- 1Chengdu University of Traditional Chinese Medicine, Chengdu, China
- 2Hospital of Chengdu University of Traditional Chinese Medicine, Chengdu, China
The imbalance of gastrointestinal microbial composition has been identified as the main factor of chronic inflammatory diseases. At present, probiotics have a beneficial effect on the microbial composition of the human gastrointestinal tract, but it is still controversial and the specific mechanism is unknown. The purpose of this network meta-analysis is to compare the mechanism of different probiotics on ulcerative colitis. PubMed, Embase, and Web of Science were searched till 16 November 2022. The SYRCLE risk bias assessment tool was used to assess the quality of the research studies. A total of 42 studies, 839 ulcerative colitis models, and 24 kinds of probiotics were finally included. The results showed that L. rhamnosus has the best effect in relieving weight loss and improving the Shannon index in the ulcerative colitis model. E. faecium has the best effect in reducing colon injury; L. reuteri has the best effect in reducing the DAI; L. acidophilus has the best effect in reducing the HIS index and increasing the expression of tight junction protein ZO-1; and L. coryniformis has the best effect in reducing the content of serum pro-inflammatory factor TNF-α. It indicated that probiotics can improve ulcerative colitis by improving histopathological manifestations, reducing inflammatory reaction, and repairing the mucosal barrier, and different probiotics showed different effects. However, considering the limitations of this study, preclinical studies that require more large samples and high-quality and more reliable and rigorous experimental designs and reports need to be conducted in the future.
Systematic Review Registration: https://www.crd.york.ac.uk/prospero/#record details, identifier CRD42022383383.
1 Introduction
Ulcerative colitis (UC) is a chronic non-specific intestinal inflammatory disease characterized by inflammatory changes of colorectal mucosa. Its etiology is not clear, and it is difficult to cure and easy to become cancerous (Ungaro et al., 2017). Epidemiological research showed that the incidence of UC is rising all over the world (Charles et al., 2010). It is generally believed that the pathogenesis of UC is the result of the interaction of heredity, immunity, microorganism, and environment. At present, the traditional treatments for UC are mainly sulfasalazine, 5-aminosalicylic acid ester, glucocorticoid, and immunosuppressants (Travis et al., 2008; Kornbluth and Sachar, 2010), but their curative effects are limited, and there are many adverse reactions such as headache, nausea, and abdominal pain, and it is expensive that most patients cannot afford it. Therefore, it is a new trend to seek a safer and more effective adjuvant therapy or alternative therapy for UC.
Intestinal flora maintains the survival and metabolism of the body by ingesting nutrients in the body, which plays an important role in regulating the nutrition, metabolism, immunity, and pathogen defense of the host, and it is an important barrier to maintain the internal environment of the body. There are 100 trillion kinds of different microorganisms in the human intestine, including bacteria, fungi, viruses, and protozoa, which constitute the intestinal microflora (Zhang et al., 2020). Intestinal flora is considered to be one of the important organs symbiotic with the body, which is important to the occurrence and development of diseases. Intestinal flora imbalance can cause systemic inflammatory response. Probiotics are a vital component of intestinal flora, including Bacteroides, Lactobacillus, and Bifidobacterium. Supplementation of probiotics can improve the intestinal environment, including regulating the composition of intestinal flora, preventing colonization of pathogenic bacteria, affecting bacterial metabolism, and regulating immune response (Zhao et al., 2020).
There is significant imbalance of intestinal flora in UC patients, which is characterized by the decrease of the types and universality of intestinal microorganisms and the difference of their spatial distribution. A series of metabolites produced by intestinal flora also affect the occurrence and development of UC (Franzosa et al., 2019). A large number of animal and clinical studies have shown that probiotics can effectively alleviate the clinical symptoms and pathological damage of UC, improve the inflammatory reaction, and restore the balance of intestinal flora (Srutkova et al., 2015; Boruel et al., 2002), and have been widely used in the treatment of gastrointestinal diseases, but probiotics do not always provide benefits to patients, the effects of different strains are quite different, and the mechanism of action is also different, which is closely related to the type and stage of the disease, the strains and doses of probiotics used. However, at present, the functions and mechanisms of different probiotics have not been discussed. In this study, the effects of 24 probiotics on the DSS-induced UC mouse model were compared, and the advantages and disadvantages of different probiotics in the treatment of ulcerative colitis were summarized, which provided evidence for the selection of clinical probiotic strains.
2 Methods
The protocol of this network meta-analysis was registered on the International Prospective Register of Systematic Reviews (PROSPERO) (Registration ID: CRD 42022383383). The whole process is based on PRISMA guidelines (Moher et al., 2015).
2.1 Search strategy
We searched PubMed, Embase, and Web of Science for animal experiments on the treatment of ulcerative colitis with probiotics before 16 November 2022. The search keywords included “Probiotics,” “Ulcerative colitis,” and “Animals.” The search was limited to the English language. The specific search strategies can be obtained in Supplementary Table S1.
2.2 Eligibility criteria
Inclusion criteria: population (P): animal model of ulcerative colitis induced by DSS; intervention (I): the intervention of the experimental group was administration of a single probiotic, by gavage or intragastric, with no restrictions on the intervention dose and intervention period; comparison (C): the control group did not intervene or placebo intervened; outcomes (O): the main outcome indicators include weight change (WC), colon length (CL), disease activity index (DAI), and historical score (HIS).
Exclusion criteria: 1) vitro studies; 2) vivo studies with humans; 3) congresses, editorials, letters, case reports, and review works; 4) papers published in a language other than English; and 5) duplicate, abstracts, unpublished reports, or incomplete data and characteristic have been disregarded.
2.3 Data extraction and quality assessment
The two authors screened the literature according to the inclusion and exclusion standard independently, and then, they extracted the following information: author, publication year, animal species (sex, n, weight), UC model induction method, study design (probiotics, administration, dose, and duration), and outcome indicators. If there were different doses of the same probiotic, the dose with the best effect was selected. When there was only SEM but no SD in the text, we converted it by the formula SD = SEM*√n (Lee et al., 2015). We used WebPlotDigitizer 4.5 software (https://automeris.io/WebPlotDigitizer) to quantify the data of that only have pictures in the text. We had tried to contact the original author when the data were unavailable. We used the SYRCLE Risk Bias Tool to evaluate the risk of bias (Hooijmans et al., 2014), including sequence generation, baseline characteristics, allocation concealment, random housing, blinding of outcome assessors, random outcome assessment, blinding of experimentalist, incomplete outcome data, selective outcome reporting, and other sources of bias, every aspect classified as high risk of bias and low risk of bias or unclear. In case of any doubt in the aforementioned process, a third author was involved.
2.4 Outcomes
The primary outcomes were WC, CL, DAI, and HIS. The secondary outcomes were tumor necrosis factor-α (TNF-α), zonula occlusion ns-1 (ZO-1), and Shannon.
2.5 Data synthesis and statistical analysis
We used R 4.2.2 to analyze the data, and Stata16 was used to make a network evidence diagram and comparison-correction funnel diagram. Mean difference (MD) and 95% credible intervals (CrIs) were used to assess continuous outcomes, except TNF-α which used standard mean difference (SMD) and 95% credible intervals (CrIs). The network meta-analysis was carried out based on the Markov chain-Monte Carlo method in the Bayesian framework, and 4 Markov chains, 1 thinning interval, 20,000 burn-in, and 50,000 iterations were set. The convergence degree of the model is satisfactory when the potential scale reduction factor (PSRF) tends to 1(Supplementary Figure S1). When there was a closed loop, the node analysis model was used to test the inconsistency.
