- 1Department of Respiratory Medicine, Hospital of Chengdu University of Traditional Chinese Medicine, Chengdu, China
- 2GCP Center, Hospital of Chengdu University of Traditional Chinese Medicine, Chengdu, China
- 3Department of Critical Care Medicine, Hospital of Chengdu University of Traditional Chinese Medicine, Chengdu, China
Background: The incidence of glycolipid metabolic diseases is extremely high worldwide, which greatly hinders people’s life expectancy and patients’ quality of life. Oxidative stress (OS) aggravates the development of diseases in glycolipid metabolism. Radical oxygen species (ROS) is a key factor in the signal transduction of OS, which can regulate cell apoptosis and contribute to inflammation. Currently, chemotherapies are the main method to treat disorders of glycolipid metabolism, but this can lead to drug resistance and damage to normal organs. Botanical drugs are an important source of new drugs. They are widely found in nature with availability, high practicality, and low cost. There is increasing evidence that herbal medicine has definite therapeutic effects on glycolipid metabolic diseases.
Objective: This study aims to provide a valuable method for the treatment of glycolipid metabolic diseases with botanical drugs from the perspective of ROS regulation by botanical drugs and to further promote the development of effective drugs for the clinical treatment of glycolipid metabolic diseases.
Methods: Using herb*, plant medicine, Chinese herbal medicine, phytochemicals, natural medicine, phytomedicine, plant extract, botanical drug, ROS, oxygen free radicals, oxygen radical, oxidizing agent, glucose and lipid metabolism, saccharometabolism, glycometabolism, lipid metabolism, blood glucose, lipoprotein, triglyceride, fatty liver, atherosclerosis, obesity, diabetes, dysglycemia, NAFLD, and DM as keywords or subject terms, relevant literature was retrieved from Web of Science and PubMed databases from 2013 to 2022 and was summarized.
Results: Botanical drugs can regulate ROS by regulating mitochondrial function, endoplasmic reticulum, phosphatidylinositol 3 kinase (PI3K)/protein kinase B (AKT), erythroid 2-related factor 2 (Nrf-2), nuclear factor κB (NF-κB), and other signaling pathways to improve OS and treat glucolipid metabolic diseases.
Conclusion: The regulation of ROS by botanical drugs is multi-mechanism and multifaceted. Both cell studies and animal experiments have demonstrated the effectiveness of botanical drugs in the treatment of glycolipid metabolic diseases by regulating ROS. However, studies on safety need to be further improved, and more studies are needed to support the clinical application of botanical drugs.
1 Introduction
Glycolipid metabolic diseases are a large group of diseases characterized by disorders of glycolipid metabolism, including many diseases, such as diabetes mellitus (DM), non-alcoholic fatty liver disease (NAFLD), obesity, and atherosclerosis (AS). This kind of disease tends to be chronic, which affects the extension of human life to a certain extent and brings a heavy burden to human health cause. According to the statistics of “The International DM Federation,” there will be 536.6 million DM patients worldwide in 2021 (Sun et al., 2022). Studies have found that about 1/4 of the global population suffers from NAFLD (Huang et al., 2021). In addition, the incidence of obesity, AS, hyperlipidemia, and other diseases presents a gradually increasing trend (Barquera et al., 2015; Bluher, 2019; Pirillo et al., 2021). Oxidative stress (OS) plays an important role in the pathogenesis of glycolipid metabolic diseases.
OS is the role of REDOX signal transduction widely existing in organisms (Sies, 2015), which is the imbalance between oxidizing free radicals and antioxidants. It is as broad and important as body PH regulation (Sies et al., 2017). Radical oxygen species (ROS) is a general term for a large group of oxidants extended from molecular oxygen, including superoxide (O2−) and hydrogen peroxide (H2O2) (Sies et al., 2022). There are two main sources of ROS in the body: one is from enzymes and the other is from mitochondria. The enzymes that generate ROS include NADPH oxidase (NOX), lipoxygenase (LOX), and nitric synthase (NOS), among which the most important is NADPH oxidase (Brown and Griendling, 2015). Mitochondrial ROS, as a by-product of the ATP production process, increases during anoxia or mitochondrial dysfunction (Shadel and Horvath, 2015). As a key factor of signal transduction in OS, ROS plays a role in regulating cell proliferation, inflammation, and body aging (Schieber and Chandel, 2014) and progressing diseases related to glucose and lipid metabolism.
2 Association of ROS with common glycolipid metabolic diseases
2.1 ROS and DM
DM is a group of complex metabolic diseases, often manifested by the abnormal metabolism of carbohydrates, fats, and proteins due to the insufficient action of insulin and pancreatic islet B cells (Farmer and Fox, 2011). In the OS mode, ROS and DM are closely related. DM promotes ROS production by reducing intracellular antioxidant levels (Vezza et al., 2021), and ROS accelerates the dysregulation of glucose metabolism and tissue damage through a series of signal transduction.
In DM, ROS can be produced by NADPH oxidase, ER stress (Leenders et al., 2021), mitochondrial stress, and abnormal fatty acid metabolism (Drose and Brandt, 2012; Qiu and Zhang, 2019; Zhou L. et al., 2021; Zhou Y. et al., 2021). Under pathological stimulation, glucose glycolysis produces a large number of ROS, resulting in elevated blood sugar and forming a vicious cycle of hyperglycemia-OS (Qiu and Zhang, 2019).
The accumulation of ROS will damage islet B cells and weaken their function (Blesia et al., 2021). ROS inhibited the expression of insulin promoter factor 1 (Pdx-1) by activating the JNK pathway (Kajimoto and Kaneto, 2004), thus reducing insulin production. In addition, ROS can induce apoptosis of islet B cells by, for example, regulating intracellular Ca2+ concentration (Gier et al., 2009) and consuming heparan sulfate proteoglycan (Dhounchak et al., 2021). In addition, ROS can further cause mitochondrial dysfunction, resulting in reduced proliferation and differentiation of islet B cells (Nahdi et al., 2017). ROS can cause not only islet B-cell dysfunction, but also insulin resistance (IR). On the one hand, ROS can induce insufficient glucose uptake and trigger IR (Bhattacharya et al., 2017) by inhibiting GLUT-4 expression (Yaribeygi et al., 2020). On the other hand, ROS can also impair insulin sensitivity and promote the occurrence of IR by interfering with signaling pathways or downregulating the concentration of signaling molecules (Cheng et al., 2021). In addition, ROS influence on mitochondrial function can lead to IR production (Yaribeygi et al., 2020). Therefore, ROS and DM have a mutually reinforcing relationship.
2.2 ROS and NAFLD
NAFLD covers a wide spectrum of liver injury pathologic spectrum, from general steatosis and steatohepatitis to liver fibrosis and cirrhosis, and it is a typical disorder of lipid metabolism. Currently, there is a “multiple shocks” hypothesis related to the pathogenesis of NAFLD, and OS is a major factor in liver injury and this disease progression (Takaki et al., 2013; Friedman et al., 2018). Abnormal lipid metabolism further promotes ROS production, whereas ROS accumulation aggravates OS, leading to the further development of NAFLD (Mansouri et al., 2018), forming a vicious cycle.
ROS in NAFLD can be produced by mitochondria, and mitochondrial fatty acid oxidative overload leads to increased mitochondrial ROS production. However, the mitochondrial electron transport chain (ETC) complex cannot be upregulated in a coordinated manner, which leads to ROS overproduction (Begriche et al., 2013). In addition, mitochondrial flavoenzymes (including pyruvate dehydrogenase, α-ketoglutarate dehydrogenase, glycerol phosphate, and electron transfer flavoprotein) in mitochondria are considered a major source of ROS (Chen et al., 2020). ER stress is also an important source of ROS in NAFLD. The increase in ROS in the ER is mainly due to the excessive utilization of reduced GSH to reduce the oxidized unfolded protein response (UPR) (Zeeshan et al., 2016) and the increase in UPR-mediated ROS production by the upregulation of CHOP activity (Fuchs et al., 2017).
On the one hand, excessive production of ROS can affect mitochondrial function and lead to abnormal fatty acid oxidation. On the other hand, ROS can damage macromolecules and lead to the production of toxic substances. In addition, ROS and gut microbiota interact to promote liver inflammation. All these contribute to the development of NAFLD (Arroyave-Ospina et al., 2021).
2.3 ROS and AS
AS is a chronic multifactorial inflammatory disease of arterial blood vessels and is the main cause of cardiovascular disease morbidity and mortality. ROS accompanies every step in the development of AS, including the expression of adhesion molecules, stimulation of vascular smooth muscle proliferation and migration, endothelial cell apoptosis, lipid oxidation, and activation of matrix metalloproteinases (Park and Oh, 2011). Matrix metalloproteinases degraded the fibrous wall of AS plaques and the basement membrane of endothelial cells. This leads to the physical destruction and shedding of plaques, ultimately leading to microvascular destruction, microbleeds, and thrombosis (Kattoor et al., 2017).
The generation of ROS in AS mainly depends on various oxidases (Forstermann et al., 2017), among which NADPH oxidases are the main ROS generators in the cardiovascular system, and the deletion of its two subtypes, NOX1 and NOX2, has been confirmed to be related to the reduction of AS in mice (Kattoor et al., 2017). In macrophages, NOX activity plays a role in endothelial adhesion molecule expression, monocyte infiltration, and vascular smooth muscle cell VSMC proliferation (Vendrov et al., 2007). In addition, elevated levels of other enzymes, such AS superoxide anions, were associated with the acceleration of AS (Madamanchi and Runge, 2007). 5/12/15-lipoxygenases are correlated with the occurrence of AS (Cervantes Gracia et al., 2017), which induce NOX activation in vascular endothelial cells and lead to OS. The end product, leukotriene, also has a pro-inflammatory effect.
ROS has multiple effects on the development of AS, including oxidative modification of lipids and DNA, endothelial dysfunction, and the stability of plaque fiber caps (Batty et al., 2022). ROS accumulation increased the content of ox-LDL and the lesion area of AS (Shih et al., 2000). ROS can damage nuclear and mitochondrial DNA (mtDNA) damage, accelerate the development of AS, and increase susceptibility to AS (Yu et al., 2013; Shah et al., 2018). It can also cause endothelial cell inflammation and damage endothelial function (Li and Mehta, 2000). In addition, ROS can promote the production of interleukin to mediate the recruitment of macrophages (Libby, 2017). All these functions play an important role in the development of AS.
2.4 ROS and other glycolipid metabolic diseases
2.4.1 Obesity
Obesity, which is usually defined as being severely over recommended weight levels, is associated with excess accumulation of fat. In obesity, excessive fat accumulation and IR promote ROS production, whereas moderate caloric restriction increases antioxidant activity (Kanikowska et al., 2021). The accumulation of ROS further promotes the occurrence of obesity and its development (Martinez-Martinez and Cachofeiro, 2022).
2.4.2 Hyperlipidemia
Hyperlipidemia is characterized by increased blood lipid levels, including increased LDL and VLDL, and decreased high-density lipoprotein particles. Hypercholesterolemia leads to ROS production; decreases the antioxidant activities of GSH, SOD, and catalase enzymes leading to homocysteine autoxidation; and weakens the intracellular PI3K/AKT pathway due to the decrease in PTP enzymes, JAK/STAT, and PARP. It accelerates endothelial dysfunction (Jamwal and Sharma, 2018). However, the high level of ROS further increases the level of ox-LDL and the severity of hyperlipidemia.
In addition, hypoglycemia (Ceriello et al., 2013) and metabolic syndrome (Ando and Fujita, 2009) are also related to ROS. The overall performance is mutual promotion and mutual influence.
In conclusion, ROS is produced in glycolipid metabolic diseases and plays an important role in the occurrence, development, and deterioration of such diseases (Figure 1). In order to improve the development process of glucose and lipid metabolism diseases, special treatment for ROS in OS is still an emerging direction.
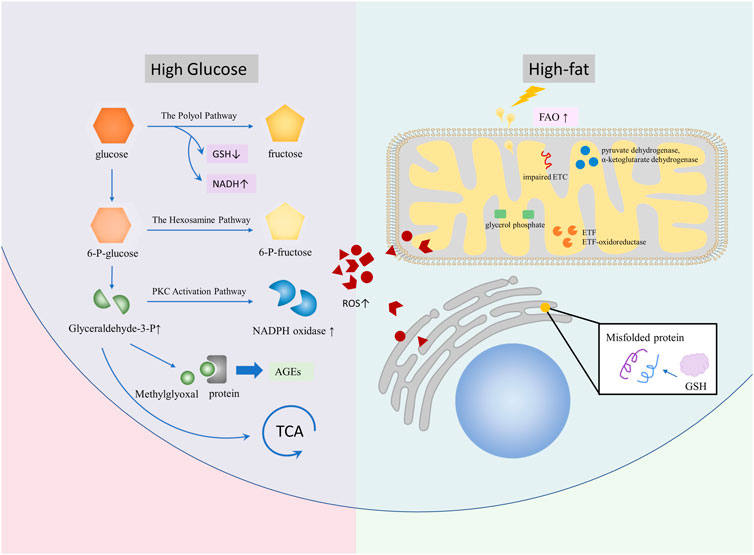
FIGURE 1. Diseases, such as DM, NAFLD, and AS, lead to glycolipid metabolism disorder and promote ROS production. In a high-sugar environment, ROS can generate more ROS via branching off pathways, such as the polyol pathway, the hexosamine pathway, the PKC activation pathway, and AGE, on the basis of glycolysis. In the high-fat environment, ROS is mainly caused by excessive oxidative stress of mitochondria and ER caused by increased FAO. A large number of ROS are involved in glycolipid metabolism, which causes complications.
Some previous drugs for the treatment of glycolipid metabolic diseases by inhibiting OS are metformin (Wang et al., 2017; Apostolova et al., 2020), sodium-glucose co-transporter-2 inhibitors (Trnovska et al., 2021), and statins for the treatment of hypercholesterolemia (Nagila et al., 2009; Carnevale et al., 2010). However, ROS as an entry point for the treatment of glycolipid metabolic diseases is still a new direction. In recent years, nano-targeted drugs targeting ROS have been developed to antagonize or eliminate ROS (Yoshitomi and Nagasaki, 2014; Saifi et al., 2021). However, nanomedicine has not been fully transformed into clinical medication due to the differences in production quality, insufficient large-scale production plans, and the need to evaluate drug safety (Zhang Z. et al., 2022). These reasons make us find safer drugs with excellent anti-OS effects, and botanical drugs give us a new choice.
To determine how botanical drugs modulate ROS in diseases of glycolipid metabolic, herb, plant medicine, Chinese herbal medicine, phytochemicals, natural medicine, phytomedicine, plant extract, botanical drug, ROS, oxygen free radicals, oxygen radical, oxidizing agent, glucose and lipid metabolism, saccharometabolism, glycometabolism, lipid metabolism, blood glucose, lipoprotein, and triglyceride, fatty “liver”, “atherosclerosis”, “obesity OR diabetes”, “dysglycemia”, “NAFLD”, and “DM” were used as keywords or subject headings to search for relevant articles in the Web of Science and PubMed databases from 2013 to 2022. A total of 680 articles were retrieved, of which 126 review articles were excluded and 554 articles were selected. Subsequently, we screened the articles and excluded those with the following conditions: 1) non-medical articles; 2) articles belonging to the scope of medicine but not to the diseases of glucose and lipid metabolism; 3) articles without animal or cell experiments; 4) articles with serious missing experimental data; 5) articles referring only to extracts but not to plant sources; and 6) articles that study combinations composed of various botanical drugs (e.g., decoctions). Finally, 89 articles were included, involving 81 kinds of botanical drugs, which summarized the species and genera of plants and the extraction methods involved in plant extraction. Unfortunately, due to the incomplete information provided by the author, five species of plants have not been found (Table 1) (the screening process is shown in Figure 2).
