- 1Department of Anesthesiology, State Key Laboratory of Oncology in Southern China, Sun Yat-sen University Cancer Center, Collaborative Innovation Center for Cancer Medicine, Guangzhou, China
- 2Department of Anesthesiology, The First Affiliated Hospital, Sun Yat-sen University, Guangzhou, China
Background: Opioid-induced hyperalgesia (OIH) is an adverse event of prolonged opioid use that increases pain intensity. The optimal drug to prevent these adverse effects is still unknown. We aimed to conduct a network meta-analysis to compare different pharmacological interventions for preventing the increase in postoperative pain intensity caused by OIH.
Methods: Several databases were searched independently for randomized controlled trials (RCTs) comparing various pharmacological interventions to prevent OIH. The primary outcomes were postoperative pain intensity at rest after 24 h and the incidence of postoperative nausea and vomiting (PONV). Secondary outcomes included pain threshold at 24 h after surgery, total morphine consumption over 24 h, time to first postoperative analgesic requirement, and shivering incidence.
Results: In total, 33 RCTs with 1711 patients were identified. In terms of postoperative pain intensity, amantadine, magnesium sulphate, pregabalin, dexmedetomidine, ibuprofen, flurbiprofen plus dexmedetomidine, parecoxib, parecoxib plus dexmedetomidine, and S (+)-ketamine plus methadone were all associated with milder pain intensity than placebo, with amantadine being the most effective (SUCRA values = 96.2). Regarding PONV incidence, intervention with dexmedetomidine or flurbiprofen plus dexmedetomidine resulted in a lower incidence than placebo, with dexmedetomidine showing the best result (SUCRA values = 90.3).
Conclusion: Amantadine was identified as the best in controlling postoperative pain intensity and non-inferior to placebo in the incidence of PONV. Dexmedetomidine was the only intervention that outperformed placebo in all indicators.
Clinical Trial Registration: https://www.crd.york.ac. uk/prospero/display_record.php?, CRD42021225361.
What is already known about this subject?
OIH is highly prevalent in surgery patients, contributing to various undesirable outcomes, such as more severe postoperative pain, increased opioid demand, and a high incidence of side effects.
In clinical routine, multiple medications with various mechanisms were proven to prevent the increase in postoperative pain caused by OIH. However, the comparative effects of different pharmacological interventions are urgently needed for a better guideline for the individualized anesthesia protocols.
What this study adds
Amantadine, magnesium sulphate, pregabalin, dexmedetomidine, ibuprofen, flurbiprofen plus dexmedetomidine, parecoxib, parecoxib plus dexmedetomidine, and S (+)-ketamine plus methadone all have statistically significantly lower pain intensity than placebo. Amantadine was the most effective compared to placebo but failed to demonstrate superiority in the incidence of PONV.
Dexmedetomidine is not the best option, but it is the most well-balanced choice because it is the only intervention that outperforms placebo in all indicators.
1 Introduction
Opioids are the most commonly used analgesics during the perioperative period as a part of balanced anesthesia. Timely opioid administration during surgery reduces the need for general anesthetics, resulting in faster recovery (Lang et al., 1996), and post-surgery patient-controlled opioid analgesia improves patient comfort and satisfaction (McNicol et al., 2015). However, a state of nociceptive sensitization with reduction in nociceptive thresholds and paradoxical increase in pain after exposure to opioids (Koppert et al., 2003), referred to as opioid-induced hyperalgesia (OIH), have been demonstrated in animal models (Minville et al., 2010), human volunteers (Vinik and Kissin, 1998) and surgical patients (Fletcher and Martinez, 2014). Patients who experience more severe postoperative pain due to nociceptive sensitization may be obliged to accept more opioids unless alternatives are considered (He et al., 2020). Furthermore, opioid-related adverse drug events have been associated with increased inpatient mortality, prolonged stay, and a high cost of hospitalization (Shafi et al., 2018).
Although the precise molecular mechanism underlying OIH is unknown, it is widely assumed to be triggered by neuroplastic changes in the peripheral and central nervous systems (Lee et al., 2011). Previous research has demonstrated that opioids contribute to the occurrence of OIH by inhibiting glutamate recapture and inducing production of pro-inflammatory molecules (Roeckel et al., 2016). The inhibition of the glutamate transporter leads to increased synaptic concentrations of glutamate, which, in turn, activates the N-methyl-D-aspartate (NMDA) receptor, thus triggering OIH (Antal et al., 2008; Arout et al., 2015). Previous electrophysiological studies also identified the rapid and persistent upregulation of NMDA receptor function by clinically relevant concentrations of remifentanil, mirroring the potential target for the pathologic activation of NMDA receptor in the intervention of OIH (Guntz et al., 2005; Zhao and Joo Daisy, 2008). Furthermore, neuroinflammation mediated by opioid-triggered release of pro-inflammatory molecules and the activation of glial cells can sensitize pain pathways, lower pain thresholds, and contribute to the development of OIH (Grace et al., 2015). Additionally, the interactions between mu and delta opioid receptors (Beaudry et al., 2015),α-2 adrenoreceptors (Mercieri et al., 2017), neurokinin-1 receptor mediated transmission (Vera-Portocarrero et al., 2007), and spinal dynorphin expression (Vanderah et al., 2001) also have been reported to play a role in the development and maintenance of OIH.
In light of these findings, clinical investigators mainly focused on manipulating the glutaminergic system through modulation of the NMDA receptor and blocking the neuroinflammation to prevent the occurrence and development of OIH. Various interventions have been explored, including NMDA receptor antagonists (amantadine (Snijdelaar Dirk et al., 2004), magnesium sulphate (Ryu et al., 2008), methadone (Tognoli et al., 2020), and ketamine (Leal et al., 2015)), non-steroidal anti-inflammatory drugs (NSAIDs) (Ibuprofen (Koo et al., 2016), flurbiprofen (Zhang et al., 2016), and parecoxib (Du et al., 2019)), opioid receptor antagonist (naloxone (Du et al., 2019)), agonist-antagonist opioid analgesics (nalbuphine (Hu et al., 2020) and buprenorphine (Mercieri et al., 2017)) and α-2 adrenoceptor agonist (dexmedetomidine (Wu et al., 2021)). These interventions, with different mechanisms of action, have been shown the potential in reduce pain intensity and the need for postoperative analgesics due to OIH. Regretfully, clinical routines are still debatable about the optimal intervention strategy to prevent the increase in postoperative pain intensity caused by OIH due to small sample sizes and varying medication dosages in existing literature (Wu et al., 2015). Importantly, the relative effects of different types of medications remain unknown.
Given these uncertainties, we conducted a systematic review and network meta-analysis of various pharmacological interventions to prevent the increase in postoperative pain intensity caused by OIH in adults following general anesthesia, hoping better to guide clinical practice for more individualized general anesthesia protocols.
2 Methods
This network meta-analysis was registered on https://www.crd.york.ac.uk/PROSPERO. The registration number is CRD42021225361.
2.1 Search strategy and selection criteria
According to PRISMA Extension Statement for Reporting of Systematic Reviews Incorporating Network Meta-analyses (Hutton et al., 2015), MEDLINE, Embase, The Cochrane Central Register of Controlled Trials, and Web of Science were searched in English with language restrictions. The search strategy combined free text words and medical subject heading (MeSH) terms to maximize the results. The following keywords were used in the search: 1) opioid, 2) hyperalgesia, and 3) magnesium, naloxone, buprenorphine, ketamine, dexmedetomidine, butorphanol, propofol, flurbiprofen, morphine, methadone, lornoxicam, nitrous oxide, parecoxib, clonidine, amantadine, nalbuphine, paracetamol, pregabalin, nefopam, acetazolamide.
2.2 Selection of studies and data extraction
Two investigators (WJX and HFC) reviewed all titles, abstracts, and full texts sequentially. Finally, eligible trials were identified, and data on eligibility, quality, and outcomes were independently retrieved. Disagreements between the two reviewers on eligibility were resolved through mutual discussion. A third reviewer (YCL) was requested for the final decision when needed. Relevant data were extracted from eligible literature using a standard extraction formula and cross-checked.
