- 1Chang Gung Memorial Hospital, Taoyuan, Taiwan
- 2Department of Dermatology, Drug Hypersensitivity Clinical and Research Center, Chang Gung Memorial Hospital, Taipei and Keelung, Taiwan
- 3Cancer Vaccine and Immune Cell Therapy Core Laboratory, Department of Medical Research, Chang Gung Memorial Hospital, Taoyuan, Taiwan
- 4Chang Gung Immunology Consortium, Chang Gung Memorial Hospital and Chang Gung University, Taoyuan, Taiwan
- 5Department of Dermatology, Xiamen Chang Gung Hospital, Xiamen, China
- 6Department of Physiology and Pharmacology, College of Medicine, Chang Gung University, Taoyuan, Taiwan
- 7Graduate Institute of Biomedical Sciences, College of Medicine, Chang Gung University, Taoyuan, Taiwan
- 8Division of Hematology and Oncology, Department of Internal Medicine, New Taipei Municipal TuCheng Hospital (Built and Operated by Chang Gung Medical Foundation), New Taipei City, Taiwan
- 9College of Medicine, Chang Gung University, Taoyuan, Taiwan
- 10Whole-Genome Research Core Laboratory of Human Diseases, Chang Gung Memorial Hospital, Keelung, Taiwan
- 11Immune-Oncology Center of Excellence, Chang Gung Memorial Hospital, Linkou, Taiwan
- 12Graduate Institute of Clinical Medical Sciences, College of Medicine, Chang Gung University, Taoyuan, Taiwan
- 13Institute of Pharmacology, School of Medicine, National Yang Ming Chiao Tung University, Taipei, Taiwan
- 14Department of Dermatology, Beijing Tsinghua Chang Gung Hospital, School of Clinical Medicine, Tsinghua University, Beijing, China
- 15Department of Dermatology, Ruijin Hospital, School of Medicine, Shanghai Jiao Tong University, Shanghai, China
- 16Genomic Medicine Core Laboratory, Chang Gung Memorial Hospital, Linkou, Taiwan
Drug-induced delayed hypersensitivity reactions (DHRs) is still a clinical and healthcare burden in every country. Increasing reports of DHRs have caught our attention to explore the genetic relationship, especially life-threatening severe cutaneous adverse drug reactions (SCARs), including acute generalized exanthematous pustulosis (AGEP), drug reactions with eosinophilia and systemic symptoms (DRESS), Stevens–Johnson syndrome (SJS), and toxic epidermal necrolysis (TEN). In recent years, many studies have investigated the immune mechanism and genetic markers of DHRs. Besides, several studies have stated the associations between antibiotics-as well as anti-osteoporotic drugs (AOD)-induced SCARs and specific human leukocyte antigens (HLA) alleles. Strong associations between drugs and HLA alleles such as co-trimoxazole-induced DRESS and HLA-B*13:01 (Odds ratio (OR) = 45), dapsone-DRESS and HLA-B*13:01 (OR = 122.1), vancomycin-DRESS and HLA-A*32:01 (OR = 403), clindamycin-DHRs and HLA-B*15:27 (OR = 55.6), and strontium ranelate (SR)-SJS/TEN and HLA-A*33:03 (OR = 25.97) are listed. We summarized the immune mechanism of SCARs, update the latest knowledge of pharmacogenomics of antibiotics- and AOD-induced SCARs, and indicate the potential clinical use of these genetic markers for SCARs prevention in this mini review article.
1 Introduction
Adverse drug reactions (ADRs) are one of the general causes of death worldwide (Shoshi et al., 2015). In America, ADRs represented the fourth leading cause of death (Insani et al., 2021). 10%–15% ADRs contribute to type B reactions which are bizarre and unexpected reaction (Wilkerson and Drug Hypersensitivity Reactions, 2022). Type B ADRs, predominantly T cell-mediated drug-induced delayed hypersensitivity reactions (DHRs), presents variable severity and clinical diagnosis, from mild skin injury such as maculopapular exanthema (MPE) to life-threatening severe cutaneous adverse drug reactions (SCARs) including acute generalized exanthematous pustulosis (AGEP), drug reactions with eosinophilia and systemic symptoms (DRESS), Stevens–Johnson syndrome (SJS), and toxic epidermal necrolysis (TEN) (Copaescu et al., 2020). SCARs are rare, but they have high mortality rates (AGEP: <5%, DRESS: 5%–10%, SJS/TEN: 10%–40%) (Bjornsson and Bjornsson, 2017; Oh et al., 2021; Huang et al., 2022). In global, the incidence of SCARs is reported to be 0.4–1.2 per million per years (Verma et al., 2013). However, the incidence rates of SCARs from European and the United States show a divergence from Asian population (Tempark et al., 2022). A Germany study revealed that the incidence of SCARs was 1.53–1.89 per million people per year (Mockenhaupt, 2012), while a Philippines study reported a prevalence of 6.25 per 10,000 people from 2011-2015 (Guzman and Paliza, 2018). Moreover, the incidence of SJS and TEN in the European population is estimated to be 1–6 and 0.4–1.2 per million people per year respectively, while the incidence of them in the Korean was 3.96–5.03 and 0.94–1.45 per million people per year respectively. SCARs can be affected by racial, genetic and drug category difference, so results from various countries should be evaluated carefully and separately (Yang et al., 2016; Duong et al., 2017; Kang et al., 2021).
AGEP, DRESS, and SJS/TEN are three important phenotypes of SCARs that we will review in this article (Yang et al., 2021). Over 90% cases of AGEP are related to drugs, especially antibiotics such as aminopenicillins (Gammoudi et al., 2018), cephalosporins (Torres-Navarro et al., 2020), sulfonamides (Spadaro et al., 2021), vancomycin (Pettit et al., 2020), pristinamycin (de Sousa et al., 2018) and quinolones (Feldmeyer et al., 2016; Martinez-De la Torre et al., 2021). Although SJS/TEN are mainly associated with anti-epileptic drugs, some kind of antibiotics including penicillins, sulfonamides, and macrolides contribute to SJS/TEN (Kloypan et al., 2021; Pejčić, 2021). Various studies of DRESS induced by antibiotics are disclosed including sulfonamides (Asyraf et al., 2022), amoxicillin (Abdin et al., 2019), minocycline (Ganeshanandan and Lucas, 2021) and vancomycin (Clark et al., 2020). Apart from antibiotics-related DHRs, strontium ranelate (SR), one of anti-osteoporotic drugs (AODs), has been reported as causative agent of SCARs (Chen et al., 2021a). Osteoporosis is represented as bone fragility by a loss of bone material and deteriorating bone-micro-architecture (Wung et al., 2021). Several AODs can be used to treat osteoporosis including bisphosphonates, selective estrogen receptor modulators, senosumab, romosozumab, SR and calcitonin (Chiodini et al., 2020). The prevalence of bisphosphonates-induced cutaneous ADRs (CADRs) is relatively low, while the incidence of SR-induced SCARS is at moderate risk (Chen et al., 2021a). In light of the severity of SCARs, it is crucial to recognize the integration of T cell and pathogenesis of antibiotics- and SR-related DHRs (Mustafa et al., 2018).
2 Mechanism of SCAR
The pathogenesis of SCARs is strongly associated with specific human leukocyte antigens (HLA), T cell receptor (TCR), drug or its metabolites and further T cell-mediated immune response (Jantararoungtong et al., 2021; Hung et al., 2022). In human genome, HLA system is the most polymorphic genetic region, resulting in presenting a variety of peptides (Negrini and Becquemont, 2017). In addition, regional and ethnic difference also express HLA alleles variation (Barbarino et al., 2015). High polymorphic and heterogenetic properties of HLA molecules enable immune system not only with an advantage to defy diverse microorganisms and antigens the host encounters but also with a disadvantage to interact with various drugs and its metabolites (Crux and Elahi, 2017). Currently, four hypothetic models for the mechanism responsible for relationship between HLA molecule-dependent manner and T cell-mediated SCARs have been proposed: altered peptide repertoire model, hapten model, pro-hapten model and pharmacological-interaction model (Miyagawa and Asada, 2021a). It is unique that these models are non-mutually exclusive, which means a specific mechanism may be prevalent for a certain drug but not for another (Negrini and Becquemont, 2017). The association between HLA alleles and SCARs in different kind of medications has increasingly been reported in recent 2 decades (Wang et al., 2022a). Recent studies show that different kinds of drugs might display single or overlapped SCARs and molecular mechanism (Villani et al., 2017). In this paragraph, we summarize the mechanism of different kinds of SCARs.
2.1 Immune mechanism of AGEP
AGEP has been characterized as T cell-mediated neutrophilic inflammatory reaction (Wang et al., 2022b). After exposure to drugs, antigen presenting cells (APCs) presents the antigen with HLA molecule to cause activation of cluster of differentiation (CD) 4 and CD 8 T cells, referred to as drug-specific T cells (Szatkowski and Schwartz, 2015). During the development of AGEP, drug-specific T cells as well as cytotoxic T lymphocyte (CTL) released cytotoxic proteins including granulysin, granzyme B, and perforin play an important role (Dotiwala et al., 2016; Feldmeyer et al., 2016). Granzyme B can induce keratinocytes’ apoptosis, leading to subcorneal vesicles formation (Verneuil et al., 2011; Feldmeyer et al., 2016). There are many mediators and cytokines involved in recruitment of neutrophils (Svoboda et al., 2022). T helper 1 cells (Th1 cells) can produce predominant cytokines including granulocyte-macrophage colony-stimulating factor (GM-CSF), tumor necrosis factor-α (TNF-α), interferon-γ (IFN-γ) to enhance neutrophil recruitment (Piipponen et al., 2020; Wang et al., 2022b). Additionally, Th17 cells can directly recruit neutrophils via secretion of IL-17 and IL-22 (Pelletier et al., 2010; Klaewsongkram et al., 2021). CXC motif chemokine ligand 8 (CXCL8), also known as interleukin-8 (IL-8), is a potent neutrophil chemotactic chemokine and is responsible for pustule formation by neutrophil aggregation (Metzemaekers et al., 2020). Moreover, IL-36 receptor antagonist (IL-36 RA) deficiency in some AGEP patients resulted in reinforcing the expression of TNF-α, CXCL8, IL-1, IL-6, IL-17, and IL-23 (Feldmeyer et al., 2016; Liu et al., 2020).
