- 1School of Pharmaceutical Engineering and Life Science, Changzhou University, Changzhou, China
- 2Medical School, Southeast University, Nanjing, China
In organisms, high glucose can cause several aspects of toxicity, including the lifespan reduction. Paeoniflorin is the major component of Paeoniaceae plants. Nevertheless, the possible effect of paeoniflorin to suppress high glucose toxicity in reducing lifespan and underlying mechanism are largely unclear. Thus, in this study, we examined the possible effect of paeoniflorin in suppressing high glucose (50 mM)-induced lifespan reduction and the underlying mechanism in Caenorhabditis elegans. Administration with 16–64 mg/L paeoniflorin could prolong the lifespan in glucose treated nematodes. Accompanied with this beneficial effect, in glucose treated nematodes, expressions of daf-2 encoding insulin receptor and its downstream kinase genes (age-1, akt-1, and akt-2) were decreased and expression of daf-16 encoding FOXO transcriptional factor was increased by 16–64 mg/L paeoniflorin administration. Meanwhile, the effect of paeoniflorin in extending lifespan in glucose treated nematodes was enhanced by RNAi of daf-2, age-1, akt-1, and akt-2 and inhibited by RNAi of daf-16. In glucose treated nematodes followed by paeoniflorin administration, the increased lifespan caused by daf-2 RNAi could be suppressed by RNAi of daf-16, suggesting that DAF-2 acted upstream of DAF-16 to regulate pharmacological effect of paeoniflorin. Moreover, in glucose treated nematodes followed by paeoniflorin administration, expression of sod-3 encoding mitochondrial Mn-SOD was inhibited by daf-16 RNAi, and the effect of paeoniflorin in extending lifespan in glucose treated nematodes could be suppressed by sod-3 RNAi. Molecular docking analysis indicated the binding potential of paeoniflorin with DAF-2, AGE-1, AKT-1, and AKT-2. Therefore, our results demonstrated the beneficial effect of paeoniflorin administration in inhibiting glucose-induced lifespan reduction by suppressing signaling cascade of DAF-2-AGE-1-AKT-1/2-DAF-16-SOD-3 in insulin signaling pathway.
Introduction
Hyperglycemia is a pathological situation with severe increase in blood glucose level in the patients (Gunst et al., 2019). This increase in blood glucose level is the major cause for type 2 diabetes (Monnier et al., 2012), and may be induced by sedentary lifestyle and excessive calories intake (Stumvoll et al., 2005; Taylor et al., 2021). Hyperglycemia is also closely associated with some other diseases, such as diabetic cardiomyopathy and renal disease (Baldwin and Apel, 2013; Jia et al., 2018). Hyperglycemia is further related to increased mortality and morbidity during neonatal period (Ramel and Rao, 2020). Chronic hyperglycemia has emerged as a global health challenge. Considering the biological activity and non-toxicity, the efforts to identify beneficial natural extracts or compounds have been conducted in order to be used for treating hyperglycemia.
Various plants and their metabolic products have been focused as an important area for identifying bioactive compounds with the health benefits, and most of these bioactive compounds are secondary metabolites and present at relatively small amount in plants (Aiello et al., 2019; Sahardi and Makpol, 2019). As a classic model animal with well-described genetic backgrounds, both molecular and metabolic pathways in Caenorhabditis elegans are highly conserved and have corresponding homologues in humans (Pir et al., 2017; Watts and Ristow, 2017; Wang, 2019). C. elegans has been shown as a powerful animal model for evaluating different aspects pharmacological effects of bioactive compounds, including anti-bacterial and fungal infections and anti-neurodegeneration diseases (such as ant-Alzheimer’s disease) (Parker et al., 2004; Griffin et al., 2017; Madende et al., 2020). During the past decade, many efforts have been made to discover bioactive compounds having anticipated pharmacological effects, such as anti-aging using the C. elegans as aging model (Collins et al., 2006; Okoro et al., 2021). In addition, due to sensitivity to environmental exposure and small volume of exposure (Wang, 2020; Xu et al., 2022a; Xu et al., 2022b; Zhao Y.-L. et al., 2023; Hua et al., 2023c), C. elegans is useful for high throughput screen of drugs or compounds (Braungart et al., 2004; O’Reilly et al., 2014).
C. elegans has been frequently applied to assess glucose-induced toxicity and to identify compounds against glucose toxicity (Fitzenberger et al., 2013; Yan et al., 2017a). In C. elegans, some components in biochemical reactions were found to have potential in reducing the glucose toxicity. For example, supplementation with carnitine (a substrate of β-oxidation) or diosgenin (a phytosterol substitute) could reduce glucose toxicity via nuclear hormone receptor DAF-12 or insulin signaling (Deusing et al., 2015a; Shanmugam et al., 2017). Besides these, some plant extracts or bioactive compounds were also found to have the potential against glucose-induced toxicity. Green tea extract enriched with catechin or blackberry leaf extract prevented the glucose-induced survival reduction (Fitzenberger et al., 2014; Deusing et al., 2015b). The anthocyanin in mulberry fruit and C-glycosides in Apios americana leaves also exhibited the protective function against the toxicity under hyperglycemic condition (Yan et al., 2017a; Yan et al., 2017b).
Paeoniflorin is the major component of total glycoside paeony. Initially, the monoterpenoid glycoside paeoniflorin was extracted in Paeonia lactiflora Pall (Zhang et al., 2019). The content of paeoniflorin in different species of Paeoniaceae ranges from 0.05% to 10.7% (Zhang X. et al., 2022). Some reports have suggested the function of paeoniflorin treatment in inhibiting glucose-induced inflammation response and oxidative injury (Shao et al., 2016; Yang et al., 2016; Zhu et al., 2017). In this study, we employed C. elegans as an animal model to further determine the possible beneficial effect of paeoniflorin against glucose toxicity in reducing lifespan and the underlying mechanism. In C. elegans, insulin signaling pathway plays a crucial function in regulating longevity (Kenyon, 2010). The insulin receptor DAF-2 regulates the longevity by activating downstream kinase cascade (AGE-1-AKT-1/2), and inhibiting FOXO transcription factor DAF-16/FOXO (Lin et al., 2001; Zheng et al., 2018). Our results suggest that administration with paeoniflorin could inhibit the glucose toxicity in reducing lifespan by suppressing insulin signaling in nematodes. Our data suggested the potential effect of paeoniflorin treatment in suppressing glucose-induced lifespan reduction.
Materials and methods
Reagent
The paeoniflorin was purchased from Yuanye Bio-Technology Co., Ltd., (Shanghai, China). The purity of paeoniflorin was ≥98%. Chemical structure of paeoniflorin is shown in Figure 1A. The stocking solution (1.024 mg/mL) was prepared by dissolving paeoniflorin into DMSO. The working solutions of paeoniflorin were prepared by diluting the stocking solution with K buffer and stored at 4°C. The control solution used in this experiment was comprised of DMSO, and diluted with K buffer in the same way as paeoniflorin solutions.
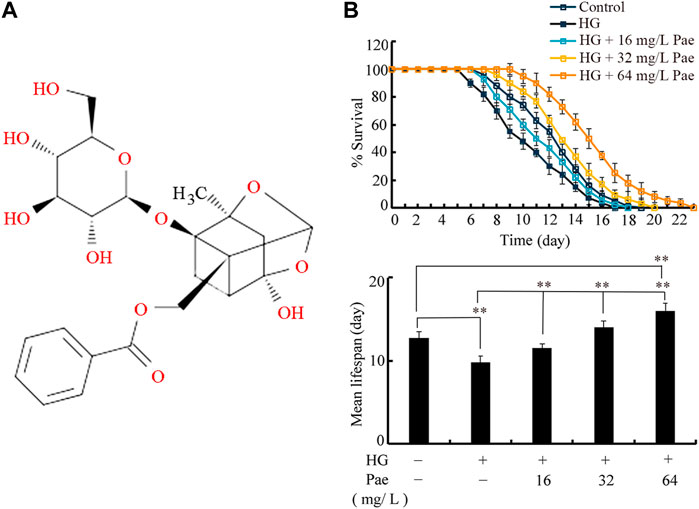
FIGURE 1. Effect of paeoniflorin administration in reducing glucose toxicity. (A) Chemical structure of paeoniflorin. (B) Effect of paeoniflorin administration on lifespan in glucose treated nematodes. Lifespan curve of HG was significantly (p < 0.01) different from control. Lifespan curves of HG + 16 mg/L Pae, HG + 32 mg/L Pae, and HG+64 mg/L Pae were significantly (p < 0.01) different from HG. Lifespan curve of HG + 64 mg/L Pae was significantly (p < 0.01) different from control. HG, high glucose (50 mM); Pae, paeoniflorin. **p < 0.01.
