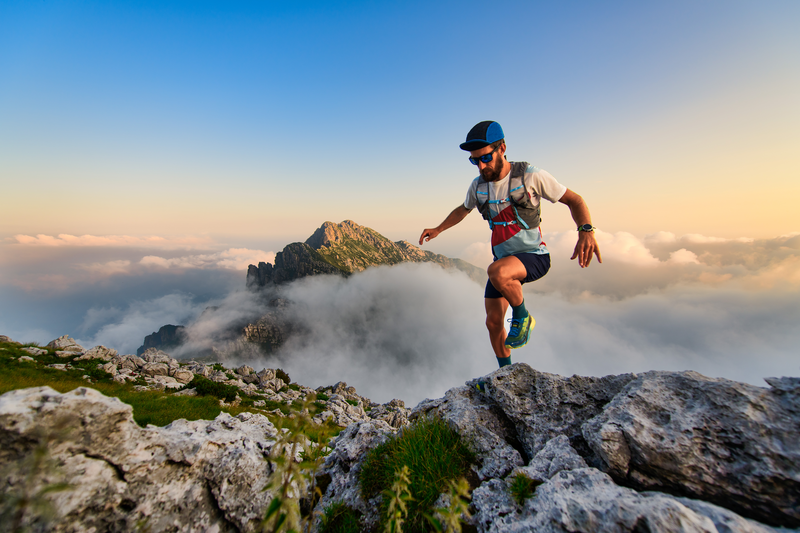
94% of researchers rate our articles as excellent or good
Learn more about the work of our research integrity team to safeguard the quality of each article we publish.
Find out more
REVIEW article
Front. Pharmacol. , 29 March 2023
Sec. Pharmacology of Anti-Cancer Drugs
Volume 14 - 2023 | https://doi.org/10.3389/fphar.2023.1159985
Cancer poses a serious threat to human health, and the search for safe and effective drugs for its treatment has aroused interest and become a long-term goal. Traditional Chinese herbal medicine (TCM), an ancient science with unique anti-cancer advantages, has achieved outstanding results in long-term clinical practice. Accumulating evidence shows that saponins are key bioactive components in TCM and have great research and development applications for their significant role in the treatment of cancer. Saponins are a class of glycosides comprising nonpolar triterpenes or sterols attached to hydrophilic oligosaccharide groups that exert antitumor effects by targeting the NF-κB, PI3Ks-Akt-mTOR, MAPK, Wnt-β-catenin, JAK-STAT3, APMK, p53, and EGFR signaling pathways. Presently, few advances have been made in physiological and pathological studies on the effect of saponins on signal transduction pathways involved in cancer treatment. This paper reviews the phytochemistry and extraction methods of saponins of TCM and their effects on signal transduction pathways in cancer. It aims to provide theoretical support for in-depth studies on the anticancer effects of saponins.
Cancer is one of the most lethal diseases caused by cells escaping homeostatic control and proliferating and differentiating abnormally. It is the second leading cause of human death worldwide (Yan et al., 2017). As of 2019, approximately 23.6 million new cancer cases and 10 million cancer deaths were reported worldwide, and the trend has been increasing annually (Kocarnik et al., 2022). Among them, lung cancer is the leading cause of morbidity and mortality among men, and global cancer data published by the International Agency for Research on Cancer (IARC) (2020) indicate that lung cancer ranks first in terms of morbidity and mortality among all malignancies (Sung et al., 2021). Breast cancer is one of the most common malignant tumors among women, with the highest incidence rate (Arnold et al., 2022). Other types of cancers, including those of the colon, esophagus, and pancreas, are also increasing yearly, thereby seriously endangering the physical and mental health of human beings. Currently, the main method of cancer treatment is chemotherapy (Nagasaka and Gadgeel., 2018), and the commonly used chemotherapeutic agents are cytotoxic and antimetabolic drugs, including adriamycin, cyclophosphamide, etc. (Khan et al., 2019); however, chemotherapeutic drugs can cause serious side effects of immunodeficiency, fatigue, diarrhea, and respiratory difficulties (Qiang et al., 2023). Therefore, the search for safe and efficient drugs or ingredients is a global concern.
Compared to traditional chemotherapeutic drugs for cancer treatment, active ingredients derived from Chinese herbal medicine (CHM) including saponins, polysaccharides, alkaloids, flavonoids, volatile oils, etc., have the advantages of multi-target synergistic effects and less toxic side effects, thus effectively inhibiting cancer cells from invading (Kumar and Jaitak., 2019) or metastasizing and differentiating (Sarwar et al., 2018; Zhou et al., 2018). Saponins are a class of glycosides with relatively complex structures and are widely found in CHM, such as Panax ginseng (Wei et al., 2020), Caulophyllum robustum Maxim (Lü et al., 2019), etc. They exert a variety of important biological activities and have a wide range of pharmacological effects; for example, antitumor (Xu et al., 2016), immunomodulatory (Bhardwaj et al., 2014), antioxidant (Wang et al., 2015), anti-inflammatory (Xiang et al., 2016), hypoglycemic (Uzayisenga et al., 2014), and therapeutic in cardiovascular diseases (Wu et al., 2019). CHM is one of the most active and fastest-progressing areas of research in traditional Chinese medicine. Saponins not only have the advantages of multi-target and multi-pathway in Chinese medicine but the active ingredients are also easy to identify and refined in modern medicine. Accumulating evidence shows that saponins prevent and treat cancer through multiple mechanisms and links, mainly including the induction of cell cycle arrest, promotion of apoptosis, induction of autophagy, anti-angiogenesis, inhibition of migration, and induction of tumor cell differentiation (Tian et al., 2020; Chen et al., 2018a; Liu et al., 2014). The potential mechanism of action of saponins against cancer is shown in Figure 1. Saponins can reduce the side effects on patients by eliminating tumor cells through apoptosis (Man et al., 2010). Ginsenosides (Yao and Guan., 2022), astragalosides (Georgieva et al., 2021), Pulsatilla chinensis saponins (Li et al., 2020), Paridis Rhizoma (Chonglou) saponins (Yu et al., 2022), Radix Bupleuri saponins (Cheng and Ying., 2021), and Zizyphus jujuba saponins (Jia et al., 2020) have significant and excellent anticancer effects. Therefore, saponins are promising drug candidates in the biomedical and pharmaceutical fields.
In recent years, owing to the in-depth study of cancer pathogenesis, tumorigenesis is found to be related to the transduction of many signaling pathways, including JAK-STAT3, NF-κB, MAPK, p53, PI3Ks-Akt-mTOR, Wnt and others, and these are considered important in regulating key functions of human tumor cells (Zhu et al., 2022; Song et al., 2022; Kobayashi et al., 2020). Modifications of various key regulatory pathways promote tumor cell metabolism, proliferation, and apoptosis, and multiple alterations in cell signaling mechanisms cause changes in cancer cells. The complex nature of cell signaling networks is a useful attempt to better understand the behavior and biological processes of tumor cells (Fu et al., 2022). The use of key signaling molecules in the pathway as targets for drug therapy has been the focus and is a fundamental direction for studying the mechanism of drug action. However, the progress of research on the effects of saponins on the corresponding cellular signal transduction pathways in the anticancer process is poorly reported, which is a matter of concern.
Taken together, saponins, as active ingredients in CHM, are effective against different kinds of tumors. This paper presents a review of the phytochemistry of the saponins of CHM, their effect on cell signal transduction pathways, and the progress of research on their extraction methods since 2014. The data presented in this review were collected from different websites, including PubMed, Citexs, Web of Science, Elsevier, and Science Direct. The keywords used in our search were “saponins”, “anticancer potential of saponins”, “biological activities of saponins”, “signal transduction pathway”, “cancer”, “triterpenoid saponins”, etc. This review aimed to sort out the key signaling pathways involved in the functional mechanism of saponins from CHM for cancer treatment, and we hope to provide scientific information for researchers and clinical workers, for the in-depth research and exploitation of saponins.
Phytochemical studies have shown that saponins can be divided into the following two major groups: triterpenoid and steroid (Fuchs et al., 2017). Among them, triterpene saponins are the most widespread, and consist of 30 carbon skeletons of triterpene glycosides in a pentacyclic structure (Augustin et al., 2011). Many common herbs including those in the Araliaceae, Leguminosae, Polygalaceae, and Campanulaceae families, etc. Contain triterpene saponins, which can be divided into tetracyclic triterpenes and pentacyclic triterpenes (Han and He., 2021). Steroid saponins are a class of steroidal glycosides of spirostane compounds combined with sugars. These do not contain carboxyl groups in the molecules and are often neutral. Steroidal saponins are raw materials for the synthesis of steroid hormones and related drugs and are widely used in the pharmaceutical industry (Passos et al., 2022). Steroidal saponins are mostly found in plants belonging to Dioscoreaceae, Agavaceae, and Scrophulariaceae, and Liliaceae, Gingeraceae, and Trilliaceae Lindl families (Dong et al., 2019). Based on their structures, they can be classified into four groups, namely, spirostanol, isosprirostanol, furostanol, and pseudospirostanol.
Saponins mainly comprise sapogenin with sugars, glyoxylates, or other organic acids. Among them, the sapogenin skeletons of triterpene saponins mainly include cycloastragenol, dammarane, oleanane, ursane, and lupane, and the sapogenin skeletons of steroidal saponins include spirostane, furostane, cholestane, and cardenolide (Zeng et al., 2022). The composition of sugars mainly includes glucose, galactose, rhamnose, arabinose, and other pentoses (Singh and Chaudhuri., 2018).
The structures of natural glycoside components are mostly in the form of hydrophobic glycosides or triterpenoids or steroids connected to glycosyl groups by glycosidic bonds, and usually, the sugar chains are attached to the sapogenins either as a unilateral sugar chain (one sugar side chain at C-3) or a bilateral sugar chain, i.e., two sugar side chains at C-3 and C-28 (Baky et al., 2022). The cytotoxic activity of saponins is associated with the presence of a free carboxyl group at C-28 and a glycosyl group at C-3 (Cho et al., 2016). Among them, the free carboxyl group at C-28 is important for antitumor activity (Chwalek et al., 2006). Saponins have large molecular weights and complex structures, and often those with similar chemical structures are subject to small differences in the number of sugar chains and the location of the linkage sugars, resulting in different biological activities. Primary saponins can be converted into hypo saponins or sapogenins by enzymatic digestion, and by the intestinal flora (Luo et al., 2020; He et al., 2019a). Most sapogenins have higher biological activity than their proto-saponin forms. For instance, the antitumor activity of ginsenosides is in the following order: sapogenins > monoglycosides > disaccharides > triglycosides > tetrasaccharides (Navarro Del Hierro et al., 2018a). Intestinal microbes are considered the “second genome” of the human body (Sommer et al., 2017), and saponins are metabolized by intestinal microorganisms to produce new components. These are absorbed into the blood and undergo biotransformation. The types of biotransformation reactions mainly include glycosyl hydrolysis, redox, acetylation, rearrangement, etc. Among them, hydrolysis of sugar groups is the most common during biotransformation (Chen et al., 2018b). New ingredients and sapogenins are produced by physiological and biochemical processes and exert therapeutic effects. This is a more profound transformation mechanism in the body, which is conducive to deepening the understanding of the material basis of saponins. Simultaneously, the complex structure of saponins makes chemical synthesis more difficult, and their biotransformation by intestinal flora yields highly active and low-toxic metabolic components, which has become a development trend in this field. The chemical structures of various triterpene and steroidal saponins described in the paper are shown in Figure 2.
