TLC plates was achieved either by exposure to iodine vapour, UV light or by spraying sulphuric acid and heating the plate at 75°C. Melting points were recorded on a Buchi B-54 apparatus. 1H and 13C NMR spectra as well as 2D NMR experiments (see Supplementary Material, Supplementary Figures S1–S22) were recorded in CDCl3, MeOH-d4, DMSO-d6 and pyridine-d5 in a JEOL ECX 500 spectrometer (Akishima, Japan) and on a Bruker ARX 400. Chemical shifts were expressed in part per million δ) relative to tetramethylsilane as internal standard. The spectroscopy data recorded were compared with data from literature, for the characterization of the chemical structures of isolated compounds which are presented in Figure 1.
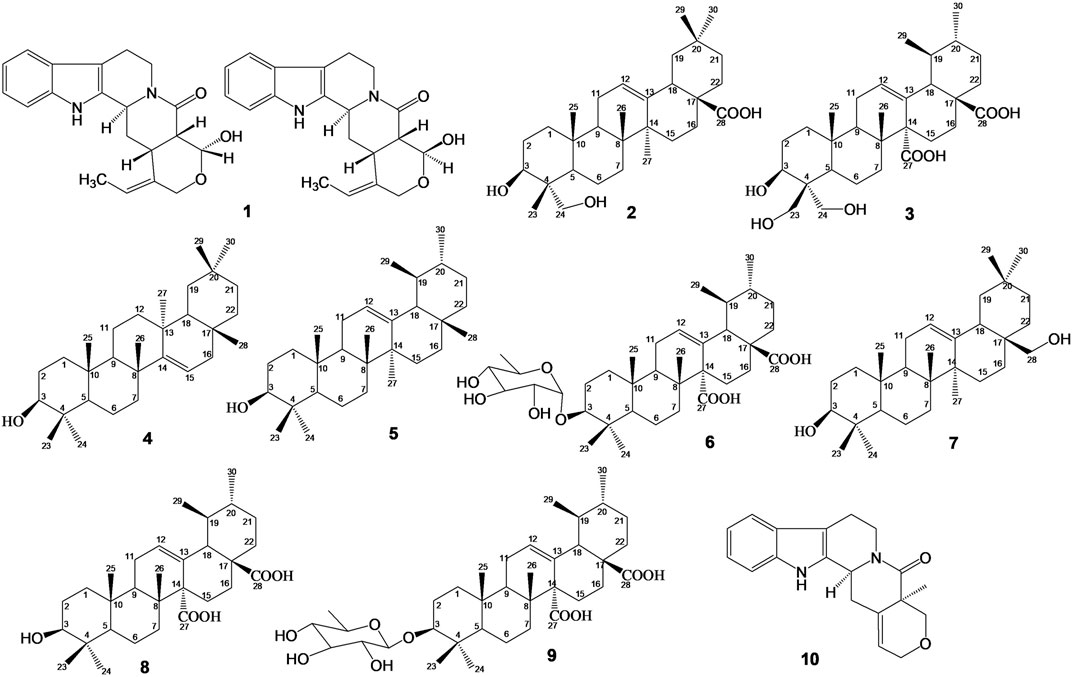
FIGURE 1. Chemical structures of isolated compounds: Mixture of Nauclealatifoline G (C20H22N2O3, 338.40 g/mol) and naucleofficine D (C20H22N2O3, 338.40 g/mol) (1), hederagenin (C30H48O4, 472.73 g/mol) (2) and chletric acid (C30H46O8, 534.69 g/mol) (3) were isolated from fruit extract of S. pobeguinii. Taraxerol (C30H50O, 426.73 g/mol) (4), α-amyrin (3β-hydroxy-urs-12-en-3-ol) (C30H50O, 426.73 g/mol) (5) and quinovic acid 3-O-[α-D-quinovopyranoside] (C36H56O9, 632.84 g/mol) (6) were isolated from leaf extract of S. pobeguinii. Erythrodiol (C30H50O2, 442.73 g/mol) (7), quinovic acid (C30H46O5, 486.69 g/mol) (8), quinovic acid 3-O-[β-D-quinovopyranoside] (C36H56O9, 632.84 g/mol) (9) and latifoliamide C (C19H20N2O2, 308.38 g/mol) (10) were isolated from bark extract of S. pobeguinii.
2.4 Cell culture
Cancerous cell lines (MCF-7: human breast adenocarcinoma cells; HepG2: human hepatocellular carcinoma cells; Caco-2: human epithelial colorectal adenocarcinoma cells; A549: human epithelial lung adenocarcinoma cells), obtained from the American Type Culture Collection (ATCC) (Rockville, MD, United States of America), were cultured in Dulbecco’s Modified Eagle’s Medium (DMEM) high glucose (4.5 g/L) containing L-glutamine (4 mM) and sodium-pyruvate (Hyclone™) supplemented with 10% (v/v) fetal bovine serum (FBS) (Capricorn Scientific GmbH, South America), and incubated at 37°C with 5% CO2 in a humidified environment. African green monkey (Vero) kidney cells (also obtained from ATCC), a non-cancerous cell line, were grown in DMEM high glucose (4.5 g/L) containing L-glutamine (Lonza, Belgium) and supplemented with 5% FBS (Capricorn Scientific GmbH, South America) and 1% gentamicin (Virbac, RSA) in the same environment as cancer cells. The RAW 264.7 murine macrophage cells (obtained from ATCC) were cultured in DMEM high glucose (4.5 g/L) containing L-glutamine (Lonza, Belgium), supplemented with 10% FBS (Capricorn Scientific GmbH, South America) and 1% penicillin/streptomycin/fungizone (PSF) solution, and kept at 37°C in a 5% CO2 humidified environment.
2.5 Antiproliferation assay
The cancerous and non-cancerous cells were seeded at a density of 104 cells per well in 96-well microtiter plates, and they were incubated overnight at 37°C with 5% CO2 in a 5% CO2 humidified environment in order to allow the attachment of cells at the bottom of the plates. Then, the cells were exposed to increasing concentrations of compounds (100, 50, 25, 10 and 5 μg/mL) dissolved in dimethyl sulfoxide (DMSO) and further diluted in fresh culture medium. In this assay, the final concentration of DMSO in the culture medium was 0.5% used as negative control while doxorubicin hydrochloride (Pfizer, United States of America) was used as a positive control. The culture plates were incubated for 48 h at 37°C in a 5% CO2 humidified environment, after which the culture medium was discarded, and replaced by 200 µL of fresh culture medium with 30 µL of 3-(4,5-dimethylthiazol-2-yl)-2,5-diphenyl-2H-tetrazolium bromide (MTT) (5 mg/mL) dissolved in phosphate buffered saline (Mosmann, 1983). After 4 h of incubation, the medium was gently removed, and the formazan crystals were solubilized in 50 µL of DMSO. The absorbance was measured at 570 nm after shaking for 1 min on a microplate reader (Synergy Multi-Mode Reader, BioTek, Winooski, United States of America).
The cell viability rate was determined at each concentration of the compound as a percentage of cells treated with DMSO at 0.5% used as negative control. The 50% inhibitory concentrations (IC50) were determined by using the non-linear regression graphical analysis of cell viability rate against the logarithm (log10) of compound concentrations with the software GraphPad Prism 6.0 (GraphPad software Inc., United States of America). The selectivity index (Supporting Material) values were calculated for each compound by dividing the IC50 of non-cancerous cells by the IC50 of cancerous cells in the same units (Mfotie Njoya et al., 2018; Mfotie Njoya et al., 2020).
