- 1Nano-biotechnology and Translational Knowledge Laboratory, Department of Applied Biology, School of Biological Sciences, University of Science and Technology Meghalaya (USTM), Techno City, Meghalaya, India
- 2Centre for Herbal Pharmacology and Environmental Sustainability, Chettinad Hospital and Research Institute, Chettinad Academy of Research and Education, kelambakkam, Tamil Nadu, India
- 3Department of Biotechnology, Yeungnam University, Gyeongsan, Republic of Korea
- 4Department of Botany, School of Biological Sciences, University of Science and Technology Meghalaya (USTM), Techno City, Meghalaya, India
- 5Learning and Development Solutions, Indegene Pvt. Ltd., Manyata Tech Park, Bangalore, India
- 6Guwahati Centre, National Institute of Electronics and Information Technology (NIELIT), Guwahati, Assam, India
- 7Department of Botany, Dhemaji College, Dhemaji, Assam, India
- 8Center of Environment Climate Change and Public Health, RUSA 2.0, Utkal University, Bhubaneswar, Odisha, India
The diabetes-associated mortality rate is increasing annually, along with the severity of its accompanying disorders that impair human health. Worldwide, several medicinal plants are frequently urged for the management of diabetes. Reports are available on the use of medicinal plants by traditional healers for their blood-sugar-lowering effects, along with scientific evidence to support such claims. The Asteraceae family is one of the most diverse flowering plants, with about 1,690 genera and 32,000 species. Since ancient times, people have consumed various herbs of the Asteraceae family as food and employed them as medicine. Despite the wide variety of members within the family, most of them are rich in naturally occurring polysaccharides that possess potent prebiotic effects, which trigger their use as potential nutraceuticals. This review provides detailed information on the reported Asteraceae plants traditionally used as antidiabetic agents, with a major focus on the plants of this family that are known to exert antioxidant, hepatoprotective, vasodilation, and wound healing effects, which further action for the prevention of major diseases like cardiovascular disease (CVD), liver cirrhosis, and diabetes mellitus (DM). Moreover, this review highlights the potential of Asteraceae plants to counteract diabetic conditions when used as food and nutraceuticals. The information documented in this review article can serve as a pioneer for developing research initiatives directed at the exploration of Asteraceae and, at the forefront, the development of a botanical drug for the treatment of DM.
1 Introduction
Diabetes mellitus (DM), or insulin-dependent diabetes, is a metabolic disorder in which the blood glucose level increases above the normal threshold (Ansari et al., 2020; Ansari et al., 2021a). In this condition, the β-cells of the islets of Langerhans of the pancreas are either unable to produce insulin or their glucose-utilizing ability is inhibited. In some cases, the cells may resist insulin uptake and utilization. This disorder is a chronic illness with a global social and economic impact (Ansari et al., 2022b). Low insulin levels affect several tissues, like skeletal muscles, adipose tissues, the kidneys, and the liver, to such an extent that they downregulate the signal transduction system and the expression of associated genes. Hyperglycemia is often accompanied by several symptoms, including extreme weight loss, polyuria, and polyphagia, and under severe conditions, it can lead to ketoacidosis, which may result in death (Kharroubi and Darwish, 2015). According to the World Health Organization (WHO), around 422 million adults suffered from DM in 2014, and it was the ninth leading cause of death globally in 2019, accounting for approximately 1.5 million deaths (WHO, 2021). Interestingly, DM is a “silent” illness that can worsen due to negligence and insufficient healthcare. Approximately 47% of diabetic individuals are unaware of their condition (Adailton da Silva et al., 2018). As a result, a DM diagnosis is often met with feelings of shock and denial, which further leads to a reluctance to undergo treatment involving important lifestyle-related changes. Many synthetic drugs are available for managing and treating type-2 DM (DM2); however, they often fail to address the complications of the disease, and most available therapies seem insufficient. Most patients require regular insulin administration, and around 75% develop CVD (Goje et al., 2014; Jayaweera et al., 2022).
Obesity and DM2 have long been linked, which explains the high prevalence of DM2 in many developed countries, with DM2 also being a substantial risk factor for CVD (Piché et al., 2020; Ansari et al., 2021c). Because obesity is frequently associated with hypertension and dyslipidemia, many high-risk obese patients have a confluence of metabolic and cardiovascular risk factors. Thus, obesity (especially in its high-risk forms) is a standard driver of altered metabolic activities that lead to DM and cardiovascular risk factors that can be targeted with pharmacotherapies (Flegal et al., 2007). The relationships between obesity and high-risk cardiometabolic risk variables, such as DM, and the consequences of cardiovascular disease have been depicted in Figure 1.
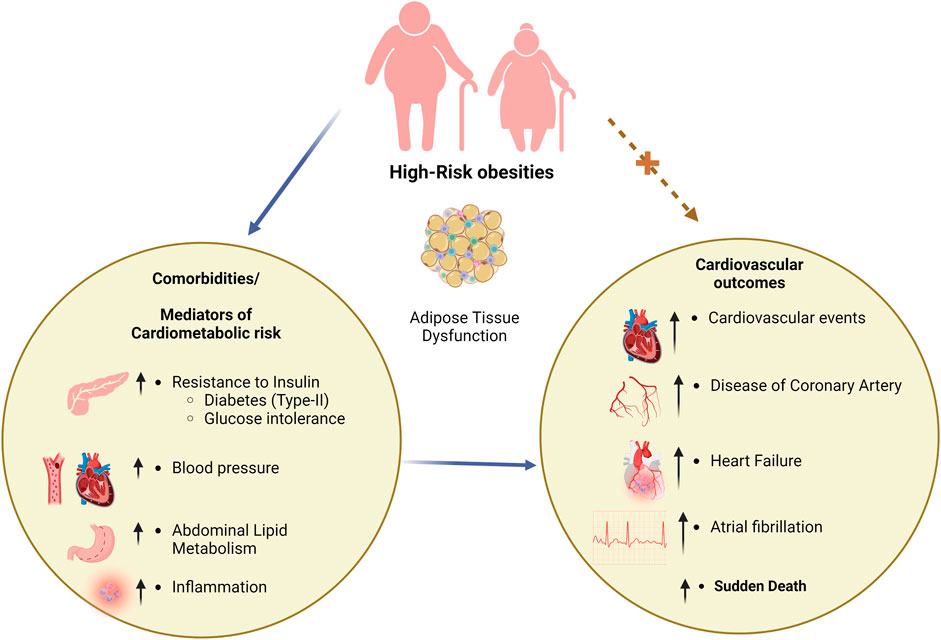
FIGURE 1. Correlation between obesity, DM, and CVD. DM; diabetes mellitus, CVD; cardiovascular disease.
The prevalence of obesity is a risk factor for developing DM2 (Flegal et al., 2007). Numerous studies using upper body, abdominal adiposity anthropometric indices, or direct imaging measurements (CT or MRI) have shown that an enlarged waistline, a higher waist-to-hip ratio, or higher levels of visceral adipose tissue (VAT) at any body mass index (BMI) level significantly raise the risk of developing DM2, in addition to overall obesity (Després, 2012). Patients with DM2 tend to be overweight and have a higher VAT than those with a similar body mass index who do not have DM. While having each of these conditions individually increases the risk of cardiovascular consequences, having both visceral or severe obesity and DM2 should exponentially increase that risk. The enhanced risk of developing cardiovascular problems and heart failure due to the increasingly common coexistence of DM2 and high-risk forms of obesity poses complex management issues. Insulin resistance, which may be partially mediated by obesity, DM2, and cardiovascular risk, is associated with ectopic and visceral adiposity. CVD mortality risk has been linked to metabolic syndrome and insulin resistance (Li et al., 2007).
The lack of available treatments and economical insufficiency to procure expensive treatments have led scientists and researchers to resort to alternative medicine. As such, botanical drugs, herbal medicines, and other dietary supplements may be suitable for treating DM.
In the present stressful world, a balanced diet and healthy lifestyle are of utmost importance. Foods fortified with high amounts of antioxidants, vitamins, and minerals are gaining tremendous importance. According to Health Line, fortified foods refer to items low in trans-fat but rich in vitamins, minerals, probiotics, fiber, and metabolites with high nutritional value and substantial health benefits (Link, 2020). A prominent issue here is that “nutraceuticals” refer to the active components present in functional foods that can be extracted, purified, and concentrated for use and have different usages apart from being “functional foods.” An online search on PubMed and Google Scholar demonstrates that diet management is an essential parameter for preventing and curing diseases such as CVD, DM, hypertension, obesity, and thyroid disorders (Chakrabartty et al., 2022). A large body of clinical evidence suggests that DM2 and its associated complications can be prevented. The risk of disease can be avoided by the consumption of foods that are rich in antioxidants, have reasonable glycemic control, regulate blood pressure, promote the growth of favorable gut microbes, lower inflammatory cytokine secretion, and activate the production of “good” enzymes and hormones (Alkhatib et al., 2017). It is interesting to note that the traditionally used Mediterranean diet (MD) is considered one of the healthiest diets for human longevity and the prevention of chronic illnesses like DM, as it is rich in secondary metabolites and other components that are recommended for the prevention of DM (Rivera et al., 2010; Mirmiran et al., 2014). Many plant families like Zingiberaceae (e.g., Alpinia galanga (L.) Wild.), Lamiaceae (e.g., Salvia officinalis L.), Caryophyllaceae (e.g., Corrigiola litoralis sub sp. foliosa (Pérez Lara) Devesa), and many others have been consumed traditionally for many generations due to their many health benefits (Ansari et al., 2021b; Ansari et., 2022a; Ansari et al., 2022d).
For a long time, plants have had a strong foothold in traditional medicine and form the basis of modern-day medications. Age-old medicine practices like Ayurveda and Unani have practitioners who rely on different plants and their parts to treat various diseases, and these treatment systems are still practiced in Southeast Asia (Ansari et al., 2022a; Ansari et al., 2022c). However, the problem with traditional medicines is the lack of documentation, authenticity, and scientific evidence. Most of the ‘bej, ‘kobiraj,’ or local healers have learned the art and knowledge of traditional healing from their ancestors, having been handed down as a legacy. According to the WHO, most of the global population relies on herbal medicines and drugs to treat diseases, from wound healing to CVD treatments. Interestingly, some of these medicinal plants find their use as one or more culinary spices and are consumed as food. One of the most common examples in this respect is the Zingiberaceae family, the natural ‘pharmacy’ of the plant kingdom and the rich flavoring ingredient of the kitchen. Alpinia nigra (Gaertn.) Burtt, a lesser explored member of this family, is traditionally used to treat gut infections caused by Fasciolis buski and is also used to prepare delicacies like ‘eromba’ and ‘deragong’ in Manipur and Tripura, respectively (Swargiary et al., 2013; Swargiary and Verma, 2015; Chakrabartty et al., 2019). Asteraceae is one such family of medicinal plants that has not been explored much but houses a wide range of plants to treat diseases (Ansari et al., 2021d).
Asteraceae, or Compositae, also known as the ‘daisy’ family, is a large family of angiosperms that harbors around 32,000 plant species with a cosmopolitan distribution, growing in all regions of the world except Antarctica. These plants have been used as medicines and as part of the human diet for ages. This family includes some well-known medicinal plants like lettuce, chamomile, and dandelion, as well as ornamental plants like daisies, sunflowers, chicory, and dahlias (Aboul Ela et al., 2012; Michel et al., 2020). Some of these plants and their parts are caffeine-free, extremely rich in vitamin C, E, and organic acids, and commonly used in salads and sandwiches. As such, these plants can be widely cultivated as sources of functional foods. Different species of Asteraceae have been reported to exhibit other pharmacological properties that can be attributed to the presence of large quantities of phytochemicals and secondary metabolites, including essential oils, lignans, tannins, flavonoids, alkaloids, phenolic acids, and saponins. Most of these plants have many sesquiterpene lactones, which impart a bitter taste (Djellouli et al., 2013; Rolnik and Olas, 2021). Secondary metabolites are responsible for antioxidant, anticancer, and antimicrobial properties; hence, the plants of the Asteraceae family can be exploited for the treatment of certain serious diseases like CVD, hypertension, and DM.
The present review aims to bridge the gap in existing literature regarding the role of several members of the family Asteraceae, particularly in preventing DM2 and diabetic wounds, and their application as fortified foods. Extensive literature is available on the role of medicinal plants in the management of lifestyle-related disorders. But this review is novel as it focuses explicitly on managing DM2 using different members of the Asteraceae family alone. Not only that, the utilization of secondary metabolites and bioactive compounds from this family as dietary supplements and fortified foods is highlighted, specifically focused on the management of DM. This review is subdivided into different sections: Section 2 describes the importance of this family in ethnopharmacology and traditional medicine. Section 3 highlights the role of the Asteraceae plant metabolites in treating and preventing DM. In Section 4, the role of different members of the Asteraceae in wound healing has been described in detail. Section 5 describes the untapped and identified compounds from Asteraceae against DM. Sections 6 and 7 discuss the prospects of research in Asteraceae (in search of novel bioactive compounds to be used as medicines and fortified foods). The challenges and opportunities to utilize and establish the Asteraceae family plants as medicines and nutraceuticals have also been discussed in Section 8, and the concluding remarks on the present study have been discussed in Section 9.
2 Asteraceae: the largest family with diverse medicinal properties
The capacity of plants to produce secondary metabolites with considerable biological activity has long made them an integral part of the evolution of medicine. Traditional medicine relies heavily on plant remedies to address various health issues. Conventional medications derived from plants are readily available to the local population, are economical, and have no side effects, as supported by many years of successful human use. In recent years, more advanced analytical techniques have been developed, identifying several new phytochemicals.
Asteraceae is one of the largest plant families, with 32,000 accepted species names under 1,690 accepted genera (POWO, 2022). A few prominent genera include Senenio (1,470 spp.), Vernonia (1,050 spp.), Cousinia (600 spp.), Eupatorium (590 spp.), Centaurea (590 spp.), Hieracium (470 spp.), Helichrysum (460 spp.), Saussurea (300 spp.), Cirsium (270 spp.), Aster (240 spp.), and Bidens (210 spp.). Many species are cultivated, including several widely used culinary botanical drugs, viz., Artemisia annua L., Blumea balsamifera (L.) DC., and Calendula officinalis L., (Rodríguez-Chavez et al., 2017). For centuries, the species of the family Asteraceae have been used worldwide as traditional medicine against various human ailments, including DM, kidney, heart, lung, liver, and skin toothache inflammation, pain, constipation, toothache, throat pain, snake bite, headache, gastrointestinal disorders, diarrhea, dysentery, tuberculosis, hepatitis, asthma, menopausal and menstrual disorders, stomach ulcers, sores, scabies, filariasis, elephantiasis, night-blindness, impotence, hair fall, jaundice, nose bleeding, allergies, viral infections, cough, bronchitis, different types of cancers, wounds and cuts, and malaria (Bessada et al., 2015; Panda and Luyten, 2018; Panda et al., 2019; Michel et al., 2020; Garcia-Oliveira et al., 2021; Woldeamanuel et al., 2022).
Artemisia is the other important member of this family, considered to comprise >500 medicinal species in the world (Martín et al., 2003; Bisht et al., 2021). Traditionally, the members of Artemisia have been used to treat several diseases like hepatitis, cancer, inflammation, DM, and infections caused by microorganisms (Asad et al., 2011). The discovery of artemisinin, which resulted in the 2015 Nobel Prize in Physiology or Medicine, has revolutionized the treatment of malaria worldwide (Panda and Luyten, 2018).
The Achillea genus is well known for its use in traditional medicine (Mohammadhosseini et al., 2017; Jarić et al., 2018). The greatest research has been done on Achillea millefolium L. Yarrow, a perennial plant native to the temperate regions of Europe and Asia. It has been utilized by humans for well over 3,000 years (Ali et al., 2017).
The genus Achyrocline (distributed across Latin America) is well-known for its medicinal properties (Retta et al., 2012; Bolson et al., 2015). Reports from ethnobotanical studies conducted in the Brazilian state of Rio Grande do Sul showed that the plant Achyrocline satureioides (Lam.) DC. is often used as a healing agent (Carvalho et al., 2018).
As early as the 13th century, Europeans used the C. officinalis (or calendula) plant to cure wounds. Since then, several cosmetic products have been created using components derived from calendula (Parente et al., 2012). In the middle ages, Calendula flowers were used for liver obstructions, snake bites, and heart strengthening (Cetkovic et al., 2004).
Since ancient times, cardoon (Cynara cardunculus L.) has played an essential role in the culinary and medicinal traditions of the Mediterranean. A wide variety of therapeutic properties, such as antioxidant, lipid-lowering, anti-inflammatory, antidiabetic (related to DM), antibacterial, and anticancer abilities, have been attributed to the various bioactive substances of Cardoon (Silva et al., 2022).
Eclipta prostrata (L.) L. is a plant that is known by several names in India and is reported to be used as a treatment for skin, respiratory (asthma), and hepatic (jaundice) conditions, gastrointestinal disorders, and spleen enlargement (Dalal et al., 2010; Jahan et al., 2014). In Nepal, people utilize its leaves and young stems to cure wounds and prevent infections (Manandhar, 2002; Adhikari et al., 2019), and many Bangladeshi ethnic groups use it to cure jaundice. Additionally, it is used to treat acidity, alopecia (Roy et al., 2008), gingivitis, fever, bodily aches, asthma, bronchitis, burns, constipation, wounds, wrinkles, edema, pimples, and other skin conditions (Kumari et al., 2006; Tewtrakul et al., 2007; Khan and Khan, 2008; Neeraja and Margaret, 2011).
Numerous communities have recognized and used the medicinal properties of the plants in the genus Pluchea (Gridling et al., 2009; Schmidt et al., 2009; Ab Rahman et al., 2014).
Solidago virgaurea L., European goldenrod (or woundwort), is a well-studied common medicinal plant across Europe and other areas. European goldenrod has been used for centuries to cure diseases of the urogenital tract (Toiu et al., 2019). In different parts of the world, its infusion or decoction is used in traditional medicine to treat bacterial infections and inflammation (Gross et al., 2002).
For a long time of people using Taraxacum sp. (dandelion) as a therapeutic herb. It is used as a food item in many countries for preventing and treating DM2. Because of its hepatic and hyperglycemic properties, dandelion has been used traditionally as a folk medicine in Russia, China, and India (Kemper, 1999). It is often used as a meal (in salads) because of its high vitamin and mineral content (Escudero et al., 2003). Dandelion is a popular traditional medicine in Turkey and Mexico used to manage DM2 (Onal et al., 2005).
Xanthium strumarium L. has been used as a medicine in China for centuries, and it is one of the most widely used herbal medications for treating rhinitis and headaches. In addition, X. strumarium has been documented as a traditional herbal remedy for various health issues in Bangladesh, including urinary tract infections, ear infections, DM, and indigestion (Islam et al., 2009).
Gundelia tournefortti L. is a plant with seeds that is often used to make pickles and acts as a diuretic (Çoruh et al., 2007). Traditional Brazilian medicine prescribes it for renal and cardiac ailments. Clinical research found that the anticoagulant properties of Emilia praetermissa Milne-Redh. helped improve hyperlipidemic disorders (Memariani et al., 2018). Another plant with significant ethnopharmacological value for wound healing is Silybum marianum (L.) Gaertn (Hudaib et al., 2008; Aziz et al., 2016; Tabari et al., 2019).
2.1 Asteraceae with particular references to traditional uses in the treatment of DM
The Asteraceae family is the group of plants that have been traditionally used to treat DM (Table 1) and has received the greatest attention. However, not all of them have been thoroughly validated by scientists. This includes Artemisia afra Jacq. ex Willd., Brachylaena elliptica (Thunb.) Less, Brachylaena discolor DC, Helichrysum gymnocomum DC, Helichrysum nudifolium (L.) Less, Helichrysum odoratissimum (L.) Sweet., and Helichrysum petiolare Hilliard & B. L. Burtt., Conyza scabrida DC., Schkuhria pinnata (Lam.) Kuntze ex Thell., Tarchonanthus camphoratus L., Pteronia divaricata (P. Bergius) Less., Vernonia oligocephala Katt., and Vernonia amygdalina Delile (Odeyemi and Bradley, 2018). A fair number of plants in this family have been shown to have a variety of effects on the body, including lowering blood sugar levels, increasing insulin production, repairing damaged pancreatic β-cells, inhibiting carbohydrate digesting enzymes, and protecting against oxidative stress (Gyang et al., 2005; Osinubi, 2006; Atangwho et al., 2008, 2013; Ebong et al., 2008; Okolie et al., 2008; Deutschländer et al., 2009; Afolayan and Sunmonu, 2010; van Huyssteen et al., 2011; Wintola and Afolayan, 2011; Mellem, 2013; Sunmonu and Afolayan, 2013; Mellem et al., 2015). Most of the anti-diabetic molecules retrieved from these plants represented saponins, flavanones, tannins, and flavonoids (aglycones) (Mukherjee et al., 2006; van Huyssteen et al., 2011; Sunmonu and Afolayan, 2013).