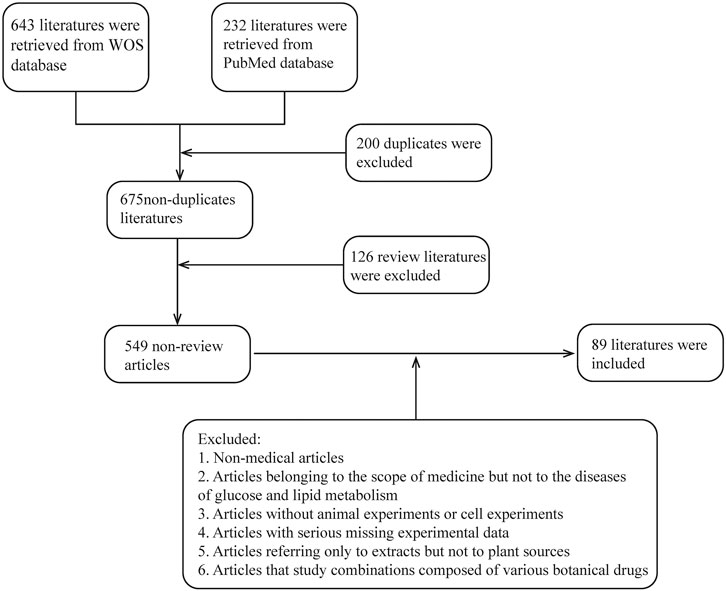
FIGURE 2. Literature screening process; review literature is automatically identified by the database.
(Botanical drugs through the following URL and the relationship between species: https://www.plantplus.cn/cn/sp/Homalium%20zeylanicum; https://www.catalogueoflife.org/; http://mpns.kew.org/mpns-portal/; http://www.plantsoftheworldonline.org).
In the following, we will explain the regulatory effects of botanical drugs on ROS regulation from the direct generation and scavenging of ROS, the regulation of related pathways, and the tolerance to reduce the adverse consequences caused by excessive ROS.
3 Multi-mechanism regulation of ROS by botanical drugs
3.1 Direct modulation of ROS production and scavenging by botanical drugs
Mitochondria, endoplasmic reticulum, and related oxidases are important sources of ROS. Botanical drugs can directly regulate the production of ROS by regulating mitochondria, NADPH oxidase, and endoplasmic reticulum stress. Botanical drugs can also play a role by regulating the activity and expression of anti-OS enzymes (Table 2) (Figure 3).
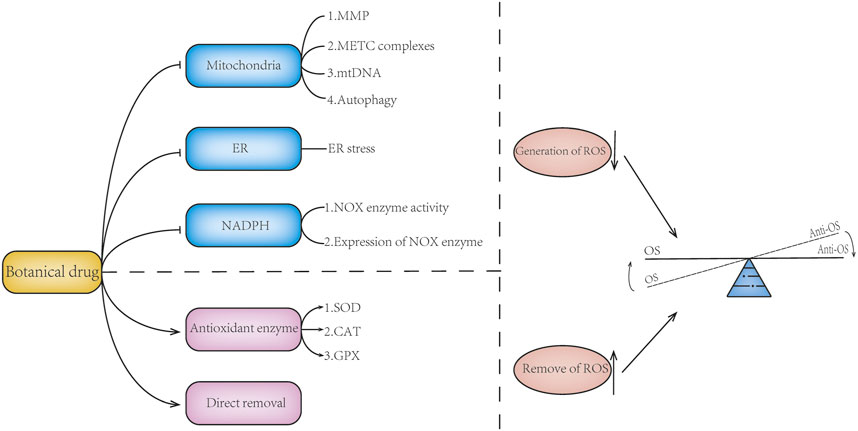
FIGURE 3. Botanical drugs directly modulate ROS generation or scavenging. Botanical drugs can regulate the balance of oxidative stress by reducing the generation of ROS and promoting the removal of ROS. Botanical drugs can reduce ROS production by regulating mitochondrial function, maintaining mitochondrial membrane potential, regulating the expression of mitochondrial ETC complex, protecting mitochondrial DNA, regulating mitophagy, improving ER stress, and reducing NOX enzymes. There are also botanical drugs that can reduce the accumulation of ROS and regulate the balance of oxidative stress by regulating the expression and activity of antioxidant enzymes and scavenging ROS. At the same time, some of the mechanisms of botanicals are not reflected in the aforementioned; for example, botanical drugs can inhibit the expression of LOX enzyme and reduce ROS production.
3.1.1 Botanical drugs reduce mitochondrial ROS production
Mitochondria are an important source of ROS in mammals. Molecular oxygen undergoes single-electron reduction through the ETC to form ROS, which is then converted to H2O2 by SOD in the mitochondrial matrix (Murphy, 2009). This effect is enhanced when cells are under hypoxia, and the ROS produced can cause damage to mitochondrial proteins and DNA (Hamanaka and Chandel, 2010; Juan et al., 2021). Botanical drugs can improve mitochondrial dysfunction and reduce ROS production by maintaining mitochondrial membrane potential (MMP), promoting the expression of mitochondrial respiratory chain complex, and reducing mitochondrial autophagy in vitro and in vivo.
3.1.1.1 Mitochondrial membrane potential
MMP abnormalities lead to ROS production. Previous studies have shown that maintaining a physiological MMP below 140 mv can reduce ROS production, whereas membrane potential hyperpolarization leads to increased ROS production in mitochondrial respiratory chain complexes Ⅰ and Ⅲ (Kaim and Dimroth, 1999; Starkov and Fiskum, 2003; Liu, 2010). Experiments have shown that botanical drugs can restore MMP and reduce ROS production. For example, plants Salvia plebeia and Pueraria lobata (Willd.) Ohwi effectively reversed MMP loss, reduced ROS production, and protected vascular endothelial cells (Gao et al., 2016; Qin et al., 2016). In vitro studies have shown that Laurus nobilis Linn. not only maintains the MMP but also increases insulin sensitivity (Bourebaba et al., 2021). Tinospora sinensis maintains MMP while reducing islet B-cell apoptosis (Banerjee et al., 2020).
3.1.1.2 Mitochondrial ETC complex
Mitochondrial ETC complexes exist in the mitochondrial and membrane space (Muller et al., 2004) and play an important role in the formation of ROS. For mitochondrial complex Ⅰ in the mitochondrial matrix, when the NADH/NAD ratio increases, the FMN binding site on complex Ⅰ decreases and superoxide is formed (Murphy, 2009). Mitochondrial complex Ⅲ is located in the inner mitochondrial membrane, which produces superoxide through the Q cycle (Hamanaka and Chandel, 2010). In vitro and in vivo studies have shown that botanical drugs can improve ROS production by regulating the expression of mitochondrial respiratory chain complexes. For example, latex protein from Calotropis procera increased the expression of mitochondrial complexes I, III, and V; improved glucose tolerance; and inhibited hepatic glucose production in mice. This makes it a potential agent for the treatment of type 2 DM (De Oliveira et al., 2021).
3.1.1.3 Mitochondrial DNA
MtDNA damage is closely related to mutation and ROS production (Passos et al., 2007). In vitro and in vivo studies have found that Trigonella foenum-graecum seeds can protect the mtDNA of 3T3-L1 adipocytes from the damage caused by ROS induced by high glucose in vitro (Luan et al., 2018). Zingiber officinale Roscoe’s steamed ginger extract can enhance the mtDNA content and increase thermogenesis to reduce the body weight of HFD-fed mice, which has a certain effect on improving obesity (Lee et al., 2021).
3.1.1.4 Mitochondrial autophagy
Excessive accumulation of ROS leads to mitochondrial autophagy (Schofield and Schafer, 2021). Studies have shown that botanical drugs can maintain mitochondrial function by reducing mitophagy. For example, Epimedium and Cordyceps sinensis reduce mitophagy in vivo (Zhang et al., 2020; Ku et al., 2021), and Hibiscus hibiscus increased mitochondrial mass and content of various cell types, activated AMPK pathway, reduced mitochondrial autophagy and alleviated OS induced by glucose lipid toxicity (Herranz-Lopez et al., 2020).
In addition, mitochondrial phosphorylation is an important mechanism for mitochondrial energy production, and electron transport and ATP formation in mitochondrial ETC are related to mitochondrial phosphorylation (Foo et al., 2022). Some botanical drugs have the effect of regulating mitochondrial phosphorylation. As mentioned earlier, the plant L. nobilis Linn. plays a role in regulating mitochondrial phosphorylation and MMP (Bourebaba et al., 2021). However, there is a close relationship between the regulation of mitochondrial dysfunction and mitochondrial phosphorylation in plants. However, few studies have focused on the effect of botanical drugs on mitochondrial phosphorylation separately.
3.1.2 Botanical drugs downregulated NADPH expression to reduce ROS production
NADPH oxidase is another major source of ROS. NOX enzymes are membrane-associated proteins that transfer electrons across biofilms, allowing molecular oxygen to accept electrons to form ROS (Bedard and Krause, 2007). The effect of botanical drugs on NOX enzymes is more common in AS. As demonstrated in vitro, treatment of HUVECs with Astragalus mongholicus can reduce the activity of the NOX enzyme and the level of ROS under oxLDL conditions (Zhu et al., 2019). In vivo studies have found that Lindera obtusiloba extract can reduce the expression of NOX oxidase subunits p22phox and p47phox and inhibit NOX activity (Ihm et al., 2021). Prosopis strombulifera, Tessaria absinthioides (Quesada et al., 2021), and Salvia miltiorrhiza significantly inhibited the expression of NOX2 and NOX4 (Zhao W. W. et al., 2017; Zhao et al., 2019), while reducing the activation of NF-κB and the expression of vascular adhesion factors and protecting vascular endothelial cells to play an anti-AS role. Regarding DN, extracts from Silybum marianum downregulated the activity of the NOX enzyme and reduced ROS production, which played a protective role in podocytes (Khazim et al., 2013).
3.1.3 Botanical drugs alleviate ER stress to reduce ROS production
The accumulation of unfolded proteins in the ER has been termed ER stress (Gardner and Walter, 2011). On the one hand, ER stress can produce ROS through NOX4 and microsomal monooxygenase systems (Zeeshan et al., 2016). On the other hand, ER stress can aggravate mitochondrial dysfunction and produce ROS by regulating Ca ions (Bhandary et al., 2012).
In vitro and in vivo experiments showed that Z. officinale and Toxicodendron vernicifluum could reduce ROS production through mTOR/SREBP1/ER stress and then improve fat metabolism to reduce body weight in high-fat diet mice (Hoang et al., 2021; Lee et al., 2021). Curcuma can alleviate ER stress and reduce MIN6 cell apoptosis by interfering with the PERK/CHOP pathway (Cao et al., 2022). In addition, Momordica charantia also has anti-ER stress and reduces ROS generation (Hsu et al., 2021).
In addition to the aforementioned mechanisms, some botanical drugs can play a role in alleviating AS by regulating the LOX enzyme, such as S. miltiorrhiza (Liu et al., 2015) and Tripterygium wilfordii Hook F. (Gu et al., 2013).
3.2 Scavenging effect of botanical drugs on ROS
Antioxidant enzymes are an important mechanism for the body to cope with the excessive accumulation of ROS. Botanical drugs can play an anti-ROS effect by activating antioxidant enzymes. At the same time, they can play an anti-ROS effect via their reaction with ROS to contribute to alleviating glycolipid metabolic diseases (Table 3).
3.2.1 Botanical drugs activate antioxidant enzymes to reduce ROS accumulation
Antioxidant enzymes are located in the middle of one of the three layers of antioxidant defense (Lei et al., 2016). These enzymes include SOD, CAT, and GPX. The SOD is a group of metal-containing enzymes that play a crucial antioxidant role in human health. ROS can be converted into H2O2 under the action of the SOD (Buettner, 2011), which is then decomposed into water by CAT and GPX (Kirkman and Gaetani, 2007; Bhabak and Mugesh, 2010).
Studies have shown that it is effective and feasible to intervene in OS-related diseases with antioxidant enzymes as the key point. For example, the use of oral antioxidant enzymes can enhance ROS clearance and reduce inflammatory responses (Zeng et al., 2021). Treating T2DM rats with Homalium zeylanicum and Padina pavonia can enhance the activity of antioxidant enzymes, reduce ROS production, and protect islet B cells (Germoush et al., 2020; Rout et al., 2020). In vivo experiments have found that Enicostemma littorale Blume can enhance the activity of antioxidant enzymes, reduce ROS production, and treat DM (Srivastava et al., 2016). Moreover, some botanical drugs can enhance the activity and expression of antioxidant enzymes and treat DM-related complications (Mihailovic et al., 2021). For example, Inonotus obliquus and Artemisia caruifolia can improve diabetic kidney damage (Li Y. et al., 2021; Sekiou et al., 2021); Parkia biglobosa seeds and Picrorhiza kurroa could improve heart damage in DM mice; and the activities of GPX, SOD, and CAT were higher after their intervention (Gimenes et al., 2018; Ogunyinka et al., 2019). The CAT was significantly increased in Hypoxis hemerocallidea diabetic rats, which showed anti-hyperglycemic and antioxidant effects (Oguntibeju et al., 2016). All these indicate that botanical drugs play a protective role against DM nephropathy (DN) or heart disease by interfering with antioxidant enzymes.
In AS, in vitro experiments found that the Romina strawberry variety (AN99.78.51), which is commonly consumed, can activate antioxidant enzymes and damage HepG2 cells (Forbes-Hernandez et al., 2017). Plants such as Amaranthus viridis and Moringa oleifera also showed excellent enhancement of antioxidant enzyme activity in vivo (Salvamani et al., 2016; Omodanisi et al., 2017). In vivo experiments found that the flower extract of Cassia auriculata could improve hyperlipidemia and the activity of various antioxidant enzymes without obvious adverse reactions (Vijayaraj et al., 2013).
3.2.2 Direct scavenging effect of botanical drugs on ROS
Antioxidants can directly or indirectly inhibit cell damage caused by OS. Antioxidants scour ROS by providing hydrogen or electron antioxidants, and ROS and reactive nitrogen are thought to be a direct pathway (Dinkova-Kostova and Talalay, 2008). Polyphenol extracts from plants have obvious advantages in the removal of ROS and play a role by removing chelating metal ions of ROS (Yahfoufi et al., 2018). For example, Alnus firma can eliminate ROS produced during fat accumulation and adipose differentiation in 3T3-L1 cells (Choi et al., 2020), and Rumex dentatus L. can improve OS in DM rats by scavenging ROS (Elsayed et al., 2020). In addition, other extracts have a significant free radical scavenging ability; for example, in vitro experiments found that Lannea coromandelica bark extract (Alam et al., 2017), Annickia polycarpa (Lartey et al., 2021), Eugenia jambolana, Galega officinalis, and Smallanthus sonchifolius also had significant ROS clearance ability (Liu et al., 2018; Hachkova et al., 2021).
ROS is a key factor in the OS. Botanical drugs can regulate OS by regulating ROS generation and clearance so that ROS levels tend to be balanced. At the same time, this effect can also play a role by indirectly regulating the up–down pathways related to ROS, which will be described as follows.
3.3 Upstream and downstream regulation related to ROS by botanical drugs
Botanical drugs can regulate ROS accumulation and improve the damage caused by ROS accumulation by regulating upstream signals of ROS, such as PI3K signaling pathway and inflammatory factor (tumor necrosis factor) TNF-α, and downstream signals, such as AMPK, NF-κB, and Nrf-2 (Figure 4).
