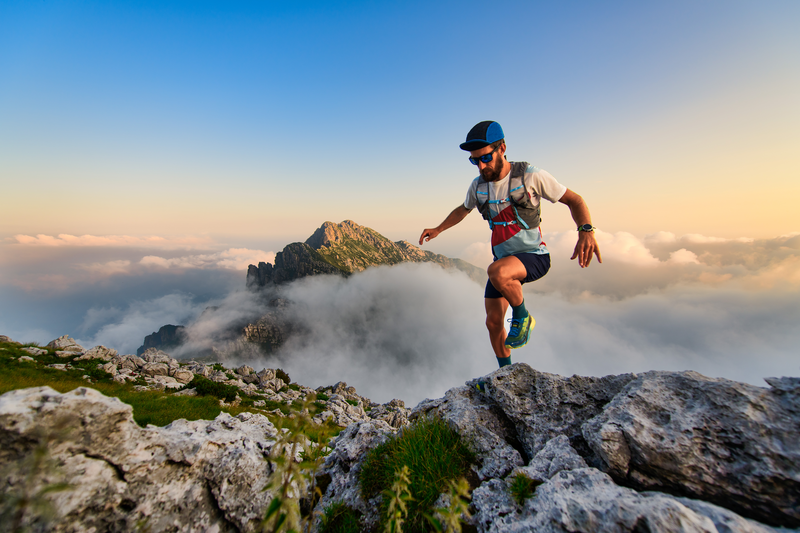
95% of researchers rate our articles as excellent or good
Learn more about the work of our research integrity team to safeguard the quality of each article we publish.
Find out more
ORIGINAL RESEARCH article
Front. Pharmacol. , 22 May 2023
Sec. Pharmacology of Anti-Cancer Drugs
Volume 14 - 2023 | https://doi.org/10.3389/fphar.2023.1159712
20 (R)-25-methoxyl-dammarane-3β, 12β, 20-triol (AD-1), a novel ginsenoside isolated from stem and leaf of Panax Notoginseng, has anticancer activity against a variety of malignant tumors. However, the pharmacological mechanism of AD-1 on colorectal cancer (CRC) remains unclear. The purpose of this study was to verify the potential mechanism of action of AD-1 against CRC through network pharmacology and experiments. A total of 39 potential targets were obtained based on the intersection of AD-1 and CRC targets, and key genes were analyzed and identified from the PPI network using Cytoscape software. 39 targets were significantly enriched in 156 GO terms and 138 KEGG pathways, among which PI3K-Akt signaling pathway was identified as one of the most enriched pathways. Based on experimental results, AD-1 can inhibit the proliferation and migration of SW620 and HT-29 cells, and induce their apoptosis. Subsequently, the HPA and UALCAN databases showed that PI3K and Akt were highly expressed in CRC. AD-1 also decreased the expressions of PI3K and Akt. In summary, these results suggest that AD-1 can play an anti-tumor role by inducing cell apoptosis and regulating PI3K-Akt signaling pathway.
Colorectal cancer (CRC) is the third most common cancer in the world, ranking the third in incidence and the second in mortality (Sung et al., 2021). Despite great progress in the treatment of CRC, the survival rate of patients with advanced CRC is still low due to poor prognosis (Gbolahan et al., 2019; Miller et al., 2019). In addition, chemotherapy has certain side effects, which can lead to drug resistance, while targeted therapy also has a certain burden of symptoms (Veenstra et al., 2018). Therefore, the development of effective anti-CRC drugs is particularly important.
Ginsenosides are the main active components of ginseng, with anti-tumor, anti-inflammatory and other pharmacological effects (Wei et al., 2020; Ren et al., 2021). 20 (R)-25-methoxyl-dammarane-3β, 12β, 20-triol (AD-1) is a novel saponin derivative isolated from the hydrolyzed saponins from the stem and leaf of panax notoginseng. It has been reported that AD-1 not only plays an anti-hepatic fibrosis role (Han et al., 2018; Li et al., 2022), but also can inhibit the activity of gastric cancer and lung cancer (Zhang et al., 2013; Zhao et al., 2016). Zhang et al. (2013) pointed out that AD-1 induced lung cancer cell arrest and apoptosis by activating p38 MAPK signaling pathway. However, the anti-CRC effect of AD-1 has not been studied. Therefore, it would be interesting to explore the roles and potential mechanisms of AD-1 in CRC.