FIGURE 2. Chemical structure of triterpene saponins and steroidal saponins involved in the paper. Among them, (A) Astragaloside IV (Molecular formula: C41H68O14), (B) Saikosaponin-A (Molecular formula: C41H66O13), and (C) TSBE (Molecular formula: C42H69O13), (D) Ziyuglycoside II (Molecular formula: C35H56O8), (E) Jujuboside B (Molecular formula: C52H84O20), (F) Momordin Ic (Molecular formula: C41H62O14), (G) Raddeanin A (Molecular formula: C46H74O16), (H) Ginsenosides Rh4 (Molecular formula: C35H58O8), and (I) Afrocyclamin A (Molecular formula: C54H86O20), (J) Pulsatilla saponin D (Molecular formula: C47H76O17), (K) Ginsenosides Rg3 (Molecular formula: C43H74O12), (L) Platycodin D (Molecular formula: C57H92O28); (M) Timosaponin AIII (Molecular formula: C39H64O13), (N) Gypenoside XL (Molecular formula: C42H70O14), (O) DT-13 (Molecular formula: C46H74O15), (P) Gypenoside XII (Molecular formula: C42H72O12), and (Q) Paris Saponin II (Molecular formula: C49H78O22), (R) Gypenoside LXXIX (Molecular formula: C42H72O14), (S) A-24 (Molecular formula: C55H90O21), (T) Ophiopogonin B (Molecular formula: C39H62O12); (U) Timosaponin A3 (Molecular formula: C39H64O13), and (V) Dioscin (Molecular formula: C45H72O16), (W) Polyphyllin D (Molecular formula: C44H70O16), and (X) Ophiopogonin D (Molecular formula: C46H74O14).
The phosphatidylinositol 3-kinase (PI3K)/protein kinase B (Akt)/mammalian target of rapamycin (mTOR) signaling pathway, plays a key role in the formation and development of various diseases, including cancer (Ediriweera et al., 2019), neurodegenerative disorders (Fakhri et al., 2021), etc. PI3K is a class of lipid kinases further classified into three subclasses, namely, PI3KI, PI3KII, and PI3KIII. Activated PI3K further catalyzes phosphatidylinositol bisphosphate (PIP2) to phosphatidylinositol trisphosphate (PIP3), and PIP3 can activate Akt (Tan., 2020). Akt is an important signaling target downstream of PI3K and is divided into three main classes, namely, Akt1, Akt2, and Akt3 (Yu et al., 2018). PI3K-Akt and AKT/mTOR signaling pathways have key roles in cell survival (Tewari et al., 2022; Xie et al., 2017). mTOR is a serine/threonine kinase, a key protein essential for life processes. mTOR is also a downstream signal of the PI3K/Akt pathway and a key component of most signaling cascades (Rong et al., 2020). The PI3K/Akt/mTOR signaling pathway affects the tumor cell cycle, apoptosis, autophagy, and angiogenesis by altering the activity of its downstream effector molecules, and therefore, it may be an effective tool for targeted cancer therapy.
TBSE, the main active ingredient of Bupleurum, induces apoptosis in human colon cancer SW480 and SW60 cells through the PI3K/Akt/mTOR signaling pathway. It reduces the expression of the anti-apoptotic member, Bcl2, causes downregulation of expressions of PI3K, Akt, mTOR, and p-PI3K, p-Akt, p-mTOR, and increases the expression of Bax, cleaved caspase-3, cleaved caspase-9 (Zhang et al., 2022). Afrocyclamin A increases the expression of p53, p21, and Bax, in addition to upregulating the expression of cleaved PARP, cleaved caspase-3, and Cyt-c but decreases the levels of Bcl-2, cyclin E, cyclin D, cyclin B, and inhibits the expressions of MMP-2 and MMP-9 (Sachan et al., 2018). PNS (Liu et al., 2022a) inhibits the proliferation of Y79 cells and induces apoptosis through the PI3K/Akt pathway. It significantly increases the levels of cleaved caspase-3, cleaved caspase-8, cleaved caspase-9, and downregulates Bcl-2, PI3K, p-Akt (THR308), p-Akt (SER473), and p-mTOR levels. Wang found that Hederacolchiside A1 could promote the expression of cleaved caspase-3 and cytochrome, downregulate bcl-2 levels, and inhibit the phosphorylation of PI3K, mTOR, Akt, and P70S6K (Wang et al., 2018a). Total secondary saponin elevated the expressions of Bax/Bcl-2, Cyt-c, caspase-3, and caspase-9 by inhibiting the proliferation of MCF-7 cells and inducing apoptosis through PI3K/Akt/mTOR signal transduction (Zhang et al., 2020). Moreover, other triterpenoid saponins, such as Jujuboside B (Li L et al., 2021) and Saikosaponin-A (Du et al., 2021) exert anti-cancer effects through the PI3K/Akt pathway.
Steroidal saponins, the material basis of higher plants like Ophiopogon japonicus (T.f) Ker-Gawl (OJ), Anemarrhena asphodeloides, and Rhizoma Paridis, inhibit cell proliferation (Wang et al., 2021a; Wang et al., 2020), induce cell cycle arrest (Long et al., 2015), and induce apoptosis (Liu et al., 2021; Song et al., 2019) through the PI3K/Akt/mTOR pathway. SSOJ significantly inhibits the expression of Ki67, p-PI3K/PI3K, p-Akt/Akt, and mTOR, and upregulates the levels of p53 and autophagy mediators (LC3-II/I ratio, ATG-3, ATG-7, and Beclin-1) (Chen et al., 2017). Paris Saponin II enhances the activities of Cyt-C, caspase 9, cleaved-caspase3, and Bax, while increasing the expressions of LC3-II and Beclin-1 by PSII but decreasing those of P62 and bcl-2, which are involved in PI3K/Akt/mTOR signaling (Zhang et al., 2016). Xu et al. showed that A-24 not only increased the expressions of cleaved-caspase-3, cleaved-caspase-8, cleaved-caspase-9, and Bax in SGC-7901 and AGS cells but also increased the levels of LC3-II and Beclin-1, and resulted in the downregulation of Bcl-2 expression, which played a role in inducing apoptosis and autophagy (Xu et al., 2020a). According to the results of in vitro research, Dioscin decreased the concentration of CHK2, cyclin B1, and CDK1 while increasing the expressions of cyclinD1, Bak, Cyt-c, caspase-3, caspase-9 in the HepG2 cells but decreasing those of bcl-2, bcl-xl and P70S6K (Zhang Y. S. et al., 2018). In summary, saponins play essential roles in anti-tumor activity by regulating the PI3K-Akt-mTOR signal transduction pathway (Figure 3B).
FIGURE 3. Signaling pathway of Chinese materia medica saponins in anti-cancer effect. (A) Wnt-β-catenin; (B) PI3Ks-Akt-mTOR; (C) p53; (D) JAK-STAT3; (E) NF-κB; (F) MAPK; (G) APMK and (H) EGFR signal transduction pathway.
The wingless-related integration site (Wnt) signaling pathway is an important intracellular signaling cascade with a regulatory role in cell proliferation, apoptosis, and differentiation in tissues and organs, and many diseases occur due to mutations or dysregulation in the Wnt signaling pathway. The widespread potential of the Wnt pathway in cancer has been reported (Duchartre et al., 2016). The Wnt signaling pathway is divided into classical (β-catenin dependent) and non-classical (β-catenin non-dependent) forms, which are key pathways that control developmental processes and histomorphogenesis (Koni et al., 2020). The dysregulation of the classical Wnt/β-catenin signaling pathway is involved in the pathological processes of many types of cancer (Zhang et al., 2018a; Cao et al., 2018; Spaan et al., 2018). β-Catenin is a switch in the Wnt/β-catenin signaling pathway, and phosphorylation of β-catenin is influenced by glycogen synthase kinase 3β (GSK3β) and casein kinase 1α (CK1α) (Nusse and Clevers., 2017). When pathway activation or degradation complexes are abnormal, β-catenin fails to phosphorylate or degrade and accumulates in the nucleus. Further, excessive concentrations of β-catenin bind to T-cell transcription factor/lymphocyte enhancer factor (TcF/LEF) and form complexes that activate the downstream target genes (cyclin D1, CDKN1A) and promote tumorigenesis and development (Zhang and Wang, 2020).
Triterpenoid saponins, like Raddeanin A (RA), inhibit proliferation and induce apoptosis in the CRC model both in vivo and in vitro. RA reduces the expression of β-catenin in the nucleus and cytoplasm along with the levels of Bcl-2, c-myc, p-GSK-3β, and cyclin D1 but promotes the expression of Bax (Wang et al., 2018b). Compound 1C is a modified version of AD-2, which is a ginsenoside isolated from P. ginseng. In LNCaP prostate cancer cells, 1C upregulates the expression of p53 but downregulated those of β-catenin, TCF-4 protein, CCND1, and C-myc (Wang et al., 2018c). In vivo and in vitro studies indicate that ginsenosides from Korean Red ginseng decrease the expressions of LEF1, CMYC, and CCND1 by inhibiting the Wnt/β-catenin signaling pathway (Ham et al., 2019). Saponins can regulate Wnt/β-catenin signal transduction and exert anti-cancer effects (Figure 3A).
The nuclear factor kappa-B (NF-κB) signaling pathway is intricately and closely linked to other cellular signaling pathways, and pathway activation is an important factor in promoting tumorigenesis and progression. Extracellular stimuli (bacteria, viruses, oncogenic molecules, etc.) can activate NF-κB. The NF-κB signaling pathway is involved in several functions related to proliferation, metastasis, and angiogenesis, which are required for cancer (Dimitrakopoulos et al., 2020). Studies have confirmed that NF-κB is a transcription factor with oncologic therapeutic potential and has been recognized for its important role in colorectal cancer (Plewka et al., 2018), gynecologic cancers (Harrington and Annunziata., 2019; Diéguez-Martinez et al., 2022), pancreatic cancer (Geismann et al., 2019), and other types of cancer. NF-κB is also an important linker between chronic inflammation and cancer and regulates the expression of a wide range of genes associated with immune and inflammatory responses. It importantly contributes to the pathogenesis of inflammation-driven diseases (Chauhan et al., 2022). NF-κB includes classical as well as non-classical pathways, comprising bridging molecules for receptor and receptor proximal signaling, the IκB kinase complex, IκB proteins (IκBα, IκBβ, IκBε, IκBγ, IκBζ, Bcl-3, p100, and p105) and NF-κB dimers (Colomer et al., 2017). NF-κB signaling plays a role in reducing cancer cell proliferation and metastases and promoting apoptosis in the pathological process of tumors by downregulating downstream genes (Soleimani et al., 2020).
The involvement of saponins in antitumor signaling contributes to a better understanding of their role in the regulation of NF-kB (Figure 3E). CBS is a triterpenoid saponin extracted from the Chinese medicinal material, Conyza blinii H. lev. In xenografted animal models of tumor and HeLa cells, CBS downregulated the expressions of nuclear-translocated p65 and molecules downstream of NF-κB (XIAP, Bcl-xL, MMP-2, MMP-9, COX-2, and cyclin D1) by inhibiting the NF-κB signaling pathway (Ma et al., 2017). RA, a triterpene saponin of Anemone raddeana Regel, possesses potent anti-tumor properties. Both in vivo and in vitro research shows that RA reduces the levels of p-IκBα, p65, MMP-2, and MMP-9 by inhibiting ROS/JNK and NF-κB signaling pathways (Ma et al., 2018). Saponins of Patrinia villosa decrease the levels of E-cadherin, N-cadherin, and NF-KBp65 in the CRC EMT model by inhibiting the NF-κB signaling pathway (Xia et al., 2018).
Paris polyphylla is usually used as a heat-clearing and detoxicating agent in traditional Chinese medicine (Tu et al., 2016; Guo et al., 2019) and Polyphyllin VII is one of the primary natural steroidal saponins in it. In vitro research indicates that Polyphyllin VII promotes the expressions of caspase-3, poly-(ADP-ribose) polymerase cleavage but suppresses those of p65, PI3K, (P)-PI3K, AKT, P-AKT, NF-κB, and P-NF-κB and inhibitor of caspase-activated DNase by attenuating the PI3K/Akt and NF-κB signaling pathways (He et al., 2020). Similarly, an in vitro experiment suggested that Paris Saponin II lowered the expressions of p65, c-myc, and cyclin D1 in HT 29 and HCT 116 cells by inhibiting the NF-κB signaling pathway, as evidenced by the suppression of IKKα phosphorylation and p65 nuclear translocation (Chen et al., 2019).