2.2 Immune mechanism of SJS/TEN
SJS and TEN are rare but life-threating dermatologic diseases and represented as T cell-mediated keratinocyte death (Arora et al., 2021). TEN is always triggered by drugs, while SJS almost triggered by drug and small part of infection (Nowsheen et al., 2021). Both are characterized by the acute onset of blister formation at the epidermal layer, and hemorrhagic and erosive lesions at the mucous layer after drug exposure (Miyagawa and Asada, 2021b). In the early stage of SJS/TEN, blister fluid over epidermis was mainly infiltrated with CTLs, Natural killer (NK) cells and NK/T cells (Neerukonda and Stagner, 2021). Initially, CTLs cause keratinocyte apoptosis and cell-cell contact-dependent epidermal damage through perforin and granzyme B pathway (Tohyama and Hashimoto, 2012). Secondly, CTLs and NK cells secrete Fas ligand (FasL) to bind Fas on keratinocyte surface and activate Fas-FasL pathway, leading to keratinocyte apoptosis (Hasegawa and Abe, 2020). Then, granulysin, secreted by CTLs and NK cells, is a pro-apoptotic protein which demonstrates widespread cytotoxicity indirectly (Su et al., 2017; Sadek et al., 2021). In addition, IL-15 secreted by keratinocyte and CTLs themselves was thought to enhance the expression of granulysin-mediated apoptosis (Stern and Divito, 2017; Neerukonda and Stagner, 2021). In the late stage, granulysin could stimulate C-C motif chemokine ligand 20 (CCL20) expression in monocytes, leading to monocyte infiltration (Tewary et al., 2010). Monocyte could not only enhance consistent CTLs’ cytotoxicity but also activate TNF-receptor 1 (TNF-R1) mediated apoptosis pathway by TNF-α secretion, resulted in further epidermal destruction (Tohyama et al., 2012; Su et al., 2017; Kuijper et al., 2020). Besides, Olsson-Brown et al. (2022) reported a mechanism of TNF-α-induced matrix metalloproteinase 9 (MMP9) expression of keratinocytes in SJS/TEN. Recently, monocytes might play an important role in keratinocyte necroptosis, another keratinocyte’s death mechanism (Saito, 2014). Necroptotic cells cause inflammation by releasing lots of pre-inflammatory cytokine, while apoptotic cells cause cell death without inflammation (Hasegawa and Abe, 2020). Multiple and detailed mechanism involved in SJS/TEN will be gradually investigated in the future.
2.3 Immune mechanism of DRESS
DRESS is a rare but severe cutaneous and systemic drug-delayed hypersensitivity reaction, mediated by T cell activation (Schunkert and Divito, 2021). Drugs, drug’s metabolites and/or coincidental viral infection such as human herpesvirus (HHV)-6 and 7, cytomegalovirus (CMV) and Epstein-Barr virus (EBV)-induced T cell activation seem to play an important role in the pathogenesis of DRESS (Chen et al., 2018a). Th2 cells and CTLs induced by DRESS activate hypersensitivity response leading to skin damage and cause organ damage, respectively (Sharifzadeh et al., 2021). Th2 cells secrete IL-4, IL-5, and IL-13 to recruit macrophages, eosinophils and mast cells, resulted in inflammatory reaction (Kang et al., 2020). IL-5 is contributed to eosinophilic differentiation and expansion at the inflammatory skin site. C-C motif chemokine 8 (CCR8) + Th2 cells belong to IL-5-enriched subgroup associated with eosinophilic inflammation (Endo et al., 2014). Besides, innate lymphoid cells (ILC) can also secrete IL-5 to increase eosinophil recruitment in the damage site such as skin, target organs and even peripheral blood (Cardones, 2020). Thymus and activation-regulated chemokine (TARC/CCL17), produced by keratinocytes, not only can recruit Th2 cells into inflammatory site, but also may be associated with disease severity (Vestergaard et al., 2000; Stirton et al., 2022). Besides, pro-inflammatory cytokines including TNF-α, IFN-γ, IL-2, IL-6, IL-15 and granulysin were reported to be associated with DRESS (Weinborn et al., 2016; Wang et al., 2022b). On the other hand, several studies revealed that DRESS is associated with regulatory T (Treg) cells and Th17 cells activation (Pichler and Brüggen, 2022). Treg cells are expanded by classical monocytes secreted IL-10 in the acute stage of DRESS, while T17 cells are proliferated by pathological monocytes in the resolution stage (Shiohara and Mizukawa, 2019). T cell shift from Treg cells to Th17 cells in the subacute phase is probably derived from IL-6 secretion from pathological monocytes (Ushigome et al., 2018).
3 HLA susceptibility to drug-induced SCAR
Several antibiotics- and strontium ranelate (SR)-induced SCARs have been proposed in recent 2 decades (Blumenthal et al., 2019), and we have illustrated in Figure 1 (Administration, 2023). We summarize the relationships between well-known antibiotics and HLA-related ADRs listed in Table 1.
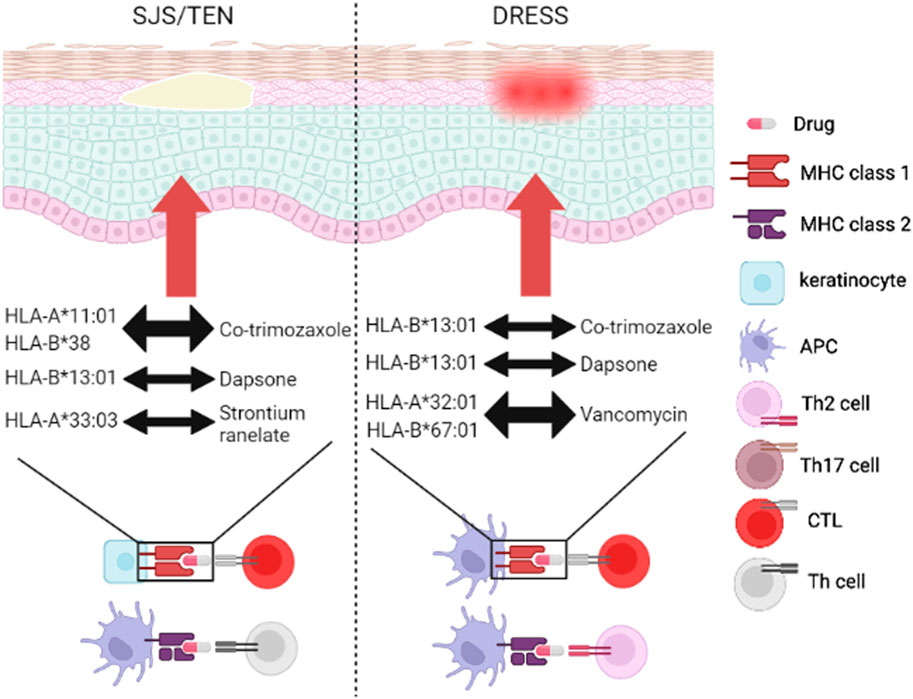
FIGURE 1. Genetic association of SCARs between HLA and drugs. The drugs (antibiotics or AODs) trigger DHRs through MHC/drug/TCR complex. SJS/TEN mainly 694 started immune mechanism by HLA/drug/CTLs and HLA/drug/Th cells, while DRESS mainly 695 started immune mechanism by HLA/drug/CTLs and HLA/drug/Th2 cells. Co-trimozaxole can induce 696 SJS/TEN and DRESS through binding to HLA-A*11:01/HLA-B*38 and HLA-B*13:01, 697 respectively. Dapsone can induce SJS/TEN and DRESS through binding to HLA-B*1301. 698 Vancomycin can induce DRESS by binding to HLA-A*32:01 and HLA-B*67:01. SR can activate 699 SJS/TEN through binding to HLA-A*33:03. Abbreviations: AOD, anti-osteoporotic drug; APC, antigen-presenting cell; CTL, cytotoxic T 701 lymphocyte; DHR, delayed hypersensitivity reaction; DRESS, drug reaction with eosinophilia and 702 systemic symptoms; HLA, human leukocyte antigen; MHC, major histocompatibility complex; SJS, 703 Stevens-Johnson syndrome; TCR, T cell receptor; TEN, toxic epidermal necrolysis; Th cell, T helper 704 cell.
3.1 Co-trimoxazole (Trimethoprim-sulfamethoxazole)
Co-trimoxazole is an antibiotics indicated for urinary tract infection and Pneumocystis jiroveci pneumonia (Haseeb et al., 2022; Jent et al., 2022). The prevalence of skin adverse reaction was 1%–4% among general population treated with co-trimoxazole (Wolkenstein et al., 1995; Masters et al., 2003). Lonjou et al. (2008) found association between HLA-B*38 and co-trimoxazole-induced SJS/TEN in European population, although ORs and statistical power showed borderline efficacy. On the contrary, a whole genome sequencing study revealed strong association between HLA-B*38:02 and co-trimoxazole-induced SJS/TEN in Chinese and Thai population. In addition, Wang et al. (2021) found association between HLA-B*15:02 and co-trimoxazole-induced SJS/TEN in Taiwanese and Thai population (Wang et al., 2021). In Thai population, HLA-C*06:02 and HLA-C*08:01 were also associated with co-trimoxazole-induced SJS/TEN (Kongpan et al., 2015). To our best knowledge, co-trimoxazole-induced DRESS has been reported to be related to HLA-B*13:01 among Chinese, Thai and Malaysian population and HLA-A*11:01 among Japanese population (Nakamura et al., 2020; Sukasem et al., 2020; Wang et al., 2021; Nakkam et al., 2022). Pratoomwun et al. (2021) found co-trimoxazole directly binding to HLA-B*13:01 induced drug-specific T cell response and further immune mechanism including IL-13, IFN-γ, granzyme B, and IL-22 secretion in Thai population.