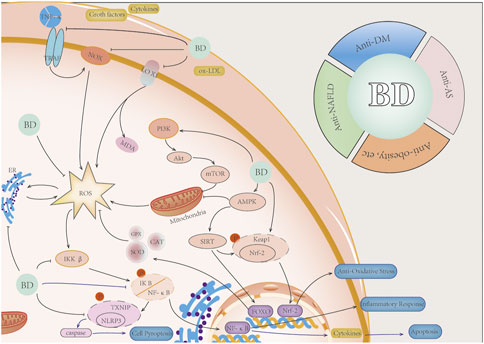
FIGURE 4. Botanical drugs regulate ROS-related pathways and corresponding anti-inflammatory effects. Botanical drugs can regulate mitochondrial function and reduce ROS production by regulating P13K/AKT/mTOR pathway. Botanical drugs can regulate TNF-α, cytokines, growth factors, and other signaling molecules and reduce the generation of ROS. Botanical drugs can regulate the AMPK pathway, improve mitochondrial energy metabolism, and reduce ROS production. Botanical drugs can regulate the Nrf-2 pathway, activate FOXO and other genes, and increase the expression of antioxidant enzyme genes. Botanical drugs can reduce ROS-induced apoptosis and caspase activation-induced pyroptosis by inhibiting the NF-κB/NLRP3 pathway. Botanical drugs eventually played a role in anti-oxidation, anti-inflammation, anti-apoptosis, and anti-pyroptosis. It has a therapeutic effect on NAFLD, DM, AS, and obesity, among others.
3.3.1 Botanical drugs regulate the upstream signal of ROS and reduce the accumulation of ROS
Botanical drugs can play a regulatory role in regulating ROS by regulating PI3K/AKT pathway and inflammatory factors (Table 4).
3.3.1.1 The PI3K/AKT pathway
Studies have shown that the PI3K/AKT pathway is correlated with ROS, and activated AKT can reduce the production of ROS caused by ischemia (Chatterjee et al., 2012). The mechanism is related to the regulation of mitochondrial function and NOX enzyme by the PI3K/AKT (Stiles, 2009; Nakanishi et al., 2014).
In recent studies, treatment targeting the PI3K/AKT pathway has a certain effect on glycolipid metabolic diseases, such as obesity and DM (Huang et al., 2018). Moderate pharmacological inhibition of the PI3K could be a therapeutic strategy for obesity and metabolic syndrome (Vanhaesebroeck et al., 2021). Moreover, PI3K/AKT is the main pathway of botanical drugs regulating OS-related glycolipid metabolic disorders. For example, botanical drugs can improve DN by regulating the PI3K pathway (Tang et al., 2021). In vitro experiments have shown that Alpinia officinarum Hance can improve the IR of HepG2 cells through the PI3K/AKT pathway (Zhang X. G. et al., 2022). Trigonella foenum-graecum seeds restored dexamethasone-induced glucose uptake in IR3T3-L1 cells by activating the AKT and AMPK (Luan et al., 2018). Rubus amabilis active ingredients treat MINI6β cells to regulate the activation of the AKT/FoxO1 pathway and play an anti-apoptotic role (Sun et al., 2020). Epimedium can reduce mitochondrial autophagy of cavernous smooth muscle cells through PI3K/AKT/mTOR pathway and improve glucose metabolism and ROS production in T2DM rats with erectile dysfunction (Zhang et al., 2020).
For AS, in vivo experiments showed that Herba Erigerontis could upregulate the expression of Hipo/FoxO3a and PI3K/AKT and reduce the occurrence of OS in vascular endothelial cells in high-fat diet rats (Fu et al., 2019). In vitro, S. miltiorrhiza Bunge has been found to reduce the production of intracellular reactive oxygen species through the PI3K/Akt/MEK1/Nrf-2 pathway and has therapeutic effects on vascular diseases (Lee et al., 2012). Lithospermum erythrorhizon and Tribulus terrestris can inhibit OS and protect vascular endothelial cells by activating the PI3K/AKT pathway and inhibiting the NF-κB pathway, and L. erythrorhizon Sieb. et Zucc. can also inhibit the NF-κB pathway activation and improve inflammation (Huang et al., 2015; Jiang et al., 2016). Ginkgo biloba Linn. extract can reduce ROS production by activating the AKT/eNOS and play a protective role in endothelial cells in vitro (Tsai et al., 2013).
Botanical drugs can reduce the excessive production of ROS; can improve IR, vascular endothelial cell injury, and islet B-cell injury by regulating the PI3K/AKT pathway; and have certain therapeutic effects on DM, AS, NAFLD, and other diseases.
3.3.1.2 TNF-α and other inflammatory factors
ROS production can be stimulated by signal molecules, such as growth factors, cytokines, and circulating exosomes (Sies and Jones, 2020). For example, TNF-α, circular RNA, and interleukin-4 induce ROS production (Lee et al., 2005; Sharma et al., 2008; Saaoud et al., 2021). Meanwhile, the crosstalk between inflammation and OS makes inflammatory factors, other information molecules, and ROS have inextricable relationships (Forrester et al., 2018). All these provide a rationale for reducing ROS production by inhibiting the expression of inflammatory factors and cytokines.
Previous studies have shown that botanical drugs have significant regulatory effects on signaling molecules, such as inflammatory factors in glucolipid metabolism diseases. In vitro and in vivo experiments have shown that botanical drugs can inhibit the overexpression of TNF, vascular adhesion factor (VCAM), and cytokines; reduce ROS generation and vascular endothelial damage caused by them; and have certain therapeutic effects on AS (Gu et al., 2013; Caliceti et al., 2017; Su et al., 2018). TNF-α can activate NOX4 to induce ROS generation, and S. miltiorrhiza can inhibit TNF-α-induced ROS generation through hydrogen bond interaction with NOX4, the production of inflammatory factors, and the release of VCAM-1 (Zhao W. W. et al., 2017). Gynura bicolor can reduce the effects of TNF-α in several ways (Goboza et al., 2019), and Catharanthus roseus can reduce TNF-α levels to reduce ROS (Hsieh et al., 2020). In vitro experiments of Prunella vulgaris and in vivo experiment of Ligusticum chuanxiong Hort. showed that both plants suppressed OS and reduced inflammation in human vascular smooth muscle cells following TNF-α treatment (Park et al., 2013; Li et al., 2014). In addition, botanical drugs can interfere with the activity of TNF-α receptor TRAF to interfere with OS. For example, Syzygium aqueum Alston was found to reduce the damage of OS and inflammation on islet B cells by downregulating the expression level of the TRAF6, NF-κB-mediated inflammation, and the effect of TNF-α (Mahmoud et al., 2021a). In addition, berberine and S. miltiorrhiza extracts can attenuate TNF-α-induced phosphorylation of IkBα, reduce inflammation and OS, and protect vascular endothelial cells (Zhao W. W. et al., 2017; Caliceti et al., 2017).
3.3.2 Botanical drugs regulate the downstream pathways of ROS and reduce the accumulation of ROS
Botanical drugs can regulate ROS downstream pathways (e.g., the AMPK pathway, the NF-κB/NLRP3 inflammasome pathway, and the Nrf-2 pathway) to reduce cell damage caused by excessive ROS, regulate inflammation, or activate the expression of antioxidant enzymes (Table 5).
3.3.2.1 The AMPK pathway
The AMPK pathway is an important regulator of energy metabolism in the body, which can regulate substance metabolism and synthesis and induce autophagy (Zhao Y. et al., 2017). Currently, all mechanisms by which the AMPK is activated by ROS are not fully understood (Ren and Shen, 2019). However, it has been shown that exogenous or glucose oxidase-generated H2O2 induces direct S-glutathionylation of cysteine residues Cys299 and Cys304 on the AMPKα subunit. It has been demonstrated that ROS can directly activate the AMPK pathway (Zmijewski et al., 2010).
Botanical drugs have a certain effect on improving lipid metabolic diseases by regulating the AMPK pathway. For example, the use of T. vernicifluum and Z. officinale extracts can effectively reduce ROS levels and highly maintain the AMPK/SIRT1 signaling, and Z. officinale extracts could inhibit hepatic dyslipidemia and regulate lipid metabolism (Hoang et al., 2021; Lee et al., 2021). In vitro and in vivo experiments have found that purpurin derived from Rubia tinctorum L. and Rubia cordifolia can exert anti-obesity effects through the AMPK pathway (Nam et al., 2019). For NAFLD, Dillenia indica leaf and Alisol A 24-acetate treatment significantly increased the expression of the AMPK/SIRT pathway, reduced ROS production, and had a certain protective effect on hepatocytes (Wu et al., 2018; Poornima et al., 2022).
Moreover, the intervention of AMPK has significant benefits in improving DM. Trigonella foenum-graecum reduces the incidence of IR and restores glucose uptake and insulin sensitivity in adipocytes by activating the AKT and AMPK, which are inhibited by dexamethasone. Phosphorylation of AKT/AMPK induced by Premna herbacea extract activates AS106 cells and increases glucose uptake by muscle cells (Li et al., 2018; Luan et al., 2018; Kashyap et al., 2021). In vivo, Aspalathus linearis was found to increase AMPK phosphorylation, reduce intracellular ROS production in islet B cells, and increase glucose uptake in myocytes (Kamakura et al., 2015). In addition, some botanical drugs can regulate the expression of adipogenic genes, such as PPAR and SREBP-1c, and reduce the production of inflammatory factors while regulating OS and AMPK (Herranz-Lopez et al., 2020; Lee et al., 2021).
3.3.2.2 The NF-κB pathway
NF-κB is an intracellular transcription factor that plays an important role in immunity and inflammation (Vallabhapurapu and Karin, 2009). Studies have shown that high glucose induces the production of ROS and stimulates the phosphorylation of IKKβ and NF-κB p65, but the phosphorylation of IKKβ is inhibited when ROS inhibitors are used, suggesting that ROS can promote the phosphorylation of IKKβ/NF-κB (Qin et al., 2016). Moreover, H2O2 can also directly regulate NF-κB (Sies and Jones, 2020).
In AS, NAFLD, and obesity, typical disorders of lipid metabolism, botanical drugs attenuate ROS and inflammation and reduce cell damage and apoptosis by regulating the NF-κB pathway. For example, in vitro experiments showed that pretreatment of HUVECs with Cnidium monnieri and Angelica gigas Nakai inhibited the NF-κB nuclear displacement caused by TNF-α stimulation and downregulated VACM expression and ROS generation (Choi et al., 2018). In vitro and in vivo experiments have found that the fruits of Scutellaria baicalensis Georgi and Rubus coreanus Miq. can reduce vascular inflammation by inhibiting ROS production and the NF-κB pathway and have a therapeutic effect on AS (Kim et al., 2013; Ku and Bae, 2015). Anoectochilus roxburghii and Cistanche tubulosa can protect vascular endothelial cells and reproductive function in diabetic rats by inhibiting the NF-κB pathway (Liu et al., 2017; Kong et al., 2018). For DM, Ganoderma lucidum can protect islet B cells by inhibiting NF-κB, JNK, and MAPK pathways (Liang et al., 2020). In vitro experiments have found that Anoectochilus roxburghii inhibits NF-κB to reduce ROS production and has a certain therapeutic effect on diabetic vasculopathy (Liu et al., 2016).
3.3.2.3 The NLRP3 inflammasome
For NLRP3 inflammasome, ROS and NF-κB activate the NLRP3 inflammasome (Tschopp and Schroder, 2010). Studies have shown that ROS can activate NLRP3 inflammasome via TXNIP, and TXNIP/NLRP3 activation is inhibited after ROS clearance (Dan Dunn et al., 2015; Mai et al., 2020). The NLRP3 inflammasome plays an important role in the development of DM, NAFLD, and AS (Hoseini et al., 2018). Excessive activation of NLRP3 will lead to the excessive release of inflammatory factors and pyrosis of cells (Jin et al., 2021a). It has been documented that AS is a key target for treating NAFLD, AS, and DM (Tang et al., 2019; Wu et al., 2021; Yu et al., 2022). Here, it has been proved that the active ingredients of botanical drugs can treat diabetes and its complications through NLRP3, and the botanical drugs and active ingredients are summarized, so we will not elaborate on this (Bai et al., 2021).
Some botanical drugs can target NLRP3 during AS and exert their effects, as found in vivo, S. plebeia can improve high glucose-mediated endothelial dysfunction by inhibiting PKCβII-related NLRP3 inflammasome activation and NF-κB signaling (Qin et al., 2016). Sophora flavescens can alleviate the NLRP3-mediated apoptosis in HUVECs stimulated by LDL (Jin et al., 2021b). Crataegus aronia inhibited the levels of NLRP3, caspase-1, and mature IL-1β in aortic tissues of high-fat diet rats and reduced the nuclear accumulation of NF-κB. Thus, it plays a role in reducing ROS (Shatoor and Al Humayed, 2020). All of the aforementioned plants can alleviate AS, which is worth exploring in depth. For NAFLD, some botanical drugs can target NLRP3 to exert anti-NAFLD effects, such as Antrodia Cinnamomea (Yen et al., 2020), Artemisia capillaris (Li B. et al., 2021), Juglans regia green husk (Fang et al., 2022), and extracts of Cannabis sativa (Jiang et al., 2021), which were found to reduce ROS, inhibit the NLRP3 in vitro and in vivo, and have a certain therapeutic effect on NAFLD.
3.3.2.4 The Nrf-2 pathway
Nrf-2 is also a nuclear factor in the cytoplasm and an important antioxidant factor in the body. It plays an important role in regulating ROS levels (Kaspar et al., 2009). The genes regulated by Nrf-2 include HO-1, GST, and NQO1, and their expressed enzymes have antioxidant effects (Liu et al., 2007). Current studies have found that targeting Nrf-2 has a clear effect on the treatment of cancer, DM and its complications, and AS (Axelsson et al., 2017; Niu et al., 2019; Sivinski et al., 2021).
In DM and its complications, botanical drugs can regulate the expression of antioxidant enzymes through Nrf-2, be antioxidant and anti-inflammatory, and reduce apoptosis. For example, in vitro experiments showed that the Nrf-2 expression was significantly enhanced and ROS was significantly reduced under the treatment of I. obliquus and G. biloba extract, which improved renal podocyte injury caused by DN (Li Y. et al., 2021; Chang et al., 2021). Similarly, the G. biloba extract reduced retinal damage in diabetic rats by activating Nrf-2 in vivo (Su et al., 2022). Hydrangea paniculata can exert beneficial effects on DN by increasing the Nrf-2 expression and inhibiting TGF-smad signaling activation (Zhang et al., 2019). In vitro experiments showed that the ethyl acetate fraction of Penthorum chinense Pursh stems could directly bind to the Keap1 protein, resulting in nuclear translocation of Nrf-2 and activation of antioxidant-related proteins (Sun et al., 2021). Scutellaria baicalensis root improves hyperglycemia-induced vascular endothelial injury by promoting the Nrf-2 nuclear enrichment (Chen et al., 2019). For DM peripheral neuropathy, Paeonia lactiflora Pall. pretreatment of RSC96 cells showed that P. lactiflora Pall. could inhibit the ROS production induced by high glucose and reduce the apoptosis of RSC96 cells through Nrf-2 (Yang et al., 2016).