The epidermal growth factor receptor (EGFR) is a transmembrane receptor glycoprotein of the tyrosine kinase family. Many cell fate-specific activities are regulated by the EGFR cell signaling pathway, including cell growth, differentiation, metabolism, and proliferation (Kyriakopoulou et al., 2018). Mutations in components of the EGFR pathway are usually closely associated with several human malignancies (Isomoto et al., 2020), and EGFR signaling is upregulated in 20% of tumors (Kang et al., 2022), inducing proliferation and inhibiting apoptosis (Guan et al., 2017). The development of molecular agents targeting the EGFR pathway offers attractive avenues for anti-tumor effects, and the presently available EGFR tyrosine kinase inhibitors in widespread clinical use are gefitinib and erlotinib (Ayati et al., 2021). Both in vivo and in vitro experiments indicate that Ginsenoside Rg3 enhances the anti-cancer cell proliferation effect of erlotinib and erlotinib-induced apoptosis. Erlotinib/ginsenoside Rg3 treatment increases the protein levels of caspase-3, caspase-9, and PARP while decreasing those of p-EGFR, p-PI3K, and p-Akt in pancreatic cancer cell lines and BALB/c nu/nu male mice by inhibiting the EGFR/PI3K/Akt signaling pathway (Jiang et al., 2017a). Ziyuglycoside II (ZYG II) is an active ingredient in the treatment of digestive system cancers (HCC, CCA, EC, and PC), and functions by inducing cell cycle arrest and activation of mitochondria-dependent apoptosis. ZYG II inhibits EGFR and ERK1/2 protein phosphorylation while enhancing the expression of cleaved caspase-3 and cleaved PARP (Zhong et al., 2021). Moreover, in vitro research suggests that Saikosaponin-d reduces the levels of EGFR, p-EGFR, MEK, p-MEK, p38, and p-P38 but promotes that of p53 in human RCC cells (769-P and 786-O) through the inhibition of the EGFR/p38 signaling pathway (Cai et al., 2017) (Figure 3H).
The Janus kinase/signal transducer and activator of the transcription 3 (JAK/STAT3) signaling pathway has an important role in tumor behavior and function. STAT3 is a common signal transducer and activator of transcription that is involved in multiple signaling cascades. STAT3 deletion is a driver of tumor growth, and metastasis (Bharadwaj et al., 2020). STAT3 is one of the key oncogenes and therapeutic targets (Wang et al., 2021b; Chai et al., 2016). Recent evidence suggests that STAT3 is a regulatory node of cancer-related inflammation and a regulator of immune checkpoint proteins (Huynh et al., 2017). Autophagy and STAT3 pathways are two important directions in tumorigenesis and progression, and both have become research hotspots for tumor mechanisms in recent years (Hu et al., 2020; Jacquet et al., 2021). Autophagy, also known as type II programmed cell death, is a biological process important for maintaining tissue stability and metabolism. Abnormalities in autophagy are accompanied by alterations in STAT3 expression, and Xu et al. showed that STAT interacts with autophagy depending on several factors including phosphorylation sites, mode of action, and subcellular localization (Xu et al., 2022). STAT3 is a member of the STAT family and an important part of the JAK/STAT3 signaling pathway. Saponins were found to exert inhibitory effects on cell proliferation and induction of apoptosis through the modulation of the STAT3 pathway (Li et al., 2018; Li X et al., 2021; Zhou et al., 2019).
JAK/STAT3 is mainly divided into receptor tyrosine kinases (RTKs), JAKs (JAK1, JAK2, JAK3, and TYK2), and signal transducer and activator of transcription (STAT), which are activated by a variety of cytokines. Among them, the JAK2/STAT3 pathway is a component of JAK/STAT signaling that is upregulated in a variety of tumor cells and is particularly involved in the development of some solid tumors through the regulation of tumor cell proliferation and apoptosis (Jiang et al., 2016; Iriki et al., 2017; Wu et al., 2017). α-Hederin, a monodesmosidic triterpenoid saponin, is isolated from the leaves of Hedera helix and exerts potential anti-tumor effects in colon cancer. In vitro studies show that α-Hederin upregulates the expression of Ecapherin but downregulates those of IL-6-induced EMT markers (N-cadherin, vimentin, fibronectin, twist, and snail) by regulating the JAK2/STAT3 pathway to intervene metastasis in colon cancer (Sun et al., 2018).
Steroidal saponins, including DT-13, dioscin, and Ophiopogonin B show anti-metastasis and anti-proliferation effects, as do triterpenoid saponins. According to in vivo and in vitro studies, DT-13, isolated from the Dwarf lilyturf tuber, inhibits the phosphorylation levels of STAT3 and AKT in breast cancer cells (DA-MB-231 and MDA-MB-468) and reduces the expressions of the Procollagen-lysine, 2-oxoglutarate 5-dioxygenase 2 (PLOD2), and two receptors (Gp130 and OBR) by inhibiting the JAK/STAT3 and PI3K/AKT signaling pathways (He et al., 2019b). Moreover, both in vivo and in vitro experiments suggest that dioscin can upregulate the expressions of IL-4 and IL-10 by regulating the JAK2/STAT3 signaling pathway, thus inhibiting metastasis of B16 cells (Kou et al., 2017). Moreover, a previous study revealed that Ophiopogonin B could downregulate the protein expression of P-STAT3 by regulating the STAT3 signaling pathway, thus inducing apoptosis and affecting the cell cycle in SKOV3 and A2780 cells (Yuan et al., 2022). Steroidal saponins, the main active ingredient in Rhizoma Paris, are mainly composed of four kinds of saponins (Paris saponin I, II, VI, and VII). It is widely applied to treat tumors in China (He et al., 2019c). Paris saponin I can lower the levels of MMP-2, p-JAK2, and STAT3 in HUVEC cells (Wang et al., 2018a) (Figure 3D).
The mitogen-activated protein kinase (MAPK) signaling pathway can affect several different biological processes in cancer, including proliferation, differentiation, apoptosis, inflammation, and immunity (Wang et al., 2021c; An et al., 2020; Zhu et al., 2020; Lu et al., 2019), and plays a non-negligible role in the development of tumors. MAPK cell signaling mainly regulates the response of tumor cells to many internal and external stimuli (Anjum et al., 2022) In several malignancies, saponins regulate the MAPK signaling pathway through the induction of apoptosis and autophagic responses, thus exerting anticancer effects. Momordin Ic, a principal triterpene saponin constituent, isolated from Fructus Kochiae directly induces autophagy of hepatocellular carcinoma cells in vitro. Momordin Ic increases Beclin1 and LC-3 protein expressions in HepG2 cells by activating ROS-mediated JNK and p38 signaling pathways and regulating the ERK signaling pathway (Mi et al., 2016). Si et al. conducted in vitro experiments using human laryngeal squamous cell carcinoma cells, Hep-2 and TU212, and demonstrated that dioscin could exert potential anti-migration and anti-invasion, cell cycle arrest, and pro-apoptosis effects by significantly downregulating the protein and mRNA levels of cyclina, CDK2, Bcl-2, MMP2, and MMP9 while upregulating those of p53, Bax, Cyto-c, and caspase-3, caspase-9, p-JNK, and p-p38 by targeting the MAPK signaling pathway (Si et al., 2016). Treatment with water-soluble Astragaloside IV (AS-IV, 20 mg/kg) prepared from the roots of Astragalus membranaceus can inhibit proliferation and invasion in vitro. Furthermore, AS-IV reduces the expressions of MMP-2/9 and VAV3 in MDA-MB-231 cells by regulating the MAPK pathway, thereby downregulating tumor cell viability and growth, which is a potential strategy for treating metastatic breast cancer (Jiang et al., 2017b). Similar observations were reported in U251 cells and tumor-bearing athymic BALB/c mice. AS-IV upregulates the levels of Ki67, MMP-2, and MMP-9 by regulating the MAPK/ERK signaling pathway, which further results in the suppression of tumor cell growth, migration, and invasion abilities (Li et al., 2017).
According to their biological functions, the MAPK family mainly includes extracellular signal-regulated kinase (ERK), p38 mitogen-activated protein kinase, and c-Jun amino-terminal kinase 1/2/3 (JNK1/2/3), and these are involved in carcinogenesis (Lei et al., 2020; Kumar et al., 2020). ERK promotes cell proliferation and is involved in apoptosis and differentiation (Xu et al., 2019). JNK can be phosphorylated and can activate several proteins (c-Myc, p53, Bcl-2 family in the mitochondria of cell death regulators, etc.), and these nuclear and non-nuclear proteins regulate many cellular responses including cellular proliferation, differentiation, and apoptosis (Bubici and Papa., 2014). p38/MAPK is a stress protein kinase with core components α, β, γ, and δ. Stress stimuli, pathogens, or pro-inflammatory factors can activate the phosphorylation of p38/MAPK, which is involved in tumor development and has key regulatory roles in eliciting apoptosis, immune responses, and inflammatory responses (Martínez-Limón et al., 2020). The three intracellular MAPK signaling pathways interact with each other to produce biological effects. Triterpenoid saponins, such as CalundulosideE (Wang et al., 2021d), platycodinD (Lei et al., 2022), and Ginsenoside Rh4 (Wu et al., 2018), and steroidal saponins, like Dioscin (Wang et al., 2014) and Polyphyllin D (Liu et al., 2022b) exert anti-tumor effects by regulating the MAPK signaling pathways (Figure 3F). (1) p38 MAPK: RLTS can activate p38 MAPK and downregulate the protein expressions of CXCR4, MMP2, and MMP9, suppress cell migration, induce apoptosis, and inhibit the proliferation of cancer cells both in vivo and in vitro (Zhan et al., 2016). Paris Saponin I (PSI) significantly reduces the levels of Bcl-2 and Bcl-xl but promotes those of p-p38 MAPK, Cyto-c, caspase-9, and caspase-3 in lung cancer cells (Liu et al., 2017). (2) ERK1/2: Timosaponin A3 decreased the levels of MMP-9, Bcl-2, Bcl-xl, and VEGF-1 (in pancreatic cancer AsPC-1 cells) by inhibiting the ERK1/2 pathway (Kim et al., 2019). (3) JNK: Protodioscin regulates the key extrinsic apoptotic pathway molecules, including Bcl-2, caspases-8/3/9, -PARP, and Bax (Lin et al., 2018). Protodioscin promotes the expression of cleaved-PARP and cleaved-caspase 3 while decreasing E-cadherin levels (Chen et al., 2022). Moreover, T-17 decreased P62 expression but increased those of P21 and Beclin-1 by regulating the JNK signaling pathway (Xu et al., 2020b).
AMP-activated protein kinase (AMPK) is a sensor of energy status and regulator of metabolism in eukaryotic cells and comprises three subunits, namely, α, β, and γ. AMPK-triggered energy imbalance can cause the development of several diseases, including diabetes, inflammation, obesity, and cancer (Paskeh et al., 2022). Among them, AMPK regulates cancer cell metabolism and is a promising anti-cancer target (Samarghandian et al., 2016). Activation of AMPK signaling is associated with an increase in AMP: ATP and ADP: ATP ratios, thus driving the stimulation of AMPK by upstream molecules (LKB1 and CAMKK) (Ashrafizadeh et al., 2021). APMK is activated in the phosphorylated state and its activation is strongly associated with improved survival of patients with breast (Henry et al., 2017), bladder (Tao et al., 2017), and colon (Wei et al., 2016) cancers. Phosphorylated AMPK activates TSC1/2 proteins, thereby inhibiting mTOR kinase activity (Bai et al., 2017). mTOR is a regulator associated with autophagy, and saponins reportedly target autophagy through the AMPK/mTOR signaling pathway to slow the malignant progression of cancer. PGB shows a noticeable pro-autophagy effect on A549 human lung carcinoma cells in vitro. Studies have revealed that PGB-activated AMPK phosphorylation inhibits mTOR and AKT activities. Furthermore, PGB increases the levels of lC3-II, Beclin-1, and Bax but decreased those of the mTOR complex (Raptor and Rictor) and Bcl-2 (Yim et al., 2016).