3.2 Penicillins
Penicillin and its derivatives are antimicrobial agents frequently used to treat a variety of bacterial infection in the world (Chang et al., 2020). Zhou et al. (2016) reported that the prevalence of penicillin allergy was 12.8%. Penicillins may cause drug hypersensitivity-related skin lesions, varying from skin rash to SCARs (Lin et al., 2014). According to Krebs et al. (2020) genome-wide associated study (GWAS), HLA-B*55:01 is a genetic biomarker for penicillin allergy in European population. Another GWAS involving 5 countries (Australia, France, Italy, Spain, and United Kingdom) concluded that HLA-DRB*10:01 is associated with immediate penicillin hypersensitivity, but not delayed penicillin hypersensitivity (Nicoletti et al., 2021). Romano et al. (2022) conducted next-generation sequencing (NGS) and found that HLA-DRB3*02:02 is associated with delayed penicillin hypersensitivity in Italian population. A case-control study unveiled that HLA-C*04:06, HLA-C*08:01, and HLA-DRB1*04:06 are associated with β-lactam delayed reaction in Thai children (Singvijarn et al., 2021).
3.3 Piperacillin-Tazobactam
Piperacillin is an extended-spectrum penicillin usually found in combination with tazobactum, a β-lactamase inhibitor (Drawz and Bonomo, 2010). Piperacillin-tazobactam could cover most of the gram-positive and gram-negative bacteria, including Pseudomonas aeruginosa (Wong et al., 2021). To our best understanding, only one observation study revealed that HLA-B*62 might be associated with piperacillin-tazobactam induced DRESS in European population (Rutkowski et al., 2017). A larger sample size may be needed to validate the above association.
3.4 Dapsone
Dapsone, a sulfone drug with anti-microbial and anti-inflammatory effects, has been used for leprosy and dermatitis herpetiformis (Reunala et al., 2021; Li et al., 2022). The prevalence of dapsone-induced SCARs was 0.5%–3.6% after 4–6 weeks treatment (Rao and Lakshmi, 2001). According to Zhang et al. (2013) GWAS study, HLA-B*13:01 is associated with dapsone-induced hypersensitivity in Chinese population. The association between HLA*B13:01 and dapsone-induced DRESS was reported in Taiwan and Thai population, respectively (Wang et al., 2013; Chen et al., 2018b; Satapornpong et al., 2021). Krismawati et al. validated HLA-B*13:01 as biomarker of dapsone-induced hypersensitivity in leprosy patients among Indonesian population (Krismawati et al., 2020). Two meta-analysis demonstrated the association between HLA-B*13:01 and dapsone-induced SCARs in Chinese and Southeastern Asian population (Tangamornsuksan and Lohitnavy, 2018; Park et al., 2020). Most studies have revealed the relationship between HLA-B*13:01 and dapsone-induced DRESS (Jung et al., 2018). Satapornpong et al. found that HLA-C*03:04 is associated with dapsone-induced SCARs, although dapsone-induced DRESS and SJS/TEN showed no significance owing to weak statistical power (Satapornpong et al., 2021). In addition, Lee et al. (2022) analyzed the clinical data warehouse from Korea and found there is no association between HLA-B*13:01 and dapsone-induced SCARs in Korean population. Zhao et al. (2021) investigated that dapsone and its metabolite nitroso-dapsone are selectively interacted with HLA-B*13:01 to activate CTLs related cytotoxicity. Recently, Jiang et al. (2022) demonstrated that HLA-B*13:01-dapsone-TCR immune molecular mechanism is formed according to pharmacological-interaction model.
3.5 Vancomycin
Vancomycin, a glycosylated peptide antibiotic, has been used mainly for resistant gram-positive bacteria-related infection (Dinu et al., 2020). According to a recent large electronic healthcare record database, the prevalence of vancomycin-induced DRESS was 39% (Pirmohamed, 2019; Wolfson et al., 2019). Furthermore, the literature on vancomycin-induced SJS/TEN is rare (Minhas et al., 2016; De Luca et al., 2020). Young et al. (2014) reported that there is no association between HLA alleles and vancomycin-induced DRESS, probably due to only three patients. HLA-A*32:01 is associated with vancomycin-induced DRESS in European population (Konvinse et al., 2019). Bellon et al. (2022) also revealed the association between HLA-A*32:01 and vancomycin-induced DRESS in Spanish population. Recently, we reported the association between HLA-A*32:01, HLA-B*07:05, HLA-B:40:06, HLA-B*67:01 and vancomycin-induced DRESS in Taiwanese population (Wang et al., 2022c). However, Lee et al. (2022) reported that there is no association between HLA-A*32:01 and vancomycin-induced SCARs in Korean population according to clinical data from Seoul National University Hospital. Nakkam et al. (2021) elucidated the possibility of cross-reactivity between vancomycin, teicoplanin, and dalbavancin in HLA*A-32:01 patients with previous vancomycin-induced DRESS. Ogese et al. (2021) reported that the direct interaction of vancomycin-induced DRESS between vancomycin and HLA*A-32:01 and further high expression of CXCR3, CCR4, IL-13, and IFN-γ.
3.6 Clindamycin
Clindamycin, a macrolide antibiotic, used for several bacterial infection including atypical pneumonia, middle ear infection and endocarditis (Dashti et al., 2022). Yang et al. (2017) reported that there is an association between HLA-B*51:01 as well as HLA-B*15:27 and clindamycin-related CADRs in Chinese population. To our best knowledge, no studies have reported the association between HLA alleles and clindamycin-induced SCARs.
3.7 Strontium ranelate (SR)
SR is used for treatment of severe osteoporosis (Yang et al., 2014). Although there is a moderate risk for SR-induced DRESS in French population (Agier et al., 2016), no further studies have reported the association between HLA alleles and SR-induced DRESS (Adwan, 2017). Lee et al. (2016) reviewed several cases in Singapore and found the association between HLA-A*33:03 as well as HLA-B*58:01 and SR-induced SJS/TEN in Chinese population. Recently, Chen et al. (2021b) demonstrated that there is an association between HLA-A*33:03 and SR-induced SJS in Taiwanese population.
4 Application of HLA testing in clinical practice
Diagnosis of drug delayed hypersensitivity reaction is often tardy leading to multiple complications and even death (Kulkarni et al., 2022). Different population and ethnicity possess varied genetic HLA molecules, accountable for different degree of drug delayed hypersensitivity reactions (Jung et al., 2018). Besides, the frequency of HLA alleles and cost of prevention and management of ADR varied from one country to another, as well (Kloypan et al., 2021).
According to the Allele Frequency Net Database (AFND), the frequency of HLA-B*13:01 is 8%–15% in Taiwan and China. Co-trimoxazole-induced DRESS can be avoided after HLA-B*13:01 testing in Asian population. Although the frequency of HLA-A*11:01 allele is lower in Japanese population compared to Chinese and Thai population (Gonzalez-Galarza et al., 2018), HLA-A*11:01 is a high risk factor involved in co-trimoxazole-induced SJS/TEN in Japanese population (Nakamura et al., 2020). Even though lots of co-trimoxazole-induced DRESS have been reported, there is still no specific HLA alleles in European population. Larger scales of studies are required to identify the relevant HLA alleles in European population (Wang et al., 2021). Another example is dapsone and HLA-B*13:01. The frequency of HLA-B*13:01 is rare in European and African population, but occurs with frequency of 2%–20% in Chinese, 7% in Thai population and 1%–12% in Indian population (Puangpetch et al., 2014; Tempark et al., 2017). Moreover, HLA-B*13:01 testing is recommended for Chinese patients with leprosy before initiating dapsone therapy (Liu et al., 2019). Owing to the relatively high frequency of HLA-A*32:01 in European population, genetic examination before vancomycin treatment can be effective to prevent vancomycin-induced DRESS (Rwandamuriye et al., 2019; Bellon et al., 2022). Hama et al. (2022) reported that the frequency of HLA-A*32:01 is 6.8% in European population and 20% of whom developed vancomycin-induced DRESS. Unlike drug-induced SJS/TEN and DRESS, there has not been a definite link between drug-induced AGEP and specific HLA genotypes (Owen and Jones, 2021). Individuals with IL-36 RA deficiency seems to being subjected to drug-induced AGEP. However, it is still unclear how IL-36 RA deficiency leads to AGEP (Gabay and Towne, 2015).