Network pharmacology is a systematic approach that combines systems biology and bioinformatics to elucidate the potential mechanisms of action of drugs (Nogales et al., 2022). In this study, we used network pharmacology to predict potential targets of AD-1 and CRC, and screened central molecules by PPI and enrichment analysis. Finally, the molecular mechanism of the above phenomenon was verified by in vitro experiments. Figure 1 depicts this workflow study.
Through PharmMapper (http://lilab.ecust.edu.cn/pharmmapper/) (Wang et al., 2017), the Swiss Target Prediction Screening (http://www.swisstargetprediction.ch/) (Daina et al., 2019) of AD-1 database of potential targets. Use UniProt (https://www.uniprot.org/) to improve the UniProt ID and gene name of the target.
Use “colorectal cancer” as the keyword in the online Mendelian inheritance in man (OMIM, https://omim.org/) (Amberger et al., 2015) database, GeneCards (https://www.genecards.org/) (Rebhan et al., 1997) database (correlation score of 20 or higher) to retrieve the CRC related targets. The results of different databases were integrated to delete duplicate target genes and finally obtain potential CRC-related targets.
Potential drug targets and CRC related genes were uploaded to VENNY2.1 website (https://bioinfogp.cnb.csic.es/tools/venny/index.html), to determine the cross genes, both the common targets for AD-1 candidate targets for the treatment of CRC.
The cross genes obtained in 2.3 were imported into the STRING database (https://string-db.org/) to construct the PPI network. Cytoscape (version 3.8.2) software and CytoHubba plug-ins are then used for visual analysis to sort the nodes in the network according to the algorithm of degree and select the top 10 core genes.
We will cross gene into the database for annotation, visualization, and integrated discovery (DAVID) (https://david.ncifcrf.gov/) (Sherman et al., 2022) to GO enrichment analysis, the database from a biological process (BP), cell composition (CC) and molecular function (MF) evaluated the goal of participation. In addition, we used KOBAS 3.0 database (http://kobas.cbi.pku.edu.cn/) (Bu et al., 2021) KEGG pathway enrichment. Then will GO and KEGG results import bioinformatics website (http://www.bioinformatics.com.cn/) for annotation and visualization.
The AD-1 structure was imported into ChemBio3D Ultra 14.0 software to minimize energy and obtain the 3D structure, which were further inputted into AutodockTools-1.5.6 and saved as “pdbqt” format. The protein 3D structure was obtained from the Protein Data Bank (PDB, http://www.rcsb.org/) and then imported into Pymol 2.3.0 to remove the protein crystallinity water, the original ligand, etc. After that, the protein structure was imported into AutoDocktools (v1.5.6) and saved as “pdbqt” format. Finally, Autodock 4.26 was used for molecular docking, and the results were visualized with PyMOL2.3.0.
AD-1 (isolated from Panax notoginseng), colorless crystals, the purity of 99.9%. Its molecular formula was ensured to be C31H56O4 by 13C NMR and ESI MS [m/z 493 (M + H)+ and 515 (M + Na)+], which was ascertained through HRE-IMS (m/z 515.4064 calcd for C31H56O4Na, 515.4076). Finally, HMBC spectrum analysis, the construction of the AD-1 was confirmed as 20 (R)-25-methoxyl-dammarane-3β, 12β, 20-triol. And then, AD-1 was dissolved in dimethyl subunit DMSO (Sigma-Aldrich, MO, United States), then divided into several centrifuge tubes and stored at −20°C for subsequent use.
The SW620 and HT-29, human CRC cell lines, were purchased from the American Type Culture Collection. These cells were cultured in DMEM medium (Biological Industries, BeitHaEmek, Israel) including 10% FBS (Biological Industries, BeitHaEmek, Israel) at 37°C in a 5% CO2.
The cells were inoculated into 96-well plates at 1 × 104 cells/well and cultured for 24 h. Subsequent incubations were performed with different concentrations of AD-1. After 24 h or 48 h, 10 μL CCK-8 solution (Beyotime Biotechnology, Jiangsu, China) was added to each well. The corresponding absorbance was measured after 2 h.
