- 1Department of Orthopedics, Shengjing Hospital of China Medical University, Shenyang, China
- 2Department of Clinical Epidemiology, Shengjing Hospital of China Medical University, Shenyang, China
Osteoarthritis (OA) is one of the most common degenerative joint diseases, often involving the entire joint. The degeneration of articular cartilage is an important feature of OA, and there is growing evidence that the mitochondrial biogenesis master regulator peroxisome proliferator-activated receptor γ coactivator 1α (PGC-1α) exert a chondroprotective effect. PGC-1α delays the development and progression of OA by affecting mitochondrial biogenesis, oxidative stress, mitophagy and mitochondrial DNA (mtDNA) replication in chondrocytes. In addition, PGC-1α can regulate the metabolic abnormalities of OA chondrocytes and inhibit chondrocyte apoptosis. In this paper, we review the regulatory mechanisms of PGC-1α and its effects on OA chondrocytes, and introduce potential drugs and novel nanohybrid for the treatment of OA which act by affecting the activity of PGC-1α. This information will help to further elucidate the pathogenesis of OA and provide new ideas for the development of therapeutic strategies for OA.
1 Introduction
Osteoarthritis (OA) is the most common degenerative disease affecting the entire joint. The global age-standardized incidence rate (ASIR) of OA is increasing by 0.32% per year, an increase of about 9% in 28 years (Quicke et al., 2022), and its prevalence is estimated to double in the next 30 years. There are approximately 500 million people with OA worldwide, with an aging population and increasing obesity, more older adults will be disabled by OA (Hunter et al., 2020). OA is primarily characterized by pathological changes in articular cartilage, bone, synovium, ligaments, muscles and periarticular fat, resulting in joint dysfunction, pain, and functional limitations (Katz et al., 2021). Several drugs have shown therapeutic potential, but few have demonstrated the ability to arrest or slow the progression of OA (Abramoff and Caldera, 2020). Arthroplasty is an effective treatment for symptomatic end-stage OA, but suffers from a poor functional prognosis following functional surgery and a limited prosthetic life span (Glyn-Jones et al., 2015).
Healthy articular cartilage is a special type of hyaline cartilage, 2–4 mm thick, without blood or lymphatic vessels or nerves (Sophia Fox et al., 2009). Chondrocytes are the only cell type present in articular cartilage, infiltrating in the extracellular matrix (ECM) (Liu et al., 2018), which is rich in type II collagen and proteoglycans, and contributing to resisting compressive loads (Guilak et al., 2018). Loss of ECM and death of chondrocytes have been shown to be central features of articular cartilage degeneration (Pascarelli et al., 2015). Chondrocyte changes are an important feature in the pathogenesis of OA, but the mechanisms of cartilage destruction and loss of joint function in OA are not fully understood (Pascarelli et al., 2015).
Normal metabolism is closely related to chondrocyte physiology, in healthy joints, chondrocytes are in a state of physiological and metabolic homeostasis (Bai et al., 2019). Since articular cartilage is avascular, chondrocytes receive nutrients and oxygen primarily through low-rate diffusion through the ECM (Guilak et al., 2018; He et al., 2020). However, aerobic glycolysis is known to coexist with anaerobic glycolysis in normal chondrocytes (Hollander and Zeng, 2019). Chondrocytes take up glucose via specific glucose transporter proteins to maintain a stable energy metabolism. In addition, Cholesterol biosynthesis has been demonstrated in animal experiments to be elevated during normal growth plate cartilage formation in rats, and this effect has also been found in human cartilage (Aguilar et al., 2009; Bernstein et al., 2010; Yang et al., 2021a). Chondrocytes are also able to sense and transport lipoproteins to regulate lipid homeostasis in cartilage and maintain the ability of chondrocytes to perform their physiological functions (Villalvilla et al., 2013).
The mitochondrial biogenesis master regulator peroxisome proliferator-activated receptor ? cofactor 1α (PGC-1α) is a 91 kDa transcription factor (Cheng et al., 2018) that is responsible for chondrocyte mitochondrial quality control (MQC), mtDNA expression, oxidative stress and metabolism, exerting a chondroprotective effect. Recent studies have shown that the expression and activity of PGC-1α are decreased in OA chondrocytes, which may be associated with degenerative changes in these cells (Wang et al., 2015).
This paper reviews the research on the effects of PGC-1α on OA chondrocytes, as well as the drugs and novel nanohybrid which may be useful for the treatment of OA by affecting PGC-1α activity, thus providing new ideas for further research into the pathogenesis, prevention and treatment of OA.
2 Chondrocyte cell death and OA
Cell death can occur in different ways and can be divided into programmed and non-programmed forms depending on the regulatory process involved (Yang et al., 2021b). Apoptosis is a highly-regulated, active process of programmed cell death and is involved in development. Apoptosis is an important process in the occurrence and development of OA, and the death of chondrocytes caused by apoptosis is positively correlated with the severity of OA (Komori, 2016). Recent studies have revealed several other types of cell death, including autophagy and ferroptosis (Sasaki et al., 2012). Ferroptosis, a newly discovered mode of programmed cell death caused by iron-dependent lipid peroxidation, has been shown to be involved in the pathogenic process of OA (Yao et al., 2021; Miao et al., 2022). In addition, most studies have proved that pyroptosis and necroptosis may be related to cartilage damage in OA (Riegger and Brenner, 2019; An et al., 2020), but the relationship between these special cell death modes and OA needs further research.
3 Regulatory pathways and molecules of PGC-1α in OA chondrocytes
3.1 AMPK/SIRT1 pathway
AMP-activated protein kinase (AMPK) is a heterotrimeric complex comprising a catalytic subunit a and two regulatory subunits ß and γ (Yan et al., 2018). As an important regulator of energy homeostasis, AMPK responds to changes in the ratio of ATP to AMP by regulating metabolic enzymes to promote ATP production and inhibit ATP consumption (Herzig and Shaw, 2018). AMPK is a recognized upstream regulator of PGC-1α, which can directly affect the activity of PGC-1α through phosphorylation (Yao et al., 2023) (Figure 1). Silent information regulator 1 (SIRT1) is a histone deacetylase that maintains cartilage homeostasis by promoting chondrocyte proliferation, differentiation and survival, and upregulating genes important for cartilage function (Almeida and Porter, 2019). SIRT1 can affect the activity of PGC-1α through acetylation, and AMPK enhances SIRT1 activity by increasing cellular NAD+ levels, and further affects the activity of PGC-1α (Cantó and Auwerx, 2009; Zheng et al., 2020) (Figure 1). PGC-1α is a master regulator of mitochondrial biogenesis and function (Wang et al., 2015), and promotes mitochondrial transcription factor A (TFAM) expression by increasing downstream transcription of nuclear respiratory factor 1 (NRF1) and nuclear respiratory factor 2 (NRF2), thereby exerting the effects of TFAM in promoting mitochondrial biogenesis and mtDNA replication (Zhang et al., 2018a).
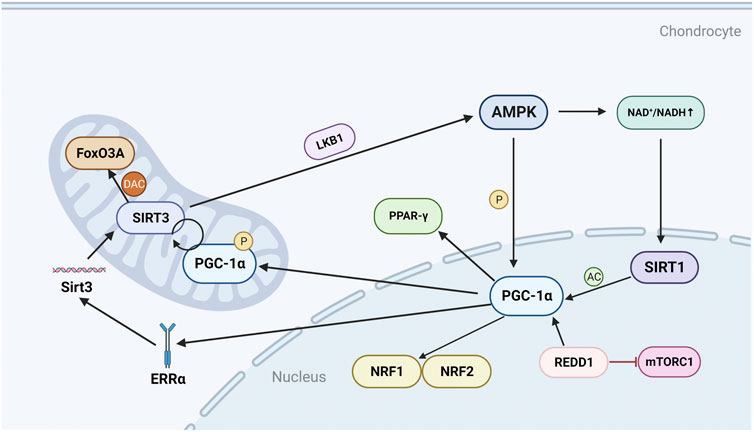
FIGURE 1. Regulatory mechanisms and molecules of PGC-1α in chondrocytes. AMPK can directly phosphorylate and activate PGC-1α, and can also activate SIRT1 by regulating NAD+/NADH, thereby acetylating and activating PGC-1α; PGC-1α can further promote the production of NRF1, NRF2 and TFAM; REDD1 is an endogenous inhibitor of mTOR and can regulate the transcriptional level of PGC-1α; PGC-1α can positively regulate SIRT3, and can also promote the expression of Sirt3 gene by mediating ERRα; SIRT3 can deacetylate FoxO3A in the mitochondrial matrix, and can also activate LKB1 to form a positive feedback loop to promote the expression of AMPK. P, phosphorylate; AC, acetylation; DAC, deacetylation.
3.2 AMPK/SIRT3 pathway
Similar to SIRT1, silent information regulator 3 (SIRT3) is an NAD + -dependent protein deacetylase that is activated when translocated to mitochondria (Ansari et al., 2017). SIRT3 is an important regulator of chondrocyte energy metabolism, and SIRT3 enhances the antioxidant activity of superoxide dismutase (SOD2) to protect mitochondria from oxidative stress, and to repair mtDNA damage by deacetylating 8-oxoguanine DNA glycosylase-1 (OGG1) (Kincaid et al., 2013; Chen et al., 2021a).
In human knee chondrocyte experiments, it was found that AMPK can regulate the level and activity of SIRT3 (Chen et al., 2018). As mentioned above, AMPK can directly regulate the expression of PGC-1α, and PGC-1α can promote the expression of Sirt3 gene by mediating the binding of estrogen-related receptor-α (ERRα) to the Sirt3 promoter (Ansari et al., 2017) (Figure 1). PGC-1α can also promote SIRT3 expression directly or indirectly through the interaction of SIRT1 and NRF2 (Kong et al., 2010). In addition, SIRT3 also affects AMPK activity by promoting the expression of liver kinase B1 (LKB1) (Li et al., 2022). LKB1 is the main kinase that catalyzes the process of AMPK activation and energy production, and the activation of AMPK further promotes the expression of PGC-1α (Yao et al., 2023). Forkhead box class O 3 A (FoxO3A) is a transcription factor of the FOXO family, and like PGC-1α, limits cellular oxidative stress by upregulating antioxidant enzymes (Zhao et al., 2014; Almeida and Porter, 2019). SIRT3 deacetylates FoxO3A in the mitochondrial matrix and binds to mtDNA, promoting the upregulation of all mitochondria-encoded genes (Zhao et al., 2014). SIRT3 may also cooperate with FoxO3A to mediate the anti-oxidative stress effects of AMPK in chondrocytes.
In general, PGC-1α exerts its ability to resist oxidation and repair DNA damage through the AMPK/SIRT3 signaling pathway and its positive feedback mechanism (Chen et al., 2021a). SIRT3 may reverse mitochondrial dysfunction in OA through LKB1/AMPK signaling.
3.3 mTOR pathway
Mechanistic target of rapamycin (mTOR) is a serine-threonine protein kinase that forms two distinct complexes, mTOR complex 1 (mTORC1) and mTOR complex 2 (mTORC2). mTORC1 is highly sensitive to rapamycin and can be inhibited by tuberous sclerosis complex 1/2 (TSC1/2) (Yang et al., 2020). mTORC1 regulates the growth and proliferation of chondrocytes, osteoblasts and osteoclasts and is therefore critical for bone metabolism (Huang et al., 2015; Zhang et al., 2017a). Activation of mTORC1 can induce OA, whereas inhibition of mTORC1 by rapamycin to activate autophagy is protective in human chondrocytes (Zhang et al., 2017b). Furthermore, activation of mTORC1 was found to induce abnormal subchondral bone formation and promote OA in a mice model (Lin et al., 2019).