3 Results
3.1 Study selection
A total of 2,707 studies were obtained, 291 studies duplicates were removed, 2,273 studies were excluded after reading the title/abstract, and 65 studies were retained after reading the full text according to the PICO inclusion criteria, and then, through further screening, 1 study was repeatedly published, we were unable to obtain the full text from 12 studies, and 10 studies had incomplete data. Finally, 42 studies were included in the network meta-analysis (Liu et al., 2011; Zakostelska et al., 2011; Chen et al., 2012; Chen et al., 2013; Pan et al., 2014; Elian et al., 2015; Lee et al., 2015; Cui et al., 2016; Kanda et al., 2016; Liu et al., 2016; Chae et al., 2018; Sun et al., 2018; Wang et al., 2018; Bian et al., 2019; Chae et al., 2019; Chen et al., 2019; Choi et al., 2019; Din et al., 2020; He et al., 2020; Hu et al., 2020; Sun et al., 2020; Yeo et al., 2020; Yu et al., 2020; Chen et al., 2021; Gao et al., 2021; Hu et al., 2021; Huang et al., 2021; Qu et al., 2021; Tong et al., 2021; Wang et al., 2021; Dong et al., 2022; Huang et al., 2022; Islam et al., 2022; Khan et al., 2022; Lee et al., 2022; Li et al., 2022; Lu et al., 2022; Ma et al., 2022; Qin et al., 2022; Shang et al., 2022; Wan et al., 2022; Wu et al., 2022). The specific selection flow chart was shown in Figure 1.
3.2 Study characteristics
A total of 42 articles were included, and the publication time was concentrated between 2011 and 2022. There were 839 ulcerative colitis animal models, including 460 in the intervention group and 379 in the control group. There were four different kinds of mice, and 14 studies used the BALB/c mice (Lee et al., 2022; Chen et al., 2013; Liu et al., 2016; Pan et al., 2014; Liu et al., 2011; Kanda et al., 2016; Cui et al., 2016; Chen et al., 2019; Zakostelska et al., 2011; Elian et al., 2015; Sun et al., 2018; Yu et al., 2020; Wang et al., 2018; Lee et al., 2015), with a total of 306 mice, 24 studies used the C57BL/6 mice (Chen et al., 2012; Chae et al., 2018; Bian et al., 2019; Chae et al., 2019; Din et al., 2020; He et al., 2020; Hu et al., 2020; Yeo et al., 2020; Chen et al., 2021; Gao et al., 2021; Hu et al., 2021; Huang et al., 2021; Qu et al., 2021; Tong et al., 2021; Dong et al., 2022; Huang et al., 2022; Islam et al., 2022; Khan et al., 2022; Lu et al., 2022; Ma et al., 2022; Qin et al., 2022; Shang et al., 2022; Wan et al., 2022; Wu et al., 2022), with a total of 436 mice, 2 studies used the ICR mice (Sun et al., 2018; Choi et al., 2019), with a total of 32 mice, 1 study used the Kunming mice (Wang et al., 2021), with 35 mice in total, and 1 study used the Sprague Dawley (SD) mice (Li et al., 2022), with a total of 20 mice. All studies adopted animal models of ulcerative colitis induced by dextran sulfate sodium (DSS), and the administration duration ranged from 7 days to 35 days. There were 24 kinds of probiotics in total, and seven studies used Bifidobacterium (B. bifidum) (Elian et al., 2015; Chae et al., 2018; Wang et al., 2018; Din et al., 2020; Dong et al., 2022; Lee et al., 2022; Shang et al., 2022), thirteen studies used Lactobacillus plantarum (L. plantarum) (Liu et al., 2011; Lee et al., 2015; Cui et al., 2016; Chae et al., 2019; Choi et al., 2019; Sun et al., 2020; Yu et al., 2020; Hu et al., 2021; Huang et al., 2021; Wang et al., 2021; Khan et al., 2022; Qin et al., 2022; Wu et al., 2022), two studies used Akkermansia muciniphila (AKK) (Bian et al., 2019; Qu et al., 2021), two studies used Lactobacillus fermentum (L. fermentum) (Cui et al., 2016; Chen et al., 2021), four studies used Lactobacillus rhamnosus (L. rhamnosus) (Yeo et al., 2020; Tong et al., 2021; Wang et al., 2021; Wan et al., 2022), three studies used Lactobacillus bulgaricus (L. bulgaricus) (Hu et al., 2020; Chen et al., 2021; Hu et al., 2021), and two studies used Lactobacillus paracasei (L. paracasei) (Pan et al., 2014; Huang et al., 2021). There was one study using Saccharomyces boulardii (S. boulardii) (Gao et al., 2021), Clostridium butyricum (C. butyricum) (Ma et al., 2022), Lactobacillus brevis (L. brevis) (Liu et al., 2016), Lactobacillus crispatus (L. crispatus) (Cui et al., 2016), Lactobacillus reuteri (L. reuteri) (Sun et al., 2018), Lactobacillus neoformans Lb-9 (LB-9) (Chae et al., 2019), Lactobacillus casei (L. casei) (Zakostelska et al., 2011), Lactobacillus kefiranofaciens M1 (L. kefiranofaciens M1) (Chen et al., 2012), Eurotium cristatum (E. cristatum) (Lu et al., 2022), Lactobacillus acidophilus (L. acidophilus) (Hu et al., 2020), Enterococcus durans (E. durans) (Kanda et al., 2016), Streptococcus thermophilus (S. thermophilus) (Chen et al., 2019), Tetragenococcus halophilus (T. halophilus) (Islam et al., 2022), Enterococcus faecalis (E. faecium) (Wang et al., 2021), Lactobacillus crustorum (L. crustorum), and Lactobacillus coryniformis (L. coryniformis) (Wang et al., 2021). A total of seven outcome indicators were analyzed, including WC, Cl, DAI, HIS, TNF-α, ZO-1, and Shannon. The specific information is presented in Table 1.
3.3 Risk of bias assessment
The ROB assessment processes and details of 10 projects in 42 studies are shown in Supplementary Table S2 and Figure 2. Randomization was used in 31 studies, and nine studies did not mention the use of random method, and two studies did not use randomization; all studies did not mention the hidden distribution sequence, so we considered it was unclear risk; all studies mentioned Random housing, so it was considered as low risk; and two studies mentioned the blind use of outcome assessment.
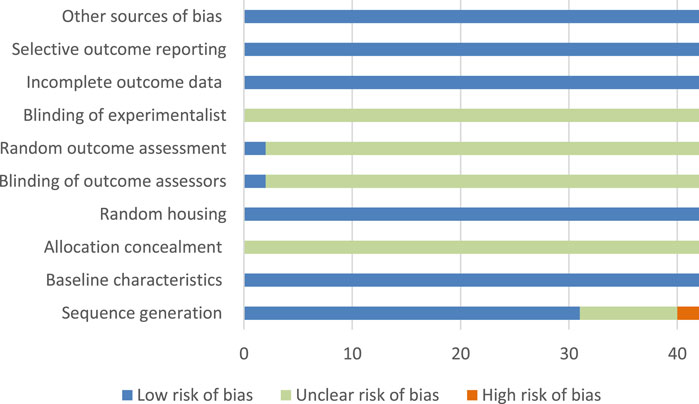
FIGURE 2. Risk of bias graph and evaluators; none of the studies mentioned the use of blind method; no research reported incomplete data, selective report bias, and other bias, so they were considered as low risk.
3.4 Outcomes
The primary outcomes were WC, CL, DAI, and HIS. The secondary outcomes were TNF-α, ZO-1, and Shannon.