2.6 Caspase-3/-7 luminescence assay
The effect of the most bioactive compounds (2), (6) and (9) were used for the analysis of caspase-3 and caspase-7 activities on MCF-7 cells by using the Caspase-Glo® 3/7 kit (Promega, Germany). In fact, MCF-7 cells were seeded at a density of 104 cells per well on 96-well microtiter plates, and the plates were incubated overnight at 37 °C in a 5% CO2 humidified environment. Then, the cells were exposed to the active compounds at different concentrations (½×IC50, IC50 and 2×IC50) or DMSO (0.5%) used as negative control, and further incubated for 18 h at 37°C in a 5% CO2 humidified environment. Thereafter, 100 µL of Caspase-Glo® 3/7 reagent was added to each well, mixed and incubated in the dark for 1 h at room temperature. The luminescence was then measured on a microplate reader (Synergy Multi-Mode Reader, BioTek, Winooski, United States of America). The caspase-3/-7 activity was expressed as fold change of cells treated with 0.5% DMSO (control).
2.7 Anti-inflammatory assays
2.7.1 Nitric oxide production inhibitory assay
Nitric oxide (NO) production was evaluated in lipopolysaccharide (LPS)-stimulated RAW 264.7 cells by measuring the influence of tested compounds on the accumulation of nitrite, an indicator of NO in the cell supernatant, which can be detected with Griess reagent (Merck, Darmstadt, Germany). Briefly, the RAW 264.7 cells at their exponential growth phase were seeded at a density of 2×104 cells per well in 96 well-microtiter plates, and they were incubated overnight at 37°C in a 5% CO2 humidified environment to allow attachment. The cells were pre-treated with tested compounds (100 μg/mL) or DMSO 0.5% (negative control) and incubated for 1 h at 37°C in a 5% CO2 humidified environment. Then, culture medium containing LPS (2 μg/mL) was added to each well and further incubated for 24 h at 37°C in a 5% CO2 humidified environment. Thereafter, 100 μL of cell supernatant from each well were transferred into a new 96-well microtiter plate and an equal volume of Griess reagent was added according to protocol described by the manufacturer. The microtiter plate was incubated for 10 min in the dark at room temperature, and the absorbance of the mixture was measured at 550 nm on a microplate reader (Synergy Multi-Mode Reader, BioTek, Winooski, United States of America). The quantity of nitrite was determined from a sodium nitrite standard curve, and the percentage of NO production was calculated based on the ability of each tested sample to inhibit nitric oxide production by LPS-stimulated RAW 264.7 cells compared to the control (cells treated with LPS without samples which was considered as 100% NO production). Additionally, the cell viability of treated cells was determined by using the MTT assay as previously described (Mosmann, 1983). The whole experiment was repeated at different concentrations (100, 50, 25, 10 and 5 μg/mL) only for tested compounds that were able to inhibit at 50% of NO production, and the IC50 values was calculated as previously described (Mfotie Njoya et al., 2023).
2.7.2 Soybean 15-LOX inhibitory assay
This assay is based on the formation of the Fe3+/xylenol orange (FOX) complex with maximal absorption at 560 nm (Pinto et al., 2007). Forty microliters of 15-LOX (final concentration: 200 UI/mL) from Glycine max (Merck, Darmstadt, Germany) was incubated with 20 µL of tested samples (100, 50, 25, 10, and 5 μg/mL) at 25°C for 5 min, with DMSO at 10% (v/v) being used as negative control. Thereafter, 40 µL of linoleic acid (final concentration, 140 μM) prepared in Tris-HCl buffer (50 mM, pH 7.4) was added, and the plates were further incubated at 25°C for 20 min in the dark. The assay was terminated by adding 100 μL of FOX reagent [sulfuric acid (30 mM), xylenol orange (100 μM), ferrous II) sulfate (100 μM), dissolved in methanol/water (9:1)], followed by the incubation of the plates at 25°C for 30 min in the dark. Finally, the absorbance was measured at 560 nm on a microplate reader (Epoch, BioTek, Winooski, United States of America). The blanks were made in the same way as tested samples except that the substrate was added after the FOX reagent. The 15-LOX inhibitory activity was calculated by using the following formula:
Abs: absorbance.
2.8 Statistical analysis
All experiments were performed in triplicate, and the results are presented as mean ± standard deviation (SD) values. The statistical analysis was done with the software GraphPad Prism 6.0 (GraphPad software Inc., United States of America) on which one-way analysis of variance (ANOVA) and Student–Newman–Keuls or Dunnett’s tests were used for the comparison of data among tested samples and/or controls. Results were considered significantly different when the p-value was greater than 0.05.
2.9 Molecular docking study
The X-ray crystal structures of putative target proteins in complex with inhibitors were retrieved from Protein Data Bank (https://www.rcsb.org/, accessed on 14 March 2023): Cyclooxygenase-2: COX-2 (PDB ID: 5KIR); Nuclear factor NF-kappa-B (p65 subunit): NFKB-p65 (PDB ID: 2RAM); Janus kinase 2: JAK2 (PDB ID: 6BBV); Signal transducer and activator of transcription 3: STAT3 (PDB ID: 6TLC); CASPASE-3 (PDB ID: 3DEI), CASPASE-7 (PDB ID: 1SHJ). PyMol v 2.0.7, was used to prepare the proteins for simulation by removing the inhibitors, water molecules, multi-chains and heteroatoms. MarvinSketch was used to draw the chemical structures of the ligands, and the Merck Molecular Force Field (MMFF94) as provided by MarvinSketch was used for energy minimization on the ligands (compounds) (Novichikhina et al., 2020). The energy minimization was carried out to make the ligands more stable near their initial states during molecular docking process. AutoDock Vina v 1.5.6 was used to add hydrogen atoms and Gasteiger charges accordingly to the ligands prior to molecular docking (Trott and Olson, 2010). The active sites of the target proteins were identified using CASTp 2.0 web-based tool (Tian et al., 2018). Further, AutoDock Vina v 1.5.6 was used to create a grid box around the identified active sites of the target proteins. Thereafter, standard precision ligand docking was performed using the same software. The best of nine binding poses based on the docking scores were visualized and captured in BIOVIA Discovery Studio Visualizer 2021 (Mfotie Njoya et al., 2023).
3 Results and discussion
3.1 Cytotoxic effect of isolated compounds and selectivity towards non-cancerous cells
The growth inhibitory effect of natural compounds isolated from leaf, fruits and bark of Sarcocephalus pobeguinii (Rubiaceae) against four cancerous cells (MCF-7, HepG2, Caco-2 and A549) and non-cancerous Vero cells was expressed as percentage of respective cells treated with DMSO (0.5%) used as negative controls. Based on the results obtained for each compound tested at different concentrations, the 50% inhibitory concentrations (IC50) were determined and presented in Table 1. It was observed that hederagenin (2), quinovic acid 3-O-[α-D-quinovopyranoside] (6) and quinovic acid 3-O-[β-D-quinovopyranoside] (9) exhibited significant (p < 0.05) antiproliferative effect against all cancerous cells, especially for (6) which showed the highest cytotoxic effect on A549 cells with IC50 of 1.96 μg/mL (3.08 µM). Additionally, (6) was also toxic to the non-cancerous Vero cells with IC50 of 6.21 μg/mL (9.81 µM). By calculating the selectivity indexes (SI) as presented in Table 2, we found that (6), isolated from leaves of S. pobeguinii, had a poor selectivity (SI ≤ 1) (except for A549 cells) towards non-cancerous Vero cells where SI was 3.17. Similarly, (9), isolated from bark of S. pobeguinii, also had poor selectivity (SI ≤ 1) for all cancer types. On the other hand, (2), isolated from fruits of S. pobeguinii, was the only compound which had acceptable selectivity (1.24 ≤ SI ≤ 5.11) for all cancer cells towards non-cancerous Vero cells thereby suggesting that this compound can selectively kill cancer cells at the IC50 without causing harmful effects on non-cancerous cells. Therefore, regarding the fact that hederagenin (2) exhibited good selectivity, this compound was tested at 10 μg/mL on all cancer cells and non-cancerous cells for 12, 24 and 48 h in order to evaluate the time-dependent cytotoxic effect on cell growth. It was observed that (2) induced the growth inhibition of different cancer cells in a time-dependent manner, and the cytotoxic effect was more pronounced on cancer cells compared to non-cancerous cells (Figure 2). In fact, the MCF-7 cells followed by A549 cells were the most sensitive to the cytotoxic effect of hederagenin (2) which was less toxic to non-cancerous Vero cells. Interestingly, hederagenin, isolated from several plants, has been previously reported to potentially inhibit the proliferation of human lung cancer cells A549 (IC50 values of 26.3 and 39 μM) (Gauthier et al., 2009; Gao et al., 2016). In the current study, hederagenin inhibits A549 growth with an IC50 of 40.11 µM which is comparable to results obtained from previous works. Additionally, hederagenin was found to be less cytotoxic to human normal skin fibroblasts (WS1) cell lines (IC50 of 77 μM) (Gauthier et al., 2009) which also confirms the fact that this compound selectively kills cancer cells with least toxic effect to normal cells. Further, hederagenin, extracted in high quantities from the fruits of Sapindus saponaria L., (Sapindaceae), has been reported to be cytotoxic to different cancer cells, and structural modifications resulted to the development of potent anti-tumour compounds (Rodriguez-Hernandez et al., 2015; Rodriguez-Hernandez et al., 2016). Overall, hederagenin was isolated for the first time from fruits of S. pobeguinii (Rubiaceae), and our data corroborates its use as a potential candidate for the development of anticancer agents.
