- 1Department of Pharmacology, School of Pharmacy, North Sichuan Medical College, Nanchong, China
- 2Nanchong Key Laboratory of Disease Prevention, Control and Detection in Livestock and Poultry, Nanchong Vocational and Technical College, Nanchong, China
- 3Department of Pharmacy, Minhang Hospital, Fudan University, Shanghai, China
Gluconeogenesis is closely related to the occurrence and development of type 2 diabetes mellitus (T2DM). Gentiopicroside (GPS) is the main active secoiridoid glycoside in Gentiana manshurica Kitagawa, which can improve chronic complications associated with diabetes and regulate glucose metabolism. However, the effects and potential mechanisms by which GPS affects T2DM understudied and poorly understood. In this study, we systematically explored the pharmacological effects of GPS on T2DM induced by a high-fat diet (HFD) and streptozotocin (STZ) as well as explored its related mechanisms. The results showed that GPS supplementation discernibly decreased blood glucose levels, food intake and water consumption, ameliorated glucose intolerance, abnormal pyruvate tolerance, insulin resistance and dyslipidemia. Furthermore, GPS discernibly ameliorated pathological morphological abnormalities of the liver and pancreas, reduced hepatic steatosis and maintain the balance between α-cells and β-cells in pancreas. Moreover, GPS significantly inhibited gluconeogenesis, as evidenced by the suppressed protein expression of phosphoenolpyruvate carboxykinase (PEPCK) and glucose 6-phosphatase (G6Pase) in the liver. Additionally, the results of Western blot analysis revealed that GPS increased p-PI3K, p-AKT, and p-FOXO1 expression levels, and decreased FOXO1 expression at protein level in the liver. Furthermore, the results of the immunostaining and Western blot analysis demonstrated that GPS supplementation increased the expression of zonula occludens-1 (ZO-1) and occludin in the ileum. Collectively, these results indicate that GPS may inhibit hepatic gluconeogenesis by regulating the PI3K/AKT/FOXO1 signaling pathway and maintain intestinal barrier integrity, and ultimately improve T2DM. Together, these findings indicate that GPS is a potential candidate drug for the prevention and treatment of T2DM, and the results of our study will provide experimental basis for further exploration of the possibility of GPS as a therapeutic agent for T2DM.
1 Introduction
Owing to rapid urbanization, the aging population, as well as lifestyle changes and improvements in society, which has resulted in an increase in the number of diabetic patients annually. More than 90% of diabetes patients are diagnosed with type 2 diabetes mellitus (T2DM) (Laakso et al., 2022), which is a chronic metabolic disease characterized by hyperglycemia, intestinal flora disorder, insulin resistance, and obesity due to insufficient insulin secretion and an ineffective insulin response, and has become disease that has caused a high public burden worldwide (Ahmad et al., 2022; Magliano et al., 2022). According to the 10th edition of the IDF Diabetes Atlas, the number of diabetic patients was 537 million worldwide in 2021. This number is estimated to rise to 643 million by 2030 and 784 million by 2045 (Sun et al., 2022). When blood glucose levels are not well regulated, it can gradually give rise to more serious chronic microvascular or macrovascular complications, which severely affect people’s quality of life and create a huge economic burden (Yingrui et al., 2022). Therefore, it is essential to identify novel therapeutic strategies and develop effective drugs that can inhibit the progression of T2DM.
Under normal circumstances, the body maintains its blood glucose level by regulating the balance between the production and absorption of glucose. The liver is one of three major target organs that is affected by insulin and plays a fundamental role in maintaining body’s blood glucose homeostasis (Liang et al., 2022). The maintenance of glucose balance in the body depends on glucose uptake by the muscles and adipose tissues, as well as glucose production by gluconeogenesis and glycogenolysis in the liver (Yan et al., 2019). The liver mainly regulates blood glucose levels by regulating hepatic glucose production (HGP), which is mainly the result of gluconeogenesis and liver glycogen breakdown, while 90% of endogenous glucose production is mediated by HGP (Fujimoto et al., 2022). The abnormal modulate of glucose production in the liver is the principal cause of hyperglycemia and insulin resistance in T2DM (Giralt et al., 2018). Notably, in the diabetic state, excessive HGP exceeds systemic glucose uptake, which in turn leads to the dysregulation of blood glucose levels. Moreover, during diabetes, gluconeogenesis levels in the liver are abnormally high and are accompanied by high levels of cholesterol and triglyceride synthesis, which eventually develops into hyperglycemia and hyperlipidemia if not controlled in time (Tseng et al., 2019). In addition, many studies have shown that HGP inhibition is an effective strategy used for the prevention and treatment of T2DM (Liu et al., 2015; Wu et al., 2021). Therefore, gluconeogenesis inhibition should be an effectual strategy for the prevention and treatment of T2DM.
Gentiopicroside (GPS) is an iridoid glycoside isolated from the perennial herbs of Gentianaceae and is widely used in China as a herbal medicine for the treatment of rheumatoid arthritis, hemiplegia, joint pain, stroke, and hypertension (Xing et al., 2021). In addition, GPS has been found to exert anti-inflammatory, anti-oxidant, and cholagogic pharmacological activities in all kinds of disease models (Wu et al., 2017; Li et al., 2018; Jin et al., 2020). In regard to diabetes and its complications, GPS significantly improved peripheral neuropathy in STZ-induced diabetic rats, and its effects may be associated with the improvement of neural blood flow and the regulation of dyslipidemia (Lu et al., 2018). GPS significantly improved diabetic retinopathy and diabetic nephropathy by inhibiting the inflammatory response and oxidative stress (Zhang et al., 2019; Xu et al., 2022). GPS activated the PI3K/AKT signaling pathway by targeting progestin and adipoQ receptor 3 (PAQR3), thereby significantly improving glycolipid metabolism disorder in high-fat fed mice (Xiao et al., 2022). GPS has been found to maintain glucose homeostasis by inhibiting gluconeogenesis in L02 cells and mice (Yang et al., 2018). In addition, GPS improved atherosclerosis in high-fat diet-induced ApoE−/− mice by modulating intestinal flora and fecal metabolites (Xie et al., 2022). Although many studies have shown that GPS can improve symptoms of hyperglycemia in diabetes, but it remains to be ascertained whether its effects are associated with gluconeogenesis and intestinal permeability. Based on the aforementioned analysis, we further investigated whether GPS improves T2DM by inhibiting gluconeogenesis and regulating the intestinal permeability.
2 Materials and methods
2.1 Reagents and antibodies
Gentiopicroside (C16H20O9, MW: 356.3, purity: ≧ 98%) and STZ (purity≧99%) were obtained from Yuanye Bio-Technology Co. Ltd (Shanghai, China). The kits used to determine triglyceride (TG), total cholesterol (TC), high-density lipoprotein cholesterol (HDL-C), and low-density lipoprotein cholesterol (LDL-C) levels were obtained from Biosino Biotechnology and Science Inc (Beijing, China). The kits for assessing alanine transaminase (ALT) and aspartate transaminase (AST) activities were purchased from the Nanjing Jiancheng Bioengineering Institute (Nanjing, China). The kit used to determine the glycosylated hemoglobin (HbA1c) level was purchased from Beijing Homa Biological Engineering Co. Ltd (Beijing, China), while kit for insulin detection was obtained from CAMILO BIOLOGICAL (Nanjing, China). Antibodies against phosphoenolpyruvate carboxylase (PEPCK), glucose 6-phosphatase (G6Pase), zonula occludens-1 (ZO-1), and occludin were purchased from Affinity Bioscience (United States). Antibodies against phosphoinositide 3-kinase (PI3K), protein kinase B (AKT) and β-actin were obtained from Wuhan Servicebio Technology Co. Ltd (Wuhan, China). Antibodies against forkhead box O1 (FOXO1), phospho-PI3K (Tyr458), phospho-AKT (Ser473), phospho-FOXO1 (Ser256) were bought from Cell Signaling Technology (Danvers, MA, United States). The Polyvinylidene difluoride (PVDF) membranes was bought from Millipore (CA, United States). Bicinchoninic acid (BCA) protein kit, RIPA lysis buffer, protease and phosphatase inhibitors, enhanced chemiluminescence (ECL) reagent, and the horseradish peroxidase (HRP)-labeled IgG were obtained from Applygen Technologies (Beijing, China).
2.2 Animals
Forty male C57BL/6 J mice (aged 8 weeks old; weighing 20–22 g) were purchased from HFK Bioscience (Beijing, China). The mice were kept in a room at a relative humidity of 55% ± 10%, a temperature of 23°C ± 2°C, and a light/dark cycle of 12 h. The mice were allowed free access to drinking water and standard laboratory food. Animals and all study protocols were performed strictly in accordance with Chinese standards and guidelines for the use of laboratory animals (GB14925-2001 and MOST 2006 a), and were carried out with the approval of the Experimental Animal Welfare Ethics Committee of the North Sichuan Medical College (Approval No. 202205).
