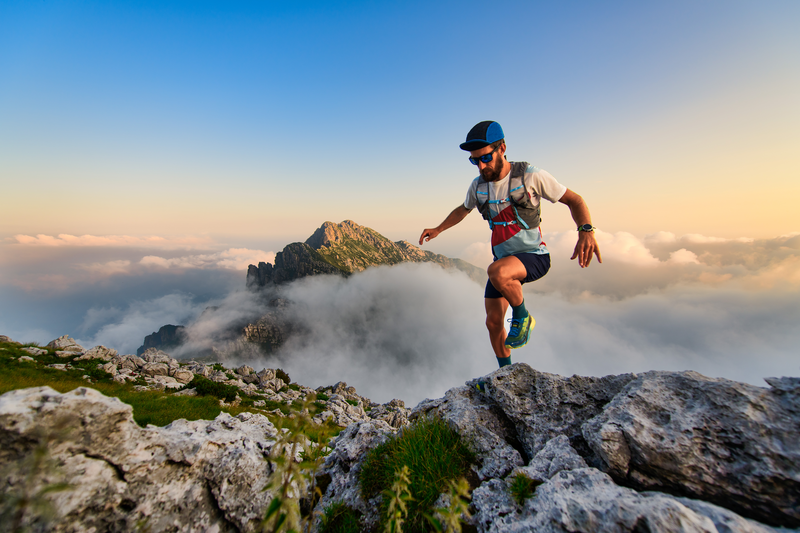
95% of researchers rate our articles as excellent or good
Learn more about the work of our research integrity team to safeguard the quality of each article we publish.
Find out more
OPINION article
Front. Pharmacol. , 04 May 2023
Sec. Translational Pharmacology
Volume 14 - 2023 | https://doi.org/10.3389/fphar.2023.1182571
The situation of opioid abuse has deteriorated in recent years. In 2019, 49,860 people died from opioid overdoses in the USA alone, a 6-fold increase since 2000 (Ko et al., 2020). Of particular concern is opioid abuse (e.g., morphine, fentanyl) by pregnant women. It was found that 6.6% of women self-reported opioid use during pregnancy in the USA, and 21.2% of them disclosed opioid misuse (Ko et al., 2020). Infants born to drug-abusing mothers are at risk of preterm delivery, poor intrauterine growth, and neonatal abstinence syndrome (NAS). Currently, Methadone Maintenance Treatment (MMT) is the recommended therapy for opioid addicts, including pregnant women (World Health Organization, 2014). However, the pharmacokinetics of methadone is greatly affected by pregnancy. Physiological changes during pregnancy (e.g., increases in total body fluid, blood volume, and body fat) and metabolic enzyme activities (CYP2B6, CYP3A4) lead to faster clearances of methadone in pregnant women (Badhan and Gittins, 2021). Consequently, a higher dosage may be required during pregnancy. Some researchers suggest a staged escalation approach, i.e., starting from the lowest pre-natal dose (30 mg once daily) to the maximum 120, 140, and 180 mg daily dose for trimester 1, 2 and 3, respectively (Badhan and Gittins, 2021). This is significantly higher than a standard dosage (15–60 mg/day) and may expose fetuses and neonates to higher risks of NAS. Indeed, of all infants born to mothers receiving MMT, 40%–90% show signs of NAS. For example, in a retrospective study of 67 women (40 received MMT at 10–20 mg/day), it was found that 43% of the infants of mothers receiving MMT required treatment for NAS (Malpas et al., 1995). Similar results were also reported in (Dryden et al., 2009). However, it was also found that there was no strong correlation between the dosage of methadone use and the severity of NAS (Malpas et al., 1995; Berghella et al., 2003).
