- 1Department of Anatomy, Jessenius Faculty of Medicine, Comenius University in Bratislava, Martin, Slovakia
- 2Clinic of Obstetrics and Gynecology, Jessenius Faculty of Medicine, Comenius University in Bratislava, Martin, Slovakia
- 3Department of Pathological Physiology, Jessenius Faculty of Medicine, Comenius University in Bratislava, Martin, Slovakia
- 4Museum of Literature in Moravia, Rajhrad, Czechia
- 5Department of Applied Ecology, Faculty of Environmental Sciences, Czech University of Life Sciences Prague, Prague, Czechia
- 6Department of Pharmacology, Faculty of Medicine, Pavol Jozef Safarik University, Kosice, Slovakia
- 7Department of Physiology and Biophysics, Weill Cornell Medicine in Qatar, Qatar Foundation, Doha, Qatar
- 8Predictive, Preventive and Personalised (3P) Medicine, Department of Radiation Oncology, University Hospital Bonn, Rheinische Friedrich-Wilhelms-Universität Bonn, Bonn, Germany
- 9Department of Medical Biology, Jessenius Faculty of Medicine, Comenius University in Bratislava, Martin, Slovakia
Significant limitations of the reactive medical approach in breast cancer management are clearly reflected by alarming statistics recorded worldwide. According to the WHO updates, breast malignancies become the leading cancer type. Further, the portion of premenopausal breast cancer cases is permanently increasing and demonstrates particularly aggressive patterns and poor outcomes exemplified by young patients with triple-negative breast cancer that lacks targeted therapy. Accumulating studies suggest the crucial role of stem cells in tumour biology, high metastatic activity, and therapy resistance of aggressive breast cancer. Therefore, targeting breast cancer stem cells is a promising treatment approach in secondary and tertiary breast cancer care. To this end, naturally occurring substances demonstrate high potential to target cancer stem cells which, however, require in-depth analysis to identify effective anti-cancer agents for cost-effective breast cancer management. The current article highlights the properties of flavonoids particularly relevant for targeting breast cancer stem cells to mitigate therapy resistance. The proposed approach is conformed with the principles of 3P medicine by applying predictive diagnostics, patient stratification and treatments tailored to the individualised patient profile. Expected impacts are very high, namely, to overcome limitations of reactive medical services improving individual outcomes and the healthcare economy in breast cancer management. Relevant clinical applications are exemplified in the paper.
1 Introduction
Breast cancer is at the forefront of incidence and mortality in women (Arnold et al., 2022). Based on the most recent GLOBOCAN statistics, in 2020, breast cancer was the most diagnosed cancer represented by 11.7% of all cancer cases and 24.5% of female cancer cases. Also, in the same year, breast cancer, the leading cause of cancer death, accounted for 15.5% of mortality in females (Sung et al., 2021). Moreover, Arnold et al. (2022) indicate the growth of newly diagnosed breast cancer cases by over 40% for incidence and by more than 50% for mortality by 2040. Current trends also highlight the rapid growth of the premenopausal cohort of BC patients. Thus, the ratio shift between post-menopausal and pre-menopausal breast cancer results in a younger breast-cancer profile compared with the previous century. Moreover, breast cancer in young women is more frequently asymptomatic, which is associated with difficulties in early diagnosis and treatment (Kudela et al., 2019). Alarming statistics are attributed to aggressive breast cancer subtypes, such as triple-negative breast cancer (TNBC) (Vaz-Luis et al., 2017; Howard and Olopade, 2021), which is more typical for premenopausal women (Kudela et al., 2019). Breast cancer, therefore, represents a severe healthcare and socio-economic problem (Mazurakova et al., 2022a).
Despite the progress in cancer management and early detection, cancer still remains a serious global problem. Cancer stem cells (CSCs) represent a sub-population of cancer cells that are characterized by self-renewal, differentiation, stemness, clonal expansion, promotion of cancer recurrence, and resistance to standard therapeutics (Wang et al., 2018; Tsai et al., 2021). The obstacle of the non-responsiveness or resistance of breast cancer to standard therapeutic options also requires in-depth analyses to identify effective anti-cancer agents applicable for adequate management of breast cancer, including the use of pleiotropic properties of phytochemicals (Mazurakova et al., 2022a). Current oncological research highlights the potential utilization of naturally occurring substances in the prevention and treatment of breast cancer (Kubatka et al., 2016; Kapinova et al., 2017; Kubatka et al., 2017; Abotaleb et al., 2018; Kapinova et al., 2019; Liskova et al., 2019; Kubatka et al., 2020a; Liskova et al., 2020a; Kubatka et al., 2020b; Liskova et al., 2020b). Flavonoids are plant secondary metabolites commonly found in vegetables, fruit, grains, or medicinal plants. Flavonoids exert potent anticancer capacity and pleiotropic effects targeting each of the multistep processes of carcinogenesis, including cancer initiation, promotion, and progression into metastatic diseases (Abotaleb et al., 2018; Jasek et al., 2019; Liskova et al., 2020a; Liskova et al., 2020b; Liskova et al., 2020c; Koklesova et al., 2021; Kubatka et al., 2021; Liskova et al., 2021; Samec et al., 2021). Therefore, flavonoids represents a promising source of phytochemicals with a potential to target CSCs.
Patient-beneficial and cost-effective management of breast cancer require the application of the principles of predictive, preventive, and personalized medicine (3PM), including advanced screening programs and targeted individualized approach in primary, secondary, and tertiary care (Bubnov et al., 2017; Fröhlich et al., 2018; Goldstein et al., 2020; Golubnitschaja et al., 2021). Here, we highlight the crucial benefits of flavonoids targeting breast cancer cells to combat the resistance of breast cancer in the framework of mainly secondary and tertiary care to improve individual outcomes and the cost-effectiveness of breast cancer management.
2 Breast cancer stem cells (BCSCs)
The stem cell theory describes the subpopulation of cancer cells entitled cancer stem cells (CSCs), also known as tumour-initiating cells. In the scope of breast cancer, CSCs can be termed breast cancer stem cells (BCSCs). BCSCs are characterized by a capability of self-renewal, plasticity, clonogenicity, and drug resistance (Liu et al., 2016), and by the presence of specific CSCs markers, including CD24, CD44, CD133, or ALDH1, among others (Ercan et al., 2011; Galoczova et al., 2018; Yousefnia et al., 2019). CSCs frequently overexpress ATB-binding cassette (ABC) efflux proteins that are responsible for the efflux of the drug outside the cell and thus contribute to the resistance to anti-cancer therapeutics. ABC transporters frequently overexpressed in BCSCs include multidrug-resistance-associated protein 1 (ABCC1, MRP1) and ATP-binding cassette superfamily G member 2 (ABCG2), also known as breast cancer resistance protein (BCRP) (Koh et al., 2019; Sridharan et al., 2019). Another molecule of the ATP-dependent proton pump family essential for the resistance of cancer cells is P-glycoprotein (P-gp), the product of MDR1 (ABCB1) gene (Nandi et al., 2022a).
The identification and isolation of CSCs from primary tumours or cancer cell lines can be performed via several methods including 1) detection of CSCs by side populations phenotypes based on high ABC transporter expression via hoechst 33,342 exclusion, 2) the enrichment of putative CSCs by sphere-formation in serum-free medium (based on the ability to form spheres in suspensions), 3) CSCs sorting by flow cytometry according to CSCs markers, and 4) isolation of CSC via measuring the activity of ALDH (Yousefnia et al., 2019; Zhang et al., 2020). Indeed, the identification of BCSCs phenotypes is based mainly on CD44, CD24, and ALDH1 while BCSCs with CD44+/CD24− and ALDH1+ phenotypes are considered as most aggressive and thus frequently associated with resistance to treatments (Zhang et al., 2020). Another method of investigating the existence of cancer cells capable of self-renewal is performed via transplantation of cells characterized by the presence of specific cell surface markers into NOD/SCID mice to observe the potential to give rise to new tumours (Ercan et al., 2011).
2.1 BCSCs: Plasticity between mesenchymal-like and epithelial-like states
Epithelial-to-mesenchymal transition (EMT) is a process by which cancer cells convert from epithelial into mesenchymal phenotype and gain migratory and invasive properties (Liskova et al., 2020c). As stated by Nallanthighal et al. (2017), EMT represents a key process of CSCs generation and maintenance of their characteristics associated also with metastasis as well as therapy resistance (Luo et al., 2015). Radio resistant MDA-MB-231 cells established by Ko et al. (2020) show promoted aggressiveness, CSCs features, and EMT. Thus, EMT-induction can also be considered a mechanism of resistance to radiotherapy or chemotherapy, as a typical characteristic of BCSCs, and both represent a target of strategies to overcome therapeutic resistance. CSCs show genetic and phenotypic heterogeneity but also a capacity to maintain plasticity to transition between mesenchymal-like and epithelial-like states regulated by tumour microenvironment (Luo et al., 2015). Mesenchymal-like BCSCs represent CD44+/CD24−/low populations that have EMT signature (low E-cadherin, high vimentin), tend to be quiescent (Luo et al., 2015; Kamble et al., 2021), and are localized at tumour-invasive edge adjacent to tumour stroma (Kotiyal and Bhattacharya, 2014). On the contrary, epithelial-like BCSCs characterized as ALDH+ populations possess signature of high E-cadherin and low vimentin, tend to be more proliferative (Luo et al., 2015; Kamble et al., 2021), and are located more centrally (Kotiyal and Bhattacharya, 2014). Despite differences between CSCs cells expressing either CD44+/CD24−/low or ALDH+, both populations are characterized by stemness, ability to re-create tumours in vivo, and a similar pattern of gene expression across molecular subtypes of breast cancer (Zhang et al., 2020). Moreover, the small population of BCSCs that express both markers (CD44+/CD24−/low and ALDH+) possess the greatest tumorigenic and metastatic capacity (Kotiyal and Bhattacharya, 2014; Zhang et al., 2020). Specific molecular breast cancer subtypes are characterized by different BCSCs populations. Basal-like tumours generally contain more CD44+/CD24−/low and ALDH1+ than luminal breast cancer subtypes. Specifically, luminal breast cancer tumours are enriched in CD44−/low/CD24+ cell population, basal/epithelial in CD44+/CD24+ cell populations, and basal/mesenchymal in CD44+/CD24−/low cell populations (Zhang et al., 2020). TNBC is a basal-like tumour representing the most aggressive molecular subtype of breast cancer that is characterized by the highest content of BCSCs with CD44+/CD24−/low and ALDH1+ and is frequently responsible for therapeutic resistance (Zhang et al., 2020).
2.2 Characteristics of BCSCs related to therapy resistance
2.2.1 Mammosphere formation
BCSCs tend to form spheroids, non-adherent spherical clusters of cells, also known as mammospheres (Liu et al., 2016). Mammospheres are considered a pre-cancerous state and an indicator of stem-ness (Bhandary et al., 2021; Patel et al., 2021), specifically self-renewal abilities and increased tumorigenicity and metastatic potential (Truong et al., 2021). Cells from mammospheres can be serially passaged and keep their multipotency (Ercan et al., 2011). Mammosphere culture of breast cancer cells is an effective way to enrich CSCs showing high tumorigenicity and chemoresistance (Wu et al., 2015). Stem-ness properties are analysed via in vitro assay in which BCSCs are enriched in suspension culture as “mammospheres” that imitate in vivo tumours (Wu et al., 2015; Manupati et al., 2017).