The activation of Nrf-2/HO-1 signaling plays an important role in protecting endothelial cells (Zhang et al., 2021). Botanical drugs can treat AS by interfering with Nrf-2, which can protect vascular endothelial cells, anti-inflammation, and anti-oxidation. For example, when Panax ginseng and Ginsenoside Rb1 were used to treat AS rats, serum NO and SOD levels were upregulated, and Nrf-2 nuclear translocation and HO-1 activation were observed. Moreover, Ginsenoside Rb1 inhibited oxLDL-induced p38 and VCAM-1 expression and reduced the adhesion of monocytes to vascular endothelial cells (Fan et al., 2016).
In addition, the intervention of botanical drugs on NAFLD can play an anti-inflammatory and anti-oxidative effect by regulating the expression of Nrf-2 and protecting liver cells. As found in in vivo and in vitro experiments, intervention with Ilex Chinensis can increase the expression of the Nrf-2 and Keap1 genes, reduce ROS production, and have a certain improvement effect on NAFLD of zebrafish larvae (Deng et al., 2021).
3.4 Botanical drugs alleviated inflammation, apoptosis, and IR caused by ROS
Abnormal levels of ROS often cause problems other than OS, such as cell apoptosis, IR, and tissue inflammation. These pathological conditions interact with OS and aggravate the development of the disease. Botanical drugs can improve these problems caused by ROS abnormalities. Later, we will explain the effects of related drugs from the perspective of regulating ROS and anti-apoptosis and improving IR and anti-inflammation (Table 6).
3.4.1 Botanical drugs regulate ROS-associated apoptosis and pyroptosis
3.4.1.1 Apoptosis
Excessive accumulation of ROS can lead to impaired mitochondrial function, lipid peroxidation, decreased ATP level, and, finally, cell necrosis (Orrenius et al., 2007). Moreover, ROS can induce apoptosis by mediating the oxidation of cardiolipin and promoting the release of cytochrome C from mitochondria. This demonstrates the bridge-like role of ROS between apoptosis, necrosis, and OS (Kaminskyy and Zhivotovsky, 2014). Botanical drugs can play an anti-apoptotic role by regulating the expression of pro- and anti-apoptotic genes. In vitro and in vivo studies have found that plants Crassocephalum crepidioides and T. sinensis can exert antioxidant and anti-apoptotic effects and reduce islet B-cell apoptosis, thereby improving DM (Bahar et al., 2017; Banerjee et al., 2020). Nepeta angustifolia C. Y. Wu can improve DN through anti-apoptotic effects (Huang et al., 2020).
3.4.1.2 Pyroptosis
Pyroptosis is a novel mode of programmed cell death characterized by the dependence on inflammatory caspases and the formation of activated gasdermin-D pores in the plasma membrane, eventually leading to cell rupture and the release of cytokines (Shi et al., 2017). NLRP3 plays an important role in pyroptosis (Sutterwala et al., 2006), so the regulation of ROS production and the activation of NLRP3 play an important role in anti-pyroptosis. In in vitro and in vivo studies of NAFLD, berberine has been shown to reduce pyroptosis by regulating the ROS/NLRP3 pathway (Mai et al., 2020).
3.4.2 Botanical drugs alleviated ROS-mediated IR
As discussed previously, the accumulation of ROS will induce IR, and botanical drugs increase cellular insulin sensitivity. In the hyperglycemia environment, L. nobilis Linn. extract could improve the decrease in INSR, AKT, and PI3K protein abundance induced by high insulin in HepG2 cells, and it alleviated IR while reducing ROS (Bourebaba et al., 2021). Premna herbacea improved IR, enhanced glucose uptake, and reduced ROS production in rat skeletal muscle cells through JNK/AKT/mTOR signaling (Kashyap et al., 2021). For T2DM, A. officinarum Hance can improve IR, as indicated by increased glucose uptake and glucose consumption in HepG2 cells, and this effect occurs through the PI3K/AKT/Nrf-2/GSK3β pathway (Zhang X. G. et al., 2022). Herba Epimedii alleviates IR by regulating the IRS1/AKT signal transduction pathway in db-/db-mice (Li et al., 2022). At the same time, this corroborates the importance of PI3K/AKT in combating IR (Huang et al., 2018).
3.4.3 The effects of botanical drugs against the inflammation associated with ROS
OS and inflammation play an important role in the development of abnormal glycolipid metabolic disease. ROS can also induce inflammation (Lei et al., 2015), so the regulation of ROS is also reflected in inflammation. Syzygium jambos bark extract protected islet B-cell in DM rats through IRS-2/AKT/GLUT4, ameliorated the elevation of TNF-α and IL-10, and exerted a regulatory effect on inflammation and OS (Mahmoud et al., 2021b). In vivo experiments have found that Dracaena cochinchinensis (Lour.) S. C. Chen and Moutan Cortex play anti-inflammatory and anti-oxidative effects by downregulating inflammatory factors and ROS levels and have certain therapeutic effects on DM (Chen et al., 2013; Zhang et al., 2014). In vivo and in vitro experiments of botanical drugs for the treatment of NAFLD have shown that berberine can control the release of inflammatory factors through ROS/NLRP3, improve the inflammation of NAFLD, protect hepatocytes, and slow the progression of the disease (Mai et al., 2020). In AS, Morinda citrifolia (Ishibashi et al., 2017), Echinodorus grandiflorus (Gasparotto et al., 2019), Dendrobium huoshanense (Fan et al., 2020), and Dendrobium officinale (Han et al., 2021) also play an antioxidant and anti-inflammatory role.
4 Conclusion and prospects
The incidence of glycolipid metabolic disease is gradually increasing, as well as the number of people affected by it, which forces people to find more ways to treat this kind of disease. As discussed earlier, ROS, as a key part of OS, plays an important role in the development of glycolipid metabolic diseases. Therefore, interfering with ROS to treat lipid metabolic diseases is a feasible and effective means in the future. For example, mitoTEMPOL and Q10 target mitochondria to reduce ROS in order to treat diabetes-related vascular damage (Graham et al., 2009; Dikalova et al., 2010) and nanoparticle drugs target ROS to improve AS (Wang et al., 2018; Zhang Z. et al., 2022) and the application of antioxidants in NAFLD (Ma et al., 2021). With this goal in view, this study summarizes the literature on botanical drugs to improve glycolipid metabolic diseases by regulating ROS from 2013 to 2022. In addition, the application parts, types of active ingredients, and extraction methods of botanical drugs involved were summarized. In addition, the mechanism of the intervention of botanical drugs on ROS to treat glycolipid metabolic diseases is briefly described, hoping this study can provide some help for the clinical use of botanical drugs.
However, there are still many deficiencies in the current research on ROS and glycolipid metabolic diseases. For example, many signaling pathways are related to OS, including but not limited to the signaling pathways discussed previously. In the future, if we continue to explore new pathways related to ROS in glycolipid metabolic diseases, or further study the cross-talk of existing pathways and develop drugs that can intervene in multiple pathways, It is believed that these may be helpful to improve OS in glycolipid metabolic diseases. These are going to be interesting new lines of research.
There are also some drawbacks to the study of botanical drugs. For example, there are more studies on the mechanism of botanical drugs or monomer components but fewer studies on adverse reactions and toxic side effects. There is insufficient clinical trial research and a lack of research on drug metabolism and kinetics. In order to promote the application of botanical drugs in glycolipid metabolic diseases and strengthen the research on the aforementioned problems, the following points are also worth developing: the extraction, processing, and storage of the effective ingredients of botanical drugs. The contents of the same active ingredient in different plants were compared. There is also the combination of a variety of plants, such as the use of herbal decoction and the combination of botanicals and chemical drugs (such as Erigeron breviscapus and enalapril to improve diabetic kidney injury; Xu et al., 2013). In addition, plant drugs do not only treat glucose and lipid metabolism diseases by regulating ROS but also include inflammation, apoptosis, cell proliferation, and other mechanisms. Therefore, the study of the combined effects of multiple mechanisms is also a good direction.
In summary, many botanical drugs have shown therapeutic effects on glycolipid metabolic diseases by regulating ROS. However, there are still many deficiencies in the current research. Although botanical drugs are still used empirically in the treatment of glucose and lipid metabolic diseases in many areas, it is obviously of great benefit to the clinical promotion of botanical drugs if a more in-depth study of the mechanism can be carried out. It is believed that better utilization of these widely available, low-cost, and complex botanical drugs will add new and powerful means for the treatment of glycolipid metabolic diseases.
Author contributions
ML, YHZ, and ST reviewed the literature, ML, YHZ, and LG wrote this manuscript. ML and YHZ draught diagrams, YZ and YL guided diagrams modification. RG, JM, and YL draw the tables, RY modified the tables. CZ and PG contributed to the manuscript revision. All authors read and approved the submitted version.
Funding
This study was funded by the fifth batch of training objects of the National TCM Clinical Excellent Talents Training Program (National TCM Renjiao Letter [2022] No. 1); “100 Talent Plan” Project of Hospital of Chengdu University of Traditional Chinese Medicine (Hospital office [2021] 42); and “Tianfu Qingcheng Plan” Tianfu Science and Technology Leading Talent Project (Cheng et al. (2021) No. 1090).
Conflict of interest
The authors declare that the research was conducted in the absence of any commercial or financial relationships that could be construed as a potential conflict of interest.
Publisher’s note
All claims expressed in this article are solely those of the authors and do not necessarily represent those of their affiliated organizations or those of the publisher, the editors, and the reviewers. Any product that may be evaluated in this article, or claim that may be made by its manufacturer, is not guaranteed or endorsed by the publisher.
References
Alam, M. B., Kwon, K. R., Lee, S. H., and Lee, S. H. (2017). Lannea coromandelica (houtt) Merr. Induces heme oxygenase 1 (HO-1) expression and reduces oxidative stress via the p38/c-Jun N-terminal kinase-nuclear factor erythroid 2-related factor 2 (p38/JNK-NRF2)-Mediated antioxidant pathway. Int. J. Mol. Sci 18 (2), 266. doi:10.3390/ijms18020266
Ando, K., and Fujita, T. (2009). Metabolic syndrome and oxidative stress. Free Radic. Biol. Med 47 (3), 213–218. doi:10.1016/j.freeradbiomed.2009.04.030
Apostolova, N., Iannantuoni, F., Gruevska, A., Muntane, J., Rocha, M., and Victor, V. M. (2020). Mechanisms of action of metformin in type 2 diabetes: Effects on mitochondria and leukocyte-endothelium interactions. Redox Biol. 34, 101517. doi:10.1016/j.redox.2020.101517
Arroyave-Ospina, J. C., Wu, Z., Geng, Y., and Moshage, H. (2021). Role of oxidative stress in the pathogenesis of non-alcoholic fatty liver disease: Implications for prevention and therapy. Antioxidants (Basel, Switz. 10 (2), 174. doi:10.3390/antiox10020174
Axelsson, A. S., Tubbs, E., Mecham, B., Chacko, S., Nenonen, H. A., Tang, Y., et al. (2017). Sulforaphane reduces hepatic glucose production and improves glucose control in patients with type 2 diabetes. Sci. Transl. Med. 9 (394), eaah4477. doi:10.1126/scitranslmed.aah4477
Bahar, E., Akter, K. M., Lee, G. H., Lee, H. Y., Rashid, H. O., Choi, M. K., et al. (2017). β-Cell protection and antidiabetic activities of Crassocephalum crepidioides (Asteraceae) Benth. S. Moore extract against alloxan-induced oxidative stress via regulation of apoptosis and reactive oxygen species (ROS). BMC COMPLEMENTARY Altern. Med. 17, 179. doi:10.1186/s12906-017-1697-0
Bai, Y., Mu, Q., Bao, X., Zuo, J., Fang, X., Hua, J., et al. (2021). Targeting NLRP3 inflammasome in the treatment of diabetes and diabetic complications: Role of natural compounds from herbal medicine. Aging Dis. 12 (7), 1587–1604. doi:10.14336/AD.2021.0318
Banerjee, A., Singh, S., Prasad, S. K., Kumar, S., Banerjee, O., Seal, T., et al. (2020). Protective efficacy of Tinospora sinensis against streptozotocin induced pancreatic islet cell injuries of diabetic rats and its correlation to its phytochemical profiles. J. Ethnopharmacol. 248, 112356. doi:10.1016/j.jep.2019.112356
Barquera, S., Pedroza-Tobías, A., Medina, C., Hernández-Barrera, L., Bibbins-Domingo, K., Lozano, R., et al. (2015). Global overview of the epidemiology of atherosclerotic cardiovascular disease. Archives Med. Res. 46 (5), 328–338. doi:10.1016/j.arcmed.2015.06.006