Several Asteraceae plants have also been reported, in Indian Ayurvedic medicines, e.g., Eclipta alba for skin diseases (Islam et al., 2012), Ageratum conyzoides, Artemisia capillaries, and Inula cappa for DM (Rai and Lalramnghinglova, 2011; Jang et al., 2015). The decoction of whole plants and roots of Blumea laciniata and B. lacera has been used to cure bronchitis, blood diseases, fever, burning sensations, and snake bites by the tribes of India (Shukla et al., 2010; Rai and Lalramnghinglova, 2011; Rosangkima and Jagetia, 2015). The extracts of Ageratum conyzoides L., Bidens pilosa L., and B. balsamifera (L.) DC. have been traditionally used as wound-healing agents and cancer treatments in India (Adebayo et al., 2010; Bartolome et al., 2013). Some species of Vernonia are most extensively reported as traditional medicine for treating diseases of the skin, central nervous system, kidney, gastrointestinal tract, metabolism, and general health (Toyang and Verpoorte, 2013; Carvalho et al., 2018).
3 Asteraceae: undisputable metabolites of interest in DM
The rate of diabetes is increasing globally, including in India, now known as the diabetic capital of the world. Traditional knowledge and the use of medicinal plants to treat severe diseases like DM are emerging again in countries like India because of the fewer side effects and treatment-associated risks. Many members of different plant families like Asteraceae, Zingiberaceae, Lamiaceae, Myrtaceae, and others have been exploited for their antidiabetic properties (Bindu and Narendhirakannan, 2019; Chakrabartty et al., 2020; Michel et al., 2020). Therefore, this review focused on studying the Asteraceae family. In the subsequent sections, five major anti-diabetic plants belonging to this family and their mechanisms of action are discussed in detail.
3.1 Taraxacum officinale (L.) Weber ex F.H.Wigg. (dandelion)
Dandelion is a folk medicine in Russia, India, and China for its hepatic and hyperglycemic properties (Wirngo et al., 2016). In many parts of the world, it is utilized as food and, sometimes, as a treatment approach for DM. This plant has bioactive compounds, such as chicoric acid, taraxasterol (TS), chlorogenic acid, and sesquiterpene lactones, that carry an anti-diabetic potential (Figure 2) (Schütz et al., 2006; González-Castejón et al., 2012). The root of the dandelion contains inulin, which includes fructooligosaccharides (FOS), and can help normalize blood sugar levels and reduce hyperglycemia. It also impacts insulin secretion and sensitivity, making it an attractive option for use as an anti-diabetic drug (Salehi et al., 2019).
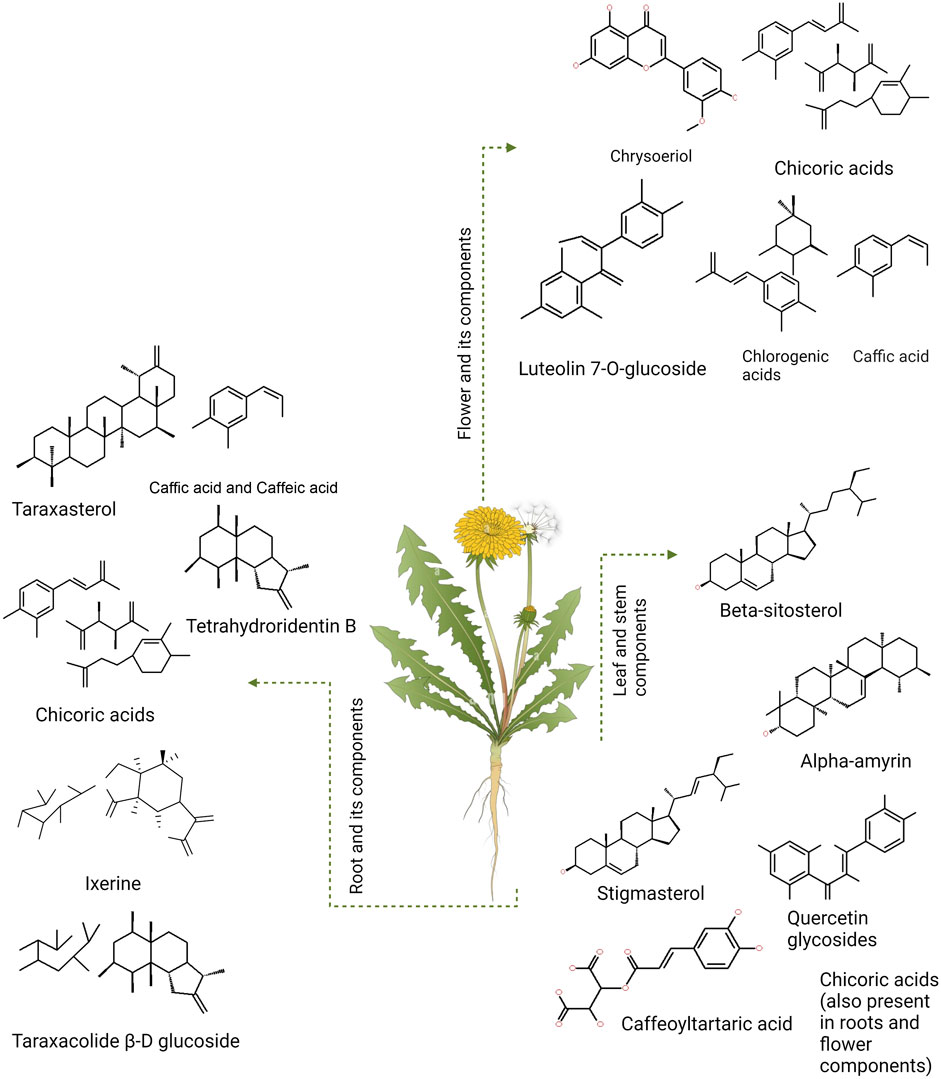
FIGURE 2. Taraxacum officinale (dandelion) and the components present different parts of the plant (flowers, stems, and leaves).
Insulin resistance is the primary cause of hyperglycemia and a hallmark of DM2 pathogenesis (Hamden et al., 2008). Another well-known mechanism that affects glucose homeostasis is oxidative stress, which is driven by auto-oxidation and protein glycation (Giugliano et al., 1996). Studies have shown that dandelion extract may stimulate the release of insulin in pancreatic β-cells (Figure 2), which subsequently counteracts the effects of hyperglycosemia (Hussain et al., 2004).
Dandelion extract exerts anti-hyperglycemic properties in non-obese diabetic mice (Petlevski et al., 2003). Chicory acid (CRA) stimulates pancreatic insulin production, which enhances glucose absorption in muscle cells (Tousch et al., 2008). CRA and TS block α-glucosidase and α-amylase, preventing the digestion of complex carbohydrates like starch. In diabetic mice, dandelion reduces plasma glucose levels, improving β-cell insulin secretion (Schütz et al., 2006). Chlorogenic acid (CGA), CRA, taraxasterol (TS), and sesquiterpene lactones (SEL) (Figure 3) have considerable promise as DM regulators. Research suggests that TS may be the strongest regulator of DM (Akashi et al., 1994; Williams et al., 1996).
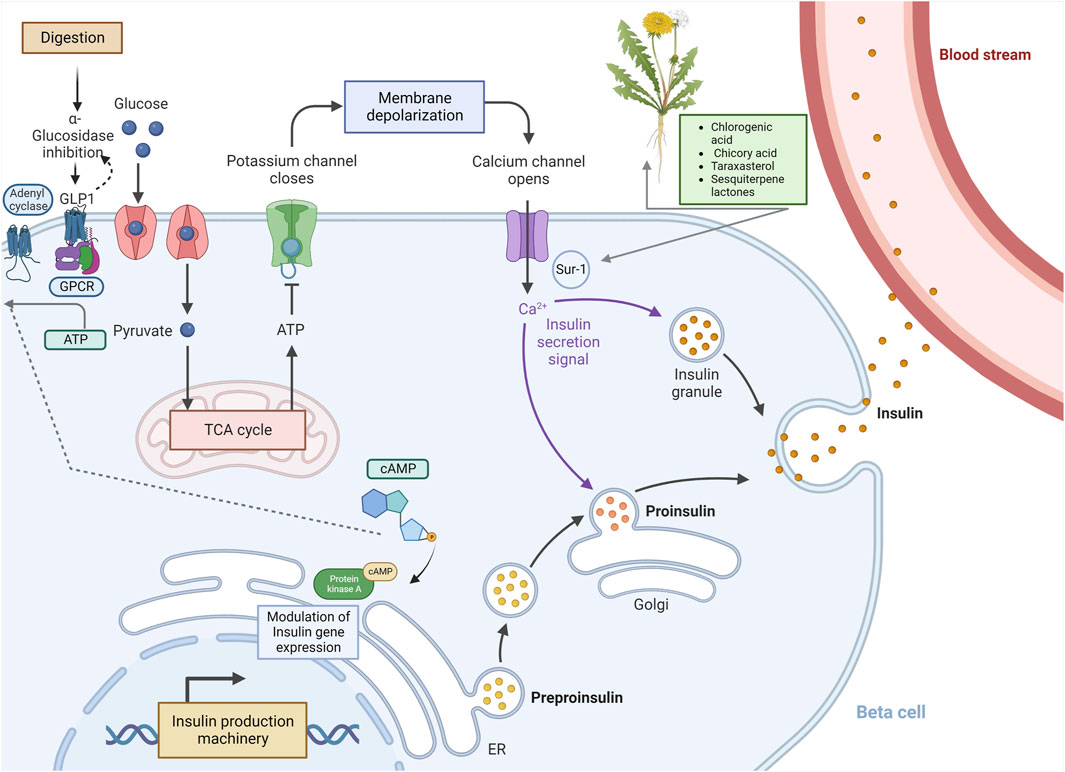
FIGURE 3. Potential biological mechanisms of Taraxacum officinale (dandelion)-derived compounds that modulate cAMP and insulin secretion. The diagram specifies the potential means of bioactive components from T. officinale (CGA, CRA, TS & SEL) on insulin secretion and the c-AMP pathway. The DM2 can give rise to several defects, including low response in β-cells (at the receptor or transportation platforms such as GULT2, SUR1, G-protein coupled receptor, and gene expression), glycolysis, Krebs’ cycle, and enzymatic digestion. Bioactive components may act directly or indirectly in a series of processes, thereby modulating and regulating DM2 defects and responses. DM2: diabetes mellitus type 2.
DM treatments produced from medicinal plants could potentially be more cost-effective and accessible after extensive study. At the same time, research into the effects and mechanisms of action of dandelion’s bioactive components has previously been conducted in vitro and in vivo. Identifying the specific bioactive components of the plant and the associated mechanisms of action in DM requires in vitro studies on clonal β-cell (INS-1E), α-cell, and human skeletal cell lines. As a result, this study can yield new information for improving diabetic treatment, which will undoubtedly lessen the global societal and economic burden caused by DM (Wirngo et al., 2016).
The efficacy and safety of the dandelion extract as a treatment for DM2 might be easily studied with clinical trials; however, those are still missing while there is clinical trial data available on the effect of this extract on other conditions such as eczema and migraines (https://clinicaltrials.gov/ct2/results?cond=Taraxacum+officinale+&term=&cntry=&state=&city=&dist=). Moreover, the bioavailability and metabolism of the individual components of the dandelion extract necessitate more studies.
3.2 Cynara cardunculus L. (Cardoon)
Abnormal blood glucose levels due to a deficiency in insulin secretion and/or insulin activity characterize DM. C. cardunculus has been studied by numerous scientists for its anti-diabetic properties and putative mechanisms of action. Ben Salem et al. (2017) tested the in vitro α-amylase inhibitory potential of C. cardunculus, and the ethanolic extract was found to be the most potent, with an IC50 value of 72.22 ug/mL (higher than that of an acarbose-specific inhibitor, which was 14.83 ug/mL). Furthermore, the scientists tested the ethanol leaf extract of C. cardunculus under alloxan-induced stress in Wistar rats to see if it had any anti-diabetic effects. For 28 days, Wistar rats were given 200 or 400 mg/kg of artichoke leaf extract (ALE) as a dietary supplement. When given to diabetic rats, ALE reduced their serum α-amylase levels, reducing their blood glucose rate. Additionally, diabetic rat lipid profiles and antioxidant activity were impacted by ALE treatment. Moreover, Kuczmannová et al. (2016) evaluated the anti-diabetic effects of Agrimonia eupatoria L. and C. altilis infusions (200 mg/L) by tracking changes in serum glucose levels, α-glucosidase inhibitory activity, advanced glycation end products (AGEs) production, and butyrylcholinesterase activity. Although artichoke extract did not affect α -glucosidase activity, it lowered serum glucose levels and prevented the production of AGEs. Similarly, significant reductions in serum glucose and triglyceride concentrations were observed in rats with streptozotocin (STZ)-induced DM, after oral administration of ALE (200 and 400 mg/kg) (Heidarian and Soofiniya, 2011). Patients with metabolic syndrome were given ALE (1800 mg/daily) supplements for 12 weeks in a clinical experiment performed by Ebrahimi-Mameghani et al. (2018). Both insulin and the homeostasis model assessment of insulin resistance (HOMA-IR) levels dropped after ALE administration (Ebrahimi-Mameghani et al., 2018). These studies validated cardoon’s anti-diabetic action, which may be connected to its chlorogenic acid due to its glucose 6-phosphate-suppressing properties (Arion et al., 1997; Loi et al., 2013), and caffeoylquinic acid, which regulates α-glucosidase activity (Matsui et al., 2006). Two clinical studies are available so far on ALE treatment for hypercholesterolemia, insulin sensitivity, obesity, and excess weight (https://clinicaltrials.gov/ct2/results?cond=Cynara+cardunculus+&term=&cntry=&state=&city=&dist=&Search=Search).
Cardoon is a Mediterranean medicinal plant with bioactive substances such as dietary fibers, sesquiterpene lactones, and phenolic compounds. Further research is needed to understand the mechanism of action of cardoon metabolites fully. Although few clinical trials have been completed already, more studies are necessary to clarify the therapeutic dose and duration of treatment (Silva et al., 2022).
3.3 Eclipta prostrata (L.) L
E. prostrata (syn. Eclipta alba (L.) Hassak) is an essential medicinal plant in the tropical and subtropical regions (Rahman et al., 2011; Feng et al., 2019; Sanyal et al., 2022; Silalahi, 2022). A whole-plant methanol extract of E. prostrata was tested for its in vitro α-amylase inhibitory activities. With an IC50 value of 322.138 ± 0.025 μg/mL, the results showed moderate effectiveness in α-amylase inhibition, suggesting a possible anti-diabetic function (Alam and Sharma, 2020; Khan et al., 2020; Timalsina and Devkota, 2021). STZ (at a dose of 70 mg/kg of body weight) was injected into the peritoneum of rats in another experiment to induce DM. Wedelolactone from E. prostrata reduced the HbA1c level in diabetic rats (10.3% ± 0.72%) in the untreated group compared to the wedelolactone-treated diabetic group (7.2% ± 0.52%). Wedelolactone was able to reverse the abnormal increase in biochemical parameters, including urea and creatinine, seen in the STZ group. After 28 days of treatment with wedelolactone, the hepatic parameters, c-peptide and insulin secreted from β-cells, were shifted toward normal levels, confirming the effectiveness of the treatment (Shahab et al., 2018; Zhang et al., 2022). The α-glucosidase activity was inhibited via a dose-dependent mechanism by the E. prostrata ethanolic extract (10 μg/mL, enzyme activity was reduced by 88.6%, IC50 = 4.5 μg/mL) (Jaiswal et al., 2012). Moreover, when treated with this extract, pancreatic β-cells are also reported to be restored and regenerated (Hemalatha et al., 2006).
E. prostrata leaf suspension (2 and 4 g/kg) was orally tested for its antidiabetic effects (Figure 4) in rats with alloxan-induced DM. The extract normalized biochemical markers that were dramatically shifted due to DM. The anti-hyperglycemic action of E. prostrata in rats was demonstrated by an increase in liver hexokinase activity and a decrease in blood glucose level and glycosylated hemoglobin as a result of decreased activity of glucose-6 phosphatase and fructose-1,6-bisphosphatase (Ananthi et al., 2003; Yadav and Yadav, 2023). Although antidiabetic effects have been observed, the molecular mechanism behind these effects is poorly understood. Two clinical studies have been made available so far on the use of this extract for the treatment of chronic hepatitis B, HIV-I-infection, and AIDS (https://clinicaltrials.gov/ct2/results?cond=Eclipta+prostrata+&term=&cntry=&state=&city=&dist=&Search=Search). Still, future clinical studies about DM are necessary.
3.4 Xanthium strumarium L
X. strumarium is a common and well-known traditional Chinese herbal medicine, usually called Cang-Er-Zi, used for thousands of years in China. The dose-dependent, powerful hypoglycemic action of WEX (15 and 30 mg/kg, i.p.) was shown in normal rats by Kupiecki et al. (1974). Researchers studied the anti-diabetic effects of caffeic acid extracted from X. strumarium in 2000, using STZ-induced hyperglycemic and insulin-resistant rat models (Figure 3). Caffeic acid (0.5–3.0 mg/kg) reduced plasma glucose levels by enhancing glucose utilization (Hsu et al., 2000). In normal-glycemic and STZ-induced hyperglycemic rats, Narendiran et al. (2011) reported that MEXs at 100 and 200 mg/kg (p.o., for 30 days) had outstanding antidiabetic action. The competitive inhibition of aldose reductase (AR) and galactitol production in rat lenses by methyl-3, 5-di-O-caffeoylquinate was previously effective in preventing diabetic complications (Yoon et al., 2013). In addition, the CFMEXL also showed significant inhibitory activity against the α-glucosidase enzyme, with an IC50 of 72 μg/mL (Khuda et al., 2014). Similarly, additional research demonstrated that MEX possessed a potent α-glucosidase inhibitory activity with an IC50 value of 15.25 μg/mL (Ingawale et al., 2018). Clinical studies are warranted to validate the active constituents of this plant as a treatment for DM.
3.5 Solidago virgaurea L
S. virgaurea (European goldenrod) is among the most researched species in its genus and is widely used for the treatment of different diseases in Germany, the Czech Republic, Poland, the Russian Federation, Ukraine, Bulgaria, Romania, the Republic of Moldova, Korea, and China. In an alloxan-induced diabetic rat model, a hydroalcoholic extract of S. virgaurea caused a decline in glycemia, TNF-α, serum α-amylase activity, and pancreatic malondialdehyde, and a rise in serum insulin, hepatic glycogen, pancreatic superoxide dismutase (SOD), and catalase activities (Fursenco et al., 2020; Sanad et al., 2022; Zehra et al., 2023). Clinical studies are warranted to further validate the active constituents of this plant as a treatment for DM.
4 The role of asteraceae in diabetic wound healing
Large lesions and/or loss of function in the affected areas result from severe wounds. The presence of pathogens like Staphylococcus aureus and Pseudomonas aeruginosa makes wound care even more complicated, especially for people with chronic DM (Carvalho et al., 2018). Hence, novel wound-healing therapies must be developed without delay. Several members of the Asteraceae family are used as ethnomedicinal plants with wound-healing properties. The pharmacological qualities of these plants have been the basis of innovative and effective medicines, and the evidence collected from traditional medicine has paved the road for their development. Plants of the Asteraceae (Figure 5) have been extensively studied. Some of their pharmacological effects have been recognized, because of their widespread occurrence and ethnopharmacological significance, such as wound-healing, anti-inflammatory, antibacterial, antioxidant, and protozoa-fighting properties (Babotă et al., 2018; Özbilgin et al., 2018; George Kallivalappil and Kuttan, 2019; Ghaderi and Sonboli, 2019). These include C. officinalis (Dinda et al., 2016), and Neurolaena lobata (L.) R.Br. ex Cass, Achillea asiatica Serg (Dorjsembe et al., 2017), Achyrocline alata (Kunth) DC.(Pereira et al., 2017), Acmella oleracea (L.) R.K.Jansen (Maria-Ferreira et al., 2014), Artemisia princeps Pampanini (Lee et al., 2014), Blumea balsamifera (L.) DC. (Pang et al., 2017), Pluchea indica (L.) Less. (Sabri and Handayani, 2022), Achyrocline satureioides (Lam.) DC. (Alerico et al., 2015), Artemisia asiatica (Pamp.) Nakai ex Kitam (Park et al., 2008), Artemisia argyi H. Lév, and Vaniot. The proliferation of keratinocytes, and extracellular matrix remodeling, have been hypothesized to be essential for successful wound healing (Speroni et al., 2002; Rosa et al., 2014). Here, we discussed the most common antidiabetic plants of the Asteraceae, their bioactive compounds, and the mechanism of action presented in Table 2.