Interestingly, Yousefnia et al. (2019) performed a study using three different breast cancer cell lines with different molecular phenotypes to enrich BCSCs. For example, treatment with docetaxel resulted in significant resistance in MDA-MB-213-derived and SKBR3-derived mammospheres. Also, MCF-7-derived mammospheres showed more resistance to docetaxel when compared with parental MCF-7 cells. Accordingly, cell lines with different molecular properties possess different mammosphere formation and CSC characteristics. Although all tested cell lines showed increased mammosphere formation efficiency, MCF-7 cell line exerted the highest mammosphere formation, which may be affected by E-cadherin expression. Also, mammospheres resistant to letrozole showed increased stemness and invasive markers, while letrozole resistance is frequently associated with CSCs (Patel et al., 2021).
2.2.2 Clonogenicity
High clonogenicity is a fundamental characteristic of BCSCs (Liu et al., 2016; Rajendran and Jain, 2018). Therefore, colony forming or clonogenic assay can be considered a measure of CSCs stemness (Esquer et al., 2020), which is performed for in vitro evaluation of the capability of a single cell to grow into a colony via clonal expansion (Rajendran and Jain, 2018). The tumour formation at the secondary site reflects the stemness potential of cancer cells, which can be tracked via detecting the colony-forming potential (Sharma et al., 2022). The exposure of tamoxifen in MCF-7 cells resistant to tamoxifen (MCF7-TR) resulted in an increased number of CSCs as observed by the increased number of ALDH+ cells accompanied by increased colony formation, a higher number of mammospheres, and alterations in epigenetic and multidrug resistance (MDR) stem cell marker genes (Kalyanaraman et al., 2020). In addition, radioresistant breast cancer cells (RT-R-MDA-MB-231) showed increased colony formation and expression of CD44 and other markers of CSCs such as Notch, ALDH1, or OCT3/4 (Ko et al., 2018).
2.2.3 Side population
Side population (SP) cells represent a sub-population of cancer cells that contain a higher proportion of CSCs or exhibit CSC-like properties, overexpress ABC transporters or other CSC markers, including Notch1, and contribute to the resistance of breast cancer cells (Britton et al., 2011; Wang et al., 2015; Liu et al., 2016). As reviewed by Britton et al. (2011), SP cells are frequently radio-resistant and chemo-resistant. Indeed, the utilization of standard chemotherapeutics frequently result in SP enrichment, thus showing an inability to target these cells (Liu et al., 2016).
2.2.4 Overactivated Nrf2
The maintenance of low ROS levels represents an essential characteristic of CSCs resulting from elevated expression of ROS-scavenging molecules and antioxidant enzymes favouring survival and resistance (Wu et al., 2015). The transcriptional factor nuclear factor erythroid 2-related factor 2 (Nrf2) is an important regulator of intracellular antioxidant responses via the regulation of transcription of genes localised in the antioxidant response element (ARE) (Kamble et al., 2021). Cancer cells are associated with a higher level of endogenous ROS when compared with normal cells. However, CSCs show high antioxidant capacity keeping ROS at lower levels (through upregulated ROS-scavenging systems such as Nrf2-mediated signalling), which promotes the stemness, survival, and drug resistance of CSC (Woo et al., 2017; Tsai et al., 2021). Above all, CSCs are characterized by constitutive activation of Nrf2 (Woo et al., 2017), which is associated with resistance to radiotherapy and chemotherapy (Kamble et al., 2021). Qin et al. (2021) describe Nrf2 upregulation and its nuclear translocation in a ROS-dependent manner as a result of exposure of TNBC cells to ionizing radiation. Thus, ROS induced by ionizing radiation can activate Nrf2, which counteracts irradiation’s killing effect. However, pharmacological inhibition of Nrf2 activation induced by ionizing radiation sensitized TNBC CSCs to irradiation. Moreover, Nrf2 knockdown suppressed the ALDH+ population, stem cell markers, and reduced radio-resistance by decreasing clonogenicity in immunocompromised mice (Kamble et al., 2021). Studies show elevated Nrf2 in chemo-resistant CSC-enriched breast tumours. Nrf2-associated antioxidant genes include, for example, heme oxygenase 1 (HO-1) (Kamble et al., 2021). Nrf2-silenced mammospheres resulted in increased cell death and reduced sphere growth associated with the repression of Nrf2 target genes. Specifically, attenuated efflux transporters (such as BCRP) and increased doxorubicin accumulation were observed in Nrf2 knockdown mammospheres when compared with control mammospheres (Ryoo et al., 2015).
2.2.5 NANOG, SOX2, OCT4
CSCs are also defined by aberrant expression or overactivation of specific molecules (such as transcription factors NANOG, SOX2, OCT4) that promote stemness and therapeutic resistance (Nandi et al., 2022a). Transcription factor SOX2 is crucial for embryonic development; however, SOX2 tends to be overexpressed in CSCs and to contribute to therapeutic resistance (Novak et al., 2020). Cancer cells with high SOX2 show CSCs features and EMT phenotype (Mauro-Lizcano et al., 2022). NANOG-CD44-MDR1 network maintains stemness and chemo-resistance. NANOG regulates SOX2 to maintain the pluripotency of breast cancer cells. Moreover, the upregulation of SOX2 and OCT4 induced by NANOG depends on upregulated CD44-MDR1 network (Nandi et al., 2022a). The binding of hyaluronic acid to CD44 induces the activation of NANOG and associated STAT-3-mediated MDR1 gene expression. Also, the binding of hyaluronic acid with CD44 supports the association between ankyrin and P-gp and multidrug flux (Bourguignon et al., 2008). Pham et al. (2011) stated that the knockdown of CD44 in CD44+CD24− cells isolated from primary cultures of malignant breast tumours resulted in the differentiation of BCSCs into non-BCSCs cells with decreased tumorigenic-potential and altered expression of some stem-cell related genes. Moreover, CD44 is associated with EMT and therapeutic resistance as characteristics of CSCs. As stated above, BCSCs are associated with developing resistance associated with the regulation of NANOG, SOX2, OCT4, CD44, and MDR1 (Nandi et al., 2022a). For example, MDA-MB-231 and MCF-7/tamoxifen-resistant cells showed overexpression of NANOG, OCT3/4, and SOX2 (Arif et al., 2015). Moreover, the expression of OCT4 and NANOG can also be promoted by Nrf2 (Tsai et al., 2021). Above all, differentiated breast cancer cells can be reprogrammed into BCSCs through the re-expression of stem-ness genes (OCT4, SOX2, NANOG, KLF4) induced by radiation (Lagadec et al., 2012; Wang et al., 2014).
Figure 1 provides an illustration of selected characteristics of BCSCs that contribute to their stem-ness and associated therapy resistance.
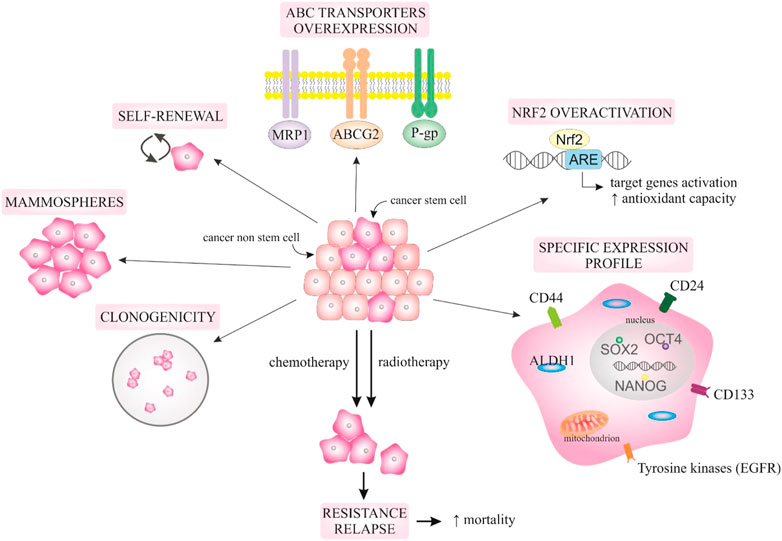
FIGURE 1. Selected BCSCs characteristics. Explanatory notes: BCSCs represent a sub-population of cancer cells that are defined by multiple characteristics including self-renewal capability, mammosphere formation, and clonogenicity. BCSCs are associated with aberrant expression of specific signalling molecules or aberrant activation of cell signalling pathways, including overexpression of ABC transporters (MRP1, ABCG2, P-gp responsible for the efflux of the drug outside the cell, overactivation of Nrf2 that increases antioxidant defence of cells, and overexpression of other markers such as CD44, CD24, EpCAM, tyrosine kinases such as EGFR, or expression transcription factors that contribute to the aggressive and resistant phenotype of BCSCs such as NANOG, SOX2, or OCT4) resulting in the regulation of target genes responsible for increased aggressiveness and therapeutic resistance that result in ineffective treatment and increased mortality of breast cancer patients.
2.2.6 Other BCSCs characteristics
Kruppel-like factor 4 (KLF4) is a product of an oncogene responsible for BCSCs maintenance, migration, and invasion. KLF4 is overexpressed in most breast cancers and is highly expressed in CSCs-enriched populations in vivo and breast cancer cell lines. Besides, KLF4 knockdown in breast cancer cells decresed the proportion of stem cells (Yu et al., 2011). Also, the abnormal expression of two main effectors of the Hippo pathway, YAP and TAZ (that mainly interacts with TEADs), are closely associated with tumorigenic processes, including the sustain of CSCs properties of self-renewal, tumour-initiating capabilities, and chemotherapy resistance particularly in TNBC. TNBC cell lines MDA-MB-231 and MDA-MB-436 frequently overexpress TAZ and are characterized by the presence of CD44+/CD24− cells (Li et al., 2018a). Furthermore, breast cancer cells with CD44+/CD24−/low phenotype (described as BCSCs) highly expressing Y-box binding protein 1 (YB-1) and P-gp are resistant to chemotherapeutics such as docetaxel, paclitaxel, or vincristine. YB-1 acts as a transcription factor in the nucleus and regulates translation in the cytoplasm; however, there is an evidence supporting the role of YB-1 in tumorigenesis, especially in the P-gp overexpression and CSCs enhancement (Li et al., 2018b). The chemokine receptor CXCR4 that is expressed in BCSCs is a crucial chemokine receptor that contributes to breast cancer metastasis, particularly of mesenchymal BCSCs. Therefore, CXCR4 also represents a target for BCSCs eradication (Sridharan et al., 2019). Other markers of CSCs include MUC1, a transmembrane glycoprotein that binds to the epidermal growth factor receptor (EGFR) family, and EpCAM that correlates with poor prognosis in numerous cancer patient types, including breast cancer (Wang et al., 2018). Moreover, Cripto-1 is a protein essential for human development in the early phases of gastrulation and is also associated with healing processes. However, Cripto-1 is upregulated in most cancer types and promotes cancer progression, metastasis, and CSCs survival (Arnouk et al., 2021). Besides, as reviewed by Tsai et al. (2021), Cripto-1 promoted EMT in mouse mammary tumours, which can be associated with EMT gene expression of CSCs to promote their self-renewal, invasion, and metastasis. Also, Cripto-1 expression is described to be associated with NANOG or OCT4 and vice versa. Cripto-1 can be considered a functional CSCs marker. Recent evidence also supports the role of epigenetics in the maintenance of CSCs. Histone modification patterns, particularly histone acetylation conducted via histone acetyltransferases and histone deacetylases (HDACs), perform necessary transcriptional regulation (Dong, 2016). In this regard, aberrantly expressed class III histone deacetylases (HDACs), also known as sirtuins (SIRTs), are also described to possess a significant role in cancer stem-ness, especially SIRT1 as the best-described SIRT (O’Callaghan and Vassilopoulos, 2017). In addition, SIRT3 and SIRT6 are also crucial for the self-renewal and tumour-initiation functions of CSCs (Sharma et al., 2022). In addition, the expression of stem cell-associated histone modifier genes, such as EZH2 contributes to self-renewal and BCSCs expansion (Jia et al., 2016). Other markers of breast cancer invasiveness and metastatic potential related to cancer stem-ness include chemokine receptor type 4 (CXCR4), which is widely expressed in various cancer types and is closely associated with migration and metastasis in breast cancer and mucin 1 (MUC1) that acts via binding to EGFR and β-catenin. Increased MUC1 also correlates with the invasiveness and metastatic potential of breast cancer. Moreover, epithelial cell adhesion molecule (EpCAM) is another marker of CSCs, which also acts on the proliferation of cancer cells (Wang et al., 2018).