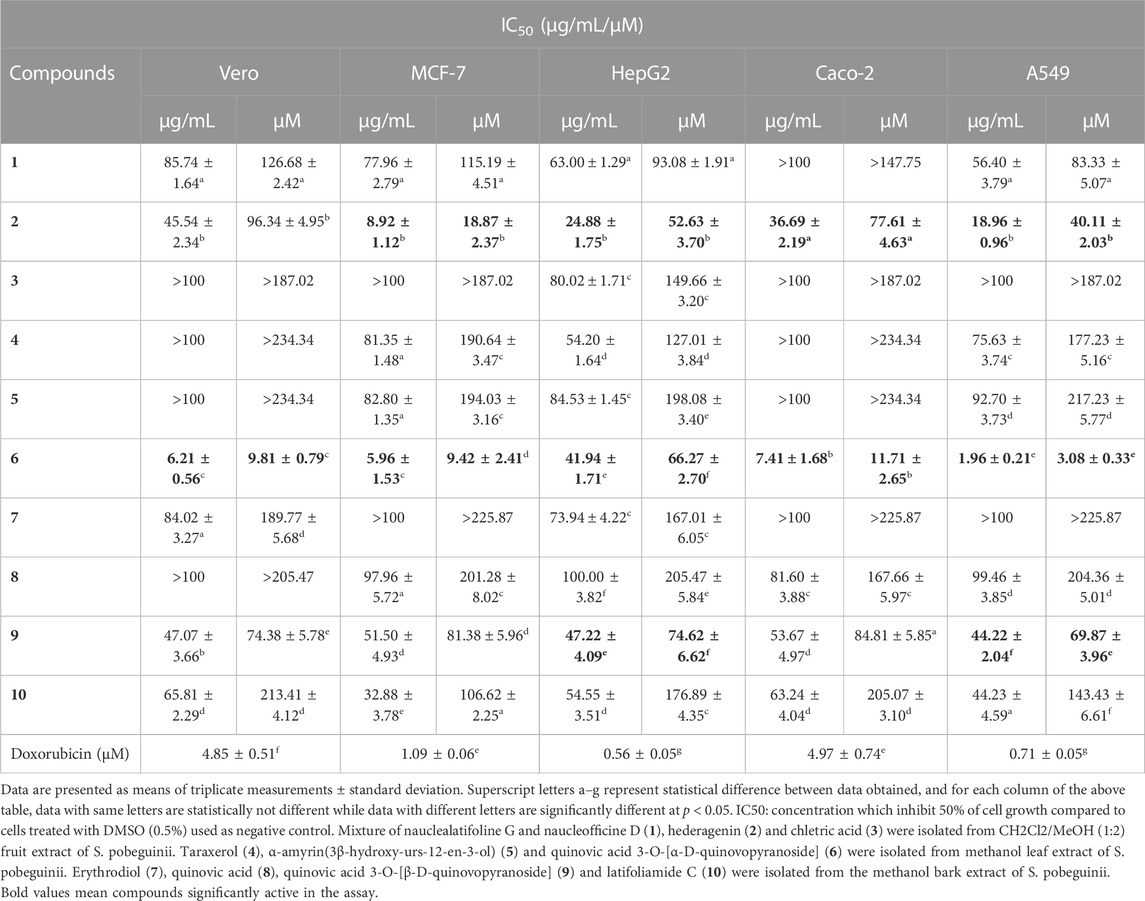
TABLE 1. Cytotoxic effects (IC50 values) of natural compounds isolated from roots, fruits, bark and leaves of Sarcocephalus pobeguinii and reference drug (doxorubicin) against cancer cell lines and non-cancerous Vero cells.
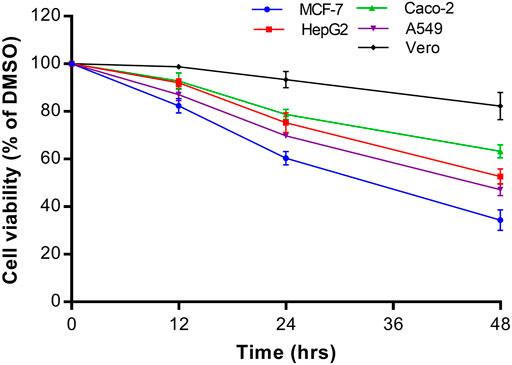
FIGURE 2. Time-dependent cytotoxic effect of hederagenin (2) at 10 μg/mL on different cancer cells (MCF-7, HepG2, Caco-2 and A549) and non-cancerous Vero cells. Data are presented as mean ± standard deviation of three independent experiments.
3.2 Structure–activity relationship
Three triterpenoids including two saponins isolated from S. pobeguinii were cytotoxic to cancer cells, and their efficacy varied according to their structural configuration or the presence of some functional groups. When compared to other triterpenoids, the cytotoxic effect of the two bioactive saponins, namely, quinovic acid 3-O-[α-D-quinovopyranoside] (6) and quinovic acid 3-O-[β-D-quinovopyranoside] (9) can be attributed to the presence of a pyranose moiety attached at position C-3. Moreover, the α anomer (6) is highly cytotoxic compared to the β anomer (9) which suggests that the configuration of the glycosidic bond (C-O-sugar bond) has an impact on the cytotoxic effect. According to several reports, the anticancer activity of triterpenoid saponins is strongly linked to the presence of functional carboxylic and hydroxyl groups on the aglycone chain, the stereo-selectivity and the type of sugar molecule attached (Nag et al., 2012; Xu et al., 2013; Elekofehinti et al., 2021; Podolak et al., 2023). In contrast, we observed from our study that despite the presence carboxylic and hydroxyl groups, other triterpenoids (3), (4), (5), (7) and (8) were less cytotoxic or inactive compared to hederagenin (2) which strongly inhibit the growth of several cancer cells. We therefore suggest that the efficacy of hederagenin might be attributed to the asymmetric carbon at position C-4 which yield a different conformation due to irregular spatial arrangements of chemical groups. This conformation might ease the interaction with molecular targets involved in the activation of cancer cell death pathways. Further, the solubility of hederagenin in culture media may also be responsible for its efficacy on cancer cells.