5 Current trends and future perspectives
With the increasing number of published studies regarding genetic polymorphisms associated with drug delayed hypersensitivity reaction, HLA alleles of SCARs could be drug-specific, ethnicity-specific and phenotypic-specific (Tempark et al., 2022). Considering the morbidity rate, mortality rate and economic burden of SCARs, it is imperative for SCAR prevention to have an efficient and effective method (Yang et al., 2021). Serum granulysin level can be not only a potential marker for the early phase of SJS/TEN, but also a predictive marker for DRESS diagnosis and prognosis (Chung et al., 2008; Saito et al., 2012). Serum IL-15 level can become a marker for SJS/TEN early diagnosis and prognosis monitoring (Su et al., 2017). In addition, serum TARC level was identified as a potential biomarker for DRESS severity and prognosis (Komatsu-Fujii et al., 2018). Moreover, epigenetic association has been found in SCARs patients (such as ITGB2 methylation associated with allopurinol-induced SCARs) (Liu et al., 2023). Recently, the high-throughput technologies including whole genome sequencing (WGS) and whole exome sequencing (WES) offer us rapid method to screen the genetic variants (Wang et al., 2022a). A number of studies have advocated the use of pharmacogenetic testing in terms of cost-effectiveness. Compared to dealing with the life-threatening severe ADR, single HLA allele testing alleviates the cost. Currently, HLA-B*57:01 screening before abacavir treatment, HLA-B*15:02 screening before cabamazepine treatment and HLA-B*58:01 screening before allopurinol treatment have been a standard operation procedure for SCARs prevention (Hung et al., 2005; Mallal et al., 2008; Fan et al., 2017). Hopefully, with the increasingly studies regarding antibiotics as well as SR and HLA alleles, the promising HLA molecules will become standard screening before antibiotics prescription.
Author contributions
C-HW, C-WW, K-CL, S-IH, and W-HC contributed to the conception. C-HW writing of the manuscript. C-WW, W-TC, K-CL, C-BC, S-IH, and W-HC reviewed and revised the manuscript. All authors contributed to the article and approved the submitted version.
Funding
This work was supported by grants from the National Science and Technology Council, Taiwan (NSTC 109-2320-B-182A-008 -MY3, 110-2320-B-182A-014-MY3, 111-2314-B-182A-113-MY3, and 111-2326-B-182A-003-), and Chang Gung Memorial Hospital (CIRPG3M0061-2, CLRPG3N0021 and CMRPG3M1081). Additionally, the Figure 1 is created with BioRender.com.
Conflict of interest
The authors declare that the research was conducted in the absence of any commercial or financial relationships that could be construed as a potential conflict of interest.
Publisher’s note
All claims expressed in this article are solely those of the authors and do not necessarily represent those of their affiliated organizations, or those of the publisher, the editors and the reviewers. Any product that may be evaluated in this article, or claim that may be made by its manufacturer, is not guaranteed or endorsed by the publisher.
References
Abdin, A. Y., Auker-Howlett, D., Landes, J., Mulla, G., Jacob, C., and Osimani, B. (2019). Reviewing the mechanistic evidence assessors E-synthesis and EBM+: A case study of amoxicillin and drug reaction with eosinophilia and systemic symptoms (DRESS). Curr. Pharm. Des. 25 (16), 1866–1880. doi:10.2174/1381612825666190628160603
Administration, U. S. F. (2023). Table of pharmacogenomic biomarkers in drug labeling. Available at: https://www.fda.gov/drugs/science-andresearch-drugs/table-pharmacogenomic-biomarkers-drug-labeling.
Adwan, M. H. (2017). Drug reaction with eosinophilia and systemic symptoms (DRESS) syndrome and the rheumatologist. Curr. Rheumatol. Rep. 19 (1), 3. doi:10.1007/s11926-017-0626-z
Agier, M. S., Boivin, N., Maruani, A., Giraudeau, B., Gouraud, A., Haramburu, F., et al. (2016). Risk assessment of drug-induced drug reaction with eosinophilia and systemic symptoms (DRESS) syndrome: A disproportionality analysis using the French pharmacovigilance database. Br. J. Dermatol 175 (5), 1067–1069. doi:10.1111/bjd.14649
Arora, R., Pande, R. K., Panwar, S., and Gupta, V. (2021). Drug-related stevens–johnson syndrome and toxic epidermal necrolysis: A review. Indian J. Crit. Care Med. 25, 575–579. doi:10.5005/jp-journals-10071-23826
Asyraf, P. A., Kusnadi, I. F., Stefanus, J., Khairinisa, M. A., and Abdulah, R. (2022). Clinical manifestations and genetic influences in sulfonamide-induced hypersensitivity. Drug Healthc. Patient Saf. 14, 113–124. doi:10.2147/DHPS.S347522
Barbarino, J. M., Kroetz, D. L., Klein, T. E., and Altman, R. B. (2015). PharmGKB summary: Very important pharmacogene information for human leukocyte antigen B. Pharmacogenet Genomics 25 (4), 205–221. doi:10.1097/FPC.0000000000000118
Bellon, T., Lerma, V., Guijarro, J., Ramírez, E., Martínez, C., Escudero, C., et al. (2022). LTT and HLA testing as diagnostic tools in Spanish vancomycin-induced DRESS cases: A case-control study. Front. Pharmacol. 13, 959321. doi:10.3389/fphar.2022.959321
Bjornsson, E. S., and Bjornsson, H. K. (2017). Mortality associated with drug-induced liver injury (DILI). Transl. Gastroenterol. Hepatol. 2, 114. doi:10.21037/tgh.2017.11.16
Blumenthal, K. G., Peter, J. G., Trubiano, J. A., and Phillips, E. J. (2019). Antibiotic allergy. Lancet 393 (10167), 183–198. doi:10.1016/S0140-6736(18)32218-9
Cardones, A. R. (2020). Drug reaction with eosinophilia and systemic symptoms (DRESS) syndrome. Clin. Dermatol 38 (6), 702–711. doi:10.1016/j.clindermatol.2020.06.008
Chang, C. J., Chen, C. B., Hung, S. I., Ji, C., and Chung, W. H. (2020). Pharmacogenetic testing for prevention of severe cutaneous adverse drug reactions. Front. Pharmacol. 11, 969. doi:10.3389/fphar.2020.00969
Chen, C.-B., Chen, Y. E., Chu, M. T., Wang, C. W., Hui, R. C. Y., Lu, C. W., et al. (2021). The risk of anti-osteoporotic agent-induced severe cutaneous adverse drug reactions and their association with HLA. J. Eur. Acad. Dermatology Venereol. 35 (3), 712–720. doi:10.1111/jdv.16924
Chen, C. B., Abe, R., Pan, R. Y., Wang, C. W., Hung, S. I., Tsai, Y. G., et al. (2018). An updated review of the molecular mechanisms in drug hypersensitivity. J. Immunol. Res. 2018, 6431694. doi:10.1155/2018/6431694
Chen, C. B., Chen, Y. E., Chu, M. T., Wang, C. W., Hui, R. C. Y., Lu, C. W., et al. (2021). The risk of anti-osteoporotic agent-induced severe cutaneous adverse drug reactions and their association with HLA. J. Eur. Acad. Dermatol Venereol. 35 (3), 712–720. doi:10.1111/jdv.16924
Chen, W. T., Wang, C. W., Lu, C. W., Chen, C. B., Lee, H. E., Hung, S. I., et al. (2018). The function of HLA-B*13:01 involved in the pathomechanism of dapsone-induced severe cutaneous adverse reactions. J. Invest. Dermatol 138, 1546–1554. doi:10.1016/j.jid.2018.02.004
Chiodini, I., Merlotti, D., Falchetti, A., and Gennari, L. (2020). Treatment options for glucocorticoid-induced osteoporosis. Expert Opin. Pharmacother. 21 (6), 721–732. doi:10.1080/14656566.2020.1721467
Chung, W. H., Hung, S. I., Yang, J. Y., Su, S. C., Huang, S. P., Wei, C. Y., et al. (2008). Granulysin is a key mediator for disseminated keratinocyte death in Stevens-Johnson syndrome and toxic epidermal necrolysis. Nat. Med. 14 (12), 1343–1350. doi:10.1038/nm.1884
Clark, D., Alomari, A. K., Burton, K., and Rahnama-Moghadam, S. (2020). A case of vancomycin-induced drug rash with eosinophilia and systemic symptoms (DRESS) syndrome with failure to respond to cyclosporine treatment. Dermatology Online J. 26 (6). doi:10.5070/d3266049312
Copaescu, A., Gibson, A., Li, Y., Trubiano, J. A., and Phillips, E. J. (2020). An updated review of the diagnostic methods in delayed drug hypersensitivity. Front. Pharmacol. 11, 573573. doi:10.3389/fphar.2020.573573
Crux, N. B., and Elahi, S. (2017). Human leukocyte antigen (HLA) and immune regulation: How do classical and non-classical HLA alleles modulate immune response to human immunodeficiency virus and hepatitis C virus infections? Front. Immunol. 8, 832. doi:10.3389/fimmu.2017.00832
Dashti, M., Al-Matrouk, A., Channanath, A., Hebbar, P., Al-Mulla, F., and Thanaraj, T. A. (2022). Distribution of HLA-B alleles and haplotypes in Qatari: Recommendation for establishing pharmacogenomic markers screening for drug hypersensitivity. Front. Pharmacol. 13, 891838. doi:10.3389/fphar.2022.891838