Retrieved data included: 1) first author, year of publication, study location, study design, sample size, gender, age, American Society of Anesthesiologists (ASA) status, types of surgery, premedication, anesthesia maintenance, intervention description, control description, dose of opioid, postoperative analgesic strategies, and 2) pain intensity in the form of the various pain scores during the 0 to 24 postoperative hours, pain threshold or normalized area of hyperalgesia during the 0 to 48 postoperative hours, cumulative morphine consumption at 24 h after surgery, time to first rescue analgesic, and incidence of postoperative opioid-related side-effects, such as postoperative nausea and vomiting (PONV), shivering, dizziness and hypotension. Dichotomous data were extracted as the number of patients (%). Continuous data were extracted in the form of mean ± standard deviation (SD).
We tried to contact the author via e-mail twice when the target data in the article were incomplete, but no responses were received. Range and median estimation (Grace et al., 2015) were used to convert the data when the standard deviation was missing.
2.3 Type of outcome measures
Our primary outcomes were postoperative pain intensity at rest after 24 h and the incidence of PONV. Postoperative pain intensity was measured using pain scores scaling from 0 (no pain) to 10 (worst possible pain). Intensity scores reported on a visual analogue scale (VAS: 0: no pain to 100: worst possible pain) were transformed to a 0–10 scale. PONV, the most common adverse event, contributes to the highly distressing experience and severe patient dissatisfaction (Myles et al., 2000; Eberhart et al., 2002), with an incidence as high as 80% in high-risk cohorts (Apfel Christian et al., 1999).
Secondary outcomes include pain thresholds at 24 h after surgery, cumulative morphine consumption over the 24 h period, time to first postoperative analgesic requirement, and shivering incidence.
2.4 Assessment of risk of bias
Two investigators (WJX and HFC) read the eligible articles independently. They assessed their methodological validity using the Cochrane Collaboration’s tool of Review Manager software (RevMan version 5.4, Cochrane Community, London, England) for evaluating the risk of bias in randomized controlled trials (RCTs), and disagreements were resolved through discussion (Higgins et al., 2011). The tool includes seven items that describe random sequence generation, allocation concealment, participants and personnel blinding, outcome assessment, blinding, incomplete outcome data, selective reporting, and other biases. Each item was assigned a risk of material bias judgment of high, low, or unclear.
2.5 Statistical analysis
For dichotomous outcomes, odds ratios (ORs) with 95% confidence intervals (CIs) were calculated, as were standardized mean differences (SMDs) or mean differences (MDs) with 95% CIs for continuous outcomes.
This network meta-analysis was performed within a frequentist framework using the STATA 16.0 (StataCorp, Texas, United States) command ‘mvmeta’ (White, 2011). First, a network geometry plot for each outcome was created, which provided a visual and concise description of the relationship between pairs of interventions (Chaimani et al., 2013). Second, the node-splitting method and loop inconsistency mode were used to assess the statistical consistency. p-value ≥0.05 or 95% CI for each closed-loop containing 0 means direct and indirect comparisons were considered consistent (van Valkenhoef et al., 2016). Third, a comparison-adjusted funnel plot was used to assess publication bias. A symmetrical graph indicated that publication bias had a low influence, whereas an asymmetric graph indicated possible publication bias. Finally, the forest plot was used to report the results of the mixed comparison of interventions and placebo, and the league table was used to illustrate all head-to-head comparisons. We assumed that 95% of CIs that did not contain 0 were statistically significant for SMDs or MDs, and those that did not have 1 were statistically significant for ORs. The two-dimensional graph is prepared to visualize comprehensive drug for placebo comparisons. The point in the lower-left portion of the coordinate system that does not intersect with the dark grey dashed line indicates that this pharmacological intervention outperforms the placebo regarding postoperative pain intensity and PONV incidence. Furthermore, the ranking probabilities of all interventions at each possible intervention rank were estimated (Chaimani et al., 2013). The treatment hierarchy was summarized and reported as the surface under the cumulative ranking curve (SUCRA) (Chaimani et al., 2013) the ranking probabilities. The higher the SUCRA value, the higher the rank of the treatment outcomes.
2.6 Inclusion and exclusion criteria
RCTs that met the following criteria were considered eligible: 1) anesthesia was induced and maintained with opioids; 2) pharmacological interventions were administered to patients at any dose before or during the operative period; and 3) pharmacological interventions were compared to the placebo.
Articles were excluded based on the following criteria: 1) combination with regional nerve block during the anesthesia induction or maintenance period, and 2) data from healthy volunteer or pediatric studies, abstracts, letters, or reviews.
3 Results
3.1 Study selection and characteristics
We identified 1,602 potentially relevant studies in total. After adjusting for duplicates and reviewing the title/abstract, the remaining 39 full-text manuscripts were reviewed. Following the study protocol, six trials were excluded due to a lack of outcome of interest (n = 4) and a combination with a regional nerve block (n = 2). In total, 33 RCTs with a total of 1711 patients were identified. Figure 1 depicts the process of literature selection.
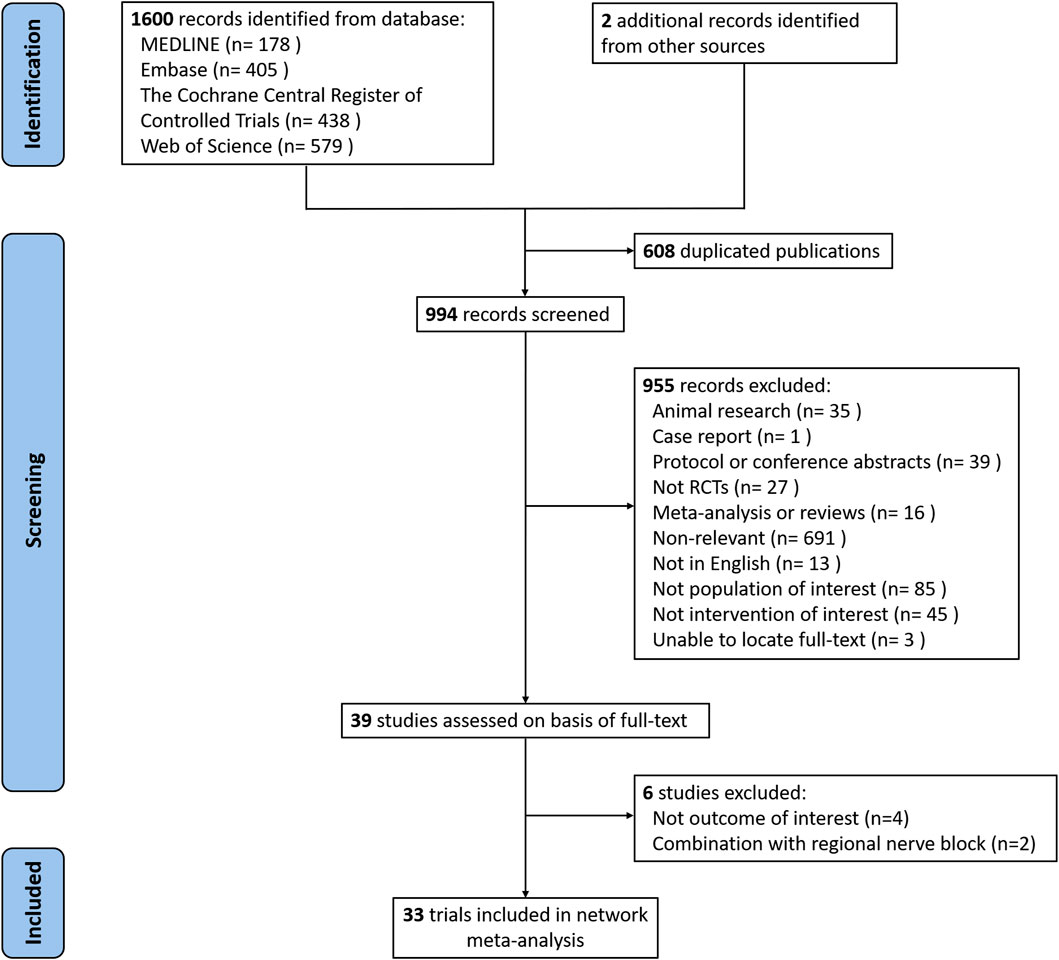
FIGURE 1. Flow chart of search strategy to identify the eligible randomized controlled trials (RCTs).
