- 1University Children’s Hospital Regensburg (KUNO), Hospital St. Hedwig of the Order of John, University of Regensburg, Regensburg, Germany
- 2Member of the Research and Development Campus Regensburg (WECARE), Hospital St. Hedwig of the Order of St. John, Regensburg, Germany
- 3Clinic of Obstetrics and Gynecology St. Hedwig, University of Regensburg, Regensburg, Germany
- 4Institute of Social Medicine and Health Economics, University of Magdeburg, Magdeburg, Germany
Introduction: Acquired QT interval prolongations due to drug side effects can result in detrimental arrhythmia. Maternal use of placenta-permeable drugs may lead to fetal exposure, thus leading to an increased risk of neonatal QT prolongation and arrhythmia.
Objectives: This study aimed to evaluate the influence of maternal QT-prolonging medication on the neonatal QT interval.
Methods: In the prospective KUNO-Kids health study, an ongoing population-based birth cohort, we classified maternal medications according to the known risk of QT interval prolongation. Effects on the neonatal QT interval were tested by linear regression analyses, correcting for perinatal confounders (birth weight, gestational age, birth mode, and age at ECG recording). Subgroup analyses were performed for selective serotonin reuptake inhibitors, proton pump inhibitors, and antihistamine dimenhydrinate. Logistic regression analysis was performed using a QTc of 450 ms as the cut-off value.
Results: A total of 2,550 pregnant women received a total of 3,990 medications, of which 315 were known to increase the risk of QT prolongation, resulting in 105 (4.1%) neonates exposed in the last month of pregnancy. Overall, the mean age of the neonates at ECG was 1.9 days and the mean QTc (Bazett) was 414 ms. Univariate (regression coefficient −2.62, p = 0.288) and multivariate (regression coefficient −3.55, p = 0.146) regression analyses showed no significant effect of fetal medication exposure on the neonatal QT interval, neither in the overall nor in the subgroup analysis. Logistic regression analysis showed no association of exposure to maternal medication with an increased risk of neonatal QT interval prolongation (OR (odds ratio) 0.34, p = 0.14).
Conclusion: The currently used maternal medication results in a relevant number of fetuses exposed to QT interval-prolonging drugs. In our cohort, exposure was found to have no effect on the neonatal QT interval.
1 Introduction
A severely prolonged QT interval is associated with life-threatening complications, such as stillbirth and sudden infant death syndrome (SIDS) in infants, and detrimental arrhythmia and sudden unexplained death (SUD) in children and adults (Schwartz et al., 1998; 2001; Arnestad et al., 2007; Tester and Ackerman, 2007; Tester et al., 2012). Approximately 10% of SIDS cases can be attributed to long QT syndrome (LQTS) (Arnestad et al., 2007), a genetic cardiac ion-channel disease with a prevalence of about 1:2000 (Schwartz et al., 2009) for which associated mutations in 17 genes have been described (Wilde et al., 2022). In addition to genetically caused LQTS, QT prolongation can also be acquired as an adverse effect of certain drugs. Through various interactions with cardiac ion channels, these drugs can prolong the QT interval and thus increase the risk for critical arrhythmia. Furthermore, genetic variants are known to influence individual susceptibility to drug-induced QT interval prolongation (Kallergis et al., 2012).
QT interval prolongation has been described for several drugs commonly taken by pregnant women, including selective serotonin reuptake inhibitors (SSRIs) (Funk and Bostwick, 2013), proton pump inhibitors (PPIs) (Moore et al., 1989; Gau et al., 2012; Bibawy et al., 2013), and antihistamine dimenhydrinate (diphenhydramine) (Poluzzi et al., 2015). Maternal drug use during pregnancy may result in fetal exposure if drugs pass the placenta. In the case of pro-arrhythmogenic drugs, this may lead to an increased risk of arrhythmia in the fetus and neonate. However, data on the influence of potential QT interval-prolonging maternal medication on the postnatal neonatal QT interval are very limited. There are some case reports on neonates with markedly prolonged postnatal QT intervals that were possibly related to exposure to maternal medication. One of these patients needed therapy for life-threatening arrhythmia and torsade de pointes, occurring after maternal intake of tricyclic antidepressants (Dubnov, 2005; Fukushima et al., 2016; Leerssen et al., 2019). For SSRIs, two case–control studies addressed the issue of neonatal QT interval prolongation after intrauterine exposure with inflicting results (Dubnov-Raz et al., 2008; Lindsay-Sutherland, 2021).
Apart from a possible influence of maternal medication on the neonatal electrocardiogram (ECG), there are other neonatal and maternal factors that may affect the neonatal QT interval. For example, studies have reported a variable effect of gestational age on neonatal repolarization (Marcellino et al., 2021; Pærregaard et al., 2021), whereas maternal age at delivery had no impact (Pærregaard et al., 2022). The interpretation in newborns is methodologically challenging as the QT interval varies with age and may change within days (Schwartz et al., 1982; Simma et al., 2020). Therefore, the aim of this study was to evaluate the influence of peripartum maternal QT interval-prolonging medication on the QT interval in the neonatal ECG, taking peripartum confounders into account.
2 Materials and methods
2.1 Study design
The KUNO-Kids health study is a population-based prospective birth cohort study carried out at the Hospital St. Hedwig of the Order of John, Regensburg, Germany, as described elsewhere (Brandstetter et al., 2019). In the presented study, we analyzed parental and neonatal demographics, maternal medication, and postnatal ECGs recorded from 3,181 participants. Written informed consent was obtained for each case. The study was approved by the Ethics Committee of the University of Regensburg (14-101-0347).
2.2 Study population
All neonates born between the beginning of the KUNO-Kids health study, 27 July 2015, and 31 December 2019 were eligible. We included neonates with a postnatal ECG and a complete documentation of the maternal medication during pregnancy. Exclusion criteria were as follows: outpatient childbirth, stillbirth, maternal age less than 18 years, or maternal German language skills inadequate to achieve informed consent.