3.4.1 Weight change
Fourteen research studies reported the outcome indicators of WC, a total of 304 UC models, involving 11 probiotics. The network evidence diagram is shown in Figure 3. Compared with the DSS group, the weight loss of 11 probiotics decreased, among which L. rhamnosus (MD = 2.87, 95% CrI (1.02, 4.73)), L. bulgaricus (MD = 1.89, 95% CrI (0.88, 2.88)), L. coryniformis (MD = 2.68, 95% CrI (0.88, 4.48)), L. plantarum (MD = 1.03,95% CrI (0.41, 1.70)), and L. fermentum (MD = 1.67,95% CrI (0.34, 2.97)) had statistical significance. Meanwhile, L. rhamnosus (MD = −2.64,95% CrI (−4.94, −0.38)) (MD = −2.82,95% CrI (−5.18, −0.46)), L. bulgaricus (MD = −1.66, 95% CrI (−3.04, −0.28)) (MD = −1.84, 95% CrI (−3.60, −0.05)), and L. coryniformis (MD = −2.45, 95% CrI (−4.70, −0.23)) (MD = −2.63, 95% CrI (−4.95, −0.33)) were obviously superior to L. crispatus and AKK in weight reduction, and there was no statistical difference compared with other probiotics. The specific data are shown in Table 2. According to the SUCRA probability results (Figure 4), L. rhamnosus (91.05%) > L. coryniformis (88.66%) > L. bulgaricus (77%) > L. fermentum (9.80%) > L. plantarum (49.73%) > C. butyricum (49.91%) > L. brevis (43.14%) > S. boulardii (32.44%) > L. crispatus (21.99%) > AKK (17.57%) > DSS (12.36%), suggesting that L. rhamnosus was the best strain to reduce the weight loss of UC.
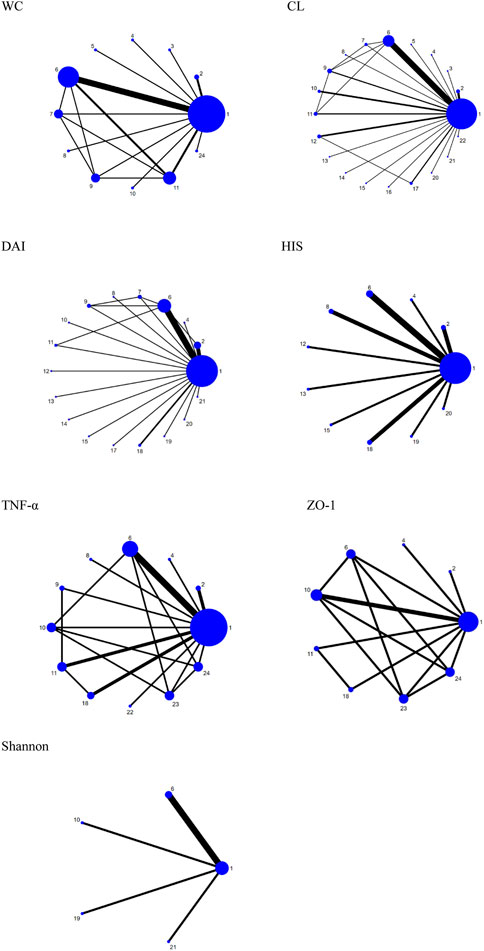
FIGURE 3. Network plots for different outcomes. 1. DSS; 2. B. bifidum; 3. S. boulardii; 4. C. butyricum; 5. L. brevis; 6. L. plantarum; 7. L. crispatus; 8. AKK; 9. L. fermentum; 10. L. rhamnosus; 11. L. bulgaricus; 12. L. paracasei; 13. L. reuteri; 14. LB-9; 15. L. casei; 16. L. kefiranofaciens M1; 17. E. cristatum; 18. L. acidophilus; 19. E. durans; 20. S. thermophilus; 21. T. halophilus; 22. E. faecium; 23. L. crustorum; and 24. L. coryniformis.
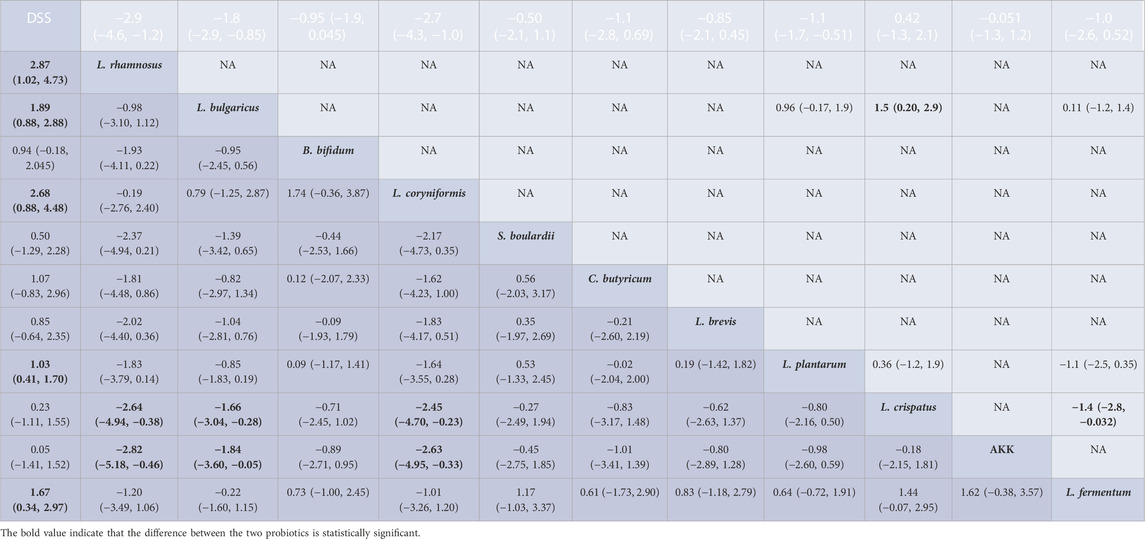
TABLE 2. League table for WC; results of the network meta-analysis are presented in the left lower half and results from pairwise meta-analysis in the upper right half. In the left lower and upper right half, mean differences lower than 0 favor the column-defining treatment, and bold font indicates significant results. NA: not available.
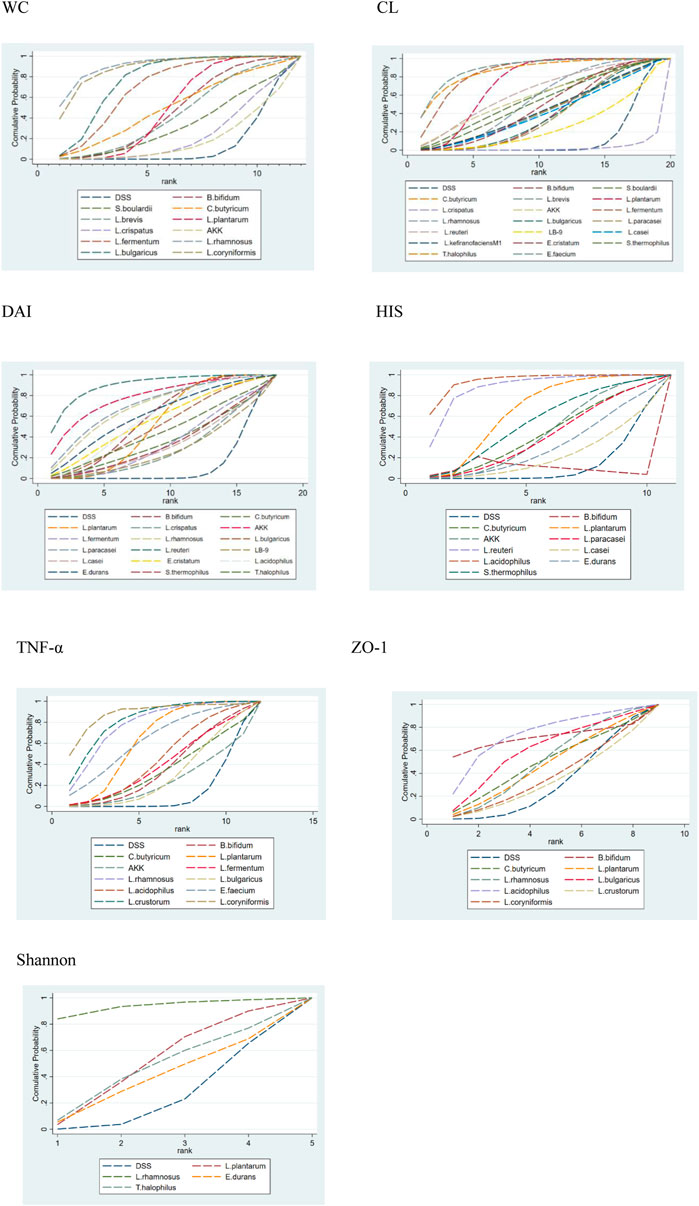
FIGURE 4. Surface under the cumulative ranking curve (SUCRA) plots for different outcomes. The vertical axis represents cumulative probabilities and the horizontal axis represents rank. 1. WC, 2. CL, 3. DAI, 4. HIS, 5. TNF-α, 6. ZO-1, and 7. Shannon.