The cells were implanted into a 6-well plate of 2 × 106 cells/well. Monolayer cells were scraped with a sterile pipette to form the wound, washed with PBS for 3 times, and cultured with AD-1 solution for 0 h, 24 h, and 48 h, then imaging examination of the wound was performed.
TCGA dataset was used to compare the expression of PI3K-Akt signaling pathway in normal and CRC tissues. Examples of proteins found in the HPA database that showed a trend of differential expression in normal and CRC tissues. The above data analysis from the UALCAN (http://ualcan.path.uab.edu/index.html) and HPA (http://www.proteinatlas.org) database.
CRC cells (2 × 106 cells/well) were inoculated in 6-well plates for 24 h and treated with different concentrations of AD-1 for 48 h. Subsequently, protein were isolated and quantified from SW620 and HT-29 cells. The required proteins were isolated on SDS-PAGE gels and shifted to PVDF membrane, closed for 1 h. Subsequent, cultivated with antibodies including Bax, Bcl-2 (Abcam, Cambridge, UK), p-PI3K, PI3K, p-Akt, Akt, and β-actin (Cell Signaling Techno-logy, Boston, MA, United States) for 24 h. The corresponding secondary antibodies were used to incubate the membrane for 1 h (Aleuronic, Beijing, China). The strip films were incubated by ECL chemiluminescence (Everbright, San Ramon, CA, United States). Finally, the membranes were developed and imaged.
All experiments were repeated three times, and the data are presented as the mean ± SD. Group means were compared using a t-test. p < 0.05 was considered statistically significant.
The chemical structure of AD-1 is shown in Figure 2A. The molecular structure of AD-1 was uploaded to PharmMapper database and Swiss Target Prediction database and 156 potential targets of AD-1 were identified. In addition, a total of 1711 CRC-related targets were obtained from the OMIM database and GeneCards database. AD-1 targets and CRC-related genes were then uploaded to VENNY2.1 to generate a Venn diagram (Figure 2B). There were 39 intersections have been identified as potential candidate targets for AD-1 against CRC (Table 1).
FIGURE 2. Targets screening involved in AD-1 for treating CRC. (A) The chemical structure of AD-1. (B) Thirty-nine overlapping target proteins between CRC-related genes and targets of AD-1.
In order to further explore the relationship between cross genes, they were uploaded to STRING database to construct PPI network, which contained 39 nodes and 109 edges (Figure 3A). Then, we upload the PPI network to Cytoscape software for visualization, and utilize CytoHubba plug-ins, the top 10 key genes (ranked from high to low) were screened by degree method: PIK3CA, MTOR, PTGS2, MET, JAK2, AR, PIK3CB, MAPK14, PIK3CD, and CYP19A1 (Figure 3B; Table 2). PIK3CA is the topmost node with a connection of 32°, which may play an important role in the anti-CRC mechanism of AD-1.
FIGURE 3. Construction of PPI network. (A) PPI network of 39 cross-target. (B) The first 10 key genes generated by (A). The darker the node color (red), the stronger the connection.
DAVID database was used to analyze the GO analysis of 39 cross genes. The 39 cross genes of AD-1 against CRC were significantly enriched in 106 BPs, 16 CCs, and 34 MFs (p < 0.05). As shown in Figure 4A, BP enrichment analysis showed that AD-1 may be involved in the regulation of signal transduction, protein phosphorylation, cell proliferation, cell migration and apoptotic process. CC enrichment analysis showed that AD-1 mainly plays a role in cytoplasm, membrane and cytosol. MF enrichment analysis suggested that the antitumor effect of AD-1 might be related to its effects on kinase activity, protein kinase activity, protein kinase binding and identical protein binding. In addition, KEGG pathway analysis was performed on KOBAS database and 138 pathways were screened (p < 0.05). It was found that metabolic pathways, pathways in cancer and PI3K-Akt signaling pathway were the top three significant pathways, with 14, 12 and 9 cross-genes, respectively (Figures 4B, C). PI3K-Akt signaling pathway plays a crucial role in regulating the development of tumor cells. Furthermore, PI3K-Akt signaling pathway is activated in malignant tumors and can directly regulate the proliferation, migration and apoptosis of tumor cells, which is a potential therapeutic target. Tan et al. (2021) pointed out that the PI3K-Akt signaling pathway is important in CRC, and found that promethazine promoted apoptosis by inhibiting the activation of PI3K-Akt signaling pathway in CRC cells. Next, we used molecular docking simulations to predict the binding capacity of AD-1 to PI3K and Akt. A lower binding energy indicates a more efficient and stable interaction between the compound and the receptor. As shown in Figures 4D, E, the binding energies of AD-1 to PI3K and Akt were −8.4 and −6.2 kcal/mol, respectively, indicating their good binding activities (Table 3).