In a recent pregnant rat model, it was shown that the methadone concentrations in the blood and brain of fetuses were 1.6 and 2.8 times higher than in dams, respectively (Kongstorp et al., 2019) (Figure 1A). The finding highlights the susceptibility of fetuses to maternal methadone exposure. Being lipophilic and with a low molecular weight (309 Da), methadone readily crosses the placenta barrier via passive diffusion. However, the return of methadone from the fetal side to the maternal side (f→m) also requires active transport by the efflux transporter P-glycoprotein (P-gp) (Nanovskaya et al., 2005). P-gp is expressed at the apical membrane of cotyledons, i.e., the maternal side (Gil et al., 2005; Nanovskaya et al., 2005). Therefore, the placenta acts as barrier that retards the backflow of methadone f→m, which causes a higher plasma concentration of methadone at the fetal side than the maternal side (Figure 1A). To assist the clearance of methadone from the fetal side, P-gp acts as the efflux transporter (f→m), on top of the limited passive perfusion. Therefore, an inhibition of P-gp would deteriorate the accumulation of methadone at the fetal side. The phenomenon has been demonstrated in experiments. For example, in an ex vivo placental perfusion experiment, it was shown that inhibition of P-gp led to a 30% increase in methadone in fetal circulation (Nanovskaya et al., 2008). Similar to its role as an efflux transporter in the placenta, P-gp is expressed at the blood-brain barrier (BBB) and acts as an efflux transporter for xenobiotics from brain tissues back to the cerebral flow. Previous studies of P-gp mediated methadone perfusion across the BBB are rarely reported. However, inhibition of P-gp greatly increased the toxicity of another synthetic opioid, fentanyl, in a rat brain model (Yu et al., 2018). The aggregated efflux effects of P-gp on the placental and BBB barriers lead to the higher concentrations in fetal brain not only higher that the maternal brain tissues, but also higher than fetal circulations in rat models (Figure 1A) (Kongstorp et al., 2019).
FIGURE 1. (A) The methadone concentrations in the blood and brain of fetuses were 1.6 and 2.8 times higher than in dams, respectively. Figure adopted from Kongstorp et al. (2019); (B) The diagram for a f-m PBPK model for methadone, and the proposed changes to incorporate the P-gp inhibition/induction kinetics. Equations for at least four compartments (the brain, intestine, placenta, and fetus) of the model need to be revised.
Based on these observations, it is hypothesized that by inducing P-gp at the placental barrier and BBB, the methadone concentration in the fetal blood and brain can be reduced. Consequently, symptoms of CNS may be alleviated. However, co-administration of a P-gp inducer (e.g., Rifamacin) with methadone also induces metabolic enzymes, e.g., CYP3A4, which can lead to a more rapid clearance of methadone and possible withdrawal syndromes in mothers. In addition, a P-gp inhibitor or inducer acting on the intestine barrier may change the bioavailability of methadone if it is administered orally (Carlos et al., 2002). This is a typical drug-drug interaction (DDI) scenario between methadone, P-gp and cytochrome P450 (CYP) enzymes in a specific population (pregnant women and fetuses), where there is a significant knowledge gap.
In this opinion paper, we first provide a brief review of some in vivo, in vitro, and ex vivo models for the role P-gp plays in methadone clearance in fetuses. We then highlight P-gp as a key in fetal-maternal physiologically based pharmacokinetic (PBPK) and ex vivo placenta perfusion models, and propose computational methods for incorporating efflux transporter kinetics in pharmacokinetic models.
As a synthetic opioid agonist, methadone is mainly eliminated via hepatic metabolism by CYP 2B6, and partly by CYP 3A4 (Garrido and Trocóniz, 1999; Kharasch, 2017). Its concentration in the blood has a long half-life (20–35 h), which is crucial to prevent withdrawal symptoms for at least 24 h. In vivo models for fetal exposure to methadone are mainly performed in animals in particular on rats (Gabrielsson et al., 1985; Chen et al., 2015; Kongstorp et al., 2019; Kongstorp et al., 2020). In these models, administering methadone is either via subcutaneous injection (Chen et al., 2015), or through an implanted osmotic minipump to keep constant opioid exposure in dams (Kongstorp et al., 2019). The dose regimen was 10 mg/kg/day, or 1.0 mL/kg of body weight to induce stable blood concentrations of 0.25 ± 0.02 μM methadone in the pregnant rats, which are comparable to the concentrations reported in pregnant women in MMT. However, in vivo data on P-gp mediated fetal exposure to methadone are rare. For example, in a literature review of fetal and offspring exposure to opioids in animals and humans, there was no mention of either inhibition or inducing P-gp (Farid et al., 2008).