3.4.2 Colon length
Thirty-four studies reported the CL, including 630 ulcerative colitis models, involving 20 kinds of probiotics. The network evidence diagram is shown in Figure 3. Compared with DSS, 19 kinds of probiotics could improve colon injury, among which are L. rhamnosus (MD = −1.22, 95% CrI (−2.21, −0.21)), T. halophilus (MD = −2.70, 95% CrI (−4.74, −0.67). −1.05)), L. plantaru (MD = −1.73, 95% CrI (−2.27, −1.20)), and L. fermentam.
(MD = −2.37, 95% CrI (−3.65, −1.11)) were statistically significant. L. bulgaricus (MD = 2.09, 95% CrI (0.13, 4.04)), L. paracasei (MD = 2.08, 95% CrI (0.10, 4.04)), L. reuteri (MD = 2.88, 95% CrI (0.44, 5.33)), E. cristatum (MD = 2.36, 95% CrI (0.29, 4.42)), B. bifidum (MD = 2.13, 95% CrI (0.21, 4.07)), S. thermophilus (MD = 2.50, 95% CrI (0.08, 4.91)), T. halophilus (MD = 4.03, 95% CrI (1.37, 6.70)), E. faecium (MD = 4.09, 95% CrI (1.68, 6.49)), S. boulardii (MD = 2.64, 95% CrI (0.18, 5.09)), L. plantarum (MD = 3.07, 95% CrI (1.37, 4.77)), and AKK (MD = −2.72, 95% CrI (−5.44, −0.01)), and L. fermentum (MD = −3.70, 95% CrI (−5.58,−1.84)) was obviously superior to L. crispatus and E. faecium is obviously superior to L. paracasei (MD = −2.02, 95% CrI (−3.99, −0.04)), LB-9 (MD = −2.50, 95% CrI (−4.89, −0.11)), and B. bifidum (MD = −1.96, 95% CrI (−3.89,−0.02)). In addition, L. fermentum was significantly better than L. bulgaricus (MD = −1.61, 95% CrI (−3.03, −0.22)), L. paracasei (MD = −1.63, 95% CrI (−3.25, −0.03)), B. bifidum (MD = −1.57, 95% CrI (−3.14,−0.01)), and L. crispatus (MD = -3.70, 95% CrI (−5.58, −1.84)). The specific data are shown in Supplementary Table S3. According to the SUCRA probability results (Figure 4), E. faecium (89.9%)>T. halophilus (87.16%)>L. fermentum (85.88%)>L. plantarum (73.45%)>L. reuteri (63.66%)>AKK (58.13%)>S. boulardii (56.68%)>L. rhamnosus (54.9%)>S. thermophilus (52.53%)>E. cristatum (48.27%)>L. kefiranofaciens M1 (44%)>C. butyricum (43.44%)>L. brevis (42.86%)>L. casei (41.16%)>B. bifidum (40.02%) > L. bulgaricus (38.64%) > L. paracasei (38.03%) > LB-9 (25.62%) > DSS (13.22%) > L. crispatus (48.27%), suggesting that E. faecium was the best strain to alleviate the colon injury of UC.
3.4.3 Disease activity index
Twenty-five studies reported DAI, a total of 472 UC models, involving 17 probiotics. The network evidence diagram is shown in Figure 3. The DAI of seven probiotics was better than that of DSS, among which L. reuteri (MD = 6.18, 95% CrI (2.08, 10.22)), L. acidophilus (MD = 2.97, 95% CrI (0.15, 5.83)), B. bifidum (MD = 2.87, 95% CrI (0.91, 4.95)), L. plantarum (MD = 2.53, 95% CrI (1.08, 3.95)), and AKK (MD = 4.96, 95% CrI (0.04, 9.87)) had statistical significance. Specific data are shown in Supplementary Table S4. According to the SUCRA probability results (Figure 4), L. reuteri (90.18%)>AKK(78.45%)>L. paracasei (71.39%)>L. rhamnosus (69.5%)> E. durans (61.46%)>L. acidophilus (58.73%)>B. bifidum (57.84%)>E. cristatum (55.96%) >L. plantarum (51.9%)>L. bulgaricus (49.89%)>T. halophilus (44.43%)>L. fermentum (40.67%)>C. butyricum (35.78%)> S. thermophilus (34.08%)>L. casei (31.96%) > L. crispatus (28.15%) > LB-9 (27.47%) > DSS (12.18%), suggesting that L. reuteri was the best strain to reduce the DAI index of UC.
3.4.4 Historical score
Fifteen studies reported HIS, a total of 246 UC models, involving 10 kinds of probiotics. The network evidence diagram is shown in Figure 3. The HIS of 10 kinds of probiotics was better than that of DSS, among which the HIS of L. reuteri (MD = 6.61, 95% CrI (2.00, 11.21)), L. acidophilus (MD = 7.56, 95% CrI (4.24, 10.79)), L. plantarum (MD = 3.58, 95% CrI (0.95, 6.33)), and L. acidophilus was significantly better than that of L. paracasei (MD = 5.75, 95% CrI (0.05, 11.29)). 12.73)), E. durans (MD = −6.43, 95% CrI (−11.99,−0.77)), and AKK (MD = −5.54, 95% CrI (−10.10,−0.86)). Supplementary Table S5 shows the specific data. According to the SUCRA probability results (Figure 4), L. acidophilus (94.42%)>L. reuteri (87.87%)>L. plantarum (65.1%)>B. bifidum (57.5%)>S. thermophilus (54.14%) > AKK(43.08%)>C. butyricum (42.56%)>L. paracasei (40.29%) > E. durans (31.04%) > L. casei (21.79%) > DSS (12.2%), suggesting that L. acidophilus was the best strain to reduce the HIS index of UC.
3.4.5 Tumor necrosis factor-α
Twelve studies reported serum TNF-α, including 279 UC models, involving 11 kinds of probiotics. Figure 3 shows the network evidence diagram. Compared with DSS, 11 kinds of probiotics could reduce the content of TNF-α in serum, among which L. rhamnosus (SMD = 5.92, 95% CrI (1.11, 10.73)), L. plantarum (SMD = 4.10, 95% CrI (1.47, 6.72)), L. crustorum (SMD = 6.42, 95% CrI (1.55, 11.28)), and L. coryniformis (SMD = 7.59, 95% CrI (2.58, 12.60)) had a statistically significant difference, L. bulgaricus (SMD = −6.38, 95% CrI (−12.45,−0.31)) and AKK (SMD = −7.12, 95% CrI (−14.22,−0.02)) were obviously superior to L. coryniformis, and the sspecific data are shown in Supplementary Table S6. According to the SUCRA probability results (Figure 4), L. coryniformis (90.4%)>L. crustorum (81.5%)>L. rhamnosus (77.8)>E. faecium (63.5%) > L. plantarum (63.1%) > L. acidophilus (45.4%) > L. fermentum (40.1%) > B. bifidum (36.3%) > C. butyricum (33.6%)>L. bulgaricus (29.8%) > AKK (24.9%)>DSS (13.4%), suggesting that L. coryniformis was the best strain to reduce the serum TNF-α content of UC.