3.3 Caspase-dependent activation by bioactive compounds
The potential mechanism of action of bioactive compounds on cellular viability was explored by quantifying the caspase-3/-7 activity in MCF-7 cells. It was found that the caspase-3/-7 activity was significantly (p < 0.05) induced in a concentration-dependent manner compared to control cells treated with DMSO 0.5% (Figure 3). Additionally, the optimal effect was observed with hederagenin (2) and quinovic acid 3-O-[α-D-quinovopyranoside] (6) tested at 2×IC50 where an induction up to 2-fold change in the activation of caspase-3/-7 activity was recorded. Caspases 3 and 7 are known as “executioners” of apoptosis, and the combined role of both caspases is crucial for the activation of apoptotic pathways (Olsson and Zhivotovsky, 2011). In fact, caspase 3 controls DNA fragmentation and morphologic changes of apoptosis, while caspase 7 is more important for the loss of cellular viability (Lakhani et al., 2006). Therefore, the induction of caspase-3/-7 activity after treatment with bioactive compounds implied that the activation of apoptotic pathways is involved in the mechanism of induced cell death in MCF-7 cells.
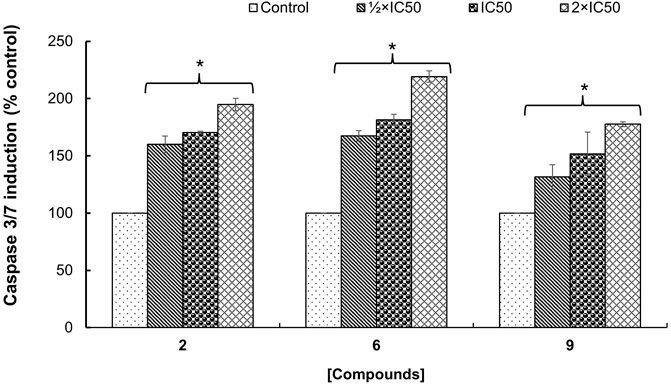
FIGURE 3. Fold change in the enzymatic activity of caspase-3/-7 expressed as percentage of control (cells treated with DMSO 0.5%) on MCF-7 cells treated with hederagenin (2), quinovic acid 3-O-[α-D-quinovopyranoside] (6) and quinovic acid 3-O-[β-D-quinovopyranoside] (9), respectively. Data are presented as mean ± SD of three independent experiments. *p < 0.05 indicate the significant difference compared to the control using the Dunnett’s test.
3.4 Anti-inflammatory effect of isolated compounds
Cancer development is known as a multistep process during which inflammation is one of the factors contributing to the promotion of cell proliferation, invasion, angiogenesis, and metastasis. Moreover, it has been proven that patients on Nonsteroidal anti-inflammatory drugs (NSAIDs) are at reduced risk of cancer development (Singh et al., 2019). One of the possible reasons is that anti-inflammatory drugs might also interfere with signaling pathways modulated in both inflammation and cancer. Therefore, we decided to investigate the anti-inflammatory effect of compounds isolated from S. pobeguinii on two experimental models. We found that all compounds (tested at 100 μg/mL) inhibited the NO production in LPS-stimulated RAW 264.7 cells, with the mixture nauclealatifoline G and naucleofficine D (1), hederagenin (2), quinovic acid 3-O-[α-D-quinovopyranoside] (6) and quinovic acid 3-O-[β-D-quinovopyranoside] (9) being the most active by reducing at least 50% of NO production (Figure 4A). However, the NO production inhibitory effect of compounds (1), (2), (6) and (9) might be attributed to their cytotoxic effect on LPS-stimulated RAW 264.7 cells as it was observed that these compounds significantly (p < 0.05) reduced the cell viability (Figure 4B). Besides, by determining the IC50 values as presented in Table 3, we found that (6) and (9) strongly inhibits NO production in a concentration-dependent manner with IC50 values of 28.71 and 77.36 µM, respectively. On the other hand, we examined the inhibitory effect of isolated compounds on the activity of 15-LOX, an enzyme which regulate the inflammatory responses via the generation of pro-inflammatory mediators known as leukotrienes. It resulted that the mixture of nauclealatifoline G and naucleofficine D (1), hederagenin (2) and chletric acid (3) strongly inhibited the activity of 15-LOX with IC50 values of 16.51, 28.20 and 41.17 µM, respectively (see Table 3). These compounds were even more active than quercetin, which had an IC50 value of 61.94 µM. Based on the fact that 15-LOX and NO are among the mediators involved in the progression of various inflammation-related diseases including cancer (Zhao et al., 2021), our study therefore identified compounds which can be considered as potential anticancer agents with additional anti-inflammatory effect for the effective management of both diseases.
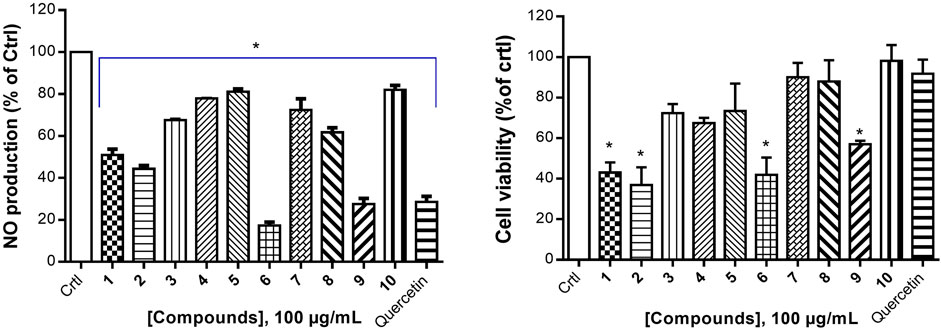
FIGURE 4. Nitric oxide production inhibition by natural compounds in LPS-stimulated RAW 264.7 cells (A) and their respective percentage of cell viability (B). The NO production inhibition was determined based on the efficacy of tested samples to reduce NO release by LPS- stimulated RAW 264.7 cells compared with the negative control (RAW 264.7 cells treated with LPS and DMSO at 0.5% without test samples), which was considered to be 100% of NO production. Quercetin was used as a positive control. Data represent the mean ± standard deviation of three independent experiments; (*) means a statistical difference (p < 0.05) between the tested samples versus the negative control (Crtl).
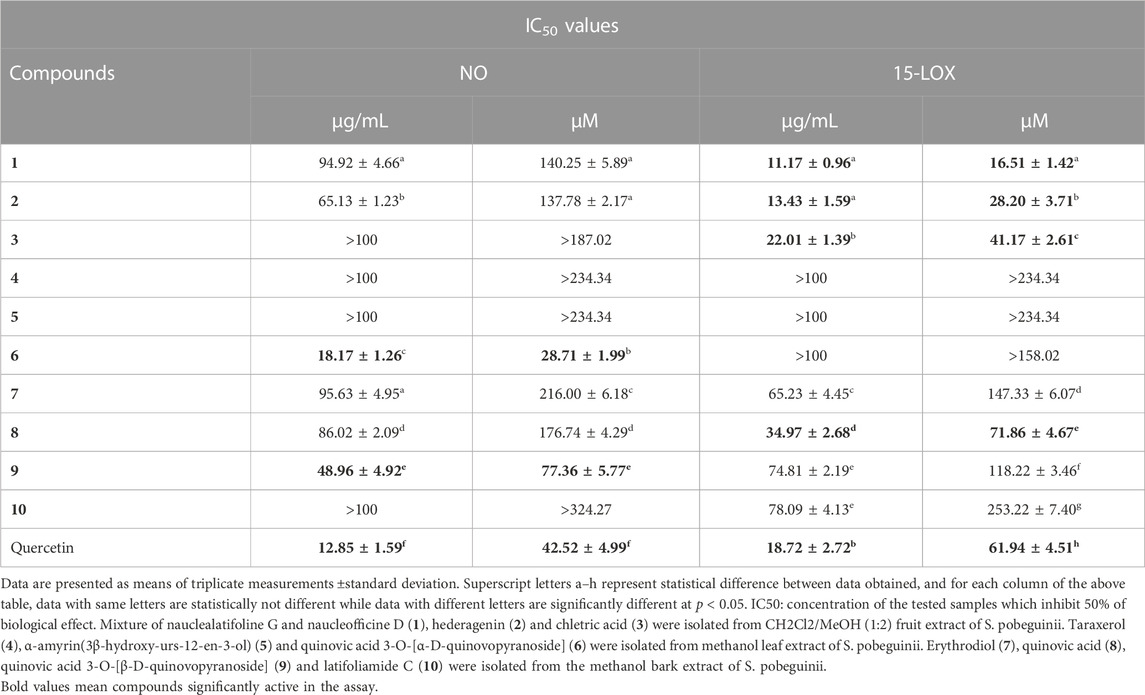
TABLE 3. Anti-inflammatory effect (IC50 values) of natural compounds isolated from fruits, leaves and bark of Sarcocephalus pobeguinii.