Monolayer BeWo cell lines have been used to investigate the permeability of opioids in the placental trophoblast (Nanovskaya et al., 2005; Mortensen et al., 2019). A study investigated the BeWo cell permeability of six opioids (Mortensen et al., 2019), and it was found that the permeability of methadone is lower than oxycodone but higher than morphine and heroin. Another study revealed methadone uptake was increased in the presence of the P-gp inhibitor cyclosporin A (Nanovskaya et al., 2005), reflecting a reduced methadone efflux. In addition, it has been shown that the basolateral to apical transport of known p-glycoprotein substrates (vinblastine, vincristine, and digoxin) is significantly greater than transport to the opposite direction (Ushigome et al., 2000). This is consistent with what has been found in the ex vivo placenta in perfusion experiments (Nanovskaya et al., 2008). Although it is rare, human intestinal cell line Caco-2 was used to investigate transporter-mediated opioid transfer in BBB. The efflux ratio of fentanyl was remarkably reduced when co-incubated with tariquidar, a P-gp inhibitor (Yu et al., 2018). However, the prediction of drug transport through the BBB in vivo may not be accurate since the BBB, and the intestinal musosa are two fundamentally different biologic barriers (Lundquist et al., 2002). Instead, in vitro models of BBB with monolayer of brain capillary endothelial cells were shown to have a good correlation with in vivo rat models (Lundquist et al., 2002).
Ex vivo placental perfusion is considered the “golden standard” for examining the transplacental properties of an investigational drug. In the experiment setup, a single unit of cotyledon is cannulated and connected to perfusates at the maternal and fetal sides separately (Nanovskaya et al., 2008) (Malek et al., 2009) (Kurosawa et al., 2020). In one such study (Nanovskaya et al., 2008), methadone concentrations were measured from the perfusate reservoirs and at sampling ports. It was found that in the maternal to fetal (m→f) perfusion route, there was a biphasic decrease in methadone concentration, i.e., a sharp drop in the first 60 min followed by a plateau in the remaining 180 min. In contrast, the f→m transperfusion showed a gradual decrease of methadone in the fetal artery. The opiate was retained by the placental tissue from both the m→f and the f→m routes.
It is worth pointing out that since placentas are usually collected postpartum, the ex vivo perfusion results only reflect the placental physiology at term, but not at the early stages of gestation. For example, the P-gp expression is lower at the apical membrane in trimester 3 than in other trimesters, which leads to a weaker efflux transport, and consequently a higher methadone accumulation in trimester 3 (Gil et al., 2005). This is unfortunate because the last stage of gestation is also the most crucial time for lowering fetal exposure to opioids and reducing the risk of NAS.
PBPK models are computational tools that quantify the time course of drug concentration in various organs and tissues, represented by compartments (Figure 1B). Fetal-maternal (f-m) PBPK models add compartments of the placenta and fetal organs into a standard model. f-m PBPK models have been developed for many drugs, including methadone (Gabrielsson et al., 1985; Ke et al., 2014). However, to our knowledge, the only pregnant rat model of methadone that has both in vivo experiments and f-m PBPK modelling was developed almost 40 years ago (Gabrielsson et al., 1985). Unfortunately, no DDI mechanism was investigated in that study.
In order to incorporate efflux transporter kinetics in the PBPK scheme shown in Figure 1B, four key compartments, namely, the brain, the placenta, the fetus and the intestine need to be revised (refer to Figure 1B). For the brain compartment, the transport-mediated DDI for BBB was implemented in two compartments, i.e., brain tissue and brain vasculature (Ball et al., 2012), where a passive diffusion term and two transport terms (representing active influx and efflux) are used to simulate the transport. A more complex four-compartment model was proposed in (Gaohua et al., 2016), where cranial and spinal cerebrospinal fluid compartments are added. The placenta compartment can be treated in a similar way, i.e., with apical and basolateral membranes in cotyledons, as has been used in an f-m PBPK model for nicotine (Amice et al., 2021). If methadone is administered orally, such as the liquid formulation being practiced in MMT clinics, then the intestine barrier needs to be implemented in the PBPK model. The pharmacokinetics of methadone in this case is different from when it is administered through infusion or intravenous injection (Chen et al., 2015). For instance, for the administration route of methadone, instead of intravenous injection, an oral dosing regimen will also be used for comparison. It is worth noting that adding the fetal liver into the fetus compartment may not be significant for methadone from ontogeny’s perspective, since some of the major metabolic enzymes (CYP2B6, CYP3A4) are still not mature in fetuses, while other enzymes such as CYP3A7 is expressed in the fetal liver that can metabolise methadone (Wolff et al., 2005). Nevertheless, the fetal liver compartment is important for some other opioids including morphine and buprenorphine, because fetal liver enzymes, e.g., SULT1A3 and SULT2A1 that are involved in these opioids’ sulfation are already active.