A total of 960 subjects were randomly assigned to pharmacological intervention and 751 to placebo. The included RCTs were published between 2002 and 2020 and included orthopedic (n = 2), urinary (n = 4), abdominal (n = 10), gynecologic (n = 9), thyroid surgery (n = 5), thoracic (n = 1) and ear-nose-throat surgery (n = 2). Table 1 describes the basic characteristics of the enrolled studies.
3.2 Risk of bias assessment
Supplementary Appendix S2 contains the details for the risk of bias assessment. The random sequence generation was specified in 24 trials (72.7%). Although 18 trials (54.5%) reported allocation concealment, one trial had a high risk of bias. Only one trial did not use blinding methods. Eight trials (24.2%) had selective reporting. No trials were found to be at high risk of bias due to incomplete outcome data and other bias. Overall, the included studies were of relatively high quality.
3.3 Network geometry of eligible comparisons
The network geometry plot (Figure 2) represents the network of eligible comparisons for postoperative pain intensity at rest at 24 h (A) and the incidence of PONV (B). The postoperative pain intensity at rest at 24 h after surgery was reported in 28 studies involving 20 treatments, and the incidence of PONV was reported in 27 studies involving 17 treatments. There was at least one placebo-controlled trial for each treatment. When a direct comparison was performed, each treatment was represented by a node and linked by an edge. More sample sizes indicate a bigger node, while more studies demonstrate a thicker edge.
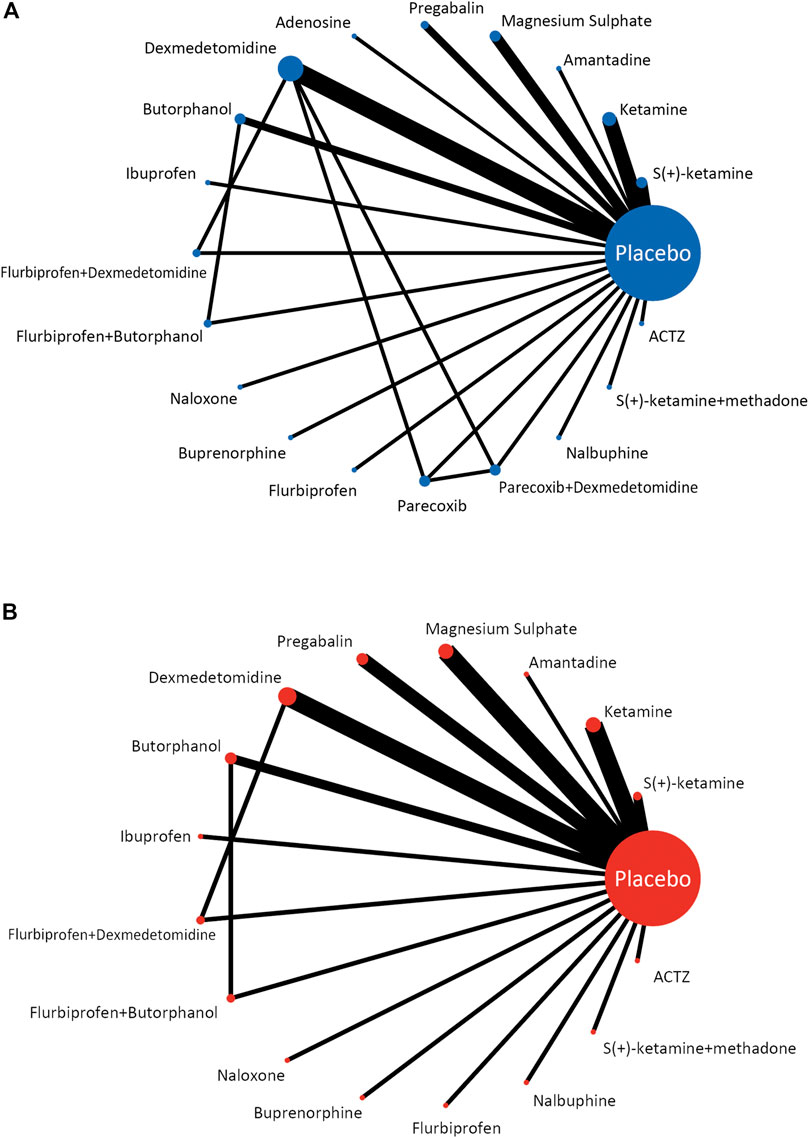
FIGURE 2. Network meta-analysis of eligible comparisons for postoperative pain intensity at rest at 24 h (A) and the incidence of PONV (B).
3.4 Results of primary outcomes
The forest plot (Figure 3) displays the network meta-analysis results for the primary outcomes. In terms of postoperative pain intensity, amantadine, magnesium sulphate, pregabalin, dexmedetomidine, ibuprofen, flurbiprofen plus dexmedetomidine, parecoxib, parecoxib plus dexmedetomidine and S (+)-ketamine plus methadone were all associated with milder pain intensity than placebo, with SMDs ranging between −3.06 (95% CI: −4.67, −1.45) for amantadine and −0.62 (95% CI: −1.23, −0.01) for magnesium sulphate. Regarding the PONV incidence, intervention with dexmedetomidine (OR = 0.25, 95% CI: 0.11, 0.54) or flurbiprofen plus dexmedetomidine (OR = 0.27, 95% CI: 0.08, 0.87) results in a lower incidence of PONV than placebo.
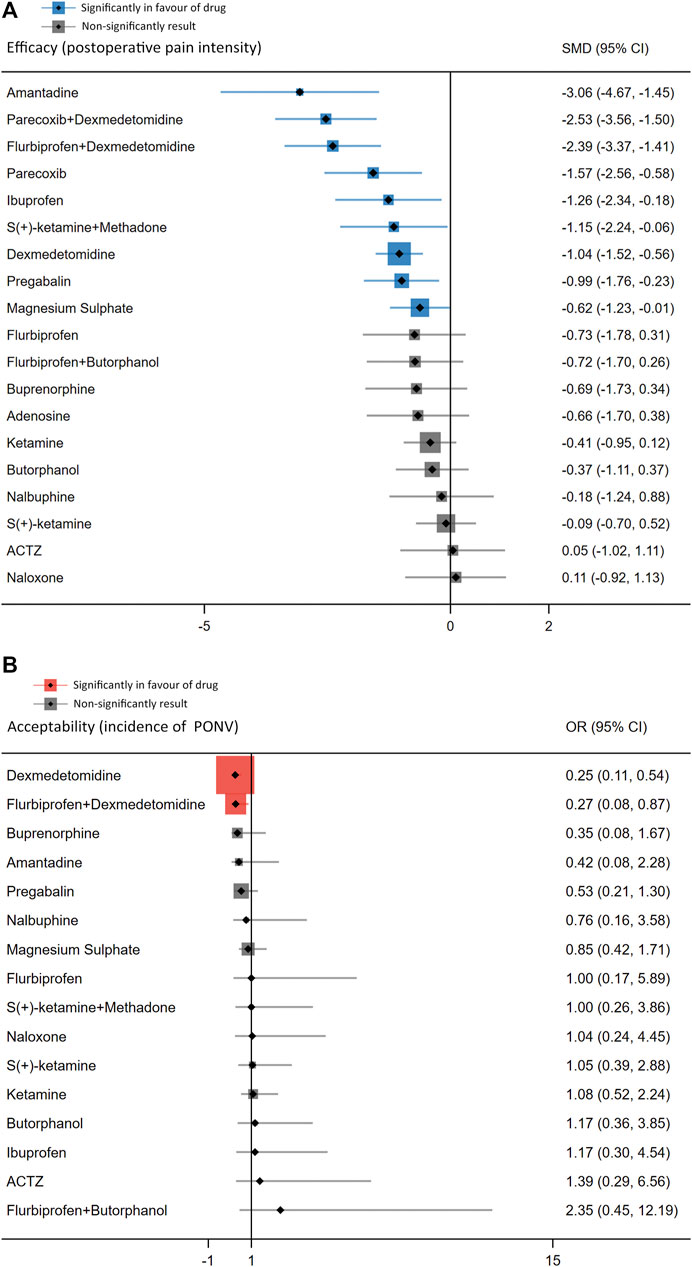
FIGURE 3. Forest plots of network meta-analysis of all trials for postoperative pain intensity at rest at 24 h (A) and the incidence of PONV (B).