In conclusion, BCSCs are responsible for cancer recurrence and treatment failure. Therefore, CSCs targeting and their elimination can be considered the most crucial approach for effective cancer management (Mauro-Lizcano et al., 2022).
3 Current research on flavonoids targeting CSCs with the potential to combat resistance
Flavonoids are polyphenols with a basic skeleton (C6-C3-C6) with two phenolic (A and B) rings and a heterocyclic pyran (C) ring (Panche et al., 2016; Liskova et al., 2020c; Koklesova et al., 2021; Mazurakova et al., 2022b; Shen et al., 2022). Based on the chemical structure, oxidation level, and substitution pattern of the C ring, flavonoids are divided into several sub-classes, including flavonols, flavones, isoflavones, anthocyanidins, flavanones, and flavan-3-ols (Liskova et al., 2021). Chalcones also belong to the flavonoid family; however, the chalcone skeleton is characterized as the initial intermediate in the biosynthesis of all flavonoids (Ninomiya et al., 2013) and is defined as the flavonoid precursor (Gao et al., 2020; Ouyang et al., 2021). Chalcones are chemically characterized by the presence of two aromatic rings and the absence of C ring and are, therefore, known as open-chain flavonoids (Ninomiya et al., 2013; Panche et al., 2016). Chalcones and flavanones, dihydrochalcones, and aurones (the isomeric of flavones) represent minor flavonoids (Ninomiya et al., 2013). Our review provides a detailed discussion on the evidence of the effects of flavonoids against BCSCs with the potential to combat the resistance of cancer cells to standard therapeutic strategies due to the close association between cancer stem-ness and drug resistance. Table 1 provides a detailed overview of flavonoids discussed in this review with their sub-classification within the class of flavonoids, chemical formula, and examples of primary natural sources.
3.1 Flavonoids targeting BCSCs
The effects of flavonoids on BCSCs are evaluated mainly in experimental, especially in vitro research. Several cell lines represent a suitable breast cancer cell model source to study BCSCs. For example, MDA-MB-231 is a TNBC cell line characterized by mesenchymal phenotype, with more than 90% of the total population expressing CD44+/CD24low/− (Hero et al., 2019). MDA-MB-468 and MDA-MB-436 represents other TNBC cell lines frequently used to study stem-cell characteristics (Li et al., 2018aLi et al., 2018a). In addition, MCF-7 is a luminal subtype breast cancer cell line (Ambrose et al., 2022) also frequently utilized to study stem-ness properties of breast cancer.
The search on most recent evaluations of the anticancer effects of flavonoids provide significant evidence on the efficacy of quercetin, kaempferol, apigenin, genistein, and naringenin (Fan et al., 2013; Li et al., 2018a; Wang et al., 2018; Hermawan et al., 2021; Sharma et al., 2022) [as the most widely naturally distributed and studied flavonoids (Calderón-Montaño et al., 2011; Kim and Park, 2020; Salehi et al., 2020)] to target BCSCs, which are closely associated with breast cancer aggressiveness, recurrence, and therapy resistance (Wang et al., 2018; Tsai et al., 2021). Quercetin is present mainly as glycoside rather than aglycone. Importantly, quercetin shows higher bioavailability than other phytochemicals (Kim and Park, 2018; Salehi et al., 2020). Quercetin inhibited proliferation, clonal expansion, mammosphere formation, and migration of MDA-MB-231 of CD44+/CD24− phenotype (BCSCs populations). Mechanistically, quercetin downregulated ALDH1, EpCAM, CXCR4, and MUC1, which are all reported as potential targets for BCSCs treatment (Wang et al., 2018) (Salehi et al., 2020).
Both kaempferol and apigenin show significant anti-stemness capacity as demonstrated via suppressed colony-forming potential and mammosphere formation in MDA-MB-468 cells in vitro. Moreover, mechanisms beyond anti-CSCs effects of kaempferol and apigenin are associated with targeting SIRT3 and SIRT6 (as demonstrated via network pharmacology), while molecular docking analysis supported the capacity of these two flavonoids as potential anti-SIRT agents to target CSCs (Sharma et al., 2022).
Moreover, the capacity of apigenin to suppress stem cell properties was demonstrated via decreased CD44+/CD24− subpopulation and mammospheres thus indicating reduced self-renewal capacity of MDA-MB-231 and MDA-MB-436 cells in vitro and suppressed tumour-initiating properties in vivo. Apigenin dose dependently inhibited YAP/TAZ and thus reversed malignant phenotype and disrupted YAP/TAZ-TEADs interactions, which was previously demonstrated to be indispensable for TAZ in maintaining stem cell traits in breast cancer cells. The authors concluded that apigenin showed potent anticancer efficacy at least partially via YAP/TAZ inhibition and decreased expression of target genes CTGF and CYR61 (Li et al., 2018a).
Moreover, significant action against BCSCs is attributed to genistein, a major soy isoflavone and naringenin, a precursor of genistein. Specifically, genistein suppressed BCSC in vitro demonstrated via reduced number and size of mammospheres and CD44+CD24– population in genistein-treated MCF-7 cells. Moreover, genistein significantly reduced tumour weight and BCSC demonstrated via ALDH when compared with control group in a xenograft model of MCF-7 cells in nude mice. Above all, the authors concluded the potent anti-BCSCs capacity of genistein to be mediated via downregulation of Hedgehog-Gli1 signalling pathway (Fan et al., 2013). In addition to Hedgehog, aberrant signalling pathways closely related to cancer stem cells include also Notch and Wnt/β-catenin (Liskova et al., 2019).
Furthermore, Hermawan et al. (2021) recently evaluated the potential therapeutic target of naringenin in BCSCs inhibition using bioinformatics study and 3D tumour-sphere in vitro modelling in breast cancer mammospheres. Among other results, naringenin inhibited mammospheres and colony formation, migration, and EMT in mammospheres. Naringenin showed the potential to inhibit BCSCs via the ability to upregulate P53 and ERα mRNA (that were found to be downregulated in mammospheres).
3.2 Flavonoids targeting BCSCs: Evidenced effects on resistance
The development of resistance to standard therapeutic strategies represents a severe obstacle of breast cancer management (Mazurakova et al., 2022a). For example, the enrichment of BCSCs following chemotherapy prevents effective management of breast cancer patients. Therefore, the eradication of BCSCs by the combination of standard cytotoxic drugs and substances specifically targeting CSCs is necessary for successful treatment (Jia et al., 2016). Flavonoids show a potent capacity to target BCSCs and therapeutic resistance, as evidenced predominantly in vitro and in vivo models of breast cancer.
3.2.1 Flavonols—Kaempferol and quercetin
Kaempferol alone or combined with a P-gp inhibitor verapamil (at a specific concentration to induce synergism) impeded pH-dependent tumour-sphere formation in vitro/ex vivo. Besides, tumour acidosis promotes CSCs phenotype and the development of therapeutic resistance. Moreover, kaempferol alone or with verapamil repressed the expression of CD44, OCT4, NANOG, and MDR1, disrupting the physical association of CD44, NANOG, and MDR1. Moreover, the dosage of this experimental candidate drug system revealed no genotoxic effects on normal breast tissues (Nandi et al., 2022a).
Similarly, kaempferol downregulated CD44, ALDH1, NANOG, MDR1, Ki67, BCL2, p53 and upregulated caspase-3 ex vivo. The authors describe the evidence on the association between p53 inactivation and the support of stem-ness and MDR1 upregulation together with the expression of other genes, thus ensuring chemo-tolerance of cancer patients via NF-κB mediated and p53 related pathways. This observation was also proved by the results on analysed ex vivo grown breast tumours via a high degree of co-expression of p53 and CD44, Ki67, ALDH1, NANOG, MDR1, BCL2, and NF-κB, thus supporting the potential of kaempferol to target specific oncogenic markers in a biomarker-driven therapeutic strategy (Nandi et al., 2022b).
Another study evaluated the impact of quercetin on MDR in breast cancer in vitro. The initial observations described overexpressed YB-1, P-gp and CD44+/CD24−/low phenotype (BCSCs) in doxorubicin-resistant MCF-7 (MCF-7/dox) cells. As stated before, YB-1 translocation significantly contributes to P-gp overexpression and CD44+/CD24−/low phenotype. Eventually, quercetin combined with docetaxel also promoted the apoptosis of MCF-7 and MCF-7/dox cells. Moreover, combined treatment of quercetin and chemotherapeutics (docetaxel, paclitaxel, and vincristine) suppressed YB-1 nuclear translocation resulting in P-gp inhibition and eliminated CD44+/CD24− cells (thus eliminating BCSCs) in both analysed cell lines. Above all, quercetin promoted the anticancer effectivity of analysed chemotherapeutics, inhibited chemotherapeutics-induced YB-1 nuclear translocation, and promoted intracellular accumulation of docetaxel in MCF-7/dox (Li et al., 2018b).
3.2.2 Flavones—Baicalein, chrysin, and luteolin
Baicalein showed efficacy as a sensitizer to overcome therapy resistance in TNBC cells in vitro. Koh et al. (2019) established treatment resistant TNBC cell model via irradiation of parental MDA-MB-231 cells (MDA-MB-231/IR); eventually, established chemo- and radio-resistant cells showed promoted invasion, migration, CSCs characteristics as well as enriched NF-κB pathway, TNF pathway, and Toll-like receptor (TLR) pathway. Moreover, transcriptome analysis revealed differential expression of several genes involved in radio- and chemo-resistance, including IFIT2. However, baicalein suppressed stem cell-like properties in chemo- and radio-resistant MDA-MB-231/IR cells and reversed IFIT2 expression.
Several anticancer therapies target the receptor tyrosine kinases (TRKs), which contribute to tumorigenesis through downstream signalling. Epidermal growth factor receptor (EGFR) is a tyrosine kinase that is overexpressed in numerous tumours and is involved in cancer cell proliferation. However, despite reduced side effects when compared with chemotherapy, EGFR inhibitors show low clinical activity. Thus, there is a need for the identification of more effective substances to target EGFR (Manupati et al., 2017). As demonstrated by Manupati et al. (2017), TNBC cancer cells (specifically MDA-MB-231) are characterized by higher EGFR expression when compared with a luminal cell line (MCF-7 cells). The authors also observed higher EGFR expression in BCSCs than in breast cancer cells. CHM-09, synthetized analogue of chrysin (isolated from Oroxylum indicum bark) with improved binding affinity to EGFR, effectively suppressed EGF-induced migration, mammosphere formation, and tri-lineage differentiation of CD24−/CD44+ breast CSCs, accompanied a reversal of EMT or the induction of MET in breast CSCs by the attenuation of EGFR signalling. The authors concluded increased responsiveness to chemotherapeutics when simultaneously treated with doxorubicin and EGFR inhibitors. Thus, EGFR represents a target of anticancer strategies due to its high expression in BCSCs (Manupati et al., 2017).