A more thorough investigation of the transport properties of methadone in the placenta would require considering several membranes that separate the maternal and fetal circulations. To date, one of the most detailed mathematical models for ex vivo placenta perfusion is that developed for metformin (Kurosawa et al., 2020) and for morphine (Ho et al., 2022). The significance of the work is that the important data of P-gp mediated methadone transport were published (Nanovskaya et al., 2008), which allows an in silico model to be calibrated. Furthermore, the parameters in in silico models can be tuned per the reduced P-gp expressions as gestation progresses (Nanovskaya et al., 2005).
In the above section, we have briefly discussed the current in vivo, in vitro, and in silico models for fetal exposure to methadone, in particular when considering the variable of efflux transporter P-gp. We also described a strategy for incorporating efflux kinetics into PBPK and ex vivo perfusion models. It is clear that none of these models is comprehensive in addressing the multiple facets of the methadone clearance mechanism in pregnant women and fetuses. Still, these models need to provide evidence-based methodology change for the current MMT practice, where clinicians rely on clinical symptoms (withdrawal or overmedication) when prescribing methadone. Efforts need to be made to fill the gaps and challenges. For example, extrapolation of in vivo placenta transfer from animal models to humans is challenging due to the anatomical and functional specificity of the placenta among different species (Myllynen and Vähäkangas, 2013). Similarly, in vitro cell line models may fall short of the prediction of in vivo drug transport (Lundquist et al., 2002). Ex vivo placenta perfusion models can only predict opioid transport properties at term, yet such properties are clearly altered by varying P-gp expression during pregnancy. While in silico models are cost-effective, they are prone to mathematical maneuver or parameter fitting when lacking data (Ho et al., 2022). Nevertheless, there is a more pressing need than ever to integrate the data and know-how gained from multiscale, multidisciplinary models in the era of the “opioid epidemic” and its treatment.
HH and EZ have made editing and intellectual contributions to the work and approved it for publication.
HH and EZ acknowledge the funding support of Chongqing Science and Technology Bureau (Grant number: cstc2022jxjl0299).
The authors declare that the research was conducted in the absence of any commercial or financial relationships that could be construed as a potential conflict of interest.
All claims expressed in this article are solely those of the authors and do not necessarily represent those of their affiliated organizations, or those of the publisher, the editors and the reviewers. Any product that may be evaluated in this article, or claim that may be made by its manufacturer, is not guaranteed or endorsed by the publisher.
Amice, B., Ho, H., Zhang, E., and Bullen, C. (2021). Physiologically based pharmacokinetic modelling for nicotine and cotinine clearance in pregnant women. Front. Pharmacol. 12, 688597. doi:10.3389/fphar.2021.688597
Badhan, R. K. S., and Gittins, R. (2021). Precision dosing of methadone during pregnancy: A pharmacokinetics virtual clinical trials study. J. Subst. Abuse Treat. 130, 108521. doi:10.1016/j.jsat.2021.108521
Ball, K., Bouzom, F., Scherrmann, J-M., Walther, B., and Declèves, X. (2012). Development of a physiologically based pharmacokinetic model for the rat central nervous system and determination of an in vitro–in vivo scaling methodology for the blood–brain barrier permeability of two transporter substrates, morphine and oxycodone. J. Pharm. Sci. 101, 4277–4292. doi:10.1002/jps.23266