C. elegans maintenance
The used C. elegans strains are listed in Supplementary Table S1. C. elegans are normally maintained on nematode growth medium (NGM) agar plates seeded with E. coli OP50 as food source according to the standard protocol (Brenner, 1974). Both C. elegans and E. coli strains were obtained from Caenorhabditis Genetics Center (CGC).
Preparation of glucose toxicity model in C. elegans
To assess the glucose toxicity, L1-larvae were treated in NGM plates fed with OP50 and containing glucose. The final concentration of added D-glucose was 50 mM in NGM plates (Shanmugam et al., 2017). To obtain synchronized L1-larval nematodes, eggs were released from pregnant hermaphrodites by treating them with solution lysis buffer (0.45 M NaOH and 2% HOCl), and allowed to further develop into L1-larvae (Zhang et al., 2022b). The nematodes were exposed to 50 mM glucose till to L4-larvae (approximately 2.5-day).
Pharmacological treatment
After the exposure of nematodes to 50 mM glucose, the nematodes were transferred into paeoniflorin solutions with the addition of OP50 to further treat for 48 h. The examined concentrations for paeoniflorin were 25, 50, and 100 mg/L, which were basically selected as previously described (Hua et al., 2023a). After the paeoniflorin treatment, the examined nematodes were transferred onto normal NGM plates for lifespan analysis. The experiments were repeated three times.
Lifespan analysis
The lifespan was analyzed as described (Zhang et al., 2022a). After glucose and paeoniflorin treatments, the survival of nematodes was counted on normal NGM plates. The survival was checked every day. The animals were counted as dead if no response of pharynx was observed after prodding with a platinum wire. To exclude the effect from offspring, we transferred the nematodes daily to new NGM plates. During the lifespan assay, median lifespan refers to the day at which 50% nematodes survive. For each treatment, 50 nematodes were tested. Three replicates were performed. Significance between lifespan curves was analyzed by Kaplan-Meier software, followed by the log-rank test.
Transcriptional expression analysis
Total RNA of nematodes was extracted using TRIZOL, and then reverse transcribed to obtain cDNA. Quality of prepared RNAs was assessed in NanoDrop One based on the ratio of OD260/OD280. The quantitative real-time polymerase chain reaction (qRT-PCR) was performed using SYBR Green Master Mix in an ABI 7500 real-time PCR system. The method of comparative CT (ΔΔ CT) was employed to evaluate transcriptional expressions of genes in C. elegans. The expressions of examined genes were expressed after normalization to reference gene tba-1 encoding tubulin (Hua et al., 2022). Three replicates were carried out. The designed primers for reference and examined genes are shown in Supplementary Table S2.
RNA interference (RNAi)
The E. coli HT115 expressing dsRNA for certain gene was used to feeding the examined nematodes (Zhao Y. et al., 2022). The RNAi feeding was carried out at the stage of pharmacological treatment with paeoniflorin. The HT115 RNAi clones for daf-2, age-1, akt-1, akt-2, daf-16, and sod-3 were obtained from Source Bioscience (Cambridge, United Kingdom) (Rual et al., 2004). HT115 expressing L4440, an empty vector, was employed as a control (Liu et al., 2022). RNAi efficiency was assessed based on qRT-PCR analysis, which is shown in Supplementary Figure S1.
Molecular docking analysis
The molecular interaction between paeoniflorin and proteins was performed using computer stimulation analysis. The structure of paeoniflorin was downloaded from the PubChem database (https://pubchem.ncbi.nlm.nih.gov/), and the structure of proteins of DAF-2, AGE-1, AKT-1, and AKT-2 were obtained from the UniProt database (https://www.rcsb.org). The Openbel software is used to convert the structures to PDB format (https://openbabel.org). Using AutoDock software (https://vina.scripps.edu/), the binding of paeoniflorin with certain proteins was stimulated, and different docking poses with the best affinity were generated. Using PyMol software (https://www.pymol.org/), image optimization and generation were performed.
Safety evaluation of paeoniflorin administration
We used lifespan, locomotion behavior, pumping rate, and brood size as endpoints to perform the safety evaluation of paeoniflorin administration. Under the normal condition, the L4-larval nematodes were treated with 16–64 mg/L paeoniflorin for 48 h. The lifespan was analyzed as described above. Locomotion behaviors of head thrash and body bend were analyzed to reflect alteration in function of motor neurons (Wang et al., 2023b; Hua et al., 2023d). A head thrash is defined as one swing of nematode body, and a body bend refers to the crawling of one wavelength (Zhao Y.-Y. et al., 2023). Fifty nematodes were assayed for each treatment. Pumping rate was used to evaluate the pharyngeal pumping, and analyzed as described (Wu et al., 2016). Nematodes were first normally maintained on NGM plate for 1-h. After that, pumping rate was assessed in 1 min intervals. Fifty nematodes were assayed for each treatment. Reproductive capacity was reflected by the endpoint of brood size (Zhao Y.-Y. et al., 2022; Hua et al., 2023e). Brood size was considered as number of offspring until nematodes end up laying eggs (Hua et al., 2023b). Thirty nematodes were assayed for each treatment.
Statistical analysis
Data are presented as means ± standard derivation (SD). SPSS 12.0 software was used for statistical analysis. Differences between different groups were analyzed by analysis of variance (ANOVA). Using SPSS Statistics 25.0 software, the significances of differences between exposure groups were analyzed using one-way or two-way analysis of variance (ANOVA) followed by Least-Significant Difference (LSD) of the post hoc test. Two-way ANOVA was used for comparing multiple factors. A probability level of 0.01 was considered statistically significant.
Results
Paeoniflorin treatment increased lifespan in nematodes after glucose exposure
In C. elegans, treatment with 50 mM glucose significantly reduced the lifespan as indicated by both lifespan curves and mean lifespan (Figure 1B). Under the background of 50 mM glucose treatment, administration with 16–64 mg/L paeoniflorin could obviously increase the lifespan of nematodes (Figure 1B). In 50 mM glucose treated nematodes, administration with 64 mg/L paeoniflorin even induced higher lifespan than control group (Figure 1B).
Paeoniflorin administration altered expressions of daf-2, age-1, akt-1, akt-2, and daf-16 in glucose treated nematodes
Considering the important function of insulin signaling pathway during aging control (Lin et al., 2001), we examined the effect of paeoniflorin administration on expressions of daf-2, age-1, akt-1, akt-2, and daf-16 in 50 mM glucose treated nematodes. Treatment with glucose (50 mM) significantly increased expressions of daf-2, age-1, akt-1, and akt-2, and decreased daf-16 expression (Figure 2). In 50 mM glucose treated nematodes, the decrease in daf-2, age-1, akt-1, and akt-2 expression and the increase in daf-16 expression could be significantly reversed by following administration with paeoniflorin (16–64 mg/L) to different degrees (Figure 2).