2.3 Exposure to maternal medication and perinatal demographics
Maternal medication during pregnancy was recorded in an interview conducted postnatally by the study team and verified by checking the maternity pass. For each drug, the month of gestation and the frequency of intake were recorded. Based on the manufacturer’s information and classification on the CredibleMeds® website (RL Woosley et al., 2022.), drugs were classified as having or not having a known risk for QT interval prolongation. Participants with maternal drugs that could not be classified due to insufficient specifications were excluded from the analysis.
Infants were defined as exposed (Med+) if a medication with a known risk of QT interval prolongation was taken by the mother at least once daily during the last month of pregnancy; otherwise, they were assigned to the unexposed (Med-) group. Among drugs with the known risk of QT interval prolongation, a subgroup analysis was performed for SSRIs, PPIs, and dimenhydrinate only.
Data on maternal demographics (age and migration background), neonatal characteristics (gestational age, birth weight, and sex), and the mode of birth (spontaneous delivery and caesarian section) were collected from patient records and a postpartum interview.
2.4 ECG
ECGs were recorded in the first week of life, as described by Simma et al. (2020), according to standard operating procedures. The 12 lead ECGs were performed with a commercially available recording device (MAC 5500 HD®, GE Healthcare, Freiburg, Germany), 10 adhesive electrodes (Ambu® BlueSensor NF50-A/12, Ambu, Bad Nauheim, Germany), and recorded at a paper with a speed of 50 mm/s including an additional rhythm recording at 25 mm/s. All ECG records were evaluated or revised by experienced pediatric cardiologists (SG and HM). The QT interval was measured manually from the onset of the Q-wave to the end of the T-wave using the tangent method. It was corrected for time (QTc) using Bazett’s formula (QTc [ms] = QT interval [ms]/(√RR [s]/1 [s])), as recommended by the guidelines for the interpretation of neonatal ECGs of the European Society of Cardiology (Schwartz, 2002). QTc was measured in lead II, and in the case of a value >440 ms, a mean of lead II, V5, and V6 was calculated according to Schwartz et al. B). The child’s age at the time of the ECG recording, QRS width, and heart rate were documented.
2.5 Statistical analysis
Parental and neonatal demographics, perinatal characteristics, and ECG parameters were entered in an electronic case report form (eCRF), and extensive plausibility checks were performed. We tested the hypothesis that fetal exposure to maternal QT-prolonging medication is associated with a prolonged neonatal QT interval using linear regression analysis. Potentially confounding variables (birth weight, gestational age, birth mode, and age at ECG) were included using a multivariate model. In a sensitivity analysis, we tested whether maternal medication is associated with a QTc interval larger than 450 ms using logistic regression analysis. The same set of confounder variables was included. All descriptive statistics and univariate and multivariate regression analyses were computed using IBM SPSS statistics (version 28).
3 Results
Out of a total of 3,181 participants in the KUNO-Kids health study, 631 were excluded because of incomplete or unclassifiable documentation of maternal medication or a lack of ECG recording. Therefore 2,550 participants were included in this analysis. A total of 22 additional participants had to be excluded in the multivariate analysis due to the incomplete dataset on perinatal demographics. A total of 2,528 participants were included in the multivariate analysis.
On average, mothers were 33 years old (SD = 4.4 years, range 18–49 years). An immigration background was present in 18.2% (n = 464) of the families. The proportion of caesarean delivery was 29.1% (n = 742). The mean birth weight of the neonates was 3366 g (SD = 503 g, range 1650 g–4985 g), and the mean gestational age was 39.5 weeks (SD = 1.5 weeks, range 33.3–43.1 weeks). An estimate of 48.7% of infants were female and 51.3% were male. The mean age of the neonates at the time of ECG was 1.9 days (SD = 0.82 days, range 1–7 days). The mean heart rate was 117.6 beats per minute (SD = 17.0, range 70–195), and QRS duration was 59 ms on average (SD = 4.3 ms, range 40–110 ms). The cohort characteristics are summarized in Table 1.
A total of 3,990 entries for maternal medication were reported and classified. In total, 315 drugs were classified as representing a known risk for QT interval prolongation. A total of 105 participants were assigned to the drug exposure group (Med+) with a daily maternal intake of a potential QT interval-prolonging drug in the last month of pregnancy. Table 2 shows a summary of the drugs assigned to the Med+ group. A total of 2,445 participants were assigned to the non-exposure group.
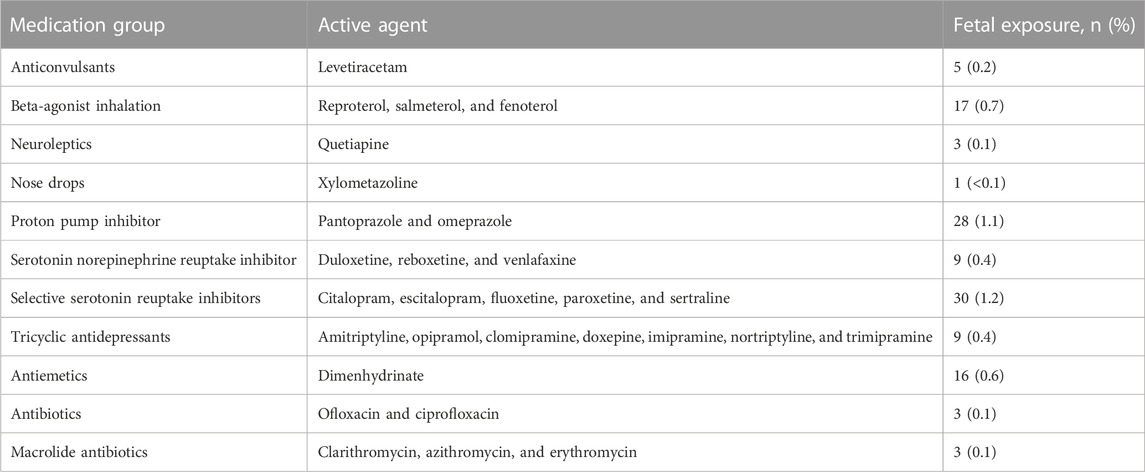
TABLE 2. Drugs assigned to the group with the known risk of QT interval prolongation and the number of participants with peripartum fetal exposure.