3.4.6 Zonula occlusion Ns-1
Five studies reported the expression of colon tight junction protein ZO-1, including 109 UC models, involving eight kinds of probiotics. The network evidence diagram is shown in Figure 3. Compared with DSS, seven probiotics could increase the expression of tight junction protein ZO-1, but the difference was not statistically significant. Supplementary Table S7 shows the specific data. According to the SUCRA probability results (Figure 4), L. acidophilus (73.68%)>B. bifidum (70.33%)> L. bulgaricus (59.94%) > L. rhamnosus (49.69%) > C. butyricum (48.73%) > L. plantarum (46.95%) > L. coryniformis (36.93%) > L. crastorum (33.21%) > DSS (30.53%), suggesting that L. acidophilus was the best strain to increase the tight junction protein ZO-1 in UC.
3.4.7 Shannon
Six studies reported the Shannon index, including 96 ulcerative colitis models, four kinds of probiotics. The network evidence diagram was shown in Figure 3. Compared with DSS, 4 probiotics can increase the Shannon index of UC, but the difference is not statistically significant. The specific data are shown in Supplementary Table S8. According to the probability results of SUCRA (Figure 4), L. rhamnosus (93.09%) > L. plantarum (49.99%) > T. halophilus (45.7%) > E. durans (38.07%) > DSS (23.15%), suggesting that L. rhamnosus was the best flora to improve the Shannon index of UC.
3.5 Publication bias
The calibration-comparison funnel chart of five outcome indicators (WC, CL, DAI, HIS, and TNF-α) was tested for publication bias, and the results showed that there was asymmetry in scattered points, which indicated that there might be a certain degree of publication bias and small sample size effect in this study. The funnel charts of all the results are shown in Figure 5.
3.6 Consistency tests
The inconsistency of WC, CL, DAI, and HIS models was analyzed by the node split method. The results showed that there is no statistical inconsistency, which indicated that the results of the aforementioned outcome indicators are reliable. Supplementary Figure S2 shows the node split analysis diagram.
4 Discussion
4.1 Research significance
In 2002, probiotics were defined by the World Health Organization as “appropriate intake of living microorganisms that are beneficial to the health of the host” (Wang and Dai, 2020), which can be divided into original bacteria, symbiotic bacteria, and fungi according to the source and mode of action of strains (Zheng et al., 2011). After more than 20 years in vivo and in vitro, clinical and animal studies had proved the positive effect of probiotics in maintaining the stability of intestinal flora and regulating immunity. Since it is safer than other conventional drugs, it has been widely used in many diseases such as respiratory system, digestive system, and autoimmune diseases, especially inflammatory bowel disease in the gastrointestinal system (Bennet, 2016). At present, a large number of studies have proved that probiotic supplementation can reduce inflammation and induce intestinal environmental balance (Srutkova et al., 2015; Boruel et al., 2002). The most commonly used probiotics include Lactobacillus, Bifidobacterium, and other probiotics such as Akkermansia muciniphila, Streptococcus thermophilus, and Escherichia coli.
Ulcerative colitis is a non-specific intestinal inflammatory disease. Studies had shown that probiotics can improve intestinal symptoms, and the treatment of UC aims at maintaining, alleviating, and preventing recurrence (Xue and Huang, 2016). At present, probiotics play a part in UC mainly from the following aspects: 1) Promoting the balance of intestinal flora: The imbalance of intestinal flora is an important reason for the occurrence and development of UC, and probiotics can compete for nutrition and produce antibacterial compounds by occupying adhesion sites on mucosa, thus resisting exogenous pathogens. 2) Maintenance of the intestinal mucosal barrier: A complete intestinal barrier is the basis for maintaining intestinal homeostasis and the most important one is the mechanical barrier. Tight junction proteins are the key components of the mechanical barrier, including occlusal proteins, closing proteins, and junction adhesion molecules. Probiotics can protect the intestinal mucosal barrier by regulating tight junction proteins. 3) Regulating immune function: Inflammation is the basis of UC. Probiotics can regulate immune response through a variety of signal pathways, reduce the production of pro-inflammatory cytokines, promote the secretion of anti-inflammatory cytokines, and then, reduce inflammatory response. 4) Enhancing the antioxidant capacity: In the UC state, excessive reactive oxygen species (ROS) can accelerate the inflammatory reaction, leading to cell apoptosis or necrosis, etc. Probiotics can reduce the oxidative stress level of colonic mucosa and protect the colonic mucosal barrier (Wang and Dai, 2020). However, not all probiotics have a good curative effect on UC, and the biological effect of probiotics depends on the strain, dose, severity of colitis, and the immune state of the host (Liu et al., 2018), so it is of great significance to seek preclinical evidence for comparing the safety and curative effect of different strains and doses to select probiotics to treat UC in clinic.
4.2 Principal finding
In this study, WC, CL, DAI, and HIS are the main outcome indexes because they are the most important and commonly used pathological indexes to evaluate experimental UC in animals. TNF-α is a pro-inflammatory factor to evaluate UC, and ZO-1 is an important protein to evaluate mucosal barrier of UC, and Shannon is an important indicator to reflect the diversity of intestinal flora of ulcerative colitis, so this study regards them as secondary outcome indicators.
This study included 42 studies, including 839 UC models, 24 probiotics. Meta-analysis showed that L. rhamnosus had the best effect in relieving weight loss and increasing the Shannon index of UC model. E. faecium had the best effect in reducing colon injury; L. reuteri had the best effect in reducing DAI; L. acidophilus had the best effect in reducing HIS and increasing the expression of tight junction protein ZO-1; and L. coryniformis had the best effect in reducing the TNF-α in serum. However, there was no statistical difference between L. rhamnosus in increasing the Shannon index and L. acidophilus in increasing the expression of tight junction protein ZO-1 compared with the untreated group.
In this study, five outcome indicators were tested for publication bias, and the results showed that there might be some publication bias and small sample size effect in this study. The consistency test of the outcome indicators WC, CL, DAI, and HIS showed that the direct and indirect research of the four outcome indicators is consistent and reliable, but there were lack face-to-face studies in this study.
4.3 Preclinical animal models
At present, rodents (rats or mice) are often used in UC model-building (Martín et al., 2017), and the most commonly used induction methods are chemical drugs (Hu et al., 2022), such as dextran sulfate sodium (DSS) and 2,4,6-trinitrobenzene sulfonic acid (TNBS). In this study, the UC model is induced by DSS. Since the UC model induced by DSS has the characteristics of convenient. The animal colitis reaction can be successfully induced by drinking DSS solution freely, and the appropriate drug concentration and administration time can be selected according to the experimental requirements. The specific mechanism is that DSS can destroy animal colon epithelial cells, damage epithelial barrier function, and produce inflammatory substances in intestinal cavity to invade lamina propria and submucosa, and then inducing abnormal immune response in animals (Wirtz et al., 2007). Therefore, the DSS model has advantages in studying the effect of probiotics on UC (Martín et al., 2017). However, the results of network meta-analysis in this study may be limited to the animal model of ulcerative colitis, which is not suitable for use in clinic directly. The difference between the preclinical ulcerative colitis animal model and clinical ulcerative colitis makes our research results need to be treated with caution.
4.4 Strengths and limitations of the study
Previous studies and meta-analysis have proved that probiotics have positive effects in improving clinical symptoms, anti-inflammation, and enhancing antioxidant capacity of UC patients (Jia et al., 2018; Kaur et al., 2020; Xie et al., 2022). In this study, the network meta-analysis was used to make preclinical analysis of different strains, which can compare the effects of different strains on ulcerative colitis. Compared with clinical research, it can explore the histopathological effects of probiotics on UC animal models, and provide reference for clinical application of probiotics in the treatment of UC.