3.5 Binding efficacy of bioactive compounds against putative molecular targets
Six putative proteins, namely, COX-2, NFκB-p65, JAK2, STAT3, CASPASE-3 and CASPASE-7 were chosen for molecular docking based on the fact that they are frequently used as therapeutic targets to regulate inflammatory signaling pathways in cancer treatment (Jha et al., 2022). As presented in Table 4, the docking score (kcal/mol) of the best binding pose was determined for each ligand against the selected proteins. According to these results, hederagenin (2), quinovic acid 3-O-[α-D-quinovopyranoside] (6) and quinovic acid 3-O-[β-D-quinovopyranoside] (9) exhibited activity within the range of −4.3 to −6.8 kcal/mol, where −6.8 kcal/mol was the most effective interaction between JAK2 and (9), and −4.3 kcal/mol was the lowest binding score between CASPASE-3 and (2). Taken individually, each of the three bioactive compounds exhibited the greatest binding efficacy (binding score above −6 kcal/mol) against JAK2 and COX-2 which suggests that these proteins are the potential molecular targets involved in their antiproliferative and anti-inflammatory effects (Figure 5). In addition, STAT3 and NFKB-p65 also interacted well with bioactive compounds. According to the literature reports, the high activation of JAK2/STAT3 signaling pathway, frequently detected in various tumors, has recently emerged as a new site for the development of novel anti-tumor agents, and these proteins (JAK2 and STAT3) are promising therapeutic targets for the treatment of many solid tumors (Huang et al., 2022; Mengie Ayele et al., 2022). Moreover, NFκB-p65 is constitutively activated in many human cancers, where it contributes to almost all steps of tumorigenesis including sustained proliferation, cell death resistance, tumor-promoting inflammation, tissue invasion, angiogenesis, and metastasis (Lin et al., 2010). As such, the NF-κB pathway is an attractive therapeutic target in a broad range of human cancers, as well as in numerous non-malignant diseases. Furthermore, COX-2, an enzyme that catalyzes the first step in the synthesis of prostanoids, is associated with inflammatory diseases and carcinogenesis (Liu et al., 2015). An overexpression of COX-2 is observed in cancers of the pancreas, breast, colorectal, stomach, and lung carcinoma. Therefore, COX-2 is considered as a significant target for the development of new anticancer agents (Mohsin et al., 2022). Among the bioactive compounds investigated, the mechanism of action of hederagenin has been extensively studied in several studies. In fact, hederagenin has been reported to have anti-tumour and anti-inflammatory effects by regulating the NFκB, PI3K/AKT and JAK2/STAT3/MAPK signalling pathways, and by reducing the expression of pro-inflammatory cytokines or enzyme production, including Tumor Necrosis Factor- α (TNF-α), interleukin 6 (IL-6), NO, prostaglandin E2 (PGE2), inducible nitric oxide synthase (iNOS) and COX-2 (Zhang et al., 2012; Lee et al., 2015; Kim et al., 2017; Yu et al., 2020; Shen et al., 2023). Our results indicate the accordance between the molecular docking and the previous studies thereby confirming the efficacy of hederagenin as a prominent lead compound for the management of inflammation and cancer development. Further investigations are required for quinovic acid 3-O-[α-D-quinovopyranoside] and quinovic acid 3-O-[β-D-quinovopyranoside] which modes of action are still not experimentally investigated.
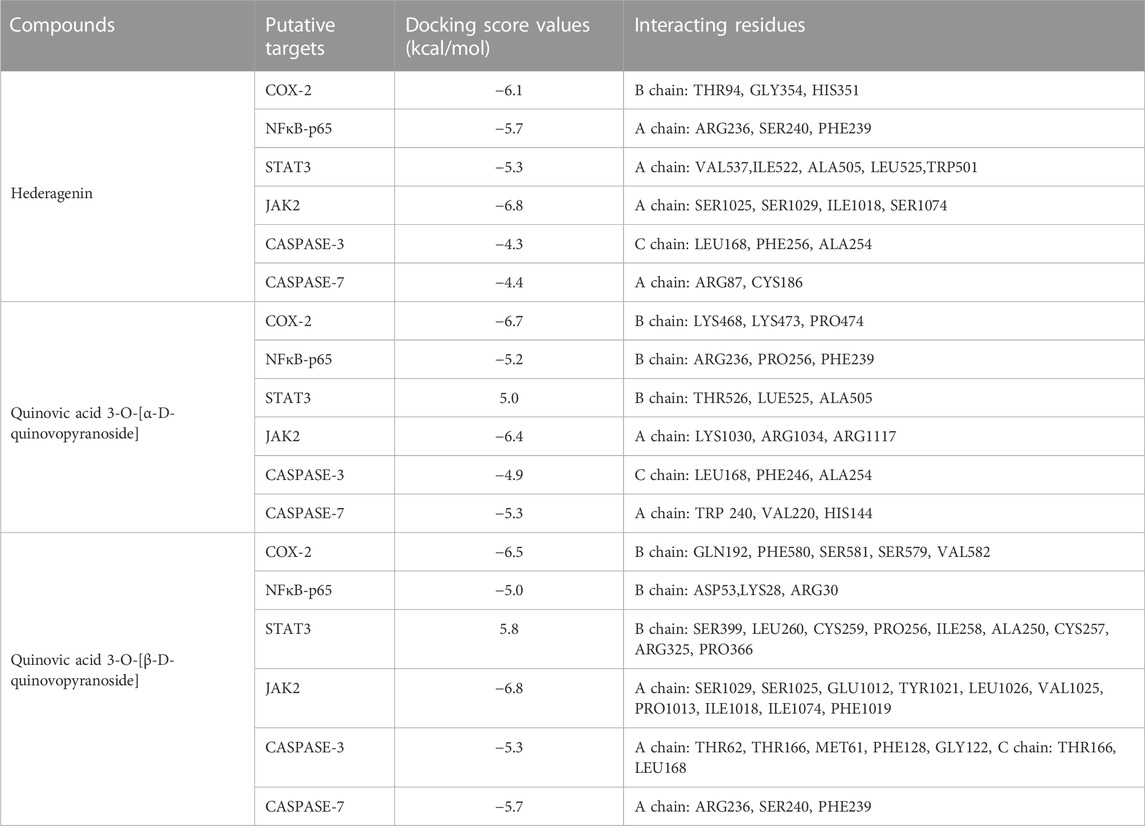
TABLE 4. Molecular docking score (kcal/mol) and interacting residues of the most bioactive compounds against six putative target proteins based on the best protein-ligand binding pose.