Mechanistically, the transcriptional level of PGC-1α is regulated by mTORC1. In skeletal muscle, mTORC1 regulates the oxidative capacity of skeletal muscle by changing the expression level of PGC-1α (Bentzinger et al., 2013). mTORC1 has also been shown to be involved in the decreased expression of PGC-1α due to Endoplasmic reticulum (ER) stress (Montori-Grau et al., 2022). In OA, development and DNA damage response-1 (REDD1), an endogenous inhibitor of mTOR, is reduced in articular cartilage (Figure 1). REDD1 controls mitochondrial biogenesis in chondrocytes by regulating the transcriptional level of PGC-1α (Alvarez-Garcia et al., 2017). Overall, although a direct link between PGC-1α and mTORC1 has been found in other tissues, the link in OA still needs to be further explored.
3.4 AMPK/PPAR-γ/PGC-1α pathway
Peroxisome proliferator-activated receptor-γ (PPAR-γ), a ligand-activated nuclear receptor, is an important target for the treatment of metabolic diseases (Wang et al., 2016). PPAR-γ-knockout mice with OA have decreased numbers of chondrocytes and increased expression of catabolic and inflammatory markers, so PPAR-γ can be used as a potential target for the treatment of OA (Vasheghani et al., 2015). PGC-1α is a transcriptional coactivator of PPAR-γ, and PPAR-γ stimulation promotes mitochondrial biogenesis by inducing PGC-1α (Zhang et al., 2021) (Figure 1). In an experiment on mice chondrocytes, it was found that the increased expression of PGC-1α and PPAR-γ could alleviate the increased expression of pro-inflammatory mediators and matrix metalloproteinases 13 (MMP-13) caused by high homocysteine (Ma et al., 2018a). The disordered expression of homocysteine may lead to mitochondrial dysfunction and oxidative stress in chondrocytes by inhibiting SIRT1 (Kalani et al., 2014). The experimental results confirmed that the disorder of homocysteine mitigated the activation of nuclear factor κB (NF-κB) pathway and reduced the expression of MMP-13, cyclooxygenase-2 (COX-2) and IL-8 in chondrocytes through AMPK/SIRT1/PGC-1α/PPAR-γ signal transduction (Ma et al., 2018a).
In addition, PPAR-γ also maintains the balance between catabolic and anabolic factors in vitro by regulating the mTOR/autophagy signaling pathway (Vasheghani et al., 2015). Given that OA is strongly associated with obesity and energy metabolism, the in vivo role of PPAR-γ in articular cartilage homeostasis requires further investigation.
4 PGC-1α reverses mitochondrial dysfunction in OA chondrocytes
4.1 PGC-1α promotes mitochondrial biogenesis in chondrocytes
Mitochondrial biogenesis is a self-renewal process that continuously provides new mitochondria through growth and differentiation, and maintains mitochondrial homeostasis by clearing damaged mitochondria (Liu et al., 2022). In OA chondrocytes, the dysfunction of mitochondrial biogenesis is mainly manifested by decreased mtDNA content, mitochondrial mass, oxygen consumption, oxidative phosphorylation (OXPHOS), and intracellular ATP levels (Wang et al., 2021a). PGC-1α is activated through the AMPK/SIRT1 signaling pathway, and increased levels of PGC-1α can promote the expression of NRF1, NRF2 and TFAM, thereby promoting mitochondrial biogenesis (Zhang et al., 2018a) (Figure 2). Omentin-1, a newly discovered metabolically regulated adipokinine, promotes mitochondrial biogenesis in chondrocytes by enhancing the expression of PGC-1α, NRF1, and TFAM (Li et al., 2020). The cytokine fibroblast growth factors (FGFs) promote mitochondrial biogenesis in a similar mode to Omentin-1, and studies have shown that FGF19 enhances mitochondrial biogenesis and fusion through upregulation of AMPKα signaling (Kan et al., 2023). FGF19 increases the expression of p-AMPKα and PGC-1α, directly promoting mitochondrial biogenesis through the AMPK/SIRT1/PGC-1α axis (Herzig and Shaw, 2018) (Figure 2). Levels of PGC-1α were also associated with the molecule regulated in REDD1, which is a key factor in AMPK-induced transcriptional activation of PGC-1α in chondrocytes. REDD1 transcriptionally activates PGC-1α to promote mitochondrial biogenesis. Conversely, OA chondrocytes lacking REDD1 have reduced mitochondrial content, ATP levels, mitochondrial biogenesis, and expression levels of PGC-1α and TFAM (Sun et al., 2021a).
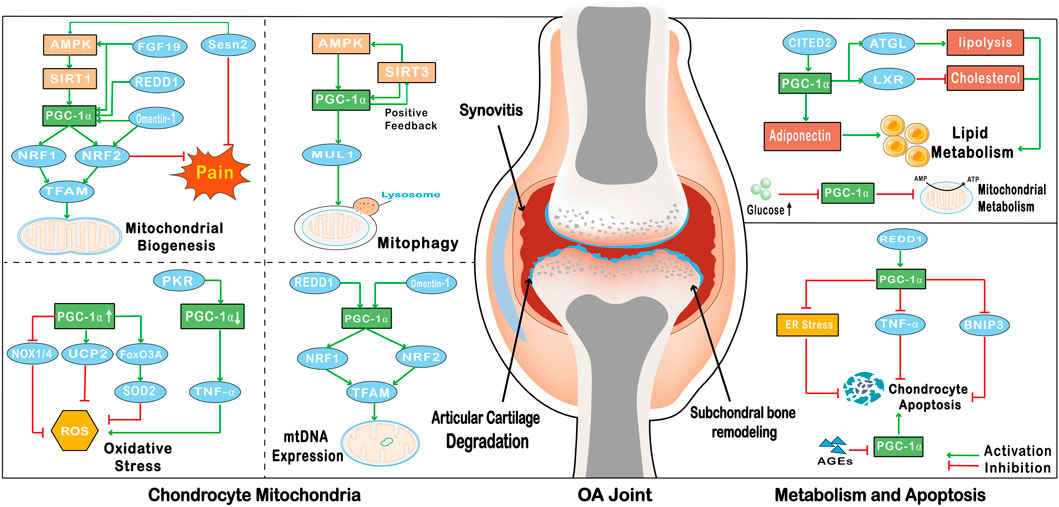
FIGURE 2. The effect of PGC-1α on OA chondrocytes. PGC-1α enhances the expression of NRF1, NRF2 and TFAM, and promotes the biogenesis of chondrocyte mitochondria; FGF19, REDD1 and Omentin-1 promote the biogenesis of chondrocyte mitochondria by promoting the expression of PGC-1α; Sesn2 reduces the pain of OA by promoting the expression of PGC-1α and NRF2; PGC-1α and SIRT3 form a positive feedback loop to promote the expression of autophagy factor MUL1 to promote mitophagy; the increase of PGC-1α expression can promote the expression of FoxO3A and UCP2 to inhibit the production of ROS; In addition, the increase of PGC-1α can also inhibit the expression of NOX1/4 to inhibit oxidative stress; On the contrary, PKR promotes the production of TNF-α by down-regulating the expression of PGC-1α to promote the production of ROS; REDD1 and Omentin-1 through affect the level of PGC-1α to promote the expression of mtDNA; PGC-1α promotes the catabolism of fatty acids and cholesterol by regulating the activity of ATGL and LXR, and CITED2 can also promote the expression of lipocalin by activating PGC-1α to regulate chondrocyte metabolism; High levels of glucose in OA chondrocytes can down-regulate the level of PGC-1α to inhibit mitochondrial metabolism; PGC-1α can be activated by REDD1 to down-regulate the level of inflammatory cytokine TNF-α to inhibit apoptosis. The effect of PGC-1α on inhibiting apoptosis can also be realized by inhibiting ER stress. In addition, the level of PGC-1α can be regulated by AGEs, and the decrease of PGC-1α level in chondrocytes can activate BNIP3 to promote apoptosis.
In addition, reduced mitochondrial biogenesis in chondrocytes may be associated with pain in OA. NRF2, produced by PGC-1α transcription, effectively relieves pain by regulating chondrocyte mitochondrial biogenesis (Sun et al., 2021a). Sestrins are a family of highly-conserved proteins induced by DNA damage and oxidative stress, and Sestrin2 (Sesn2) is a member of the Sestrin family. Overexpression of Sesn2 alleviates pain in monoiodoacetate-induced OA rats through AMPK/PGC-1α-mediated mitochondrial biogenesis (Sun et al., 2022) (Figure 2). Sesn2 also activates NRF2 with the help of PGC-1α expression and reduces ROS production, which may contribute to the relief of OA pain (Sun et al., 2022). Therefore, PGC-1α alleviates mitochondrial dysfunction in OA chondrocytes by promoting mitochondrial biogenesis, and can reduce the pain of OA.
4.2 PGC-1α promotes mitophagy in chondrocytes
Mitophagy is a specialized form of autophagy that functions to regulate the turnover of dysfunctional mitochondria and maintain mitochondrial homeostasis (Sun et al., 2021b). Additionally, mitophagy reduces the production of ROS and inhibits the activation of inflammatory factors in OA. The lack of mitophagy is an important factor in the development of OA disease, leading to chondrocyte death, imbalance of ECM homeostasis and cartilage degeneration (Hu et al., 2020).
In recent years, several studies have shown a link between PGC-1α and chondrocyte mitophagy. PGC-1α activates SIRT3 and regulates mitophagy through an AMPK/SIRT3 positive feedback loop (Chen et al., 2021a). In contrast, reduced expression levels of PGC-1α in OA chondrocytes activate parkin RBR E3 ubiquitin protein ligase (PRKN)-independent mitophagy via upregulation of Bcl-2/adenovirus E1B 19-kDa interacting protein (BNIP3), stimulating cartilage degradation and chondrocyte apoptosis (Kim et al., 2021). Furthermore, overexpression of PGC-1α suppresses FoxO3-mediated transcriptional activity, which further promotes the expression of various autophagy factors such as mitochondrial ubiquitin ligase 1 (MUL1) (Olmos et al., 2013) (Figure 2). One study has shown that dysregulation of mitophagy due to abnormalities in the AMPK/SIRT1/PGC-1α signaling pathway is a causative agent of sarcopenic obesity (Ryu et al., 2020), which may be associated with complications of OA, and therefore decreased levels of PGC-1α may induce and exacerbate OA by affecting mitophagy.
4.3 PGC-1α reduces oxidative stress in chondrocytes
Mitochondrial damage is associated with ROS production, which can be increased by a variety of factors including inflammatory cytokines, mechanical stress, and aging (Wang et al., 2021a). Increased ROS production and downregulation of SOD2 induce oxidative stress, which in turn leads to mitochondrial damage (Loeser et al., 2016). Loss of mitochondrial membrane potential in damaged mitochondria leads to decreased ATP production and increased mitochondrial membrane permeability, which is an important factor in chondrocyte senescence (Sun et al., 2021b). In addition, ROS induce oxidative stress and impair mitochondrial biogenesis, which has been shown to be associated with the development of chronic pain (Gao et al., 2022).
The activity of PGC-1α is inhibited in OA, leading to increased ROS production and oxidative stress. Double-stranded RNA-dependent protein kinase R (PKR) is an interferon-inducible kinase associated with cartilage degeneration that occurs in arthritic disease (Ma et al., 2018b). Increased PKR mediates activation of the inflammatory cytokine TNF-α by inhibiting the expression of PGC-1α, leading to increased oxidative stress and apoptosis in chondrocytes (Ma et al., 2018b). Activation of AMPK and SIRT1 modulates the activity of PGC-1α, thereby reducing oxidative stress and pro-metabolic responses in chondrocytes from OA patients (Wang et al., 2020), and relieving pain in patients with OA. PGC-1α and FoxO3A also limit cellular oxidative stress by upregulating antioxidant enzymes, including SOD2 and catalase (Zhao et al., 2014) (Figure 2). In addition, PGC-1α increases ATP production and reduces ROS production by altering the structure of the mitochondrial respiratory complex (Cunningham et al., 2007). PGC-1α also reverses the loss of chondrocyte phenotype by decreasing NADPH oxidase1/4 (NOX1/4) expression and increasing uncoupling protein 2 (UCP2) expression (Miao et al., 2017) (Figure 2). Consequently, PGC-1α has the ability to inhibit oxidative stress by reducing ROS production in chondrocytes, which is beneficial in delaying the further development of OA.