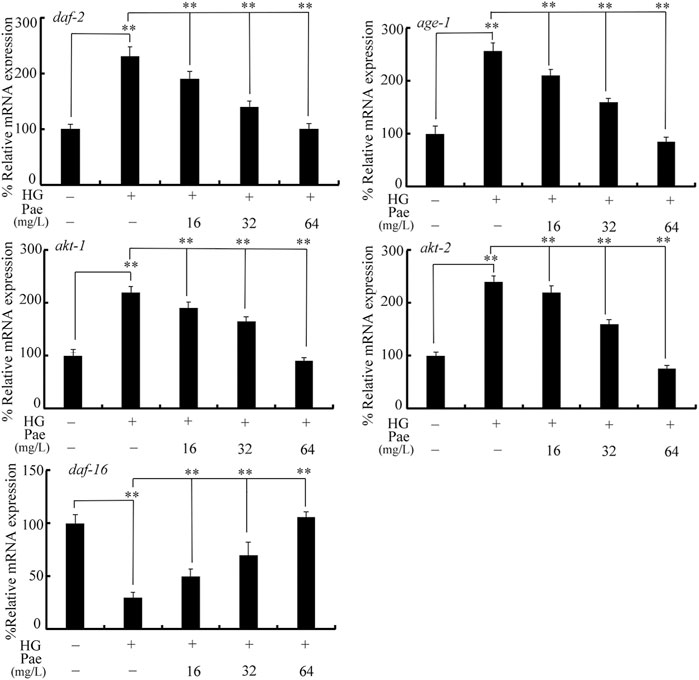
FIGURE 2. Effect of paeoniflorin administration on expressions of daf-2, age-1, akt-1, akt-2, and daf-16 in 50 mM glucose treated nematodes. HG, high glucose (50 mM); Pae, paeoniflorin. **p < 0.01.
Paeoniflorin administration affected expression of DAF-16::GFP in glucose treated nematodes
We further used transgenic strain of TJ356 to investigate effect of paeoniflorin administration on DAF-16::GFP expression in 50 mM glucose treated nematodes. Treatment with 50 mM glucose caused increase in DAF-16::GFP translocation in the nucleus and decrease in relative fluorescence intensity of DAF-16::GFP (Figure 3). In 50 mM glucose treated nematodes, administration with 16–64 mg/L paeoniflorin could cause the change of DAF-16::GFP translocation from nucleus to cytoplasm and increase in relative fluorescence intensity of DAF-16::GFP (Figure 3).
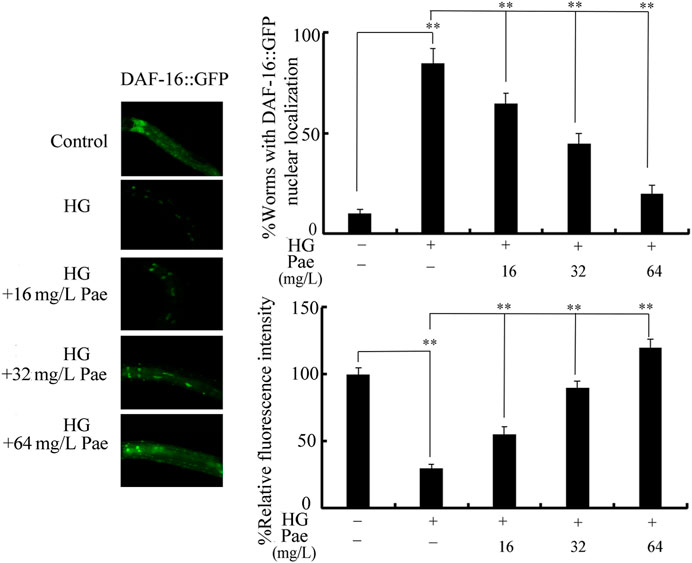
FIGURE 3. Effect of paeoniflorin administration on expression of DAF-16::GFP in 50 mM glucose treated nematodes. For each treatment, 50 nematodes were tested. HG, high glucose (50 mM); Pae, paeoniflorin. **p < 0.01.
RNAi of daf-2, age-1, akt-1, akt-2, and daf-16 altered the pharmacological effect of paeoniflorin in increasing lifespan in glucose treated nematodes
To determine the exact function of insulin signaling pathway, we investigated the effect of daf-2, age-1, akt-1, akt-2, and daf-16 RNAi on the role of paeoniflorin (64 mg/L) in increasing lifespan in 50 mM glucose treated nematodes. After glucose treatment followed by paeoniflorin administration, the lifespan was significantly increased by RNAi of daf-2, age-1, akt-1, and akt-2 compared to wild-type, and meanwhile the lifespan was significantly decreased by daf-16 RNAi compared to wild-type (Figure 4). Therefore, the insulin signaling pathway was involved in controlling pharmacological effect of paeoniflorin in increasing lifespan in glucose treated nematodes.
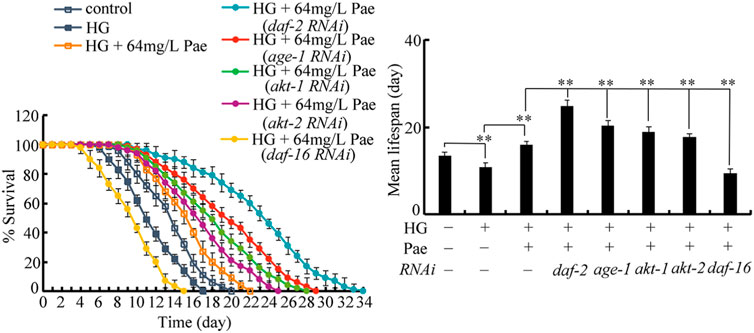
FIGURE 4. Effect of daf-2, age-1, akt-1, akt-2, and daf-16 RNAi on pharmacological effect of paeoniflorin (64 mg/L) in increasing lifespan in 50 mM glucose treated nematodes. Lifespan curve of HG was significantly (p < 0.01) different from control. Lifespan curve of HG + 64 mg/L Pae was significantly (p < 0.01) different from HG. Lifespan curves of HG + Pae + daf-2 (RNAi), HG + Pae + age-1 (RNAi), HG + Pae + akt-1 (RNAi), HG + Pae + akt-2 (RNAi), and HG + Pae + daf-16 (RNAi) were significantly (p < 0.01) different from HG + Pae. HG, high glucose (50 mM); Pae, paeoniflorin. **p < 0.01.
Genetic interaction between DAF-2 and DAF-16 in regulating pharmacological effect of paeoniflorin in increasing lifespan in glucose treated nematodes
To determine the genetic interaction between DAF-2 and DAF-16 in regulating the pharmacological effect of paeoniflorin, double RNAi of daf-2 and daf-16 was performed after the 50 mM glucose treatment. In glucose treated nematodes followed by paeoniflorin administration, the lifespan of daf-16(RNAi);daf-2(RNAi) nematodes was similar to that of daf-16(RNAi) nematodes (Figure 5), which suggested that DAF-16 acted downstream of DAF-2 to regulate the pharmacological effect of paeoniflorin in increasing lifespan in glucose treated nematodes.
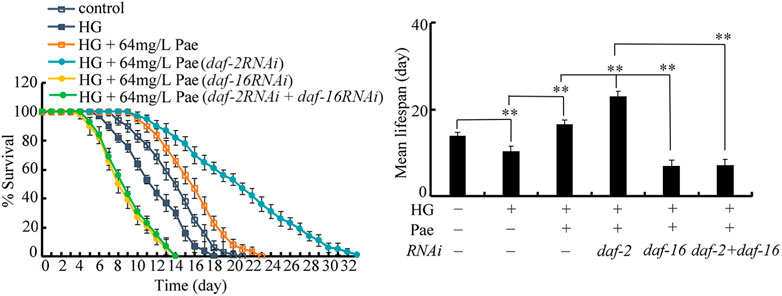
FIGURE 5. Genetic interaction between daf-2 and daf-16 in regulating function of paeoniflorin (64 mg/L) in increasing lifespan in 50 mM glucose treated nematodes. Lifespan curve of HG was significantly (p < 0.01) different from control. Lifespan curve of HG + 64 mg/L Pae was significantly (p < 0.01) different from HG. Lifespan curves of HG + Pae + daf-2 (RNAi) and HG + Pae + daf-16 (RNAi) were significantly (p < 0.01) different from HG + Pae. Lifespan curve of HG + Pae + daf-16 (RNAi); daf-2 (RNAi) was significantly (p < 0.01) different from HG + Pae + daf-2(RNAi). HG, high glucose (50 mM); Pae, paeoniflorin. **p < 0.01.