GIT, an important component of Tribulus longipetalus, belongs to a family of steroidal saponins. Both in vivo and in vitro experiments indicate that GIT promotes Bax expression, activities of cleaved Caspase-3 and PARP, and enhances LC3II and P-AMPK expressions in lung cancer cells through the AMPK and AKT signaling pathways, thereby initiating autophagy. Thus, it is a potential apoptosis and autophagy inducer (Liu et al., 2022c). Moreover, DT-13 activates AMPK phosphorylation and reduces the expressions of p-mTOR, p-p70S6K, GLUT1, and p-4EBP1 in the HCT-15 and HT-29 cells (Wei et al., 2019). Tetracyclic triterpenoid AS-IV exerts anti-tumor effects by reducing tumor cell growth, invasion, migration, and angiogenesis. An in vivo study showed that AS-IV inhibited the levels of M2 surface marker (CD206) and macrophage markers (PPARγ and Arg-1), MMP9, MMP10, and MMP14. It also significantly downregulated the levels of IL-10 and TGF-β by targeting the AMPK signaling pathway (Xu et al., 2018) (Figure 3G).
The p53 tumor suppressor is a transcription factor that induces apoptosis. When Afrocyclamin A was added to human prostate cancer cells, the expressions of p53, p21, and Bax were found to increase. The results showed that Afrocyclamin A could effectively induce apoptosis (Sachan et al., 2018). The treatment of HCC cells with dioscin showed that the expressions of P53 and P21 increased while those of CHK2, cyclin B1, and CDK1 were inhibited. Dioscin could effectively block the cell cycle in the G2/M phase through the p53 signaling pathway (Zhang et al., 2018a). These results show that saponins are engaged in the p53 signaling pathway (Figure 3C).
In summary, saponins play essential roles in anti-tumor effects through the regulation of NF-κB, PI3Ks-Akt-mTOR, MAPK, Wnt-β-catenin, JAK-STAT3, APMK, p53, and EGFR signal transduction pathways (Figure 3). The saponins summarized in this review are mainly divided into monomer saponins and total saponin extracts as shown in Tables 1, 2.
TABLE 1. Monomer saponins against cerebral ischemia-reperfusion injury. Up arrows indicate upregulation, while the down arrows indicate downregulation.
TABLE 2. Total saponin extracts against cancer. Up arrows indicate upregulation, while the down arrows indicate downregulation.
Saponins are the main active ingredients of many herbal plants and have a wide range of biological effects. Several extraction methods have been employed to obtain saponins, which are mainly divided into two categories, namely, traditional extraction and green extraction processes (Figure 4). The choice of either method depends mainly on the focus of the study; for saponin isolation and pharmacological activity studies, traditional methods are preferred, while for quantitative and optimization studies, green techniques are employed (Choon et al., 2014). Traditional extraction methods include maceration, soxhlet extraction, and reflux extraction (Ramli et al., 2019). These are dependent on solubility and require high solvent and time depletion (Yusoff et al., 2022), and therefore, have prompted scientific researchers to invent green extraction methods such as ultrasound-assisted, microwave-assisted, and accelerated solvent extraction methods. Ultrasound reportedly increases the solubility of saponins and improves their bioavailability (Navarro Del Hierro et al., 2018b). Ultrasound-assisted extraction has characteristics of less solvent requirement, shorter time, and higher extraction yields, making it a promising alternative method (Fu et al., 2021). With the advancement of scientific research, the exploration of saponin-like components in herbal medicines has increased, the perception of saponins has been redefined to some extent, and their development and application value have been improved.
Cancer is a complex pathological process with multifactorial involvement and multiple implicated pathways. The activation or inhibition of many cell signal transduction pathways drives the genesis and development of tumors, and saponins derived from CHM interact with them to inhibit cancer. An ongoing understanding of the role of saponins on signaling pathways involved in cancer development and progression will help us fight cancer in a more precise manner. Therefore, the development of saponins to act against different targets of cancer is of extreme importance. This review summarizes the classification, composition, structure, and extraction methods of saponins. Moreover, the function of saponins in regulating NF-κB, PI3Ks-Akt-mTOR, MAPK, Wnt-β-catenin, JAK-STAT3, APMK, p53, and EGFR signaling pathways are summarized, which may be useful for broadening their anti-cancer activity spectrum. This is expected to enhance researchers’ understanding of the anti-tumor effects of herbal saponins to a certain extent.
Saponins of CHM are a large class of ingredients with low side effects, low cost, easy availability, and significant antitumor activity, and are new antitumor agents worthy of further research and development. This review provides good evidence for its potential application in tumor treatment and the improvement of related diseases. However, existing studies still have some limitations. First, most of the research on Chinese herbal saponins is limited to in vitro cell-based or animal experiments, and there is a lack of relevant scientific and standardized clinical experimental studies. The effectiveness and safety of saponins for humans need to be verified. Therefore, in future investigations, clinical studies on the involvement of saponins in cancer prevention and treatment through multiple cell signaling pathways should be conducted to provide a more reliable theoretical basis for their clinical promotion. Second, clinical antitumor drugs are often combined, and in the studies summarized herein, we found that saponins had outstanding anticancer effects when combined with other drugs (Erlotinib/Ginsenoside Rg3, Jiang et al., 2017b), so synergistic effects of between saponins and other components and the mechanisms that regulate the relevant cell signaling pathways warrant further investigation. Saponins are characterized by low bioavailability and corresponding limitations in clinical application, which may be aided by chemical modification and artificial synthesis, as mentioned above (Compound 1C); therefore, in future research, combining modern technical means and existing research results, multidisciplinary crossover in-depth study of the anti-cancer mechanism of saponins, and development of prominent efficacy and clearer action targets of anti-tumor drugs should be explored. The depth and breadth of research on the treatment of cancer by Chinese herbal saponins should be increased in subsequent studies to reflect their role and value in the prevention and treatment of cancer and better promote the development and utilization of these CHM resources.
Writing, manuscript preparation, and overall conception: MZ; Project administration, Funding acquisition: QW and HK; Supervision, resources, and analysis: YS, HB, YW, and BY All authors read and approved the final manuscript.
This work was supported by the Chief Scientist of Qi-Huang Project of National Traditional Chinese Medicine Inheritance and Innovation “One Hundred Million” Talent Project [Grant Number: (2021) No. 7]. Qi-Huang Scholar of National Traditional Chinese Medicine Leading Talents Support Program [Grant Number: (2018) No. 284]. National Famous Old Traditional Chinese Medicine Experts Inheritance Studio Construction Program of National Administration of TCM [Grant Number: (2022) No. 75]. The Seventh Batch of National Famous Old Traditional Chinese Medicine Experts Experience Heritage Construction Program of National Administration of TCM [Grant Number: (2022) No.76]. Heilongjiang Touyan Innovation Team Program [Grant Number: (2019) No. 5].
The authors declare that the research was conducted in the absence of any commercial or financial relationships that could be construed as a potential conflict of interest.
All claims expressed in this article are solely those of the authors and do not necessarily represent those of their affiliated organizations, or those of the publisher, the editors and the reviewers. Any product that may be evaluated in this article, or claim that may be made by its manufacturer, is not guaranteed or endorsed by the publisher.
An, L., Zhao, J., Sun, X., Zhou, Y., and Zhao, Z. (2020). S-allylmercaptocysteine inhibits mucin overexpression and inflammation via MAPKs and PI3K-Akt signaling pathways in acute respiratory distress syndrome. Pharmacol. Res. 159, 105032. doi:10.1016/j.phrs.2020.105032
Anjum, J., Mitra, S., Das, R., Alam, R., Mojumder, A., Emran, T. B., et al. (2022). A renewed concept on the MAPK signaling pathway in cancers: Polyphenols as a choice of therapeutics. Pharmacol. Res. 184, 106398. doi:10.1016/j.phrs.2022.106398
Arnold, M., Morgan, E., Rumgay, H., Mafra, A., Singh, D., Laversanne, M., et al. (2022). Current and future burden of breast cancer: Global statistics for 2020 and 2040. Breastedinbg. Scotl. 66, 15–23. doi:10.1016/j.breast.2022.08.010
Ashrafizadeh, M., Mirzaei, S., Hushmandi, K., Rahmanian, V., Zabolian, A., Raei, M., et al. (2021). Therapeutic potential of AMPK signaling targeting in lung cancer: Advances, challenges and future prospects. Life Sci. 278, 119649. doi:10.1016/j.lfs.2021.119649
Augustin, J. M., Kuzina, V., Andersen, S. B., and Bak, S. (2011). Molecular activities, biosynthesis and evolution of triterpenoid saponins. Phytochemistry 72 (6), 435–457. doi:10.1016/j.phytochem.2011.01.015
Ayati, A., Moghimi, S., Toolabi, M., and Foroumadi, A. (2021). Pyrimidine-based EGFR TK inhibitors in targeted cancer therapy. Eur. J. Med. Chem. 221, 113523. doi:10.1016/j.ejmech.2021.113523
Bai, L., Mei, X., Shen, Z., Bi, Y., Yuan, Y., Guo, Z., et al. (2017). Netrin-1 improves functional recovery through autophagy regulation by activating the AMPK/mTOR signaling pathway in rats with spinal cord injury. Sci. Rep. 7, 42288. doi:10.1038/srep42288
Baky, M. H., Elsaid, M. B., and Farag, M. A. (2022). Phytochemical and biological diversity of triterpenoid saponins from family sapotaceae: A comprehensive review. Phytochemistry 202, 113345. doi:10.1016/j.phytochem.2022.113345