4.4 PGC-1α promotes the replication and gene expression of mtDNA in chondrocytes
Alterations in mitochondrial genetics are an important contributor to the development of OA. Increased ROS also leads to damage of mtDNA, causing a severe imbalance in redox and metabolic activity, which disrupts the homeostasis of articular cartilage (He et al., 2020). Reduced PGC-1α activity in OA is closely associated with decreased mitochondrial biogenesis and mtDNA content. PGC-1α activates NRF1 and NRF2 and promotes TFAM expression. Activation of PGC-1α, NRF1 and TFAM contributes to transcription and replication of mtDNA and the generation of new mitochondria (Kim et al., 2021). In addition, Omentin-1 promotes the expression of mtDNA, mRNA transcripts and mitochondrial proteins through the activation of PGC-1α (Li et al., 2020) (Figure 2). In conclusion, altered mitochondrial genetics is also a feature of OA, and like mitochondrial dysfunction, mtDNA damage in OA can be treated by affecting PGC-1α activity in chondrocytes.
5 Relationship between PGC-1α and abnormal metabolism of chondrocytes in OA
Abnormal chondrocyte metabolism is risk factor for OA and can have a direct systemic impact on the joints (Zheng et al., 2021). In the OA setting, the main components of the ECM, aggrecan and type II collagen, are reduced due to inhibition of chondrocyte synthetic activity (Wei et al., 2021). In OA cartilage, the rate of anaerobic glycolysis is increased and changes in key enzymes and glycolytic processes lead to the production and accumulation of excess lactic acid by chondrocytes, creating an acidic microenvironment (Maneiro et al., 2003). The acidic microenvironment has been shown to inhibit matrix synthesis in chondrocytes and potentially promote cartilage degeneration in OA (High et al., 2019). On the other hand, OA chondrocytes exhibit intracellular lipid deposition and the amount of lipid deposition is positively correlated with the severity of OA (Zheng et al., 2021).
Studies have shown PGC-1α regulates chondrocyte metabolism through the AMPK/SIRT1/PGC-1α signaling pathway, and has the ability to block chondrocyte pre-catabolic reactions (Zhao et al., 2014). PGC-1α is involved in the inhibition of advanced glycation end products (AGEs)-induced NF-κB activation and inflammatory cytokine-induced catabolic responses in chondrocytes (Li et al., 2021a; Yang et al., 2022). In contrast, decreased levels of PGC-1α in OA chondrocytes, possibly due to high levels of glucose promoting glycolysis and inhibiting oxidative phosphorylation, lead to impaired mitochondrial metabolism and trigger mitochondrial dysfunction (Minguzzi et al., 2018).
In addition to glucose metabolism, PGC-1α is also involved in the regulation of abnormal lipid metabolism in OA. PGC-1α is activated by SIRT1 in response to adipose triglyceride lipase (ATGL)-mediated increases in lipolysis (Khan et al., 2015). Activation of PGC-1α by PPAR-γ promotes Liver X receptors (LXR) expression, reduces cholesterol deposition on the joint surface, and maintains normal joint function and bone development (Ratneswaran et al., 2017). PGC-1α, which can be activated by Glu/Asp rich carboxy-terminal domain 2 (CITED2), plays a critical role in regulating load-induced adiponectin and inhibiting adiponectin expression in human infrapatellar fat pad-derived adipose stem cells/preadipocytes (Liu et al., 2019). In conclusion, PGC-1α is associated with obesity-induced OA and is involved in the regulation of abnormal glucose metabolism and lipid metabolism in OA chondrocytes (Ryu et al., 2020) (Figure 2).
6 Relationship between PGC-1α and chondrocyte apoptosis in OA
As mentioned above, increased ROS production and decreased SOD2 levels in OA chondrocytes lead to mitochondrial damage. Depolarization of mitochondria leads to the release of apoptotic factors such as cytochrome c (Cyt-c), apoptosis-inducing factor and capase-9 from the intermembrane space of mitochondria into the cytoplasm, resulting in apoptosis (Ansari et al., 2018). Numerous studies in recent years have revealed the relationship between autophagy, ER stress and chondrocyte apoptosis, indicating that autophagy may inhibit chondrocyte apoptosis by reducing ROS production through clearance of damaged mitochondria (Nugent et al., 2009). Increased ROS also induces ER stress, and sustained ER stress triggers the apoptotic pathway (Kim and Kim, 2018).
PGC-1α inhibits oxidative stress by reducing ROS production, blocking the activation of ER stress, and thus reducing chondrocyte apoptosis (Feng et al., 2019) (Figure 2). PGC-1α may also alleviate mitochondrial dysfunction in chondrocytes and reduce chondrocyte apoptosis by promoting mitochondrial biogenesis and accelerating the replication and expression of mtDNA. Conversely, decreased levels of PGC-1α may contribute to TNF-α induces chondrocyte apoptosis through the accumulation of oxidative stress via the PKR/p38 MAPK/p53/AKT/PGC-1α signaling pathway (Goldring, 2000). As an important mediator of OA, AGEs induce OA is by downregulating PGC-1α levels leading to increased oxidative stress, inflammation and apoptosis (Yu et al., 2022). Expression of REDD1 is regulated by PGC-1α, and Redd1−/− mice have increased rates of apoptosis and increased indicators of cell death in knee chondrocytes (Alvarez-Garcia et al., 2017) (Figure 2). A study on homocysteine and OA showed that homocysteine dose-dependently inhibited the expression of AMPK/SIRT1/PGC-1α signaling in chondrocytes to promote chondrocyte apoptosis (Ma et al., 2018a). PGC-1α also has a role in promoting cartilage formation and differentiation, which may be achieved through interaction with the transcription factor sry-related high mobility group-box 9 (SOX9) (Kawakami et al., 2005). SOX9 is a key transcription factor in chondrocytes, co-expression of SOX9 and PGC-1α also induces the expression of other chondrogenic genes (Kawakami et al., 2005).
7 Potential drugs and strategies to treat OA by affecting the activity of PGC-1α
PGC-1α is important to the mitochondrial function and energy metabolism of chondrocytes, and also delays the degeneration of articular cartilage by regulating the process of cell death. Improving the antioxidant capacity of chondrocytes and reversing mitochondrial dysfunction in OA chondrocytes by affecting PGC-1α levels may be a potential therapeutic strategy for OA. Therefore, it is necessary to develop new drugs and novel nanohybrid based on altering the activity of PGC-1α to improve the function of OA chondrocytes (Figure 3) (Table 1).
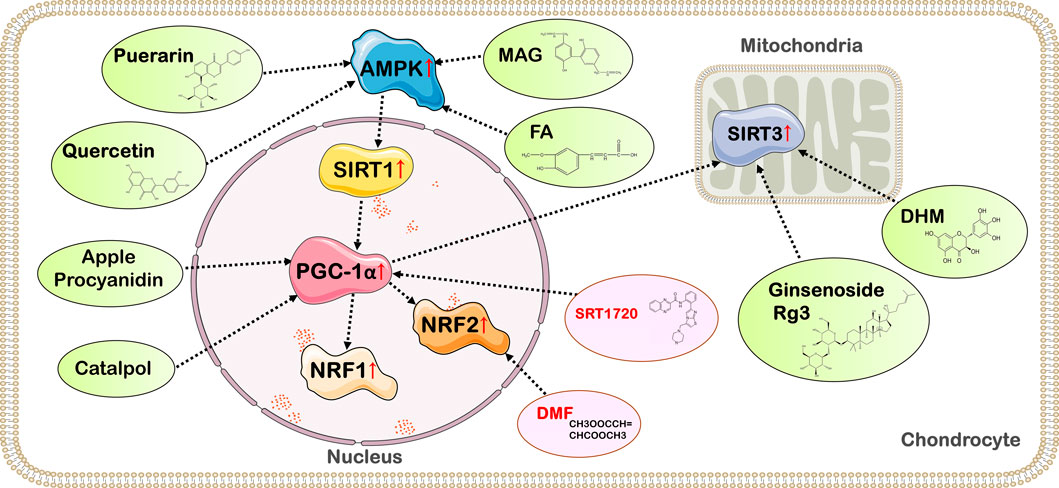
FIGURE 3. Targets of action of drugs with therapeutic effects on OA by affecting the activity of PGC-1α. Quercetin, FA, MAG, geraniin, and Br in RB@MPMW promote PGC-1α expression and delay the deterioration of OA by activating the AMPK/SIRT1/PGC-1α signaling pathway; Apple Procyanidins and catalpol can affect PGC-1α activity and ameliorate mitochondrial damage in OA chondrocytes; Ginsenoside Rg3 and DHM exert anti-inflammatory and chondrocyte homeostatic effects through the PGC-1α/SIRT3 pathway; SRT1720, an activator of SIRT1, exerts chondroprotective effects by activating SIRT1 and PGC-1α; DMF enhances NRF2 expression to promote mitochondrial biogenesis in chondrocytes.
7.1 Natural products
7.1.1 Quercetin
Quercetin (3,3′,4′,5,7-pentahydroxyflavone) is a naturally occurring flavonoid found in many types of fruits and vegetables with anti-inflammatory, antioxidant, and anti-osteoporotic effects (Li et al., 2021b). In addition, quercetin alleviates cartilage degradation and thus OA by modulating chondrocyte autophagy (Lv et al., 2022), inflammation (Wang et al., 2021b), oxidative stress (Hu et al., 2019a), and apoptosis (Li et al., 2021b). Quercetin reduces the production of ROS and increases the expression levels of glutathione and glutathione peroxidase in OA rats. Quercetin also upregulates SOD and tissue inhibitor of metalloproteinase 1 while downregulating MMP-13 to attenuate oxidative stress and inhibit the degradation of cartilage extracellular matrix (Wei et al., 2019). Another animal study suggested that quercetin affects the characteristics and composition of the gut microbiota and metabolism of OA rats (Lan et al., 2021). Taken together, these data suggest that quercetin alleviates the deterioration of OA through multiple mechanisms.
In a rat model study of OA, researchers found that ROS production was reduced and mitochondrial biogenesis was improved after quercetin administration. In addition, the expression of p-AMPK, SIRT1, PGC-1α, NRF1, NRF2, and TFAM was also enhanced after quercetin application. Therefore, the antioxidative and mitochondrial dysfunction-reversing effects of quercetin may be achieved through the AMPK/SIRT1/PGC-1α signaling pathway (Qiu et al., 2018). Another animal study found that quercetin can also inhibit ER stress by activating the AMPK/SIRT1 signaling pathway, thereby inhibiting chondrocyte apoptosis, alleviating and eliminating articular cartilage degeneration, and thus treating OA (Feng et al., 2019). As mentioned above, quercetin may increase the expression of PGC-1α through the AMPK/SIRT1 signaling pathway, exerting the potential of treating OA in the mitochondrial pathway. However, most of the current studies on quercetin and OA use rat models, and further research is needed to explore its therapeutic potential and safety on human OA chondrocytes.
7.1.2 Magnolol (MAG)
Magnolol (MAG) is extracted from a Chinese medicinal herb named Magnolia officinalis. A study on the link between MAG and OA showed that MAG exerted chondroprotective effects by inhibiting the production of inflammatory mediators as well as the degradation of OA chondrocyte proteoglycans and type II collagen (Hu et al., 2019b).
The effect of MAG on PGC-1α activity may be another mediator of its effects in OA. MAG increased PGC-1α expression in human chondrocytes in a dose-dependent manner and also alleviated IL-1β-induced mitochondrial dysfunction, oxidative stress and inflammation through the AMPK/SIRT1/PGC-1α signaling pathway and maintained the balance of ECM synthesis and catabolism in human chondrocytes (Liu et al., 2020). Therefore, MAG can be extracted and developed as a potential drug for OA.