The league table (Figure 4) illustrates head-to-head comparisons of all pharmacological intervention strategies and placebo for postoperative pain intensity (lower left portion) and PONV incidence (upper right portion). The results of pairwise comparisons are expressed as SMD (95% CI) and OR (95% CI), respectively. The two-dimensional graph (Figure 5) reveals that only dexmedetomidine and flurbiprofen plus dexmedetomidine outperform placebo in terms of postoperative pain intensity and PONV incidence.
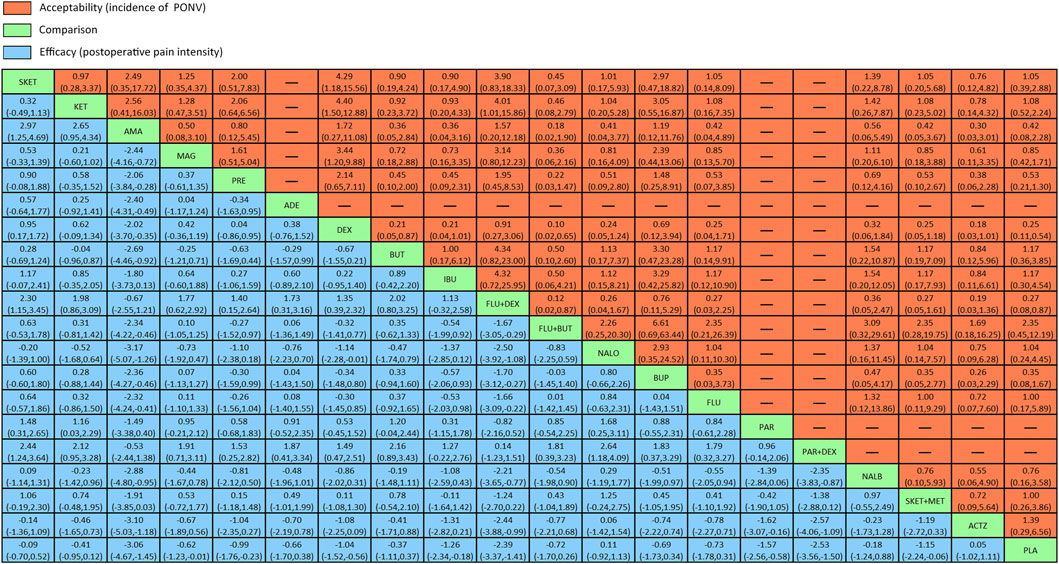
FIGURE 4. League table of head-to-head comparisons for postoperative pain intensity at rest at 24 h and the incidence of PONV of all pharmacological interventions and placebo. PLA = placebo. SKET = S (+)-ketamine. KET = ketamine. AMA = amantadine. MAG = magnesium sulphate. PRE = pregabalin. ADE = adenosine. DEX = dexmedetomidine. BUT = butorphanol. IBU = ibuprofen. FLU + DEX = flurbiprofen + dexmedetomidine. FLU + BUT = flurbiprofen + butorphanol. NALO = naloxone. BUP = buprenorphine. FLU = flurbiprofen. PAR = parecoxib. PAR + DEX = parecoxib + dexmedetomidine. NALB = nalbuphine. SKET + MET = S (+)-ketamine + methadone.
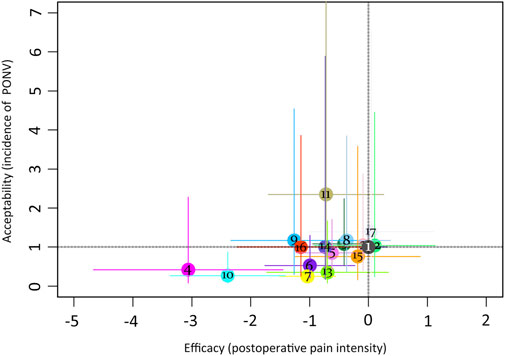
FIGURE 5. Two-dimensional graphs for postoperative pain intensity at rest at 24 h and the incidence of PONV. 1 = placebo; 2 = S (+)-ketamine; 3 = ketamine; 4 = amantadine; 5 = magnesium sulphate; 6 = pregabalin; 7 = dexmedetomidine; 8 = butorphanol; 9 = ibuprofen; 10 = flurbiprofen + dexmedetomidine; 11 = flurbiprofen + butorphanol; 12 = naloxone; 13 = buprenorphine; 14 = flurbiprofen; 15 = nalbuphine; 16 = S (+)-ketamine + methadone; 17 = ACTZ.
In the ranking probability plot (Supplementary Appendix S4, Figure 4), amantadine appeared to be the best agent for postoperative pain intensity among all 20 treatments with a SUCRA value of 96.2. In terms of PONV incidence, it was determined that dexmedetomidine appeared to be the best option among all 17 PONV treatments, with a SUCRA value of 90.3.
3.5 Results of secondary outcomes
3.5.1 Pain threshold at 24 h after surgery
Ten studies involving 11 interventions reported pain thresholds 24 h after surgery (measured by QST and in g) (Supplementary Appendix S4 and Supplementary Figure 4.1.1). Butorphanol (SMD = 2.43, 95% CI: 1.65, 3.22), magnesium sulphate (SMD = 1.01, 95% CI: 0.14, 1.88) and dexmedetomidine (SMD = 1.01, 95% CI: 0.14, 1.88) have higher pain thresholds than placebo at 24 h after surgery (Supplementary Appendix S4 and Supplementary Figure 4.1.2). The league table (Supplementary Appendix S4 and Supplementary Figure 4.1.3) illustrates the comparison of each intervention to one another. Flurbiprofen plus dexmedetomidine was ranked first in the ranking probability plot (Supplementary Appendix S4 and Supplementary Figure 4.1.4) among 11 interventions with a SUCRA value of 98.1.
3.5.2 Cumulative morphine consumption over the 24 h
A total of 14 studies with 11 interventions reported cumulative morphine consumption over a 24 h period (Supplementary Appendix S4 and Supplementary Figure 4.2.1). Flurbiprofen (SMD = −17.36, 95% CI: −22.13, −12.59) and dexmedetomidine (SMD = −11.83, 95% CI: −17.77, −5.90) caused more morphine consumption at 24 h after surgery than placebo (Supplementary Appendix S4 and Supplementary Figure 4.2.2). The league table (Supplementary Appendix S4 and Supplementary Figure 4.2.3) compares the outcomes of each intervention to one another. Flurbiprofen plus dexmedetomidine was ranked first in the ranking probability plot (Supplementary Appendix S4 and Supplementary Figure 4.2.4) among 11 interventions with a SUCRA value of 100.
3.5.3 The time to first postoperative analgesic requirement
The time to the first postoperative analgesic requirement was reported in 14 studies involving 13 interventions (Supplementary Appendix S4 and Supplementary Figure 4.3.1). When compared with placebo, flurbiprofen plus dexmedetomidine (MD = 43.05, 95% CI: 28.49, 57.60), adenosine (MD = 26.90, 95% CI: 11.98, 41.82), magnesium sulphate (MD = 23.29, 95% CI: 12.27, 34.30) and dexmedetomidine (MD = 11.39, 95% CI: 0.93, 21.84) have a longer time to require first postoperative analgesic (Supplementary Appendix S5 and Supplementary Figure 5.3.2). The league table (Supplementary Appendix S4 and Supplementary Figure 4.3.3) compares the outcome of each intervention to one another. Flurbiprofen plus dexmedetomidine was ranked first in the ranking probability plot (Supplementary Appendix S4 and Supplementary Figure 4.3.4) among 13 interventions with SUCRA value of 98.5.
3.5.4 Incidence of shivering
Nine studies involving nine interventions reported the incidence of shivering (Supplementary Appendix S4 and Supplementary Figure 4.4.1). Dexmedetomidine (OR = 0.16, 95% CI: 0.06, 0.43), flurbiprofen plus dexmedetomidine (OR = 0.12, 95% CI: 0.03, 0.49), magnesium sulphate (OR = 0.07, 95% CI: 0.02, 0.36) and S (+) -ketamine (OR = 0.05, 95% CI: 0.00, 0.99) have a lower incidence of shivering than placebo (Supplementary Appendix S4 and Supplementary Figure 4.4.2). The league table (Supplementary Appendix S4 and Supplementary Figure 4.4.3) compares the outcomes of each intervention to one another. S (+) -ketamine was ranked highest in the ranking probability plot (Supplementary Appendix S4 and Supplementary Figure 4.4.4) among nine interventions with SUCRA value of 82.0.