2.3 Experimental design
The establishment of T2DM mouse model referred to the following literature with a small change (Yan et al., 2018; Zhang et al., 2018; Xiao et al., 2022; Xie et al., 2022). The 30 mice were adaptively fed for a week, and were then administered a high-fat diet (HFD, 60% fat), while the other 10 mice were fed on a normal diet for 8 weeks. Subsequently, the HFD mice were fasted for 6 h and injected with STZ (50 mg/kg) dissolved in an ice-cold citrate buffer (0.1 M, pH 4.5), on 4 consecutive days. The mice that were given a normal diet were intraperitoneally injected with a citric acid buffer. After 3 days, the fasting blood glucose (FBG) level of each mouse was measured, and mice with FBG >200 mg/dL were regarded as diabetic mice and were selected for further study. The diabetic mice were divided into two groups, based on their blood glucose level and body weight: 1) the diabetic model group (Mod); and 2) the GPS intragastric administration treatment group (GPS, 50 mg/kg). The dosage selected was based on previously published articles (Lu et al., 2018; Xiao et al., 2020; Xiao et al., 2022; Xu et al., 2022; Zou et al., 2022). Mice that were administered a normal diet were used as the normal control group (Nor). After the mice were divided into groups, GPS was dissolved in 0.5% sodium carboxymethyl cellulose (CMC-Na) to create a suspension, and the suspension was administered through gavage for 7 weeks, once a day. The mice in the Nor and Mod groups were orally administered a 0.5% CMC-Na suspension. Then, random blood glucose (RBG), FBG, and body weight were measured weekly during the experiment. After 7 weeks of treatment, the mice were fasted for 6 h, and after being administered pentobarbital sodium anesthesia, whole blood samples were collected through retroorbital puncture and were allowed to stand at room temperature for 2 h. Then, serum was collected through centrifugation at 4°C and 6,000 g for 10 min, and frozen until the analysis was conducted. The liver, pancreas and small intestine were immediately removed, and a few of these samples were fixed in 4% neutral paraformaldehyde for histological analysis, while the rest were quickly frozen for different analyses. The flow chart of the mice treatment procedure is depicted in Figure 1A.
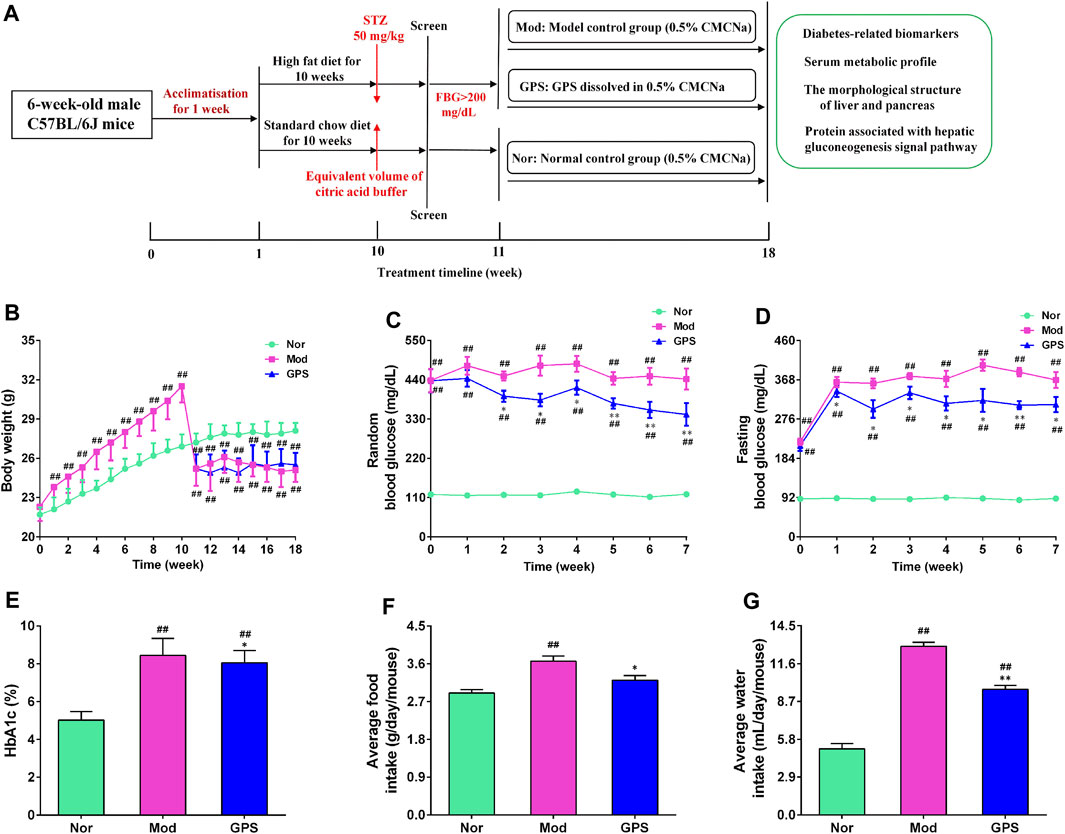
FIGURE 1. GPS attenuated metabolic disorders in HFD and STZ-induced diabetic mice (A) The flowchart of treatments administered to the mice (B) Body weight (C) RBG concentrations (D) FBG concentration (E) HbA1c (F) Average food intake (G) Average water concentrations. Data are presented as means ± SEM, n = 10. #p < 0.05; ##p < 0.01 vs. Nor group; *p < 0.05; **p < 0.01 vs. Mod group.
2.4 Oral glucose tolerance test (OGTT), insulin tolerance test (ITT), and pyruvate tolerance test (PTT)
The OGTT, ITT, and PTT were conducted during weeks 6 and 7. The mice were fasted 10 h before OGTT and then glucose (2 g/kg) was administered through gavage. Thereafter, blood glucose levels of all the mice were measured at 30, 60, 90, and 120 min after glucose loading. The mice were fasted for 6 h before ITT and were intraperitoneally injected with 0.75 IU/kg insulin. Afterwards, blood glucose levels of all the mice were measured at 30, 60, 90, and 120 min after insulin injection. The mice were fasted 10 h before PTT and were given pyruvate (1.5 g/kg) through gavage. Thereafter, the blood glucose levels of all the mice were measured at 30, 60, 90, and 120 min after pyruvate loading. The area under the curve (AUC) was calculated.
2.5 Biochemical analysis
The serum LDL-C, HDL-C, TC, TG, ALT, AST and insulin levels were assayed using commercial detection kits in accordance with the manufacturer’s instructions. The homeostasis model assessment-insulin resistance (HOMA-IR) and insulin sensitivity index (ISI) were calculated according to the following formulas: HOMA-IR = Insulin concentration (ng/mL) × FBG (mmol/L)/22.5, ISI = 1/[FBG (mmol/L) × fasting serum insulin (ng/mL)].
2.6 Histopathological analysis
After the fresh liver tissue samples were fixed for 24 h, they were embedded in paraffin and cut into 5 μm thick slices. Following the manufacturer’s instructions, the slices were stained with hematoxylin and eosin (H&E), as well as periodic acid-Schiff staining (PAS) and oil red staining. Finally, all slices were scanned under a scanning microscope (Leica, Aperio CS2, United States) and analyzed.
2.7 Immunofluorescence assay
After the mice were sacrificed, the pancreas was harvested, and fixed in 10% formalin, embedded in paraffin and cut into 5 μm sections, to make slides that were subsequently stained with anti-insulin and glucagon antibodies, in the same manner as previously described (Li et al., 2017). Finally, the ImageJ software was used to calculate the fluorescence intensity of insulin and glucagon.
2.8 Immunohistochemistry assay
After the mice were sacrificed, a portion of the liver and ileum was removed, fixed in 4% paraformaldehyde for 24 h, embedded in paraffin, and cut into 5-μm-thick sections using a microtome, followed by staining with the relevant antibodies (Zhong et al., 2019). The images were captured under a scanner and the staining positive area were calculated with ImageJ.
2.9 Western blotting analysis
A certain mass of tissue was weighed, and the RIPA lysis buffer containing protease inhibitor was added. After the total proteins were extracted, its concentration was determined using the BCA method. Then, the protein concentrations of all the samples were adjusted to the same concentration, and 5 × loading buffer was added. Afterward, the samples were denatured in boiling water at 100°C for 10 min. An equal amount of the denatured proteins was separated using sodium dodecyl sulfate-polyacrylamide gel electrophoresis and transferred onto the PVDF membrane. After blocking with 5% skimmed milk powder for 2 h at room temperature, the membranes were incubated with the corresponding antibodies at 4°C, overnight. After washing thrice, the samples were incubated with the second antibody coupled with horseradish peroxidase for 2 h at room temperature. After washing, the bands were observed using an ECL luminescent reagent under a chemiluminescent system. Then, the protein band densities were analyzed using Image Lab 3.0 software.
2.10 Statistical analysis
Data are presented herein as mean ± standard error of the mean (SEM). We analyzed the data using one-way analysis of variance along with Bonferroni’s correction. Differences with a p < 0.05 were considered to indicate statistical significance.
3 Results
3.1 GPS attenuated metabolic disorders in HFD and STZ-induced diabetic mice
To explore the effect of GPS on the metabolism of diabetic mice, the weight, food intake, water consumption, and blood glucose level of all the mice were recorded once a week. The mice that were fed a normal diet steadily gained weight during the study, while the body weight of the HFD mice increased obviously from the first week to the 10th week, and was higher than that of the Nor group. Following STZ injection, the body weight of the HFD mice decreased significantly, and was observably lower than that of the Nor group. During the administration, the results showed that GPS had no effect on the body weight of the diabetic mice (Figure 1A). The RBG, FBG, HbA1c, food intake, and water intake of mice were markedly increased in the Mod group compared with the Nor group (Figures 1B–F), indicating that the metabolism of mice was disordered in the former group. Relative to the Mod group, GPS markedly decreased the RBG of the diabetic mice from the second week (Figure 1B), significantly decreased the FBG of diabetic mice from the first week (Figure 1C), and decreased the HbA1c (Figure 1D), food consumption (Figure 1E), and water consumption (Figure 1F) of the diabetic mice. All in all, these findings indicate that GPS significantly improves metabolic disorders in diabetic mice.