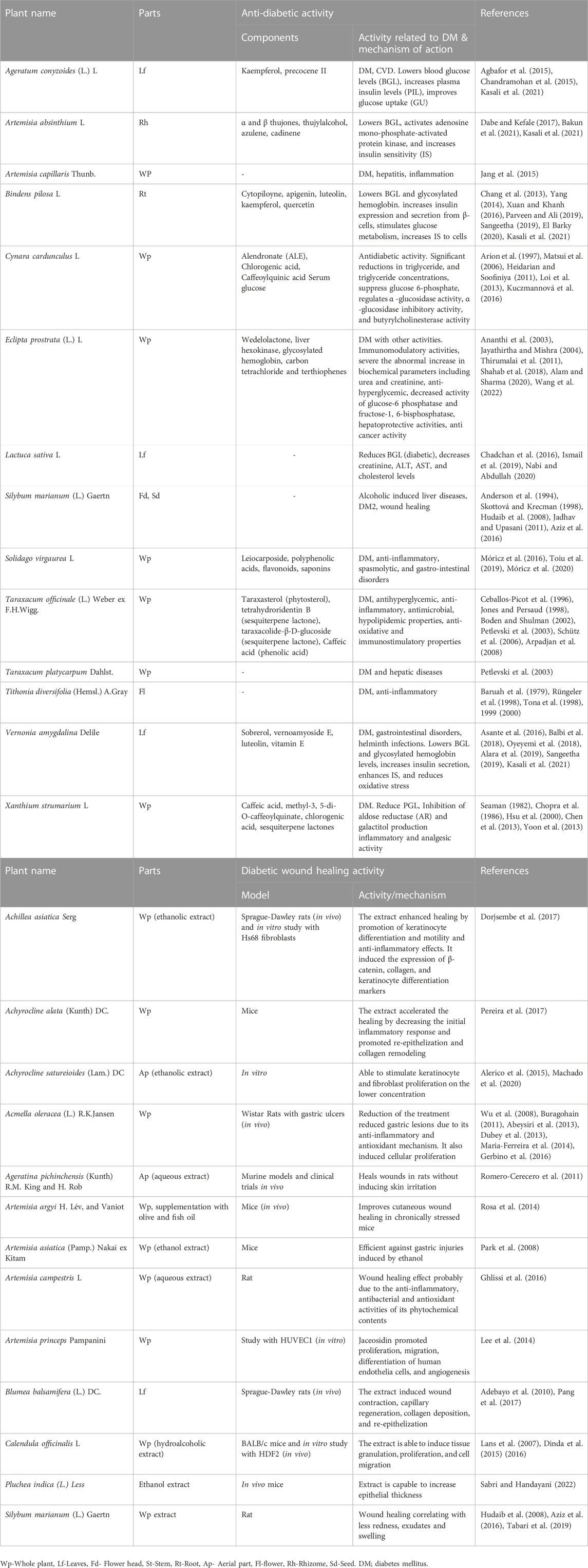
TABLE 2. Plants/compounds of the Asteraceae family that possess anti-diabetic and diabetic wound healing activities.
B. balsamifera is used in traditional Asian medicine as Ainaxiang, rich in volatile compounds such as L-borneol (a primary constituent), terpenoids, fatty acids, phenols, alcohols, aldehydes, ethers, ketones, pyridines, furans, and alkanes (de Boer and Cotingting, 2014). The volatile oil improved angiogenesis, collagen and epithelium deposition, and granular tissue development in injured Kun-Ming mice. This effect on wound healing and cell proliferation was linked to increased neuropeptide production (Pang et al., 2014a; Pang et al., 2014b). The volatile oil speeds up the recovery of Sprague-Dawley rats with burns by releasing growth factors and reducing pro-inflammatory cytokines (TNF and IL-1) (Fan et al., 2015). One week after the treatment with flavonoid-rich B. balsamifera leaf extract on Sprague-Dawley rat skin wounds, wound contraction, capillary regeneration, collagen deposition, and re-epithelialization occurred. These changes increased the expression of VEGF, TGF-β1, and CD68 in rat wound tissues.
S. marianum, also known as silymarin, is a wound-healing herb (Aziz et al., 2016). Increased epithelization and reduced inflammation are two benefits of silymarin, as observed in albino rats with excision wounds. Its extract has been shown to prevent oxidative damage in fibroblasts caused by lipopolysaccharides (Sharifi et al., 2012; Sharifi et al., 2013). In a similar vein, the flavonoid silibinin, which is derived from the silymarin herb, has been shown to speed up wound healing in rats by increasing the production of the extracellular matrix components stromelysin hydroxyproline, glycosaminoglycans, and collagen (Tabandeh et al., 2013). The cutaneous toxicity of nitrogen mustard is mitigated in mice by silibinin (Balszuweit et al., 2013); this further helps lower oxidative stress and inflammation (Jain et al., 2015). Another study found that a silibinin-based gel, applied topically for 14 days, helped wound healing by hastening epithelization, collagen production, and granulation tissue deposition (Samanta et al., 2016).
C. officinalis has been used in Europe as a wound treatment since the 13th century (Cioinac, 2016). Many cosmetic and personal care products include their components. In one study, an ethanolic extract from C. officinalis flowers and its dichloromethane and hexane fractions increased angiogenesis in embryonated eggs and rats with skin lesions. This effect on the vasculature was due to inflammatory cell infiltration and collagen deposition (Parente et al., 2012). C. officinalis tincture increased fibroblast proliferation and migration via the PI3K and FAK/Akt pathways. This extract included mostly flavonol glycosides (Dinda et al., 2015). Moreover, human keratinocytes treated with C. officinalis flower extracts (n-hexane and ethanolic extracts) boosted IL-8 and NF-kB expression and migratory cell capacity. The extract also inhibited collagenase action in human dermal fibroblasts. It is hypothesized that flavonoids and saponins are responsible for these effects (Nicolaus et al., 2017). The hydroglycolic extract of C. officinalis (Plenusdermax) enhanced wound epithelization, reducing healing time in venous leg ulcer patients (Ahmad et al., 2012; Buzzi et al., 2016). Another study found that low-intensity laser therapy combined with C. officinalis oil reduces lesions in diabetic foot ulcers (Carvalho et al., 2016).
Traditional medicine uses the Achillea genus for therapeutic purposes (Mohammadhosseini et al., 2017). A. millefolium is a herb native to Europe and Asia that has been utilized for over 3,000 years (Ali et al., 2017). Its anti-inflammatory, antioxidant, antifungal, and therapeutic activities are attributed to sesquiterpenes and phenolic compounds in this plant (Karamenderes and Apaydin, 2003; Fierascu et al., 2015). An in vitro study with human skin fibroblasts showed that the hydroalcoholic extract from the aerial parts of A. millefolium induces cell proliferation (Ghobadian et al., 2015). More recently, oil extracts from aerial parts of A. millefolium reduced skin irritation in healthy individuals (Tadić et al., 2017). A. asiatica (syn. of A. millefolium var. Manshurica Kitam), also called Mongolian yarrow, has therapeutic properties. In vitro, treatment of the aerial portions of this plant with Hs68 fibroblasts stimulated collagen synthesis via transformation of growth factor-mediated pathways. The same extract increased β-catenin, Akt, and keratinocyte differentiation markers, boosting keratinocyte differentiation and motility. Chlorogenic acid, apigenin-7-O-glucoside, and schaftoside are connected to the A. asiatica ethanolic extract’s therapeutic benefits (Dorjsembe et al., 2017).
P. indica is an evergreen shrub predominantly distributed in South Africa and Thailand. P. indica tea is widely recommended for promoting health in Southeast Asia. The findings suggest that ingesting this substance may potentially mitigate weight gain and hyperglycemia in rats fed a high-fat diet. It may be helpful in the treatment of hyperglycemia, dyslipidemia, obesity, and wound healing (Sirichaiwetchakoon et al., 2020; Sabri and Handayani, 2022). DM1 and DM2 are characterized by β-cell loss, and emerging evidence indicates that apoptosis is the mechanism by which β-cells are lost in both types. The ethanolic extracts of P. indica leaves were observed to decrease blood glucose levels in vivo (Nopparat et al., 2019). The leaf of P. indica has been observed to mitigate the severity of DM phenotypes induced by STZ. The untreated mice administered STZ exhibited elevated levels of hepatic malondialdehyde and reduced levels of superoxide dismutase and catalase. The administration of P. indica leaf extract at a dosage of 100 mg for 8 weeks resulted in the dissolution of the aforementioned symptoms (Nopparat et al., 2020). According to a search, P. indica tea can potentially mitigate hyperglycemia and dyslipidemia in individuals with pre-DM without causing any adverse effects on the kidneys, liver, or blood. The leaf of this plant can be utilized to produce a tea or herbal medication that may aid in preventing hyperglycemia and dyslipidemia (Sirichaiwetchakoon et al., 2021).
Another plant with ethnopharmacological relevance is Artemisia princeps. It is traditionally used to treat inflammatory-related diseases and has had its properties scientifically proven in various in vitro and in vivo models (Min et al., 2009). Jaceosidin is extracted from this plant, which has also been identified as the main constituent of another species, Artemisia argyi, with ethnomedicinal use as a healing agent (Li et al., 2018). It can inhibit the production of pro-inflammatory mediators such as TNF-α, IL-1β, and PGE2 (Min et al., 2009). In vitro, this flavonoid induces the proliferation, migration, and differentiation of human umbilical vascular endothelial cells. It also stimulates the formation of microvessels in rat aortic tissue, and this effect has been associated with the activation of VEGFR2/FAK/PI3K/AKT/NF-κB signaling pathways (Ali et al., 2017). Overall, all these studies suggest that jaceosidin is an interesting pro-angiogenic compound. Isosecotanapartholide, isolated from A. princeps, also has in vitro cell proliferating properties (Ali et al., 2017).
Artemisia pichinchensis is an ethnopharmacologically important plant in Mexico. Its pharmacological activities have been confirmed in murine models and clinical trials for diseases such as onychomycosis (Romero-Cerecero et al., 2009) and interdigital tinea pedis (Romero-Cerecero et al., 2012b, 2017). The first investigation demonstrated that A. pichinchensis aerial extract cures lesions in rats without causing skin irritation (Romero-Cerecero et al., 2011). Bio-guided purification showed that 7-O-(-D-glucopyranosyl)-galactin is the main component of A. pichinchensis that aids cell proliferation (Romero-Cerecero et al., 2013). Later, two extracts (aqueous and hexane) with standardized 7-O-(-D-glucopyranosyl)-galactin concentrations promoted skin lesion healing in diabetic rats (Romero-Cerecero et al., 2014). Human clinical investigations evaluated this plant’s therapeutic capabilities to treat chronic venous leg ulcers (Romero-Cerecero et al., 2012a). In another trial, diabetic patients with foot ulcers used a lotion containing A. pichinchensis extract that decreased healing time and lesion size, although no significant improvements were identified. The scientists attributed this to sample size, concluding that a larger clinical trial could prove A. pichinchensis’s effect on wound healing (Romero-Cerecero et al., 2015).
Achyrocline plants are crucial in Latin American traditional medicine (Bolson et al., 2015). In Rio Grande do Sul, Brazil, A. satureioides is utilized for healing. Extracts from the plant’s aerial portions trigger HaCaT keratinocyte growth (Alerico et al., 2015; Machado et al., 2020). Wistar rats showed efficient healing from treatment with A. satureioides essential oil applied to hydroxyethyl cellulose films (Yamane et al., 2016). A. alata and A. satureioides flower extracts were used to treat skin lesions in mice. Both extracts demonstrated positive benefits, but A. alata extract accelerated wound closure, resulting in faster healing (Balestrin et al., 2021, 2022; Salazar-Gómez and Alonso-Castro, 2022). Higher phenolic chemicals in A. alata caused this effect. Animals treated with A. alata extract had fewer mast cells at the site of inflammation, greater re-epithelization and granulation, and reduced initial inflammation (Pereira et al., 2017).
A. oleracea is a Brazilian plant used to heal skin and gastrointestinal ailments (da Rocha et al., 2018). A. oleracea’s rhamnogalacturonan inhibits ethanol-induced stomach ulcers in rats (Nascimento et al., 2013). Later studies showed that this chemical protects against acute (intraperitoneal) and chronic (oral) ethanol-induced damage. Rhamnogalacturonan improved gastric cell proliferation, mucus content, inflammation, and oxidative stress (Maria-Ferreira et al., 2014). Another study developed hydroxyethyl cellulose (HEC) films with A. oleracea ethanolic extract and A. satureioides essential oil. The HEC films containing these two plant components promoted wound healing in Wistar rats by increasing collagen deposition. α-Humulene and spilanthol were found to be responsible for the wound healing properties of these plants (Yamane et al., 2016).
Artemisia’s pharmacological potential has also been investigated in healing models. A. asiatica extract proved effective against ethanol-induced stomach injuries (Park et al., 2008). A. argyi repaired oral rat ulcers (Yin et al., 2017). Another study found that Artemisia montana (Nakai) Pamp essential oil promotes keratinocyte proliferation and collagen production. In vivo, tests demonstrated that A. montana essential oil enhances wound healing in rats (Yoon et al., 2014). A. campestris aqueous extract decreased inflammatory cell populations in the wound and promoted wound healing (Ghlissi et al., 2016).
5 Molecular antidiabetic phyto-compound database: DiaNat-DB
DiaNat-DB is an original and one-of-a-kind database (first version) focusing on anti-diabetic natural products. The most recent version (the first one) includes 336 different compounds. An examination of the database’s features and structures revealed that the molecules contained within DiaNat-DB have, in general, characteristics like those of medicines. In addition, scaffolds are highlighted in the DiaNat-DB database because we could determine which structural scaffolds are present in antihyperglycemic and hypoglycemic actions by correlating the most common chemotypes with biological activity. This gave us insight into which compounds had these activities and were approachable for future drug development. In biochemical tests, the significant structural variety and complexity of the compounds contained in DiaNat-DB hint at the possibility (Figure 6) that the database could produce molecules with a high level of target selectivity. Finally, the hit expansion analysis enabled it to extend from 336 compounds to more than eight thousand analogs. These analogs constitute the starting points of lead optimization programs that are inspired by natural products. The general public can access DiaNat-DB which provides a significant direction in the integration of chemical and biological information for the discovery of natural anti-diabetic drugs. Compound databases are an essential component of chemo informatics as well as several other informatics-related disciplines that have significantly impacted the fields of chemistry, biology, and biomedical sciences (López-López et al., 2021; Madariaga-Mazón et al., 2021).
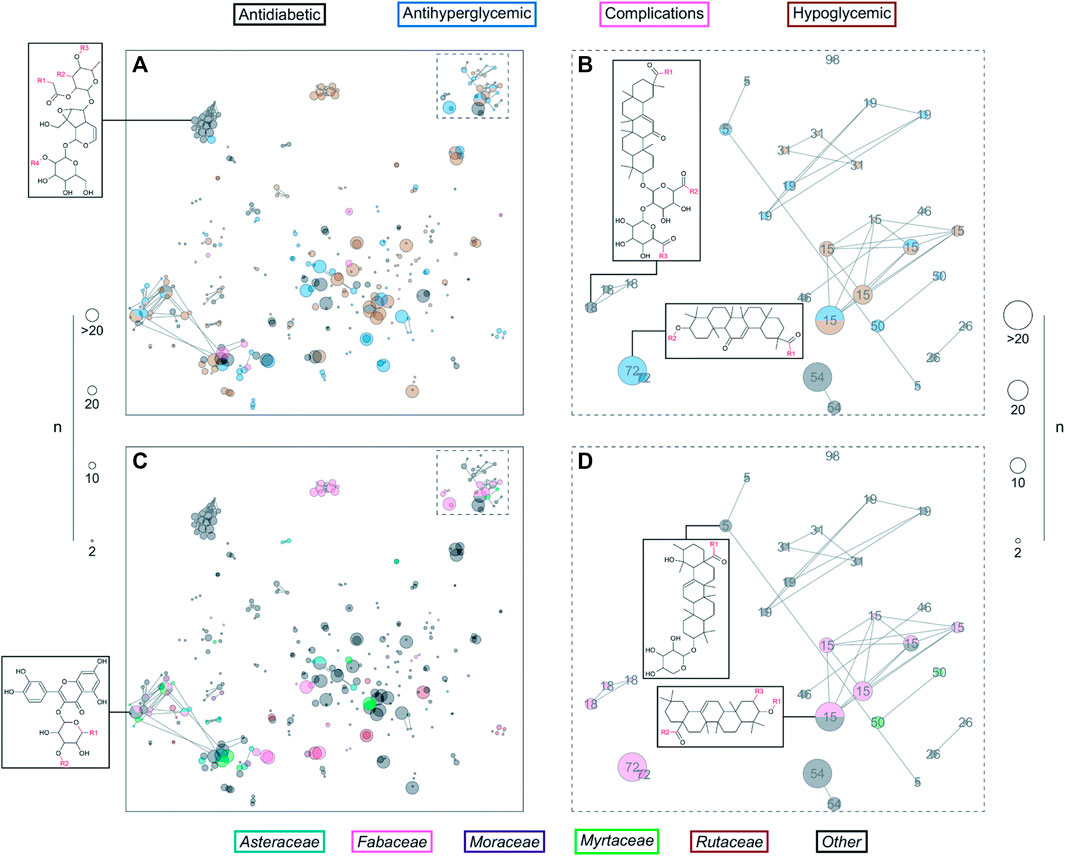
FIGURE 6. DiaNat-DB and ZINC15 analogs in a constellation diagram. The size of the dot shows the number of molecules mapped to the center. Colored dots represent DiaNat-DB compounds (A, B) based on the type of activity they are known to have, and (C, D) based on the plant family from which they were derived (Madariaga-Mazón et al., 2021). (CC-BY common attribute license).
6 Role of asteraceae as dietary supplements/nutraceuticals to control DM
Since the link between nutrition, health, and happiness has been discovered, food is now seen as more than just a way to fill the stomach and ward against deficiencies; it is also considered a significant means by which people travel toward their health and happiness goals. With rising global prosperity, improved access to information on how to eat safely and healthily has increased overall life expectancy. In 2016, the WHO found that a high percentage of adults were overweight or obese. This frequency has increased among children and adolescents (WHO, 2018). Considering the current pandemic context, in which many people with chronic non-communicable diseases are at risk (Stefan et al., 2020), the dissemination and contribution to increasing the knowledge of important foods that improve health and satiety, which are easily accessible by the larger population who are living with low income, is crucial (Hobbs, 2020). Non-starchy tubers have been mostly overlooked for a long time because of low utilization and a lack of information about their cultivation worldwide, in contrast to the perennially popular starchy roots, tubers, and potatoes. Although this was the case previously, things have changed thanks to several studies and reports emphasizing the significance of prebiotic fibers in food, and tubers (Kocsis et al., 2007) and chicory (Pilon et al., 2006) have gained some commercial value.
In recent decades, there has been a rise in the use of nutraceuticals, defined as “dietary supplements or foods containing a broad kind of plant extracts, pure chemicals, and, more generally, functional compounds” (Colitti and Grasso, 2014). Nutraceuticals can address the immune system, obesity, aging, oxidative stress, etc. Non-starchy Asteraceae tubers contain prebiotic oligofructose and inulin (Flamm et al., 2001), while sunflower, safflower, chamomile, lettuce, and mug wort have broad commercial uses in the food and medicinal industries. All these can be utilized as dietary supplements and nutraceuticals for health benefits and the overall wellbeing of diabetic patients.
7 Recent advancements and the prospects of flavonoids in the management of DM
Flavonoids have been widely investigated as antidiabetic agents in cells, animals, human studies, and clinical trials. Two recent reviews published by Ansari and co-workers (Ansari et al., 2022a; 2022c) elaborately discussed flavonoids from products of natural origin as antidiabetic agents and the role of quercetin in the treatment and management of DM2. The authors examined how flavonoids exert their antidiabetic activity via different mechanistic pathways [see Figure 7] (Ansari et al., 2022a). As per the role of Asteraceae in DM control, what kind of flavonoids and their mechanistic pathway are debatable? Literature research revealed that dietary polyphenols prevent several diabetic-related complications, such as vascular dysfunction, nephropathy, retinopathy, neuropathy, cardiomyopathy, coronary diseases, and renal failure. There are reports available regarding polyphenols preventing and managing DM2 via insulin-dependent approaches.