In addition, luteolin at various concentrations inhibited stemness-related proteins, ALDH1 activity, sphere formation as well as Nrf2, SIRT3, Cripto-1, and HO-1 in MDA-MB-231 cells and promoted their chemosensitivity to taxol. In conclusion, luteolin diminished the hallmarks of BC stem-ness by Nrf2-mediated pathway (Tsai et al., 2021).
3.2.3 Flavanols—EGCG and ampelopsin
Recent research supports the role of lipid metabolism in BCSCs promotion and chemo-resistance. An enzyme fatty acid synthase (FASN) is involved in de novo synthesis of the most abundant fatty acid palmitate. Indeed, several cancer types overexpress FASN, while the blockage of FASN resulted in the inhibition of cancer progression, overcoming resistance, and promoting the effectiveness of chemotherapy in vitro and in vivo. The association between FASN and drug resistance is based on the new phospholipid synthesis for membrane renovation and plasticity (Giró-Perafita et al., 2019). FASN has been shown to be overexpressed in most tumour tissues of a cohort of 100 TNBC patients (Giró-Perafita et al., 2017; Giró-Perafita et al., 2019). The flavanol epigallocatechin-3-gallate (EGCG) is the main catechin of green tea (Nagle et al., 2006). Giró-Perafita et al. (2019) studied the effects of EGCG and its synthetic derivatives on the FASN inhibition in BCSC-enriched populations resistant to doxorubicin (MDA-MB-231DXR) and paclitaxel (MDA-MB-231PTR). Eventually, the mammosphere-forming capacity of tested TNBC cells in vitro was significantly inhibited after the treatment with G28, the derivative of EGCG.
Recently, Truong et al. (2021) evaluated the effects of ampelopsin [also known as dihydromyricetin (Weng et al., 2017)] in TNBC cell line MDA-MB-231/IR, characterized by better stem-ness features, chemo-resistance, and radio-resistance when compared with parental MDA-MB-231 cells. Specifically, ampelopsin at different concentrations inhibited stem-ness features mammosphere formation, CD44+/CD24−/low and ALDH+ populations, invasion, migration, and EMT markers in resistant breast MDA-MB-231/IR cells. Moreover, ampelopsin inhibited oxidative phosphorylation in the same cell line. Indeed, CSCs show flexibility in switching between glycolysis and oxidative phosphorylation; however, recent evidence describes enhanced oxidative phosphorylation to maintain the stemness of CSCs and resistance to DNA damage. Also, the authors concluded that ampelopsin prevented TNF-α/NF-κB signalling in breast CSCs (Truong et al., 2021).
3.2.4 Chalcones—Xanthohumol and cardamonin
Similarly, a prenylated chalcone xanthohumol enhanced the sensitivity of doxorubicin-resistant MCF-7/ADR cells to docetaxel demonstrated via significantly reduced IC50 value when compared with docetaxel alone. Also, when compared with the effects on parental cells sensitive to docetaxel, the authors concluded that the synergistic effects of xanthohumol and docetaxel were not as apparent as in docetaxel-resistant cells. In addition, xanthohumol at different concentrations reduced SP cell fractions, alleviated clonogenicity, suppressed mammosphere formation and migration in a concentration-dependent manner, reduced stem cell markers, including CD44+/CD24− populations, ABCG2 and Notch1 in doxorubicin-resistant MCF-7/ADR cells; indeed, these effects were accompanied by inhibited viability, induced apoptosis, and cell cycle arrest (Liu et al., 2016).
Cardamonin, a cardamom-derived chalcone reduced colony-forming capacity, CD44high/CD24−/low subpopulations, and expression of CSC-associated genes, and stem cell-associated modifier genes in chemotherapy-enriched breast cancer stem-like SUM-190 cells. These results highlight the potential of cardamonin to target BCSCs after chemotherapy completion; moreover, cardamonin seems to act on diminishing CSC properties rather than reducing CSC populations, thus promoting the conversion of BCSCs into non-CSCs. In addition, the combinatory treatment with chemotherapy and cardamonin mitigated CSCs phenotype. Cardamonin also abrogated inflammatory cytokines closely related to CSCs development and diminished the activation of NF- κB, IκBα, and Stat3 in vitro. Moreover, cardamonin combined with doxorubicin enhanced the efficiency of chemotherapy in vivo via suppressing tumour growth and reducing CSCs, eliminated doxorubicin-enriched CSCs, and diminished doxorubicin-upregulated stemness genes (ALDH1, SOX2, OCT4) (Jia et al., 2016).
Table 2 provides a detailed overview of the above-discussed flavonoids that exert either the potential to combat BCSCs or their capability to target BCSCs with evidenced effects on therapeutic resistance as the crucial obstacle in the effective management of breast cancer.
3.3 Plant extracts rich in flavonoids
In addition to isolated flavonoids, a mixture of numerous phytochemicals within the whole plant also exert potent anticancer effects via their synergistic or additive action (Kubatka et al., 2015; Kapinova et al., 2017; Kubatka et al., 2017; Kubatka et al., 2020a; Kubatka et al., 2020b; Liskova et al., 2020c). For example, a recent study demonstrated the potent anticancer effectivity of polyphenols isolated from Artemisia annua L. to inhibit CSC markers, β-catenin and matrix metalloproteinase 9 (MMP-9), all of which were overexpressed in radio-resistant MDA-MB-231 cells in vitro (Ko et al., 2020). Moreover, extract of Viola odorata characterized by numerous phytochemicals, including flavonoids luteolin, quercetin, apigenin, or kaempferol, showed potent anticancer effects on breast cancer cells (MCF-7, SKBR3) and derived BCSCs. Specifically, the extract of Viola odorata suppressed colony formation, migration, and invasion of derived mammospheres more than in corresponding breast cancer cells. Moreover, the extract inhibited tumorigenicity in evaluated breast cancer cells and derived mammospheres in chicken embryos in vivo (Yousefnia et al., 2020). Woo et al. (2017) observed that CSCs derived from breast cancer cells MCF-7 with CD44high/CD24low phenotype form mammospheres and overexpress Nrf2 when compared with parental MCF-7 cells. Chestnut is rich in numerous polyphenols, including flavonoids (Tuyen et al., 2017). Chestnut (Castanea crenata) leaf extract suppressed nuclear translocation of Nrf2, suppressed ARE-luciferase activity, and reduced protein expression of Nrf2-downstream gene HO-1 in MCF-7 derived CSCs. Chestnut leaf extract also suppressed colony formation of CSCs, while more effective inhibition of clonogenicity was observed after the combinatory treatment with paclitaxel. Therefore, CSCs treatment with chestnut leaf extract combined with paclitaxel showed more efficacy in reducing the viability of CSC when compared with paclitaxel alone; however, such combinatory treatment showed no significant effects on MCF-7 cells (Woo et al., 2017). Also, solvent fractions of selected medicinal plants (Vernonia leopoldi, Clematis simensis) (Tuasha et al., 2020), characterized by the presence of numerous phytochemicals, including flavonoids (Teshome et al., 2022; Tuasha et al., 2022), showed a potent capacity to reduce ALDH+ subpopulations of JIMT-1 cells, and/or reduce colony formation, and/or reduced migration, and/or inhibit TNF-α-induced NF-κB nuclear translocation (Tuasha et al., 2020). Many other plant extracts show significant capacity to suppress CSCs in breast cancer models, for example, pomegranate extract targeting BCSCs and EMT (Nallanthighal et al., 2017) or hexane extract of Garcinia quaesita fruits inducing apoptosis of BCSCs isolated from MDA-MB-231 cells (Colamba Pathiranage et al., 2021) with the potential to increase the effectiveness of therapeutics.
In conclusion, above discussed preclinical evidence on the effects of flavonoids against BCSCs can represent an essential cornerstone for further clinical evaluations. Figure 2 provides an illustrated overview of BCSCs markers/characteristics that are associated with the resistance of breast cancer to therapeutics and are most frequently demonstrated to be modulated by flavonoids in most current in vitro and in vivo studies.
4 Flavonoids: Limitations and potential in clinical evaluations
Here we provided a detailed overview of current evidence on the effects of flavonoids to target BCSCs to overcome breast cancer resistance to standard therapeutics. Above discussed study results focused primarily on in vitro analyses of the anticancer efficacy of flavonoids (Jia et al., 2016; Woo et al., 2017; Truong et al., 2021; Nandi et al., 2022b; Sharma et al., 2022). However, the discussion on the advantages and limitations of flavonoids as well as the currents state of their evaluation in clinical studies is essential to provide a comprehensive review anti-cancer effects of flavonoids targeted against BCSCs.
Naturally occurring phytochemicals are advantageous due to their pluripotency, minimal or no side effects, and cost-effectiveness (Liskova et al., 2020c; Koklesova et al., 2021; Liskova et al., 2021; Samec et al., 2021). However, the anticancer capability of in vivo or in a clinical setting is associated with numerous complications. Firstly, flavonoids are characterized by low bioavailability due to their characteristics and extensive metabolism (Liskova et al., 2020c). For example, dihydromyricetin is generally associated with poor bioavailability due to the hydrophilic character (Li et al., 2017). Flavonoid metabolites are generally associated with reduced bioactivity when compared with parental compounds (Thilakarathna and Rupasinghe, 2013). Flavonoids present most abundantly in our diet may not reach target organs. On the contrary, several flavonoid metabolites are associated with more potent bioactivity than their precursors (Murota et al., 2018; Liskova et al., 2020c). The utilization of flavonoids as health-promoting agents also highly depends on individual characteristics such as age, sex, habitual diet, genotype, and gut microbiome, which plays an essential role in the absorption, distribution, tissue exposure, and elimination of phytochemicals (Liskova et al., 2020c). Still, the current scientific community also analyses the approaches to improve the bioavailability and utilization of flavonoids against cancer, for example, via absorption enhancers, structural transformation, carrier complexes, or nanotechnology, among others (Thilakarathna and Rupasinghe, 2013; Zhao et al., 2019).
Despite the peculiarities associated with limited bioavailability of phytochemicals, current evidence on the evaluation of the effects of phytochemicals in clinical settings still provide at least promising results (Mazurakova et al., 2022a; Mazurakova et al., 2022c). Lazzeroni et al. (2017) demonstrated that oral administration of a lecithin formulation of a caffeine-free green tea catechin extract (Greenselect Phytosome) increases EGCG bioavailability, while EGCG can be detectable in breast tumour tissues of early breast cancer patients. Also, free EGCG plasma levels significantly correlated with Ki67 decrease in tumour tissues (Lazzeroni et al., 2017). Above all, as reviewed previously, phytochemicals showed potential in the prevention of breast cancer in terms of dietary patterns and breast cancer risk or in the mitigation of side effects of cancer therapies (for example, silymarin reducing radiodermatitis or EGCG reducing acute skin damage induced by radiation) (Zhu et al., 2016; Karbasforooshan et al., 2019; Mazurakova et al., 2022a). Moreover, flavonoids showed efficacy in enhancing the effectiveness of conventional therapeutic strategies demonstrated in clinical studies, for example, EGCG enhanced radiotherapy in breast cancer patients and affected cell proliferation, invasion, and cell cycle among others (Zhang et al., 2012). In addition, green tea extract, Polyphenon E resulted in non-significant decrease of VEGF by 11.5% at 2 months (p = 0.02) and 13.9% at 4 months (p = 0.05) in hormone-receptor negative breast cancer patients (Crew et al., 2015). In addition, the overweight after breast cancer treatment can increase the risk of recurrent disease; however, decaffeinated green tea taken for 6 months was associated with slightly reduced body weight and improved HDL and glucose homeostasis in overweight breast cancer survivors (Stendell-Hollis et al., 2010). On the contrary, Wang et al. (2009) demonstrated no association of dietary intake of flavonols (quercetin, kaempferol, myricetin), flavones (apigenin and luteolin), or flavonoid-rich food and incidence of cancer in middle-aged and older women. Therefore, despite above-discussed evidence, the potential of flavonoids in clinical research cannot be definitely confirmed. As an example, despite that epidemiological studies predominantly highlight the potential of soy (that contains isoflavones genistein and daidzein) in breast cancer prevention, the effects of soy on breast cancer is not clearly understood yet (Shike et al., 2014). A six-months intervention of mixed soy isoflavones in healthy, high-risk adult Western women did not result in reduced epithelial proliferation thus suggesting inefficacy in breast cancer prevention (Khan et al., 2012). On the contrary, the consumption of soy products is associated with lower risk of breast cancer in BRCA mutations carriers (Ko et al., 2013) or with reduced fibroglandular breast tissue, a breast cancer risk marker (Lu et al., 2022). Moreover, as demonstrated in a population-based study on TNBC cohort, long-term pre-diagnosis consumption of soy, a rich source of isoflavones, may lead in increased expression of tumour suppressors and decreased expression of oncogenes in breast tumour tissue (Guo et al., 2016).