Overall, mean neonatal QTc was 414 ms (SD = 24.4 ms, range 321–549 ms). The mean QTc of participants with or without exposure to maternal QT interval-prolonging medication was 412 ms (SD = 20.4 ms, range 349–487 ms) and 414 ms (SD = 24.6 ms, range 321–549 ms), respectively, (Figure 1). Using 450 ms as the cut-off, the QTc interval was prolonged in 4.6% (n = 117) of the participants (4.6%), with a rate of 4.7% (n = 114) in the Med- group and 2.9% (n = 3) in the Med+ group. Univariate analysis showed no statistically significant association between the neonatal QT interval and exposure to maternal medication (regression coefficient −2.62, p = 0.288). To control the possible influence of confounding variables, we performed a multivariate analysis, including birth weight, gestational age, birth mode, and age of the neonate at the time of the ECG recording. Table 3 shows that even after controlling these confounding variables, there was no significant association between exposure to maternal medication and the neonatal QT interval (regression coefficient −3.55, p = 0.146). Each variable considered as a confounder showed a statistically significant association with the postnatal QT interval using the multivariate model. In detail, the postnatal QT interval was decreasing with increasing gestational age (regression coefficient −2.67, p < 001) and with increasing age of the infants at the time of the ECG (regression coefficient −2.37, p < 0.001). An increase in birth weight was associated with a longer QT interval (regression coefficient 0.003, p = 0.003). A caesarean section was associated with a significantly longer postnatal QT interval (regression coefficient 3.03, p = 0.006).
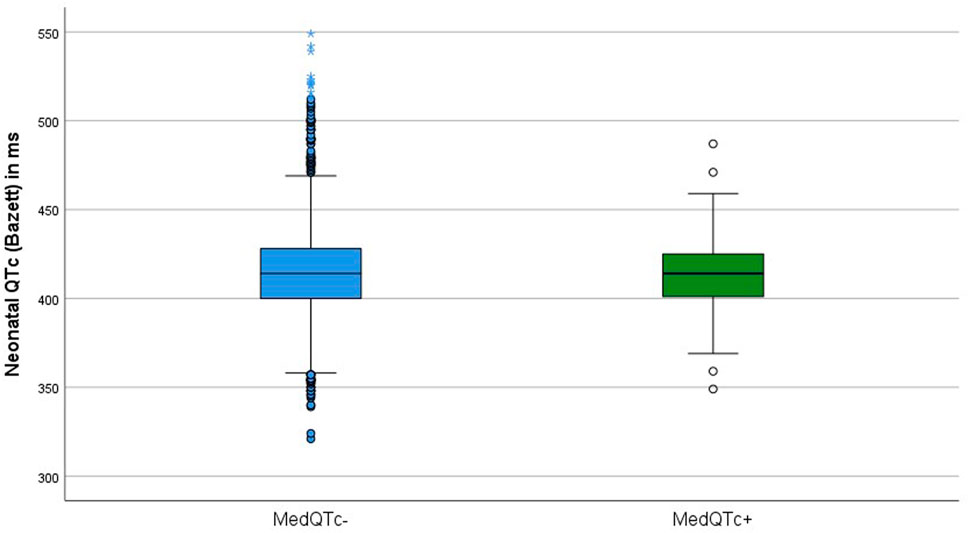
FIGURE 1. Boxplot of the neonatal QTc intervals of neonates prenatally exposed (Med+ n = 105) and unexposed (Med- n = 2,445) to QT interval-prolonging maternal medication.
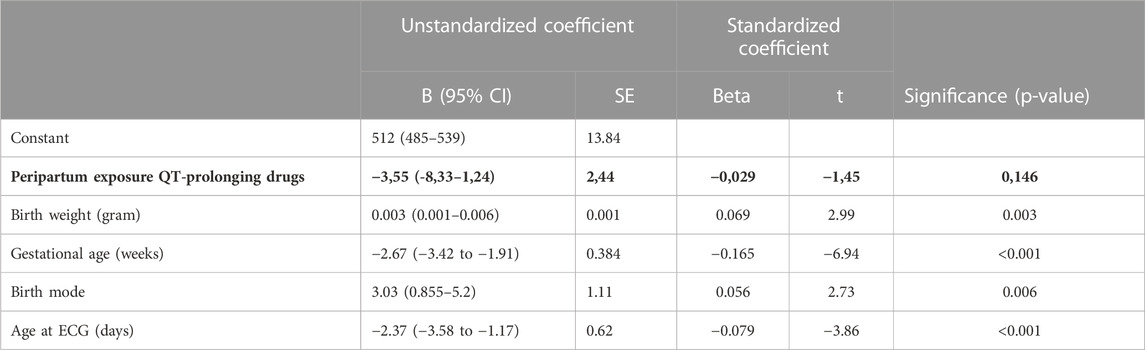
TABLE 3. Multivariate linear regression analysis on the effect of exposure to peripartum maternal QT-prolonging drugs on the neonatal QT interval (highlighted in bold letters), correcting for perinatal confounders such as birth weight (in gram), gestational age (in weeks), the mode of delivery (caesarean section/spontaneous delivery), and age of the neonate at the ECG recording (in days). Total n = 2,528 participants.