Batty, M., Bennett, M. R., and Yu, E. (2022). The role of oxidative stress in atherosclerosis. Cells 11 (23), 3843. doi:10.3390/cells11233843
Bedard, K., and Krause, K.-H. (2007). The NOX family of ROS-generating NADPH oxidases: Physiology and pathophysiology. Physiol. Rev. 87 (1), 245–313. doi:10.1152/physrev.00044.2005
Begriche, K., Massart, J., Robin, M.-A., Bonnet, F., and Fromenty, B. (2013). Mitochondrial adaptations and dysfunctions in nonalcoholic fatty liver disease. Hepatol. Baltim. Md) 58 (4), 1497–1507. doi:10.1002/hep.26226
Bhabak, K. P., and Mugesh, G. (2010). Functional mimics of glutathione peroxidase: Bioinspired synthetic antioxidants. Accounts Chem. Res. 43 (11), 1408–1419. doi:10.1021/ar100059g
Bhandary, B., Marahatta, A., Kim, H.-R., and Chae, H.-J. (2012). An involvement of oxidative stress in endoplasmic reticulum stress and its associated diseases. Int. J. Mol. Sci. 14 (1), 434–456. doi:10.3390/ijms14010434
Bhattacharya, S., Khan, M. M., Ghosh, C., Bank, S., and Maiti, S. (2017). The role of Dermcidin isoform-2 in the occurrence and severity of Diabetes. Sci. Rep. 7 (1), 8252. doi:10.1038/s41598-017-07958-3
Blesia, V., Patel, V. B., Al-Obaidi, H., Renshaw, D., and Zariwala, M. G. (2021). Excessive iron induces oxidative stress promoting cellular perturbations and insulin secretory dysfunction in MIN6 beta cells. Cells 10 (5), 1141. doi:10.3390/cells10051141
Blüher, M. (2019). Obesity: Global epidemiology and pathogenesis. Nat. Rev. Endocrinol. 15 (5), 288–298. doi:10.1038/s41574-019-0176-8
Bourebaba, N., Kornicka-Garbowska, K., Marycz, K., Bourebaba, L., and Kowalczuk, A. (2021). Laurus nobilis ethanolic extract attenuates hyperglycemia and hyperinsulinemia-induced insulin resistance in HepG2 cell line through the reduction of oxidative stress and improvement of mitochondrial biogenesis - possible implication in pharmacotherapy. MITOCHONDRION 59, 190–213. doi:10.1016/j.mito.2021.06.003
Brown, D. I., and Griendling, K. K. (2015). Regulation of signal transduction by reactive oxygen species in the cardiovascular system. Circulation Res. 116 (3), 531–549. doi:10.1161/CIRCRESAHA.116.303584
Buettner, G. R. (2011). Superoxide dismutase in redox biology: The roles of superoxide and hydrogen peroxide. Anti-cancer Agents Med. Chem. 11 (4), 341–346. doi:10.2174/187152011795677544
Caliceti, C., Rizzo, P., Ferrari, R., Fortini, F., Aquila, G., Leoncini, E., et al. (2017). Novel role of the nutraceutical bioactive compound berberine in lectin-like OxLDL receptor 1-mediated endothelial dysfunction in comparison to lovastatin. Nutr. METABOLISM Cardiovasc. Dis. 27 (6), 552–563. doi:10.1016/j.numecd.2017.04.002
Cao, Z. H., Wu, Z. A., Duan, T. T., Tang, C. F., Huang, D., and Hu, X. B. (2022). Curcumin ameliorates HO-induced injury through SIRT1-PERK-CHOP pathway in pancreatic beta cells. ACTA BIOCHIMICA BIOPHYSICA SINICA 54 (3), 370–377. doi:10.3724/abbs.2022004
Carnevale, R., Pignatelli, P., Di Santo, S., Bartimoccia, S., Sanguigni, V., Napoleone, L., et al. (2010). Atorvastatin inhibits oxidative stress via adiponectin-mediated NADPH oxidase down-regulation in hypercholesterolemic patients. Atherosclerosis 213 (1), 225–234. doi:10.1016/j.atherosclerosis.2010.08.056
Ceriello, A., Novials, A., Ortega, E., Canivell, S., La Sala, L., Pujadas, G., et al. (2013). Glucagon-like peptide 1 reduces endothelial dysfunction, inflammation, and oxidative stress induced by both hyperglycemia and hypoglycemia in type 1 diabetes. Diabetes Care 36 (8), 2346–2350. doi:10.2337/dc12-2469
Cervantes Gracia, K., Llanas-Cornejo, D., and Husi, H. (2017). CVD and oxidative stress. J. Clin. Med. 6 (2), 22. doi:10.3390/jcm6020022
Chang, T. T., Chen, Y. A., Li, S. Y., and Chen, J. W. (2021). Nrf-2 mediated heme oxygenase-1 activation contributes to the anti-inflammatory and renal protective effects of Ginkgo biloba extract in diabetic nephropathy. J. Ethnopharmacol. 266, 113474. doi:10.1016/j.jep.2020.113474
Chatterjee, S., Browning, E. A., Hong, N., DeBolt, K., Sorokina, E. M., Liu, W., et al. (2012). Membrane depolarization is the trigger for PI3K/Akt activation and leads to the generation of ROS. Am. J. Physiology. Heart Circulatory Physiology 302 (1), H105–H114. doi:10.1152/ajpheart.00298.2011
Chen, F., Xiong, H., Wang, J., Ding, X., Shu, G., and Mei, Z. (2013). Antidiabetic effect of total flavonoids from Sanguis draxonis in type 2 diabetic rats. J. Ethnopharmacol. 149 (3), 729–736. doi:10.1016/j.jep.2013.07.035
Chen, G., Chen, X. J., Niu, C., Huang, X. Z., An, N., Sun, J., et al. (2019). Baicalin alleviates hyperglycemia-induced endothelial impairment via Nrf2. J. Endocrinol. 240 (1), 81–98. doi:10.1530/JOE-18-0457
Chen, Z., Tian, R., She, Z., Cai, J., and Li, H. (2020). Role of oxidative stress in the pathogenesis of nonalcoholic fatty liver disease. Free Radic. Biol. Med. 152, 116–141. doi:10.1016/j.freeradbiomed.2020.02.025
Cheng, Y.-C., Chiu, Y.-M., Dai, Z.-K., and Wu, B.-N. (2021). Loganin ameliorates painful diabetic neuropathy by modulating oxidative stress, inflammation and insulin sensitivity in streptozotocin-nicotinamide-induced diabetic rats. Cells 10 (10), 2688. doi:10.3390/cells10102688
Choi, E. S., Yoon, J. J., Han, B. H., Jeong, D. H., Lee, Y. J., Kang, D. G., et al. (2018). Ligustilide attenuates vascular inflammation and activates Nrf2/HO-1 induction and, NO synthesis in HUVECs. PHYTOMEDICINE 38, 12–23. doi:10.1016/j.phymed.2017.09.022
Choi, Y. E., Choi, S. I., Han, X. G., Men, X., Jang, G. W., Kwon, H. Y., et al. (2020). Enhancement of immune activities of mixtures with sasa quelpaertensis Nakai and Ficus erecta var. sieboldii. ANTIOXIDANTS 9 (12), 868. doi:10.3390/foods9070868
Dan Dunn, J., Alvarez, L. A., Zhang, X., and Soldati, T. (2015). Reactive oxygen species and mitochondria: A nexus of cellular homeostasis. Redox Biol. 6, 472–485. doi:10.1016/j.redox.2015.09.005
De Oliveira, K. A., Araujo, H. N., De Lima, T. I., Oliveira, A. G., Favero-Santos, B. C., Guimaraes, D., et al. (2021). Phytomodulatory proteins isolated from Calotropis procera latex promote glycemic control by improving hepatic mitochondrial function in HepG2 cells. SAUDI Pharm. J. 29 (9), 1061–1069. doi:10.1016/j.jsps.2021.07.008
Deng, Y., Ma, J., Weng, X., Wang, Y. Q., Li, M. R., Yang, T. T., et al. (2021). Kaempferol-3-O-Glucuronide ameliorates non-alcoholic steatohepatitis in high-cholesterol-diet-induced larval zebrafish and HepG2 cell models via regulating oxidation stress. LIFE-BASEL 11 (5), 445. doi:10.3390/life11050445
Dhounchak, S., Popp, S. K., Brown, D. J., Laybutt, D. R., Biden, T. J., Bornstein, S. R., et al. (2021). Heparan sulfate proteoglycans in beta cells provide a critical link between endoplasmic reticulum stress, oxidative stress and type 2 diabetes. PloS One 16 (6), e0252607. doi:10.1371/journal.pone.0252607
Dikalova, A. E., Bikineyeva, A. T., Budzyn, K., Nazarewicz, R. R., McCann, L., Lewis, W., et al. (2010). Therapeutic targeting of mitochondrial superoxide in hypertension. Circulation Res. 107 (1), 106–116. doi:10.1161/CIRCRESAHA.109.214601
Dinkova-Kostova, A. T., and Talalay, P. (2008). Direct and indirect antioxidant properties of inducers of cytoprotective proteins. Mol. Nutr. Food Res. 52, S128–S138. doi:10.1002/mnfr.200700195
Dröse, S., and Brandt, U. (2012). Molecular mechanisms of superoxide production by the mitochondrial respiratory chain. Adv. Exp. Med. Biol. 748, 145–169. doi:10.1007/978-1-4614-3573-0_6
Elsayed, R. H., Kamel, E. M., Mahmoud, A. M., El-Bassuony, A. A., Bin-Jumah, M., Lamsabhi, A., et al. (2020). Rumex dentatus L. phenolics ameliorate hyperglycemia by modulating hepatic key enzymes of carbohydrate metabolism, oxidative stress and PPAR gamma in diabetic rats. FOOD Chem. Toxicol. 138, 111202. doi:10.1016/j.fct.2020.111202
Fan, J., Liu, D., He, C., Li, X., and He, F. (2016). Inhibiting adhesion events by Panax notoginseng saponins and Ginsenoside Rb1 protecting arteries via activation of Nrf2 and suppression of p38 - VCAM-1 signal pathway. J. Ethnopharmacol. 192, 423–430. doi:10.1016/j.jep.2016.09.022
Fan, X. C., Han, J. C., Zhu, L. J., Chen, Z. P., Li, J. J., Gu, Y., et al. (2020). Protective Activities of Dendrobium huoshanense C. Z. Tang et S. J. Cheng Polysaccharide against High-Cholesterol Diet-Induced Atherosclerosis in Zebrafish. OXIDATIVE Med. Cell. Longev. 2020, 8365056. doi:10.1155/2020/8365056
Fang, Q., Li, X., Wang, M., Qiao, X., Huang, F., Hu, C., et al. (2022). Walnut green husk ethanol extract improves gut microbiota and their metabolites associated with NLRP3 in non-alcoholic steatohepatitis. Food and Funct. 13 (11), 6387–6403. doi:10.1039/d2fo00012a
Farmer, A., and Fox, R. (2011). Diagnosis, classification, and treatment of diabetes. BMJ Clin. Res. ed.) 342, d3319. doi:10.1136/bmj.d3319
Foo, J., Bellot, G., Pervaiz, S., and Alonso, S. (2022). Mitochondria-mediated oxidative stress during viral infection. Trends Microbiol. 30 (7), 679–692. doi:10.1016/j.tim.2021.12.011
Forbes-Hernandez, T. Y., Gasparrini, M., Afrin, S., Cianciosi, D., Gonzalez-Paramas, A. M., Santos-Buelga, C., et al. (2017). Strawberry (cv. Romina) methanolic extract and anthocyanin-enriched fraction improve lipid profile and antioxidant status in HepG2 cells. Int. J. Mol. Sci. 18 (6), 1149. doi:10.3390/ijms18061149
Forrester, S. J., Kikuchi, D. S., Hernandes, M. S., Xu, Q., and Griendling, K. K. (2018). Reactive oxygen species in metabolic and inflammatory signaling. Circulation Res. 122 (6), 877–902. doi:10.1161/CIRCRESAHA.117.311401
Förstermann, U., Xia, N., and Li, H. (2017). Roles of vascular oxidative stress and nitric oxide in the pathogenesis of atherosclerosis. Circulation Res. 120 (4), 713–735. doi:10.1161/CIRCRESAHA.116.309326
Friedman, S. L., Neuschwander-Tetri, B. A., Rinella, M., and Sanyal, A. J. (2018). Mechanisms of NAFLD development and therapeutic strategies. Nat. Med. 24 (7), 908–922. doi:10.1038/s41591-018-0104-9
Fu, Y. F., Sun, S. Y., Sun, H. J., Peng, J. Y., Ma, X. D., Bao, L. C., et al. (2019). Scutellarin exerts protective effects against atherosclerosis in rats by regulating the Hippo-FOXO3A and PI3K/AKT signaling pathways. J. Cell. PHYSIOLOGY 234 (10), 18131–18145. doi:10.1002/jcp.28446
Fuchs, C. D., Claudel, T., Scharnagl, H., Stojakovic, T., and Trauner, M. (2017). FXR controls CHOP expression in steatohepatitis. FEBS Lett. 591 (20), 3360–3368. doi:10.1002/1873-3468.12845
Gao, Y., Wang, X., and He, C. (2016). An isoflavonoid-enriched extract from Pueraria lobata (kudzu) root protects human umbilical vein endothelial cells against oxidative stress induced apoptosis. J. Ethnopharmacol. 193, 524–530. doi:10.1016/j.jep.2016.10.005
Gardner, B. M., and Walter, P. (2011). Unfolded proteins are Ire1-activating ligands that directly induce the unfolded protein response. Sci. (New York, N.Y.) 333 (6051), 1891–1894. doi:10.1126/science.1209126
Gasparotto, F. M., Palozi, R. A. C., da Silva, C. H. F., Pauli, K. B., Donadel, G., Lourenco, B., et al. (2019). Antiatherosclerotic properties of Echinodorus grandiflorus (cham. and schltdl) micheli: From antioxidant and lipid-lowering effects to an anti-inflammatory role. J. Med. FOOD 22 (9), 919–927. doi:10.1089/jmf.2019.0017
Germoush, M. O., Elgebaly, H. A., Hassan, S., Kamel, E. M., Bin-Jumah, M., and Mahmoud, A. M. (2020). Consumption of terpenoids-rich Padina pavonia extract attenuates hyperglycemia, insulin resistance and oxidative stress, and Upregulates PPAR gamma in a rat model of type 2 diabetes. ANTIOXIDANTS 9 (1), 22. doi:10.3390/antiox9010022
Gier, B., Krippeit-Drews, P., Sheiko, T., Aguilar-Bryan, L., Bryan, J., Düfer, M., et al. (2009). Suppression of KATP channel activity protects murine pancreatic beta cells against oxidative stress. J. Clin. Investigation 119 (11), 3246–3256. doi:10.1172/JCI38817
Gimenes, R., Gimenes, C., Rosa, C. M., Xavier, N. P., Campos, D. H. S., Fernandes, A. A. H., et al. (2018). Influence of apocynin on cardiac remodeling in rats with streptozotocin-induced diabetes mellitus. Cardiovasc. Diabetol. 17, 15. doi:10.1186/s12933-017-0657-9
Goboza, M., Aboua, Y. G., Chegou, N., and Oguntibeju, O. O. (2019). Vindoline effectively ameliorated diabetes-induced hepatotoxicity by docking oxidative stress, inflammation and hypertriglyceridemia in type 2 diabetes-induced male Wistar rats. Biomed. Pharmacother. 112, 108638. doi:10.1016/j.biopha.2019.108638
Graham, D., Huynh, N. N., Hamilton, C. A., Beattie, E., Smith, R. A. J., Cochemé, H. M., et al. (2009). Mitochondria-targeted antioxidant MitoQ10 improves endothelial function and attenuates cardiac hypertrophy. Hypertens. (Dallas, Tex, 1979) 54 (2), 322–328. doi:10.1161/HYPERTENSIONAHA.109.130351
Gu, L., Bai, W., Li, S., Zhang, Y., Han, Y., Gu, Y., et al. (2013). Celastrol prevents atherosclerosis via inhibiting LOX-1 and oxidative stress. PLoS One 8 (6), e65477. doi:10.1371/journal.pone.0065477
Hachkova, H., Nagalievska, M., Soliljak, Z., Kanyuka, O., Kucharska, A. Z., Sokol-Letowska, A., et al. (2021). Medicinal plants Galega officinalis L. And Yacon leaves as potential sources of antidiabetic drugs. ANTIOXIDANTS 10 (9), 1362. doi:10.3390/antiox10091362
