- 1Center for Plastic and Reconstructive Surgery, Department of Orthopedics, Zhejiang Provincial People’s Hospital, Affiliated People’s Hospital Hangzhou Medical College, Hangzhou, China
- 2The Second School of Clinical Medicine, Zhejiang Chinese Medical University, Hangzhou, China
- 3Department of Orthopedics, Zhejiang Provincial People’s Hospital Bijie Hospital, Bijie, Guizhou, China
Intervertebral disc degeneration (IVDD) refers to the aging and degenerative diseases of intervertebral disc components such as nucleus pulposus, annulus fibrosus, and cartilage endplate, and is the main cause of chronic low back pain. Over the past few years, many researchers around the world concerned that the degeneration of nucleus pulposus (NP) cells plays the main role in IVDD. The degeneration of NP cells is caused by a series of pathological processes, including oxidative stress, inflammatory response, apoptosis, abnormal proliferation, and autophagy. Interestingly, many studies have found a close relationship between the senescence of NP cells and the progression of NP degeneration. The classical aging pathways also have been confirmed to be involved in the pathological process of IVDD. Moreover, several anti-aging drugs have been used to treat IVDD by inhibiting NP cells senescence, such as proanthocyanidins, resveratrol and bone morphogenetic protein 2. Therefore, this article will systematically list and discuss aging, cell senescence, the pathogenesis and targeted therapies of IVDD, in order to provide new ideas for the treatment of IVDD in the future.
1 Introduction
Low back pain (LBP) is one of the most common clinical health problems, experienced by people of all ages, and as the number one cause of disability worldwide, it imposes significant healthcare costs on society (Hoy et al., 2010; Vos et al., 2015; Maher et al., 2017; Clark and Horton, 2018; Hartvigsen et al., 2018). As the most important cause of LBP, intervertebral disc degeneration (IVDD) refers to the pathophysiological process of the progressive structural failure of intervertebral disc (IVD) (Adams and Roughley, 2006; Zhang et al., 2021c). The pathogenesis of IVDD is complex and mainly includes aging, inflammation, oxidative stress, mechanical stress and genetic factors (Sharma, 2018; Li et al., 2020; Wen et al., 2022; Xin et al., 2022). All of these factors promote degradation of the IVD extracellular matrix (ECM), leading to dysfunction and structural damage of the IVD (Risbud and Shapiro, 2014; Wang D. et al., 2020; Zhang G. Z. et al., 2021). Nevertheless, the mechanisms of IVDD are still not well established. An increasing number of researchers are dedicated to clarify the pathogenesis of IDD.
The IVD consists of mainly three parts: the nucleus pulposus (NP), annulus fibrosus (AF), and two cartilaginous endplates (CEP) (Clouet et al., 2009). The NP consists of NP cells and ECM, which mainly includes type II collagen (Col II) and proteoglycans (Pattappa et al., 2012; Rustenburg et al., 2018). The dynamic balance of ECM composition and content determines the structural and functional integrity of the NP(Xin et al., 2022). ECM catabolic enzymes show high expression in degenerating NP cells, such as matrix metalloproteinases (MMPs) and a disintegrin and metalloprotease with thrombospondin motifs (ADAMTS), ultimately leading to ECM degradation (Nagase and Kashiwagi, 2003; Wang et al., 2015; Sun et al., 2022). Therefore, we should pay attention to the dynamic balance of ECM anabolism and catabolism, which is the key to the treatment of IVDD.
Studies have found that the prevalence of IVDD increases dramatically with age, with a 50% difference in the prevalence of intervertebral disc disease between people in their 50s and 70s (Miller et al., 1988). Histological studies have shown that IVD cells begin to senesce as early as the second decade of life (Dowdell et al., 2017). New evidence from clinical and animal model studies suggests that senescent IVD Cells, particularly senescent NP Cells, accumulate in aging and degenerating IVD, which is considered a new hallmark and major cause of IVDD (Dowdell et al., 2017; Zhang K. et al., 2021; Sun et al., 2021). The role of senescent NP Cells in IVDD has become clearer in recent years, and its related literature is growing. The causative mechanisms of aging as a major cause of IVDD deserve to be explored and studied.
Cellular senescence is characterized by irreversible cell cycle arrest and senescence-associated secretory phenotype (SASP). Senescent cells (SCs) with SASP secrete numerous factors such as pro-inflammatory cytokines and chemokines, growth regulators, angiogenic factors, and MMP(Patil et al., 2019b; Gorgoulis et al., 2019). In studies over the past few years, it has been found that SASP produced by SCs in IVDCs can disrupt the metabolic balance in the ECM(Wang and Tissenbaum, 2006; Wu Y. et al., 2022). It was shown that the expression levels of ECM proteases (MMP-13, ADAMTS-4 and ADAMTS-5) were significantly increased in aging IVD, along with degradation of more Col II and proteoglycan (Wang and Tissenbaum, 2006; Bedore et al., 2014). Therefore, it is important to know the specific pathways of how aging occurs in IVD Cells, and to provide ideas for targeted therapies for IVDD. Currently recognized classical molecular signaling pathways of cellular senescence include mTOR, AMPK, NF-κB, Sirtuins, P16, and p53 (Rayess et al., 2012; Osorio et al., 2016; Van de Ven et al., 2017; Ou and Schumacher, 2018; Stallone et al., 2019; Ge et al., 2022). Based on the above-mentioned signaling pathways, there have been many studies investigating the effects of cellular senescence in IVDD (Zhang et al., 2019; Che et al., 2020; Yurube et al., 2020; Shao et al., 2021). In addition, anti-aging drugs have been studied for the treatment of IVDD, such as proanthocyanidins, resveratrol, and bone morphogenetic protein 2 (Tan et al., 2019; Chen H.-W. et al., 2022; Liu et al., 2022).
This review provides an overview of the relationship between aging in IVD Cells and IVDD, summarizes the research progress of some classical pathways of aging in IVDD and strategies for anti-cellular aging, and discusses the future direction of clinical application.
2 Mechanism of intervertebral disc degeneration
The IVD consists of three layers: AF, central NP and CEP (Colombini et al., 2008). AF is mainly used to protect the NP from freeing from the IVD when the spine is under high load. In IVD, nutrition penetrates into the NP through the CEP, so the NP, as a tissue lacking blood vessels and nerves, is very slow and difficult to repair after injury (Sampara et al., 2018). Although the NP Cells account for only 1% of the volume of the IVD, they play the most important role in the physiology and biomechanics of the IVD (Vo et al., 2016; Lu et al., 2020). NP Cells regulate the metabolism of ECM and together constitute NP. The ECM of the NP consists mainly of Col II, and proteoglycans, which are used to counteract and transmit the axial pressure of the spine during loading (Chen et al., 2020; Xi et al., 2020). ECM of NP is important to maintain the structural and functional integrity of the IVD and to help prevent IVDD (Qiu et al., 2019). Studies have shown that the imbalance between ECM anabolism and catabolism play an important role in IVDD, ultimately leading to the loss of proteoglycans and hydration (Sampara et al., 2018).
IVDD, a common and complex disease, is associated with multiple pathological factors. Several studies have shown that multiple pathological factors such as inflammation, oxidative stress, mitochondrial dysfunction, and abnormal mechanical loading are involved in the development of IVDD (Feng et al., 2016; Ao et al., 2019; Chen et al., 2020; Zhang et al., 2020). These pathological factors lead to the degradation of ECM through various pathways and finally cause IVDD (Figure 1). Studies have demonstrated that inflammatory mediators such as IL-1β disrupt the metabolic homeostasis of the ECM(Wang L. et al., 2020). In recent years, there is increasing evidence that mitochondrial dysfunction increases the production of reactive oxygen species (ROS), and that excess ROS activate IVDD by regulating matrix metabolism, pro-inflammatory phenotype, autophagy, senescence, and apoptosis of IVDC (Davalli et al., 2016; Feng et al., 2017b; Kang et al., 2020; Cao et al., 2022). Studies have demonstrated that when the IVD is subjected to abnormal mechanical stress, it promotes the degradation of ECM by IVDCs and the development of IVDD (Vergroesen et al., 2015).
3 Aging, cell senescence
3.1 Cellular senescence
Aging is a process in which the tissues and organs of an organism undergo irreversible functional decline and weakening of the regulation of internal environmental homeostasis as they age, and gradually tend to die (Kennedy et al., 2014; Fulop et al., 2019). Hayflick et al. found that human fibroblasts exhibit a limited ability to divide cells before entering irreversible growth arrest, known as replicating aging. Thus they hypothesized that the gradual loss of cell proliferation eventually leads to tissue aging (Hayflick and Moorhead, 1961). Cellular senescence is a state of irreversible cell cycle arrest in cells driven by continuous exogenous and endogenous stress and injury (Wu Y. et al., 2022) (Figure 2 A). SCs, a product of cellular aging process, have special biological characteristics and functions, and are one of the important causes of impaired tissue regeneration, chronic aging-related diseases and organismal aging. There is no single marker with absolute specificity for senescent cells. The combination of cytoplasmic (e.g., senescence-associated β-Galactosidase (SA-β-Gal), lipofuscin), nuclear (e.g., p16INK4A, p21WAF1/Cip1, Ki-67) is now commonly used for the detection of SCs(Childs et al., 2015; Wu Y. et al., 2022). Currently, microscopic imaging is the method of choice for detecting aging. In addition, single-cell assays, such as immunostaining, in situ hybridization, and multicolor (imaging) flow cytometry, are also used to detect SCs(Gorgoulis et al., 2019). A quantitative study of SCs by Anat Biran et al. found significantly more SCs in older mice than in younger mice (Biran et al., 2017). The accumulation of SCs with age has a negative impact since the immune system fails to completely remove them (Kowald et al., 2020). Short-term cellular senescence is essentially harmless because the immune system will completely remove it, however long-term cellular senescence can lead to inflammation and disease (Herranz and Gil, 2018). In addition to irreversible cell cycle arrest, changes in chromatin, gene expression, organelles and cell morphology are also characteristic of cellular senescence (Gorgoulis et al., 2019). Meanwhile the extrinsic activity of SCs is extensively associated with the activation of SASP, which enhances the effects of intrinsic cell proliferation arrest and leads to impaired tissue regeneration, age-related chronic diseases and organismal aging (Di Micco et al., 2021).
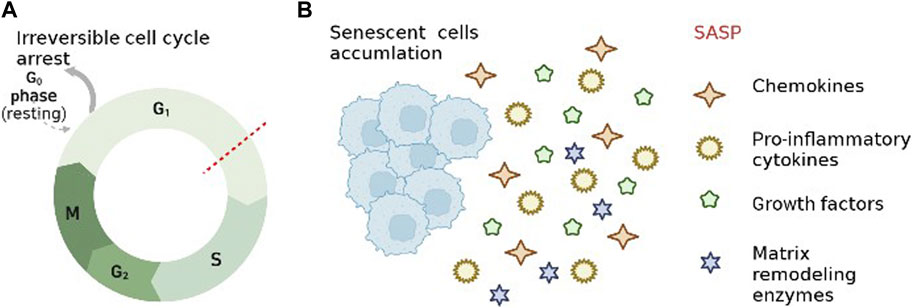
FIGURE 2. Characteristics of cellular senescence. (A). Irreversible cell cycle arrest. (B). senescence-associated secretory phenotype.