3.2 GPS ameliorated glucose homeostasis in HFD and STZ-induced diabetic mice
To further investigate the effect of GPS on glucose metabolism, OGTT, ITT, and PTT experiments were conducted during the study. As anticipated, the results of the OGTT and ITT experiments showed that blood glucose at each time point and the AUC of the diabetic mice in the Mod group were remarkably higher, compared with the Nor group (Figures 2A–D), indicating that the diabetic mice had developed abnormal glucose tolerance and insulin resistance. GPS supplementation significantly decreased blood glucose levels at each time point and the AUC of diabetic mice during the two tests (Figures 2A–D). To determine whether the improvement of glucose homeostasis exerted by GPS was associated with gluconeogenesis, we immediately performed a pyruvate tolerance test. The results demonstrated that GPS supplementation resulted in significantly lower blood glucose levels following pyruvate injection (Figure 2E), as well as a significantly smaller AUC (Figure 2F), relative to the Mod group. Next, the insulin content of the serum was measured, and the HOMA-IR and ISI indexes were calculated. The data demonstrated that GPS decreased the serum insulin content of the diabetic mice, but there was no statistical difference (Figure 2G). In addition, GPS also significantly decreased the HOMA-IR index and increased ISI index (Figures 2H,I). Taken together, these results indicate that GPS significantly alleviated glucose metabolism disorders in HFD and STZ-induced diabetic mice.
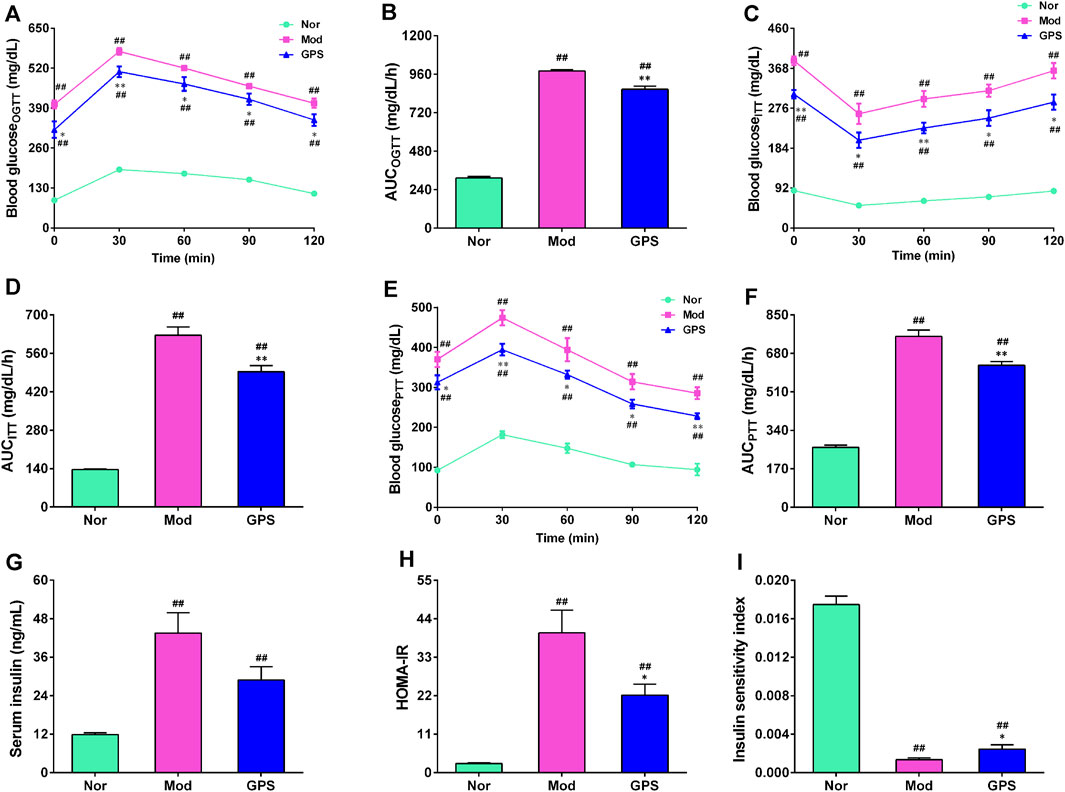
FIGURE 2. GPS ameliorated glucose homeostasis in HFD and STZ-induced diabetic mice (A) OGTT in mice (B) AUC of OGTT in mice (C) ITT in mice (D) AUC of ITT in mice (E) PTT in mice (F) AUC of PTT in mice (G) Serum insulin (H) HOMA-IR in mice (I) Insulin sensitivity index (ISI) in mice. Data are presented as mean ± SEM, n = 10. #p < 0.05; ##p < 0.01 vs. Nor group; *p < 0.05; **p < 0.01 vs. Mod group.
3.3 GPS attenuated islet morphology in HFD and STZ-induced diabetic mice
To ascertain whether GPS can protect the islets of diabetic mice from hyperglycemia, immunofluorescence staining and HE staining were performed on the islets. As illustrated in Figure 3, relative to the Nor group, the insulin content in the islets of the diabetic mice in Mod group were significantly downregulate, while the glucagon content were markedly upregulated. However, after 7 weeks of GPS treatment, these abnormal changes had been discernibly reversed (Figure 3A). At the same time, the HE staining results also showed that GPS could significantly improve hyperglycemia-induced pancreatic islet injury (Figure 3A). The statistical results of immunofluorescence staining intensity showed that the ratio of the insulin positive area to the islet area was decreased remarkably, while the ratio of the glucagon positive area to the pancreatic islet area was increased remarkably, and the mean fluorescence intensity ratio of insulin to glucagon in the diabetic mice of the Mod group was remarkably decreased, relative to the Nor group mice, indicating an imbalance between α-cells and β-cells. However, these abnormal changes were significantly alleviated following GPS treatment (Figures 3B–D). In summary, these data indicate that GPS plays a pivotal role in maintaining insulin-glucagon homeostasis and improving islet function in HFD and STZ-induced diabetic mice.
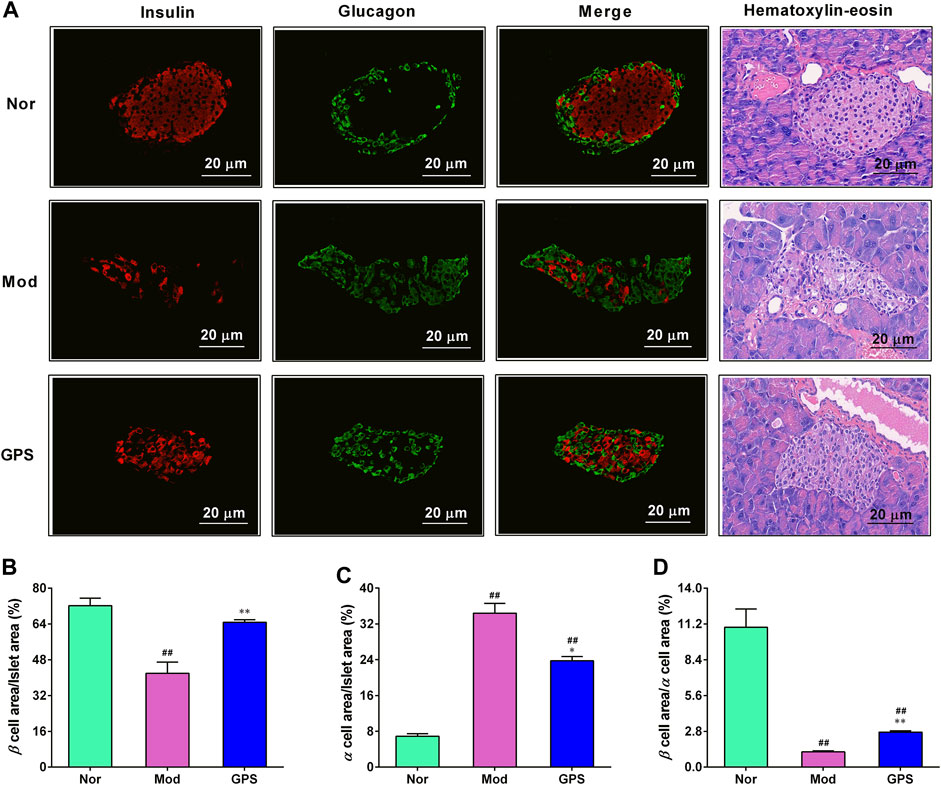
FIGURE 3. GPS attenuated islet morphology in HFD and STZ-induced diabetic mice (A) Representative images of insulin (red) and glucagon (green) immunofluorescence staining of the pancreatic islets. Magnification of all images is at × 200 (B) Insulin-positive area to the total islet area (C) Glucagon-positive area to the total islet area (D) The ratio of insulin to the glucagon. Data are presented as mean ± SEM, n = 4. #p < 0.05; ##p < 0.01 vs. Nor group; *p < 0.05; **p < 0.01 vs. Mod group.
3.4 GPS ameliorated dyslipidemia in HFD and STZ-induced diabetic mice
Dyslipidemia is closely associated with the occurrence and development of T2DM (Goldberg et al., 2022). Therefore, the indexes of lipid metabolism in the serum were determined. As illustrated in Figure 4, relative to the Nor group, the serum TG, TC, and LDL-C contents of the diabetic mice in the Mod group were remarkably increased (Figures 4A,B,D), while the HDL-C content was significantly decreased (Figure 4C), indicating that the diabetic mice suffered from observable lipid disorders. However, following GPS treatment for 7 weeks, the serum TG, TC, and LDL-C contents of the diabetic mice decreased significantly (Figures 4A,B,D), and the HDL-C content increased remarkably, compared with the Mod group (Figure 4C). Notably, compared with the Mod group, GPS significantly reduced the kidney index and liver index of the diabetic mice (Figures 4E,F). These results suggest that the amelioration of diabetic symptoms as a result of GPS treatment may be associated with the improvement in blood lipid disorders.