FIGURE 4. GO and KEGG pathway analysis of intersecting genes. (A) GO enrichment analysis, including BP, CC, and MF. (B) Bubble diagram of KEGG pathway enrichment. (C) GO chord diagram. (D, E) The docking mode of AD-1 binding with target proteins; (D) PI3K, (E) Akt.
To verify the anti-proliferation effect of AD-1 on CRC cells, CCK8 was used to detect the proliferation ability of SW620 and HT-29 cells treated with AD-1 for 24 and 48 h. The results showed that AD-1 significantly inhibited the proliferation of SW620 and HT-29 cells. The IC50 values of SW620 and HT-29 cells treated with AD-1 for 24 h were 36.22 µM, 15.52 µM, respectively. The IC50 values of SW620 and HT-29 cells treated with AD-1 for 48 h were 18.65 µM, 11.07 µM, respectively (Figure 5A). Therefore, 0, 5, 10, 20 µM concentration was selected for 48 h for subsequent study. In addition, wound healing assay was performed to evaluate the effect of AD-1 on the migration ability of SW620 and HT-29 cells. The results showed that with the increase of AD-1 concentration, the migration ability of SW620 and HT-29 cells was significantly inhibited (Figure 5B).
FIGURE 5. AD-1 inhibited CRC cell proliferation, migration and induced apoptosis. (A) SW620 and HT-29 cell proliferation was measured after treatment with AD-1 at different concentrations for 24 and 48 h. (B) The ability of SW620 and HT-29 cells to migrate was examined by wound healing assay. (C,D) The protein expressions of Bcl-2, Bax and β-actin were evaluated by western blot. *: vs. the control group, *p < 0.05, **p < 0.01.
Bcl-2 family proteins, including Bcl-2 and Bax, are key regulatory genes that initiate apoptosis. To detect the effect of AD-1 on apoptosis of SW620 and HT-29 cells, the levels of apoptosis-related proteins were detected by western blot. The results showed that AD-1 significantly decreased the expression of Bcl-2 protein, but increased the expression of Bax protein in a dose-dependent manner (Figures 5C, D). Besides, AD-1 dramatically increased the percentage of apoptosis cells (Supplementary Figure S1). These results suggested that AD-1 could efficiently induce apoptosis of CRC cells.
These results indicated that AD-1 could significantly inhibit cell growth and migration, and induce cell apoptosis. According to the results of network pharmacological analysis, the PI3K-Akt signaling pathway is involved in the anti-CRC mechanism of AD-1. To test this hypothesis, we analyzed the correlation between the PI3K-Akt signaling pathway and CRC using the HPA and UALCAN databases. We analyzed PI3K and Akt expression at the protein level in normal and CRC tissues using data from the HPA. Notably, CRC tissue was highly positive in multiple patients, primarily in the cytoplasm/membrane (Figure 6A). In addition, mRNA expressions of PI3K and Akt in CRC tissues were significantly higher than those in normal tissues (Figure 6B). It is suggested that PI3K and Akt are highly expressed in CRC.
FIGURE 6. Validation of PI3K and Akt genes in HPA and UALCAN databases. (A) The representative immunohistochemical images of PI3K and Akt in normal tissue and CRC tissue. The right picture is a magnified image corresponding to (×400). (B) The expression of PI3K and Akt in UALCAN database. Tumors are shown in red and normal tissues in blue. *: vs. the control group, *p < 0.05, **p < 0.01.