3.2 SASP
As another feature of cellular senescence, SASP refers to the state of senescent cells that secrete different chemokines, pro-inflammatory cytokines, growth factors and matrix remodeling enzymes (Shelton et al., 1999; Rodier et al., 2009) (Figure 2B). SASP-related proteins, such as TNF-α, IL-6, MMPs, monocyte chemotactic protein-1 and IGF binding proteins, increase over time with aging in multiple tissues (Freund et al., 2010). It has been shown that the secretion of SCs is constantly changing during the development of cellular senescence because SASP is regulated at multiple levels (transcription, translation, and secretion) (Hoare et al., 2016; Malaquin et al., 2016). Furthermore SASP induces neighboring cells to senesce and amplify SASP by autocrine and paracrine means (Herranz and Gil, 2018; Patil et al., 2018). The inflammatory microenvironment generated by SASP via autocrine and paracrine secretion has been identified as a key step in the development of age-related diseases, such as IVDD, osteoarthritis, Osteoporosis (Birch et al., 2015; Feng et al., 2016; Jeon et al., 2017). The inflammatory mediators of SASP are powerful drivers of tumor progression, and early studies have shown that SASP can increase tumor angiogenesis and promote malignant cell proliferation and metastasis (Krtolica et al., 2001; Coppé et al., 2006). SASP is a double-edged sword in specific cases (Herranz and Gil, 2018). On the one hand, it accelerates cellular senescence through paracrine secretion (inducing non-malignantly proliferating neighboring cells to undergo senescence), and on the other hand, it can reinforce senescent growth arrest through autocrine secretion, such as suppression of senescent tumors (Hubackova et al., 2012; Nelson et al., 2012; Acosta et al., 2013).
4 Effects of senescent cells on intervertebral disc cells
To date, IVDD is known to be driven by loss of IVD Cells, anabolic factor deficiency, and activation of matrix degrading enzymes (Risbud and Shapiro, 2014; Wang et al., 2016). The proportion of senescent NP Cells was significantly increased in degenerated IVDs, and the lifespan of senescent NP Cells was shorter compared to normal NP Cells (Jeong et al., 2014). Compared to younger patients, NP samples from older IVDD patients showed a higher proportion of senescent NP Cells, higher p53 and p21 expression, higher SA-β-Gal activity and lower telomere length (Kim et al., 2009). Senescent NP Cells in SASP secrete a variety of substances including cytokines, chemokines, growth factors and ECM proteases, among which ECM proteases (MMPs and ADAMTS) cause excessive breakdown of ECM and the microenvironment of NP, thus greatly contributing to the development of IVDD (Wang and Tissenbaum, 2006; Bedore et al., 2014). Therefore, it is particularly crucial to investigate the pathological factors that lead to NP Cells senescence.
4.1 Etiology of nucleus pulposus cellular senescence
There are many causes of cellular senescence, and we summarize the most important pathological factors that lead to NP Cells senescence. (Figure 3).
4.1.1 Inflammation
Chronic inflammation, considered as a sign of aging, is a risk factor for age-related diseases (Franceschi et al., 2000; López-Otín et al., 2013; Ferrucci and Fabbri, 2018). During IVDD pathology, NP Cells produce excess inflammatory factors (such as IL-1β and TNF-α) that trigger the subsequent pathogenic process of aging (Le Maitre et al., 2005; Le Maitre et al., 2007).
D Purmerrur et al. performed SA-β-Gal staining and qPCR assay to evaluate the senescence and ECM homeostasis (Purmessur et al., 2013). The data showed that the proportion of positive SA-β-Gal stained cells was significantly greater in the TNF-α group than in the control group, moreover, the gene expression of aggrecan, type Ⅰ collagen (Col Ⅰ), and Col Ⅱ was significantly downregulated in the NP. Therefore, the author hypothesized that TNF-α may induce senescence and disrupt the microenvironmental homeostasis of IVD Cells through some mechanism. In another study, the authors explored the differences between senescence and puncture-induced IVDD models in rats (He et al., 2022). The relationship between inflammation and senescence in NP Cells was studied and evaluated by immunohistochemical detection of changes in ECM (Col I, Col II) and detection of inflammation-related indicators (TNF-α). Immunohistological results showed a higher percentage of TNF-α positive cells in the senescent-group compared to the punctured-group. The investigators used alcian blue and safranin o staining to observe the composition and content of ECM in IVD. The histological results revealed a decrease in the stained area of IVD in both the senescent and punctured groups, and the punctured group had the smallest stained area of IVD. Aging-induced and puncture-induced IVDD manifestations were different, with chronic inflammatory indicators rising in the senescent group and the punctured group suffering from severe ECM loss. TNF-α induced inflammation plays a great role in senescence of NP Cells and ECM homeostasis. Kang et al. used TNF-α and IL-18 in mouse NP to mimic the inflammatory environment and construct an IVDD model (Li et al., 2019). They found a higher proportion of NP Cells senescence and senescence markers (P16 and p53) in treated NP. The investigators also used the CCK-8 assay to assess cell proliferation and found that TNF-α-induced NP Cells had significantly lower proliferative capacity. Moreover, the result of flow cytometry of NP Cells revealed a significant increase in the G0/G1 phase and a significant decrease in the S phase. These data mean inflammatory factors induce senescence in NP Cells, as evidenced by reduced cell proliferation capacity and cell cycle arrest.
The specific pathogenesis of obesity-induced IVDD has been under investigation. Interestingly, Sunli Hu et al. isolated NP tissue from patients with different levels of IVDD and evaluated the proportion of senescent NP Cells and the amount of chemerin in the NP(Hu et al., 2020). They found that the levels of senescent NP Cells and Chemerin increased significantly with increasing degree of IVDD. Thus, Hu et al. speculated that aging of NP Cells may be associated with chemerin. Several previous studies have shown that the NF-kB pathway plays an important role in obesity-related inflammation. Therefore, Hu detected the expression levels of p65 and AKT in chemerin induced senescent NP Cells. Western blotting showed that chemerin stimulation significantly increased the expression levels of p65 and AKT. And the investigators found that the use of Lv-shTLR4 reversed chemerin-induced activation of the NF-κB signaling pathway, and Lv-shCMKLR1 reduced AKT phosphorylation and SA-β-Gal-positive NP Cells. Therefore, the authors concluded that chemerin activates the NF-κB signaling pathway through its receptors CMKLR1 and TLR4, releasing inflammatory mediators, leading to cellular senescence.
Apart from this, according to previous studies, other pro-inflammatory mediators, including, IL-2, IL-4, IL-8, IL-10, IL-17, IFN-γ, chemokines and prostaglandin 2 promote aging of NP Cells, which leads to the development of IVDD (Wuertz and Haglund, 2013; Risbud and Shapiro, 2014; Ohtori et al., 2015). However, their role of IVDD still needs to be explored.
4.1.2 Mitochondrial dysfunction and oxidative stress
Mitochondria, an evolutionary product of aerobic bacterial invasion of eukaryotic cells a billion years ago, have a separate genome for the synthesis of ATP. As a complex organelle, mitochondria are not only energy workstations, but also regulate cellular senescence (Zhang et al., 2022). The most classical mitochondria-induced senescence-related pathways available are P38/MAPK, P16-RPB and P53 (Van Deursen, 2014). Subsequently, more and more researchers identified mitochondrial dysfunction as being associated with NP Cells cellular senescence. A study by Xiaolong Xu et al. investigated the correlation between senescence and mitochondrial dysfunction in NP Cells (Xu et al., 2019). Progerin, a truncated unprocessed lamin A protein, causes tissue aging and degeneration. Xu found that progerin levels increased with IVDD progression in human and rats NP tissue. Researchers infected rat NP Cells with mCherry-progerin-expressing or vector-only-expressing lentiviruses. They found that progerin expression decreased the levels of the nuclear structure-associated proteins lamin B1 and lamina-associated polypeptide2, and the extent of lysine 27 trimethylation on heterochromatin-associated histone 3, but increased the expression of serine-139 phosphorylated H2AX and the proportion of SA-β-Gal-positive cells. At the same time, the progerin group exhibited elevated ROS levels, disruption of mitochondrial membrane potential, decreased ATP production, and reduced activity of mitochondrial complex enzymes compared to the vector control group. Overall, these data clearly indicate that upregulation of progerin expression in NP cells induces severe oxidative stress and mitochondrial dysfunction, triggering senescence-related defects and apoptosis in vitro. To explore the link between progerin overexpression and IVDD progression, Xu et al. constructed LmnaG609G knock-in mice (progerin overaccumulation). And mouse tissue samples were analyzed histologically and levels of progerin were assessed using Western blotting. Mitochondrial function was measured by analyzing mitochondrial membrane potential and ATP levels. The results showed that excessive progerin expression in vivo induced NP senescence and mitochondrial dysfunction. Overexpression of progerin in NP Cells induces mitochondrial dysfunction, leading to cellular senescence.
ROS accumulate in large amounts as a byproduct of ATP synthesis due to mitochondrial dysfunction. The large accumulation of ROS in NP Cells can also leads to senescence (Feng et al., 2017a). Wang et al. showed that oxidative stress can induce mitochondrial dysfunction and exacerbate the aging of NP Cells (Wang Y. et al., 2018). Phosphatase and Tensin homolog-induced putative kinase protein 1 is a mitochondria-targeted serine/threonine kinase that protects against mitochondrial dysfunction and mitochondrial quality control by activating PINK1/Parkin-mediated mitophagy. The investigators induced oxidative stress in human NP Cells by H2O2. And the senescence of NP Cells was detected after targeted silencing of PINK1 by short hairpin RNA. It was found that downregulation of PINK1 in H2O2-treated NP Cells resulted in a significant increase in the proportion of senescent NP Cells. PINK1 plays a protective role in the clearance of damaged mitochondria and mitigation of NP Cells senescence under oxidative stress by a mechanism related to the regulation of mitochondrial autophagy. Liang et al. conducted a similar study and further confirmed that oxidative stress promotes aging in NP Cells (Kang et al., 2019). The investigators used tert-butyl hydroperoxide (TBHP) to induce oxidative stress and mitochondrial dysfunction in rat NP Cells. The results show TBHP increase the levels of Bax, cleaved caspase-3, cleaved caspase-9 and cytoplasmic cytochrome c and decrease the levels of Bcl-2 and mitochondrial cytochrome c in NP Cells. However, curcumin pretreatment attenuated these changes and reduced the proportion of senescence in NP Cells. Moreover, Liang also found higher phosphorylation level of AMPK and ULK1 and lower phosphorylation of mTOR in the western blotting results. All these data suggest that the AMPK/mTOR/ULK1 pathway is involved in oxidative stress related NP cells senescence.
4.1.3 Mechanical stress
Abnormal mechanical stress is an important risk factor for IVDD, and cellular senescence is a pathological alteration of IVDD. However, the molecular mechanisms of mechanical stress-induced cellular senescence have not been fully elucidated.
