- 1Department of Cardiology, Affiliated Hangzhou First People’s Hospital, Zhejiang University School of Medicine, Hangzhou, Zhejiang, China
- 2Department of Laboratory Medicine, Affiliated Hangzhou First People’s Hospital, Zhejiang University School of Medicine, Hangzhou, Zhejiang, China
Immune checkpoint inhibitors (ICIs), including cytotoxic T-lymphocyte antigen 4 (CTLA-4), programmed cell death 1 (PD-1), and its ligand 1 (PD-L1), have improved the survival in multiple types of cancers; however, ICIs may cause cardiovascular toxicity. Although rare, ICI-mediated cardiotoxicity is an extremely serious complication with a relatively high mortality. In this review, we discuss the underlying mechanism and clinical manifestations of cardiovascular toxicity induced by ICIs. According to previous studies, multiple signaling pathways are involved in myocarditis induced by ICIs. Further, we summarize the clinical trials of drugs for the treatment of ICI-associated myocarditis. Although these drugs have shown the beneficial effects of alleviating cardiac function and reducing mortality rates, their efficacy is not optimal. Finally, we discuss the therapeutic potential of some novel compounds as well as the underlying mechanisms of their action.
1 Introduction
Although some of the main cancer therapies, such as chemotherapy and radiation therapy, have significantly improved long-term survival rates, an increased risk of cardiac dysfunction has been observed in cancer survivors (Kostakou et al., 2019). Immune checkpoint inhibitors (ICIs), which represent the most notable breakthrough in cancer therapy, have shown significant clinical efficacy with reduced adverse events (Gong et al., 2018). Although rare, cardiovascular toxicities associated with ICIs are often serious complications with a relatively high mortality (Lyon et al., 2018).
The therapeutic mechanism of ICIs is based on targeting certain immunoregulatory signaling molecules, including cytotoxic T-lymphocyte antigen 4 (CTLA-4), programmed cell death 1 (PD-1), and its ligand 1 (PD-L1), which activate T cells and inhibit the growth of tumor cells (Ribas and Wolchok, 2018). So far, eight agents, including one CTLA-4-blocking antibody (ipilimumab), three PD-1-blocking antibodies (nivolumab, pembrolizumab, cemiplimab, and dostarlimab), and three PD-L1-blocking antibodies (atezolizumab, avelumab, and durvalumab) have been approved for clinical use by the United States Food and Drug Administration (US FDA) (Wu et al., 2022). However, reports on immune-mediated cardiovascular toxicities have been increasing, and up to 80% of patients treated with ICIs may experience such adverse events and even death (Mir et al., 2018). Indeed, the rate of various adverse cardiac events, such as cardiovascular arrest, cardiogenic shock, and myocardial infarction, in patients treated with ICIs is four times greater than that in patients without ICI treatment (Moslehi et al., 2018). Therefore, multiple immunosuppressant drugs, such as methylprednisolone, prednisone, infliximab, anti-thymocyte globulin, mycophenolate mofetil rituximab, and tacrolimus, that attenuate the cardiotoxicity induced by ICIs have been widely investigated (Brahmer et al., 2018; Chen et al., 2021a; Kennedy et al., 2022); however, the efficacy of these drugs is not optimal and their use requires immediate termination of ICI treatment.
We searched the PubMed, Embase, Cochrane Library, and China National Knowledge Infrastructure (CNKI) electronic databases for the following terms: 1) “immune checkpoint inhibitors” or “PD-1” or “PD-L1” or “CTLA-4” and 2) “myocarditis” or “cardiotoxicity”. The searches were limited to studies published in English or Chinese. The final literature searches were performed on 5 Dec 2022.
In this review, we summarize the pathogenic mechanism of ICI-induced cardiotoxicity and evaluate the commonly used cardioprotective drugs. We also review the putative molecular mechanism underlying the effects of new drugs used to treat cardiotoxicity induced by ICIs in preclinical studies.
2 Molecular mechanisms of cardiotoxicity induced by CTLA-4 axis
CTLA-4 is expressed almost exclusively on T cells and binds to B7 molecules on antigen presenting cells (APCs) to promote tumor growth by inhibiting T cell immune response. Anti-CTLA-4 antibodies enhance immune responses and suppress neoantigen expression by activating the binding of CD28 to B7 and that of T cell receptor to major histocompatibility complex molecules, resulting in tumor cell elimination (Hu et al., 2019). The multiple processes involved in the mechanism of CTLA-4 blockade-associated cardiotoxicity, including fibrosis and inflammation, are summarized in Figure 1.
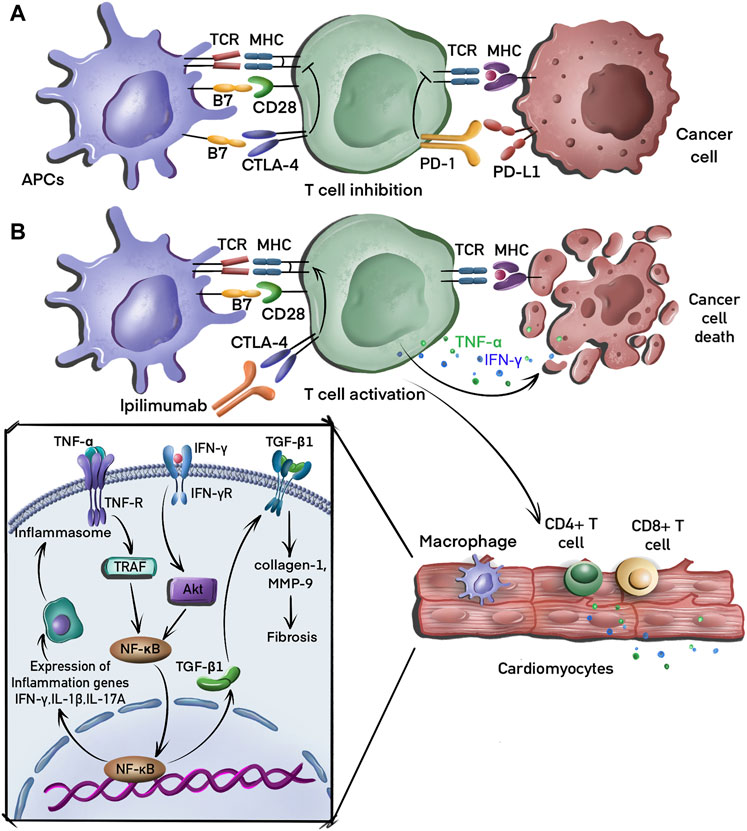
FIGURE 1. Molecular mechanisms of tumor evasion and cardiotoxicity induced by CTLA-4 blockade. (A) T cells recognize major histocompatibility complex (MHC) molecules on antigen presenting cells (APC), leading to the activation of these T cells, which then migrate to the tumor bed where they recognize cancer cells via the interaction between the MHC complex and T cell receptors (TCR) on the T cell. T cells receive inhibitory signals induced by the binding of CTLA-4 to B7 and PD-1 to PD-L1. (B) CTLA-4 inhibitor (ipilimumab) binds to CTLA-4 to activate T cells that eliminate tumor cell by secreting interferon-γ (IFN-γ) and tumor necrosis factor-alpha (TNF-α). CD4+ and CD8+ T cells also infiltrate the myocardial tissue, releasing TNF-α, which induces the production of proinflammatory cytokines, including IFN-γ, interleukin (IL)-2, and IL-17A via the activation of the nuclear factor-kappa B (NF-κB) signaling pathway. IFN-γ can also induce fibrosis by promoting the expression of transforming growth factor-beta 1 (TGF-β1).
2.1 Inflammation
In a preclinical mouse model, CTLA-4 knockout induced T cell and macrophage infiltration in myocardial tissues that destroyed cardiomyocytes, leading to metabolic failure with high mortality (Wei et al., 2021). The activation of T cells and macrophages induces a proinflammatory phenotype in cardiac and vascular tissues by increasing the expression of nuclear factor-kappa B (NF-κB), the NLRP3 inflammasome, and MyD88 (Quagliariello et al., 2022). The blockade of CTLA-4 in cardiomyocytes also led to an increased levels of proinflammatory cytokines, including those of interferon-gamma (IFN-γ), tumor necrosis factor-alpha (TNF-α), interleukin (IL)-2, and IL-17A in the myocardium (Han et al., 2012). Although the underlying mechanism is unclear, it is hypothesized that CTLA-4 blockade leads to the differentiation of inflammatory effector CD4+ T cells that migrate back to the heart and increase the expression of these proinflammatory cytokines (Lichtman, 2013). Finally, CTLA-4 blockade therapy induces endothelial activation to increase the expression of intercellular adhesion molecule 1 (ICAM1) in the aortic endothelium. As the ligand for β2-integrin molecules, ICAM1 plays an important role in inflammation (Poels et al., 2020). The reduction of ICAM1 expression has been shown to decrease cardiac inflammation and ameliorate systolic dysfunction during pressure overload (Yoshida et al., 2015).
2.2 Fibrosis
Several clinical cases of interstitial fibrosis in myocardial tissue have been identified in endomyocardial biopsies after CTLA-4 inhibitor treatment (Heinzerling et al., 2016); however, the underlying mechanism is unknown. A preclinical study showed that the depletion of T cells enhances silica-induced fibrosis by increasing the secretion of transforming growth factor-beta 1 (TGF-β1), which is associated with the suppression of CTLA-4 expression (Liu et al., 2010). CTLA-4 blocking agents also increase myocardial fibrosis by modulating the expression of galectin-3, collagen 1, and matrix metalloproteinase 9 (MMP-9) (Quagliariello et al., 2022). By contrast, a recent study demonstrated that CTLA4-Ig-mediated immunosuppression limits subepithelial fibrosis (Khan et al., 2022). Further studies are required to elucidate the regulatory mechanism of CTLA-4 inhibitors.