SOD-3 acts as downstream target of DAF-16 to regulate pharmacological effect of paeoniflorin in increasing lifespan in glucose treated nematodes
During the control of stress response, SOD-3 is a primary target of DAF-16 (Shao et al., 2019; Wang, 2019). Using transgenic strain CF1553, the decrease in SOD-3::GFP expression caused by 50 mM glucose could be suppressed by administration with 16–64 mg/L paeoniflorin (Figure 6A). The function of paeoniflorin (64 mg/L) in increasing SOD-3::GFP expression in 50 mM glucose treated nematodes was inhibited by RNAi of daf-16 (Figure 6A). Meanwhile, we observed that the role of paeoniflorin (64 mg/L) in increasing lifespan in 50 mM glucose treated nematodes was suppressed by RNAi of sod-3 (Figure 6B).
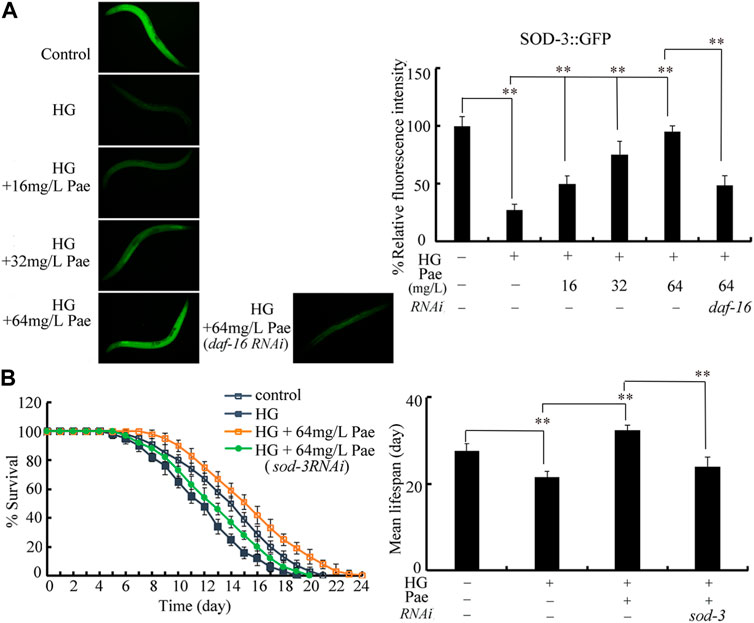
FIGURE 6. Role of SOD-3 in regulating function of paeoniflorin (64 mg/L) in increasing lifespan in 50 mM glucose treated nematodes. (A) Effect of daf-16 RNAi on SOD-3::GFP expression in 50 mM glucose treated nematodes after paeoniflorin (64 mg/L) administration. (B) Effect of sod-3 RNAi on lifespan in 50 mM glucose treated nematodes after paeoniflorin (64 mg/L) administration. HG, high glucose (50 mM); Pae, paeoniflorin. **p < 0.01.
Binding potential between paeoniflorin and DAF-2, AGE-1, AKT-1, and AKT-2
To further confirm the relationships between paeoniflorin and DAF-2 and its downstream kinases, molecular docking method was used to investigate the specific binding sites of paeoniflorin in DAF-2, AGE-1, AKT-1, and AKT-2. The molecular docking analysis showed that paeoniflorin potentially interacts with the amino acid residues of asparagine (Asn)-652, isoleucine (Ile)-651, and valine (Val)-653 in DAF-2, the amino acid residues of lysine (Lys)-1060, arginine (Arg)-1065, asparagine (Asn)-1173, and glutamine (Gln)-128 in AGE-1, amino acid residues of asparagine (Asn)-126, alanine (Ala)-125, and lysine (Lys)-68 in AKT-1, and amino acid residues of asparagine (Asn)-5 and leucine (Leu)-8 and (Leu)-55 in AKT-2 via hydrogen bonding (Figure 7A). The docked stable confirmations showed the binding energies between paeoniflorin and DAF-2, AGE-1, AKT-1, and AKT-2 were −7.6, −8.3, −8, and −8.4 kcal/mol, respectively (Figure 7B). These results suggested the binding potentials of paeoniflorin to DAF-2 and its downstream kinases.
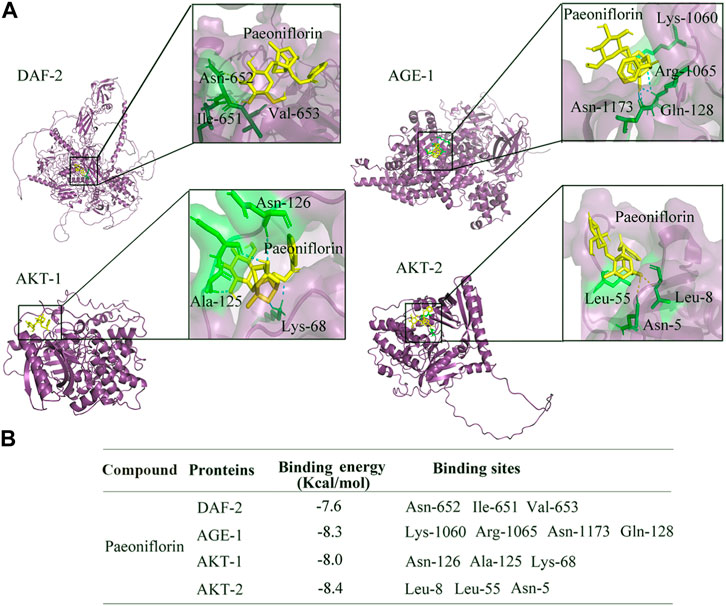
FIGURE 7. Binding potential of paeoniflorin with DAF-2, AGE-1, AKT-1, and AKT-2. (A) Molecular docking between paeoniflorin and DAF-2, AGE-1, AKT-1, or AKT-2. (B) The binding energy of paeoniflorin bound to the active sites of DAF-2, AGE-1, AKT-1, or AKT-2.
Safety evaluation of paeoniflorin administration on nematodes
Finally, we selected lifespan, locomotion behavior, pumping rate, and brood size as endpoints to evaluate the possible safety of paeoniflorin administration at these aspects in nematodes. Under the normal condition, administration with 16–64 mg/L paeoniflorin did not obviously affect lifespan, locomotion behavior reflected by body bend and head thrash, pumping rate, and brood size (Figures 8A–D).
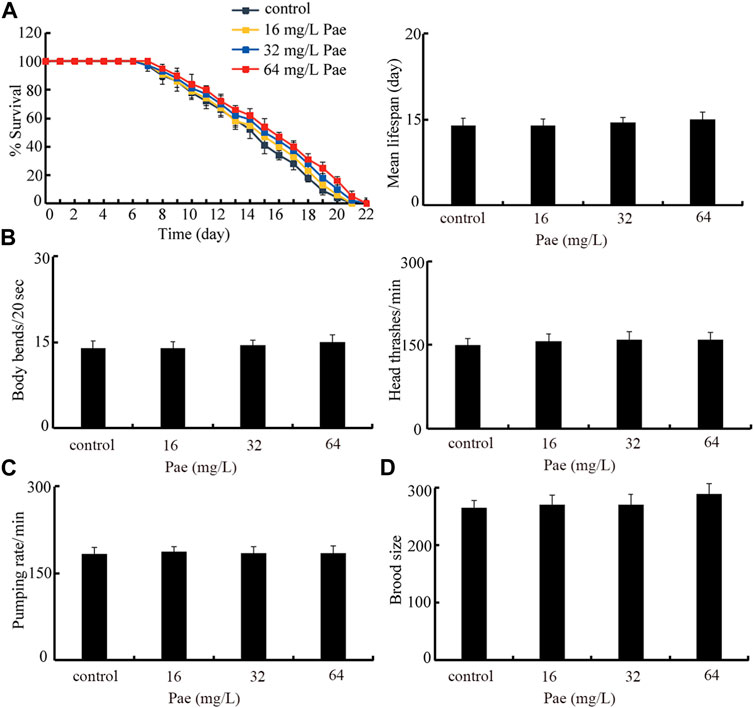
FIGURE 8. Safety assessment of 16–64 mg/L paeoniflorin on lifespan (A), locomotion behavior (B), pumping rate (C), and brood size (D). Pae, paeoniflorin.