In a study addressing mechanical stress and aging of NP Cells, Qiujuan Xing et al. constructed models of abnormal stress by performing forelimb amputations on mice (Xing et al., 2010). Quantitative immunohistochemical analysis showed that the mRNA levels of p16INK4a, RB, PTEN, p27KIP, p19ARF and RAGE were upregulated, and the mRNA of p21 was significantly downregulated. The highest levels of SA-β-GaL activity and significant increased cell cycle protein D1 mRNA levels were detected in rats with 9 months of amputation. The researchers hypothesized that the abnormal stress caused by amputation affected the function of cell cycle regulatory proteins and accelerated the aging of NP Cells. In another study, Yiyang Wang et al. found that overload mechanical compression induces oxidative stress, mitochondrial dysfunction and aging in human NP Cells (Wang et al., 2021). The investigators explored the association between mechanical stress and aging of NP Cells by providing different mechanical compressive loads to NP Cells. Mitochondrial phagocytosis can reduce oxidative stress-induced senescence in NP Cells by recycling damaged mitochondria. Western blotting results showed that high pressure loading treatments downregulated the expression of PINK1 and LC3II/I and upregulated the expression of Parkin and P62. At the same time, mitochondria showed shrinkage and enhanced membrane density in NP Cells treated with high pressure loading. All of these indicate that high pressure loading leads to impaired mitochondrial phagocytosis. Moreover, the proportion of senescence in NP Cells was significantly increased under high pressure. High mechanical load accelerates aging of NP Cells by inhibiting mitochondrial function and increasing oxidative stress.
Periosteal proteins and the associated classical pathway (NF-κB) are previously known to play a very critical role in stress-related cellular pathology. Wu et al. explored the mechanism of the role of periosteal proteins and pathways in mechanical stress-induced NP Cells senescence (Wu J. et al., 2022). They detected the expression of periostin and NF-κB/p65 in mechanically stressed-induced senescent NP Cells, and the results suggested that it is not only transcriptionally upregulated by NF-κB/p65, but also promotes catabolism in NP Cells by activating NF-κB. Therefore, the authors suggest that abnormal stress can regulate the aging process of NP Cells through periosteal proteins and NF-κB positive and negative feedback pathways.
What’s more, the Piezo1 ion channel in IVD Cells senses changes in mechanical stress and converts mechanical signals into chemical signals, which can also alert the process of IVDD (Zhao et al., 2018). Sheng et al. evaluated the role of Piezo1 in mechanical stress-mediated IVDD (Shi et al., 2022). Immunofluorescence staining results showed increased gene and protein levels of Piezo1 after high-intensity stress. In addition, qPCR and protein blotting results showed that high-intensity stress treatment also amplified the expression of classical senescence markers p53 and p16INK4a in NP Cells. Meanwhile, the investigators found that high-intensity stress not only increased the expression of inflammatory cytokines TNF-α, IL-6 and IL-1β, but also induced mitochondrial dysfunction by decreasing mitochondrial membrane potential and oxygen consumption rate in a time-dependent manner. Excessive mechanical stress increases pro-inflammatory cytokines by upregulating piezo1, which induces mitochondrial dysfunction and thus triggers aging of human NP Cells by inhibiting autophagy. Since Piezo1 is a non-selective cation channel and many studies have demonstrated that Ca2+ entry into Piezo1 promotes pro-inflammatory cytokine secretion and mitochondrial dysfunction, the authors speculate that Ca2+ influx plays a key role in Piezo1 signaling (Blythe et al., 2019; Yang et al., 2019).
4.1.4 DNA damage
There are many mechanisms regarding DNA damage leading to aging, including through activation of signaling responses that block transcription and other DNA metabolism (Fagagna et al., 2003; Vermeij et al., 2016). DNA damage, as one of macromolecular damage, causes cells to undergo senescence is time-dependent accumulation (Yousefzadeh et al., 2021). Accumulated genomic and mitochondrial DNA damage causes stress-induced premature senescence (SIPS) in cells (Vo et al., 2016).
In IVDD, DNA damage is also involved in the aging process of NP Cells. It was found that DNA damage in IVD can promote aging by blocking the cell cycle and reducing the ability of cells to proliferate (Dimozi et al., 2015). The investigators used subcytotoxic concentrations of hydrogen peroxide (H2O2) to simulate oxidative stress and construct a model of DNA damage in NP Cells. Immunohistological results showed elevated expression levels of p53, p16INK4a and p21WAF1 in H2O2-induced senescent NP Cells, and pRb showed hypophosphorylation. Flow cytometry and SA-β-Gal staining results show that DNA-damaged NP Cells exhibit cell cycle arrest and an increased proportion of senescence. DNA damage leads to delayed G1 cell cycle and reduced cell proliferation through activation of the ATM-Chk2-p53-p21WAF1-pRb pathway. Nasto et al. found that rat with DNA defects were more susceptible to senescence of NP Cells and IVDD (Nasto et al., 2013). Researchers induced DNA damage with subtoxic doses of the chemotherapeutic agent mechlorethamine in wild-type and DNA repair-deficient Ercc1-/Δ mice. They found that mechlorethamine, an anticancer chemotherapeutic agent, accelerated the loss of ECM in IVD and greatly enhanced cellular senescence and apoptosis. And this effect was more pronounced in DNA repair-deficient Ercc1-/Δ mice than in Wt mice. These findings provide strong evidence that DNA damage can drive degenerative changes associated with IVDD even in normal hosts.
5 Anti-cellular senescence strategies
5.1 Reduction of senescent cells formation
To address the above triggering factors of aging in NP Cells, the following will summarize the therapies to relieve aging in NP Cells through four aspects, such as attenuating the inflammatory response, resisting oxidative stress and preventing mechanical stress, inhibiting DNA damage.
5.1.1 Reduced inflammatory response
Inflammation as a driver of aging induction in NP Cells, current anti-inflammatory therapeutic studies in IVDD focus on inhibition of inflammatory pathways (e.g., NF-κB) and downregulation of inflammatory factors and enzymes (e.g., IL-1β, TNF-α).
NF-κB, a classical pathway of aging in NP Cells, can be used as a target for IVDD aging therapy. Huang et al. used a cationic polymer brush-coated carbon nanotube siRNA delivery platform to transduce LINC02569 siRNA to NP Cells and found that it significantly attenuated the IL-1β-induced inflammatory response and delayed the aging of NP Cells by blocking the NF-κB signaling pathway (Huang Y. et al., 2022). Xiaoliang Bai et al. assessed the role of higenamine in IL-1β-induced inflammation in human senescent NP Cells (Bai et al., 2019). The investigators isolated NP Cells from the IVD of IVDD patients and induced a mock inflammatory environment with IL-1β. By examination, higenamine was found to increase the cell viability of IL-1β-induced senescent NP Cells. Higenamine significantly attenuated the increased production of ECM degrading enzymes, as well as the IL-1β-induced production of a series of inflammatory factors such as nitric oxide synthase, nitric oxide, Prostaglandin E2, cyclooxygenase-2, TNF-α, and IL-2. Moreover, they examined the expression of IκBα, NF-κB, p65 and p-p65 using western blot. The results showed that higenamine attenuated IL-1β-induced p65 expression and NF-κB degradation in NP Cells. This indicates that higenamine also inhibits IL-1β-induced activation of the NF-κB signaling pathway in NP Cells, which is useful for inhibiting aging in NP Cells.
In Xin Huang’s study, IL-1β was used to mimic the inflammatory environment in IVDD, and it was found that the addition of Omentin-1 improved IL-1β-induced senescence in NP Cells, G1 phase cell cycle arrest and reduced ECM synthesis in NP Cells (Huang X. et al., 2022). These findings suggest that Omentin-1 plays an important function in protecting NP Cells against senescence and has the potential for IVDD gene targeting therapy. Li et al. explored the role of resveratrol in inflammation-induced senescence of NP Cells (Li et al., 2019). It was found that resveratrol partially reversed the effects of inflammatory cytokines (e.g., IL-1β, TNF-α) on the senescence of NP Cells, such as increased SA-β-Gal activity and ROS content, promotion of G0/1 cell cycle arrest, and gene/protein expression were upregulated. This suggests that resveratrol can effectively inhibit cellular senescence in an inflammatory environment. Qianchen Zhi et al. found that Dehydrolactone (DHE) partially attenuated TNF-α-induced ECM degradation and senescence of NP Cells (Chen et al., 2021). DHE is a natural sesquiterpene lactone isolated from medicinal plants with anti-inflammatory properties. Firstly, they investigated the role of DHE in TNF-α-induced activation of inflammatory signaling pathways and cellular senescence in vitro. Western blotting and SA-β-Gal staining showed that DHE inhibited the activation of NF-κB and MAPK inflammatory signaling pathways and ameliorated TNF-α-induced activation of STING-TBK1/NF-κB signaling-induced senescence in NP Cells. Subsequently, they measured the therapeutic effect of DHE in a mouse model of lumbar instability and found that DHE significantly attenuated the structural damage of the IVD, as evidenced by a significant reduction in the expression of TNF-α and IL-1β and the degradation of aggregated glycans within the IVD. The ability of DHE to alleviate TNF-α-induced ECM degradation and aging of NP Cells suggests that DHE is very promising for the treatment of IVDD.
5.1.2 Resistance to oxidative stress
Oxidative stress as a risk factor for causing IVDD and aging inducing factor for NP Cells, therefore anti-aging treatment for oxidative stress in IVDD is necessary.
Shuo Zhang et al. found that heat shock protein 70 (HSP70) has a role in alleviating the aging of NP Cells in IVDD (Zhang et al., 2021d). They treated NP Cells with TBHP in vitro to mimic oxidative stress in NP Cells and used the HSP70 inducer TRC051384 to assess the cytoprotective effect of HSP70. SA-β-Gal staining and protein blotting analysis showed that HSP70 inhibited p53/p21-mediated senescence in NP Cells. And western blotting results showed that HSP70 could downregulate the JNK/c-Jun pathway (a pro-apoptotic and pro-inflammatory pathway). These indicate HSP70 impeded oxidative stress-induced senescence in human NP Cells by inhibiting the JNK/c-Jun pathway. Recently, goldenseal glycosides were found to inhibit TBHP-induced senescence and mitochondrial dysfunction in NP Cells by activating Nrf2, thereby inhibiting the deleterious effects induced by oxidative stress and enhancing the antioxidant capacity of NP Cells (Wang et al., 2019). In a similar vein, apigenin was found to protect NP Cells against TBHP-induced senescence and ECM degradation (Xie et al., 2022). Apigenin promotes nuclear translocation of transcription factor EB via AMPK/mTOR signaling pathway to alleviate TBHP-induced disruption of autophagosome-lysosome fusion and lysosome dysfunction, thereby protecting cells from oxidative stress (Liang et al., 2021; Xie et al., 2022).
SIRT1 has been shown to regulate cellular oxidative stress and can modulate the expression of p53 and p16 through deacetylation in NP Cells to mitigate the progression of aging (He et al., 2019). He et al. investigated the role and mechanism of action of SIRT1 in oxidative stress-induced senescence in rat NP Cells (He et al., 2019). The investigators used sublethal concentrations of H2O2 to induce senescence in rat NP Cells, and SRT1720 to activate SIRT1. Simultaneously, FoxO1 and Akt were inhibited by AS1842856 and MK-2206, respectively, to explore the role of the Akt-FoxO1-SIRT1 axis in rat NP Cells. Fluorescence quantitative PCR analysis showed that the protein expression of SIRT1 was gradually downregulated with increasing H2O2 concentration. Immunofluorescence revealed that the PI3K/Akt pathway was activated during this oxidative stress, and the activated phosphorylated Akt (Ser473) exerted post-translational modifications at the Ser1 locus and promoted FoxO1 phosphorylation. Activation of SIRT1 using SRT1720 showed downregulation reflecting senescence-related proteins (p53, p21, p16 and p-Rb), decreased expression of pro-inflammatory cytokines (TNF-α, IL-1β, IL-6 and IL-8), reduced G0/G1 phase arrest, and reduced proliferation and SA-β-Gal positive cells. SIRT1 ameliorated oxidative stress-induced senescence in rat NP cells, which is regulated by the Akt-FoxO1 pathway.