Hamanaka, R. B., and Chandel, N. S. (2010). Mitochondrial reactive oxygen species regulate cellular signaling and dictate biological outcomes. Trends Biochem. Sci. 35 (9), 505–513. doi:10.1016/j.tibs.2010.04.002
Han, J., Dong, J., Zhang, R., Zhang, X., Chen, M., Fan, X., et al. (2021). Dendrobium catenatum lindl. Water extracts attenuate atherosclerosis. Mediat. Inflamm. 2021, 9951946. doi:10.1155/2021/9951946
Herranz-Lopez, M., Olivares-Vicente, M., Gallego, E. R., Encinar, J. A., Perez-Sanchez, A., Ruiz-Torres, V., et al. (2020). Quercetin metabolites from Hibiscus sabdariffa contribute to alleviate glucolipotoxicity-induced metabolic stress in vitro. FOOD Chem. Toxicol. 144, 111606. doi:10.1016/j.fct.2020.111606
Hoang, T. H., Yoon, Y., Park, S. A., Lee, H. Y., Peng, C., Kim, J. H., et al. (2021). IBF-R, a botanical extract of Rhus verniciflua controls obesity in which AMPK-SIRT1 axis and ROS regulatory mechanism are involved in mice. J. Funct. FOODS 87, 104804. doi:10.1016/j.jff.2021.104804
Hoseini, Z., Sepahvand, F., Rashidi, B., Sahebkar, A., Masoudifar, A., and Mirzaei, H. (2018). NLRP3 inflammasome: Its regulation and involvement in atherosclerosis. J. Cell. Physiology 233 (3), 2116–2132. doi:10.1002/jcp.25930
Hsieh, S. L., Wang, J. J., Su, K. H., Kuo, Y. L., Hsieh, S. C., and Wu, C. C. (2020). Suppressive effects of the Gynura bicolor ether extract on endothelial permeability and leukocyte transmigration in human endothelial cells induced by TNF-α. EVIDENCE-BASED COMPLEMENTARY Altern. Med. 2020, 9413724. doi:10.1155/2020/9413724
Hsu, J. Y., Lin, H. H., Chyau, C. C., Wang, Z. H., and Chen, J. H. (2021). Aqueous extract of pepino leaves ameliorates palmitic acid-induced hepatocellular lipotoxicity via inhibition of endoplasmic reticulum stress and apoptosis. ANTIOXIDANTS 10 (6), 903. doi:10.3390/antiox10060903
Huang, C. S., Lin, A. H., Yang, T. C., Liu, K. L., Chen, H. W., and Lii, C. K. (2015). Shikonin inhibits oxidized LDL-induced monocyte adhesion by suppressing NFκB activation via up-regulation of PI3K/Akt/Nrf2-dependent antioxidation in EA.hy926 endothelial cells. Biochem. Pharmacol. 93 (3), 352–361. doi:10.1016/j.bcp.2014.12.005
Huang, D. Q., El-Serag, H. B., and Loomba, R. (2021). Global epidemiology of NAFLD-related HCC: Trends, predictions, risk factors and prevention. Nat. Rev. Gastroenterology Hepatology 18 (4), 223–238. doi:10.1038/s41575-020-00381-6
Huang, S., Tan, M., Guo, F., Dong, L. S., Liu, Z. M., Yuan, R. Y., et al. (2020). Nepeta angustifolia C. Y. Wu improves renal injury in HFD/STZ-induced diabetic nephropathy and inhibits oxidative stress-induced apoptosis of mesangial cells. J. Ethnopharmacol. 255, 112771. doi:10.1016/j.jep.2020.112771
Huang, X., Liu, G., Guo, J., and Su, Z. (2018). The PI3K/AKT pathway in obesity and type 2 diabetes. Int. J. Biol. Sci. 14 (11), 1483–1496. doi:10.7150/ijbs.27173
Ihm, S. H., Park, S. H., Lee, J. O., Kim, O. R., Park, E. H., Kim, K. R., et al. (2021). A standardized Lindera obtusiloba extract improves endothelial dysfunction and attenuates plaque development in hyperlipidemic ApoE-knockout mice. PLANTS-BASEL 10 (11), 2493. doi:10.3390/plants10112493
Ishibashi, Y., Matsui, T., Isami, F., Abe, Y., Sakaguchi, T., Higashimoto, Y., et al. (2017). N-butanol extracts of Morinda citrifolia suppress advanced glycation end products (AGE)-induced inflammatory reactions in endothelial cells through its anti-oxidative properties. BMC COMPLEMENTARY Altern. Med. 17, 137. doi:10.1186/s12906-017-1641-3
Jamwal, S., and Sharma, S. (2018). Vascular endothelium dysfunction: A conservative target in metabolic disorders. Inflamm. Res. Official J. Eur. Histamine Res. Soc. 67 (5), 391–405. doi:10.1007/s00011-018-1129-8
Jiang, X., Gu, Y., Huang, Y., Zhou, Y., Pang, N., Luo, J., et al. (2021). CBD alleviates liver injuries in alcoholics with high-fat high-cholesterol diet through regulating NLRP3 inflammasome-pyroptosis pathway. Front. Pharmacol. 12, 724747. doi:10.3389/fphar.2021.724747
Jiang, Y. H., Yang, C. H., Li, W., Wu, S., Meng, X. Q., and Li, D. N. (2016). Aqueous extracts of Tribulus terrestris protects against oxidized low-density lipoprotein-induced endothelial dysfunction. Chin. J. Integr. Med. 22 (3), 193–200. doi:10.1007/s11655-015-2321-0
Jin, X., Fu, W., Zhou, J., Shuai, N., Yang, Y., and Wang, B. (2021a). Oxymatrine attenuates oxidized low-density lipoprotein-induced HUVEC injury by inhibiting NLRP3 inflammasome-mediated pyroptosis via the activation of the SIRT1/Nrf2 signaling pathway. Int. J. Mol. Med. 48 (4), 187. doi:10.3892/ijmm.2021.5020
Jin, X., Fu, W., Zhou, J. X., Shuai, N. N., Yang, Y., and Wang, B. (2021b). Oxymatrine attenuates oxidized low-density lipoprotein-induced HUVEC injury by inhibiting NLRP3 inflammasome-mediated pyroptosis via the activation of the SIRT1/Nrf2 signaling pathway. Int. J. Mol. Med. 48 (4), 187. doi:10.3892/ijmm.2021.5020
Juan, C. A., Pérez de la Lastra, J. M., Plou, F. J., and Pérez-Lebeña, E. (2021). The chemistry of reactive oxygen species (ROS) revisited: Outlining their role in biological macromolecules (DNA, lipids and proteins) and induced pathologies. Int. J. Mol. Sci. 22 (9), 4642. doi:10.3390/ijms22094642
Kaim, G., and Dimroth, P. (1999). ATP synthesis by F-type ATP synthase is obligatorily dependent on the transmembrane voltage. EMBO J. 18 (15), 4118–4127. doi:10.1093/emboj/18.15.4118
Kajimoto, Y., and Kaneto, H. (2004). Role of oxidative stress in pancreatic beta-cell dysfunction. Ann. N. Y. Acad. Sci. 1011, 168–176. doi:10.1007/978-3-662-41088-2_17
Kamakura, R., Son, M. J., de Beer, D., Joubert, E., Miura, Y., and Yagasaki, K. (2015). Antidiabetic effect of green rooibos (Aspalathus linearis) extract in cultured cells and type 2 diabetic model KK-A(y) mice. Cytotechnology 67 (4), 699–710. doi:10.1007/s10616-014-9816-y
Kaminskyy, V. O., and Zhivotovsky, B. (2014). Free radicals in cross talk between autophagy and apoptosis. Antioxidants Redox Signal. 21 (1), 86–102. doi:10.1089/ars.2013.5746
Kanikowska, D., Kanikowska, A., Swora-Cwynar, E., Grzymisławski, M., Sato, M., Bręborowicz, A., et al. (2021). Moderate caloric restriction partially improved oxidative stress markers in obese humans. Antioxidants (Basel, Switz. 10 (7), 1018. doi:10.3390/antiox10071018
Kashyap, B., Barge, S. R., Bharadwaj, S., Deka, B., Rahman, S., Ghosh, A., et al. (2021). Evaluation of therapeutic effect of Premna herbacea in diabetic rat and isoverbascoside against insulin resistance in L6 muscle cells through bioenergetics and stimulation of JNK and AKT/mTOR signaling cascade. PHYTOMEDICINE 93, 153761. doi:10.1016/j.phymed.2021.153761
Kaspar, J. W., Niture, S. K., and Jaiswal, A. K. (2009). Nrf2:INrf2 (Keap1) signaling in oxidative stress. Free Radic. Biol. Med. 47 (9), 1304–1309. doi:10.1016/j.freeradbiomed.2009.07.035
Kattoor, A. J., Pothineni, N. V. K., Palagiri, D., and Mehta, J. L. (2017). Oxidative stress in atherosclerosis. Curr. Atheroscler. Rep. 19 (11), 42. doi:10.1007/s11883-017-0678-6
Khazim, K., Gorin, Y., Cavaglieri, R. C., Abboud, H. E., and Fanti, P. (2013). The antioxidant silybin prevents high glucose-induced oxidative stress and podocyte injury in vitro and in vivo. Am. J. Physiol. Ren. Physiol. 305 (5), F691–F700. doi:10.1152/ajprenal.00028.2013
Kim, S., Kim, C. K., Lee, K. S., Kim, J. H., Hwang, H., Jeoung, D., et al. (2013). Aqueous extract of unripe Rubus coreanus fruit attenuates atherosclerosis by improving blood lipid profile and inhibiting NF-κB activation via phase II gene expression. J. Ethnopharmacol. 146 (2), 515–524. doi:10.1016/j.jep.2013.01.016
Kirkman, H. N., and Gaetani, G. F. (2007). Mammalian catalase: A venerable enzyme with new mysteries. Trends Biochem. Sci. 32 (1), 44–50. doi:10.1016/j.tibs.2006.11.003
Kong, Z. L., Johnson, A., Ko, F. C., He, J. L., and Cheng, S. C. (2018). Effect of Cistanche tubulosa extracts on male reproductive function in streptozotocin-nicotinamide-induced diabetic rats. NUTRIENTS 10 (10), 1562. doi:10.3390/nu10101562
Ku, C. W., Ho, T. J., Huang, C. Y., Chu, P. M., Ou, H. C., and Hsieh, P. L. (2021). Cordycepin attenuates palmitic acid-induced inflammation and apoptosis of vascular endothelial cells through mediating PI3K/Akt/eNOS signaling pathway. Am. J. Chin. Med. 49 (07), 1703–1722. doi:10.1142/S0192415X21500804
Ku, S. K., and Bae, J. S. (2015). Baicalin, baicalein and wogonin inhibits high glucose-induced vascular inflammation in vitro and in vivo. BMB Rep. 48 (9), 519–524. doi:10.5483/bmbrep.2015.48.9.017
Lartey, N. L., Asare-Anane, H., Ofori, E. K., Antwi, S., Asiedu-Larbi, J., Ayertey, F., et al. (2021). Antidiabetic activity of aqueous stem bark extract of Annickia polycarpa in alloxan-induced diabetic mice. J. TRADITIONAL COMPLEMENTARY Med. 11 (2), 109–116. doi:10.1016/j.jtcme.2020.02.001
Lee, G. H., Peng, C., Jeong, S. Y., Park, S. A., Lee, H. Y., Hoang, T. H., et al. (2021). Ginger extract controls mTOR-SREBP1-ER stress-mitochondria dysfunction through AMPK activation in obesity model. J. Funct. FOODS 87, 104628. doi:10.1016/j.jff.2021.104628
Lee, N. K., Choi, Y. G., Baik, J. Y., Han, S. Y., Jeong, D.-W., Bae, Y. S., et al. (2005). A crucial role for reactive oxygen species in RANKL-induced osteoclast differentiation. Blood 106 (3), 852–859. doi:10.1182/blood-2004-09-3662
Lee, S. E., Jeong, S. I., Yang, H., Jeong, S. H., Jang, Y. P., Park, C. S., et al. (2012). Extract of Salvia miltiorrhiza (Danshen) induces Nrf2-mediated heme oxygenase-1 expression as a cytoprotective action in RAW 264.7 macrophages. J. Ethnopharmacol. 139 (2), 541–548. doi:10.1016/j.jep.2011.11.046
Leenders, F., Groen, N., de Graaf, N., Engelse, M. A., Rabelink, T. J., de Koning, E. J. P., et al. (2021). Oxidative stress leads to β-cell dysfunction through loss of β-cell identity. Front. Immunol. 12, 690379. doi:10.3389/fimmu.2021.690379
Lei, X. G., Zhu, J.-H., Cheng, W.-H., Bao, Y., Ho, Y.-S., Reddi, A. R., et al. (2016). Paradoxical roles of antioxidant enzymes: Basic mechanisms and health implications. Physiol. Rev. 96 (1), 307–364. doi:10.1152/physrev.00010.2014
Lei, Y., Wang, K., Deng, L., Chen, Y., Nice, E. C., and Huang, C. (2015). Redox regulation of inflammation: Old elements, a new story. Med. Res. Rev. 35 (2), 306–340. doi:10.1002/med.21330
Li, B., Wang, R., Wang, L., Zhang, G., and Zhang, Y. (2021a). Capillin protects against non-alcoholic steatohepatitis through suppressing NLRP3 inflammasome activation and oxidative stress. Immunopharmacol. Immunotoxicol. 43 (6), 778–789. doi:10.1080/08923973.2021.1984520
Li, C. M., Guo, Y. Q., Dong, X. L., Li, H., Wang, B., Wu, J. H., et al. (2014). Ethanolic extract of rhizome of Ligusticum chuanxiong Hort. (chuanxiong) enhances endothelium-dependent vascular reactivity in ovariectomized rats fed with high-fat diet. Food Funct. 5 (10), 2475–2485. doi:10.1039/c4fo00211c
Li, D., and Mehta, J. L. (2000). Upregulation of endothelial receptor for oxidized LDL (LOX-1) by oxidized LDL and implications in apoptosis of human coronary artery endothelial cells: Evidence from use of antisense LOX-1 mRNA and chemical inhibitors. Arteriosclerosis, Thrombosis, Vasc. Biol. 20 (4), 1116–1122. doi:10.1161/01.atv.20.4.1116
Li, G., Luan, G. X., He, Y. F., Tie, F. F., Wang, Z. H., Suo, Y. R., et al. (2018). Polyphenol stilbenes from fenugreek (Trigonella foenum-graecum L) seeds improve insulin sensitivity and mitochondrial function in 3T3-L1 adipocytes. OXIDATIVE Med. Cell. Longev. 2018, 7634362. doi:10.1155/2018/7634362
Li, Y. Q., Li, Y. L., Chen, N. N., Feng, L. Y., Gao, J. M., Zeng, N., et al. (2022). Icariside II exerts anti-type 2 diabetic effect by targeting pparα/γ: Involvement of ROS/NF-κB/IRS1 signaling pathway. ANTIOXIDANTS 11 (9), 1705. doi:10.3390/antiox11091705
Li, Y., Zhou, Y., Wu, J., Li, J. D., and Yao, H. K. (2021b). Phelligridin D from Inonotus obliquus attenuates oxidative stress and accumulation of ECM in mesangial cells under high glucose via activating Nrf2. J. Nat. Med. 75 (4), 1021–1029. doi:10.1007/s11418-021-01534-w
Liang, H. H., Pan, Y. N., Teng, Y. L., Yuan, S. L., Wu, X., Yang, H. J., et al. (2020). A proteoglycan extract from Ganoderma Lucidum protects pancreatic beta-cells against STZ-induced apoptosis. Biosci. Biotechnol. Biochem. 84 (12), 2491–2498. doi:10.1080/09168451.2020.1805718
Libby, P. (2017). Interleukin-1 beta as a target for atherosclerosis therapy: Biological basis of CANTOS and beyond. J. Am. Coll. Cardiol. 70 (18), 2278–2289. doi:10.1016/j.jacc.2017.09.028
Liu, F. F., Ma, H., Wang, G. H., Liu, W. X., Seeram, N. P., Mu, Y., et al. (2018). Phenolics from Eugenia jambolana seeds with advanced glycation endproduct formation and alpha-glucosidase inhibitory activities. FOOD and Funct. 9 (8), 4246–4254. doi:10.1039/c8fo00583d
Liu, Q., Qiao, A. M., Yi, L. T., Liu, Z. L., and Sheng, S. M. (2016). Protection of kinsenoside against AGEs-induced endothelial dysfunction in human umbilical vein endothelial cells. Life Sci. 162, 102–107. doi:10.1016/j.lfs.2016.08.022