To further test this hypothesis, we evaluated the expression levels of PI3K, p-PI3K, Akt, and p-Akt in SW620 and HT-29 cells after AD-1 intervention. The results showed that AD-1 significantly inhibited the phosphorylation levels of PI3K and Akt (Figures 7A, B), indicating that the PI3K-Akt pathway was inhibited by AD-1 in CRC cells. Next, we further explored the role of the PI3K-Akt signaling pathway in AD-1 mediated regulation of CRC. We used PI3K inhibitor LY294002 (20 μM) to block the activity of PI3K-Akt, and PI3K activator 740Y-P (5 μM) to activate the activity of PI3K-Akt. We found that the inhibitor not only inhibited the activation of PI3K-Akt pathway, but also promoted the inhibition of this pathway by AD-1 (Figures 7C, D). The activation of PI3K-Akt pathway induced by PI3K activator 740Y-P can be reversed by the addition of AD-1 (Figures 7E, F). These results indicate that AD-1 is involved in the proliferation of CRC cells by regulating the PI3K-Akt signaling pathway.
FIGURE 7. AD-1 inhibits the activation of PI3K-Akt signaling pathway. (A, B) The protein expression of p-PI3K, PI3K, p-Akt, and Akt was detected by western blot. (C, D) CRC cells were pretreated with PI3K inhibitor LY294002, the effects on PI3K-Akt signaling pathway were measured. (E, F) CRC cells were pretreated with PI3K activator 740Y-P, the effects on PI3K-Akt signaling pathway were measured. *: vs. the control group, *p < 0.05, **p < 0.01. #:vs. the LY294002 group or 740Y-P group, #p < 0.05, ##p < 0.01.
In recent years, more and more attention has been paid to the application of Chinese herbal medicine, which has become a new trend in tumor treatment. Many studies have shown that AD-1 can inhibit cell growth and promote apoptosis in tumor cells such as gastric cancer, lung cancer, breast cancer (Wang et al., 2012; Zhang et al., 2013; Zhao et al., 2016). AD-1 has the characteristics of low toxicity and multi-target prevention and treatment of CRC. The theoretical concept based on network pharmacology is similar to the multi-component and multi-target of traditional Chinese medicine, which is an effective and clear method to reveal the targets of drugs and diseases. Therefore, this study aimed to elucidate the effects of AD-1 on CRC and further verify the mechanism predicted by network pharmacology using in vitro experiments.
First, we identified 39 crossover targets from 156 potential AD-1 targets and 1711 CRC-related targets. Then, PPI network was constructed and 10 hub genes were found. These genes are involved in the regulation of signal transduction, protein phosphorylation, cell proliferation, cell migration and apoptosis process. Based on KEGG pathway analysis, PI3K-Akt pathway was one of the most important pathways with 9 enriched cross genes. Molecular docking analysis confirmed that AD-1 had a high binding potential with PI3K and Akt. Therefore, we speculate that AD-1 may affect CRC cell proliferation, migration, and apoptosis by binding to some core proteins in the PI3K-Akt signaling pathway.
Based on the above network pharmacological research, we conducted the verification of relevant experiments. In our study, AD-1 showed some anti-proliferative effects on CRC cells by cell viability measurements. In addition, AD-1 inhibits CRC cell migration. The Bcl-2 protein family is closely related to the mitochondrial apoptosis pathway, and Bax is a regulatory factor of Bcl-2, which can change the changes of mitochondrial permeability and activate apoptosis-related proteins (Hird et al., 2019). AD-1 can induce apoptosis of a variety of cancer cells, such as BGC-823, SGC-7901, MSN-28 cells (Zhao et al., 2016), lung cell carcinoma A549 and H292 cells (Zhang et al., 2013), and breast cancer MCF7 and MDA-MB-231 cells (Wang et al., 2012). Here, we assessed whether AD-1 induced apoptosis in CRC cells. The results showed that AD-1 significantly reduced the protein expression of Bcl-2 and increased the protein level of Bax, suggesting that AD-1 could induce apoptosis of SW620 and HT-29 cells and regulate the death of CRC cells. In conclusion, AD-1 has anti-proliferation and pro-apoptosis effects on CRC cells, and its specific mechanism is worthy of further study.