Discussion
The paeoniflorin has multiple aspects of pharmacological effects, including anti-inflammation, neuroprotective effect, and anticancer (Xiang et al., 2020; Zhou et al., 2020; Hong et al., 2022). In C. elegans, paeoniflorin was first observed to have function in inhibiting age-onset Aβ proteotoxicity by partially regulating oxidative stress response (Ai et al., 2018). Recently, it was found that paeoniflorin treatment could attenuate polystyrene nanoparticle-induced damage on reproductive capacity and germline development (Hua et al., 2023a). In addition, paeoniflorin treatment was helpful for nematodes against Pseudomonas aeruginosa infection and biofilm formation (Wang et al., 2023a). In this study, we further observed that treatment with 16–64 mg/L paeoniflorin could inhibit the glucose toxicity in reducing lifespan in nematodes (Figure 1). Previous observations on the pharmacological effect of paeoniflorin treatment against glucose-induced oxidative injury partially support our finding (Yang et al., 2016). Our data suggested the novel pharmacological effect of paeoniflorin treatment against glucose toxicity.
In C. elegans, although several signaling pathways have been identified to be required for control of longevity (Lapierre and Hansen, 2012; Martins et al., 2016; Blackwell et al., 2019), the insulin signaling pathway plays the central role and is an evolutionarily conserved mechanism (Barbieri et al., 2003; Murphy and Hu, 2013). In this insulin signaling pathway, the activated insulin receptor DAF-2 suppresses the longevity by increasing downstream several kinases (AGE-1, AKT-1, and AKT-2) and inhibiting FOXO transcriptional factor DAF-16 (Braeckman and Vanfleteren, 2007). In glucose treated nematodes, we observed the increase in daf-2, age-1, akt-1, and akt-2 expressions and decrease in daf-16 expression (Figure 2), which suggested that the observed lifespan reduction by glucose treatment was associated with the activation of insulin signaling. Moreover, in the glucose treated nematodes, 16–64 mg/L paeoniflorin administration could further obviously decrease daf-2, age-1, akt-1, and akt-2 expressions and increase daf-16 expression (Figure 2). Meanwhile, Moreover, in the glucose treated nematodes, we also observed the increase in DAF-16::GFP expression after paeoniflorin administration (Figure 3). That is, the observed beneficial effect of paeoniflorin against glucose toxicity on lifespan was due to the suppression in signaling cascade of DAF-2-AGE-1-AKT-1/2 and the activation of DAF-16 in nematodes.
In glucose treated nematodes, the obvious increase in translocation of DAF-16::GFP into nucleus was observed (Figure 3). The increase in translocation of DAF-16::GFP into nucleus has been also detected in nematodes exposed to other stresses (such as simulated microgravity) or pollutants (such as graphene oxide) (Zhao et al., 2016; Kong et al., 2019), suggesting that the translocation of DAF-16::GFP into nucleus is a normally formed response to stresses or pollutants. That is, the stresses and toxicants with certain degree of toxicity can induce both the decrease in fluorescence intensity of DAF-16::GFP and the translocation of DAF-16::GFP into nucleus. Moreover, the following administration with 16–64 mg/L paeoniflorin could suppress this translocation of DAF-16::GFP into nucleus in glucose treated nematodes (Figure 3). This implied the important function of paeoniflorin against toxicity of stresses, including the glucose treatment.
The functional analysis demonstrated that the beneficial effect of paeoniflorin against glucose toxicity in reducing lifespan could be enhanced by RNAi of daf-2, age-1, akt-1, and akt-2, and inhibited by RNAi of daf-16 (Figure 4). These observations confirmed the functions of DAF-2, AGE-1, AKT-1/2, and DAF-16 in regulating the pharmacological effect of paeoniflorin in inhibiting glucose toxicity. The insulin signaling was also required for controlling the pharmacological effects of compounds or plant extracts in extending lifespan under normal condition (Wang et al., 2018; Zeng et al., 2021; Zhou et al., 2021). For example, the DAF-16/DAF-2 insulin signaling regulated the pharmacological effect of sulforaphane in promoting lifespan and healthspan (Qi et al., 2021). That is, under both the normal and stress conditions, the insulin signaling is required for the control of pharmacological effect of compounds in extending longevity in nematodes.
The genetic interaction analysis indicated that, in glucose treated nematodes, DAF-2 acted upstream of DAF-16 to regulate the pharmacological effect of paeoniflorin in extending lifespan (Figure 5). Similarly, DAF-2 could function upstream of DAF-16 to control pharmacological effect of luteolin to promote bacterial pathogen resistance (Xiao et al., 2023). DAF-2 could also act upstream of DAF-16 to regulate toxicity on lifespan induced by nanoplastic particle and simulated microgravity (Kong et al., 2019; Shao et al., 2019; Liu et al., 2022). Therefore, the signaling cascade of DAF-2-DAF-16 is a conserved mechanism for the regulation of both pharmacological effects of compounds and stress response in nematodes.
During the control of pharmacological effect of paeoniflorin, SOD-3/Mn-SOD was identified as the downstream target of DAF-16. Two lines of evidence were raised in this study. Firstly, the increase in SOD-3::GFP expression induced by 16–64 mg/L paeoniflorin in glucose treated nematodes could be suppressed by RNAi of daf-16 (Figure 6A). Secondly, the pharmacological effect of paeoniflorin in extending lifespan in glucose treated nematodes could be inhibited by RNAi of sod-3 (Figure 6B). Therefore, the DAF-16 could target to SOD-3 to affect the pharmacological effect of paeoniflorin on lifespan in glucose treated nematodes. SOD-3 was also identified to act as downstream target of DAF-16 during the control of pharmacological effect of sulforaphane and blueberry extract in extending the lifespan (Wang et al., 2018; Qi et al., 2021).
In this study, the molecular docking analysis indicated the binding potentials of paeoniflorin to DAF-2, AGE-1, AKT-1, and AKT-2 (Figure 7). That is, the administrated paeoniflorin can bind to both insulin receptor DAF-2 and its downstream three kinases, which provides an important signal amplification mechanism for paeoniflorin to exert its pharmacological effect in nematodes. Moreover, this further supported the molecular mechanism that paeoniflorin could extend lifespan in glucose treated nematodes by inhibiting the signaling cascade of DAF-2-AGE-1-AKT-1/2, which further activated the DAF-16 and its target of SOD-3.
Finally, with the aid of several endpoints including lifespan, locomotion behavior, pumping rate, and brood size, our results suggested the safety of paeoniflorin administration in nematodes (Figure 8). The safe property of paeoniflorin administration has also been shown in other reports (Li et al., 2016; Ngo et al., 2019). Paeoniflorin is one of the main bioactive ingredients in the blood for Xuebijing injection with the safety to be used to treat the sepsis in the clinical (Li et al., 2018; Xiao et al., 2018), which indirectly supports the safety of paeoniflorin administration.
Conclusion
Together, using the high-glucose model in C. elegans, we examined the potential of paeoniflorin administration against glucose toxicity in reducing lifespan. Our results demonstrated the potential of 16–64 mg/L paeoniflorin against glucose toxicity in reducing lifespan. This beneficial effect of paeoniflorin administration was associated with the inhibition of insulin signaling pathway reflected by the decrease in daf-2, age-1, akt-1, and akt-2 expressions and the increase in daf-16 expression. During the control of pharmacological effect of paeoniflorin, DAF-2 acted upstream of DAF-16. After pharmacological treatment, the DAF-16 further activated its target of SOD-3, suggesting the requirement of DAF-2-AGE-1-AKT-1/2-DAF-16-SOD-3 signaling cascade for formation of pharmacological effect of paeoniflorin in inhibiting glucose toxicity. In this signaling cascade, molecular docking analysis indicates the binding potential of paeoniflorin to DAF-2, AGE-1, AKT-1, and AKT-2. Our data highlights the potential of paeoniflorin administration in inhibiting toxicity of high glucose on longevity of organisms.
Data availability statement
The datasets presented in this study can be found in online repositories. The names of the repository/repositories and accession number(s) can be found in the article/Supplementary Material.
Author contributions
TL performed the experiments. ZZ and DW conceived the idea. DW wrote the manuscript. All authors contributed to the article and approved the submitted version.