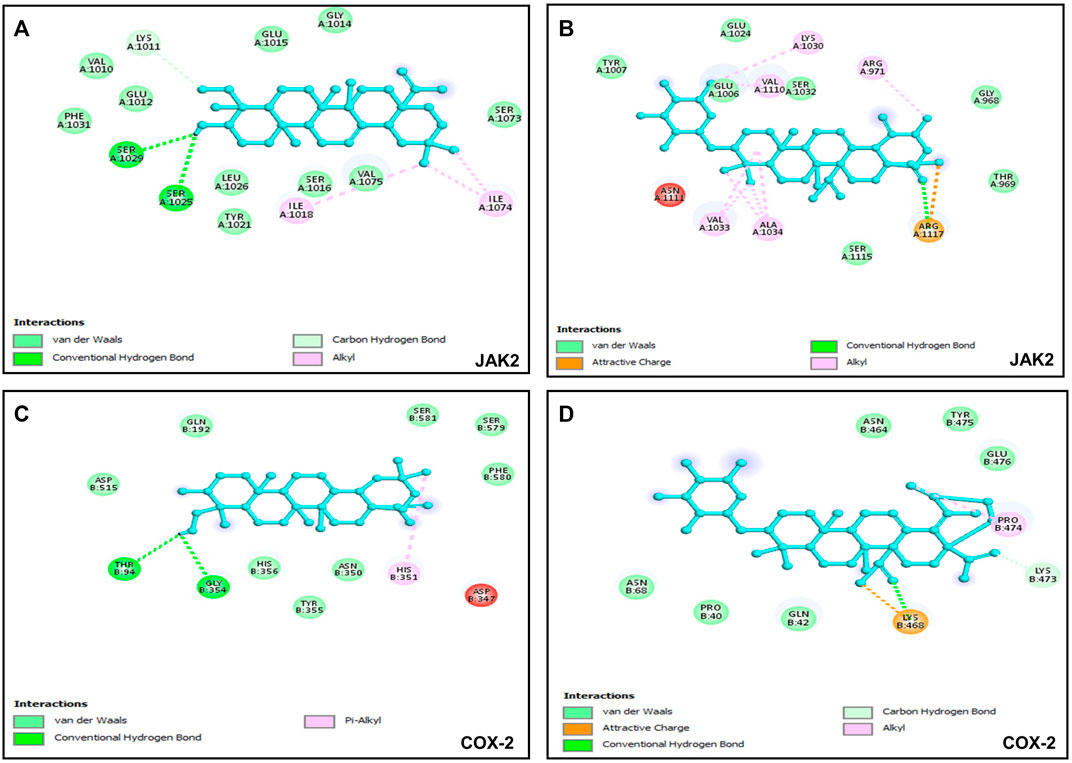
FIGURE 5. Visualization of binding interactions by using BIOVIA Discovery Studio Visualizer 2021 software; (A) protein-ligand interactions between JAK2 and hederagenin (−6.8 kcal/mol); (B) protein-ligand interactions between JAK2 and quinovic acid 3-O-[α-D-quinovopyranoside] (−6.4 kcal/mol); (C) protein-ligand interactions between COX-2 and hederagenin (−6.1 kcal/mol); (D) protein-ligand interactions between COX-2 and quinovic acid 3-O-[α-D-quinovopyranoside] (−6.7 kcal/mol).
4 Conclusion
This study reported the isolation of ten natural compounds from leaf, fruit and bark of Sarcocephalus pobeguinii, and described their antiproliferative and anti-inflammatory effects as well as exploration of potential target proteins of bioactive compounds. Hederagenin (2), quinovic acid 3-O-[α-D-quinovopyranoside] (6) and quinovic acid 3-O-[β-D-quinovopyranoside] (9) exhibited antiproliferative effect against all cancerous cells by inducing apoptosis via caspase-3/-7 activation. Among these bioactive compounds, hederagenin, isolated for the first time from the fruits of S. pobeguinii, selectively kills cancer cells with additional anti-inflammatory potential, and it interacts with JAK2 and COX-2 suggesting these proteins as its potential molecular targets. The molecular docking results were in agreement with previously reported experimental data thereby confirming hederagenin as a prominent drug candidate to tackle cancer progression. Furthermore, apart from hederagenin, nauclealatifoline G and naucleofficine D (1), and chletric acid (3) strongly inhibited the activity of 15-LOX as compared to quercetin, which opens further research direction aiming to investigate experimentally their mode of action on molecular targets of inflammation.
Data availability statement
The original contributions presented in the study are included in the article/Supplementary Material, further inquiries can be directed to the corresponding authors.
Author contributions
EMN conceptualized the study design; EMN, AM, BN, and JA participated in data collection and in conducting the study. AM, PM, and BN analyzed the spectroscopic data. EMN and AM wrote the original draft of the manuscript. EMN and CC contributed to the statistical analysis of data. LM, TM, and SM provided facility and funding to carried out this study. All authors contributed to the article and approved the submitted version.
Funding
This research was funded by the Central University of Technology operational expenses (RES. 10/22/04 awarded to EMN) and the National Research Foundation (NRF), South Africa, through the Incentive Funding for Rated Researchers (awarded to LM). The APC was funded by the Central University of Technology research expenses (TM).
Acknowledgments
EN is very grateful to the Central University of Technology for the Track1 Postdoctoral fellowship.
Conflicts of interest
The authors declare that the research was conducted in the absence of any commercial or financial relationships that could be construed as a potential conflict of interest.
Publisher’s note
All claims expressed in this article are solely those of the authors and do not necessarily represent those of their affiliated organizations, or those of the publisher, the editors and the reviewers. Any product that may be evaluated in this article, or claim that may be made by its manufacturer, is not guaranteed or endorsed by the publisher.
Supplementary material
The Supplementary Material for this article can be found online at: https://www.frontiersin.org/articles/10.3389/fphar.2023.1205414/full#supplementary-material
References
Agomuoh, A. A., Ata, A., Udenigwe, C. C., Aluko, R. E., and Irenus, I. (2013). Novel indole alkaloids from Nauclea latifolia and their renin-inhibitory activities. Chem. Biodivers. 10 (3), 401–410. doi:10.1002/cbdv.201200023
Aktar, F., Abul Kaisar, M., Hamidul Kabir, A. N. M., Hasanand, C. M., and Rashid, M. A. (2009). Phytochemical and biological investigations of ixora arborea. Dhaka Univ. J. Pharm. Sci. 8 (2), 161–166. doi:10.3329/dujps.v8i2.6031
Amjad, M. T., Chidharla, A., and Kasi, A. (2022). Cancer chemotherapy. StatPearls: Treasure Island FL.