De Luca, J. F., Holmes, N. E., and Trubiano, J. A. (2020). Adverse reactions to vancomycin and cross-reactivity with other antibiotics. Curr. Opin. Allergy Clin. Immunol. 20 (4), 352–361. doi:10.1097/ACI.0000000000000665
de Sousa, M., Bueno, C. S., Mendoza Lopez, R., de Almeida, E. M., Cecatto, R., and de Brito, C. M. (2018). Postbreast cancer surgery outpatient rehabilitation program: Analysis of clinical profile, impact, and direct medical costs. Indian J. Dermatology 63 (1), 22–29. doi:10.4103/jisprm.jisprm_17_18
Dinu, V., Lu, Y., Weston, N., Lithgo, R., Coupe, H., Channell, G., et al. (2020). The antibiotic vancomycin induces complexation and aggregation of gastrointestinal and submaxillary mucins. Sci. Rep. 10 (1), 960. doi:10.1038/s41598-020-57776-3
Dotiwala, F., Mulik, S., Polidoro, R. B., Ansara, J. A., Burleigh, B. A., Walch, M., et al. (2016). Killer lymphocytes use granulysin, perforin and granzymes to kill intracellular parasites. Nat. Med. 22 (2), 210–216. doi:10.1038/nm.4023
Drawz, S. M., and Bonomo, R. A. (2010). Three decades of beta-lactamase inhibitors. Clin. Microbiol. Rev. 23 (1), 160–201. doi:10.1128/CMR.00037-09
Duong, T. A., Valeyrie-Allanore, L., Wolkenstein, P., and Chosidow, O. (2017). Severe cutaneous adverse reactions to drugs. Lancet 390 (10106), 1996–2011. doi:10.1016/S0140-6736(16)30378-6
Endo, Y., Hirahara, K., Yagi, R., Tumes, D. J., and Nakayama, T. (2014). Pathogenic memory type Th2 cells in allergic inflammation. Trends Immunol. 35 (2), 69–78. doi:10.1016/j.it.2013.11.003
Fan, W. L., Shiao, M. S., Hui, R. C. Y., Su, S. C., Wang, C. W., Chang, Y. C., et al. (2017). HLA association with drug-induced adverse reactions. J. Immunol. Res. 2017, 3186328. doi:10.1155/2017/3186328
Feldmeyer, L., Heidemeyer, K., and Yawalkar, N. (2016). Acute generalized exanthematous pustulosis: Pathogenesis, genetic background, clinical variants and therapy. Int. J. Mol. Sci. 17 (8), 1214. doi:10.3390/ijms17081214
Gabay, C., and Towne, J. E. (2015). Regulation and function of interleukin-36 cytokines in homeostasis and pathological conditions. J. Leukoc. Biol. 97 (4), 645–652. doi:10.1189/jlb.3RI1014-495R
Gammoudi, R., Ben Salem, C., Boussofara, L., Fathallah, N., Ghariani, N., Slim, R., et al. (2018). Acute generalized exanthematous pustulosis induced by oxacillin confirmed by patch testing. Contact Dermat. 79 (2), 108–110. doi:10.1111/cod.13005
Ganeshanandan, L., and Lucas, M. (2021). Drug reaction with eosinophilia and systemic symptoms: A complex interplay between drug, T cells, and herpesviridae. Int. J. Mol. Sci. 22 (3), 1127. doi:10.3390/ijms22031127
Gonzalez-Galarza, F. F., McCabe, A., Melo Dos Santos, E. J., Takeshita, L., Ghattaoraya, G., Jones, A. R., et al. (2018). Allele frequency Net database. Methods Mol. Biol. 1802, 49–62. doi:10.1007/978-1-4939-8546-3_4
Guzman, A. I., and Paliza, A. C. (2018). Epidemiology of severe cutaneous adverse drug reactions in a university hospital: A five-year review. J. Med. Univ. Santo Tomas 2 (1), 171–184. doi:10.35460/2546-1621.2017-0031
Hama, N., Abe, R., Gibson, A., and Phillips, E. J. (2022). Drug-induced hypersensitivity syndrome (DIHS)/Drug reaction with eosinophilia and systemic symptoms (DRESS): Clinical features and pathogenesis. J. Allergy Clin. Immunol. Pract. 10 (5), 1155–1167 e5. doi:10.1016/j.jaip.2022.02.004
Haseeb, A., Abourehab, M. A. S., Almalki, W. A., Almontashri, A. M., Bajawi, S. A., Aljoaid, A. M., et al. (2022). Trimethoprim-sulfamethoxazole (bactrim) dose optimization in Pneumocystis jirovecii pneumonia (PCP) management: A systematic review. Int. J. Environ. Res. Public Health 19 (5), 2833. doi:10.3390/ijerph19052833
Hasegawa, A., and Abe, R. (2020). Recent advances in managing and understanding Stevens-Johnson syndrome and toxic epidermal necrolysis. F1000Res 9.
Huang, P. W., Chiou, M. H., Chien, M. Y., Chen, W. W., and Chu, C. Y. (2022). Analysis of severe cutaneous adverse reactions (SCARs) in taiwan drug-injury relief system: 18-year results. J. Formos. Med. Assoc. 121 (8), 1397–1405. doi:10.1016/j.jfma.2021.09.025
Hung, S.-I., Chung, W. H., Liou, L. B., Chu, C. C., Lin, M., Huang, H. P., et al. (2005). HLA-B*5801 allele as a genetic marker for severe cutaneous adverse reactions caused by allopurinol. Proc. Natl. Acad. Sci. 102 (11), 4134–4139. doi:10.1073/pnas.0409500102
Hung, S.-I., Preclaro, I. A. C., Chung, W. H., and Wang, C. W. (2022). Immediate hypersensitivity reactions induced by COVID-19 vaccines: Current trends, potential mechanisms and prevention strategies. Biomedicines 10 (6), 1260. doi:10.3390/biomedicines10061260
Insani, W. N., Whittlesea, C., Alwafi, H., Man, K. K. C., Chapman, S., and Wei, L. (2021). Prevalence of adverse drug reactions in the primary care setting: A systematic review and meta-analysis. PLoS One 16 (5), e0252161. doi:10.1371/journal.pone.0252161
Jantararoungtong, T., Tempark, T., Koomdee, N., Medhasi, S., and Sukasem, C. (2021). Genotyping HLA alleles to predict the development of severe cutaneous adverse drug reactions (SCARs): State-of-the-art. Expert Opin. Drug Metab. Toxicol. 17 (9), 1049–1064. doi:10.1080/17425255.2021.1946514
Jent, P., Berger, J., Kuhn, A., Trautner, B. W., Atkinson, A., and Marschall, J. (2022). Antibiotics for preventing recurrent urinary tract infection: Systematic review and meta-analysis. Open Forum Infect. Dis. 9 (7), ofac327. doi:10.1093/ofid/ofac327
Jiang, H., Wang, C. W., Wang, Z., Dai, Y., Zhu, Y., Lee, Y. S., et al. (2022). Functional and structural characteristics of HLA-B*13:01-mediated specific T cells reaction in dapsone-induced drug hypersensitivity. J. Biomed. Sci. 29 (1), 58. doi:10.1186/s12929-022-00845-8
Jung, J. W., Kim, J. Y., Park, I. W., Choi, B. W., and Kang, H. R. (2018). Genetic markers of severe cutaneous adverse reactions. Korean J. Intern Med. 33 (5), 867–875. doi:10.3904/kjim.2018.126
Kang, D. Y., Yun, J., Lee, S. Y., Koh, Y. I., Sim, D. W., Kim, S., et al. (2021). A nationwide study of severe cutaneous adverse reactions based on the multicenter registry in Korea. J. Allergy Clin. Immunol. Pract. 9 (2), 929–936 e7. doi:10.1016/j.jaip.2020.09.011
Kang, S. Y., Kim, J., Ham, J., Cho, S. H., Kang, H. R., and Kim, H. Y. (2020). Altered T cell and monocyte subsets in prolonged immune reconstitution inflammatory syndrome related with DRESS (drug reaction with eosinophilia and systemic symptoms). Asia Pac Allergy 10 (1), e2. doi:10.5415/apallergy.2020.10.e2
Klaewsongkram, J., Buranapraditkun, S., Thantiworasit, P., Rerknimitr, P., Tuchinda, P., Chularojanamontri, L., et al. (2021). The role of in vitro detection of drug-specific mediator-releasing cells to diagnose different phenotypes of severe cutaneous adverse reactions. Allergy Asthma Immunol. Res. 13 (6), 896–907. doi:10.4168/aair.2021.13.6.896
Kloypan, C., Koomdee, N., Satapornpong, P., Tempark, T., Biswas, M., and Sukasem, C. (2021). A comprehensive review of HLA and severe cutaneous adverse drug reactions: Implication for clinical pharmacogenomics and precision medicine. Pharmaceuticals 14 (11), 1077. doi:10.3390/ph14111077
Komatsu-Fujii, T., Chinuki, Y., Niihara, H., Hayashida, K., Ohta, M., Okazaki, R., et al. (2018). The thymus and activation-regulated chemokine (TARC) level in serum at an early stage of a drug eruption is a prognostic biomarker of severity of systemic inflammation. Allergol. Int. 67 (1), 90–95. doi:10.1016/j.alit.2017.06.001
Kongpan, T., Mahasirimongkol, S., Konyoung, P., Kanjanawart, S., Chumworathayi, P., Wichukchinda, N., et al. (2015). Candidate HLA genes for prediction of co-trimoxazole-induced severe cutaneous reactions. Pharmacogenet Genomics 25 (8), 402–411. doi:10.1097/FPC.0000000000000153
Konvinse, K. C., Trubiano, J. A., Pavlos, R., James, I., Shaffer, C. M., Bejan, C. A., et al. (2019). HLA-A*32:01 is strongly associated with vancomycin-induced drug reaction with eosinophilia and systemic symptoms. J. Allergy Clin. Immunol. 144 (1), 183–192. doi:10.1016/j.jaci.2019.01.045
Krebs, K., Bovijn, J., Zheng, N., Lepamets, M., Censin, J. C., Jürgenson, T., et al. (2020). Genome-wide study identifies association between HLA-B(*)55:01 and self-reported penicillin allergy. Am. J. Hum. Genet. 107 (4), 612–621. doi:10.1016/j.ajhg.2020.08.008
Krismawati, H., Irwanto, A., Pongtiku, A., Irwan, I. D., Maladan, Y., Sitanggang, Y. A., et al. (2020). Validation study of HLA-B*13:01 as a biomarker of dapsone hypersensitivity syndrome in leprosy patients in Indonesia. PLoS Negl. Trop. Dis. 14 (10), e0008746. doi:10.1371/journal.pntd.0008746