3 Molecular mechanisms of cardiotoxicity induced by PD-1/PD-L1 blockade
PD-1 and PD-L1 are important targets expressed on most immune cells, including T cells and B cells. Tumor neoantigens released by cancer cells are captured and presented to T cells by APCs. However, PD-L1, which is expressed at high levels on cancer cells, binds to PD-1 on T cells, resulting in inhibitory checkpoint signaling that inhibits their activation, thereby allowing unregulated tumor growth. PD-1 blocking antibodies suppress the interaction of PD-1 with PD-L1, resulting in enhanced T cell cytotoxicity and ultimately in tumor cell elimination (Varricchi et al., 2017). The mechanisms considered to play an important role in cardiotoxicity induced by PD-1/PD-L1 blockade are presented in Figure 2.
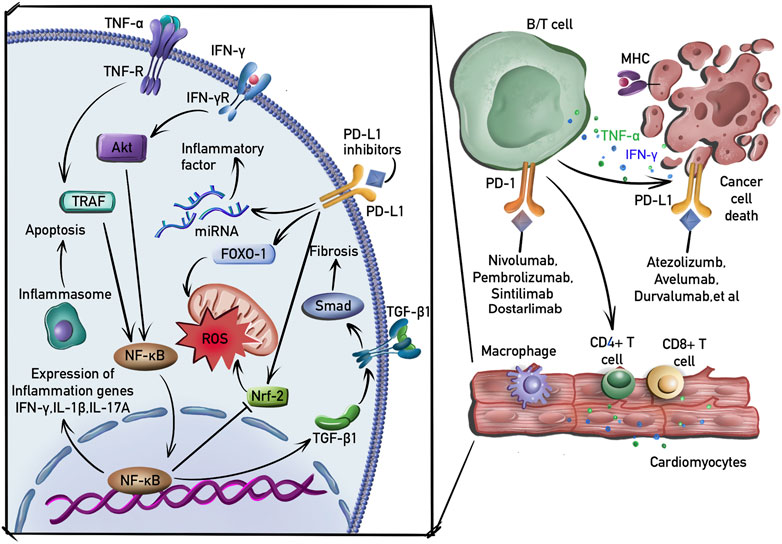
FIGURE 2. Molecular mechanisms of cardiotoxicity induced by PD-1/PD-L1 blockade. B and T cells are activated by PD-1 or PD-L1 inhibitors. Macrophages, CD4+ and CD4+ T cells then infiltrate the myocardial tissue. In addition to the induction of inflammation and fibrosis by activating NF-κB and TGF-β1 signaling pathway, PDL-1 inhibitors induce the production of ROS by suppressing nuclear factor erythroid 2-related factor-2 (Nrf-2) and upregulating transcriptional factor forkhead box-1 (FOXO-1). PD-1 inhibitors also activate macrophages and induce the expression of proinflammatory cytokines by regulating the expression of miRNAs.
3.1 Inflammation
It has been reported that the cardioprotective effects of the PD-1/PD-L1 interaction are mediated by suppressing the production of inflammatory factors and that the disturbance of this interaction neutralizes this protective effect to cause cardiotoxicity (Seko et al., 2007). The inhibition of the PD-1/PD-L1 interaction in an animal model has been shown to induce the expression of several proinflammatory factors, including TNF-α and IL-1β, leading to cardiomyocyte apoptosis (Chen et al., 2022). PD-1/PD-L1 blockade also induces cardiac inflammation by increasing the expression of IFN-γ and promoting cardiomyocyte apoptosis via a mechanism that involves the reduction of p-Akt expression (Tay et al., 2020). In addition, this inflammatory cardiotoxicity was associated with the infiltration of CD4+/CD8+ T cells (Ji et al., 2019). Furthermore, PD-L1 blockade reduced B-cell production of the anti-inflammatory cytokine IL-10, thereby limiting inflammatory responses (Wang and Tan, 2020).
Inflammation is a key process in the development of atherosclerosis, which is characterized by the accumulation of lipids and IFN-γ-producing T cells in atherosclerotic plaques (Buono et al., 2005). The stimulation of the PD-1 pathway delayed atherosclerosis progression by decreasing the frequency of atherogenic IFN-γ T cells (Grievink et al., 2021). However, PD-1/PD-L1 blockade also accelerated the progression of atherosclerosis by inducing a predominantly T cell-driven inflammation (Sun et al., 2020).
3.2 Fibrosis
PD-1(−/−) mice develop atrial myocardial fibrosis (Fu et al., 2013). Although the underlying mechanism is not yet known, one clinical study showed that the concentrations of TGF-β1 were increased in patients with lower expression of PD-1 (Zhang et al., 2022c). In addition, decreased PD-1/PD-L1 expression was correlated with decreased TGF-β1 in a previous animal study (Zhang et al., 2015), whereas increased PD-1 expression induced TGF-β1 expression (Celada et al., 2018). It is well known that PD-1 blockade activates the proliferation of regulatory T cells (Kamada et al., 2019), which play an important role in the progression of fibrosis by activating TGF-β1 production (Kagawa et al., 2022). TGF-β1 is a primary factor that drives fibrosis via the activation of Smad- and non-Smad-based signaling pathways that produce myofibroblasts (Meng et al., 2016). PD-1 inhibitors also increase the expression of fibronectin, a major pro-fibrotic cytokine, in cardiomyocytes; fibronectin plays an important role in the fibrotic process (Quagliariello et al., 2022).
3.3 Oxidative stress
T cell activation can lead to increased reactive oxygen species (ROS) production, resulting in oxidative stress, which is involved in PD-1/PD-L1 blockade-induced cardiotoxicity (Aboelella et al., 2021). ROS production is increased in the atrial myocytes of PD-1(−/−) mice (Fu et al., 2013). In addition, PD-1 blockade-induced oxidative stress is associated with an increase in IFN-γ-positive macrophages (Liu et al., 2021). IFN-γ induces ROS production by suppressing the PI3K/Akt/nuclear factor erythroid 2-related factor-2 (Nrf-2) pathway, resulting in reduced antioxidant activity (Niu et al., 2021). Furthermore, the inhibition of PD-1 results in the upregulated expression of transcriptional factor forkhead box-1 (FOXO-1), which promotes ROS production by limiting mitochondrial glucose oxidation and increasing lipid oxidation (Chistiakov et al., 2017).
3.4 Other mechanisms
Studies have demonstrated that ICI-induced cardiotoxicity is associated with the regulation of miRNA expression. For instance, PD-1 inhibitor treatment profoundly increased the expression of miR-34a-5p, which targets the serine/threonine-protein phosphatase 1 regulatory subunit 10, to induce cardiac senescence (Xia et al., 2020a). PD-1 inhibitors also induce macrophage polarization and the expression of some proinflammatory cytokines by activating miR-34a expression (Xia et al., 2020b).
4 Clinical evaluations of therapy against ICI-induced cardiotoxicity
ICI-associated myocarditis has a high rate of morbidity and mortality (Lyon et al., 2018). For instance, one observational study demonstrated that nearly 50% of all patients with ICI-induced myocarditis experienced a major adverse cardiac event, such as cardiovascular death, cardiogenic shock, or complete heart block (Mahmood et al., 2018b). Therefore, electrocardiogram (ECG), natriuretic peptides, and cardiac troponin measurements are recommended in all patients before starting ICI therapy according to the 2022 ESC Guidelines on cardio-oncology (Lyon et al., 2022). In addition, the management of ICI-induced cardiovascular toxicity should be performed depending on the severity of the cardiotoxicity, with termination of ICI in cancer patients with fulminant or nonfulminant ICI-associated myocarditis as the first consideration. Furthermore, such patients should be hospitalized and allocated a level 2 or 3 bed with continuous ECG monitoring. For milder ICI-mediated cardiotoxicity, the treatment strategies include immunosuppression to alleviate inflammatory changes and supportive therapy to address cardiac complications (Hu et al., 2019). Clinical studies on the efficacy of drug therapies for ICI-induced cardiotoxicity are summarized in Supplementary Table S1.
4.1 Methylprednisolone
In one clinical study, the rate of ICI-associated myocarditis reached approximately 25%, although only 2 of 35 patients exhibited the symptoms of a major adverse cardiac event after receiving 1,000 mg methylprednisolone (Mahmood et al., 2018b). Methylprednisolone is a common steroid that exerts rapid immunosuppressive effects by suppressing T cell function. Despite many case reports on these effects, a large randomized controlled trial is required to assess the efficacy of methylprednisolone in ameliorating cardiotoxicity induced by ICIs (Supplementary Table S1).
Sintilimab, a PD-1-specific monoclonal antibody, has been reported in numerous clinical studies and is widely used to treat multiple tumor types. Several case reports on the therapeutic efficacy of methylprednisolone in sintilimab-induced myocarditis and cardiac injury have been published (Xing et al., 2020; Bi et al., 2021; Lin et al., 2022); however, some patients who received sintilimab did not survive even after methylprednisolone treatment (Chen et al., 2021b).
Pembrolizumab is another PD-1 inhibitor approved by the US FDA in 2014 and has been used in many counties (Szuchan et al., 2020; Arangalage et al., 2021; Cao et al., 2021; Nguyen et al., 2022; Su et al., 2022; Yang et al., 2022). In cases of pembrolizumab-induced cardiotoxicity, patients present with the clinical symptoms of chest pain, shortness of breath, and dyspnea after treatment, with dramatically increased levels of myocardial injury markers, which decline to normal levels after methylprednisolone treatment (Supplementary Table S1). Methylprednisolone has been shown to ameliorate the symptoms of myocarditis and acute heart failure induced by other PD-1 inhibitors, including camrelizumab, nivolumab, tislelizumab, and toripalimab (Monge et al., 2018; Fazel and Jedlowski, 2019; Bai et al., 2021; Hu et al., 2021; Jespersen et al., 2021; Komatsu et al., 2021; Luo et al., 2021; Wang et al., 2021; Zhang et al., 2022a). These patients commonly exhibit the symptoms of chest pain and shortness of breath approximately 1 or 2 weeks after PD-1 inhibitor treatment. Methylprednisolone usually alleviates these symptoms and reduces the myocardial injury markers in serum; however, some patients still die owing to cardiac dysfunction and other reasons (Monge et al., 2018; Jespersen et al., 2021; Komatsu et al., 2021; Wang et al., 2021; Zhang et al., 2022a). Therefore, Methylprednisolone therapy requires optimization. Finally, methylprednisolone has been shown to suppress myocarditis induced by the CTLA-4 inhibitor ipilimumab (Fazel and Jedlowski, 2019).