7.1.3 Ferulic acid (FA)
Ferulic acid (3-methoxy-4-hydroxycinnamic acid; FA), a phenolic substance widely found in plants (Klepacka and Łiu, 2006), is one of the most common natural products found in vegetable, and is an important active ingredient in many traditional Chinese medicines (Chaudhary et al., 2019). FA plays a role in the response to oxidative stress, inflammation, vascular endothelial damage, fibrosis, apoptosis and platelet aggregation, and is involved in the treatment of various diseases throughout the body (Chaudhary et al., 2019; Li et al., 2021c).
One of the mechanisms of FA treatment for OA is the inhibition of IL-1β-induced chondrocyte degeneration through the AMPK/SIRT1/PGC-1α signaling pathway. FA inhibits the production of IL-6, prostaglandin E2, nitrite, Collagen I, runt-related transcription factor 2, MMP-1, MMP-3 and MMP-13, suppresses oxidative stress, attenuates IL-1β-induced OA chondrocyte degeneration, and enhances expression of type II collagen and aggrecan through activation of PGC-1α (Du et al., 2021). Moreover, FA also prevents degradation of the ECM, inhibits the inflammatory response, and delays the onset and progression of OA (Zhou et al., 2021). The current study confirmed that FA, as a nutritional supplement for patients with OA, may have a potential therapeutic effect on OA by inhibiting inflammation (Du et al., 2021), but its clinical application value needs to be further explored.
7.1.4 Puerarin
Puerarin (C21H20O9) is the main bioactive component isolated from the plant Pueraria montana var. Lobata (Zhou et al., 2014). Puerarin has antioxidant, anti-inflammatory, neuroprotective and anti-apoptotic activities, as well as lowering blood sugar and improving microcirculation. In OA, Puerarin has been shown to inhibit ECM degradation, relieve pain, and reduce cartilage destruction (Peng et al., 2019; Chen et al., 2021b; Li et al., 2021d). In addition, Puerarin was found to inhibit the production of the inflammatory factors IL-1β, IL-6 and TNF-α and increase type II collagen content in a rat OA model established by anterior cruciate ligament transection (Ma et al., 2020).
Although Puerarin has the ability to treat a variety of diseases, its molecular mechanisms and targets are not fully understood. There is evidence that the effect of Puerarin on OA rats may be achieved through the AMPK/PGC-1α pathway (Wang et al., 2018a). Experimental results in rat models have shown that puerarin can promote chondrocyte mitochondrial biogenesis through the AMPK/PGC-1α pathway, restore mitochondrial dysfunction in OA chondrocytes (Wang et al., 2018a), while reducing mechanical nociceptive hypersensitivity and cartilage damage in OA rats. In addition, in Puerarin-fed OA mice chondrocytes, the researchers found that Puerarin may inhibit the activation of NF-κB pathway and the degradation of ECM by regulating the level of NRF2 (Chen et al., 2021b). The activation of NF-κB pathway can enhance the inflammatory response of cells, leading to inflammatory injury and apoptosis (Tak and Firestein, 2001). Therefore, Puerarin is a dietary supplement with potential for the treatment of OA, but its role in the treatment of OA in humans needs further clinical verification.
7.1.5 Apple procyanidin
Apple polyphenols (apple Procyanidin) are compounds of several polyphenols obtained from unripe apples and have been shown to have cardioprotective (Cicero et al., 2017), anti-inflammatory and anti-proliferative properties. An animal study showed that apple polyphenols reduced the severity of OA by inhibiting oxidative stress and the expression of TNF-α and MMP-13. Apple polyphenols also inhibited synovial inflammation in OA by enhancing cell proliferation and hyaluronic acid production (Kobayashi et al., 2022). Oral administration of apple polyphenols to mice was also shown to prevent articular cartilage degeneration caused by mitochondrial dysfunction. In addition, apple polyphenols were able to promote mitochondrial biogenesis and proteoglycan biosynthesis in chondrocytes and enhance aggrecan upregulation in primary chondrocytes, which may be achieved by affecting PGC-1α activity (Masuda et al., 2018). Mechanistically, apple polyphenols enhance mitochondrial dehydrogenase activity and mitochondrial DNA copy number, and promote PGC-1α expression to promote mitochondrial biogenesis. Apple polyphenols also improve mitochondrial depolarization impaired by SOD2 loss by affecting PGC-1α activity (Masuda et al., 2018). These results demonstrate that apple polyphenols may be food components with effects on maintaining joint cartilage health.
7.1.6 Ginsenoside Rg3
Ginsenoside Rg3 is a steroidal saponin isolated from ginseng and is the main active component of ginseng (Liu et al., 2021). Recent studies have shown that Rg3 has various biological activities such as anti-inflammatory and anti-cancer (Xia et al., 2022). In the experimental model of human OA aging chondrocytes, Ginsenoside Rg3 has the effect of anti-aging and protecting cartilage (So et al., 2013). A new study suggests that activation of the SIRT1/PGC-1α/SIRT3 pathway by Rg3 inhibits TNF-α-induced cyclophilin D acetylation, reduces mitochondrial dysfunction and ROS accumulation, thereby ameliorating TNF-α-induced apoptosis (Ma et al., 2021). Additionally, Rg3 suppresses TNF-α-stimulated p38 MAPK phosphorylation and NF-κB activation through SIRT1/PGC-1α/SIRT3 signaling, and inhibits TNF-α-induced increases in production of IL-8 and MMP-9 (Ma et al., 2021). The SIRT1/PGC-1α/SIRT3 pathway may be the main mechanism via which Rg3 acts to inhibit expression of the inflammatory cytokine TGF-α, so Ginsenoside Rg3 has the potential to alleviate the inflammatory response and cartilage degeneration in OA patients.
7.1.7 Catalpol
Catalpol, an active ingredient from the traditional Chinese herbal medicine Di-Huang (Rehmannia glutinosa Libosch or Chinese foxglove), has potential antioxidant and hypoglycemic effects (Bhattamisra et al., 2021). Previous studies have shown that in chondrocytes, catalpol can attenuate IL-1β-induced inflammatory response and apoptosis in rat chondrocytes by inhibiting the NF-κB pathway (Zeng et al., 2019). In addition, catalpol was also found to enhance mitochondrial biogenesis in human chondrocytes through a dose-dependent increase in phosphorylation of cAMP/response element-binding protein (CREB) to promote the expression of PGC-1α, NRF1 and TFAM (Chen et al., 2022a). In addition, catalpol improves mitochondrial ATP production, Cyt-c oxidase activity, and respiratory rate. Although the current study shows that catalpol has chondroprotective effect, further detailed mechanism is needed before it can be used for the treatment and prevention of OA.
7.1.8 Dihydromyricetin (DHM)
DHM is a flavonoid with modulatory metabolic, anti-inflammatory, antioxidant, antitumor, pyroptosis-reducing and cardioprotective effects (Zhang et al., 2018b; Cheng et al., 2020; Sun et al., 2020). DHM increases SIRT3 and PGC-1α levels in a dose-dependent manner through the AMPK/SIRT3/PGC-1α signaling pathway, improves antioxidant capacity and mitochondrial fusion in chondrocytes, increases the levels of aggrecan and type II collagen, maintains chondrocyte homeostasis and prevents chondrocyte degeneration (Wang et al., 2018b). The level of PGC-1α is positively correlated with the activity of SIRT3, and DHM also activates SIRT3 by increasing the level of PGC-1α to regulate the mitochondrial dynamics and mitophagy in chondrocytes (Mao et al., 2020), providing a new idea for the treatment of OA.
7.2 Chemical drugs
7.2.1 Dimethyl fumarate (DMF)
Dimethyl fumarate (DMF) is a fumarate ester with cytoprotective, anti-inflammatory and antioxidant properties, induces protein succinylation, which leads to inactivation of cysteine-rich proteins (Saidu et al., 2019). As mentioned above, PGC-1α can activate NRF2 to regulate mitochondrial biogenesis, and DMF mainly plays a role by regulating the level of NRF2. Experimental animal studies have shown that DMF improves renal injury and cognitive deficits by activating NRF2 to prevent ferroptosis (Yang et al., 2021c; Yan et al., 2021). For OA, DMF inhibits IL-1β expression by activating NRF2, thereby attenuating destabilization of the medial meniscus-induced OA in mice (Chen et al., 2022b). Furthermore, DMF induced mitochondrial biogenesis and attenuated pain-related behaviors in a rat model of OA by activating NRF2 (Gao et al., 2022). In human chondrocytes, experiments have shown that DMF can inhibit OA-induced degradation of type II collagen, suggesting that DMF treatment may be a potential chondroprotective strategy (Li et al., 2014).
7.2.2 SRT1720
SRT1720 is a synthetic compound that activates SIRT1((Minor et al., 2011; Svensson et al., 2015)) and has been implicated in the regulation of neurogenesis (Iwata et al., 2020) and angiogenesis (Dadwal et al., 2021) through activation of SIRT1. It has also been shown that SRT1720 can reduce pain due to bone cancer (Li et al., 2019) and intervertebral disc degeneration (Zhang et al., 2020) by activating SIRT1. In OA, intraperitoneal injection of SRT1720 has been shown to slow the progression of experimental OA in mice (Nishida et al., 2018). SRT1720 also exerts a chondroprotective effect by increasing the level of SIRT1 to regulate the expression of lymphoid enhancer-binding factor 1 (LEF-1) and related inflammatory factors in OA (135). In addition, an animal study on rabbit chondrocytes suggested that SRT1720 inhibited OA cell apoptosis by activating SIRT1 and PGC-1α, thereby protecting chondrocytes and promoting the expression of cartilage matrix (Liu et al., 2016). In conclusion, the mechanism of action of SRT1720 is not fully understood so far, but its chondroprotective effect provides a new strategy for the treatment of OA.
7.3 Novel nanohybrid
7.3.1 Cartilage-targeting peptide-modified dual-drug delivery nanoplatform with NIR laser (RB@MPMW)
Cartilage-targeted drug delivery is an effective strategy for the treatment of OA, and the design of novel drug delivery systems has been an important direction of OA treatment research in recent years (Xiong et al., 2021). As mentioned above, PGC-1α has a role in regulating mitochondrial function and chondrocyte energy metabolism. Cartilage-targeted peptide-modified near-infrared laser dual drug delivery nanoplatform is a recently reported novel material for the treatment of OA that regulates chondrocyte energy metabolism through sustained phosphorylation of AMPK by bilirubin (Br) (Idelman et al., 2015; Vítek, 2020) and thus promotes activation of PGC-1α. The platform loads rapamycin in mesopores, Br on the metal organic framework shell, and type II collagen-targeting peptide bound to the above nanocarrier surface. Near-infrared laser stimulation releases both rapamycin and Br drugs continuously. Br acts as an activator of AMPK and activates PGC-1α by activating the AMPK/SIRT1/PGC-1α signaling pathway, exerting the ability of PGC-1α to scavenge ROS and inhibit apoptosis (Xue et al., 2021). Activation of PGC-1α also inhibits the action of the inflammatory cytokine IL-β and regulates the energy metabolism of chondrocytes (Xue et al., 2021), thus preventing cartilage degeneration in vivo.
8 Discussion
Results of recent studies suggest that PGC-1α, as a master regulator of mitochondrial biogenesis and metabolism, may be a candidate target for the treatment of OA. PGC-1α is activated by multiple pathways and signaling molecules in chondrocytes to regulate mitochondrial biogenesis, mitophagy, and mtDNA replication and expression. In addition, PGC-1α can inhibit oxidative stress and chondrocyte apoptosis to maintain cartilage homeostasis. PGC-1α is also involved in the regulation of abnormal energy metabolism and lipid metabolism in OA chondrocytes, and inhibits the catabolic reactions of chondrocytes. The chondroprotective effect of PGC-1α has also been demonstrated in drug experiments, and multiple effectors have shown beneficial effects in experimental models of OA by activating PGC-1α in chondrocytes. However, the value of target-activating PGC-1α effectors and novel nanohybrids in clinical applications needs further investigation. The role of PGC-1α on other metabolic pathways of chondrocytes and other pathological changes of OA is still unclear. Therefore, in the future experimental research and clinical treatment of OA, PGC-1α and its related pathways and regulatory molecules deserve special attention.