Above all, here we provided an overview of the available results of the utilization of flavonoids in clinical settings, especially in enhancing the effectiveness or mitigating the side effects of conventional therapy (Mazurakova et al., 2022a). Still, despite proved anti-cancer potential of flavonoids (or phytochemicals in general) in affecting each of the multistep process of carcinogenesis in experimental in vitro and in vivo settings (Kapinova et al., 2017; Jasek et al., 2019; Liskova et al., 2020a; Liskova et al., 2020c; Koklesova et al., 2021; Koklesova et al., 2021; Liskova et al., 2021; Samec et al., 2021), the research evidence on the potential of flavonoids in clinical studies is generally limited, while the clinical evaluation of potent effectiveness of flavonoids in targeting BCSCs is not available. Nevertheless, the precise clinical evaluation of the anti-cancer effectiveness of phytochemicals is highly required for improvement of cancer management and a shift from reactive to 3PM medicine. BCSCs represents a crucial target in cancer management due to their role in cancer initiation, progression, invasiveness, metastasis, and therapeutic resistance. The potential utilization of flavonoids targeting BCSCs requires precise evaluation of multiple approaches, including the precise mechanisms of action of flavonoids in specific conditions as well as the characteristics of the individual patient.
5 Conclusion and future outlooks
The appropriate management of breast cancer requires strategies to be beneficial for the patient and society as a whole. Primary care represents the protection of individuals against cancer development. Secondary care is associated with protection against the development of metastatic disease and tertiary care is targeted against cascading complications related to palliative care and chronic disease management (Liskova et al., 2020c). The evidence in detail discussed in this review supports flavonoids’ great potential, especially in secondary care of breast cancer patients, due to the capacity of flavonoids to combat resistance and thus could potentially provide more beneficial therapeutic outcomes for breast cancer patients. The non-responsiveness of cancer patients to treatment strategies or relapse of the disease after initial responses or after years of remission urgently highlight the need of novel strategies to be identified. The incidence of breast cancer is alarming, and the decreasing age at the diagnosis of breast cancer further support the need to improve cancer management in the framework of 3PM.
Above presented research data are considered highly beneficial for innovative strategies in the framework of 3P medicine promoting predictive approach, targeted prevention, and personalisation of medical services (Mazurakova et al., 2022a). Relevant clinical applications can be exemplified by
- Hypoxia-induced breast cancer stem cells (Zhang et al., 2016). 3PM approach may consider relevant phenotyping such as patients stratified by Flammer syndrome demonstrating evident microcirculation deficits associated with systemic ischemia-reperfusion events and particularly aggressive breast cancer with poor outcomes (Bubnov et al., 2017; Golubnitschaja, 2017).
- Treated cancer patients exposed to environmental stress leading to mitochondrial injury and mitochondrial DNA (mtDNA) extracellular release (Sansone et al., 2017). In both cases, 3PM approach is based on individualised patient profiling, predictive diagnostics and therapies tailored to the person. To this end, systemic effects are well reflected in the mitochondrial health status—the regular control of which is highly recommended for predictive and preventive purposes (Koklesova et al., 2022).
Data availability statement
The datasets presented in this study can be found in online repositories. The names of the repository/repositories and accession number(s) can be found in the article/supplementary material.
Author contributions
AM was responsible for the conception. The manuscript was drafted by AM, DV, LK, MS, M.Š, MK, and EK who also performed the literature search. Manuscript was critically revised by PK, DB, STSH, and KB. OG has contributed with PPPM/3P medicine expertise and data interpretation in the framework of PPPM. PK, OG, and DB provided skilled assistance and supervised the overall preparation of the manuscript. The figures were designed and prepared by LK. All authors have read and agreed to the published version of the manuscript.
Funding
This work was supported by the Scientific Grant Agency of the Ministry of Education of the Slovak Republic under the Contracts No. VEGA 1/0045/23.
Conflict of interest
The authors declare that the research was conducted in the absence of any commercial or financial relationships that could be construed as a potential conflict of interest.
Publisher’s note
All claims expressed in this article are solely those of the authors and do not necessarily represent those of their affiliated organizations, or those of the publisher, the editors and the reviewers. Any product that may be evaluated in this article, or claim that may be made by its manufacturer, is not guaranteed or endorsed by the publisher.
References
Abotaleb, M., Samuel, S. M., Varghese, E., Varghese, S., Kubatka, P., Liskova, A., et al. (2018), Flavonoids in cancer and apoptosis. Cancers (Basel) 11. Available at: https://www.ncbi.nlm.nih.gov/pmc/articles/PMC6357032/.
Ambrose, J. M., Veeraraghavan, V. P., Vennila, R., Rupert, S., Sathyanesan, J., Meenakshisundaram, R., et al. (2022). Comparison of mammosphere formation from stem-like cells of normal breast, malignant primary breast tumors, and MCF-7 cell line. J. Egypt. Natl. Cancer Inst. 34, 51. doi:10.1186/s43046-022-00152-1
Arif, K., Hussain, I., Rea, C., and El-Sheemy, M. (2015). The role of Nanog expression in tamoxifen-resistant breast cancer cells. Onco Targets Ther. 8, 1327–1334. doi:10.2147/OTT.S67835
Arnold, M., Morgan, E., Rumgay, H., Mafra, A., Singh, D., Laversanne, M., et al. (2022). Current and future burden of breast cancer: Global statistics for 2020 and 2040. Breast 66, 15–23. doi:10.1016/j.breast.2022.08.010
Arnouk, H., Yum, G., and Shah, D. (2021). Cripto-1 as a key factor in tumor progression, epithelial to mesenchymal transition and cancer stem cells. Int. J. Mol. Sci. 22, 9280. doi:10.3390/ijms22179280
Bhandary, L., Bailey, P. C., Chang, K. T., Underwood, K. F., Lee, C. J., Whipple, R. A., et al. (2021). Lipid tethering of breast tumor cells reduces cell aggregation during mammosphere formation. Sci. Rep. Nat. Publ. Group 11, 3214. doi:10.1038/s41598-021-81919-9
Bie, B., Sun, J., Guo, Y., Li, J., Jiang, W., Yang, J., et al. (2017). Baicalein: A review of its anti-cancer effects and mechanisms in hepatocellular carcinoma. Biomed. Pharmacother. 93, 1285–1291. doi:10.1016/j.biopha.2017.07.068
Bourguignon, L. Y. W., Peyrollier, K., Xia, W., and Gilad, E. (2008). Hyaluronan-CD44 interaction activates stem cell marker nanog, stat-3-mediated MDR1 gene expression, and ankyrin-regulated multidrug efflux in breast and ovarian tumor cells. J. Biol. Chem. 283, 17635–17651. doi:10.1074/jbc.M800109200
Britton, K. M., Kirby, J. A., Lennard, T. W. J., and Meeson, A. P. (2011). Cancer stem cells and side population cells in breast cancer and metastasis. Cancers (Basel) 3, 2106–2130. doi:10.3390/cancers3022106
Bubnov, R., Polivka, J., Zubor, P., Konieczka, K., and Golubnitschaja, O. (2017). Pre-metastatic niches” in breast cancer: Are they created by or prior to the tumour onset? “Flammer syndrome” relevance to address the question. EPMA J. 8, 141–157. doi:10.1007/s13167-017-0092-8
Calderón-Montaño, J. M., Burgos-Morón, E., Pérez-Guerrero, C., and López-Lázaro, M. (2011). A review on the dietary flavonoid kaempferol. Mini Rev. Med. Chem. 11, 298–344. doi:10.2174/138955711795305335
Colamba Pathiranage, V., Lowe, J. N., Rajagopalan, U., Ediriweera, M. K., Senathilake, K., Piyathilaka, P., et al. (2021). Hexane extract of Garcinia quaesita fruits induces apoptosis in breast cancer stem cells isolated from triple negative breast cancer cell line MDA-MB-231. Nutr. Cancer 73, 845–855. doi:10.1080/01635581.2020.1773511
Crew, K. D., Ho, K. A., Brown, P., Greenlee, H., Bevers, T. B., Arun, B., et al. (2015). Effects of a green tea extract, Polyphenon E, on systemic biomarkers of growth factor signalling in women with hormone receptor-negative breast cancer. J. Hum. Nutr. Diet. 28, 272–282. doi:10.1111/jhn.12229
Dong, Z. (2016). Function of sirtuins in cancer stem cells. Int. J. Stem Cell Res. Ther. 3, doi:10.23937/2469-570x/1410024
Eng, Q. Y., Thanikachalam, P. V., and Ramamurthy, S. (2018). Molecular understanding of Epigallocatechin gallate (EGCG) in cardiovascular and metabolic diseases. J. Ethnopharmacol. 210, 296–310. doi:10.1016/j.jep.2017.08.035
Ercan, C., van Diest, P. J., and Vooijs, M. (2011). Mammary development and breast cancer: The role of stem cells. Curr. Mol. Med. 11, 270–285. doi:10.2174/156652411795678007
Esquer, H., Zhou, Q., Abraham, A. D., and LaBarbera, D. V. (2020). Advanced high-content-screening applications of clonogenicity in cancer. SLAS Discov. 25, 734–743. doi:10.1177/2472555220926921
Fan, P., Fan, S., Wang, H., Mao, J., Shi, Y., Ibrahim, M. M., et al. (2013). Genistein decreases the breast cancer stem-like cell population through Hedgehog pathway. Stem Cell Res. Ther. 4, 146. doi:10.1186/scrt357
Fröhlich, H., Patjoshi, S., Yeghiazaryan, K., Kehrer, C., Kuhn, W., and Golubnitschaja, O. (2018). Premenopausal breast cancer: Potential clinical utility of a multi-omics based machine learning approach for patient stratification. EPMA J. 9, 175–186. doi:10.1007/s13167-018-0131-0