Bharadwaj, U., Kasembeli, M. M., Robinson, P., and Tweardy, D. J ( (2020). Targeting Janus kinases and signal transducer and activator of transcription 3 to treat inflammation, fibrosis, and cancer: Rationale, progress, and caution. Pharmacol. Rev. 72 (2), 486–526. doi:10.1124/pr.119.018440
Bhardwaj, J., Chaudhary, N., Seo, H. J., Kim, M. Y., Shin, T. S., and Kim, J. D. (2014). Immunomodulatory effect of tea saponin in immune T-cells and T-lymphoma cells via regulation of Th1, Th2 immune response and MAPK/ERK2 signaling pathway. Immunopharmacol. Immunotoxicol. 36 (3), 202–210. doi:10.3109/08923973.2014.909849
Bubici, C., and Papa, S. (2014). JNK signalling in cancer: In need of new, smarter therapeutic targets. Br. J. Pharmacol. 171 (1), 24–37. doi:10.1111/bph.12432
Cai, C., Zhang, H., Ou, Y., Jiang, Y., Zhong, D., Qi, H., et al. (2017). Saikosaponin-d suppresses cell growth in renal cell carcinoma through EGFR/p38 signaling pathway. Neoplasma 64 (4), 518–525. doi:10.4149/neo_2017_405
Cao, M. Q., You, A. B., Zhu, X. D., Zhang, W., Zhang, Y. Y., Zhang, S. Z., et al. (2018). miR-182-5p promotes hepatocellular carcinoma progression by repressing FOXO3a. J. Hematol. Oncol. 11 (1), 12. doi:10.1186/s13045-018-0555-y
Chai, E. Z., Shanmugam, M. K., Arfuso, F., Dharmarajan, A., Wang, C., Kumar, A. P., et al. (2016). Targeting transcription factor STAT3 for cancer prevention and therapy. Pharmacol. Ther. 162, 86–97. doi:10.1016/j.pharmthera.2015.10.004
Chauhan, A., Islam, A. U., Prakash, H., and Singh, S. (2022). Phytochemicals targeting NF-κB signaling: Potential anti-cancer interventions. J. Pharm. analysis 12 (3), 394–405. doi:10.1016/j.jpha.2021.07.002
Chen, J., Yuan, J., Zhou, L., Zhu, M., Shi, Z., Song, J., et al. (2017). Regulation of different components from Ophiopogon japonicus on autophagy in human lung adenocarcinoma A549Cells through PI3K/Akt/mTOR signaling pathway. Biomed. Pharmacother. 87, 118–126. doi:10.1016/j.biopha.2016.12.093
Chen, M. Y., Shao, L., Zhang, W., Wang, C. Z., Zhou, H. H., Huang, W. H., et al. (2018b). Metabolic analysis of Panax notoginseng saponins with gut microbiota-mediated biotransformation by HPLC-DAD-Q-TOF-MS/MS. J. Pharm. Biomed. analysis 150, 199–207. doi:10.1016/j.jpba.2017.12.011
Chen, M., Ye, K., Zhang, B., Xin, Q., Li, P., Kong, A. N., et al. (2019). Paris Saponin II inhibits colorectal carcinogenesis by regulating mitochondrial fission and NF-κB pathway. Pharmacol. Res. 139, 273–285. doi:10.1016/j.phrs.2018.11.029
Chen, T., Li, B., Qiu, Y., Qiu, Z., and Qu, P. (2018a). Functional mechanism of Ginsenosides on tumor growth and metastasis. Saudi J. Biol. Sci. 25 (5), 917–922. doi:10.1016/j.sjbs.2018.01.012
Chen, Y. R., Wang, S. C., Huang, S. P., Su, C. C., Liu, P. L., Cheng, W. C., et al. (2022). Protodioscin inhibits bladder cancer cell migration and growth, and promotes apoptosis through activating JNK and p38 signaling pathways. Biomed. Pharmacother. = Biomedecine Pharmacother. 156, 113929. doi:10.1016/j.biopha.2022.113929
Cheng, T., and Ying, M. (2021). Antitumor effect of Saikosaponin A on human neuroblastoma cells. BioMed Res. Int. 2021, 5845554. doi:10.1155/2021/5845554
Cho, Y. J., Woo, J. H., Lee, J. S., Jang, D. S., Lee, K. T., and Choi, J. H. (2016). Eclalbasaponin II induces autophagic and apoptotic cell death in human ovarian cancer cells. J. Pharmacol. Sci. 132 (1), 6–14. doi:10.1016/j.jphs.2016.02.006
Choon, Y. C., Salman, H. A. K., and Sulaiman, R. (2014). Extraction and quantification of saponins: A review. Food Res. Int. 59, 16–40. doi:10.1016/j.foodres.2014.01.057
Chwalek, M., Lalun, N., Bobichon, H., Plé, K., and Voutquenne-Nazabadioko, L. (2006). Structure-activity relationships of some hederagenin diglycosides: Haemolysis, cytotoxicity and apoptosis induction. Biochimica biophysica acta 1760 (9), 1418–1427. doi:10.1016/j.bbagen.2006.05.004
Colomer, C., Marruecos, L., Vert, A., Bigas, A., and Espinosa, L. (2017). NF-κB members left home: NF-κB-Independent roles in cancer. Biomedicines 5 (2), 26. null. doi:10.3390/biomedicines5020026
Diéguez-Martínez, N., Espinosa-Gil, S., Yoldi, G., Megías-Roda, E., Bolinaga-Ayala, I., Viñas-Casas, M., et al. (2022). The ERK5/NF-κB signaling pathway targets endometrial cancer proliferation and survival. Cell. Mol. life Sci. CMLS 79 (10), 524. doi:10.1007/s00018-022-04541-6
Dimitrakopoulos, F. D., Kottorou, A. E., Kalofonou, M., and Kalofonos, H. P ( (2020). The fire within: NF-κB involvement in non-small cell lung cancer. Cancer Res. 80 (19), 4025–4036. doi:10.1158/0008-5472.CAN-19-3578
Dong, J., Liang, W., Wang, T., Sui, J., Wang, J., Deng, Z., et al. (2019). Saponins regulate intestinal inflammation in colon cancer and IBD. Pharmacol. Res. 144, 66–72. doi:10.1016/j.phrs.2019.04.010
Du, J., Song, D., Cao, T., Li, Y., Liu, J., Li, B., et al. (2021). Saikosaponin-A induces apoptosis of cervical cancer through mitochondria- and endoplasmic reticulum stress-dependent pathway in vitro and in vivo: Involvement of PI3K/AKT signaling pathway. Cell cycleGeorget. Tex.) 20 (21), 2221–2232. doi:10.1080/15384101.2021.1974791
Duchartre, Y., Kim, Y. M., and Kahn, M. (2016). The Wnt signaling pathway in cancer. Crit. Rev. oncology/hematology 99, 141–149. doi:10.1016/j.critrevonc.2015.12.005
Ediriweera, M. K., Tennekoon, K. H., and Samarakoon, S. R. (2019). Role of the PI3K/AKT/mTOR signaling pathway in ovarian cancer: Biological and therapeutic significance. Seminars cancer Biol. 59, 147–160. doi:10.1016/j.semcancer.2019.05.012
Fakhri, S., Iranpanah, A., Gravandi, M. M., Moradi, S. Z., Ranjbari, M., Majnooni, M. B., et al. (2021). Natural products attenuate PI3K/Akt/mTOR signaling pathway: A promising strategy in regulating neurodegeneration. Phytomedicine Int. J. phytotherapy Phytopharm. 91, 153664. doi:10.1016/j.phymed.2021.153664
Fu, D., Hu, Z., Xu, X., Dai, X., and Liu, Z. (2022). Key signal transduction pathways and crosstalk in cancer: Biological and therapeutic opportunities. Transl. Oncol. 26, 101510. doi:10.1016/j.tranon.2022.101510
Fu, X., Wang, D., Belwal, T., Xie, J., Xu, Y., Zou, L., et al. (2021). Natural deep eutectic solvent enhanced pulse-ultrasonication assisted extraction as a multi-stability protective and efficient green strategy to extract anthocyanin from blueberry pomace. LWT - Food Sci. Technol. 144, 111220. doi:10.1016/j.lwt.2021.111220
Fuchs, H., Niesler, N., Trautner, A., Sama, S., Jerz, G., Panjideh, H., et al. (2017). Glycosylated triterpenoids as endosomal escape enhancers in targeted tumor therapies. Biomedicines 5 (2), 14. doi:10.3390/biomedicines5020014
Geismann, C., Schäfer, H., Gundlach, J. P., Hauser, C., Egberts, J. H., Schneider, G., et al. (2019). NF-κB dependent chemokine signaling in pancreatic cancer. Cancers 11 (10), 1445. doi:10.3390/cancers11101445)
Georgieva, A., Popov, G., Shkondrov, A., Toshkova, R., Krasteva, I., Kondeva-Burdina, M., et al. (2021). Antiproliferative and antitumour activity of saponins from Astragalus glycyphyllos on myeloid Graffi tumour. J. Ethnopharmacol. 267, 113519. doi:10.1016/j.jep.2020.113519
Guan, H., Du, Y., Ning, Y., and Cao, X. (2017). A brief perspective of drug resistance toward EGFR inhibitors: The crystal structures of EGFRs and their variants. Future Med. Chem. 9 (7), 693–704. doi:10.4155/fmc-2016-0222
Guo, J., Gao, Y., Wang, Y., Liu, W. J., Zhou, J., and Wang, Z. (2019). Application of herbal medicines with heat-clearing property to anti-microinflammation in the treatment of diabetic kidney disease. Evidence-based complementary Altern. Med. 2019, 6174350. doi:10.1155/2019/6174350
Ham, S. W., Kim, J. K., Jeon, H. Y., Kim, E. J., Jin, X., Eun, K., et al. (2019). Korean Red ginseng extract inhibits glioblastoma propagation by blocking the Wnt signaling pathway. J. Ethnopharmacol. 236, 393–400. doi:10.1016/j.jep.2019.03.031
Han, B., and He, C. (2021). Targeting autophagy using saponins as a therapeutic and preventive strategy against human diseases. Pharmacol. Res. 166, 105428. doi:10.1016/j.phrs.2021.105428
Harrington, B. S., and Annunziata, C. M. (2019). NF-κB signaling in ovarian cancer. Cancers 11 (8), 1182. null. doi:10.3390/cancers11081182
He, H., Xu, C., Zheng, L., Wang, K., Jin, M., Sun, Y., et al. (2020). Polyphyllin VII induces apoptotic cell death via inhibition of the PI3K/Akt and NF-κB pathways in A549 human lung cancer cells. Mol. Med. Rep. 21 (2), 597–606. doi:10.3892/mmr.2019.10879
He, J., Wei, X., Li, S., Quan, X., Li, R., Du, H., et al. (2019b). DT-13 suppresses breast cancer metastasis by modulating PLOD2 in the adipocytes microenvironment. Phytomedicine Int. J. phytotherapy Phytopharm. 59, 152778. doi:10.1016/j.phymed.2018.12.001
He, J., Yu, S., Guo, C., Tan, L., Song, X., Wang, M., et al. (2019c). Polyphyllin I induces autophagy and cell cycle arrest via inhibiting PDK1/Akt/mTOR signal and downregulating cyclin B1 in human gastric carcinoma HGC-27 cells. Biomed. Pharmacother. = Biomedecine Pharmacother. 117, 109189. doi:10.1016/j.biopha.2019.109189
He, Y., Hu, Z., Li, A., Zhu, Z., Yang, N., Ying, Z., et al. (2019a). Recent advances in biotransformation of saponins. Mol. (Basel, Switz. 24 (13), 2365. null. doi:10.3390/molecules24132365
Henry, W. S., Laszewski, T., Tsang, T., Beca, F., Beck, A. H., McAllister, S. S., et al. (2017). Aspirin suppresses growth in PI3K-mutant breast cancer by activating AMPK and inhibiting mTORC1 signaling. Cancer Res. 77 (3), 790–801. doi:10.1158/0008-5472.CAN-16-2400
Hu, H., Zhang, Q., Chen, W., Wu, T., Liu, S., Li, X., et al. (2020). MicroRNA-301a promotes pancreatic cancer invasion and metastasis through the JAK/STAT3 signaling pathway by targeting SOCS5. Carcinogenesis 41 (4), 502–514. doi:10.1093/carcin/bgz121
Huynh, J., Etemadi, N., Hollande, F., Ernst, M., and Buchert, M. (2017). The JAK/STAT3 axis: A comprehensive drug target for solid malignancies. Seminars cancer Biol. 45, 13–22. doi:10.1016/j.semcancer.2017.06.001
Iriki, T., Ohnishi, K., Fujiwara, Y., Horlad, H., Saito, Y., Pan, C., et al. (2017). The cell-cell interaction between tumor-associated macrophages and small cell lung cancer cells is involved in tumor progression via STAT3 activation. Lung cancer 106, 22–32. doi:10.1016/j.lungcan.2017.01.003