4.2 Prednisone
Prednisone is another glucocorticoid steroid widely used for the treatment of ICI-induced myocarditis (Supplementary Table S1). Compared with receiving intravenous methylprednisolone, the effective time of oral prednisone is relatively short and dependent on the metabolic activation of the liver. In the clinic, prednisone is preferred for the treatment of patients with moderate immune responses after methylprednisolone treatment. Prednisone combined with another immunosuppressive agent usually provides benefit in patients with cancer (Nasr et al., 2018; Yamaguchi et al., 2018; Agrawal et al., 2019; Guo et al., 2020; von Itzstein et al., 2020; Luo et al., 2021), although some patients do not survive (Konstantina et al., 2019). Furthermore, one case report showed that prednisone alone improved cardiac function in a patient with ICI-induced myocarditis (Miyauchi et al., 2021).
4.3 Infliximab
As some patients cannot tolerate high-dose corticosteroids, other immunosuppressive drugs, such as infliximab, can be regarded as second-line therapies (Hu et al., 2019). The monoclonal antibody infliximab suppresses inflammatory responses by specifically binding and neutralizing TNF-α. Several case reports have described the ability of infliximab treatment to successfully ameliorate ICI-induced myocardial injury (Supplementary Table S1). In some cases, infliximab treatment could eliminated arrhythmias and cardiac dysfunction (Martinez-Calle et al., 2018; Padegimas et al., 2019). However, one retrospective study demonstrated that patients treated with infliximab were those with persistent evidence of cardiac dysfunction such as heart failure and ventricular tachycardia and that two of the four patients died from septic shock 2–3 months after the initial treatment (Zhang et al., 2021). Furthermore, in one case report, methylprednisolone combined with a high dosage of infliximab (10 mg/kg) did not improve the condition of a patient who ultimately died in the hospital (Gallegos et al., 2019). Therefore, for patients with symptoms of heart failure, a lower dose of infliximab (5 mg/kg) may be safer (Chung et al., 2003).
4.4 Immunoglobulins
Immunoglobulins, which are widely used to treat fulminant myocarditis and acute inflammatory cardiomyopathy, function by suppressing the expression of inflammatory and proinflammatory cytokines at the mRNA level (Shioji et al., 2001). Indeed, in some cases, intravenous immunoglobulins successfully suppressed severe myocarditis induced by ICIs (Supplementary Table S1). Similar to the situation with infliximab, immunoglobulins are used as a second-line therapy if methylprednisolone or other drugs are ineffective in treating ICI-induced cardiotoxicity (Norwood et al., 2017; Nasr et al., 2018; Yamaguchi et al., 2018; Agrawal et al., 2019; Balanescu et al., 2020; Yanase et al., 2021; Yang et al., 2021). However, immunoglobulins also failed in some patients (Chen et al., 2018; Agrawal et al., 2019; Imai et al., 2019; Ono et al., 2022; Saishu et al., 2022). Although the reason for differences in the effectiveness of methylprednisolone in some cases is unclear, we hypothesize that these differences are caused by variations in the underlying mechanism of ICI-induced cardiotoxicity in the different types of cancers.
4.5 Anti-thymocyte globulin (ATG)
ATG is a polyclonal antibody that modulates immune responses by inducing T lymphocyte depletion and commonly used to prevent acute allograft rejection (Valdez-Ortiz et al., 2015). ATG has been reported to improve cardiac function impaired by ICIs (Supplementary Table S1). In one case, ATG temporarily improved cardiac function in a patient with ongoing shock and hypotension (Jain et al., 2018). In some patients, ATG has produced a favorable outcome when combined with other drugs (Tay et al., 2017; Itzhaki Ben Zadok et al., 2019). However, it does not produce beneficial effects in some patients, especially in those with melanoma (Agrawal et al., 2019; Arora et al., 2020) or who received chemotherapy/radiotherapy prior to ATG treatment (McDowall et al., 2019). Therefore, further clinical investigations are required to fully elucidate the mechanism underlying the protective effects of ATG against ICI-cardiotoxicity and identify patients who are likely to benefit.
4.6 Mycophenolate mofetil
Similar to ATG, mycophenolate mofetil is used to prevent acute allograft rejection by inhibiting the proliferation of T and B lymphocytes. In some case reports, mycophenolate mofetil combined with a high dosage of methylprednisolone successfully suppressed fulminant immune-mediated myocarditis in patients with advanced endometrial cancer and melanoma (Mahmood et al., 2018a; Esfahani et al., 2019; Liu et al., 2020). Interestingly, one study showed that mycophenolate mofetil alone could rescue cardiac function impaired by PD-1 and CTL4 inhibitors (Reddy et al., 2017). However, in another case, this drug therapy decreased the levels of a cardiac injury biomarker, although the patient died because of respiratory failure (Yogasundaram et al., 2021). Moreover, one patient who received 10-month chemotherapy treatment with mycophenolate mofetil died (Konstantina et al., 2019).
4.7 Alemtuzumab
Alemtuzumab is a monoclonal antibody that binds to CD52, a protein present on the surface of multiple immune cells. One case report showed that alemtuzumab was used as an adjuvant medication for a patient with melanoma who suffered myocarditis for PD-1 therapy; the treatment gradually reduced cardiac biomarkers (Esfahani et al., 2019).
4.8 Abatacept
Abatacept is an agonist of CTLA-4 that alleviates nivolumab-induced myocarditis in lung cancer treatment (Salem et al., 2019) as well as pembrolizumab-induced myocarditis in melanoma treatment (Liu et al., 2020).
4.9 Tocilizumab
Tocilizumab is an IL-6 receptor inhibitor that plays an important role in ICI-associated myocarditis. One case report showed that intravenous tocilizumab was administered for one patient with lung cancer who developed myocarditis after nivolumab and ipilimumab treatments which was attenaued by Tocilizumab (Doms et al., 2020).
4.10 Plasmapheresis
Plasmapheresis, which is commonly used for therapeutic plasma exchange, is now used as a third-line approach for treating ICI-induced myocarditis owing to its ability to rapidly remove circulating immune complexes from the blood of the patient (Patel et al., 2021). Plasmapheresis also successfully suppresses immune responses induced by ICIs in combination with other drugs (Frigeri et al., 2018; Nasr et al., 2018; Liu et al., 2020; Yanase et al., 2021; Yogasundaram et al., 2021).
5 Therapeutic mechanisms of other agents used for ICI-induced cardiotoxicity
There is now a large body of evidence showing that chemical substances and natural products from Chinese herbs can attenuate cardiotoxicity induced by ICIs (Table 1).
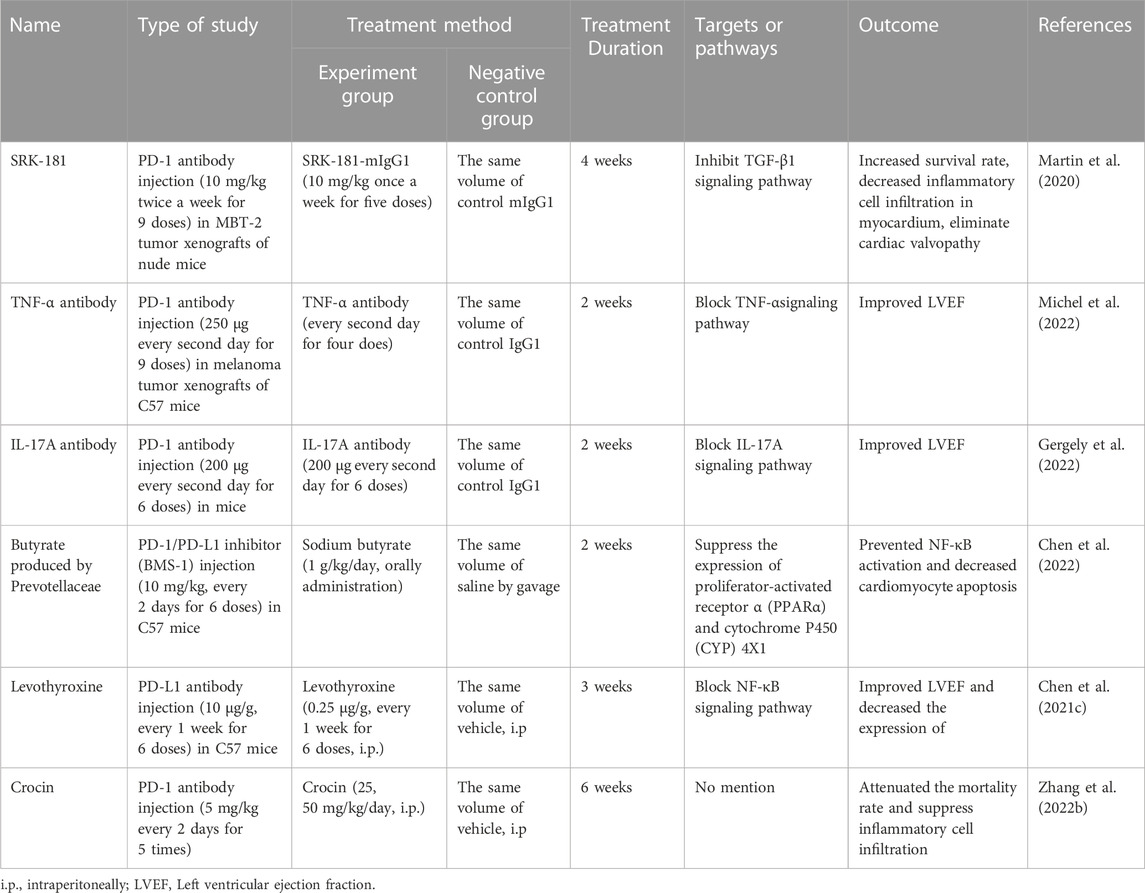
TABLE 1. The mechanisms by which some chemical substances and natural products treat ICIs-induced cardiotoxicity.