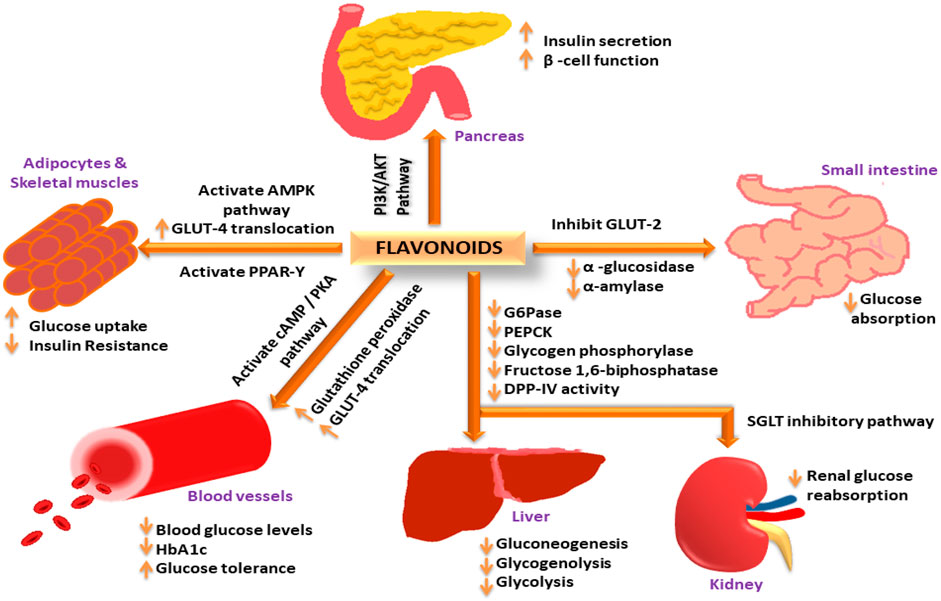
FIGURE 7. “Flavonoids exerting antidiabetic activity via different mechanistic pathways: Flavonoids increase insulin secretion and improve β-cell function via the PI3K/AKT signalling pathway; increase GLUT-4 translocation through AMPK activation to increase glucose uptake in adipose tissues and skeletal muscles; activate PPAR-γ expression to decrease insulin resistance; activate cAMP/PKA pathway to reduce blood glucose levels and improve glucose tolerance; increase glutathione peroxidase activity to reduce HbA1c levels; decrease glucose 6-phosphate, PEPCK, glycogen phosphorylase, fructose 1,6-biphosphatase and DPP-IV activity in the liver to decrease gluconeogenesis, glycogenolysis, and glycolysis; inhibit SGLT pathway in the kidney to decrease renal glucose reabsorption; inhibit GLUT-2, α-amylase, and α-glucosidase activity to reduce glucose absorption in the small intestine (Ansari et al., 2022a) (CC-BY common attribute license).
As discussed previously, it is important to search for unique dietary polyphenols from the Asteraceae family to develop nutraceuticals that can be used to control DM. The common flavonoids most frequently isolated from the plants of this family are isoflavones, hydroxycinnamic acids, and caffeoylquinic acids.
A significant hypoglycemic effect was observed from dietary flavonoids via the inhibition of digestive enzymes (Xiao et al., 2013b; 2013a), regulation of intestinal microbiota (Gowd et al., 2019), and prevention of advanced glycation end-product formation (Xie and Chen, 2013), among other mechanisms. By controlling glucose metabolism, hepatic enzyme activities, and lipid profiles, flavonoids mitigate the development of DM and its complications (Al-Ishaq et al., 2019; Zhao et al., 2019). Flavonoids’ hydroxyl groups on rings B and C, as well as their unsaturated 2, 3-bond in conjugation with a 4-carbonyl group, help prevent the development of digestive enzymes and AGEs, but their methyl and glycosidic moieties are counterproductive (Xiao et al., 2013b, 2013a; Xie and Chen, 2013). However, it remains unclear how the structure of flavonoids in the diet contributes to their hypoglycemic effects in animals. Rarely has the antidiabetic action of flavonoid C-glycosides been reported in human or clinical studies.
Epidemiological studies have found strong evidence linking isoflavone consumption to a reduced risk of developing DM (Konishi et al., 2019). Isoflavones inhibit carbohydrate digestion and glucose uptake in the small intestine, leading to hypoglycemic effects in Goto-Kakizaki diabetic rats (Jin et al., 2018) and slowing the progression of renal interstitial fibrosis in diabetic nephropathic rats (Liu et al., 2018). By lowering blood sugar levels, protecting pancreatic cells from damage, reducing inflammation and oxidative stress, and blocking the Maillard process and arabinogalactan protein (AGPs) formation, isoflavones proved to be the most effective hypoglycemic agents (Chen et al., 2018a; 2018b). In addition, DM complications such as cardiovascular disease, nephropathy, retinopathy, neuropathy, and so on are all improved by puerarin. DM2 patients can benefit from taking genistein because it significantly reduces hyperglycemia (Fu et al., 2012; Rockwood et al., 2019), increases β-cell proliferation and decreases apoptosis (Gilbert and Liu, 2013), reduces cardiac inflammation and oxidative stress (Gupta et al., 2015), and strengthens bones (Odle et al., 2017; Wang et al., 2019).
In vivo, studies using STZ, alloxan, and high-fructose and high-fat diets to cause DM2 have shown that several major hydroxycinnamic acids, like ferulic acid, caffeic acid, and chlorogenic acid, have significant effects on blood sugar levels. The primary phenolic component, chlorogenic acid, is known to lessen the likelihood of developing DM2. Changes in the heart, liver, and metabolism were slowed by a diet high in chlorogenic acid (Bhandarkar et al., 2019). CGA was reported to improve both sensorineural auditory function and DM consequences in animal models (Hong et al., 2017; Mei et al., 2018). CGA can reduce fasting plasma glucose and hemoglobin A1c levels in mice with advanced DM (Jin et al., 2015). Still, little data suggests that chlorogenic acid-rich decaffeinated coffee can regulate animal blood sugar levels (Faraji, 2018). To a lesser extent, chlorogenic acid inhibited carbohydrate-digesting enzymes in mice (Yambe-Silavwe and Williamson, 2018) and had a modest effect on both fasting blood glucose and blood glucose levels in oral glucose tolerance tests (Igarashi and Takahashi, 2017). Diet-induced obese mice do not benefit from chlorogenic acid protection against metabolic syndrome characteristics when fed a high-fat diet (Mubarak et al., 2013).
The 5-caffeoylquinic acids have been shown to block both human salivary and pancreatic α-amylase, which suggests that they may help decrease glucose absorption in the intestines following a meal in overweight or diabetic individuals (Narita and Inouye, 2009; Nurul Islam et al., 2013). Although pharmacokinetic studies have shown that caffeoylquinic acids are poorly absorbed from the gastrointestinal system (Lafay et al., 2006), various investigations have been conducted to determine if caffeoylquinic acids can affect the metabolism of peripheral tissues. In vitro studies have shown that caffeoylquinic acids competitively block liver glucose-6-phosphatase, the enzyme responsible for the final reaction in gluconeogenesis. It was hypothesized (Arion et al., 1997; Hemmerle et al., 1997; Henry-Vitrac et al., 2010) that caffeoylquinic acids could exert hypoglycemic activity by suppressing hepatic glucose synthesis. One possible explanation for this finding is that the amount of caffeoylquinic acid in liver cells was too low to inhibit Glucose-6-phosphatase (Bassoli et al., 2015) effectively. Recent research has shown that chlorogenic acid, along with several caffeoylquinic acid derivatives, acts as a noncompetitive inhibitor of protein tyrosine phosphatase 1B, and of all the caffeoylquinic acids, it is the most powerful inhibitor, with a Ki value of around 15 micromolar (Chen et al., 2014; Zhang et al., 2018). These findings indicate that caffeoylquinic acids may help increase insulin sensitivity in people who are overweight or have DM2. Treatment with CGA increases glucose uptake in insulin-sensitive and insulin-resistant adipocytes, supporting the former theory (Alonso-Castro et al., 2008).
Long-term use of caffeoylquinic acids decreases blood sugar levels, boosts insulin responsiveness (Reis et al., 2018), lessens hepatic insulin resistance (Lecoultre et al., 2014), lowers serum lipids, and promotes weight loss (Martínez-López et al., 2019). Extracts high in caffeoylquinic acids are suggested by traditional medicine for the treatment of DM and obesity, and these findings corroborate the idea that caffeoylquinic acids profoundly affect human energy metabolism (Spínola and Castilho, 2017; Xie et al., 2019).
8 Dietary polyphenols and the gut microbiome for DM
Gut microbiota makeup is linked to chronic illnesses (e.g., obesity, DM2, CVD, and nonalcoholic steatohepatitis) and is a new factor in DM management. Larsen et al. (2010) compared the intestinal microbiota of DM2 and nondiabetic patients. Firmicutes and Clostridia were dramatically reduced, while β-proteobacteria, positively linked with plasma glucose, were highly concentrated in DM2 patients’ feces compared to those of non-diabetic people. Food affects gut microbial diversity. According to animal studies and clinical trials, polyphenols and polyphenol-rich diets lower DM2 and/or its consequences (Nie et al., 2019). Polyphenols and their microbial metabolites can improve glucose metabolism by modulating the gut microbiota (Cardona et al., 2013). Few studies have investigated how polyphenols in the diet might affect the microbiome of people with DM. It is important to remember that studies of gut microorganisms have been identified as a novel field of research for generating new techniques to combat DM2.
Recent proposals for Akkermansia as a new biomarker of intestinal health suggest that its regulation may have favorable impacts on DM2 (Akkermansia has been documented to have effects on obesity and gut inflammation) (Delzenne et al., 2015; Blandino et al., 2016). Polyphenols were also discovered to influence Akkermansia positively (Everard & Cani, 2013). One study found that polyphenols stimulate the development of Bifidobacteria (Bialonska et al., 2010). Another study found that samples from diabetic patients had fewer Faecalibacterium prausnitzii and butyrate-producing bacteria than those from healthy individuals (Qin et al., 2012). Bacteroidetes-to-Firmicutes ratios are positively associated with decreased glucose tolerance (Larsen et al., 2010). Flavonoid metabolites 3,4-dihydroxyphenylacetic acid and 3-hydroxyphenylpropionic acid may promote pancreatic β-cell survival and function (Fernández-Millán et al., 2014). Polyphenol microbial metabolites may influence bile acid synthesis, affecting host metabolism.
In a nutshell, research has demonstrated a powerful interaction between polyphenols and the gut microbiota, both of which can protect against DM. Overall, the dietary polyphenols act on the gut microbiota and lower the ill-effects of DM in the following ways: (1) Polyphenols alter the gut microbiota by encouraging the expansion of good bacteria, including Akkermansia, Bifidobacteria, and Faecalibacterium prausnitzii; (2) bioactivity against DM is increased not only by polyphenols but also by their resulting microbial metabolites; and (3) more research is needed to determine how polyphenols and the microbes that metabolize them affect the microbiota in the digestive tract. Polyphenols reduce DM2 and diabetic consequences in animals (see Figure 8). It is unclear how DM2 affects polyphenol bioavailability and bioactivity, and understanding this mechanism will enhance clinical outcomes. The challenge is to produce enough excellent products to improve with the health of millions of people.
9 Challenges and opportunities of asteraceae medicinal plants in the management of DM
A wide variety of plants belonging to different families are available worldwide that are useful for treating many lifestyle-related disorders; this includes plants from the Asteraceae family too. There is no denying that plants from the Asteraceae family have demonstrated extensive use in traditional and modern medicinal systems due to their unique phytochemicals and bioactive compounds. This family has yielded many beneficial drugs (FDA approved) that have been and continue to be utilized for cancer, parasitic infections, and inflammation, among other diseases. However, despite their age-old use as traditional medicine, several compounds in this family lack scientific validation and, hence, have not been established or approved for treatment to date. The lack of sufficient documentation makes these plants unreliable for use. Also, the phytochemistry and essential oil content of many members of the Asteraceae are not well defined, and some plants have not even been studied yet.
9.1 Loss of traditional knowledge
The disappearance of traditional knowledge in developing countries is alarming. There should be preservation and awareness regarding the importance of indigenous knowledge and medicinal plants; a lot of valuable plants are becoming victims of deforestation and urbanization. As such, ethno-medicine and ethno-pharmacology should be introduced early in the curriculum of schools and colleges to make young people aware of ethnic practices. Government and non-government organizations should collaborate for the plantation and preservation of valuable medicinal plants, especially in developing countries. Since Asteraceae is a large family and many of the plants used by the indigenous peoples are not well explored, more work is needed for documentation to yield potent, commercially available botanically sourced antidiabetic drugs.
9.2 Safety
Safety concerns are another major issue with herbal drugs. The effects can be severe and detrimental if the appropriate dosage is not determined, as active compounds are toxic at high doses. The administration, distribution, metabolism, and excretion (ADME) of a compound that may act as a drug needs to be studied as it determines the bioavailability or fate of the compound; this stands true for all compounds (synthetic and herbal) that can be developed as drugs in the future. The FDA, United States, has designed a set of guidelines for all pharmaceutical companies and drug manufacturers to strictly adhere to for proper manufacturing, safety, and toxicity labeling. This ensures that all information is available to consumers to make an informed choice or purchase. However, several countries do not have appropriate regulations for quality control and risk assessment of bioactive compounds from natural sources. Coupled with the lack of consistency, misinformation between different countries across the globe can lead to disastrous effects and prevent the establishment of plant-based compounds as therapeutic products and nutraceuticals.
9.3 Bioassay guided purification
Extraction and characterization of bioactive compounds is a major challenge to drug discovery and development, even though high-end techniques, combinatorial chemistry, and high-throughput screening are well-developed. Most importantly, a minuscule number of “pure” compounds are obtained after lengthy, exhaustive procedures, which is insufficient for bioactivity screening. Though organic chemistry and synthesis can be applied to overcome this limitation, this process is not economically feasible. Also, there is a vast disparity in the activity of the natural compounds in vivo and in vitro; this can be attributed to the complex metabolic and synergistic pathways within the living systems that bring about their promising results in vivo but are extremely difficult and nearly impossible to replicate in vitro (Chakrabartty, 2022; Chakrabartty et al., 2022). As a result, drug discovery and the development of some potential and promising lead compounds take a backseat. Few studies are followed up by bioassay guided purification, so more research is necessary for scientific validation.
9.4 Lack of R & D on natural products in the pharmaceutical industries
Like many other families of medicinal plants, Asteraceae also show promising results for treating lifestyle-related disorders like DM and hypertension. However, the preliminary results do not highlight the actual underlying mechanisms of action of the different extracts and compounds. Hence, focused research is the need of the hour; extensive research is required on mechanisms, toxicity, risk assessment, safety, ADME, drug dosage, and formulation development. Such studies may attract the attention of big pharmaceutical companies to fund the subsequent research and commercialization of the potential drug leads from Asteraceae and all other medicinally important plants.
10 Conclusion and prospects
Lifestyle-related disorders have become commonplace in today’s world owing to the hectic and sedentary lifestyle, stress, and lack of awareness or consciousness of one’s health. Asteraceae is traditionally used worldwide to treat various diseases, including infections, wound healing, liver dysfunction, and other disorders. Complex secondary metabolites play a major role in bringing out these activities. Despite the excessive scientific studies in the search for new antidiabetic drugs, more work is needed to yield potent, commercially available drugs based on medicinal plants. Indeed, the scientific community and pharmaceutical companies have failed to provide the required attention to this plant family to explore therapeutic products, although a Nobel Prize has already been awarded. Although several plants from this family were extensively studied, some of the species important in traditional medicine have still hardly been studied for their bioactivity. Therefore, the present review aims to encourage in-depth exploration of different members of the Asteraceae family for the treatment of DM, guided by folk and traditional knowledge. The daisy family exerts antioxidant, hepatoprotective, vasodilation, antidiabetic, and wound healing effects, which further prevent major diseases like CVDs, liver cirrhosis, and DM. Most of these studies are still in the preliminary stages of in vitro and in vivo experiments, while clinical trials are lacking. No doubt, a few studies related to flavonoids and their mode of action in the treatment and management of DM are available, but pharmacokinetics/pharmacodynamics in laboratory animals and clinical trials are warranted to investigate their effects, including the mechanisms of action.
The information documented in this review article can serve as a pioneer for developing research initiatives directed at the exploration of the Asteraceae family at the forefront of developing a botanical drug to be used as a treatment for DM. With a shift towards plant-based and herbal products for nutrition and wellbeing, there is a high chance that consumers may easily opt for natural products for food and medicine in the long run.
Author contributions
YM, AM, and AN: conceptualized the manuscript, Write and edit the original draft; SM: prepared the images, BS and JP: data collection, Write and edit part of the manuscript, IC and SP: Write and edit the draft. All authors contributed to the article and approved the submitted version.
Funding
YM and JP is indebted their sincere thanks to the SERB-DST, Govt. of India for supporting the Nano-biotechnology and Translational Knowledge Laboratory through research grant no. SRG/2022/000641 at University of Science and Technology Meghalaya, India.
Acknowledgments
Authors are thankful to their respective institutions for providing research facility during developing this scientific manuscript. SP is thankful to the RUSA 2.0 for supporting ECCPH, Utkal University.
Conflict of interest
IC was employed by the Learning and Development Solutions, Indegene Pvt. Ltd., Manyata Tech Park.
The remaining authors declare that the research was conducted in the absence of any commercial or financial relationships that could be construed as a potential conflict of interest.
Publisher’s note
All claims expressed in this article are solely those of the authors and do not necessarily represent those of their affiliated organizations, or those of the publisher, the editors and the reviewers. Any product that may be evaluated in this article, or claim that may be made by its manufacturer, is not guaranteed or endorsed by the publisher.
References
Ab Rahman, M. R., Abdul Razak, F., and Mohd Bakri, M. (2014). Evaluation of wound closure activity of Nigella sativa, Melastoma malabathricum, Pluchea indica, and Piper sarmentosum extracts on scratched monolayer of human gingival fibroblasts. Evid. Based. Complement. Altern. Med. 2014, 190342. doi:10.1155/2014/190342
Abeysiri, G. R. P. I., Dharmadasa, R. M., Abeysinghe, D. C., and Samarasinghe, K. (2013). Screening of phytochemical, physico-chemical and bioactivity of different parts of Acmella oleraceae Murr. (Asteraceae), a natural remedy for toothache. Ind. Crops Prod. 50, 852–856. doi:10.1016/j.indcrop.2013.08.043
Aboul Ela, M., El-Lakany, A., and Ali Hijazi, M. (2012). “General introduction on family Asteracea,” in Phytochemicals - a global perspective of their role in nutrition and health (London: InTechOpen), 375–400. doi:10.5772/28956
Adailton da Silva, J., Fagundes de Souza, E. C., Echazú Böschemeier, A. G., Maia da Costa, C. C., Souza Bezerra, H., and Lopes Cavalcante Feitosa, E. E. (2018). Diagnosis of diabetes mellitus and living with a chronic condition: Participatory study. BMC Public Health 18, 699. doi:10.1186/s12889-018-5637-9
Adebayo, A. H., Tan, N. H., Akindahunsi, A. A., Zeng, G. Z., and Zhang, Y. M. (2010). Anticancer and antiradical scavenging activity of Ageratum conyzoides L. (Asteraceae). Pharmacogn. Mag. 6, 62–66. doi:10.4103/0973-1296.59968
Adhikari, M., Thapa, R., Kunwar, R. M., Devkota, H. P., and Poudel, P. (2019). Ethnomedicinal uses of plant resources in the Machhapuchchhre rural municipality of Kaski district, Nepal. Med. 6, 69. doi:10.3390/medicines6020069
Afolayan, J. A., and Sunmonu, T. (2010). In vivo studies on antidiabetic plants used in South African herbal medicine. J. Clin. Biochem. Nutr. 47, 98–106. doi:10.3164/jcbn.09-126R
Agbafor, K., Onuoha, S., Ominyi, M., Orinya, O., and Ezeani, N. (2015). Antidiabetic, hypolipidemic and antiathrogenic properties of leaf extracts of Ageratum conyzoides in streptozotocin-induced diabetic rats. Int. J. Curr. Microbiol. App. Sci. 4, 816–824.