4 Discussion
Because of the morbidity concealment, complex pathogenesis, and treatment uncertainty of OIH, the best strategy is to avoid it. This is the first systematic review and network meta-analysis to compare various pharmacological interventions and investigate the best strategy for preventing the increase in postoperative pain caused by OIH in adults following general anesthesia. The following aspects of the 20 treatments were compared and analyzed: pain intensity, opioid-related adverse effects, pain threshold, time first to rescue analgesia, and morphine consumption. We identified that no such perfect drug performs best in all indicators. This emphasizes the significance of individualized treatment selection and a multimodal approach.
Our findings reveal that amantadine, magnesium sulphate, pregabalin, dexmedetomidine, ibuprofen, flurbiprofen plus dexmedetomidine, parecoxib, parecoxib plus dexmedetomidine and S (+)-ketamine plus methadone all have the potential to prevent the increase in postoperative pain intensity, with amantadine appearing to be the best option among the 20 interventions studies. Although the mechanisms underlying OIH are not fully understood. Preclinical models implicate the glutaminergic system and pathological NMDA receptor activation in the development of central sensitization (Mao et al., 1994; Mao et al., 2002; Zhao et al., 2012). Amantadine, magnesium sulphate, methadone, and S (+)-ketamine are known to be the NMDA receptor’s antagonists, where its primary effects are thought to occur. Wu L et al. found that perioperative administration of NMDA receptor antagonists effectively reduced postoperative pain intensity and morphine consumption (Wu et al., 2015), without evident psychological effects. However, our findings suggest that amantadine may be the best option when either ketamine or S (+)-ketamine fails to show significant superiority in preventing the rise of postoperative pain intensity. A possible explanation for this discrepancy is that Wu L et al.'s conclusion requires extraordinary caution in interpretation due to high heterogeneity even after subgroup analysis. The studies involved were small (only 14 studies included 3 drugs which directly act on NMDA receptors), with possible overestimation of the risk of Type II statistical error. However, the effect of an intervention may be influenced to varying degrees by other factors in NMDA. Therefore, we suggest that future studies should consider confirming the findings of our meta-analysis.
Ibuprofen, flurbiprofen, and parecoxib are NSAIDs that have potent anti-inflammatory, analgesic, and antipyretic activities and are used globally. One of their primary mechanisms of action is the inhibition of cyclo-oxygenase (COX), an enzyme involved in the biosynthesis of prostaglandins and thromboxane (Bacchi et al., 2012). Prostaglandins have been demonstrated to modulate nociceptive processing (Baba et al., 2001) and stimulate the release of the excitatory amino acid glutamate in the dorsal horns of the spinal cord (O’Rielly Darren and Loomis Christopher, 2006). Moreover, COX constitutively expressed in the spinal cord and is activated in response to peripheral stimuli that cause pain, primarily through the involvement of NMDA and substance P signaling (Yaksh and Malmberg, 1993). As such, NSAIDs have been demonstrated to inhibit the heightened sensitivity to pain triggered by the activation of spinal NMDA and substance P receptors (Malmberg and Yaksh, 1992; Yaksh and Malmberg, 1993). Clinical studies or meta-analyses about the effect of COX inhibitors on OIH are still lacking, even though it has been proved in animal models (Li et al., 2018; Peng et al., 2019) and human volunteers (Koppert et al., 2004; Lenz et al., 2011).
It has been indicated that opioid-induced pronociceptive effects are caused by central and peripheral nervous system sensitization, similar to the mechanism of hyperalgesia associated with nerve injury (Mao et al., 1995). Pregabalin is a 3-substituted analogue of γ-aminobutyric acid used to treat neuropathic pain (Guay, 2005) with the side effects of dizziness and drowsiness. It has a similar structure and mechanism of action to gabapentin but has fewer side effects (Ben-Menachem, 2004). Pregabalin binds strongly to the α2δ-1 subunit of voltage-gated calcium channels. This binding impairs channel trafficking and reduces the release of various neurotransmitters, including glutamate, noradrenaline, and substance P (Bannister et al., 2011). These effects result in interactions with spino-bulbo-spinal loop-comprising projection neurons in the superficial dorsal horn and brainstem, leading to facilitation of 5-hydroxytryptamine3 receptor-mediated effects in pain modulation. It has been indicated that pregabalin reduce hyperalgesia and allodynia in human volunteers (Chizh et al., 2007) and rat models (Field et al., 1999). However, A J Lederer et al. reviewed the effects of pregabalin on OIH and concluded that, despite strong support by theoretical considerations, the recommendation as a clinical use still lacks clinical evidence (Lederer et al., 2011). Stoicia et al. reached a similar conclusion, stating that applying gabapentin in mitigating OIH still requires support from large-scale standardized patient studies (Stoicea et al., 2015).
Dexmedetomidine is a potent and highly selective α-2 adrenoceptor agonist with sympatholytic, sedative, amnestic, and analgesic properties (Khan et al., 1999). Its anti-hyperalgesia effects are closely associated with NMDA receptors. Animal studies reveal that dexmedetomidine modulates spinal cord NMDA receptor activation by suppressing tyrosine phosphorylation of NR2B in the superficial spinal cord, which was found to be upregulated during remifentanil-induced hyperalgesia (Zheng et al., 2012). Furthermore, another study has provided evidence supporting the prevention of OIH by dexmedetomidine through the regulation of spinal NMDA receptors, as well as the levels of protein kinase C (PKC) and calcium/calmodulin-dependent protein kinase II (CaMKII), both of which are involved in neuronal signaling (Yuan et al., 2017). Similarly, its anti-hyperalgesia effect in clinical practice requires further investigation.
The findings of the present meta-analysis also revealed that dexmedetomidine and flurbiprofen plus dexmedetomidine are interventions associated with a lower PONV incidence compared to placebo. It is worth noting that flurbiprofen alone has no superior effect. This appears to imply that dexmedetomidine plays a significant role in preventing PONV, consistent with previous studies (Le Bot et al., 2015; Jin et al., 2017; Grape et al., 2019). Jin S et al. (Jin et al., 2017) investigated the effect of dexmedetomidine on PONV in patients undergoing general anesthesia. They identified that dexmedetomidine (irrespective of administration mode) had a significantly lower incidence of PONV than placebo. It was thought that this additional antiemetic effect of α2 agonists might be explained by inhibiting catecholamines by parasympathetic tone, even though the biological basis remains unknown. Alternatively, dexmedetomidine may reduce intraoperative anesthetics and opioids, which have been considered risk factors for PONV (Gan et al., 2020).
The treatment risk/benefit ratio is an important consideration in clinical decision-making. Our findings revealed that, while there is the best option in every index, dexmedetomidine is the only pharmacological intervention that outperformed placebo in all indicators. In addition, the multifaceted benefit of dexmedetomidine in improving the quality of emergence from anesthesia (Aouad et al., 2019), reducing postoperative delirium incidence (Duan et al., 2018), enhancing recovery after surgery (Kaye et al., 2020) and providing organ-protective effects (Bao and Tang, 2020) has already been fully demonstrated and widely accepted. Despite the side effects of hypotension and bradycardia, it is difficult to deny that dexmedetomidine is an attractive anesthetic adjuvant (Weerink et al., 2017).
This network meta-analysis had several possible limitations. First, because multiple interventions were included in the analysis, several had data from only one study, resulting in a relatively small sample size, which could have led to possible bias and overestimation of the treatment effect. Second, some non-pharmacological interventions, such as gradual withdrawal of remifentanil (Comelon et al., 2016), opioid rotation (Mercadante and Arcuri, 2005) and combination with a regional nerve block (Rivat et al., 2013), were not included in the comparison. Third, it is important to acknowledge that despite conducting comprehensive literature research prior to designing the retrieval strategy and considering commonly used drugs in clinical anesthesia, there is a possibility that our study may have omitted other drugs that have been investigated for their effects on OIH. Finally, there was variation in gender, opioid dosage, timing, administration regimens, surgery duration, and anesthesia maintenance. These disparities limit the amount of data pooled in a meta-analysis, posing significant challenges in interpreting and applying the results.