Galoczova, M., Coates, P., and Vojtesek, B. (2018). STAT3, stem cells, cancer stem cells and p63. Cell Mol. Biol. Lett. 23, 12. doi:10.1186/s11658-018-0078-0
Gao, F., Huang, G., and Xiao, J. (2020). Chalcone hybrids as potential anticancer agents: Current development, mechanism of action, and structure-activity relationship. Med. Res. Rev. 40, 2049–2084. doi:10.1002/med.21698
Giró-Perafita, A., Rabionet, M., Planas, M., Feliu, L., Ciurana, J., Ruiz-Martínez, S., et al. (2019). EGCG-derivative G28 shows high efficacy inhibiting the mammosphere-forming capacity of sensitive and resistant TNBC models. Molecules 24, 1027. doi:10.3390/molecules24061027
Giró-Perafita, A., Sarrats, A., Pérez-Bueno, F., Oliveras, G., Buxó, M., Brunet, J., et al. (2017). Fatty acid synthase expression and its association with clinico-histopathological features in triple-negative breast cancer. Oncotarget 8, 74391–74405. doi:10.18632/oncotarget.20152
Goldstein, E., Yeghiazaryan, K., Ahmad, A., Giordano, F. A., Fröhlich, H., and Golubnitschaja, O. (2020). Optimal multiparametric set-up modelled for best survival outcomes in palliative treatment of liver malignancies: Unsupervised machine learning and 3 PM recommendations. EPMA J. 11, 505–515. doi:10.1007/s13167-020-00221-2
Golubnitschaja, O. (2017). Feeling cold and other underestimated symptoms in breast cancer: Anecdotes or individual profiles for advanced patient stratification? EPMA J. 8, 17–22. doi:10.1007/s13167-017-0086-6
Golubnitschaja, O., Liskova, A., Koklesova, L., Samec, M., Biringer, K., Büsselberg, D., et al. (2021). Caution, “normal” BMI: Health risks associated with potentially masked individual underweight-EPMA position paper 2021. EPMA J. 1–22, 243–264. doi:10.1007/s13167-021-00251-4
Guo, X., Cai, Q., Bao, P., Wu, J., Wen, W., Ye, F., et al. (2016). Long-term soy consumption and tumor tissue microRNA and gene expression in triple negative breast cancer. Cancer 122, 2544–2551. doi:10.1002/cncr.29981
Han, J. M., Kim, H. L., and Jung, H. J. (2021). Ampelopsin inhibits cell proliferation and induces apoptosis in HL60 and K562 leukemia cells by downregulating AKT and NF-κB signaling pathways. Int. J. Mol. Sci. 22, 4265. doi:10.3390/ijms22084265
Harish, V., Haque, E., Śmiech, M., Taniguchi, H., Jamieson, S., Tewari, D., et al. (2021). Xanthohumol for human malignancies: Chemistry, pharmacokinetics and molecular targets. Int. J. Mol. Sci. 22, 4478. doi:10.3390/ijms22094478
Hermawan, A., Ikawati, M., Jenie, R. I., Khumaira, A., Putri, H., Nurhayati, I. P., et al. (2021). Identification of potential therapeutic target of naringenin in breast cancer stem cells inhibition by bioinformatics and in vitro studies. Saudi Pharm. J. 29, 12–26. doi:10.1016/j.jsps.2020.12.002
Hero, T., Bühler, H., Kouam, P. N., Priesch-Grzeszowiak, B., Lateit, T., and Adamietz, I. A. (2019). The triple-negative breast cancer cell line MDA-MB 231 is specifically inhibited by the ionophore salinomycin. Anticancer research. Int. Inst. Anticancer Res. 39, 2821–2827. doi:10.21873/anticanres.13410
Howard, F. M., and Olopade, O. I. (2021). Epidemiology of triple-negative breast cancer: A review. Cancer J. 27, 8–16. doi:10.1097/PPO.0000000000000500
Imran, M., Rauf, A., Abu-Izneid, T., Nadeem, M., Shariati, M. A., Khan, I. A., et al. (2019). Luteolin, a flavonoid, as an anticancer agent: A review. Biomed. Pharmacother. 112, 108612. doi:10.1016/j.biopha.2019.108612
Jasek, K., Kubatka, P., Samec, M., Liskova, A., Smejkal, K., Vybohova, D., et al. (2019). DNA methylation status in cancer disease: Modulations by plant-derived natural compounds and dietary interventions. Biomolecules 9, 289. doi:10.3390/biom9070289
Jia, D., Tan, Y., Liu, H., Ooi, S., Li, L., Wright, K., et al. (2016). Cardamonin reduces chemotherapy-enriched breast cancer stem-like cells in vitro and in vivo. Oncotarget 7, 771–785. doi:10.18632/oncotarget.5819
Kalyanaraman, A., Gnanasampanthapandian, D., Shanmughan, P., Kishore, P., Ramalingam, S., Arunachalam, R., et al. (2020). Tamoxifen induces stem-like phenotypes and multidrug resistance by altering epigenetic regulators in ERα+ breast cancer cells. Stem Cell Investig. 7, 20. doi:10.21037/sci-2020-020
Kamble, D., Mahajan, M., Dhat, R., and Sitasawad, S. (2021). Keap1-Nrf2 pathway regulates ALDH and contributes to radioresistance in breast cancer stem cells. Cells. Multidiscip. Digit. Publ. Inst. 10, 83.
Kapinova, A., Kubatka, P., Liskova, A., Baranenko, D., Kruzliak, P., Matta, M., et al. (2019). Controlling metastatic cancer: The role of phytochemicals in cell signaling. J. Cancer Res. Clin. Oncol. 145, 1087–1109. doi:10.1007/s00432-019-02892-5
Kapinova, A., Stefanicka, P., Kubatka, P., Zubor, P., Uramova, S., Kello, M., et al. (2017). Are plant-based functional foods better choice against cancer than single phytochemicals? A critical review of current breast cancer research. Biomed. Pharmacother. 96, 1465–1477. doi:10.1016/j.biopha.2017.11.134
Karbasforooshan, H., Hosseini, S., Elyasi, S., Fani Pakdel, A., and Karimi, G. (2019). Topical silymarin administration for prevention of acute radiodermatitis in breast cancer patients: A randomized, double-blind, placebo-controlled clinical trial. Phytother. Res. 33, 379–386. doi:10.1002/ptr.6231
Khan, S. A., Chatterton, R. T., Michel, N., Bryk, M., Lee, O., Ivancic, D., et al. (2012). Soy isoflavone supplementation for breast cancer risk reduction: A randomized phase II trial. Cancer Prev. Res. (Phila). 5, 309–319. doi:10.1158/1940-6207.CAPR-11-0251
Kim, J. K., and Park, S. U. (2018). Quercetin and its role in biological functions: An updated review. EXCLI J. 17, 856–863. doi:10.17179/excli2018-1538
Kim, J. K., and Park, S. U. (2020). Recent studies on kaempferol and its biological and pharmacological activities. EXCLI J. 19, 627–634.
Ko, K-P., Kim, S-W., Ma, S. H., Park, B., Ahn, Y., Lee, J. W., et al. (2013). Dietary intake and breast cancer among carriers and noncarriers of BRCA mutations in the Korean Hereditary Breast Cancer Study. Am. J. Clin. Nutr. 98, 1493–1501. doi:10.3945/ajcn.112.057760
Ko, Y. S., Jin, H., Lee, J. S., Park, S. W., Chang, K. C., Kang, K. M., et al. (2018). Radioresistant breast cancer cells exhibit increased resistance to chemotherapy and enhanced invasive properties due to cancer stem cells, Oncol. Rep. 40 (6), 3752–3762. doi:10.3892/or.2018.6714
Ko, Y. S., Jung, E. J., Go, S-I., Jeong, B. K., Kim, G. S., Jung, J-M., et al. (2020). Polyphenols extracted from Artemisia annua L. Exhibit anti-cancer effects on radio-resistant MDA-MB-231 human breast cancer cells by suppressing stem cell phenotype, β-catenin, and MMP-9. Molecules 25, 1916. doi:10.3390/molecules25081916
Koh, S. Y., Moon, J. Y., Unno, T., and Cho, S. K. (2019). Baicalein suppresses stem cell-like characteristics in radio- and chemoresistant MDA-MB-231 human breast cancer cells through up-regulation of IFIT2. Nutrients 11. Available at: https://www.ncbi.nlm.nih.gov/pmc/articles/PMC6471144/.
Koklesova, L., Liskova, A., Samec, M., Zhai, K., Al-Ishaq, R. K., Bugos, O., et al. (2021). Protective effects of flavonoids against mitochondriopathies and associated pathologies: Focus on the predictive approach and personalized prevention. Int. J. Mol. Sci. 22, 8649. doi:10.3390/ijms22168649
Koklesova, L., Mazurakova, A., Samec, M., Kudela, E., Biringer, K., Kubatka, P., et al. (2022). Mitochondrial health quality control: Measurements and interpretation in the framework of predictive, preventive, and personalized medicine. EPMA J. 13, 177–193. doi:10.1007/s13167-022-00281-6
Kotiyal, S., and Bhattacharya, S. (2014). Breast cancer stem cells, EMT and therapeutic targets. Biochem. Biophysical Res. Commun. 453, 112–116. doi:10.1016/j.bbrc.2014.09.069
Kubatka, P., Kapinová, A., Kello, M., Kruzliak, P., Kajo, K., Výbohová, D., et al. (2016). Fruit peel polyphenols demonstrate substantial anti-tumour effects in the model of breast cancer. Eur. J. Nutr. 55, 955–965. doi:10.1007/s00394-015-0910-5
Kubatka, P., Kapinová, A., Kružliak, P., Kello, M., Výbohová, D., Kajo, K., et al. (2015). Antineoplastic effects of Chlorella pyrenoidosa in the breast cancer model. Nutrition 31, 560–569. doi:10.1016/j.nut.2014.08.010
Kubatka, P., Kello, M., Kajo, K., Samec, M., Jasek, K., Vybohova, D., et al. (2020). Chemopreventive and therapeutic efficacy of Cinnamomum zeylanicum L. bark in experimental breast carcinoma: Mechanistic in vivo and in vitro analyses. Molecules 25 (6),1399. doi:10.3390/molecules25061399
Kubatka, P., Kello, M., Kajo, K., Samec, M., Liskova, A., Jasek, K., et al. (2020). Rhus coriaria L. (Sumac) demonstrates oncostatic activity in the therapeutic and preventive model of breast carcinoma. Int. J. Mol. Sci. 22, 183. doi:10.3390/ijms22010183
Kubatka, P., Mazurakova, A., Samec, M., Koklesova, L., Zhai, K., Al-Ishaq, R., et al. (2021). Flavonoids against non-physiologic inflammation attributed to cancer initiation, development, and progression-3PM pathways. EPMA J. 12, 559–587. doi:10.1007/s13167-021-00257-y
Kubatka, P., Uramova, S., Kello, M., Kajo, K., Kruzliak, P., Mojzis, J., et al. (2017). Antineoplastic effects of clove buds (Syzygium aromaticum L.) in the model of breast carcinoma. J. Cell Mol. Med. 21, 2837–2851. doi:10.1111/jcmm.13197
Kudela, E., Samec, M., Kubatka, P., Nachajova, M., Laucekova, Z., Liskova, A., et al. (2019). Breast cancer in young women: Status quo and advanced disease management by a predictive, preventive, and personalized approach. Cancers (Basel) 11. Available at: https://www.ncbi.nlm.nih.gov/pmc/articles/PMC6896106/.