Zhang et al. found that Butein can be used as a therapeutic agent for diabetic IVDD patients by delaying the aging of NP Cells (Zhang et al., 2019). The investigators performed diabetic modeling in rats by intraperitoneal injection of streptozotocin. Senescence of NP Cells was measured by SA-β-Gal staining and found to be significantly increased in a dose-dependent manner after glucose treatment, and protein blotting results showed that high concentrations of glucose significantly promoted the expression of classical senescence-associated proteins p16INK4a and p21WAF1. Also increased acetylation of P53 and decreased expression of Sirt1 were found in both in vivo NP tissues and in vitro hyperglycemic NP Cells of diabetic patients. After the use of aging inhibitors of Sirt1, protein blotting results showed that the expression of Sirt1 was decreased and the acetyl-p53/p53 ratio was increased. This is in contrast to the results of butein action, indicating that butein can improve aging of NP Cells and IVDD in diabetic rats through the Sirt1/P53 axis.
Targeting mitochondria with oxidative stress dysfunction and applying antioxidants that target damaged mitochondria can help inhibit IVDD. Jianle Wang et al. found that Polydatin (PD) was able to reduce the level of NP Cell senescence by alleviating mitochondrial dysfunction (Wang J. et al., 2018). In vitro, NP Cells were pretreated with TNF-α to induce senescence while treated with PD. The results showed that PD reduced the number of SA-β-Gal-positive cells and the expression of p53 and p16 in TNF-treated NP Cells. Meanwhile, researchers collected NP tissues for histological and immunofluorescence analysis after 4 weeks of treatment with PD after puncture induction of IVDD in rats. Increased MitoSOX fluorescence of NP Cells in the TNF-α group suggested that TNF-α could exacerbate oxidative stress in NP Cells. However, MitoSOX fluorescence was significantly reduced in the PD group, indicating that PD promotes ROS clearance in NP Cells. In addition, the investigators examined the levels of Nrf2 in the nucleus. Immunofluorescence of Nrf2 showed that PD promoted Nrf2 translocation to the nucleus in a dose-dependent manner. Taken together, PD ameliorates IVDD in a rat model by promoting Nrf2 activity and inhibiting the senescence of NP Cells. Liang Kang et al. modeled IVDD by compression and found that MitoQ could significantly inhibit mitochondrial ROS production in NP Cells (Kang et al., 2020). Song et al. reported that protection of mitochondrial redox homeostasis by restoring the function of sirtuin 3 could attenuate IVDD (Song et al., 2018).
5.1.3 Avoidance of excessive mechanical stress
Prevention of excessive mechanical stress is a promising way to slow down the process of IVDD. Actions with high IVD loads, such as torsion, bending, should be minimized or avoided (Walter et al., 2011; Chan et al., 2013). There is evidence that antibodies to periostin interrupt the PIEZO1-induced self-amplifying loop of NF-κB and periostin, delaying the production of senescent cells and may serve as potential therapeutic agents for IVDD (Wu J. et al., 2022). There is a lack of studies on the application of antibodies to periosteal proteins in IVDD models.
5.1.4 Inhibition of DNA damage
It has been demonstrated that DNA damage as a driver of IVD aging significantly accelerates IVDD in mice (Wang et al., 2012). Despite the existence of complex repair mechanisms in cells, DNA damage continues to accumulate in NP Cells over time (Vo et al., 2016). Lack of gene repair mechanisms always leads to aging in multiple organ systems, such as IVDD (Hasty et al., 2003). It was found that DNA repair-deficient Ercc1/mice had an earlier onset of IVDD compared to normal mice, as evidenced by loss of proteoglycans, increased senescent NP Cells, and reduced disc height (Vo et al., 2010; Vo et al., 2013). Application of telomeric antisense oligonucleotides to a mouse model of accelerated senescence syndrome effectively reduced DNA damage response activation, senescence marker levels and SASP induction, and improved tissue homeostasis (Di Micco et al., 2021). The effectiveness of this method, although not confirmed in IVDD models, provides a research direction for the treatment of IVDD caused by cellular senescence due to telomeric DNA damage response. Apart from this, studies of exposed genotoxic stress in humans and mice have shown that DNA damage from ionizing radiation and smoking accelerates the development of IVDD (Nasto et al., 2013). Reducing or avoiding exposure to ionizing radiation and smoking therefore help to inhibit cellular aging and IVDD.
5.2 Removal of senescent cells
As one of the main modalities of ‘senotherapeutic’, ‘senolytics’ refers to pharmaceutical agents that can eliminate SCs. The combination of dasatinib (D) and quercetin (Q) has been better studied in recent years. And studies have reported that D can remove senescent adipose stem cells and Q can kill senescent endothelial cells and osteoblasts (Chen B. et al., 2022; Wu Y. et al., 2022). The combination of the D and Q can increase the content of proteoglycans in mouse IVD and is useful for eliminating SCs in CEP (Nasto et al., 2013; Chen B. et al., 2022). Emanuel J et al. prevented or treated age-related IVDD in 6-, 14-, and 18-month-old wild-type C57BL/6 mice targeted for senescent cells with weekly injections of a combination of D and Q. Histological analysis of lumbar discs from the 6- and 14-month D + Q cohorts showed significant reductions in aging markers such as p16INK4a and p19ARF and better preservation of tissue and cellular morphology, suggesting that D + Q treatment can effectively inhibit IVDD (Novais et al., 2021). The latest study has found that curcumin and its metabolite o-vanillin, a member of the “senolytics”, have been shown to eliminate SCs in IVD and to reduce IVDD (Cherif et al., 2019; Mannarino et al., 2021). Studies have demonstrated that the cell cycle suppressor p16INK4a is essential for the induction and maintenance of IVD senescence (Liu et al., 2019). Hosni Cherif et al. validated the drug’s ability to eliminate SCs by injecting RG-7112 into the central region of intact human IVD (Cherif et al., 2020). Immunohistochemical evaluation of p16Ink4a showed a significant reduction in the number of aging NP Cells after RG7112 treatment. Patil et al. found extensive reductions in both MMP13 expression and ECM degradation in NP after elimination of transgenic p16INK4a senescent NP Cells using ganciclovir (Patil et al., 2019a). This suggests that elimination of senescent NP Cells with high expression of p16INK4a can significantly slow down the aging process and reduce IVDD.
5.3 Others
Cell cycle arrest as one of the main features of cellular senescence, therefore inhibition of cell cycle arrest in senescent NP Cells is helpful for improving IVDD. Kunkun Sheng et al. measured the cell cycle of NP cells by flow cytometry, and β-galactosidase staining was used to study the senescence of NP cells. The results showed that p-coumaric acid significantly inhibited H2O2-induced cell cycle arrest and SA-β-Gal activity in a dose-dependent manner. Taken together, p-coumaric acid attenuated H2O2-induced oxidative stress and cellular senescence, suggesting a potential therapeutic use of p-coumaric acid in IVDD.
SASP is the main way in which SCs accelerate IVDD, so SASP inhibitors can prevent the cell-extrinsic effects of SCs(Di Micco et al., 2021). P16INK4a is considered to be a major regulator of SASP, and elimination of p16INK4a is able to reduce SASP(Novais et al., 2019). Activation of cannabinoid type 2 receptors inhibits p16INK4a protein and SASP, thereby restoring ECM metabolic homeostasis and reducing IVDD (Du et al., 2022). It was shown that TWIST1/2 protein supplementation also contributes to the partial reversal of the aging phenotype of NP Cells and facilitates the intervention of IVDD (Qiu et al., 2020). Both o-vanillin and curcumin effectively inhibited the SASP of NP Cells while eliminating senescent NP Cells, thus significantly increasing the synthesis of proteoglycans and Col II in NP(Cherif et al., 2019; Cherif et al., 2020). In addition, compounds that modulate NF-κB signaling, including metformin, apigenin, kaempferol, and BAY11-7,082, have also been shown to reduce SASP production, with apigenin reversing the imbalance between ECM synthesis and degradation by promoting the expression of Col II and proteoglycan and inhibiting the expression of matrix degrading enzymes (Di Micco et al., 2021; Xie et al., 2022). In conclusion, SASP inhibitors, unlike drugs that alleviate senescence-associated proliferative arrest, are particularly important in inhibiting senescent cellular intervention in the development of IVDD.
6 Discussion
IVDD is one of the leading causes of lumbar degenerative disease and has significant socioeconomic effects. SCs accumulate with age, leading to the normal aging process as well as age-related diseases. In healthy IVDs, the balance between anabolic and catabolic processes maintains ECM homeostasis; however, aging NP Cells develop SASP, which leads to an imbalance in expression between catabolic factors (e.g., MMPs and ADAMTS) and anabolic mediators (e.g., growth factors), ultimately leading to loss of ECM homeostasis and subsequent IVDD (Zeiter et al., 2005; Zhao et al., 2007; Sowa et al., 2008). Among the imaging manifestations of degenerative IVD, the NP, as the earliest and most widely degenerated structure, has the greatest impact on IVDD with its altered ECM component. Available studies have reported that DNA damage, inflammation, oxidative stress, and mechanical stress are all predisposing factors for IVDD, and all interact with aging. However, aging as one of the main causes of induction of IVDD, the initiating factors that cause NP Cells to undergo aging are still unclear. Therefore, in future studies on the correlation between aging and IVDD, more attention can be paid to the inducing factors and loci of action that trigger aging in NP Cells. Mitochondria are the main source of energy supply for IVD Cells and a major contributor to ROS production. Therefore, the stability of mitochondrial function is very important for IVD Cells. Mitochondrial dysfunction leads to oxidative stress, cell death and premature cellular senescence, all of which are associated with IVDD (Saberi et al., 2021). Studies have shown that depletion of dysfunctional mitochondrial sirtuins triggers cellular senescence (Wiley et al., 2016). Moreover, SCs are characterized by changes in mitochondrial mass, membrane potential and mitochondrial morphology (Chapman et al., 2019). Recent studies have shown that increased oxidative stress in SCs is associated with the accumulation of dysfunctional mitochondria (Di Micco et al., 2021). Mitochondria serve as a common site of action for a variety of IVDD causative factors, therefore mitochondria may be the focus of future IVDD research. Cellular senescence is mainly characterized by irreversible cell cycle arrest and SASP, and several drugs are available to treat IVDD by eliminating SCs and inhibiting SASP. This paper lists current anti-aging therapies for IVDD by combining the aging etiology of IVD Cells in IVDD and classical pathways of aging such as mTOR, AMPK, NF-κB, Sirtuins, P16, and p53 (Table 1). Hopefully, it could provide a little help for future IVDD research.