Immunoglobulins that bind to CTLA-4 and PD1/PD-L1 have been shown to ameliorate myocarditis (Wei et al., 2021). The CTLA-4-Ig abatacept attenuates ICI-associated myocarditis by blocking T cell stimulation through its ability to bind to B7 ligands. Abatacept treatment significantly decreased myocardial immune infiltrates and reduced mortality in PD-1(−/−) mice (Wei et al., 2021). Abatacept has also been beneficial in some clinical cases of ICI-induced cardiotoxicity (Salem et al., 2019; Liu et al., 2020).
TGF-β1 plays an important role in fibrosis induced by CTLA-4 and PD-1/PD-L1 inhibitors (Liu et al., 2010; Kagawa et al., 2022). SRK-181, a highly selective antibody inhibitor of TGF-β1, has been shown to increase intratumoral CD8+ T cells and decrease the frequency of immunosuppressive myeloid cells after anti-PD-1 treatment in an animal model (Martin et al., 2020). In addition, the blockade of TGF-β1 using a specific monoclonal antibody reduced tumor sizes (Terabe et al., 2017). Therefore, the combination of anti-TGF-β1 and anti-PD-1 has been implicated as a promising therapy for ICI-induced cardiotoxicity.
TNF-α has been predicted to mediate the cardiotoxic effects of ICI therapy. Increased cardiac TNF-α level was detected in tumor-bearing mice receiving anti-PD1 therapy, and TNF-α blockade improved the ejection fraction by enhancing the expression of lymphocyte-activation gene 3 and T cell immunoglobulin and mucin-domain containing-3 (TIM3), thereby enhancing the efficacy of anti-PD1 therapy (Michel et al., 2022).
IL-17A, which is an inflammatory cytokine produced primarily by Th17 cells, is activated by anti-PD1 treatment (Dulos et al., 2012). Furthermore, IL-17A was found to be associated with ventricular dilation and dysfunction in a mouse model of autoimmune myocarditis (Baldeviano et al., 2010). A recent study revealed that treatment with anti-IL17A antibody prevented the decline in ejection fraction and alleviated the cardiac dysfunction induced by PD-1 inhibitor (Gergely et al., 2022).
PD-1/PD-L1 inhibitors lead to gut microbiota dysbiosis, including the depletion of prevotellaceae and low production of microbial butyrate. Prevotellaceae enrichment or butyrate supplementation alleviated PD-1/PD-L1 inhibitor-induced myocardial enzyme leakage and myocardial apoptosis (Chen et al., 2022). These effects were associated with the upregulation of PPARα–CYP4X1 axis and downregulation of IL-1β and TNF-α in colonic macrophages. These findings provide a notable target for improving the safety of immunotherapy.
Interestingly, one study showed that levothyroxine rescued mice from ICI-caused mortality (Chen et al., 2021c). Cardiac function was significantly impaired at the early stage after PD-L1 inhibitor injection, with decreased cardiac ejection function and cardiac electrical conduction as well as a reduction in the body temperature, and these symptoms were effectively relieved by levothyroxine. However, the underlying mechanism of this effect remains to be elucidated.
Crocin, which is a major apo-carotenoid derived from saffron, partially reversed ICI-related myocarditis by improving cardiac function and alleviating myocardial injury. Mechanistically, it was shown that crocin inhibits theNLRP3 inflammasome and cleaved gasdermin D (GSDMD) and IL-1β (Zhang et al., 2022b). Therefore, we believe that Chinese herbs should be considered as promising therapeutic agents for the treatment of cardiotoxicity induced by ICIs.
6 Discussion
The pathogenesis of ICI-induced myocarditis is complex as multiple signaling pathways are activated by the infiltration of B and T lymphocytes as well as macrophages. Current immunosuppressive agents function mainly by suppressing B and T lymphocyte function or inhibiting inflammatory responses; however, according to the case reports reviewed here (Supplementary Table S1), approximately 50% of patients still do not survive such treatments. Although there is no widely accepted theory to account for the underlying mechanisms, we believe that once the symptoms of myocarditis present, the downstream signaling pathways, including inflammation (Han et al., 2012; Ji et al., 2019), fibrosis (Quagliariello et al., 2022), oxidative stress (Liu et al., 2021), and even apoptosis (Chen et al., 2022), are all activated. However, myocyte fibrosis/necrosis is a common histopathologic finding in patients with cancer treated with ICIs (Touat et al., 2018; Gallegos et al., 2019; Padegimas et al., 2019; Quagliariello et al., 2019); therefore, the suppression of B and T lymphocytes alone may not stop the immune response. Some preclinical studies showed that the blockade of signaling pathways significantly increased the survival rate and improved the cardiac function of patients with ICI-induced cytotoxicity (Supplementary Table S1). Therefore, we hypothesize that an alternative therapy combined with these immunosuppressive agents and blockade inhibitors will prove beneficial; however, this remains to be confirmed in clinical trials.
In this review, we reviewed 71 patients with cancer (40 male and 31 female) treated with anti-PD-1/PD-L1 and anti-CTLA-4 inhibitors. These patients were diagnosed with metastatic melanoma (n = 22), lung cancer (n = 10), thymoma (9), renal cell carcinoma (n = 6), esophagogastric carcinoma (n = 3), breast cancer (n = 2), gastric cancer (n = 2), myelodysplastic syndrome (n = 2), prostate cancer (n = 2), squamous cell cancer (n = 2), urothelial cancer (n = 2), advanced endometrial cancer (n = 1), chordoma (n = 1), colonic carcinoma (n = 1), glioblastoma (n = 1), laryngeal cancer (n = 1), lymphoma (n = 1), mesothelioma (n = 1), myeloma (n = 1), and ovarian adenocarcinoma (n = 1). The common symptoms included chest pain, chest tightness, dyspnea (shortness of breath), fatigue, muscle weakness, and occasional fever (Yamaguchi et al., 2018; Konstantina et al., 2019; Balanescu et al., 2020; Guo et al., 2020; Ono et al., 2022; Yang et al., 2022). Two patients even presented with a rash in the extremities or chest (Konstantina et al., 2019; Cao et al., 2021). All the patients were diagnosed with myocarditis via multiple techniques, including cardiac biomarkers in serum, ECG, echocardiography, cardiac magnetic resonance, or cardiac computed tomography (Supplementary Table S1).
After diagnosis, ICIs were stopped and immunosuppressive agents were immediately applied, including high-dose methylprednisolone, prednisone, infliximab, immunoglobulin, ATG, mycophenolate mofetil, alemtuzumab, abatacept, plasmapheresis, tocilizumab, rituximab, tacrolimus, or methotrexate (Supplementary Table S1). Of 41 patients treated initially with methylprednisolone, 25 (61%) were alive after subsequent immunosuppressive therapy (Supplementary Table S1), whereas of 16 patients who received methylprednisolone treatment alone, only 7 (43%) were alive with a decline in cardiac biomarkers and improved cardiac function (Xing et al., 2020; Bai et al., 2021; Bi et al., 2021; Hu et al., 2021; Lin et al., 2022; Su et al., 2022; Yang et al., 2022). Thus, it appears that a combination of multiple drugs can promote overall survival, suggesting that when the effects of methylprednisolone treatment alone are unsatisfactory, outcomes are improved by adding other immunosuppressive agents. Furthermore, six patients were treated initially with prednisone, followed by other immunosuppressive agents; three (50%) were alive after immunosuppressive therapy (Nasr et al., 2018; Yamaguchi et al., 2018; Agrawal et al., 2019) and two patients were alive after receiving prednisone alone (Guo et al., 2020; Miyauchi et al., 2021).
Some other organ systems are affected by ICIs, including the gastrointestinal tract, skin, lung, and liver (Weber et al., 2017), with reports of patient deaths caused by respiratory failure (Arora et al., 2020; Komatsu et al., 2021; Saishu et al., 2022), pulmonary encephalopathy (Chen et al., 2021b), infection (Konstantina et al., 2019; Padegimas et al., 2019), and even multiorgan failure (Chen et al., 2018; Agrawal et al., 2019). Therefore, the effects of ICIs on the function of other organs should be considered.
Conclusion
Herein, we reviewed the case reports of ICI-induced myocarditis, which is a serious adverse event with a high fatality rate. Although one retrospective study demonstrated that the 1-year survival was significantly higher in patients with cancer who received ICI-based immunosuppressive therapy (Joseph et al., 2020), this strategy remains to be optimized.
The limitations of our review should be noted. First, the majority of the clinical information was based on case reports and there is a lack of large-scale, multicenter, randomized, and controlled clinical trials on the use of immunosuppressive agents to treat ICIs-induced cardiotoxicity. Second, there was wide variation in the characteristics of the referred cases, including age, tumor type, and stage. In addition, follow-up after discharge was not performed in some cases, whereas others were followed-up for several months. Third, a large proportion of the published cases focused on suppressing the immune response in the myocardium, although the function of other organs was also affected. Finally, there were no tumor controls without ICI therapy in these published cases. To overcome these limitations, we believe that future research studies should be focused on at least two aspects. First, large-scale, multicenter, and randomized controlled trials are urgently required to investigate the efficacy of different immunosuppressive agents on different tumor types. Second, products derived from Chinese herbs offer several outstanding advantages that indicate their promise as a therapeutic option. Since, natural products usually have few side effects compared with synthetic drugs. In addition, natural products also have multiple pharmacological activities, providing enhanced beneficial effects owing to the involvement of multiple signaling pathways in the process of ICI-induced myocarditis.