Isomoto, K., Haratani, K., Hayashi, H., Shimizu, S., Tomida, S., Niwa, T., et al. (2020). Impact of EGFR-TKI treatment on the tumor immune microenvironment in EGFR mutation-positive non-small cell lung cancer. Clin. cancer Res. official J. Am. Assoc. Cancer Res. 26 (8), 2037–2046. doi:10.1158/1078-0432.CCR-19-2027
Jacquet, M., Guittaut, M., Fraichard, A., and Despouy, G. (2021). The functions of atg8-family proteins in autophagy and cancer: Linked or unrelated? Autophagy 17 (3), 599–611. doi:10.1080/15548627.2020.1749367
Jia, M. M., Li, Y. Q., Xu, K. Q., Zhang, Y. Y., Tan, S. M., Zhang, Q., et al. (2020). Jujuboside B promotes the death of acute leukemia cell in a RIPK1/RIPK3/MLKL pathway-dependent manner. Eur. J. Pharmacol. 876, 173041. doi:10.1016/j.ejphar.2020.173041
Jiang, J., Liu, Y., Tang, Y., Li, L., Zeng, R., Zeng, S., et al. (2016). ALDH1A1 induces resistance to CHOP in diffuse large B-cell lymphoma through activation of the JAK2/STAT3 pathway. OncoTargets Ther. 9, 5349–5360. doi:10.2147/OTT.S107957
Jiang, J., Yuan, Z., Sun, Y., Bu, Y., Li, W., and Fei, Z. (2017a). Ginsenoside Rg3 enhances the anti-proliferative activity of erlotinib in pancreatic cancer cell lines by downregulation of EGFR/PI3K/Akt signaling pathway. Biomed. Pharmacother. 96, 619–625. doi:10.1016/j.biopha.2017.10.043
Jiang, K., Lu, Q., Li, Q., Ji, Y., Chen, W., and Xue, X. (2017b). Astragaloside IV inhibits breast cancer cell invasion by suppressing Vav3 mediated Rac1/MAPK signaling. Int. Immunopharmacol. 42, 195–202. doi:10.1016/j.intimp.2016.10.001
Kang, J. J., Ko, A., Kil, S. H., Mallen-St Clair, J., Shin, D. S., Wang, M. B., et al. (2022). EGFR pathway targeting drugs in head and neck cancer in the era of immunotherapy. Rev. cancer 1878 (1), 188827. doi:10.1016/j.bbcan.2022.188827
Khan, T., Date, A., Chawda, H., and Patel, K. (2019). Polysaccharides as potential anticancer agents-A review of their progress. Carbohydr. Polym. 210, 412–428. doi:10.1016/j.carbpol.2019.01.064
Kim, Y., Kim, K. H., Lee, I. S., Park, J. Y., Na, Y. C., Chung, W. S., et al. (2019). Apoptosis and G2/M cell cycle arrest induced by a timosaponin A3 from Anemarrhena asphodeloides Bunge on AsPC-1 pancreatic cancer cells. Phytomedicine Int. J. phytotherapy Phytopharm. 56, 48–56. doi:10.1016/j.phymed.2018.08.006
Kobayashi, Y., Lim, S. O., and Yamaguchi, H. (2020). Oncogenic signaling pathways associated with immune evasion and resistance to immune checkpoint inhibitors in cancer. Seminars cancer Biol. 65, 51–64. doi:10.1016/j.semcancer.2019.11.011
Kocarnik, J. M., Compton, K., Dean, F. E., Fu, W., Gaw, B. L., et al. (2022). Cancer incidence, mortality, years of life lost, years lived with disability, and disability-adjusted life years for 29 cancer groups from 2010 to 2019: A systematic analysis for the global burden of disease study 2019. JAMA Oncol. 8 (3), 420–444. doi:10.1001/jamaoncol.2021.6987
Koni, M., Pinnarò, V., and Brizzi, M. F ( (2020). The Wnt signalling pathway: A tailored target in cancer. Int. J. Mol. Sci. 21 (20), 7697. doi:10.3390/ijms21207697)
Kou, Y., Ji, L., Wang, H., Wang, W., Zheng, H., Zou, J., et al. (2017). Connexin 43 upregulation by dioscin inhibits melanoma progression via suppressing malignancy and inducing M1 polarization. Int. J. cancer 141 (8), 1690–1703. doi:10.1002/ijc.30872
Kumar, A., and Jaitak, V. (2019). Natural products as multidrug resistance modulators in cancer. Eur. J. Med. Chem. 176, 268–291. doi:10.1016/j.ejmech.2019.05.027
Kumar, S., Principe, D. R., Singh, S. K., Viswakarma, N., Sondarva, G., Rana, B., et al. (2020). Mitogen-activated protein kinase inhibitors and T-cell-dependent immunotherapy in cancer. Pharm. (Basel, Switz. 13 (1), 9. null. doi:10.3390/ph13010009
Kyriakopoulou, K., Kefali, E., Piperigkou, Z., Bassiony, H., and Karamanos, N. K. (2018). Advances in targeting epidermal growth factor receptor signaling pathway in mammary cancer. Cell. Signal. 51, 99–109. doi:10.1016/j.cellsig.2018.07.010
Lei, J., Fu, Y., Zhuang, Y., and Zhang, K. (2020). Sema4D aggravated LPS-induced injury via activation of the MAPK signaling pathway in ATDC5 chondrocytes. BioMed Res. Int. 2020, 8691534. doi:10.1155/2020/8691534
Lei, J., Zhao, J., Long, M. Y., Cao, X. W., and Wang, F. J. (2022). In addition to its endosomal escape effect, platycodin D also synergizes with ribosomal inactivation protein to induce apoptosis in hepatoma cells through AKT and MAPK signaling pathways. Chemico-biological Interact. 364, 110058. doi:10.1016/j.cbi.2022.110058
Li, B., Wang, F., Liu, N., Shen, W., and Huang, T. (2017). Astragaloside IV inhibits progression of glioma via blocking MAPK/ERK signaling pathway. Biochem. biophysical Res. Commun. 491 (1), 98–103. doi:10.1016/j.bbrc.2017.07.052
Li L, L., Chen, M., Li, G., and Cai, R. (2021). Raddeanin A induced apoptosis of non-small cell lung cancer cells by promoting ROS-mediated STAT3 inactivation. Tissue & Cell 71, 101577. doi:10.1016/j.tice.2021.101577
Li X, Xiaoling, Chen, M., Yao, Z., Du, H., Zhang, T., Wang, H., et al. (2021). Jujuboside B induces mitochondrial-dependent apoptosis in colorectal cancer through ROS-mediated PI3K/Akt pathway in vitro and in vivo. J. Funct. Foods 87, 104796. doi:10.1016/J.JFF.2021.104796
Li, Y. H., Zou, M., Han, Q., Deng, L. R., and Weinshilboum, R. M . (2020). Therapeutic potential of triterpenoid saponin anemoside B4 from Pulsatilla chinensis. Pharmacol. Res. 160, 105079. doi:10.1016/j.phrs.2020.105079
Li, Y., Ye, Y., and Chen, H. (2018). Astragaloside IV inhibits cell migration and viability of hepatocellular carcinoma cells via suppressing long noncoding RNA ATB. Biomed. Pharmacother. 99, 134–141. doi:10.1016/j.biopha.2017.12.108
Lin, C. L., Lee, C. H., Chen, C. M., Cheng, C. W., Chen, P. N., Ying, T. H., et al. (2018). Protodioscin induces apoptosis through ROS-mediated endoplasmic reticulum stress via the JNK/p38 activation pathways in human cervical cancer cells. Cell. physiology Biochem. Int. J. Exp. Cell. physiology, Biochem. Pharmacol. 46 (1), 322–334. doi:10.1159/000488433
Liu, H., Li, X., Duan, Y., Xie, J. B., and Piao, X. L ( (2021). Mechanism of gypenosides of Gynostemma pentaphyllum inducing apoptosis of renal cell carcinoma by PI3K/AKT/mTOR pathway. J. Ethnopharmacol. 271, 113907. doi:10.1016/j.jep.2021.113907
Liu, J., Liu, Y., Li, H., Wei, C., Mao, A., Liu, W., et al. (2022b). Polyphyllin D induces apoptosis and protective autophagy in breast cancer cells through JNK1-Bcl-2 pathway. J. Ethnopharmacol. 282, 114591. doi:10.1016/j.jep.2021.114591
Liu, J., Zhang, C., Jia, B., Dong, K., Li, M., Qiu, D., et al. (2022a). Panax notoginseng saponins induce apoptosis in retinoblastoma Y79 cells via the PI3K/AKT signalling pathway. Exp. eye Res. 216, 108954. doi:10.1016/j.exer.2022.108954
Liu, Q., Chen, W., Jiao, Y., Hou, J., Wu, Q., Liu, Y., et al. (2014). Pulsatilla saponin A, an active molecule from Pulsatilla chinensis, induces cancer cell death and inhibits tumor growth in mouse xenograft models. J. Surg. Res. 188 (2), 387–395. doi:10.1016/j.jss.2014.01.026
Liu, T., Li, Y., Sun, J., Tian, G., and Shi, Z. (2022c). Gitogenin suppresses lung cancer progression by inducing apoptosis and autophagy initiation through the activation of AMPK signaling. Int. Immunopharmacol. 111, 108806. doi:10.1016/j.intimp.2022.108806
Liu, Z., Zheng, Q., Chen, W., Wu, M., Pan, G., Yang, K., et al. (2017). Chemosensitizing effect of Paris Saponin I on Camptothecin and 10-hydroxycamptothecin in lung cancer cells via p38 MAPK, ERK, and Akt signaling pathways. Eur. J. Med. Chem. 125, 760–769. doi:10.1016/j.ejmech.2016.09.066
Long, F. Y., Chen, Y. S., Zhang, L., Kuang, X., Yu, Y., Wang, L. F., et al. (2015). Pennogenyl saponins induce cell cycle arrest and apoptosis in human hepatocellular carcinoma HepG2 cells. J. Ethnopharmacol. 162, 112–120. doi:10.1016/j.jep.2014.12.065
Lu, M., Wang, Y., and Zhan, X. (2019). The MAPK pathway-based drug therapeutic targets in pituitary adenomas. Front. Endocrinol. 10, 330. doi:10.3389/fendo.2019.00330
Lü, S., Zhao, S., Zhao, M., Guo, Y., Li, G., Yang, B., et al. (2019). Systematic screening and characterization of prototype constituents and metabolites of triterpenoid saponins of Caulopphyllum robustum Maxim using UPLC-LTQ Orbitrap MS after oral administration in rats. J. Pharm. Biomed. analysis 168, 75–82. doi:10.1016/j.jpba.2019.02.005
Luo, Z., Xu, W., Zhang, Y., Di, L., and Shan, J. (2020). A review of saponin intervention in metabolic syndrome suggests further study on intestinal microbiota. Pharmacol. Res. 160, 105088. doi:10.1016/j.phrs.2020.105088
Ma, B., Zhu, J., Zhao, A., Zhang, J., Wang, Y., Zhang, H., et al. (2018). Raddeanin A, a natural triterpenoid saponin compound, exerts anticancer effect on human osteosarcoma via the ROS/JNK and NF-κB signal pathway. Toxicol. Appl. Pharmacol. 353, 87–101. doi:10.1016/j.taap.2018.05.025
Ma, L., Liu, H., Qin, P., Hu, C., Man, S., Li, Y., et al. (2017). Saponin fraction isolated from Conyza blinii H.Lév. demonstrates strong anti-cancer activity that is due to its NF-κB inhibition. Biochem. biophysical Res. Commun. 483 (1), 779–785. doi:10.1016/j.bbrc.2016.12.066
Man, S., Gao, W., Zhang, Y., Huang, L., and Liu, C. (2010). Chemical study and medical application of saponins as anti-cancer agents. Fitoterapia 81 (7), 703–714. doi:10.1016/j.fitote.2010.06.004
Martínez-Limón, A., Joaquin, M., Caballero, M., Posas, F., and de Nadal, E. (2020). The p38 pathway: From biology to cancer therapy. Int. J. Mol. Sci. 21 (6), 1913. doi:10.3390/ijms21061913)
Mi, Y., Xiao, C., Du, Q., Wu, W., Qi, G., and Liu, X. (2016). Momordin Ic couples apoptosis with autophagy in human hepatoblastoma cancer cells by reactive oxygen species (ROS)-mediated PI3K/Akt and MAPK signaling pathways. Free Radic. Biol. Med. 90, 230–242. doi:10.1016/j.freeradbiomed.2015.11.022
Nagasaka, M., and Gadgeel, S. M. (2018). Role of chemotherapy and targeted therapy in early-stage non-small cell lung cancer. Expert Rev. anticancer Ther. 18 (1), 63–70. doi:10.1080/14737140.2018.1409624
Navarro Del Hierro, J., Herrera, T., García-Risco, M. R., Fornari, T., Reglero, G., and Martin, D. (2018b). Ultrasound-assisted extraction and bioaccessibility of saponins from edible seeds: Quinoa, lentil, fenugreek, soybean and lupin. Food Res. Int. Ott. Ont.) 109, 440–447. doi:10.1016/j.foodres.2018.04.058