In addition, there are still some limitations in this study: 1) The overall quality of the included studies is medium, some studies do not describe the random sequence generation method in detail, and most studies do not mention blind method, distribution concealment, and registration of animal experiments. 2) Some data in this study were obtained indirectly through data extraction tools, which might lead to measurement deviation. 3) By drawing the funnel diagram, it was found that there was a certain degree of publication bias and small sample size effect in this study. 4) In order to improve the research quality, only the English literature was included in this study, which might lead to language bias. 5) The heterogeneity of this study was high, we tried doing the sensitivity analysis and the results were stable. We analyze the heterogeneity might come from animal strains, induction time, disease severity, and so on. 6) The results of this study might only be applicable to preclinical animal models of ulcerative colitis, and the differences between animals and humans make it necessary to be cautious when applying the results of this study in clinic. 7) This study lacks of face-to-face comparison between different probiotics.
5 Conclusion
In summary, the results of network meta-analysis showed that different probiotics have different effects on the ulcerative colitis model, and some probiotics have definite effects on improving the histopathological manifestations of UC and reducing inflammatory reaction. However, considering the limitations of this study, preclinical studies that need more large samples and high-quality and more reliable and rigorous experimental designs need to be conducted in the future.
Data availability statement
The original contributions presented in the study are included in the article/Supplementary Material; further inquiries can be directed to the corresponding authors.
Author contributions
WJ contributed to the study design, manuscript conception, and writing; HA and QH were responsible for data extraction; CL and XH conducted the data analyses and quality assessment; and ZJ and YZ checked and revised at any stage of this manuscript. All authors contributed to the article and approved the submitted version.
Funding
This study was supported by the Sichuan Provincial Administration of Traditional Chinese Medicine (Grant nos. 2021MS133 and 2021MS061).
Conflict of interest
The authors declare that the research was conducted in the absence of any commercial or financial relationships that could be construed as a potential conflict of interest.
Publisher’s note
All claims expressed in this article are solely those of the authors and do not necessarily represent those of their affiliated organizations, or those of the publisher, the editors, and the reviewers. Any product that may be evaluated in this article, or claim that may be made by its manufacturer, is not guaranteed or endorsed by the publisher.
Supplementary material
The Supplementary Material for this article can be found online at: https://www.frontiersin.org/articles/10.3389/fphar.2023.1187911/full#supplementary-material
References
Bennet, W. J. (2016). Quantitative risk-beneft analysis of probiotic use for iritable bowel syndrome and inflammatory bowel disease. Druo Saf. 39 (4), 295–305.
Bian, X., Wu, W., Yang, L., Lv, L., Wang, Q., Li, Y., et al. (2019). Administration of Akkermansia muciniphila ameliorates dextran sulfate sodium-induced ulcerative colitis in mice. Front. Microbiol. 10, 2259. doi:10.3389/fmicb.2019.02259
Boruel, N., Carol, M., Casellas, F., Antolín, M., de Lara, F., Espín, E., et al. (2002). Increased mucosal tumour necrosis factor alpha production in Crohn's disease can be downregulated ex vivo by probiotic bacteria. Gut 51 (5), 659–664. doi:10.1136/gut.51.5.659
Chae, J. M., Chang, M. H., Heo, W., Cho, H. T., Lee, D. H., Hwang, B. B., et al. (2019). LB-9, novel probiotic lactic acid bacteria, ameliorates dextran sodium sulfate-induced colitis in mice by inhibiting TNF-α-mediated apoptosis of intestinal epithelial cells. J. Med. Food 22, 271–276. doi:10.1089/jmf.2018.4236
Chae, J. M., Heo, W., Cho, H. T., Lee, D. H., Kim, J. H., Rhee, M. S., et al. (2018). Effects of orally-administered Bifidobacterium animalis subsp. lactis strain BB12 on dextran sodium sulfate-induced colitis in mice. J. Microbiol. Biotechnol. 28, 1800–1805. doi:10.4014/jmb.1805.05072
Charles, N. B., Michael, F., rabshuis, J. H. K., Henry, C., Eliakim, R., Suleiman, F., et al. (2010). World gastroenterology organization's practice guide on diagnosis and treatment of inflammatory bowel disease. Gastroenterology 15 (09), 548–558.
Chen, L. L., Zou, Y. Y., Lu, F. G., Li, F. J., and Lian, G. H. (2013). Efficacy profiles for different concentrations of Lactobacillus acidophilus in experimental colitis. World J. Gastroenterology 19, 5347–5356. doi:10.3748/wjg.v19.i32.5347
Chen, Y. P., Hsiao, P. J., Hong, W. S., Dai, T. Y., and Chen, M. J. (2012). Lactobacillus kefiranofaciens M1 isolated from milk kefir grains ameliorates experimental colitis in vitro and in vivo. J. Dairy Sci. 95, 63–74. doi:10.3168/jds.2011-4696
Chen, Y., Zhang, M., and Ren, F. (2019). A role of exopolysaccharide produced by Streptococcus thermophilus in the intestinal inflammation and mucosal barrier in caco-2 monolayer and dextran sulphate sodium-induced experimental murine colitis. Molecules 24, 513. doi:10.3390/molecules24030513
Chen, Z., Yi, L., Pan, Y., Long, X., Mu, J., Yi, R., et al. (2021). Lactobacillus fermentum ZS40 ameliorates inflammation in mice with ulcerative colitis induced by dextran sulfate sodium. Front. Pharmacol. 12, 700217. doi:10.3389/fphar.2021.700217
Choi, S. H., Lee, S. H., Kim, M. G., Lee, H. J., and Kim, G. B. (2019). Lactobacillus plantarum CAU1055 ameliorates inflammation in lipopolysaccharide-induced RAW264.7 cells and a dextran sulfate sodium-induced colitis animal model. J. Dairy Sci. 102, 6718–6725. doi:10.3168/jds.2018-16197
Cui, Y., Wei, H., Lu, F., Liu, X., Liu, D., Gu, L., et al. (2016). Different effects of three selected Lactobacillus strains in dextran sulfate sodium-induced colitis in BALB/c mice. PLoS One 11, 0148241. doi:10.1371/journal.pone.0148241
Din, A. U., Hassan, A., Zhu, Y., Zhang, K., Wang, Y., Li, T., et al. (2020). Inhibitory effect of Bifidobacterium bifidum ATCC 29521 on colitis and its mechanism. J. Nutr. Biochem. 79, 108353. doi:10.1016/j.jnutbio.2020.108353
Dong, Y., Liao, W., Tang, J., Fei, T., Gai, Z., and Han, M. (2022). Bifidobacterium BLa80 mitigates colitis by altering gut microbiota and alleviating inflammation. Amb. Express 12, 67. doi:10.1186/s13568-022-01411-z
Elian, S. D., Souza, E. L., Vieira, A. T., Teixeira, M. M., Arantes, R. M., Nicoli, J. R., et al. (2015). Bifidobacterium longum subsp. infantis BB-02 attenuates acute murine experimental model of inflammatory bowel disease. Exp. Model. Inflamm. bowel Dis. 6, 277–286. doi:10.3920/BM2014.0070
Franzosa, E. A., Sirota-Madi, A., Avila-Pacheco, J., Fornelos, N., Haiser, H. J., Reinker, S., et al. (2019). Gut microbiome structure and metabolic activity in inflammatory bowel disease. Nat. Microbiol. 4 (2), 293–305. doi:10.1038/s41564-018-0306-4
Gao, H., Li, Y., Sun, J., Xu, H., Wang, M., Zuo, X., et al. (2021). Saccharomyces boulardii ameliorates dextran sulfate sodium-induced ulcerative colitis in mice by regulating NF-κB and Nrf2 signaling pathways. Oxid. Med. Cell. Longev. 2021, 1622375. doi:10.1155/2021/1622375
He, W., Ni, W., and Zhao, J. (2020). Enterococcus faecium alleviates gut barrier injury by restoring the balance of the microbiota in C57BL/6 mice with dextran sulfate sodium-induced ulcerative colitis. J. Dig. Dis. 21, 41.