Due to limitations to the sample size, subgroup analyses were performed for PPIs, SSRIs, and dimenhydrinate only, with 26, 29, and 16 exposed participants, respectively. Compared with unexposed neonates, no subgroup showed significantly prolonged QT intervals in the neonatal ECG. After exposure ro PPIs and SSRIs the mean postnatal QTc was shorter, with 408 ms (SD = 15 ms, range 369 ms–435 ms) and 408 ms (SD = 27 ms, range 349 ms–471 ms), respectively. After maternal intake of dimenhydrinate, the mean neonatal QTc interval was slightly longer at 419 ms (SD = 11.3 ms, range 398 ms–433 ms). In multivariate analysis, these differences were also not significant for any subgroup (Table 4).
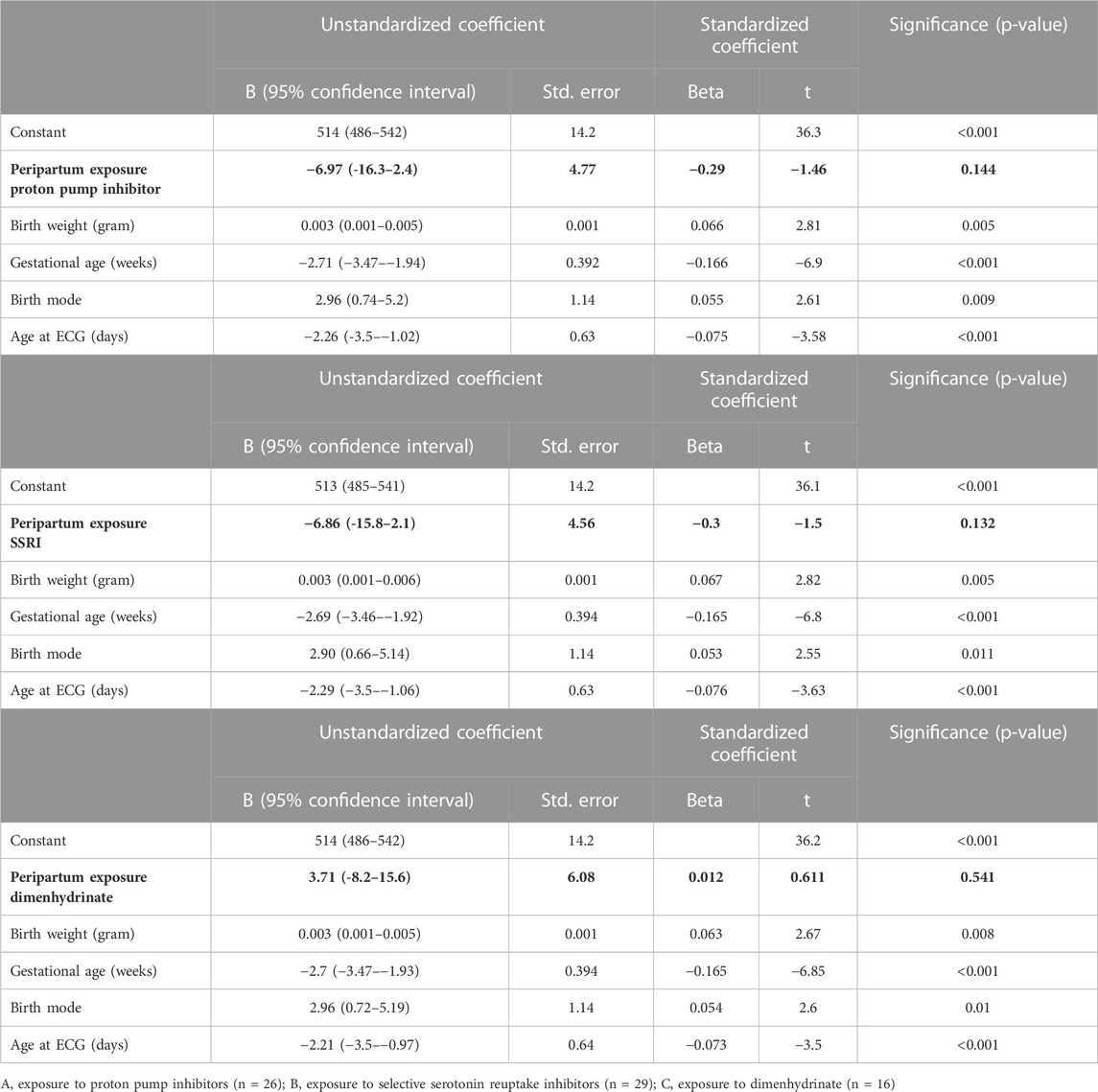
TABLE 4. Multivariate subgroup analysis of the effect of exposure to the respective peripartum maternal drugs on the neonatal QT interval (highlighted in bold letters), correcting for perinatal confounders such as birth weight (in gram), gestational age (in weeks), the mode of delivery (caesarean section/spontaneous delivery), and age of the neonate at the ECG recording (in days).
Increased genetic susceptibility to drugs that induce QT interval prolongation in some individuals at risk could lead to an increased rate of QT prolongation without significantly affecting the QT interval of the whole group of exposed neonates. To test whether exposure to maternal QT prolonging medication is associated with an increased rate of prolonged neonatal QT interval, a logistic regression analysis was additionally performed using a QTc of 450 ms as the cut-off value. In this analysis, controlling gestational age, birth weight, birth mode, and age at ECG as confounders, exposure to maternal medication was not associated with an increased chance of neonatal QTc prolongation above 450 ms (OR (odds ratio) 0.34, 95% confidence interval 0.083–1,415, p = 0.14) (Table 5).