Author contributions
YX and LX conceptualized the research work. YW and LX performed extensive literature search. YW wrote the manuscript draft. LX critically revised the manuscript. All authors contributed to the article and approved the submitted version.
Funding
This work was supported by the Medical and Health Science and Technology Program of Zhejiang Province (2022RC227), the Construction Fund of Key Medical Disciplines of Hangzhou (OO20200121) and the Medical and Health Science and Technology Project of Hangzhou City (Z20200135).
Conflict of interest
The authors declare that the research was conducted in the absence of any commercial or financial relationships that could be construed as a potential conflict of interest.
Publisher’s note
All claims expressed in this article are solely those of the authors and do not necessarily represent those of their affiliated organizations, or those of the publisher, the editors and the reviewers. Any product that may be evaluated in this article, or claim that may be made by its manufacturer, is not guaranteed or endorsed by the publisher.
Supplementary material
The Supplementary Material for this article can be found online at: https://www.frontiersin.org/articles/10.3389/fphar.2023.1161243/full#supplementary-material
References
Aboelella, N. S., Brandle, C., Kim, T., Ding, Z. C., and Zhou, G. (2021). Oxidative stress in the tumor microenvironment and its relevance to cancer immunotherapy. Cancers (Basel) 13 (5), 986. doi:10.3390/cancers13050986
Agrawal, N., Khunger, A., Vachhani, P., Colvin, T. A., Hattoum, A., Spangenthal, E., et al. (2019). Cardiac toxicity associated with immune checkpoint inhibitors: Case series and review of the literature. Case Rep. Oncol. 12 (1), 260–276. doi:10.1159/000498985
Arangalage, D., Pavon, A. G., Ozdemir, B. C., Michielin, O., Schwitter, J., and Monney, P. (2021). Acute cardiac manifestations under immune checkpoint inhibitors-beware of the obvious: A case report. Eur. Heart J. Case Rep. 5 (8), ytab262. doi:10.1093/ehjcr/ytab262
Arora, P., Talamo, L., Dillon, P., Gentzler, R. D., Millard, T., Salerno, M., et al. (2020). Severe combined cardiac and neuromuscular toxicity from immune checkpoint blockade: An institutional case series. Cardiooncology 6, 21. doi:10.1186/s40959-020-00076-6
Bai, J., Li, D., Yang, P., Xu, K., Wang, Y., Li, Q., et al. (2021). Camrelizumab-related myocarditis and myositis with myasthenia gravis: A case report and literature review. Front. Oncol. 11, 778185. doi:10.3389/fonc.2021.778185
Balanescu, D. V., Donisan, T., Palaskas, N., Lopez-Mattei, J., Kim, P. Y., Buja, L. M., et al. (2020). Immunomodulatory treatment of immune checkpoint inhibitor-induced myocarditis: Pathway toward precision-based therapy. Cardiovasc Pathol. 47, 107211. doi:10.1016/j.carpath.2020.107211
Baldeviano, G. C., Barin, J. G., Talor, M. V., Srinivasan, S., Bedja, D., Zheng, D., et al. (2010). Interleukin-17A is dispensable for myocarditis but essential for the progression to dilated cardiomyopathy. Circ. Res. 106 (10), 1646–1655. doi:10.1161/CIRCRESAHA.109.213157
Bi, H., Ren, D., Wang, Q., Ding, X., and Wang, H. (2021). Immune checkpoint inhibitor-induced myocarditis in lung cancer patients: A case report of sintilimab-induced myocarditis and a review of the literature. Ann. Palliat. Med. 10 (1), 793–802. doi:10.21037/apm-20-2449
Brahmer, J. R., Lacchetti, C., Schneider, B. J., Atkins, M. B., Brassil, K. J., Caterino, J. M., et al. (2018). Management of immune-related adverse events in patients treated with immune checkpoint inhibitor therapy: American society of clinical oncology clinical practice guideline. J. Clin. Oncol. 36 (17), 1714–1768. doi:10.1200/JCO.2017.77.6385
Buono, C., Binder, C. J., Stavrakis, G., Witztum, J. L., Glimcher, L. H., and Lichtman, A. H. (2005). T-bet deficiency reduces atherosclerosis and alters plaque antigen-specific immune responses. Proc. Natl. Acad. Sci. U. S. A. 102 (5), 1596–1601. doi:10.1073/pnas.0409015102
Cao, J., Li, Q., Zhi, X., Yang, F., Zhu, W., Zhou, T., et al. (2021). Pembrolizumab-induced autoimmune stevens-johnson syndrome/toxic epidermal necrolysis with myositis and myocarditis in a patient with esophagogastric junction carcinoma: A case report. Transl. Cancer Res. 10 (8), 3870–3876. doi:10.21037/tcr-21-470
Celada, L. J., Kropski, J. A., Herazo-Maya, J. D., Luo, W., Creecy, A., Abad, A. T., et al. (2018). PD-1 up-regulation on CD4+ T cells promotes pulmonary fibrosis through STAT3-mediated IL-17A and TGF-β1 production. Sci. Transl. Med. 10 (460), eaar8356. doi:10.1126/scitranslmed.aar8356
Chen, D. H., Tyebally, S., Malloupas, M., Roylance, R., Spurrell, E., Raja, F., et al. (2021a). Cardiovascular disease amongst women treated for breast cancer: Traditional cytotoxic chemotherapy, targeted therapy, and radiation therapy. Curr. Cardiol. Rep. 23 (3), 16. doi:10.1007/s11886-021-01446-x
Chen, Q., Huang, D. S., Zhang, L. W., Li, Y. Q., Wang, H. W., and Liu, H. B. (2018). Fatal myocarditis and rhabdomyolysis induced by nivolumab during the treatment of type B3 thymoma. Clin. Toxicol. (Phila) 56 (7), 667–671. doi:10.1080/15563650.2017.1401079
Chen, Y., Jia, Y., Liu, Q., Shen, Y., Zhu, H., Dong, X., et al. (2021b). Myocarditis related to immune checkpoint inhibitors treatment: Two case reports and literature review. Ann. Palliat. Med. 10 (7), 8512–8517. doi:10.21037/apm-20-2620
Chen, Y., Liu, Y., Wang, Y., Chen, X., Wang, C., Chen, X., et al. (2022). Prevotellaceae produces butyrate to alleviate PD-1/PD-L1 inhibitor-related cardiotoxicity via PPARα-CYP4X1 axis in colonic macrophages. J. Exp. Clin. Cancer Res. 41 (1), 1. doi:10.1186/s13046-021-02201-4
Chen, Z., Wang, M., Gao, S., Guo, H., Wang, G., and Zhou, G. (2021c). Cardiotoxicity of anti-PD-L1 antibody and the effect of levothyroxine in attenuating the related mortality in mice. Zhongguo Fei Ai Za Zhi 24 (6), 394–403. doi:10.3779/j.issn.1009-3419.2021.102.23
Chistiakov, D. A., Orekhov, A. N., and Bobryshev, Y. V. (2017). The impact of FOXO-1 to cardiac pathology in diabetes mellitus and diabetes-related metabolic abnormalities. Int. J. Cardiol. 245, 236–244. doi:10.1016/j.ijcard.2017.07.096
Chung, E. S., Packer, M., Lo, K. H., Fasanmade, A. A., Willerson, J. T., and Anti, T. N. F. T. A. C. H. F. I. (2003). Randomized, double-blind, placebo-controlled, pilot trial of infliximab, a chimeric monoclonal antibody to tumor necrosis factor-alpha, in patients with moderate-to-severe heart failure: Results of the anti-TNF therapy against congestive heart failure (ATTACH) trial. Circulation 107 (25), 3133–3140. doi:10.1161/01.CIR.0000077913.60364.D2
Doms, J., Prior, J. O., Peters, S., and Obeid, M. (2020). Tocilizumab for refractory severe immune checkpoint inhibitor-associated myocarditis. Ann. Oncol. 31 (9), 1273–1275. doi:10.1016/j.annonc.2020.05.005
Dulos, J., Carven, G. J., van Boxtel, S. J., Evers, S., Driessen-Engels, L. J., Hobo, W., et al. (2012). PD-1 blockade augments Th1 and Th17 and suppresses Th2 responses in peripheral blood from patients with prostate and advanced melanoma cancer. J. Immunother. 35 (2), 169–178. doi:10.1097/CJI.0b013e318247a4e7
Esfahani, K., Buhlaiga, N., Thebault, P., Lapointe, R., Johnson, N. A., and Miller, W. H. (2019). Alemtuzumab for immune-related myocarditis due to PD-1 therapy. N. Engl. J. Med. 380 (24), 2375–2376. doi:10.1056/NEJMc1903064
Fazel, M., and Jedlowski, P. M. (2019). Severe myositis, myocarditis, and myasthenia gravis with elevated anti-striated muscle antibody following single dose of ipilimumab-nivolumab therapy in a patient with metastatic melanoma. Case Rep. Immunol. 2019, 2539493. doi:10.1155/2019/2539493
Frigeri, M., Meyer, P., Banfi, C., Giraud, R., Hachulla, A. L., Spoerl, D., et al. (2018). Immune checkpoint inhibitor-associated myocarditis: A new challenge for cardiologists. Can. J. Cardiol. 34 (1), e1–e92. doi:10.1016/j.cjca.2017.09.025