Overall, this systematic review and network meta-analysis provides the most comprehensive summary of the comparative effect of various pharmacological interventions on improving the intensity of postoperative pain caused by OIH.
5 Conclusion
In summary, a meta-analysis of eligible RCTs identified that amantadine was the best at preventing an increase in postoperative pain and non-inferior to placebo in the incidence of PONV. In contrast, dexmedetomidine was the only intervention superior to placebo in all indicators.
Data availability statement
The original contributions presented in the study are included in the article/Supplementary Material, further inquiries can be directed to the corresponding authors.
Author contributions
W-JX and WL proposed and designed the study. Y-CL, J-SH, C-FF, and H-FC provided experimental research. W-JX and Y-CL participated in data analysis. W-JX and J-SH wrote and revised the manuscript. All authors contributed to the article and approved the submitted version.
Conflict of interest
The authors declare that the research was conducted in the absence of any commercial or financial relationships that could be construed as a potential conflict of interest.
Publisher’s note
All claims expressed in this article are solely those of the authors and do not necessarily represent those of their affiliated organizations, or those of the publisher, the editors and the reviewers. Any product that may be evaluated in this article, or claim that may be made by its manufacturer, is not guaranteed or endorsed by the publisher.
Supplementary material
The supplementary material for this article can be found online at: https://www.frontiersin.org/articles/10.3389/fphar.2023.1199794/full#supplementary-material
References
Antal, M., Fukazawa, Y., Eördögh, M., Muszil, D., Molnár, E., Itakura, M., et al. (2008). Numbers, densities, and colocalization of AMPA- and NMDA-type glutamate receptors at individual synapses in the superficial spinal dorsal horn of rats. J. Neurosci. 28, 9692–9701. doi:10.1523/JNEUROSCI.1551-08.2008
Aouad, M. T., Zeeni, C., Al Nawwar, R., Siddik-Sayyid, S. M., Barakat, H. B., Elias, S., et al. (2019). Dexmedetomidine for improved quality of emergence from general anesthesia: A dose-finding study. Anesth. Analgesia 129, 1504–1511. doi:10.1213/ANE.0000000000002763
Apfel Christian, C., Läärä, E., Koivuranta, M., Greim, C-A., and Roewer, N. (1999). A simplified risk score for predicting postoperative nausea and vomiting: Conclusions from cross-validations between two centers. Anesthesiology 91, 693–700. doi:10.1097/00000542-199909000-00022
Arout, C. A., Caldwell, M., Rossi, G., and Kest, B. (2015). Spinal and supraspinal N-methyl-D-aspartate and melanocortin-1 receptors contribute to a qualitative sex difference in morphine-induced hyperalgesia. Physiol. Behav. 147, 364–372. doi:10.1016/j.physbeh.2015.05.006
Baba, H., Kohno, T., Moore, K. A., and Woolf, C. J. (2001). Direct activation of rat spinal dorsal horn neurons by prostaglandin E2. J. Neurosci. 21, 1750–1756. doi:10.1523/JNEUROSCI.21-05-01750.2001
Bacchi, S., Palumbo, P., Sponta, A., and Coppolino, M. F. (2012). Clinical pharmacology of non-steroidal anti-inflammatory drugs: A review. Antiinflamm. Antiallergy Agents Med. Chem. 11, 52–64. doi:10.2174/187152312803476255
Bannister, K., Sikandar, S., Bauer, C. S., Dolphin, A. C., Porreca, F., and Dickenson, A. H. (2011). Pregabalin suppresses spinal neuronal hyperexcitability and visceral hypersensitivity in the absence of peripheral pathophysiology. Anesthesiology 115, 144–152. doi:10.1097/ALN.0b013e31821f6545
Bao, N., and Tang, B. (2020). Organ-protective effects and the underlying mechanism of dexmedetomidine. Mediat. Inflamm. 2020, 6136105–05. doi:10.1155/2020/6136105
Beaudry, H., Gendron, L., and Morón, J. A. (2015). Implication of delta opioid receptor subtype 2 but not delta opioid receptor subtype 1 in the development of morphine analgesic tolerance in a rat model of chronic inflammatory pain. Eur. J. Neurosci. 41, 901–907. doi:10.1111/ejn.12829
Ben-Menachem, E. (2004). Pregabalin pharmacology and its relevance to clinical practice. Epilepsia 45 (6), 13–18. doi:10.1111/j.0013-9580.2004.455003.x
Chaimani, A., Higgins, J. P. T., Mavridis, D., Spyridonos, P., and Salanti, G. (2013). Graphical tools for network meta-analysis in STATA. PLoS One 8, e76654–e54. doi:10.1371/journal.pone.0076654
Chizh, B. A., Göhring, M., Tröster, A., Quartey, G. K., Schmelz, M., and Koppert, W. (2007). Effects of oral pregabalin and aprepitant on pain and central sensitization in the electrical hyperalgesia model in human volunteers. Br. J. Anaesth. 98, 246–254. doi:10.1093/bja/ael344
Comelon, M., Raeder, J., Stubhaug, A., Nielsen, C. S., Draegni, T., and Lenz, H. (2016). Gradual withdrawal of remifentanil infusion may prevent opioid-induced hyperalgesia. Br. J. Anaesth. 116, 524–530. doi:10.1093/bja/aev547
Du, X., Song, F., Zhang, X., and Ma, S. (2019). Protective efficacy of combined use of parecoxib and dexmedetomidine on postoperative hyperalgesia and early cognitive dysfunction after laparoscopic cholecystectomy for elderly patients. Acta Cir. Bras. 34, e201900905. doi:10.1590/s0102-865020190090000005
Duan, X., Coburn, M., Rossaint, R., Sanders, R. D., Waesberghe, J. V., and Kowark, A. (2018). Efficacy of perioperative dexmedetomidine on postoperative delirium: Systematic review and meta-analysis with trial sequential analysis of randomised controlled trials. Br. J. Anaesth. 121, 384–397. doi:10.1016/j.bja.2018.04.046
Eberhart, L. H. J., Mauch, M., Morin, A. M., Wulf, H., and Geldner, G. (2002). Impact of a multimodal anti-emetic prophylaxis on patient satisfaction in high-risk patients for postoperative nausea and vomiting. Anaesthesia 57, 1022–1027. doi:10.1046/j.1365-2044.2002.02822.x
Field, M. J., Bramwell, S., Hughes, J., and Singh, L. (1999). Detection of static and dynamic components of mechanical allodynia in rat models of neuropathic pain: Are they signalled by distinct primary sensory neurones? PAIN 83, 303–311. doi:10.1016/s0304-3959(99)00111-6
Fletcher, D., and Martinez, V. (2014). Opioid-induced hyperalgesia in patients after surgery: A systematic review and a meta-analysis. Br. J. Anaesth. 112, 991–1004. doi:10.1093/bja/aeu137
Gan, T. J., Belani, K. G., Bergese, S., Chung, F., Diemunsch, P., Habib, A. S., et al. (2020). Fourth consensus guidelines for the management of postoperative nausea and vomiting. Anesth. Analgesia 131, 411–448. doi:10.1213/ANE.0000000000004833
Grace, P. M., Maier, S. F., and Watkins, L. R. (2015). Opioid-induced central immune signaling: Implications for opioid analgesia. Headache 55, 475–489. doi:10.1111/head.12552
Grape, S., Kirkham, K. R., Frauenknecht, J., and Albrecht, E. (2019). Intra-operative analgesia with remifentanil vs. dexmedetomidine: A systematic review and meta-analysis with trial sequential analysis. Anaesthesia 74, 793–800. doi:10.1111/anae.14657
Guay, D. R. P. (2005). Pregabalin in neuropathic pain: A more “pharmaceutically elegant” gabapentin? Am. J. Geriatric Pharmacother. 3, 274–287. doi:10.1016/j.amjopharm.2005.12.008