Ahmad, H., Khan, I., and Wahid, A. (2012). Antiglycation and antioxidation properties of Juglans regia and Calendula officinalis: Possible role in reducing diabetic complications and slowing down ageing. J. Tradit. Chin. Med. 32, 411–414. doi:10.1016/s0254-6272(13)60047-3
Akashi, T., Furuno, T., Takahashi, T., and Ayabe, S.-I. (1994). Biosynthesis of triterpenoids in cultured cells, and regenerated and wild plant organs of Taraxacum officinale. Phytochemistry 36, 303–308. doi:10.1016/S0031-9422(00)97065-1
Al-Ishaq, R. K., Abotaleb, M., Kubatka, P., Kajo, K., and Büsselberg, D. (2019). Flavonoids and their anti-diabetic effects: Cellular mechanisms and effects to improve blood sugar levels. Biomolecules 9, 430. doi:10.3390/biom9090430
Alam, N., and Sharma, K. (2020). Estimation of phenolic content, flavonoid content, antioxidant, and α-amylase inhibitory activity of some selected plants from Siraha district Nepal. Asian J. Pharm. Clin. Res. 13, 18–23. doi:10.22159/ajpcr.2020.v13i4.36734
Alara, O. R., Abdurahman, N. H., Ukaegbu, C. I., and Kabbashi, N. A. (2019). Extraction and characterization of bioactive compounds in Vernonia amygdalina leaf ethanolic extract comparing Soxhlet and microwave-assisted extraction techniques. J. Taibah Univ. Sci. 13, 414–422. doi:10.1080/16583655.2019.1582460
Alerico, G. C., Beckenkamp, A., Vignoli-Silva, M., Buffon, A., and von Poser, G. L. (2015). Proliferative effect of plants used for wound healing in Rio Grande do Sul state, Brazil. J. Ethnopharmacol. 176, 305–310. doi:10.1016/j.jep.2015.11.001
Ali, S. I., Gopalakrishnan, B., and Venkatesalu, V. (2017). Pharmacognosy, phytochemistry and pharmacological properties of Achillea millefolium L.: A review. Phytother. Res. 31, 1140–1161. doi:10.1002/ptr.5840
Alkhatib, A., Tsang, C., Tiss, A., Bahorun, T., Arefanian, H., Barake, R., et al. (2017). Functional foods and lifestyle approaches for diabetes prevention and management. Nutrients 9, 1310–1318. doi:10.3390/nu9121310
Alonso-Castro, A. J., Miranda-Torres, A. C., González-Chávez, M. M., and Salazar-Olivo, L. A. (2008). Cecropia obtusifolia Bertol and its active compound, chlorogenic acid, stimulate 2-NBDglucose uptake in both insulin-sensitive and insulin-resistant 3T3 adipocytes. J. Ethnopharmacol. 120, 458–464. doi:10.1016/j.jep.2008.09.019
Ananthi, J., Prakasam, A., and Pugalendi, K. V. (2003). Antihyperglycemic activity of Eclipta alba leaf on alloxan-induced diabetic rats. Yale J. Biol. Med. 76, 97–102.
Anderson, D., Yu, T. W., Phillips, B. J., and Schmezer, P. (1994). The effect of various antioxidants and other modifying agents on oxygen-radical-generated DNA damage in human lymphocytes in the COMET assay. Mutat. Res. 307, 261–271. doi:10.1016/0027-5107(94)90300-x
Ansari, P., Akther, S., Hannan, J. M. A., Seidel, V., Nujat, N. J., and Abdel-Wahab, Y. H. A. (2022a). Pharmacologically active phytomolecules isolated from traditional antidiabetic plants and their therapeutic role for the management of diabetes mellitus. Molecules 27, 4278. doi:10.3390/molecules27134278
Ansari, P., Azam, S., Hannan, J. M. A., Flatt, P. R., and Abdel Wahab, Y. H. A. (2020). Anti-hyperglycaemic activity of Hibiscus rosasinensis leaves is partly mediated by inhibition of carbohydrate digestion and absorption, and enhancement of insulin secretion. J. Ethnopharmacol. 253, 112647. doi:10.1016/j.jep.2020.112647
Ansari, P., Choudhury, S. T., and Abdel-Wahab, Y. H. A. (2022d). Insulin secretory actions of ethanol extract of Eucalyptus citriodora Leaf, including plasma DPP-IV and GLP-1 levels in high-fat-fed rats, as well as characterization of biologically effective phytoconstituents. Metabolites 12, 757. doi:10.3390/metabo12080757
Ansari, P., Choudhury, S. T., Seidel, V., Rahman, A. B., Aziz, M. A., Richi, A. E., et al. (2022c). Therapeutic potential of quercetin in the management of type-2 diabetes mellitus. Life 12, 1146. doi:10.3390/life12081146
Ansari, P., Flatt, P. R., Harriott, P., and Abdel-Wahab, Y. H. A. (2021a). Anti-hyperglycaemic and insulin-releasing effects of Camellia sinensis leaves and isolation and characterisation of active compounds. Br. J. Nutr. 126, 1149–1163. doi:10.1017/S0007114520005085
Ansari, P., Flatt, P. R., Harriott, P., and Abdel-Wahab, Y. H. A. (2022b). Insulin secretory and antidiabetic actions of Heritiera fomes bark together with isolation of active phytomolecules. PLoS One 17, e0264632. doi:10.1371/journal.pone.0264632
Ansari, P., Flatt, P. R., Harriott, P., and Abdel-Wahab, Y. H. A. (2021b). Insulinotropic and antidiabetic properties of Eucalyptus citriodora leaves and isolation of bioactive phytomolecules. J. Pharm. Pharmacol. 73, 1049–1061. doi:10.1093/jpp/rgab030
Ansari, P., Flatt, P. R., Harriott, P., Hannan, J. M. A., and Abdel-Wahab, Y. H. A. (2021c). Identification of multiple pancreatic and extra-pancreatic pathways underlying the glucose-lowering actions of Acacia arabica Bark in type-2 diabetes and isolation of active phytoconstituents. Plants 10, 1190. doi:10.3390/plants10061190
Ansari, P., Hannon-Fletcher, M. P., Flatt, P. R., and Abdel-Wahab, Y. H. A. (2021d). Effects of 22 traditional anti-diabetic medicinal plants on DPP-IV enzyme activity and glucose homeostasis in high-fat fed obese diabetic rats. Biosci. Rep. 41, BSR20203824. doi:10.1042/BSR20203824
Arion, W. J., Canfield, W. K., Ramos, F. C., Schindler, P. W., Burger, H. J., Hemmerle, H., et al. (1997). Chlorogenic acid and hydroxynitrobenzaldehyde: New inhibitors of hepatic glucose 6-phosphatase. Arch. Biochem. Biophys. 339, 315–322. doi:10.1006/abbi.1996.9874
Arpadjan, S., Çelik, G., Taşkesen, S., and Güçer, Ş. (2008). Arsenic, cadmium and lead in medicinal herbs and their fractionation. Food Chem. Toxicol. 46, 2871–2875. doi:10.1016/j.fct.2008.05.027
Asad, M. H., Hussain, M. S., and Murtaza, G. (2011). Enlisting the scientifically unnoticed medicinal plants of Pakistan as a source of novel therapeutic agents showing anti-venom activity. Afr. J. Pharm. Pharmacol. 5, 2292–2305.
Asante, D.-B., Effah-Yeboah, E., Barnes, P., Abban, H. A., Ameyaw, E. O., Boampong, J. N., et al. (2016). Antidiabetic effect of young and old ethanolic leaf extracts of Vernonia amygdalina: A comparative study. J. Diabetes Res. 2016, 8252741. doi:10.1155/2016/8252741
Atangwho, I., Ebong, P. E., Egbung, E., Eteng, M., and Eyong, E. (2008). Effect of Vernonia amygdalina Del. on liver function in alloxan-induced hyperglycaemic rats. J. Pharm. Bioresour. 4, 25–31. doi:10.4314/jpb.v4i1.32107
Atangwho, I. J., Egbung, G. E., Ahmad, M., Yam, M. F., and Asmawi, M. Z. (2013). Antioxidant versus anti-diabetic properties of leaves from Vernonia amygdalina Del. growing in Malaysia. Food Chem. 141, 3428–3434. doi:10.1016/j.foodchem.2013.06.047
Aziz, M. A., Adnan, M., Begum, S., Azizullah, A., Nazir, R., and Iram, S. (2016). A review on the elemental contents of Pakistani medicinal plants: Implications for folk medicines. J. Ethnopharmacol. 188, 177–192. doi:10.1016/j.jep.2016.05.011
Babotă, M., Mocan, A., Vlase, L., Crișan, O., Ielciu, I., Gheldiu, A.-M., et al. (2018). Phytochemical analysis, antioxidant and antimicrobial activities of Helichrysum arenarium (L) Moench. and Antennaria dioica (L) Gaertn. flowers. Molecules 23, 409. doi:10.3390/molecules23020409
Bakun, P., Czarczynska-Goslinska, B., Goslinski, T., and Lijewski, S. (2021). In vitro and in vivo biological activities of azulene derivatives with potential applications in medicine. Med. Chem. Res. 30, 834–846. doi:10.1007/s00044-021-02701-0
Balbi, M. E., Tonin, F. S., Mendes, A. M., Borba, H. H., Wiens, A., Fernandez-Llimos, F., et al. (2018). Antioxidant effects of vitamins in type 2 diabetes: A meta-analysis of randomized controlled trials. Diabetol. Metab. Syndr. 10, 18. doi:10.1186/s13098-018-0318-5
Balestrin, L. A., Back, P. I., Marques, M. da S., Araújo, G. de M. S., Carrasco, M. C. F., Batista, M. M., et al. (2022). Effect of hydrogel containing Achyrocline satureioides (Asteraceae) extract-loaded nanoemulsions on wound healing activity. Pharmaceutics 14, 2726. doi:10.3390/pharmaceutics14122726
Balestrin, L. A., Kreutz, T., Fachel, F. N. S., Bidone, J., Gelsleichter, N. E., Koester, L. S., et al. (2021). Achyrocline satureioides (lam) DC (asteraceae) extract-loaded nanoemulsions as a promising topical wound healing delivery system: In vitro assessments in human keratinocytes (HaCaT) and HET-CAM irritant potential. Pharmaceutics 13, 1241. doi:10.3390/pharmaceutics13081241
Balszuweit, F., John, H., Schmidt, A., Kehe, K., Thiermann, H., and Steinritz, D. (2013). Silibinin as a potential therapeutic for sulfur mustard injuries. Chem. Biol. Interact. 206, 496–504. doi:10.1016/j.cbi.2013.06.010
Bartolome, A. P., Villaseñor, I. M., and Yang, W.-C. (2013). Bidens pilosa L. (Asteraceae): Botanical properties, traditional uses, phytochemistry, and pharmacology. Evid. Based. Complement. Altern. Med. 2013, 340215. doi:10.1155/2013/340215
Baruah, N. C., Sharma, R. P., Madhusudanan, K. P., Thyagarajan, G., Herz, W., and Murari, R. (1979). Sesquiterpene lactones of Tithonia diversifolia. Stereochemistry of the tagitinins and related compounds. J. Org. Chem. 44, 1831–1835. doi:10.1021/jo01325a018
Ben Salem, M., Ben Abdallah Kolsi, R., Dhouibi, R., Ksouda, K., Charfi, S., Yaich, M., et al. (2017). Protective effects of Cynara scolymus leaves extract on metabolic disorders and oxidative stress in alloxan-diabetic rats. BMC Complement. Altern. Med. 17, 328. doi:10.1186/s12906-017-1835-8
Bessada, S. M. F., Barreira, J. C. M., and Oliveira, M. B. P. P. (2015). Asteraceae species with most prominent bioactivity and their potential applications: A review. Ind. Crops Prod. 76, 604–615. doi:10.1016/j.indcrop.2015.07.073
Bhandarkar, N. S., Brown, L., and Panchal, S. K. (2019). Chlorogenic acid attenuates high-carbohydrate, high-fat diet-induced cardiovascular, liver, and metabolic changes in rats. Nutr. Res. 62, 78–88. doi:10.1016/j.nutres.2018.11.002
Bialonska, D., Ramnani, P., Kasimsetty, S. G., Muntha, K. R., Gibson, G. R., and Ferreira, D. (2010). The influence of pomegranate by-product and punicalagins on selected groups of human intestinal microbiota. Int. J. Food Microbiol. 140, 175–182. doi:10.1016/j.ijfoodmicro.2010.03.038
Bindu, J., and Narendhirakannan, R. T. (2019). Role of medicinal plants in the management of diabetes mellitus: A review. 3 Biotech. 9, 4. doi:10.1007/s13205-018-1528-0
Bisht, D., Kumar, D., Kumar, D., Dua, K., and Chellappan, D. K. (2021). Phytochemistry and pharmacological activity of the genus Artemisia. Arch. Pharm. Res. 44, 439–474. doi:10.1007/s12272-021-01328-4
Blandino, G., Inturri, R., Lazzara, F., Di Rosa, M., and Malaguarnera, L. (2016). Impact of gut microbiota on diabetes mellitus. Diabetes Metab. 42, 303–315. doi:10.1016/j.diabet.2016.04.004
Boden, G., and Shulman, G. I. (2002). Free fatty acids in obesity and type 2 diabetes: Defining their role in the development of insulin resistance and beta-cell dysfunction. Eur. J. Clin. Invest. 32 (3), 14–23. doi:10.1046/j.1365-2362.32.s3.3.x
Bolson, M., Hefler, S. M., Dall’Oglio Chaves, E. I., Gasparotto Junior, A., and Cardozo Junior, E. L. (2015). Ethno-medicinal study of plants used for treatment of human ailments, with residents of the surrounding region of forest fragments of Paraná, Brazil. J. Ethnopharmacol. 161, 1–10. doi:10.1016/j.jep.2014.11.045
Buragohain, J. (2011). Ethnomedicinal plants used by the ethnic communities of tinsukia district of Assam, India. Recent Res. Sci. Technol. 3, 31–42.
Buzzi, M., de Freitas, F., and de Barros Winter, M. (2016). Therapeutic effectiveness of a Calendula officinalis extract in venous leg ulcer healing. J. Wound Care 25, 732–739. doi:10.12968/jowc.2016.25.12.732
Cardona, F., Andrés-Lacueva, C., Tulipani, S., Tinahones, F. J., and Queipo-Ortuño, M. I. (2013). Benefits of polyphenols on gut microbiota and implications in human health. J. Nutr. Biochem. 24, 1415–1422. doi:10.1016/j.jnutbio.2013.05.001
Carvalho, A. F. M. de, Feitosa, M. C. P., Coelho, N. P. M. de F., Rebêlo, V. C. N., Castro, J. G. de, Sousa, P. R. G. de, et al. (2016). Low-level laser therapy and Calendula officinalis in repairing diabetic foot ulcers. Rev. Esc. Enferm. Usp. 50, 628–634. doi:10.1590/S0080-623420160000500013
Carvalho, A. R., Diniz, R. M., Suarez, M. A. M., Figueiredo, C. S. S. S., Zagmignan, A., Grisotto, M. A. G., et al. (2018). Use of some Asteraceae plants for the treatment of wounds: From ethnopharmacological studies to scientific evidences. Front. Pharmacol. 9, 784. doi:10.3389/fphar.2018.00784
Ceballos-Picot, I., Witko-Sarsat, V., Merad-Boudia, M., Nguyen, A. T., Thévenin, M., Jaudon, M. C., et al. (1996). Glutathione antioxidant system as a marker of oxidative stress in chronic renal failure. Free Radic. Biol. Med. 21, 845–853. doi:10.1016/0891-5849(96)00233-x
Cetkovic, G. S., Djilas, S. M., Čanadanović-Brunet, J. M., and Tumbas, V. T. (2004). Antioxidant properties of marigold extracts. Food Res. Int. 37, 643–650. doi:10.1016/j.foodres.2004.01.010
Chadchan, K. S., Jargar, J. G., and Das, S. N. (2016). Anti-diabetic effects of aqueous prickly lettuce (Lactuca scariola Linn) leaves extract in alloxan-induced male diabetic rats treated with nickel (II). J. Basic Clin. Physiol. Pharmacol. 27, 49–56. doi:10.1515/jbcpp-2015-0038
Chakrabartty, I., Kumar, N., Boruah, P., Katiyar, V., Hakeem, K. R., and Rangan, L. (2020). Physico-rheological characterization of organically derived seed samples from Alpinia nigra (Gaertn) B. L. Burtt, an ethnic medicinal plant of Northeast India. Ind. Crop. Prod. 152, 112560. doi:10.1016/j.indcrop.2020.112560
Chakrabartty, I., Mohanta, Y. K., Nongbet, A., Mohanta, T. K., Mahanta, S., Das, N., et al. (2022). Exploration of Lamiaceae in cardio vascular diseases and functional foods: Medicine as food and food as medicine. Front. Pharmacol. 13, 894814. doi:10.3389/fphar.2022.894814
Chakrabartty, I. (2022). “Plant metabolic engineering for a futuristic economy,” in Metabolic engineering in plants (Springer Nature), 391–404. doi:10.1007/978-981-16-7262-0_18
Chakrabartty, I., Vijayasekhar, A., and Rangan, L. (2019). Therapeutic potential of labdane diterpene isolated from Alpinia nigra: Detailed hemato-compatibility and antimicrobial studies. Nat. Prod. Res. 35, 1000–1004. doi:10.1080/14786419.2019.1610756
Chandramohan, G., Al-Numair, K. S., Alsaif, M. A., and Veeramani, C. (2015). Antidiabetic effect of kaempferol a flavonoid compound, on streptozotocin-induced diabetic rats with special reference to glycoprotein components. Prog. Nutr. 17, 50–57.
Chang, C. L.-T., Liu, H.-Y., Kuo, T.-F., Hsu, Y.-J., Shen, M.-Y., Pan, C.-Y., et al. (2013). Antidiabetic effect and mode of action of cytopiloyne. Evid. Based. Complement. Altern. Med. 2013, 685642. doi:10.1155/2013/685642
Chen, B., Ma, L., Wang, X., Shen, Y., and Jia, X. (2013). Simultaneous determination of 5 phenolic acids in fried Fructus xanthii from different production sites and its dispensing granules by using ultra-pressure liquid chromatography. Pharmacogn. Mag. 9, 103–108. doi:10.4103/0973-1296.111245
Chen, J., Mangelinckx, S., Ma, L., Wang, Z., Li, W., and De Kimpe, N. (2014). Caffeoylquinic acid derivatives isolated from the aerial parts of Gynura divaricata and their yeast α-glucosidase and PTP1B inhibitory activity. Fitoterapia 99, 1–6. doi:10.1016/j.fitote.2014.08.015
Chen, X., Wang, L., Fan, S., Song, S., Min, H., Wu, Y., et al. (2018a). Puerarin acts on the skeletal muscle to improve insulin sensitivity in diabetic rats involving μ-opioid receptor. Eur. J. Pharmacol. 818, 115–123. doi:10.1016/j.ejphar.2017.10.033
Chen, X., Yu, J., and Shi, J. (2018b). Management of diabetes mellitus with Puerarin, a natural isoflavone from Pueraria lobata. Am. J. Chin. Med. 46, 1771–1789. doi:10.1142/S0192415X18500891
Chopra, R. N., Naya, S. L., and Chopra, I. C. (1986). Glossary of indian medicinal plants. New Delhi: Counc. Sci. Ind. Res., 259.
Cioinac, S. E. (2016). Use of calendula cream balm to medicate the feet of diabetic patients: Case series. Int. J. Nurs. Sci. 3, 102–112. doi:10.1016/j.ijnss.2015.12.001
Colitti, M., and Grasso, S. (2014). Nutraceuticals and regulation of adipocyte life: Premises or promises. Biofactors 40, 398–418. doi:10.1002/biof.1164
Çoruh, N., Sagˇdıçogˇlu Celep, A. G., Özgökçe, F., and İşcan, M. (2007). Antioxidant capacities of Gundelia tournefortii L. extracts and inhibition on glutathione-S-transferase activity. Food Chem. 100, 1249–1253. doi:10.1016/j.foodchem.2005.12.008
da Rocha, C. F., de Medeiros Souza Lima, Y., Carvalho, H. O., Pinto, R. C., Ferreira, I. M., Castro, A. N., et al. (2018). Action of the hydroethanolic extract of the flowers of Acmella oleracea (L) R.K. Jansen on the reproductive performance of wistar females rats: A popular female aphrodisiac from the amazon. J. Ethnopharmacol. 214, 301–308. doi:10.1016/j.jep.2017.12.024
Dabe, N. E., and Kefale, A. T. (2017). Antidiabetic effects of Artemisia species: A systematic review. Anc. Sci. Life 36, 175–181. doi:10.4103/asl.ASL_87_17
Dalal, S., Kataria, S., Sastry, K., and Rana, S. (2010). Phytochemical screening of methanolic extract and antibacterial activity of active principles of hepatoprotective herb, Eclipta alba. Ethnobot. Leafl. 2010, 3.
de Boer, H. J., and Cotingting, C. (2014). Medicinal plants for women’s healthcare in Southeast Asia: A meta-analysis of their traditional use, chemical constituents, and pharmacology. J. Ethnopharmacol. 151, 747–767. doi:10.1016/j.jep.2013.11.030
Delzenne, N. M., Cani, P. D., Everard, A., Neyrinck, A. M., and Bindels, L. B. (2015). Gut microorganisms as promising targets for the management of type 2 diabetes. Diabetologia 58, 2206–2217. doi:10.1007/s00125-015-3712-7
Després, J. P. (2012). Body fat distribution and risk of cardiovascular disease: An update. Circulation 126, 1301–1313. doi:10.1161/CIRCULATIONAHA.111.067264
Deutschländer, M., Lall, N., and de Venter, M. (2009). Plant species used in the treatment of diabetes by South African traditional healers: An inventory. Pharm. Biol. 47, 348–365. doi:10.1080/13880200902752959
Dinda, M., Dasgupta, U., Singh, N., Bhattacharyya, D., and Karmakar, P. (2015). PI3K-mediated proliferation of fibroblasts by Calendula officinalis tincture: Implication in wound healing. Phytother. Res. 29, 607–616. doi:10.1002/ptr.5293
Dinda, M., Mazumdar, S., Das, S., Ganguly, D., Dasgupta, U. B., Dutta, A., et al. (2016). The water fraction of Calendula officinalis hydroethanol extract stimulates in vitro and in vivo proliferation of dermal fibroblasts in wound healing. Phytother. Res. 30, 1696–1707. doi:10.1002/ptr.5678
Djellouli, M., Moussaoui, A., Benmehdi, H., Laid, Z., Abdelghani, B., Messaouda, B., et al. (2013). Ethnopharmacological study and phytochemical screening of three plants (Asteraceae family) from the region of South west Algeria. Asian J. Nat. Appl. Sci. 2, 59–65.