In recent years, the PI3K-Akt signaling pathway is widely present in a variety of cell signal transduction pathways, and is one of the hot spots in the research of malignant tumors (Dong et al., 2018). PI3K is an intracellular phosphatidyl inositol kinase composed of a catalytic subunit p110 and a regulatory subunit p85. PI3K can be activated by receptors on the cell surface to produce the second messenger phosphatidylinosite-3, 4, 5-triphosphate (PIP3), which can further activate downstream effector factors such as Akt and participate in the regulation of cell proliferation, migration, apoptosis and other signal transduction (Vanhaesebroeck et al., 2010; Ruicci et al., 2019). A large number of studies have shown that the occurrence and development of various diseases, including cancer, may be related to the abnormal activation of this pathway, such as colon cancer, non-small cell lung cancer and prostate cancer (Jiang et al., 2021; Zhu et al., 2021; Alharbi et al., 2022). In this study, we used network pharmacology to predict the potential anti-CRC mechanism of AD-1. The results showed that PI3K-Akt pathway has 9 enriched cross genes and central gene PIK3CA, suggesting that this pathway may be a key pathway involved in the anti-CRC mechanism of AD-1. To test this hypothesis, we searched the UALCAN and HPA databases and found that PI3K and Akt were highly expressed in CRC. In addition, the protein expressions of PI3K, p-PI3K, Akt, and p-Akt were detected by western blot. These results are consistent with our findings above that AD-1 significantly reduces the phosphorylation of PI3K and Akt, suggesting that PI3K-Akt may be an effective phosphorylation pathway.
In conclusion, our results suggest that AD-1 inhibits SW620 and HT-29 cell proliferation and promotes apoptosis by regulating the PI3K-Akt signaling pathway. We believe that AD-1 may be a promising therapeutic agent for CRC.
The original contributions presented in the study are included in the article/Supplementary Material, further inquiries can be directed to the corresponding authors.
Study design: JL; Data collection: JL, FL; Statistical analysis: JL, FL, YZ, and DJ; writing—review and editing: JL, FL, and DJ. All authors listed have made a substantial, direct, and intellectual contribution to the work and approved it for publication.
This work was supported by the National Natural Science Foundation of China (Grant no. 81860288).
The authors declare that the research was conducted in the absence of any commercial or financial relationships that could be construed as a potential conflict of interest.
All claims expressed in this article are solely those of the authors and do not necessarily represent those of their affiliated organizations, or those of the publisher, the editors and the reviewers. Any product that may be evaluated in this article, or claim that may be made by its manufacturer, is not guaranteed or endorsed by the publisher.
The Supplementary Material for this article can be found online at: https://www.frontiersin.org/articles/10.3389/fphar.2023.1159712/full#supplementary-material
Alharbi, K. S., Shaikh, M. A. J., Almalki, W. H., Kazmi, I., Al-Abbasi, F. A., Alzarea, S. l., et al. (2022). PI3K/Akt/mTOR pathways inhibitors with potential prospects in non-small-cell lung cancer. J. Environ. Pathol. Toxicol. Oncol. 41, 85–102. doi:10.1615/JEnvironPatholToxicolOncol.2022042281
Amberger, J. S., Bocchini, C. A., Schiettecatte, F., Scott, A. F., and Hamosh, A. (2015). OMIM.org: Online Mendelian Inheritance in Man (OMIM®), an online catalog of human genes and genetic disorders. Nucleic. acids. Res. 43, D789–D798. doi:10.1093/nar/gku1205