Guntz, E., Dumont, H., Roussel, C., Gall, D., Dufrasne, F., Cuvelier, L., et al. (2005). Effects of remifentanil on N -methyl-d-aspartate receptor: An electrophysiologic study in rat spinal cord. Anesthesiology 102, 1235–1241. doi:10.1097/00000542-200506000-00025
He, W., Wu, Z., Zu, L., Sun, H., and Yang, X. (2020). Application of erector spinae plane block guided by ultrasound for postoperative analgesia in breast cancer surgery: A randomized controlled trial. Cancer Commun. 40, 122–125. doi:10.1002/cac2.12013
Higgins, J. P. T., Altman, D. G., Gøtzsche, P. C., Jüni, P., Moher, D., Oxman, A. D., et al. (2011). The Cochrane Collaboration’s tool for assessing risk of bias in randomised trials. BMJ 343, d5928. doi:10.1136/bmj.d5928
Hu, J., Chen, S., Zhu, M., Wu, Y., Wang, P., Chen, J., et al. (2020). Preemptive nalbuphine attenuates remifentanil-induced postoperative hyperalgesia after laparoscopic cholecystectomy: A prospective randomized double-blind clinical trial. J. Pain Res. 13, 1915–1924. doi:10.2147/JPR.S257018
Hutton, B., Salanti, G., Caldwell, D. M., Chaimani, A., Schmid, C. H., Cameron, C., et al. (2015). The PRISMA extension statement for reporting of systematic reviews incorporating network meta-analyses of health care interventions: Checklist and explanations. Ann. Intern. Med. 162, 777–784. doi:10.7326/M14-2385
Jin, S., Liang, D. D., Chen, C., Zhang, M., and Wang, J. (2017). Dexmedetomidine prevent postoperative nausea and vomiting on patients during general anesthesia: A PRISMA-compliant meta analysis of randomized controlled trials. Medicine 96, e5770. doi:10.1097/MD.0000000000005770
Kaye, A. D., Chernobylsky, D. J., Thakur, P., Siddaiah, H., Kaye, R. J., Eng, L. K., et al. (2020). Dexmedetomidine in enhanced recovery after surgery (ERAS) protocols for postoperative pain. Curr. Pain Headache Rep. 24, 21. doi:10.1007/s11916-020-00853-z
Khan, Z. P., Ferguson, C. N., and Jones, R. M. (1999). Alpha-2 and imidazoline receptor agonistsTheir pharmacology and therapeutic role. Anaesthesia 54, 146–165. doi:10.1046/j.1365-2044.1999.00659.x
Koo, C. H., Cho, Y. J., Hong, D. M., Jeon, Y., and Kim, T. K. (2016). Influence of high-dose intraoperative remifentanil with intravenous ibuprofen on postoperative morphine consumption in patients undergoing pancreaticoduodenectomy: A randomized trial. J. Clin. Anesth. 35, 47–53. doi:10.1016/j.jclinane.2016.07.017
Koppert, W., Sittl, R., Scheuber, K., Alsheimer, M., Schmelz, M., and Schüttler, J. (2003). Differential modulation of remifentanil-induced analgesia and postinfusion hyperalgesia by S -ketamine and clonidine in humans. Anesthesiology 99, 152–159. doi:10.1097/00000542-200307000-00025
Koppert, W., Wehrfritz, A., Körber, N., Sittl, R., Albrecht, S., Schüttler, J., et al. (2004). The cyclooxygenase isozyme inhibitors parecoxib and paracetamol reduce central hyperalgesia in humans. PAIN 108, 148–153. doi:10.1016/j.pain.2003.12.017
Lang, E., Kapila, A., Shlugman, D., Hoke, J. F., Sebel, P. S., and Glass, P. S. A. (1996). Reduction of isoflurane minimal alveolar concentration by remifentanil. Anesthesiology 85, 721–728. doi:10.1097/00000542-199610000-00006
Le Bot, A., Michelet, D., Hilly, J., Maesani, M., Dilly, M. P., Brasher, C., et al. (2015). Efficacy of intraoperative dexmedetomidine compared with placebo for surgery in adults: A meta-analysis of published studies. Minerva Anestesiol. 81, 1105–1117.
Leal, P. C., Salomão, R., Brunialti, M. K., and Sakata, R. K. (2015). Evaluation of the effect of ketamine on remifentanil-induced hyperalgesia: A double-blind, randomized study. J. Clin. Anesth. 27, 331–337. doi:10.1016/j.jclinane.2015.02.002
Lederer, A. J., Bornemann-Cimenti, H., Wejbora, M., Kern-Pirsch, C., Michaeli, K., and Sandner-Kiesling, A. (2011). Pregabalin and postoperative hyperalgesia: A review. Der Schmerz 25, 12–18. doi:10.1007/s00482-010-1008-x
Lee, M., Silverman, S. M., Hansen, H., Patel, V. B., and Manchikanti, L. (2011). A comprehensive review of opioid-induced hyperalgesia. Pain Physician 14, 145–161. doi:10.36076/ppj.2011/14/145
Lenz, H., Raeder, J., Draegni, T., Heyerdahl, F., Schmelz, M., and Stubhaug, A. (2011). Effects of COX inhibition on experimental pain and hyperalgesia during and after remifentanil infusion in humans. PAIN 152, 1289–1297. doi:10.1016/j.pain.2011.02.007
Li, Q. B., Chang, L., Ye, F., Luo, Q. H., Tao, Y. X., and Shu, H. H. (2018). Role of spinal cyclooxygenase-2 and prostaglandin E2 in fentanyl-induced hyperalgesia in rats. Br. J. Anaesth. 120, 827–835. doi:10.1016/j.bja.2017.11.103
Malmberg, A., and Yaksh, T. (1992). Hyperalgesia mediated by spinal glutamate or substance P receptor blocked by spinal cyclooxygenase inhibition. Science 257, 1276–1279. doi:10.1126/science.1381521
Mao, J., Price, D., and Mayer, D. (1994). Thermal hyperalgesia in association with the development of morphine tolerance in rats: Roles of excitatory amino acid receptors and protein kinase C. J. Neurosci. 14, 2301–2312. doi:10.1523/JNEUROSCI.14-04-02301.1994
Mao, J., Price, D. D., and Mayer, D. J. (1995). Mechanisms of hyperalgesia and morphine tolerance: A current view of their possible interactions. PAIN 62, 259–274. doi:10.1016/0304-3959(95)00073-2
Mao, J., Sung, B., Ji, R-R., and Lim, G. (2002). Chronic morphine induces downregulation of spinal glutamate transporters: Implications in morphine tolerance and abnormal pain sensitivity. J. Neurosci. 22, 8312–8323. doi:10.1523/JNEUROSCI.22-18-08312.2002
McNicol, E. D., Ferguson, M. C., and Hudcova, J. (2015). Patient controlled opioid analgesia versus non-patient controlled opioid analgesia for postoperative pain. Cochrane Database Syst. Rev. 2015, CD003348–CD48. doi:10.1002/14651858.CD003348.pub3
Mercadante, S., and Arcuri, E. (2005). Hyperalgesia and opioid switching. Am. J. Hospice Palliat. Medicine® 22, 291–294. doi:10.1177/104990910502200411
Mercieri, M., Palmisani, S., De Blasi, R. A., D'Andrilli, A., Naccarato, A., Silvestri, B., et al. (2017). Low-dose buprenorphine infusion to prevent postoperative hyperalgesia in patients undergoing major lung surgery and remifentanil infusion: A double-blind, randomized, active-controlled trial. Br. J. Anaesth. 119, 792–802. doi:10.1093/bja/aex174
Minville, V., Fourcade, O., Girolami, J. P., and Tack, I. (2010). Opioid-induced hyperalgesia in a mice model of orthopaedic pain: Preventive effect of ketamine. Br. J. Anaesth. 104, 231–238. doi:10.1093/bja/aep363
Myles, P. S., Williams, D. L., Hendrata, M., Anderson, H., and Weeks, A. M. (2000). Patient satisfaction after anaesthesia and surgery: Results of a prospective survey of 10,811 patients. Br. J. Anaesth. 84, 6–10. doi:10.1093/oxfordjournals.bja.a013383
O’Rielly Darren, D., and Loomis Christopher, W. (2006). Increased expression of cyclooxygenase and nitric oxide isoforms, and exaggerated sensitivity to prostaglandin E2, in the rat lumbar spinal cord 3 Days after L5–L6 spinal nerve ligation. Anesthesiology 104, 328–337. doi:10.1097/00000542-200602000-00019