Dorjsembe, B., Lee, H. J., Kim, M., Dulamjav, B., Jigjid, T., and Nho, C. W. (2017). Achillea asiatica extract and its active compounds induce cutaneous wound healing. J. Ethnopharmacol. 206, 306–314. doi:10.1016/j.jep.2017.06.006
Dubey, S., Maity, S., Singh, M., Saraf, S. A., and Saha, S. (2013). Phytochemistry, pharmacology and toxicology of Spilanthes acmella: A review. Adv. Pharmacol. Sci. 2013, 423750. doi:10.1155/2013/423750
Ebong, P., Atangwho, I., Eyong, E., and Egbung, E. (2008). The antidiabetic efficacy of combined extracts from two continental plants: Azadirachta indica (A Juss) (neem) and Vernonia amygdalina (del) (african bitter leaf). Am. J. Biochem. Biotechnol. 4, 239–244. doi:10.3844/ajbbsp.2008.239.244
Ebrahimi-Mameghani, M., Asghari-Jafarabadi, M., and Rezazadeh, K. (2018). TCF7L2-rs7903146 polymorphism modulates the effect of artichoke leaf extract supplementation on insulin resistance in metabolic syndrome: A randomized, double-blind, placebo-controlled trial. J. Integr. Med. 16, 329–334. doi:10.1016/j.joim.2018.05.006
El Barky, A. (2020). The Potential role of apigenin in diabetes mellitus. Int. J. Clin. Case Rep. Rev. 3 (1). doi:10.31579/2690-4861/032
Escudero, N. L., De Arellano, M. L., Fernández, S., Albarracín, G., and Mucciarelli, S. (2003). Taraxacum officinale as a food source. Plant Foods Hum. Nutr. 58, 1–10. doi:10.1023/B:QUAL.0000040365.90180.b3
Fan, Z.-W., Pang, Y.-X., Wang, K., Yu, F.-L., Wang, D., Yang, Q., et al. (2015). Blumea balsamifera oil for the acceleration of healing of burn injuries. Molecules 20, 17166–17179. doi:10.3390/molecules200917166
Faraji, H. (2018). Effect of decaffeinated coffee-enriched chlorogenic acid on blood glucose levels in healthy controls: A systematic review. Int. J. Prev. Med. 9, 112. doi:10.4103/ijpvm.IJPVM_343_17
Feng, L., Zhai, Y.-Y., Xu, J., Yao, W.-F., Cao, Y.-D., Cheng, F.-F., et al. (2019). A review on traditional uses, phytochemistry and pharmacology of Eclipta prostrata (L) L. J. Ethnopharmacol. 245, 112109. doi:10.1016/j.jep.2019.112109
Fernández-Millán, E., Ramos, S., Alvarez, C., Bravo, L., Goya, L., and Martín, M. Á. (2014). Microbial phenolic metabolites improve glucose-stimulated insulin secretion and protect pancreatic beta cells against tert-butyl hydroperoxide-induced toxicity via ERKs and PKC pathways. Food Chem. Toxicol. Int. J. Publ. Br. Ind. Biol. Res. Assoc. 66, 245–253. doi:10.1016/j.fct.2014.01.044
Fierascu, I., Ungureanu, C., Avramescu, S. M., Fierascu, R. C., Ortan, A., Soare, L. C., et al. (2015). In vitro antioxidant and antifungal properties of Achillea millefolium L. Rom. Biotechnol. Lett. 20, 10626–10636.
Flamm, G., Glinsmann, W., Kritchevsky, D., Prosky, L., and Roberfroid, M. (2001). Inulin and oligofructose as dietary fiber: A review of the evidence. Crit. Rev. Food Sci. Nutr. 41, 353–362. doi:10.1080/20014091091841
Flegal, K. M., Graubard, B. I., Williamson, D. F., and Gail, M. H. (2007). Cause-specific excess deaths associated with underweight, overweight, and obesity. JAMA 298, 2028–2037. doi:10.1001/jama.298.17.2028
Fu, Z., Gilbert, E. R., Pfeiffer, L., Zhang, Y., Fu, Y., and Liu, D. (2012). Genistein ameliorates hyperglycemia in a mouse model of nongenetic type 2 diabetes. Appl. Physiol. Nutr. Metab. 37, 480–488. doi:10.1139/h2012-005
Fursenco, C., Calalb, T., Uncu, L., Dinu, M., and Ancuceanu, R. (2020). Solidago virgaurea L.: A review of its ethnomedicinal uses, phytochemistry, and pharmacological activities. Biomolecules 10, 1619–1631. doi:10.3390/biom10121619
Garcia-Oliveira, P., Barral, M., Carpena, M., Gullón, P., Fraga-Corral, M., Otero, P., et al. (2021). Traditional plants from Asteraceae family as potential candidates for functional food industry. Food Funct. 12, 2850–2873. doi:10.1039/D0FO03433A
George Kallivalappil, G., and Kuttan, G. (2019). Evaluation of the anti-inflammatory and urotoxicity ameliorative effects of γ-humulene containing active fraction of Emilia sonchifolia (L) DC. Inflammopharmacology 27, 409–420. doi:10.1007/s10787-017-0423-3
Gerbino, A., Schena, G., Milano, S., Milella, L., Barbosa, A. F., Armentano, F., et al. (2016). Spilanthol from Acmella oleracea lowers the intracellular levels of cAMP impairing NKCC2 phosphorylation and water channel AQP2 membrane expression in mouse kidney. PLoS One 11, e0156021. doi:10.1371/journal.pone.0156021
Ghaderi, A., and Sonboli, A. (2019). Chemical composition and antimicrobial activity of the essential oil of Tanacetum walteri (Anthemideae-Asteraceae) from Iran. Nat. Prod. Res. 33, 1787–1790. doi:10.1080/14786419.2018.1434640
Ghlissi, Z., Sayari, N., Kallel, R., Bougatef, A., and Sahnoun, Z. (2016). Antioxidant, antibacterial, anti-inflammatory and wound healing effects of Artemisia campestris aqueous extract in rat. Biomed. Pharmacother. 84, 115–122. doi:10.1016/j.biopha.2016.09.018
Ghobadian, Z., Ahmadi, M. R. H., Rezazadeh, L., Hosseini, E., Kokhazadeh, T., and Ghavam, S. (2015). In vitro evaluation of Achillea Millefolium on the production and stimulation of human skin fibroblast cells (HFS-PI-16). Med. Arch. (Sarajevo, Bosnia Herzeg. 69, 212–217. doi:10.5455/medarh.2015.69.212-217
Gilbert, E. R., and Liu, D. (2013). Anti-diabetic functions of soy isoflavone genistein: Mechanisms underlying its effects on pancreatic β-cell function. Food Funct. 4, 200–212. doi:10.1039/c2fo30199g
Giugliano, D., Ceriello, A., and Paolisso, G. (1996). Oxidative stress and diabetic vascular complications. Diabetes Care 19, 257–267. doi:10.2337/diacare.19.3.257
Goje, L. J., Maisamari, C. A., Maigari, F. U., Ghamba, P. E., Goji, A. D. T., and Mshelia, P. P. (2014). The hypoglycemic and hypolipidemic effects of the aqueous extract of Vernonia amygdalina leaves on alloxan induced diabetic albino rats. Int. J. Sci. 3, 5–11.
González-Castejón, M., Visioli, F., and Rodriguez-Casado, A. (2012). Diverse biological activities of dandelion. Nutr. Rev. 70, 534–547. doi:10.1111/j.1753-4887.2012.00509.x
Gowd, V., Karim, N., Shishir, M. R. I., Xie, L., and Chen, W. (2019). Dietary polyphenols to combat the metabolic diseases via altering gut microbiota. Trends food Sci. Technol. 93, 81–93. doi:10.1016/j.tifs.2019.09.005
Gridling, M., Stark, N., Madlener, S., Lackner, A., Popescu, R., Benedek, B., et al. (2009). In vitro anti-cancer activity of two ethno-pharmacological healing plants from Guatemala pluchea odorata and Phlebodium decumanum. Int. J. Oncol. 34, 1117–1128. doi:10.3892/ijo_00000239
Gross, S. C., Goodarzi, G., Watabe, M., Bandyopadhyay, S., Pai, S. K., and Watabe, K. (2002). Antineoplastic activity of Solidago virgaurea on prostatic tumor cells in an SCID mouse model. Nutr. Cancer 43, 76–81. doi:10.1207/S15327914NC431_9
Gupta, S. K., Dongare, S., Mathur, R., Mohanty, I. R., Srivastava, S., Mathur, S., et al. (2015). Genistein ameliorates cardiac inflammation and oxidative stress in streptozotocin-induced diabetic cardiomyopathy in rats. Mol. Cell. Biochem. 408, 63–72. doi:10.1007/s11010-015-2483-2
Gyang, S., Nyam, D., and Sokomba, E. (2005). Hypoglycaemic activity of Vernonia amygdalina (chloroform extract) in normoglycaemic and alloxan-induced hyperglycaemic rats. J. Pharm. Biores. 1, 61–66. doi:10.4314/jpb.v1i1.32050
Hamden, K., Carreau, S., Boujbiha, M. A., Lajmi, S., Aloulou, D., Kchaou, D., et al. (2008). Hyperglycaemia, stress oxidant, liver dysfunction and histological changes in diabetic male rat pancreas and liver: Protective effect of 17 beta-estradiol. Steroids 73, 495–501. doi:10.1016/j.steroids.2007.12.026
Heidarian, E., and Soofiniya, Y. (2011). Hypolipidemic and hypoglycemic effects of aerial part of cynara scolymus in streptozotocin-induced diabetic rats. J. Med. Plants Res. 5, 2717–2723.
Hemalatha, S., Ayyappan, T. M., Shanmugam, S., Nagavalli, D., and Shrivijayakirubha, T. (2006). Evaluation of antidiabetic and diuretic activity of polyherbal formulation. Indian J. Tradit. Knowl. 5, 468–470.
Hemmerle, H., Burger, H. J., Below, P., Schubert, G., Rippel, R., Schindler, P. W., et al. (1997). Chlorogenic acid and synthetic chlorogenic acid derivatives: Novel inhibitors of hepatic glucose-6-phosphate translocase. J. Med. Chem. 40, 137–145. doi:10.1021/jm9607360
Henry-Vitrac, C., Ibarra, A., Roller, M., Mérillon, J.-M., and Vitrac, X. (2010). Contribution of chlorogenic acids to the inhibition of human hepatic glucose-6-phosphatase activity in vitro by Svetol, a standardized decaffeinated green coffee extract. J. Agric. Food Chem. 58, 4141–4144. doi:10.1021/jf9044827
Hobbs, J. E. (2020). Food supply chains during the COVID-19 pandemic. Can. J. Agric. Econ. 68, 171–176. doi:10.1111/cjag.12237
Hong, B. N., Nam, Y. H., Woo, S. H., and Kang, T. H. (2017). Chlorogenic acid rescues sensorineural auditory function in a diabetic animal model. Neurosci. Lett. 640, 64–69. doi:10.1016/j.neulet.2017.01.030
Hsu, F. L., Chen, Y. C., and Cheng, J. T. (2000). Caffeic acid as active principle from the fruit of Xanthium strumarium to lower plasma glucose in diabetic rats. Planta Med. 66, 228–230. doi:10.1055/s-2000-8561
Hudaib, M., Mohammad, M., Bustanji, Y., Tayyem, R., Yousef, M., Abuirjeie, M., et al. (2008). Ethnopharmacological survey of medicinal plants in Jordan, Mujib nature reserve and surrounding area. J. Ethnopharmacol. 120, 63–71. doi:10.1016/j.jep.2008.07.031
Hussain, Z., Waheed, A., Qureshi, R. A., Burdi, D. K., Verspohl, E. J., Khan, N., et al. (2004). The effect of medicinal plants of Islamabad and Murree region of Pakistan on insulin secretion from INS-1 cells. Phytother. Res. 18, 73–77. doi:10.1002/ptr.1372
Igarashi, K., and Takahashi, M. (2017). Vegetable soup produced by mixing steamed weet potato with rice malt and component chlogenic acid ameliorate diabetes in KK-[A.sup.y] Mice. Curr. Top. Nutraceutical Res. 15, 151–161.
Ingawale, A. S., Sadiq, M. B., Nguyen, L. T., and Ngan, T. B. (2018). Optimization of extraction conditions and assessment of antioxidant, α-glucosidase inhibitory and antimicrobial activities of Xanthium strumarium L. fruits. Biocatal. Agric. Biotechnol. 14, 40–47. doi:10.1016/j.bcab.2018.02.004
Islam, M. R., Uddin, M. Z., Rahman, M. S., Tutul, E., Rahman, M. Z., Hassan, M. A., et al. (2009). Ethnobotanical, phytochemical and toxicological studies of Xanthium strumarium L. Bangladesh Med. Res. Counc. Bull. 35, 84–90. doi:10.3329/bmrcb.v35i3.3658
Islam, M. T., Das, P. R., Kabir, M. H., Akter, S., Khatun, Z., Haque, M. M., et al. (2012). Acanthaceae and Asteraceae family plants used by folk medicinal practitioners for treatment of malaria in Chittagong and Sylhet Divisions of Bangladesh. Am. J. Sustain. Agric. 6, 146–152.
Ismail, H., Gillespie, A. L., Calderwood, D., Iqbal, H., Gallagher, C., Chevallier, O. P., et al. (2019). The health promoting bioactivities of Lactuca sativa can be enhanced by genetic modulation of plant secondary metabolites. Metabolites 9, 97. doi:10.3390/metabo9050097
Jadhav, G. B., and Upasani, C. D. (2011). Antihypertensive effect of Silymarin on DOCA salt induced hypertension in unilateral nephrectomized rats. Orient. Pharm. Exp. Med. 11, 101–106. doi:10.1007/s13596-011-0018-2
Jahan, R., Al-Nahain, A., Majumder, S., and Rahmatullah, M. (2014). Ethnopharmacological significance of Eclipta alba (L) hassk. (Asteraceae). Int. Sch. Res. Not. 2014, 385969. doi:10.1155/2014/385969
Jain, A. K., Tewari-Singh, N., Inturi, S., Kumar, D., Orlicky, D. J., Agarwal, C., et al. (2015). Flavanone silibinin treatment attenuates nitrogen mustard-induced toxic effects in mouse skin. Toxicol. Appl. Pharmacol. 285, 71–78. doi:10.1016/j.taap.2015.03.009
Jaiswal, N., Bhatia, V., Srivastava, S. P., Srivastava, A. K., and Tamrakar, A. K. (2012). Antidiabetic effect of Eclipta alba associated with the inhibition of α-glucosidase and aldose reductase. Nat. Prod. Res. 26, 2363–2367. doi:10.1080/14786419.2012.662648
Jang, E., Kim, B.-J., Lee, K.-T., Inn, K.-S., and Lee, J.-H. (2015). A survey of therapeutic effects of Artemisia capillaris in liver diseases. Evid. Based. Complement. Altern. Med. 2015, 728137. doi:10.1155/2015/728137
Jarić, S., Kostić, O., Mataruga, Z., Pavlović, D., Pavlović, M., Mitrović, M., et al. (2018). Traditional wound-healing plants used in the Balkan region (Southeast Europe). J. Ethnopharmacol. 211, 311–328. doi:10.1016/j.jep.2017.09.018
Jayathirtha, M. G., and Mishra, S. H. (2004). Preliminary immunomodulatory activities of methanol extracts of Eclipta alba and Centella asiatica. Phytomedicine 11, 361–365. doi:10.1078/0944711041495236
Jayaweera, U., Akowuah, G. A., Ling, C. Y., Kumar, P. V., and Herapathdeniya, S. (2022). Diabetes and Vernonia amygdalina delile (asteraceae). Biointerface Res. Appl. Chem. 12, 4496–4517. doi:10.33263/BRIAC124.44964517
Jin, S., Chang, C., Zhang, L., Liu, Y., Huang, X., and Chen, Z. (2015). Chlorogenic acid improves late diabetes through adiponectin receptor signaling pathways in db/db mice. PLoS One 10, e0120842. doi:10.1371/journal.pone.0120842
Jin, T., Song, Z., Weng, J., and Fantus, I. G. (2018). Curcumin and other dietary polyphenols: Potential mechanisms of metabolic actions and therapy for diabetes and obesity. Am. J. Physiol. Endocrinol. Metab. 314, E201–E205. doi:10.1152/ajpendo.00285.2017
Jones, P. M., and Persaud, S. J. (1998). Protein kinases, protein phosphorylation, and the regulation of insulin secretion from pancreatic β-cells. Endocr. Rev. 19, 429–461. doi:10.1210/edrv.19.4.0339
Karamenderes, C., and Apaydin, S. (2003). Antispasmodic effect of Achillea nobilis L. subsp. sipylea (O. Schwarz) Bässler on the rat isolated duodenum. J. Ethnopharmacol. 84, 175–179. doi:10.1016/s0378-8741(02)00296-9
Kasali, F. M., Kadima, J. N., Peter, E. L., Mtewa, A. G., Ajayi, C. O., Tusiimire, J., et al. (2021). Antidiabetic medicinal plants used in democratic republic of Congo: A critical review of ethnopharmacology and bioactivity data. Front. Pharmacol. 12, 757090. doi:10.3389/fphar.2021.757090
Khan, A. V., and Khan, M. A. (2008). Ethnomedicinal uses of Eclipta prostrta linn. Indian J. Tradit. Knowl. 7, 316–320.
Khan, Z. A., Khare, S., and Dubey, B. K. (2020). Comparative extraction, phytochemical screening and in vitro biological activities of Eclipta prostrata extract. J. Drug Deliv. Ther. 10, 148–152. doi:10.22270/jddt.v10i4-s.4263
Kharroubi, A. T., and Darwish, H. M. (2015). Diabetes mellitus: The epidemic of the century. World J. Diabetes 6, 850–867. doi:10.4239/wjd.v6.i6.850
Khuda, F., Iqbal, Z., Khan, A., ZakiullahShah, Y., and Khan, A. (2014). Report: Screening of selected medicinal plants for their enzyme inhibitory potential - a validation of their ethnopharmacological uses. Pak. J. Pharm. Sci. 27, 593–596.
Kocsis, L., Liebhard, P., and Praznik, W. (2007). Effect of seasonal changes on content and profile of soluble carbohydrates in tubers of different varieties of Jerusalem artichoke (Helianthus tuberosus L). J. Agric. Food Chem. 55, 9401–9408. doi:10.1021/jf0717485
Konishi, K., Wada, K., Yamakawa, M., Goto, Y., Mizuta, F., Koda, S., et al. (2019). Dietary soy intake is inversely associated with risk of type 2 diabetes in Japanese women but not in men. J. Nutr. 149, 1208–1214. doi:10.1093/jn/nxz047
Kuczmannová, A., Balažová, A., Račanská, E., Kameníková, M., Fialová, S., Majerník, J., et al. (2016). Agrimonia eupatoria L. and Cynara cardunculus L. water infusions: Comparison of anti-diabetic activities. Molecules 21, 564. doi:10.3390/molecules21050564
Kumari, C. S., Govindasamy, S., and Sukumar, E. (2006). Lipid lowering activity of Eclipta prostrata in experimental hyperlipidemia. J. Ethnopharmacol. 105, 332–335. doi:10.1016/j.jep.2005.10.031
Kupiecki, F. P., Ogzewalla, C. D., and Schell, F. M. (1974). Isolation and characterization of a hypoglycemic agent from Xanthium strumarium. J. Pharm. Sci. 63, 1166–1167. doi:10.1002/jps.2600630736
Lafay, S., Morand, C., Manach, C., Besson, C., and Scalbert, A. (2006). Absorption and metabolism of caffeic acid and chlorogenic acid in the small intestine of rats. Br. J. Nutr. 96, 39–46. doi:10.1079/bjn20061714
Lans, C., Turner, N., Khan, T., and Brauer, G. (2007). Ethnoveterinary medicines used to treat endoparasites and stomach problems in pigs and pets in British Columbia, Canada. Vet. Parasitol. 148, 325–340. doi:10.1016/j.vetpar.2007.06.014
Larsen, N., Vogensen, F. K., van den Berg, F. W. J., Nielsen, D. S., Andreasen, A. S., Pedersen, B. K., et al. (2010). Gut microbiota in human adults with type 2 diabetes differs from non-diabetic adults. PLoS One 5, e9085. doi:10.1371/journal.pone.0009085
Lecoultre, V., Carrel, G., Egli, L., Binnert, C., Boss, A., MacMillan, E. L., et al. (2014). Coffee consumption attenuates short-term fructose-induced liver insulin resistance in healthy men. Am. J. Clin. Nutr. 99, 268–275. doi:10.3945/ajcn.113.069526
Lee, T. H., Jung, H., Park, K. H., Bang, M. H., Baek, N.-I., and Kim, J. (2014). Jaceosidin, a natural flavone, promotes angiogenesis via activation of VEGFR2/FAK/PI3K/AKT/NF-κB signaling pathways in endothelial cells. Exp. Biol. Med. (Maywood). 239, 1325–1334. doi:10.1177/1535370214533883
Li, C., Ford, E. S., McGuire, L. C., and Mokdad, A. H. (2007). Association of metabolic syndrome and insulin resistance with congestive heart failure: Findings from the third national health and nutrition examination survey. J. Epidemiol. Community Health 61, 67–73. doi:10.1136/jech.2006.048173
Li, S., Zhou, S., Yang, W., and Meng, D. (2018). Gastro-protective effect of edible plant Artemisia argyi in ethanol-induced rats via normalizing inflammatory responses and oxidative stress. J. Ethnopharmacol. 214, 207–217. doi:10.1016/j.jep.2017.12.023
Liu, C. L., Yan, L., Cai, K. R., Sun, K., Qi, Y., Han, Y. L., et al. (2018). Effects of soybean isoflavones on Wnt/β-catenin and the TGF-β1 signaling pathway in renal tissue of type 2 diabetic rats. J. Biol. Regul. Homeost. Agents 32, 455–464.