Elekofehinti, O. O., Iwaloye, O., Olawale, F., and Ariyo, E. O. (2021). Saponins in cancer treatment: Current progress and future prospects. Pathophysiology 28 (2), 250–272. doi:10.3390/pathophysiology28020017
Fatima, N., Tapondjou, L. A., Lontsi, D., Sondengam, B. L., Atta Ur, R., and Choudhary, M. I. (2002). Quinovic acid glycosides from Mitragyna stipulosa-first examples of natural inhibitors of snake venom phosphodiesterase I. Nat. Prod. Lett. 16 (6), 389–393. doi:10.1080/10575630290033169
Gao, Y., He, C., Bi, W., Wu, G., and Altman, E. (2016). Bioassay guided fractionation identified hederagenin as a major cytotoxic agent from cyclocarya paliurus leaves. Planta Med. 82 (1-2), 171–179. doi:10.1055/s-0035-1557900
Gauthier, C., Legault, J., Girard-Lalancette, K., Mshvildadze, V., and Pichette, A. (2009). Haemolytic activity, cytotoxicity and membrane cell permeabilization of semi-synthetic and natural lupane- and oleanane-type saponins. Bioorg. Med. Chem. 17 (5), 2002–2008. doi:10.1016/j.bmc.2009.01.022
Haudecoeur, R., Peuchmaur, M., Peres, B., Rome, M., Taiwe, G. S., Boumendjel, A., et al. (2018). Traditional uses, phytochemistry and pharmacological properties of african Nauclea species: A review. J. Ethnopharmacol. 212, 106–136. doi:10.1016/j.jep.2017.10.011
Hibino, S., Kawazoe, T., Kasahara, H., Itoh, S., Ishimoto, T., Sakata-Yanagimoto, M., et al. (2021). Inflammation-induced tumorigenesis and metastasis. Int. J. Mol. Sci. 22 (11), 5421. doi:10.3390/ijms22115421
Huang, B., Lang, X., and Li, X. (2022). The role of IL-6/JAK2/STAT3 signaling pathway in cancers. Front. Oncol. 12, 1023177. doi:10.3389/fonc.2022.1023177
Jha, N. K., Arfin, S., Jha, S. K., Kar, R., Dey, A., Gundamaraju, R., et al. (2022). Re-establishing the comprehension of phytomedicine and nanomedicine in inflammation-mediated cancer signaling. Semin. Cancer Biol. 86 (2), 1086–1104. doi:10.1016/j.semcancer.2022.02.022
Jiofack, T., Ayissi, I., Fokunang, C., Guedje, N., and Kemeuze, V. (2009). Ethnobotany and phytomedicine of the upper Nyong valley forest in Cameroon. Afr. J. Pharm. Pharmacol. 3 (4), 144–150. doi:10.5897/AJPP.9000140
Joshi, B. S., Singh, K. L., and Raja, R. (1999). Complete assignments of 1H and 13C NMR spectra of the pentacyclic triterpene hederagenin from Nigella sativa Linn. Magnetic Reson. Chem. 37, 295–298. doi:10.1002/(sici)1097-458x(199904)37:4<295:aid-mrc457>3.0.co;2-z
Karou, S. D., Tchacondo, T., Ilboudo, D. P., and Simpore, J. (2011). Sub-saharan rubiaceae: A review of their traditional uses, phytochemistry and biological activities. Pak. J. Biol. Sci. PJBS 14 (3), 149–169. doi:10.3923/pjbs.2011.149.169
Kim, G. J., Song, D. H., Yoo, H. S., Chung, K. H., Lee, K. J., and An, J. H. (2017). Hederagenin supplementation alleviates the pro-inflammatory and apoptotic response to alcohol in rats. Nutrients 9 (1), 41. doi:10.3390/nu9010041
Kuete, V., and Efferth, T. (2010). Cameroonian medicinal plants: Pharmacology and derived natural products. Front. Pharmacol. 1, 123. doi:10.3389/fphar.2010.00123
Kuete, V., Sandjo, L. P., Mbaveng, A. T., Seukep, J. A., Ngadjui, B. T., and Efferth, T. (2015). Cytotoxicity of selected Cameroonian medicinal plants and Nauclea pobeguinii towards multi-factorial drug-resistant cancer cells. BMC Complement. Altern. Med. 15, 309. doi:10.1186/s12906-015-0841-y
Lai, H., Liu, Y., Wu, J., Cai, J., Jie, H., Xu, Y., et al. (2022). Targeting cancer-related inflammation with non-steroidal anti-inflammatory drugs: Perspectives in pharmacogenomics. Front. Pharmacol. 13, 1078766. doi:10.3389/fphar.2022.1078766
Lakhani, S. A., Masud, A., Kuida, K., Porter, G. A., Booth, C. J., Mehal, W. Z., et al. (2006). Caspases 3 and 7: Key mediators of mitochondrial events of apoptosis. Science 311 (5762), 847–851. doi:10.1126/science.1115035
Lee, C. W., Park, S. M., Zhao, R., Lee, C., Chun, W., Son, Y., et al. (2015). Hederagenin, a major component of Clematis mandshurica Ruprecht root, attenuates inflammatory responses in RAW 264.7 cells and in mice. Int. Immunopharmacol. 29 (2), 528–537. doi:10.1016/j.intimp.2015.10.002
Lin, Y., Bai, L., Chen, W., and Xu, S. (2010). The NF-kappaB activation pathways, emerging molecular targets for cancer prevention and therapy. Expert Opin. Ther. targets 14 (1), 45–55. doi:10.1517/14728220903431069
Liu, B., Qu, L., and Yan, S. (2015). Cyclooxygenase-2 promotes tumor growth and suppresses tumor immunity. Cancer Cell. Int. 15, 106. doi:10.1186/s12935-015-0260-7
Mantovani, A., Allavena, P., Sica, A., and Balkwill, F. (2008). Cancer-related inflammation. Nature 454 (7203), 436–444. doi:10.1038/nature07205
Mengie Ayele, T., Tilahun Muche, Z., Behaile Teklemariam, A., Bogale Kassie, A., and Chekol Abebe, E. (2022). Role of JAK2/STAT3 signaling pathway in the tumorigenesis, chemotherapy resistance, and treatment of solid tumors: A systemic review. J. Inflamm. Res. 15, 1349–1364. doi:10.2147/JIR.S353489
Mesia, G. K., Tona, G. L., Penge, O., Lusakibanza, M., Nanga, T. M., Cimanga, R. K., et al. (2005). Antimalarial activities and toxicities of three plants used as traditional remedies for malaria in the democratic republic of Congo: Croton mubango, Nauclea pobeguinii and pyrenacantha staudtii. Ann. Trop. Med. Parasitol. 99 (4), 345–357. doi:10.1179/136485905X36325
Mfotie Njoya, E., Eloff, J. N., and McGaw, L. J. (2018). Croton gratissimus leaf extracts inhibit cancer cell growth by inducing caspase 3/7 activation with additional anti-inflammatory and antioxidant activities. BMC Complement. Altern. Med. 18 (1), 305. doi:10.1186/s12906-018-2372-9
Mfotie Njoya, E., Maza, H. L. D., Mkounga, P., Koert, U., Nkengfack, A. E., and McGaw, L. J. (2020). Selective cytotoxic activity of isolated compounds from Globimetula dinklagei and Phragmanthera capitata (Loranthaceae). Z Naturforsch C J. Biosci. 75 (5-6), 135–144. doi:10.1515/znc-2019-0171
Mfotie Njoya, E., Maza, H. L. D., Swain, S. S., Chukwuma, C. I., Mkounga, P., Nguekeu Mba, Y. M., et al. (2023). Natural compounds isolated from african mistletoes (loranthaceae) exert anti-inflammatory and acetylcholinesterase inhibitory potentials: In vitro and in silico studies. Appl. Sci. 13 (4), 2606. doi:10.3390/app13042606
Mfotie Njoya, E., Munvera, A. M., Mkounga, P., Nkengfack, A. E., and McGaw, L. J. (2017). Phytochemical analysis with free radical scavenging, nitric oxide inhibition and antiproliferative activity of Sarcocephalus pobeguinii extracts. BMC Complement. Altern. Med. 17 (1), 199. doi:10.1186/s12906-017-1712-5
Mohamed, M. (1999). Quinovic acid glycosides from Zygophyllum aegyptium. Bull. Pharm. Sci. 22 (1), 47–53. doi:10.21608/bfsa.1999.66076
Mohsin, N. U. A., Aslam, S., Ahmad, M., Irfan, M., Al-Hussain, S. A., and Zaki, M. E. A. (2022). Cyclooxygenase-2 (COX-2) as a target of anticancer agents: A review of novel synthesized scaffolds having anticancer and COX-2 inhibitory potentialities. Pharm. (Basel) 15 (12), 1471. doi:10.3390/ph15121471
Mosmann, T. (1983). Rapid colorimetric assay for cellular growth and survival: Application to proliferation and cytotoxicity assays. J. Immunol. methods 65 (1-2), 55–63. doi:10.1016/0022-1759(83)90303-4