Fu, G., Cao, Y., Lu, J., Li, J., Liu, L., Wang, H., et al. (2013). Programmed cell death-1 deficiency results in atrial remodeling in C57BL/6 mice. Int. J. Mol. Med. 31 (2), 423–429. doi:10.3892/ijmm.2012.1218
Gallegos, C., Rottmann, D., Nguyen, V. Q., and Baldassarre, L. A. (2019). Myocarditis with checkpoint inhibitor immunotherapy: Case report of late gadolinium enhancement on cardiac magnetic resonance with pathology correlate. Eur. Heart J. Case Rep. 3 (1), yty149. doi:10.1093/ehjcr/yty149
Gergely, T. G., Kucsera, D., Toth, V. E., Kovacs, T., Sayour, N. V., Drobni, Z. D., et al. (2022). Characterization of immune checkpoint inhibitor-induced cardiotoxicity reveals interleukin-17A as a driver of cardiac dysfunction after anti-PD-1 treatment. Br. J. Pharmacol. 180, 740–761. doi:10.1111/bph.15984
Gong, J., Chehrazi-Raffle, A., Reddi, S., and Salgia, R. (2018). Development of PD-1 and PD-L1 inhibitors as a form of cancer immunotherapy: A comprehensive review of registration trials and future considerations. J. Immunother. Cancer 6 (1), 8. doi:10.1186/s40425-018-0316-z
Grievink, H. W., Smit, V., Verwilligen, R. A. F., Bernabe Kleijn, M. N. A., Smeets, D., Binder, C. J., et al. (2021). Stimulation of the PD-1 pathway decreases atherosclerotic lesion development in ldlr deficient mice. Front. Cardiovasc Med. 8, 740531. doi:10.3389/fcvm.2021.740531
Guo, C. W., Alexander, M., Dib, Y., Lau, P. K. H., Weppler, A. M., Au-Yeung, G., et al. (2020). A closer look at immune-mediated myocarditis in the era of combined checkpoint blockade and targeted therapies. Eur. J. Cancer 124, 15–24. doi:10.1016/j.ejca.2019.09.009
Han, B., Jiang, H., Liu, Z., Zhang, Y., Zhao, L., Lu, K., et al. (2012). CTLA4-Ig relieves inflammation in murine models of coxsackievirus B3-induced myocarditis. Can. J. Cardiol. 28 (2), 239–244. doi:10.1016/j.cjca.2011.11.014
Heinzerling, L., Ott, P. A., Hodi, F. S., Husain, A. N., Tajmir-Riahi, A., Tawbi, H., et al. (2016). Cardiotoxicity associated with CTLA4 and PD1 blocking immunotherapy. J. Immunother. Cancer 4, 50. doi:10.1186/s40425-016-0152-y
Hu, J. R., Florido, R., Lipson, E. J., Naidoo, J., Ardehali, R., Tocchetti, C. G., et al. (2019). Cardiovascular toxicities associated with immune checkpoint inhibitors. Cardiovasc Res. 115 (5), 854–868. doi:10.1093/cvr/cvz026
Hu, X., Wei, Y., and Shuai, X. (2021). Case report: Glucocorticoid effect observation in a ureteral urothelial cancer patient with ICI-associated myocarditis and multiple organ injuries. Front. Immunol. 12, 799077. doi:10.3389/fimmu.2021.799077
Imai, R., Ono, M., Nishimura, N., Suzuki, K., Komiyama, N., and Tamura, T. (2019). Fulminant myocarditis caused by an immune checkpoint inhibitor: A case report with pathologic findings. J. Thorac. Oncol. 14 (2), e36–e38. doi:10.1016/j.jtho.2018.10.156
Itzhaki Ben Zadok, O., Ben-Avraham, B., Nohria, A., Orvin, K., Nassar, M., Iakobishvili, Z., et al. (2019). Immune-checkpoint inhibitor-induced fulminant myocarditis and cardiogenic shock. JACC CardioOncol 1 (1), 141–144. doi:10.1016/j.jaccao.2019.07.004
Jain, V., Mohebtash, M., Rodrigo, M. E., Ruiz, G., Atkins, M. B., and Barac, A. (2018). Autoimmune myocarditis caused by immune checkpoint inhibitors treated with antithymocyte globulin. J. Immunother. 41 (7), 332–335. doi:10.1097/CJI.0000000000000239
Jespersen, M. S., Fano, S., Stenor, C., and Moller, A. K. (2021). A case report of immune checkpoint inhibitor-related steroid-refractory myocarditis and myasthenia gravis-like myositis treated with abatacept and mycophenolate mofetil. Eur. Heart J. Case Rep. 5 (11), ytab342. doi:10.1093/ehjcr/ytab342
Ji, C., Roy, M. D., Golas, J., Vitsky, A., Ram, S., Kumpf, S. W., et al. (2019). Myocarditis in cynomolgus monkeys following treatment with immune checkpoint inhibitors. Clin. Cancer Res. 25 (15), 4735–4748. doi:10.1158/1078-0432.CCR-18-4083
Joseph, A., Simonaggio, A., Stoclin, A., Vieillard-Baron, A., Geri, G., Oudard, S., et al. (2020). Immune-related adverse events: A retrospective look into the future of oncology in the intensive care unit. Ann. Intensive Care 10 (1), 143. doi:10.1186/s13613-020-00761-w
Kagawa, K., Sato, S., Koyama, K., Imakura, T., Murakami, K., Yamashita, Y., et al. (2022). The lymphocyte-specific protein tyrosine kinase-specific inhibitor A-770041 attenuates lung fibrosis via the suppression of TGF-beta production in regulatory T-cells. PLoS One 17 (10), e0275987. doi:10.1371/journal.pone.0275987
Kamada, T., Togashi, Y., Tay, C., Ha, D., Sasaki, A., Nakamura, Y., et al. (2019). PD-1(+) regulatory T cells amplified by PD-1 blockade promote hyperprogression of cancer. Proc. Natl. Acad. Sci. U. S. A. 116 (20), 9999–10008. doi:10.1073/pnas.1822001116
Kennedy, O. J., Kicinski, M., Valpione, S., Gandini, S., Suciu, S., Blank, C. U., et al. (2022). Prognostic and predictive value of beta-blockers in the EORTC 1325/KEYNOTE-054 phase III trial of pembrolizumab versus placebo in resected high-risk stage III melanoma. Eur. J. Cancer 165, 97–112. doi:10.1016/j.ejca.2022.01.017
Khan, M. A., Shamma, T., Altuhami, A., Ahmed, H. A., Assiri, A. M., and Broering, D. C. (2022). CTLA4-Ig mediated immunosuppression favors immunotolerance and restores graft in mouse airway transplants. Pharmacol. Res. 178, 106147. doi:10.1016/j.phrs.2022.106147
Komatsu, M., Hirai, M., Kobayashi, K., Hashidate, H., Fukumoto, J., Sato, A., et al. (2021). A rare case of nivolumab-related myasthenia gravis and myocarditis in a patient with metastatic gastric cancer. BMC Gastroenterol. 21 (1), 333. doi:10.1186/s12876-021-01904-4
Konstantina, T., Konstantinos, R., Anastasios, K., Anastasia, M., Eleni, L., Ioannis, S., et al. (2019). Fatal adverse events in two thymoma patients treated with anti-PD-1 immune check point inhibitor and literature review. Lung Cancer 135, 29–32. doi:10.1016/j.lungcan.2019.06.015
Kostakou, P. M., Kouris, N. T., Kostopoulos, V. S., Damaskos, D. S., and Olympios, C. D. (2019). Cardio-oncology: A new and developing sector of research and therapy in the field of cardiology. Heart Fail Rev. 24 (1), 91–100. doi:10.1007/s10741-018-9731-y
Lichtman, A. H. (2013). The heart of the matter: Protection of the myocardium from T cells. J. Autoimmun. 45, 90–96. doi:10.1016/j.jaut.2013.05.004
Lin, Y., Yuan, X., and Chen, L. (2022). Immune myocarditis related to sintilimab treatment in a patient with advanced lung adenocarcinoma: A case report. Front. Cardiovasc Med. 9, 955527. doi:10.3389/fcvm.2022.955527
Liu, F., Liu, J., Weng, D., Chen, Y., Song, L., He, Q., et al. (2010). CD4+CD25+Foxp3+ regulatory T cells depletion may attenuate the development of silica-induced lung fibrosis in mice. PLoS One 5 (11), e15404. doi:10.1371/journal.pone.0015404
Liu, S., Chan, J., Brinc, D., Gandhi, S., Izenberg, A., Delgado, D., et al. (2020). Immune checkpoint inhibitor-associated myocarditis with persistent troponin elevation despite abatacept and prolonged immunosuppression. JACC CardioOncol 2 (5), 800–804. doi:10.1016/j.jaccao.2020.10.013
Liu, X., Weng, X., Xiao, W., Xu, X., Chen, Y., and Chen, P. (2021). Pharmacological and genetic inhibition of PD-1 demonstrate an important role of PD-1 in ischemia-induced skeletal muscle inflammation, oxidative stress, and angiogenesis. Front. Immunol. 12, 586429. doi:10.3389/fimmu.2021.586429
Luo, Y. B., Tang, W., Zeng, Q., Duan, W., Li, S., Yang, X., et al. (2021). Case report: The neuromusclar triad of immune checkpoint inhibitors: A case report of myositis, myocarditis, and myasthenia gravis overlap following toripalimab treatment. Front. Cardiovasc Med. 8, 714460. doi:10.3389/fcvm.2021.714460
Lyon, A. R., Lopez-Fernandez, T., Couch, L. S., Asteggiano, R., Aznar, M. C., Bergler-Klein, J., et al. (2022). 2022 ESC Guidelines on cardio-oncology developed in collaboration with the European hematology association (EHA), the European society for therapeutic radiology and oncology (ESTRO) and the international cardio-oncology society (IC-OS). Eur. Heart J. 43 (41), 4229–4361. doi:10.1093/eurheartj/ehac244