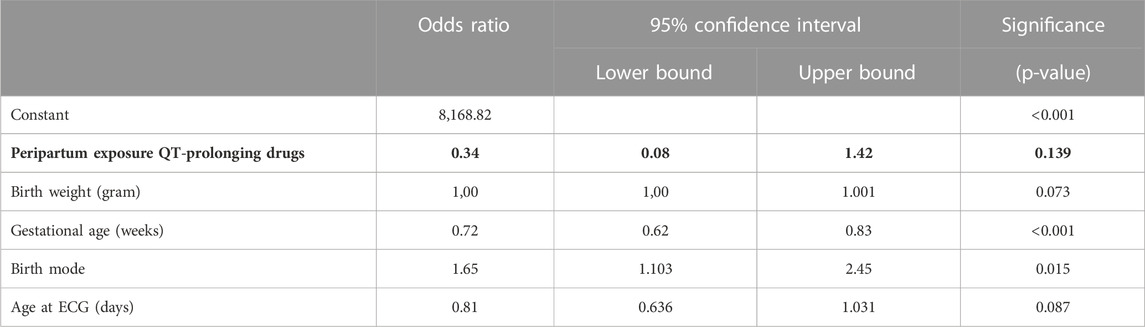
TABLE 5. Logistic regression analysis on the effect of exposure to peripartum maternal QT-prolonging drugs (highlighted in bold letters) on the rate of neonatal QT interval prolongation (QTc > 450 ms), correcting for perinatal confounders such as birth weight (in gram), gestational age (in weeks), the mode of delivery (caesarean section/spontaneous delivery), and the age of the neonate at the ECG recording (in days).
4 Discussion
Through various pathophysiological mechanisms, drugs can lead to QT interval prolongation and subsequently to an increased risk of critical arrhythmias, mostly through interactions with cardiac ion channels. Studies in adults estimate that 5%–7% of ventricular tachycardia, ventricular fibrillation, and sudden cardiac death are due to drug-induced LQTS and torsade de pointes (Kallergis et al., 2012). Maternal drug use during pregnancy may result in fetal exposure via the placenta.
The aim of this prospective birth cohort study was to investigate the influence of the peripartum maternal use of potential QT interval-prolonging medication on the neonatal ECG. As a main finding, there was no significant effect on the mean neonatal QT interval and on the rate of infants with QT prolongation.
In our cohort, a relevant number of infants (n = 105, 4.1%) were exposed to potential QT interval-prolonging drugs by maternal intake in the last 4 weeks of pregnancy, for which placental transfer is known. Most frequent was the maternal use of SSRIs, PPIs, beta-agonist inhalation, and dimenhydrinate, some of which are common over-the-counter medications in Germany.
Despite heterogeneous exposure due to the use of a variety of different drugs in our cohort, in a first general approach, we tested the effect of all potential QT interval-prolonging drugs in a combined analysis. No significant change in the mean neonatal QT interval after exposure to maternal potential QT interval prolonging-drugs was found. The rate of neonates with a QTc interval greater than 450 ms was also not significantly higher in the exposed group than that in the unexposed neonates. This cut-off value has been recommended by Saul et al. (2014) for follow-up ECGs and further evaluation. The majority of ECGs in our study were recorded on the second and third days after birth (mean age = 1.9 days, SD = 0.82 days). Therefore, we cannot exclude that possible effects on the neonatal QT interval may already have disappeared over time due to decreasing drug levels in the neonate. In our institution, most neonates are discharged from the maternity ward on the third day of birth. Thus, our study indicates that at this time point, there was no increased risk of arrhythmia or need for further diagnostic evaluation due to drug-induced QT prolongation for the infants.
Second, since numerous additional factors may have an influence on the neonatal QT interval, we performed a multivariate analysis to correct for confounding factors. This analysis also showed no significant influence of maternal QT interval-prolonging medication on the neonatal QT interval. In contrast, there was a significant association between the confounding factors of gestational age, birth weight, birth mode, and timing of the ECG with neonatal QT intervals in our setting.
Transient postnatal QT interval prolongation and high dynamics in the first few days with normalization over the course of days have been described (Schwartz et al., 1982; Simma et al., 2020); thus not surprisingly, in this analysis, the neonatal QT interval decreased with increasing postnatal age of the neonates. An influence of gestational age on the QT interval has been studied previously, with various effects depending on the level of prematurity and the timing of the ECG recording (Séguéla et al., 2012; Marcellino et al., 2021; Pærregaard et al., 2021). The study of Marcellino et al. showed no difference in the QT interval with respect to gestational age. Pærregaard et al. demonstrated a slight prolongation of the QT interval in infants at >41 compared with <35 weeks of gestation. In contrast, Séguéla et al. found that in preterm infants, QT intervals increased up to a peak at 32 weeks of gestation and became shorter again thereafter. In our study, neonates from 33 to 43 weeks of gestation were included, and in the multivariate approach similar to the latter study, there seemed to be a decrease in the postnatal QT interval with increasing gestational age. Furthermore, in our analysis, increasing birth weight was associated with an increasing QT interval, but this effect was rather small, with an average of a 3-ms increase in the QT interval per kilogram of weight. Previous studies did not find such a difference (Séguéla et al., 2012; Pærregaard et al., 2021). One possible explanation for the effect in our data might be that we did not correct for gestational diabetes of the mother. After this complication of pregnancy, prolonged QT and QT dispersion have been described and neonates also tend to be heavier (Al-Biltagi et al., 2021). Interestingly, our analysis seemed to show a difference in the neonatal QT interval with respect to the mode of delivery, spontaneous delivery vs. cesarean section, although the observed effect was small (increase in QTc by 3.03 ms in infants after cesarean section vs. spontaneous delivery). In the logistic regression analysis, the rate of neonates with a QTc interval above 450 ms was also significantly increased in children born by cesarean section compared to spontaneous delivery (odds ratio 1.65; Table 5). Several underlying causes are conceivable due to complex interactions, such as perinatal stress and corresponding neurohumoral activation, maternal medication during anesthesia, and an increased risk of neonatal respiratory distress. Further analyses including these factors and differentiating between primary and secondary cesarean sections are needed to evaluate whether the birth mode indeed has an impact on the neonatal QT interval and what mechanisms might cause such an impact. To the best of our knowledge, no data have been published on this topic. As gestational age, birth weight, birth mode, and timing of the ECG were not the primary exposure variables in this analysis, these findings need to be verified in further studies.