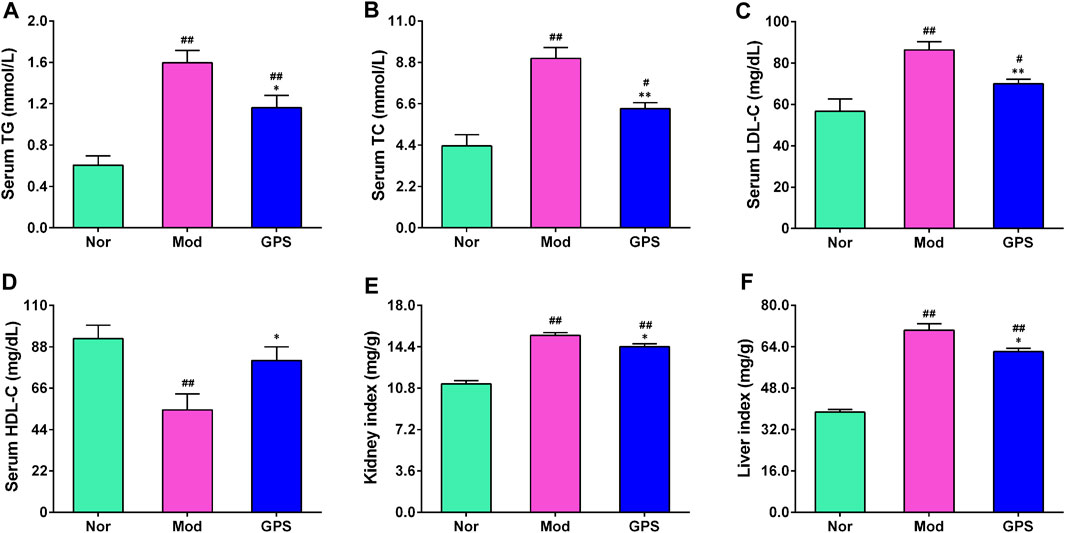
FIGURE 4. GPS ameliorated dyslipidemia in HFD and STZ-induced diabetic mice (A) Serum TG levels (B) Serum TC levels (C) Serum HDL-C levels (D) Serum LDL-C levels (E) Kidney index (F) Liver index. Data are presented as mean ± SEM, n = 10. #p < 0.05; ##p < 0.01 vs. Nor group; *p < 0.05; **p < 0.01 vs. Mod group.
3.5 GPS mitigated hepatic steatosis in HFD and STZ-induced diabetic mice
Hepatic steatosis plays a fundamental role in the occurrence and development of type 2 diabetes Lee et al., 2022a. Therefore, HE staining, PAS staining, and oil red staining were performed on the liver. As illustrated in Figure 5A, the results of the H&E staining demonstrated that, relative to the Nor group, hepatocytes of the Mod group were filled with many vacuolated round lipid droplets of different sizes, and that the hepatocytes were significantly swollen, damaged, disorganized, and infiltrated by inflammatory cells. After 7 weeks of treatment with GPS, the abnormal changes in the liver of diabetic mice were found to have improved evidently (Figure 5A). The results of PAS staining also showed that GPS significantly improved structural and morphological abnormalities, and increased glycogen accumulation in the liver of the diabetic mice (Figure 5A). The results of the oil red staining revealed that GPS treatment could remarkably decrease liver fat accumulation in diabetic mice (Figure 5A), and the quantitative analysis results of ImageJ further confirm this result (Figure 5B). Furthermore, GPS treatment also remarkably decreased the TG and TC levels in the liver of the diabetic mice (Figures 5C, D), and significantly weakened ALT and AST activities in the serum (Figures 5E, F). Together, the above results suggest that the improvement of type 2 diabetes symptoms caused by GPS might be associated with the decrease in hepatic steatosis in mice.
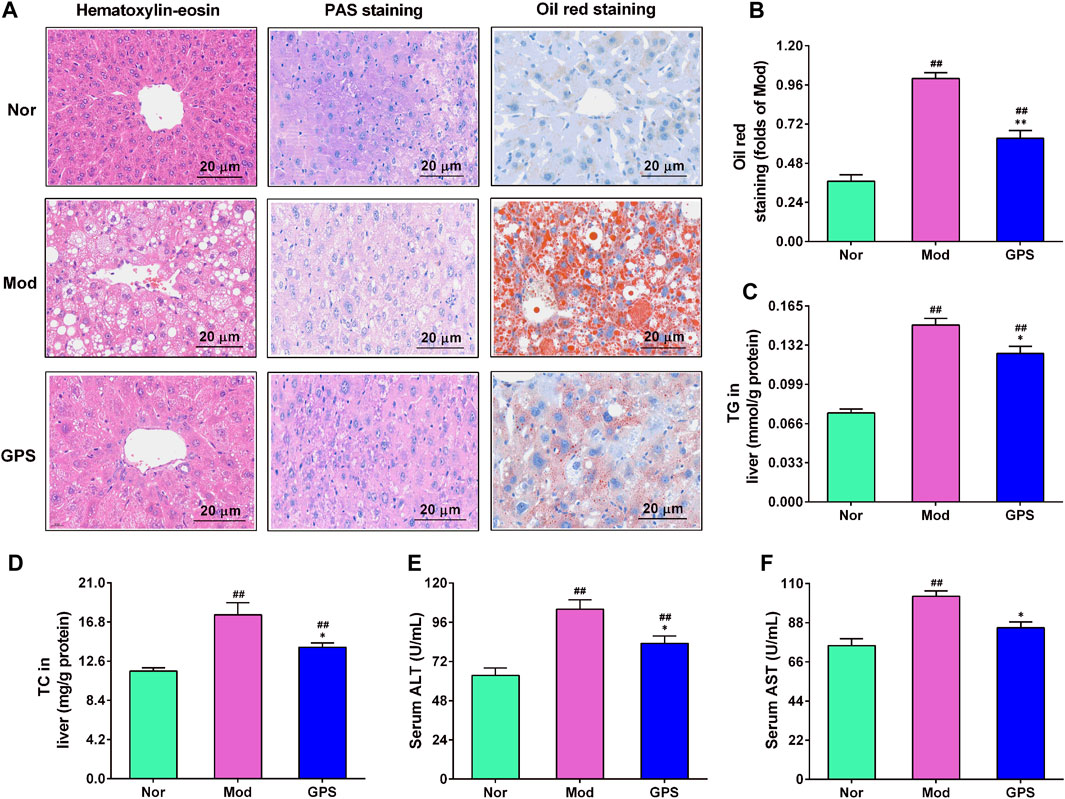
FIGURE 5. GPS mitigated hepatic steatosis in HFD and STZ-induced diabetic mice (A) Representative images of hematoxylin-eosin (HE), periodic acid-Schiff (PAS), and oil red staining of the liver of the diabetic mice, n = 4. Magnification of all images were at ×200 (B) Statistical analysis of oil red level, n = 4 (C) TG in the liver, n = 10 (D) TC in the liver, n = 10 (E) Serum ALT activities. N = 10 (F) Serum AST activities, n = 10. Data are presented as mean ± SEM. #p < 0.05; ##p < 0.01 vs. Nor group; *p < 0.05; **p < 0.01 vs. Mod group.
3.6 GPS inhibited hepatic gluconeogenesis by regulating the PI3K/AKT/FOXO1 signaling pathway in HFD and STZ-induced diabetic mice
To investigate whether GPS-induced improvements of the symptoms of type 2 diabetes were associated with the inhibition of gluconeogenesis, the expression levels of PEPCK and G6Pase were evaluated using immunohistochemistry and Western blotting assays. As shown in Figures 6A–C, the results of the Western blotting analysis illustrated that the protein expression levels of PEPCK and G6Pase in the liver of the Mod group mice were remarkably elevated, compared with the Nor group, and that 50 mg/kg GPS significantly suppressed the expression of PEPCK and G6Pase. The PI3K/AKT/FOXO1 signaling pathway is closely associated with gluconeogenesis. To evaluate whether the GPS-induced gluconeogenesis inhibition was associated with this signaling pathway, Western blotting analysis was performed to detect the expression levels of associated proteins in the liver of the diabetic mice. As illustrated in Figure 6A–G, relative to the Nor group mice, the protein levels of p-PI3K, p-AKT, and p-FOXO1 were remarkably downregulated, along with the upregulation of FOXO1 expression in liver of the Mod group mice. However, after 7 weeks of GPS treatment, the abnormal changes associated with this signaling pathway-related protein in liver of the diabetic mice had improved significantly, relative to the Mod group (Figure 6A–G). Collectively, all these data indicate that GPS significantly inhibits gluconeogenesis by regulating the PI3K/AKT/FOXO1 signaling pathway in liver of the diabetic mice, indicating that this may be the potential mechanism by which GPS treatment improves symptoms of type 2 diabetes.