7 Conclusion
In conclusion, in this article, we discuss the etiology of IVDD and highlight the relevant role of senescent NP Cells on IVDD. The SASP of senescent NP Cells leads to an imbalance in IVD metabolic homeostasis and subsequently causes alterations in ECM composition in NP as a major cause of IVDD, and we describe in detail the mechanism of its role in IVDD. In addition, we listed some classical pathways that cause cellular senescence and anti-aging IVDD therapies, expecting to provide some help for the future clinical diagnosis and treatment of IVDD. Meanwhile, as SCs have been shown to be associated with multiple disease spectrums, studying SCs will also have a broad impact on the treatment of multiple systemic diseases.
Author contributions
JX: Conceptualization, Methodology, Data Curation, and Writing-Original Draft; TS: Methodology and Data Curation; JL: Data Curation and Formal analysis; CX, and JZ: Supervision, Project administration, Funding acquisition, and Writing Review and Editing.
Funding
This study was supported by grants from Science Technology Department of Zhejiang Province (LGD22H060004 to JZ) and Department of Education of Zhejiang Province (Y202249216 to JZ) and Zhejiang Provincial People’s Hospital (ZRY019A002 to JZ).
Conflict of interest
The authors declare that the research was conducted in the absence of any commercial or financial relationships that could be construed as a potential conflict of interest.
Publisher’s note
All claims expressed in this article are solely those of the authors and do not necessarily represent those of their affiliated organizations, or those of the publisher, the editors and the reviewers. Any product that may be evaluated in this article, or claim that may be made by its manufacturer, is not guaranteed or endorsed by the publisher.
Abbreviations
IVDD, Intervertebral Disc Degeneration; NP, Nucleus Pulposus; LBP, Low Back Pain; IVD, Intervertebral Disc; ECM, Extracellular Matrix; AF, Annulus Fibrosus; CEP, Cartilaginous Endplate; Col II, Type II collagen; MMPs, Matrix Metalloproteinases; ADAMTS, A Disintegrin and Metalloprotease with Thrombospondin Motifs; SASP, Senescence-associated Secretory Phenotype; SCs, Senescent Cells; ROS, Reactive Oxygen Species; SA-β-Gal, Senescence-associated β-Galactosidase; Col Ⅰ, Type Ⅰ collagen; TBHP, Tert-butyl hydroperoxide; DHE, Dehydrolactone; HSP, Heat Shock Protein 70; PD, Polydatin; D, Dasatinib; Q, Quercetin; PCA, P-coumaric acid.
References
Acosta, J. C., Banito, A., Wuestefeld, T., Georgilis, A., Janich, P., Morton, J. P., et al. (2013). A complex secretory program orchestrated by the inflammasome controls paracrine senescence. Nat. Cell Biol. 15 (8), 978–990. doi:10.1038/ncb2784
Adams, M. A., and Roughley, P. J. (2006). What is intervertebral disc degeneration, and what causes it? Spine 31 (18), 2151–2161. doi:10.1097/01.brs.0000231761.73859.2c
Ao, X., Wang, L., Shao, Y., Chen, X., Zhang, J., Chu, J., et al. (2019). Development and characterization of a novel bipedal standing mouse model of intervertebral disc and facet joint degeneration. Clin. Orthop. Relat. Res. 477 (6), 1492–1504. doi:10.1097/CORR.0000000000000712
Bai, X., Ding, W., Yang, S., and Guo, X. (2019). Higenamine inhibits IL-1β-induced inflammation in human nucleus pulposus cells. Biosci. Rep. 39 (6). doi:10.1042/bsr20190857
Bedore, J., Leask, A., and Séguin, C. A. (2014). Targeting the extracellular matrix: Matricellular proteins regulate cell–extracellular matrix communication within distinct niches of the intervertebral disc. Matrix Biol. 37, 124–130. doi:10.1016/j.matbio.2014.05.005
Biran, A., Zada, L., Abou Karam, P., Vadai, E., Roitman, L., Ovadya, Y., et al. (2017). Quantitative identification of senescent cells in aging and disease. Aging Cell 16 (4), 661–671. doi:10.1111/acel.12592
Birch, J., Anderson, R. K., Correia-Melo, C., Jurk, D., Hewitt, G., Marques, F. M., et al. (2015). DNA damage response at telomeres contributes to lung aging and chronic obstructive pulmonary disease. Am. J. Physiology-Lung Cell. Mol. Physiology 309 (10), L1124–L1137. doi:10.1152/ajplung.00293.2015
Blythe, N. M., Muraki, K., Ludlow, M. J., Stylianidis, V., Gilbert, H. T. J., Evans, E. L., et al. (2019). Mechanically activated Piezo1 channels of cardiac fibroblasts stimulate p38 mitogen-activated protein kinase activity and interleukin-6 secretion. J. Biol. Chem. 294 (46), 17395–17408. doi:10.1074/jbc.RA119.009167
Cao, G., Yang, S., Cao, J., Tan, Z., Wu, L., Dong, F., et al. (2022). The role of oxidative stress in intervertebral disc degeneration. Oxid. Med. Cell Longev. 2022, 2166817. doi:10.1155/2022/2166817
Chan, S. C., Walser, J., Käppeli, P., Shamsollahi, M. J., Ferguson, S. J., and Gantenbein-Ritter, B. (2013). Region specific response of intervertebral disc cells to complex dynamic loading: An organ culture study using a dynamic torsion-compression bioreactor. PloS one 8 (8), e72489. doi:10.1371/journal.pone.0072489
Chapman, J., Fielder, E., and Passos, J. F. (2019). Mitochondrial dysfunction and cell senescence: Deciphering a complex relationship. FEBS Lett. 593 (13), 1566–1579. doi:10.1002/1873-3468.13498
Che, H., Li, J., Li, Y., Ma, C., Liu, H., Qin, J., et al. (2020). p16 deficiency attenuates intervertebral disc degeneration by adjusting oxidative stress and nucleus pulposus cell cycle. Elife 9, e52570. doi:10.7554/eLife.52570
Chen, B., Zhu, R., Hu, H., Zhan, M., Wang, T., Huang, F., et al. (2022a). Elimination of senescent cells by senolytics facilitates bony endplate microvessel formation and mitigates disc degeneration in aged mice. Front. Cell Dev. Biol. 10, 853688. doi:10.3389/fcell.2022.853688
Chen, F., Liu, H., Wang, X., Li, Z., Zhang, J., Pei, Y., et al. (2020). Melatonin activates autophagy via the NF-κB signaling pathway to prevent extracellular matrix degeneration in intervertebral disc. Osteoarthr. Cartil. 28 (8), 1121–1132. doi:10.1016/j.joca.2020.05.011
Chen, H.-W., Liu, M.-Q., Zhang, G.-Z., Zhang, C.-Y., Wang, Z.-H., Lin, A.-X., et al. (2022b). Proanthocyanidins inhibit the apoptosis and aging of nucleus pulposus cells through the PI3K/Akt pathway delaying intervertebral disc degeneration. Connect. Tissue Res. 63, 650–662. doi:10.1080/03008207.2022.2063121
Chen, Z., Yang, X., Zhou, Y., Liang, Z., Chen, C., Han, C., et al. (2021). Dehydrocostus lactone attenuates the senescence of nucleus pulposus cells and ameliorates intervertebral disc degeneration via inhibition of STING-TBK1/NF-κB and MAPK signaling. Front. Pharmacol. 12, 641098. doi:10.3389/fphar.2021.641098
Cherif, H., Bisson, D. G., Jarzem, P., Weber, M., Ouellet, J. A., and Haglund, L. (2019). Curcumin and o-vanillin exhibit evidence of senolytic activity in human IVD cells in vitro. J. Clin. Med. 8 (4), 433. doi:10.3390/jcm8040433
Cherif, H., Bisson, D. G., Mannarino, M., Rabau, O., Ouellet, J. A., and Haglund, L. (2020). Senotherapeutic drugs for human intervertebral disc degeneration and low back pain. Elife 9, e54693. doi:10.7554/eLife.54693
Childs, B. G., Durik, M., Baker, D. J., and Van Deursen, J. M. (2015). Cellular senescence in aging and age-related disease: From mechanisms to therapy. Nat. Med. 21 (12), 1424–1435. doi:10.1038/nm.4000
Clark, S., and Horton, R. (2018). Low back pain: A major global challenge. Lancet 391 (10137), 2302. doi:10.1016/S0140-6736(18)30725-6
Clouet, J., Vinatier, C., Merceron, C., Pot-Vaucel, M., Hamel, O., Weiss, P., et al. (2009). The intervertebral disc: From pathophysiology to tissue engineering. Jt. Bone Spine 76 (6), 614–618. doi:10.1016/j.jbspin.2009.07.002
Colombini, A., Lombardi, G., Corsi, M. M., and Banfi, G. (2008). Pathophysiology of the human intervertebral disc. Int. J. Biochem. Cell Biol. 40 (5), 837–842. doi:10.1016/j.biocel.2007.12.011
Coppé, J.-P., Kauser, K., Campisi, J., and Beauséjour, C. M. (2006). Secretion of vascular endothelial growth factor by primary human fibroblasts at senescence. J. Biol. Chem. 281 (40), 29568–29574. doi:10.1074/jbc.M603307200
Davalli, P., Mitic, T., Caporali, A., Lauriola, A., and D’Arca, D. (2016). “ROS, cell senescence, and novel molecular mechanisms in aging and age-related diseases,” in Oxidative medicine and cellular longevity 2016.
Di Micco, R., Krizhanovsky, V., Baker, D., and d’Adda di Fagagna, F. (2021). Cellular senescence in ageing: From mechanisms to therapeutic opportunities. Nat. Rev. Mol. Cell Biol. 22 (2), 75–95. doi:10.1038/s41580-020-00314-w
Dimozi, A., Mavrogonatou, E., Sklirou, A., and Kletsas, D. (2015). Oxidative stress inhibits the proliferation, induces premature senescence and promotes a catabolic phenotype in human nucleus pulposus intervertebral disc cells. Eur. Cell Mater 30 (89), 89–102. doi:10.22203/ecm.v030a07
Dowdell, J., Erwin, M., Choma, T., Vaccaro, A., Iatridis, J., and Cho, S. K. (2017). Intervertebral disk degeneration and repair. Neurosurgery 80 (3S), S46–S54. doi:10.1093/neuros/nyw078
Du, J., Xu, M., Kong, F., Zhu, P., Mao, Y., Liu, Y., et al. (2022). Cb2r attenuates intervertebral disc degeneration by delaying nucleus pulposus cell senescence through ampk/gsk3β pathway. Aging Dis. 13 (2), 552–567. doi:10.14336/AD.2021.1025
Fagagna, F. d. A. d., Reaper, P. M., Clay-Farrace, L., Fiegler, H., Carr, P., Von Zglinicki, T., et al. (2003). A DNA damage checkpoint response in telomere-initiated senescence. Nature 426 (6963), 194–198. doi:10.1038/nature02118
Feng, C., Liu, H., Yang, M., Zhang, Y., Huang, B., and Zhou, Y. (2016). Disc cell senescence in intervertebral disc degeneration: Causes and molecular pathways. Cell cycle 15 (13), 1674–1684. doi:10.1080/15384101.2016.1152433
Feng, C., Yang, M., Lan, M., Liu, C., Zhang, Y., Huang, B., et al. (2017a). “Ros: Crucial intermediators in the pathogenesis of intervertebral disc degeneration,” in Oxidative medicine and cellular longevity 2017.