Conflict of interest
The authors declare that the research was conducted in the absence of any commercial or financial relationships that could be construed as a potential conflict of interest.
Publisher’s note
All claims expressed in this article are solely those of the authors and do not necessarily represent those of their affiliated organizations, or those of the publisher, the editors and the reviewers. Any product that may be evaluated in this article, or claim that may be made by its manufacturer, is not guaranteed or endorsed by the publisher.
Supplementary material
The Supplementary Material for this article can be found online at: https://www.frontiersin.org/articles/10.3389/fphar.2023.1202379/full#supplementary-material
References
Ai, L., Yang, F., Song, J., Chen, Y., Xiao, L., Wang, Q., et al. (2018). Inhibition of Abeta proteotoxicity by paeoniflorin in Caenorhabditis elegans through regulation of oxidative and heat shock stress responses. Rejuvenation Res. 21, 304–312. doi:10.1089/rej.2017.1966
Aiello, P., Sharghi, M., Mansourkhani, S. M., Ardekan, A. P., Jouybari, L., Daraei, N., et al. (2019). Medicinal plants in the prevention and treatment of colon cancer. Oxid. Med. Cell. Longev. 2019, 2075614. doi:10.1155/2019/2075614
Baldwin, D., and Apel, J. (2013). Management of hyperglycemia in hospitalized patients with renal insufficiency or steroid-induced diabetes. Curr. Diab. Rep. 13, 114–120. doi:10.1007/s11892-012-0339-7
Barbieri, M., Bonafè, M., Franceschi, C., and Paolisso, G. (2003). Insulin/IGF-I-signaling pathway: An evolutionarily conserved mechanism of longevity from yeast to humans. Am. J. Physiol. Endocrinol. Metab. 285, E1064–E1071. doi:10.1152/ajpendo.00296.2003
Blackwell, T. K., Sewell, A. K., Wu, Z., and Han, M. (2019). TOR signaling in Caenorhabditis elegans development, metabolism, and aging. Genetics 213, 329–360. doi:10.1534/genetics.119.302504
Braeckman, B. P., and Vanfleteren, J. R. (2007). Genetic control of longevity in C. elegans. Exp. Gerontol. 42, 90–98. doi:10.1016/j.exger.2006.04.010
Braungart, E., Gerlach, M., Riederer, P., Baumeister, R., and Hoener, M. C. (2004). Caenorhabditis elegans MPP + model of Parkinson's disease for high-throughput drug screenings. Neurodegener. Dis. 1, 175–183. doi:10.1159/000080983
Brenner, S. (1974). The genetics of Caenorhabditis elegans. Genetics 77, 71–94. doi:10.1093/genetics/77.1.71
Collins, J. J., Evason, K., and Kornfeld, K. (2006). Pharmacology of delayed aging and extended lifespan of Caenorhabditis elegans. Exp. Gerontol. 41, 1032–1039. doi:10.1016/j.exger.2006.06.038
Deusing, D. J., Beyrer, M., Fitzenberger, E., and Wenzel, U. (2015a). Carnitine protects the nematode Caenorhabditis elegans from glucose-induced reduction of survival depending on the nuclear hormone receptor DAF-12. Biochem. Biophys. Res. Commu. 460, 747–752. doi:10.1016/j.bbrc.2015.03.101
Deusing, D. J., Winter, S., Kler, A., Kriesl, E., Bonnlander, B., Wenzel, U., et al. (2015b). A catechin-enriched green rea extract prevents glucose-induced survival reduction in Caenorhabditis elegans through sir-2.1 an uba-1 dependent hormesis. Fitoterapai 102, 163–170. doi:10.1016/j.fitote.2015.03.005
Fitzenberger, E., Boll, M., and Wenzel, U. (2013). Impairment of the proteasome is crucial for glucose-induced lifespan reduction in the mev-1 mutant of Caenorhabditis elegans. Biochim. Biophys. Acta 1832, 565–573. doi:10.1016/j.bbadis.2013.01.012
Fitzenberger, E., Deusing, D. J., Wittkop, A., Kler, A., Kriesl, E., Bonnlander, B., et al. (2014). Effect of plant extracts on the reversal of glucose-induced impairment of stress-resistance in Caenorhabditis elegans. Plant Foods Hum. mutr. 69, 78–84. doi:10.1007/s11130-013-0399-0
Griffin, E. F., Caldwell, K. A., and Caldwell, G. A. (2017). Genetic and pharmacological discovery for Alzheimer's disease using Caenorhabditis elegans. ACS Chem. Neurosci. 8, 2596–2606. doi:10.1021/acschemneuro.7b00361
Gunst, J., De Bruyn, A., and Van den Berghe, G. (2019). Glucose control in the ICU. Curr. Opin. Anaesthesiol. 32, 156–162. doi:10.1097/ACO.0000000000000706
Hong, H., Lu, X., Wu, C., Chen, J., Chen, C., Zhang, J., et al. (2022). A review for the pharmacological effects of paeoniflorin in the nervous system. Front. Pharmacol. 13, 898955. doi:10.3389/fphar.2022.898955
Hua, X., Cao, C., Zhang, L., and Wang, D.-Y. (2023b). Activation of FGF signal in germline mediates transgenerational toxicity of polystyrene nanoparticles at predicted environmental concentrations in Caenorhabditis elegans. J. Hazard. Mat. 451, 131174. doi:10.1016/j.jhazmat.2023.131174
Hua, X., Feng, X., Hua, Y.-S., and Wang, D.-Y. (2023a). Paeoniflorin attenuates polystyrene nanoparticle-induced reduction in reproductive capacity and increase in germline apoptosis through suppressing DNA damage checkpoints in Caenorhabditis elegans. Sci. Total Environ. 871, 162189. doi:10.1016/j.scitotenv.2023.162189
Hua, X., Feng, X., Liang, G.-Y., Chao, J., and Wang, D.-Y. (2023d). Exposure to 6-PPD quinone at environmentally relevant concentrations causes abnormal locomotion behaviors and neurodegeneration in Caenorhabditis elegans. Environ. Sci. Technol. 57, 4940–4950. doi:10.1021/acs.est.2c08644
Hua, X., Feng, X., Liang, G.-Y., Chao, J., and Wang, D.-Y. (2023e). Long-term exposure to 6-PPD quinone reduces reproductive capacity by enhancing germline apoptosis associated with activation of both DNA damage and cell corpse engulfment in Caenorhabditis elegans. J. Hazard. Mat. 454, 131495. doi:10.1016/j.jhazmat.2023.131495
Hua, X., Feng, X., Liang, G.-Y., Chao, J., and Wang, D.-Y. (2023c). Long-term exposure to tire-derived 6-PPD quinone causes intestinal toxicity by affecting functional state of intestinal barrier in Caenorhabditis elegans. Sci. Total Environ. 861, 160591. doi:10.1016/j.scitotenv.2022.160591