Author contributions
HW had the idea for the article and wrote the paper. JS and MY performed the literature search and data analysis. YX and YW reviewed the manuscript and approved the final version. All authors read and approved the final manuscript.
Funding
This work was supported by the National Natural Science Foundation of China (grant number 31900847), the China Postdoctoral Science Foundation (grant number 2019M66169), the Liaoning Province Doctoral Start-up Fund (grant number 2019JH3/10100299), the 345 Talent Project of Shengjing Hospital of China Medical University (grant number M0271).
Conflict of interest
The authors declare that the research was conducted in the absence of any commercial or financial relationships that could be construed as a potential conflict of interest.
Publisher’s note
All claims expressed in this article are solely those of the authors and do not necessarily represent those of their affiliated organizations, or those of the publisher, the editors and the reviewers. Any product that may be evaluated in this article, or claim that may be made by its manufacturer, is not guaranteed or endorsed by the publisher.
References
Abramoff, B., and Caldera, F. E. (2020). Osteoarthritis: Pathology, diagnosis, and treatment options. Med. Clin. North Am. 104 (2), 293–311. doi:10.1016/j.mcna.2019.10.007
Aguilar, A., Wu, S., and De Luca, F. (2009). P450 oxidoreductase expressed in rat chondrocytes modulates chondrogenesis via cholesterol- and Indian Hedgehog-dependent mechanisms. Endocrinology 150 (6), 2732–2739. doi:10.1210/en.2009-0043
Almeida, M., and Porter, R. M. (2019). Sirtuins and FoxOs in osteoporosis and osteoarthritis. Bone 121, 284–292. doi:10.1016/j.bone.2019.01.018
Alvarez-Garcia, O., Matsuzaki, T., Olmer, M., Plate, L., Kelly, J. W., and Lotz, M. K. (2017). Regulated in development and DNA damage response 1 deficiency impairs autophagy and mitochondrial biogenesis in articular cartilage and increases the severity of experimental osteoarthritis. Arthritis Rheumatol. 69 (7), 1418–1428. doi:10.1002/art.40104
An, S., Hu, H., Li, Y., and Hu, Y. (2020). Pyroptosis plays a role in osteoarthritis. Aging Dis. 11 (5), 1146–1157. doi:10.14336/AD.2019.1127
Ansari, A., Rahman, M. S., Saha, S. K., Saikot, F. K., Deep, A., and Kim, K. H. (2017). Function of the SIRT3 mitochondrial deacetylase in cellular physiology, cancer, and neurodegenerative disease. Aging Cell 16 (1), 4–16. doi:10.1111/acel.12538
Ansari, M. Y., Khan, N. M., Ahmad, I., and Haqqi, T. M. (2018). Parkin clearance of dysfunctional mitochondria regulates ROS levels and increases survival of human chondrocytes. Osteoarthr. Cartil. 26 (8), 1087–1097. doi:10.1016/j.joca.2017.07.020
Bai, Y., Gong, X., Dou, C., Cao, Z., and Dong, S. (2019). Redox control of chondrocyte differentiation and chondrogenesis. Free Radic. Biol. Med. 132, 83–89. doi:10.1016/j.freeradbiomed.2018.10.443
Bentzinger, C. F., Lin, S., Romanino, K., Castets, P., Guridi, M., Summermatter, S., et al. (2013). Differential response of skeletal muscles to mTORC1 signaling during atrophy and hypertrophy. Skelet. Muscle 3 (1), 6. doi:10.1186/2044-5040-3-6
Bernstein, P., Sticht, C., Jacobi, A., Liebers, C., Manthey, S., and Stiehler, M. (2010). Expression pattern differences between osteoarthritic chondrocytes and mesenchymal stem cells during chondrogenic differentiation. Osteoarthr. Cartil. 18 (12), 1596–1607. doi:10.1016/j.joca.2010.09.007
Bhattamisra, S. K., Koh, H. M., Lim, S. Y., Choudhury, H., and Pandey, M. (2021). Molecular and biochemical pathways of catalpol in alleviating diabetes mellitus and its complications. Biomolecules 11 (2), 323. doi:10.3390/biom11020323
Cantó, C., and Auwerx, J. (2009). PGC-1alpha, SIRT1 and AMPK, an energy sensing network that controls energy expenditure. Curr. Opin. Lipidol. 20 (2), 98–105. doi:10.1097/MOL.0b013e328328d0a4
Chaudhary, A., Jaswal, V. S., Choudhary, S., Ajay Sharma, S., Beniwal, V., et al. (2019). Ferulic acid: A promising therapeutic phytochemical and recent patents advances. Recent Pat. Inflamm. Allergy Drug Discov. 13 (2), 115–123. doi:10.2174/1872213X13666190621125048
Chen, D., Guo, J., and Li, L. (2022). Catalpol promotes mitochondrial biogenesis in chondrocytes. Arch. Physiol. Biochem. 128 (3), 802–808. doi:10.1080/13813455.2020.1727927
Chen, J., Chen, Z., Yuan, P., Huang, H., Wang, J., Shi, P., et al. (2022). ERK1 loss accelerates the progression of osteoarthritis in aged mice via NRF2/BACH1 signaling. Biochem. Biophys. Res. Commun. 622, 129–135. doi:10.1016/j.bbrc.2022.07.012
Chen, L. Y., Wang, Y., Terkeltaub, R., and Liu-Bryan, R. (2018). Activation of AMPK-SIRT3 signaling is chondroprotective by preserving mitochondrial DNA integrity and function. Osteoarthr. Cartil. 26 (11), 1539–1550. doi:10.1016/j.joca.2018.07.004
Chen, X., Huang, C., Sun, H., Hong, H., Jin, J., Bei, C., et al. (2021). Puerarin suppresses inflammation and ECM degradation through Nrf2/HO-1 axis in chondrocytes and alleviates pain symptom in osteoarthritic mice. Food Funct. 12 (5), 2075–2089. doi:10.1039/d0fo03076g
Chen, Y., Wu, Y. Y., Si, H. B., Lu, Y. R., and Shen, B. (2021). Mechanistic insights into AMPK-SIRT3 positive feedback loop-mediated chondrocyte mitochondrial quality control in osteoarthritis pathogenesis. Pharmacol. Res. 166, 105497. doi:10.1016/j.phrs.2021.105497
Cheng, C. F., Ku, H. C., and Lin, H. (2018). PGC-1α as a pivotal factor in lipid and metabolic regulation. Int. J. Mol. Sci. 19 (11), 3447. doi:10.3390/ijms19113447
Cheng, Q. C., Fan, J., Deng, X. W., Liu, H. C., Ding, H. R., Fang, X., et al. (2020). Dihydromyricetin ameliorates chronic liver injury by reducing pyroptosis. World J. Gastroenterol. 26 (41), 6346–6360. doi:10.3748/wjg.v26.i41.6346
Cicero, A. F. G., Caliceti, C., Fogacci, F., Giovannini, M., Calabria, D., Colletti, A., et al. (2017). Effect of apple polyphenols on vascular oxidative stress and endothelium function: A translational study. Mol. Nutr. Food Res. 61 (11), 1700373. doi:10.1002/mnfr.201700373
Cunningham, J. T., Rodgers, J. T., Arlow, D. H., Vazquez, F., Mootha, V. K., and Puigserver, P. (2007). mTOR controls mitochondrial oxidative function through a YY1-PGC-1alpha transcriptional complex. Nature 450 (7170), 736–740. doi:10.1038/nature06322
Dadwal, U. C., Bhatti, F. U. R., Awosanya, O. D., Staut, C. A., Nagaraj, R. U., Perugini, A. J., et al. (2021). The effects of SRT1720 treatment on endothelial cells derived from the lung and bone marrow of young and aged, male and female mice. Int. J. Mol. Sci. 22 (20), 11097. doi:10.3390/ijms222011097
Du, K., Fang, X., and Li, Z. (2021). Ferulic acid suppresses interleukin-1β-induced degeneration of chondrocytes isolated from patients with osteoarthritis through the SIRT1/AMPK/PGC-1α signaling pathway. Immun. Inflamm. Dis. 9 (3), 710–720. doi:10.1002/iid3.424
Feng, K., Chen, Z., Pengcheng, L., Zhang, S., and Wang, X. (2019). Quercetin attenuates oxidative stress-induced apoptosis via SIRT1/AMPK-mediated inhibition of ER stress in rat chondrocytes and prevents the progression of osteoarthritis in a rat model. J. Cell Physiol. 234 (10), 18192–18205. doi:10.1002/jcp.28452
Gao, S. J., Li, D. Y., Liu, D. Q., Sun, J., Zhang, L. Q., Wu, J. Y., et al. (2022). Dimethyl fumarate attenuates pain behaviors in osteoarthritis rats via induction of nrf2-mediated mitochondrial biogenesis. Mol. Pain 18, 17448069221124920. doi:10.1177/17448069221124920
Glyn-Jones, S., Palmer, A. J., Agricola, R., Price, A. J., Vincent, T. L., Weinans, H., et al. (2015). Osteoarthr. Lancet. 386 (9991), 376–387. doi:10.1016/S0140-6736(14)60802-3
Goldring, M. B. (2000). The role of the chondrocyte in osteoarthritis. Arthritis Rheum. 43 (9), 1916–1926. doi:10.1002/1529-0131(200009)43:9<1916::AID-ANR2>3.0.CO;2-I
Guilak, F., Nims, R. J., Dicks, A., Wu, C. L., and Meulenbelt, I. (2018). Osteoarthritis as a disease of the cartilage pericellular matrix. Matrix Biol. 71-72, 40–50. doi:10.1016/j.matbio.2018.05.008
He, Y., Makarczyk, M. J., and Lin, H. (2020). Role of mitochondria in mediating chondrocyte response to mechanical stimuli. Life Sci. 263, 118602. doi:10.1016/j.lfs.2020.118602
Herzig, S., and Shaw, R. J. (2018). Ampk: Guardian of metabolism and mitochondrial homeostasis. Nat. Rev. Mol. Cell Biol. 19 (2), 121–135. doi:10.1038/nrm.2017.95
High, R. A., Ji, Y., Ma, Y. J., Tang, Q., Murphy, M. E., Du, J., et al. (2019). In vivo assessment of extracellular pH of joint tissues using acidoCEST-UTE MRI. Quant. Imaging Med. Surg. 9 (10), 1664–1673. doi:10.21037/qims.2019.08.11
Hollander, J. M., and Zeng, L. (2019). The emerging role of glucose metabolism in cartilage development. Curr. Osteoporos. Rep. 17 (2), 59–69. doi:10.1007/s11914-019-00506-0
Hu, S., Zhang, C., Ni, L., Huang, C., Chen, D., Shi, K., et al. (2020). Stabilization of HIF-1α alleviates osteoarthritis via enhancing mitophagy. Cell Death Dis. 11 (6), 481. doi:10.1038/s41419-020-2680-0
Hu, X., Feng, G., Meng, Z., Ma, L., and Jin, Q. (2021). The protective mechanism of SIRT1 on cartilage through regulation of LEF-1. BMC Musculoskelet. Disord. 22 (1), 642. doi:10.1186/s12891-021-04516-x
Hu, Y., Gui, Z., Zhou, Y., Xia, L., Lin, K., and Xu, Y. (2019). Quercetin alleviates rat osteoarthritis by inhibiting inflammation and apoptosis of chondrocytes, modulating synovial macrophages polarization to M2 macrophages. Free Radic. Biol. Med. 145, 146–160. doi:10.1016/j.freeradbiomed.2019.09.024