Feng, C., Zhang, Y., Yang, M., Lan, M., Liu, H., Huang, B., et al. (2017b). “Oxygen-sensing Nox4 generates genotoxic ROS to induce premature senescence of nucleus pulposus cells through MAPK and NF-κB pathways,” in Oxidative medicine and cellular longevity 2017.
Ferrucci, L., and Fabbri, E. (2018). Inflammageing: Chronic inflammation in ageing, cardiovascular disease, and frailty. Nat. Rev. Cardiol. 15 (9), 505–522. doi:10.1038/s41569-018-0064-2
Franceschi, C., Bonafè, M., Valensin, S., Olivieri, F., De Luca, M., Ottaviani, E., et al. (2000). Inflamm-aging: An evolutionary perspective on immunosenescence. Ann. N. Y. Acad. Sci. 908 (1), 244–254. doi:10.1111/j.1749-6632.2000.tb06651.x
Freund, A., Orjalo, A. V., Desprez, P.-Y., and Campisi, J. (2010). Inflammatory networks during cellular senescence: Causes and consequences. Trends Mol. Med. 16 (5), 238–246. doi:10.1016/j.molmed.2010.03.003
Fulop, T., Larbi, A., Khalil, A., Cohen, A. A., and Witkowski, J. M. (2019). Are we ill because we age? Front. physiology 10, 1508. doi:10.3389/fphys.2019.01508
Ge, Y., Zhou, M., Chen, C., Wu, X., and Wang, X. (2022). Role of AMPK mediated pathways in autophagy and aging. Biochimie 195, 100–113. doi:10.1016/j.biochi.2021.11.008
Gorgoulis, V., Adams, P. D., Alimonti, A., Bennett, D. C., Bischof, O., Bishop, C., et al. (2019). Cellular senescence: Defining a path forward. Cell 179 (4), 813–827. doi:10.1016/j.cell.2019.10.005
Hartvigsen, J., Hancock, M. J., Kongsted, A., Louw, Q., Ferreira, M. L., Genevay, S., et al. (2018). What low back pain is and why we need to pay attention. Lancet 391 (10137), 2356–2367. doi:10.1016/S0140-6736(18)30480-X
Hasty, P., Campisi, J., Hoeijmakers, J., Van Steeg, H., and Vijg, J. (2003). Aging and genome maintenance: Lessons from the mouse? Science 299 (5611), 1355–1359. doi:10.1126/science.1079161
Hayflick, L., and Moorhead, P. S. (1961). The serial cultivation of human diploid cell strains. Exp. Cell Res. 25 (3), 585–621. doi:10.1016/0014-4827(61)90192-6
He, J., Zhang, A., Song, Z., Guo, S., Chen, Y., Liu, Z., et al. (2019). The resistant effect of SIRT1 in oxidative stress-induced senescence of rat nucleus pulposus cell is regulated by Akt-FoxO1 pathway. Biosci. Rep. 39 (5). doi:10.1042/BSR20190112
He, S., Zhang, Y., Zhou, Z., Shao, X., Chen, K., Dai, S., et al. (2022). Similarity and difference between aging and puncture-induced intervertebral disc degeneration. J. Orthop. Res. 40 (11), 2565–2575. doi:10.1002/jor.25281
Herranz, N., and Gil, J. (2018). Mechanisms and functions of cellular senescence. J. Clin. investigation 128 (4), 1238–1246. doi:10.1172/JCI95148
Hoare, M., Ito, Y., Kang, T.-W., Weekes, M. P., Matheson, N. J., Patten, D. A., et al. (2016). NOTCH1 mediates a switch between two distinct secretomes during senescence. Nat. Cell Biol. 18 (9), 979–992. doi:10.1038/ncb3397
Hoy, D., March, L., Brooks, P., Woolf, A., Blyth, F., Vos, T., et al. (2010). Measuring the global burden of low back pain. Best Pract. Res. Clin. rheumatology 24 (2), 155–165. doi:10.1016/j.berh.2009.11.002
Hu, S., Shao, Z., Zhang, C., Chen, L., Mamun, A. A., Zhao, N., et al. (2020). Chemerin facilitates intervertebral disc degeneration via TLR4 and CMKLR1 and activation of NF-kB signaling pathway. Aging (Albany NY) 12 (12), 11732–11753. doi:10.18632/aging.103339
Huang, X., Chen, C., Chen, Y., Xu, J., and Liu, L. (2022a). Omentin-1 alleviate interleukin-1β(IL-1β)-induced nucleus pulposus cells senescence. Bioengineered 13 (5), 13849–13859. doi:10.1080/21655979.2022.2084495
Huang, Y., Yang, J., Liu, X., Wang, X., Zhu, K., Ling, Z., et al. (2022b). Cationic polymer brush-modified carbon nanotube-meditated eRNA LINC02569 silencing attenuates nucleus pulposus degeneration by blocking NF-κB signaling pathway and alleviate cell senescence. Front. Cell Dev. Biol. 9, 837777. doi:10.3389/fcell.2021.837777
Hubackova, S., Krejcikova, K., Bartek, J., and Hodny, Z. (2012). IL1-and TGFβ-Nox4 signaling, oxidative stress and DNA damage response are shared features of replicative, oncogene-induced, and drug-induced paracrine ‘bystander senescence. Aging (Albany NY) 4 (12), 932–951. doi:10.18632/aging.100520
Jeon, O. H., Kim, C., Laberge, R.-M., Demaria, M., Rathod, S., Vasserot, A. P., et al. (2017). Local clearance of senescent cells attenuates the development of post-traumatic osteoarthritis and creates a pro-regenerative environment. Nat. Med. 23 (6), 775–781. doi:10.1038/nm.4324
Jeong, S.-W., Lee, J.-S., and Kim, K.-W. (2014). In vitro lifespan and senescence mechanisms of human nucleus pulposus chondrocytes. Spine J. 14 (3), 499–504. doi:10.1016/j.spinee.2013.06.099
Kang, L., Liu, S., Li, J., Tian, Y., Xue, Y., and Liu, X. (2020). The mitochondria-targeted anti-oxidant MitoQ protects against intervertebral disc degeneration by ameliorating mitochondrial dysfunction and redox imbalance. Cell Prolif. 53 (3), e12779. doi:10.1111/cpr.12779
Kang, L., Xiang, Q., Zhan, S., Song, Y., Wang, K., Zhao, K., et al. (2019). Restoration of autophagic flux rescues oxidative damage and mitochondrial dysfunction to protect against intervertebral disc degeneration. Oxid. Med. Cell Longev. 2019, 7810320. doi:10.1155/2019/7810320
Kennedy, B. K., Berger, S. L., Brunet, A., Campisi, J., Cuervo, A. M., Epel, E. S., et al. (2014). Geroscience: Linking aging to chronic disease. Cell 159 (4), 709–713. doi:10.1016/j.cell.2014.10.039
Kim, K. W., Chung, H. N., Ha, K. Y., Lee, J. S., and Kim, Y. Y. (2009). Senescence mechanisms of nucleus pulposus chondrocytes in human intervertebral discs. Spine J. 9 (8), 658–666. doi:10.1016/j.spinee.2009.04.018
Kowald, A., Passos, J. F., and Kirkwood, T. B. (2020). On the evolution of cellular senescence. Aging Cell 19 (12), e13270. doi:10.1111/acel.13270
Krtolica, A., Parrinello, S., Lockett, S., Desprez, P.-Y., and Campisi, J. (2001). Senescent fibroblasts promote epithelial cell growth and tumorigenesis: A link between cancer and aging. Proc. Natl. Acad. Sci. 98 (21), 12072–12077. doi:10.1073/pnas.211053698
Le Maitre, C. L., Freemont, A. J., and Hoyland, J. A. (2005). The role of interleukin-1 in the pathogenesis of human intervertebral disc degeneration. Arthritis Res. Ther. 7 (4), R732–R745. doi:10.1186/ar1732
Le Maitre, C. L., Hoyland, J. A., and Freemont, A. J. (2007). Catabolic cytokine expression in degenerate and herniated human intervertebral discs: IL-1beta and TNFalpha expression profile. Arthritis Res. Ther. 9 (4), R77. doi:10.1186/ar2275
Li, X., Lin, F., Wu, Y., Liu, N., Wang, J., Chen, R., et al. (2019). Resveratrol attenuates inflammation environment-induced nucleus pulposus cell senescence in vitro. Biosci. Rep. 39 (5). doi:10.1042/bsr20190126
Li, Z., Shao, Z., Chen, S., Huang, D., Peng, Y., Chen, S., et al. (2020). TIGAR impedes compression-induced intervertebral disc degeneration by suppressing nucleus pulposus cell apoptosis and autophagy. J. Cell. physiology 235 (2), 1780–1794. doi:10.1002/jcp.29097
Liang, H., Liu, Z., Wang, Y., Wang, D., Tian, J., Duan, C., et al. (2021). The monocyte to high-density lipoprotein cholesterol ratio and outcomes in type 2 diabetes mellitus patients with non-ST-segment elevation acute coronary syndrome. Ann. Transl. Med. 9 (17), 1627. doi:10.21037/atm-21-4876
Liu, J.-Y., Souroullas, G. P., Diekman, B. O., Krishnamurthy, J., Hall, B. M., Sorrentino, J. A., et al. (2019). Cells exhibiting strong p16 INK4a promoter activation in vivo display features of senescence. Proc. Natl. Acad. Sci. 116 (7), 2603–2611. doi:10.1073/pnas.1818313116
Liu, M. y., Zhang, L., Zang, W. d., Zhang, K. g., Li, H. j., and Gao, Y. z. (2022). Pharmacological effects of resveratrol in intervertebral disc degeneration: A literature review. Orthop. Surg. 14 (12), 3141–3149. doi:10.1111/os.13560
López-Otín, C., Blasco, M. A., Partridge, L., Serrano, M., and Kroemer, G. (2013). The hallmarks of aging. Cell 153 (6), 1194–1217. doi:10.1016/j.cell.2013.05.039
Lu, X., Liu, Y., and Zhang, Z. (2020). Matrilin-3 alleviates extracellular matrix degradation of nucleus pulposus cells via induction of IL-1 receptor antagonist. Eur. Rev. Med. Pharmacol. Sci. 24 (10), 5231–5241. doi:10.26355/eurrev_202005_21305
Maher, C., Underwood, M., and Buchbinder, R. (2017). Non-specific low back pain. Lancet 389 (10070), 736–747. doi:10.1016/S0140-6736(16)30970-9
Malaquin, N., Martinez, A., and Rodier, F. (2016). Keeping the senescence secretome under control: Molecular reins on the senescence-associated secretory phenotype. Exp. Gerontol. 82, 39–49. doi:10.1016/j.exger.2016.05.010
Mannarino, M., Cherif, H., Li, L., Sheng, K., Rabau, O., Jarzem, P., et al. (2021). Toll-like receptor 2 induced senescence in intervertebral disc cells of patients with back pain can be attenuated by o-vanillin. Arthritis Res. Ther. 23 (1), 117–214. doi:10.1186/s13075-021-02504-z
Miller, J. A., Schmatz, C., and Schultz, A. (1988). Lumbar disc degeneration: Correlation with age, sex, and spine level in 600 autopsy specimens. Spine 13 (2), 173–178. doi:10.1097/00007632-198802000-00008