Kuijper, E. C., French, L. E., Tensen, C. P., Vermeer, M. H., and Bouwes Bavinck, J. N. (2020). Clinical and pathogenic aspects of the severe cutaneous adverse reaction epidermal necrolysis (EN). J. Eur. Acad. Dermatol Venereol. 34 (9), 1957–1971. doi:10.1111/jdv.16339
Kulkarni, M. P., Chinta, S., Sosa, F., Nasr, R., and Kelly, P. (2022). Drug rash with eosinophilia and systemic symptoms (DRESS) syndrome due to vancomycin. Cureus 14 (6), e26219. doi:10.7759/cureus.26219
Lee, H. Y., Shen, M. X., Lim, Y. L., Tay, Y. K., Chan, M. M. F., Pang, S. M., et al. (2016). Increased risk of strontium ranelate-related SJS/TEN is associated with HLA. Osteoporos. Int. 27 (8), 2577–2583. doi:10.1007/s00198-016-3568-9
Lee, K. H., Kang, D. Y., Kim, H. H., Kim, Y. J., Kim, H. J., Kim, J. H., et al. (2022). Reducing severe cutaneous adverse and type B adverse drug reactions using pre-stored human leukocyte antigen genotypes. Clin. Transl. Allergy 12 (1), e12098. doi:10.1002/clt2.12098
Li, X., Li, G., Yang, J., Jin, G., Shao, Y., Li, Y., et al. (2022). Drug resistance (dapsone, rifampicin, ofloxacin) and resistance-related gene mutation features in leprosy patients: A systematic review and meta-analysis. Int. J. Mol. Sci. 23 (20), 12443. doi:10.3390/ijms232012443
Lin, Y. F., Yang, C. H., Sindy, H., Lin, J. Y., Rosaline Hui, C. Y., Tsai, Y. C., et al. (2014). Severe cutaneous adverse reactions related to systemic antibiotics. Clin. Infect. Dis. 58 (10), 1377–1385. doi:10.1093/cid/ciu126
Liu, H., Wang, Z., Bao, F., Wang, C., Sun, L., Zhang, H., et al. (2019). Evaluation of prospective HLA-B*13:01 screening to prevent dapsone hypersensitivity syndrome in patients with leprosy. JAMA Dermatol 155 (6), 666–672. doi:10.1001/jamadermatol.2018.5360
Liu, Y., Wang, C. W., Chen, C. B., Yu, K. H., Wu, Y. J., Choon, S. E., et al. (2023). DNA methylation of ITGB2 contributes to allopurinol hypersensitivity. Clin. Immunol. 248, 109250. doi:10.1016/j.clim.2023.109250
Liu, Z. J., Tian, Y. T., Shi, B. Y., Zhou, Y., and Jia, X. S. (2020). Association between mutation of interleukin 36 receptor antagonist and generalized pustular psoriasis: A PRISMA-compliant systematic review and meta-analysis. Med. Baltim. 99 (45), e23068. doi:10.1097/MD.0000000000023068
Lonjou, C., Borot, N., Sekula, P., Ledger, N., Thomas, L., Halevy, S., et al. (2008). A European study of HLA-B in Stevens-Johnson syndrome and toxic epidermal necrolysis related to five high-risk drugs. Pharmacogenet Genomics 18 (2), 99–107. doi:10.1097/FPC.0b013e3282f3ef9c
Mallal, S., Phillips, E., Carosi, G., Molina, J. M., Workman, C., Tomazic, J., et al. (2008). HLA-B*5701 screening for hypersensitivity to abacavir. N. Engl. J. Med. 358 (6), 568–579. doi:10.1056/NEJMoa0706135
Martinez-De la Torre, A., van Weenen, E., Kraus, M., Weiler, S., Feuerriegel, S., and Burden, A. M. (2021). A network analysis of drug combinations associated with acute generalized exanthematous pustulosis (AGEP). J. Clin. Med. 10 (19), 4486. doi:10.3390/jcm10194486
Masters, P. A., O'Bryan, T. A., Zurlo, J., Miller, D. Q., and Joshi, N. (2003). Trimethoprim-sulfamethoxazole revisited. Archives Intern. Med. 163 (4), 402–410. doi:10.1001/archinte.163.4.402
Metzemaekers, M., Gouwy, M., and Proost, P. (2020). Neutrophil chemoattractant receptors in health and disease: Double-edged swords. Cell Mol. Immunol. 17 (5), 433–450. doi:10.1038/s41423-020-0412-0
Minhas, J. S., Wickner, P. G., Long, A. A., Banerji, A., and Blumenthal, K. G. (2016). Immune-mediated reactions to vancomycin: A systematic case review and analysis. Ann. Allergy Asthma Immunol. 116 (6), 544–553. doi:10.1016/j.anai.2016.03.030
Miyagawa, F., and Asada, H. (2021). Chemokines in severe cutaneous adverse reactions (SCARs). Biomolecules 11 (6), 847. doi:10.3390/biom11060847
Miyagawa, F., and Asada, H. (2021). Current perspective regarding the immunopathogenesis of drug-induced hypersensitivity syndrome/drug reaction with eosinophilia and systemic symptoms (DIHS/DRESS). Int. J. Mol. Sci. 22 (4), 2147. doi:10.3390/ijms22042147
Mockenhaupt, M. (2012). Epidemiology of cutaneous adverse drug reactions. Chem. Immunol. Allergy 97, 1–17. doi:10.1159/000335612
Mustafa, S. S., Ostrov, D., and Yerly, D. (2018). Severe cutaneous adverse drug reactions: Presentation, risk factors, and management. Curr. Allergy Asthma Rep. 18 (4), 26. doi:10.1007/s11882-018-0778-6
Nakamura, R., Ozeki, T., Hirayama, N., Sekine, A., Yamashita, T., Mashimo, Y., et al. (2020). Association of HLA-A*11:01 with sulfonamide-related severe cutaneous adverse reactions in Japanese patients. J. Invest. Dermatol 140 (8), 1659–1662. doi:10.1016/j.jid.2019.12.025
Nakkam, N., Gibson, A., Mouhtouris, E., Konvinse, K. C., Holmes, N. E., Chua, K. Y., et al. (2021). Cross-reactivity between vancomycin, teicoplanin, and telavancin in patients with HLA-A *32:01-positive vancomycin-induced DRESS sharing an HLA class II haplotype. J. Allergy Clin. Immunol. 147 (1), 403–405. doi:10.1016/j.jaci.2020.04.056
Nakkam, N., Saksit, N., Konyoung, P., Amornpinyo, W., Khunarkornsiri, U., Purimart, D., et al. (2022). Associations of HLA and drug-metabolizing enzyme genes in co-trimoxazole-induced severe cutaneous adverse reactions. Drug Metabolism Pharmacokinet. 47, 100480. doi:10.1016/j.dmpk.2022.100480
Neerukonda, V. K., and Stagner, A. M. (2021). Stevens johnson syndrome: A review of a vision and life-threatening mucocutaneous disease including histopathology with updates on pathogenesis and genetic risk factors. Semin. Ophthalmol. 36 (4), 270–281. doi:10.1080/08820538.2021.1893764
Negrini, S., and Becquemont, L. (2017). HLA-associated drug hypersensitivity and the prediction of adverse drug reactions. Pharmacogenomics 18 (15), 1441–1457. doi:10.2217/pgs-2017-0090
Nicoletti, P., Carr, D. F., Barrett, S., McEvoy, L., Friedmann, P. S., Shear, N. H., et al. (2021). Beta-lactam-induced immediate hypersensitivity reactions: A genome-wide association study of a deeply phenotyped cohort. J. Allergy Clin. Immunol. 147 (5), 1830–1837 e15. doi:10.1016/j.jaci.2020.10.004
Nowsheen, S., Lehman, J. S., and El-Azhary, R. A. (2021). Differences between Stevens-Johnson syndrome versus toxic epidermal necrolysis. Int. J. Dermatol 60 (1), 53–59. doi:10.1111/ijd.15287
Ogese, M. O., Lister, A., Gardner, J., Meng, X., Alfirevic, A., Pirmohamed, M., et al. (2021). Deciphering adverse drug reactions: In vitro priming and characterization of vancomycin-specific T cells from healthy donors expressing HLA-A*32:01. Toxicol. Sci. 183 (1), 139–153. doi:10.1093/toxsci/kfab084
Oh, D. A. Q., Yeo, Y. W., Choo, K. J. L., Pang, S. M., Oh, C. C., and Lee, H. Y. (2021). Acute generalized exanthematous pustulosis: Epidemiology, clinical course, and treatment outcomes of patients treated in an Asian academic medical center. JAAD Int. 3, 1–6. doi:10.1016/j.jdin.2020.12.004
Olsson-Brown, A., Yip, V., Ogiji, E. D., Jolly, C., Ressel, L., Sharma, A., et al. (2022). TNF-α‒Mediated keratinocyte expression and release of matrix metalloproteinase 9: Putative mechanism of pathogenesis in stevens‒johnson syndrome/toxic epidermal necrolysis. J. Investigative Dermatology. doi:10.1016/j.jid.2022.11.024
Owen, C. E., and Jones, J. M. (2021). Recognition and management of severe cutaneous adverse drug reactions (including drug reaction with eosinophilia and systemic symptoms, stevens-johnson syndrome, and toxic epidermal necrolysis). Med. Clin. North Am. 105 (4), 577–597. doi:10.1016/j.mcna.2021.04.001
Park, H. J., Park, J. W., Kim, S. H., Choi, S. Y., Kim, H. K., Jung, C. G., et al. (2020). The HLA-B*13:01 and the dapsone hypersensitivity syndrome in Korean and asian populations: Genotype- and meta-analyses. Expert Opin. Drug Saf. 19 (10), 1349–1356. doi:10.1080/14740338.2020.1796965
Pejčić, A. V. (2021). Stevens-johnson syndrome and toxic epidermal necrolysis associated with the use of macrolide antibiotics: A review of published cases. Int. J. Dermatology 60 (1), 12–24. doi:10.1111/ijd.15144
Pelletier, M., Maggi, L., Micheletti, A., Lazzeri, E., Tamassia, N., Costantini, C., et al. (2010). Evidence for a cross-talk between human neutrophils and Th17 cells. Blood 115 (2), 335–343. doi:10.1182/blood-2009-04-216085
Pettit, C., Trinidad, J., and Kaffenberger, B. (2020). A case of vancomycin-induced acute generalized exanthematous pustulosis confirmed by patch testing. J. Clin. aesthetic dermatology 13, 35–36.