Loi, B., Fantini, N., Colombo, G., Gessa, G. L., Riva, A., Bombardelli, E., et al. (2013). Reducing effect of a combination of Phaseolus vulgaris and Cynara scolymus extracts on food intake and glycemia in rats. Phytother. Res. 27, 258–263. doi:10.1002/ptr.4704
López-López, E., Bajorath, J., and Medina-Franco, J. L. (2021). Informatics for chemistry, biology, and biomedical sciences. J. Chem. Inf. Model. 61, 26–35. doi:10.1021/acs.jcim.0c01301
Machado, V. S., Camponogara, C., Oliveira, S. M., Baldissera, M. D., Sagrillo, M. R., Gundel, S. D. A. S., et al. (2020). Topical hydrogel containing Achyrocline satureioides oily extract (free and nanocapsule) has anti-inflammatory effects and thereby minimizes irritant contact dermatitis. An. Acad. Bras. Cienc. 92, e20191066. doi:10.1590/0001-3765202020191066
Madariaga-Mazón, A., Naveja, J. J., Medina-Franco, J. L., Noriega-Colima, K. O., and Martinez-Mayorga, K. (2021). DiaNat-DB: A molecular database of antidiabetic compounds from medicinal plants. RSC Adv. 11, 5172–5178. doi:10.1039/D0RA10453A
Maria-Ferreira, D., da Silva, L. M., Mendes, D. A. G. B., Cabrini, D. A., Nascimento, A. M., Iacomini, M., et al. (2014). Rhamnogalacturonan from Acmella oleracea (L) R.K. Jansen: Gastroprotective and ulcer healing properties in rats. PLoS One 9, e84762. doi:10.1371/journal.pone.0084762
Martín, J., Torrell, M., Korobkov, A. A., and Vallès, J. (2003). Palynological features as a systematic marker in Artemisia L. and related genera (Asteraceae, Anthemideae) - II: Implications for subtribe artemisiinae delimitation. Plant Biol. 5, 85–93. doi:10.1055/s-2003-37979
Martínez-López, S., Sarriá, B., Mateos, R., and Bravo-Clemente, L. (2019). Moderate consumption of a soluble green/roasted coffee rich in caffeoylquinic acids reduces cardiovascular risk markers: Results from a randomized, cross-over, controlled trial in healthy and hypercholesterolemic subjects. Eur. J. Nutr. 58, 865–878. doi:10.1007/s00394-018-1726-x
Matsui, T., Ogunwande, I. A., Abesundara, K. J. M., and Matsumoto, K. (2006). Anti-hyperglycemic potential of natural products. Mini Rev. Med. Chem. 6, 349–356. doi:10.2174/138955706776073484
Mei, X., Zhou, L., Zhang, T., Lu, B., Sheng, Y., and Ji, L. (2018). Chlorogenic acid attenuates diabetic retinopathy by reducing VEGF expression and inhibiting VEGF-mediated retinal neoangiogenesis. Vasc. Pharmacol. 101, 29–37. doi:10.1016/j.vph.2017.11.002
Mellem, J., Baijnath, H., and Odhav, B. (2015). Antidiabetic potential of Brachylaena discolor. Afr. J. Tradit. Complement. Altern. Med. 12, 38. doi:10.4314/ajtcam.v12i1.6
Mellem, J. J. (2013). “Isolation and characterization of the leaves of Brachylaena discolor extract as an anti-diabetic agent,”. Thesis (Durban Uninersity).
Memariani, Z., Moeini, R., Hamedi, S. S., Gorji, N., and Mozaffarpur, S. A. (2018). Medicinal plants with antithrombotic property in Persian medicine: A mechanistic review. J. Thromb. Thrombolysis 45, 158–179. doi:10.1007/s11239-017-1580-3
Michel, J., Abd Rani, N. Z., and Husain, K. (2020). A Review on the potential use of medicinal plants from asteraceae and Lamiaceae plant family in cardiovascular diseases. Front. Pharmacol. 11, 852. doi:10.3389/fphar.2020.00852
Min, S.-W., Kim, N.-J., Baek, N.-I., and Kim, D.-H. (2009). Inhibitory effect of eupatilin and jaceosidin isolated from Artemisia princeps on carrageenan-induced inflammation in mice. J. Ethnopharmacol. 125, 497–500. doi:10.1016/j.jep.2009.06.001
Mirmiran, P., Bahadoran, Z., and Azizi, F. (2014). Functional foods-based diet as a novel dietary approach for management of type 2 diabetes and its complications: A review. World J. Diabetes 5, 267–281. doi:10.4239/wjd.v5.i3.267
Mohammadhosseini, M., Sarker, S. D., and Akbarzadeh, A. (2017). Chemical composition of the essential oils and extracts of Achillea species and their biological activities: A review. J. Ethnopharmacol. 199, 257–315. doi:10.1016/j.jep.2017.02.010
Móricz, Á. M., Jamshidi-Aidji, M., Krüzselyi, D., Darcsi, A., Böszörményi, A., Csontos, P., et al. (2020). Distinction and valorization of 30 root extracts of five goldenrod (Solidago) species. J. Chromatogr. A 1611, 460602. doi:10.1016/j.chroma.2019.460602
Móricz, Á. M., Ott, P. G., Häbe, T. T., Darcsi, A., Böszörményi, A., Alberti, Á., et al. (2016). Effect-directed discovery of bioactive compounds followed by highly targeted characterization, isolation and identification, exemplarily shown for Solidago virgaurea. Anal. Chem. 88, 8202–8209. doi:10.1021/acs.analchem.6b02007
Mostafa, I., Abd El-Aziz, E., Hafez, S., and El-Shazly, A. (2013). Chemical constituents and biological activities of Galinsoga parviflora cav. (Asteraceae) from Egypt. Z. Naturforsch. C 68, 285–292. doi:10.1515/znc-2013-7-805
Mubarak, A., Hodgson, J. M., Considine, M. J., Croft, K. D., and Matthews, V. B. (2013). Supplementation of a high-fat diet with chlorogenic acid is associated with insulin resistance and hepatic lipid accumulation in mice. J. Agric. Food Chem. 61, 4371–4378. doi:10.1021/jf400920x
Mukherjee, P. K., Maiti, K., Mukherjee, K., and Houghton, P. J. (2006). Leads from Indian medicinal plants with hypoglycemic potentials. J. Ethnopharmacol. 106, 1–28. doi:10.1016/j.jep.2006.03.021
Nabi, R., and Abdullah, M. (2020). Effect of quercetin on parenchymatous organ of the alloxan induced diabetes in male rats. Int. J. Res. Med. Sci. 8, 3809. doi:10.18203/2320-6012.ijrms20204862
Narendiran, S., Mohanambal, E., Kumar, P. S., Shankar, M., Kuttimani, T., and Vijayakumar, B. (2011). Study of anti diabetic and anti oxidant activities of methanolic extract of Xanthium strumarium (Linn) stems on diabetic rats. J. Pharm. Res. 4, 3728.
Narita, Y., and Inouye, K. (2009). Kinetic analysis and mechanism on the inhibition of chlorogenic acid and its components against porcine pancreas alpha-amylase isozymes I and II. Agric. Food Chem. 57, 9218–9225. doi:10.1021/jf9017383
Nascimento, A. M., de Souza, L. M., Baggio, C. H., Werner, M. F. de P., Maria-Ferreira, D., da Silva, L. M., et al. (2013). Gastroprotective effect and structure of a rhamnogalacturonan from Acmella oleracea. Phytochemistry 85, 137–142. doi:10.1016/j.phytochem.2012.08.024
Neeraja, P. V., and Margaret, E. (2011). Eclipta alba (L) hassk: A valuable medicinal herb. Int. J. Curr. Pharm. Rev. Res. 2, 188–197.
Nicolaus, C., Junghanns, S., Hartmann, A., Murillo, R., Ganzera, M., and Merfort, I. (2017). In vitro studies to evaluate the wound healing properties of Calendula officinalis extracts. J. Ethnopharmacol. 196, 94–103. doi:10.1016/j.jep.2016.12.006
Nie, Q., Chen, H., Hu, J., Fan, S., and Nie, S. (2019). Dietary compounds and traditional Chinese medicine ameliorate type 2 diabetes by modulating gut microbiota. Crit. Rev. Food Sci. Nutr. 59, 848–863. doi:10.1080/10408398.2018.1536646
Nopparat, J., Nualla-Ong, A., and Phongdara, A. (2019). Ethanolic extracts of Pluchea indica (L) leaf pretreatment attenuates cytokine-induced β-cell apoptosis in multiple low-dose streptozotocin-induced diabetic mice. PLoS One 14, e0212133. doi:10.1371/journal.pone.0212133
Nopparat, J., Nualla-Ong, A., and Phongdara, A. (2020). Treatment with Pluchea indica (L) Less. leaf ethanol extract alleviates liver injury in multiple low-dose streptozotocin-induced diabetic BALB/c mice. Exp. Ther. Med. 20, 1385–1396. doi:10.3892/etm.2020.8877
Nurul Islam, M., Jung, H. A., Sohn, H. S., Kim, H. M., and Choi, J. S. (2013). Potent α-glucosidase and protein tyrosine phosphatase 1B inhibitors from Artemisia capillaris. Arch. Pharm. Res. 36, 542–552. doi:10.1007/s12272-013-0069-7
Odeyemi, S., and Bradley, G. (2018). Medicinal plants used for the traditional management of diabetes in the Eastern Cape, South Africa: Pharmacology and toxicology. Molecules 23, 2759. doi:10.3390/molecules23112759
Odle, B., Dennison, N., Al-Nakkash, L., Broderick, T. L., and Plochocki, J. H. (2017). Genistein treatment improves fracture resistance in obese diabetic mice. BMC Endocr. Disord. 17, 1. doi:10.1186/s12902-016-0144-4
Okolie, U. V., Okeke, C. E., Oli, J. M., and Ehiemere, I. O. (2008). Hypoglycemic indices of Vernonia amygdalina on postprandial blood glucose concentration of healthy humans. Afr. J. Biotechnol. 7, 4581–4585.
Onal, S., Timur, S., Okutucu, B., and Zihnioğlu, F. (2005). Inhibition of alpha-glucosidase by aqueous extracts of some potent antidiabetic medicinal herbs. Prep. Biochem. Biotechnol. 35, 29–36. doi:10.1081/PB-200041438
Osinubi, A. A. A. (2006). Effects of Vernonia amygdalina and Chlorpropamide on blood glusoce. Med. J. Islam. World Acad. Sci. 16 (3), 115–119.
Oyeyemi, I. T., Akinlabi, A. A., Adewumi, A., Aleshinloye, A. O., and Oyeyemi, O. T. (2018). Vernonia amygdalina: A folkloric herb with anthelminthic properties. Beni-Suef Univ. J. Basic Appl. Sci. 7, 43–49. doi:10.1016/j.bjbas.2017.07.007
Özbilgin, S., Akkol, E. K., Ergene Öz, B., Ilhan, M., Saltan, G., Acıkara, Ö. B., et al. (2018). In vivo activity assessment of some Tanacetum species used as traditional wound healer along with identification of the phytochemical profile by a new validated HPLC method. Iran. J. Basic Med. Sci. 21, 145–152. doi:10.22038/IJBMS.2018.24258.6055
Panda, S. K., da Silva, L. C. N., Sahal, D., and Leonti, M. (2019). Editorial: Ethnopharmacological studies for the development of drugs with special reference to Asteraceae. Front. Pharmacol. 10, 955. doi:10.3389/fphar.2019.00955
Panda, S. K., and Luyten, W. (2018). Antiparasitic activity in Asteraceae with special attention to ethnobotanical use by the tribes of Odisha, India. Parasite 25, 10. doi:10.1051/parasite/2018008
Pang, Y., Wang, D., Fan, Z., Chen, X., Yu, F., Hu, X., et al. (2014a). Blumea balsamifera-a phytochemical and pharmacological review. Molecules 19, 9453–9477. doi:10.3390/molecules19079453
Pang, Y., Wang, D., Hu, X., Wang, H., Fu, W., Fan, Z., et al. (2014b). Effect of volatile oil from Blumea Balsamifera (L) DC. leaves on wound healing in mice. J. Tradit. Chin. Med. 34, 716–724. doi:10.1016/s0254-6272(15)30087-x
Pang, Y., Zhang, Y., Huang, L., Xu, L., Wang, K., Wang, D., et al. (2017). Effects and mechanisms of total flavonoids from Blumea balsamifera (L) DC. on skin wound in rats. Int. J. Mol. Sci. 18, 2766. doi:10.3390/ijms18122766
Parente, L. M. L., Lino Júnior, R. de S., Tresvenzol, L. M. F., Vinaud, M. C., de Paula, J. R., and Paulo, N. M. (2012). Wound healing and anti-inflammatory effect in animal models of Calendula officinalis L. growing in Brazil. Evid. Based. Complement. Altern. Med. 2012, 375671. doi:10.1155/2012/375671
Park, S. W., Oh, T. Y., Kim, Y. S., Sim, H., Park, S. J., Jang, E. J., et al. (2008). Artemisia asiatica extracts protect against ethanol-induced injury in gastric mucosa of rats. J. Gastroenterol. Hepatol. 23, 976–984. doi:10.1111/j.1440-1746.2008.05333.x
Parveen, G., and Ali, M. (2019). Extraction isolation and phytochemical screening of leaves and stems of Bidens pilosa and evaluation of antifungal potential of extracts. IOSR J. Pharm. Biol. Sci. 14, 2319–7676. doi:10.9790/3008-1404027385
Pereira, L. X., Silva, H. K. C., Longatti, T. R., Silva, P. P., Di Lorenzo Oliveira, C., de Freitas Carneiro Proietti, A. B., et al. (2017). Achyrocline alata potentiates repair of skin full thickness excision in mice. J. Tissue Viability 26, 289–299. doi:10.1016/j.jtv.2017.09.005
Petlevski, R., Hadzija, M., Slijepcević, M., Juretić, D., and Petrik, J. (2003). Glutathione S-transferases and malondialdehyde in the liver of NOD mice on short-term treatment with plant mixture extract P-9801091. Phytother. Res. 17, 311–314. doi:10.1002/ptr.1128
Piché, M.-E., Tchernof, A., and Després, J.-P. (2020). Obesity phenotypes, diabetes, and cardiovascular diseases. Circ. Res. 126, 1477–1500. doi:10.1161/CIRCRESAHA.120.316101
Pilon, L., Oetterer, M., Gallo, C. R., and Spoto, M. H. F. (2006). Shelf life of minimally processed carrot and green pepper. Cienc. Tecnol. Aliment. 26, 150–158. doi:10.1590/S0101-20612006000100025
Qin, J., Li, Y., Cai, Z., Li, S., Zhu, J., Zhang, F., et al. (2012). A metagenome-wide association study of gut microbiota in type 2 diabetes. Nature 490, 55–60. doi:10.1038/nature11450
Rahman, M., Rahman, M. Z., Begum, B., Chowdhury, R., Islam, S., and Rashid, M. (2011). Antidiabetic principle from Eclipta prostrata. Lat. Am. J. Pharm. 30, 1656–1660.
Rai, P. K., and Lalramnghinglova, H. (2011). Threatened and less known ethnomedicinal plants of an indo-Burma hotspot region: Conservation implications. Environ. Monit. Assess. 178, 53–62. doi:10.1007/s10661-010-1670-6
Reis, C. E. G., Dórea, J. G., and da Costa, T. H. M. (2018). Effects of coffee consumption on glucose metabolism: A systematic review of clinical trials. J. Tradit. Complement. Med. 9, 184–191. doi:10.1016/j.jtcme.2018.01.001
Retta, D., Dellacassa, E., Villamil, J., Suárez, S. A., and Bandoni, A. L. (2012). Marcela, a promising medicinal and aromatic plant from Latin America: A review. Ind. Crops Prod. 38, 27–38. doi:10.1016/j.indcrop.2012.01.006
Rivera, G., Bocanegra-García, V., and Monge, A. (2010). Traditional plants as source of functional foods: A review plantas tradicionales como fuente de alimentos funcionales: Una revisión. CYTA - J. Food 8, 159–167. doi:10.1080/19476330903322978
Rockwood, S., Mason, D., Lord, R., Lamar, P., Prozialeck, W., and Al-Nakkash, L. (2019). Genistein diet improves body weight, serum glucose and triglyceride levels in both male and female ob/ob mice. Diabetes. Metab. Syndr. Obes. 12, 2011–2021. doi:10.2147/DMSO.S216312
Rodríguez-Chávez, J. L., Egas, V., Linares, E., Bye, R., Hernández, T., Espinosa-García, F. J., et al. (2017). Mexican arnica (Heterotheca inuloides Cass. Asteraceae: Astereae): Ethnomedical uses, chemical constituents and biological properties. J. Ethnopharmacol. 195, 39–63. doi:10.1016/j.jep.2016.11.021
Rolnik, A., and Olas, B. (2021). The plants of the asteraceae family as agents in the protection of human health. Int. J. Mol. Sci. 22, 3009–3010. doi:10.3390/ijms22063009
Romero-Cerecero, O., Islas-Garduño, A. L., Zamilpa, A., and Tortoriello, J. (2017). Effectiveness of Ageratina pichinchensis extract in patients with vulvovaginal candidiasis. A randomized, double-blind, and controlled pilot study. Phytother. Res. 31, 885–890. doi:10.1002/ptr.5802
Romero-Cerecero, O., Román-Ramos, R., Zamilpa, A., Jiménez-Ferrer, J. E., Rojas-Bribiesca, G., and Tortoriello, J. (2009). Clinical trial to compare the effectiveness of two concentrations of the Ageratina pichinchensis extract in the topical treatment of onychomycosis. J. Ethnopharmacol. 126, 74–78. doi:10.1016/j.jep.2009.08.007
Romero-Cerecero, O., Zamilpa, A., Díaz-García, E. R., and Tortoriello, J. (2014). Pharmacological effect of Ageratina pichinchensis on wound healing in diabetic rats and genotoxicity evaluation. J. Ethnopharmacol. 156, 222–227. doi:10.1016/j.jep.2014.09.002
Romero-Cerecero, O., Zamilpa, A., González-Cortazar, M., Alonso-Cortés, D., Jiménez-Ferrer, E., Nicasio-Torres, P., et al. (2013). Pharmacological and chemical study to identify wound-healing active compounds in Ageratina pichinchensis. Planta Med. 79, 622–627. doi:10.1055/s-0032-1328462
Romero-Cerecero, O., Zamilpa, A., Jiménez-Ferrer, E., and Tortoriello, J. (2012b). Therapeutic effectiveness of Ageratina pichinchensis on the treatment of chronic interdigital tinea pedis: A randomized, double-blind clinical trial. J. Altern. Complement. Med. 18, 607–611. doi:10.1089/acm.2011.0319
Romero-Cerecero, O., Zamilpa, A., and Tortoriello, J. (2015). Effectiveness and tolerability of a standardized extract from Ageratina pichinchensis in patients with diabetic foot ulcer: A randomized, controlled pilot study. Planta Med. 81, 272–278. doi:10.1055/s-0034-1396315
Romero-Cerecero, O., Zamilpa-Álvarez, A., Jiménez-Ferrer, E., and Tortoriello, J. (2012a). Exploratory study on the effectiveness of a standardized extract from Ageratina pichinchensis in patients with chronic venous leg ulcers. Planta Med. 78, 304–310. doi:10.1055/s-0031-1280448
Romero-Cerecero, O., Zamilpa-Álvarez, A., Ramos-Mora, A., Alonso-Cortés, D., Jiménez-Ferrer, J. E., Huerta-Reyes, M. E., et al. (2011). Effect on the wound healing process and in vitro cell proliferation by the medicinal Mexican plant Ageratina pichinchensis. Planta Med. 77, 979–983. doi:10.1055/s-0030-1250743
Rosa, A. dos S., Bandeira, L. G., Monte-Alto-Costa, A., and Romana-Souza, B. (2014). Supplementation with olive oil, but not fish oil, improves cutaneous wound healing in stressed mice. Wound repair Regen. 22, 537–547. doi:10.1111/wrr.12191
Rosangkima, G., and Jagetia, G. (2015). In vitro anticancer screening of medicinal plants of Mizoram state, India, against Dalton’S lymphoma, Mcf-7 and Hela cells. Int. J. Recent Sci. Res. 6, 5648–5653.