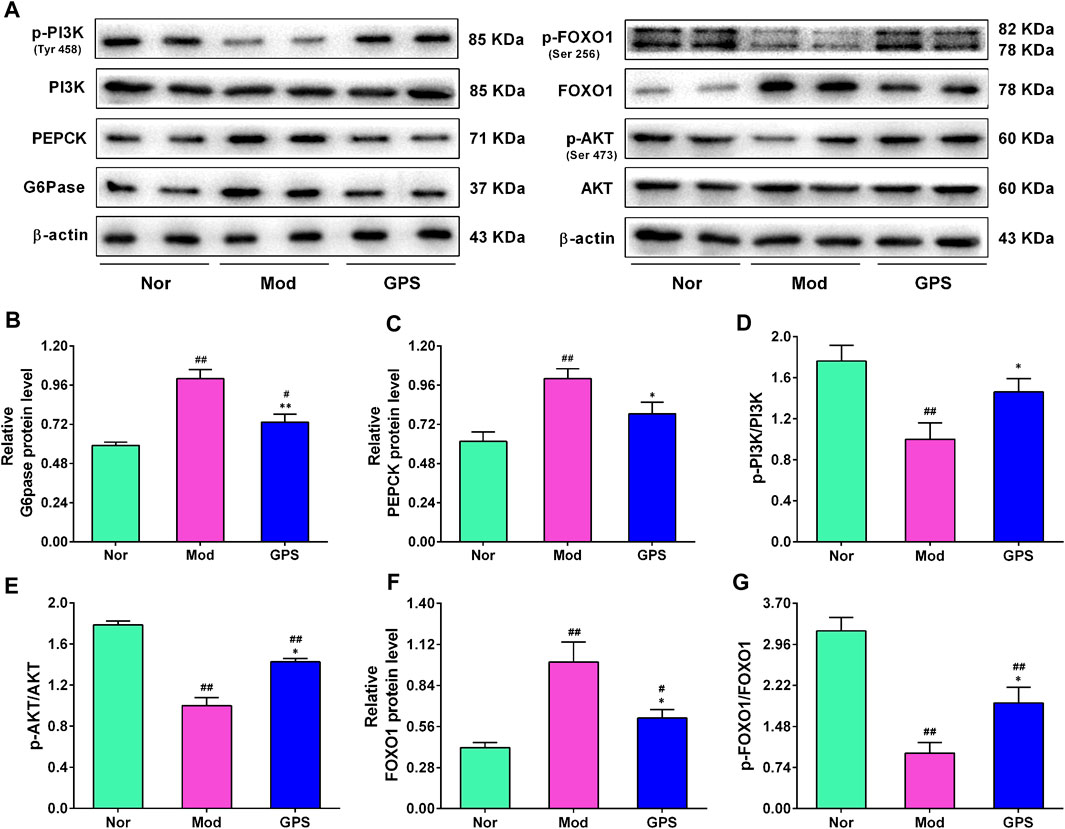
FIGURE 6. GPS inhibited hepatic gluconeogenesis by regulating the PI3K/AKT/FOXO1 signaling pathway in HFD and STZ-induced diabetic mice (A) Representative Western blotting analysis results of G6Pase, PEPCK, PI3K, p-PI3K, AKT, p-AKT, FOXO1, and p-FOXO1 (B) Relative optical density of G6Pase levels (C) Relative optical density of PEPCK levels (D) The ratio of p-PI3K/PI3K (E) The ratio of p-AKT/AKT (F) Relative optical density of FOXO1 expression (G) The ratio of p-FOXO1/FOXO1. Data are presented as mean ± SEM, n = 6. #p < 0.05; ##p < 0.01 vs. Nor group; *p < 0.05; **p < 0.01 vs. Mod group.
3.7 GPS enhanced the intestinal integrity in HFD and STZ-induced diabetic mice
To study whether GPS is beneficial for the intestinal barrier function of type 2 diabetic mice, the ileum of the mice were analyzed using immunohistochemistry. As illustrated in Figures 7A–C, relative to the Nor group, positive staining of ZO-1 and occludin in ileum of the Mod group were lighter. However, after 7 weeks of GPS treatment, the positive staining of these two indicators became darker, compared with the Mod group. To further verify the immunohistochemistry results, the protein expression of ZO-1 and occludin in the ileum were evaluated using Western blotting. The results demonstrated that GPS remarkably increased the protein expression of ZO-1 and occludin in the ileum of the diabetic mice, relative to the Mod group (Figures 7D–F), in agreement with results of the immunohistochemistry. Taken together, all these data indicate that GPS can help maintain the integrity of the intestinal barrier in type 2 diabetic mice.
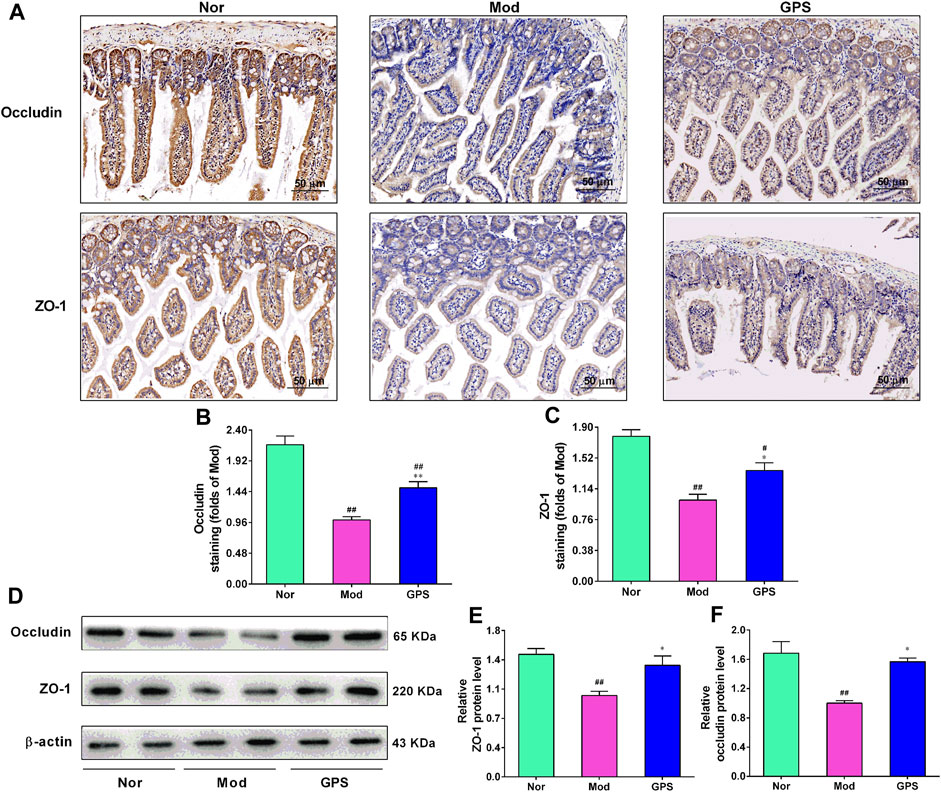
FIGURE 7. GPS enhanced intestinal integrity in HFD and STZ-induced diabetic mice (A) Representative images of immunohistochemical staining performed to determine ZO-1 and occludin expression levels in the ileum of the diabetic mice, n = 4. The magnification of all images is at × 200 (B) Statistical analysis of occluding protein expression, n = 4 (C) Statistical analysis of ZO-1 protein expression, n = 4 (D) Representative protein bands of ZO-1 and occludin, n = 6 (E) Relative optical density of ZO-1 levels, n = 6 (F) Relative optical density of occludin levels, n = 6. Data are presented as mean ± SEM. #p < 0.05; ##p < 0.01 vs. Nor group; *p < 0.05; **p < 0.01 vs. Mod group.
4 Discussion
T2DM is chiefly characterized by the decrease in insulin secretion or decreased sensitivity of target organs to insulin, which in turn leads to hyperglycemia, hyperlipidemia, and the increase in glucose production in the liver (Dao et al., 2023). Its typical clinical manifestations are polydipsia, polyphagia, polyuria, and weight loss. T2DM and its complications are one of the principal causes of morbidity and mortality worldwide and are currently on the rise, and have seriously affected the quality of life and imposed a very heavy economic burden on society (Faselis et al., 2020). At present, the main methods of treatment used for T2DM are insulin injection and the oral administration of one or more hypoglycemic agents (Ke et al., 2022). Although wide range of drugs with certain curative effects are available, the number of patients with T2DM continues to increase annually. Owing to the ineffective control of T2DM, new treatment strategies are increasingly needed. GPS can improve liver diseases, such as alcoholic liver disease (Li et al., 2018; Yang et al., 2020), cholestatic liver disease (Tang et al., 2016), and drug-induced liver injury (Han et al., 2018). In addition, other studies have found that GPS can alleviate diabetes (Xiao et al., 2022), diabetic retinopathy (Zhang et al., 2019), diabetic nephropathy (Xiao et al., 2020), and peripheral neuropathy (Lu et al., 2018). However, the metabolic pathway through which GPS improves T2DM and gluconeogenesis, and regulates gluconeogenesis remains has not been elucidated as yet. At present, the HFD/STZ-induced T2DM model had been widely used to study the activity and mechanism of various drugs for diabetes. In this study, we explored the potential effects and mechanism by which GPS treatment acts on gluconeogenesis in type 2 diabetic mice, and determined whether the inhibition of GPS during gluconeogenesis can be mediated by regulating the PI3K/AKT/FOXO1 signaling pathway.