Nagase, H., and Kashiwagi, M. (2003). Aggrecanases and cartilage matrix degradation. Arthritis Res. Ther. 5 (2), 94–103. doi:10.1186/ar630
Nasto, L. A., Wang, D., Robinson, A. R., Clauson, C. L., Ngo, K., Dong, Q., et al. (2013). Genotoxic stress accelerates age-associated degenerative changes in intervertebral discs. Mech. ageing Dev. 134 (1-2), 35–42. doi:10.1016/j.mad.2012.11.002
Nelson, G., Wordsworth, J., Wang, C., Jurk, D., Lawless, C., Martin-Ruiz, C., et al. (2012). A senescent cell bystander effect: Senescence-induced senescence. Aging Cell 11 (2), 345–349. doi:10.1111/j.1474-9726.2012.00795.x
Novais, E. J., Diekman, B. O., Shapiro, I. M., and Risbud, M. V. (2019). p16Ink4a deletion in cells of the intervertebral disc affects their matrix homeostasis and senescence associated secretory phenotype without altering onset of senescence. Matrix Biol. 82, 54–70. doi:10.1016/j.matbio.2019.02.004
Novais, E. J., Tran, V. A., Johnston, S. N., Darris, K. R., Roupas, A. J., Sessions, G. A., et al. (2021). Long-term treatment with senolytic drugs Dasatinib and Quercetin ameliorates age-dependent intervertebral disc degeneration in mice. Nat. Commun. 12 (1), 5213. doi:10.1038/s41467-021-25453-2
Ohtori, S., Inoue, G., Miyagi, M., and Takahashi, K. (2015). Pathomechanisms of discogenic low back pain in humans and animal models. spine J. 15 (6), 1347–1355. doi:10.1016/j.spinee.2013.07.490
Osorio, F., Soria-Valles, C., Santiago-Fernández, O., Freije, J., and López-Otín, C. (2016). NF-κB signaling as a driver of ageing. Int. Rev. Cell Mol. Biol. 326, 133–174. doi:10.1016/bs.ircmb.2016.04.003
Ou, H.-L., and Schumacher, B. (2018). DNA damage responses and p53 in the aging process. Blood, J. Am. Soc. Hematol. 131 (5), 488–495. doi:10.1182/blood-2017-07-746396
Patil, P., Dong, Q., Wang, D., Chang, J., Wiley, C., Demaria, M., et al. (2019a). Systemic clearance of p16INK4a-positive senescent cells mitigates age-associated intervertebral disc degeneration. Aging Cell 18 (3), e12927. doi:10.1111/acel.12927
Patil, P., Falabella, M., Saeed, A., Lee, D., Kaufman, B., Shiva, S., et al. (2019b). Oxidative stress-induced senescence markedly increases disc cell bioenergetics. Mech. ageing Dev. 180, 97–106. doi:10.1016/j.mad.2019.04.006
Patil, P., Niedernhofer, L. J., Robbins, P. D., Lee, J., Sowa, G., and Vo, N. (2018). Cellular senescence in intervertebral disc aging and degeneration. Curr. Mol. Biol. Rep. 4, 180–190. doi:10.1007/s40610-018-0108-8
Pattappa, G., Li, Z., Peroglio, M., Wismer, N., Alini, M., and Grad, S. (2012). Diversity of intervertebral disc cells: Phenotype and function. J. Anat. 221 (6), 480–496. doi:10.1111/j.1469-7580.2012.01521.x
Purmessur, D., Walter, B. A., Roughley, P. J., Laudier, D. M., Hecht, A. C., and Iatridis, J. (2013). A role for TNFα in intervertebral disc degeneration: A non-recoverable catabolic shift. Biochem. Biophys. Res. Commun. 433 (1), 151–156. doi:10.1016/j.bbrc.2013.02.034
Qiu, H., Bian, W., Zhang, L., Mei, N., Wu, Y., Wei, Y., et al. (2020). Inhibition of p53/p21 by TWIST alleviates TNF-α induced nucleus pulposus cell senescence in vitro. Eur. Rev. Med. Pharmacol. Sci. 24 (24), 12645–12654. doi:10.26355/eurrev_202012_24161
Qiu, X., Zhuang, M., Lu, Z., Liu, Z., Cheng, D., Zhu, C., et al. (2019). RIPK1 suppresses apoptosis mediated by TNF and caspase-3 in intervertebral discs. J. Transl. Med. 17 (1), 135–214. doi:10.1186/s12967-019-1886-3
Rayess, H., Wang, M. B., and Srivatsan, E. S. (2012). Cellular senescence and tumor suppressor gene p16. Int. J. cancer 130 (8), 1715–1725. doi:10.1002/ijc.27316
Risbud, M. V., and Shapiro, I. M. (2014). Role of cytokines in intervertebral disc degeneration: Pain and disc content. Nat. Rev. Rheumatol. 10 (1), 44–56. doi:10.1038/nrrheum.2013.160
Rodier, F., Coppé, J.-P., Patil, C. K., Hoeijmakers, W. A., Muñoz, D. P., Raza, S. R., et al. (2009). Persistent DNA damage signalling triggers senescence-associated inflammatory cytokine secretion. Nat. Cell Biol. 11 (8), 973–979. doi:10.1038/ncb1909
Rustenburg, C. M., Emanuel, K. S., Peeters, M., Lems, W. F., Vergroesen, P. P. A., and Smit, T. H. (2018). Osteoarthritis and intervertebral disc degeneration: Quite different, quite similar. JOR spine 1 (4), e1033. doi:10.1002/jsp2.1033
Saberi, M., Zhang, X., and Mobasheri, A. (2021). Targeting mitochondrial dysfunction with small molecules in intervertebral disc aging and degeneration. Geroscience 43, 517–537. doi:10.1007/s11357-021-00341-1
Sampara, P., Banala, R. R., Vemuri, S. K., Av, G. R., and Gpv, S. (2018). Understanding the molecular biology of intervertebral disc degeneration and potential gene therapy strategies for regeneration: A review. Gene Ther. 25 (2), 67–82. doi:10.1038/s41434-018-0004-0
Shao, Z., Wang, B., Shi, Y., Xie, C., Huang, C., Chen, B., et al. (2021). Senolytic agent Quercetin ameliorates intervertebral disc degeneration via the Nrf2/NF-κB axis. Osteoarthr. Cartil. 29 (3), 413–422. doi:10.1016/j.joca.2020.11.006
Sharma, A. (2018). The role of adipokines in intervertebral disc degeneration. Med. Sci. 6 (2), 34. doi:10.3390/medsci6020034
Shelton, D. N., Chang, E., Whittier, P. S., Choi, D., and Funk, W. D. (1999). Microarray analysis of replicative senescence. Curr. Biol. 9 (17), 939–945. doi:10.1016/s0960-9822(99)80420-5
Shi, S., Kang, X. J., Zhou, Z., He, Z. M., Zheng, S., and He, S. S. (2022). Excessive mechanical stress-induced intervertebral disc degeneration is related to Piezo1 overexpression triggering the imbalance of autophagy/apoptosis in human nucleus pulpous. Arthritis Res. Ther. 24 (1), 119. doi:10.1186/s13075-022-02804-y
Song, Y., Li, S., Geng, W., Luo, R., Liu, W., Tu, J., et al. (2018). Sirtuin 3-dependent mitochondrial redox homeostasis protects against AGEs-induced intervertebral disc degeneration. Redox Biol. 19, 339–353. doi:10.1016/j.redox.2018.09.006
Sowa, G., Vadalà, G., Studer, R., Kompel, J., Iucu, C., Georgescu, H., et al. (2008). Characterization of intervertebral disc aging: Longitudinal analysis of a rabbit model by magnetic resonance imaging, histology, and gene expression. Spine 33 (17), 1821–1828. doi:10.1097/BRS.0b013e31817e2ce3
Stallone, G., Infante, B., Prisciandaro, C., and Grandaliano, G. (2019). mTOR and aging: an old fashioned dress. Int. J. Mol. Sci. 20 (11), 2774. doi:10.3390/ijms20112774
Sun, Y., Lyu, M., Lu, Q., Cheung, K., and Leung, V. (2022). Current perspectives on nucleus pulposus fibrosis in disc degeneration and repair. Int. J. Mol. Sci. 23 (12), 6612. doi:10.3390/ijms23126612
Sun, Y., Wang, X., Fu, G., and Geng, X. (2021). MicroRNA-199a-5p accelerates nucleus pulposus cell apoptosis and IVDD by inhibiting SIRT1-mediated deacetylation of p21. Mol. Therapy-Nucleic Acids 24, 634–645. doi:10.1016/j.omtn.2021.02.009
Tan, Y., Yao, X., Dai, Z., Wang, Y., and Lv, G. (2019). Bone morphogenetic protein 2 alleviated intervertebral disc degeneration through mediating the degradation of ECM and apoptosis of nucleus pulposus cells via the PI3K/Akt pathway. Int. J. Mol. Med. 43 (1), 583–592. doi:10.3892/ijmm.2018.3972
Van de Ven, R. A., Santos, D., and Haigis, M. C. (2017). Mitochondrial sirtuins and molecular mechanisms of aging. Trends Mol. Med. 23 (4), 320–331. doi:10.1016/j.molmed.2017.02.005
Van Deursen, J. M. (2014). The role of senescent cells in ageing. Nature 509 (7501), 439–446. doi:10.1038/nature13193
Vergroesen, P.-P., Kingma, I., Emanuel, K. S., Hoogendoorn, R. J., Welting, T. J., van Royen, B. J., et al. (2015). Mechanics and biology in intervertebral disc degeneration: A vicious circle. Osteoarthr. Cartil. 23 (7), 1057–1070. doi:10.1016/j.joca.2015.03.028
Vermeij, W., Dollé, M., Reiling, E., Jaarsma, D., Payan-Gomez, C., Bombardieri, C. R., et al. (2016). Restricted diet delays accelerated ageing and genomic stress in DNA-repair-deficient mice. Nature 537 (7620), 427–431. doi:10.1038/nature19329
Vo, N., Niedernhofer, L. J., Nasto, L. A., Jacobs, L., Robbins, P. D., Kang, J., et al. (2013). An overview of underlying causes and animal models for the study of age-related degenerative disorders of the spine and synovial joints. J. Orthop. Res. 31 (6), 831–837. doi:10.1002/jor.22204
Vo, N., Seo, H. Y., Robinson, A., Sowa, G., Bentley, D., Taylor, L., et al. (2010). Accelerated aging of intervertebral discs in a mouse model of progeria. J. Orthop. Res. 28 (12), 1600–1607. doi:10.1002/jor.21153
Vo, N. V., Hartman, R. A., Patil, P. R., Risbud, M. V., Kletsas, D., Iatridis, J. C., et al. (2016). Molecular mechanisms of biological aging in intervertebral discs. J. Orthop. Res. 34 (8), 1289–1306. doi:10.1002/jor.23195
Vos, T., Barber, R. M., Bell, B., Bertozzi-Villa, A., Biryukov, S., Bolliger, I., et al. (2015). Global, regional, and national incidence, prevalence, and years lived with disability for 301 acute and chronic diseases and injuries in 188 countries, 1990–2013: A systematic analysis for the global burden of disease study 2013. lancet 386 (9995), 743–800. doi:10.1016/S0140-6736(15)60692-4