Roy, R. K., Thakur, M., and Dixit, V. K. (2008). Hair growth promoting activity of Eclipta alba in male albino rats. Arch. Dermatol. Res. 300, 357–364. doi:10.1007/s00403-008-0860-3
Rüngeler, P., Lyss, G., Castro, V., Mora, G., Pahl, H. L., and Merfort, I. (1998). Study of three sesquiterpene lactones from Tithonia diversifolia on their anti-inflammatory activity using the transcription factor NF-kappa B and enzymes of the arachidonic acid pathway as targets. Planta Med. 64, 588–593. doi:10.1055/s-2006-957527
Sabri, E., and Handayani, R. (2022). Effect of Beluntas (Pluchea indica (L) Less) leaves ethanol extract of incision wound and healing in mice. Mus musculus L. 2022, 171–177. doi:10.5220/0010138200002775
Salazar-Gómez, A., and Alonso-Castro, A. J. (2022). Medicinal plants from Latin America with wound healing activity: Ethnomedicine, phytochemistry, preclinical and clinical studies-A review. Pharm. (Basel) 15, 1095. doi:10.3390/ph15091095
Salehi, B., Ata, A., V Anil Kumar, N., Sharopov, F., Ramírez-Alarcón, K., Ruiz-Ortega, A., et al. (2019). Antidiabetic potential of medicinal plants and their active components. Biomolecules 9, 551. doi:10.3390/biom9100551
Samanta, R., Pattnaik, A. K., Pradhan, K. K., Mehta, B. K., Pattanayak, S. P., and Banerjee, S. (2016). Wound healing activity of Silibinin in mice. Pharmacogn. Res. 8, 298–302. doi:10.4103/0974-8490.188880
Sanad, F. A.-A., Ahmed, S. F., and El-Tantawy, W. H. (2022). Antidiabetic and hypolipidemic potentials of Solidago virgaurea extract in alloxan-induced diabetes type 1. Arch. Physiol. Biochem. 128, 716–723. doi:10.1080/13813455.2020.1722705
Sangeetha, R. (2019). Luteolin in the management of type 2 diabetes mellitus. Curr. Res. Nutr. Food Sci. 7, 393–398. doi:10.12944/CRNFSJ.7.2.09
Sanyal, R., Nandi, S., Mandal, S., Dewanjee, S., Al Tawaha, A. R., Bursal, E., et al. (2022). “Eclipta prostrata (L.) L.: Traditional use, phytochemistry, and pharmacology,” in Medicinal plants of the Asteraceae family (SpringerLink), 173–195. doi:10.1007/978-981-19-6080-2_11
Schmidt, C., Fronza, M., Goettert, M., Geller, F., Luik, S., Flores, E. M. M., et al. (2009). Biological studies on Brazilian plants used in wound healing. J. Ethnopharmacol. 122, 523–532. doi:10.1016/j.jep.2009.01.022
Schütz, K., Carle, R., and Schieber, A. (2006). Taraxacum -a review on its phytochemical and pharmacological profile. J. Ethnopharmacol. 107, 313–323. doi:10.1016/j.jep.2006.07.021
Seaman, F. C. (1982). Sesquiterpene lactones as taxonomic characters in the asteraceae. Bot. Rev. 48, 121–594. doi:10.1007/BF02919190
Shahab, U., Faisal, M., Alatar, A. A., and Ahmad, S. (2018). Impact of wedelolactone in the anti-glycation and anti-diabetic activity in experimental diabetic animals. IUBMB Life 70, 547–552. doi:10.1002/iub.1744
Sharifi, R., Pasalar, P., Kamalinejad, M., Dehpour, A. R., Tavangar, S. M., Paknejad, M., et al. (2013). The effect of silymarin (Silybum marianum) on human skin fibroblasts in an in vitro wound healing model. Pharm. Biol. 51, 298–303. doi:10.3109/13880209.2012.721789
Sharifi, R., Rastegar, H., Kamalinejad, M., Dehpour, A. R., Tavangar, S. M., Paknejad, M., et al. (2012). Effect of topical application of silymarin (Silybum marianum) on excision wound healing in albino rats. Acta Med. Iran. 50, 583–588.
Shukla, A. N., Srinivastan, S., and Rawat, A. K. S. (2010). An ethnobotanical study of medicinal plants of Rewa district, Madhya Pradesh. Indian J. Tradit. Knowl. 9, 191–202.
Silalahi, M. (2022). Eclipta prostrata (L) L (uses and bioactivities). GSC Biol. Pharm. Sci. 18, 1–7. doi:10.30574/gscbps.2022.18.1.0371
Silva, L. R., Jacinto, T. A., and Coutinho, P. (2022). Bioactive compounds from cardoon as health promoters in metabolic disorders. Foods 11, 336–337. doi:10.3390/foods11030336
Sirichaiwetchakoon, K., Churproong, S., Kupittayanant, S., and Eumkeb, G. (2021). The effect of Pluchea indica (L) less. Tea on blood glucose and lipid profile in people with prediabetes: A randomized clinical trial. J. Altern. Complement. Med. 27, 669–677. doi:10.1089/acm.2020.0246
Sirichaiwetchakoon, K., Lowe, G. M., Kupittayanant, S., Churproong, S., and Eumkeb, G. (2020). Pluchea indica (L) Less. tea ameliorates hyperglycemia, dyslipidemia, and obesity in high fat diet-fed mice. Evid. Based. Complement. Altern. Med. 2020, 8746137. doi:10.1155/2020/8746137
Skottová, N., and Krecman, V. (1998). Silymarin as a potential hypocholesterolaemic drug. Physiol. Res. 47, 1–7.
Speroni, E., Govoni, P., Guizzardi, S., Renzulli, C., and Guerra, M. C. (2002). Anti-inflammatory and cicatrizing activity of Echinacea pallida Nutt. root extract. J. Ethnopharmacol. 79, 265–272. doi:10.1016/s0378-8741(01)00391-9
Spínola, V., and Castilho, P. C. (2017). Evaluation of Asteraceae herbal extracts in the management of diabetes and obesity. Contribution of caffeoylquinic acids on the inhibition of digestive enzymes activity and formation of advanced glycation end-products (in vitro). Phytochemistry 143, 29–35. doi:10.1016/j.phytochem.2017.07.006
Stefan, N., Birkenfeld, A. L., Schulze, M. B., and Ludwig, D. S. (2020). Obesity and impaired metabolic health in patients with COVID-19. Nat. Rev. Endocrinol. 16, 341–342. doi:10.1038/s41574-020-0364-6
Sunmonu, T. O., and Afolayan, A. J. (2013). Evaluation of antidiabetic activity and associated toxicity of Artemisia afra aqueous extract in wistar rats. Evid. Based. Complement. Altern. Med. 2013, 929074. doi:10.1155/2013/929074
Swargiary, A., Roy, B., and Ronghang, B. (2013). Partial characterisation of alkaline phosphatase in Fasciolopsis buski – An intestinal fluke treated with crude extract of Alpinia nigra (Zingiberaceae). J. Pharm. Technol. Drug Res. 2, 5. doi:10.7243/2050-120X-2-5
Swargiary, A., and Verma, A. K. (2015). Investigation on the binding affinities of different anthelmintic drugs on the 3D Model protein structure of acetylcholinesterase of Schistosoma mansoni: An in silico approach. Br. Biomed. Bull. 3, 020–033.
Tabandeh, M. R., Oryan, A., Mohhammad-Alipour, A., and Tabatabaei-Naieni, A. (2013). Silibinin regulates matrix metalloproteinase 3 (stromelysine1) gene expression, hexoseamines and collagen production during rat skin wound healing. Phytother. Res. 27, 1149–1153. doi:10.1002/ptr.4839
Tabari, S. A., Carpi, S., Polini, B., Nieri, P., Esfahani, M. L., Moghadamnia, A. A., et al. (2019). Topical application of silymarin enhances cutaneous wound healing in rats. South Afr. J. Bot. 124, 494–498. doi:10.1016/j.sajb.2019.06.004
Tadić, V., Arsić, I., Zvezdanović, J., Zugić, A., Cvetković, D., and Pavkov, S. (2017). The estimation of the traditionally used yarrow (Achillea millefolium L. Asteraceae) oil extracts with anti-inflamatory potential in topical application. J. Ethnopharmacol. 199, 138–148. doi:10.1016/j.jep.2017.02.002
Tewtrakul, S., Subhadhirasakul, S., Cheenpracha, S., and Karalai, C. (2007). HIV-1 protease and HIV-1 integrase inhibitory substances from Eclipta prostrata. Phytother. Res. 21, 1092–1095. doi:10.1002/ptr.2252
Thirumalai, T., David, E., Therasa, S. V., and Elumalai, E. K. (2011). Restorative effect of Eclipta alba in CCl4 induced hepatotoxicity in male albino rats. Asian Pac. J. Trop. Dis. 1, 304–307. doi:10.1016/S2222-1808(11)60072-8
Timalsina, D., and Devkota, H. P. (2021). Eclipta prostrata (L) L. (Asteraceae): Ethnomedicinal uses, chemical constituents, and biological activities. Biomolecules 11, 1738. doi:10.3390/biom11111738
Toiu, A., Vlase, L., Vodnar, D. C., Gheldiu, A.-M., and Oniga, I. (2019). Solidago graminifolia L. Salisb. (Asteraceae) as a valuable source of bioactive polyphenols: HPLC profile, in vitro antioxidant and antimicrobial potential. Molecules 24, 2666. doi:10.3390/molecules24142666
Tona, L., Kambu, K., Mesia, K., Cimanga, K., Apers, S., De Bruyne, T., et al. (1999). Biological screening of traditional preparations from some medicinal plants used as antidiarrhoeal in Kinshasa, Congo. Phytomedicine 6, 59–66. doi:10.1016/S0944-7113(99)80036-1
Tona, L., Kambu, K., Ngimbi, N., Cimanga, K., and Vlietinck, A. J. (1998). Antiamoebic and phytochemical screening of some Congolese medicinal plants. J. Ethnopharmacol. 61, 57–65. doi:10.1016/S0378-8741(98)00015-4
Tona, L., Kambu, K., Ngimbi, N., Mesia, K., Penge, O., Lusakibanza, M., et al. (2000). Antiamoebic and spasmolytic activities of extracts from some antidiarrhoeal traditional preparations used in Kinshasa, Congo. Phytomedicine 7, 31–38. doi:10.1016/S0944-7113(00)80019-7
Tousch, D., Lajoix, A.-D., Hosy, E., Azay-Milhau, J., Ferrare, K., Jahannault, C., et al. (2008). Chicoric acid, a new compound able to enhance insulin release and glucose uptake. Biochem. Biophys. Res. Commun. 377, 131–135. doi:10.1016/j.bbrc.2008.09.088
Toyang, N., and Verpoorte, R. (2013). A review of the medicinal potentials of plants of the genus Vernonia (asteraceae). J. Ethnopharmacol. 146, 681–723. doi:10.1016/j.jep.2013.01.040
van Huyssteen, M., Milne, P. J., Campbell, E. E., and van de Venter, M. (2011). Antidiabetic and cytotoxicity screening of five medicinal plants used by traditional African health practitioners in the Nelson Mandela Metropole, South Africa. Afr. J. Tradit. Complement. Altern. Med. 8, 150–158. doi:10.4314/ajtcam.v8i2.63202
Wang, X.-Q., Liu, R.-P., Wang, J., Luo, D., Li, Y.-H., Jiang, H., et al. (2022). Wedelolactone facilitates the early development of parthenogenetically activated porcine embryos by reducing oxidative stress and inhibiting autophagy. PeerJ 10, e13766. doi:10.7717/peerj.13766
Wang, Z., Gao, Z., Wang9, A., Jia, L., Zhang, X., Fang, M., et al. (2019). Comparative oral and intravenous pharmacokinetics of phlorizin in rats having type 2 diabetes and in normal rats based on phase II metabolism. Food Funct. 10, 1582–1594. doi:10.1039/C8FO02242A
WHO (2018). Obesity and overweight - facts. Available at: http://www.who.int/en/news-room/fact-sheets/detail/obesity-and-overweight.
Williams, C. A., Goldstone, F., and Greenham, J. (1996). Flavonoids, cinnamic acids and coumarins from the different tissues and medicinal preparations of Taraxacum officinale. Phytochemistry 42, 121–127. doi:10.1016/0031-9422(95)00865-9
Wintola, O. A., and Afolayan, A. J. (2011). Phytochemical constituents and antioxidant activities of the whole leaf extract of Aloe ferox. Mill. Pharmacogn. Mag. 7, 325–333. doi:10.4103/0973-1296.90414
Wirngo, F. E., Lambert, M. N., and Jeppesen, P. B. (2016). The physiological effects of dandelion (Taraxacum officinale) in type 2 diabetes. Rev. Diabet. Stud. 13, 113–131. doi:10.1900/RDS.2016.13.113
Woldeamanuel, M. M., Geda, M. K., Mohapatra, S., Bastia, T. K., Rath, P., and Panda, A. K. (2022). Ethnobotanical study of endemic and non-endemic medicinal plants used by indigenous people in environs of gullele botanical garden addis ababa, central Ethiopia: A major focus on asteraceae family. Front. Pharmacol. 13, 1020097. doi:10.3389/fphar.2022.1020097
Wu, L.-C., Fan, N.-C., Lin, M.-H., Chu, I.-R., Huang, S.-J., Hu, C.-Y., et al. (2008). Anti-inflammatory effect of spilanthol from Spilanthes acmella on murine macrophage by down-regulating LPS-induced inflammatory mediators. J. Agric. Food Chem. 56, 2341–2349. doi:10.1021/jf073057e
Xiao, J., Kai, G., Yamamoto, K., and Chen, X. (2013a). Advance in dietary polyphenols as α-glucosidases inhibitors: A review on structure-activity relationship aspect. Crit. Rev. Food Sci. Nutr. 53, 818–836. doi:10.1080/10408398.2011.561379
Xiao, J., Ni, X., Kai, G., and Chen, X. (2013b). A review on structure-activity relationship of dietary polyphenols inhibiting α-amylase. Crit. Rev. Food Sci. Nutr. 53, 497–506. doi:10.1080/10408398.2010.548108
Xie, M., Chen, G., Wan, P., Dai, Z., Zeng, X., and Sun, Y. (2019). Effects of dicaffeoylquinic acids from Ilex kudingcha on lipid metabolism and intestinal microbiota in high-fat-diet-fed mice. J. Agric. Food Chem. 67, 171–183. doi:10.1021/acs.jafc.8b05444
Xie, Y., and Chen, X. (2013). Structures required of polyphenols for inhibiting advanced glycation end products formation. Curr. Drug Metab. 14, 414–431. doi:10.2174/1389200211314040005
Xuan, T. D., and Khanh, T. D. (2016). Chemistry and pharmacology of Bidens pilosa: An overview. J. Pharm. Investig. 46, 91–132. doi:10.1007/s40005-016-0231-6
Yadav, N. K., and Yadav, R. (2023). Medicinal effects, phytochemistry, pharmacology of Euphorbia prostrata and promising molecular mechanisms. Chin. J. Integr. Med. doi:10.1007/s11655-023-3544-0
Yamane, L. T., de Paula, E., Jorge, M. P., de Freitas-Blanco, V. S., Junior, Í. M., Figueira, G. M., et al. (2016). Acmella oleracea and Achyrocline satureioides as sources of natural products in topical wound care. Evid. Based. Complement. Altern. Med. 2016, 3606820. doi:10.1155/2016/3606820
Yambe-Silavwe, H., and Williamson, G. (2018). Chlorogenic and phenolic acids are only very weak inhibitors of human salivary α-amylase and rat intestinal maltase activities. Food Res. Int. 113, 452–455. doi:10.1016/j.foodres.2018.07.038
Yang, W.-C. (2014). Botanical, pharmacological, phytochemical, and toxicological aspects of the antidiabetic plant Bidens pilosa L. Evid. Based. Complement. Altern. Med. 2014, 698617. doi:10.1155/2014/698617
Yin, S., Yan, Y., Huang, T., Guan, J., Wu, L., and Li, K. (2017). Therapeutic effect of Artemisia argyi on oral ulcer in rats. Zhong Nan Da Xue Xue Bao. Yi Xue Ban. 42, 824–830. doi:10.11817/j.issn.1672-7347.2017.07.014
Yoon, H. N., Lee, M. Y., Kim, J.-K., Suh, H.-W., and Lim, S. S. (2013). Aldose reductase inhibitory compounds from Xanthium strumarium. Arch. Pharm. Res. 36, 1090–1095. doi:10.1007/s12272-013-0123-5
Yoon, M.-S., Won, K.-J., Kim, D. Y., Hwang, D., Yoon, S. W., Kim, B., et al. (2014). Skin regeneration effect and chemical composition of essential oil from Artemisia montana. Nat. Prod. Commun. 9, 1619–1622. doi:10.1177/1934578x1400901123
Zehra, A. S., Bhattarai, P., Zhang, J., Liu, Y., Parveen, Z., Sajid, M., et al. (2023). In vitro and in vivo evaluation of the antidiabetic activity of Solidago virgaurea extracts. Curr. Bioact. Compd. 19, 68–78. doi:10.2174/1573407218666220615143502
Zhang, J., Sasaki, T., Li, W., Nagata, K., Higai, K., Feng, F., et al. (2018). Identification of caffeoylquinic acid derivatives as natural protein tyrosine phosphatase 1B inhibitors from Artemisia princeps. Bioorg. Med. Chem. Lett. 28, 1194–1197. doi:10.1016/j.bmcl.2018.02.052
Zhang, L., Zheng, C., Xu, T., and Du, L. (2022). Antihyperglycemic, antihyperlipidemic, and antioxidant effects of Eclipta prostrata L. aqueous extract in streptozotocin-induced diabetic rats. Sains Malays. 51, 3359–3370. doi:10.17576/jsm-2022-5110-20
Zhao, C., Yang, C., Wai, S. T. C., Zhang, Y., P Portillo, M., Paoli, P., et al. (2019). Regulation of glucose metabolism by bioactive phytochemicals for the management of type 2 diabetes mellitus. Crit. Rev. Food Sci. Nutr. 59, 830–847. doi:10.1080/10408398.2018.1501658
Glossary
Keywords: Asteraceae, diabetes, drug development, DiaNat-DB, ethnomedicine, nutraceuticals
Citation: Mohanta YK, Mishra AK, Nongbet A, Chakrabartty I, Mahanta S, Sarma B, Panda J and Panda SK (2023) Potential use of the Asteraceae family as a cure for diabetes: A review of ethnopharmacology to modern day drug and nutraceuticals developments. Front. Pharmacol. 14:1153600. doi: 10.3389/fphar.2023.1153600
Received: 29 January 2023; Accepted: 29 June 2023;
Published: 03 August 2023.
Edited by:
Habib Yaribeygi, Semnan University of Medical Sciences, IranReviewed by:
Armando Caceres, Galileo University, GuatemalaPrawej Ansari, Independent University, Bangladesh
Copyright © 2023 Mohanta, Mishra, Nongbet, Chakrabartty, Mahanta, Sarma, Panda and Panda. This is an open-access article distributed under the terms of the Creative Commons Attribution License (CC BY). The use, distribution or reproduction in other forums is permitted, provided the original author(s) and the copyright owner(s) are credited and that the original publication in this journal is cited, in accordance with accepted academic practice. No use, distribution or reproduction is permitted which does not comply with these terms.
*Correspondence: Sujogya Kumar Panda, sujogyapanda@utkaluniversity.ac.in; Awdhesh Kumar Mishra, awadhesh.biotech07@gmail.com
†These authors have contributed equally to this work