Hu, Z. C., Luo, Z. C., Jiang, B. J., Fu, X., Xuan, J. W., Li, X. B., et al. (2019). The protective effect of magnolol in osteoarthritis: In vitro and in vivo studies. Front. Pharmacol. 10, 393. doi:10.3389/fphar.2019.00393
Huang, B., Wang, Y., Wang, W., Chen, J., Lai, P., Liu, Z., et al. (2015). mTORC1 prevents preosteoblast differentiation through the notch signaling pathway. PLoS Genet. 11 (8), e1005426. doi:10.1371/journal.pgen.1005426
Hunter, D. J., March, L., and Chew, M. (2020). Osteoarthritis in 2020 and beyond: A lancet commission. Lancet 396 (10264), 1711–1712. doi:10.1016/S0140-6736(20)32230-3
Idelman, G., Smith, D. L. H., and Zucker, S. D. (2015). Bilirubin inhibits the up-regulation of inducible nitric oxide synthase by scavenging reactive oxygen species generated by the toll-like receptor 4-dependent activation of NADPH oxidase. Redox Biol. 5, 398–408. doi:10.1016/j.redox.2015.06.008
Iwata, R., Casimir, P., and Vanderhaeghen, P. (2020). Mitochondrial dynamics in postmitotic cells regulate neurogenesis. Science 369 (6505), 858–862. doi:10.1126/science.aba9760
Kalani, A., Kamat, P. K., Voor, M. J., Tyagi, S. C., and Tyagi, N. (2014). Mitochondrial epigenetics in bone remodeling during hyperhomocysteinemia. Mol. Cell Biochem. 395 (1-2), 89–98. doi:10.1007/s11010-014-2114-3
Kan, S., Pi, C., Zhang, L., Guo, D., Niu, Z., Liu, Y., et al. (2023). FGF19 increases mitochondrial biogenesis and fusion in chondrocytes via the AMPKα-p38/MAPK pathway. Cell Commun. Signal 21 (1), 55. doi:10.1186/s12964-023-01069-5
Katz, J. N., Arant, K. R., and Loeser, R. F. (2021). Diagnosis and treatment of hip and knee osteoarthritis: A review. Jama 325 (6), 568–578. doi:10.1001/jama.2020.22171
Kawakami, Y., Tsuda, M., Takahashi, S., Taniguchi, N., Esteban, C. R., Zemmyo, M., et al. (2005). Transcriptional coactivator PGC-1alpha regulates chondrogenesis via association with Sox9. Proc. Natl. Acad. Sci. U. S. A. 102 (7), 2414–2419. doi:10.1073/pnas.0407510102
Khan, S. A., Sathyanarayan, A., Mashek, M. T., Ong, K. T., Wollaston-Hayden, E. E., and Mashek, D. G. (2015). ATGL-catalyzed lipolysis regulates SIRT1 to control PGC-1α/PPAR-α signaling. Diabetes 64 (2), 418–426. doi:10.2337/db14-0325
Kim, C., and Kim, B. (2018). Anti-cancer natural products and their bioactive compounds inducing ER stress-mediated apoptosis: A review. Nutrients 10 (8), 1021. doi:10.3390/nu10081021
Kim, D., Song, J., and Jin, E. J. (2021). BNIP3-Dependent mitophagy via PGC1α promotes cartilage degradation. Cells 10 (7), 1839. doi:10.3390/cells10071839
Kincaid, B., Bossy-Wetzel, E., and young, F. (2013). Forever young: SIRT3 a shield against mitochondrial meltdown, aging, and neurodegeneration. Front. Aging Neurosci. 5, 48. doi:10.3389/fnagi.2013.00048
Klepacka, J., and Łiu, F. (2006). Ferulic acid and its position among the phenolic compounds of wheat. Crit. Rev. Food Sci. Nutr. 46 (8), 639–647. doi:10.1080/10408390500511821
Kobayashi, M., Harada, S., Fujimoto, N., and Nomura, Y. (2022). Apple polyphenols exhibits chondroprotective changes of synovium and prevents knee osteoarthritis. Biochem. Biophys. Res. Commun. 614, 120–124. doi:10.1016/j.bbrc.2022.05.016
Komori, T. (2016). Cell death in chondrocytes, osteoblasts, and osteocytes. Int. J. Mol. Sci. 17 (12), 2045. doi:10.3390/ijms17122045
Kong, X., Wang, R., Xue, Y., Liu, X., Zhang, H., Chen, Y., et al. (2010). Sirtuin 3, a new target of PGC-1alpha, plays an important role in the suppression of ROS and mitochondrial biogenesis. PLoS One 5 (7), e11707. doi:10.1371/journal.pone.0011707
Lan, H., Hong, W., Qian, D., Peng, F., Li, H., Liang, C., et al. (2021). Quercetin modulates the gut microbiota as well as the metabolome in a rat model of osteoarthritis. Bioengineered 12 (1), 6240–6250. doi:10.1080/21655979.2021.1969194
Li, D., Rui, Y. X., Guo, S. D., Luan, F., Liu, R., and Zeng, N. (2021). Ferulic acid: A review of its pharmacology, pharmacokinetics and derivatives. Life Sci. 284, 119921. doi:10.1016/j.lfs.2021.119921
Li, G., Rao, H., and Xu, W. (2021). Puerarin plays a protective role in chondrocytes by activating Beclin1-dependent autophagy. Biosci. Biotechnol. Biochem. 85 (3), 621–625. doi:10.1093/bbb/zbaa078
Li, J., Wang, Y., Chen, D., and Liu-Bryan, R. (2022). Oral administration of berberine limits post-traumatic osteoarthritis development and associated pain via AMP-activated protein kinase (AMPK) in mice. Osteoarthr. Cartil. 30 (1), 160–171. doi:10.1016/j.joca.2021.10.004
Li, M. Y., Ding, J. Q., Tang, Q., Hao, M. M., Wang, B. H., Wu, J., et al. (2019). SIRT1 activation by SRT1720 attenuates bone cancer pain via preventing Drp1-mediated mitochondrial fission. Biochim. Biophys. Acta Mol. Basis Dis. 1865 (3), 587–598. doi:10.1016/j.bbadis.2018.12.017
Li, Q., Wen, Y., Wang, L., Chen, B., Chen, J., Wang, H., et al. (2021). Hyperglycemia-induced accumulation of advanced glycosylation end products in fibroblast-like synoviocytes promotes knee osteoarthritis. Exp. Mol. Med. 53 (11), 1735–1747. doi:10.1038/s12276-021-00697-6
Li, W., Wang, Y., Tang, Y., Lu, H., Qi, Y., Li, G., et al. (2021). Quercetin alleviates osteoarthritis progression in rats by suppressing inflammation and apoptosis via inhibition of IRAK1/NLRP3 signaling. J. Inflamm. Res. 14, 3393–3403. doi:10.2147/JIR.S311924
Li, Y., Tang, J., and Hu, Y. (2014). Dimethyl fumarate protection against collagen II degradation. Biochem. Biophys. Res. Commun. 454 (2), 257–261. doi:10.1016/j.bbrc.2014.10.005
Li, Z., Zhang, Y., Tian, F., Wang, Z., Song, H., Chen, H., et al. (2020). Omentin-1 promotes mitochondrial biogenesis via PGC1α-AMPK pathway in chondrocytes. Arch. Physiol. Biochem. 25, 1–7.
Lin, C., Liu, L., Zeng, C., Cui, Z. K., Chen, Y., Lai, P., et al. (2019). Activation of mTORC1 in subchondral bone preosteoblasts promotes osteoarthritis by stimulating bone sclerosis and secretion of CXCL12. Bone Res. 7, 5. doi:10.1038/s41413-018-0041-8
Liu, B., Lei, M., Hu, T., Yu, F., Xiao, D. M., and Kang, H. (2016). Inhibitory effects of SRT1720 on the apoptosis of rabbit chondrocytes by activating SIRT1 via p53/bax and NF-κB/PGC-1α pathways. J. Huazhong Univ. Sci. Technol. Med. Sci. 36 (3), 350–355. doi:10.1007/s11596-016-1590-y
Liu, C., Wang, B., Xiao, L., Li, Y., Xu, L., Zhao, Z., et al. (2018). Protective effects of the pericellular matrix of chondrocyte on articular cartilage against the development of osteoarthritis. Histol. Histopathol. 33 (8), 757–764. doi:10.14670/HH-11-967
Liu, D., Cai, Z. J., Yang, Y. T., Lu, W. H., Pan, L. Y., Xiao, W. F., et al. (2022). Mitochondrial quality control in cartilage damage and osteoarthritis: New insights and potential therapeutic targets. Osteoarthr. Cartil. 30 (3), 395–405. doi:10.1016/j.joca.2021.10.009
Liu, L., He, Z., Xu, L., Lu, L., Feng, H., Leong, D. J., et al. (2019). CITED2 mediates the mechanical loading-induced suppression of adipokines in the infrapatellar fat pad. Ann. N. Y. Acad. Sci. 1442 (1), 153–164. doi:10.1111/nyas.14025
Liu, W., Zhang, S. X., Ai, B., Pan, H. F., Zhang, D., Jiang, Y., et al. (2021). Ginsenoside Rg3 promotes cell growth through activation of mTORC1. Front. Cell Dev. Biol. 9, 730309. doi:10.3389/fcell.2021.730309
Liu, Z., Zhang, H., Wang, H., Wei, L., Niu, L., Xu, H. M., et al. (2020). Natural variation and evolutionary dynamics of transposable elements in Brassica oleracea based on next-generation sequencing data. J. Interferon Cytokine Res. 40 (3), 145–151. doi:10.1038/s41438-020-00367-0
Loeser, R. F., Collins, J. A., and Diekman, B. O. (2016). Ageing and the pathogenesis of osteoarthritis. Nat. Rev. Rheumatol. 12 (7), 412–420. doi:10.1038/nrrheum.2016.65
Lv, S., Wang, X., Jin, S., Shen, S., Wang, R., and Tong, P. (2022). Quercetin mediates TSC2-RHEB-mTOR pathway to regulate chondrocytes autophagy in knee osteoarthritis. Gene 820, 146209. doi:10.1016/j.gene.2022.146209
Ma, C. H., Chiua, Y. C., Wu, C. H., Jou, I. M., Tu, Y. K., Hung, C. H., et al. (2018). Homocysteine causes dysfunction of chondrocytes and oxidative stress through repression of SIRT1/AMPK pathway: A possible link between hyperhomocysteinemia and osteoarthritis. Redox Biol. 15, 504–512. doi:10.1016/j.redox.2018.01.010
Ma, C. H., Chou, W. C., Wu, C. H., Jou, I. M., Tu, Y. K., Hsieh, P. L., et al. (2021). Ginsenoside Rg3 attenuates TNF-α-induced damage in chondrocytes through regulating SIRT1-mediated anti-apoptotic and anti-inflammatory mechanisms. Antioxidants (Basel) 10 (12), 1972. doi:10.3390/antiox10121972
Ma, C. H., Wu, C. H., Jou, I. M., Tu, Y. K., Hung, C. H., Hsieh, P. L., et al. (2018). PKR activation causes inflammation and MMP-13 secretion in human degenerated articular chondrocytes. Redox Biol. 14, 72–81. doi:10.1016/j.redox.2017.08.011
Ma, T. W., Wen, Y. J., Song, X. P., Hu, H. L., Li, Y., Bai, H., et al. (2020). Puerarin inhibits the development of osteoarthritis through antiinflammatory and antimatrix-degrading pathways in osteoarthritis-induced rat model. Phytother. Res. 35, 2579–2593. doi:10.1002/ptr.6988
Maneiro, E., Martín, M. A., de Andres, M. C., López-Armada, M. J., Fernández-Sueiro, J. L., del Hoyo, P., et al. (2003). Mitochondrial respiratory activity is altered in osteoarthritic human articular chondrocytes. Arthritis Rheum. 48 (3), 700–708. doi:10.1002/art.10837