Due to the heterogeneity of maternal drugs and the sample size of the respective medication groups, we performed a subgroup analysis for SSRIs, PPIs, and dimenhydrinate only. Although there are case reports of neonates with the prolonged QT interval associated with the maternal SSRI intake (Dubnov et al., 2005; Leerssen et al., 2019), two case–control studies performed on this topic had inflicting results. Although Dubnov-Raz et al. found a significant increase in the QT interval in intrauterine-exposed neonates, a recent study by Lindsay–Sutherland showed no differences in the two groups (Dubnov-Raz et al., 2008; Lindsay-Sutherland, 2021). The results in our cohort go in line with the latter study, showing no significant effect of SSRI exposure. No cases of neonatal QT interval prolongation after the maternal use of PPIs or dimenhydrinate have been described, and to the best of our knowledge, no studies addressing the effect of intrauterine exposure to these agents on the neonatal QT time have been conducted so far. In our analysis, there was no significant effect of intrauterine exposure on the neonatal QT interval in these two subgroups. Limiting in our setting, despite the considerably large cohort, the number of exposed infants in the respective subgroups was small.
This is, to the best of our knowledge, the first cohort study to investigate the possible effect of maternal medication with the known risk of QT interval prolongation in a multivariate analysis, taking important perinatal confounding factors into account. In summary, in our cohort, there was no significant effect on the neonatal QT interval, either in the overall or in the subgroup analyses, which adds important data on possible effects of maternal therapy with regard to this scarcely investigated neonatal adverse effect. Further studies with larger case numbers are necessary in order to have a more substantial database on the safety of these therapies and to clarify some of the contradictory previous study results.
In this analysis, the maternal medication use more than 4 weeks before delivery was defined as non-exposure (Med-). Although it is unlikely, it cannot be excluded that substances with a very long half-life still have residual activity and thus lead to fetal exposure at the time of birth. In addition, the prolonged use of long-acting drugs and subsequent accumulation can lead to increased fetal exposure.
As genetic variants are known to influence susceptibility for acquired QT interval prolongation (Kallergis et al., 2012), our results cannot be generalized to populations with different ethnic distributions. Furthermore, our results might be biased because of the single-center study design as maternal diseases and medication intake in our cohort may not match those of the general population. Future studies will need to take these factors into account when discussing individual aspects of medication safety.
5 Conclusion
A relevant number of women take potential QT-interval prolonging drugs during pregnancy and until delivery.
In our cohort, intrauterine exposure showed no effect on the neonatal QT interval and on the rate of infants with QT prolongation.
Further studies with larger case numbers are needed in order to have a more substantial database on the safety of these therapies.
Several perinatal confounders might have an influence on the neonatal QT interval. More data are needed to consider them appropriately in future studies or interpretation of neonatal ECGs in the clinical setting.
Data availability statement
The raw data supporting the conclusions of this article will be made available by the authors, without undue reservation.
Ethics statement
The studies involving human participants were reviewed and approved by the Ethics Committee of the University of Regensburg, Regensburg, Germany. Written informed consent to participate in this study was provided by the participants’ legal guardian/next of kin.
Author contributions
Study design: MK, CA, HM, and SG. Data collection: HM, MD, AP, SG, MK, MM, and AK. Statistical analysis and data interpretation: HM, SG, SB, and MK. Manuscript writing: HM, MD, SW, SG, and MK. Final approval: HM, MK, MM, SW, SB, and SG. All authors contributed to the article and approved the submitted version.
Funding
The KUNO-Kids study is funded by research grants of the EU (HEALS: 603946) and the German Federal Ministry for Education and Research (SYSINFLAME: 01ZX1306E). Further financial support was provided by the University Children’s Hospital of the University of Regensburg (KUNO-Clinics) and the clinic “St. Hedwig” (Hospital “Barmherzige Brüder Regensburg”).