Hua, X., Zhao, Y., Yuan, Y.-J., Zhang, L., Bian, Q., and Wang, D.-Y. (2022). Nanoplastics cause transgenerational toxicity through inhibiting germline microRNA mir-38 in C. elegans. J. Hazard. Mat. 437, 129302. doi:10.1016/j.jhazmat.2022.129302
Jia, G., Whaley-Connell, A., and Sowers, J. R. (2018). Diabetic cardiomyopathy: A hyperglycaemia- and insulin-resistance-induced heart disease. Diabetologia 61, 21–28. doi:10.1007/s00125-017-4390-4
Kong, Y., Liu, H.-L., Li, W.-J., and Wang, D.-Y. (2019). Intestine-specific activity of insulin signaling pathway in response to microgravity stress in Caenorhabditis elegans. Caenorhabditis Elegans. Biochem. Biophys. Res. Commun. 517, 278–284. doi:10.1016/j.bbrc.2019.07.067
Lapierre, L. R., and Hansen, M. (2012). Lessons from C. elegans: Signaling pathways for longevity. Trends Endocrinol. Metab. 23, 637–644. doi:10.1016/j.tem.2012.07.007
Li, C., Wang, P., Zhang, L., Li, M., Lei, X., Liu, S., et al. (2018). Efficacy and safety of xuebijing injection (a Chinese patent) for sepsis: A meta-analysis of randomized controlled trials. J. Ethnopharmacol. 224, 512–521. doi:10.1016/j.jep.2018.05.043
Li, X., Shi, F., Zhang, R., Sun, C., Gong, C., Jian, L., et al. (2016). Pharmacokinetics, safety, and tolerability of amygdalin and paeoniflorin after single and multiple intravenous infusions of Huoxue-Tongluo lyophilized powder for injection in healthy Chinese volunteers. Clin. Ther. 38, 327–337. doi:10.1016/j.clinthera.2015.12.005
Lin, K., Hsin, H., Libina, N., and Kenyon, C. (2001). Regulation of the Caenorhabditis elegans longevity protein DAF-16 by insulin/IGF-1 and germline signaling. Nat. Genet. 28, 139–145. doi:10.1038/88850
Liu, H.-L., Zhao, Y.-L., Hua, X., and Wang, D.-Y. (2022). Induction of transgenerational toxicity is associated with the activated germline insulin signals in nematodes exposed to nanoplastic at predicted environmental concentrations. Ecotoxicol. Environ. Saf. 243, 114022. doi:10.1016/j.ecoenv.2022.114022
Madende, M., Albertyn, J., Sebolai, O., and Pohl, C. H. (2020). Caenorhabditis elegans as a model animal for investigating fungal pathogenesis. Med. Microbiol. Immunol. 209, 1–13. doi:10.1007/s00430-019-00635-4
Martins, R., Lithgow, G. J., and Link, W. (2016). Long live FOXO: Unraveling the role of FOXO proteins in aging and longevity. Aging Cell 15, 196–207. doi:10.1111/acel.12427
Monnier, L., Colette, C., and Owens, D. (2012). The glycemic triumvirate and diabetic complications: Is the whole greater than the sum of its component parts? Diabetes Res. Clin. Pract. 95, 303–311. doi:10.1016/j.diabres.2011.10.014
Murphy, C. T., and Hu, P. J. (2013). Insulin/insulin-like growth factor signaling in C. elegans. WormBook 26, 1–43. doi:10.1895/wormbook.1.164.1
Ngo, T., Kim, K., Bian, Y., Noh, H., Lim, K. M., Chung, J. H., et al. (2019). Antithrombotic effects of paeoniflorin from Paeonia suffruticosa by selective inhibition on shear stress-induced platelet aggregation. Int. J. Mol. Sci. 20, 5040. doi:10.3390/ijms20205040
O'Reilly, L. P., Luke, C. J., Perlmutter, D. H., Silverman, G. A., and Pak, S. C. (2014). C. elegans in high-throughput drug discovery. Adv. Drug Deliv. Rev. 69-70, 247–253. doi:10.1016/j.addr.2013.12.001
Okoro, N. O., Odiba, A. S., Osadebe, P. O., Omeje, E. O., Liao, G., Fang, W., et al. (2021). Bioactive phytochemicals with anti-aging and lifespan extending potentials in Caenorhabditis elegans. Molecules 26, 7323. doi:10.3390/molecules26237323
Parker, J. A., Holbert, S., Lambert, E., Abderrahmane, S., and Néri, C. (2004). Genetic and pharmacological suppression of polyglutamine-dependent neuronal dysfunction in Caenorhabditis elegans. J. Mol. Neurosci. 23, 61–68. doi:10.1385/JMN:23:1-2:061
Pir, G. J., Choudhary, B., and Mandelkow, E. (2017). Caenorhabditis elegans models of tauopathy. FASEB J. 31, 5137–5148. doi:10.1096/fj.201701007
Qi, Z., Ji, H., Le, M., Li, H., Wieland, A., Bauer, S., et al. (2021). Sulforaphane promotes C. elegans longevity and healthspan via DAF-16/DAF-2 insulin/IGF-1 signaling. Aging 13, 1649–1670. doi:10.18632/aging.202512
Ramel, S., and Rao, R. (2020). Hyperglycemia in extremely preterm infants. Neoreviews 21, e89–e97. doi:10.1542/neo.21-2-e89
Rual, J. F., Ceron, J., Koreth, J., Hao, T., Nicot, A. S., Hirozane-Kishikawa, T., et al. (2004). Toward improving Caenorhabditis elegans phenome mapping with an ORFeome-based RNAi library. Genome Res. 14, 2162–2168. doi:10.1101/gr.2505604
Sahardi, N. F. N. M., and Makpol, S. (2019). Ginger (Zingiber officinale Roscoe) in the prevention of ageing and degenerative diseases: Review of current evidence. Evid.-Based Complement. Altern. Med. 2019, 5054395. doi:10.1155/2019/5054395
Shanmugam, G., Mohankumar, A., Kalaiselvi, D., Nivitha, S., Murugesh, E., Shanmughavel, P., et al. (2017). Diosgenin a phytosterol substitute for cholesterol, prolongs the lifespan and mitigates glucose toxicity via DAF-16/FOXO and GST-4 in Caenorhabditis elegans. Biomed. Pharmacother. 95, 1693–1703. doi:10.1016/j.biopha.2017.09.096
Shao, H.-M., Han, Z.-Y., Krasteva, N., and Wang, D.-Y. (2019). Identification of signaling cascade in the insulin signaling pathway in response to nanopolystyrene particles. Nanotoxicology 13, 174–188. doi:10.1080/17435390.2018.1530395
Shao, Y., Xu, X., Li, Y., Qi, X., Wang, K., Wu, Y., et al. (2016). Paeoniflorin inhibits high glucose-induced macrophage activation through TLR2-dependent signal pathways. J. Ethnopharmacol. 193, 377–386. doi:10.1016/j.jep.2016.08.035
Stumvoll, M., Goldstein, B. J., and van Haeften, T. W. (2005). Type 2 diabetes: Principles of pathogenesis and therapy. Lancet 365, 1333–1346. doi:10.1016/S0140-6736(05)61032-X
Taylor, S. I., Yazdi, Z. S., and Beitelshees, A. L. (2021). Pharmacological treatment of hyperglycemia in type 2 diabetes. J. Clin. Invest. 131, e142243. doi:10.1172/JCI142243
Wang, D.-Y. (2020). Exposure toxicology in Caenorhabditis elegans. Springer Nature Singapore Pte Ltd.
Wang, D.-Y. (2019). Molecular toxicology in Caenorhabditis elegans. Springer Nature Singapore Pte Ltd.