Hooijmans, C. R., Rovers, M. M., de Vries, R. B., Leenaars, M., Ritskes-Hoitinga, M., and Langendam, M. W. (2014). SYRCLE’s risk of bias tool for animal studies. BMC Med. Res. Method 14, 43. doi:10.1186/1471-2288-14-43
Hu, T., Fan, Y., Long, X., Pan, Y., Mu, J., Tan, F., et al. (2021). Protective effect of Lactobacillus plantarum YS3 on dextran sulfate sodium-induced colitis in C57BL/6J mice. J. Food Biochem. 45, e13632. doi:10.1111/jfbc.13632
Hu, T., Wang, H., Xiang, C., Mu, J., and Zhao, X. (2020). Preventive effect of Lactobacillus acidophilus XY27 on DSS-induced ulcerative colitis in mice. Drug Des. Devel Ther. 14, 5645–5657. doi:10.2147/DDDT.S284422
Hu, Y., Lan, T. X., Chen, X. X., Xiong, W., Tang, S. Q., Jia, B., et al. (2022). Research progress of animal model of ulcerative colitis. laboratory animal Comp. Med. 42 (03), 220–228.
Huang, J., Yang, Z., Li, Y., Chai, X., Liang, Y., Lin, B., et al. (2021). Lactobacillus paracasei R3 protects against dextran sulfate sodium (DSS)-induced colitis in mice via regulating Th17/Treg cell balance. J. Transl. Med. 19, 356. doi:10.1186/s12967-021-02943-x
Huang, Y. Y., Wu, Y. P., Jia, X. Z., Lin, J., Xiao, L. F., Liu, D. M., et al. (2022). Lactiplantibacillus plantarum DMDL 9010 alleviates dextran sodium sulfate (DSS)-induced colitis and behavioral disorders by facilitating microbiota-gut-brain axis balance. Food Funct. 13, 411–424. doi:10.1039/d1fo02938j
Islam, S. M. S., Ryu, H. M., and Sohn, S. (2022). Tetragenococcus halophilus alleviates intestinal inflammation in mice by altering gut microbiota and regulating dendritic cell activation via CD83. Cells 11, 1903. doi:10.3390/cells11121903
Jia, K., Tong, X., Wang, R., and Song, X. (2018). The clinical effects of probiotics for inflammatory bowel disease: A meta-analysis. Medicine 97 (51), e13792. doi:10.1097/MD.0000000000013792
Kanda, T., Nishida, A., Ohno, M., Imaeda, H., Shimada, T., Inatomi, O., et al. (2016). Enterococcus durans TN-3 induces regulatory T cells and suppresses the development of dextran sulfate sodium (DSS)-Induced experimental colitis. Plos One 11, 0159705. doi:10.1371/journal.pone.0159705
Kaur, L., Gordon, M., Baines, P. A., Iheozor-Ejiofor, Z., Sinopoulou, V., and Akobeng, A. K. (2020). Probiotics for induction of remission in ulcerative colitis. Cochrane Database Syst. Rev. 3, 005573. doi:10.1002/14651858.CD005573.pub3
Khan, I., Wei, J. S., Li, A. P., Liu, Z. R., Yang, P. R., Jing, Y. P., et al. (2022). Lactobacillus plantarum strains attenuated DSS-induced colitis in mice by modulating the gut microbiota and immune response. Int. Microbiol. 25, 587–603. doi:10.1007/s10123-022-00243-y
Kornbluth, A., and Sachar, D. B. (2010). Practice parameters committee of the American college of gastroenterology ulcerative colitis practice guidelines in adults: American college of gastroenterology, practice parameters committee. Am. J. Gastroenterol. 105, 501–523. doi:10.1038/ajg.2009.727
Lee, D. K., Lee, J. S., and Lee, S. (2015). Standard deviation and standard error of the mean. Korean J. Anesth. 68 (3), 220–223. doi:10.4097/kjae.2015.68.3.220
Lee, H. A., Bong, Y. J., Kim, H., Jeong, J. K., Kim, H. Y., Lee, K. W., et al. (2015). Effect of nanometric Lactobacillus plantarum in kimchi on dextran sulfate sodium-induced colitis in mice. J. Med. Food 18, 1073–1080. doi:10.1089/jmf.2015.3509
Lee, S. Y., Lee, B. H., Park, J. H., Park, M. S., Ji, G. E., and Sung, M. K. (2022). Bifidobacterium bifidum BGN4 paraprobiotic supplementation alleviates experimental colitis by maintaining gut barrier and suppressing nuclear factor kappa B activation signaling molecules. J. Med. Food 25, 146–157. doi:10.1089/jmf.2021.K.0150
Li, P., Chen, G., Zhang, J., Pei, C., Chen, Y., Gong, J., et al. (2022). Live Lactobacillus acidophilus alleviates ulcerative colitis via the SCFAs/mitophagy/NLRP3 inflammasome axis. Food Funct. 13, 2985–2997. doi:10.1039/d1fo03360c
Liu, Y., Alookaran, J., and Rhoads, J. (2018). Probiotics in autoimmune and inflammatory disorders. Nutrients 10 (10), 1537. doi:10.3390/nu10101537
Liu, Y. W., Ong, W. K., Su, Y. W., Hsu, C. C., Cheng, T. H., and Tsai, Y. C. (2016). Anti-inflammatory effects of Lactobacillus brevis K65 on RAW 264.7 cells and in mice with dextran sulphate sodium-induced ulcerative colitis. Benef. Microbes 7, 387–396. doi:10.3920/BM2015.0109
Liu, Y. W., Su, Y. W., Ong, W. K., Cheng, T. H., and Tsai, Y. C. (2011). Oral administration of Lactobacillus plantarum K68 ameliorates DSS-induced ulcerative colitis in BALB/c mice via the anti-inflammatory and immunomodulatory activities. Int. Immunopharmacol. 11, 2159–2166. doi:10.1016/j.intimp.2011.09.013
Lu, X., Jing, Y., Zhang, N., and Cao, Y. (2022). Eurotium cristatum, a probiotic fungus from fuzhuan brick tea, and its polysaccharides ameliorated DSS-induced ulcerative colitis in mice by modulating the gut microbiota. J. Agric. Food Chem. 70, 2957–2967. doi:10.1021/acs.jafc.1c08301
Ma, L., Shen, Q., Lyu, W., Lv, L., Wang, W., Yu, M., et al. (2022). Clostridium butyricum and its derived extracellular vesicles modulate gut homeostasis and ameliorate acute experimental colitis. Microbiol. Spectr. 10, 0136822. doi:10.1128/spectrum.01368-22
Martín, R., Chain, F., Miquel, S., Motta, J. P., Vergnolle, N., Sokol, H., et al. (2017). Using murine colitis models to analyze probiotics–host interactions. FEMS Microbiol. Rev. 41, S49–S70. doi:10.1093/femsre/fux035
Moher, D., Shamseer, L., Clarke, M., Ghersi, D., Liberati, A., Petticrew, M., et al. (2015). Preferred reporting items for systematic review and meta-analysis protocols (PRISMA-P) 2015 statement. Syst. Rev. 4 (1), 1–9. doi:10.1186/2046-4053-4-1
Pan, T., Guo, H. Y., Zhang, H., Liu, A. P., Wang, X. X., and Ren, F. Z. (2014). Oral administration of Lactobacillus paracasei alleviates clinical symptoms of colitis induced by dextran sulphate sodium salt in BALB/c mice. Benef. Microbes 5, 315–322. doi:10.3920/BM2013.0041
Qin, S., Huang, Z., Wang, Y., Pei, L., and Shen, Y. (2022). Probiotic potential of Lactobacillus isolated from horses and its therapeutic effect on DSS-induced colitis in mice. Microb. Pathog. 165, 105216. doi:10.1016/j.micpath.2021.105216