Acknowledgments
The authors would like to thank all families who participated in the KUNO-Kids birth cohort study and all medical students, nurses, midwives, physicians, and researchers who facilitated the recruitment of participants and data assessment. Furthermore, they would like to thank all members of the KUNO-Kids study group: Andreas Ambrosch (Institute of Laboratory Medicine, Microbiology and Hygiene, Barmherzige Brüder Hospital, Regensburg, Germany), Petra A. Arndt (ZNL Transfer Center of Neuroscience and Learning, University of Ulm, Ulm, Germany), Andrea Baessler (Department of Internal Medicine II, Regensburg University Medical Center, Regensburg, Germany), Mark Berneburg (Department of Dermatology, University Medical Centre Regensburg, Regensburg, Germany), Stephan Böse-O’Reilly (University Children’s Hospital Regensburg (KUNO), Hospital St. Hedwig of the Order of St. John, Regensburg, Germany), Romuald Brunner (Clinic of Child and Adolescent Psychiatry, Psychosomatics and Psychotherapy, Bezirksklinikum Regensburg (medbo), Regensburg, Germany), Wolfgang Buchalla (Department of Conservative Dentistry and Periodontology, University Hospital Regensburg, University of Regensburg, Regensburg, Germany), Sara Fill Malfertheiner (Clinic of Obstetrics and Gynecology St. Hedwig, University of Regensburg, Regensburg, Germany), André Franke (Institute of Clinical Molecular Biology, Christian-Albrechts-University of Kiel, Kiel, Germany), Sebastian Häusler (Clinic of Obstetrics and Gynecology St. Hedwig, University of Regensburg, Regensburg, Germany), Iris Heid (Department of Genetic Epidemiology, University of Regensburg, Regensburg, Germany), Stefanie Heinze (Bavarian Health and Food Safety Authority (LGL), Munich, Germany), Wolfgang Högler (Department of Pediatrics and Adolescent Medicine, Johannes Kepler University Linz, Linz, Austria), Sebastian Kerzel (Department of Pediatric Pneumology and Allergy, University Children’s Hospital Regensburg (KUNO), Hospital St. Hedwig of the Order of St. John, Regensburg, Germany), Michael Koller (Center for Clinical Studies, University Hospital Regensburg, Regensburg, Germany), Michael Leitzmann (Department of Epidemiology and Preventive Medicine, University of Regensburg, Regensburg, Germany), David Rothfuß (City of Regensburg, Coordinating Center for Early Interventions, Regensburg, Germany), Wolfgang Rösch (Department of Pediatric Urology, University Medical Center, Regensburg, Germany), Bianca Schaub (Pediatric Allergology, Department of Pediatrics, Dr. von Hauner Children’s Hospital, University Hospital, LMU Munich, Munich, Germany), Birgit Seelbach-Göbel (Clinic of Obstetrics and Gynecology St. Hedwig, University of Regensburg, Regensburg, Germany), Stephan Weidinger (Department of Dermatology, Venereology and Allergy, University Hospital Schleswig-Holstein, Campus Kiel, Kiel, Germany), and Sven Wellmann (Department of Neonatology, University Children’s Hospital Regensburg (KUNO), Hospital St. Hedwig of the Order of St. John, Regensburg, Germany).
Conflict of interest
The authors declare that the research was conducted in the absence of any commercial or financial relationships that could be construed as a potential conflict of interest.
Publisher’s note
All claims expressed in this article are solely those of the authors and do not necessarily represent those of their affiliated organizations, or those of the publisher, the editors, and the reviewers. Any product that may be evaluated in this article, or claim that may be made by its manufacturer, is not guaranteed or endorsed by the publisher.
References
Al-Biltagi, M., El razaky, O., and El Amrousy, D. (2021). Cardiac changes in infants of diabetic mothers. WJD 12, 1233–1247. doi:10.4239/wjd.v12.i8.1233
Arnestad, M., Crotti, L., Rognum, T. O., Insolia, R., Pedrazzini, M., Ferrandi, C., et al. (2007). Prevalence of long-QT syndrome gene variants in sudden infant death syndrome. Circulation 115, 361–367. doi:10.1161/CIRCULATIONAHA.106.658021
Bibawy, J. N., Parikh, V., Wahba, J., Barsoum, E. A., Lafferty, J., Kowalski, M., et al. (2013). Pantoprazole (proton pump inhibitor) contributing to torsades de Pointes storm. Circ Arrhythmia Electrophysiol. 6. doi:10.1161/CIRCEP.112.000101
Brandstetter, S., Toncheva, A. A., Niggel, J., Wolff, C., Gran, S., Seelbach-Göbel, B., et al. (2019). KUNO-kids birth cohort study: Rationale, design, and cohort description. Mol. Cell. Pediatr. 6, 1. doi:10.1186/s40348-018-0088-z
Dubnov, G., Fogelman, R., and Merlob, P. (2005). Prolonged QT interval in an infant of a fluoxetine treated mother. Archives Dis. Child. 90, 972–973. doi:10.1136/adc.2004.064618
Dubnov-Raz, G., Juurlink, D. N., Fogelman, R., Merlob, P., Ito, S., Koren, G., et al. (2008). Antenatal use of selective serotonin-reuptake inhibitors and QT interval prolongation in newborns. Pediatrics 122, e710–e715. doi:10.1542/peds.2008-0658
Fukushima, N., Nanao, K., Fukushima, H., Namera, A., and Miura, M. (2016). A neonatal prolonged QT syndrome due to maternal use of oral tricyclic antidepressants. Eur. J. Pediatr. 175, 1129–1132. doi:10.1007/s00431-016-2722-x
Funk, K. A., and Bostwick, J. R. (2013). A comparison of the risk of QT prolongation among SSRIs. Ann. Pharmacother. 47, 1330–1341. doi:10.1177/1060028013501994
Gau, J.-T., Yang, Y.-X., Chen, R., and Kao, T.-C. (2012). Uses of proton pump inhibitors and hypomagnesemia: Gastric acid suppressants and magnesium. Pharmacoepidemiol Drug Saf. 21, 553–559. doi:10.1002/pds.3224
Kallergis, E. M., Goudis, C. A., Simantirakis, E. N., Kochiadakis, G. E., and Vardas, P. E. (2012). Mechanisms, risk factors, and management of acquired long QT syndrome: A comprehensive review. Sci. World J. 2012, 212178–8. doi:10.1100/2012/212178