Walter, B., Korecki, C., Purmessur, D., Roughley, P., Michalek, A., and Iatridis, J. (2011). Complex loading affects intervertebral disc mechanics and biology. Osteoarthr. Cartil. 19 (8), 1011–1018. doi:10.1016/j.joca.2011.04.005
Wang, D., He, X., Wang, D., Peng, P., Xu, X., Gao, B., et al. (2020a). Quercetin suppresses apoptosis and attenuates intervertebral disc degeneration via the SIRT1-autophagy pathway. Front. Cell Dev. Biol. 8, 613006. doi:10.3389/fcell.2020.613006
Wang, D., Nasto, L. A., Roughley, P., Leme, A. S., Houghton, A., Usas, A., et al. (2012). Spine degeneration in a murine model of chronic human tobacco smokers. Osteoarthr. Cartil. 20 (8), 896–905. doi:10.1016/j.joca.2012.04.010
Wang, F., Cai, F., Shi, R., Wang, X. H., and Wu, X. T. (2016). Aging and age related stresses: A senescence mechanism of intervertebral disc degeneration. Osteoarthr. Cartil. 24 (3), 398–408. doi:10.1016/j.joca.2015.09.019
Wang, J., Huang, C., Lin, Z., Pan, X., Chen, J., Zheng, G., et al. (2018a). Polydatin suppresses nucleus pulposus cell senescence, promotes matrix homeostasis and attenuates intervertebral disc degeneration in rats. J. Cell Mol. Med. 22 (11), 5720–5731. doi:10.1111/jcmm.13848
Wang, L., Gu, Y., Zhao, H., Chen, R., Chen, W., Qi, H., et al. (2020b). Dioscin attenuates interleukin 1β (IL-1β)-induced catabolism and apoptosis via modulating the toll-like receptor 4 (Tlr4)/nuclear factor kappa B (NF-κB) signaling in human nucleus pulposus cells. Med. Sci. Monit. Int. Med. J. Exp. Clin. Res. 26, e923386–e923381. doi:10.12659/MSM.923386
Wang, W.-J., Yu, X.-H., Wang, C., Yang, W., He, W.-S., Zhang, S.-J., et al. (2015). MMPs and ADAMTSs in intervertebral disc degeneration. Clin. Chim. Acta 448, 238–246. doi:10.1016/j.cca.2015.06.023
Wang, Y., Hu, Y., Wang, H., Liu, N., Luo, L., Zhao, C., et al. (2021). Deficiency of MIF accentuates overloaded compression-induced nucleus pulposus cell oxidative damage via depressing mitophagy. Oxid. Med. Cell Longev. 2021, 6192498. doi:10.1155/2021/6192498
Wang, Y., Shen, J., Chen, Y., Liu, H., Zhou, H., Bai, Z., et al. (2018b). PINK1 protects against oxidative stress induced senescence of human nucleus pulposus cells via regulating mitophagy. Biochem. biophysical Res. Commun. 504 (2), 406–414. doi:10.1016/j.bbrc.2018.06.031
Wang, Y., and Tissenbaum, H. A. (2006). Overlapping and distinct functions for a Caenorhabditis elegans SIR2 and DAF-16/FOXO. Mech. ageing Dev. 127 (1), 48–56. doi:10.1016/j.mad.2005.09.005
Wang, Y., Zuo, R., Wang, Z., Luo, L., Wu, J., Zhang, C., et al. (2019). Kinsenoside ameliorates intervertebral disc degeneration through the activation of AKT-ERK1/2-Nrf2 signaling pathway. Aging (Albany NY) 11 (18), 7961–7977. doi:10.18632/aging.102302
Wen, P., Zheng, B., Zhang, B.-F., Ma, T., Hao, L., and Zhang, Y. (2022). The role of ageing and oxidative stress in intervertebral disc degeneration. Front. Mol. Biosci. 9, 1052878. doi:10.3389/fmolb.2022.1052878
Wiley, C. D., Velarde, M. C., Lecot, P., Liu, S., Sarnoski, E. A., Freund, A., et al. (2016). Mitochondrial dysfunction induces senescence with a distinct secretory phenotype. Cell metab. 23 (2), 303–314. doi:10.1016/j.cmet.2015.11.011
Wu, J., Chen, Y., Liao, Z., Liu, H., Zhang, S., Zhong, D., et al. (2022a). Self-amplifying loop of NF-κB and periostin initiated by PIEZO1 accelerates mechano-induced senescence of nucleus pulposus cells and intervertebral disc degeneration. Mol. Ther. 30 (10), 3241–3256. doi:10.1016/j.ymthe.2022.05.021
Wu, Y., Shen, S., Shi, Y., Tian, N., Zhou, Y., and Zhang, X. (2022b). Senolytics: Eliminating senescent cells and alleviating intervertebral disc degeneration. Front. Bioeng. Biotechnol. 10, 823945. doi:10.3389/fbioe.2022.823945
Wuertz, K., and Haglund, L. (2013). Inflammatory mediators in intervertebral disk degeneration and discogenic pain. Glob. spine J. 3 (3), 175–184. doi:10.1055/s-0033-1347299
Xi, Y., Ma, J., and Chen, Y. (2020). PTEN promotes intervertebral disc degeneration by regulating nucleus pulposus cell behaviors. Cell Biol. Int. 44 (2), 583–592. doi:10.1002/cbin.11258
Xie, C., Shi, Y., Chen, Z., Zhou, X., Luo, P., Hong, C., et al. (2022). Apigenin alleviates intervertebral disc degeneration via restoring autophagy flux in nucleus pulposus cells. Front. Cell Dev. Biol. 9, 787278. doi:10.3389/fcell.2021.787278
Xin, J., Wang, Y., Zheng, Z., Wang, S., Na, S., and Zhang, S. (2022). Treatment of intervertebral disc degeneration. Orthopaedic Surgery.
Xing, Q.-J., Liang, Q.-Q., Bian, Q., Ding, D.-F., Cui, X.-J., Shi, Q., et al. (2010). Leg amputation accelerates senescence of rat lumbar intervertebral discs. Spine 35 (23), E1253–E1261. doi:10.1097/BRS.0b013e3181e7d087
Xu, X., Wang, D., Zheng, C., Gao, B., Fan, J., Cheng, P., et al. (2019). Progerin accumulation in nucleus pulposus cells impairs mitochondrial function and induces intervertebral disc degeneration and therapeutic effects of sulforaphane. Theranostics 9 (8), 2252–2267. doi:10.7150/thno.30658
Yang, Q., Zhou, Y., Wang, J., Fu, W., and Li, X. (2019). Study on the mechanism of excessive apoptosis of nucleus pulposus cells induced by shRNA-Piezo1 under abnormal mechanical stretch stress. J. Cell Biochem. 120 (3), 3989–3997. doi:10.1002/jcb.27683
Yousefzadeh, M., Henpita, C., Vyas, R., Soto-Palma, C., Robbins, P., and Niedernhofer, L. (2021). DNA damage—How and why we age? Elife 10, e62852. doi:10.7554/eLife.62852
Yurube, T., Ito, M., Kakiuchi, Y., Kuroda, R., and Kakutani, K. (2020). Autophagy and mTOR signaling during intervertebral disc aging and degeneration. JOR spine 3 (1), e1082. doi:10.1002/jsp2.1082
Zeiter, S., Bishop, N., and Ito, K. (2005). Significance of the mechanical environment during regeneration of the intervertebral disc. Eur. Spine J. 14, 874–879. doi:10.1007/s00586-005-0957-8
Zhang, C., Peng, X., Wang, F., Xie, Z., Chen, L., and Wu, X. (2022). Update on the correlation between mitochondrial dysfunction and intervertebral disk degeneration. DNA Cell Biol. 41 (3), 257–261. doi:10.1089/dna.2021.1012
Zhang, G.-Z., Deng, Y.-J., Xie, Q.-Q., Ren, E.-H., Ma, Z.-J., He, X.-G., et al. (2020). Sirtuins and intervertebral disc degeneration: Roles in inflammation, oxidative stress, and mitochondrial function. Clin. Chim. acta 508, 33–42. doi:10.1016/j.cca.2020.04.016
Zhang, G. Z., Liu, M. Q., Chen, H. W., Wu, Z. L., Gao, Y. C., Ma, Z. J., et al. (2021a). NF-κB signalling pathways in nucleus pulposus cell function and intervertebral disc degeneration. Cell Prolif. 54 (7), e13057. doi:10.1111/cpr.13057
Zhang, K., Zhang, Y., Zhang, C., and Zhu, L. (2021b). Upregulation of P53 promotes nucleus pulposus cell apoptosis in intervertebral disc degeneration through upregulating NDRG2. Cell Biol. Int. 45 (9), 1966–1975. doi:10.1002/cbin.11650
Zhang, S., Hu, B., Liu, W., Wang, P., Lv, X., Chen, S., et al. (2021c). The role of structure and function changes of sensory nervous system in intervertebral disc-related low back pain. Osteoarthr. Cartil. 29 (1), 17–27. doi:10.1016/j.joca.2020.09.002
Zhang, S., Liu, W., Wang, P., Hu, B., Lv, X., Chen, S., et al. (2021d). Activation of HSP70 impedes tert-butyl hydroperoxide (t-BHP)-induced apoptosis and senescence of human nucleus pulposus stem cells via inhibiting the JNK/c-Jun pathway. Mol. Cell Biochem. 476 (5), 1979–1994. doi:10.1007/s11010-021-04052-1
Zhang, Z., Lin, J., Nisar, M., Chen, T., Xu, T., Zheng, G., et al. (2019). “The Sirt1/P53 axis in diabetic intervertebral disc degeneration pathogenesis and therapeutics,” in Oxidative medicine and cellular longevity 2019.
Zhao, C.-Q., Wang, L.-M., Jiang, L.-S., and Dai, L.-Y. (2007). The cell biology of intervertebral disc aging and degeneration. Ageing Res. Rev. 6 (3), 247–261. doi:10.1016/j.arr.2007.08.001
Keywords: aging, cell senescence, pathogensis, targeted therapies of aging, intervertebral disc degeneration
Citation: Xu J, Shao T, Lou J, Zhang J and Xia C (2023) Aging, cell senescence, the pathogenesis and targeted therapies of intervertebral disc degeneration. Front. Pharmacol. 14:1172920. doi: 10.3389/fphar.2023.1172920
Received: 24 February 2023; Accepted: 25 April 2023;
Published: 05 May 2023.
Edited by:
Chen-he Zhou, Zhejiang University, ChinaReviewed by:
Chawon Yun, Northwestern University, United StatesZhaozhi Li, The First Affiliated Hospital of Shandong First Medical University, China
Mengchen Yin, Shanghai University of Traditional Chinese Medicine, China
Xiaobin Wang, The Second Xiangya Hospital of Central South University, China
Copyright © 2023 Xu, Shao, Lou, Zhang and Xia. This is an open-access article distributed under the terms of the Creative Commons Attribution License (CC BY). The use, distribution or reproduction in other forums is permitted, provided the original author(s) and the copyright owner(s) are credited and that the original publication in this journal is cited, in accordance with accepted academic practice. No use, distribution or reproduction is permitted which does not comply with these terms.
*Correspondence: Chen Xia, 497077831@qq.com; Jun Zhang, spinezhangjun@aliyun.com
†These authors have contributed equally to this work