Navarro del Hierro, J, Herrera, T, Fornari, T, Reglero, G., and Martin, Diana (2018a). The gastrointestinal behavior of saponins and its significance for their bioavailability and bioactivities. J. Funct. Foods 40, 484–497. doi:10.1016/j.jff.2017.11.032
Nusse, R., and Clevers, H. (2017). Wnt/β-Catenin signaling, disease, and emerging therapeutic modalities. Cell 169 (6), 985–999. doi:10.1016/j.cell.2017.05.016
Paskeh, M. D. A., Asadi, A., Mirzaei, S., Hashemi, M., Entezari, M., Raesi, R., et al. (2022). Targeting AMPK signaling in ischemic/reperfusion injury: From molecular mechanism to pharmacological interventions. Cell. Signal. 94, 110323. doi:10.1016/j.cellsig.2022.110323
Passos, F. R. S., Araújo-Filho, H. G., Monteiro, B. S., Shanmugam, S., Araújo, A. A. S., Almeida, J. R. G. D. S., et al. (2022). Anti-inflammatory and modulatory effects of steroidal saponins and sapogenins on cytokines: A review of pre-clinical research. Phytomedicine Int. J. phytotherapy Phytopharm. 96, 153842. doi:10.1016/j.phymed.2021.153842
Plewka, D., Plewka, A., Miskiewicz, A., Morek, M., and Bogunia, E. (2018). Nuclear factor-kappa B as potential therapeutic target in human colon cancer. J. Cancer Res. Ther. 14 (3), 516–520. doi:10.4103/0973-1482.180607
Qiang, M., Cai, P., Ao, M., Li, X., Chen, Z., and Yu, L. (2023). Polysaccharides from Chinese materia medica: Perspective towards cancer management. Int. J. Biol. Macromol. 224, 496–509. doi:10.1016/j.ijbiomac.2022.10.139
Ramli, N. H., Yusup, S., Quitain, A. T., Johari, K., and Kueh, B. W. B. (2019). Optimization of saponin extracts using microwave-assisted extraction as a sustainable biopesticide to reduce Pomacea canaliculata population in paddy cultivation. Sustain. Chem. Pharm. 11, 23–35. doi:10.1016/j.scp.2018.12.002
Rong, L., Li, Z., Leng, X., Li, H., Ma, Y., Chen, Y., et al. (2020). Salidroside induces apoptosis and protective autophagy in human gastric cancer AGS cells through the PI3K/Akt/mTOR pathway. Biomed. Pharmacother. 122, 109726. doi:10.1016/j.biopha.2019.109726
Sachan, R., Kundu, A., Jeon, Y., Choi, W. S., Yoon, K., Kim, I. S., et al. (2018). Afrocyclamin A, a triterpene saponin, induces apoptosis and autophagic cell death via the PI3K/Akt/mTOR pathway in human prostate cancer cells. Phytomedicine Int. J. phytotherapy Phytopharm. 51, 139–150. doi:10.1016/j.phymed.2018.10.012
Samarghandian, S., Azimi-Nezhad, M., Borji, A., and Farkhondeh, T. (2016). Effect of crocin on aged rat kidney through inhibition of oxidative stress and proinflammatory state. Phytotherapy Res. PTR 30 (8), 1345–1353. doi:10.1002/ptr.5638
Sarwar, M. S., Zhang, H. J., and Tsang, S. W. (2018). Perspectives of plant natural products in inhibition of cancer invasion and metastasis by regulating multiple signaling pathways. Curr. Med. Chem. 25 (38), 5057–5087. doi:10.2174/0929867324666170918123413
Si, L., Zheng, L., Xu, L., Yin, L., Han, X., Qi, Y., et al. (2016). Dioscin suppresses human laryngeal cancer cells growth via induction of cell-cycle arrest and MAPK-mediated mitochondrial-derived apoptosis and inhibition of tumor invasion. Eur. J. Pharmacol. 774, 105–117. doi:10.1016/j.ejphar.2016.02.009
Singh, D., and Chaudhuri, P. K. (2018). Structural characteristics, bioavailability and cardioprotective potential of saponins. Integr. Med. Res. 7 (1), 33–43. doi:10.1016/j.imr.2018.01.003
Soleimani, A., Rahmani, F., Ferns, G. A., Ryzhikov, M., Avan, A., and Hassanian, S. M ( (2020). Role of the NF-κB signaling pathway in the pathogenesis of colorectal cancer. Gene 726, 144132. doi:10.1016/j.gene.2019.144132
Sommer, F., Anderson, J. M., Bharti, R., Raes, J., and Rosenstiel, P. (2017). The resilience of the intestinal microbiota influences health and disease. Nat. Rev. Microbiol. 15 (10), 630–638. doi:10.1038/nrmicro.2017.58
Song, X., Wei, C., and Li, X. (2022). The signaling pathways associated with breast cancer bone metastasis. Front. Oncol. 12, 855609. doi:10.3389/fonc.2022.855609
Song, X. Y., Han, F. Y., Chen, J. J., Wang, W., Zhang, Y., Yao, G. D., et al. (2019). Timosaponin AIII, a steroidal saponin, exhibits anti-tumor effect on taxol-resistant cells in vitro and in vivo. Steroids 146, 57–64. doi:10.1016/j.steroids.2019.03.009
Spaan, I., Raymakers, R. A., van de Stolpe, A., and Peperzak, V. (2018). Wnt signaling in multiple myeloma: A central player in disease with therapeutic potential. J. Hematol. Oncol. 11 (1), 67. doi:10.1186/s13045-018-0615-3
Sun, D., Shen, W., Zhang, F., Fan, H., Xu, C., Li, L., et al. (2018). α-Hederin inhibits interleukin 6-induced epithelial-to-mesenchymal transition associated with disruption of JAK2/STAT3 signaling in colon cancer cells. Biomed. Pharmacother. 101, 107–114. doi:10.1016/j.biopha.2018.02.062
Sung, H., Ferlay, J., Siegel, R. L., Laversanne, M., Soerjomataram, I., Jemal, A., et al. (2021). Global cancer statistics 2020: GLOBOCAN estimates of incidence and mortality worldwide for 36 cancers in 185 countries. CA a cancer J. Clin. 71 (3), 209–249. doi:10.3322/caac.21660
Tan, A. C. (2020). Targeting the PI3K/Akt/mTOR pathway in non-small cell lung cancer (NSCLC). Thorac. cancer 11 (3), 511–518. doi:10.1111/1759-7714.13328
Tao, T., He, C., Deng, J., Huang, Y., Su, Q., Peng, M., et al. (2017). A novel synthetic derivative of quercetin, 8-trifluoromethyl-3,5,7,3',4'- O -pentamethyl-quercetin, inhibits bladder cancer growth by targeting the AMPK/mTOR signaling pathway. Oncotarget 8 (42), 71657–71671. doi:10.18632/oncotarget.17799
Tewari, D., Patni, P., Bishayee, A., Sah, A. N., and Bishayee, A. (2022). Natural products targeting the PI3K-Akt-mTOR signaling pathway in cancer: A novel therapeutic strategy. Seminars cancer Biol. 80, 1–17. doi:10.1016/j.semcancer.2019.12.008
Tian, Y., Gong, G. Y., Ma, L. L., Wang, Z. Q., Song, D., and Fang, M. Y. (2020). Anti-cancer effects of Polyphyllin I: An update in 5 years. Chemico-biological Interact. 316, 108936. doi:10.1016/j.cbi.2019.108936
Tu, X., Deng, Y., Chen, J., Hu, Q., He, C., Jordan, J. B., et al. (2016). Screening study on the anti-angiogenic effects of Traditional Chinese Medicine - Part I: Heat-clearing and detoxicating TCM. J. Ethnopharmacol. 194, 280–287. doi:10.1016/j.jep.2016.09.010
Uzayisenga, R., Ayeka, P. A., and Wang, Y. (2014). Anti-diabetic potential of Panax notoginseng saponins (PNS): A review. Phytotherapy Res. PTR 28 (4), 510–516. doi:10.1002/ptr.5026
Wang, D., Wu, Z., Zhao, C., Yang, X., Wei, H., Liu, M., et al. (2021c). KP-10/Gpr54 attenuates rheumatic arthritis through inactivating NF-κB and MAPK signaling in macrophages. Pharmacol. Res. 171, 105496. doi:10.1016/j.phrs.2021.105496
Wang, S., Chen, X., Cheng, J., Cai, T., Wu, X., Cheng, Z., et al. (2021d). Calunduloside E inhibits HepG2 cell proliferation and migration via p38/JNK-HMGB1 signalling axis. J. Pharmacol. Sci. 147 (1), 18–26. doi:10.1016/j.jphs.2021.05.005
Wang, T., Di, G., Yang, L., Dun, Y., Sun, Z., Wan, J., et al. (2015). Saponins from Panax japonicus attenuate D-galactose-induced cognitive impairment through its anti-oxidative and anti-apoptotic effects in rats. J. Pharm. Pharmacol. 67 (9), 1284–1296. doi:10.1111/jphp.12413
Wang, W., Liu, Y., You, L., Sun, M., Qu, C., Dong, X., et al. (2020). Inhibitory effects of Paris saponin I, II, Ⅵ and Ⅶ on HUVEC cells through regulation of VEGFR2, PI3K/AKT/mTOR, Src/eNOS, PLCγ/ERK/MERK, and JAK2-STAT3 pathways. Biomed. Pharmacother. 131, 110750. doi:10.1016/j.biopha.2020.110750
Wang, X., Su, G. Y., Zhao, C., Qu, F. Z., Wang, P., and Zhao, Y. Q ( (2018c). Anticancer activity and potential mechanisms of 1C, a ginseng saponin derivative, on prostate cancer cells. J. ginseng Res. 42 (2), 133–143. doi:10.1016/j.jgr.2016.12.014
Wang, Y-E, Xu, K, Wen-Hua, Y, You, B-G, Zhang, M-Y, Zhu, Z-C, et al. (2018a). Hederacolchiside A1 suppresses proliferation of tumor cells by inducing apoptosis through modulating PI3K/Akt/mTOR signaling pathway. Chin. Herb. Med. 10 (02), 215–222. doi:10.1016/j.jff.2021.104796
Wang, Y., Bao, X., Zhao, A., Zhang, J., Zhang, M., Zhang, Q., et al. (2018b). Raddeanin A inhibits growth and induces apoptosis in human colorectal cancer through downregulating the Wnt/β-catenin and NF-κB signaling pathway. Life Sci. 207, 532–549. doi:10.1016/j.lfs.2018.06.035
Wang, Y., He, Q. Y., and Chiu, J. F ( (2014). Dioscin induced activation of p38 MAPK and JNK via mitochondrial pathway in HL-60 cell line. Eur. J. Pharmacol. 735, 52–58. doi:10.1016/j.ejphar.2014.04.018
Wang, Z., Hui, C., and Xie, Y. (2021b). Natural STAT3 inhibitors: A mini perspective. Bioorg. Chem. 115, 105169. doi:10.1016/j.bioorg.2021.105169
Wang, Z., Xu, J., Wang, Y., Xiang, L., and He, X. (2021a). Total saponins from Tupistra chinensis baker inhibits growth of human gastric cancer cells in vitro and in vivo. J. Ethnopharmacol. 278, 114323. doi:10.1016/j.jep.2021.114323
Wei, G., Yang, F., Wei, F., Zhang, L., Gao, Y., Qian, J., et al. (2020). Metabolomes and transcriptomes revealed the saponin distribution in root tissues of Panax quinquefolius and Panax notoginseng. J. ginseng Res. 44 (6), 757–769. doi:10.1016/j.jgr.2019.05.009
Wei, X. H., Lin, S. S., Liu, Y., Zhao, R. P., Khan, G. J., Du, H. Z., et al. (2016). DT-13 attenuates human lung cancer metastasis via regulating NMIIA activity under hypoxia condition. Oncol. Rep. 36 (2), 991–999. doi:10.3892/or.2016.4879
Wei, X., Mao, T., Li, S., He, J., Hou, X., Li, H., et al. (2019). DT-13 inhibited the proliferation of colorectal cancer via glycolytic metabolism and AMPK/mTOR signaling pathway. Phytomedicine Int. J. phytotherapy Phytopharm. 54, 120–131. doi:10.1016/j.phymed.2018.09.003
Wu, Q., Deng, J., Fan, D., Duan, Z., Zhu, C., Fu, R., et al. (2018). Ginsenoside Rh4 induces apoptosis and autophagic cell death through activation of the ROS/JNK/p53 pathway in colorectal cancer cells. Biochem. Pharmacol. 148, 64–74. doi:10.1016/j.bcp.2017.12.004
Wu, Q., Zhou, X., Huang, D., Ji, Y., and Kang, F. (2017). IL-6 enhances osteocyte-mediated osteoclastogenesis by promoting JAK2 and RANKL activity in vitro. Cell. physiology Biochem. Int. J. Exp. Cell. physiology, Biochem. Pharmacol. 41 (4), 1360–1369. doi:10.1159/000465455
Wu, T., Jia, Z., Dong, S., Han, B., Zhang, R., Liang, Y., et al. (2019). Panax notoginseng saponins ameliorate leukocyte adherence and cerebrovascular endothelial barrier breakdown upon ischemia-reperfusion in mice. J. Vasc. Res. 56 (1), 1–10. doi:10.1159/000494935