Pichler, W. J., and Brüggen, M.-C. (2022). Viral infections and drug hypersensitivity. Allergy 78, 60–70. doi:10.1111/all.15558
Piipponen, M., Li, D., and Landén, N. X. (2020). The immune functions of keratinocytes in skin wound healing. Int. J. Mol. Sci. 21 (22), 8790. doi:10.3390/ijms21228790
Pirmohamed, M. (2019). HLA- and immune-mediated adverse drug reactions: Another hit with vancomycin. J. Allergy Clin. Immunol. 144 (1), 44–45. doi:10.1016/j.jaci.2019.04.009
Pratoomwun, J., Thomson, P., Jaruthamsophon, K., Tiyasirichokchai, R., Jinda, P., Rerkpattanapipat, T., et al. (2021). Characterization of T-cell responses to SMX and SMX-NO in Co-trimoxazole hypersensitivity patients expressing HLA-B*13:01. Front. Immunol. 12, 658593. doi:10.3389/fimmu.2021.658593
Puangpetch, A., Koomdee, N., Chamnanphol, M., Jantararoungtong, T., Santon, S., Prommas, S., et al. (2014). HLA-B allele and haplotype diversity among Thai patients identified by PCR-SSOP: Evidence for high risk of drug-induced hypersensitivity. Front. Genet. 5, 478. doi:10.3389/fgene.2014.00478
Rao, P. N., and Lakshmi, T. S. (2001). Increase in the incidence of dapsone hypersensitivity syndrome--an appraisal. Lepr. Rev. 72 (1), 57–62. doi:10.5935/0305-7518.20010009
Reunala, T., Hervonen, K., and Salmi, T. (2021). Dermatitis herpetiformis: An update on diagnosis and management. Am. J. Clin. Dermatol 22 (3), 329–338. doi:10.1007/s40257-020-00584-2
Romano, A., Oussalah, A., Chery, C., Guéant-Rodriguez, R. M., Gaeta, F., Cornejo-Garcia, J. A., et al. (2022). Next-generation sequencing and genotype association studies reveal the association of HLA-DRB3*02:02 with delayed hypersensitivity to penicillins. Allergy 77 (6), 1827–1834. doi:10.1111/all.15147
Rutkowski, K., Taylor, C., and Wagner, A. (2017). HLA B62 as a possible risk factor for drug reaction with eosinophilia and systemic symptoms to piperacillin/tazobactam. J. Allergy Clin. Immunol. Pract. 5 (3), 829–830. doi:10.1016/j.jaip.2016.10.008
Rwandamuriye, F. X., Chopra, A., Konvinse, K. C., Choo, L., Trubiano, J. A., Shaffer, C. M., et al. (2019). A rapid allele-specific assay for HLA-A*32:01 to identify patients at risk for vancomycin-induced drug reaction with eosinophilia and systemic symptoms. J. Mol. Diagn 21 (5), 782–789. doi:10.1016/j.jmoldx.2019.04.006
Sadek, M., Iqbal, O., Siddiqui, F., Till, S., Mazariegos, M., Campbell, E., et al. (2021). The role of IL-13, IL-15 and granulysin in the pathogenesis of stevens-johnson syndrome/toxic epidermal necrolysis. Clin. Appl. Thromb. Hemost. 27, 1076029620950831. doi:10.1177/1076029620950831
Saito, N., Abe, R., Yoshioka, N., Murata, J., Fujita, Y., and Shimizu, H. (2012). Prolonged elevation of serum granulysin in drug-induced hypersensitivity syndrome. Br. J. Dermatol 167 (2), 452–453. doi:10.1111/j.1365-2133.2012.10921.x
Saito, N. (2014). An annexin A1–FPR1 interaction contributes to necroptosis of keratinocytes in severe cutaneous adverse drug reactions. Sci. Transl. Med. 6, 245ra95.
Satapornpong, P., Pratoomwun, J., Rerknimitr, P., Klaewsongkram, J., Nakkam, N., Rungrotmongkol, T., et al. (2021). HLA-B*13:01 is a predictive marker of dapsone-induced severe cutaneous adverse reactions in Thai patients. Front. Immunol. 12, 661135. doi:10.3389/fimmu.2021.661135
Schunkert, E. M., and Divito, S. J. (2021). Updates and insights in the diagnosis and management of DRESS syndrome. Curr. Dermatol Rep. 10 (4), 192–204. doi:10.1007/s13671-021-00348-z
Sharifzadeh, S., Mohammadpour, A. H., Tavanaee, A., and Elyasi, S. (2021). Antibacterial antibiotic-induced drug reaction with eosinophilia and systemic symptoms (DRESS) syndrome: A literature review. Eur. J. Clin. Pharmacol. 77 (3), 275–289. doi:10.1007/s00228-020-03005-9
Shiohara, T., and Mizukawa, Y. (2019). Drug-induced hypersensitivity syndrome (DiHS)/drug reaction with eosinophilia and systemic symptoms (DRESS): An update in 2019. Allergol. Int. 68 (3), 301–308. doi:10.1016/j.alit.2019.03.006
Shoshi, A., Hoppe, T., Kormeier, B., Ogultarhan, V., and Hofestädt, R. (2015). GraphSAW: A web-based system for graphical analysis of drug interactions and side effects using pharmaceutical and molecular data. BMC Med. Inf. Decis. Mak. 15, 15. doi:10.1186/s12911-015-0139-5
Singvijarn, P., Manuyakorn, W., Mahasirimongkol, S., Wattanapokayakit, S., Inunchot, W., Wichukchinda, N., et al. (2021). Association of HLA genotypes with Beta-lactam antibiotic hypersensitivity in children. Asian Pac J. Allergy Immunol. 39 (3), 197–205. doi:10.12932/AP-271118-0449
Spadaro, A., Gartland, R., and Conlon, L. W. (2021). Images in emergency medicine: Acute generalized exanthematous pustulosis. J. Emerg. Med. 61 (3), e48–e49. doi:10.1016/j.jemermed.2021.03.007
Stern, R. S., and Divito, S. J. (2017). Stevens-johnson syndrome and toxic epidermal necrolysis: Associations, outcomes, and pathobiology-thirty years of progress but still much to Be done. J. Invest. Dermatol 137 (5), 1004–1008. doi:10.1016/j.jid.2017.01.003
Stirton, H., N.H. Shear, and R.P. Dodiuk-Gad, (2022). Drug reaction with eosinophilia and systemic symptoms (DReSS)/Drug-Induced hypersensitivity syndrome (DiHS)—readdressing the DReSS. Biomedicines 10, 999.