Mao, X., Fu, P., Wang, L., and Xiang, C. (2020). Mitochondria: Potential targets for osteoarthritis. Front. Med. (Lausanne). 7, 581402. doi:10.3389/fmed.2020.581402
Masuda, I., Koike, M., Nakashima, S., Mizutani, Y., Ozawa, Y., Watanabe, K., et al. (2018). Apple procyanidins promote mitochondrial biogenesis and proteoglycan biosynthesis in chondrocytes. Sci. Rep. 8 (1), 7229. doi:10.1038/s41598-018-25348-1
Miao, Y., Chen, Y., Xue, F., Liu, K., Zhu, B., Gao, J., et al. (2022). Contribution of ferroptosis and GPX4's dual functions to osteoarthritis progression. EBioMedicine 76, 103847. doi:10.1016/j.ebiom.2022.103847
Miao, Y., Dong, Y., Huang, P., Zhao, X., Huang, Z., Yao, J., et al. (2017). Increasing UCP2 expression and decreasing NOX1/4 expression maintain chondrocyte phenotype by reducing reactive oxygen species production. Oncotarget 8 (38), 63750–63763. doi:10.18632/oncotarget.18908
Minguzzi, M., Cetrullo, S., D'Adamo, S., Silvestri, Y., Flamigni, F., and Borzì, R. M. (2018). Emerging players at the intersection of chondrocyte loss of maturational arrest, oxidative stress, senescence and low-grade inflammation in osteoarthritis. Oxid. Med. Cell Longev. 2018, 3075293. doi:10.1155/2018/3075293
Minor, R. K., Baur, J. A., Gomes, A. P., Ward, T. M., Csiszar, A., Mercken, E. M., et al. (2011). SRT1720 improves survival and healthspan of obese mice. Sci. Rep. 1, 70. doi:10.1038/srep00070
Montori-Grau, M., Aguilar-Recarte, D., Zarei, M., Pizarro-Delgado, J., Palomer, X., and Vázquez-Carrera, M. (2022). Endoplasmic reticulum stress downregulates PGC-1α in skeletal muscle through ATF4 and an mTOR-mediated reduction of CRTC2. Cell Commun. Signal 20 (1), 53. doi:10.1186/s12964-022-00865-9
Nishida, K., Matsushita, T., Takayama, K., Tanaka, T., Miyaji, N., Ibaraki, K., et al. (2018). Intraperitoneal injection of the SIRT1 activator SRT1720 attenuates the progression of experimental osteoarthritis in mice. Bone Jt. Res. 7 (3), 252–262. doi:10.1302/2046-3758.73.BJR-2017-0227.R1
Nugent, A. E., Speicher, D. M., Gradisar, I., McBurney, D. L., Baraga, A., Doane, K. J., et al. (2009). Advanced osteoarthritis in humans is associated with altered collagen VI expression and upregulation of ER-stress markers Grp78 and bag-1. J. Histochem Cytochem 57 (10), 923–931. doi:10.1369/jhc.2009.953893
Olmos, Y., Sánchez-Gómez, F. J., Wild, B., García-Quintans, N., Cabezudo, S., Lamas, S., et al. (2013). SirT1 regulation of antioxidant genes is dependent on the formation of a FoxO3a/PGC-1α complex. Antioxid. Redox Signal 19 (13), 1507–1521. doi:10.1089/ars.2012.4713
Pascarelli, N. A., Collodel, G., Moretti, E., Cheleschi, S., and Fioravanti, A. (2015). Changes in ultrastructure and cytoskeletal aspects of human normal and osteoarthritic chondrocytes exposed to interleukin-1β and cyclical hydrostatic pressure. Int. J. Mol. Sci. 16 (11), 26019–26034. doi:10.3390/ijms161125936
Peng, L., Xie, Z., Pei, J., Wang, B., Gao, Y., and Qu, Y. (2019). Puerarin alters the function of monocytes/macrophages and exhibits chondroprotection in mice. Mol. Med. Rep. 19 (4), 2876–2882. doi:10.3892/mmr.2019.9936
Qiu, L., Luo, Y., and Chen, X. (2018). Quercetin attenuates mitochondrial dysfunction and biogenesis via upregulated AMPK/SIRT1 signaling pathway in OA rats. Biomed. Pharmacother. 103, 1585–1591. doi:10.1016/j.biopha.2018.05.003
Quicke, J. G., Conaghan, P. G., Corp, N., and Peat, G. (2022). Osteoarthritis year in review 2021: Epidemiology and therapy. Osteoarthr. Cartil. 30 (2), 196–206. doi:10.1016/j.joca.2021.10.003
Ratneswaran, A., Sun, M. M., Dupuis, H., Sawyez, C., Borradaile, N., and Beier, F. (2017). Nuclear receptors regulate lipid metabolism and oxidative stress markers in chondrocytes. J. Mol. Med. Berl. 95 (4), 431–444. doi:10.1007/s00109-016-1501-5
Riegger, J., and Brenner, R. E. (2019). Evidence of necroptosis in osteoarthritic disease: Investigation of blunt mechanical impact as possible trigger in regulated necrosis. Cell Death Dis. 10 (10), 683. doi:10.1038/s41419-019-1930-5
Ryu, J. Y., Choi, H. M., Yang, H. I., and Kim, K. S. (2020). Dysregulated autophagy mediates sarcopenic obesity and its complications via AMPK and PGC1α signaling pathways: Potential involvement of gut dysbiosis as a pathological link. Int. J. Mol. Sci. 21 (18), 6887. doi:10.3390/ijms21186887
Saidu, N. E. B., Kavian, N., Leroy, K., Jacob, C., Nicco, C., Batteux, F., et al. (2019). Dimethyl fumarate, a two-edged drug: Current status and future directions. Med. Res. Rev. 39 (5), 1923–1952. doi:10.1002/med.21567
Sasaki, H., Takayama, K., Matsushita, T., Ishida, K., Kubo, S., Matsumoto, T., et al. (2012). Autophagy modulates osteoarthritis-related gene expression in human chondrocytes. Arthritis Rheum. 64 (6), 1920–1928. doi:10.1002/art.34323
So, M. W., Lee, E. J., Lee, H. S., Koo, B. S., Kim, Y. G., Lee, C. K., et al. (2013). Protective effects of ginsenoside Rg3 on human osteoarthritic chondrocytes. Mod. Rheumatol. 23 (1), 104–111. doi:10.1007/s10165-012-0635-8
Sophia Fox, A. J., Bedi, A., and Rodeo, S. A. (2009). The basic science of articular cartilage: Structure, composition, and function. Sports Health 1 (6), 461–468. doi:10.1177/1941738109350438
Sun, J., Li, J. Y., Zhang, L. Q., Li, D. Y., Wu, J. Y., Gao, S. J., et al. (2021). Nrf2 activation attenuates chronic constriction injury-induced neuropathic pain via induction of PGC-1α-mediated mitochondrial biogenesis in the spinal cord. Oxid. Med. Cell Longev. 2021, 9577874. doi:10.1155/2021/9577874
Sun, J., Song, F. H., Wu, J. Y., Zhang, L. Q., Li, D. Y., Gao, S. J., et al. (2022). Sestrin2 overexpression attenuates osteoarthritis pain via induction of AMPK/PGC-1α-mediated mitochondrial biogenesis and suppression of neuroinflammation. Brain Behav. Immun. 102, 53–70. doi:10.1016/j.bbi.2022.02.015
Sun, K., Jing, X., Guo, J., Yao, X., and Guo, F. (2021). Mitophagy in degenerative joint diseases. Autophagy 17 (9), 2082–2092. doi:10.1080/15548627.2020.1822097
Sun, Z., Lu, W., Lin, N., Lin, H., Zhang, J., Ni, T., et al. (2020). Dihydromyricetin alleviates doxorubicin-induced cardiotoxicity by inhibiting NLRP3 inflammasome through activation of SIRT1. Biochem. Pharmacol. 175, 113888. doi:10.1016/j.bcp.2020.113888
Svensson, K., Schnyder, S., Albert, V., Cardel, B., Quagliata, L., Terracciano, L. M., et al. (2015). Resveratrol and SRT1720 elicit differential effects in metabolic organs and modulate systemic parameters independently of skeletal muscle peroxisome proliferator-activated receptor γ Co-activator 1α (PGC-1α). J. Biol. Chem. 290 (26), 16059–16076. doi:10.1074/jbc.M114.590653
Tak, P. P., and Firestein, G. S. (2001). NF-kappaB: A key role in inflammatory diseases. J. Clin. Invest. 107 (1), 7–11. doi:10.1172/JCI11830
Vasheghani, F., Zhang, Y., Li, Y. H., Blati, M., Fahmi, H., Lussier, B., et al. (2015). PPARγ deficiency results in severe, accelerated osteoarthritis associated with aberrant mTOR signalling in the articular cartilage. Ann. Rheum. Dis. 74 (3), 569–578. doi:10.1136/annrheumdis-2014-205743
Villalvilla, A., Gómez, R., Largo, R., and Herrero-Beaumont, G. (2013). Lipid transport and metabolism in healthy and osteoarthritic cartilage. Int. J. Mol. Sci. 14 (10), 20793–20808. doi:10.3390/ijms141020793
Vítek, L. (2020). Bilirubin as a signaling molecule. Med. Res. Rev. 40 (4), 1335–1351. doi:10.1002/med.21660
Wang, F. S., Kuo, C. W., Ko, J. Y., Chen, Y. S., Wang, S. Y., Ke, H. J., et al. (2020). Irisin mitigates oxidative stress, chondrocyte dysfunction and osteoarthritis development through regulating mitochondrial integrity and autophagy. Antioxidants (Basel). 9 (9), 810. doi:10.3390/antiox9090810
Wang, J., Wang, K., Huang, C., Lin, D., Zhou, Y., Wu, Y., et al. (2018). SIRT3 activation by dihydromyricetin suppresses chondrocytes degeneration via maintaining mitochondrial homeostasis. Int. J. Biol. Sci. 14 (13), 1873–1882. doi:10.7150/ijbs.27746
Wang, L., Shan, H., Wang, B., Wang, N., Zhou, Z., Pan, C., et al. (2018). Puerarin attenuates osteoarthritis via upregulating AMP-activated protein kinase/proliferator-activated receptor-γ coactivator-1 signaling pathway in osteoarthritis rats. Pharmacology 102 (3-4), 117–125. doi:10.1159/000490418
Wang, S., Dougherty, E. J., and Danner, R. L. (2016). PPARγ signaling and emerging opportunities for improved therapeutics. Pharmacol. Res. 111, 76–85. doi:10.1016/j.phrs.2016.02.028
Wang, X. P., Xie, W. P., Bi, Y. F., Wang, B. A., Song, H. B., Wang, S. L., et al. (2021). Quercetin suppresses apoptosis of chondrocytes induced by IL-1β via inactivation of p38 MAPK signaling pathway. Exp. Ther. Med. 21 (5), 468. doi:10.3892/etm.2021.9899
Wang, Y., Chen, L. Y., and Liu-Bryan, R. (2021). Mitochondrial biogenesis, activity, and DNA isolation in chondrocytes. Methods Mol. Biol. 2245, 195–213. doi:10.1007/978-1-0716-1119-7_14
Wang, Y., Zhao, X., Lotz, M., Terkeltaub, R., Liu-Bryan, R., Pan, H., et al. (2015). Comparison of arthrodesis with total contact casting for midfoot ulcerations associated with charcot neuroarthropathy. Arthritis Rheumatol. 67 (8), 2141–2148. doi:10.12659/MSM.893677
Wei, B., Zhang, Y., Tang, L., Ji, Y., Yan, C., and Zhang, X. (2019). Protective effects of quercetin against inflammation and oxidative stress in a rabbit model of knee osteoarthritis. Drug Dev. Res. 80 (3), 360–367. doi:10.1002/ddr.21510