Nag, S. A., Qin, J. J., Wang, W., Wang, M. H., Wang, H., and Zhang, R. (2012). Ginsenosides as anticancer agents: In vitro and in vivo activities, structure-activity relationships, and molecular mechanisms of action. Front. Pharmacol. 3, 25. doi:10.3389/fphar.2012.00025
Novichikhina, N., Ilin, I., Tashchilova, A., Sulimov, A., Kutov, D., Ledenyova, I., et al. (2020). Synthesis, docking, and in vitro anticoagulant activity assay of hybrid derivatives of pyrrolo[3,2,1-ij]Quinolin-2(1H)-one as new inhibitors of factor xa and factor XIa. Molecules 25 (8), 1889. doi:10.3390/molecules25081889
Ntie-Kang, F., Lifongo, L. L., Mbaze, L. M., Ekwelle, N., Owono Owono, L. C., Megnassan, E., et al. (2013). Cameroonian medicinal plants: A bioactivity versus ethnobotanical survey and chemotaxonomic classification. BMC Complement. Altern. Med. 13, 147. doi:10.1186/1472-6882-13-147
Olsson, M., and Zhivotovsky, B. (2011). Caspases and cancer. Cell. death Differ. 18 (9), 1441–1449. doi:10.1038/cdd.2011.30
Peczek, P., Gajda, M., Rutkowski, K., Fudalej, M., Deptala, A., and Badowska-Kozakiewicz, A. M. (2022). Cancer-associated inflammation: Pathophysiology and clinical significance. J. Cancer Res. Clin. Oncol. 149, 2657–2672. doi:10.1007/s00432-022-04399-y
Pinto, M. C., Tejeda, A., Duque, A. L., and Macias, P. (2007). Determination of lipoxygenase activity in plant extracts using a modified ferrous oxidation-xylenol orange assay. J. Agric. food Chem. 55 (15), 5956–5959. doi:10.1021/jf070537x
Podolak, I., Grabowska, K., Sobolewska, D., Wrobel-Biedrawa, D., Makowska-Was, J., and Galanty, A. (2023). Saponins as cytotoxic agents: An update (2010–2021). Part II—triterpene saponins. Phytochem. Rev. 22, 113–167. doi:10.1007/s11101-022-09830-3
Rayburn, E. R., Ezell, S. J., and Zhang, R. (2009). Anti-inflammatory agents for cancer therapy. Mol. Cell. Pharmacol. 1 (1), 29–43. doi:10.4255/mcpharmacol.09.05
Rodriguez-Hernandez, D., Demuner, A. J., Barbosa, L. C., Csuk, R., and Heller, L. (2015). Hederagenin as a triterpene template for the development of new antitumor compounds. Eur. J. Med. Chem. 105, 57–62. doi:10.1016/j.ejmech.2015.10.006
Rodriguez-Hernandez, D., Demuner, A. J., Barbosa, L. C., Heller, L., and Csuk, R. (2016). Novel hederagenin-triazolyl derivatives as potential anti-cancer agents. Eur. J. Med. Chem. 115, 257–267. doi:10.1016/j.ejmech.2016.03.018
Shen, Y., Teng, L., Qu, Y., Huang, Y., Peng, Y., Tang, M., et al. (2023). Hederagenin suppresses inflammation and cartilage degradation to ameliorate the progression of osteoarthritis: An in vivo and in vitro study. Inflammation 46 (2), 655–678. doi:10.1007/s10753-022-01763-5
Singh, N., Baby, D., Rajguru, J. P., Patil, P. B., Thakkannavar, S. S., and Pujari, V. B. (2019). Inflammation and cancer. Ann. Afr. Med. 18 (3), 121–126. doi:10.4103/aam.aam_56_18
Sung, H., Ferlay, J., Siegel, R. L., Laversanne, M., Soerjomataram, I., Jemal, A., et al. (2021). Global cancer statistics 2020: GLOBOCAN estimates of incidence and mortality worldwide for 36 cancers in 185 countries. CA Cancer J. Clin. 71 (3), 209–249. doi:10.3322/caac.21660
Takahashi, K., and Takani, M. (1978). Studies on the constituents of the medicinal plants. XXI. Constituents of the leaves of Clethra barbinervis Sieb. et Zucc. (2) and the 13C-nuclear magnetic resonance spectra of 19.ALPHA. hydroxyurs-12-en-28-oic acid type of triterpenoids. Bulletin 26, 2689–2693. doi:10.1248/cpb.26.2689
Tian, W., Chen, C., Lei, X., Zhao, J., and Liang, J. (2018). CASTp 3.0: Computed atlas of surface topography of proteins. Nucleic acids Res. 46 (1), W363–W7. doi:10.1093/nar/gky473
Trott, O., and Olson, A. J. (2010). AutoDock Vina: Improving the speed and accuracy of docking with a new scoring function, efficient optimization, and multithreading. J. Comput. Chem. 31 (2), 455–461. doi:10.1002/jcc.21334
Viet, T. D., Xuan, T. D., and Anh, H. (2021). α-Amyrin and β-amyrin isolated from Celastrus hindsii leaves and their antioxidant, anti-xanthine oxidase, and anti-tyrosinase potentials. Molecules 26 (23), 7248. doi:10.3390/molecules26237248
Xu, K., Shu, Z., Xu, Q. M., Liu, Y. L., Li, X. R., Wang, Y. L., et al. (2013). Cytotoxic activity of Pulsatilla chinensis saponins and their structure-activity relationship. J. Asian Nat. Prod. Res. 15 (6), 680–686. doi:10.1080/10286020.2013.790901
Yen, C. K., Keng, C. W., Hasnah, O., Ibrahim, E., and Mohammad, Z. A. (2013). Chemical constituents and biological activities of Strobilanthes crispus. Rec. Nat. Prod. 7, 59–64.
Yepez, A. M., de Ugaz, O. L., Alvarez, C. M., De Feo, V., Aquino, R., De Simone, F., et al. (1991). Quinovic acid glycosides from Uncaria guianensis. Phytochemistry 30 (5), 1635–1637. doi:10.1016/0031-9422(91)84223-f
Yu, H., Song, L., Cao, X., Li, W., Zhao, Y., Chen, J., et al. (2020). Hederagenin attenuates cerebral ischaemia/reperfusion injury by regulating MLK3 signalling. Front. Pharmacol. 11, 1173. doi:10.3389/fphar.2020.01173
Yüce, I., Agnaniet, H., and Morlock, G. E. (2019). New antidiabetic and free-radical scavenging potential of strictosamide in Sarcocephalus pobeguinii ground bark extract via effect-directed analysis. ACS Omega 4 (3), 5038–5043. doi:10.1021/acsomega.8b02462
Zappavigna, S., Cossu, A. M., Grimaldi, A., Bocchetti, M., Ferraro, G. A., Nicoletti, G. F., et al. (2020). Anti-inflammatory drugs as anticancer agents. Int. J. Mol. Sci. 21 (7), 2605. doi:10.3390/ijms21072605
Zhang, L. J., Cheng, J. J., Liao, C. C., Cheng, H. L., Huang, H. T., Kuo, L. M., et al. (2012). Triterpene acids from Euscaphis japonica and assessment of their cytotoxic and anti-NO activities. Planta Med. 78 (14), 1584–1590. doi:10.1055/s-0032-1315040
Keywords: Sarcocephalus pobeguinii, hederagenin, inflammation, cancer, cytotoxicity, selective index, apoptosis, docking score
Citation: Mfotie Njoya E, Ndemangou B, Akinyelu J, Munvera AM, Chukwuma CI, Mkounga P, Mashele SS, Makhafola TJ and McGaw LJ (2023) In vitro antiproliferative, anti-inflammatory effects and molecular docking studies of natural compounds isolated from Sarcocephalus pobeguinii (Hua ex Pobég). Front. Pharmacol. 14:1205414. doi: 10.3389/fphar.2023.1205414
Received: 13 April 2023; Accepted: 12 June 2023;
Published: 21 June 2023.
Edited by:
Ochuko Lucky Erukainure, University of the Free State, South AfricaReviewed by:
Laiba Arshad, Forman Christian College, PakistanMd Afjalus Siraj, Yale University, United States
Copyright © 2023 Mfotie Njoya, Ndemangou, Akinyelu, Munvera, Chukwuma, Mkounga, Mashele, Makhafola and McGaw. This is an open-access article distributed under the terms of the Creative Commons Attribution License (CC BY). The use, distribution or reproduction in other forums is permitted, provided the original author(s) and the copyright owner(s) are credited and that the original publication in this journal is cited, in accordance with accepted academic practice. No use, distribution or reproduction is permitted which does not comply with these terms.
*Correspondence: Emmanuel Mfotie Njoya, bWZvdGllZnJAeWFob28uZnI=, ZW5qb3lhQGN1dC5hYy56YQ==; Tshepiso J. Makhafola, am1ha2hhZm9sYUBjdXQuYWMuemE=