Bu, D. C., Luo, H. T., Huo, P. P., Wang, Z. H., Zhang, S., He, Z. H., et al. (2021). KOBAS-I: Intelligent prioritization and exploratory visualization of biological functions for gene enrichment analysis. Nucleic. acids. Res. 49, W317–W325. doi:10.1093/nar/gkab447
Daina, A., Michielin, O., and Zoete, V. (2019). SwissTargetPrediction: Updated data and new features for efficient prediction of protein targets of small molecules. Nucleic. acids. Res. 47, W357–W364. doi:10.1093/nar/gkz382
Dong, P. P., Hao, F. M., Dai, S. F., and Tian, L. (2018). Combination therapy Eve and Pac to induce apoptosis in cervical cancer cells by targeting PI3K/AKT/mTOR pathways. J. Recept. Signal. Transduct. Res. 38, 83–88. doi:10.1080/10799893.2018.1426610
Gbolahan, O., and O'Neil, B. (2019). Update on systemic therapy for colorectal cancer: Biologics take sides. Transl. Gastroenterol. Hepatol. 4, 9. doi:10.21037/tgh.2019.01.12
Han, X., Song, J., Lian, L. H., Yao, Y. L., Shao, D. Y., Fan, Y., et al. (2018). Ginsenoside 25-OCH3-PPD promotes activity of LXRs to ameliorate P2X7R-mediated NLRP3 inflammasome in the development of hepatic fibrosis. J. Agric. Food. Chem. 66, 7023–7035. doi:10.1021/acs.jafc.8b01982
Hird, A. W., and Tron, A. E. (2019). Recent advances in the development of Mcl-1 inhibitors for cancer therapy. Pharmacol. Ther. 198, 59–67. doi:10.1016/j.pharmthera.2019.02.007
Jiang, T., Wang, H. Y., Liu, L. Y., Song, H., Zhang, Y., Wang, J. Q., et al. (2021). CircIL4R activates the PI3K/AKT signaling pathway via the miR-761/TRIM29/PHLPP1 axis and promotes proliferation and metastasis in colorectal cancer. Mol. Cancer. 20, 167. doi:10.1186/s12943-021-01474-9
Li, T., Chen, Y., Li, Y., Chen, G., Zhao, Y. Q., and Su, G. Y. (2022). Antifibrotic effect of AD-1 on lipopolysaccharide-mediatedfibroblast injury in L929 cells andbleomycin-induced pulmonaryfibrosis in mice. Food. Funct. 13, 7650–7665. doi:10.1039/d1fo04212b
Miller, K. D., Nogueira, L., Mariotto, A. B., Rowland, J. H., Yabroff, K. R., Alfano, C. M., et al. (2019). Cancer treatment and survivorship statistics, 2019. Ca. Cancer. J. . Clin. 69, 363–385. doi:10.3322/caac.21565
Nogales, C., Mamdouh, Z. M., List, M., Kiel, C., Casas, A. I., and Schmidt, H. H. H. W. (2022). Network pharmacology: Curing causal mechanisms instead of treating symptoms. Trends. Pharmacol. Sci. 43, 136–150. doi:10.1016/j.tips.2021.11.004
Rebhan, M., Chalifa-Caspi, V., Prilusky, J., and Lancet, D. (1997). GeneCards: Integrating information about genes, proteins and diseases. Trends. Genet. 13, 163. doi:10.1016/s0168-9525(97)01103-7
Ren, B., Feng, J. P., Yang, N., Guo, Y. J., Chen, C., and Qin, Q. (2021). Ginsenoside Rg3 attenuates angiotensin II-induced myocardial hypertrophy through repressing NLRP3 inflammasome and oxidative stress via modulating SIRT1/NF-κB pathway. Int. Immunopharmacol. 98, 107841. doi:10.1016/j.intimp.2021.107841
Ruicci, K. M., Plantinga, P., Pinto, N., Khan, M. L., Stecho, W., Dhaliwal, S. S., et al. (2019). Disruption of the RICTOR/mTORC2 complex enhances the response of head and neck squamous cell carcinoma cells to PI3K inhibition. Mol. Oncol. 13, 2160–2177. doi:10.1002/1878-0261.12558
Sherman, B. T., Hao, M., Qiu, J., Jiao, X., Baseler, M. W., Lane, H. C., et al. (2022). David: A web server for functional enrichment analysis and functional annotation of gene lists (2021 update). Nucleic. acids. Res. 50, W216–W221. doi:10.1093/nar/gkac194
Sung, H., Ferlay, J., Siegel, R. L., Laversanne, M., Soerjomataram, I., Jemal, A., et al. (2021). Global cancer statistics 2020: Globocan estimates of incidence and mortality worldwide for 36 cancers in 185 countries. Ca. Cancer. J. Clin. 71, 209–249. doi:10.3322/caac.21660