Lagadec, C., Vlashi, E., Della Donna, L., Dekmezian, C., and Pajonk, F. (2012). Radiation-induced reprogramming of breast cancer cells. Stem Cells 30, 833–844. doi:10.1002/stem.1058
Lazzeroni, M., Guerrieri-Gonzaga, A., Gandini, S., Johansson, H., Serrano, D., Cazzaniga, M., et al. (2017). A presurgical study of lecithin formulation of green tea extract in women with early breast cancer. Cancer Prev. Res. (Phila). 10, 363–370. doi:10.1158/1940-6207.CAPR-16-0298
Li, H., Li, Q., Liu, Z., Yang, K., Chen, Z., Cheng, Q., et al. (2017). The versatile effects of dihydromyricetin in health. Evid. Based Complement. Altern. Med. 2017, 1053617. doi:10.1155/2017/1053617
Li, S., Zhao, Q., Wang, B., Yuan, S., Wang, X., and Li, K. (2018). Quercetin reversed MDR in breast cancer cells through down-regulating P-gp expression and eliminating cancer stem cells mediated by YB-1 nuclear translocation. Phytother. Res. 32, 1530–1536. doi:10.1002/ptr.6081
Li, W., and Xiao, H. (2021). Dihydromyricetin alleviates high glucose-induced oxidative stress and apoptosis in human retinal pigment epithelial cells by downregulating miR-34a expression</p>, 14. DMSO. Dove Press, 387–397.
Li, Y-W., Xu, J., Zhu, G-Y., Huang, Z-J., Lu, Y., Li, X-Q., et al. (2018). Apigenin suppresses the stem cell-like properties of triple-negative breast cancer cells by inhibiting YAP/TAZ activity. Cell Death Discov. 4, 105. doi:10.1038/s41420-018-0124-8
Liskova, A., Koklesova, L., Samec, M., Smejkal, K., Samuel, S. M., Varghese, E., et al. (2020). Flavonoids in cancer metastasis. Cancers (Basel) [Internet]. 2020 [cited 2020 Oct 24];12. Available from: https://www.ncbi.nlm.nih.gov/pmc/articles/PMC7352928/.
Liskova, A., Koklesova, L., Samec, M., Varghese, E., Abotaleb, M., Samuel, S. M., et al. (2020). Implications of flavonoids as potential modulators of cancer neovascularity. J. Cancer Res. Clin. Oncol. 146, 3079–3096. doi:10.1007/s00432-020-03383-8
Liskova, A., Kubatka, P., Samec, M., Zubor, P., Mlyncek, M., Bielik, T., et al. (2019). Dietary phytochemicals targeting cancer stem cells. Molecules 24. Available at: https://www.ncbi.nlm.nih.gov/pmc/articles/PMC6429493/.
Liskova, A., Samec, M., Koklesova, L., Brockmueller, A., Zhai, K., Abdellatif, B., et al. (2021). Flavonoids as an effective sensitizer for anti-cancer therapy: Insights into multi-faceted mechanisms and applicability towards individualized patient profiles. EPMA J. 12, 155–176. doi:10.1007/s13167-021-00242-5
Liskova, A., Stefanicka, P., Samec, M., Smejkal, K., Zubor, P., Bielik, T., et al. (2020). Dietary phytochemicals as the potential protectors against carcinogenesis and their role in cancer chemoprevention. Clin. Exp. Med. 20, 173–190. doi:10.1007/s10238-020-00611-w
Liu, M., Yin, H., Qian, X., Dong, J., Qian, Z., and Miao, J. (2016). Xanthohumol, a prenylated chalcone from hops, inhibits the viability and stemness of doxorubicin-resistant MCF-7/ADR cells. Molecules 22, 36. doi:10.3390/molecules22010036
Lu, L-J. W., Chen, N-W., Brunder, D. G., Nayeem, F., Nagamani, M., Nishino, T. K., et al. (2022). Soy isoflavones decrease fibroglandular breast tissue measured by magnetic resonance imaging in premenopausal women: A 2-year randomized double-blind placebo controlled clinical trial. Clin. Nutr. ESPEN 52, 158–168. doi:10.1016/j.clnesp.2022.10.007
Luo, M., Brooks, M., and Wicha, M. S. (2015). Epithelial-mesenchymal plasticity of breast cancer stem cells: Implications for metastasis and therapeutic resistance. Curr. Pharm. Des. 21, 1301–1310. doi:10.2174/1381612821666141211120604
Manupati, K., Dhoke, N. R., Debnath, T., Yeeravalli, R., Guguloth, K., Saeidpour, S., et al. (2017). Inhibiting epidermal growth factor receptor signalling potentiates mesenchymal-epithelial transition of breast cancer stem cells and their responsiveness to anticancer drugs. FEBS J. 284, 1830–1854. doi:10.1111/febs.14084
Mauro-Lizcano, M., Sotgia, F., and Lisanti, M. P. (2022). SOX2-high cancer cells exhibit an aggressive phenotype, with increases in stemness, proliferation and invasion, as well as higher metabolic activity and ATP production. Aging (Albany NY) 14, 9877–9889. doi:10.18632/aging.204452
Mazurakova, A., Koklesova, L., Samec, M., Kudela, E., Kajo, K., Skuciova, V., et al. (2022). Anti-breast cancer effects of phytochemicals: Primary, secondary, and tertiary care. EPMA J. 13, 315–334. doi:10.1007/s13167-022-00277-2
Mazurakova, A., Koklesova, L., Samec, M., Kudela, E., Sivakova, J., Pribulova, T., et al. (2022). Flavonoids exert potential in the management of hypertensive disorders in pregnancy. Pregnancy Hypertens. 29, 72–85. doi:10.1016/j.preghy.2022.06.007
Mazurakova, A., Samec, M., Koklesova, L., Biringer, K., Kudela, E., Al-Ishaq, R. K., et al. (2022). Anti-prostate cancer protection and therapy in the framework of predictive, preventive and personalised medicine - comprehensive effects of phytochemicals in primary, secondary and tertiary care. EPMA J. 13, 461–486. doi:10.1007/s13167-022-00288-z
Murota, K., Nakamura, Y., and Uehara, M. (2018). Flavonoid metabolism: The interaction of metabolites and gut microbiota. Biosci. Biotechnol. Biochem. 82, 600–610. doi:10.1080/09168451.2018.1444467
Nagle, D. G., Ferreira, D., and Zhou, Y-D. (2006). Epigallocatechin-3-gallate (EGCG): Chemical and biomedical perspectives. Phytochemistry 67, 1849–1855. doi:10.1016/j.phytochem.2006.06.020
Nallanthighal, S., Elmaliki, K. M., and Reliene, R. (2017). Pomegranate extract alters breast cancer stem cell properties in association with inhibition of epithelial-to-mesenchymal transition. Nutr. Cancer 69, 1088–1098. doi:10.1080/01635581.2017.1359318
Nandi, S. K., Pradhan, A., Das, B., Das, B., Basu, S., Mallick, B., et al. (2022). Kaempferol attenuates viability of ex-vivo cultured post-NACT breast tumor explants through downregulation of p53 induced stemness, inflammation and apoptosis evasion pathways. Pathol. Res. Pract. 237, 154029. doi:10.1016/j.prp.2022.154029
Nandi, S. K., Roychowdhury, T., Chattopadhyay, S., Basu, S., Chatterjee, K., Choudhury, P., et al. (2022). Deregulation of the CD44-NANOG-MDR1 associated chemoresistance pathways of breast cancer stem cells potentiates the anti-cancer effect of Kaempferol in synergism with Verapamil. Toxicol. Appl. Pharmacol. 437, 115887. doi:10.1016/j.taap.2022.115887
Nawaz, J., Rasul, A., Shah, M. A., Hussain, G., Riaz, A., Sarfraz, I., et al. (2020). Cardamonin: A new player to fight cancer via multiple cancer signaling pathways. Life Sci. 250, 117591. doi:10.1016/j.lfs.2020.117591
Naz, S., Imran, M., Rauf, A., Orhan, I. E., Shariati, M. A., et al. Iahtisham-Ul-Haq null (2019). Chrysin: Pharmacological and therapeutic properties. Life Sci. 235, 116797. doi:10.1016/j.lfs.2019.116797
Ninomiya, M., and Koketsu, M. (2013). “Minor flavonoids (chalcones, flavanones, dihydrochalcones, and aurones),” in Natural products: Phytochemistry, botany and metabolism of alkaloids, phenolics and terpenes [internet]. Editors K. G. Ramawat, and J-M. Mérillon (Berlin, Heidelberg: Springer), 1867–1900. doi:10.1007/978-3-642-22144-6_62
Novak, D., Hüser, L., Elton, J. J., Umansky, V., Altevogt, P., and Utikal, J. (2020). SOX2 in development and cancer biology. Seminars Cancer Biol. 67, 74–82. doi:10.1016/j.semcancer.2019.08.007
O’Callaghan, C., and Vassilopoulos, A. (2017). Sirtuins at the crossroads of stemness, aging, and cancer. Aging Cell 16, 1208–1218. doi:10.1111/acel.12685
Ouyang, Y., Li, J., Chen, X., Fu, X., Sun, S., and Wu, Q. (2021). Chalcone derivatives: Role in anticancer therapy. Biomolecules 11, 894. doi:10.3390/biom11060894
Panche, A. N., Diwan, A. D., and Chandra, S. R. (2016). Flavonoids: An overview. J. Nutr. Sci. [Internet] 5.
Patel, J. R., Gallegos, K. M., Walker, R. R., Davidson, A. M., Davenport, I., and Tilghman, S. L. (2021). Mammospheres of letrozole-resistant breast cancer cells enhance breast cancer aggressiveness. Oncol. Lett. 22, 620. doi:10.3892/ol.2021.12881
Pham, P. V., Phan, N. L. C., Nguyen, N. T., Truong, N. H., Duong, T. T., Le, D. V., et al. (2011). Differentiation of breast cancer stem cells by knockdown of CD44: Promising differentiation therapy. J. Transl. Med. 9, 209. doi:10.1186/1479-5876-9-209
Qin, S., He, X., Lin, H., Schulte, B. A., Zhao, M., Tew, K. D., et al. (2021). Nrf2 inhibition sensitizes breast cancer stem cells to ionizing radiation via suppressing DNA repair. Free Radic. Biol. Med. 169, 238–247. doi:10.1016/j.freeradbiomed.2021.04.006
Rajendran, V., and Jain, M. V. (2018). In vitro tumorigenic assay: Colony forming assay for cancer stem cells. Methods Mol. Biol. 1692, 89–95. doi:10.1007/978-1-4939-7401-6_8
Ryoo, I-G., Choi, B-H., and Kwak, M-K. (2015). Activation of NRF2 by p62 and proteasome reduction in sphere-forming breast carcinoma cells. Oncotarget 6, 8167–8184. doi:10.18632/oncotarget.3047
Salehi, B., Fokou, P. V. T., Sharifi-Rad, M., Zucca, P., Pezzani, R., Martins, N., et al. (2019). The therapeutic potential of naringenin: A review of clinical trials. Pharm. (Basel) 12, E11. doi:10.3390/ph12010011
Salehi, B., Machin, L., Monzote, L., Sharifi-Rad, J., Ezzat, S. M., Salem, M. A., et al. (2020). Therapeutic potential of quercetin: New insights and perspectives for human health. ACS Omega 5, 11849–11872. doi:10.1021/acsomega.0c01818
Samec, M., Liskova, A., Koklesova, L., Mersakova, S., Strnadel, J., Kajo, K., et al. (2021)., .Flavonoids targeting HIF-1: Implications on cancer metabolism. Multidiscip. Digit. Publ. Inst. 13, 130
Sansone, P., Savini, C., Kurelac, I., Chang, Q., Amato, L. B., Strillacci, A., et al. (2017). Packaging and transfer of mitochondrial DNA via exosomes regulate escape from dormancy in hormonal therapy-resistant breast cancer. Proc. Natl. Acad. Sci. U. S. A. 114, E9066–E9075. doi:10.1073/pnas.1704862114
Sharifi-Rad, J., Quispe, C., Imran, M., Rauf, A., Nadeem, M., Gondal, T. A., et al. (2021). Genistein: An integrative overview of its mode of action, pharmacological properties, and health benefits. Oxid. Med. Cell Longev. 2021, 3268136. doi:10.1155/2021/3268136
Sharma, A., Sinha, S., Keswani, H., and Shrivastava, N. (2022). Kaempferol and Apigenin suppresses the stemness properties of TNBC cells by modulating Sirtuins. Mol. Divers 26, 3225–3240. doi:10.1007/s11030-022-10384-x
Shen, N., Wang, T., Gan, Q., Liu, S., Wang, L., and Jin, B. (2022). Plant flavonoids: Classification, distribution, biosynthesis, and antioxidant activity. Food Chem. 383, 132531. doi:10.1016/j.foodchem.2022.132531
Shike, M., Doane, A. S., Russo, L., Cabal, R., Reis-Filho, J. S., Gerald, W., et al. (2014). The effects of soy supplementation on gene expression in breast cancer: A randomized placebo-controlled study. J. Natl. Cancer Inst. 106, dju189. doi:10.1093/jnci/dju189
Sridharan, S., Howard, C. M., Tilley, A. M. C., Subramaniyan, B., Tiwari, A. K., Ruch, R. J., et al. (2019). Novel and alternative targets against breast cancer stemness to combat chemoresistance. Frontiers in Oncology [Internet]. 2019 [cited 2023 Feb 2];9. Available from: https://www.frontiersin.org/articles/10.3389/fonc.2019.01003.