Peng, Y., Zang, T., Zhou, L., Ni, K., and Zhou, X. (2019). COX-2 contributed to the remifentanil-induced hyperalgesia related to ephrinB/EphB signaling. Neurological Res. 41, 519–527. doi:10.1080/01616412.2019.1580459
Rivat, C., Bollag, L., and Richebé, P. (2013). Mechanisms of regional anaesthesia protection against hyperalgesia and pain chronicization. Curr. Opin. Anesthesiol. 26, 621–625. doi:10.1097/01.aco.0000432511.08070.de
Roeckel, L. A., Le Coz, G. M., Gavériaux-Ruff, C., and Simonin, F. (2016). Opioid-induced hyperalgesia: Cellular and molecular mechanisms. Neuroscience 338, 160–182. doi:10.1016/j.neuroscience.2016.06.029
Ryu, J. H., Kang, M. H., Park, K. S., and Do, S. H. (2008). Effects of magnesium sulphate on intraoperative anaesthetic requirements and postoperative analgesia in gynaecology patients receiving total intravenous anaesthesia. Br. J. Anaesth. 100, 397–403. doi:10.1093/bja/aem407
Shafi, S., Collinsworth, A. W., Copeland, L. A., Ogola, G. O., Qiu, T., Kouznetsova, M., et al. (2018). Association of opioid-related adverse drug events with clinical and cost outcomes among surgical patients in a large integrated health care delivery system. JAMA Surg. 153, 757–763. doi:10.1001/jamasurg.2018.1039
Snijdelaar Dirk, G., Koren, G., and Katz, J. (2004). Effects of perioperative oral amantadine on postoperative pain and morphine consumption in patients after radical prostatectomy: Results of a preliminary study. Anesthesiology 100, 134–141. doi:10.1097/00000542-200401000-00022
Stoicea, N., Russell, D., Weidner, G., Durda, M., Joseph, N. C., Yu, J., et al. (2015). Opioid-induced hyperalgesia in chronic pain patients and the mitigating effects of gabapentin. Front. Pharmacol. 6, 104. doi:10.3389/fphar.2015.00104
Tognoli, E., Proto, P. L., Motta, G., Galeone, C., Mariani, L., and Valenza, F. (2020). Methadone for postoperative analgesia: Contribution of N-methyl-D-aspartate receptor antagonism: A randomised controlled trial. Eur. J. Anaesthesiol. 37, 934–943. doi:10.1097/EJA.0000000000001217
van Valkenhoef, G., Dias, S., Ades, A. E., and Welton, N. J. (2016). Automated generation of node-splitting models for assessment of inconsistency in network meta-analysis. Res. Synthesis Methods 7, 80–93. doi:10.1002/jrsm.1167
Vanderah, T. W., Ossipov, M. H., Lai, J., Malan, T. P., and Porreca, F. (2001). Mechanisms of opioid-induced pain and antinociceptive tolerance: Descending facilitation and spinal dynorphin. Pain 92, 5–9. doi:10.1016/s0304-3959(01)00311-6
Vera-Portocarrero, L. P., Zhang, E. T., King, T., Ossipov, M. H., Vanderah, T. W., Lai, J., et al. (2007). Spinal NK-1 receptor expressing neurons mediate opioid-induced hyperalgesia and antinociceptive tolerance via activation of descending pathways. Pain 129, 35–45. doi:10.1016/j.pain.2006.09.033
Vinik, H. R., and Kissin, I. (1998). Rapid development of tolerance to analgesia during remifentanil infusion in humans. Anesth. Analgesia 86, 1307–1311. doi:10.1097/00000539-199806000-00033
Weerink, M. A. S., Struys, M. M. R. F., Hannivoort, L. N., Barends, C. R. M., Absalom, A. R., and Colin, P. (2017). Clinical pharmacokinetics and pharmacodynamics of dexmedetomidine. Clin. Pharmacokinet. 56, 893–913. doi:10.1007/s40262-017-0507-7
White, I. R. (2011). Multivariate random-effects meta-regression: Updates to mvmeta. Stata J. 11, 255–270. doi:10.1177/1536867x1101100206
Wu, L., Huang, X., and Sun, L. (2015). The efficacy of N-methyl-d-aspartate receptor antagonists on improving the postoperative pain intensity and satisfaction after remifentanil-based anesthesia in adults: A meta-analysis. J. Clin. Anesth. 27, 311–324. doi:10.1016/j.jclinane.2015.03.020
Wu, Z., Yu, J., Lin, Q., Li, H., Zhang, T., Tan, H., et al. (2021). Effects of an intraoperative intravenous bolus dose of dexmedetomidine on remifentanil-induced postinfusion hyperalgesia in patients undergoing thyroidectomy: A double-blind randomized controlled trial. Anesth. Analgesia 132, 320–328. doi:10.1213/ANE.0000000000005003
Yaksh, T. L., and Malmberg, A. B. (1993). Spinal actions of NSAIDS in blocking spinally mediated hyperalgesia: The role of cyclooxygenase products. Agents Actions Suppl. 41, 89–100.
Yuan, Y., Sun, Z., Chen, Y., Zheng, Y., Xie, K. L., He, Y., et al. (2017). Prevention of remifentanil induced postoperative hyperalgesia by dexmedetomidine via regulating the trafficking and function of spinal NMDA receptors as well as PKC and CaMKII level in vivo and in vitro. PLoS One 12, e0171348. doi:10.1371/journal.pone.0171348
Zhang, L., Shu, R., Zhao, Q., Li, Y., Yu, Y., and Wang, G. (2016). Preoperative butorphanol and flurbiprofen axetil therapy attenuates remifentanil-induced hyperalgesia after laparoscopic gynaecological surgery: A randomized double-blind controlled trial. Br. J. Anaesth. 117, 504–511. doi:10.1093/bja/aew248
Zhao, M., and Joo Daisy, T. (2008). Enhancement of spinal N -Methyl-d-aspartate receptor function by remifentanil action at δ-opioid receptors as a mechanism for acute opioid-induced hyperalgesia or tolerance. Anesthesiology 109, 308–317. doi:10.1097/ALN.0b013e31817f4c5d
Zhao, Y-L., Chen, S-R., Chen, H., and Pan, H-L. (2012). Chronic opioid potentiates presynaptic but impairs postsynaptic N-Methyl-d-aspartic acid receptor activity in spinal cords: Implications for opioid hyperalgesia and tolerance. J. Biol. Chem. 287, 25073–25085. doi:10.1074/jbc.M112.378737
Zheng, Y., Cui, S., Liu, Y., Zhang, J., Zhang, W., Zhang, J., et al. (2012). Dexmedetomidine prevents remifentanil-induced postoperative hyperalgesia and decreases spinal tyrosine phosphorylation of N-methyl-d-aspartate receptor 2B subunit. Brain Res. Bull. 87, 427–431. doi:10.1016/j.brainresbull.2012.01.009
Keywords: opioid-induced hyperalgesia, pharmacological interventions, general anesthesia, network meta-analysis, postoperative pain, postoperative nausea and vomiting
Citation: Xie W-J, Hong J-S, Feng C-F, Chen H-F, Li W and Li Y-C (2023) Pharmacological interventions for preventing opioid-induced hyperalgesia in adults after opioid-based anesthesia: a systematic review and network meta-analysis. Front. Pharmacol. 14:1199794. doi: 10.3389/fphar.2023.1199794
Received: 04 April 2023; Accepted: 12 June 2023;
Published: 22 June 2023.
Edited by:
Tomoya Tachi, Nagoya City University, JapanReviewed by:
Shamseddin Ahmadi, University of Kurdistan, IranLin Zhang, Sichuan University, China
Salvador Romero Molina, Hospital Clínico Universitario Virgen de la Victoria, Spain
Copyright © 2023 Xie, Hong, Feng, Chen, Li and Li. This is an open-access article distributed under the terms of the Creative Commons Attribution License (CC BY). The use, distribution or reproduction in other forums is permitted, provided the original author(s) and the copyright owner(s) are credited and that the original publication in this journal is cited, in accordance with accepted academic practice. No use, distribution or reproduction is permitted which does not comply with these terms.
*Correspondence: Wei Li, Liw@sysucc.org.cn; Yong-Chun Li, liyongch@sysucc.org.cn