Su, S. C., Mockenhaupt, M., Wolkenstein, P., Dunant, A., Le Gouvello, S., Chen, C. B., et al. (2017). Interleukin-15 is associated with severity and mortality in stevens-johnson syndrome/toxic epidermal necrolysis. J. Invest. Dermatol 137 (5), 1065–1073. doi:10.1016/j.jid.2016.11.034
Sukasem, C., Pratoomwun, J., Satapornpong, P., Klaewsongkram, J., Rerkpattanapipat, T., Rerknimitr, P., et al. (2020). Genetic association of Co-Trimoxazole-Induced severe cutaneous adverse reactions is phenotype-specific: HLA class I genotypes and haplotypes. Clin. Pharmacol. Ther. 108 (5), 1078–1089. doi:10.1002/cpt.1915
Svoboda, S. A., Bisbee, E. L., Bender, N., and Motaparthi, K. (2022). Acute generalized exanthematous pustulosis sine pustules: A case series. JAAD Case Rep. 23, 24–26. doi:10.1016/j.jdcr.2022.02.009
Szatkowski, J., and Schwartz, R. A. (2015). Acute generalized exanthematous pustulosis (AGEP): A review and update. J. Am. Acad. Dermatol 73 (5), 843–848. doi:10.1016/j.jaad.2015.07.017
Tangamornsuksan, W., and Lohitnavy, M. (2018). Association between HLA-B*1301 and dapsone-induced cutaneous adverse drug reactions: A systematic review and meta-analysis. JAMA Dermatol 154 (4), 441–446. doi:10.1001/jamadermatol.2017.6484
Tempark, T., John, S., Rerknimitr, P., Satapornpong, P., and Sukasem, C. (2022). Drug-induced severe cutaneous adverse reactions: Insights into clinical presentation, immunopathogenesis, diagnostic methods, treatment, and pharmacogenomics. Front. Pharmacol. 13, 832048. doi:10.3389/fphar.2022.832048
Tempark, T., Satapornpong, P., Rerknimitr, P., Nakkam, N., Saksit, N., Wattanakrai, P., et al. (2017). Dapsone-induced severe cutaneous adverse drug reactions are strongly linked with HLA-B*13: 01 allele in the Thai population. Pharmacogenet Genomics 27 (12), 429–437. doi:10.1097/FPC.0000000000000306
Tewary, P., Yang, D., de la Rosa, G., Li, Y., Finn, M. W., Krensky, A. M., et al. (2010). Granulysin activates antigen-presenting cells through TLR4 and acts as an immune alarmin. Blood 116 (18), 3465–3474. doi:10.1182/blood-2010-03-273953
Tohyama, M., and Hashimoto, K. (2012). Immunological mechanisms of epidermal damage in toxic epidermal necrolysis. Curr. Opin. Allergy Clin. Immunol. 12 (4), 376–382. doi:10.1097/ACI.0b013e328355b865
Tohyama, M., Watanabe, H., Murakami, S., Shirakata, Y., Sayama, K., Iijima, M., et al. (2012). Possible involvement of CD14+ CD16+ monocyte lineage cells in the epidermal damage of Stevens–Johnson syndrome and toxic epidermal necrolysis. Br. J. Dermatology 166 (2), 322–330. doi:10.1111/j.1365-2133.2011.10649.x
Torres-Navarro, I., Abril-Pérez, C., Roca-Ginés, J., Sánchez-Arráez, J., and Botella-Estrada, R. (2020). A case of cefditoren-induced acute generalized exanthematous pustulosis during COVID-19 pandemics. Severe cutaneous adverse reactions are an issue. J. Eur. Acad. Dermatology Venereol. 34 (10), e537–e539. doi:10.1111/jdv.16664
Ushigome, Y., Mizukawa, Y., Kimishima, M., Yamazaki, Y., Takahashi, R., Kano, Y., et al. (2018). Monocytes are involved in the balance between regulatory T cells and Th17 cells in severe drug eruptions. Clin. Exp. Allergy 48 (11), 1453–1463. doi:10.1111/cea.13252
Verma, R., Vasudevan, B., and Pragasam, V. (2013). Severe cutaneous adverse drug reactions. Med. J. Armed Forces India 69 (4), 375–383. doi:10.1016/j.mjafi.2013.01.007
Verneuil, L., Leboeuf, C., Vidal, J. S., Ratajczak, P., Comoz, F., Ameisen, J. C., et al. (2011). Endothelial damage in all types of T-lymphocyte–mediated drug-induced eruptions. Archives Dermatology 147 (5), 579–584. doi:10.1001/archdermatol.2011.104
Vestergaard, C., Bang, K., Gesser, B., Yoneyama, H., Matsushima, K., and Larsen, C. G. (2000). A Th2 chemokine, TARC, produced by keratinocytes may recruit CLA+CCR4+ lymphocytes into lesional atopic dermatitis skin. J. Invest. Dermatol 115 (4), 640–646. doi:10.1046/j.1523-1747.2000.00115.x
Villani, A., Baldo, A., De Fata Salvatores, G., Desiato, V., Ayala, F., and Donadio, C. (2017). Acute localized exanthematous pustulosis (ALEP): Review of literature with report of case caused by amoxicillin-clavulanic acid. Dermatol Ther. (Heidelb) 7 (4), 563–570. doi:10.1007/s13555-017-0206-1
Wang, C. W., Divito, S. J., Chung, W. H., and Hung, S. I. (2022). Advances in the pathomechanisms of delayed drug hypersensitivity. Immunol. Allergy Clin. North Am. 42 (2), 357–373. doi:10.1016/j.iac.2022.01.002
Wang, C. W., Lin, W. C., Chen, W. T., Chen, C. B., Lu, C. W., Hou, H. H., et al. (2022). Associations of HLA-A and HLA-B with vancomycin-induced drug reaction with eosinophilia and systemic symptoms in the Han-Chinese population. Front. Pharmacol. 13, 954596. doi:10.3389/fphar.2022.954596
Wang, C. W., Preclaro, I. A. C., Lin, W. H., and Chung, W. H. (2022). An updated review of genetic associations with severe adverse drug reactions: Translation and implementation of pharmacogenomic testing in clinical practice. Front. Pharmacol. 13, 886377. doi:10.3389/fphar.2022.886377
Wang, C. W., Tassaneeyakul, W., Chen, C. B., Chen, W. T., Teng, Y. C., Huang, C. Y., et al. (2021). Whole genome sequencing identifies genetic variants associated with co-trimoxazole hypersensitivity in Asians. J. Allergy Clin. Immunol. 147 (4), 1402–1412. doi:10.1016/j.jaci.2020.08.003
Wang, H., Yan, L., Zhang, G., Chen, X., Yang, J., Li, M., et al. (2013). Association between HLA-B*1301 and dapsone-induced hypersensitivity reactions among leprosy patients in China. J. Invest. Dermatol 133 (11), 2642–2644. doi:10.1038/jid.2013.192
Weinborn, M., Barbaud, A., Truchetet, F., Beurey, P., Germain, L., and Cribier, B. (2016). Histopathological study of six types of adverse cutaneous drug reactions using granulysin expression. Int. J. Dermatol 55 (11), 1225–1233. doi:10.1111/ijd.13350
Wilkerson, R. G., and Drug Hypersensitivity Reactions, (2022). Drug hypersensitivity reactions. Emerg. Med. Clin. North Am. 40 (1), 39–55. doi:10.1016/j.emc.2021.09.001
Wolfson, A. R., Zhou, L., Phadke, N. A., Chow, O. A., and Blumenthal, K. G. (2019). Drug reaction with eosinophilia and systemic symptoms (DRESS) syndrome identified in the electronic health record allergy module. J. Allergy Clin. Immunol. Pract. 7 (2), 633–640. doi:10.1016/j.jaip.2018.08.013
Wolkenstein, P., Charue, D., Laurent, P., Revuz, J., Roujeau, J. C., and Bagot, M. (1995). Metabolic predisposition to cutaneous adverse drug reactions: Role in toxic epidermal necrolysis caused by sulfonamides and anticonvulsants. Archives Dermatology 131 (5), 544–551. doi:10.1001/archderm.131.5.544
Wong, J. C., Au, E. Y., Yeung, H. H., Lau, C. S., and Li, P. H. (2021). Piperacillin-tazobactam allergies: An exception to usual penicillin allergy. Allergy Asthma Immunol. Res. 13 (2), 284–294. doi:10.4168/aair.2021.13.2.284
Wung, C. H., Chung, C. Y., Wu, P. Y., Huang, J. C., Tsai, Y. C., Chen, S. C., et al. (2021). Associations between metabolic syndrome and obesity-related indices and bone mineral density T-score in hemodialysis patients. J. Pers. Med. 11 (8), 775. doi:10.3390/jpm11080775
Yang, C. Y., Chen, C. H., Wang, H. Y., Hsiao, H. L., Hsiao, Y. H., and Chung, W. H. (2014). Strontium ranelate related stevens-johnson syndrome: A case report. Osteoporos. Int. 25 (6), 1813–1816. doi:10.1007/s00198-014-2688-3
Yang, M. S., Lee, J. Y., Kim, J., Kim, G. W., Kim, B. K., Kim, J. Y., et al. (2016). Incidence of stevens-johnson syndrome and toxic epidermal necrolysis: A nationwide population-based study using national health insurance database in Korea. PLoS One 11 (11), e0165933. doi:10.1371/journal.pone.0165933
Yang, S.-C., Chen, C. B., Lin, M. Y., Zhang, Z. Y., Jia, X. Y., Huang, M., et al. (2021). Genetics of severe cutaneous adverse reactions. Front. Med. 8, 652091. doi:10.3389/fmed.2021.652091
Yang, Y., Chen, S., Yang, F., Zhang, L., Alterovitz, G., Zhu, H., et al. (2017). HLA-B*51:01 is strongly associated with clindamycin-related cutaneous adverse drug reactions. Pharmacogenomics J. 17 (6), 501–505. doi:10.1038/tpj.2016.61
Young, S., Ojaimi, S., Dunckley, H., Douglas, M. W., Kok, J., Fulcher, D. A., et al. (2014). Vancomycin-associated drug reaction with eosinophilia and systemic symptoms syndrome. Intern Med. J. 44 (7), 694–696. doi:10.1111/imj.12462
Zhang, F. R., Liu, H., Irwanto, A., Fu, X. A., Li, Y., Yu, G. Q., et al. (2013). HLA-B*13:01 and the dapsone hypersensitivity syndrome. N. Engl. J. Med. 369 (17), 1620–1628. doi:10.1056/NEJMoa1213096
Zhao, Q., Almutairi, M., Tailor, A., Lister, A., Harper, N., Line, J., et al. (2021). HLA class-II‒restricted CD8(+) T cells contribute to the promiscuous immune response in dapsone-hypersensitive patients. J. Invest. Dermatol 141 (10), 2412–2425 e2. doi:10.1016/j.jid.2021.03.014
Keywords: delayed hypersensitivity reactions, human leukocyte antigens, Stevens-Johnson syndrome, toxic epidermal necrosis, drug reactions with eosinophilia and systemic symptoms
Citation: Wung C-H, Wang C-W, Lai K-C, Chen C-B, Chen W-T, Hung S-I, Chung W-H and Taiwan Severe Cutaneous Adverse Reaction Consortium (2023) Current understanding of genetic associations with delayed hypersensitivity reactions induced by antibiotics and anti-osteoporotic drugs. Front. Pharmacol. 14:1183491. doi: 10.3389/fphar.2023.1183491
Received: 10 March 2023; Accepted: 12 April 2023;
Published: 26 April 2023.
Edited by:
Vijay Suppiah, University of South Australia, AustraliaReviewed by:
Ingrid Fricke-Galindo, Instituto Nacional de Enfermedades Respiratorias-México (INER), MexicoCopyright © 2023 Wung, Wang, Lai, Chen, Chen, Hung and Chung, Taiwan Severe Cutaneous Adverse Reaction Consortium. This is an open-access article distributed under the terms of the Creative Commons Attribution License (CC BY). The use, distribution or reproduction in other forums is permitted, provided the original author(s) and the copyright owner(s) are credited and that the original publication in this journal is cited, in accordance with accepted academic practice. No use, distribution or reproduction is permitted which does not comply with these terms.
*Correspondence: Shuen-Iu Hung, hungshueniu@gmail.com; Wen-Hung Chung, wenhungchung@yahoo.com
†These authors have contributed equally to this work