Lyon, A. R., Yousaf, N., Battisti, N. M. L., Moslehi, J., and Larkin, J. (2018). Immune checkpoint inhibitors and cardiovascular toxicity. Lancet Oncol. 19 (9), e447–e458. doi:10.1016/S1470-2045(18)30457-1
Mahmood, S. S., Chen, C. L., Shapnik, N., Krishnan, U., Singh, H. S., and Makker, V. (2018a). Myocarditis with tremelimumab plus durvalumab combination therapy for endometrial cancer: A case report. Gynecol. Oncol. Rep. 25, 74–77. doi:10.1016/j.gore.2018.05.014
Mahmood, S. S., Fradley, M. G., Cohen, J. V., Nohria, A., Reynolds, K. L., Heinzerling, L. M., et al. (2018b). Myocarditis in patients treated with immune checkpoint inhibitors. J. Am. Coll. Cardiol. 71 (16), 1755–1764. doi:10.1016/j.jacc.2018.02.037
Martin, C. J., Datta, A., Littlefield, C., Kalra, A., Chapron, C., Wawersik, S., et al. (2020). Selective inhibition of TGFβ1 activation overcomes primary resistance to checkpoint blockade therapy by altering tumor immune landscape. Sci. Transl. Med. 12 (536), eaay8456. doi:10.1126/scitranslmed.aay8456
Martinez-Calle, N., Rodriguez-Otero, P., Villar, S., Mejias, L., Melero, I., Prosper, F., et al. (2018). Anti-PD1 associated fulminant myocarditis after a single pembrolizumab dose: The role of occult pre-existing autoimmunity. Haematologica 103 (7), e318–e321. doi:10.3324/haematol.2017.185777
McDowall, L. M., Fernando, S. L., Ange, N., Yun, J., and Chia, K. K. M. (2019). Immune checkpoint inhibitor-mediated myocarditis and ventricular tachycardia storm. Hear. Case Rep. 5 (10), 497–500. doi:10.1016/j.hrcr.2019.06.006
Meng, X. M., Nikolic-Paterson, D. J., and Lan, H. Y. (2016). TGF-Β: The master regulator of fibrosis. Nat. Rev. Nephrol. 12 (6), 325–338. doi:10.1038/nrneph.2016.48
Michel, L., Helfrich, I., Hendgen-Cotta, U. B., Mincu, R. I., Korste, S., Mrotzek, S. M., et al. (2022). Targeting early stages of cardiotoxicity from anti-PD1 immune checkpoint inhibitor therapy. Eur. Heart J. 43 (4), 316–329. doi:10.1093/eurheartj/ehab430
Mir, H., Alhussein, M., Alrashidi, S., Alzayer, H., Alshatti, A., Valettas, N., et al. (2018). Cardiac complications associated with checkpoint inhibition: A systematic review of the literature in an important emerging area. Can. J. Cardiol. 34 (8), 1059–1068. doi:10.1016/j.cjca.2018.03.012
Miyauchi, Y., Naito, H., Tsunemori, H., Tani, R., Hasui, Y., Miyake, Y., et al. (2021). Myocarditis as an immune-related adverse event following treatment with ipilimumab and nivolumab combination therapy for metastatic renal cell carcinoma: A case report. J. Med. Case Rep. 15 (1), 508. doi:10.1186/s13256-021-03097-6
Monge, C., Maeng, H., Brofferio, A., Apolo, A. B., Sathya, B., Arai, A. E., et al. (2018). Myocarditis in a patient treated with nivolumab and PROSTVAC: A case report. J. Immunother. Cancer 6 (1), 150. doi:10.1186/s40425-018-0473-0
Moslehi, J. J., Salem, J. E., Sosman, J. A., Lebrun-Vignes, B., and Johnson, D. B. (2018). Increased reporting of fatal immune checkpoint inhibitor-associated myocarditis. Lancet 391 (10124), 933. doi:10.1016/S0140-6736(18)30533-6
Nasr, F., El Rassy, E., Maalouf, G., Azar, C., Haddad, F., Helou, J., et al. (2018). Severe ophthalmoplegia and myocarditis following the administration of pembrolizumab. Eur. J. Cancer 91, 171–173. doi:10.1016/j.ejca.2017.11.026
Nguyen, L. S., Bretagne, M., Arrondeau, J., Zahr, N., Ederhy, S., Abbar, B., et al. (2022). Reversal of immune-checkpoint inhibitor fulminant myocarditis using personalized-dose-adjusted abatacept and ruxolitinib: Proof of concept. J. Immunother. Cancer 10 (4), e004699. doi:10.1136/jitc-2022-004699
Niu, L. T., Wang, Y. Q., Wong, C. C. L., Gao, S. X., Mo, X. D., and Huang, X. J. (2021). Targeting IFN-gamma-inducible lysosomal thiol reductase overcomes chemoresistance in AML through regulating the ROS-mediated mitochondrial damage. Transl. Oncol. 14 (9), 101159. doi:10.1016/j.tranon.2021.101159
Norwood, T. G., Westbrook, B. C., Johnson, D. B., Litovsky, S. H., Terry, N. L., McKee, S. B., et al. (2017). Smoldering myocarditis following immune checkpoint blockade. J. Immunother. Cancer 5 (1), 91. doi:10.1186/s40425-017-0296-4
Ono, R., Iwai, Y., Yamazaki, T., Takahashi, H., Hori, Y., Fukushima, K., et al. (2022). Nivolumab-induced myositis and myocarditis with positive anti-titin antibody and anti-voltage-gated potassium channel Kv1.4 antibody. Intern Med. 61 (19), 2973–2979. doi:10.2169/internalmedicine.8772-21
Padegimas, A., Agarwal, P., Fleitman, J., Carver, J., Rao, S., Mather, P., et al. (2019). Case series of ventricular tachycardia and myocarditis from programmed cell-death protein-1 inhibitor treated with infliximab. JACC Clin. Electrophysiol. 5 (8), 989–992. doi:10.1016/j.jacep.2019.05.001
Patel, R. P., Parikh, R., Gunturu, K. S., Tariq, R. Z., Dani, S. S., Ganatra, S., et al. (2021). Cardiotoxicity of immune checkpoint inhibitors. Curr. Oncol. Rep. 23 (7), 79. doi:10.1007/s11912-021-01070-6
Poels, K., van Leent, M. M. T., Reiche, M. E., Kusters, P. J. H., Huveneers, S., de Winther, M. P. J., et al. (2020). Antibody-mediated inhibition of CTLA4 aggravates atherosclerotic plaque inflammation and progression in hyperlipidemic mice. Cells 9 (9), 1987. doi:10.3390/cells9091987
Quagliariello, V., Passariello, M., Coppola, C., Rea, D., Barbieri, A., Scherillo, M., et al. (2019). Cardiotoxicity and pro-inflammatory effects of the immune checkpoint inhibitor Pembrolizumab associated to Trastuzumab. Int. J. Cardiol. 292, 171–179. doi:10.1016/j.ijcard.2019.05.028
Quagliariello, V., Passariello, M., Di Mauro, A., Cipullo, C., Paccone, A., Barbieri, A., et al. (2022). Immune checkpoint inhibitor therapy increases systemic SDF-1, cardiac DAMPs Fibronectin-EDA, S100/Calgranulin, galectine-3, and NLRP3-MyD88-chemokine pathways. Front. Cardiovasc Med. 9, 930797. doi:10.3389/fcvm.2022.930797
Reddy, N., Moudgil, R., Lopez-Mattei, J. C., Karimzad, K., Mouhayar, E. N., Somaiah, N., et al. (2017). Progressive and reversible conduction disease with checkpoint inhibitors. Can. J. Cardiol. 33 (10), e13. doi:10.1016/j.cjca.2017.05.026
Ribas, A., and Wolchok, J. D. (2018). Cancer immunotherapy using checkpoint blockade. Science 359 (6382), 1350–1355. doi:10.1126/science.aar4060
Saishu, Y., Yoshida, T., Seino, Y., and Nomura, T. (2022). Nivolumab-related myasthenia gravis with myositis requiring prolonged mechanical ventilation: A case report. J. Med. Case Rep. 16 (1), 61. doi:10.1186/s13256-022-03286-x
Salem, J. E., Allenbach, Y., Vozy, A., Brechot, N., Johnson, D. B., Moslehi, J. J., et al. (2019). Abatacept for severe immune checkpoint inhibitor-associated myocarditis. N. Engl. J. Med. 380 (24), 2377–2379. doi:10.1056/NEJMc1901677
Seko, Y., Yagita, H., Okumura, K., Azuma, M., and Nagai, R. (2007). Roles of programmed death-1 (PD-1)/PD-1 ligands pathway in the development of murine acute myocarditis caused by coxsackievirus B3. Cardiovasc Res. 75 (1), 158–167. doi:10.1016/j.cardiores.2007.03.012
Shioji, K., Kishimoto, C., and Sasayama, S. (2001). Fc receptor-mediated inhibitory effect of immunoglobulin therapy on autoimmune giant cell myocarditis: Concomitant suppression of the expression of dendritic cells. Circ. Res. 89 (6), 540–546. doi:10.1161/hh1801.096263
Su, L., Liu, C., Wu, W., Cui, Y., Wu, M., and Chen, H. (2022). Successful therapy for myocarditis concomitant with complete heart block after pembrolizumab treatment for head and neck squamous cell carcinoma: A case report with literature review. Front. Cardiovasc Med. 9, 898756. doi:10.3389/fcvm.2022.898756
Sun, Y., Li, L., Wu, Y., and Yang, K. (2020). PD-1/PD-L1 in cardiovascular disease. Clin. Chim. Acta 505, 26–30. doi:10.1016/j.cca.2020.02.019
Szuchan, C., Elson, L., Alley, E., Leung, K., Camargo, A. L., Elimimian, E., et al. (2020). Checkpoint inhibitor-induced myocarditis and myasthenia gravis in a recurrent/metastatic thymic carcinoma patient: A case report. Eur. Heart J. Case Rep. 4 (3), 1–8. doi:10.1093/ehjcr/ytaa051