It is well recognized that HFD combined with a low-dose STZ injection is sufficient to establish a model of insulin resistance with typical pathological features of T2DM (Srinivasan et al., 2005). In our study, the RBG, FBG, HbA1c, food intake, and water consumption of mice in the Mod group were found to be markedly upregulated than those in the Nor group, while the body weight of the mice was remarkably downregulated, relative to the Nor group following the injection of STZ. These characteristics are consistent with that of clinically diabetic patients. These characteristics may be a result of impaired energy metabolism and insufficient energy supply caused by body’s insufficient utilization of glucose, which leads to the increase in food and water consumption in the diabetic mice. In addition, abnormal glucose metabolism also leads to excessive consumption of muscle, fat, and protein tissues, which in turn leads to weight loss and a further increase in FBG. However, GPS treatment could discernibly improve these abnormal changes, which is in accordance with the results of previous studies (Xiao et al., 2022). Furthermore, the sensitivity of the liver, muscle, and fat of T2DM patients to insulin is reduced, and the ability of the body to handle glucose is also significantly reduced, indicating obvious signs of insulin resistance and abnormal glucose tolerance (Lee et al., 2022b). On the other hand, long-term hyperglycemia caused by the overloading of the pancreas, resulting in the secretion of an excessive quantity of insulin to maintain blood sugar, which leads to impaired pancreatic function and abnormal islet structure (Wolpin et al., 2013). To further study the effects of GPS on glucose metabolism and the pancreatic morphology in mice with type 2 diabetes, we determined the levels of the relevant indicators. The results showed that OGTT, ITT, and the pancreatic morphology of the mice in the GPS group had improved remarkably. These results indicate that GPS exerts a potential therapeutic effect on type 2 diabetes induced by HFD/STZ.
Dyslipidemia is very common among T2DM patients. It is usually one of the early manifestations of insulin resistance (IR), sometimes even before the diagnosis of diabetes. Therefore, IR in type 2 diabetes is relative to dyslipidemia (Bahiru et al., 2021). Moreover, IR and insulin secretion deficiency are the main causes of dyslipidemia in type 2 diabetes. When IR occurs, the synthesis of lipoprotein lipase in the body is reduced, which in turn reduces the hydrolysis of TG, which leads to lipid metabolism disorders (Kim and Song, 2022). In addition, high levels of TG require a higher level of insulin to compensate for the decrease in insulin sensitivity, which further aggravates IR, leading to a vicious circle (Fan et al., 2018). In this study, we observed a significant improvement in blood lipid disorders in the mice treated with GPS. The liver is a central organ for regulating lipid metabolism and maintaining energy homeostasis. If the lipid content exceeds the metabolic capacity of the liver, lipid is stored in the liver in the form of lipid droplets, which may cause long-term liver inflammation, insulin resistance, and diabetes (Heeren and Scheja, 2021). However, persistent hyperglycemia promotes abnormal TG deposition in the liver cells, which further promotes liver steatosis (Zhang et al., 2018). Therefore, we also investigated the effect of GPS treatment on liver steatosis and found that GPS could significantly slow down lipid accumulation in the liver of the type 2 diabetic mice. However, we failed to investigate the mechanism through which GPS improves hepatic steatosis and will be examined through further research in the future.
The pathophysiological mechanism that leads to elevated blood glucose involves a variety of tissues and cells, as the liver is very closely associated with T2DM. Among these mechanisms, the increase in the production of endogenous glucose through gluconeogenesis is regarded as one of the main causes of high FBG in T2DM (Liu et al., 2021). At present, a large number of studies have shown that inhibition of liver gluconeogenesis is effective in reducing blood glucose, which is also a reliable direction for the development of anti-diabetic drugs (Petersen et al., 2017). During fasting, blood glucose is mainly derived from gluconeogenesis and glycogen decomposition, while in patients with T2DM with long-term fasting, blood glucose is mainly derived from gluconeogenesis, indicating that gluconeogenesis plays a crucial role in the development of T2DM (Rizza, 2010). In our PTT experiment, the gluconeogenesis of mice in the Mod group increased significantly, while GPS treatment could significantly inhibit gluconeogenesis. Therefore, there is a need to better understand the molecular mechanism underlying the inhibition of gluconeogenesis induced by GPS. The PI3K/Akt pathway is one of the most important signal transduction pathways that operate under the action of insulin (Huang et al., 2018). The PI3K signaling molecule regulates glucose uptake and metabolism by regulating glycogen synthesis and gluconeogenesis, while dysfunctional PI3K leads to an imbalance in glucose metabolism (Zhu et al., 2023). AKT is a direct downstream molecule regulated by PI3K. It is also a key enzyme for regulating glucose metabolism. AKT can be directly activated by insulin to mediate various biological effects associated with glucose metabolism (Sun et al., 2019). FOXO1 is a transcription factor that plays a fundamental role in the regulation of HGP and insulin sensitivity. Studies have shown that elevated FOXO1 expression in the liver may lead to an increase in FBG in mice (Van Berendoncks et al., 2015), while the inhibition of FOXO1 expression in the liver may delay the progression of diabetes and fatty liver (Herder et al., 2016). G6Pase and PEPCK are rate-limiting enzymes that are involved in gluconeogenesis (Zhu et al., 2021). PEPCK catalyzes oxaloacetate to phosphoenolpyruvate, and then converts it to glucose. The function of G6Pase is to hydrolyze glucose 6 phosphate into glucose and inorganic phosphate, and the glucose is finally transported by transporters (Sun et al., 2021). In T2DM, PI3K/Akt activity is inhibited, and the gene expression of gluconeogenesis-related genes (PEPCK and G6Pase), is enhanced by FOXO1. In this study, GPS treatment was shown to increase the expression of p-PI3K, p-AKT, and p-FOXO1 at protein level, and also to inhibit the expression of PEPCK and G6Pase at protein level in the liver of diabetic mice, indicating that GPS treatment may be a viable manner of improving IR and hyperglycemia by regulating the PI3K/AKT/FOXO1 signaling pathway to inhibit gluconeogenesis.
T2DM and intestinal permeability interact with each other. On the one hand, the ecological imbalance of intestinal microflora and T2DM will lead to the impairment of intestinal permeability (Rehman et al., 2022). On the other hand, the increased intestinal permeability will promote the progress of T2DM (Cao et al., 2020). Moreover, as important structural proteins with tight junction, ZO-1 and occludin play important roles in maintaining intestinal permeability and regulating intestinal barrier function (Sharma and Tripathi, 2019). ZO-1 and occludin block proteins and cytoskeletons by connecting tight junction proteins. When abnormally expressed, these will damage the intestinal mechanical barrier and promote the occurrence and development of diseases (König et al., 2016). Furthermore, it has also been found that T2DM is usually accompanied by increased intestinal permeability, as evidenced by decreased protein of tight junction (Xu et al., 2019; Cao et al., 2020; Rehman et al., 2022). Herein, we found that GPS significantly increased the expression of protein ZO-1 and occludin in the ileum of diabetic mice, indicating that GPS may have an impact on intestinal flora of diabetic mice. However, we have not deeply studied the reason why GPS reduces intestinal permeability. Therefore, future study will thus be focused on clarification of the echanism of GPS in improving T2DM from intestinal permeability.
There remain certain limitations for our research. Firstly, the effects of GPS on the proteins and genes related to insulin resistance and fat accumulation in the liver of the HFD and STZ-induced diabetic mice were not studied, which made the research in this field lacking. Secondly, muscle and adipose tissue are the target organs of insulin and also play a pivotal role in maintaining glucose homeostasis. However, we did not investigate the effects of GPS on these two organs. Thirdly, the effect of GPS on gluconeogenesis and the possible mechanism were not studied in the corresponding cell model. In addition, when the research was carried out on HFD and STZ-induced diabetic mice, there were no positive control drugs and more dose groups, and the effects of GPS on all aspects of normal mice at such dose were not investigated. It would be more convincing and more meaningful if supported by these relevant data. Lastly, we found that GPS played a certain role in improving the intestinal barrier, but we did not explore the possible mechanisms including the intestinal flora and intestinal microecology. Nevertheless, further investigation of DPDS is highly desirable because it has many known pharmacological effects and unknown pharmacological effects that warrant further investigation. Moreover, further research is needed to better understand the protective mechanism of GPS for T2DM.
In summary, our findings clearly indicated that GPS treatment can improve symptoms of the type 2 diabetic mice to a certain extent. The underlying mechanism of action involved may be that GPS induces the enhancement of the phosphorylation of FOXO1 by activating PI3K/AKT in the liver of diabetic mice, and to further inhibit the expression levels of G6Pase and PEPCK, thus inhibiting gluconeogenesis, and may also be related to the enhancement of intestinal integrity (Figure 8). The findings of this study provide a direct experimental basis for the role and potential mechanism by which GPS exerts its anti-T2DM effects. Although GPS has been found to have a variety of pharmacological effects, it is still a long way from clinical application.
Data availability statement
The raw data supporting the conclusion of this article will be made available by the authors, without undue reservation.
Ethics statement
The animal study was reviewed and approved by the Ethics Committee of North Sichuan Medical College.
Author contributions
XW designed and conducted the research, analyzed the data, and wrote the manuscript. DL and XH participated in experiments. NG supervised the whole project, supplied academic and technical support, and reviewed the manuscript. All authors contributed to the article and approved the submitted version.
Funding
The present study was supported by Youth Science Fund of Sichuan Natural Science Foundation (No. 2023NSFSC1676), the Municipal-school Cooperative Scientific Research Project (No. 22SXZRKX0012), the Innovation and Entrepreneurship Training Program for College Students in Sichuan Province (No. 202210634012), the North Sichuan Medical College PhD Research Fund (CBY21-QD16), and the Shanghai Sailing Program (No. 22YF1439800).
Conflict of interest
The authors declare that the research was conducted in the absence of any commercial or financial relationships that could be construed as a potential conflict of interest.
Publisher’s note
All claims expressed in this article are solely those of the authors and do not necessarily represent those of their affiliated organizations, or those of the publisher, the editors and the reviewers. Any product that may be evaluated in this article, or claim that may be made by its manufacturer, is not guaranteed or endorsed by the publisher.