Liu, S.-S. (2010). Mitochondrial Q cycle-derived superoxide and chemiosmotic bioenergetics. Ann. N. Y. Acad. Sci. 1201, 84–95. doi:10.1111/j.1749-6632.2010.05632.x
Liu, Y., Kern, J. T., Walker, J. R., Johnson, J. A., Schultz, P. G., and Luesch, H. (2007). A genomic screen for activators of the antioxidant response element. Proc. Natl. Acad. Sci. U. S. A. 104 (12), 5205–5210. doi:10.1073/pnas.0700898104
Liu, Z. L., Zhang, J. G., Liu, Q., Yi, L. T., Li, Y. M., and Li, Y. (2017). The vascular protective effects of Anoectochilus roxburghii polysaccharose under high glucose conditions. J. Ethnopharmacol. 202, 192–199. doi:10.1016/jjep.2017.03.012
Liu, Z., Xu, S., Huang, X., Wang, J., Gao, S., Li, H., et al. (2015). Cryptotanshinone, an orally bioactive herbal compound from danshen, attenuates atherosclerosis in apolipoprotein E-deficient mice: Role of lectin-like oxidized LDL receptor-1 (LOX-1). Br. J. Pharmacol. 172 (23), 5661–5675. doi:10.1111/bph.13068
Luan, G. X., Wang, Y. W., Wang, Z. H., Zhou, W. N., Hu, N., Li, G., et al. (2018). Flavonoid glycosides from fenugreek seeds regulate glycolipid metabolism by improving mitochondrial function in 3T3-L1 adipocytes in vitro. J. Agric. FOOD Chem. 66 (12), 3169–3178. doi:10.1021/acs.jafc.8b00179
Ma, Y., Lee, G., Heo, S.-Y., and Roh, Y.-S. (2021). Oxidative stress is a key modulator in the development of nonalcoholic fatty liver disease. Antioxidants (Basel, Switz. 11 (1), 91. doi:10.3390/antiox11010091
Madamanchi, N. R., and Runge, M. S. (2007). Mitochondrial dysfunction in atherosclerosis. Circulation Res. 100 (4), 460–473. doi:10.1161/01.RES.0000258450.44413.96
Mahmoud, M. F., Abdelaal, S., Mohammed, H. O., El-Shazly, A. M., Daoud, R., Abdelfattah, M. A. O., et al. (2021a). Syzygium aqueum (Burm.f) Alston prevents streptozotocin-induced pancreatic beta cells damage via the TLR-4 signaling pathway. Front. Pharmacol. 12, 769244. doi:10.3389/fphar.2021.769244
Mahmoud, M. F., Abdelaal, S., Mohammed, H. O., El-Shazly, A. M., Daoud, R., El Raey, M. A., et al. (2021b). Syzygium jambos extract mitigates pancreatic oxidative stress, inflammation and apoptosis and modulates hepatic IRS-2/AKT/GLUT4 signaling pathway in streptozotocin-induced diabetic rats. Biomed. Pharmacother. = Biomedecine Pharmacother. 142, 112085. doi:10.1016/j.biopha.2021.112085
Mai, W. J., Xu, Y. Z., Xu, J. H., Zhao, D., Ye, L. Y., Yu, G. X., et al. (2020). Berberine inhibits nod-like receptor family pyrin domain containing 3 inflammasome activation and pyroptosis in nonalcoholic steatohepatitis via the ROS/TXNIP Axis. Front. Pharmacol. 11, 185. doi:10.3389/fphar.2020.00185
Mansouri, A., Gattolliat, C.-H., and Asselah, T. (2018). Mitochondrial dysfunction and signaling in chronic liver diseases. Gastroenterology 155 (3), 629–647. doi:10.1053/j.gastro.2018.06.083
Martínez-Martínez, E., and Cachofeiro, V. (2022). Oxidative stress in obesity. Antioxidants (Basel, Switz. 11 (4), 639. doi:10.3390/antiox11040639
Mihailović, M., Dinić, S., Arambašić Jovanović, J., Uskoković, A., Grdović, N., and Vidaković, M. (2021). The influence of plant extracts and phytoconstituents on antioxidant enzymes activity and gene expression in the prevention and treatment of impaired glucose homeostasis and diabetes complications. Antioxidants (Basel, Switz. 10 (3), 480. doi:10.3390/antiox10030480
Muller, F. L., Liu, Y., and Van Remmen, H. (2004). Complex III releases superoxide to both sides of the inner mitochondrial membrane. J. Biol. Chem. 279 (47), 49064–49073. doi:10.1074/jbc.M407715200
Murphy, M. P. (2009). How mitochondria produce reactive oxygen species. Biochem. J. 417 (1), 1–13. doi:10.1042/BJ20081386
Nagila, A., Permpongpaiboon, T., Tantrarongroj, S., Porapakkham, P., Chinwattana, K., Deakin, S., et al. (2009). Effect of atorvastatin on paraoxonase1 (PON1) and oxidative status. Pharmacol. Rep. P. R. 61 (5), 892–898. doi:10.1016/s1734-1140(09)70146-x
Nahdi, A. M. T. A., John, A., and Raza, H. (2017). Elucidation of molecular mechanisms of streptozotocin-induced oxidative stress, apoptosis, and mitochondrial dysfunction in rin-5F pancreatic β-cells. Oxidative Med. Cell. Longev. 2017, 7054272. doi:10.1155/2017/7054272
Nakanishi, A., Wada, Y., Kitagishi, Y., and Matsuda, S. (2014). Link between PI3K/AKT/PTEN pathway and NOX proteinin diseases. Aging Dis. 5 (3), 203–211. doi:10.14336/AD.2014.0500203
Nam, W., Nam, S. H., Kim, S. P., Levin, C., and Friedman, M. (2019). Anti-adipogenic and anti-obesity activities of purpurin in 3T3-L1 preadipocyte cells and in mice fed a high-fat diet. BMC COMPLEMENTARY Altern. Med. 19 (1), 364. doi:10.1186/s12906-019-2756-5
Niu, N., Xu, S., Xu, Y., Little, P. J., and Jin, Z.-G. (2019). Targeting mechanosensitive transcription factors in atherosclerosis. Trends Pharmacol. Sci. 40 (4), 253–266. doi:10.1016/j.tips.2019.02.004
Oguntibeju, O. O., Meyer, S., Aboua, Y. G., and Goboza, M. (2016). Hypoxis hemerocallidea significantly reduced hyperglycaemia and hyperglycaemic-induced oxidative stress in the liver and kidney tissues of streptozotocin-induced diabetic male wistar rats. Evid. Based Complement. Altern. Med. 2016, 8934362. doi:10.1155/2016/8934362
Ogunyinka, B. I., Oyinloye, B. E., Osunsanmi, F. O., Kolanisi, U., Opoku, A. R., and Kappo, A. P. (2019). Protein isolate from Parkia biglobosa seeds improves dyslipidaemia and cardiac oxidative stress in streptozotocin-induced diabetic rats. ANTIOXIDANTS 8 (10), 481. doi:10.3390/antiox8100481
Omodanisi, E. I., Aboua, Y. G., and Oguntibeju, O. O. (2017). Assessment of the anti-hyperglycaemic, anti-inflammatory and antioxidant activities of the methanol extract of Moringa oleifera in diabetes-induced nephrotoxic male wistar rats. MOLECULES 22 (4), 439. doi:10.3390/molecules22040439
Orrenius, S., Gogvadze, V., and Zhivotovsky, B. (2007). Mitochondrial oxidative stress: Implications for cell death. Annu. Rev. Pharmacol. Toxicol. 47, 143–183. doi:10.1146/annurev.pharmtox.47.120505.105122
Park, J.-G., and Oh, G. T. (2011). The role of peroxidases in the pathogenesis of atherosclerosis. BMB Rep. 44 (8), 497–505. doi:10.5483/bmbrep.2011.44.8.497
Park, S. H., Koo, H. J., Sung, Y. Y., and Kim, H. K. (2013). The protective effect of Prunella vulgaris ethanol extract against vascular inflammation in TNF-α-stimulated human aortic smooth muscle cells. BMB Rep. 46 (7), 352–357. doi:10.5483/bmbrep.2013.46.7.214
Passos, J. F., Saretzki, G., and von Zglinicki, T. (2007). DNA damage in telomeres and mitochondria during cellular senescence: Is there a connection? Nucleic Acids Res. 35 (22), 7505–7513. doi:10.1093/nar/gkm893
Pirillo, A., Casula, M., Olmastroni, E., Norata, G. D., and Catapano, A. L. (2021). Global epidemiology of dyslipidaemias. Nat. Rev. Cardiol. 18 (10), 689–700. doi:10.1038/s41569-021-00541-4
Poornima, M. S., Sindhu, G., Billu, A., Sruthi, C. R., Nisha, P., Gogoi, P., et al. (2022). Pretreatment of hydroethanolic extract of Dillenia indica L. attenuates oleic acid induced NAFLD in HepG2 cells via modulating SIRT-1/p-LKB-1/AMPK, HMGCR and PPAR-α signaling pathways. J. Ethnopharmacol. 292, 115237. doi:10.1016/j.jep.2022.115237
Qin, W. W., Xi, J. X., He, B. Q., Zhang, B. B., Luan, H. L., and Wu, F. H. (2016). Ameliorative effects of hispidulin on high glucose-mediated endothelial dysfunction via inhibition of PKC beta II-associated NLRP3 inflammasome activation and NF-kappa B signaling in endothelial cells. J. Funct. FOODS 27, 392–405. doi:10.1016/j.jff.2016.09.021
Qiu, F., and Zhang, Y.-Q. (2019). Metabolic effects of mulberry branch bark powder on diabetic mice based on GC-MS metabolomics approach. Nutr. Metabolism 16, 10. doi:10.1186/s12986-019-0335-x
Quesada, I., de Paola, M., Alvarez, M. S., Hapon, M. B., Gamarra-Luques, C., and Castro, C. (2021). Antioxidant and anti-atherogenic properties of prosopis strombulifera and Tessaria absinthioides aqueous extracts: Modulation of NADPH oxidase-derived reactive oxygen species. Front. PHYSIOLOGY 12, 662833. doi:10.3389/fphys.2021.662833
Ren, Y., and Shen, H.-M. (2019). Critical role of AMPK in redox regulation under glucose starvation. Redox Biol. 25, 101154. doi:10.1016/j.redox.2019.101154
Rout, D., Dash, U. C., Kanhar, S., Swain, S. K., and Sahoo, A. K. (2020). The modulatory role of prime identified compounds in the bioactive fraction of Homalium zeylanicum in high-fat diet fed-streptozotocin-induced type 2 diabetic rats. J. Ethnopharmacol. 260, 113099. doi:10.1016/j.jep.2020.113099
Saaoud, F., Drummer, I. V, C., Shao, Y., Sun, Y., Lu, Y., Xu, K., et al. (2021). Circular RNAs are a novel type of non-coding RNAs in ROS regulation, cardiovascular metabolic inflammations and cancers. Pharmacol. Ther. 220, 107715. doi:10.1016/j.pharmthera.2020.107715
Saifi, M. A., Seal, S., and Godugu, C. (2021). Nanoceria, the versatile nanoparticles: Promising biomedical applications. J. Control. Release Official J. Control. Release Soc. 338, 164–189. doi:10.1016/j.jconrel.2021.08.033
Salvamani, S., Gunasekaran, B., Shukor, M. Y., Abu Bakar, M. Z., and Ahmad, S. A. (2016). Phytochemical investigation, hypocholesterolemic and anti-atherosclerotic effects of Amaranthus viridis leaf extract in hypercholesterolemia-induced rabbits. RSC Adv. 6 (39), 32685–32696. doi:10.1039/c6ra04827g
Schieber, M., and Chandel, N. S. (2014). ROS function in redox signaling and oxidative stress. Curr. Biol. CB 24 (10), R453–R462. doi:10.1016/j.cub.2014.03.034
Schofield, J. H., and Schafer, Z. T. (2021). Mitochondrial reactive oxygen species and mitophagy: A complex and nuanced relationship. Antioxidants Redox Signal. 34 (7), 517–530. doi:10.1089/ars.2020.8058
Sekiou, O., Boumendjel, M., Taibi, F., Tichati, L., Boumendjel, A., and Messarah, M. (2021). Nephroprotective effect of Artemisia herba alba aqueous extract in alloxan-induced diabetic rats. J. Tradit. Complement. Med. 11 (1), 53–61. doi:10.1016/j.jtcme.2020.01.001
Shadel, G. S., and Horvath, T. L. (2015). Mitochondrial ROS signaling in organismal homeostasis. Cell 163 (3), 560–569. doi:10.1016/j.cell.2015.10.001
Shah, A., Gray, K., Figg, N., Finigan, A., Starks, L., and Bennett, M. (2018). Defective base excision repair of oxidative DNA damage in vascular smooth muscle cells promotes atherosclerosis. Circulation 138 (14), 1446–1462. doi:10.1161/CIRCULATIONAHA.117.033249
Sharma, P., Chakraborty, R., Wang, L., Min, B., Tremblay, M. L., Kawahara, T., et al. (2008). Redox regulation of interleukin-4 signaling. Immunity 29 (4), 551–564. doi:10.1016/j.immuni.2008.07.019
Shatoor, A. S., and Al Humayed, S. (2020). The protective effect of crataegus aronia against high-fat diet-induced vascular inflammation in rats entails inhibition of the NLRP-3 inflammasome pathway. Cardiovasc. Toxicol. 20 (1), 82–99. doi:10.1007/s12012-019-09534-9
Shi, J., Gao, W., and Shao, F. (2017). Pyroptosis: Gasdermin-Mediated programmed necrotic cell death. Trends Biochem. Sci. 42 (4), 245–254. doi:10.1016/j.tibs.2016.10.004
Shih, D. M., Xia, Y. R., Wang, X. P., Miller, E., Castellani, L. W., Subbanagounder, G., et al. (2000). Combined serum paraoxonase knockout/apolipoprotein E knockout mice exhibit increased lipoprotein oxidation and atherosclerosis. J. Biol. Chem. 275 (23), 17527–17535. doi:10.1074/jbc.M910376199
Sies, H., Belousov, V. V., Chandel, N. S., Davies, M. J., Jones, D. P., Mann, G. E., et al. (2022). Defining roles of specific reactive oxygen species (ROS) in cell biology and physiology. Nat. Rev. Mol. Cell Biol. 23 (7), 499–515. doi:10.1038/s41580-022-00456-z
Sies, H., Berndt, C., and Jones, D. P. (2017). Oxidative stress. Annu. Rev. Biochem. 86, 715–748. doi:10.1146/annurev-biochem-061516-045037
Sies, H., and Jones, D. P. (2020). Reactive oxygen species (ROS) as pleiotropic physiological signalling agents. Nat. Rev. Mol. Cell Biol. 21 (7), 363–383. doi:10.1038/s41580-020-0230-3
Sies, H. (2015). Oxidative stress: A concept in redox biology and medicine. Redox Biol. 4, 180–183. doi:10.1016/j.redox.2015.01.002
Sivinski, J., Zhang, D. D., and Chapman, E. (2021). Targeting NRF2 to treat cancer. Seminars Cancer Biol. 76, 61–73. doi:10.1016/j.semcancer.2021.06.003
Srivastava, A., Bhatt, N. M., Patel, T. P., Dadheech, N., Singh, A., and Gupta, S. (2016). Anti-apoptotic and cytoprotective effect of Enicostemma littorale against oxidative stress in Islets of Langerhans. Pharm. Biol. 54 (10), 2061–2072. doi:10.3109/13880209.2016.1141222
Starkov, A. A., and Fiskum, G. (2003). Regulation of brain mitochondrial H2O2 production by membrane potential and NAD(P)H redox state. J. Neurochem. 86 (5), 1101–1107. doi:10.1046/j.1471-4159.2003.01908.x
Stiles, B. L. (2009). PI-3-K and AKT: Onto the mitochondria. Adv. Drug Deliv. Rev. 61 (14), 1276–1282. doi:10.1016/j.addr.2009.07.017
Su, Q., Dong, J., Zhang, D. L., Yang, L., and Roy, R. (2022). Protective effects of the bilobalide on retinal oxidative stress and inflammation in streptozotocin-induced diabetic rats. Appl. Biochem. Biotechnol. 194 (12), 6407–6422. doi:10.1007/s12010-022-04012-5