Tay, R. Y., Blackley, E., McLean, C., Moore, M., Bergin, P., Gill, S., et al. (2017). Successful use of equine anti-thymocyte globulin (ATGAM) for fulminant myocarditis secondary to nivolumab therapy. Br. J. Cancer 117 (7), 921–924. doi:10.1038/bjc.2017.253
Tay, W. T., Fang, Y. H., Beh, S. T., Liu, Y. W., Hsu, L. W., Yen, C. J., et al. (2020). Programmed cell death-1: Programmed cell death-ligand 1 interaction protects human cardiomyocytes against T-cell mediated inflammation and apoptosis response in vitro. Int. J. Mol. Sci. 21 (7), 2399. doi:10.3390/ijms21072399
Terabe, M., Robertson, F. C., Clark, K., De Ravin, E., Bloom, A., Venzon, D. J., et al. (2017). Blockade of only TGF-beta 1 and 2 is sufficient to enhance the efficacy of vaccine and PD-1 checkpoint blockade immunotherapy. Oncoimmunology 6 (5), e1308616. doi:10.1080/2162402X.2017.1308616
Touat, M., Maisonobe, T., Knauss, S., Ben Hadj Salem, O., Hervier, B., Aure, K., et al. (2018). Immune checkpoint inhibitor-related myositis and myocarditis in patients with cancer. Neurology 91 (10), e985–e994. doi:10.1212/WNL.0000000000006124
Valdez-Ortiz, R., Bestard, O., Llaudo, I., Franquesa, M., Cerezo, G., Torras, J., et al. (2015). Induction of suppressive allogeneic regulatory T cells via rabbit antithymocyte polyclonal globulin during homeostatic proliferation in rat kidney transplantation. Transpl. Int. 28 (1), 108–119. doi:10.1111/tri.12448
Varricchi, G., Galdiero, M. R., Marone, G., Criscuolo, G., Triassi, M., Bonaduce, D., et al. (2017). Cardiotoxicity of immune checkpoint inhibitors. ESMO Open 2 (4), e000247. doi:10.1136/esmoopen-2017-000247
von Itzstein, M. S., Khan, S., Popat, V., Lu, R., Khan, S. A., Fattah, F. J., et al. (2020). Statin intolerance, anti-HMGCR antibodies, and immune checkpoint inhibitor-associated myositis: A "Two-Hit" autoimmune toxicity or clinical predisposition? Oncologist 25 (8), e1242–e1245. doi:10.1634/theoncologist.2019-0911
Wang, F., Liu, Y., Xu, W., Zhang, C., Lv, J., and Ma, S. (2021). Fulminant myocarditis induced by immune checkpoint inhibitor nivolumab: A case report and review of the literature. J. Med. Case Rep. 15 (1), 336. doi:10.1186/s13256-021-02934-y
Wang, Z., and Tan, F. (2020). The blockade of PD-1/PD-L1 pathway promotes the apoptosis of CD19(+) CD25(+) Bregs and suppresses the secretion of IL-10 in patients with allergic rhinitis. Scand. J. Immunol. 91 (2), e12836. doi:10.1111/sji.12836
Weber, J. S., Hodi, F. S., Wolchok, J. D., Topalian, S. L., Schadendorf, D., Larkin, J., et al. (2017). Safety profile of nivolumab monotherapy: A pooled analysis of patients with advanced melanoma. J. Clin. Oncol. 35 (7), 785–792. doi:10.1200/JCO.2015.66.1389
Wei, S. C., Meijers, W. C., Axelrod, M. L., Anang, N. A. S., Screever, E. M., Wescott, E. C., et al. (2021). A genetic mouse model recapitulates immune checkpoint inhibitor-associated myocarditis and supports a mechanism-based therapeutic intervention. Cancer Discov. 11 (3), 614–625. doi:10.1158/2159-8290.CD-20-0856
Wu, Q., Qian, W., Sun, X., and Jiang, S. (2022). Small-molecule inhibitors, immune checkpoint inhibitors, and more: FDA-approved novel therapeutic drugs for solid tumors from 1991 to 2021. J. Hematol. Oncol. 15 (1), 143. doi:10.1186/s13045-022-01362-9
Xia, W., Chen, H., Chen, D., Ye, Y., Xie, C., and Hou, M. (2020a). PD-1 inhibitor inducing exosomal miR-34a-5p expression mediates the cross talk between cardiomyocyte and macrophage in immune checkpoint inhibitor-related cardiac dysfunction. J. Immunother. Cancer 8 (2), e001293. doi:10.1136/jitc-2020-001293
Xia, W., Zou, C., Chen, H., Xie, C., and Hou, M. (2020b). Immune checkpoint inhibitor induces cardiac injury through polarizing macrophages via modulating microRNA-34a/Kruppel-like factor 4 signaling. Cell Death Dis. 11 (7), 575. doi:10.1038/s41419-020-02778-2
Xing, Q., Zhang, Z. W., Lin, Q. H., Shen, L. H., Wang, P. M., Zhang, S., et al. (2020). Myositis-myasthenia gravis overlap syndrome complicated with myasthenia crisis and myocarditis associated with anti-programmed cell death-1 (sintilimab) therapy for lung adenocarcinoma. Ann. Transl. Med. 8 (5), 250. doi:10.21037/atm.2020.01.79
Yamaguchi, S., Morimoto, R., Okumura, T., Yamashita, Y., Haga, T., Kuwayama, T., et al. (2018). Late-onset fulminant myocarditis with immune checkpoint inhibitor nivolumab. Can. J. Cardiol. 34 (6), e1. doi:10.1016/j.cjca.2018.03.007
Yanase, T., Moritoki, Y., Kondo, H., Ueyama, D., Akita, H., and Yasui, T. (2021). Myocarditis and myasthenia gravis by combined nivolumab and ipilimumab immunotherapy for renal cell carcinoma: A case report of successful management. Urol. Case Rep. 34, 101508. doi:10.1016/j.eucr.2020.101508
Yang, Y., Wu, Q., Chen, L., Qian, K., and Xu, X. (2022). Severe immune-related hepatitis and myocarditis caused by PD-1 inhibitors in the treatment of triple-negative breast cancer: A case report. Ann. Transl. Med. 10 (7), 424. doi:10.21037/atm-22-1284
Yang, Z. X., Chen, X., Tang, S. Q., and Zhang, Q. (2021). Sintilimab-induced myocarditis overlapping myositis in a patient with metastatic thymoma: A case report. Front. Cardiovasc Med. 8, 797009. doi:10.3389/fcvm.2021.797009
Yogasundaram, H., Alhumaid, W., Chen, J. W., Church, M., Alhulaimi, N., Kimber, S., et al. (2021). Plasma exchange for immune checkpoint inhibitor-induced myocarditis. CJC Open 3 (3), 379–382. doi:10.1016/j.cjco.2020.11.004
Yoshida, Y., Shimizu, I., Katsuumi, G., Jiao, S., Suda, M., Hayashi, Y., et al. (2015). p53-Induced inflammation exacerbates cardiac dysfunction during pressure overload. J. Mol. Cell Cardiol. 85, 183–198. doi:10.1016/j.yjmcc.2015.06.001
Zhang, B., Gyawali, L., Liu, Z., Du, H., and Yin, Y. (2022a). Camrelizumab-related lethal arrhythmias and myasthenic crisis in a patient with metastatic thymoma. Case Rep. Cardiol. 2022, 4042909. doi:10.1155/2022/4042909
Zhang, H., Lin, J., Shen, Y., Pan, J., Wang, C., and Cheng, L. (2022b). Protective effect of crocin on immune checkpoint inhibitors-related myocarditis through inhibiting NLRP3 mediated pyroptosis in cardiomyocytes via NF-κB pathway. J. Inflamm. Res. 15, 1653–1666. doi:10.2147/JIR.S348464
Zhang, R. S., Padegimas, A., Murphy, K. M., Evans, P. T., Peters, C. J., Domenico, C. M., et al. (2021). Treatment of corticosteroid refractory immune checkpoint inhibitor myocarditis with infliximab: A case series. Cardiooncology 7 (1), 13. doi:10.1186/s40959-021-00095-x
Zhang, S., Tao, X., Wang, L., Chen, H., Zhao, L., Sun, J., et al. (2022c). Downregulation of programmed death-1 pathway promoting CD8 + T cell cytotoxicity in primary biliary cholangitis. Dig. Dis. Sci. 67 (7), 2981–2993. doi:10.1007/s10620-021-07165-1
Keywords: immune checkpoint inhibitors, programmed cell death 1, myocarditis, cardiotoxicity, inflammation, fibrosis, cytotoxic T-lymphocyte antigen 4
Citation: Wu Y, Xu Y and Xu L (2023) Drug therapy for myocarditis induced by immune checkpoint inhibitors. Front. Pharmacol. 14:1161243. doi: 10.3389/fphar.2023.1161243
Received: 08 February 2023; Accepted: 05 May 2023;
Published: 25 May 2023.
Edited by:
Tamer M. A. Mohamed, University of Louisville, United StatesReviewed by:
Hongsong Zhang, Nanjing Medical University, ChinaJiangyun Luo, Shanghai Jiao Tong University, China
Copyright © 2023 Wu, Xu and Xu. This is an open-access article distributed under the terms of the Creative Commons Attribution License (CC BY). The use, distribution or reproduction in other forums is permitted, provided the original author(s) and the copyright owner(s) are credited and that the original publication in this journal is cited, in accordance with accepted academic practice. No use, distribution or reproduction is permitted which does not comply with these terms.
*Correspondence: Yizhou Xu, xyzhzsyxnk@163.com; Linhao Xu, xulinhaoluck@163.com