Qu, S., Fan, L., Qi, Y., Xu, C., Hu, Y., Chen, S., et al. (2021). Akkermansia muciniphila alleviates dextran sulfate sodium (DSS)-Induced acute colitis by NLRP3 activation. Microbiol. Spectr. 9, 0073021. doi:10.1128/Spectrum.00730-21
Shang, J., Yang, S., Tang, Z., Chen, Y., Duan, B., and Meng, X. (2022). Bifidobacterium bifidum H3-R2 and its molecular communication within the context of ulcerative colitis. J. Agric. Food Chem. 70, 11678–11688. doi:10.1021/acs.jafc.2c02909
Srutkova, D., Schwarzer, M., Hudcovic, T., Zakostelska, Z., Drab, V., Spanova, A., et al. (2015). Bifidobacterium longum CCM 7952 promotes epithelial barrier function and prevents acute DSS-induced colitis in strictly strain-specific manner. PLoS One 10 (7), 0134050. doi:10.1371/journal.pone.0134050
Sun, M. C., Zhang, F. C., Yin, X., Cheng, B. J., Zhao, C. H., Wang, Y. L., et al. (2018). Lactobacillus reuteri F-9-35 prevents DSS-induced colitis by inhibiting proinflammatory gene expression and restoring the gut microbiota in mice. J. Food Sci. 83, 2645–2652. doi:10.1111/1750-3841.14326
Sun, M., Liu, Y., Song, Y., Gao, Y., Zhao, F., Luo, Y., et al. (2020). The ameliorative effect of Lactobacillus plantarum-12 on DSS-induced murine colitis. Food Funct. 11, 5205–5222. doi:10.1039/d0fo00007h
Tong, L., Zhang, X., Hao, H., Liu, Q., Zhou, Z., Liang, X., et al. (2021). Lactobacillus rhamnosus GG derived extracellular vesicles modulate gut microbiota and attenuate inflammatory in DSS-induced colitis mice. Nutrients 13, 3319. doi:10.3390/nu13103319
Travis, S. P., Stange, E. F., Le´mann, M., Oresland, T., Bemelman, W. A., Chowers, Y., et al. (2008). European evidence-based Consensus on the management of ulcerative colitis: Current management. J. Crohns Colitis 2, 24–62. doi:10.1016/j.crohns.2007.11.002
Ungaro, R., Mehandru, S., Allen, P. B., Peyrin-Biroulet, L., and Colombel, J. F. (2017). Ulcerative colitis. Lancet 389 (10080), 1756–1770. doi:10.1016/S0140-6736(16)32126-2
Wan, C., Qian, W. W., Liu, W., Pi, X., Tang, M. T., Wang, X. L., et al. (2022). Exopolysaccharide from Lactobacillus rhamnosus ZFM231 alleviates DSS-induced colitis in mice by regulating gut microbiota. J. Sci. Food Agric. 102, 7087–7097. doi:10.1002/jsfa.12070
Wang, T., Sun, H., Chen, J., Luo, L., Gu, Y., Wang, X., et al. (2021). Anti-adhesion effects of Lactobacillus strains on caco-2 cells against Escherichia coli and their application in ameliorating the symptoms of dextran sulfate sodium-induced colitis in mice. Probiotics Antimicrob. Proteins 13, 1632–1643. doi:10.1007/s12602-021-09774-8
Wang, Y., Guo, Y., Chen, H., Wei, H., and Wan, C. (2018). Potential of Lactobacillus plantarum ZDY2013 and Bifidobacterium bifidum WBIN03 in relieving colitis by gut microbiota, immune, and anti-oxidative stress. Can. J. Microbiol. 64, 327–337. doi:10.1139/cjm-2017-0716
Wang, Y. J., and Dai, C. B. (2020). Application of probiotics in inflammatory bowel disease. Med. Inf. 33 (22), 42–44.
Wirtz, S., Neufert, C., Weigmann, B., and Neurath, M. F. (2007). Chemically induced mouse models of intestinal inflammation. Nat. Protoc. 2, 541–546. doi:10.1038/nprot.2007.41
Wu, Y., Li, A., Liu, H., Zhang, Z., Zhang, C., Ma, C., et al. (2022). Lactobacillus plantarum HNU082 alleviates dextran sulfate sodium-induced ulcerative colitis in mice through regulating gut microbiome. Food Funct. 13, 10171–10185. doi:10.1039/d2fo02303b
Xie, F., Li, S., Fan, Y., Li, W., Lv, Q., Sun, X., et al. (2022). Efficacy and safety of Bifidobacterium quadruple viable bacteria combined with mesalamine against UC management: A systematic review and meta-analysis. Oxid. Med. Cell. Longev. 4, 8272371. doi:10.1155/2022/8272371
Xue, A. J., and Huang, Y. (2016). Therapeutic effect of probiotics in inflammatory bowel disease. Microbiol. Infect. 11 (05), 312–316.
Yeo, S., Park, H., Seo, E., Kim, J., Kim, B. K., Choi, I. S., et al. (2020). Anti-inflammatory and gut microbiota modulatory effect ofLactobacillus rhamnosusStrain LDTM 7511 in a dextran sulfate sodium-induced colitis murine model. Microorganisms 8, 845. doi:10.3390/microorganisms8060845
Yu, P., Ke, C., Guo, J., Zhang, X., and Li, B. (2020). Lactobacillus plantarum L15 alleviates colitis by inhibiting LPS-mediated NF-κB activation and ameliorates DSS-induced gut microbiota dysbiosis. Front. Immunol. 11, 575173. doi:10.3389/fimmu.2020.575173
Zakostelska, Z., Kverka, M., Klimesova, K., Rossmann, P., Mrazek, J., Kopecny, J., et al. (2011). Lysate of probiotic Lactobacillus casei DN-114 001 ameliorates colitis by strengthening the gut barrier function and changing the gut microenvironment. Gut Microenviron. 6, 27961. doi:10.1371/journal.pone.0027961
Zhang, N. N., Zhang, H., and Jiang, L. Q. (2020). A novel method of sacral nerve stimulation for colonic inflammation[J]. Neurogastroenterol. Motil. 32 (6), 138.
Zhao, Y., Zeng, Y. D., Wang, H., Zhou, M., and Sun, N. (2020). Probiotics and MicroRNA: Their roles in the host-microbe interactions. Front. Microbio 11, 604462. doi:10.3389/fmicb.2020.604462
Keywords: probiotics, ulcerative colitis, preclinical evidence, systematic review, network meta-analysis
Citation: Jin W, Ai H, Huang Q, Li C, He X, Jin Z and Zuo Y (2023) Preclinical evidence of probiotics in ulcerative colitis: a systematic review and network meta-analysis. Front. Pharmacol. 14:1187911. doi: 10.3389/fphar.2023.1187911
Received: 16 March 2023; Accepted: 30 May 2023;
Published: 09 June 2023.
Edited by:
Irfan A. Rather, Yeungnam University, Republic of KoreaReviewed by:
Hilal Parray, University of Texas Southwestern Medical Center, United StatesSuriya Rehman, Imam Abdulrahman Bin Faisal University, Saudi Arabia
Copyright © 2023 Jin, Ai, Huang, Li, He, Jin and Zuo. This is an open-access article distributed under the terms of the Creative Commons Attribution License (CC BY). The use, distribution or reproduction in other forums is permitted, provided the original author(s) and the copyright owner(s) are credited and that the original publication in this journal is cited, in accordance with accepted academic practice. No use, distribution or reproduction is permitted which does not comply with these terms.
*Correspondence: Zhao Jin, dr.jinzhao@cdutcm.edu.cn; Yuling Zuo, zuozuoe@126.com