Tan, X. Y., Gong, L. Y., Li, X. Y., Zhang, X. Y., Sun, J. H., Luo, X. H., et al. (2021). Promethazine inhibits proliferation and promotes apoptosis in colorectal cancer cells by suppressing the PI3K/AKT pathway. Biomed. Pharmacother. 143, 112174. doi:10.1016/j.biopha.2021.112174
Vanhaesebroeck, B., Guillermet-Guibert, J., Graupera, M., and Bilanges, B. (2010). The emerging mechanisms of isoform-specific PI3K signalling. Nat. Rev. Mol. Cell. Biol. 11, 329–341. doi:10.1038/nrm2882
Veenstra, C. M., and Krauss, J. C. (2018). Emerging systemic therapies for colorectal cancer. Clin. Colon. Rectal. Surg. 31, 179–191. doi:10.1055/s-0037-1602238
Wang, W., Zhang, X., Qin, J. J., Voruganti, S., Ashok Nag, S., Wang, M. H., et al. (2012). Natural product ginsenoside 25-OCH3-PPD inhibits breast cancer growth and metastasis through down-regulating MDM2. Plos. One. 7, e41586. doi:10.1371/journal.pone.0041586
Wang, X., Shen, Y. H., Wang, S. W., Li, S. L., Zhang, W. L., Liu, X. F., et al. (2017). PharmMapper 2017 update: A web server for potential drug target identification with a comprehensive target pharmacophore database. Nucleic. acids. Res. 45, W356–W360. doi:10.1093/nar/gkx374
Wei, Y. G., Yang, H. X., Zhu, C. H., Deng, J. J., and Fan, D. D. (2020). Hypoglycemic effect of ginsenoside Rg5 mediated partly by modulating gut microbiota dysbiosis in diabetic db/db mice. J. Agric. Food. Chem. 68, 5107–5117. doi:10.1021/acs.jafc.0c00605
Zhang, L. H., Jia, Y. L., Lin, X. X., Zhang, H. Q., Dong, X. W., Zhao, J. M., et al. (2013). AD-1, a novel ginsenoside derivative, shows anti-lung cancer activity via activation of p38 MAPK pathway and generation of reactive oxygen species. Biochim. Biophys. Acta 1830, 4148–4159. doi:10.1016/j.bbagen.2013.04.008 (
Zhao, C., Su, G. Y., Wang, X. D., Zhang, X. S., Guo, S., and Zhao, Y. Q. (2016). Antitumor activity of ginseng sapogenins, 25-OH-PPD and 25-OCH3-PPD, on gastric cancer cells. Biotechnol. Lett. 38, 43–50. doi:10.1007/s10529-015-1964-4
Keywords: AD-1, colorectal cancer, network pharmacology, cell apoptosis, PI3K-Akt signaling pathway
Citation: Li J, Li F, Zhao Y and Jin D (2023) Integrating network pharmacology and experimental validation to explore the effect and mechanism of AD-1 in the treatment of colorectal cancer. Front. Pharmacol. 14:1159712. doi: 10.3389/fphar.2023.1159712
Received: 06 February 2023; Accepted: 11 May 2023;
Published: 22 May 2023.
Edited by:
Seong-Gyu Ko, Kyung Hee University, Republic of KoreaReviewed by:
Meng Tian, Sichuan University, ChinaCopyright © 2023 Li, Li, Zhao and Jin. This is an open-access article distributed under the terms of the Creative Commons Attribution License (CC BY). The use, distribution or reproduction in other forums is permitted, provided the original author(s) and the copyright owner(s) are credited and that the original publication in this journal is cited, in accordance with accepted academic practice. No use, distribution or reproduction is permitted which does not comply with these terms.
*Correspondence: Yuqing Zhao, enlxNDg4NUAxMjYuY29t; Dan Jin, MDAwMDAwMjA0OEB5YnUuZWR1LmNu
†These authors have contributed equally to this work
Disclaimer: All claims expressed in this article are solely those of the authors and do not necessarily represent those of their affiliated organizations, or those of the publisher, the editors and the reviewers. Any product that may be evaluated in this article or claim that may be made by its manufacturer is not guaranteed or endorsed by the publisher.
Research integrity at Frontiers
Learn more about the work of our research integrity team to safeguard the quality of each article we publish.