Su, Q., Sun, Y. H., Ye, Z. L., Yang, H. F., Kong, B. H., and Li, L. (2018). Pinocembrin protects endothelial cells from oxidized LDL-induced injury. CYTOKINE 111, 475–480. doi:10.1016/j.cyto.2018.05.033
Sun, H., Saeedi, P., Karuranga, S., Pinkepank, M., Ogurtsova, K., Duncan, B. B., et al. (2022). IDF Diabetes Atlas: Global, regional and country-level diabetes prevalence estimates for 2021 and projections for 2045. Diabetes Res. Clin. Pract. 183, 109119. doi:10.1016/j.diabres.2021.109119
Sun, M., Zhu, T., Tong, J., Caidan, R., Wang, K., Kai, G., et al. (2020). Screening active components from Rubus amabilis for pancreatic β-cells protection. Pharm. Biol. 58 (1), 674–685. doi:10.1080/13880209.2020.1787467
Sun, Y. R., He, L. B., Wang, W., Wang, T. Y., Hua, W., Li, T. T., et al. (2021). Polyphenols from Penthorum chinense Pursh. Attenuates high glucose-induced vascular inflammation through directly interacting with Keap1 protein. J. Ethnopharmacol. 268, 113617. doi:10.1016/j.jep.2020.113617
Sutterwala, F. S., Ogura, Y., Szczepanik, M., Lara-Tejero, M., Lichtenberger, G. S., Grant, E. P., et al. (2006). Critical role for NALP3/CIAS1/Cryopyrin in innate and adaptive immunity through its regulation of caspase-1. Immunity 24 (3), 317–327. doi:10.1016/j.immuni.2006.02.004
Takaki, A., Kawai, D., and Yamamoto, K. (2013). Multiple hits, including oxidative stress, as pathogenesis and treatment target in non-alcoholic steatohepatitis (NASH). Int. J. Mol. Sci. 14 (10), 20704–20728. doi:10.3390/ijms141020704
Tang, G., Duan, F., Li, W., Wang, Y., Zeng, C., Hu, J., et al. (2019). Metformin inhibited Nod-like receptor protein 3 inflammasomes activation and suppressed diabetes-accelerated atherosclerosis in apoE-/- mice. Biomed. Pharmacother. = Biomedecine Pharmacother. 119, 109410. doi:10.1016/j.biopha.2019.109410
Tang, G., Li, S., Zhang, C., Chen, H., Wang, N., and Feng, Y. (2021). Clinical efficacies, underlying mechanisms and molecular targets of Chinese medicines for diabetic nephropathy treatment and management. Acta Pharm. Sin. B 11 (9), 2749–2767. doi:10.1016/j.apsb.2020.12.020
Trnovska, J., Svoboda, P., Pelantova, H., Kuzma, M., Kratochvilova, H., Kasperova, B. J., et al. (2021). Complex positive effects of SGLT-2 inhibitor empagliflozin in the liver, kidney and adipose tissue of hereditary hypertriglyceridemic rats: Possible contribution of attenuation of cell senescence and oxidative stress. Int. J. Mol. Sci. 22 (19), 10606. doi:10.3390/ijms221910606
Tsai, H. Y., Huang, P. H., Lin, F. Y., Chen, J. S., Lin, S. J., and Chen, J. W. (2013). Ginkgo biloba extract reduces high-glucose-induced endothelial reactive oxygen species generation and cell adhesion molecule expression by enhancing HO-1 expression via Akt/eNOS and p38 MAP kinase pathways. Eur. J. Pharm. Sci. 48 (4-5), 803–811. doi:10.1016/j.ejps.2013.01.002
Tschopp, J., and Schroder, K. (2010). NLRP3 inflammasome activation: The convergence of multiple signalling pathways on ROS production? Nat. Rev. Immunol. 10 (3), 210–215. doi:10.1038/nri2725
Vallabhapurapu, S., and Karin, M. (2009). Regulation and function of NF-kappaB transcription factors in the immune system. Annu. Rev. Immunol. 27, 693–733. doi:10.1146/annurev.immunol.021908.132641
Vanhaesebroeck, B., Perry, M. W. D., Brown, J. R., André, F., and Okkenhaug, K. (2021). PI3K inhibitors are finally coming of age. Nat. Rev. Drug Discov. 20 (10), 741–769. doi:10.1038/s41573-021-00209-1
Vendrov, A. E., Hakim, Z. S., Madamanchi, N. R., Rojas, M., Madamanchi, C., and Runge, M. S. (2007). Atherosclerosis is attenuated by limiting superoxide generation in both macrophages and vessel wall cells. Arteriosclerosis, Thrombosis, Vasc. Biol. 27 (12), 2714–2721. doi:10.1161/ATVBAHA.107.152629
Vezza, T., de Marañón, A. M., Canet, F., Díaz-Pozo, P., Marti, M., D'Ocon, P., et al. (2021). MicroRNAs and oxidative stress: An intriguing crosstalk to Be exploited in the management of type 2 diabetes. Antioxidants (Basel, Switz. 10 (5), 802. doi:10.3390/antiox10050802
Vijayaraj, P., Muthukumar, K., Sabarirajan, J., and Nachiappan, V. (2013). Antihyperlipidemic activity of Cassia auriculata flowers in triton WR 1339 induced hyperlipidemic rats. Exp. Toxicol. Pathol. 65 (1-2), 135–141. doi:10.1016/j.etp.2011.07.001
Wang, Q., Zhang, M., Torres, G., Wu, S., Ouyang, C., Xie, Z., et al. (2017). Metformin suppresses diabetes-accelerated atherosclerosis via the inhibition of drp1-mediated mitochondrial fission. Diabetes 66 (1), 193–205. doi:10.2337/db16-0915
Wang, Y., Li, L., Zhao, W., Dou, Y., An, H., Tao, H., et al. (2018). Targeted therapy of atherosclerosis by a broad-spectrum reactive oxygen species scavenging nanoparticle with intrinsic anti-inflammatory activity. ACS Nano 12 (9), 8943–8960. doi:10.1021/acsnano.8b02037
Wu, C. Q., Jing, M. H., Yang, L. J., Jin, L., Ding, Y. C., Lu, J., et al. (2018). Alisol A 24-acetate ameliorates nonalcoholic steatohepatitis by inhibiting oxidative stress and stimulating autophagy through the AMPK/mTOR pathway. CHEMICO-BIOLOGICAL Interact. 291, 111–119. doi:10.1016/j.cbi.2018.06.005
Wu, M., Yang, Z., Zhang, C., Shi, Y., Han, W., Song, S., et al. (2021). Inhibition of NLRP3 inflammasome ameliorates podocyte damage by suppressing lipid accumulation in diabetic nephropathy. Metabolism Clin. Exp. 118, 154748. doi:10.1016/j.metabol.2021.154748
Xu, X. X., Zhang, W., Zhang, P., Qi, X. M., Wu, Y. G., and Shen, J. J. (2013). Superior renoprotective effects of the combination of breviscapine with enalapril and its mechanism in diabetic rats. Phytomedicine 20 (10), 820–827. doi:10.1016/j.phymed.2013.03.027
Yahfoufi, N., Alsadi, N., Jambi, M., and Matar, C. (2018). The immunomodulatory and anti-inflammatory role of polyphenols. NUTRIENTS 10 (11), 1618. doi:10.3390/nu10111618
Yang, X., Yao, W., Shi, H., Liu, H., Li, Y., Gao, Y., et al. (2016). Paeoniflorin protects Schwann cells against high glucose induced oxidative injury by activating Nrf2/ARE pathway and inhibiting apoptosis. J. Ethnopharmacol. 185, 361–369. doi:10.1016/j.jep.2016.03.031
Yaribeygi, H., Sathyapalan, T., Atkin, S. L., and Sahebkar, A. (2020). Molecular mechanisms linking oxidative stress and diabetes mellitus. Oxidative Med. Cell. Longev. 2020, 8609213. doi:10.1155/2020/8609213
Yen, I. C., Lin, J.-C., Chen, Y., Tu, Q.-W., and Lee, S.-Y. (2020). Antrodia Cinnamomea attenuates non-alcoholic steatohepatitis by suppressing NLRP3 inflammasome activation in vitro and in vivo. Am. J. Chin. Med. 48 (8), 1859–1874. doi:10.1142/S0192415X20500937
Yoshitomi, T., and Nagasaki, Y. (2014). Reactive oxygen species-scavenging nanomedicines for the treatment of oxidative stress injuries. Adv. Healthc. Mater. 3 (8), 1149–1161. doi:10.1002/adhm.201300576
Yu, E., Calvert, P. A., Mercer, J. R., Harrison, J., Baker, L., Figg, N. L., et al. (2013). Mitochondrial DNA damage can promote atherosclerosis independently of reactive oxygen species through effects on smooth muscle cells and monocytes and correlates with higher-risk plaques in humans. Circulation 128 (7), 702–712. doi:10.1161/CIRCULATIONAHA.113.002271
Yu, L., Hong, W., Lu, S., Li, Y., Guan, Y., Weng, X., et al. (2022). The NLRP3 inflammasome in non-alcoholic fatty liver disease and steatohepatitis: Therapeutic targets and treatment. Front. Pharmacol. 13, 780496. doi:10.3389/fphar.2022.780496
Zeeshan, H. M. A., Lee, G. H., Kim, H.-R., and Chae, H.-J. (2016). Endoplasmic reticulum stress and associated ROS. Int. J. Mol. Sci. 17 (3), 327. doi:10.3390/ijms17030327
Zeng, Z., He, X., Li, C., Lin, S., Chen, H., Liu, L., et al. (2021). Oral delivery of antioxidant enzymes for effective treatment of inflammatory disease. Biomaterials 271, 120753. doi:10.1016/j.biomaterials.2021.120753
Zhang, J., Li, S., Li, S., Zhang, S. Q., Wang, Y. H., Jin, S. P., et al. (2020). Effect of icariside II and metformin on penile erectile function, glucose metabolism, reaction oxygen species, superoxide dismutase, and mitochondrial autophagy in type 2 diabetic rats with erectile dysfunction. Transl. Androl. UROLOGY 9 (2), 355–366. doi:10.21037/tau.2020.02.07
Zhang, M. H., Feng, L., Zhu, M. M., Gu, J. F., Jiang, J., Cheng, X. D., et al. (2014). The anti-inflammation effect of Moutan Cortex on advanced glycation end products-induced rat mesangial cells dysfunction and High-glucose-fat diet and streptozotocin-induced diabetic nephropathy rats. J. Ethnopharmacol. 151 (1), 591–600. doi:10.1016/j.jep.2013.11.015
Zhang, Q., Liu, J., Duan, H., Li, R., Peng, W., and Wu, C. (2021). Activation of Nrf2/HO-1 signaling: An important molecular mechanism of herbal medicine in the treatment of atherosclerosis via the protection of vascular endothelial cells from oxidative stress. J. Adv. Res. 34, 43–63. doi:10.1016/j.jare.2021.06.023
Zhang, S., Wang, W. D., Ma, J., Sheng, L., Zhang, D. M., and Chen, X. G. (2019). Coumarin glycosides from Hydrangea paniculata slow down the progression of diabetic nephropathy by targeting Nrf2 anti-oxidation and smad2/3-mediated profibrosis. PHYTOMEDICINE 57, 385–395. doi:10.1016/j.phymed.2018.12.045
Zhang, X. G., Liu, A. X., Zhang, Y. X., Zhou, M. Y., Li, X. Y., Fu, M. H., et al. (2022a). A diarylheptanoid compound from Alpinia officinarum Hance ameliorates high glucose-induced insulin resistance by regulating PI3K/AKT-Nrf2-GSK3 beta signaling pathways in HepG2 cells. J. Ethnopharmacol. 295, 115397. doi:10.1016/j.jep.2022.115397
Zhang, Z., Dalan, R., Hu, Z., Wang, J.-W., Chew, N. W., Poh, K.-K., et al. (2022b). Reactive oxygen species scavenging nanomedicine for the treatment of ischemic heart disease. Adv. Mater. Deerf. Beach, Fla.) 34 (35), e2202169. doi:10.1002/adma.202202169
Zhao, W. W., Feng, H. T., Guo, S. H., Han, Y. T., and Chen, X. P. (2017a). Danshenol A inhibits TNF-alpha-induced expression of intercellular adhesion molecule-1 (ICAM-1) mediated by NOX4 in endothelial cells. Sci. Rep. 7, 12953. doi:10.1038/s41598-017-13072-1
Zhao, W. W., Yuan, Y., Zhao, H. Y., Han, Y. T., and Chen, X. P. (2019). Aqueous extract of Salvia miltiorrhiza Bunge-Radix Puerariae herb pair ameliorates diabetic vascular injury by inhibiting oxidative stress in streptozotocin-induced diabetic rats. FOOD Chem. Toxicol. 129, 97–107. doi:10.1016/j.fct.2019.04.018
Zhao, Y., Hu, X., Liu, Y., Dong, S., Wen, Z., He, W., et al. (2017b). ROS signaling under metabolic stress: Cross-talk between AMPK and AKT pathway. Mol. Cancer 16 (1), 79. doi:10.1186/s12943-017-0648-1
Zhou, L., Liu, Y., Wang, Z., Liu, D., Xie, B., Zhang, Y., et al. (2021a). Activation of NADPH oxidase mediates mitochondrial oxidative stress and atrial remodeling in diabetic rabbits. Life Sci. 272, 119240. doi:10.1016/j.lfs.2021.119240
Zhou, Y., Zhou, C., Zhang, X., Vong, C. T., Wang, Y., and Cheang, W. S. (2021b). Coptisine attenuates diabetes-associated endothelial dysfunction through inhibition of endoplasmic reticulum stress and oxidative stress. Mol. (Basel, Switz. 26 (14), 4210. doi:10.3390/molecules26144210
Zhu, Z. S., Li, J. Y., and Zhang, X. R. (2019). Astragaloside IV protects against oxidized low-density lipoprotein (ox-LDL)-Induced endothelial cell injury by reducing oxidative stress and inflammation. Med. Sci. Monit. 25, 2132–2140. doi:10.12659/MSM.912894
Zmijewski, J. W., Banerjee, S., Bae, H., Friggeri, A., Lazarowski, E. R., and Abraham, E. (2010). Exposure to hydrogen peroxide induces oxidation and activation of AMP-activated protein kinase. J. Biol. Chem. 285 (43), 33154–33164. doi:10.1074/jbc.M110.143685
Glossary
Keywords: botanical drugs, glycolipid metabolic diseases, oxidative stress, radical oxygen species, mitochondria function, nicotinamide adenine dinucleotide phosphate hydrogen oxidase, signaling pathways
Citation: Luo M, Zheng Y, Tang S, Gu L, Zhu Y, Ying R, Liu Y, Ma J, Guo R, Gao P and Zhang C (2023) Radical oxygen species: an important breakthrough point for botanical drugs to regulate oxidative stress and treat the disorder of glycolipid metabolism. Front. Pharmacol. 14:1166178. doi: 10.3389/fphar.2023.1166178
Received: 14 February 2023; Accepted: 21 April 2023;
Published: 12 May 2023.
Edited by:
Cailu Lin, Monell Chemical Senses Center, United StatesReviewed by:
Mengmeng Zhang, Shaanxi University of Chinese Medicine, ChinaYunbin Jiang, Southwest University, China
Copyright © 2023 Luo, Zheng, Tang, Gu, Zhu, Ying, Liu, Ma, Guo, Gao and Zhang. This is an open-access article distributed under the terms of the Creative Commons Attribution License (CC BY). The use, distribution or reproduction in other forums is permitted, provided the original author(s) and the copyright owner(s) are credited and that the original publication in this journal is cited, in accordance with accepted academic practice. No use, distribution or reproduction is permitted which does not comply with these terms.
*Correspondence: Peiyang Gao, Z2FvcHk5MzBAMTI2LmNvbQ==; Chuantao Zhang, emhhbmdjaHVhbnRhb0BjZHV0Y20uZWR1LmNu
†These authors have contributed equally to this work and share first authorship