Xia, L., Zhang, B., Yan, Q., and Ruan, S. (2018). Effects of saponins of patrinia villosa against invasion and metastasis in colorectal cancer cell through NF-κB signaling pathway and EMT. Biochem. biophysical Res. Commun. 503 (3), 2152–2159. doi:10.1016/j.bbrc.2018.08.005
Xiang, L., Yi, X., Wang, Y., and He, X. (2016). Antiproliferative and anti-inflammatory polyhydroxylated spirostanol saponins from Tupistra chinensis. Sci. Rep. 6, 31633. doi:10.1038/srep31633
Xie, Z. Z., Li, M. M., Deng, P. F., Wang, S., Wang, L., Lu, X. P., et al. (2017). Paris saponin-induced autophagy promotes breast cancer cell apoptosis via the Akt/mTOR signaling pathway. Chemico-biological Interact. 264, 1–9. doi:10.1016/j.cbi.2017.01.004
Xu, F., Cui, W. Q., Wei, Y., Cui, J., Qiu, J., Hu, L. L., et al. (2018). Astragaloside IV inhibits lung cancer progression and metastasis by modulating macrophage polarization through AMPK signaling. J. Exp. Clin. cancer Res. CR 37 (1), 207. doi:10.1186/s13046-018-0878-0
Xu, J., Wang, Z., Huang, Y., Wang, Y., Xiang, L., and He, X. (2020b). A spirostanol saponin isolated from Tupistra chinensis Baker simultaneously induces apoptosis and autophagy by regulating the JNK pathway in human gastric cancer cells. Steroids 164, 108737. doi:10.1016/j.steroids.2020.108737
Xu, J., Zhang, J., Mao, Q. F., Wu, J., and Wang, Y. (2022). The interaction between autophagy and JAK/STAT3 signaling pathway in tumors. Front. Genet. 13, 880359. doi:10.3389/fgene.2022.880359
Xu, J., Zhang, M., Lin, X., Wang, Y., and He, X. (2020a). A steroidal saponin isolated from Allium chinense simultaneously induces apoptosis and autophagy by modulating the PI3K/Akt/mTOR signaling pathway in human gastric adenocarcinoma. Steroids 161, 108672. doi:10.1016/j.steroids.2020.108672
Xu, X. H., Li, T., Fong, C. M., Chen, X., Chen, X. J., Wang, Y. T., et al. (2016). Saponins from Chinese medicines as anticancer agents. Mol. (Basel, Switz. 21 (10), 1326. null. doi:10.3390/molecules21101326
Xu, X., Xu, Y., Zhang, Q., Yang, F., Yin, Z., Wang, L., et al. (2019). Porcine epidemic diarrhea virus infections induce apoptosis in Vero cells via a reactive oxygen species (ROS)/p53, but not p38 MAPK and SAPK/JNK signalling pathways. Veterinary Microbiol. 232, 1–12. doi:10.1016/j.vetmic.2019.03.028
Yan, X., Qi, M., Li, P., Zhan, Y., and Shao, H. (2017). Apigenin in cancer therapy: Anti-cancer effects and mechanisms of action. Cell & Biosci. 7, 50. doi:10.1186/s13578-017-0179-x
Yao, W., and Guan, Y. (2022). Ginsenosides in cancer: A focus on the regulation of cell metabolism. Biomed. Pharmacother. 156, 113756. doi:10.1016/j.biopha.2022.113756
Yim, N. H., Hwang, Y. H., Liang, C., and Ma, J. Y ( (2016). A platycoside-rich fraction from the root of Platycodon grandiflorum enhances cell death in A549 human lung carcinoma cells via mainly AMPK/mTOR/AKT signal-mediated autophagy induction. J. Ethnopharmacol. 194, 1060–1068. doi:10.1016/j.jep.2016.10.078
Yu, L. L., Wang, S., Wang, J., Yan, H., Ni, W., and Liu, H. Y ( (2022). Steroidal saponin components and their cancer cell cytotoxicity from Paris rugosa. Phytochemistry 204, 113452. doi:10.1016/j.phytochem.2022.113452
Yu, Y., Wu, X., Pu, J., Luo, P., Ma, W., Wang, J., et al. (2018). Lycium barbarum polysaccharide protects against oxygen glucose deprivation/reoxygenation-induced apoptosis and autophagic cell death via the PI3K/Akt/mTOR signaling pathway in primary cultured hippocampal neurons. Biochem. biophysical Res. Commun. 495 (1), 1187–1194. doi:10.1016/j.bbrc.2017.11.165
Yuan, S., Xu, Y., Yi, T., and Wang, H. (2022). The anti-tumor effect of OP-B on ovarian cancer in vitro and in vivo, and its mechanism: An investigation using network pharmacology-based analysis. J. Ethnopharmacol. 283, 114706. doi:10.1016/j.jep.2021.114706
Yusoff, I. M., Mat Taher, Z., Rahmat, Z., and Chua, L. S. (2022). A review of ultrasound-assisted extraction for plant bioactive compounds: Phenolics, flavonoids, thymols, saponins and proteins. Food Res. Int. Ott. Ont.) 157, 111268. doi:10.1016/j.foodres.2022.111268
Zeng, M., Zhang, R., Yang, Q., Guo, L., Zhang, X., Yu, B., et al. (2022). Pharmacological therapy to cerebral ischemia-reperfusion injury: Focus on saponins. Biomed. Pharmacother. = Biomedecine Pharmacother. 155, 113696. doi:10.1016/j.biopha.2022.113696
Zhan, Y., Liu, R., Wang, W., Li, J., Yang, X. O., and Zhang, Y. (2016). Total saponins isolated from Radix et Rhizoma Leonticis suppresses tumor cells growth by regulation of PI3K/Akt/mTOR and p38 MAPK pathways. Environ. Toxicol. Pharmacol. 41, 39–44. doi:10.1016/j.etap.2015.10.008
Zhang, D., Zhang, Q., Zheng, Y., and Lu, J. (2020). Anti-breast cancer and toxicity studies of total secondary saponin from Anemone raddeana Rhizome on MCF-7 cells via ROS generation and PI3K/AKT/mTOR inactivation. J. Ethnopharmacol. 259, 112984. doi:10.1016/j.jep.2020.112984
Zhang, L., Man, S., Wang, Y., Liu, J., Liu, Z., Yu, P., et al. (2016). Paris Saponin II induced apoptosis via activation of autophagy in human lung cancer cells. Chemico-biological Interact. 253, 125–133. doi:10.1016/j.cbi.2016.05.016
Zhang, M., Weng, W., Zhang, Q., Wu, Y., Ni, S., Tan, C., et al. (2018b). The lncRNA NEAT1 activates Wnt/β-catenin signaling and promotes colorectal cancer progression via interacting with DDX5. J. Hematol. Oncol. 11 (1), 113. doi:10.1186/s13045-018-0656-7
Zhang, X., Liu, Z., Chen, S., Li, H., Dong, L., and Fu, X. (2022). A new discovery: Total Bupleurum saponin extracts can inhibit the proliferation and induce apoptosis of colon cancer cells by regulating the PI3K/Akt/mTOR pathway. J. Ethnopharmacol. 283, 114742. doi:10.1016/j.jep.2021.114742
Zhang, Y. S., Ma, Y. L., Thakur, K., Hussain, S. S., Wang, J., Zhang, Q., et al. (2018a). Molecular mechanism and inhibitory targets of dioscin in HepG2 cells. Food Chem. Toxicol. Int. J. Publ. Br. Industrial Biol. Res. Assoc. 120, 143–154. doi:10.1016/j.fct.2018.07.016
Zhang, Y., and Wang, X. (2020). Targeting the Wnt/β-catenin signaling pathway in cancer. J. Hematol. Oncol. 13 (1), 165. doi:10.1186/s13045-020-00990-3
Zhong, Y., Li, X. Y., Zhou, F., Cai, Y. J., Sun, R., and Liu, R. P ( (2021). Ziyuglycoside II inhibits the growth of digestive system cancer cells through multiple mechanisms. Chin. J. Nat. Med. 19 (5), 351–363. doi:10.1016/S1875-5364(21)60033-X
Zhou, H., Sun, Y., Zheng, H., Fan, L., Mei, Q., Tang, Y., et al. (2019). Paris saponin VII extracted from trillium tschonoskii suppresses proliferation and induces apoptosis of human colorectal cancer cells. J. Ethnopharmacol. 239, 111903. doi:10.1016/j.jep.2019.111903
Zhou, X., Yue, G. G., Tsui, S. K., Pu, J., Fung, K. P., and Lau, C. B ( (2018). Elaborating the role of natural products on the regulation of autophagy and their potentials in breast cancer therapy. Curr. cancer drug targets 18 (3), 239–255. doi:10.2174/1568009617666170330124819
Zhu, Y., Zhu, C., Yang, H., Deng, J., and Fan, D. (2020). Protective effect of ginsenoside Rg5 against kidney injury via inhibition of NLRP3 inflammasome activation and the MAPK signaling pathway in high-fat diet/streptozotocin-induced diabetic mice. Pharmacol. Res. 155, 104746. doi:10.1016/j.phrs.2020.104746
Zhu, Z., McGray, A. J. R., Jiang, W., Lu, B., Kalinski, P., and Guo, Z. S ( (2022). Improving cancer immunotherapy by rationally combining oncolytic virus with modulators targeting key signaling pathways. Mol. cancer 21 (1), 196. doi:10.1186/s12943-022-01664-z
Akt Protein kinase B;
AMPK AMP-activated protein kinase;
AS-IV Astragaloside IV;
Bcl-xl B-cell lymphoma-extra-large;
Bax Bcl-2-associated X;
Bcl-2 B-cell lymphoma-2;
Cyt-c Cytochrome c;
CDK1 cyclin dependent kinase 1;
Cyclin cell cycle protein;
CK1α casein kinase 1α;
CHM Chinese herbal medicine;
EGFR Epidermal growth factor receptor;
EMT epithelial-mesenchymal transition;
ERK extracellular signal-regulated kinase;
EGFR epidermal growth factor receptor;
JAK Janus Kinase;
GSK3β glycogen synthase kinase 3β;
JNK c-Jun N-terminal kinase;
LC3 Microtubule-associated protein light chain 3;
MAPK mitogen-activated protein kinase;
MMP matrix metalloproteinase;
mTOR mammalian target of rapamycin;
NF-κB Nuclear factor kappa B;
NF-κB nuclear factor kappa-B;
PIP2 phosphatidylinositol bisphosphate;
PIP3 phosphatidylinositol trisphosphate;
p70S6K Ribosomal protein S6 kinase;
TcF/LEF T-cell transcription factor/lymphocyte enhancer factor;
PARP Poly (ADP-ribose) polymerase;
p-Akt Phosphorylated Protein kinase B;
PI3K phosphoinositide 3-kinase;
STAT3 signal transducer and activator of transcription-3;
TCM Traditional Chinese herbal medicine;
Wnt The wingless-related integration site
Keywords: triterpenoid saponins, signal transduction pathway, anti-cancer, Chinese herbal medicine, steroid saponins
Citation: Zhu M, Sun Y, Bai H, Wang Y, Yang B, Wang Q and Kuang H (2023) Effects of saponins from Chinese herbal medicines on signal transduction pathways in cancer: A review. Front. Pharmacol. 14:1159985. doi: 10.3389/fphar.2023.1159985
Received: 06 February 2023; Accepted: 16 March 2023;
Published: 29 March 2023.
Edited by:
Azhar Rasul, Government College University, PakistanReviewed by:
Ping Wang, Shanghai University of Traditional Chinese Medicine, ChinaCopyright © 2023 Zhu, Sun, Bai, Wang, Yang, Wang and Kuang. This is an open-access article distributed under the terms of the Creative Commons Attribution License (CC BY). The use, distribution or reproduction in other forums is permitted, provided the original author(s) and the copyright owner(s) are credited and that the original publication in this journal is cited, in accordance with accepted academic practice. No use, distribution or reproduction is permitted which does not comply with these terms.
*Correspondence: Qiuhong Wang, cWh3YW5nQGhsanVjbS5uZXQ=; Haixue Kuang, aHhrdWFuZzE1QGhvdG1haWwuY29t
Disclaimer: All claims expressed in this article are solely those of the authors and do not necessarily represent those of their affiliated organizations, or those of the publisher, the editors and the reviewers. Any product that may be evaluated in this article or claim that may be made by its manufacturer is not guaranteed or endorsed by the publisher.
Research integrity at Frontiers
Learn more about the work of our research integrity team to safeguard the quality of each article we publish.