Wei, W., He, S., Wang, Z., Dong, J., Xiang, D., Li, Y., et al. (2021). LINC01534 promotes the aberrant metabolic dysfunction and inflammation in IL-1β-simulated osteoarthritic chondrocytes by targeting miR-140-5p. Cartilage 13, 898s–907s. doi:10.1177/1947603519888787
Xia, J., Ma, S., Zhu, X., Chen, C., Zhang, R., Cao, Z., et al. (2022). Versatile ginsenoside Rg3 liposomes inhibit tumor metastasis by capturing circulating tumor cells and destroying metastatic niches. Sci. Adv. 8 (6), eabj1262. doi:10.1126/sciadv.abj1262
Xiong, W., Lan, Q., Liang, X., Zhao, J., Huang, H., Zhan, Y., et al. (2021). Cartilage-targeting poly(ethylene glycol) (PEG)-formononetin (FMN) nanodrug for the treatment of osteoarthritis. J. Nanobiotechnology 19 (1), 197. doi:10.1186/s12951-021-00945-x
Xue, S., Zhou, X., Sang, W., Wang, C., Lu, H., Xu, Y., et al. (2021). Cartilage-targeting peptide-modified dual-drug delivery nanoplatform with NIR laser response for osteoarthritis therapy. Bioact. Mater 6 (8), 2372–2389. doi:10.1016/j.bioactmat.2021.01.017
Yan, N., Xu, Z., Qu, C., and Zhang, J. (2021). Dimethyl fumarate improves cognitive deficits in chronic cerebral hypoperfusion rats by alleviating inflammation, oxidative stress, and ferroptosis via NRF2/ARE/NF-κB signal pathway. Int. Immunopharmacol. 98, 107844. doi:10.1016/j.intimp.2021.107844
Yan, Y., Zhou, X. E., Xu, H. E., and Melcher, K. (2018). Structure and physiological regulation of AMPK. Int. J. Mol. Sci. 19 (11), 3534. doi:10.3390/ijms19113534
Yang, H., Wen, Y., Zhang, M., Liu, Q., Zhang, H., Zhang, J., et al. (2020). MTORC1 coordinates the autophagy and apoptosis signaling in articular chondrocytes in osteoarthritic temporomandibular joint. Autophagy 16 (2), 271–288. doi:10.1080/15548627.2019.1606647
Yang, J., Hu, S., Bian, Y., Yao, J., Wang, D., Liu, X., et al. (2021). Targeting cell death: Pyroptosis, ferroptosis, apoptosis and necroptosis in osteoarthritis. Front. Cell Dev. Biol. 9, 789948. doi:10.3389/fcell.2021.789948
Yang, Q., Shi, Y., Jin, T., Duan, B., and Wu, S. (2022). Advanced glycation end products induced mitochondrial dysfunction of chondrocytes through repression of ampkα-SIRT1-PGC-1α pathway. Pharmacology 107 (5-6), 298–307. doi:10.1159/000521720
Yang, Y., Cai, F., Zhou, N., Liu, S., Wang, P., Zhang, S., et al. (2021). Dimethyl fumarate prevents ferroptosis to attenuate acute kidney injury by acting on NRF2. Clin. Transl. Med. 11 (4), e382. doi:10.1002/ctm2.382
Yang, Y., Wei, J., Li, J., Cui, Y., Zhou, X., and Xie, J. (2021). Lipid metabolism in cartilage and its diseases: A concise review of the research progress. Acta Biochim. Biophys. Sin. (Shanghai). 53 (5), 517–527. doi:10.1093/abbs/gmab021
Yao, Q., Wu, X., Tao, C., Gong, W., Chen, M., Qu, M., et al. (2023). Osteoarthritis: Pathogenic signaling pathways and therapeutic targets. Signal Transduct. Target Ther. 8 (1), 56. doi:10.1038/s41392-023-01330-w
Yao, X., Sun, K., Yu, S., Luo, J., Guo, J., Lin, J., et al. (2021). Chondrocyte ferroptosis contribute to the progression of osteoarthritis. J. Orthop. Transl. 27, 33–43. doi:10.1016/j.jot.2020.09.006
Yu, M., Wang, D., Chen, X., Zhong, D., and Luo, J. (2022). BMSCs-derived mitochondria improve osteoarthritis by ameliorating mitochondrial dysfunction and promoting mitochondrial biogenesis in chondrocytes. Stem Cell Rev. Rep. 18, 3092–3111. doi:10.1007/s12015-022-10436-7
Zeng, Y. F., Wang, R., Bian, Y., Chen, W. S., and Peng, L. (2019). Catalpol attenuates IL-1β induced matrix catabolism, apoptosis and inflammation in rat chondrocytes and inhibits cartilage degeneration. Med. Sci. Monit. 25, 6649–6659. doi:10.12659/MSM.916209
Zhang, G. Z., Deng, Y. J., Xie, Q. Q., Ren, E. H., Ma, Z. J., He, X. G., et al. (2020). Sirtuins and intervertebral disc degeneration: Roles in inflammation, oxidative stress, and mitochondrial function. Clin. Chim. Acta 508, 33–42. doi:10.1016/j.cca.2020.04.016
Zhang, H., Wang, H., Zeng, C., Yan, B., Ouyang, J., Liu, X., et al. (2017). mTORC1 activation downregulates FGFR3 and PTH/PTHrP receptor in articular chondrocytes to initiate osteoarthritis. Osteoarthr. Cartil. 25 (6), 952–963. doi:10.1016/j.joca.2016.12.024
Zhang, J., Chen, Y., Luo, H., Sun, L., Xu, M., Yu, J., et al. (2018). Recent update on the pharmacological effects and mechanisms of dihydromyricetin. Front. Pharmacol. 9, 1204. doi:10.3389/fphar.2018.01204
Zhang, X., Bu, Y., Zhu, B., Zhao, Q., Lv, Z., Li, B., et al. (2018). Global transcriptome analysis to identify critical genes involved in the pathology of osteoarthritis. Bone Jt. Res. 7 (4), 298–307. doi:10.1302/2046-3758.74.BJR-2017-0245.R1
Zhang, Y., Xu, S., Li, K., Tan, K., Liang, K., Wang, J., et al. (2017). mTORC1 inhibits NF-κB/NFATc1 signaling and prevents osteoclast precursor differentiation, in vitro and in mice. J. Bone Min. Res. 32 (9), 1829–1840. doi:10.1002/jbmr.3172
Zhang, Z., Zhang, X., Meng, L., Gong, M., Li, J., Shi, W., et al. (2021). Pioglitazone inhibits diabetes-induced atrial mitochondrial oxidative stress and improves mitochondrial biogenesis, dynamics, and function through the PPAR-γ/PGC-1α signaling pathway. Front. Pharmacol. 12, 658362. doi:10.3389/fphar.2021.658362
Zhao, X., Petursson, F., Viollet, B., Lotz, M., Terkeltaub, R., and Liu-Bryan, R. (2014). Peroxisome proliferator-activated receptor γ coactivator 1α and FoxO3A mediate chondroprotection by AMP-activated protein kinase. Arthritis Rheumatol. 66 (11), 3073–3082. doi:10.1002/art.38791
Zheng, L., Zhang, Z., Sheng, P., and Mobasheri, A. (2021). The role of metabolism in chondrocyte dysfunction and the progression of osteoarthritis. Ageing Res. Rev. 66, 101249. doi:10.1016/j.arr.2020.101249
Zheng, Z., Bian, Y., Zhang, Y., Ren, G., and Li, G. (2020). Metformin activates AMPK/SIRT1/NF-κB pathway and induces mitochondrial dysfunction to drive caspase3/GSDME-mediated cancer cell pyroptosis. Cell Cycle 19 (10), 1089–1104. doi:10.1080/15384101.2020.1743911
Zhou, W., Chen, B., Shang, J., and Li, R. (2021). Ferulic acid attenuates osteoporosis induced by glucocorticoid through regulating the GSK-3β/Lrp-5/ERK signalling pathways. Physiol. Int. 108 (3), 317–341. doi:10.1556/2060.2021.00180
Zhou, Y. X., Zhang, H., and Peng, C. (2014). Puerarin: A review of pharmacological effects. Phytother. Res. 28 (7), 961–975. doi:10.1002/ptr.5083
Glossary
PGC-1α peroxisome proliferator-activated receptor γ cofactor 1α
mtDNA mitochondrial DNA
OA osteoarthritis
ASIR age-standardized incidence rate
ECM extracellular matrix
MQC mitochondrial quality control
AMPK AMP-activated protein kinase
SIRT1 silent information regulator 1
TFAM mitochondrial transcription factor A
NRF1 nuclear respiratory factor 1
NRF2 nuclear respiratory factor 2
SIRT3 silent information regulator 3
SOD2 superoxide dismutases 2
OGG1 8-oxoguanine DNA glycosylase-1
ERRα estrogen-related receptor-α
LKB1 liver kinase B1
FoxO3A forkhead box class O 3A
mTOR mechanistic target of rapamycin
mTORC1 mTOR complex 1
mTORC2 mTOR complex 2
TSC1/2 tuberous sclerosis complex 1/2
ER endoplasmic reticulum
REDD1 regulated in development and DNA damage response 1
PPAR-γ peroxisome proliferator-activated receptor-γ
MMP-13 matrix metalloproteinase-13
NF-κB nuclear factor κB
COX-2 cyclooxygenase-2
OXPHOS oxidative phosphorylation
FGFs fibroblast growth factors
Sesn2 Sestrin2
PRKN parkin RBR E3 ubiquitin protein ligase
BNIP3 Bcl-2/adenovirus E1B 19 kDa interacting protein 3
MUL1 mitochondrial ubiquitin ligase 1
PKR double-stranded RNA-dependent protein kinase R
NOX1/4 NADPH oxidase1/4
UCP2 uncoupling protein 2
AGEs advanced glycation end products
ATGL adipose triglyceride lipase
LXR liver X receptor
CITED2 CBP/p300 interacting transactivator with Glu/Asp rich carboxy-terminal domain 2
Cyt-c cytochrome c
SOX9 sry-related high mobility group-box 9
MAG magnolol
FA ferulic acid
CREB cAMP/responsive element-binding protein
DHM dihydromyricetin
DMF dimethyl fumarate
LEF-1 lymphoid enhancer-binding factor 1
RB@MPMW cartilage-targeting peptide-modified dual-drug delivery nanoplatform with near-infrared laser
Br bilirubin
Keywords: osteoarthritis, chondrocyte, PGC- 1α, metabolism, apoptosis, mitochondria
Citation: Wang H, Su J, Yu M, Xia Y and Wei Y (2023) PGC-1α in osteoarthritic chondrocytes: From mechanism to target of action. Front. Pharmacol. 14:1169019. doi: 10.3389/fphar.2023.1169019
Received: 18 February 2023; Accepted: 30 March 2023;
Published: 06 April 2023.
Edited by:
Sukanya Saha, National Institute of Environmental Health Sciences (NIH), United StatesReviewed by:
Pritam Sadhukhan, Johns Hopkins University, United StatesSourav Panja, Vanderbilt University Medical Center, United States
Tarunendu Mapder, Bristol Myers Squibb, United States
Tarun Barbhuyan, The University of Iowa, United States
Copyright © 2023 Wang, Su, Yu, Xia and Wei. This is an open-access article distributed under the terms of the Creative Commons Attribution License (CC BY). The use, distribution or reproduction in other forums is permitted, provided the original author(s) and the copyright owner(s) are credited and that the original publication in this journal is cited, in accordance with accepted academic practice. No use, distribution or reproduction is permitted which does not comply with these terms.
*Correspondence: Yang Xia, eXhpYUBjbXUuZWR1LmNu; Yingliang Wei, eWx3ZWlAY211LmVkdS5jbg==