Wang, H., Liu, J., Li, T., and Liu, R. (2018). Blueberry extract promotes longevity and stress tolerance via DAF-16 in Caenorhabditis elegans. Food Funct. 9, 5273–5282. doi:10.1039/c8fo01680a
Wang, Y.-X., Yuan, X.-A., Zhou, R., Bu, Y.-Q., and Wang, D.-Y. (2023b). Combinational exposure to hydroxyatrazine increases neurotoxicity of polystyrene nanoparticles on Caenorhabditis elegans. Sci. Total Environ. 880, 163283. doi:10.1016/j.scitotenv.2023.163283
Wang, Y.-X., Zhang, L., Yuan, X.-A., and Wang, D.-Y. (2023a). Treatment with paeoniflorin increases lifespan of Pseudomonas aeruginosa infected Caenorhabditis elegans by inhibiting bacterial accumulation in intestinal lumen and biofilm formation. Front. Pharmacol. 14, 1114219. doi:10.3389/fphar.2023.1114219
Watts, J. L., and Ristow, M. (2017). Lipid and carbohydrate metabolism in Caenorhabditis elegans. Genetics 207, 413–446. doi:10.1534/genetics.117.300106
Wu, Q.-L., Zhi, L.-T., Qu, Y.-Y., and Wang, D.-Y. (2016). Quantum dots increased fat storage in intestine of Caenorhabditis elegans by influencing molecular basis for fatty acid metabolism. Nanomedicine Nanotechnol. Biol. Med. 12, 1175–1184. doi:10.1016/j.nano.2016.01.016
Xiang, Y., Zhang, Q., Wei, S., Huang, C., Li, Z., and Gao, Y. (2020). Paeoniflorin: A monoterpene glycoside from plants of paeoniaceae family with diverse anticancer activities. J. Pharm. Pharmacol. 72, 483–495. doi:10.1111/jphp.13204
Xiao, S., Luo, L., Liu, X., Zhou, Y., Liu, H., and Huang, Z. (2018). Curative efficacy and safety of traditional Chinese medicine xuebijing injections combined with ulinastatin for treating sepsis in the Chinese population: A meta-analysis. Medicine 97, e10971. doi:10.1097/MD.0000000000010971
Xiao, Y., Zhang, L., Zhu, X., Qin, Y., Yu, C., Jiang, N., et al. (2023). Luteolin promotes pathogen resistance in Caenorhabditis elegans via DAF-2/DAF-16 insulin-like signaling pathway. Int. Immunopharmacol. 115, 109679. doi:10.1016/j.intimp.2023.109679
Xu, R.-R., Hua, X., Rui, Q., and Wang, D.-Y. (2022a). Alteration in Wnt signaling mediates induction of transgenerational toxicity of polystyrene nanoplastics in C. elegans. NanoImpact 28, 100425. doi:10.1016/j.impact.2022.100425
Xu, R.-R., Hua, X., Rui, Q., and Wang, D.-Y. (2022b). Polystyrene nanoparticles caused dynamic alteration in mitochondrial unfolded protein response from parents to the offspring in C. elegans. Chemosphere 308, 136154. doi:10.1016/j.chemosphere.2022.136154
Yan, F., Chen, X., and Zheng, X. (2017a). Protective effect of mulberry fruit anthocyanin on human hepatocyte cells (LO2) and Caenorhabditis elegans under hyperglycemic conditions. Food Res. Int. 102, 213–224. doi:10.1016/j.foodres.2017.10.009
Yan, F., Yang, Y., Yu, L., and Zheng, X. (2017b). Effect of C-glycosides from Apios americana leaves against oxidative stress during hyperglycemia through regulating mitogen-activated protein kinases and nuclear factor erythroid 2-related factor 2. J. Agric. Food Chem. 65, 7457–7466. doi:10.1021/acs.jafc.7b03163
Yang, X., Yao, W., Shi, H., Liu, H., Li, Y., Gao, Y., et al. (2016). Paeoniflorin protects Schwann cells against high glucose induced oxidative injury by activating Nrf2/ARE pathway and inhibiting apoptosis. J. Ethnopharmacol. 185, 361–369. doi:10.1016/j.jep.2016.03.031
Zeng, W., Tan, L., Han, C., Zheng, Z., Wu, G., Luo, H., et al. (2021). Trigonelline extends the lifespan of C. elegans and delays the progression of age-related diseases by activating AMPK, DAF-16, and HSF-1. Oxid. Med. Cell. Longev. 2021, 7656834. doi:10.1155/2021/7656834
Zhang, L., Wang, S.-T., Zhao, Y.-L., Nurdebek, B., Bu, Y.-Q., and Wang, D.-Y. (2022b). Long-term exposure to polystyrene nanoparticles causes transgenerational toxicity by affecting the function and expression of MEV-1 and DAF-2 signals in Caenorhabditis elegans. Caenorhabditis elegans NanoImpact 26, 100403. doi:10.1016/j.impact.2022.100403
Zhang, L., Wang, Y.-X., Cao, C., Zhu, Y.-K., Huang, W., Yang, Y., et al. (2022a). Beneficial effect of Xuebijing against Pseudomonas aeruginosa infection in Caenorhabditis elegans. Front. Pharmacol. 13, 949608. doi:10.3389/fphar.2022.949608
Zhang, X., Zhai, Y., Yuan, J., and Hu, Y. (2019). New insights into paeoniaceae used as medicinal plants in China. Sci. Rep. 9, 18469. doi:10.1038/s41598-019-54863-y
Zhang, X., Zuo, J., Wang, Y., Duan, H., Yuan, J., and Hu, Y. (2022c). Paeoniflorin in Paeoniaceae: Distribution, influencing factors, and biosynthesis. Front. Plant Sci. 13, 980854. doi:10.3389/fpls.2022.980854
Zhao, Y.-L., Chen, J.-Y., Wang, R., Pu, X.-X., and Wang, D.-Y. (2023a). A review of transgenerational and multigenerational toxicology in the in vivo model animal Caenorhabditis elegans. J. Appl. Toxicol. 43, 122–145. doi:10.1002/jat.4360
Zhao, Y.-Y., Hua, X., Rui, Q., and Wang, D.-Y. (2023b). Exposure to multi-walled carbon nanotubes causes suppression in octopamine signal associated with transgenerational toxicity induction in C. elegans. Chemosphere 318, 137986. doi:10.1016/j.chemosphere.2023.137986
Zhao, Y.-Y., Xu, R.-R., Hua, X., Rui, Q., and Wang, D.-Y. (2022b). Multi-walled carbon nanotubes induce transgenerational toxicity associated with activation of germline long non-coding RNA linc-7 in C. elegans. Chemosphere 301, 134687. doi:10.1016/j.chemosphere.2022.134687
Zhao, Y., Hua, X., Bian, Q., and Wang, D.-Y. (2022a). Nanoplastic exposure at predicted environmental concentrations induces activation of germline Ephrin signal associated with toxicity formation in the Caenorhabditis elegans offspring. Toxics 10, 699. doi:10.3390/toxics10110699
Zhao, Y., Yang, R., Rui, Q., and Wang, D. (2016). Intestinal insulin signaling encodes two different molecular mechanisms for the shortened longevity induced by graphene oxide in Caenorhabditis elegans. Sci. Rep. 6, 24024. doi:10.1038/srep24024
Zheng, S., Chiu, H., Boudreau, J., Papanicolaou, T., Bendena, W., and Chin-Sang, I. (2018). A functional study of all 40 Caenorhabditis elegans insulin-like peptides. J. Biol. Chem. 293, 16912–16922. doi:10.1074/jbc.RA118.004542
Zhou, L., Liu, J., Bu, L., Liao, D., Cheng, S., and Zheng, X. (2021). Curcumin acetylsalicylate extends the lifespan of Caenorhabditis elegans. Molecules 26, 6609. doi:10.3390/molecules26216609
Zhou, Y., Gong, X., Zhang, H., and Peng, C. (2020). A review on the pharmacokinetics of paeoniflorin and its anti-inflammatory and immunomodulatory effects. Biomed. Pharmacother. 130, 110505. doi:10.1016/j.biopha.2020.110505
Zhu, S., Liu, B., Hao, M., Fan, Y., Qian, C., Teng, P., et al. (2017). Paeoniflorin suppressed high glucose-induced retinal microglia MMP-9 expression and inflammatory response via inhibition of TLR4/NF-κB pathway through upregulation of SOCS3 in diabetic retinopathy. Inflammation 40, 1475–1486. doi:10.1007/s10753-017-0571-z
Keywords: paeoniflorin, glucose toxicity, C. elegans, pharmacological, insulin signaling
Citation: Liu T, Zhuang Z and Wang D (2023) Paeoniflorin mitigates high glucose-induced lifespan reduction by inhibiting insulin signaling in Caenorhabditis elegans. Front. Pharmacol. 14:1202379. doi: 10.3389/fphar.2023.1202379
Received: 08 April 2023; Accepted: 12 June 2023;
Published: 19 June 2023.
Edited by:
Rong-Rong He, Jinan University, ChinaReviewed by:
Qinli Ruan, Nanjing University of Chinese Medicine, ChinaKang Qiao, Shandong Agricultural University, China
Yonggang Liu, Beijing University of Chinese Medicine, China
Copyright © 2023 Liu, Zhuang and Wang. This is an open-access article distributed under the terms of the Creative Commons Attribution License (CC BY). The use, distribution or reproduction in other forums is permitted, provided the original author(s) and the copyright owner(s) are credited and that the original publication in this journal is cited, in accordance with accepted academic practice. No use, distribution or reproduction is permitted which does not comply with these terms.
*Correspondence: Ziheng Zhuang, Y2N6dXp6aEAxNjMuY29t; Dayong Wang, ZGF5b25nd0BzZXUuZWR1LmNu