Berghella, V., Lim, P. J., Hill, M. K., Cherpes, J., Chennat, J., and Kaltenbach, K. (2003). Maternal methadone dose and neonatal withdrawal. Am. J. Obstetrics Gynecol. 189, 312–317. doi:10.1067/S0002-9378(03)00520-9
Carlos, M. A., Souich, P. D., Carlos, R., Suarez, E., Lukas, J. C., and Calvo, R. (2002). Effect of omeprazole on oral and intravenous RS-methadone pharmacokinetics and pharmacodynamics in the rat. J. Pharm. Sci. 91, 1627–1638. doi:10.1002/jps.10031
Chen, H-H., Chiang, Y-C., Yuan, Z. F., Kuo, C-C., Lai, M-D., Hung, T-W., et al. (2015). Buprenorphine, methadone, and morphine treatment during pregnancy: Behavioral effects on the offspring in rats. NDT 11, 609–618. doi:10.2147/NDT.S70585
Dryden, C., Young, D., Hepburn, M., and Mactier, H. (2009). Maternal methadone use in pregnancy: Factors associated with the development of neonatal abstinence syndrome and implications for healthcare resources. BJOG Int. J. Obstetrics Gynaecol. 116, 665–671. doi:10.1111/j.1471-0528.2008.02073.x
Farid, W. O., Dunlop, S. A., Tait, R. J., and Hulse, G. K. (2008). The effects of maternally administered methadone, buprenorphine and naltrexone on offspring: Review of human and animal data. Curr. Neuropharmacol. 6, 125–150. doi:10.2174/157015908784533842
Gabrielsson, J. L., Johansson, P., Bondesson, U., and Paalzow, L. K. (1985). Analysis of methadone disposition in the pregnant rat by means of a physiological flow model. J. Pharmacokinet. Biopharm. 13, 355–372. doi:10.1007/BF01061474
Gaohua, L., Neuhoff, S., Johnson, T. N., Rostami-Hodjegan, A., and Jamei, M. (2016). Development of a permeability-limited model of the human brain and cerebrospinal fluid (CSF) to integrate known physiological and biological knowledge: Estimating time varying CSF drug concentrations and their variability using in vitro data. Drug Metabolism Pharmacokinet. 31, 224–233. doi:10.1016/j.dmpk.2016.03.005
Garrido, M. J., and Trocóniz, I. F. (1999). Methadone: A review of its pharmacokinetic/pharmacodynamic properties. J. Pharmacol. Toxicol. Methods 42, 61–66. doi:10.1016/S1056-8719(00)00043-5
Gil, S., Saura, R., Forestier, F., and Farinotti, R. (2005). P-glycoprotein expression of the human placenta during pregnancy. Placenta 26, 268–270. doi:10.1016/j.placenta.2004.05.013
Ho, H., Zhang, S., Kurosawa, K., and Chiba, K. (2022). In silico modeling for ex vivo placental transfer of morphine. J. Clin. Pharmacol. 62, 140–146. doi:10.1002/jcph.2105
Ke, A. B., Nallani, S. C., Zhao, P., Rostami-Hodjegan, A., and Unadkat, J. D. (2014). Expansion of a PBPK model to predict disposition in pregnant women of drugs cleared via multiple CYP enzymes, including CYP2B6, CYP2C9 and CYP2C19. Br. J. Clin. Pharmacol. 77, 554–570. doi:10.1111/bcp.12207
Kharasch, E. D. (2017). Current concepts in methadone metabolism and transport. Clin. Pharmacol. Drug Dev. 6, 125–134. doi:10.1002/cpdd.326
Ko, J. Y., D’Angelo, D. V., Haight, S. C., Morrow, B., Cox, S., Salvesen von Essen, B., et al. (2020). Vital signs: Prescription opioid pain reliever use during pregnancy — 34 U.S. Jurisdictions, 2019. MMWR Morb. Mortal. Wkly. Rep. 69, 897–903. doi:10.15585/mmwr.mm6928a1
Kongstorp, M., Bogen, I. L., Stiris, T., and Andersen, J. M. (2019). High accumulation of methadone compared with buprenorphine in fetal rat brain after maternal exposure. J. Pharmacol. Exp. Ther. 371, 130–137. doi:10.1124/jpet.119.259531
Kongstorp, M., Bogen, I. L., Stiris, T., and Andersen, J. M. (2020). Prenatal exposure to methadone or buprenorphine impairs cognitive performance in young adult rats. Drug Alcohol Dependence 212, 108008. doi:10.1016/j.drugalcdep.2020.108008
Kurosawa, K., Chiba, K., Noguchi, S., Nishimura, T., and Tomi, M. (2020). Development of a pharmacokinetic model of transplacental transfer of metformin to predict in vivo fetal exposure. Drug Metab. Dispos. 48, 1293–1302. doi:10.1124/dmd.120.000127