References
Ahmad, E., Lim, S., Lamptey, R., Webb, D. R., and Davies, M. J. (2022). Type 2 diabetes. Lancet 400, 1803–1820. doi:10.1016/S0140-6736(22)01655-5
Bahiru, E., Hsiao, R., Phillipson, D., and Watson, K. E. (2021). Mechanisms and treatment of dyslipidemia in diabetes. Curr. Cardiol. Rep. 23, 26. doi:10.1007/s11886-021-01455-w
Cao, H., Li, C., Lei, L., Wang, X., Liu, S., Liu, Q., et al. (2020). Stachyose improves the effects of berberine on glucose metabolism by regulating intestinal microbiota and short-chain fatty acids in spontaneous type 2 diabetic KKAy mice. Front. Pharmacol. 11, 578943. doi:10.3389/fphar.2020.578943
Dao, L., Choi, S., and Freeby, M. (2023). Type 2 diabetes mellitus and cognitive function: Understanding the connections. Curr. Opin. Endocrinol. Diabetes. Obes. 30, 7–13. doi:10.1097/MED.0000000000000783
Fan, Y., He, Z., Wang, W., Li, J., Hu, A., Li, L., et al. (2018). Tangganjian decoction ameliorates type 2 diabetes mellitus and nonalcoholic fatty liver disease in rats by activating the IRS/PI3K/AKT signaling pathway. Biomed. Pharmacother. 106, 733–737. doi:10.1016/j.biopha.2018.06.089
Faselis, C., Katsimardou, A., Imprialos, K., Deligkaris, P., Kallistratos, M., and Dimitriadis, K. (2020). Microvascular complications of type 2 diabetes mellitus. Curr. Vasc. Pharmacol. 18, 117–124. doi:10.2174/1570161117666190502103733
Fujimoto, M., Yokoyama, M., Kiuchi, M., Hosokawa, H., Nakayama, A., Hashimoto, N., et al. (2022). Liver group 2 innate lymphoid cells regulate blood glucose levels through IL-13 signaling and suppression of gluconeogenesis. Nat. Commun. 13, 5408. doi:10.1038/s41467-022-33171-6
Giralt, A., Denechaud, P. D., Lopez-Mejia, I. C., Delacuisine, B., Blanchet, E., Bonner, C., et al. (2018). E2F1 promotes hepatic gluconeogenesis and contributes to hyperglycemia during diabetes. Mol. Metab. 11, 104–112. doi:10.1016/j.molmet.2018.02.011
Goldberg, R. B. (2022). Dyslipidemia in diabetes: When and how to treat? Endocrinol. Metab. Clin. North. Am. 51, 603–624. doi:10.1016/j.ecl.2022.02.011
Han, H., Xu, L., Xiong, K., Zhang, T., and Wang, Z. (2018). Exploration of hepatoprotective effect of gentiopicroside on alpha-naphthylisothiocyanate-induced cholestatic liver injury in rats by comprehensive proteomic and metabolomic signatures. Cell. Physiol. biochem. 49, 1304–1319. doi:10.1159/000493409
Heeren, J., and Scheja, L. (2021). Metabolic-associated fatty liver disease and lipoprotein metabolism. Mol. Metab. 50, 101238. doi:10.1016/j.molmet.2021.101238
Herder, C., Færch, K., Carstensen-Kirberg, M., Lowe, G. D., Haapakoski, R., Witte, D. R., et al. (2016). Biomarkers of subclinical inflammation and increases in glycaemia, insulin resistance and beta-cell function in non-diabetic individuals: The whitehall II study. Eur. J. Endocrinol. 175, 367–377. doi:10.1530/EJE-16-0528
Huang, X., Liu, G., Guo, J., and Su, Z. (2018). The PI3K/AKT pathway in obesity and type 2 diabetes. Int. J. Biol. Sci. 14, 1483–1496. doi:10.7150/ijbs.27173
Jin, M., Feng, H., Wang, Y., Yan, S., Shen, B., Li, Z., et al. (2020). Gentiopicroside ameliorates oxidative stress and lipid accumulation through nuclear factor erythroid 2-related factor 2 activation. Oxid. Med. Cell. Longev. 2020, 2940746. doi:10.1155/2020/2940746
Ke, C., Narayan, K. M. V., Chan, J. C. N., Jha, P., and Shah, B. R. (2022). Pathophysiology, phenotypes and management of type 2 diabetes mellitus in Indian and Chinese populations. Nat. Rev. Endocrinol. 18, 413–432. doi:10.1038/s41574-022-00669-4
Kim, H. K., and Song, J. (2022). Hypothyroidism and diabetes-related dementia: Focused on neuronal dysfunction, insulin resistance, and dyslipidemia. Int. J. Mol. Sci. 23, 2982. doi:10.3390/ijms23062982
König, J., Wells, J., Cani, P. D., García-Ródenas, C. L., MacDonald, T., Mercenier, A., et al. (2016). Human intestinal barrier function in health and disease. Clin. Transl. Gastroenterol. 7, e196. doi:10.1038/ctg.2016.54
Laakso, M., and Fernandes, S. L. (2022). Genetics of type 2 diabetes: Past, present, and future. Nutrients 14, 3201. doi:10.3390/nu14153201
Lee, C. H., Lui, D. T., and Lam, K. S. (2022a). Non-alcoholic fatty liver disease and type 2 diabetes: An update. J. Diabetes. Investig. 13, 930–940. doi:10.1111/jdi.13756
Lee, S. H., Park, S. Y., and Choi, C. S. (2022b). Insulin resistance: From mechanisms to therapeutic strategies. Diabetes. Metab. J. 46, 15–37. doi:10.4093/dmj.2021.0280
Li, C., Yang, M., Hou, G., Liu, S., Huan, Y., Yu, D., et al. (2017). A human glucagon-like peptide-1-albumin recombinant protein with prolonged hypoglycemic effect provides efficient and beneficial control of glucose metabolism in diabetic mice. Biol. Pharm. Bull. 40, 1399–1408. doi:10.1248/bpb.b17-00169
Li, X., Zhang, Y., Jin, Q., Xia, K. L., Jiang, M., Cui, B. W., et al. (2018). Liver kinase B1/AMP-activated protein kinase-mediated regulation by gentiopicroside ameliorates P2X7 receptor-dependent alcoholic hepatosteatosis. Br. J. Pharmacol. 175, 1451–1470. doi:10.1111/bph.14145
Liang, J. Q., Liu, C., Zhang, W. X., and Chen, S. Y. (2022). Interaction between hepatokines and metabolic diseases. Yi Chuan 44, 853–866. doi:10.16288/j.yczz.22-218
Liu, T. Y., Shi, C. X., Gao, R., Sun, H. J., Xiong, X. Q., Ding, L., et al. (2015). Irisin inhibits hepatic gluconeogenesis and increases glycogen synthesis via the PI3K/Akt pathway in type 2 diabetic mice and hepatocytes. Clin. Sci. (Lond). 129, 839–850. doi:10.1042/CS20150009
Liu, Y., Qiu, Y., Chen, Q., Han, X., Cai, M., and Hao, L. (2021). Puerarin suppresses the hepatic gluconeogenesis via activation of PI3K/Akt signaling pathway in diabetic rats and HepG2 cells. Biomed. Pharmacother. 137, 111325. doi:10.1016/j.biopha.2021.111325
Lu, Y., Yao, J., Gong, C., Wang, B., Zhou, P., Zhou, S., et al. (2018). Gentiopicroside ameliorates diabetic peripheral neuropathy by modulating PPAR- Γ/AMPK/ACC signaling pathway. Cell. Physiol. biochem. 50, 585–596. doi:10.1159/000494174
Magliano, D. J., and Boyko, E. J. (2021). IDF diabetes atlas 10th edition scientific committee. IDF DIABETES ATLAS. https://www.ncbi.nlm.nih.gov/books/NBK581934/.