Leerssen, E. C. M., Tak, R. O., and Breur, J. M. P. J. (2019). Severe transient neonatal long QT syndrome due to maternal paroxetine usage: A case report. Cardiol. Young 29, 1300–1301. doi:10.1017/S1047951119001938
Lindsay-Sutherland, M. (2021). The effect of antenatal selective serotonin reuptake inhibitor exposure on the corrected QT interval of neonates. J. Perinatol. 41, 1515–1518. doi:10.1038/s41372-021-01057-y
Marcellino, A., Luchetti, J., Raponi, M., Falsaperla, R., Pirone, C., Fares, M. K., et al. (2021). Single-centre retrospective analysis of the best timing for the QTc interval length assessment in neonates. BMJ Paediatr. Open 5, e001026. doi:10.1136/bmjpo-2021-001026
Moore, J., Flynn, R. J., Sampaio, M., Wilson, C. M., and Gillon, K. R. W. (1989). Effect of single-dose omeprazole on intragastric acidity and volume during obstetric anaesthesia. Anaesthesia 44, 559–562. doi:10.1111/j.1365-2044.1989.tb11441.x
Pærregaard, M. M., Hartmann, J., Vøgg, O., Pietersen, A., Boyd, H. A., Raja, A. A., et al. (2022). The impact of maternal age on the neonatal electrocardiogram. Neonatology 119, 629–637. doi:10.1159/000524439
Pærregaard, M. M., Hvidemose, S. O., Pihl, C., Sillesen, A.-S., Parvin, S. B., Pietersen, A., et al. (2021). Defining the normal QT interval in newborns: The natural history and reference values for the first 4 weeks of life. EP Eur. 23, 278–286. doi:10.1093/europace/euaa143
Poluzzi, E., Raschi, E., Godman, B., Koci, A., Moretti, U., Kalaba, M., et al. (2015). Pro-arrhythmic potential of oral antihistamines (H1): Combining adverse event reports with drug utilization data across Europe. PLoS ONE 10, e0119551. doi:10.1371/journal.pone.0119551
Saul, J. P., Schwartz, P. J., Ackerman, M. J., and Triedman, J. K. (2014). Rationale and objectives for ECG screening in infancy. Heart rhythm. 11, 2316–2321. doi:10.1016/j.hrthm.2014.09.047
Schwartz, P., Garson, A., Paul, T., Stramba-Badiale, M., Vetter, V. L., Wren, C., et al. (2002). Guidelines for the interpretation of the neonatal electrocardiogram A task force of the European Society of Cardiology. Eur. Heart J. 23, 1329–1344. doi:10.1053/euhj.2002.3274
Schwartz, P. J., Montemerlo, M., Facchini, M., Salice, P., Rosti, D., Poggio, G., et al. (1982). The QT interval throughout the first 6 months of life: A prospective study. Circulation 66, 496–501. doi:10.1161/01.CIR.66.3.496
Schwartz, P. J., Priori, S. G., Spazzolini, C., Moss, A. J., Vincent, G. M., Napolitano, C., et al. (2001). Genotype-phenotype correlation in the long-QT syndrome: Gene-specific triggers for life-threatening arrhythmias. Circulation 103, 89–95. doi:10.1161/01.CIR.103.1.89
Schwartz, P. J., Stramba-Badiale, M., Crotti, L., Pedrazzini, M., Besana, A., Bosi, G., et al. (2009). Prevalence of the congenital long-QT syndrome. Circulation 120, 1761–1767. doi:10.1161/CIRCULATIONAHA.109.863209
Schwartz, P. J., Stramba-Badiale, M., Segantini, A., Austoni, P., Bosi, G., Giorgetti, R., et al. (1998). Prolongation of the QT interval and the sudden infant death syndrome. N. Engl. J. Med. 338, 1709–1714. doi:10.1056/NEJM199806113382401
Séguéla, P.-E., Rozé, J.-C., and Gournay, V. (2012). Evolution of the QT interval in premature infants: A preliminary study. Cardiol. Young 22, 430–435. doi:10.1017/S1047951111001958
Simma, A., Potapow, A., Brandstetter, S., Michel, H., Melter, M., Seelbach-Göbel, B., et al. (2020). Electrocardiographic screening in the first days of life for diagnosing long QT syndrome: Findings from a birth cohort study in Germany. Neonatology 117, 756–763. doi:10.1159/000511572
Tester, D. J., and Ackerman, M. J. (2007). Postmortem long QT syndrome genetic testing for sudden unexplained death in the young. J. Am. Coll. Cardiol. 49, 240–246. doi:10.1016/j.jacc.2006.10.010
Tester, D. J., Medeiros-Domingo, A., Will, M. L., Haglund, C. M., and Ackerman, M. J. (2012). Cardiac Channel molecular autopsy: Insights from 173 consecutive cases of autopsy-negative sudden unexplained death referred for postmortem genetic testing. Mayo Clin. Proc. 87, 524–539. doi:10.1016/j.mayocp.2012.02.017
Wilde, A. A. M., Amin, A. S., and Postema, P. G. (2022). Diagnosis, management and therapeutic strategies for congenital long QT syndrome. Heart 108, 332–338. doi:10.1136/heartjnl-2020-318259
Keywords: acquired long QT, neonate, KUNO-Kids, maternal medication, ECG
Citation: Michel H, Potapow A, Dechant M-J, Brandstetter S, Wellmann S, Köninger A, Melter M, Apfelbacher C, Kabesch M, Gerling S and the KUNO-Kids study group (2023) Effect of QT interval-prolonging drugs taken in pregnancy on the neonatal QT interval. Front. Pharmacol. 14:1193317. doi: 10.3389/fphar.2023.1193317
Received: 24 March 2023; Accepted: 18 July 2023;
Published: 07 August 2023.
Edited by:
Catherine M. T. Sherwin, Wright State University, United StatesReviewed by:
Christof Schaefer, Charité University Medicine Berlin, GermanyAshwin Karanam, Pfizer, United States
Copyright © 2023 Michel, Potapow, Dechant, Brandstetter, Wellmann, Köninger, Melter, Apfelbacher, Kabesch and Gerling. This is an open-access article distributed under the terms of the Creative Commons Attribution License (CC BY). The use, distribution or reproduction in other forums is permitted, provided the original author(s) and the copyright owner(s) are credited and that the original publication in this journal is cited, in accordance with accepted academic practice. No use, distribution or reproduction is permitted which does not comply with these terms.
*Correspondence: Holger Michel, holger.michel@barmherzige-regensburg.de