Stendell-Hollis, N. R., Thomson, C. A., Thompson, P. A., Bea, J. W., Cussler, E. C., and Hakim, I. A. (2010). Green tea improves metabolic biomarkers, not weight or body composition: A pilot study in overweight breast cancer survivors. J. Hum. Nutr. Diet. 23, 590–600. doi:10.1111/j.1365-277X.2010.01078.x
Sung, H., Ferlay, J., Siegel, R. L., Laversanne, M., Soerjomataram, I., Jemal, A., et al. (2021). Global cancer statistics 2020: GLOBOCAN estimates of incidence and mortality worldwide for 36 cancers in 185 countries. CA A Cancer J. Clin. 71, 209–249. doi:10.3322/caac.21660
Teshome, N., Degu, A., Ashenafi, E., Ayele, E., and Abebe, A. (2022). Evaluation of wound healing and anti-inflammatory activity of hydroalcoholic leaf extract of Clematis simensis fresen (ranunculaceae). Clin. Cosmet. Investig. Dermatol 15, 1883–1897. doi:10.2147/CCID.S384419
Thilakarathna, S. H., and Rupasinghe, H. P. V. (2013). Flavonoid bioavailability and attempts for bioavailability enhancement. Nutrients 5, 3367–3387. doi:10.3390/nu5093367
Truong, V. N-P., Nguyen, Y. T-K., and Cho, S-K. (2021). Ampelopsin suppresses stem cell properties accompanied by attenuation of oxidative phosphorylation in chemo- and radio-resistant MDA-MB-231 breast cancer cells. Pharm. (Basel) 14, 794. doi:10.3390/ph14080794
Tsai, K-J., Tsai, H-Y., Tsai, C-C., Chen, T-Y., Hsieh, T-H., Chen, C-L., et al. (2021). Luteolin inhibits breast cancer stemness and enhances chemosensitivity through the nrf2-mediated pathway. Molecules 26, 6452. doi:10.3390/molecules26216452
Tuasha, N., Escobar, Z., Seifu, D., Gadisa, E., Petros, B., Sterner, O., et al. (2022). Cytotoxic and other bioactivities of a novel and known sesquiterpene lactones isolated from Vernonia leopoldi (Sch. Bip. ex Walp.) Vatke in breast cancer cell lines. Toxicol. Rep. 9, 382–392. doi:10.1016/j.toxrep.2022.02.011
Tuasha, N., Seifu, D., Gadisa, E., Petros, B., and Oredsson, S. (2020). Solvent fractions of selected Ethiopian medicinal plants used in traditional breast cancer treatment inhibit cancer stem cells in a breast cancer cell line. BMC Complement. Med. Ther. 20, 366. doi:10.1186/s12906-020-03154-5
Tuli, H. S., Tuorkey, M. J., Thakral, F., Sak, K., Kumar, M., Sharma, A. K., et al. (2019). Molecular mechanisms of action of genistein in cancer: Recent advances. Front. Pharmacol. 10, 1336. doi:10.3389/fphar.2019.01336
Tuyen, P. T., Xuan, T. D., Khang, D. T., Ahmad, A., Quan, N. V., Tu, Anh T. T., et al. (2017). Phenolic Compositions and Antioxidant Properties in Bark, Flower, Inner Skin, Kernel and Leaf Extracts of Castanea crenata Sieb. et Zucc. Zucc. Antioxidants (Basel) 6, 31. doi:10.3390/antiox6020031
Vaz-Luis, I., Lin, N. U., Keating, N. L., Barry, W. T., Winer, E. P., and Freedman, R. A. (2017). Factors associated with early mortality among patients with de novo metastatic breast cancer: A population-based study. Oncologist 22, 386–393. doi:10.1634/theoncologist.2016-0369
Voon, F-L., Sulaiman, M. R., Akhtar, M. N., Idris, M. F., Akira, A., Perimal, E. K., et al. (2017). Cardamonin (2′,4′-dihydroxy-6′-methoxychalcone) isolated from Boesenbergia rotunda (L.) Mansf. inhibits CFA-induced rheumatoid arthritis in rats. Eur. J. Pharmacol. 794, 127–134. doi:10.1016/j.ejphar.2016.11.009
Wang, L., Lee, I-M., Zhang, S. M., Blumberg, J. B., Buring, J. E., and Sesso, H. D. (2009). Dietary intake of selected flavonols, flavones, and flavonoid-rich foods and risk of cancer in middle-aged and older women. Am. J. Clin. Nutr. 89, 905–912. doi:10.3945/ajcn.2008.26913
Wang, M., Wang, Y., and Zhong, J. (2015). Side population cells and drug resistance in breast cancer. Mol. Med. Rep. 11, 4297–4302. doi:10.3892/mmr.2015.3291
Wang, R., Yang, L., Li, S., Ye, D., Yang, L., Liu, Q., et al. (2018). Quercetin inhibits breast cancer stem cells via downregulation of aldehyde dehydrogenase 1A1 (ALDH1A1), chemokine receptor type 4 (CXCR4), mucin 1 (MUC1), and epithelial cell adhesion molecule (EpCAM). Med. Sci. Monit. 24, 412–420. doi:10.12659/msm.908022
Wang, Y., Li, W., Patel, S. S., Cong, J., Zhang, N., Sabbatino, F., et al. (2014). Blocking the formation of radiation-induced breast cancer stem cells. Oncotarget 5, 3743–3755. doi:10.18632/oncotarget.1992
Weng, L., Zhang, H., Li, X., Zhan, H., Chen, F., Han, L., et al. (2017). Ampelopsin attenuates lipopolysaccharide-induced inflammatory response through the inhibition of the NF-κB and JAK2/STAT3 signaling pathways in microglia. Int. Immunopharmacol. 44, 1–8. doi:10.1016/j.intimp.2016.12.018
Woo, Y., Oh, J., and Kim, J-S. (2017). Suppression of Nrf2 activity by chestnut leaf extract increases chemosensitivity of breast cancer stem cells to paclitaxel. Nutrients 9, 760. doi:10.3390/nu9070760
Wu, T., Harder, B. G., Wong, P. K., Lang, J. E., and Zhang, D. D. (2015). Oxidative stress, mammospheres and Nrf2-new implication for breast cancer therapy? Mol. Carcinog. 54, 1494–1502. doi:10.1002/mc.22202
Yousefnia, S., Ghaedi, K., Seyed Forootan, F., and Nasr Esfahani, M. H. (2019). Characterization of the stemness potency of mammospheres isolated from the breast cancer cell lines. Tumour Biol. 41, 1010428319869101. doi:10.1177/1010428319869101
Yousefnia, S., Naseri, D., Seyed Forootan, F., Tabatabaeian, M., Moattar, F., Ghafghazi, T., et al. (2020). Suppressive role of Viola odorata extract on malignant characters of mammosphere-derived breast cancer stem cells. Clin. Transl. Oncol. 22, 1619–1634. doi:10.1007/s12094-020-02307-9
Yu, F., Li, J., Chen, H., Fu, J., Ray, S., Huang, S., et al. (2011). Kruppel-like factor 4 (KLF4) is required for maintenance of breast cancer stem cells and for cell migration and invasion. Oncogene 30, 2161–2172. doi:10.1038/onc.2010.591
Zhang, C., Samanta, D., Lu, H., Bullen, J. W., Zhang, H., Chen, I., et al. (2016). Hypoxia induces the breast cancer stem cell phenotype by HIF-dependent and ALKBH5-mediated m6A-demethylation of NANOG mRNA. Proc. Natl. Acad. Sci. U. S. A. 113, E2047–E2056. doi:10.1073/pnas.1602883113
Zhang, G., Wang, Y., Zhang, Y., Wan, X., Li, J., Liu, K., et al. (2012). Anti-cancer activities of tea epigallocatechin-3-gallate in breast cancer patients under radiotherapy. Curr. Mol. Med. 12, 163–176. doi:10.2174/156652412798889063
Zhang, X., Powell, K., and Li, L. (2020). Breast cancer stem cells: Biomarkers, identification and isolation methods, regulating mechanisms, cellular origin, and beyond. Cancers (Basel) 12, 3765. doi:10.3390/cancers12123765
Zhao, J., Yang, J., and Xie, Y. (2019). Improvement strategies for the oral bioavailability of poorly water-soluble flavonoids: An overview. Int. J. Pharm. 570, 118642. doi:10.1016/j.ijpharm.2019.118642
Zhou, Y., Shu, F., Liang, X., Chang, H., Shi, L., Peng, X., et al. (2014). Ampelopsin induces cell growth inhibition and apoptosis in breast cancer cells through ROS generation and endoplasmic reticulum stress pathway. PLoS One 9, e89021. doi:10.1371/journal.pone.0089021
Keywords: breast cancer, resistance, non-responsiveness, cancer stem cells, predictive, preventive and personalised medicine, secondary and tertiary care
Citation: Mazurakova A, Koklesova L, Vybohova D, Samec M, Kudela E, Biringer K, Šudomová M, Hassan STS, Kello M, Büsselberg D, Golubnitschaja O and Kubatka P (2023) Therapy-resistant breast cancer in focus: Clinically relevant mitigation by flavonoids targeting cancer stem cells. Front. Pharmacol. 14:1160068. doi: 10.3389/fphar.2023.1160068
Received: 06 February 2023; Accepted: 27 March 2023;
Published: 06 April 2023.
Edited by:
Vijayasteltar B. Liju, Ben-Gurion University of the Negev, IsraelReviewed by:
Ajaikumar B. Kunnumakkara, Indian Institute of Technology Guwahati, IndiaManu Prasad, Memorial Sloan Kettering Cancer Center, United States
Copyright © 2023 Mazurakova, Koklesova, Vybohova, Samec, Kudela, Biringer, Šudomová, Hassan, Kello, Büsselberg, Golubnitschaja, Kubatka. This is an open-access article distributed under the terms of the Creative Commons Attribution License (CC BY). The use, distribution or reproduction in other forums is permitted, provided the original author(s) and the copyright owner(s) are credited and that the original publication in this journal is cited, in accordance with accepted academic practice. No use, distribution or reproduction is permitted which does not comply with these terms.
*Correspondence: Peter Kubatka, cGV0ZXIua3ViYXRrYUB1bmliYS5zaw==; Alena Mazurakova, YWxlbmEubWF6dXJha292YUB1bmliYS5zaw==