Petersen, M. C., Vatner, D. F., and Shulman, G. I. (2017). Regulation of hepatic glucose metabolism in health and disease. Nat. Rev. Endocrinol. 13, 572–587. doi:10.1038/nrendo.2017.80
Rehman, A. U., Siddiqui, N. Z., Farooqui, N. A., Alam, G., Gul, A., Ahmad, B., et al. (2022). Morchella esculenta mushroom polysaccharide attenuates diabetes and modulates intestinal permeability and gut microbiota in a type 2 diabetic mice model. Front. Nutr. 9, 984695. doi:10.3389/fnut.2022.984695
Rizza, R. A. (2010). Pathogenesis of fasting and postprandial hyperglycemia in type 2 diabetes: Implications for therapy. Diabetes 59, 2697–2707. doi:10.2337/db10-1032
Sharma, S., and Tripathi, P. (2019). Gut microbiome and type 2 diabetes: Where we are and where to go? J. Nutr. Biochem. 63, 101–108. doi:10.1016/j.jnutbio.2018.10.003
Srinivasan, K., Viswanad, B., Asrat, L., Kaul, C. L., and Ramarao, P. (2005). Combination of high-fat diet-fed and low-dose streptozotocin-treated rat: A model for type 2 diabetes and pharmacological screening. Pharmacol. Res. 52, 313–320. doi:10.1016/j.phrs.2005.05.004
Sun, H., Liu, X., Long, S. R., Teng, W., Ge, H., Wang, Y., et al. (2019). Antidiabetic effects of pterostilbene through PI3K/Akt signal pathway in high fat diet and STZ-induced diabetic rats. Eur. J. Pharmacol. 859, 172526. doi:10.1016/j.ejphar.2019.172526
Sun, H., Saeedi, P., Karuranga, S., Pinkepank, M., Ogurtsova, K., Duncan, B. B., et al. (2022). IDF Diabetes Atlas: Global, regional and country-level diabetes prevalence estimates for 2021 and projections for 2045. Diabetes Res. Clin. Pract. 183, 109119. doi:10.1016/j.diabres.2021.109119
Sun, X., Cao, Z., Ma, Y., Shao, Y., Zhang, J., Yuan, G., et al. (2021). Resveratrol attenuates dapagliflozin-induced renal gluconeogenesis via activating the PI3K/Akt pathway and suppressing the FoxO1 pathway in type 2 diabetes. Food Funct. 12, 1207–1218. doi:10.1039/d0fo02387f
Tang, X., Yang, Q., Yang, F., Gong, J., Han, H., Yang, L., et al. (2016). Target profiling analyses of bile acids in the evaluation of hepatoprotective effect of gentiopicroside on ANIT-induced cholestatic liver injury in mice. J. Ethnopharmacol. 194, 63–71. doi:10.1016/j.jep.2016.08.049
Tseng, Y. H., Chang, C. W., Chiang, W., and Hsieh, S. C. (2019). Adlay bran oil suppresses hepatic gluconeogenesis and attenuates hyperlipidemia in type 2 diabetes rats. J. Med. Food. 22, 22–28. doi:10.1089/jmf.2018.4237
Van Berendoncks, A. M., Stensvold, D., Garnier, A., Fortin, D., Sente, T., Vrints, C. J., et al. (2015). Disturbed adiponectin - AMPK system in skeletal muscle of patients with metabolic syndrome. Eur. J. Prev. Cardiol. 22, 203–205. doi:10.1177/2047487313508034
Wang, G., Song, J., Huang, Y., et al. (2022). Lactobacillus plantarum SHY130 isolated from yak yogurt attenuates hyperglycemia in C57BL/6J mice by regulating the enteroinsular axis. Food Funct. 13 (2), 675–687. doi:10.1039/d1fo02387j
Wolpin, B. M., Bao, Y., Qian, Z. R., Wu, C., Kraft, P., Ogino, S., et al. (2013). Hyperglycemia, insulin resistance, impaired pancreatic β-cell function, and risk of pancreatic cancer. J. Natl. Cancer. Inst. 105, 1027–1035. doi:10.1093/jnci/djt123
Wu, F., Shao, Q., Xia, Q., Hu, M., Zhao, Y., Wang, D., et al. (2021). A bioinformatics and transcriptomics based investigation reveals an inhibitory role of Huanglian-Renshen-Decoction on hepatic glucose production of T2DM mice via PI3K/Akt/FoxO1 signaling pathway. Phytomedicine 83, 153487. doi:10.1016/j.phymed.2021.153487
Wu, S., Ning, Y., Zhao, Y., Sun, W., Thorimbert, S., Dechoux, L., et al. (2017). Research progress of natural product gentiopicroside - a secoiridoids compound. Mini. Rev. Med. Chem. 17, 62–77. doi:10.2174/1389557516666160624124127
Xiao, H., Sun, X., Lin, Z., Yang, Y., Zhang, M., Xu, Z., et al. (2022). Gentiopicroside targets PAQR3 to activate the PI3K/AKT signaling pathway and ameliorate disordered glucose and lipid metabolism. Acta. Pharm. Sin. B 12, 2887–2904. doi:10.1016/j.apsb.2021.12.023
Xiao, H., Sun, X., Liu, R., Chen, Z., Lin, Z., Yan, g. Y., et al. (2020). Gentiopicroside activates the bile acid receptor Gpbar1 (TGR5) to repress NF-kappaB pathway and ameliorate diabetic nephropathy. Pharmacol. Res. 151, 104559. doi:10.1016/j.phrs.2019.104559
Xie, B., Zu, X., Wang, Z., Xu, X., Liu, G., and Liu, R. (2022). Ginsenoside Rc ameliorated atherosclerosis via regulating gut microbiota and fecal metabolites. Front. Pharmacol. 13, 990476. doi:10.3389/fphar.2022.990476
Xing, S., Nong, F., Qin, J., Huang, H., Zhan, R., and Chen, W. (2021). Gentiopicroside produces endothelium-independent vasodilation by deactivating the PI3K/Akt/Rho-Kinase pathway in isolated rat thoracic aorta. Biomed. Res. Int. 2021, 5565748. doi:10.1155/2021/5565748
Xu, J., Liang, R., Zhang, W., Tian, K., Li, J., Chen, X., et al. (2019). Faecalibacterium prausnitzii-derived microbial anti-inflammatory molecule regulates intestinal integrity in diabetes mellitus mice via modulating tight junction protein expression. J. Diabetes 12, 224–236. doi:10.1111/1753-0407.12986
Xu, Z., Zhang, M., Wang, Y., Chen, R., Xu, S., Sun, X., et al. (2022). Gentiopicroside ameliorates diabetic renal tubulointerstitial fibrosis via inhibiting the AT1R/CK2/NF-κB pathway. Front. Pharmacol. 13, 848915. doi:10.3389/fphar.2022.848915
Yan, H., Yang, W., Zhou, F., Li, X., Pan, Q., Shen, Z., et al. (2019). Estrogen improves insulin sensitivity and suppresses gluconeogenesis via the transcription factor foxo1. Diabetes 68, 291–304. doi:10.2337/db18-0638
Yan, J., Wang, C., Jin, Y., Meng, Q., Liu, Q., Liu, Z., et al. (2018). Catalpol ameliorates hepatic insulin resistance in type 2 diabetes through acting on AMPK/NOX4/PI3K/AKT pathway. Pharmacol. Res. 130, 466–480. doi:10.1016/j.phrs.2017.12.026
Yang, H. X., Shang, Y., Jin, Q., Wu, Y. L., Liu, J., Qiao, C. Y., et al. (2020). Gentiopicroside ameliorates the progression from hepatic steatosis to fibrosis induced by chronic alcohol intake. Biomol. Ther. Seoul. 28, 320–327. doi:10.4062/biomolther.2020.008
Yang, S. Q., Chen, Y. D., Li, H., Hui, X., and Gao, W. Y. (2018). Geniposide and gentiopicroside suppress hepatic gluconeogenesis via regulation of AKT-FOXO1 pathway. Arch. Med. Res. 49, 314–322. doi:10.1016/j.arcmed.2018.10.005
Yin, G. W., Zheng, L., Guo, Y. L., and Hong, J. W. (2022). Research progress of active ingredients of Scutellaria baicalensis in the treatment of type 2 diabetes and its complications. Biomed. Pharmacother. 148, 112690. doi:10.1016/j.biopha.2022.112690
Zhang, C., Deng, J., Liu, D., Tuo, X., Xiao, L., Lai, B., et al. (2018). Nuciferine ameliorates hepatic steatosis in high-fat diet/streptozocin-induced diabetic mice through a PPARα/PPARγ coactivator-1α pathway. Br. J. Pharmacol. 175, 4218–4228. doi:10.1111/bph.14482
Zhang, X., Shi, E., Yang, L., Fu, W., and Zhou, X. (2019). Gentiopicroside attenuates diabetic retinopathy by inhibiting inflammation, oxidative stress, and NF-κB activation in rat model. Eur. J. Inflamm. 17, 205873921984783. doi:10.1177/2058739219847837
Zhong, C., Qiu, S., Li, J., Shen, J., Zu, Y., Shi, J., et al. (2019). Ellagic acid synergistically potentiates inhibitory activities of chemotherapeutic agents to human hepatocellular carcinoma. Phytomedicine 59, 152921. doi:10.1016/j.phymed.2019.152921
Zhu, X., He, Y., Zhang, Q., Ma, D., and Zhao, H. (2023). Lead induced disorders of lipid metabolism and glycometabolism in the liver of developmental Japanese quails (Coturnix japonica) via inhibiting PI3K/Akt signaling pathway. Comp. Biochem. Physiol. C. Toxicol. Pharmacol. 263, 109489. doi:10.1016/j.cbpc.2022.109489
Zhu, Y. X., Hu, H. Q., Zuo, M. L., Mao, L., Song, G. L., Li, T. M., et al. (2021). Effect of oxymatrine on liver gluconeogenesis is associated with the regulation of PEPCK and G6Pase expression and AKT phosphorylation. Biomed. Rep. 15, 56. doi:10.3892/br.2021.1432
Keywords: gentiopicroside, type 2 diabetes mellitus, hepatic gluconeogenesis, PI3K/akt pathway, FOxO1
Citation: Wang X, Long D, Hu X and Guo N (2023) Gentiopicroside modulates glucose homeostasis in high-fat-diet and streptozotocin-induced type 2 diabetic mice. Front. Pharmacol. 14:1172360. doi: 10.3389/fphar.2023.1172360
Received: 23 February 2023; Accepted: 30 June 2023;
Published: 04 August 2023.
Edited by:
Åke Sjöholm, Gävle Hospital, SwedenReviewed by:
Waseem El-Huneidi, University of Sharjah, United Arab EmiratesYifan Bao, Johnson and Johnson, United States
Copyright © 2023 Wang, Long, Hu and Guo. This is an open-access article distributed under the terms of the Creative Commons Attribution License (CC BY). The use, distribution or reproduction in other forums is permitted, provided the original author(s) and the copyright owner(s) are credited and that the original publication in this journal is cited, in accordance with accepted academic practice. No use, distribution or reproduction is permitted which does not comply with these terms.
*Correspondence: Nan Guo, guon@fudan.edu.cn