Lundquist, S., Renftel, M., Brillault, J., Fenart, L., Cecchelli, R., and Dehouck, M-P. (2002). Prediction of drug transport through the blood-brain barrier in vivo: A comparison between two in vitro cell models. Pharm. Res. 19, 976–981. doi:10.1023/A:1016462205267
Malek, A., Obrist, C., Wenzinger, S., and von Mandach, U. (2009). The impact of cocaine and heroin on the placental transfer of methadone. Reproductive Biol. Endocrinol. 7, 61. doi:10.1186/1477-7827-7-61
Malpas, T. j., Darlow, B. a., Lennox, R., and Horwood, L. j. (1995). Maternal methadone dosage and neonatal withdrawal. Aust. N. Z. J. Obstetrics Gynaecol. 35, 175–177. doi:10.1111/j.1479-828X.1995.tb01863.x
Mortensen, N. P., Caffaro, M. M., Snyder, R. W., Yueh, Y. L., and Fennell, T. R. (2019). Placental trophoblast transfer of opioids following exposures to individual or mixtures of opioids in vitro. Exp. Biol. Med. (Maywood) 244, 846–849. doi:10.1177/1535370219851109
Myllynen, P., and Vähäkangas, K. (2013). Placental transfer and metabolism: An overview of the experimental models utilizing human placental tissue. Toxicol. Vitro 27, 507–512. doi:10.1016/j.tiv.2012.08.027
Nanovskaya, T., Nekhayeva, I., Karunaratne, N., Audus, K., Hankins, G. D. V., and Ahmed, M. S. (2005). Role of P-glycoprotein in transplacental transfer of methadone. Biochem. Pharmacol. 69, 1869–1878. doi:10.1016/j.bcp.2005.03.030
Nanovskaya, T. N., Nekhayeva, I. A., Hankins, G. D. V., and Ahmed, M. S. (2008). Transfer of methadone across the dually perfused preterm human placental lobule. Am. J. Obstetrics Gynecol. 198, 126.e1–e4. doi:10.1016/j.ajog.2007.06.073
Ushigome, F., Takanaga, H., Matsuo, H., Yanai, S., Tsukimori, K., Nakano, H., et al. (2000). Human placental transport of vinblastine, vincristine, digoxin and progesterone: Contribution of P-glycoprotein. Eur. J. Pharmacol. 408, 1–10. doi:10.1016/S0014-2999(00)00743-3
Wolff, K., Boys, A., Rostami-Hodjegan, A., Hay, A., and Raistrick, D. (2005). Changes to methadone clearance during pregnancy. Eur. J. Clin. Pharmacol. 61, 763–768. doi:10.1007/s00228-005-0035-5
World Health Organization (2014). Guidelines for the identification and management of substance use and substance use disorders in pregnancy.
Keywords: methadone, opioids, PBPK, P-glycoprotein, model
Citation: Ho H and Zhang E (2023) P-glycoprotein efflux transporter: a key to pharmacokinetic modeling for methadone clearance in fetuses. Front. Pharmacol. 14:1182571. doi: 10.3389/fphar.2023.1182571
Received: 10 March 2023; Accepted: 18 April 2023;
Published: 04 May 2023.
Edited by:
Elena Ramírez, University Hospital La Paz, SpainReviewed by:
Tomohiro Nishimura, Keio University, JapanCopyright © 2023 Ho and Zhang. This is an open-access article distributed under the terms of the Creative Commons Attribution License (CC BY). The use, distribution or reproduction in other forums is permitted, provided the original author(s) and the copyright owner(s) are credited and that the original publication in this journal is cited, in accordance with accepted academic practice. No use, distribution or reproduction is permitted which does not comply with these terms.
*Correspondence: Harvey Ho, aGFydmV5LmhvQGF1Y2tsYW5kLmFjLm56; En Zhang, WmhhbmdlbkBjcWlmZGMub3JnLmNu
Disclaimer: All claims expressed in this article are solely those of the authors and do not necessarily represent those of their affiliated organizations, or those of the publisher, the editors and the reviewers. Any product that may be evaluated in this article or claim that may be made by its manufacturer is not guaranteed or endorsed by the publisher.
Research integrity at Frontiers
Learn more about the work of our research integrity team to safeguard the quality of each article we publish.